- 1College of Ecology and Environment, Hainan University, Haikou, China
- 2Forest Dynamics, Swiss Federal Institute for Forest, Snow and Landscape Research WSL, Birmensdorf, Switzerland
- 3Department of Environmental Systems Science, Institute of Terrestrial Ecosystems, ETH Zürich, Zürich, Switzerland
- 4Forest Soils and Biogeochemistry, Swiss Federal Institute for Forest, Snow and Landscape Research WSL, Birmensdorf, Switzerland
- 5Key Laboratory of Geographical Processes and Ecological Security in Changbai Mountains, Ministry of Education, School of Geographical Sciences, Northeast Normal University, Changchun, Jilin, China
- 6College of Life Science, Hebei University, Baoding, Hebei, China
Many carbon-related physiological questions in plants such as carbon (C) limitation or starvation have not yet been resolved thoroughly due to the lack of suitable experimental methodology. As a first step towards resolving these problems, we conducted infusion experiments with bonsai trees (Ficus microcarpa) and young maple trees (Acer pseudoplatanus) in greenhouse, and with adult Scots pine trees (Pinus sylvestris) in the field, that were “fed” with 13C-labelled glucose either through the phloem or the xylem. We then traced the 13C-signal in plant organic matter and respiration to test whether trees can take up and metabolize exogenous sugars infused. Ten weeks after infusion started, xylem but not phloem infusion significantly increased the δ13C values in both aboveground and belowground tissues of the bonsai trees in the greenhouse, whereas xylem infusion significantly increased xylem δ13C values and phloem infusion significantly increased phloem δ13C values of the adult pines in the field experiment, compared to the corresponding controls. The respiration measurement experiment with young maple trees showed significantly increased δ13C-values in shoot respired CO2 at the time of four weeks after xylem infusion started. Our results clearly indicate that trees do translocate and metabolize exogenous sugars infused, and because the phloem layer is too thin, and thus xylem infusion can be better operated than phloem infusion. This tree infusion method developed here opens up new avenues and has great potential to be used for research on the whole plant C balance and its regulation in response to environmental factors and extreme stress conditions.
1 Introduction
Trees rely on photosynthesis to gain carbon, which is a core resource allocated among different tissues to grow, reproduce, defend, and maintain metabolic functions (Körner, 2006; Maguire and Kobe, 2015; Hartmann and Trumbore, 2016). Photosynthetic carbon may be passively (Sala et al., 2010) or actively (Li et al., 2018) stored in the form of sugars and starch as nonstructural carbohydrates (NSC) in response to environmental stress, thus the plant tissue NSC levels reflect the balance between C-gain (or supply) and C-utilization (or demand) (Chapin et al., 1990; Kozlowski, 1992; Li et al., 2002; Hoch et al., 2003; Körner, 2003; Dietze et al., 2014; O’Brien et al., 2014; Hartmann and Trumbore, 2016; Martínez-Vilalta et al., 2016; Sapes et al., 2021). If plant’s C demand exceeds the C supply, the plant’s C storage will be depleted with time and the plant may suffer from a carbon deficit, which may result in decreasing growth rate and increasing mortality of plants.
In the debate about either the alpine treeline formation or the drought-induced forest mortality, carbon shortage or carbon starvation has been proposed and are still being debated as the underlying physiological mechanisms. Studies based on natural experiments suggested carbon source limitation (Wardle, 1993; Li et al., 2002; Li et al., 2008a; Li et al., 2008b; Li et al., 2018; Wang et al., 2021) or growth sink limitation (Körner, 1998; Hoch and Körner, 2009; Harsch and Bader, 2011) as the possible physiological mechanisms for tree growth under low temperature at the alpine treelines. Similarly, hydraulic failure and carbon starvation have been proposed as possible physiological mechanisms to explain the water deficit-caused forest dieback (McDowell et al., 2008; McDowell et al., 2011; Mcdowell et al., 2016; Choat et al., 2018). The carbon starvation or limitation hypothesis assumes that mortality of drought- or low temperature-stressed trees occurs because trees’ carbon production of photosynthesis cannot meet its minimum demand for survival (Li et al., 2008b; McDowell and Sevanto, 2010). Measurements of tissue NSC levels reveal, however, inconsistent results. For example, as Hoch and Körner (2003) did not find any carbon shortage in treeline trees, other studies clearly indicated a carbon limitation in roots of plants at their upper limits (Li et al., 2008a; Li et al., 2008b; Genet et al., 2011; Li et al., 2018; Wang et al., 2021) and under water deficit (Galvez et al., 2013; Sevanto and Dickman, 2015; Hagedorn et al., 2016; Joseph et al., 2020; Schönbeck et al., 2020). Moreover, in both the treeline and drought cases, carbon limitation may occur with growth limitation (treeline) or hydraulic failure (drought) together (Adams et al., 2017), and thus, we actually don’t know which one is the primary and which is the following secondary mechanisms leading to mortality. If non-structural carbohydrates could be introduced into stressed trees, and if trees can accept, distribute and metabolize such exogenous sugars added, it is then possible to distinguish carbon limitation from growth limitation or hydraulic failure.
We therefore ask whether plants, analogously to nutritionally deficit sick humans and animals, can accept and use exogenous sugars infused, because sugars in the nature are merely produced by plant photosynthesis (endogenous sugars)? If yes, we, then, can develop a new methodology (i.e. exogenous sugar addition) to open up new avenues for research on the whole plant carbon balance and its regulations, and potentially improve vitality of historic and landmark trees suffering from severe damage in the practice.
Since more than 50 years, some previous activities have tried to develop plant injection methods and techniques to inject chemical substances into tree’s stem for pests control (Feng, 1972; Feng, 1979; Eggers et al., 2005; Feng and Ji, 2009; Zeng et al., 2010; Hijaz et al., 2020), which has been recently reviewed by Li and Nangong (2022). Injection of plant nutrients (Grabau et al., 1986; Schon and Blevins, 1987) and hormones (Philipson, 1985; Ross and Bower, 1991; Eriksson et al., 1998; Smith, 1998; Kong et al., 2008) for growth regulations has also been applied. Other authors demonstrated the possibility of supplying albino corn plants (Spoehr, 1942) and tomato plants (Went and Carter, 1948) with sucrose, or feeding trees (Picea abies [L.] Karst) with amino acids (Gessler et al., 2003) through the cut ends of the leaves.
Overall, there are only a few studies worldwide that injected (fast, short time treatment) or infused (slow, longer time treatment) sugars into plant stems, and these very limited experiments concentrated only on a few agricultural species, i.e. soybean (Hayati et al., 1995; Abdin et al., 1998; Zhou et al., 2000), corn plants (Zea mays L.) (Boyle et al., 1991a; Boyle et al., 1991b; Ma et al., 1994; Zhou and Smith, 1996; Zhou et al., 1997), sweet and grain sorghum (Tarpley et al., 1994), and chickpea (Cicer arietinum L.) (Khan et al., 2017). They generally found that sugar injection or infusion increased yield but decreased plant photosynthesis. On the other hand, probably due to the hardness and rigidity of wood and bark, the possibility and methodology of sugar infusion or injection with woody plants have rarely been studied, tested only in Quercus alba (McLaughlin et al., 1980) using the circumferential trough technique (Auerbach et al., 1964), Citrus unshiu (Iglesias et al., 2003) with a low-pressure injection system (Iglesias et al., 2001), and in Quercus virginiana (Martinez-Trinidad et al., 2009) using the so-called macro-injection system (Eggers et al., 2005). They did not trace the transport and allocation of the injected/infused sugars within a tree, and thus, we actually do not know how the exogenous sugars added are transported and allocated in plants.
It is well known that water movement in xylem is unidirectional upwards from roots to aerial parts of trees, whereas the movement of photosynthetic carbon products in phloem is bidirectional both downwards and upwards from source (leaves or storage) to sink. As many studies indicated that carbon shortage occurs mainly in roots (Li et al., 2008a; Li et al., 2008b; Genet et al., 2011; Galvez et al., 2013; Sevanto and Dickman, 2015; Hagedorn et al., 2016; Li et al., 2018; Joseph et al., 2020; Schönbeck et al., 2020; Wang et al., 2021) mentioned above, it is thus reasonable that sugar addition into phloem may be more effective than into xylem of stressed trees, to improve the root carbon supply and balance.
We therefore, carried out the present pilot study to test whether trees take up, translocate and metabolize infused exogenous sugars. Using common infusion method and equipment for human and animals, we experimentally tested to infuse 13C-labelled glucose through either phloem or xylem into bonsai trees (Ficus microcarpa) in greenhouse, and also into adult Pinus sylvestris trees in the field in 2021. Three and ten weeks after infusion started, we took samples to analyze the δ13C values in organic matter. We hypothesize that xylem infusion increases the δ13C values in leaves and wood above the infusion point due to unidirectional upward transport in xylem, whereas phloem infusion increases the overall δ13C values of plants due to bidirectional movement in phloem (Hypothesis I). This, in other words, means that trees do take up infused exogenous sugars. Moreover, we expect that xylem infusion will works better than phloem infusion (Hypothesis II) because the latter may be very difficult to be operated due to the very thin phloem layer. Based on the results gained above in 2021, we conducted a third experiment with young Acer pseudoplatanus trees in 2022. We first infused the trees with 13C-labelled glucose, and then measured the shoot dark respiration four weeks after infusion started, to test our third hypothesis that plants use the infused exogenous sugars for respiration (Hypothesis III) to provide energy for physiological processes and growth of trees.
