- 1Key Laboratory of Cultivation and Protection for Non-Wood Forest Trees, Ministry of Education, Central South University of Forestry and Technology, Changsha, China
- 2CAS Key Laboratory of Plant Germplasm Enhancement and Specialty Agriculture, Wuhan Botanical Garden, The Innovative Academy of Seed Design, Chinese Academy of Sciences, Wuhan, China
- 3Center of Economic Botany, Core Botanical Gardens, Chinese Academy of Sciences, Wuhan, China
- 4University of Chinese Academy of Sciences, Beijing, China
The seed oil of tung tree is rich in a-eleostearic acid (ESA), which endows tung oil with the characteristic of an excellently dry oil. The stearoyl-acyl carrier protein δ9 desaturase (SAD) is a rate-limiting enzyme that converts the stearic acid to the oleic acid, the substrate for the production of the α-ESA. However, the function of the two predicted VfSAD1 and VfSAD2 genes in the tung tree has not been determined. In this study, quantitative real-time PCR (qRT-PCR) analysis showed that VfSAD1 and VfSAD2 were expressed in multiple organs of tung tree but were highly expressed in the seed during the oil rapid accumulation period. Heterologous expression of VfSAD1 and VfSAD2 could promote the production of oleic acid and its derivatives in Arabidopsis thaliana and yeast BY4741, indicating that VfSAD1 and VfSAD2 possess the stearoyl-ACP desaturases function. Furthermore, both VfSAD1 and VfSAD2 could significantly improve seed oil accumulation in Arabidopsis. VfSAD1 could also significantly promote the oil accumulation in the yeast BY4741 strain. In addition, overexpression of VfSAD1 and VfSAD2 enhanced the tolerance of yeast and Arabidopsis seedlings to low temperature stress. This study indicates that the two VfSAD genes play a vital role in the process of oil accumulation and fatty acid biosynthesis in the tung tree seed, and both of them could be used for molecular breeding in tung tree and other oil crops.
1 Introduction
Tung tree (Vernicia fordii) is an oil-bearing woody plant of Euphorbiaceae. The oil extracted from the fruits of the tung tree, also called tung oil, is a superior drying oil, which is widely used in multiple aspects of the industry. Due to its economic value, tung tree has been cultivated in China for over 1,000 years in history. The plant’s oil quality is largely determined by the composition of fatty acid. The α-eleostearic acid (α-ESA, 18:3Δ9cis, 11trans, 13trans) is an unusual fatty acid, which contains three conjugated unsaturated double bonds (Dyer et al., 2002). It accounts for more than 80% of fatty acid components in the tung oil and thus endows the tung oil with active chemical activity.
Triacylglycerol is the main storage form of plant oil and the most important energy supplier for plant growth and development. Lots of efforts have been made to detect the complex molecular mechanism for seed oil accumulation (Shockey et al., 2016; Zhang et al., 2021; Nam et al., 2022; Yang et al., 2022). At this background, tung tree genome was published (Cui et al., 2018; Zhang et al., 2019), which benefited the deep investigation of tung oil accumulation at the molecular level. Tung oil quickly accumulated from early August to late September (Galli et al., 2014). Meanwhile, transcriptome analysis revealed that a large body of the genes involved in oil biosynthesis and accumulation were upregulated and kept a high expression pattern during the oil rapid accumulation period and then was sharply downregulated at the late period of oil accumulation (Cui et al., 2018; Zhang et al., 2019). The tung tree genotype with the higher seed oil content, to a large degree, was due to the extended phase of the high expression level of those global genes involved in oil biosynthesis and accumulation (Zhang et al., 2018). In addition, efforts were also made to detect the critical transcript factors that were involved in tung oil accumulation during the oil accumulation period (Zhang et al., 2018; Zhang et al., 2021). The biosynthetic pathway of tung oil is shown in Supplementary Figure S1. In the seed of tung tree, the oleic acid synthesized in the plastid is transported to the ER, where the oleic acid is further modified by the FAD2 and FADX enzymes to form the α-ESA (Dyer et al., 2002). The fatty acid molecules in the ER were then assembled into TAG mainly by two pathways, the Kenney pathway and the PC-derived DAG pathway (Li-Beisson et al., 2013). Up to now, little is known about the molecular mechanism responsible for the high percentage of the α-ESA in tung oil (van Erp et al., 2015; Yurchenko et al., 2017). Thus, there is an urgent need to investigate the function of the components in the tung oil biosynthetic complex.
Stearoyl-acyl carrier protein Δ9 desaturase (SAD) is an important rate-limiting enzyme that controls the desaturation of stearic acid to the oleic acid during the process of fatty acid de novo synthesis in the plastid. Up to now, the desaturases of SAD derived from various plants have been investigated by heterologous expression (Li et al., 2020). The oleic acid produced by the SAD could be assembled into different lipid classes. Thus, the SAD genes actually participated in multiple biological processes during the growth and development of plants. In Arabidopsis, it was proven that the SAD members participated in the embryo development, elaboration of the embryonic cuticle, and storage of lipid production during the seed maturation phase (Kazaz et al., 2020). During the oil rapid accumulation period, two predicted SAD genes were highly expressed in the seeds of tung tree. However, the function of the SAD genes from tung tree during seed oil accumulation has not been studied. In this study, the VfSAD1 and VfSAD2 are proven to be the stearoyl-ACP Desaturases. It could improve the lipid accumulation in the seed of Arabidopsis and enhance the tolerance of Arabidopsis seedlings to low-temperature stress. Similar results were obtained by the heterologous expression of the two VfSAD genes in the yeast BY4741 strains. This study indicated that the two SAD genes should play a crucial role in the seed oil accumulation of tung tree.
2 Materials and methods
2.1 Plant materials and methods
Tung tree fruits were collected from tung tree germplasm nursery established in Wuhan, Hubei, China, in 2021. Lipid accumulation in tung tree seeds began in July, accumulated rapidly in August, and stabilized in September. We aim to focus on the conversion of saturated fatty acids to unsaturated fatty acids. Based on this process, we selected five representative lipid accumulation periods. Tung fruits were collected at five stages of seed development (including 26 Jul, 2 August, 16 August, 30 August, and 13 September). Three fruits were taken each time, each fruit as one biological repeat. The endosperm tissue was ground into powder in liquid nitrogen for oil content measurement. Arabidopsis thaliana was grown in the greenhouse at 26°C and 60% relative humidity with a 14-h light/10-h dark–light cycle.
2.2 RNA isolation, inversion, and real-time quantitative PCR
Total RNA was extracted from the seeds of tung fruit an RNA extraction kit (BioTeck Corporation, China). RNA purity and RNA concentrations were detected by a nanophotometer (IMPLEN, CA, USA). According to the manufacturer’s instructions, the first-strand cDNA was reverse-transcribed using PrimeScript™ RT Kit (Cat. RR047A, TaKaRa, Japan). The reaction solution contained SYBR® PremixExTaq™ II (Tli RNaseH Plus) (TaKaRa, Japan). The gene-specific primers used for qPCR are shown in Supplementary Table S1. Applied Biosystems™ QuantStudio™ 6 Flex Real-Time PCR System was used in the experiment, with a total of three replicates. Reaction conditions were 95°C for 30 s, 95°C for 10 s, 60°C for 30 s, and 40 cycles. After the final cycle, the melting curve was analyzed, rising from 60°C to 95°C with an increase of 0.3°C/5s. The 2−ΔΔCT method was used to analyze the fluorescence quantitative PCR data (Livak and Schmittgen, 2001).
2.3 Sequence analysis of VfSAD1 and VfSAD2 proteins from Vernicia fordii
The physical and chemical properties were analyzed by using ProtParam online tool (http://web.expasy.org/protparam/). Signal peptide prediction SignalP4.0 server (http://www.cbs.dtu.dk/services/Sig-nalP/) was to predict VfSAD1 and VfSAD2 amino acid sequence of the splice site if there was a potential signal peptide and its place. The online software protscale was used to analyze the hydrophobicity of VfSAD1 and VfSAD2. Tmpred website was used to predict the transmembrane domain of VfSAD1 and VfSAD2 proteins and at the same time submit the amino acid sequence to online analysis software for protein secondary structure prediction (https://www.predictprotein.org/). The tertiary structure of proteins was modeled by SWISS-MODEL prediction and modeling (https://swissmodel.expasy.Org). Multiple protein sequences were aligned with Geneious software, and the alignment results were compared with MAGE7.0 software to construct a phylogenetic tree using the neighbor-joining method.
