- 1Henan Academy of Crop Molecular Breeding, Henan Academy of Agricultural Sciences, Zhengzhou, China
- 2Qiqihar Branch of Heilongjiang Academy of Agricultural Sciences, Qiqihar, Heilongjiang, China
- 3College of Agricultural, State Key Laboratory for Conservation and Utilization of Subtropical Agro-Bioresources, Guangxi University, Nanning, China
- 4National Centre for Plant Breeding, Xinxiang, China
Soybean cyst nematode (SCN, Heterodera glycines, HG) is one of the severe pests in plant-parasitic nematodes, which impairs root development and causes severe losses in soybean production worldwide. Breeding SCN-resistant cultivars is an important measure for securing harvests without affecting the environment, as can be done with pesticides. The majority of genetic resources for plant pest resistances are found in wild or closely related species which are often difficult to use in breeding due to crossing barriers or close linkage with unfavorable agronomic traits. In this study, 12 soybean cultivars were evaluated for their marker haplotype at the rhg1 and Rhg4 SCN resistance loci and their SCN resistance tested against multiple races in environmentally controlled bioassays. The results showed that all 12 cultivars displayed Peking-type resistance marker haplotypes and all of them proved to be resistant to multiple SCN races. These cultivars provide potential for improving H. glycines resistance of soybean as donor parent in breeding and can contribute to reduce SCN field populations as part of a sustainable agriculture management.
Introduction
Soybean (Glycine max) plays an important role in agricultural production (Hannah Ritchie and Roser, 2021), which is not only the source of vegetable protein and edible oil for human food but also the source of feed for animals and use in industrial processes. Plant pests and diseases are important factors limiting soybean yield, and the soybean cyst nematode (SCN, Heterodera glycines, HG) is one of the major plant-parasitic nematodes in soybean production. The yield losses caused by SCN in soybean production range from 10 to 30% on average each year (Koenning and Wrather, 2010; Bradley et al., 2021; Peng et al., 2021).
SCNs invade the soybean root as juveniles. The female larvae initiate in the root vascular cylinder, a so-called syncytium as a unique feeding site providing the nutrition for the nematode’s further growth and development (Klink et al., 2009). Through a feeding tube which is associated at the end of the nematode stylet and connected to the endoplasmic reticulum (ER) of the developing syncytium, the nematode secretes small molecules into the syncytium (Klink et al., 2007; Ali et al., 2013; Holbein et al., 2016). Subsequently, transcriptional and metabolic reprogramming invokes the compatible or incompatible interaction of the soybean host with the SCN. In incompatible interactions, the nematode’s development is arrested in the second-stage juvenile (J2) or third-stage juvenile (J3). In compatible interactions, the nematode can complete its life cycle within 4–5 weeks and turn into a solid cyst filled with hundreds of eggs after a male fertilized the female (Sobczak et al., 1999; Holbein et al., 2016; Mitchum, 2016).
Breeding soybean varieties with both high level of resistance and high yield offers an economic and ecological sustainable alternative to control SCN. In recent years, several reports from Missouri, USA, and the Huang-Huai Valley, China (Lian et al., 2016; Howland et al., 2018; Meinhardt et al., 2021) showed that formerly predominant SCN races shifted into more virulence races by cultivar-depending selection and that this evolution is expected to happen in many important soybean-growing areas. Even new virulent populations can develop like X12, which is a highly virulent SCN population found in Shanxi province, China (Lian et al., 2017; Lian et al., 2019b).
Although chemical and biological control agents including fungi and bacteria have been identified to be effective to manage this pest, environmental and economic concerns limit these approaches (Lin et al., 2022). Moreover, soybean is still being cultivated in small areas with low technology input or in fields with weak soil fertility by marginal farmers like in some parts of China. Thus, most of the management strategies for controlling SCN are seldom followed by such farmers or in soybean productions with low yield and revenue.
Therefore, it is desirable to identify resistance sources with broad-spectrum resistance to SCN and favorable agronomic traits, especially for yield traits, and utilize them to breed new resistant cultivars for long-term management of this devastating pest. Researchers tried to introduce strong SCN resistance sources such as PI 437654 and ZDD 2315 (Lian et al., 2017), but practical benefits from such germplasms were low due to the close linkage between resistance genes and adverse agronomic traits. So far, only very few accessions, like PI 88788 (around 90% of all resistant varieties are based on this resistance source), PI 209332, and Peking, were successfully used in the commercial soybean varieties for SCN resistance (Winter et al., 2006; Mitchum, 2016). However, more and more reports have shown that the sole use of resistances with single resistant genes such as PI 88788 is inefficient over time due to the development or selection of virulent nematode field populations (Niblack et al., 2008; Mitchum, 2016; Lin et al., 2022).