2 Methodology development: Materials, equipment, and methods
2.1 Greenhouse experiment with bonsai plants
We selected bonsai trees of Ficus microcarpa potted in 21 cm (upper diameter) x 18.5 cm (height) x 15 cm (bottom diameter) pots filled with Ficus Mix soil (SYBotanicA, Bemmel, The Netherlands) for a greenhouse experiment at the Swiss Federal Institute for Forest, Snow and Landscape Research WSL, Birmensdorf, Switzerland (Figure 1A). Bonsai trees of F. microcarpa were selected because they are thick enough with thick xylem and especially thick bark, so that both phloem (Figure 1B) and xylem (Figure 1C) infusion may be possible. One week prior to sugar infusion treatment, ~85% of the leaves from each of the 27 F. microcarpa plants were removed (i.e. only ~15% of leaves in the upper crown were left intact; Figure 1A) to limit photosynthesis and thus to create a carbon shortage in plants.
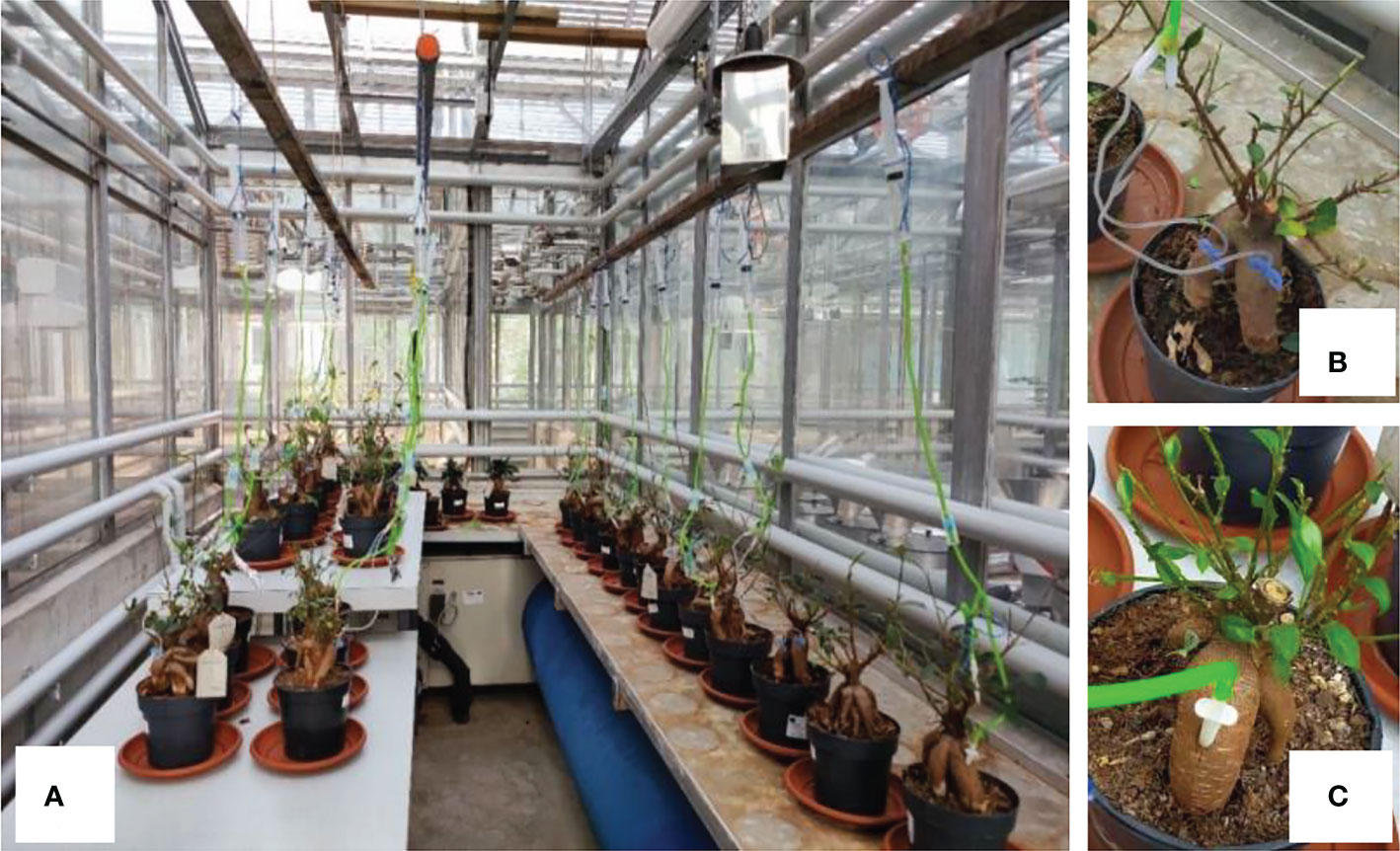
Figure 1 Glucose infusion experiment with Ficus microcarpa bonsai plants in WSL greenhouse (A). Each of the three treatments, i.e. phloem infusion (B), xylem infusion (C), and control, had 9 plants. The infusion set consists of a 20 mL-syringe, a 120 cm-long rubber tube with a flow regulator and two thin infusion needles for phloem infusion (B), or one thick plastic needle for xylem infusion (C).
On April 11-12, 2021, the sugar solutions were prepared. We dissolved 10 g 13C-labeled D-glucose powder (13C6, 99 atom % 13C, Sigma-Aldrich, Buchs, Switzerland) in 20 mL distilled water as 13C-labelled glucose solution (~1:2 for sugar: water). We also dissolved 2 kg non-labelled D-glucose powder (Carl-Roth, Karlsruhe, Germany) in 3 L distilled water as non-labelled glucose solution (~1:1.5 for sugar: water). The δ13C value of the non-labelled D-glucose is –26.00‰ ± 0.015‰, and is thus very close to the natural 13C abundance (–26.00‰ δ13C) in European trees (Taylor et al., 2003; Zeller et al., 2007). This ensures that any 13C-labelling effects of the study should thus be solely derived from the 13C-labelled glucose. The solution was filtered aseptically in an aseptic platform before infusion.
The infusion set consists of a 20 mL-syringe, a 120 cm-long rubber tube with a flow regulator and two thin infusion needles (size 0.65 x 19 mm; Venofix® Safety G23, Venipuncture set) for phloem infusion (Figure 1B), or one thick plastic needle (size 4 x 20 mm; Henan Zhengzhou Green Cube Garden Engineering Co., Ltd., China) for xylem infusion (Figure 1C). Before infusion started, we first poured 15 mL non-labeled glucose into each syringe (Figure 1A). Nine plants were randomly selected for one of the three treatments, i.e. control, phloem infusion (Figure 1B), and xylem infusion (Figure 1C) on April 13, 2021. For phloem infusion, the thin, sharp needles were directly inserted into the phloem parallel to the stem surface (Figure 1B). For xylem infusion, we first used an electric drill (Bosch Professional GSR 12V-15, Germany) to drill a hole (~10 mm in depth, and 3.5 mm in diameter) in a 45° angle into the stem, and then the thick plastic infusion needle was inserted into that hole (Figure 1C), and the whole contact surface was immediately sealed with an adhesive layer (Cementit Universal adhesive, Switzerland). To effectively use the expensive, limited 13C-labelled glucose, we used a 1 mL-syringe to inject 1 mL 13C-labelled glucose into the lower segment of the rubber tube of each infusion set one day later (April 14, 2021), and the hole on the rubber tube was immediately sealed with an adhesive layer. The initial level of sugar solution in each syringe was marked on the syringe and recorded. The controls were kept intact to measure the natural 13C abundance.
We took leaves, shoots and fine roots (<2 mm in diameter) samples twice during the infusion process: the first sampling date was three weeks later (May 4, 2021) when a visible reduction in the initial level of sugar solution in syringes was observed, and the second sampling date was ten weeks later (June 22, 2021) after infusion started. Leaves, shoots that emerged acropetally above the infusion point and fine roots with white color were collected from each sample plant and immediately transferred and stored at -20°C. We hypothesized that most of the thin white colored roots may be of post-infusion origin, and therefore that they most likely utilized and incorporated the infused exogenous sugars. To collect fine roots, we carefully pulled out the plants from pots, cut the outer white-colored roots, and carefully put the plants back into the pots. Samples were taken only from the bonsai trees, on which the syringe showed a visible lower level of the sugar solution compared to the initial level marked. Only 3 to 5 individuals out of the 9 replicates showed a visible decreased sugar solution level, and the decreasing velocity was normally < 3 mL within the 1st 3 weeks and < 5 mL within the 10 weeks. Thus, the replicates for laboratory and statistical analyses were n=3–5 (see Figure 2).