2.4 Subcellular localization
The Agrobacterium infection method was applied to infuse Agrobacterium containing 35S: VfSAD1-GFP, 35S: VfSAD1-GFP, and Agrobacterium containing plasmid location Marker 217: mCherry into the lower epidermal cells of wild-type tobacco leaves, respectively. After dark culture for 24 h at room temperature and 48 h under normal light, the co-localization of proteins encoded by VfSAD1 and VfSAD2 genes and chloroplast Marker 217: mCherry was observed under laser confocal three-dimensional scanner (TCS SP8).
2.5 Measurement of oil content and fatty acid components
The oil content of tung tree seeds was measured using gas chromatography (GC, Agilent 7820A) according to the method of Zhang et al. (2018). The measurement of oil content and fatty acid components in the mature seed of Arabidopsis was referred to Li-Beisson et al. (2013). The total oil content of Saccharomyces cerevisiae BY4741 in 20 ml of SD-Trp liquid medium was measured. Analyses of fatty acid composition and oil content in BY4741 were referred to Wu et al. (2018). The fatty acid percentage is calculated according to the percentage of fatty acid peak area. The oil content is calculated by the following formula:
Note: The internal standard dosage in this study was 20 mg for Arabidopsis seeds, 50–80 mg for tung tree seeds (select specific dosage according to different lipid content), and 50 mg for yeast strains.
The error bar represents the standard deviation ( ± SD) for setting three biological replicates. For example, statistical analysis of data such as oil content and 1,000-grain weight of seeds was conducted by using t-test (* means p< 0.05; ** means p< 0.01). A comparison between groups was conducted by one-way ANOVA test (FA component), and the different letters above the bar chart indicated significant differences (p< 0.05).
2.6 Low-temperature stress treatments of transgenic yeasts
VfSAD1/2 was used to construct the pESC-Trp expression vector in yeast experiments; after extracting the plasmid, the yeast strain BY4741 was transformed to verify the function of SAD dehydrogenase.
In this experiment, three to five repeated bacterial liquids and colonies were selected, screened by glucose medium, and cleaned with sterile water, and underwent a galactose-induced expression program, at 17°C and 30°C, and the OD600 value of the colony was focused on the performance after 12 h. The solid medium culture was a unified dilution concentration multiple (100, 10−1, 10−2, 10−3, 10−4, 10−5, and 10−6).
27 Low-temperature stress treatments of transgenic Arabidopsis
In this study, the Columbia ecotype of Arabidopsis thaliana was used as control. According to the method of Huang et al. (2022), transgenic Arabidopsis seeds were sterilized, and 25 seeds (5×5 rows) were placed on MS solid medium (MS 4.43 g/L+ sucrose 10 g/L + agar 8 g/L) using toothpicks. To achieve consistent germination and growth of Arabidopsis seeds, the seeds were placed in 4°C darkness for 2 days for vernalization (vernalization treatment). After fully absorbing water, all seeds were placed in a plant room (24°C under a 16-h light/8-h dark cycle with a photon flux of 240 µmol/m/s) for a week. Arabidopsis thaliana materials were placed in an incubator at −8°C for 1–3 h, the phenotype was observed, growth was recovered at 24°C for 3 days, and then the survival rate was calculated.
3 Results
3.1 Molecular identification and sequence analysis of VfSAD1 and VfSAD2 genes from tung tree seed
We identified three SAD members using the genome data, but only two were upregulated in the lipid synthesis pathway. These two genes were predicted as stearoyl-acyl carrier protein Δ9 desaturase (SAD). Based on tung tree genomic data, the sequences of the two genes, named VfSAD1 and VfSAD2, were obtained. The open reading frame length of VfSAD1 was 1,191 bp, encoding 397 amino acids, and the open reading frame length of VfSAD2 was 1,176 bp, encoding 386 amino acids (Supplementary Table S2). Subcellular localization analysis revealed that VfSAD1 and VfSAD2 were both located on chloroplasts (Figure 1A). Using ProtParam online tool analysis, the molecular formula of VfSAD1 is C3601H6013N1191O1507S247, and its molecular weight is approximately 45.54 kDa. The molecular formula of VfSAD2 is C3541H5908N1176O1477S255, and its molecular weight is approximately 43.82 kDa. Other parameters of physicochemical properties of VfSAD1 and VfSAD2 proteins are listed in Supplementary Table S2. The protein secondary structure of VfSAD1 and VfSAD2 was analyzed using online software (https://www.predictprotein.org/). The results showed that VfSAD1 and VfSAD2 proteins had a similar secondary structure (Supplementary Figure S2A). In addition, multiple sequence alignment showed that VfSAD1 and VfSAD2 proteins are highly conserved among plant SAD members (Supplementary Figure S3A). Furthermore, the phylogenetic tree of VfSADs was constructed using the adjacency method through MAGE5.0 software. As shown in Figure 1B, VfSAD1 was closely related to Vernicia montana Lour, and VfSAD2 was closely related to Ricinus communis.
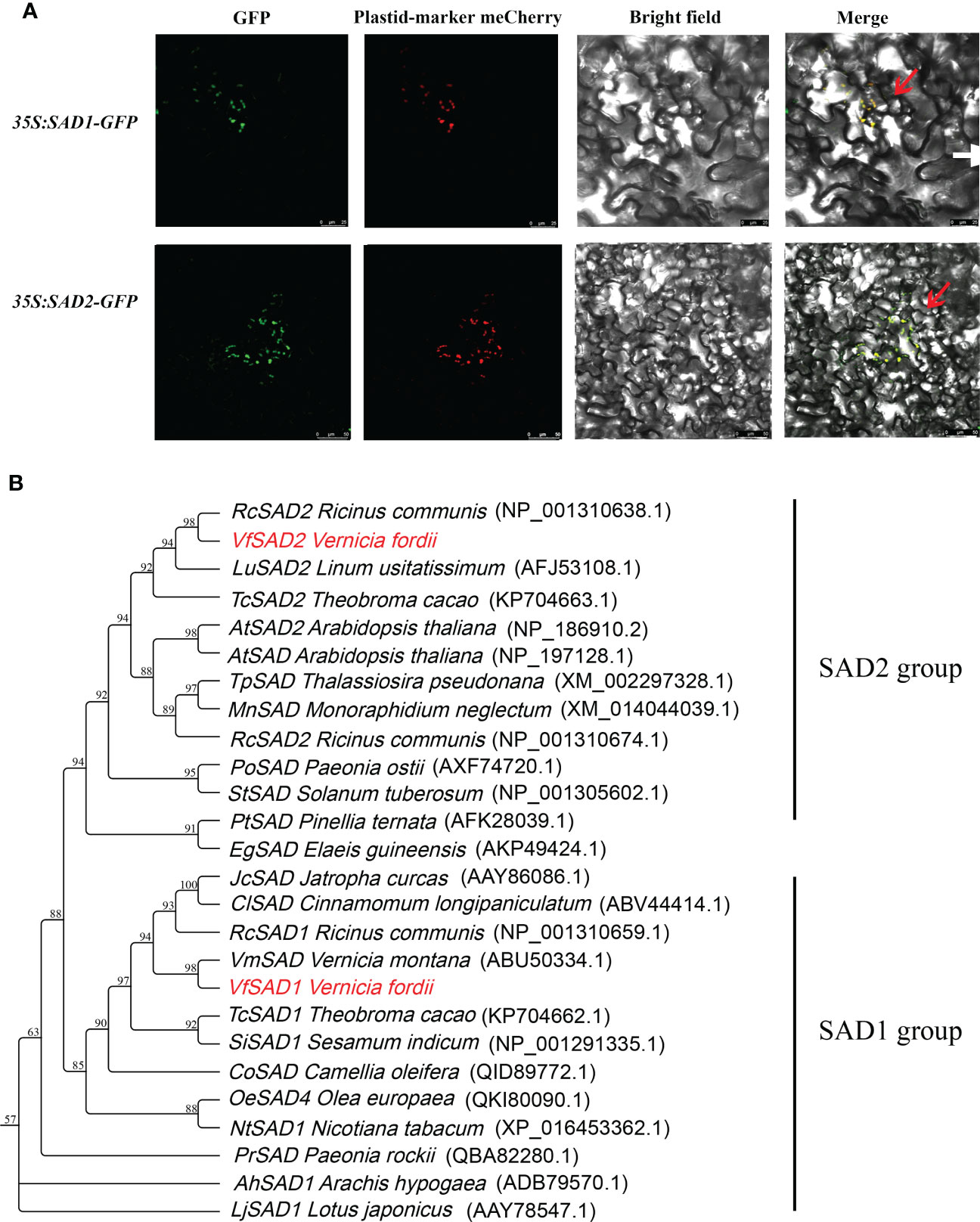
Figure 1 The characteristic analysis of VfSAD1 and VfSAD2 protein. (A) Subcellular localization of VfSAD1 and VfSAD2 proteins. (B) Phylogenetic tree analysis of SAD proteins from tung tree and other species.
