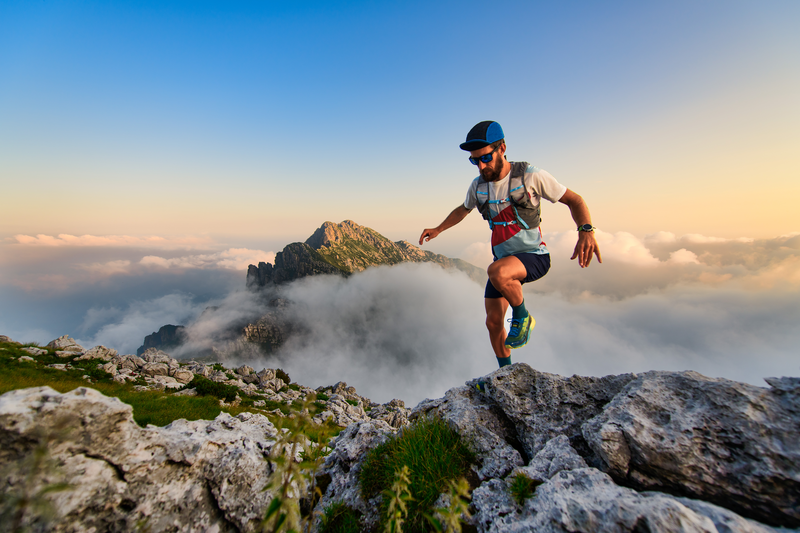
94% of researchers rate our articles as excellent or good
Learn more about the work of our research integrity team to safeguard the quality of each article we publish.
Find out more
REVIEW article
Front. Plant Sci. , 23 March 2023
Sec. Plant Abiotic Stress
Volume 14 - 2023 | https://doi.org/10.3389/fpls.2023.1141138
This article is part of the Research Topic Environmental Pollutants in Agroecosystem: Toxicity, Mechanism, and Remediation View all 11 articles
Cadmium is one of the most toxic heavy metal elements that seriously threaten food safety and agricultural production worldwide. Because of its high solubility, cadmium can easily enter plants, inhibiting plant growth and reducing crop yield. Therefore, finding a way to alleviate the inhibitory effects of cadmium on plant growth is critical. Silicon, the second most abundant element in the Earth’s crust, has been widely reported to promote plant growth and alleviate cadmium toxicity. This review summarizes the recent progress made to elucidate how silicon mitigates cadmium toxicity in plants. We describe the role of silicon in reducing cadmium uptake and transport, improving plant mineral nutrient supply, regulating antioxidant systems and optimizing plant architecture. We also summarize in detail the regulation of plant water balance by silicon, and the role of this phenomenon in enhancing plant resistance to cadmium toxicity. An in-depth analysis of literature has been conducted to identify the current problems related to cadmium toxicity and to propose future research directions.
Cadmium is a nonessential, highly toxic, heavy metal element and a widespread environmental pollutant that is persistent, nonbiodegradable and bioaccumulative even at low concentrations (Hussain et al., 2019). Upon entering the food chain, cadmium seriously threatens the health of animals and humans (Krämer and Chardonnens, 2001; Fulekar et al., 2009; Liu et al., 2019). Contamination of soil with cadmium severely affects plant growth resulting in leaf yellowing, stunted growth, reduced yield, reduced enzyme activity, reactive oxygen species (ROS) accumulation and protein denaturation (Bovetl et al., 2003; Fan et al., 2010; Gallego et al., 2012; Rizwan et al., 2017; Rizwan et al., 2018; Hamid et al., 2019). Owing to its high mobility in soil-plant systems, cadmium is easily absorbed by plants. Therefore, a reliable strategy to reduce cadmium toxicity and minimize its accumulation in plants is urgently needed to improve plant growth and ensure food safety.
After oxygen (47%), silicon is the second most abundant element (27.7%) in the Earth’s crust. Although silicon is not essential for the survival of terrestrial higher plants, it plays a variety of roles to ehhance plant growth, especially under biotic and abiotic stress conditions (Epstein, 1994; Luyckx et al., 2017). Silicon can improve soil properties, such as enhancing soil microbial communities, regulating nutrient cycling, enhancing soil water retention and providing a more favorable growing environment for plants (Bhardwaj et al., 2022). Silicon is abundant in nature and often exists in inert forms that are inaccessible to plants. In contrast, soluble, bioavailable monosilicic/protosilicic acid [Si(OH)4/H4SiO4] is typically present in soil at concentrations of (0.1–0.6 mM) (Epstein, 1994). Plants absorb silicon from the soil exclusively in the form of H4SiO4, which is the only plant-available form of silicon in the soil. After absorption, H4SiO4 is transported radially into the root cortex via the cytoplasm or plasmodesmata (Hodson and Sangster, 1989; Ma et al., 2006). Once H4SiO4 is loaded into the xylem, it is rapidly translocated to the aboveground plant parts through the transpiration stream (Lux et al., 2020). In organs such as stems and leaves, H4SiO4 is unloaded from the xylem into the extracellular apoplastic space. Subsequently, through transpiration and a series of biochemical reactions, H4SiO4 forms insoluble silica molecules and is ultimately deposited as a polymer of hydrated amorphous silica (SiO2-nH2O) in the cell lumen, cell wall, intercellular space or between the epidermal cell wall and the cuticle (Hodson and Sangster, 1989; Ma et al., 2006).
Silicon has been shown to benefit plant growth and reduce the toxic effects of cadmium (Ma and Yamaji, 2008; Gao et al., 2018; Hajiboland et al., 2018; Kollárová et al., 2019; Gheshlaghpour et al., 2021). In this review, recent research on the mechanism of silicon-induced mitigation of cadmium toxicity in plants is summarized. To date, studies on the mechanism of silicon-mediated mitigation of cadmium toxicity have mainly focused on reducing cadmium uptake and transport, increasing antioxidant properties, improving photosynthesis, promoting nutrient uptake and maintaining the integrity of the cell structure. This review highlights the novel hypothesis that silicon alleviates cadmium-induced damage to plants through the ability to alter water relations to dilute the cadmium concentration in plant tissues.
Cadmium accumulation can severely inhibit plant growth. However, plant biomass and morphological traits, such as leaf length, leaf area, root length, root volume and root tip number, are enhanced by silicon under cadmium toxicity (Farooq et al., 2013; Rahman et al., 2021c; Seyed, 2022) (Table 1). Disruption of photosynthesis is a major impact of cadmium toxicity, which can be alleviated by silicon, for example, in rice (Nwugo and Huerta, 2008; Li et al., 2018), cucumber (Feng et al., 2010), cotton (Farooq et al., 2013), maize (Vaculík et al., 2015), wheat (Sun et al., 2016; Shi et al., 2018) and lupin (Sun et al., 2016). Chloroplasts are organelles that perform photosynthesis, and their ultrastructure plays a vital role in this process. With the accumulation of cadmium in tissues, the concentration of ROS increases, and the cell membrane system is damaged, especially when the chloroplast membrane is damaged, which will inhibit photosynthesis (Feng et al., 2010; Pereira AS et al., 2018). Silicon application has been reported to alleviate cadmium-induced cell ultrastructure damage in a variety of plant species (Zhao et al., 2022).
In rice, silicon ameliorates cadmium-induced changes in chloroplast ultrastructure by ensuring the integrity of chloroplasts and membranes (Guo et al., 2018). In addition, silicon-enhanced regeneration of cell walls by maize protoplasts may also be used to maintain chloroplast structure (Kollárová et al., 2019). Cadmium negatively affects the formation of cystoids in the chloroplasts of bundle sheath cells, which is mitigated by silicon and the improved cystoid formation may contribute to enhance photosynthesis and then increase biomass (Vaculík et al., 2015). Cadmium inhibits the activity of chlorophyll synthase and increases the activity of chlorophyllase, thus decreasing chlorophyll content (Ekmekçi et al., 2008; Vaculík et al., 2015). Silicon increases the content of photosynthetic pigments in tobacco under cadmium toxicity and improves the efficiency of light energy utilization (Feng et al., 2010; Luyckx et al., 2021). Cadmium toxicity also causes changes in leaf structure, stomatal size and density, which ultimately inhibit photosynthesis (Shi and Cai, 2008). However, in the presence of silicon, stomatal density is increased and results in a high gas diffusion rate (Cipriano et al., 2021). Moreover, silicon deposition is beneficial for the plant to maintain an erect habit, especially under stress and to promote leaf positioning favorable for light interception and efficient photosynthesis (Epstein, 1994).
The phytotoxic effects of cadmium present in soil are not exerted until it is absorbed by plants and transported to various tissues and organs. There are two means for cadmium to enter the root: the apoplast pathway and the symplast pathway (Redjala et al., 2009; Song et al., 2017). In the apoplast pathway, cadmium present in the soil is absorbed by plants roots via free diffusion; however, instead of entering the cells, cadmium passes through voids in the cell walls of rhizodermis and cortex to enter the xylem and phloem (Degryse et al., 2006; Tao et al., 2019). The symplast pathway refers to the transport of cadmium through plasmodesmata from one cell (protoplast) to the other using cytoplasmic continuum; and cadmium transport across membranes, this process is mediated by transporters located in the cell wall (Kreszies et al., 2018). Silicon interferes with these two transport pathways in various ways, inhibiting the accumulation of cadmium in plants, thereby reducing its harmfulness to many plants (Kabir et al., 2016; Song et al., 2021).
The formation of a physical barrier in the apoplast space reduce cadmium transport rates, thereby reducing the accumulation and distribution of cadmium in the cytoplasm (Ye et al., 2012; Zhang et al., 2014; Luyckx et al., 2017; Riaz et al., 2022). Silicon creates a physical barrier through the formation of specific cells (siliceous cells) that reduce the uptake and transport of cadmium in plants (Ma and Yamaji, 2008). Siliccon is deposited in root tissues through three main modes: (1) impregnation of the endodermal cell wall; (2) formation of silica aggregates associated with the inner tangential wall of the endodermal cells;and (3) formation of silica aggregates or phytoliths in specific cells associated with thick-walled sclerenchymatous tissues (Lux et al., 2020). After its absorption by plant roots exclusively as H4SiO4, silicon binds to cell wall components including hemicellulose, pectin, callose, cellulose and dextran to form SiO2 precipitates (Guerriero et al., 2016). Experiments on rice suspension cells show that hemicellulosic polysaccharides in the cell wall exhibit greater silicon-binding capacity than pectic polysaccharides (Ma et al., 2015). Silicon significantly increases the content of total polysaccharides and their components (pectin and cellulose) in the root cell wall and improves the ability of cadmium to bind to pectin and cellulose, which decreases cadmium transport efficiency through the apoplast pathway (Cai et al., 2022). The specific amino acid composition of cell-wall-localized proteins may also lead to silicification (Guerriero et al., 2016). Silicon increases the cation exchange capacity of the cell wall; cadmium binds to the cell wall to a greater extent in the presence of silicon than in its absence (Lukačová et al., 2013). Heavy deposition of silicon near the cortex, as aggregates or phytoliths, may reduce the cell wall porosity of the internal tissues of the root, especially in the inner cortex (Lux et al., 2020). Massive deposition of SiO2 near the endodermis physically blocks plasmodesmata flow through the root, thereby inhibiting the plasmodesmata transport of cadmium to reduce its toxic effects on plant cells (Shi et al., 2005).
