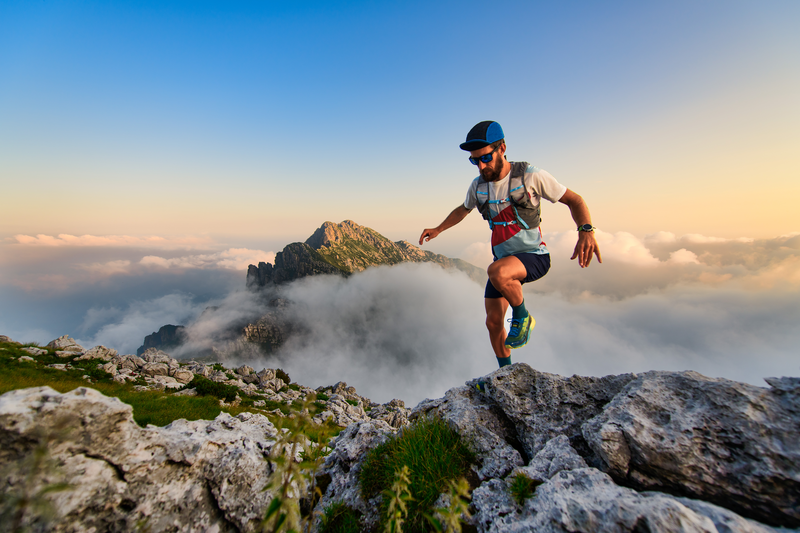
95% of researchers rate our articles as excellent or good
Learn more about the work of our research integrity team to safeguard the quality of each article we publish.
Find out more
ORIGINAL RESEARCH article
Front. Plant Sci. , 02 June 2023
Sec. Plant Symbiotic Interactions
Volume 14 - 2023 | https://doi.org/10.3389/fpls.2023.1134995
This article is part of the Research Topic Effects of Plant-Microbiome Interactions on Phyto- and Bio-Remediation Capacity, Volume II View all 7 articles
Arbuscular mycorrhizal fungi (AMF) play a key role in terrestrial ecosystems, while the ecological restoration application of AMF in mining areas has been progressively gaining attention. This study simulated a low nitrogen (N) environment in copper tailings mining soil to explore inoculative effects of four AMF species on the eco-physiological characteristics of Imperata cylindrica, and provided plant-microbial symbiote with excellent resistance to copper tailings. Results show that N, soil type, AMF species, and associated interactions significantly affected ammonium (), nitrate nitrogen (), and total nitrogen (TN) content and photosynthetic characteristics of I. cylindrica. Additionally, interactions between soil type and AMF species significantly affected the biomass, plant height, and tiller number of I. cylindrica. Rhizophagus irregularis and Glomus claroideun significantly increased TN and content in the belowground components I. cylindrica in non-mineralized sand. Moreover, the inoculation of these two fungi species significantly increased belowground content in mineralized sand. The net photosynthetic rate positively correlated to aboveground total carbon (TC) and TN content under the high N and non-mineralized sand treatment. Moreover, Glomus claroideun and Glomus etunicatum inoculation significantly increased both net photosynthetic and water utilization rates, while F. mosseae inoculation significantly increased the transpiration rate under the low N treatment. Additionally, aboveground total sulfur (TS) content positively correlated to the intercellular carbon dioxide (CO2) concentration, stomatal conductance, and the transpiration rate under the low N sand treatment. Furthermore, G. claroideun, G. etunicatum, and F. mosseae inoculation significantly increased aboveground and belowground TC content of I. cylindrica, while G. etunicatum significantly increased belowground content. Average membership function values of all physiological and ecological I. cylindrica indexes infected with AMF species were higher compared to the control group, while corresponding values of I. cylindrica inoculated with G. claroideun were highest overall. Finally, comprehensive evaluation coefficients were highest under both the low N and high N mineralized sand treatments. This study provides information on microbial resources and plant-microbe symbionts in a copper tailings area, while aiming to improve current nutrient-poor soil conditions and ecological restoration efficiency in copper tailings areas.
Arbuscular mycorrhizal fungi (AMF) are the most widely distributed endophytic and mycorrhiza fungal group and the key microbes which affect terrestrial ecosystems (Gao et al., 2022). Studies have shown that different AMF communities can utilize different soil spatial resources, leading to host plant resource niche differentiation (Li, 2021). AMF can also improve plant stress resistance, which effectively enhances plant resistance to disease, drought, waterlogging, salt and alkali content, heavy metals, weeds, and high and low temperatures (Rivero et al., 2018; Li et al., 2019; Wang et al., 2020; Han et al., 2022; Zhu et al., 2022). Moreover, AMF promotes the nutrient absorption and water-use efficiency of host plants, improves their photosynthetic and osmoregulatory capacity, and contributes to improvements of their antioxidant capacity and drought resistance (Estrada et al., 2013; Han et al., 2022). Furthermore, AMF can directly or indirectly improve the stress resistance of host plants in many aspects. For example, AMF can improve plant water absorption, which would otherwise be difficult for root systems to absorb through their mycelial networks, while improving the overall water and nutritional status of plants, being instrumental in their nutritional status under stress. Through means of regulating soil microecology in the rhizosphere via improvements in soil organic matter (SOM) and microbial levels (Kong, 2021), exogenous mycelia can promote water absorption and regulate the transmission of plant root chemical signals (Green et al., 1998). This subsequently promotes the rapid transmission of water and nutrients to aboveground plant components, reduces stomatal conductance and transpiration rates, improves the photosynthetic capacity of plants, and regulates the osmotic capacity of plants to better cope with drought (Zhu et al., 2015). Moreover, damage to the cytoplasmic membrane can be alleviated by regulating the ion balance of plant cells under stress (Cao et al., 2015).
Symbiosis between plant roots and AMF can help improve plant nitrogen (N) and phosphorus (P) absorption efficiency (Zhou et al., 2021). AMF can symbiotically secrete various enzymes (Saia et al., 2014) and organic acids (Tawaraya et al., 2006) with plants to promote availability of P and N (as well as other nutrient) in soil. Moreover, AMF can help symbionts to form mycelial networks and bridges between plants (Whitfield, 2007). The vast surface area of mycelia can also effectively improve plant and soil interactions and promote root activity (Balogh-Brunstad et al., 2008). Mycelial bridges can directly transfer N that will subsequently be directly absorbed into the host root system. This can also affect N redistribution (Govindarajulu et al., 2005). Additionally, AMF can alter species composition and productivity under N application practices while increasing the relative abundance and aboveground biomass of plants (Zhang et al., 2016).