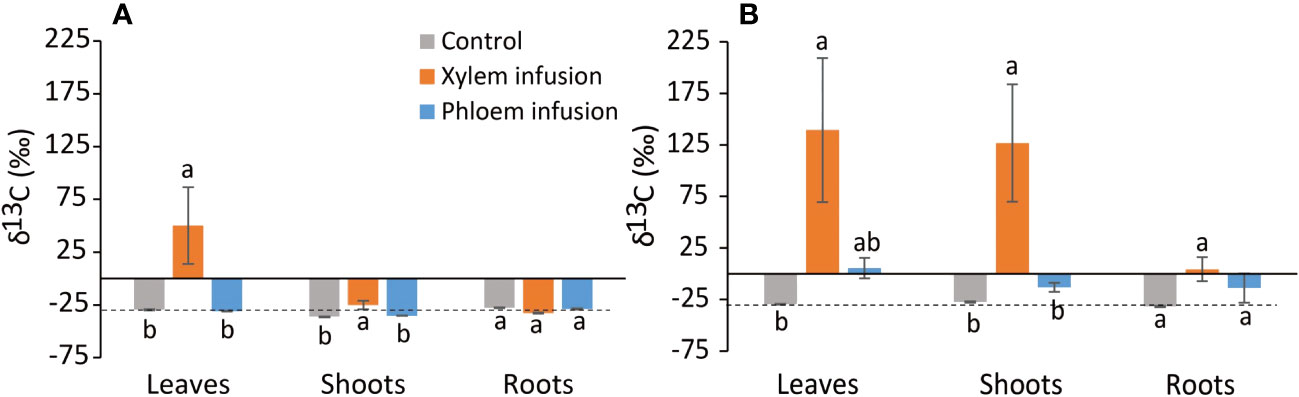
Figure 2 Effects (n = 3 – 5, mean value ± 1 SE) of xylem infusion (orange color) and phloem infusion (blue) with 13C-labelled glucose into Ficus microcarpa bonsai trees compared to controls (grey) under greenhouse conditions (see Figure 1). Samples were taken three (A) and ten (B) weeks after the infusion started. The dashed lines correspond to the average natural 13C abundance (~ -26‰ δ13C) in European trees (Taylor et al., 2003; Zeller et al., 2007). Different lowercase letters indicate significant differences in δ13C values of tissue (i.e., leaves, shoots, or roots) among the treatment xylem infusion, phloem infusion and controls (P < 0.05).
2.2 Field experiment with adult pine trees
The field study was carried out in a mature Scots pine (Pinus sylvestris L.) forest located in a dry valley Pfynwald (~600 mm precipitation per year; 46° 18′ N, 7° 36′ E, 615 m above sea level), Valais, Switzerland. Eighteen Scots pine trees (~120 years old, ~11 m in height and ~30 cm in diameter at breast height) grown in similar ambient condition were selected, six out of the 18 trees were randomly assigned for one of the three treatments, i.e. phloem infusion (Figure 3B), xylem infusion (Figure 3C), and intact controls.
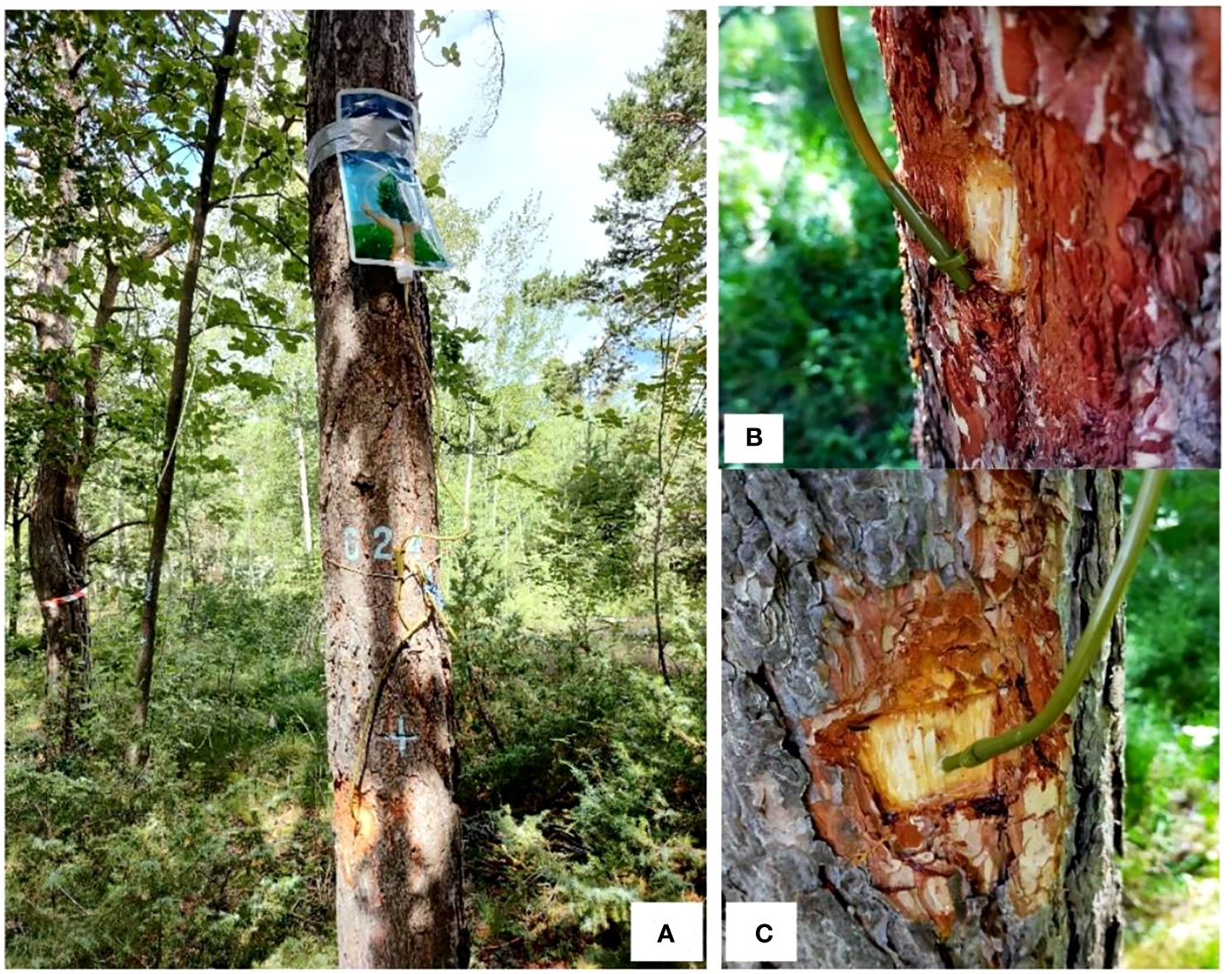
Figure 3 Glucose infusion experiment with adult Scots pine (Pinus sylvestris) trees (A), for phloem infusion (B) and xylem infusion (C) in Pfynwald, Valais, Switzerland. For phloem infusion (B), a hole (~20 mm in depth, and 3.5 mm in diameter) between the bark and xylem, parallel to the stem surface, was drilled with an electric drill, then the thick plastic infusion needle was inserted into that hole. For xylem infusion (C), we also used an electric drill to drill a hole (~20 mm in depth, and 3.5 mm in diameter) in a 45° angle into the stem, and then the thick plastic infusion needle was inserted into that hole.
The infusion set consists of a 1.5 L tree infusion bag, a 70 cm-long rubber tube and a thick plastic needle (size 4 x 20 mm; Henan Zhengzhou Green Cube Garden Engineering Co., Ltd., China; Figure 3). Both the non-labelled and the 13C-labelled glucose solutions used here were prepared as those used in the greenhouse experiment described above. Each infusion bag was filled with 150 mL non-labelled glucose solution. Phloem infusion (Figure 3B) and xylem infusion (Figure 3C) started on June 28-29, 2021. For phloem infusion, we first used an electric drill (Bosch Professional GSR 12V-15, Germany) to drill a hole (~20 mm in depth, and 3.5 mm in diameter) between the bark and xylem, parallel to the stem surface, then the thick plastic infusion needle was inserted into that hole (Figure 3B), and the whole contact surface was immediately sealed with an adhesive layer (Cementit Universal adhesive, Switzerland). For xylem infusion, we also used the electric drill to drill a hole (~20 mm in depth, and 3.5 mm in diameter) in a 45° angle into the stem, then the thick plastic infusion needle was inserted into that hole (Figure 3C), and the whole contact surface was immediately sealed with an adhesive layer (Cementit Universal adhesive, Switzerland). The infusion point (position) was at the stem height of 80-100 cm (Figure 3A). One week later (on July 7, 2021), we used a 1 mL-syringe to inject 0.8 mL 13C-labelled glucose solution into the lower segment of each 70 cm-long rubber tube, and the hole on the tube was immediately sealed with an adhesive layer. Thus, the 13C-labelled glucose of 0.8 mL was not diluted in the 150 mL non-labelled glucose solution but concentrated in the lower segment of the rubber tube close to the infusion point. The initial level of sugar solution in each infusion bag was marked on the bag and recorded. The controls were kept intact to measure the natural 13C abundance.
Similar to sampling in the greenhouse experiment described above, we took samples also two times during the infusion process. The 1st sampling date was also three weeks later (July 28, 2021) and the 2nd sampling date was ten weeks later (September 15, 2021) after 13C-labelled glucose was added. Samples collected included phloem and the very outer xylem layer, which we assume to be the most active xylem wood. The phloem and xylem samples were taken with treering increment borers (1 cm in diameter, Haglöf Sweden) from the stem height of ~30 cm above (above-sample) and below (below-sample) the infusion point at the 1st sampling date, and of ~50 cm above and below the infusion point at the 2nd sampling date for each infused tree. For the controls, we collected samples at ~1 m (1st sampling) and ~1.3 m (2nd sampling) stem height of each control tree. To obtain materials necessary for 13C analysis, 3-5 cores (~1 cm long each) were collected from each stem height level for each sampling date. The bark was removed, and the phloem and xylem were separately collected. Collected samples were kept in polybags in an ice-box carrier, transported to the laboratory and stored at -20°C. Samples were taken only from the treated trees, on which the infusion bag showed a visible lower level of the sugar solution compared to the initial level marked. Only 3 individuals out of the 6 replicates showed a visible decreased sugar solution level (the decreasing velocity could not be estimated due to lack of scale on the bags), and thus, the replicates for laboratory and statistical analyses were n=3 (see Figure 4).