After years of research for the identification of SCN resistance resources with high agronomical value, a total of 12 soybean cultivars with multiple SCN resistances were identified in advanced breeding germplasms from China and in cultivars submitted for the national/provincial variety registration system in China. These cultivars have relatively high yield compared with Peking, Pickett, PI 88788, etc., and they are not derived from the single locus (rhg1) resistance source PI 88788. These results provide valuable genotypic and phenotypic information and methods for developing elite SCN resistant cultivars.
Materials and methods
Plant materials
12 SCN cultivars were previously identified as SCN resistant from larger collections of cultivar candidates or known cultivars. Zhonghuang 57, Handou 10, and Cangdou 11 derived from Huang-Huai Valley, China. Zhonghuang 57 and Handou 10 are known resistant cultivars with resistance to race 2 (Lian et al., 2019a; Wei et al., 2022). Cangdou 11 is screened by SCN bioassays with race 1 from a collection of 640 cultivar candidates. The other nine cultivars used in this study derived from Northeast China. Heinong 531 is a known resistant cultivar with resistance to race 3 (Wang et al., 2022). Heinong 84 is a cultivar showing rgh1 and Rgh4 resistance marker loci (Lian et al., 2022). Fengdou 1, Nenfeng 15, Nenfeng 17 (Chen et al., 2023), Nenfeng 18 (Chen et al., 2023), Nenfeng 19, Nongqingdou 24, and Qinong 5 derived from soybean breeder Dr. M. Yuan and were selected with race 3 in the field. The resistant and susceptible control cultivars Peking, PI 88788, PI 437654, and Lee were maintained by the soybean research group at the Henan Academy of Agricultural Sciences, Institute for Crops Molecular Breeding, Zhengzhou, China.
SCN purification and inbreeding, propagation, and maintenance
Single SCN cysts were isolated from previously race tested soil samples (Lian et al., 2019b), which were selected for their potentially high uniformity to be infected with a single SCN race. Single cysts were used to inoculate susceptible Lee seedlings growing in soil sterilized for 2 h at 120°C. After four times of single-cyst propagation, SCN populations were considered as sufficiently inbred and race uniformity confirmed by race testing according to the SCN race model of Riggs (Riggs and Schmitt, 1988). Individual race populations of race 1, 2, 3, 4, and 5 were separately bulk multiplied several times, and cysts were stored in sterilized soil at 16°C in a dark room. The race populations were maintained in greenhouse culture pots on susceptible Lee plants, growing in soil from previous maintenance cultivation. SCN race 1, 2, 3, 4, and 5 are the most common races in the soybean production areas of China and worldwide (Lian et al., 2016).
Nematode egg preparation
The adult SCN female cysts were washed off with pressure water from 30-day-old soybean roots placed on an 850-µm-pore sieve nested over a 250-µm-pore sieve. The eggs were released from inside the cysts using a rubber stopper on the surface of a 250-µm-pore sieve nested over a 210-µm-pore and an additional 25-µm-pore sieve (Faghihi and Ferris, 2000). Eventually, the eggs were collected on the 25-µm-pore sieve. Sucrose centrifugation with 20% sucrose/water solution was applied to separate the eggs from debris (Jenkins, 1964). The eggs were rinsed with water on a 25-µm-pore sieve to remove the sucrose solution and purified at 2,500 rpm. The eggs’ concentration was adjusted to 1,250 eggs/ml before use in experiments.
Nematode resistance tests
The seeds used for resistance tests were germinated for 3 days in vermiculite at 25°C in the dark. The inoculation and infection of SCN to the soybean seedlings were carried out around 4 days after transferring single, germinated seedlings into cups (upper diameter × lower diameter × height: 70 mm × 50 mm × 90 mm) filled with sterilized soil. Lee was used as the SCN susceptible control. A total of 2,500 eggs were added with a membrane pump to each plant root by applying two times within 24 h 1,250 eggs with each 1-ml inoculation solution (eggs in water). Four weeks after infection, the roots were harvested and the cysts washed off as described above. The collected cysts were counted under an Olympus SZX16 stereomicroscope (Olympus, Japan). The average number of cysts per plant was calculated over five plants per soybean accession in each experiment and finally over three completely replicated experiments. A female index (FI) was calculated by dividing the average number of cysts on Lee (susceptible control) with the average number of cysts for the test accession (cultivar) multiplied by 100 for each experiment (replication), and finally, the overall average FI was calculated over the replications. According to Riggs and Schmitt (1988), a cultivar was designated resistant to SCN if the FI was ≤10%, whereas the cultivar was designated as moderate resistant, moderate susceptible, and susceptible to SCN with the FI values in the range of 10% < FI ≤ 30%, 30% < FI ≤ 60%, and FI > 60%, respectively.