In addition, the chemically heterogeneous walls resulting from silicon modification may provide additional binding sites for cadmium (Liu et al., 2013a). In rice and maize, silicon application enhances cell wall suberization and lignification and promotes Casparian strip formation (Fleck et al., 2011; Vaculík et al., 2012). In wheat plants exposed to long-term cadmium stress, silicon promoted suberin deposition in the root endodermis and decreased cadmium concentration in the apoplastic fluid of the shoot by almost 38% (Wu et al., 2019). Additionally, the ratio of root endothelium cell wall to the whole cell volume was higher in the cadmium+silicon treatment than in the cadmium-only treatment (Lukačová et al., 2013).
More than 90% of the H4SiO4 taken up by roots is transported to the aboveground organs, along with the transpiration water flow, through the xylem. In this process, silicon is also deposited in the leaf epidermal cell wall, which decreases the efficiency of cadmium transport (Lux et al., 2003; Keller et al., 2015). When cadmium reaches the leaf, silica aggregates in the cell wall, as a physical barrier, suppressing cadmium from entering the cells (Farnezi et al., 2020).
In addition to the formation of physical barriers in the cell wall, silicon also affects the expression of genes involved in cadmium uptake and transport. The main cadmium transporter genes encode proteins belonging to the ZRT-like protein (ZIP), IRT-like protein (IRT), natural resistance-associated macrophage protein (Nramp), yellow stripe-like transporter (YSL), low affinity cation transporter (LCT), P-type adenosine triphosphatase (ATPase, HMA) and cation diffusion facilitator (CDF) families. Among these proteins, ZIPs, IRTs, Nramps, YSLs and LCTs mediate cadmium uptake from the soil by roots and its transport to aboveground plant parts, while HMAs and CDFs are involved in the efflux of cadmium to the cytoplasm or its transport into the vacuoles. Nramp5, a key transporter protein for cadmium uptake by the root system, is located at the plasma membrane and is highly expressed in the root maturation zone, responsible for the transport of cadmium from the outside into the root cells (Sasaki et al., 2012; Riaz et al., 2021). Silicon downregulates the expression of Nramp5 in rice cells under cadmium toxicity (Ma et al., 2015). Treatment of rice suspension cells with silicon nanoparticles (SiNPs) reduces the expression levels of the genes encoding the cadmium transport proteins OsLCT1 and OsNramp5 (Cui et al., 2017). Due to the unique N-terminal sequence, the ATPase in plants is also named as HMAs. Among them, HMA3 is the main protein responsible for the transport of cadmium chelates into vesicles (Mohabubul Haque et al., 2022). The OsHMA3 mediates the sequestration of cadmium in the root cell vacuole (Riaz et al., 2021). The transport of cadmium into vesicles may be an important mechanism for cadmium detoxification in plants (Li et al., 2021). Consistent with this, silicon upregulates the expression of OsHMA3 in the root system (Cai et al., 2022). Cadmium toxicity increases the expression of four genes (Nramp5, Nramp1, HMA2 and HMA3) that encode cadmium transporter proteins in rice. Exogenous application of silicon downregulates the expression of these four genes under cadmium stress (Chen et al., 2019). A homolog of OsHMA3, OsHMA2, localizes to the plasma membrane of root pericycle cells and is involved in the root-to-shoot translocation of cadmium; however, silicon downregulates the activity of OsHMA2 (Shao et al., 2017). Located in the rice bast, OsLCT1 mediates the transport of cadmium to the grain (Uraguchi et al., 2011). Silicon reduces the promotion of OsLCT1 expression by cadmium (Greger et al., 2016). Thus, silicon application can minimize cadmium-induced damage to plants by reducing the uptake and transport of cadmium (Rizwan et al., 2012; Vaculík et al., 2012).
The coprecipitation of silicon and cadmium in soil reduces the availability of soil cadmium (Zhao et al., 2020). Silicon improves soil physical and chemical properties, reduces soil acidity (i.e., increases soil pH) and coprecipitates with heavy metals in the soil, forming metal-silicate complexes, to reduce the uptake of heavy metals by plants (Khan et al., 2021; Bhardwaj et al., 2022). Soluble silicates hydrolyze in soil solution and produce gel-like metasilicic acid, which binds heavy metals and changes the form of the metal from toxic to non-toxic (Bhat et al., 2019). Consistent with this result, the addition of SiNPs to cadmium-contaminated soil decreases the content of biologically effective cadmium in the soil (Hussain et al., 2019). Studies show that silicon-rich amendments fix copper, cadmium, and zinc in polymetallic acidic soils by increasing soil pH, and that metals are mainly in the form of silicates, phosphates, and hydroxides during amendment treatment exist (Gu et al., 2011). The use of silicon in the form of calcium silicate also changes the distribution of cadmium and zinc in soils, which are present in more stable forms, such as complexes with organic matter and crystalline iron oxide (Da Cunha et al., 2008). Among the four amendments tested on cadmium-contaminated soil, silicon showed the greatest reduction in the concentration of fast-acting cadmium (56.28%), greatly changed the soil microbial community (especially acidophilus and chiguria) and significantly reduced the bioavailability of cadmium to plants (Song et al., 2021).
The coprecipitation of silicon and metals in the cell walls of roots and leaves is an additional means of mitigating metal toxicity (Figure 1) (Wang et al., 2000; Ye et al., 2012; Cai et al., 2022). Cadmium and zinc co-precipitate with silicon in the cell walls of epidermal, ectodermal, endodermal, mesocolonial sheath and xylem cells in maize roots (Da Cunha and Do Nascimento, 2009). Similarly, the coprecipitation of silicon with cadmium in rice stems reduces the metal concentration in the leaves (Gu et al., 2011). Silicon coprecipitates with cadmium and zinc in the leaf cell cytoplasm and vesicles to form Si-metal complexes that contribute to cellular detoxification (Da Cunha and Do Nascimento, 2009). Increased silicon content in the cell wall may provide additional cadmium-binding sites (Cui et al., 2017). In the rice cell walls, electrostatic interactions between the silicon–hemicellulose matrix and cadmium cations, co-deposition of silicon with cadmium inhibit cadmium ion uptake and lead to in vivo detoxification of cadmium (Ma et al., 2015). This mechanism explains how silicon inhibits cadmium uptake and transport at the single-cell level. Moreover, silicon application may lead to an increase in root secretions, which can reduce heavy metal uptake by the roots because of the chelation of heavy metals (Keller et al., 2015).
Figure 1 Mechanisms of silicon on reducing cadmium toxicity. The underlying mechanism includes (і) enhancing plant growth by promoting photosynthesis and nutrition uptake; (ii) reduction of cadmium accumulation due to silicon decreases cadmium uptake&transport gene expression and its deposition forms a physical barrier; (iii) the co-precipitation, compartmentalization of Cd2+. Cartoon pictures were created with BioRender.com.
Vacuoles are important storage organelles for many ions, and high-level accumulation of toxic ions in vacuoles is an important strategy employed by plants to enhance heavy metal tolerance (Sterckeman and Thomine, 2020).
Phytochelatins (PCs) are a class of small biological molecules, with the structure (γ-Glu-Cys)n-Gly (n=2~11), synthesized by plant chelating peptide synthase (PCS) upon plant exposure to cadmium or other metals and oxygen-containing anions (Satofuka et al., 2001; Vestergaard et al., 2008). In plant cells, cadmium usually binds to PCs to form cadmium-PC complexes, which are then transported to and sequestered in vesicles to avoid further damage to cellular organelles (Shanmugaraj et al., 2019). Silicon increases the concentration of cadmium bound to PCs in rice (Cai et al., 2022). Similar result was also proved that the concentration of PCs with expression of OsPCS1 in roots of cadmium-stressed was significantly induced when subjected to silicon treatment (Bari et al., 2020). PC2 and PC3 are the main PCs that synthesized in the cytoplasm of root cells after cadmium exposure (Volland et al., 2013). Silicon promotes the production of PC2 and PC3, thereby sequestering more PC-bound cadmium in the vacuole and limiting the migration of cadmium from roots to shoots (Wei et al., 2021). Glutathione both acts as a precursor for production of PCs which chelate cadmium in cells to form PC-cadmium complexes and also promotes the transport of these complexes into the vacuoles to reduce the cadmium concentration in the cytoplasm (Huang et al., 2021; Luyckx et al., 2021). Silicon increases glutathione and PC synthesis, allowing the plants to efficiently cope with oxidative stress through the improvement of cadmium sequestration on thiol groups in the roots (Luyckx et al., 2021). Stimulation of glutathione synthesis by silicon under cadmium stress was also found in rice (Farooq et al., 2016), sugar beet (Kabir et al., 2021), maize (Singh et al., 2019), wheat (Thind et al., 2020), and pepper (Kaya et al., 2020).
Heavy metals form complexes with PCs, which are transported to and sequestered in vesicles by ATP-binding cassette (ABC) transporters (Sharma et al., 2016). OsHMA3, a heavy metal ATPase, is a cadmium efflux protein to the vesicles and facilitates cadmium sequestration in the root vesicles (Sasaki et al., 2014). Rice under cadmium toxicity was mostly up-regulated in ABC transporter proteins after silicon treatment, which may contribute to the compartmentalization of cadmium in vesicles (Sun et al., 2022). The addition of SiNPs increases the expression of rice heavy metal ATPase 3 (OsHMA3) localized in the vacuole membrane and increases cadmium translocation into the vacuoles, thus reducing the effects of cadmium toxicity (Cui et al., 2017). Another heavy metal binding ligand, metallothioneins (MTs), have also been found in many plant species (Cobbett and Goldsbrough, 2002).