3.2 The mRNA levels of VfSAD1 and VfSAD2 were largely upregulated in the seed during the oil accumulation period
The expression of VfSAD1/2 gene in different tissue parts and seed kernels at five different developmental stages was analyzed by quantitative real-time PCR (qRT-PCR). The accumulation of oil in the seeds of Aleurites mainly comes from the endosperm gradually expanded at the later stage of kernel maturation. As shown in Figures 2G and H, the VfSAD1/2 gene was expressed in the kernel, flower, stem, and leaf of tung tree, and the expression level in the kernel was higher than that in other tissues. In this study, seed kernel expression was detected at the five developmental stages (Figure 2). The expression level of VfSAD1 and VfSAD2 genes began to increase gradually on 26 July and increased rapidly to the highest level on 30 August (at the stage of lipid synthesis). After reaching the peak, the expression level of VfSAD1 and VfSAD2 genes decreased rapidly to the lowest level on 3 September (at the end of lipid accumulation). Based on the expression patterns of VfSAD genes, it was concluded that VfSAD1 and VfSAD2 may be involved in lipid synthesis and storage during the development of tung tree seeds.
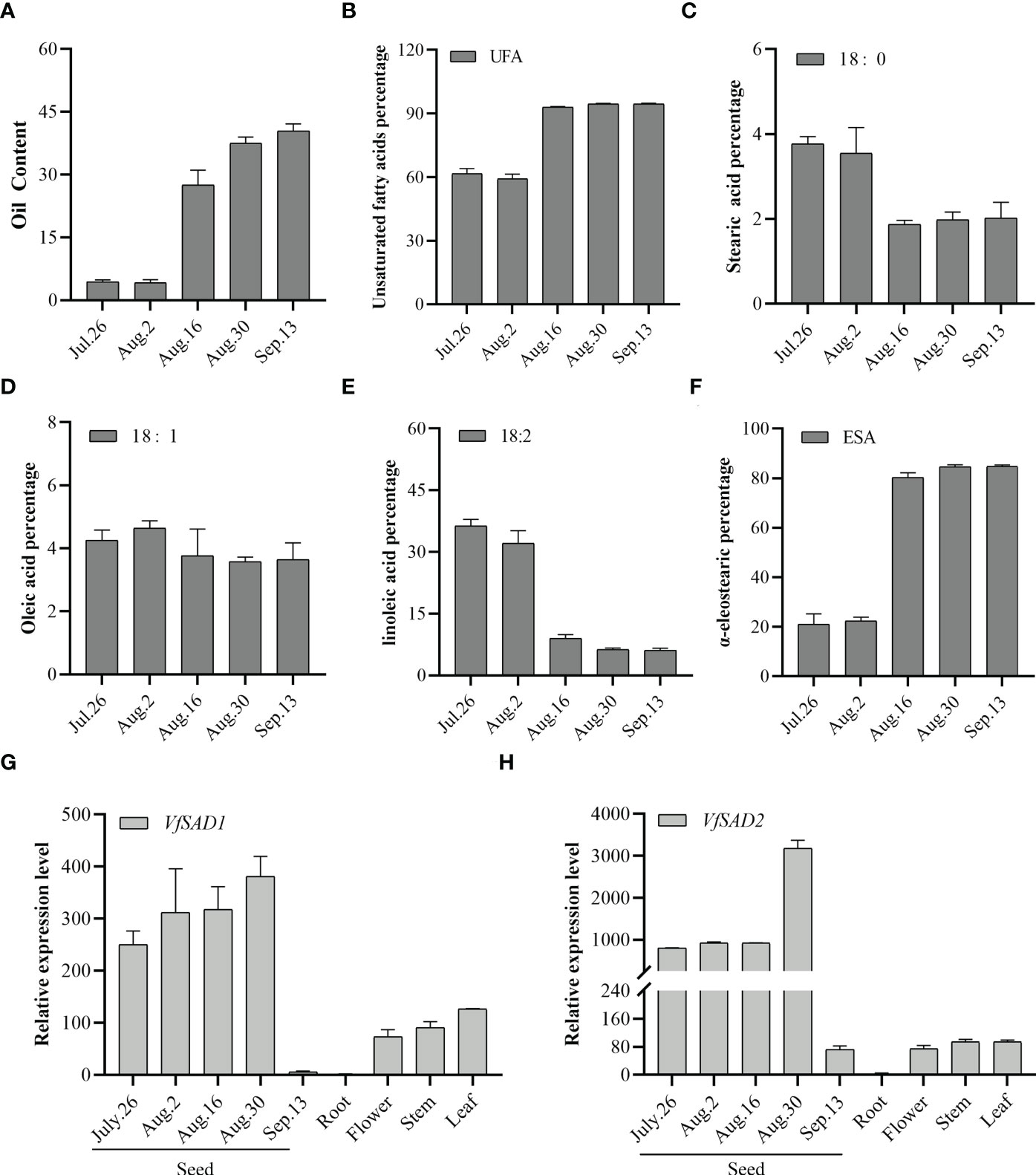
Figure 2 Oil content in the developing seed and the expression level of the two SAD genes in the multiple tissues of tung tree. (A) Oil content in the seeds from multiples development stages; (B–F) percentage of unsaturated fatty acid (B), stearic acid (C), oleic acid (D), linoleic acid (E), and α-eleostearic (F) in the seeds from multiples development stages; (G, H) the relative expression level of VfSAD1 (G) and VfSAD2 (H) in the different tissues of tung tree. The error bar represents the standard deviation (± SD) for setting three biological replicates.
By analyzing the samples from the five periods, it was found that before 2 August, the lipid content was low (Figure 2A), and the unsaturated fatty acids accounted for approximately 60% of the total fatty acids (Figure 2B). But since 16 August, lipids have begun to accumulate in large quantities, with unsaturated fatty acids increased sharply to 90%. The unsaturated fatty acids in IL tung oil mainly include oleic acid, linoleic acid, and α-ESA. As shown in Figures 2C–E, oleic acid content was stable. Linoleic acid content decreased sharply since 16 August, but alpha-ESA content increased dramatically and accounted for more than 80% of the total fatty acids (Figure 2F). The results showed that stearic acid could be efficiently converted to oleic acid, and oleic acid could be efficiently converted to α-ESA. Oil accumulation and fatty acid conversion tended to stop at a late stage of seed development. Similarly, according to Figures 2G, H, VfSAD1/2 expression decreased sharply. These results suggested that the VfSAD1 and VfSAD2 genes are mainly involved in regulating the transformation of saturated fatty acid stearic acid in tung tree seeds, thereby increasing the amount of biosynthesis of unsaturated fatty acid–oleic acid and other products.
3.3 Overexpression of VfSAD1 and VfSAD2 could change the composition of fatty acids
The VfSAD1 and VfSAD2 were investigated in the S. cerevisiae BY4741 to determine the biochemical function. Thus, VfSAD1 and VfSAD2 were transferred into BY4741, respectively. The fatty acid in the oil of BY4741 contained palmitic acid, palmitoleic acid, stearic acid, and oleic acid (Figure 3A). The fatty acid profile demonstrated that VfSAD1 and VfSAD2 altered the percentage of the fatty acid component in the oil of BY4741. The content of oleic acid changed the most, increasing by approximately 10% (Figure 3B) in the BY4741 strain containing VfSAD1 or VfSAD2 gene. In addition, the stearic acid was significantly increased, and the palmitic acid and palmitoleic acid were significantly decreased in the BY4741 containing VfSAD1 or VfSAD2 gene in comparison with that containing the empty plasmid. The result reflected that VfSAD1 and VfSAD2 promoted the production of oleic acid and thus led to the percentage alteration of the palmitic acid, palmitoleic acid, and stearic acid in the lipid of BY4741.