2.3 Respiration measurement experiment with maple saplings
A third experiment with Acer pseudoplatanus young trees (170 – 200 cm in height, 1.0 – 1.5 cm in diameter at breast height) infused with 13C-labelled glucose was conducted, to test whether plants use the infused exogenous sugars for respiration to provide energy for physiological processes and growth. Using the same infusion set and methods including sugar solutions mentioned above for the field experiment, 3 maple trees were infused with 13C-labelled glucose into xylem, and 3 trees were used as controls. Each infused tree was treated with 1 mL 13C-labelled glucose plus 30 mL non-labelled glucose, with the same procedure as the field experiment described above. The infusion started on April 14, 2022. Four weeks after infusion started, we tightly wrapped up the upper crown (incl. leaves and small branches) above the infusion point with a black plastic bag (70 L) connected with an input and an output rubber tube. These tubes were then tightly connected to a G2131‐i isotopic‐CO2 gas analyzer (Picarro, USA), to measure the dark respiratory 13CO2 of the upper crown for 20 minutes each (Picarro, 2011).
2.4 Laboratory analysis
The bulk 13C content of dried and ground samples was analyzed with an elemental analyzer (IsoEarth, Sercon, Crewe, UK) and isotope ratio mass spectrometer (HS2022, Sercon, Crewe, UK) in the WSL stable isotope lab (https://www.wsl.ch/en/about-wsl/instrumented-field-sites-and-laboratories/laboratories/isotope-laboratory.html). The resulting isotope ratios were converted to δ13C in per mil by reference to the international standard VPDB with a precision of better than 0.2‰ (Brooks et al., 2022).
2.5 Data analysis
After the data normality test, we first analyzed the effects of treatment, sampling date (time), and their interaction on tissue δ13C levels for the greenhouse experiment with bonsai trees and the field experiment with adult pine trees, respectively. The results showed that the sampling date had significant effects on the tissue δ13C levels in 4 out of 7 cases (see Tables 1, 2). We, therefore, tested the treatment effects for each experiment within each sampling date separately. We used one-way ANOVA to analyze the treatment (control, xylem infusion, phloem infusion) effects on δ13C values within each tissue type (leaves, shoots, roots) for each sampling date for the greenhouse experiment (Figure 2), while we performed two-way ANOVAs to test the effects of treatment (control, xylem infusion, phloem infusion), tissue position (above or below the infusion point), and their interaction on δ13C values within each tissue type (xylem, phloem) for each sampling date for the field experiment (Figures 4). One-way ANOVA was used to test the difference in the dark respiratory 13CO2 of the upper crown between the controls and trees infused with 13C-labelled glucose (Figure 5).

Table 1 Effects of treatment (control, xylem infusion, phloem infusion) and sampling date (3 weeks and 10 weeks after the infusion started), and their interaction on δ13C values in leaves, shoots and roots of bonsai Ficus microcarpa trees, using the linear mixed-effects models.

Table 2 Effects of treatment (control, xylem infusion, phloem infusion) and sampling date (3 weeks and 10 weeks after the infusion started), and their interaction on δ13C values in xylem (sampled above and below the infusion point) and phloem (above and below the infusion point) of adult Pinus sylvestris trees, using the linear mixed-effects models.
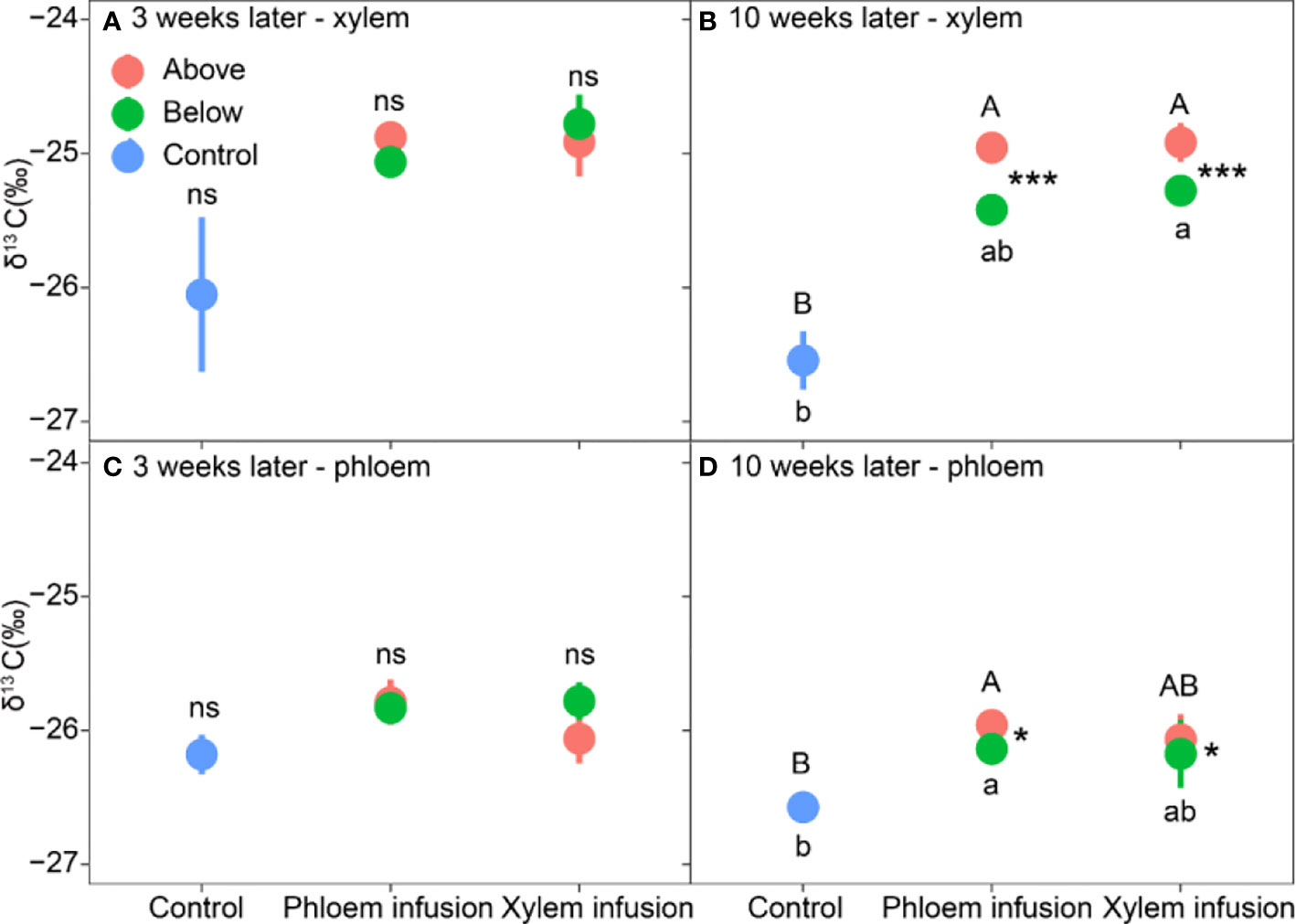
Figure 4 Effects of 13C-labelled glucose infusion into phloem and xylem tissues of adult Pinus sylvestris trees compared to controls (n = 3, mean value ± 1 SE). Tissues were collected from positions above (red color) and below (green) the infusion point at the time of three weeks (A, C; leaf panel) and ten weeks (B, D; right panel) after the infusion started. Note different capital letters indicate significant differences in δ13C values among ‘above’-samples and controls, and different lowercase letters indicate significant differences in δ13C values among ‘below’-samples and controls. * (P < 0.05) and *** (P < 0.001) denote significant differences in δ13C values between ‘above’ and ‘below’-samples within a treatment.