High concentrations of cadmium reduce the ability of plants to absorb and transport nutrients and disrupt mineral metabolism, leading to nutrient deficiencies (Luyckx et al., 2021). Cadmium competes with mineral nutrient ions for the same transport system, resulting in the shortage of nutrients required for plant growth and development (Alcántara et al., 1994; Sarwar et al., 2010; Nazar et al., 2012). Silicon facilitates the uptake and utilization of plant nutrients was summarized in Table 2. This may be one of the reasons why silicon promotes plant growth under cadmium toxicity (Pilon et al., 2013; Hernandez-Apaolaza, 2014). Exogenous application of silicon promotes the uptake of nutrients by plant roots, including mineral elements such as Zn, Fe, Mn, Ca, Mg, P, and K, thereby offsetting the shortage caused by cadmium and thus promoting plant growth (Keller et al., 2015; Wang et al., 2015; Rahman et al., 2021c). The effect of silicon on the concentration of mineral elements varies depending on the crop type, plant organism and type of mineral element. In poplar callus cells, silicon attenuates plasma membrane damage caused by cadmium toxicity and promotes the uptake and transport of nutrients including Ca, K, Mg, P, Fe, Zn, and Mn after 3 and 9 weeks of cadmium treatment (Kučerová et al., 2020). In hemp, cadmium stress increases the content of Ca, P and S in stems and leaves and that of Fe in roots but decreases the content of Fe in leaves. Additionally, silicon greatly increases Fe concentration in the leaf and alleviates cadmium-induced reduction in Ca concentration in the root and cadmium-induced accumulation of P in the shoot (Luyckx et al., 2021). On the contrary, silicon has no significant impact on S accumulation in the shoot under cadmium stress (Luyckx et al., 2021). Foliar application of silicon increases the concentration of N and P; but decreases the concentration of Na. Because silicon has no effect on the concentration of K, treatment with silicon decreases the ratio of K+/Na+ (Rady et al., 2019). In ajwain, cadmium decreases Fe, Mg, K and Ca both in shoot and root (Javed et al., 2020).
Although some studies have been conducted to reveal the effect of silicon on plant mineral elements by silicon under cadmium stress, most of them only demonstrated the results, lacking the exploration of the deep mechanism analysis. Thus, how silicon regulates the uptake of mineral elements and how these mineral elements enhance cadmium resistance in plants remain largely unclear.
The concentration of ROS in plant cells is normally low and poses little danger to cellular functioning. However, upon exposure to heavy metal toxicity, ROS concentrations increase dramatically, triggering a series of physiological and biochemical changes, which eventually cause yield reduction and even plant death. Cadmium increases the production of ROS in plants and causes oxidative stress (Schutzendubel et al., 2001; Farooq et al., 2013). Under cadmium toxicity, large amounts of ROS, including singlet oxygen (1O2), superoxide ion (), hydrogen peroxide (H2O2) and hydroxyl radical (OH−), accumulate in plant cells (Gallego et al., 2012; Liu et al., 2013b). Plants synthesize antioxidant enzymes, such as superoxide dismutase (SOD), peroxidase (POD), catalase (CAT), ascorbate peroxidase (APX) and guaiacol peroxidase (GPX), to cope with the adverse effects of high ROS accumulation under cadmium stress (Li et al., 2018). Silicon can enhance antioxidant capacity of plants (Figure 2). Silicon dioxide nanoparticles stimulate the antioxidant defense system in wheat and rice (Tripathi et al., 2017). The application of silicon increases the activities of SOD, POD, CAT, APX and GPX in different wheat cultivars under cadmium toxicity and the degree of enhancement in antioxidant enzyme activity is higher in cadmium-tolerant cultivars than in cadmium-sensitive cultivars (Naeem et al., 2018; Hussain et al., 2019). Foliar spray of silicon on rice plants under cadmium toxicity increases the branch POD and SOD activities and decreases CAT activity (Wang et al., 2015). Similarly, in ginger, silicon increases SOD, POD and CAT activities under cadmium toxicity (Chen et al., 2020). Exogenous silicon treatment increases SOD, CAT and APX activities in cucumber leaves. However, in tomato leaves, silicon increases only SOD activity and decreases other antioxidant enzyme activities under cadmium toxicity (Wu et al., 2015). Moreover, silicon promotes the rapid accumulation of polyamines, scavenges free radicals and prevents heavy metal-induced oxidative damage (Das et al., 2022). Spray application of silicon onto the leaf surface enhances the production of glutamyl kinase (the first committed enzyme in the proline biosynthesis pathway) and decreases the production of proline oxidase (responsible for the denaturation of proline molecules) to promote proline synthesis and help plants to cope with oxidative stress (Elnaz et al., 2021).
Figure 2 Silicon-induced antioxidant defense system mitigates the toxic effects of cadmium. Cartoon pictures were created with BioRender.com.
By contrast, some studies demonstrated that silicon reduces antioxidant enzyme activity under cadmium stress. For instance, compared to cadmium treatment alone, the presence of silicon reduces the SOD, POD, CAT and APX activity in Solanum nigrum L. (Liu et al., 2013b). In wheat, cadmium stress increased SOD, CAT and POD activities by 77.18%, 76.95% and 108.33%, respectively, compared with the control, whereas foliar application of 4.50 mM silicon improved the SOD, POD and CAT activities by 23.91%, 32.01% and 69.76%, respectively (Heile et al., 2021). These contrasting results may be caused by differences in plant species, cultivars, age, silicon source (silicon or nano-silicon), treatment time and experimental conditions (Table 3).
Water is the most important constituent of living organisms. Every plant cell requires water for survival. Without water, plants cannot perform photosynthesis (the process of food production), respiration nutrient translocation to different plant parts. Adverse environmental conditions such as drought, salt, evaporation, chilling and heavy metal toxicity can disturb plant water balance, which in turn inhibits plant growth (Bray, 1997; Tchounwou et al., 2012).
Cadmium is mainly present in the soil as the divalent cation (Cd2+), which is absorbed by the root system and then translocated to the shoot through the xylem along the transpiration stream (Sterckeman and Thomine, 2020). Because transpiration pull is the main driving force for cadmium transport from the root system to the aboveground parts, lower transpiration rate is likely to reduce cadmium accumulation in the leaf (Uraguchi et al., 2009; Zulfiqar et al., 2021). The application of silicon significantly reduces stomatal conductance and subsequently constrains the transpiration rate, which reduces cadmium transport from roots to shoots in wheat (Naeem et al., 2018). Similarly, silicon reduces stomatal conductance and transpiration rate in Cannabis sativa under cadmium stress (Luyckx et al., 2021). In rice, cadmium significantly reduces transpiration, and the reduction in transpiration increases further upon the addition of silicon. Interestingly, the transpiration rate of plants treated with 0.2 mM silicon is significantly lower than that of plants treated with 0.6 mM silicon (Nwugo and Huerta, 2008). Rizwan et al. (2016) indicated that silicon-induced reduction in transpiration would reduce cadmium translocation to shoots. Additionally, silicon application decreases transpiration rate by 65% and 42% in - cadmium and + cadmium plants, respectively (Nwugo and Huerta, 2011).
Contrary to the above result, there are accumulating experiments proved that under cadmium stress silicon enhances gas exchange indices (especially represented by transpiration rate and stomatal conductance) in rice (Table 4; Gao et al., 2018; Li et al., 2018; Rehman et al., 2019; Rizwan et al., 2019; Sohail et al., 2019; Huang et al., 2021; Mapodzeke et al., 2021), wheat (Alzahrani et al., 2018; Shi et al., 2018; Ali et al., 2019; Hussain et al., 2019), peas (Jan et al., 2018), beans (Rady et al., 2019; Ahmad et al., 2021; El-Saadony et al., 2021), maize (Sohail et al., 2019), gladiolus (Zaheer et al., 2018), pepper (Kaya et al., 2020), ajwain (Javed et al., 2020), cabbage (Yang et al., 2018). In comparison with control (non-stressed) conditions, cadmium stress conditions reduce photosynthetic rate (37.29%), transpiration rate (37.28%), stomatal conductance (38.09%) and chlorophyll content (14.13%). Silicon supplementation improves plant tolerance to cadmium stress. The greatest influence on all physio-biochemical attributes was noticed in plants supplemented with 4.50 mM silicon under cadmium stress. In the presence of 4.50 mM silicon, the most promising level, increases in the photosynthetic rate (45.77%), rate of transpiration (38.60%), stomatal conductance (42.85%), chlorophyll contents (45.77%) and water use efficiency (7.77%) compared with the relevant control (Heile et al., 2021). In rice, foliar spray of silicon reduces the accumulation of cadmium in leaves but increases the transpiration rate and stomatal conductance of leaves, compared with the control, under cadmium stress. Furthermore, structural equation modeling indicated that transpiration rate and stomatal conductance have negative effects on cadmium concentration in rice (Gao et al., 2018). Compared with the control (no-stress condition), cadmium stress reduces the transpiration rate and stomatal conductance of wheat leaves, but external silicon application significantly alleviates the inhibitory effect of cadmium on these indicators and subsequently enhances the water use efficiency (WUE) of plants (Howladar et al., 2018). Notably, in this study, relative leaf water content (RWC), a common indicator of leaf water balance, was also enhanced by silicon under stress (Howladar et al., 2018). Similar results have also been reported in bean (Rady et al., 2019; Ahmad et al., 2021; El-Saadony et al., 2021), pea (Jan et al., 2018) and pepper (Kaya et al., 2020). Silicon addition maintains RWC in wheat cells and tissues under cadmium poisoning (Alzahrani et al., 2018; Heile et al., 2021). The addition of silicon significantly increases RWC to maintain cell expansion pressure and protects plants from wilting and cell relaxation to mitigate plant cadmium toxicity (Rahman et al., 2021a). In addition, and more importantly, an increase in the leaf water content by silicon could dilute the cadmium concentration, subsequently reduce cadmium toxicity in plants (Kaya et al., 2020).
Plant leaf water content depends on the dynamic balance between water loss from leaves and water uptake by roots; thus, changes in both these processes affect the plant water status. Transpiration is the primary mode of water loss from shoots (Bernacchi and Vanloocke, 2015; Wang et al., 2018). As mentioned above, silicon-induced decrease in transpiration not only reduces the translocation of cadmium from roots to leaves but also reduces leaf water loss, resulting in higher RWC than the cadmium treatment (Chen et al., 2018).
In addition to water loss, water uptake also influences the RWC (Steudle, 2000; Liu et al., 2014). The RWC of wheat significantly decreases under drought, salinity and cadmium stresses. However, the presence of silicon significantly reduces the destructive effects of the above stresses on RWC. According to these data, we can infer that the mitigation effect of silicon on RWC is similar in the three stress conditions. In other words, the effect of silicon on leaf water content is independent of the stress type (Alzahrani et al., 2018). Therefore, we can hypothesize that the mechanism of silicon on enhancing water balance revealed in other stresses may also apply to cadmium toxicity. Liu et al. (2014) revealed that silicon application maintains the water balance in sorghum by elevating root water uptake under stress conditions. This phenomenon has been confirmed in other studies (Liu et al., 2015; Zhu et al., 2015; Chen et al., 2016).
Water channel proteins play a major role in regulating root water uptake and shoot water transport under stress (Hachez et al., 2012). Silicon-mediated increase in the expression of the plasma membrane intrinsic protein water channel protein (PIP) gene is associated with an increase in root hydraulic conductivity and water uptake (Rios et al., 2017). An effect of silicon on aquaporin gene expression has been observed in sorghum (Liu et al., 2014), cucumber (Zhu et al., 2015) and barley (Celikkol and Erkan, 2016). In tobacco, we recently observed that the expression of NtPIP genes was strongly enhanced under cadmium stress (unpublished data). In addition to the expression of aquaporin genes, the content of aquaporin proteins is also influenced by silicon. In rapeseed plants under drought stress, silicon application promotes the expression of BnPIP1, BnPIP2-1-7 and BnTIP1;1 and the accumulation of aquaporins (Gao et al., 2006; Saja-Garbarz et al., 2022). These findings show that water channel protein transport activity can be regulated at the post-transcriptional level. The effect of silicon on plant water balance under cadmium stress is summarized in Figure 3.