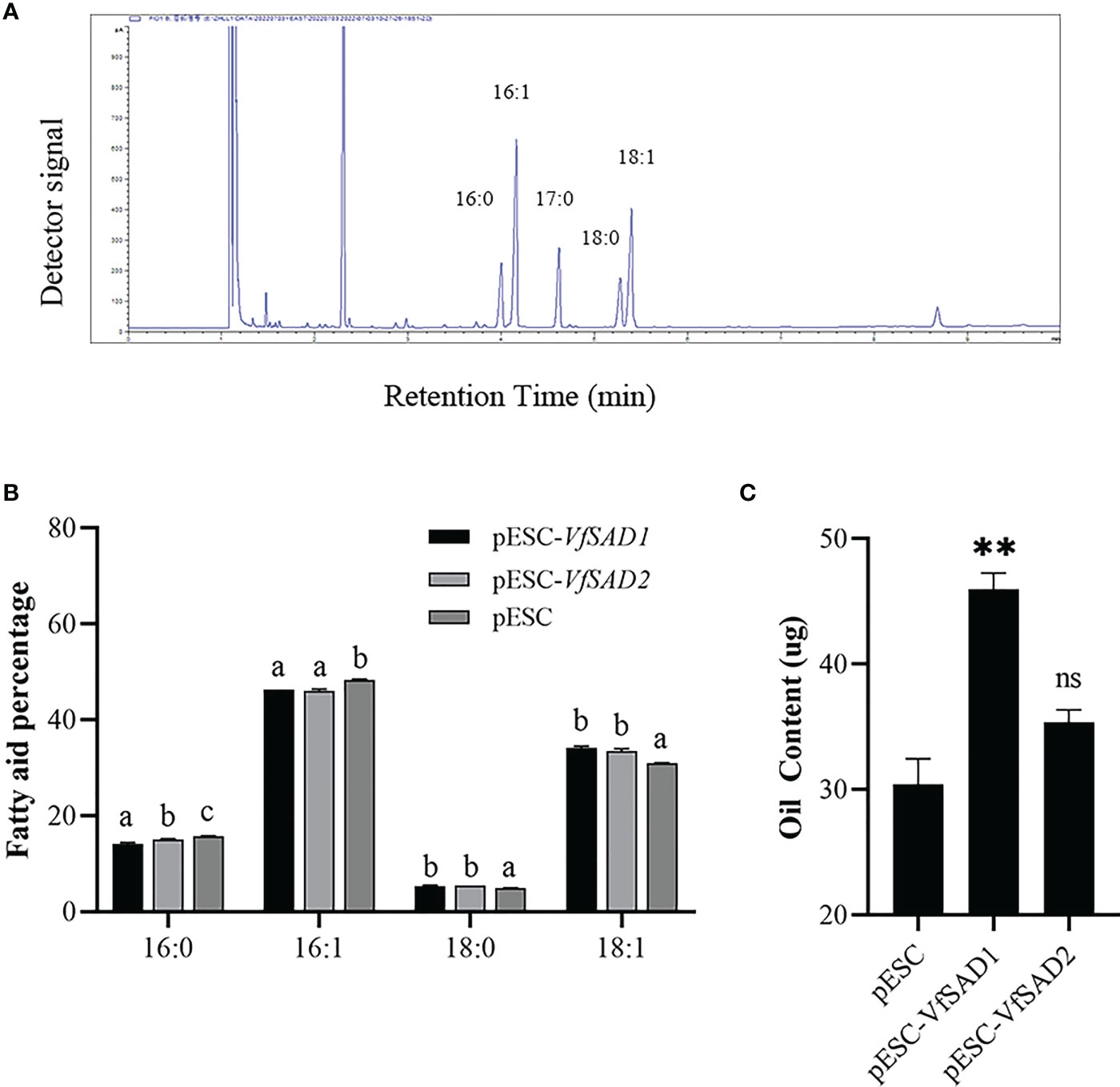
Figure 3 The VfSAD1/2 improves oil content and alters the percentage of the fatty acid component in the S. cerevisiae BY4741. (A) GC-FID analysis of fatty acid methyl esters derived from neutral lipids isolated from S. cerevisiae BY4741. (B) The percentage of fatty acid components in the wild-type BY4741 containing the empty plastid and transgenic BY4741 with the overexpression of VfSAD1 or VfSAD2. (C) Oil content in the wild-type BY4741 and transgenic BY4741 containing VfSAD1 or VfSAD2. The error bar represents the standard deviation (± SD) for setting three biological replicates. Significant difference was detected by t-test (* indicates p< 0.05; ** indicates p< 0.01). A comparison between groups was conducted by one-way ANOVA test (FA component), and the different letters above the bar chart indicate significant differences (p< 0.05).
To further confirm the biochemical function of VfSAD1 and VfSAD2, overexpression was performed in the fad3fae1 double mutant of the accession Landsberg (Ler) of A. thaliana. The fatty acid components were analyzed in the seed of VfSAD1/2-overexpression (VfSAD1/2-OE) lines and the fad3fae1 line. The oleic acid and linoleic acid accounted for the largest proportion in the fad3fae1 line. Compared with the fad3fae1 line, oleic acid content was not obviously changed in the seeds of VfSAD1/2-OE lines, but its derivation, linoleic acid, was significantly increased. The palmitic acid and stearic acid content were also significantly decreased in the seeds of VfSAD1/2-OE lines (Figure 4A). The result reflected that VfSAD1 and VfSAD2 promoted the production of oleic acid that was further converted to linoleic acid in the seed of VfSAD1/2-OE lines. Thus, the results above revealed that the two SADs of tung tree were the stearoyl-ACP desaturases.
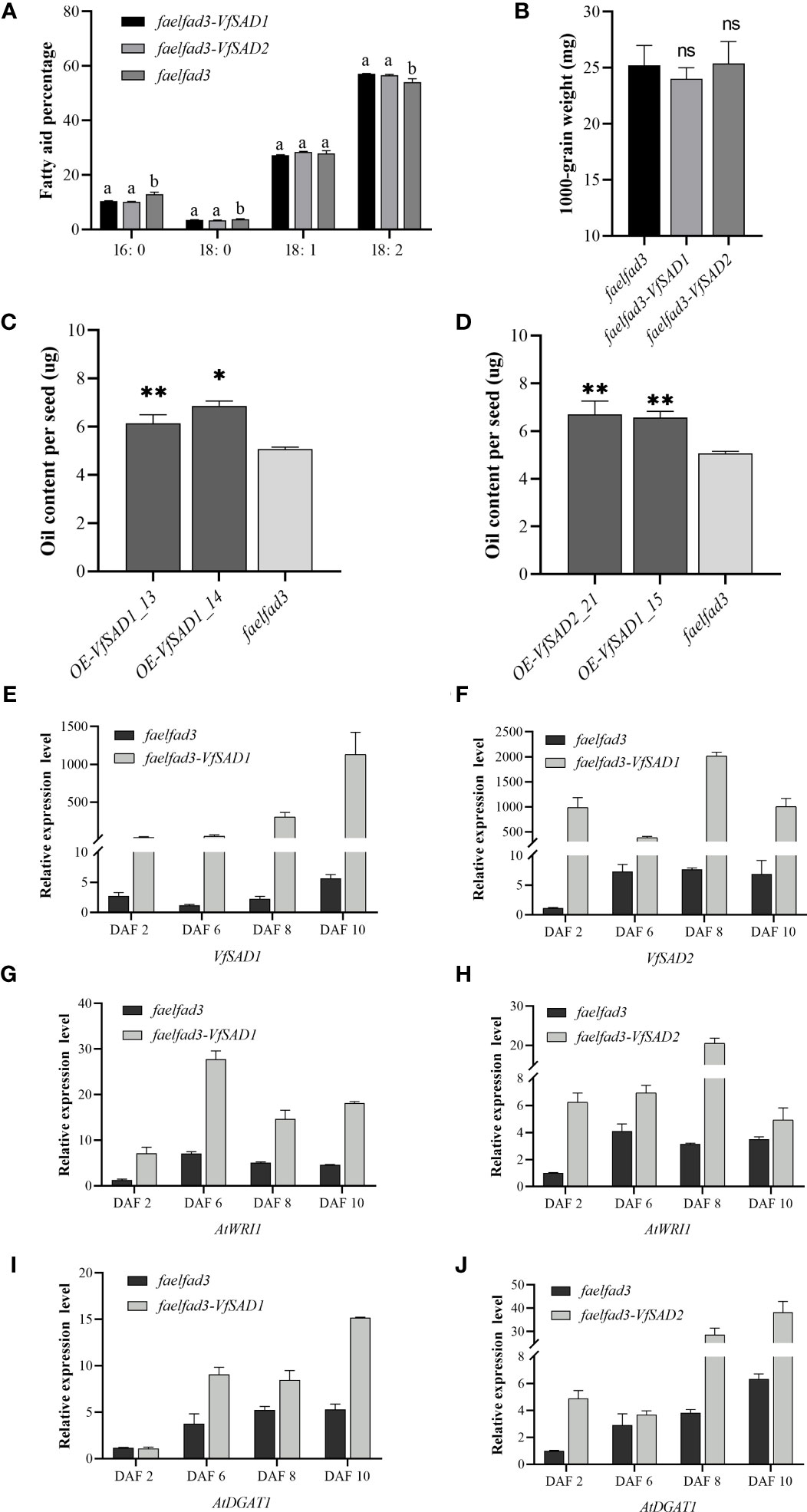
Figure 4 The overexpression of VfSAD1/2 altered the percentage of fatty acid components and oil content in the seeds of Arabidopsis. (A) Fatty acid composition in seeds of SAD1-OE line, SAD2-OE line, and fad3fae1 line. (B) The 1,000-grain weight in seeds of SAD1-OE line, SAD2-OE line, and fad3fae1 line. (C) Oil content in seeds of SAD1-OE lines and fad3fae1 line. (D) Oil content in seeds of SAD2-OE lines and fad3fae1 line. (E) The expression pattern of VfSAD1 in the SAD1-OE line and fad3fae1 line. (F) The expression pattern of VfSAD2 in the SAD2-OE line and fad3fae1 line. (G) The expression pattern of AtWRI1 in the SAD1-OE line and fad3fae1 line. (H) The expression pattern of AtWRI1 in the SAD2-OE line and fad3fae1 line. (I) The expression pattern of AtDGAT1 in the SAD1-OE line and fad3fae1 line. (J) The expression pattern of AtDGAT1 in the SAD2-OE line and fad3fae1 line. The error bar represents the standard deviation (± SD) for setting three biological replicates. Significant difference was detected by t-test (* indicates p< 0.05; ** indicates p< 0.01). A comparison between groups was conducted by one-way ANOVA test (FA component), and the different letters above the bar chart indicate significant differences (p< 0.05).