The role that AMF play is important for host plant photosynthetic processes (Xu, 2021). Studies have shown that AMF inoculation can effectively improve the photosynthetic capacity and carbon (C) assimilation efficiency of plants under drought stress (Metwally et al., 2019; Ye et al., 2022). Additionally, AMF can significantly increase the net photosynthetic rate of host plants, increase dry matter accumulation in plants, and enhanced plant drought resistance. Currently, it remains unclear how AMF affect photosynthetic plant processes (Xu, 2017). According to Huang et al. (2011), AMF mainly improves the nutrient absorption of host plants, which in turn helps promote the accumulation of sufficient amounts of N and P for effective photosynthesis. Additionally, Ludwig-Miiller (2010) reported that AMF inoculation may affect hormone (i.e., abscisic acid [ABA]) levels of host plants that regulate stomatal conductance, thus impacting photosynthetic efficiency (Ludwig-Miiller, 2010). Additionally, AMF species differ regarding their effect on eco-physiological host characteristics. One study found that Rhizoglomus aggregatum, Glomus etunicatum, Glomus claroideun, and Funneliformis constrictus can improve plant growth and photosynthesis (Wang et al., 2022). Among these, R. aggregatum plays a dominant role in promoting seedling height and G. etunicatum and G. claroideun play a dominant role in promoting root regeneration. Moreover, R. aggregatum, G. etunicatum, and G. claroideun can maximize the net photosynthetic rates of plants. On the other hand, F. mosseae can effectively alleviate a decline in the photosynthetic capacity of host plants under stress conditions (Wang et al., 2022).
Technological-based AMF approaches used in the ecological restoration of mining areas have gradually been gaining attention in recent years due to their low cost and high efficiency (Bi and Xie, 2021). For example, AMF can be used to increase vegetation survival rates while improving land reclamation efficiency (Druille et al., 2013; Hao et al., 2014). AMF not only have a positive effect on plant nutrient absorption and enzyme activities, but also can enhance the stability of soil aggregates, improve soil permeability and water retention, and boost overall soil quality (Yang et al., 2016; Choi et al., 2018). The Zhongtiao Mountains copper mining region, Shanxi Province, is North China’s largest, producing 7 million tons of copper annually. It is the largest non-coal underground mining area in China. This mining region produces vast amounts of copper tailings, resulting in severe pollution and damage to the local ecological environment. Previous studies have reported that nutrient levels are low in copper tailings ore. Imperata cylindrica is the dominant grass species in this region, and may form a symbiotic relationship with AMF during phytoremediation (Jia et al., 2022). Based on this hypothesis, we simulated the low N conditions of this copper tailings region to explore how four different AMF inoculation species types will affect the eco-physiological characteristics of I. cylindrica. For this study, we screened out plant-microbial symbiont strains to improve resistance in copper tailings areas, to enhance the status quo of nutrient scarcity, and to increase the efficiency of ecological restoration in copper tailings areas.
The plant selected for this study (I. cylindrica) is a perennial herbaceous cogon grass species with a glabrous, erect stem that grows up to 80 cm tall. Prior to the study, I. cylindrica seeds were soaked in 10% hydrogen peroxide (H2O2) for 10 min before being rinsed several times with sterile water to kill miscellaneous bacteria on the seed surface (Zhang et al., 2015). The seeds of I. cylindrica were provide by Clover (Beijing) Ecological Technology Co., Ltd. Four AMF species were used in this study: Glomus claroideun (GC), Glomus etunicatum (GE), Rhizophagus irregularis (RI), and Funneliformis mosseae (FM). These AMF species originally provided by the Institute of Plant Nutrition and Resources, Beijing Academy of Agriculture and Forestry Sciences. Sorghum was used for strain propagation under greenhouse conditions. The soil types used for this study were typical river sand (i.e., here referred to as non-mineralized sand) and sand obtained from the copper tailings area (i.e., here referred to as mineralized sand). Impurities such as large stones and leaf detritus were removed using a 2 mm sieve and then autoclaved to eliminate any mycorrhizal fungi (or other microbe) influence in the soil samples.
A 2×5×2 completely randomized three-factor block design was used for the experiment. The first factor was the soil matrix itself: sand from the copper tailings area (i.e., mineralized sand) and sand obtained from a typical river system (i.e., non-mineralized sand). The second factor was the four AMF inocula (i.e., GC, GE, RI, FM), including a control where no inoculant was used. The third factor was the nutrient treatments, namely, the low nitrogen (LN) treatment and the high nitrogen (HN) treatment. Each treatment was replicated fivefold (i.e., 100 pots in total). Urea was used for N inoculation. Compared to the LN group, the amount of inoculum in the HN group was greater by a factor of 10, which was in accordance with the lowest N content measured in copper tailings dam (Xin et al., 2016). The nutrient solution was in the form of a Hoagland solution.
The sterilized matrix was weighed in a plastic pot (21 cm × 12 cm) filled to two-thirds of the way. The AMF inoculant (100 g) was added to the AMF treatment and then spread onto a sterilized matrix. The same amount of sterilized inoculant was added to the treatment where no inoculant was added (i.e., the control) before being covered with the sterilized matrix (2 cm). In total, 30 I. cylindrica seeds were sowed in each pot. After one month growth, the I. cylindrica seedlings were thinned to 15 in each pot. We randomly altered the position of each pot every two weeks under a three-month planting cycle in greenhouse, which was set to a temperature of 20°C at night, 25°C during the day and 50% moisture under natural light.