Molecular marker analysis of the SCN resistance loci rhg1/Rhg4
rhg1 and Rhg4 are the only two QTLs in soybean that have been consistently mapped in a variety of soybean germplasm sources so far, and the involved genes were identified and sequenced (Liu et al., 2012; Liu et al., 2017; Peng et al., 2021). These are also the two most prominent major resistance QTLs used in commercial germplasm (Peng et al., 2021; Lin et al., 2022). Kompetitive allele-specific PCR (KASP) markers for rgh1 and Rgh4, developed by Shi et al. (2015), were applied to scan the presence/absence of these two major resistance QTLs in our cultivar collection. Of each cultivar, three plants were subjected to the marker analysis. Susceptible control cultivar Lee and the resistance control germplasms Peking, PI 88788, and PI437654 were used as marker control. Plants were genotyped with the two markers GSM381 and GSM383 for the rhg1 locus on chromosome 18 and marker GSM191 for the Rhg4 locus on chromosome 8 to determine the SCN resistance marker haplotype.
Results
Main agronomic traits of the 12 cultivars
The 12 cultivars were derived from Northeast China or the Huang-Huai Valley in Central China, the two major soybean-producing areas in China, and were registered as cultivars between 2004 and 2021 (Table 1). According to the official cultivar registration data, the cultivars Heinong 531, Fengdou 1, Nenfeng 15, Nenfeng 19, and Nongqingdou 24 are resistant to race 3; Zhonghuang 57 is displayed as SCN resistant but without any information about the test race; Nenfeng 15, Nenfeng 18, Nenfeng 19, Qinong 5, Handou 10, and Handou 11 do not have SCN testing results. The soybean yield of the 12 cultivars varied from 1,857 to 3,135 kg/ha in regional trials and from 1,942 to 2,911 kg/ha in prerelease trials. The oil content and protein content varied from 19.6 to 22.9% and from 37.8 to 42.6%, respectively. All the 12 cultivars are yellow seeded and have a higher 100-seed weight between 16 and 23.9 g as required for food use (Table 1). None of these registered varieties with SCN resistance have been well released in the local production area (Duan and Peng, 2014; Peng et al., 2021) except “Heinong 84”, which accounted for 4% of the soybean planting area in Heilongjiang in 2019 (Lian et al., 2022).
Evaluation of the SCN resistance using race 1 to 5
We evaluated the SCN resistance of the 12 cultivars in detail by testing with race 1, race 2, race 3, race 4, and race 5 in separate bioassays (Table 2). All 12 cultivars proved to be resistant to race 1, race 3, and race 5 except for Heinong 84 which was sensitive to race 5. All of the 12 identified cultivars showed various levels of resistance to race 2, i.e., one resistant cultivar, one moderately resistant cultivar, four