3.4 Overexpression of VfSAD1 and VfSAD2 could improve oil accumulation
To detect the influence of the overexpression of VfSAD1 and VfSAD2 on oil accumulation, the oil content in the yeast and seed of Arabidopsis was measured. In the yeast, the oil content was significantly increased in the BY4741containing VfSAD1 gene, with an increase of 30%–34% but was not in the BY4741 strain containing VfSAD2 gene (Figure 3C). In Arabidopsis, the seed oil content was significantly improved in the VfSAD1-OE and VfSAD2-OE lines than that in the fad3fae1 line (Figures 4C, D). Compared with fad3fae1, the oil content was increased by 21.1% and 35.3% in the three homozygous VfSAD1-OE lines, respectively (Figure 4C). The oil content was increased by 29.4% and 32.2% in the three homozygous VfSAD2-OE lines, respectively (Figure 4D). In addition, it was found that seed weight was not obviously altered in the VfSAD1/2-OE lines in comparison to the fad3fae1 line (Figure 4B). The results further indicated that overexpression of VfSAD1 and VfSAD2 promoted the accumulation of oil.
3.5 The expression of AtWRI1 and AtDGAT1 was upregulated in the VfSAD1/2-OE lines
WRI1 is a master transcriptome factor that directly regulates multiple genes involved in the fatty acid de novo biosynthesis (To et al., 2012). DGAT1 is one of the diacylglycerol acyltransferases, which has been proven to play a vital role in seed oil accumulation in Arabidopsis (Zou et al., 1999). To detect the molecular mechanism for the oil content improvement in the VfSAD1/2-OE lines, the gene expression patterns of AtWRI1 and AtDGAT1 were detected in the developing silique of Arabidopsis. The developing siliques were sampled at 2, 6, 8, and 10 days after flowering (DAF). The VfSAD1/2 showed high expression in the siliques of VfSAD1/2-OE lines at the four development periods (Figures 4E, F). As shown in Figures 4G–J, the expression levels of the AtWRI1 and AtDGAT1 in the siliques of VfSAD1/2-OE lines were all significantly upregulated in at least two of the four development periods in comparison to that in the fad3fae1 line. The results indicated that the improved oil content in the VfSAD1/2-OE lines should be due to the upregulation of the genes involved in the oil biosynthesis pathway.
3.6 VfSAD1 and VfSAD2 could enhance the tolerance of yeast and Arabidopsis seedlings to the low temperature stress
The increase in unsaturated FAs content in the cell membrane enhanced the membrane lipid fluidity and thus could increase the tolerance of the cell to the low temperature (Xiao et al., 2022). The results above showed that the overexpression of VfSAD1/2 promoted the production of oleic acid and/or its derivation in the BY4741 and Arabidopsis. The optimal growth temperature for yeast is 28°C–30°C. In order to detect the tolerance of transgenic BY4741 strain with the overexpression of the VfSAD1 or VfSAD2 gene to the low temperature, two temperature values, 17°C and 30°C, were selected for the experiment. At 30°C, there was no difference in growth performance between wild-type BY4741 and transgenic BY4741 at six diluted concentrations (Figure 5A). However, under the condition of 17°C, the growth activity of transgenic BY4741 was significantly better than that of the wild type (Figure 5B).
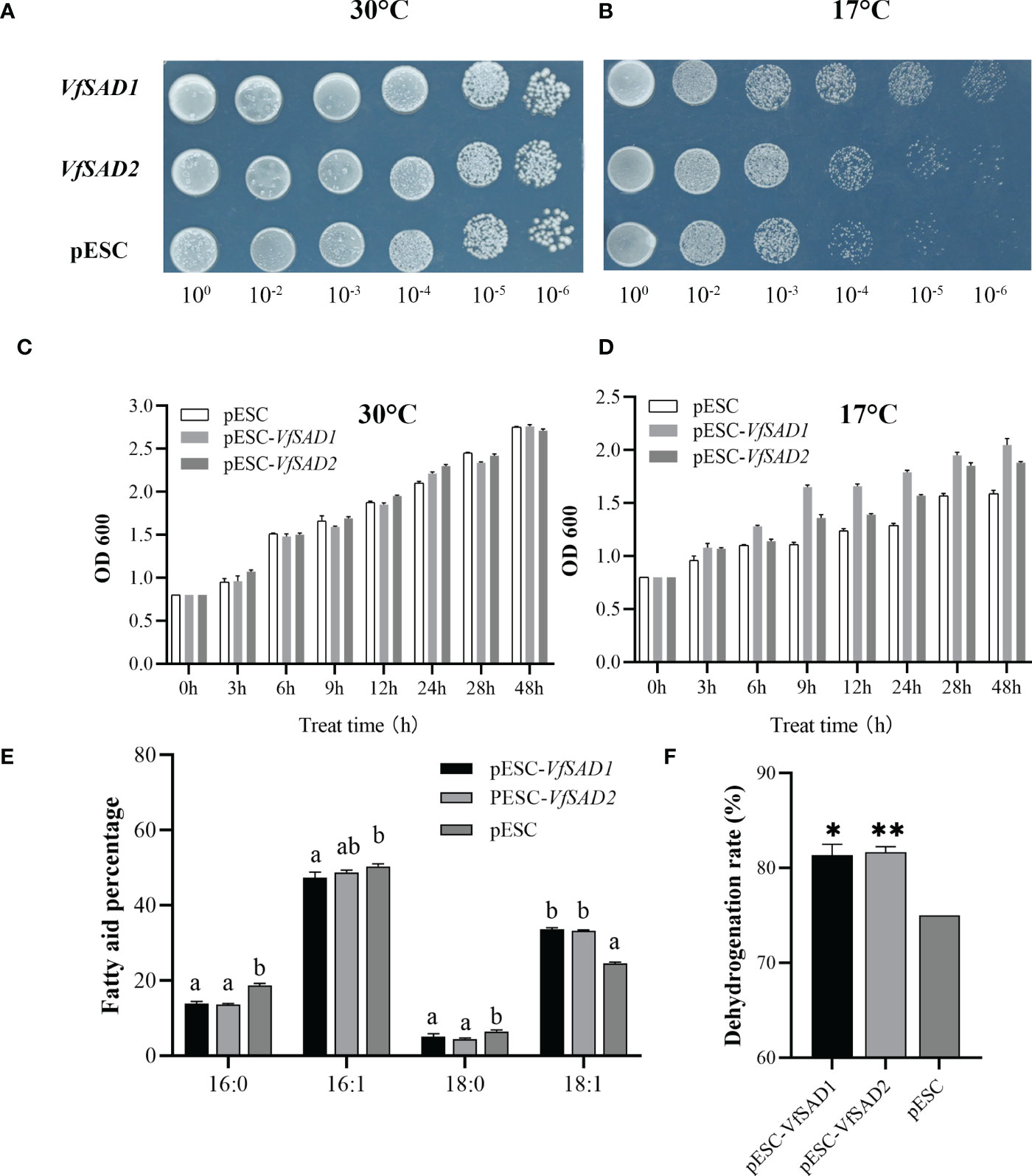
Figure 5 VfSAD1 and VfSAD2 enhance the tolerance of yeast to low temperature stress. (A, B) Yeast colonies at 30°C (A) and 17°C (B); (C, D) OD values of yeast liquid cultures treated at 30°C (C) and 17°C (D); (E) fatty acid fractions in the BY4741 treated at 17°C; (F) conversion of unsaturated fatty acids in the BY4741 treated at 17°C. The error bar represents the standard deviation (± SD) for setting three biological replicates. Significant difference was detected by t-test (* indicates p< 0.05; ** indicates p< 0.01). A comparison between groups was conducted by one-way ANOVA test (FA component), and the different letters above the bar chart indicate significant differences (p< 0.05).