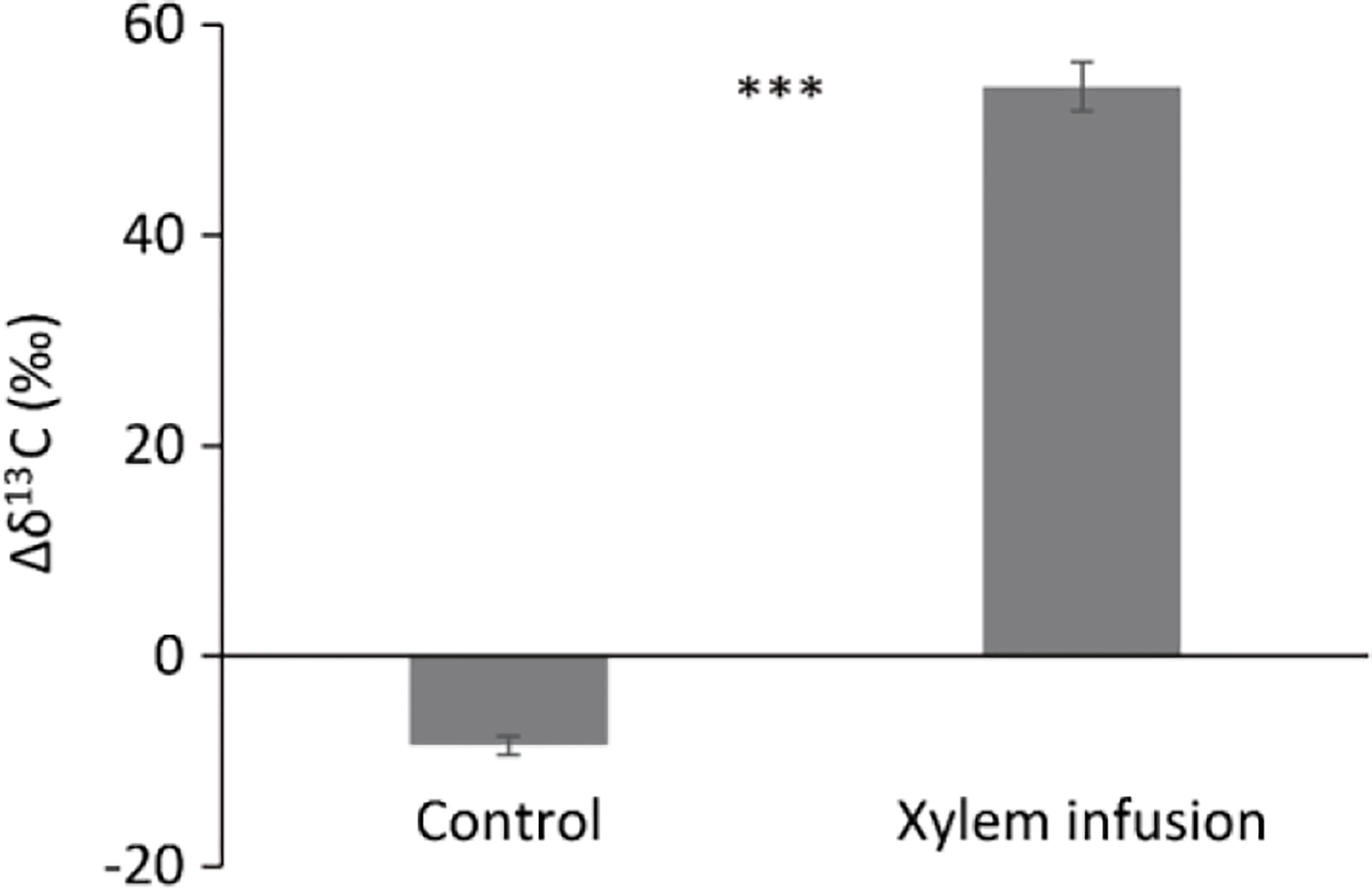
Figure 5 Carbon isotopic composition (δ13C) in shoot dark respirated CO2 in non-treated Acer pseudoplatanus young trees (controls) and trees xylem-infused with 13C-labelled glucose (n = 3, mean value ± 1 SE). Δδ13C (‰) = shoot dark respirated δ13C (‰) minus the ambient air δ13C (‰). *** (P < 0.001) denote significant differences in Δδ13C values between xylem infusion and control.
3 Results
3.1 Greenhouse experiment
Infusion treatment significantly affected leaf δ13C values (P = 0.005) but not root δ13C values (P > 0.05), while the infusion treatment interacted with sampling date to significantly affect shoot δ13C (P = 0.018; Table 1). Across the experiment period, the δ13C values in the control plants corresponded to the average level of the natural 13C abundance of ~ -26‰ (δ13C) in European trees (Figures 2A, B). Three and ten weeks after the start of the xylem infusion, δ13C values significantly increased in leaves and shoots but not in roots, compared to controls (Figures 2A, B). Compared to control plants, phloem infusion did not change the overall δ13C level in bonsai trees three weeks after the infusion started (Figure 2A), but it tended to increase the tissue δ13C level ten weeks after infusion started (Figure 2B).
3.2 Field experiment
Infusion treatment significantly affected the δ13C values in both xylem and phloem collected from both above and below the infusion point (all P < 0.05; Table 2). Only “below-” phloem δ13C significantly varied with time (P = 0.007; Table 2), and ‘below-’ xylem δ13C was marginally significantly affected by time (P = 0.065; Table 2). Three weeks after the infusion started, both xylem and phloem infusion tended to increase (P > 0.05) the δ13C values in xylem and phloem tissue below and above the infusion point compared to controls (Figures 4A, C). Ten weeks after the infusion started, the xylem and phloem δ13C values did not differ between phloem and xylem infusion (Figures 4B, D), but both xylem and phloem infusion increased or tended to increase the δ13C values in xylem and phloem tissues taken from the positions both above and below the infusion position, compared to the controls (Figures 4B, D). Moreover, ten weeks after the infusion started, the ‘above-’ xylem and ‘above-’ phloem tissues had significantly higher δ13C values than the respective ‘below-’ xylem and ‘below-’ phloem tissues for both phloem and xylem infusion (Figures 4B, D).
3.3 Respiration experiment
Compared to controls, xylem infusion of 13C-labelled glucose highly significantly increased the Δδ13C values of shoot dark respiration (Figure 5), indicating that exogenous sugar was metabolized and used by trees for respiration to produce adenosine triphosphate (ATP) for the physiological processes and tree growth.
4 Discussion
4.1 Trees take up, translocate, and metabolize infused exogenous sugars
Our results clearly demonstrated that the infused exogenous sugars were taken up and translocated to above- and belowground tissues within a tree (Figures 2, 4), and the respiration experiment confirmed that the exogenous sugars were further metabolized and respired, potentially to provide energy for the physiological processes and tree growth (Figure 5).
The present study aimed to develop a method for slowly, longer-lasting addition (i.e. infusion) of exogenous sugars to stressed trees. So far there have been only very few limited (probably 3 only) previous experiments with trees for sugar addition (McLaughlin et al., 1980; Iglesias et al., 2003; Martı́nez-Trinidad et al., 2009) mentioned above in the section introduction. Those studies, unlike the present study, attempted to fast inject sugars into trees through a hole drilled into a tree trunk or a trough drilled around a tree trunk, and filled with sugar solutions (McLaughlin et al., 1980; Iglesias et al., 2003; Martı́nez-Trinidad et al., 2009). They all, however, indicated that trees seemed to accept the exogenous sugars. For instance, McLaughlin et al. (1980) introduced 14C-labelled sucrose into the stem of a Quercus alba tree (~15 cm in diameter at breast height) and subsequently detected and measured the 14CO2 respiration from soils around that tree, indicating that the 14C-labelled sucrose was accepted by the tree and downward allocated to the roots. Iglesias et al. (2003) injected sucrose into stems of Citrus unshiu trees and found that sucrose supplementation promoted citrus fruit set by >10%. Martı́nez-Trinidad et al. (2009) found that trunk sugar injection decreased the stress and improved the growth and vitality of mature (16-20 cm in diameter at breast height) Quercus virginiana urban trees under stressful conditions. Sugar injection into crop plants also generally led to increased yield and stress-tolerance (Boyle et al., 1991a; Boyle et al., 1991b; Ma et al., 1994; Tarpley et al., 1994; Hayati et al., 1995; Ma et al., 1995; Zhou and Smith, 1996; Zhou et al., 1997; Abdin et al., 1998; Zhou et al., 2000; Khan et al., 2017). Sorochan (2002) stated that exogenous sugars added can mix with the endogenous carbohydrate pool and be utilized for metabolic processes. Our study suggests that the newly developed methodology will open up new avenues for research on the whole plant C balance and its regulation, and have important implications for practice (e.g. providing additional sugars to historic and landmark trees suffering from severe damage) and future research areas.
It is also worthy to mention that, in the field experiment carried out in the Swiss dry valley Pfynwald (~600 mm precipitation per year), samples taken on September 15 (ten weeks after the infusion started) showed somewhat lower δ13C levels than those taken on July 28 (three weeks) (Figures 4B, D). For instance, the control trees showed higher δ13C levels in tissues sampled on July 28 (-26.1‰ – -26.2‰; Figures 4A, C) than those in tissues sampled on September 15 (-26.5‰ – -26.6‰; Figures 4B, D). The higher δ13C in July (less negative) is a result of summer drought-induced stomatal closure, and the somewhat lower δ13C in September is related to decreased temperature and improved soil water conditions, and thus increased openness of stomata (Ehleringer et al., 1992; Eilmann et al., 2010; Timofeeva et al., 2017).
Reduced stomatal conductance or stomatal closure in response to drought stress in July led to reduced CO2 concentration in intercellular cavities of leaves (Ci) (Schulze, 1986), relative to atmospheric CO2 concentration (Ca), and thus to decreased leaf (Ci/Ca), which have been found to be closely correlated with increases (less negative values) in δ13C of newly formed photosynthates (Brugnoli et al., 1988; Farquhar et al., 1989).
4.2 Xylem infusion vs. phloem infusion
Xylem infusion but not phloem infusion led to increased tissue δ13C levels in the greenhouse experiment with bonsai Ficus trees (Figure 2), and the respiration experiment with young maple trees showed also highly significant effects of xylem infusion on the Δδ13C values of shoot dark respiration (Figure 5). These results suggest that xylem infusion is more effective than phloem infusion (see Figures 4–5), especially for young trees with very thin phloem layer (see Figures 2, 5). This point will be further discussed below in section 4.4. (Advantages and limitations).
Phloem infusion significantly increased δ13C values in xylem tissues collected above the infusion point (Figure 4B), and it significantly enhanced the δ13C values in phloem tissues collected both above and below the infusion point (Figure 4D), indicating both upward and downward movement of the infused sugars in phloem. This result is reasonable because phloem consisting of living cells can carry materials both up and down the plant body (Ciffroy and Tanaka, 2018), to allocate photosynthetic carbon products bidirectionally from carbon source (leaves or storage) to sink. In the present study, we might have created a strong C source at the point of C infusion and thus transport in both directions is explainable.