Figure 3 Silicon-diluted cadmium concentration by maintaining the water balance (increasing root water uptake and decreasing leaf water loss). Cartoon pictures were created with BioRender.com.
There is increasing evidence that silicon can improve plant growth under exposure to cadmium toxicity. The reported mechanisms include complexation and coprecipitation of silicon with cadmium in different plant organs, compartmentalization of cadmium in different subcellular organelles, structural alteration of the plant, reduction of cadmium content, regulation of cadmium transporter gene expression, improvement of plant mineral nutrient supply (Figure 1), enhancement of ROS scavenging (Figure 2) and maintenance of leaf water balance (Figure 3).
However, the molecular mechanism underlying the effect of silicon on plant reactions remains unknown. Further research is needed to determine whether silicon directly or indirectly participates in plant physiological responses or gene expression regulation under cadmium stress. An increasing number of studies show that the function of silicon is mediated by signaling messengers, such as plant hormones, ROS and Ca2+. Thus, the crosstalk between silicon and signaling messengers may constitute an important research focus to elucidate the mechanism of silicon-mediated increase in cadmium tolerance in plants.
LH: Writing-original draft; SJ: Visualization, Writing-original draft; YZ: Writing-review & editing; XW: Methodology; LZ: Writing-review & editing; PL: Conceptualization, Writing review & editing, Funding acquisition. All authors contributed to the article and approved the submitted version.
Foundation of Modern Agricultural Technology Industry System of Shandong Province (SDAIT-25-07) and Taishan brand cigarette high-quality core raw material development and application in Shandong (202102004).
The authors are grateful to Long Yang, Professor of College of Plant Protection, Shandong Agricultural University, for providing essential suggestions and language help.
The authors declare that the research was conducted in the absence of any commercial or financial relationships that could be construed as a potential conflict of interest.
All claims expressed in this article are solely those of the authors and do not necessarily represent those of their affiliated organizations, or those of the publisher, the editors and the reviewers. Any product that may be evaluated in this article, or claim that may be made by its manufacturer, is not guaranteed or endorsed by the publisher.
Ahmad, A., Yasin, N. A., Khan, W. U., Akram, W., Wang, R., Shah, A. A., et al. (2021). Silicon assisted ameliorative effects of iron nanoparticles against cadmium stress: attaining new equilibrium among physiochemical parameters, antioxidative machinery, and osmoregulators of Phaseolus lunatus. Plant Physiol. Biochem. 166, 874–886. doi: 10.1016/j.plaphy.2021.06.016
Alcántara, E., Romera, F. J., Cañete, M., de la Guardia, M. D. (1994). Effects of heavy metals on both induction and function of root fe(lll) reductase in fe-deficient cucumber (Cucumis sativus l.) plants. J. Exp. Bot. 45, 1893–1898. doi: 10.1093/jxb/45.12.1893
Ali, S., Rizwan, M., Hussain, A., ur Rehman, M. Z., Ali, B., Yousaf, B., et al. (2019). Silicon nanoparticles enhanced the growth and reduced the cadmium accumulation in grains of wheat (Triticum aestivum l.). Plant Physiol. Biochem. 140, 1–8. doi: 10.1016/j.plaphy.2019.04.041
Alzahrani, Y., Kusvuran, A., Alharby, H. F., Kusvuran, S., Rady, M. M. (2018). The defensive role of silicon in wheat against stress conditions induced by drought, salinity or cadmium. Ecotoxicol. Environ. Saf. 154, 187–196. doi: 10.1016/j.ecoenv.2018.02.057
Ashraf, M., Aziz, A., Kausar, R., Sahazad, S. M. (2019). Efficiency of different amendments for the mitigation of cadmium toxicity in sunflower (Helianthus annuus l.). J. Soil Sci. Plant Biol. 2019, 73–86. doi: 10.33513/JSPB/1901-08
Azam, S. K., Karimi, N., Souri, Z., Vaculík, M. (2021). Multiple effects of silicon on alleviation of arsenic and cadmium toxicity in hyperaccumulator Isatis cappadocica desv. Plant Physiol. Biochem. 168, 177–187. doi: 10.1016/j.plaphy.2021.09.027
Bari, M. A., Prity, S. A., Das, U., Akther, M. S., Sajib, S. A., Reza, M. A., et al. (2020). Silicon induces phytochelatin and ROS scavengers facilitating cadmium detoxification in rice. Plant Biol. 22, 472–479. doi: 10.1111/plb.13090
Batool, T., Javied, S., Ashraf, K., Sultan, K., Zaman, Q. U., Haider, F. U. (2022). Alleviation of cadmium stress by silicon supplementation in peas by the modulation of morpho-Physio-Biochemical variables and health risk assessment. LIFE-BASEL 12, 1479. doi: 10.3390/life12101479
Bernacchi, C. J., Vanloocke, A. (2015). Terrestrial ecosystems in a changing environment: A dominant role for water. Annu. Rev. Plant Biol. 66, 599–622. doi: 10.1146/annurev-arplant-043014-114834
Bhardwaj, S., Sharma, D., Singh, S., Ramamurthy, P. C., Verma, T., Pujari, M., et al. (2022). Physiological and molecular insights into the role of silicon in improving plant performance under abiotic stresses. Plant Soil 2022, 1–19. doi: 10.1007/s11104-022-05395-4
Bhat, J. A., Shivaraj, S. M., Singh, P., Navadagi, D. B., Tripathi, D. K., Dash, P. K., et al. (2019). Role of silicon in mitigation of heavy metal stresses in crop plants. Plants 8, 71. doi: 10.3390/plants8030071
Bovetl, L., Eggmann, T., Meylan Bettex, M., Polier, J., Kammer, P., Marin, E., et al. (2003). Transcript levels of AtMRPs after cadmium treatment: induction of AtMRP3. Plant Cell Environ. 26, 371–381. doi: 10.1046/j.1365-3040.2003.00968.x
Bray, E. A. (1997). Plant responses to water deficit. Trends Plant Sci. 2, 48–54. doi: 10.1016/S1360-1385(97)82562-9
Cai, Y., Pan, B., Liu, B., Cai, K., Tian, J., Wang, W. (2022). The cd sequestration effects of rice roots affected by different Si management in cd-contaminated paddy soil. Sci. Total Environ. 849, 157718. doi: 10.1016/j.scitotenv.2022.157718
Carneiro, J. M. T., Chacón-Madrid, K., Galazzi, R. M., Campos, B. K., Arruda, S. C. C., Azevedo, R. A., et al. (2017). Evaluation of silicon influence on the mitigation of cadmium-stress in the development of arabidopsis thaliana through total metal content, proteomic and enzymatic approaches. J. Trace Elem. Med. Biol. 44, 50–58. doi: 10.1016/j.jtemb.2017.05.010
Celikkol, A. U., Erkan, I. E. (2016). Silicon induced antioxidative responses and expression of BOR2 and two PIP family aquaporin genes in barley grown under boron toxicity. Plant Mol. Biol. Rep. 34, 318–326. doi: 10.1007/s11105-015-0923-5
Chen, D., Cao, B., Wang, S., Liu, P., Deng, X., Yin, L., et al. (2016). Silicon moderated the K deficiency by improving the plant-water status in sorghum. Sci. Rep. 6, 1–14. doi: 10.1038/srep22882
Chen, D., Chen, D., Xue, R., Long, J., Lin, X., Lin, Y., et al. (2019). Effects of boron, silicon and their interactions on cadmium accumulation and toxicity in rice plants. J. Hazard. Mater. 367, 447–455. doi: 10.1016/j.jhazmat.2018.12.111
Chen, Z., Zhang, J., Xu, K., Cao, B. (2020). Alleviating effects of silicon on cadmium toxicity in ginger (Zingiber officinale Roscoe). Eur. J. Hortic. Sci. 86, 469–479. doi: 10.17660/eJHS.2021/86.5.3
Chen, R., Zhang, C., Zhao, Y., Huang, Y., Liu, Z. (2018). Foliar application with nano-silicon reduced cadmium accumulation in grains by inhibiting cadmium translocation in rice plants. Environ. Sci. pollut. Res. 25, 2361–2368. doi: 10.1007/s11356-017-0681-z
Cipriano, R., Martins, J. P. R., Conde, L. T., Moreira, S. W., Clairvil, E., Braga, P. D. C. D., et al. (2021). Anatomical, physiological, and biochemical modulations of silicon in Aechmea blanchetiana (Bromeliaceae) cultivated in vitro in response to cadmium. Plant Cell Tissue Organ Cult. 147, 271–285. doi: 10.1007/s11240-021-02122-2
Cobbett, C., Goldsbrough, P. (2002). Phytochelatins and metallothioneins: roles in heavy metal detoxification and homeostasis. Annu. Rev. Plant Biol. 53, 159–182. doi: 10.1146/annurev.arplant.53.100301.135154
Cui, J., Liu, T., Li, F., Yi, J., Liu, C., Yu, H. (2017). Silica nanoparticles alleviate cadmium toxicity in rice cells: Mechanisms and size effects. Environ. pollut. 228, 363–369. doi: 10.1016/j.envpol.2017.05.014
Da Cunha, K. P. V., Do Nascimento, C. W. A. (2009). Silicon effects on metal tolerance and structural changes in maize (Zea mays l.) grown on a cadmium and zinc enriched soil. Water Air Soil pollut. 197, 323–330. doi: 10.1007/s11270-008-9814-9
Da Cunha, K. P. V., Do Nascimento, C. W. A., Da Silva, A. J. (2008). Silicon alleviates the toxicity of cadmium and zinc for maize (Zea mays l.) grown on a contaminated soil. J. Plant Nutr. Soil Sci. 171, 849–853. doi: 10.1002/jpln.200800147
Das, S., Majumder, B., Biswas, A. K. (2022). Comparative study on the influence of silicon and selenium to mitigate arsenic induced stress by modulating TCA cycle, GABA, and polyamine synthesis in rice seedlings. Ecotoxicology 31, 468–489. doi: 10.1007/s10646-022-02524-8