Plant height and tiller number were measured at harvest time. Plant specimens were first oven-dried at 105°C for 30 min, and then further oven-dried at 65°C to a constant weight for biomass determination. Additionally, I. cylindrica roots were washed with clean water, immersed in a 10% potassium hydroxide (KOH) solution, and treated in a 90°C water bath for 1 h. Root samples were treated in a hydrochloric acid (HCl) solution for 3–5 min after allowing to cool and then washed with distilled water to remove pigments. Roots were sectioned into approximately 1 cm segments, stained with 0.05% Aniline Blue WS, treated in a 90°C water bath for 30 min, washed with clean water, and finally placed under a microscope for observation, where plant mycorrhizal infection rates were calculated (Liu et al., 2015). Equation (1) was used to calculate the mycorrhizal infection rate (MIR):
MIR denotes the root mycorrhizal infection rate (%); NS denotes the number of mycorrhizal segments; TNS denotes the total number of root segments.
Spore density was measured through the wet sieving and sucrose gradient centrifugation procedures and was expressed as the number of AMF spores isolated from 100 g of air-dried soil (Ren, 2017).
Oven-dried plant samples were ground using a ball mill. Following this, total carbon (STC), total nitrogen (STN), and total sulfur (STS) content within aboveground plant components and total carbon (RTC), total nitrogen (RTN), and total sulfur (RTS) content in belowground plant components were measured using an elemental analyzer (vario MACRO cube, Germany). Ammonium () and nitrate nitrogen () were measured using an automated discontinuous chemical analyzer (DeChem-Tech, CleverChem380, Germany).
To measure photosynthetic pigments, we weighed leaf material (0.5 g) before soaking it in a 20 mL mixed solution, with a 1:1 acetone to ethanol ratio. Absorbance was measured at 663 nm, 645 nm, 440 nm, 644 nm, and 662 nm using a microplate reader after seven days under darkened conditions. Following this, the photosynthetic pigment content was calculated using equations (2), (3), (4), and (5):
where V represents the volume of the ethanol and acetone mixed solution, and w represents the weight of the leaf material.
Photosynthetic characteristics were measured using a photosynthetic apparatus (i.e., the CIRAS-3 Portable Photosynthesis System) on a sunny day eight weeks after the plant culture was first established. Light intensity was set at 1200 μmol/(m2·s), and the temperature was set at 25°C. The first fully expanded new leaves were selected for determination. Five replicates of each treatment were made. The photosynthetic indexes used were the net photosynthetic rate (Pn), the intercellular CO2 concentration (Ci), stomatal conductance (Gs), water-use efficiency (WUE), water vapor pressure deficit (VPD), and the transpiration rate (Tr).
SPSS.25 was used for statistical analysis. Duncan’s multiple range test was used as a post hoc test to determine differences between the different bacterial treatments. Origin 2021 was used to visualize statistical results. Equation (6) was used to calculate the membership functions (Jin et al., 2018):
where X is the measured value; Xmax is the maximum value; Xmin is the minimum value. Membership function values were collected and their average value was calculated for this comprehensive evaluation.
Results from Multi-Way ANOVA showed that belowground biomass, aboveground biomass, plant height, and tiller number of I. cylindrica were significantly affected by interactions between soil type and AMF infection type. Moreover, N, soil type, and AMF infection type all significantly affected I. cylindrica root infection rates and tiller numbers (P< 0.05, Table 1). The mycorrhizal infection rate of GE and RI inoculant in non-mineralized sand was significantly higher compared to the LN and HN treatments. The mycorrhizal infection rate of the GE inoculant under the HN treatment was significantly higher compared to the LN treatment (P< 0.05, Figure 1). Spore density of the four different AMF species was significantly higher under the non-mineralized sand and the HN treatment compared to the LN treatment (P< 0.05, Figure 1). After GC inoculation, spore density was significantly higher under the mineralized sand and the HN treatment compared to the LN treatment (P< 0.05, Figure 1). AMF infection promoted the shoot biomass in mineralized sand (P< 0.05, Figure 2).
Table 1 Multi-way ANOVA of different nitrogen and AMF infection on plant growth and the infection characteristics of I. cylindrica.
Figure 1 Infection rate of I. cylindrica and spore density in non-mineralized sand (A, C) and mineralized sand (B, D). MIR is mycorrhizal infection rate. Different lowercase letters representing AMF species and different nitrogen had significant effects on infection characteristics and spore density (P<0.05).
Figure 2 Effects of AMF inoculation on growth characteristics of I. cylindrica in non-mineralized sand (A–D) and mineralized sand (E–H). Different lowercase letters represent significant differences (P< 0.05).
Results showed that N, soil type, AMF infection, and associated interactions significantly affected , , and total nitrogen (TN) content (P< 0.05, Tables 2, 3), which also significantly affected below ground and TN content in I. cylindrica (P< 0.05, Table 3). The FM inoculant under the LN treatment significantly increased aboveground total carbon (TC) content in non-mineralized sand (P< 0.05, Figure 3), while the RI inoculant significantly increased belowground TN content (P< 0.05, Figure 4). Moreover, the GE and FM inocula under the HN treatment significantly increased the TN content of belowground I. cylindrica components in mineralized sand (P< 0.05, Figure 4). The GE inoculant under the HN treatment significantly increased the TN content of aboveground I. cylindrica components in non-mineralized sand (P< 0.05, Figure 3), while the FM inoculant under the LN treatment significantly increased the corresponding TN content in aboveground I. cylindrica components. The GC, GE, and FM inocula under the LN treatment significantly increased the TC content in belowground I. cylindrica components in mineralized sand (P< 0.05, Figure 4). The GE inoculant under the HN treatment significantly increased the content of aboveground I. cylindrica components in mineralized sand (P< 0.05). Furthermore, content in aboveground I. cylindrica components under the LN treatment was significantly higher compared to the control in mineralized sand (P< 0.05) (Figure 3).
Table 2 Multi-way ANOVA of different nitrogen treatments and AMF infection on aboveground nutrient characteristics of I cylindrica.
Table 3 Multi-way ANOVA of different nitrogen and AMF infection on underground nutrient characteristics of I cylindrica.
Figure 3 Effects of AMF inoculation on aboveground nutrient characteristics of I. cylindric in non-mineralized sand (A–E) and mineralized sand (F–J). STN is shoot total nitrogen, STC is shoot total carbon, STS is shoot total sulfur. Different lowercase letters represent significant differences (P< 0.05).
Figure 4 Effects of AMF inoculation on underground nutrient characteristics of I. cylindric in non-mineralized sand (A–E) and mineralized sand (F–J). RTN is root total nitrogen, RTC is root total carbon, RTS is root total sulfur. Different lowercase letters represent significant differences (P< 0.05).