moderately susceptible cultivars, and six susceptible cultivars. Related to race 4, only Zhonghuang 57 showed a clear resistance reaction, whereas two cultivars were moderately susceptible and the other nine cultivars proved to be susceptible against race 4. The combined race results showed that only Zhonghuang 57 proved resistant against all five races (resistance type like PI 437654), Heinong 531, Fengdou 1, Nenfeng 15, Nenfeng 19, and Nongqingdou 24 which turned out to be resistant to race 1, 3, and 5 (resistance type like Peking), and Heinong 84 resistant to race 1 and 3 (incomplete resistance type like Peking).
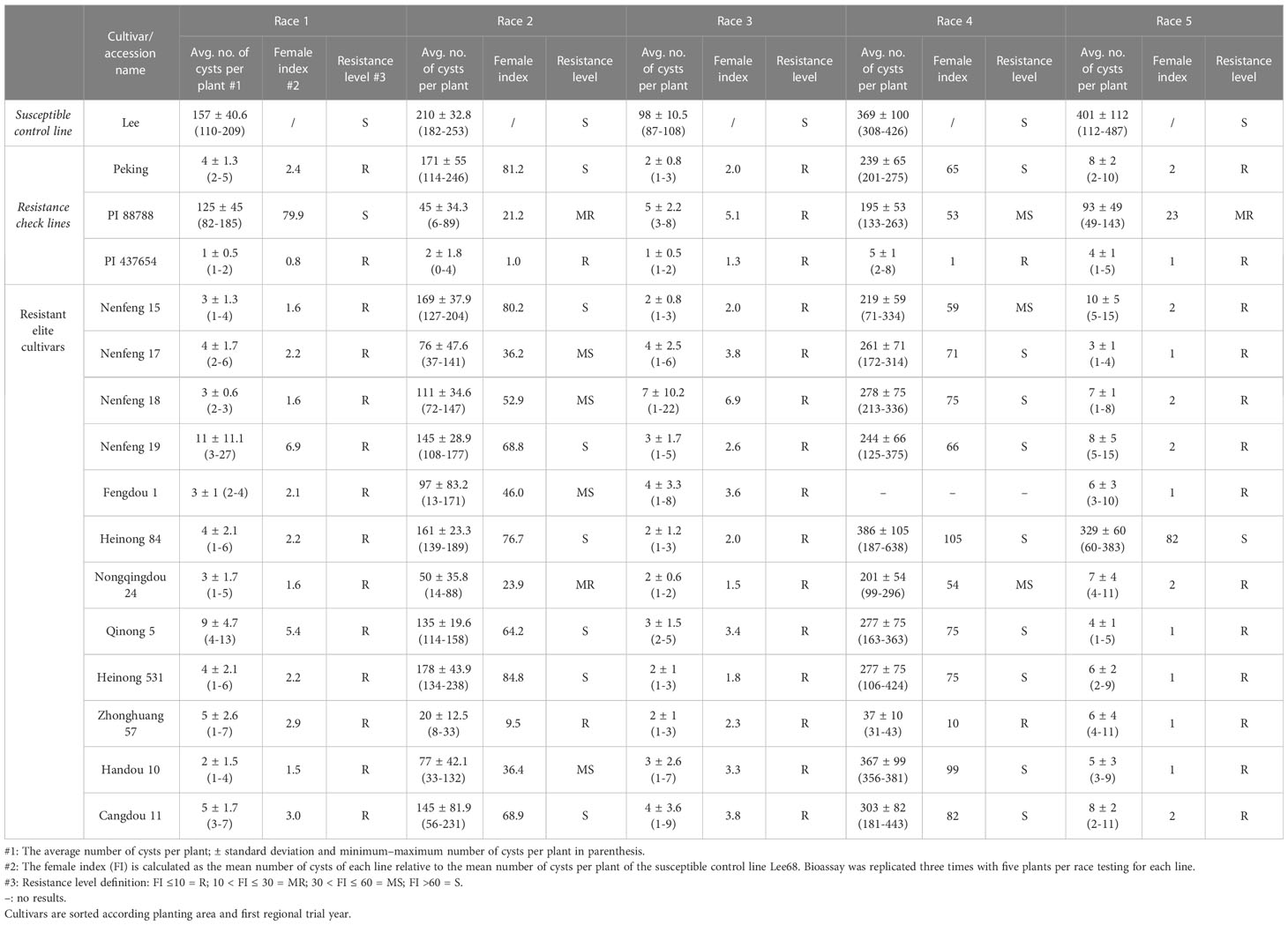
Table 2 The race testing results of the resistant elite cultivars/accessions to five soybean cyst nematode races.
KASP molecular marker assay results
We performed KASP rhg1 and Rhg4 genotyping for the 12 cultivars and the control lines of the bioassay (Table 3). All of the 12 cultivars and the control lines Peking and PI 437654 carry the Peking-source marker haplotype for the rhg1 and Rhg4 resistance alleles (GSM381=G, GSM383=G, GSM191=G).
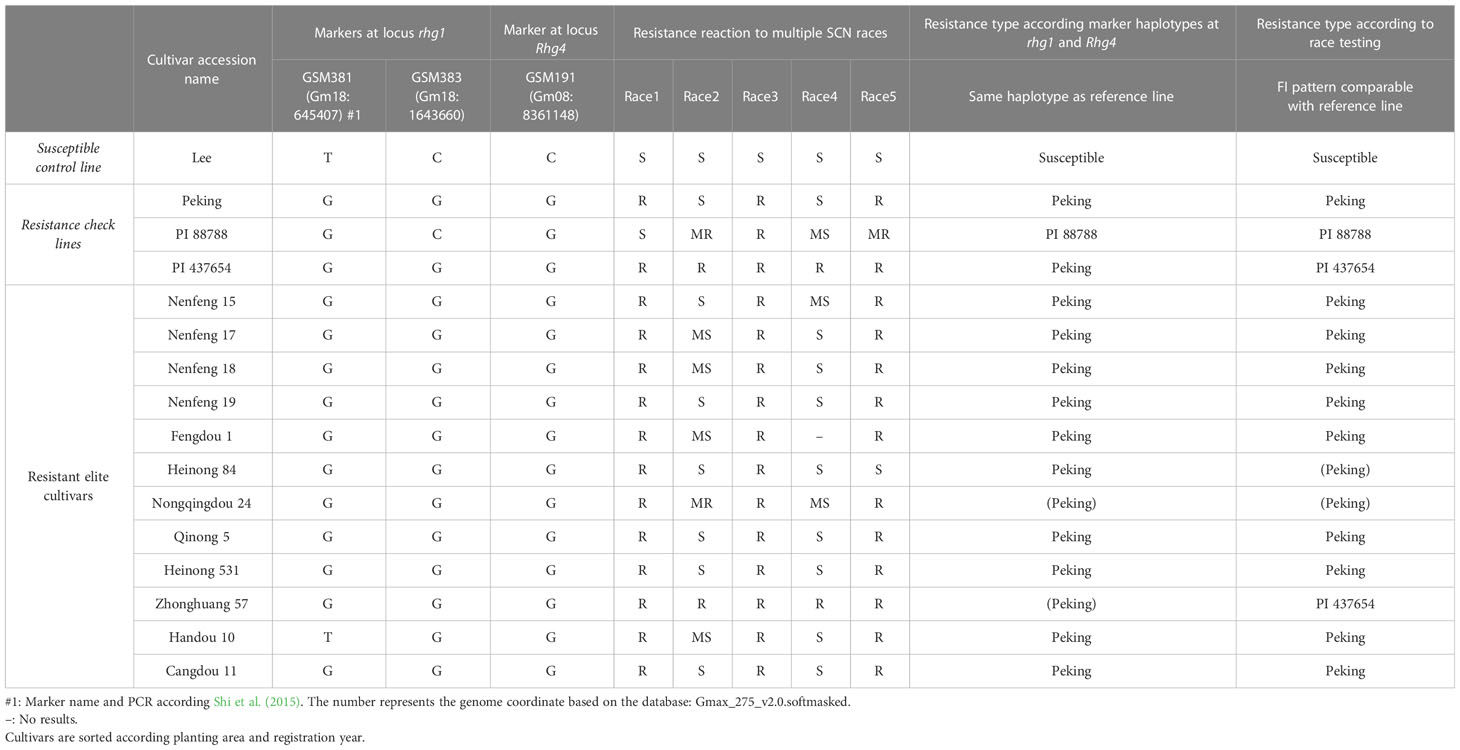
Table 3 Marker haplotypes at SCN loci rhg1 and Rhg4, race testing phenotypes, and the corresponding resistance types of the 12 soybean cultivars and four known soybean reference lines.
Discussion
In this study, 12 cultivars were genotyped for the known SCN resistance QTLs rhg1 and Rhg4 (Concibido et al., 2004; Cook et al., 2012; Liu et al., 2012; Liu et al., 2017). All 12 cultivars belong to the SCN resistance conferring the Peking-type marker haplotype and provide resistance against race 1, race 3, and race 5 (Tables 2 , 3) (Cook et al., 2012; Liu et al., 2012; Cook et al., 2014; Liu et al., 2017). Furthermore, in environmentally controlled SCN bioassays, the 12 cultivars were additionally phenotyped for resistance against SCN race 1–5 and all cultivars showed resistance against race 1, race 3, and race 5 except Heinong 84 which proved sensitive to race 5. All of the 12 cultivars have better yield compared with the control cultivars (1,942–2911 kg/ha in the prerelease trial). Although the yields of these SCN-resistant cultivars are already improved and competitive with the susceptible controls in registration trials in China, further yield and quality improvement can be facilitated using the molecular markers, as described in this study. Furthermore, the SCN bioassay results proved these cultivars as multiresistant, and there is a good chance that simultaneous resistances against race 1, race 3, and race 5 can be maintained during yield and quality breeding by applying the described molecular markers simplifying the selection and improving selection gain. Multiplex SCN resistance is also a valuable preventive measure against resistance breakdown and shift of virulence in the host populations (Meinhardt et al., 2021; Lin et al., 2022).