Furthermore, the time-course optical density (OD) value of the two kinds of yeast was measured. The initial OD values of the two types of yeast were adjusted to 0.8. At 30°C, there was no obvious difference in OD value between the two types of yeasts (Figure 5C) at the different growth stages. The OD values of transgenic BY4741 containing VfSAD1 or VfSAD2 were obviously lower at 17°C than that at 30°C since growth for 6 h. However, the OD values of transgenic BY4741 containing VfSAD1 or VfSAD2 were significantly higher than that of the wild-type BY4741 at each sampling time at the 17°C (Figure 5D). The results reflected that the low temperature decreased the growth rate of yeast, but the overexpression of VfSAD1/2 could partly recover the growth rate of yeast. The oleic acid percentage in the transgenic BY4741 containing VfSAD1 or VfSAD2 was increased by 10% than that in the wild-type BY4741 at 30°C (Figure 3A). Here, at 17°C, the oleic acid was further increased by 13.7% in the transgenic BY4741 containing VfSAD1 or VfSAD2 than that in the wild-type BY4741, and other three fatty acids, the palmitic acid, palmitoleic acid, and saturated acid, were all significantly decreased than that in the wild-type BY4741 (Figures 5E, F). This result reflected that more oleic acid molecules were produced in the transgenic BY4741 under low temperature.
To understand the biological function of VfSAD1/2 in regulating cold tolerance, three VfSAD1/2-overexpressing (OE) Arabidopsis lines with relatively high expression were selected for assessing cold tolerance. No conspicuous difference in phenotype was observed between transgenic Arabidopsis plants and WT under normal conditions (Figures 6A, B). After the treatment at −8°C for 90 min, the wild Arabidopsis leaves showed severe wilting, whereas the VfSAD1-OE Arabidopsis leaves exhibited slight wilting. In the subsequent 3 days of recovery culture, the wild-type Arabidopsis plants almost died (Figures 6A, C), but the VfSAD1-OE Arabidopsis grew larger. The average survival rate of the three strains of VfSAD1 was 98%, 98.1%, and 91.75%, respectively, and the average survival rate of WT was 36.1% (Figure 6B). Little difference was observed between transgenic Arabidopsis plants and WT after the treatment at −8°C for 90 min. Thus, the VfSAD2-OE Arabidopsis was treated at −8°C for 2 h and then recovered at 24°C for 3 days. The phenotype of the VfSAD2-OE Arabidopsis to the low temperature was similar to that of the VfSAD1-OE Arabidopsis. The average survival rate of the three transgenic plant lines was 79.4%, 88%, and 16%, respectively, and the average survival rate of the WT was 30.5% (Figure 6D). Therefore, tolerance to low temperature was significantly improved in the 7-day-old plantlet of A. thaliana with overexpression of VfSAD1 or VfSAD2 gene (Figure 6). The results above indicated that under low temperature conditions, the overexpression of the VfSAD1 or VfSAD2 could improve the tolerance of the cell to the low temperature stress.
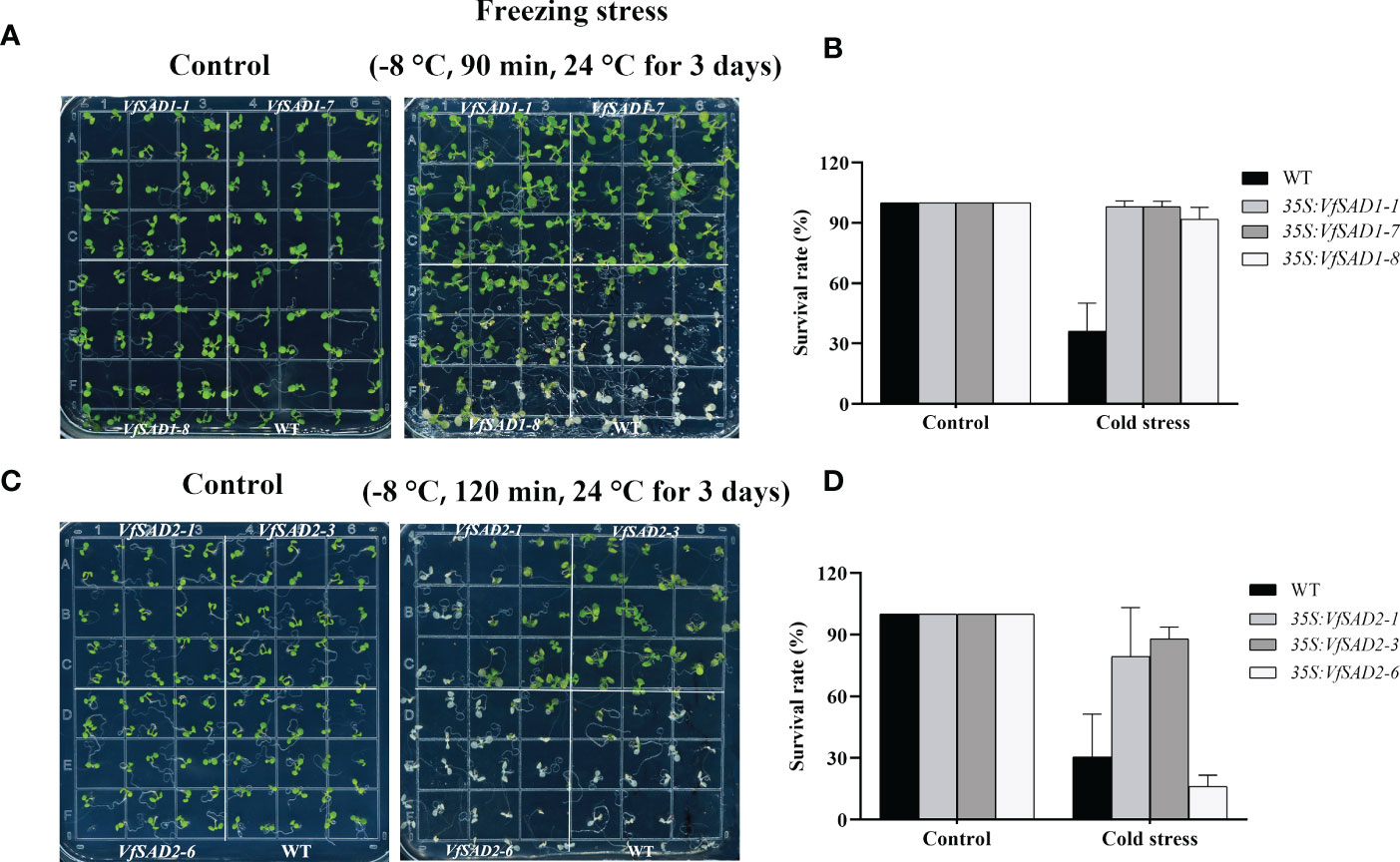
Figure 6 VfSAD1 and VfSAD2 enhance the tolerance of Arabidopsis seedlings to low temperature stress. (A, B) Phenotypes (A) and survival rates (B) of the VfSAD1-overexpressing and WT Arabidopsis plants under normal and freezing treatments (−8°C for 90 min); (C, D) phenotypes (C) and survival rates (D) of the VfSAD2-overexpressing and WT Arabidopsis plants under normal and freezing treatments (−8°C for 120 min). The error bar represents the standard deviation (± SD) for setting three biological replicates.
4 Discussion
There are usually one to four members of the SAD gene for each plant species according to other existing studies. In this study, we identified three SAD members using the genome data, but only two were upregulated in the lipid synthesis pathway, so we annotated and downloaded the sequences of VfSAD1 and VfSAD2 using genomic data to focus on their functional characterization (Zhang et al., 2019). Phylogenetic analysis showed that SAD1 and SAD2 genes were clustered into different groups, suggesting genetic differentiation between SAD1 and SAD2, which is consistent with the report of Li et al. (2022). Despite the genetic differentiation between the SAD1 and SAD2, we observed that they displayed similar functions in fatty acid biosynthesis. Overexpression of VfSAD1 or VfSAD2 can improve oleic acid content and cold resistance in transgenic yeast and Arabidopsis. During low-temperature acclimation of higher plants, polyunsaturated FAs have been found to increase in many plant species (De Palma et al., 2008; Wang et al., 2013). The researchers found that the SAD genes were upregulated and the content of unsaturated fatty acid increased in response to the low temperature (Ding et al., 2016; Hernández et al., 2019; Zhou et al., 2019).