The GC inoculant in non-mineralized sand significantly increased the content in belowground I. cylindrica components (P< 0.05). The GC and RI inocula significantly increased the content in belowground I. cylindrica components in mineralized sand (P< 0.05). The GC inoculant under the HN treatment significantly increased the content in belowground I. cylindrica components (P< 0.05, Figure 4), while the GE inoculant under the LN treatment significantly increased the content in belowground I. cylindrica components in mineralized sand (P< 0.05).
AMF inoculation and associated interactions with N significantly affected photosynthetic pigments of I. cylindrica (P< 0.05, Table 4). AMF inoculation significantly increased the chlorophyll a content of I. cylindrica under the LN treatment in both mineralized and non-mineralized sand (P< 0.05) (Figure 5).
Table 4 Multi-way ANOVA of different nitrogen treatments and AMF infection on the photosynthetic pigment content of I. cylindrica.
Figure 5 Effect of AMF inoculation on photosynthetic pigment content of I. cylindric in non-mineralized sand (A–D) and mineralized sand (E–H). Different lowercase letters represent significant differences (P< 0.05).
Furthermore, N, soil type, AMF infection, and associated interactions significantly affected the photosynthetic rate, the intercellular CO2 concentration, the transpiration rate, and the water vapor pressure deficit of I. cylindrica (P< 0.05, Table 5). AMF inoculation significantly increased the intercellular CO2 concentration of I. cylindrica in non-mineralized sand (P< 0.05, Figure 6). The GE and RI inocula under the LN treatment significantly increased the water vapor pressure deficit of I. cylindrica in mineralized sand, while the FM inoculant under the HN treatment significantly increased the water utilization rate of I. cylindrica (P< 0.05, Figure 6). The GC and GE inocula under the HN treatment significantly increased the net photosynthetic rate and the water utilization rate of I. cylindrica in non-mineralized sand, while the FM inoculant under the LN treatment significantly increased the transpiration rate of I. cylindrica (P< 0.05, Figure 6).
Table 5 Multi-way ANOVA of different nitrogen treatments and AMF infection on the photosynthetic parameters of I. cylindrica.
Figure 6 Effects of AMF inoculation on photosynthetic parameters of I. cylindric in non-mineralized sand (A–F) and mineralized sand (G–L). Ci is intercellular CO2 concentration, Gs is stomatal conductance, VPD is vapor pressure deficit, Pn is net photosynthetic rate, Tr is evaporation rate, WUE is water use efficiency. Different lowercase letters represent significant differences (P< 0.05).
The net photosynthetic rate positively correlated with aboveground TC and TN content (P< 0.05), while water-use efficiency significantly and positively correlated with belowground content (P< 0.05) under the HN treatment in non-mineralized sand (Table 6). The transpiration rate significantly and positively correlated with aboveground TC content, aboveground TN content, and aboveground total sulfur (TS) content under the LN treatment in non-mineralized sand (P< 0.05) (Table 6).
Table 6 Correlation analysis between photosynthetic characteristics and physicochemical properties of I. cylindrica under different treatments.
The net photosynthetic rate significantly and positively correlated with aboveground and TN content under the HN treatment in mineralized sand (P< 0.05). The transpiration rate positively correlated with aboveground content and negatively correlated with aboveground TS content (P< 0.05) (Table 6). The net photosynthetic rate significantly and negatively correlated with and TN content in I. cylindrica under the LN treatment in mineralized sand, while belowground TS content significantly and positively correlated with Ci, Gs, and Tr (P< 0.05, Table 6).
Membership function analysis is a method to comprehensively evaluate material based on multiple indexes, which avoids any bias based on a single index, evaluate each I. cylindrica index more comprehensively, and allow test results to be more scientifically based and reliable. This study comprehensively evaluated eco-physiological characteristics of I. cylindrica based on a photosynthetic index, a growth index, and aboveground and belowground nutrient content. Larger coefficient values signify better plant growth. Results showed that the average membership function value of I. cylindrica under AMF inoculation was higher than the control. The average membership function value of the GC inoculant was highest, while the comprehensive evaluation coefficient was highest under both the HN and LN treatments in mineralized sand (Table 7). The average membership function value of the RI inoculant was highest, while the average values of the membership function growth index and the photosynthetic index of the GE inoculant were highest under the LN treatment in non-mineralized sand (Table 7).
AMF play a crucial role in plant nutrient absorption and stress resistance (Zhang, 2013). Our study found that AMF species significantly affected belowground and aboveground biomass, tiller number, plant height, and mycorrhizal infection rates of I. cylindrica, which was consistent with a previous study (Huang, 2020). This may be because the extraradical mycelium network of AMF can penetrate areas inaccessible to plant roots, subsequently expanding the area of nutrient absorption. Additionally, the extraradical mycelium network can connect to the cortex of plants to form arbuscular structures (Ge et al., 2020), which is advantageous when water and mineral nutrients are transferred via plant shoots for purposes of growth and metabolism, promoting biomass accumulation (Ren et al., 2014; Zhang et al., 2018; Teng et al., 2020). The mycorrhizal infection rate can reflect symbiotic intensity between AMF and host plants (Qin, 2022), while infection rates will directly affect the ability of AMF to obtain C from host plants for its own growth requirements, thus affecting spore germination and hyphal growth (Cai, 2017). This study found that the spore density of the GC inoculant was significantly higher under the HN treatment in mineralized sand compared to the corresponding LN treatment, indicating that AMF inoculation was conducive to the germination and growth of fungal spores. Moreover, the N content of soil also affected AMF growth. This is consistent with results from a previous study (Cai, 2017). AMF infection rates will differ under different environmental factors, such as the available mineral nutrients, organic matter content, and soil pH in different regions. Studies have shown that AMF inoculation can significantly increase mycorrhizal infection rates, that excessively high N applications are not conducive to mycorrhizal infection, and that more significant root mycorrhizal infection rates will occur under LN levels. Additionally, mycorrhizal infections will vary among different plant species and different N application levels (Wang, 2012).