The special features of the 12 cultivars identified in this study are the multi-race SCN resistance and the relatively high yield, which are both simultaneously required for a sustainable cultivation of soybeans in the presence of SCN. Despite the yield advantage of the new SCN-resistant cultivars in the registration trials, only the cultivar Heinong 84 of the 12 cultivars was grown on 4% of Heilongjiang’s soybean planting area in 2019 whereas others have very little planting area as yet (Lian et al., 2022). Heinong 531 was just released in 2021 belonging to maturity group(MG I, Northeast China growing area) with 2,752 kg/ha in the 1-year prerelease trial and a yield increase of 12.0% compared with the local cultivars (Wang et al., 2022). Heinong 531 is the cultivar with the highest expectation of all the cultivars of MG I regarding SCN control and yield. In another study, Handou 10 showed an FI value of 28.0 and was described as MR (Wei et al., 2022). Here, Handou 10 achieved an FI of 36.4 which corresponds to an MS reaction. In general, the number of harvested cysts is environmentally affected by temperature, humidity, watering, etc., and even when the bioassay is carried out in an environmentally controlled climate room, there are minimal differences between different pots, experiments, and environments. It is not unexpected for a quantitative trait like FI that average values are varying to some degree. In this case, the overall conclusion is not much different regarding that MS and MR are intermediate scores which are neither resistant nor fully susceptible. Zhonghuang 57 also showed resistance to race 2 and race 4, two races that increasingly spread in soybean-growing areas worldwide (Xu et al., 2021; Lian et al., 2022). With resistances against all five races, Zhonghuang 57 is the most resistant cultivar in our study material and this multiplex resistance is comparable with one of the most resistant genetic resources for SCN resistance, PI 437654 (Shi et al., 2015). This result also shows the limitation of our molecular markers which are not able to differentiate between Peking-type resistance and PI 437654-type resistance. Zhonghuang 57 had a relatively good yield with 2,779 kg/ha in the prerelease trial in 2009 and a yield increase of 1.3% when compared with Huang-Huai Valley local cultivars (MG III). By using marker-assisted selection with the described KASP markers, this cultivar with that excellent SCN resistance profile is a valid candidate for further yield and quality increase to achieve market competitiveness for MG III cultivars and the Huang-Huai Valley production area.