The function of a SAD gene is usually considered to catalyze the formation of oleic acid with stearic acid as the substrate. However, we found that overexpression of both VfSADs could increase oil content in addition to oleic acid content in transgenic Arabidopsis. Actually, Kazaz et al. (2020) reported that decreased SAD gene expression leads to the decreased oil content in Arabidopsis seeds. Association analysis further confirmed that GhSAD4 plays an important role in cottonseed oil composition (Shang et al., 2017), which supports our finding that SAD is also involved in oil accumulation. Interestingly, we also found that AtWRI1 and AtDGAT1 were upregulated in VfSAD transgenic Arabidopsis. AtWRI1 and AtDGAT1 are two key genes involved in oil biosynthesis. A WRI1 transcription factor is considered as a “master regulator” that controls the transcription of almost all key enzymes converting sucrose to FA (Liu et al., 2019). For instance, seed oil decreased significantly in Arabidopsis wri1 mutants (An et al., 2017), and overexpression of WRI1 increased seed oil content in several plant species (Snell et al., 2019; Fei et al., 2020; Sanchez et al., 2022). The DGAT protein family catalyzes the final step in triacylglycerol (TAG) biosynthesis, which is considered to be the rate-limiting step in TAG accumulation (Shockey et al., 2006; Cao et al., 2013). According to the study of Zhang et al. (2019), DGAT2 was considered to be the most important DGAT gene for TAG biosynthesis in tung tree seeds. Since the expression of SAD causes the first step of the saturated fatty acid dehydrogenation reaction, we speculate that this reaction may accelerate the whole oil biosynthesis pathway, and then, the expression level of WRI1 and DGAT1 are upregulated.
In addition, we noted that VfSAD1 showed a higher expression level than VfSAD2 in developing tung tree seeds, suggesting that VfSAD1 may play a more important role than VfSAD2 in oil and fatty acid biosynthesis. However, overexpression of the two genes in Arabidopsis generated opposite results, namely, the transgenic Arabidopsis with SAD2 produced higher oil content and oleic acid content than the VfSAD1 carrying Arabidopsis. This inconsistent finding may be due to two reasons. First, expression patterns imply a biological function for genes, while only higher expression does not represent stronger biological function, since the function of genes is also related to the posttranslational modification of proteins. Second, it is generally believed that the integration site of transgenic gene in the host chromosome is random. Exogenous DNA can be inserted into any chromosome of the plant genome, or any site of a chromosome, or there is no fixed insertion site, but it often has priority insertion characteristics for the transcription active region. Thus, the phenotype of transgenic lines is not only related to the function of exogenous genes but also to the insertion sites in the host genome. To compare the significance of the two VfSADs in oil and fatty acid biosynthesis, in fact, gene knockout dependent on genetic transformation system is the most efficient method. This transformation system, however, has not yet been resolved. Based on our present results, both VfSADs seem to play a key role with almost identical efficiency in oil and fatty acid biosynthesis. In our previous study, we have developed a method of Agrobacterium rhizogenes-mediated transgenic hairy root induction from the stem of tung tree (Jia et al., 2022), which laid an important foundation for the construction of a genetic transformation system. The two VfSADs could be used for tung tree genetic improvements, e.g., increase seed oil content and oleic acid proportion or improve cold resistance once the genetic transformation system of tung tree is successfully established in the future.
5 Conclusion
The two predicted VfSAD1 and VfSAD2 were highly expressed in the seed of tung tree during the oil rapid accumulation period. The function of two predicted SAD genes was investigated in Arabidopsis and yeast. The results showed that the VfSAD1 and VfSAD2 are the stearoyl-ACP desaturases. It could improve the lipid accumulation in Arabidopsis seed and yeast and enhance the tolerance of yeast and Arabidopsis seedlings to the low temperature stress. This study indicated that the two SAD genes should play a vital role in the process of oil accumulation in the seed of tung tree.
Data availability statement
The datasets presented in this study can be found in online repositories. The names of the repository/repositories and accession number(s) can be found in the article/Supplementary Material.
Author contributions
LZ and LLZ contributed to conception and design of the study. LZ contributed to supervision and writing—reviewing and editing. JC organized the database. JG performed the statistical analysis by using software. JC wrote the first draft of the manuscript and performed validation and visualization. LZ, LLZ, and JC wrote sections of the manuscript. All authors contributed to manuscript revision, read, and approved the submitted version.
Funding
This work was supported by the National Natural Science Foundation of China (NSFC) (Grant Nos. 31971692 and 31500267), the Youth Innovation Promotion Association of the Chinese Academy of Sciences (2021–2024), and National Key R&D Program of China (Grant No. 2017YFD0600703).
Conflict of interest
The authors declare that the research was conducted in the absence of any commercial or financial relationships that could be construed as a potential conflict of interest.
Publisher’s note
All claims expressed in this article are solely those of the authors and do not necessarily represent those of their affiliated organizations, or those of the publisher, the editors and the reviewers. Any product that may be evaluated in this article, or claim that may be made by its manufacturer, is not guaranteed or endorsed by the publisher.
Supplementary material
The Supplementary Material for this article can be found online at: https://www.frontiersin.org/articles/10.3389/fpls.2023.1144853/full#supplementary-material
References
An, D., Kim, H., Ju, S., Go, Y. S., Kim, H. U., Suh, M. C. (2017). Expression of camelina WRINKLED1 isoforms rescue the seed phenotype of the arabidopsis wri1 mutant and increase the triacylglycerol content in tobacco leaves. Front. Plant Sci. 8. doi: 10.3389/fpls.2017.00034
Cao, H. P., Shockey, J. M., Klasson, K. T., Chapital, D. C., Mason, C. B., Scheffler, B. E. (2013). Developmental regulation of diacylglycerol acyltransferase family gene expression in tung tree tissues. PloS One 8 (10), e76946. doi: 10.1371/journal.pone.0076946
Cui, P., Lin, Q., Fang, D. M., Zhang, L. L., Li, R. J., Cheng, J. Y., et al. (2018). Tung tree (Vernicia fordii, hemsl.) genome and transcriptome sequencing reveals Co-ordinate up-regulation of fatty acid beta-oxidation and triacylglycerol biosynthesis pathways during eleostearic acid accumulation in seeds. Plant Cell Physiol. 59 (10), 1990–2003. doi: 10.1093/pcp/pcy117
De Palma, M., Grillo, S., Massarelli, I., Costa, A., Balogh, G., Vigh, L., et al. (2008). Regulation of desaturase gene expression, changes in membrane lipid composition and freezing tolerance in potato plants. Mol. Breed. 21 (1), 15–26. doi: 10.1007/s11032-007-9105-y
Ding, Z. T., Shen, J. Z., Pan, L. L., Wang, Y. U., Li, Y. S., Wang, Y., et al. (2016). CsSAD: a fatty acid desaturase gene involved in abiotic resistance in camellia sinensis (L.). Genet. Mol. Res. 15 (1), 15017512. doi: 10.4238/gmr.15017512
Dyer, J. M., Chapital, D. C., Kuan, J. C., Mullen, R. T., Turner, C., McKeon, T. A., et al. (2002). Molecular analysis of a bifunctional fatty acid conjugase/desaturase from tung. implications for the evolution of plant fatty acid diversity. Plant Physiol. 130 (4), 2027–2038. doi: 10.1104/pp.102.010835
Fei, W., Yang, S., Hu, J., Yang, F., Qu, G., Peng, D., et al. (2020). Research advances of WRINKLED1 (WRI1) in plants. Funct. Plant Biol. 47 (3), 185–194. doi: 10.1071/fp19225