Being one of the three essential elements limiting plant growth and development, N is a key chemical element of plant organic matter (Cai, 2017), while its availability will be affected by soil type, N form type, etc. (Liu et al., 2019). AMF species not only absorb and from the surrounding environment and transfer them to host plants (Hodge et al., 2001), they also accelerate organic matter decomposition and improve plant N absorption by secreting enzymes from extraradical hypha. This study found that N content, soil type, AMF infection type, and associated interactions significantly affected the , , and TN content of I. cylindrica. Hawkins et al. (2000) reported that absorption (per unit weight) by FM mycelia was significantly higher than that of , with a value greater by a factor of 15. The absorption rate (per unit weight) by mycelia was higher compared to that of . Similarly, in non-mineralized sand the GC inoculant significantly increased the content in belowground components of I. cylindrica in this study, while the GC and RI inocula in mineralized sand also significantly increased the content in belowground components of I. cylindrica. Using mineralized sand as a substrate, content in belowground components of I. cylindrica significantly increased in the GC inoculant under the HN treatment, while the content in belowground components of I. cylindrica significantly increased in the GE inoculant under the LN treatment. The reason behind differences in AMF absorption between these two inorganic N forms could be that requires less energy for absorption and assimilation compared to . The absorption process of the latter is as follows: it first reduces to NH3 and then enters into the GS/GOGAT pathway, requiring both energy consumption and reductase participation (Wu and Ca, 2022). However, can directly enter the GS/GOGAT pathway under conditions of low energy consumption. For , via the plant root diffusion process (i.e., where it is absorbed [Sun et al., 2005]), absorption is more difficult due to mycorrhizal associations. This is because of its high mobility. On the other hand, mobility is less robust, forming in the soil within the enrichment region (Smith, 2010), making it easier for roots to absorb outside the hyphae.
Photosynthesis is the fundamental means by which plants synthesize organic matter and obtain energy (Zhu et al., 2010). In this study, we found that N, soil type, AMF infection, and associated interactions significantly affected the net photosynthetic rate, the intercellular CO2 concentration, the transpiration rate, and the water vapor pressure deficit of I. cylindrica. Studies have found that AMF inoculation can also significantly increase chlorophyll content in plant leaves (Sannazzaro et al., 2006; Sheng et al., 2008; Zhu et al., 2010; Liu et al., 2011). Results from this study showed that AMF inoculation under the LN treatment significantly increased the chlorophyll a content of I. cylindrica. This may be because AMF inoculation helps I. cylindrica to obtain the water and nutrients necessary for metabolic photosynthetic processes to take place in belowground components, subsequently promoting chlorophyll synthesis and enhancing the photosynthetic capacity of plant leaves. Additionally, the net photosynthetic rate directly reflects the assimilation capacity of leaves (per unit area), which is an important indicator in measuring the photosynthetic capacity of plants (Hu et al., 2020). Plants provide the AMF photosynthate that most benefits them, and AMF also tends to provide soil nutrients to plants that deliver the most photosynthate for their usage (Kiers et al., 2011). Studies have also shown that AMF symbiosis can promote photosynthetic rates, transpiration rates, and a means for host plants to uptake water (Gavito et al., 2019; Puschel et al., 2020), which can improve the photosynthetic capacity of plants, although still regulated by environmental conditions and available nutrient elements. Similarly, the average membership function values of each I. cylindrica index inoculated with AMF were higher compared to the control. For non-mineralized sand, the net photosynthetic rate of I. cylindrica inoculated with GC and GE under the HN treatment significantly increased, while the net photosynthetic rate positively correlated with aboveground TC and TN content. The transpiration rate of I. cylindrica inoculated with FM under the LN treatment increased significantly. This may be because N enhances the enzyme activities associated with the photosynthetic electron transport chain while promoting photosynthesis, and P is an important enzyme component that is necessary for plant photosynthesis and ATP synthesis. AMF inoculation promotes N and P absorption and utilization in I. cylindrica, subsequently promoting plant photosynthesis (Evans and Von Caemmerer, 1996; Wu and Zhao, 2010; Wang et al., 2016). Moreover, S plays a key role in the synthesis and metabolism of photosynthetic pigments and proteases (Shao, 2004). In this study, AMF inoculation significantly increased the S content in aboveground I. cylindrica components, and this significantly and positively correlated with Ci, Gs, and Tr in LN mineralized sand, which was beneficial to the synthesis of various plant proteins, chlorophyll and carotenoid content, and stress resistance. In conclusion, different AMF inoculation had significant effects on the eco-physiological characteristics of I. cylindrica under differing soil nitrogen conditions. AMF strains can improve plant physiological characteristics to varying degrees.
The original contributions presented in the study are included in the article/supplementary material. Further inquiries can be directed to the corresponding author.
TJ conceived and designed the experiments. MZ, YY, and YZ performed the experiments. BC contributed new reagents. YW, XL, LZ, and TJ wrote the manuscript. All authors read and approved the manuscript. All authors contributed to the article.
This study was supported by the National Natural Science Foundation of China (Grant No. 32171524), Shanxi Province Science and Technology Innovation base construction project (grant no. YDZJSX2022B001), Scientific and Technological Innovation Programs of Higher Education Institutions in Shanxi (Grant No.2019L0005), and Shanxi Province Foundation for Returnees (Grant No. 2021-018), Shanxi Province Graduate Education Innovation Project (Grant No. 2022Y121).
The authors declare that the research was conducted in the absence of any commercial or financial relationships that could be construed as a potential conflict of interest.
All claims expressed in this article are solely those of the authors and do not necessarily represent those of their affiliated organizations, or those of the publisher, the editors and the reviewers. Any product that may be evaluated in this article, or claim that may be made by its manufacturer, is not guaranteed or endorsed by the publisher.
Balogh-Brunstad, Z., Keller, C. K., Dickinson, J. T., Stevens, F., Li, C. Y., Bormann, B. T. (2008). Biotite weathering and nutrient uptake by ectomycorrhizal fungus, suillus tomentosus, in liquid-culture experiments. Geochimica Et Cosmochimica Acta 72, 2601–2618. doi: 10.1016/j.gca.2008.04.003
Bi, Y. L., Xie, l.l. (2021). Functions of arbuscular mycorrhizal fungi and dark septate endophytes in ecological restoration. Acta Microbiologica Sin. 61, 58–67. doi: 10.13343/j.cnki.wsxb.20200171
Cai, X. Z. (2017). Effects of nitrogen addition on arbuscular mycorrhizal fungi and nitrogen unptake of Chinese fir seedlings. Fujian Normal Univ.