Of the 12 SCN-resistant varieties described in this study, Heinong 84 was bred by combining molecular marker-assisted selection with traditional pedigree breeding, whereas the other varieties were only selected through traditional pedigree breeding. In the cultivar registration system, it is shown that each one of the parents of Nongqingdou 24, Fengdou 1, and Zhonghuang57 was resistant against SCN. There is no further information about the parents for these cultivars. Previous SCN resistance screening studies were often carried out with only one race like race 3 with no specific virulence, whereas in this study, the most common reported races in the soybean production area worldwide, race 1 to race 5, were included. In contrast to most resistance screening studies, the 12 soybean cultivars included here are proven of agronomic value with competitive yield, oil and protein content, and seed coat color, which makes them more easily accessible for modern breeding than other genetic resources like wild soybean species or minimally adapted landraces. Moreover, with Zhonghuang 57 we identified a cultivar with broad resistance against all five races which goes beyond the popular resistance sources like PI 88788 (rgh1-b) and Peking (rgh1-a, Rgh4). Zhonghuang 57 can be used in areas where race 2 or race 4 are of major yield concern. Nematode populations can overcome the resistance of a single resistance locus within a decade like it was described for PI 88788, the most known and used SCN-resistant source (Niblack et al., 2008; Howland et al., 2018; Meinhardt et al., 2021). Unique resistance types that are different from PI 88788 and best, based on multiple resistance loci like Zhonghuang 57, will be valuable for developing soybean cultivars with potentially durable resistance to SCN.
Cultivars with the same genetic sources of resistance do not necessarily have the same levels of resistance in the field (Niblack et al., 2002). Therefore, before using one of these varieties as resistance donor in a new breeding program, it is advised to confirm the SCN resistance performance in infested fields. Marker-assisted selection (MAS) of the SCN resistance and molecular marker background selection or straightforward line selection can be applied to implement the multiple SCN resistances identified in this study into favorable elite soybean cultivars. MAS directly improves selection gain, reducing breeding cost, and less “false” selected or “escapes” can happen as compared with solely field or bioassay selection. Potential linkage drags with undesirable traits should not cause any problems for cultivation or further improvement through breeding since the cultivar level has already been reached. This identification would be critical to achieving a better understanding of the molecular architecture of resistance to H. glycines. Our data suggest that there is a tremendous genetic potential available to improve soybean cultivars with resistance against H. glycines by using agronomically adapted and competitive G. max genetic breeding resources, as described in this study.
Data availability statement
The original contributions presented in the study are included in the article/supplementary material. Further inquiries can be directed to the corresponding authors.
Author contributions
YL and WL designed the experiments. MY supplied most of the cultivars. YL produced the data. YL drafted the manuscript with contributions from GK, HW, JL, BD, JW, and WL. All authors read and approved the submitted version.
Funding
This study was supported by Joint Fund for S&T Research and Development Plan of Henan in China (222301420028) and HeNan Province Key S&T Special Projects (221100110300).
Acknowledgments
We extend our sincere gratitude to Richard W. Madsen, Professor Emeritus of Statistics, University of Missouri-Columbia, United States, for his help in editing the text.
Conflict of interest
The authors declare that the research was conducted in the absence of any commercial or financial relationships that could be construed as a potential conflict of interest.
Publisher’s note
All claims expressed in this article are solely those of the authors and do not necessarily represent those of their affiliated organizations, or those of the publisher, the editors and the reviewers. Any product that may be evaluated in this article, or claim that may be made by its manufacturer, is not guaranteed or endorsed by the publisher.