Degryse, F., Smolders, E., Merckx, R. (2006). Labile cd complexes increase cd availability to plants. Environ. Sci. Technol. 40, 830–836. doi: 10.1021/es050894t
Ekmekçi, Y., Tanyolaç, D., Ayhan, B. (2008). Effects of cadmium on antioxidant enzyme and photosynthetic activities in leaves of two maize cultivars. J. Plant Physiol. 165, 600–611. doi: 10.1016/j.jplph.2007.01.017
Elnaz, F. M., Afsaneh, Y., Marzieh, B. (2021). Foliar-applied silicon nanoparticles mitigate cadmium stress through physio-chemical changes to improve growth, antioxidant capacity, and essential oil profile of summer savory (Satureja hortensis l.). Plant Physiol. Biochem. 165, 71–79. doi: 10.1016/j.plaphy.2021.04.040
El-Okkiah, S. A. F., El-Tahan, A. M., Ibrahim, O. M., Taha, M. A., Korany, S. M., Alsherif, E. A., et al. (2022). Under cadmium stress, silicon has a defensive effect on the morphology, physiology, and anatomy of pea (Pisum sativum l.) plants. Front. Plant Sci. 13. doi: 10.3389/fpls.2022.997475
El-Saadony, M. T., Desoky, E. S. M., Saad, A. M., Eid, R. S., Selem, E., Elrys, A. S. (2021). Biological silicon nanoparticles improve Phaseolus vulgaris l. yield and minimize its contaminant contents on a heavy metals-contaminated saline soil. J. Environ. Sci. 106, 1–14. doi: 10.1016/j.jes.2021.01.012
Epstein, E. (1994). The anomaly of silicon in Plant biology. Proc. Natl. Acad. Sci. U. S. A. 91, 11–17. doi: 10.1073/pnas.91.1.11
Fan, J., Hu, Z., Ziadi, N., Xia, X., Wu, C. (2010). Excessive sulfur supply reduces cadmium accumulation in brown rice (Oryza sativa l.). Environ. pollut. 158, 409–415. doi: 10.1016/j.envpol.2009.08.042
Farnezi, M. M. D., Silva, E. D., Dos Santos, L. L., Silva, A. C., Grazziotti, P. H., Prochnow, J. T., et al. (2020). Potential of grasses in phytolith production in soils contaminated with cadmium. Plants 9, 109. doi: 10.3390/plants9010109
Farooq, M. A., Ali, S., Hameed, A., Ishaque, W., Mahmood, K., Iqbal, Z. (2013). Alleviation of cadmium toxicity by silicon is related to elevated photosynthesis, antioxidant enzymes; suppressed cadmium uptake and oxidative stress in cotton. Ecotoxicol. Environ. Saf. 96, 242–249. doi: 10.1016/j.ecoenv.2013.07.006
Farooq, M. A., Detterbeck, A., Clemens, S., Dietz, K. (2016). Silicon-induced reversibility of cadmium toxicity in rice. J. Exp. Bot. 67, 3573–3585. doi: 10.1093/jxb/erw175
Farooqi, Z. U.R., Murtaza, G., Bibi, S., Sabir, M., Saifullah, O. G., Ahmad, I., et al. (2022). Immobilization of cadmium in soil-plant system through soil and foliar applied silicon. Int. J. Phytorem. 24, 1193–1204. doi: 10.1080/15226514.2021.2024133
Feng, J., Shi, Q., Wang, X., Wei, M., Yang, F., Xu, H. (2010). Silicon supplementation ameliorated the inhibition of photosynthesis and nitrate metabolism by cadmium (Cd) toxicity in Cucumis sativus l. Sci. Hortic. 123, 521–530. doi: 10.1016/j.scienta.2009.10.013
Fleck, A. T., Nye, T., Repenning, C., Stahl, F., Zahn, M., Schenk, M. K. (2011). Silicon enhances suberization and lignification in roots of rice (Oryza sativa). J. Exp. Bot. 62, 2001–2011. doi: 10.1093/jxb/erq392
Fulekar, M. H., Singh, A., Bhaduri, A. M. (2009). Genetic engineering strategies for enhancing phytoremediation of heavy metals. Afr. J. Biotechnol. 8, 529–535. doi: 10.1016/j.mrgentox.2008.11.010
Gallego, S. M., Pena, L. B., Barcia, R. A., Azpilicueta, C. E., Iannone, M. F., Rosales, E. P., et al. (2012). Unravelling cadmium toxicity and tolerance in plants: Insight into regulatory mechanisms. Environ. Exp. Bot. 83, 33–46. doi: 10.1016/j.envexpbot.2012.04.006
Gao, M., Zhou, J., Liu, H., Zhang, W., Hu, Y., Liang, J., et al. (2018). Foliar spraying with silicon and selenium reduces cadmium uptake and mitigates cadmium toxicity in rice. Sci. Total Environ. 631, 1100–1108. doi: 10.1016/j.scitotenv.2018.03.047
Gao, X., Zou, C., Wang, L., Zhang, F. (2006). Silicon decreases transpiration rate and conductance from stomata of maize plants. J. Plant Nutr. 29, 1637–1647. doi: 10.1080/01904160600851494
Garg, N., Singh, S. (2018). Arbuscular mycorrhiza rhizophagus irregularis and silicon modulate growth, proline biosynthesis and yield in Cajanus cajan l. millsp. (pigeonpea) genotypes under cadmium and zinc stress. J. Plant Growth Regul. 37, 46–63. doi: 10.1007/s00344-017-9708-4
Gheshlaghpour, J., Asghari, B., Khademian, R., Sedaghati, B. (2021). Silicon alleviates cadmium stress in basil (Ocimum basilicum l.) through alteration of phytochemical and physiological characteristics. Ind. Crops Prod. 163, 113338. doi: 10.1016/j.indcrop.2021.113338
Greger, M., Kabir, A. H., Landberg, T., Maity, P. J., Lindberg, S. (2016). Silicate reduces cadmium uptake into cells of wheat. Environ. pollut. 211, 90–97. doi: 10.1016/j.envpol.2015.12.027
Gu, H., Qiu, H., Tian, T., Zhan, S., Deng, T., Chaney, R. L., et al. (2011). Mitigation effects of silicon rich amendments on heavy metal accumulation in rice (Oryza sativa l.) planted on multi-metal contaminated acidic soil. Chemosphere 83, 1234–1240. doi: 10.1016/j.chemosphere.2011.03.014
Guerriero, G., Hausman, J., Legay, S. (2016). Silicon and the plant extracellular matrix. Front. Plant Sci. 7. doi: 10.3389/fpls.2016.00463
Guo, L., Chen, A., He, N., Yang, D., Liu, M. (2018). Exogenous silicon alleviates cadmium toxicity in rice seedlings in relation to cd distribution and ultrastructure changes. J. Soils Sediments. 18, 1691–1700. doi: 10.1007/s11368-017-1902-2
Hachez, C., Veselov, D., Ye, Q., Reinhardt, H., Knipfer, T., Fricke, W., et al. (2012). Short-term control of maize cell and root water permeability through plasma membrane aquaporin isoforms. Plant Cell Environ. 35, 185–198. doi: 10.1111/j.1365-3040.2011.02429.x
Hajiboland, R., Moradtalab, N., Eshaghi, Z., Feizy, J. (2018). Effect of silicon supplementation on growth and metabolism of strawberry plants at three developmental stages. N. Z. J. Crop Hortic. Sci. 46, 144–161. doi: 10.1080/01140671.2017.1373680
Hamid, Y., Tang, L., Sohail, M. I., Cao, X., Hussain, B., Aziz, M. Z., et al. (2019). An explanation of soil amendments to reduce cadmium phytoavailability and transfer to food chain. Sci. Total Environ. 660, 80–96. doi: 10.1016/j.scitotenv.2018.12.419
Hasanuzzaman, M., Nahar, K., Anee, T. I., Fujita, M. (2017). Exogenous silicon attenuates cadmium-induced oxidative stress in brassica napus l. by modulating AsA-GSH pathway and glyoxalase system. Front. Plant Sci. 8, 1061–1070. doi: 10.3389/fpls.2017.01061
Heile, A. O., Zaman, Q. U., Aslam, Z., Hussain, A., Aslam, M., Saleem, M. H., et al. (2021). Alleviation of cadmium phytotoxicity using silicon fertilization in wheat by altering antioxidant metabolism and osmotic adjustment. SUSTAINABILITY-BASEL 13, 11317. doi: 10.3390/su132011317
Hernandez-Apaolaza, L. (2014). Can silicon partially alleviate micronutrient deficiency in plants? a review. Planta 240, 447–458. doi: 10.1007/s00425-014-2119-x
Hodson, M. J., Sangster, A. G. (1989). X-Ray microanalysis of the seminal root of sorghum bicolor with particular reference to silicon. Ann. Bot. 64, 659–667. doi: 10.1093/oxfordjournals.aob.a087891
Howladar, S. M., Al-Robai, S. A., Al-Zahrani, F. S., Howladar, M. M., Aldhebiani, A. Y. (2018). Silicon and its application method effects on modulation of cadmium stress responses in Triticum aestivum (L.) through improving the antioxidative defense system and polyamine gene expression. Ecotoxicol. Environ. Saf. 159, 143–152. doi: 10.1016/j.ecoenv.2018.05.004
Huang, H., Rizwan, M., Li, M., Song, F., Zhou, S., He, X., et al. (2019). Comparative efficacy of organic and inorganic silicon fertilizers on antioxidant response, Cd/Pb accumulation and health risk assessment in wheat (Triticum aestivum L.). Environ. Pollut. 255, 113146–113155. doi: 10.1016/j.envpol.2019.113146
Huang, H., Li, M., Rizwan, M., Dai, Z., Yuan, Y., Hossain, M. M. (2021). Synergistic effect of silicon and selenium on the alleviation of cadmium toxicity in rice plants. J. Hazard. Mater. 401, 123393–123404. doi: 10.1016/j.jhazmat.2020.123393
Huang, F., Wen, X., Cai, Y., Cai, K. (2018). Silicon-mediated enhancement of heavy metal tolerance in rice at different growth stages. Int. J. Environ. Res. Public Health 15, 2193–2209. doi: 10.3390/ijerph15102193
Hussain, A., Rizwan, M., Ali, Q., Ali, S. (2019). Seed priming with silicon nanoparticles improved the biomass and yield while reduced the oxidative stress and cadmium concentration in wheat grains. Environ. Sci. pollut. Res. Int. 26, 7579–7588. doi: 10.1007/s11356-019-04210-5
Jan, S., Alyemeni, M. N., Wijaya, L., Alam, P., Siddique, K. H., Ahmad, P. (2018). Interactive effect of 24-epibrassinolide and silicon alleviates cadmium stress via the modulation of antioxidant defense and glyoxalase systems and macronutrient content in Pisum sativum l. seedlings. BMC Plant Biol. 18, 1–18. doi: 10.1186/s12870-018-1359-5