Cao, Y. P., Dai, P., Dai, S. Y., He, C. X. (2015). Effects of arbuscular mycorrhizal fungi (AMF) on seedling growth and na+ ,K+ ,Ca2+ ,Mg2+ contents and distribution in asparagus under salt stress. Chin. J. Chin. J. Of Ecol. 34, 1699–1704. doi: 10.13292/j.1000-4890.2015.0157
Choi, J., Summers, W., Paszkowski, U. (2018). Mechanisms underlying establishment of arbuscular mycorrhizal symbioses. Annu. Rev. Of Phytopathol. 56, 135–160. doi: 10.1146/annurev-phyto-080516-035521
Druille, M., Omacini, M., Golluscio, R. A., Cabello, M. N. (2013). Arbuscular mycorrhizal fungi are directly and indirectly affected by glyphosate application. Appl. Soil Ecol. 72, 143–149. doi: 10.1016/j.apsoil.2013.06.011
Estrada, B., Aroca, R., Barea, J. M., Ruiz-Lozano, J. M. (2013). Native arbuscular mycorrhizal fungi isolated from a saline habitat improved maize antioxidant systems and plant tolerance to salinity. Plant Sci. 201, 42–51. doi: 10.1016/j.plantsci.2012.11.009
Evans, J. R., Von Caemmerer, S. (1996). Carbon dioxide diffusion inside leaves. Plant Physiol. 110, 339–346. doi: 10.1104/pp.110.2.339
Gao, Z. Q., Xie, T. L., Ai, J. G., Mei, L. L. (2022). Effects of arbuscular mycorrhizal fungi on stoichiometric characteristics of Leymus chinensis under nitrogen addition. Chin. J. Of Grassland 44, 113–118. doi: 10.16742/j.zgcdxb.20210270
Gavito, M. E., Jakobsen, I., Mikkelsen, T. N., Mora, F. (2019). Direct evidence for modulation of photosynthesis by an arbuscular mycorrhiza-induced carbon sink strength. New Phytol. 223, 896–907. doi: 10.1111/nph.15806
Ge, S. P., Jiang, X. C., Wang, L. Y., Yu, J. Q., Zhou, Y. H. (2020). Recent advances in the role and mechanism of arbuscular mycorrhiza-induced improvement of abiotic stress tolerance in horticultural plants. Acta Hortic. Sin. 47, 1752–1776. doi: 10.16420/j.issn.0513-353x.2020-0479
Govindarajulu, M., Pfeffer, P. E., Jin, H. R., Abubaker, J., Douds, D. D., Allen, J. W., et al. (2005). Nitrogen transfer in the arbuscular mycorrhizal symbiosis. Nature 435, 819–823. doi: 10.1038/nature03610
Green, C. D., Stodola, A., Auge, R. M. (1998). Transpiration of detached leaves from mycorrhizal and nonmycorrhizal cowpea and rose plants given varying abscisic acid, pH, calcium, and phosphorus. Mycorrhiza 8, 93–99. doi: 10.1007/s005720050218
Han, Y. Y., Lou, X., Zhang, W. R., Xu, T. Y., Tang, M. (2022). Arbuscular mycorrhizal fungi enhanced drought resistance of populus cathayana by regulating the 14-3-3 family rrotein genes. Microbiol. Spectr. 10, e0245621. doi: 10.1128/SPECTRUM.02456-21
Hao, X. J., Hong, J. P., Zhang, T. Q., Li, J. R., Gao, W. J., Zheng, Z. M. (2014). Effects of arbuscular mycorrhizal fungal inoculation and phosphorus (P) addition on maize p utilization and growth in reclaimed soil of a mining area. Commun. In Soil Sci. And Plant Anal. 45, 2413–2428. doi: 10.1080/00103624.2014.912295
Hawkins, H. J., Johansen, A., George, E. (2000). Uptake and transport of organic and inorganic nitrogen by arbuscular mycorrhizal fungi. Plant And Soil 226, 275–285. doi: 10.1023/A:1026500810385
Hodge, A., Campbell, C. D., Fitter, A. H. (2001). An arbuscular mycorrhizal fungus accelerates decomposition and acquires nitrogen directly from organic material. Nature 413, 297–299. doi: 10.1038/35095041
Hu, A. S., Xiao, D. D., Zhang, X. D., Wang, W. C., Li, K. C., Sun, Y. (2020). Effects of salt stress on growth and photosynthetic fluorescence of two strains of Malus robusta rehd. Non-Wood For. Res. 38, 208–215. doi: 10.14067/j.cnki.1003-8981.2020.03.024
Huang, D. (2020). Functional analysis of arbuscular mycorrhizal fungi in apple under drought stress. Northwest A&F Univ.
Huang, Z., Zou, Z. R., He, C. X., He, Z. Q., Zhang, Z. B., Li, J. M. (2011). Physiological and photosynthetic responses of melon (Cucumis melo l.) seedlings to three glomus species under water deficit. Plant And Soil 339, 391–399. doi: 10.1007/s11104-010-0591-z
Jia, T., Wang, Y., Liang, X. X., Guo, T. Y. (2022). Effect of AM fungi inoculation on litter bacterial community characteristics under heavy metal stress. Microorganisms 10, 206. doi: 10.3390/microorganisms10020206
Jin, Y., Wang, Y. Z., Chen, J. J., Guo, Q., Xu, B. (2018). Comparison of salt tolerance of 14 alfalfa hybrid combinations. Pratacultural Sci. 35, 2931–2939. doi: 10.11829/j.issn.1001-0629.2017-0497
Kiers, E. T., Duhamel, M., Beesetty, Y., Mensah, J. A., Franken, O., Verbruggen, E., et al. (2011). Reciprocal rewards stabilize cooperation in the mycorrhizal symbiosis. Science 333, 880–882. doi: 10.1126/science.1208473
Kong, Y. H. (2021). Effects of orange peel extract on the growth of citrus seedlings inoculated with arbuscular mycorrhizal fungi. Southwest Univ. doi: 10.27684/d.cnki.gxndx.2021.001816
Li, S. X. (2021). Physiological mechanisms of arbuscular mycorrhizal fungi (AMF) enhancing low temperature resilience in maize. Northeast Forestry Univ.