Galli, V., Guzman, F., Messias, R. S., Koerbes, A. P., Silva, S. D. A., Margis-Pinheiro, M., et al. (2014). Transcriptome of tung tree mature seeds with an emphasis on lipid metabolism genes. Tree Genet. Genomes 10 (5), 1353–1367. doi: 10.1007/s11295-014-0765-6
Hernández, M. L., Sicardo, M. D., Alfonso, M., Martínez-Rivas, J. M. (2019). Transcriptional regulation of stearoyl-acyl carrier protein desaturase genes in response to abiotic stresses leads to changes in the unsaturated fatty acids composition of olive mesocarp. Front. Plant Sci. 10. doi: 10.3389/fpls.2019.00251
Huang, X., Cao, L., Fan, J., Ma, G., Chen, L. (2022). CdWRKY2-mediated sucrose biosynthesis and CBF-signalling pathways coordinately contribute to cold tolerance in bermudagrass. Plant Biotechnol. J. 20 (4), 660–675. doi: 10.1111/pbi.13745
Jia, H., Chen, J., Zhang, L., Zhang, L. (2022). The first report on transgenic hairy root induction from the stem of tung tree (Vernicia fordii). Plants-Basel 11 (10), 1315. doi: 10.3390/plants11101315
Kazaz, S., Barthole, G., Domergue, F., Ettaki, H., To, A., Vasselon, D., et al. (2020). Differential activation of partially redundant Delta9 stearoyl-ACP desaturase genes is critical for omega-9 monounsaturated fatty acid biosynthesis during seed development in arabidopsis. Plant Cell 32 (11), 3613–3637. doi: 10.1105/tpc.20.00554
Li, L., Li, Y., Wang, R., Chao, L., Xiu, Y., Wang, H. (2020). Characterization of the stearoyl-ACP desaturase gene (PoSAD) from woody oil crop paeonia ostii var. lishizhenii in oleic acid biosynthesis. Phytochemistry 178, 112480. doi: 10.1016/j.phytochem.2020.112480
Li, T., Sun, Y., Chen, Y., Gao, Y., Gao, H., Liu, B., et al. (2022). Characterisation of two novel genes encoding delta 9 fatty acid desaturases (CeSADs) for oleic acid accumulation in the oil-rich tuber of cyperus esculentus. Plant Sci. 319, 111243. doi: 10.1016/j.plantsci.2022.111243
Li-Beisson, Y., Shorrosh, B., Beisson, F., Andersson, M. X., Arondel, V., Bates, P. D., et al. (2013). Acyl-lipid metabolism. Arabidopsis Book 11, e0161. doi: 10.1199/tab.0161
Liu, H., Zhai, Z., Kuczynski, K., Keereetaweep, J., Schwender, J., Shanklin, J. (2019). WRINKLED1 regulates BIOTIN ATTACHMENT DOMAIN-CONTAINING proteins that inhibit fatty acid Synthesis1 OPEN. Plant Physiol. 181 (1), 55–62. doi: 10.1104/pp.19.00587
Livak, K. J., Schmittgen, T. D. (2001). Analysis of relative gene expression data using real-time quantitative PCR and the 2(-delta delta C(T)) method. Methods 25 (4), 402–408. doi: 10.1006/meth.2001.1262
Nam, J. W., Lee, H. G., Do, H., Kim, H. U., Seo, P. J. (2022). Transcriptional regulation of triacylglycerol accumulation in plants under environmental stress conditions. J. Exp. Bot. 73 (9), 2905–2917. doi: 10.1093/jxb/erab554
Sanchez, R., Gonzalez-Thuillier, I., Venegas-Caleron, M., Garces, R., Salas, J. J., Martinez-Force, E. (2022). The sunflower WRINKLED1 transcription factor regulates fatty acid biosynthesis genes through an AW box binding sequence with a particular base bias. Plants-Basel 11 (7), 972. doi: 10.3390/plants11070972
Shang, X., Cheng, C., Ding, J., Guo, W. (2017). Identification of candidate genes from the SAD gene family in cotton for determination of cottonseed oil composition. Mol. Genet. Genomics 292 (1), 173–186. doi: 10.1007/s00438-016-1265-1
Shockey, J. M., Gidda, S. K., Chapital, D. C., Kuan, J. C., Dhanoa, P. K., Bland, J. M., et al. (2006). Tung tree DGAT1 and DGAT2 have nonredundant functions in triacylglycerol biosynthesis and are localized to different subdomains of the endoplasmic reticulum. Plant Cell 18 (9), 2294–2313. doi: 10.1105/tpc.106.043695
Shockey, J., Regmi, A., Cotton, K., Adhikari, N., Browse, J., Bates, P. D. (2016). Identification of arabidopsis GPAT9 (At5g60620) as an essential gene involved in triacylglycerol biosynthesis. Plant Physiol. 170 (1), 163–179. doi: 10.1104/pp.15.01563
Snell, P., Grimberg, A., Carlsson, A. S., Hofvander, P. (2019). WRINKLED1 is subject to evolutionary conserved negative autoregulation. Front. Plant Sci. 10. doi: 10.3389/fpls.2019.00387
To, A., Joubes, J., Barthole, G., Lecureuil, A., Scagnelli, A., Jasinski, S., et al. (2012). WRINKLED transcription factors orchestrate tissue-specific regulation of fatty acid biosynthesis in arabidopsis. Plant Cell 24 (12), 5007–5023. doi: 10.1105/tpc.112.106120
van Erp, H., Shockey, J., Zhang, M., Adhikari, N. D., Browse, J. (2015). Reducing isozyme competition increases target fatty acid accumulation in seed triacylglycerols of transgenic arabidopsis. Plant Physiol. 168 (1), 36–46. doi: 10.1104/pp.114.254110
Wang, H., Cao, F., Zhang, W., Wang, G., Yu, W. (2013). Cloning and expression of stearoyl-ACP desaturase and two oleate desaturases genes from ginkgo biloba l. Plant Mol. Biol. Rep. 31 (3), 633–648. doi: 10.1007/s11105-012-0525-4
Wu, P., Zhang, L. L., Feng, T., Lu, W. Y., Zhao, H. Y., Li, J. Z., et al. (2018). A conserved glycine is identified to be essential for desaturase activity of IpFAD2s by analyzing natural variants from idesia polycarpa. Int. J. Mol. Sci. 19 (12), 3932. doi: 10.3390/ijms19123932
Xiao, R. X., Zou, Y. R., Guo, X. R., Li, H., Lu, H. (2022). Fatty acid desaturases (FADs) modulate multiple lipid metabolism pathways to improve plant resistance. Mol. Biol. Rep. 49 (10), 9997–10011. doi: 10.1007/s11033-022-07568-x
Yang, Y., Kong, Q., Lim, A. R. Q., Lu, S., Zhao, H., Guo, L., et al. (2022). Transcriptional regulation of oil biosynthesis in seed plants: Current understanding, applications, and perspectives. Plant Commun. 3 (5), 100328. doi: 10.1016/j.xplc.2022.100328
Yurchenko, O., Shockey, J. M., Gidda, S. K., Silver, M. I., Chapman, K. D., Mullen, R. T., et al. (2017). Engineering the production of conjugated fatty acids in arabidopsis thaliana leaves. Plant Biotechnol. J. 15 (8), 1010–1023. doi: 10.1111/pbi.12695
Zhang, L., Liu, M., Long, H., Dong, W., Pasha, A., Esteban, E., et al. (2019). Tung tree (Vernicia fordii) genome provides a resource for understanding genome evolution and improved oil production. Genomics Proteomics Bioinf. 17 (6), 558–575. doi: 10.1016/j.gpb.2019.03.006
Zhang, L., Wu, P., Li, W., Feng, T., Shockey, J., Chen, L., et al. (2021). Triacylglycerol biosynthesis in shaded seeds of tung tree (Vernicia fordii) is regulated in part by homeodomain leucine zipper 21. Plant J. 1086), 1735-1753 doi: 10.1111/tpj.15540
Zhang, L. L., Wu, P., Lu, W. Y., Lu, S. Y. (2018). Molecular mechanism of the extended oil accumulation phase contributing to the high seed oil content for the genotype of tung tree (Vernicia fordii). BMC Plant Biol. 18(1), 248. doi: 10.1186/s12870-018-1458-3
Zhou, R., Liu, P., Li, D., Zhang, Y., Wang, L., Zhang, X., et al. (2019). Cloning and functional characterization of sesame SiSAD gene. Sci. Agricult. Sin. 52 (10), 1678–1685. doi: 10.3864/j.issn.0578-1752.2019.10.002
Keywords: tung tree (Vernicia fordii), stearoyl-acyl carrier prote in Δ9 desaturase (SAD), oleic Acid, the α-eleostearic acid, oil accumulation
Citation: Chen J, Gao J, Zhang L and Zhang L (2023) Tung tree stearoyl‐acyl carrier protein Δ9 desaturase improves oil content and cold resistance of Arabidopsis and Saccharomyces cerevisiae. Front. Plant Sci. 14:1144853. doi: 10.3389/fpls.2023.1144853
Received: 15 January 2023; Accepted: 20 February 2023;
Published: 07 March 2023.
Edited by:
Junhua Peng, Spring Valley Agriscience Co., Ltd, ChinaReviewed by:
Yu Wang, Northeast Forestry University, ChinaChun Li, Henan Academy of Agricultural Sciences (HNAAS), China
Hua Zhao, Huazhong Agricultural University, China
Jing Ren, Dezhou University, China
Copyright © 2023 Chen, Gao, Zhang and Zhang. This is an open-access article distributed under the terms of the Creative Commons Attribution License (CC BY). The use, distribution or reproduction in other forums is permitted, provided the original author(s) and the copyright owner(s) are credited and that the original publication in this journal is cited, in accordance with accepted academic practice. No use, distribution or reproduction is permitted which does not comply with these terms.
*Correspondence: Lin Zhang, triwoodtim918@126.com; Lingling Zhang, zhanglingling@wbgcas.cn