Javed, M. T., Saleem, M. H., Aslam, S., Rehman, M., Iqbal, N., Begum, R., et al. (2020). Elucidating silicon-mediated distinct morpho-physio-biochemical attributes and organic acid exudation patterns of cadmium stressed ajwain (Trachyspermum ammi l.). Plant Physiol. Biochem. 157, 23–37. doi: 10.1016/j.plaphy.2020.10.010
Kabir, A. H., Das, U., Rahman, M. A., Lee, K. W. (2021). Silicon induces metallochaperone-driven cadmium binding to the cell wall and restores redox status through elevated glutathione in cd-stressed sugar beet. Physiol. Plant 171, 352–368. doi: 10.1111/ppl.13424
Kabir, A. H., Hossain, M. M., Khatun, M. A., Mandal, A., Haider, S. A. (2016). Role of silicon counteracting cadmium toxicity in alfalfa (Medicago sativa l.). Front. Plant Sci. 7, 1117–1129. doi: 10.3389/fpls.2016.01117
Kaya, C., Akram, N. A., Ashraf, M., Alyemeni, M. N., Ahmad, P. (2020). Exogenously supplied silicon (Si) improves cadmium tolerance in pepper (Capsicum annuum l.) by up-regulating the synthesis of nitric oxide and hydrogen sulfide. J. Biotechnol. 316, 35–45. doi: 10.1016/j.jbiotec.2020.04.008
Keller, C., Rizwan, M., Davidian, J. C., Pokrovsky, O. S., Bovet, N., Chaurand, P., et al. (2015). Effect of silicon on wheat seedlings (Triticum turgidum l.) grown in hydroponics and exposed to 0 to 30 µM Cu. Planta 241, 847–860. doi: 10.1007/s00425-014-2220-1
Khan, I., Awan, S. A., Rizwan, M., Ali, S., Hassan, M. J., Brestic, M., et al. (2021). Effects of silicon on heavy metal uptake at the soil-plant interphase: A review. Ecotoxicol. Environ. Saf. 222, 112510. doi: 10.1016/j.ecoenv.2021.112510
Koleva, L., Umar, A., Yasin, N. A., Shah, A. A., Siddiqui, M. H., Alamri, S., et al. (2022). Iron oxide and silicon nanoparticles modulate mineral nutrient homeostasis and metabolism in cadmium-stressed Phaseolus vulgaris. Front. Plant Sci. 13. doi: 10.3389/fpls.2022.806781
Kollárová, K., Kusa, Z., Vatehova-Vivodova, Z., Liskova, D. (2019). The response of maize protoplasts to cadmium stress mitigated by silicon. Ecotoxicol. Environ. Saf. 170, 488–494. doi: 10.1016/j.ecoenv.2018.12.016
Krämer, U., Chardonnens, A. N. (2001). The use of transgenic plants in the bioremediation of soils contaminated with trace elements. Appl. Microbiol. Biotechnol. 55, 661–672. doi: 10.1007/s002530100631
Kreszies, T., Schreiber, L., Ranathunge, K. (2018). Suberized transport barriers in arabidopsis, barley and rice roots: From the model plant to crop species. J. Plant Physiol. 227, 75–83. doi: 10.1016/j.jplph.2018.02.002
Kučerová, D., Labancová, E., Vivodová, Z., Kollárová, K. (2020). The modulation of ion homeostasis by silicon in cadmium treated poplar callus cells. Environ. Sci. pollut. Res. Int. 27, 2857–2867. doi: 10.1007/s11356-019-07054-1
Li, L., Ai, S., Li, Y., Wang, Y., Tang, M. (2018). Exogenous silicon mediates alleviation of cadmium stress by promoting photosynthetic activity and activities of antioxidative enzymes in rice. J. Plant Growth Regul. 37, 602–611. doi: 10.1007/s00344-017-9758-7
Li, C., Zhang, T., Feng, P., Li, D., Brestic, M., Liu, Y., et al. (2021). Genetic engineering of glycinebetaine synthesis enhances cadmium tolerance in BADH-transgenic tobacco plants via reducing cadmium uptake and alleviating cadmium stress damage. Environ. Exp. Bot. 191, 104602. doi: 10.1016/j.envexpbot.2021.104602
Liu, J., Li, N., Zhang, W., Wei, X., Tsang, D. C. W., Sun, Y., et al. (2019). Thallium contamination in farmlands and common vegetables in a pyrite mining city and potential health risks. Environ. pollut. 248, 906–915. doi: 10.1016/j.envpol.2019.02.092
Liu, J., Ma, J., He, C., Li, X., Zhang, W., Xu, F., et al. (2013a). Inhibition of cadmium ion uptake in rice (Oryza sativa) cells by a wall-bound form of silicon. New Phytol. 200, 691–699. doi: 10.1111/nph.12494
Liu, P., Yin, L., Deng, X., Wang, S., Tanaka, K., Zhang, S. (2014). Aquaporin-mediated increase in root hydraulic conductance is involved in silicon-induced improved root water uptake under osmotic stress in Sorghum bicolor l. J. Exp. Bot. 65, 4747–4756. doi: 10.1093/jxb/eru220
Liu, P., Yin, L., Wang, S., Zhang, M., Deng, X., Zhang, S., et al. (2015). Enhanced root hydraulic conductance by aquaporin regulation accounts for silicon alleviated salt-induced osmotic stress in Sorghum bicolor l. Environ. Exp. Bot. 111, 42–51. doi: 10.1016/j.envexpbot.2014.10.006
Liu, J., Zhang, H., Zhang, Y., Chai, T. (2013b). Silicon attenuates cadmium toxicity in Solanum nigrum l. by reducing cadmium uptake and oxidative stress. Plant Physiol. Biochem. 68, 1–7. doi: 10.1016/j.plaphy.2013.03.018
Lu, Y., Ma, J., Teng, Y., He, J., Christie, P., Zhu, L., et al. (2018). Effect of silicon on growth, physiology, and cadmium translocation of tobacco (Nicotiana tabacum l.) in cadmium-contaminated soil. Pedosphere 28, 680–689. doi: 10.1016/S1002-0160(17)60417-X
Lukačová, Z., Avubová, R., Kohanová, J., Lux, A. (2013). Silicon mitigates the cd toxicity in maize in relation to cadmium translocation, cell distribution, antioxidant enzymes stimulation and enhanced endodermal apoplasmic barrier development. Plant Growth Regul. 70, 89–103. doi: 10.1007/s10725-012-9781-4
Lux, A., Lukačová, Z., Vaculík, M., Avubová, R., Kohanová, J., Soukup, M., et al. (2020). Silicification of root tissues. Plants 9, 111. doi: 10.3390/plants9010111
Lux, A., Luxová, M., Abe, J., Morita, S., Inanaga, S., Abe, J. (2003). Silicification of bamboo (Phyllostachys heterocycla mitf.) root and leaf. Plant Soil. 255, 85–91. doi: 10.1023/A:1026157424794
Luyckx, M., Hausman, J., Blanquet, M., Guerriero, G., Lutts, S. (2021). Silicon reduces cadmium absorption and increases root-to-shoot translocation without impacting growth in young plants of hemp (Cannabis sativa l.) on a short-term basis. Environ. Sci. pollut. Res. 28, 37963–37977. doi: 10.1007/s11356-021-12912-y
Luyckx, M., Hausman, J., Lutts, S., Guerriero, G. (2017). Silicon and plants: current knowledge and technological perspectives. Front. Plant Sci. 8. doi: 10.3389/fpls.2017.00411
Ma, J., Cai, H., He, C., Zhang, W., Wang, L. (2015). A hemicellulose-bound form of silicon inhibits cadmium ion uptake in rice (Oryza sativa) cells. New Phytol. 206, 1063–1074. doi: 10.1111/nph.13276
Ma, J. F., Tamai, K., Yamaji, N., Mitani, N., Konishi, S., Katsuhara, M., et al. (2006). A silicon transporter in rice. Nature 440, 688–691. doi: 10.1038/nature04590
Ma, J. F., Yamaji, N. (2008). Functions and transport of silicon in plants. Cell. Mol. Life Sci. 65, 3049–3057. doi: 10.1007/s00018-008-7580-x
Mapodzeke, J. M., Adil, M. F., Wei, D., Joan, H. I., Ouyang, Y., Shamsi, I. H. (2021). Modulation of key physio-biochemical and ultrastructural attributes after synergistic application of zinc and silicon on rice under cadmium stress. Plants 10, 87. doi: 10.3390/plants10010087
Mohabubul Haque, A. F. M., Gohari, G., El-Shehawi, A. M., Dutta, A. K., Elseehy, M. M., Kabir, A. H. (2022). Genome-wide identification, characterization and expression profiles of heavy metal ATPase 3 (HMA3) in plants. J. King Saud Univ. Eng. Sci. 34, 101730. doi: 10.1016/j.jksus.2021.101730
Naeem, A., Zia-Ur-Rehman, M., Akhtar, T., Zia, M. H., Aslam, M. (2018). Silicon nutrition lowers cadmium content of wheat cultivars by regulating transpiration rate and activity of antioxidant enzymes. Environ. pollut. 242, 126–135. doi: 10.1016/j.envpol.2018.06.069
Nazar, R., Iqbal, N., Masood, A., Khan, M. I. R., Syeed, S., Khan, N. A. (2012). Cadmium toxicity in plants and role of mineral nutrients in its alleviation. Am. J. Plant Sci. 3, 1476–1489. doi: 10.4236/ajps.2012.310178
Nwugo, C. C., Huerta, A. J. (2008). Silicon-induced cadmium resistance in rice (Oryza sativa). J. Plant Nutr. Soil Sci. 171, 841–848. doi: 10.1002/jpln.200800082
Nwugo, C. C., Huerta, A. J. (2011). The effect of silicon on the leaf proteome of rice (Oryza sativa l.) plants under cadmium-stress. J. Proteome Res. 10, 518–528. doi: 10.1021/pr100716h
Pereira, T. S., Pereira, T. S., Souza, C. L. F. D., Lima, E. J. A., Batista, B. L., Lobato, A. K. D. S. (2018). Silicon deposition in roots minimizes the cadmium accumulation and oxidative stress in leaves of cowpea plants. Physiol. Mol. Biol. Plants. 24, 99–114. doi: 10.1007/s12298-017-0494-z
Pereira, A. S., Severo Dorneles, A. O., Bernardy, K., Sasso, V. M., Bernardy, D., Possebom, G., et al. (2018). Selenium and silicon reduce cadmium uptake and mitigate cadmium toxicity in Pfaffia glomerata (Spreng.) pedersen plants by activation antioxidant enzyme system. Environ. Sci. pollut. Res. 25, 18548–18558. doi: 10.1007/s11356-018-2005-3
Pereira, A. S., Bortolin, G. S., Dorneles, A. O. S., Meneghello, G. E., Do Amarante, L., Mauch, C. R. (2021). Silicon seed priming attenuates cadmium toxicity in lettuce seedlings. Environ. Sci. Pollut. Res. 28, 21101–21109. doi: 10.1007/s11356-020-12249-y
Pilon, C., Soratto, R. P., Moreno, L. A. (2013). Effects of soil and foliar application of soluble silicon on mineral nutrition, gas exchange, and growth of potato plants. Crop Sci. 53, 1605–1614. doi: 10.2135/cropsci2012.10.0580