Li, J. Q., Meng, B., Chai, H., Yang, X. C., Song, W. Z., Li, S. X., et al. (2019). Arbuscular mycorrhizal fungi alleviate drought stress in c-3 (Leymus chinensis) and c-4 (Hemarthria altissima) grasses via altering antioxidant enzyme activities and photosynthesis. Front. In Plant Sci. 10. doi: 10.3389/fpls.2019.00499
Liu, A. R., Chen, S. C., Liu, Y. Y., Li, Y. N., He, C. X. (2011). Effects of AM fungi on leaf photosynthetic physiological parameters and antioxidant enzyme activities under low temperature. Acta Ecologica Sin. 31, 3497–3503. doi: CNKI:SUN:STXB.0.2011-12-030
Liu, H., Chen, W., Zhou, Y., Li, X., Ren, A. Z., Gao, Y. B. (2015). Effects of endophyte and arbuscular mycorrhizal fungi on growth of Leymus chinensis. Chin. J. Of Plant Ecol. 39, 477–485. doi: 10.17521/cjpe.2015.0046
Liu, Y. Y., Zhang, L., Wang, S., Liu, D. H., Zhang, N. M., Zhang, J. H., et al. (2019). Effects of nitrogen and inoculation of indigenous arbuscular mycorrhizal fungi on soil enzyme activity and nitrogen utilization of cucumber under intercropping conditions. Mycosystema 38, 1965–1975. doi: 10.13346/j.mycosystema.190221
Ludwig-Miiller, J. (2010). Hormonal responses in host plants triggered by arbuscular mycorrhizal fungi (Springer), 169–190. doi: 10.1007/978-90-481-9489-6_8
Metwally, A., Azooz, M., Nafady, N., EL-Enany, A. (2019). Arbuscular mycorrhizal symbiosis alleviates drought stress imposed on wheat plants (Triticum aestivum l.). Appl. Ecol. And Environ. Res. 17, 13713–13727. doi: 10.15666/aeer/1706_137131372
Puschel, D., Bitterlich, M., Rydlova, J., Jansa, J. (2020). Facilitation of plant water uptake by an arbuscular mycorrhizal fungus: a Gordian knot of roots and hyphae. Mycorrhiza 30, 299–313. doi: 10.1007/s00572-020-00949-9
Qin, S. F. (2022). Mechanisms of alleviating the growth inhibition of maize by aluminum in acidic soils by arbuscular mycorrhizal fungi. Guangxi Univ.
Ren, J. T. (2017). Biodiversity and distribution of arbuscular mycorrhizal fungi (AMF) and other soli microorganism of aeolian sandy coal mining subsidence area. Inner Mongolia Univ. doi: 10.3969/j.issn.1673-5021.2014.06.013
Ren, A. T., Lu, W. H., Yang, J. J., Liu, H. L., Ma, C. H. (2014). Effects of arbusular mycorrhizal fungi (AMF) on growth of alfalfa and phosphorus utilization under different p levels. Chin. J. Of Grassland 36, 72–78. doi: 10.3969/j.issn.1673-5021.2014.06.013
Rivero, J., Alvarez, D., Flors, V., Azcon-Aguilar, C., Pozo, M. J. (2018). Root metabolic plasticity underlies functional diversity in mycorrhiza-enhanced stress tolerance in tomato. New Phytol. 220, 1322–1336. doi: 10.1111/nph.15295
Saia, S., Benitez, E., Garcia-Garrido, J. M., Settanni, L., Amato, G., Giambalvo, D. (2014). The effect of arbuscular mycorrhizal fungi on total plant nitrogen uptake and nitrogen recovery from soil organic material. J. Of Agric. Sci. 152, 370–378. doi: 10.1017/S002185961300004X
Sannazzaro, A. I., Ruiz, O. A., Alberto, E. O., Menendez, A. B. (2006). Alleviation of salt stress in lotus glaber by glomus intraradices. Plant And Soil 285, 279–287. doi: 10.1007/s11104-006-9015-5
Shao, L. L. (2004). Effects of sulfur nutrition on physiology anti-drought parameters of crops. Northwest A&F Univ.
Sheng, M., Tang, M., Chen, H., Yang, B. W., Zhang, F. F., Huang, Y. H. (2008). Influence of arbuscular mycorrhizae on photosynthesis and water status of maize plants under salt stress. Mycorrhiza 18, 287–296. doi: 10.1007/s00572-008-0180-7
Smith, S. S. E. (2010). Mycorrhizas of autotrophic higher plants. Biol. Rev. 4, 475–510. doi: 10.1111/j.1469-185X.1980.tb00701.x
Sun, T. C., Li, S. Q., Shao, M. A. (2005). Effects of long-term fertilization on distribution of organic matters and nitrogen in cinnamon soil aggregates. Scientia Agricultura Sin. 4, 1841–1848. doi: 10.13287/j.1001-9332.2007.0364
Tawaraya, K., Naito, M., Wagatsuma, T. (2006). Solubilization of insoluble inorganic phosphate by hyphal exudates of arbuscular mycorrhizal fungi. J. OF Plant Nutr. 29, 657–665. doi: 10.1080/01904160600564428
Teng, Q. M., Zhang, Z. F., Li, H. Y., Xu, G. P., Zhou, L. W., Huang, Y. Q. (2020). Effects of arbuscular mycorrhizal fungi on growth, photosynthesis characteristics and mineral nutrition of Arundo donax under cd stress. Soils 52, 1212–1221. doi: 10.13758/j.cnki.tr.2020.06.016
Wang, J. (2012). Effect of AMF and rhizobium inoculation on growth and nutrient uptake of alfalfa at different fertilizer levels. Beijing Forestry Univ.