References
Ali, M. A., Abbas, A., Kreil, D. P., Bohlmann, H. (2013). Overexpression of the transcription factor RAP2.6 leads to enhanced callose deposition in syncytia and enhanced resistance against the beet cyst nematode heterodera schachtii in arabidopsis roots. BMC Plant Biol. 13, 47. doi: 10.1186/1471-2229-13-47
Bradley, C. A., Allen, T., Sisson, A. J., Bergstrom, G. C., Wise, K. (2021). Soybean yield loss estimates due to diseases in the united states and Ontario, Canada from 2015-2019. Plant Health Prog 22, 483–495. doi: 10.1094/PHP-01-21-0013-RS
Chen, J., Zhou, Y., Hu, Y., Zhao, D., Zhou, C., Shi, R., et al. (2023). Evaluation soybean cultivars for reaction to Heterodera glycines populations HG types 7 and 1.3.4.7 in northeast China. Life 13, 248. doi: 10.3390/life13010248
Concibido, V. C., Diers, B. W., Arelli, P. R. (2004). A decade of QTL mapping for cyst nematode resistance in soybean. Crop Sci. 44, 1121–1131. doi: 10.2135/cropsci2004.1121
Cook, D. E., Bayless, A. M., Wang, K., Guo, X., Song, Q., Jiang, J., et al. (2014). Distinct copy number, coding sequence, and locus methylation patterns underlie Rhg1-mediated soybean resistance to soybean cyst nematode. Plant Physiol. 165, 630–647. doi: 10.1104/pp.114.235952
Cook, D. E., Lee, T. G., Guo, X., Melito, S., Wang, K., Bayless, A. M., et al. (2012). Copy number variation of multiple genes at Rhg1 mediates nematode resistance in soybean. Science 338, 1206–1209. doi: 10.1126/science.1228746
Duan, Y. X., Peng, D. L. (2014). “Soybean cyst nematode,” in Institute of plant protection, Chinese academy of agricultural sciences, Chinese society of plant protection, editor. plant disease and pests on China agricultural crops, 3rd ed (Beijing: China agriculture press), 1039–1046.
Faghihi, J., Ferris, J. M. (2000). An efficient new device to release eggs from Heterodera glycines. J. Nematol. 32, 411–413.
Holbein, J., Grundler, F. M. W., Siddique, S. (2016). Plant basal resistance to nematodes: an update. J. Exp. Bot. 67, 2049–2061. doi: 10.1093/jxb/erw005
Howland, A., Monnig, N., Mathesius, J., Nathan, M., Mitchum, M. G. (2018). Survey of Heterodera glycines population densities and virulence phenotypes during 2015-2016 in Missouri. Plant Dis. 102, 2407–2410. doi: 10.1094/PDIS-04-18-0650-SR
Jenkins, W. R. (1964). A rapid centrifugal-flotation technique for separating nematodes from soil. Plant Dis. Rep. 48, 692.
Klink, V. P., Hosseini, P., Matsye, P., Alkharouf, N. W., Matthews, B. F. (2009). A gene expression analysis of syncytia laser microdissected from the roots of the Glycine max (soybean) genotype PI 548402 (Peking) undergoing a resistant reaction after infection by Heterodera glycines (soybean cyst nematode). Plant Mol. Biol. 71, 525–567. doi: 10.1007/s11103-009-9539-1
Klink, V. P., Overall, C. C., Alkharouf, N. W., MacDonald, M. H., Matthews, B. F. (2007). A time-course comparative microarray analysis of an incompatible and compatible response by glycine max (soybean) to Heterodera glycines (soybean cyst nematode) infection. Planta 226, 1423–1447. doi: 10.1007/s00425-007-0581-4
Koenning, S. R., Wrather, J. A. (2010). Suppression of soybean yield potential in the continental united states by plant diseases from 2006 to 2009. Plant Health Prog. 11. doi: 10.1094/PHP-2010-1122-01-RS
Lian, Y., Guo, J. Q., Li, H. C., Wu, Y. K., Wei, H., Wang, J. S., et al. (2017). A new race (X12) of soybean cyst nematode in China. J. Nematol. 49, 321–326. doi: 10.21307/jofnem-2017-079
Lian, Y., Koch, G., Bo, D., Wang, J., Nguyen, H. T., Li, C., et al. (2022). The spatial distribution and genetic diversity of the soybean cyst nematode, Heterodera glycines, in China: It is time to take measures to control soybean cyst nematode. Front. Plant Sci., 927773. doi: 10.3389/fpls.2022.927773
Lian, Y., Li, H. C., Li, J. Y., Wang, J. S., Wei, H., Lei, C. F., et al. (2019a). Distribution of soybean cyst nematode resistance allele Rhg1 and Rhg4 in Huang-huai soybean varieties. Scientia Agricultura Sin. 52, 2559–2566. doi: 10.3864/j.issn.0578-1752.2019.15.001
Lian, Y., Wang, J. S., Li, H. C., Wei, H., Li, J. Y., Wu, Y. K., et al. (2016). Race distribution of soybean cyst nematode in the main soybean producing area of Huang-huai rivers valley. Acta Agronomica Sin. 42, 1479–1486. doi: 10.1111/1755-0998.13068