Interestingly, xylem infusion tended to increase the δ13C values in roots (Figure 4B) and in xylem tissues collected below the infusion point (Figures 3B, D), indicating a downward movement of the sugars infused in xylem. Theoretically, this result should not occur since the xylem sap flows unidirectionally upwards (Dixon and Joly, 1894). However, in agreement with our results, bidirectional movement and exchange of many compounds in xylem have also been demonstrated (Gessler et al., 2003; Hijaz et al., 2020). Tattar and Tattar (1999), and Tattar (2009) found both upward and downward movement of xylem-injected mobile dye solution in 8 different tree species with 5 cm to 25 cm in stem diameter at breast height, and they explained those as results caused by the tension break of the water column in the xylem due to the injection wound, which may force the injected solution to move in both upward and downward directions. This explanation may also be valid for our experiment (see Figure 3), leading to a ‘dysfunctional’ water movement. Moreover, xylem and phloem streams are neighbored and connected, and thus a diffusive exchange may occur between the two transport systems (Gessler et al., 1998; Gessler et al., 2003; Ciffroy and Tanaka, 2018), which may lead to or contribute to the increased δ13C values in roots under xylem infusion (Figure 2B) and in xylem below the xylem infusion point (Figures 4B, D). On the other hand, the results may also reflect an infusion time-dependent effect. This is when the xylem infusion time is long enough, the δ13C signals may move upwards along with the xylem sap flow to leaves firstly, and then downwards along with the phloem sap flow from leaves to roots. It has also been proposed that this two-way movement may be related to the season-dependent leaf transpiration and stress: during the active growing seasons or suitable conditions stem injection may show stronger upward movement, while injections in fall, winter or strong stress (e.g. drought) may result in more downward movement for temperate deciduous trees (Tattar and Tattar, 1999). All these raise the urgent necessity of further studies to explain the theoretical background and the implementation of infusion or injection.
4.3 Experiments with young trees vs. adult trees
The labelled δ13C values in the young bonsai trees (Figure 2, see also Figure 1) were much higher (up to +160‰) than those in the adult pine trees (Figure 4, see also Figure 3), which, certainly, is a result of the dilution effects. We added 1 mL 13C-labelled glucose to each infusion set for each small Ficus bonsai tree (Figure 1), whereas we added only 0.8 mL 13C-labelled glucose to each infusion set for each large pine tree (Figure 3). The 13C concentration is diluted by the large biomass (Li et al., 2001), leading to lower δ13C values in tissues of the large pine trees compared to those in the small bonsai Ficus trees.
In the greenhouse experiment with small bonsai Ficus trees, the leaves had much higher δ13C levels than the shoots (Figure 2B). This is due to the fact that leaves always have much higher levels of mobile carbohydrates and thus higher δ13C levels (Li et al., 2001). Moreover, in a defoliation experiment with Pinus cembra trees, Li et al. (2002) found a compensatory mechanism that the defoliated trees prioritize to allocate mobile carbohydrates (thus higher δ13C levels) for formation and expansion of new foliage, to compensate for the foliage defoliated and to restore the photosynthesis. In the field experiment with adult pine trees, both phloem infusion and xylem infusion resulted in significantly higher δ13C levels in ‘above-’ tissues than ‘below-’ tissues ten weeks after the infusion started (Figures 4B, D), indicating a prevailing upward movement of the sugars infused, which has been detailly discussed above in section 4.2 (Xylem infusion vs. phloem infusion).
4.4 Advantages and limitations
Here we successfully developed a new technique to infuse exogenous (13C-labelled) sugar solution into xylem and phloem tissues of young and mature trees using common infusion equipment for human and animals. However, only 40% (greenhouse experiment) and 50% (field experiment) of the infused trees showed a visible reduction in the initial level of sugar solution after three and ten weeks of infusion, which raises the urgent necessity of further improvement of the infusion method and equipment. Our results indicated that xylem infusion is more feasible and effective than phloem infusion for woody plants (Figures 4–5). It is very difficult to exactly and successfully insert the very thin infusion needle (see Figure 1B) into the very thin phloem layer on the one hand, and on the other hand the pinhole of such very thin infusion needles can very easily be blocked during the infusion process. Compared to the very thin phloem layer, the thick xylem structure allows to be easily operated with infusion on the one side, and on the other side xylem infusion is also more effective than phloem infusion, to infuse a significant amount of sugars into big trees.
The limitations of the present study are that we, using the current equipment, could not control the infusion velocity, and thus do not know the effects of infusion velocity on the infusion effectiveness. Because the price of 13C-labelled sucrose is very expensive (> 6200 CHF/g), we did not compare the difference in uptake effectiveness of glucose with sucrose in the present pilot study, although sucrose is the typical form of sugars that is used to translocate carbon and energy in plants. Moreover, the present study did not try to understand the physiological mechanisms for uptake and allocation of the infused sugars and its relations to environmental stress and growth. Our further experiments will consider these limitations for in-depth understanding of the functioning of carbon in stressed trees.
5 Prospects and conclusions
The experiments conducted in the present study clearly indicated that trees do take up, translocate and metabolize the exogenous sugars infused, and xylem infusion can be better operated than phloem infusion (especially for young trees), which fully support our three hypotheses (see Introduction). This methodology developed in the present study allows to “feed” trees with sugars, and the tracer allows to study when and where the exogenous carbon is utilized, which can be used to study ecophysiolgoical processes related to carbon starvation or source-sink carbon balances. The method could also be combined with 18O, 15N, and/or 2H-labelling to trace carbon, nutrient, and water movement simultaneously, which will open up new avenues for research on the whole plant resource balance and its regulation in relation to environmental stress, and have great potential for future physiological research areas.
Data availability statement
The original contributions presented in the study are included in the article/supplementary material. Further inquiries can be directed to the corresponding author.
Author contributions
M-HL conceived the ideas, designed the methodology, and established the experiments. Y-LZ, YY, and M-HL carried out the experiment. Y-LZ and YY collected and analyzed the data, wrote the manuscript. M-HL led the writing of the manuscript. All authors contributed to the article and approved the submitted version.
Funding
This study was partly supported by an Innovative Partnership Grants (No.: IPG 03-052020 to M-HL) granted by the Swiss State Secretariat for Education, Research and Innovation, and an Internal Innovative Project (No.: 202102N2100 to M-HL) granted by the Swiss Federal Institute for Forest, Snow and Landscape Research WSL. The research stay of Y-LZ (Grant No.: 202103270001) and YY (No.: 201906620095) was partly supported by the Chinese Scholarship Council. Open access funding provided by WSL - Swiss Federal Institute For Forest, Snow And Landscape Research.
Acknowledgments
We thank Ao Wang and Peng Zhang for helping in the field and lab work, Dr. Haoyu Diao for checking the statistical analysis, Dr. Ivano Brunner and Dr. Frank Hagedorn for discussion, and Dr. Mathias Mayer, Dr. Patrick Schleppi, Alois Zürcher, and Alessandro Schlumpf for technical support. We would also like to thank the two reviewers for their insightful suggestions and valuable comments.
Conflict of interest
The authors declare that the research was conducted in the absence of any commercial or financial relationships that could be construed as a potential conflict of interest.
Publisher’s note
All claims expressed in this article are solely those of the authors and do not necessarily represent those of their affiliated organizations, or those of the publisher, the editors and the reviewers. Any product that may be evaluated in this article, or claim that may be made by its manufacturer, is not guaranteed or endorsed by the publisher.
References
Abdin, O. A., Zhou, X., Coulman, B. E., Cloutier, D., Faris, M. A., Smith, D. L. (1998). Effect of sucrose supplementation by stem injection on the development of soybean plants. J. Exp. Bot. 49, 2013–2018. doi: 10.1093/jxb/49.329.2013
Adams, H. D., Zeppel, M. J., Anderegg, W. R., Hartmann, H., Landhäusser, S. M., Tissue, D. T., et al. (2017). A multi-species synthesis of physiological mechanisms in drought-induced tree mortality. Nat. Ecol. Evol. 1, 1285–1291. doi: 10.1038/s41559-017-0248-x
Auerbach, S. I., Olson, J. S., Waller, H. D. (1964). Landscape investigations using cæsium-137. Nature 201, 761–764. doi: 10.1038/201761a0
Boyle, M. G., Boyer, J. S., Morgan, P. W. (1991a). Stem infusion of liquid culture medium prevents reproductive failure of maize at low water potential. Crop Sci. 31, 1246–1252. doi: 10.2135/cropsci1991.0011183X003100050033x
Boyle, M. G., Boyer, J. S., Morgan, P. W. (1991b). Stem infusion of maize plants. Crop Sci. 31, 1241–1245. doi: 10.2135/cropsci1991.0011183X003100050032x
Brooks, J. R., Rugh, W. D., Werner, R. A. (2022). “Tree-ring stable isotope measurements: The role of quality assurance and quality control to ensure high quality data,” in Stable isotopes in tree rings: Inferring physiological, climatic and environmental responses. Eds. Siegwolf, R. T. W., Brooks, J. R., Roden, J., Saurer, M. (Cham: Springer International Publishing), 191–213.
Brugnoli, E., Hubick, K. T., von Caemmerer, S., Wong, S. C., Farquhar, G. D. (1988). Correlation between the carbon isotope discrimination in leaf starch and sugars of C(3) plants and the ratio of intercellular and atmospheric partial pressures of carbon dioxide. Plant Physiol. 88, 1418–1424. doi: 10.1104/pp.88.4.1418
Chapin, F. S., III, Schulze, E., Mooney, H. A. (1990). The ecology and economics of storage in plants. Annu. Rev. Ecol. systematics 21, 423–447. doi: 10.1146/annurev.es.21.110190.002231
Choat, B., Brodribb, T. J., Brodersen, C. R., Duursma, R. A., López, R., Medlyn, B. E. (2018). Triggers of tree mortality under drought. Nature 558, 531–539. doi: 10.1038/s41586-018-0240-x
Ciffroy, P., Tanaka, T. (2018). “Modelling the fate of chemicals in plants,” in Modelling the fate of chemicals in the environment and the human body. Eds. Ciffroy, P., Tediosi, A., Capri, E. (Cham: Springer International Publishing), 167–189.