Wang, F. Y., Adams, C. A., Yang, W. W., Sun, Y. H., Shi, Z. Y. (2020). Benefits of arbuscular mycorrhizal fungi in reducing organic contaminant residues in crops: implications for cleaner agricultural production. Crit. Rev. In Environ. Sci. And Technol. 50, 1580–1612. doi: 10.1080/10643389.2019.1665945
Wang, X. Y., Peng, L. Q., Jin, Z. X. (2016). Effects of AMF inoculation on growth and photosynthetic physiological characteristics of Sinocalycanthus chinensis under conditions of simulated warming. Acta Ecologica Sin. 36, 5204–5214. doi: 10.5846/stxb201501220177
Wang, X. L., Zhao, Y. W., Kong, X. N., Cao, Z. L. (2022). Isolation and identification of mycorrhizal fungi in rhizosphere and their effect on growth and photosynthetic characteristics of Eucalyptus globulus seedlings. Acta Agriculturae Zhejiangensis 34, 1015–1023. doi: 10.3969/j.issn.1004-1524.2022.05.16
Whitfield, J. (2007). Fungal roles in soil ecology: underground networking. Nature 449, 136–138. doi: 10.1038/449136a
Wu, Y. S., Ca, B. Y. (2022). Research progress on mechanism of plant phosphorus absorption mediated by arbuscular mycorrhizal fungi. Shandong Arricultural Sci. 54, 137–143. doi: 10.14083/j.issn.1001-4942.2022.08.020
Wu, W., Zhao, J. (2010). Advances on plants’nitrogen assimilation and utilization. Chin. Agric. Sci. Bullrtin 26, 75–78. doi: CNKI:SUN:ZNTB.0.2010-13-018
Xin, X. J., Liu, L., Shen, J. F., Zhao, N. X., Gao, Y. B. (2016). Interactions between genotypic number and nitrogen addition on soil microbial communities in the population of Leymus chinensis. Acta Ecologica Sin. 36, 3923–3932. doi: 10.5846/stxb201506251289
Xu, P. H. (2017). Effects of arbuscular mycorrhizal fungi on growth and drought resistance of the plant under water stress. Northwest A&F Univ.
Xu, D. L. (2021). Diversity of mycorrhizal and symbiotic microoganisms of six rare plants in western ordos desert. Inner Mongolia Univ.
Yang, H. S., Xu, J. L., Guo, Y., Koide, R. T., Dai, Y. J., Xu, M. M., et al. (2016). Predicting plant response to arbuscular mycorrhizas: the role of host functional traits. Fungal Ecol. 20, 79–83. doi: 10.1016/j.funeco.2015.12.001
Ye, Q. H., Wang, H., Li, H. (2022). Arbuscular mycorrhizal fungi improve growth, photosynthetic activity, and chlorophyll fluorescence of vitis vinifera l. cv. ecolly under drought stress. Agronomy-Basel 12, 1563. doi: 10.3390/AGRONOMY12071563
Zhang, G. Y. (2013). Effects of different agricultural practices on community siructure and colonization rate of arbuscular mycorrhizal fungi. Nanjing Agric. Univ.
Zhang, X. S., Meng, X. Y., Wang, W., Meng, C. L., Liu, Z. Q., Wang, Z. J. (2015). Effects of arbuscular mycorrhizal fungi on growth of ryegrass seedling in cadmium contaminated soils. Soils And Fertilizers Sci. In China 6, 122–127. doi: 10.11838/sfsc.20150620
Zhang, T., Yang, X., Guo, R., Guo, J. X. (2016). Response of AM fungi spore population to elevated temperature and nitrogen addition and their influence on the plant community composition and productivity. Sci. Rep. 6, 24749. doi: 10.1038/srep24749
Zhang, Z. F., Zhang, J. C., Zhou, L. W., Xu, G. P., Li, Y. Q. (2018). Effects of arbuscular mycorrhizal fungi on the growth of afforestation seedlings in a rocky desertification area. Chin. J. Of Ecol. 37, 2927–2934. doi: 10.13292/j.1000-4890.201810.030
Zhou, J. Q., Zhang, Y., Zhang, M. Y., Bo, Y., Ji, W., Sun, J. (2021). Effect of arbuscular mycorrhizal fungi on reducing nitrogen fertilizer dosage in substrate cultivation of lettuce and spinach. China Vegetables 10, 57–65. doi: 10.19928/j.cnki.1000-6346.2021.1039
Zhu, B. L., Gao, T. T., Zhang, D. N., Ding, K., Li, C., Ma, F. W. (2022). Functions of arbuscular mycorrhizal fungi in horticultural crops. Scientia Hortic. 303, 111219. doi: 10.1016/j.scienta.2022.111219
Zhu, X. C., Song, F. B., Xu, H. W. (2010). Effects of arbuscular mycorrhizal fungi on photosynthetic characteristics od maize under low temperature stress. Chin. J. Of Appl. Ecol. 21, 470–475. doi: 10.13287/j.1001-9332.2010.0017
Keywords: arbuscular mycorrhizal fungi, inoculation, eco-physiological characteristics, membership functions, copper tailings areas
Citation: Jia T, Zhang Y, Yao Y, Wang Y, Liang X, Zheng M, Zhao L and Chai B (2023) Effects of AMF inoculation on the eco-physiological characteristics of Imperata cylindrica under differing soil nitrogen conditions. Front. Plant Sci. 14:1134995. doi: 10.3389/fpls.2023.1134995
Received: 31 December 2022; Accepted: 11 May 2023;
Published: 02 June 2023.
Edited by:
Angela Cicatelli, University of Salerno, ItalyReviewed by:
Everlon Cid Rigobelo, São Paulo State University, BrazilCopyright © 2023 Jia, Zhang, Yao, Wang, Liang, Zheng, Zhao and Chai. This is an open-access article distributed under the terms of the Creative Commons Attribution License (CC BY). The use, distribution or reproduction in other forums is permitted, provided the original author(s) and the copyright owner(s) are credited and that the original publication in this journal is cited, in accordance with accepted academic practice. No use, distribution or reproduction is permitted which does not comply with these terms.
*Correspondence: Tong Jia, amlhdG9uZ0BzeHUuZWR1LmNu
Disclaimer: All claims expressed in this article are solely those of the authors and do not necessarily represent those of their affiliated organizations, or those of the publisher, the editors and the reviewers. Any product that may be evaluated in this article or claim that may be made by its manufacturer is not guaranteed or endorsed by the publisher.
Research integrity at Frontiers
Learn more about the work of our research integrity team to safeguard the quality of each article we publish.