Rady, M. M., Elrys, A. S., El-Maati, M. F. A. (2019). Desoky EM. interplaying roles of silicon and proline effectively improve salt and cadmium stress tolerance in Phaseolus vulgaris plant. Plant Physiol. Biochem. 139, 558–568. doi: 10.1016/j.plaphy.2019.04.025
Rahman, M. F., Ghosal, A., Alam, M. F., Kabir, A. H. (2017). Remediation of cadmium toxicity in field peas ( Pisum sativum L.) through exogenous silicon. Ecotoxicol. Environ. Saf. 135, 165–172. doi: 10.1016/j.ecoenv.2016.09.019
Rahman, S., Xuebin, Q., Kamran, M., Yasin, G., Cheng, H., Rehim, A., et al. (2021a). Silicon elevated cadmium tolerance in wheat (Triticum aestivum l.) by endorsing nutrients uptake and antioxidative defense mechanisms in the leaves. Plant Physiol. Biochem. 166, 148–159. doi: 10.1016/j.plaphy.2021.05.038
Rahman, S., Xuebin, Q., Yasin, G., Cheng, H., Mehmood, F., Zain, M., et al. (2021b). Role of silicon on root morphological characters of wheat (Triticum aestivum l.) plants grown under cd-contaminated nutrient solution. Acta Physiol. Plant 43, 1–13. doi: 10.1007/s11738-021-03228-y
Rahman, S., Xuebin, Q., Zhao, Z., Du, Z., Imtiaz, M., Mehmood, F., et al. (2021c). Alleviatory effects of silicon on the morphology, physiology, and antioxidative mechanisms of wheat (Triticum aestivum l.) roots under cadmium stress in acidic nutrient solutions. Sci. Rep. 11, 1–12. doi: 10.1038/s41598-020-80808-x
Redjala, T., Sterckeman, T., Morel, J. L. (2009). Cadmium uptake by roots: Contribution of apoplast and of high- and low-affinity membrane transport systems. Environ. Exp. Bot. 67, 235–242. doi: 10.1016/j.envexpbot.2009.05.012
Rehman, M. Z., Rizwan, M., Rauf, A., Ayub, M. A., Ali, S., Qayyum, M. F., et al. (2019). Split application of silicon in cadmium (Cd) spiked alkaline soil plays a vital role in decreasing cd accumulation in rice (Oryza sativa l.) grains. Chemosphere 226, 454–462. doi: 10.1016/j.chemosphere.2019.03.182
Riaz, M., Kamran, M., Fahad, S., Wang, X. (2022). Nano-silicon mediated alleviation of cd toxicity by cell wall adsorption and antioxidant defense system in rice seedlings. Plant Soil 2022, 1–15. doi: 10.1007/s11104-022-05588-x
Riaz, M., Kamran, M., Rizwan, M., Ali, S., Parveen, A., Malik, Z., et al. (2021). Cadmium uptake and translocation: selenium and silicon roles in cd detoxification for the production of low cd crops: a critical review. Chemosphere 273, 129690. doi: 10.1016/j.chemosphere.2021.129690
Rios, J. J., Martínez-Ballesta, M. C., Ruiz, J. M., Blasco, B., Carvajal, M. (2017). Silicon-mediated improvement in plant salinity tolerance: The role of aquaporins. Front. Plant Sci. 8. doi: 10.3389/fpls.2017.00948
Rizwan, M., Ali, S., Hussain, A., Ali, Q., Shakoor, M. B., Zia-Ur-Rehman, M., et al. (2017). Effect of zinc-lysine on growth, yield and cadmium uptake in wheat (Triticum aestivum l.) and health risk assessment. Chemosphere 187, 35–42. doi: 10.1016/j.chemosphere.2017.08.071
Rizwan, M., Ali, S., Malik, S., Adrees, M., Qayyum, M. F., Alamri, S. A., et al. (2019). Effect of foliar applications of silicon and titanium dioxide nanoparticles on growth, oxidative stress, and cadmium accumulation by rice (Oryza sativa). Acta Physiol. Plant 41, 1–12. doi: 10.1007/s11738-019-2828-7
Rizwan, M., Ali, S., Rehman, M. Z. U., Rinklebe, J., Tsang, D. C. W., Bashir, A., et al. (2018). Cadmium phytoremediation potential of brassica crop species: A review. Sci. Total Environ. 631, 1175–1191. doi: 10.1016/j.scitotenv.2018.03.104
Rizwan, M., Meunier, J. D., Davidian, J. C. (2016). Silicon alleviates cd stress of wheat seedlings (Triticum turgidum l. cv. Claudio) grown in hydroponics. Environ. Sci. pollut. Res. 23, 1414–1427. doi: 10.1007/s11356-015-5351-4
Rizwan, M., Meunier, J., Miche, H., Keller, C. (2012). Effect of silicon on reducing cadmium toxicity in durum wheat (Triticum turgidum l. cv. Claudio w.) grown in a soil with aged contamination. J. Hazard. Mater. 209, 326–334. doi: 10.1016/j.jhazmat.2012.01.033
Saber, N. E., Abdel-Rahman, M. M., Mabrouk, M. E. M. (2022). Silicon alleviates cadmium toxicity in Triticum aestivum l. plants by modulating antioxidants, nutrient uptake, and gene expression. Egypt. J. Bot. 62, 319–336. doi: 10.21608/ejbo.2021.59947.1618
Saja-Garbarz, D., Libik-Konieczny, M., Fellner, M., Jurczyk, B., Janowiak, F. (2022). Silicon-induced alterations in the expression of aquaporins and antioxidant system activity in well-watered and drought-stressed oilseed rape. Plant Physiol. Biochem. 174, 73–86. doi: 10.1016/j.plaphy.2022.01.033
Saleem, M. H., Parveen, A., Khan, S. U., Hussain, I., Wang, X., Alshaya, H., et al. (2022). Silicon fertigation regimes attenuates cadmium toxicity and phytoremediation potential in two maize (Zea mays l.) cultivars by minimizing its uptake and oxidative stress. SUSTAINABILITY-BASEL 14, 1462. doi: 10.3390/su14031462
Sarwar, N., Malhi, S. S., Zia, M. H., Naeem, A., Bibi, S., Farid, G. (2010). Role of mineral nutrition in minimizing cadmium accumulation by plants. J. Sci. Food Agric. 90, 925–937. doi: 10.1002/jsfa.3916
Sasaki, A., Yamaji, N., Ma, J. F. (2014). Overexpression of osHMA3 enhances cd tolerance and expression of zn transporter genes in rice. J. Exp. Bot. 65, 6013–6021. doi: 10.1093/jxb/eru340
Sasaki, A., Yamaji, N., Yokosho, K., Ma, J. F. (2012). Nramp5 is a major transporter responsible for manganese and cadmium uptake in rice. Plant Cell. 24, 2155–2167. doi: 10.1105/tpc.112.096925
Satofuka, H., Fukui, T., Takagi, M., Atomi, H., Imanaka, T. (2001). Metal-binding properties of phytochelatin-related peptides. J. Inorg. Biochem. 8, 595–602. doi: 10.1016/S0162-0134(01)00223-9
Schutzendubel, A., Schwanz, P., Teichmann, T., Gross, K., Langenfeld-Heyser, R., Godbold, D. L., et al. (2001). Cadmium-induced changes in antioxidative systems, hydrogen peroxide content, and differentiation in scots pine roots. Plant Physiol. 127, 887–898. doi: 10.1104/pp.127.3.887
Seyed, M. (2022). “Silicon and nano-silicon mediated heavy metal stress tolerance in plants,” in Silicon and nano-silicon in environmental stress management and crop quality improvement (United States of America: Academic Press), 181–191. doi: 10.1016/B978-0-323-91225-9.00012-1
Shanmugaraj, B. M., Malla, A., Ramalingam, S. (2019). Cadmium stress and toxicity in plants: an overview. Cadmium Toxic. Tolerance Plants 2019, 1–17. doi: 10.1016/B978-0-12-814864-8.00001-2
Shao, J. F., Che, J., Yamaji, N., Shen, R. F., Ma, J. F. (2017). Silicon reduces cadmium accumulation by suppressing expression of transporter genes involved in cadmium uptake and translocation in rice. J. Exp. Bot. 68, 5641–5651. doi: 10.1093/jxb/erx364
Sharma, S. S., Dietz, K., Mimura, T. (2016). Vacuolar compartmentalization as indispensable component of heavy metal detoxification in plants. Plant Cell Environ. 39, 1112–1126. doi: 10.1111/pce.12706
Shi, G., Cai, Q. (2008). Photosynthetic and anatomic responses of peanut leaves to cadmium stress. Photosynthetica 4, 627–630. doi: 10.1007/s11099-008-0107-8
Shi, G., Cai, Q., Liu, C., Wu, L. (2010). Silicon alleviates cadmium toxicity in peanut plants in relation to cadmium distribution and stimulation of antioxidative enzymes. Plant Growth Regul. 61, 45–52. doi: 10.1007/s10725-010-9447-z
Shi, Z., Yang, S., Han, D., Zhou, Z., Li, X., Liu, Y., et al. (2018). Silicon alleviates cadmium toxicity in wheat seedlings (Triticum aestivum l.) by reducing cadmium ion uptake and enhancing antioxidative capacity. Environ. Sci. pollut. Res. 25, 7638–7646. doi: 10.1007/s11356-017-1077-9
Shi, X., Zhang, C., Wang, H., Zhang, F. (2005). Effect of Si on the distribution of cd in rice seedlings. Plant Soil. 272, 53–60. doi: 10.1007/s11104-004-3920-2
Singh, S., Singh, V. P., Prasad, S. M., Sharma, S., Ramawat, N., Dubey, N. K., et al. (2019). Interactive effect of silicon (Si) and salicylic acid (SA) in maize seedlings and their mechanisms of cadmium (Cd) toxicity alleviation. J. Plant Growth Regul. 38, 1587–1597. doi: 10.1007/s00344-019-09958-1
Sohail, M. I., ur Rehman, M. Z., Rizwan, M., Yousaf, B., Ali, S., ul Haq, M. A., et al. (2019). Efficiency of various silicon rich amendments on growth and cadmium accumulation in field grown cereals and health risk assessment. Chemosphere 244, 125481. doi: 10.1016/j.chemosphere.2019.125481
Song, Y., Jin, L., Wang, X. (2017). Cadmium absorption and transportation pathways in plants. Int. J. Phytorem. 19, 133–141. doi: 10.1080/15226514.2016.1207598
Song, A., Li, Z., Wang, E., Xu, D., Wang, S., Bi, J., et al. (2021). Supplying silicon alters microbial community and reduces soil cadmium bioavailability to promote health wheat growth and yield. Sci. Total Environ. 796, 148797. doi: 10.1016/j.scitotenv.2021.148797
Srivastava, R. K., Pandey, P., Rajpoot, R., Rani, A., Gautam, A., Dubey, R. S. (2015). Exogenous application of calcium and silica alleviates cadmium toxicity by suppressing oxidative damage in rice seedlings. Protoplasma. 252, 959–975. doi: 10.1007/s00709-014-0731-z