Dietze, M. C., Sala, A., Carbone, M. S., Czimczik, C. I., Mantooth, J. A., Richardson, A. D., et al. (2014). Nonstructural carbon in woody plants. Annu. Rev. Plant Biol. 65, 667–687. doi: 10.1146/annurev-arplant-050213-040054
Dixon, H. H., Joly, J. (1894). On the ascent of sap. Annuals Bot. 8, 468–470. doi: 10.1093/oxfordjournals.aob.a090723
Eggers, J., Juzwik, J., Bernick, S., Mordaunt, L. (2005). Evaluation of propiconazole operational treatments of oaks for oak wilt control. Res. Note NC-390. St. Paul MN: US Department Agriculture For. Service North Cent. Res. Station. 6, 390. doi: 10.2737/NC-RN-390
Ehleringer, J. R., Phillips, S. L., Comstock, J. P. (1992). Seasonal variation in the carbon isotopic composition of desert plants. Funct. Ecol. 6, 396–404. doi: 10.2307/2389277
Eilmann, B., Buchmann, N., Siegwolf, R., Saurer, M., Cherubini, P., Rigling, A. (2010). Fast response of scots pine to improved water availability reflected in tree-ring width and δ13C. Plant Cell Environ. 33, 1351–1360. doi: 10.1111/j.1365-3040.2010.02153.x
Eriksson, U., Jansson, G., Almqvist, C. (1998). Seed and pollen production after stem injections of gibberellin A4/7 in field-grown seed orchards of pinus sylvestris. Can. J. For. Res. 28, 340–346. doi: 10.1139/x97-219
Farquhar, G. D., Ehleringer, J. R., Hubick, K. T. (1989). Carbon isotope discrimination and photosynthesis. Annu. Rev. Plant Physiol. Plant Mol. Biol. 40, 503–537. doi: 10.1146/annurev.pp.40.060189.002443
Feng, J. (1972). Application of plant infusion in fruit tree production. Bull. Agric. Sci. Technol. 3, 27–31.
Feng, J., Ji, J. (2009). Application of tree bottle infusion in first aid of drought resistance of fruit trees. Water Saving Irrigation 7, 54–55.
Galvez, D. A., Landhausser, S. M., Tyree, M. T. (2013). Low root reserve accumulation during drought may lead to winter mortality in poplar seedlings. New Phytol. 198, 139–148. doi: 10.1111/nph.12129
Genet, M., Li, M., Luo, T., Fourcaud, T., Clément-Vidal, A., Stokes, A. (2011). Linking carbon supply to root cell-wall chemistry and mechanics at high altitudes in abies georgei. Ann. Bot. 107, 311–320. doi: 10.1093/aob/mcq237
Gessler, A., Schneider, S., Weber, P., Hanemann, U., Rennenberg, H. (1998). Soluble n compounds in trees exposed to high loads of n: A comparison between the roots of Norway spruce (Picea abies) and beech (Fagus sylvatica) trees grown under field conditions. New Phytol. 138, 385–399. doi: 10.1046/j.1469-8137.1998.00134.x
Gessler, A., Weber, P., Schneider, S., Rennenberg, H. (2003). Bidirectional exchange of amino compounds between phloem and xylem during long-distance transport in Norway spruce trees (Picea abies [L.] karst). J. Exp. Bot. 54, 1389–1397. doi: 10.1093/jxb/erg146
Grabau, L. J., Blevins, D. G., Minor, H. C. (1986). Stem infusions enhanced methionine content of soybean storage protein. Plant Physiol. 82, 1013–1018. doi: 10.1104/pp.82.4.1013
Hagedorn, F., Joseph, J., Peter, M., Luster, J., Pritsch, K., Geppert, U., et al. (2016). Recovery of trees from drought depends on belowground sink control. Nat. Plants 2, 16111. doi: 10.1038/nplants.2016.111
Harsch, M. A., Bader, M. Y. (2011). Treeline form – a potential key to understanding treeline dynamics. Global Ecol. Biogeogr. 20, 582–596. doi: 10.1111/j.1466-8238.2010.00622.x
Hartmann, H., Trumbore, S. (2016). Understanding the roles of nonstructural carbohydrates in forest trees–from what we can measure to what we want to know. New Phytol. 211, 386–403. doi: 10.1111/nph.13955
Hayati, R., Egli, D. B., Crafts-Brandner, S. J. (1995). Carbon and nitrogen supply during seed filling and leaf senescence in soybean. Crop Sci. 35, 1063–1069. doi: 10.2135/cropsci1995.0011183X003500040024x
Hijaz, F., Nehela, Y., Al-Rimawi, F., Vincent, C. I., Killiny, N. (2020). The role of the xylem in oxytetracycline translocation within citrus trees. Antibiotics 9, 691. doi: 10.3390/antibiotics9100691
Hoch, G., Körner, C. (2003). The carbon charging of pines at the climatic treeline: a global comparison. Oecologia 135, 10–21. doi: 10.1007/s00442-002-1154-7
Hoch, G., Körner, C. (2009). Growth and carbon relations of tree line forming conifers at constant vs. variable low temperatures. J. Ecol. 97, 57–66. doi: 10.1111/j.1365-2745.2008.01447.x
Hoch, G., Richter, A., Körner, C. (2003). Non-structural carbon compounds in temperate forest trees. Plant Cell Environ. 26, 1067–1081. doi: 10.1046/j.0016-8025.2003.01032.x
Iglesias, D. J., Tadeo, F. R., Legaz, F., Primo-Millo, E., Talon, M. (2001). In vivo sucrose stimulation of colour change in citrus fruit epicarps: Interactions between nutritional and hormonal signals. Physiol. Plantarum 112, 244–250. doi: 10.1034/j.1399-3054.2001.1120213.x
Iglesias, D. J., Tadeo, F. R., Primo-Millo, E., Talon, M. (2003). Fruit set dependence on carbohydrate availability in citrus trees. Tree Physiol. 23, 199–204. doi: 10.1093/treephys/23.3.199
Joseph, J., Gao, D., Backes, B., Bloch, C., Brunner, I., Gleixner, G., et al. (2020). Rhizosphere activity in an old-growth forest reacts rapidly to changes in soil moisture and shapes whole-tree carbon allocation. Proc. Natl. Acad. Sci. 117, 24885–24892. doi: 10.1073/pnas.2014084117
Khan, H. A., Siddique, K. H. M., Colmer, T. D. (2017). Vegetative and reproductive growth of salt-stressed chickpea are carbon-limited: sucrose infusion at the reproductive stage improves salt tolerance. J. Exp. Bot. 68, 2001–2011. doi: 10.1093/jxb/erw177
Kong, L., Abrams, S. R., Owen, S. J., Graham, H., von Aderkas, P. (2008). Phytohormones and their metabolites during long shoot development in Douglas-fir following cone induction by gibberellin injection. Tree Physiol. 28, 1357–1364. doi: 10.1093/treephys/28.9.1357
Körner, C. (1998). A re-assessment of high elevation treeline positions and their explanation. Oecologia 115, 445–459. doi: 10.1007/s004420050540
Körner, C. (2003). Carbon limitation in trees. J. Ecol. 91, 4–17. doi: 10.1046/j.1365-2745.2003.00742.x
Körner, C. (2006). Plant CO2 responses: an issue of definition, time and resource supply. New Phytol. 172, 393–411. doi: 10.1111/j.1469-8137.2006.01886.x
Kozlowski, T. (1992). Carbohydrate sources and sinks in woody plants. botanical Rev. 58, 107–222. doi: 10.1007/BF02858600
Li, M., Hoch, G., Körner, C. (2001). Spatial variability of mobile carbohydrates within pinus cembra trees at the alpine treeline. Phyton 41, 203–213.