Lian, Y., Wei, H., Wang, J. S., Lei, C. F., Li, H. C., Li, J. Y., et al. (2019b). Chromosome-level reference genome of X12, a highly virulent race of the soybean cyst nematode Heterodera glycines. Mol. Ecol. Resour. 19, 1637–1646. doi: 10.3724/SP.J.1006.2016.01479
Lin, F., Chhapekar, S. S., Vieira, C. C., Da Silva, M. P., Rojas, A., Lee, D., et al. (2022). Breeding for disease resistance in soybean: a global perspective. Theor. Appl. Genet 135, 3773–3872. doi: 10.1007/s00122-022-04101-3
Liu, S., Kandoth, P. K., Lakhssassi, N., Kang, J., Colantonio, V., Heinz, R., et al. (2017). The soybean GmSNAP18 gene underlies two types of resistance to soybean cyst nematode. Nat. Commun. 8, 14822. doi: 10.1038/ncomms14822
Liu, S., Kandoth, P. K., Warren, S. D., Yeckel, G., Heinz, R., Alden, J., et al. (2012). A soybean cyst nematode resistance gene points to a new mechanism of plant resistance to pathogens. Nature 492, 256–260. doi: 10.1038/nature11651
Meinhardt, C., Howland, A., Ellersieck, M., Scaboo, A., Diers, B., Mitchum, M. A.-O. (2021). Resistance gene pyramiding and rotation to combat widespread soybean cyst nematode virulence. Plant Dis. 105, 3238–3243. doi: 10.1094/PDIS-12-20-2556-RE
Mitchum, M. G. (2016). Soybean resistance to the soybean cyst nematode Heterodera glycines: an update. Phytopathology 106, 1444–1450. doi: 10.1094/PHYTO-06-16-0227-RVW
Niblack, T. L., Arelli, P. R., Noel, G. R., Opperman, C. H., Orf, J. H. (2002). A revised classification scheme for genetically diverse populations of Heterodera glycines. J. Nematol. 34, 279–288.
Niblack, T. L., Colgrove, A. L., Bond, J. P. (2008). Shift in virulence of soybean cyst nematode is associated with use of resistance from PI 88788. Plant Health Prog 9, 29. doi: 10.1094/PHP-2008-0118-01-RS
Peng, D., Jiang, R., Peng, H., Liu, S. M. (2021). Soybean cyst nematodes: a destructive threat to soybean production in China. Phytopathol. Res. 3, 19. doi: 10.1186/s42483-021-00095-w
Riggs, R. D., Schmitt, D. P. (1988). Complete characterization of the race scheme for Heterodera glycines. J. Nematol. 20, 392–395.
Shi, Z., Liu, S. M., Noe, J., Arelli, P., Meksem, K., Li, Z. L. (2015). SNP identification and marker assay development for high-throughput selection of soybean cyst nematode resistance. BMC Genomics 16, 314. doi: 10.1186/s12864-015-1531-3
Sobczak, M., Golinowski, W., Grundler, F. M. W. (1999). Ultrastructure of feeding plugs and feeding tubes formed by Heterodera schachtii. Nematology 1, 363–374. doi: 10.1163/156854199508351
Wang, J., Kong, L. A., Zhang, L., Shi, X., Yu, B., Li, J., et al. (2022). Breeding a soybean cultivar heinong 531 with Peking-type cyst nematode resistance, enhanced yield and high seed-oil contents. Phytopathology 112, 1345–1349. doi: /10.1094/PHYTO-08-21-0347-R
Wei, H., Lian, Y., Li, J., Li, H., Song, Q., Wu, Y., et al. (2022). Identification of candidate genes controlling soybean cyst nematode resistance in “Handou 10” based on genome and transcriptome analyzes 13. doi: 10.3389/fpls.2022.860034
Winter, S. M. J., Rajcan, I., Shelp, B. J. (2006). Soybean cyst nematode: Challenges and opportunities. Can. J. Plant Sci. 86, 25–32. doi: 10.4141/P05-072
Keywords: soybean cyst nematode, Heterodera glycines, soybean, resistance, Glycine max
Citation: Lian Y, Yuan M, Wei H, Li J, Ding B, Wang J, Lu W and Koch G (2023) Identification of resistant sources from Glycine max against soybean cyst nematode. Front. Plant Sci. 14:1143676. doi: 10.3389/fpls.2023.1143676
Received: 13 January 2023; Accepted: 16 February 2023;
Published: 07 March 2023.
Edited by:
Shiming Liu, Institute of Plant Protection (CAAS), ChinaReviewed by:
Dan Zhang, Henan Agricultural University, ChinaYongqing Jiao, Henan Agricultural University, China
Copyright © 2023 Lian, Yuan, Wei, Li, Ding, Wang, Lu and Koch. This is an open-access article distributed under the terms of the Creative Commons Attribution License (CC BY). The use, distribution or reproduction in other forums is permitted, provided the original author(s) and the copyright owner(s) are credited and that the original publication in this journal is cited, in accordance with accepted academic practice. No use, distribution or reproduction is permitted which does not comply with these terms.
*Correspondence: Weiguo Lu, MTIzYmVhbkAxNjMuY29t; Georg Koch, Z2VvcmdrQHdlYi5kZQ==
†These authors have contributed equally to this work