Sterckeman, T., Thomine, S. (2020). Mechanisms of cadmium accumulation in plants. Crit. Rev. Plant Sci. 39, 322–359. doi: 10.1080/07352689.2020.1792179
Steudle, E. (2000). Water uptake by roots: effects of water deficit. J. Exp. Bot. 51, 1531–1542. doi: 10.1093/jexbot/51.350.1531
Sun, D., Hussain, H. I., Yi, Z., Rookes, J. E., Kong, L., Cahill, D. M. (2016). Mesoporous silica nanoparticles enhance seedling growth and photosynthesis in wheat and lupin. Chemosphere 152, 81–91. doi: 10.1016/j.chemosphere.2016.02.096
Sun, C., Liang, X., Gong, X., Chen, H., Liu, X., Zhang, S., et al. (2022). Comparative transcriptomics provide new insights into the mechanisms by which foliar silicon alleviates the effects of cadmium exposure in rice. J. Environ. Sci. 115, 294–307. doi: 10.1016/j.jes.2021.07.030
Tao, Q., Jupa, R., Liu, Y., Luo, J., Li, J., Kováč, J., et al. (2019). Abscisic acid-mediated modifications of radial apoplastic transport pathway play a key role in cadmium uptake in hyperaccumulatorSedum alfredii. Plant Cell Environ. 2019 42, 1425–1440. doi: 10.1111/pce.13506
Tchounwou, P. B., Yedjou, C. G., Patlolla, A. K. (2012). Heavy metal toxicity and the environment. Molecular Clin. Environ. Toxicol 101, 133–164. doi: 10.1007/978-3-7643-8340-4_6
Thind, S., Hussain, I., Ali, S., Hussain, S., Rasheed, R., Ali, B., et al. (2020). Physiological and biochemical bases of foliar silicon-induced alleviation of cadmium toxicity in wheat. J. Soil Sci. Plant Nutr. 20, 2714–2730. doi: 10.1007/s42729-020-00337-4
Thind, S., Hussain, I., Ali, S., Rasheed, R., Ashraf, M. A. (2021a). Silicon application modulates growth, physio-chemicals, and antioxidants in wheat (Triticum aestivum l.) exposed to different cadmium regimes. Dose-Response 19, 1–15. doi: 10.1177/15593258211014646
Thind, S., Hussain, I., Rasheed, R., Ashraf, M. A., Perveen, A., Ditta, A., et al. (2021b). Alleviation of cadmium stress by silicon nanoparticles during different phenological stages of ujala wheat variety. Arabian J. Geosci. 14, 1–15. doi: 10.1007/s12517-021-07384-w
Tripathi, D. K., Singh, S., Singh, V. P., Prasad, S. M., Dubey, N. K., Chauhan, D. K. (2017). Silicon nanoparticles more effectively alleviated UV-b stress than silicon in wheat (Triticum aestivum) seedlings. Plant Physiol. Biochem. 110, 70–81. doi: 10.1016/j.plaphy.2016.06.026
Uraguchi, S., Kamiya, T., Sakamoto, T., Kasai, K., Sato, Y., Nagamura, Y., et al. (2011). Low-affinity cation transporter (OsLCT1) regulates cadmium transport into rice grains. Proc. Natl. Acad. Sci. 108, 20959–20964. doi: 10.1073/pnas.1116531109
Uraguchi, S., Mori, S., Kuramata, M., Kawasaki, A., Arao, T., Ishikawa, S. (2009). Root-to-shoot cd translocation via the xylem is the major process determining shoot and grain cadmium accumulation in rice. J. Exp. Bot. 60, 2677–2688. doi: 10.1093/jxb/erp119
Vaculík, M., Landberg, T., Greger, M., Luxová, M., Stoláriková, M., Lux, A. (2012). Silicon modifies root anatomy, and uptake and subcellular distribution of cadmium in young maize plants. Ann. Bot. 110, 433–443. doi: 10.1093/aob/mcs039
Vaculík, M., Pavlovic, A., Lux, A. (2015). Silicon alleviates cadmium toxicity by enhanced photosynthetic rate and modified bundle sheath’s cell chloroplasts ultrastructure in maize. Ecotoxicol. Environ. Saf. 120, 66–73. doi: 10.1016/j.ecoenv.2015.05.026
Vestergaard, M. D., Matsumoto, S., Nishikori, S., Shiraki, K., Hirata, K., Takagi, M. (2008). Chelation of cadmium ions by phytochelatin synthase: role of the cystein-rich c-terminal. Anal. Sci. 24, 277–281. doi: 10.2116/analsci.24.277
Volland, S., Schaumlöffel, D., Dobritzsch, D., Krauss, G., Lütz-Meindl, U. (2013). Identification of phytochelatins in the cadmium-stressed conjugating green alga Micrasterias denticulata. Chemosphere 91, 448–454. doi: 10.1016/j.chemosphere.2012.11.064
Wang, L., Wang, Y., Chen, Q., Cao, W., Li, M., Zhang, F. (2000). Silicon induced cadmium tolerance of rice seedlings. J. Plant Nutr. 23, 1397–1406. doi: 10.1080/01904160009382110
Wang, S., Wang, F., Gao, S. (2015). Foliar application with nano-silicon alleviates cd toxicity in rice seedlings. Environ. Sci. pollut. Res. 22, 2837–2845. doi: 10.1007/s11356-014-3525-0
Wang, Z., Zhou, Z., Wang, X., Chen, Z. (2018). Relationships between transpiration, water loss, and air conditions during physiological drying. Drying Technol. 36, 245–254. doi: 10.1080/07373937.2017.1326499
Wei, W., Peng, H., Xie, Y., Wang, X., Huang, R., Chen, H., et al. (2021). The role of silicon in cadmium alleviation by rice root cell wall retention and vacuole compartmentalization under different durations of cd exposure. Ecotoxicol. Environ. Saf. 226, 112810. doi: 10.1016/j.ecoenv.2021.112810
Wu, J., Guo, J., Hu, Y., Gong, H. (2015). Distinct physiological responses of tomato and cucumber plants in silicon-mediated alleviation of cadmium stress. Front. Plant Sci. 6. doi: 10.3389/fpls.2015.00453
Wu, Z., Xu, S., Shi, H., Zhao, P., Liu, X., Li, F., et al. (2018). Comparison of foliar silicon and selenium on cadmium absorption, compartmentation, translocation and the antioxidant system in Chinese flowering cabbage. Ecotoxicol. Environ. Saf. 166, 157–164. doi: 10.1016/j.ecoenv.2018.09.085
Wu, J., Mock, H., Giehl, R. F. H., Pitann, B., Mühling, K. H. (2019). Silicon decreases cadmium concentrations by modulating root endodermal suberin development in wheat plants. J. Hazard. Mater. 364, 581–590. doi: 10.1016/j.jhazmat.2018.10.052
Yang, W. J., Wang, Q. Y., Sun, J. Y. (2018). Alleviation mechanism of exogenous silicon in cabbages under cadmium stress. Acta Botanica Boreali-Occidentalia Sinica. 38, 1088–1098. doi: 10.7606/j.issn.1000-4025.2018.06.1088
Ye, J., Yan, C., Liu, J., Lu, H., Liu, T., Song, Z. (2012). Effects of silicon on the distribution of cadmium compartmentation in root tips of kandelia obovata (S., l.) yong. Environ. pollut. 162, 369–373. doi: 10.1016/j.envpol.2011.12.002
Zaheer, M. M., Yasin, N. A., Ahmad, S. R., Khan, W. U., Ahmad, A., Ali, A., et al. (2018). Amelioration of cadmium stress in gladiolus (Gladiolus grandiflora l.) by application of potassium and silicon. J. Plant Nutr. 4, 461–476. doi: 10.1080/01904167.2017.1385808
Zhang, Q., Yan, C., Liu, J., Lu, H., Duan, H., Du, J., et al. (2014). Silicon alleviation of cadmium toxicity in mangrove (Avicennia marina) in relation to cadmium compartmentation. J. Plant Growth Regul. 33, 233–242. doi: 10.1007/s00344-013-9366-0
Zhao, Y., Liu, M., Guo, L., Yang, D., He, N., Ying, B., et al. (2020). Influence of silicon on cadmium availability and cadmium uptake by rice in acid and alkaline paddy soils. J. Soils Sediments. 20, 2343–2353. doi: 10.1007/s11368-020-02597-0
Zhao, K., Yang, Y., Zhang, L., Zhang, J., Zhou, Y., Huang, H., et al. (2022). Silicon-based additive on heavy metal remediation in soils: Toxicological effects, remediation techniques, and perspectives. Environ. Res. 205, 112244. doi: 10.1016/j.envres.2021.112244
Zhu, Y., Xu, X., Hu, Y., Han, W., Yin, J., Li, H., et al. (2015). Silicon improves salt tolerance by increasing root water uptake in Cucumis sativus l. Plant Cell Rep. 34, 1629–1646. doi: 10.1007/s00299-015-1814-9
Zong, H., Guo, X., Song, N., Liu, J., Wang, F., Gai, L. (2022). Mitigation of cadmium toxicity in edible rape (Brassica rapa l.) by combined application of chitosan and silicon. J. Plant Growth Regul. 2022, 1–10. doi: 10.1007/s00344-022-10752-9
Keywords: antioxidant, cadmium accumulation, cadmium toxicity, silicon, water balance
Citation: Hou L, Ji S, Zhang Y, Wu X, Zhang L and Liu P (2023) The mechanism of silicon on alleviating cadmium toxicity in plants: A review. Front. Plant Sci. 14:1141138. doi: 10.3389/fpls.2023.1141138
Received: 10 January 2023; Accepted: 08 March 2023;
Published: 23 March 2023.
Edited by:
Muhammad Musa Khan, Zhejiang University, ChinaReviewed by:
Atique ur Rehman, Bahauddin Zakariya University, PakistanCopyright © 2023 Hou, Ji, Zhang, Wu, Zhang and Liu. This is an open-access article distributed under the terms of the Creative Commons Attribution License (CC BY). The use, distribution or reproduction in other forums is permitted, provided the original author(s) and the copyright owner(s) are credited and that the original publication in this journal is cited, in accordance with accepted academic practice. No use, distribution or reproduction is permitted which does not comply with these terms.
*Correspondence: Peng Liu, bGl1cGVuZzIwMDNAc2RhdS5lZHUuY24=
Disclaimer: All claims expressed in this article are solely those of the authors and do not necessarily represent those of their affiliated organizations, or those of the publisher, the editors and the reviewers. Any product that may be evaluated in this article or claim that may be made by its manufacturer is not guaranteed or endorsed by the publisher.
Research integrity at Frontiers
Learn more about the work of our research integrity team to safeguard the quality of each article we publish.