Li, M. H., Hoch, G., Korner, C. (2002). Source/sink removal affects mobile carbohydrates in pinus cembra at the Swiss treeline. Trees-Structure Funct. 16, 331–337. doi: 10.1007/s00468-002-0172-8
Li, M.-H., Jiang, Y., Wang, A., Li, X., Zhu, W., Yan, C.-F., et al. (2018). Active summer carbon storage for winter persistence in trees at the cold alpine treeline. Tree Physiol. 38, 1345–1355. doi: 10.1093/treephys/tpy020
Li, M., Nangong, Z. (2022). Precision trunk injection technology for treatment of huanglongbing (HLB)-affected citrus trees–a review. J. Plant Dis. Prot. 129, 15–34. doi: 10.1007/s41348-021-00510-6
Li, M. H., Xiao, W. F., Shi, P. L., Wang, S. G., Zhong, Y. D., Liu, X. L., et al. (2008a). Nitrogen and carbon source-sink relationships in trees at the Himalayan treelines compared with lower elevations. Plant Cell Environ. 31, 1377–1387. doi: 10.1111/j.1365-3040.2008.01848.x
Li, M. H., Xiao, W. F., Wang, S. G., Cheng, G. W., Cherubini, P., Cai, X. H., et al. (2008b). Mobile carbohydrates in Himalayan treeline trees i. evidence for carbon gain limitation but not for growth limitation. Tree Physiol. 28, 1287–1296. doi: 10.1093/treephys/28.8.1287
Ma, B. L., Dwyer, L. M., Leibovitch, S., Smith, D. L. (1995). Effect of peduncle-perfused nitrogen, sucrose, and growth regulators on barley and wheat amino acid composition. Commun. Soil Sci. Plant Anal. 26, 969–982. doi: 10.1080/00103629509369349
Ma, B., Dwyer, L., Smith, D. (1994). Evaluation of peduncle perfusion for in vivo studies of carbon and nitrogen distribution in cereal crops. Crop Sci. 34, 1584–1588. doi: 10.2135/cropsci1994.0011183X003400060030x
Maguire, A. J., Kobe, R. K. (2015). Drought and shade deplete nonstructural carbohydrate reserves in seedlings of five temperate tree species. Ecol. Evol. 5, 5711–5721. doi: 10.1002/ece3.1819
Martínez-Trinidad, T., Watson, W. T., Arnold, M. A., Lombardini, L., Appel, D. N. (2009). Carbohydrate injections as a potential option to improve growth and vitality of live oaks. J. Arboriculture 35, 142. doi: 10.48044/jauf.2009.025
Martínez-Vilalta, J., Sala, A., Asensio, D., Galiano, L., Hoch, G., Palacio, S., et al. (2016). Dynamics of non-structural carbohydrates in terrestrial plants: a global synthesis. Ecol. Monogr. 86, 495–516. doi: 10.1002/ecm.1231
McDowell, N. G., Beerling, D. J., Breshears, D. D., Fisher, R. A., Raffa, K. F., Stitt, M. (2011). The interdependence of mechanisms underlying climate-driven vegetation mortality. Trends Ecol. Evol. 26, 523–532. doi: 10.1016/j.tree.2011.06.003
McDowell, N., Pockman, W. T., Allen, C. D., Breshears, D. D., Cobb, N., Kolb, T., et al. (2008). Mechanisms of plant survival and mortality during drought: why do some plants survive while others succumb to drought? New Phytol. 178, 719–739. doi: 10.1111/j.1469-8137.2008.02436.x
McDowell, N. G., Sevanto, S. (2010). The mechanisms of carbon starvation: How, when, or does it even occur at all? New Phytol. 186, 264–266. doi: 10.1111/j.1469-8137.2010.03232.x
Mcdowell, N. G., Williams, A., Xu, C., Pockman, W., Dickman, L., Sevanto, S., et al. (2016). Multi-scale predictions of massive conifer mortality due to chronic temperature rise. Nat. Climate Change 6, 295–300. doi: 10.1038/nclimate2873
McLaughlin, S. B., McConathy, R. K., Barnes, R. L., Edwards, N. T. (1980). Seasonal changes in energy allocation by white oak (Quercusalba). Can. J. For. Res. 10, 379–388. doi: 10.1139/x80-063
O’Brien, M. J., Leuzinger, S., Philipson, C. D., Tay, J., Hector, A. (2014). Drought survival of tropical tree seedlings enhanced by non-structural carbohydrate levels. Nat. Climate Change 4, 710–714. doi: 10.1038/nclimate2281
Philipson, J. J. (1985). The promotion of flowering in large field-grown sitka spruce by girdling and stem injections of gibberellin A4/7. Can. J. For. Res. 15, 166–170. doi: 10.1139/x85-027
Picarro (2011). Picarro G2131-i δ 13C high-precision isotopic CO2 CRDS analyzer (Santa Clara, California, USA: Picarro Inc.).
Ross, S. D., Bower, R. C. (1991). Promotion of seed production in Douglas-fir grafts by girdling + gibberellin A4/7 stem injection, and effect of retreatment. New Forests 5, 23–34. doi: 10.1007/BF00037548
Sala, A., Piper, F., Hoch, G. (2010). Physiological mechanisms of drought-induced tree mortality are far from being resolved. New Phytol. 186, 274–281. doi: 10.1111/j.1469-8137.2009.03167.x
Sapes, G., Demaree, P., Lekberg, Y., Sala, A. (2021). Plant carbohydrate depletion impairs water relations and spreads via ectomycorrhizal networks. New Phytol. 229, 3172–3183. doi: 10.1111/nph.17134
Schon, M. K., Blevins, D. G. (1987). Boron stem infusions stimulate soybean yield by increasing pods on lateral branches. Plant Physiol. 84, 969–971. doi: 10.1104/pp.84.4.969
Schönbeck, L., Gessler, A., Schaub, M., Rigling, A., Hoch, G., Kahmen, A., et al. (2020). Soil nutrients and lowered source:sink ratio mitigate effects of mild but not of extreme drought in trees. Environ. Exp. Bot. 169, 103905. doi: 10.1016/j.envexpbot.2019.103905
Schulze, E. D. (1986). Carbon dioxide and water vapor exchange in response to drought in the atmosphere and in the soil. Annu. Rev. Plant Physiol. 37, 247–274. doi: 10.1146/annurev.pp.37.060186.001335
Sevanto, S., Dickman, L. T. (2015). Where does the carbon go?–plant carbon allocation under climate change. Tree Physiol. 35, 581–584. doi: 10.1093/treephys/tpv059
Smith, R. F. (1998). Effects of stem injections of gibberellin A4/7 and paclobutrazol on sex expression and the within-crown distribution of seed and pollen cones in black spruce (Picea mariana). Can. J. For. Res. 28, 641–651. doi: 10.1139/x98-034
Sorochan, J. C. (2002). Sugar in shade: The effects of exogenous fructose applications to turfgrass under reduced light conditions (Ann Arbor, United States: Michigan State University).
Spoehr, H. A. (1942). The culture of albino maize. Plant Physiol. 17, 397–410. doi: 10.1104/pp.17.3.397
Tarpley, L., Vietor, D. M., Miller, F. R. (1994). Internodal compartmentation of stem-infused [14C]Sucrose in sweet and grain sorghum. Crop Sci. 34, 1116–1120. doi: 10.2135/cropsci1994.0011183X003400040052x
Tattar, T. A. (2009). “Injection, infusion, and systemic movement in trees,” in Proceedings of the national oak wilt symposium; 2007 June 4–7 (Austin, TX: Texas Forest Service), 169–173.
Tattar, T. A., Tattar, S. J. (1999). Evidence for the downward movement of materials injected into trees. J. Arboriculture 25, 325–332. doi: 10.48044/jauf.1999.043
Taylor, A. F. S., Fransson, P. M., Högberg, P., Högberg, M. N., Plamboeck, A. H. (2003). Species level patterns in 13C and 15N abundance of ectomycorrhizal and saprotrophic fungal sporocarps. New Phytol. 159, 757–774. doi: 10.1046/j.1469-8137.2003.00838.x
Timofeeva, G., Treydte, K., Bugmann, H., Rigling, A., Schaub, M., Siegwolf, R., et al. (2017). Long-term effects of drought on tree-ring growth and carbon isotope variability in scots pine in a dry environment. Tree Physiol. 37, 1028–1041. doi: 10.1093/treephys/tpx041
Wang, X., Yu, F. H., Jiang, Y., Li, M. H. (2021). Carbon and nutrient physiology in shrubs at the upper limits: a multispecies study. J. Plant Ecol. 14, 301–309. doi: 10.1093/jpe/rtaa097
Wardle, P. (1993). “Causes of alpine timberline: a review of the hypotheses,” in Forest development in cold climates (Laugarvatn, Iceland: Plenum Press), 89–103.
Went, F. W., Carter, M. (1948). Growth response of tomato plants to applied sucrose. Am. J. Bot. 35, 95–106. doi: 10.1002/j.1537-2197.1948.tb05192.x
Zeller, B., Brechet, C., Maurice, J.-P., Le Tacon, F. (2007). 13C and 15N isotopic fractionation in trees, soils and fungi in a natural forest stand and a Norway spruce plantation. Ann. For. Sci. 64, 419–429. doi: 10.1051/forest:2007019
Zeng, F., Yi, C., Mei, Z., Gao, X., Zhang, Z. (2010). Technology of infusion dropper for tomato plants in spring plant protection area. Vegetables Yangtze River 1, 28–29.
Zhou, X. M., Abdin, O. A., Coulman, B. E., Clontier, D. C., Faris, M. A., Smith, D. L. (2000). Carbon and nitrogen supplementation to soybean through stem injection and its effect on soybean plant senescence. J. Plant Nutr. 23, 605–616. doi: 10.1080/01904160009382044
Zhou, X. M., Dutilleul, P., MacKenzie, A. F., Madramootoo, C. A., Smith, D. L. (1997). Effects of stem-injected sucrose on grain production, dry matter distribution, and chlorophyll fluorescence of field-grown corn plants. J. Agron. Crop Sci. 178, 65–71. doi: 10.1111/j.1439-037X.1997.tb00352.x
Keywords: 13C-labelled glucose, exogenous sugars, carbon limitation, carbon physiology, methodology, tree infusion
Citation: Zhang Y-L, Yang Y, Saurer M, Schaub M, Gessler A, Lehmann MM, Rigling A, Walser M, Stierli B, Hajjar N, Christen D and Li M-H (2023) Sugar infusion into trees: A novel method to study tree carbon relations and its regulations. Front. Plant Sci. 14:1142595. doi: 10.3389/fpls.2023.1142595
Received: 11 January 2023; Accepted: 14 February 2023;
Published: 23 February 2023.
Edited by:
Deliang Kong, Henan Agricultural University, ChinaCopyright © 2023 Zhang, Yang, Saurer, Schaub, Gessler, Lehmann, Rigling, Walser, Stierli, Hajjar, Christen and Li. This is an open-access article distributed under the terms of the Creative Commons Attribution License (CC BY). The use, distribution or reproduction in other forums is permitted, provided the original author(s) and the copyright owner(s) are credited and that the original publication in this journal is cited, in accordance with accepted academic practice. No use, distribution or reproduction is permitted which does not comply with these terms.
*Correspondence: Mai-He Li, bWFpaGUubGlAd3NsLmNo
†These authors have contributed equally to this work