- 1Department of Agricultural College, State Key Laboratory for Conservation and Utilization of Subtropical Agro-bioresources, Guangxi Key Laboratory of Sugarcane Biology, Guangxi University, Nanning, Guangxi, China
- 2College of Agronomy, Jiangxi Agricultural University, Nanchang, Jiangxi, China
- 3Herbarium, Royal Botanic Gardens, Kew, London, United Kingdom
- 4South China Botanical Garden, Chinese Academy of Sciences, Guangzhou, Guangdong, China
There are about 140 species of Callicarpa L. 1753 (Lamiaceae), with more species richness in tropical to subtropical Asia and the New World. The genus might provide an insight into the amphi-Pacific disjunction pattern of tropical and subtropical vegetation. This study has greatly improved the phylogenetic underpinning for Callicarpa, derived from more inclusive taxonomic samplings, and employing data on both two-nuclear and eight-chloroplast regions. To address time and patterns of diversification in Callicarpa, we conducted divergence time and biogeographic analyses, and inferred shifts in the distribution areas across the phylogenetic clades. Our phylogenetic results show that Callicarpa is monophyletic with respect to the groups considered, and eight well-supported primary clades were discerned in the combined analyses. Our estimates indicated that the crown group of Callicarpa originates around the Late-Eocene (ca. 36.23 Ma) and diversification within most clades is concentrated in the Miocene and continued to the Pleistocene. In addition, our biogeographic analyses suggested that the probable ancestor of the Callicarpa crown clade originated in East Asia and Southeast Asia. Multiple dispersal and vicariance events contributed to the current distribution of the taxa. Furthermore, this genus expanded eastward out of East and Southeast Asia to the New World by long-distance dispersal, which inspired us to better understand the amphi-Pacific disjunct distribution.
1 Introduction
Understanding how distributions of organisms have been shaped is a fundamental question in biogeography and the use of molecular clocks and fossil records introduced a timeframe for the evolution of the taxa (Smith and Peterson, 2002). Complex interactions between abiotic and biotic factors and geological-tectonic settings have played an important role in this process. Amphi-Pacific disjunctions is a striking distribution pattern in biogeography, and temperate elements between eastern Asia and North America have been extensively studied in plants (Wen, 2001; Donoghue and Smith, 2004; Wen et al., 2016). These have intrigued many botanists and biogeographers to produce more studies of Northern Hemisphere botanical biogeography (Gaynor et al., 2020; Zhang et al., 2020b; Zhou et al., 2020). Wen (2001) suggested that the disjunction pattern was largely caused by complex processes such as dispersal, extinction, speciation, vicariance, and stasis. The relevant disjunction patterns are common in plants, and many temperate forest groups originated and diversified within East Asia, followed by movements out of Asia to the New World (Wen, 1999; Manos and Donoghue, 2001; Milne and Abbott, 2002; Donoghue and Smith, 2004). Two major hypotheses have been proposed to explain amphi-Pacific tropical disjunctions —the boreotropics hypothesis and the West Gondwanan vicariance hypothesis (Yang et al., 2017). The boreotropics hypothesis postulates a continuous belt of tropical to subtropical forest at middle to northern latitudes of the Northern Hemisphere, and the continents were connected by the Bering and North Atlantic land bridges during the early Cenozoic (Wolfe, 1975; Tiffney, 1985; Lavin and Luckow, 1993; Wen et al., 2016). Recently, an increased focus on the amphi-Pacific tropical (subtropical) disjunction of taxa has supplemented our knowledge of how this distribution pattern has been achieved. Dendropanax (Araliaceae) is disjunctly distributed in tropical to subtropical Asia and the Neotropics, and Li and Wen (2013) hypothesized that the genus originated in the Old World and migrated to the New World via the North Atlantic land bridges in the early Tertiary. Yang et al. (2018) suggested a Eurasian origin of Sabiaceae (with an amphi-Pacific tropical disjunct distribution) in the late Cretaceous, and a boreotropical range expansion during the Paleogene and a long distance dispersal from Central America to tropical Asia during the Neogene and Quaternary boundary in Kingsboroughia alba. Lian et al. (2020) inferred that the formation and breakup of the boreotropical floral may have been responsible for the amphi-Pacific disjunct distribution within Pachygoneae. The West Gondwanan vicariance hypothesis postulates a tropical origin and expansion in southern West Gondwana followed by vicariance from tectonic separation into South America and Africa (Yang et al., 2017), and has not been applied to any taxa showing the amphi-Pacific tropical distribution pattern. Similar distribution patterns were also reported within groups such as Leydigiopsis species (Van Damme and Sinev, 2013), Symplocaceae (Fritsch et al., 2015), Diplazium (Wei et al., 2015), and diving beetles (Toussaint et al., 2017). Also, disjunct species ranges could be explained by long-distance dispersal and it is known that a great variety of processes can move seeds by anemochory, hydrochory, autochory, ectozoochory, and endozoochory (Van Der Pijl, 1982; Higgins et al., 2003).
Callicarpa (Lamiaceae), with the nickname ‘beauty berry’, was first described by Linnaeus (1753). The genus takes its name because of its attractive purple fruits usually displaying in the autumn (Figure 1). There are about 140 species of Callicarpa in temperate, subtropical, and tropical Asia, America, Australia, and the Pacific Islands (Leeratiwong et al., 2007; Bramley, 2009; Bramley, 2013; Ma et al., 2016). However, regional diversity is variable and the genus is more species rich in the Old World, particularly with ca. 51 species in Malaysia (Bramley, 2013) and ca. 48 species in China (Chen and Gilbert, 1994). The current distribution range of Callicarpa recognized species over the world is characterized by a typical East Asia-Southeast Asia/North America disjunction, especially in tropical and subtropical regions on both sides of the Pacific Ocean. Under this biogeographic pattern, differentiation history of Callicarpa might be a good candidates for biogeographic studies in pantropical plants. A tropical and subtropical amphi-Pacific disjunction is among the most fascinating distribution patterns, and what might have been responsible for this pattern is the focus of most research into this disjunction. East and Southeast Asia are pivotal, having two of the highest levels of species diversity in the Northern Hemisphere owing to their geological and climatological history (Latham and Ricklefs, 1993; Wu and Wu, 1996; Myers et al., 2000; Tan et al., 2020; Zhang et al., 2020b). During their evolutionary history, Callicarpa may have exhibited significant species diversification in Southeast Asia and East Asia. Equally importantly, most species of this genus are much-valued traditional medicinal plants, and research has tended to focus on several of them at present, such as C. bodinieri, C. macrophylla, C. kwangtungensis, C. nudiflora, and C. integerrima (Wu et al., 2018; Ma et al., 2022). Based on current research results, Callicarpa produce abundant flavonoid, terpenoid, and phenylethanol glycosides, and have significant pharmacological effects on the prevention and treatment of health disorders such as inflammation, menoxenia, hematuria, and scrofula (Tu et al., 2013; Yang et al., 2021).
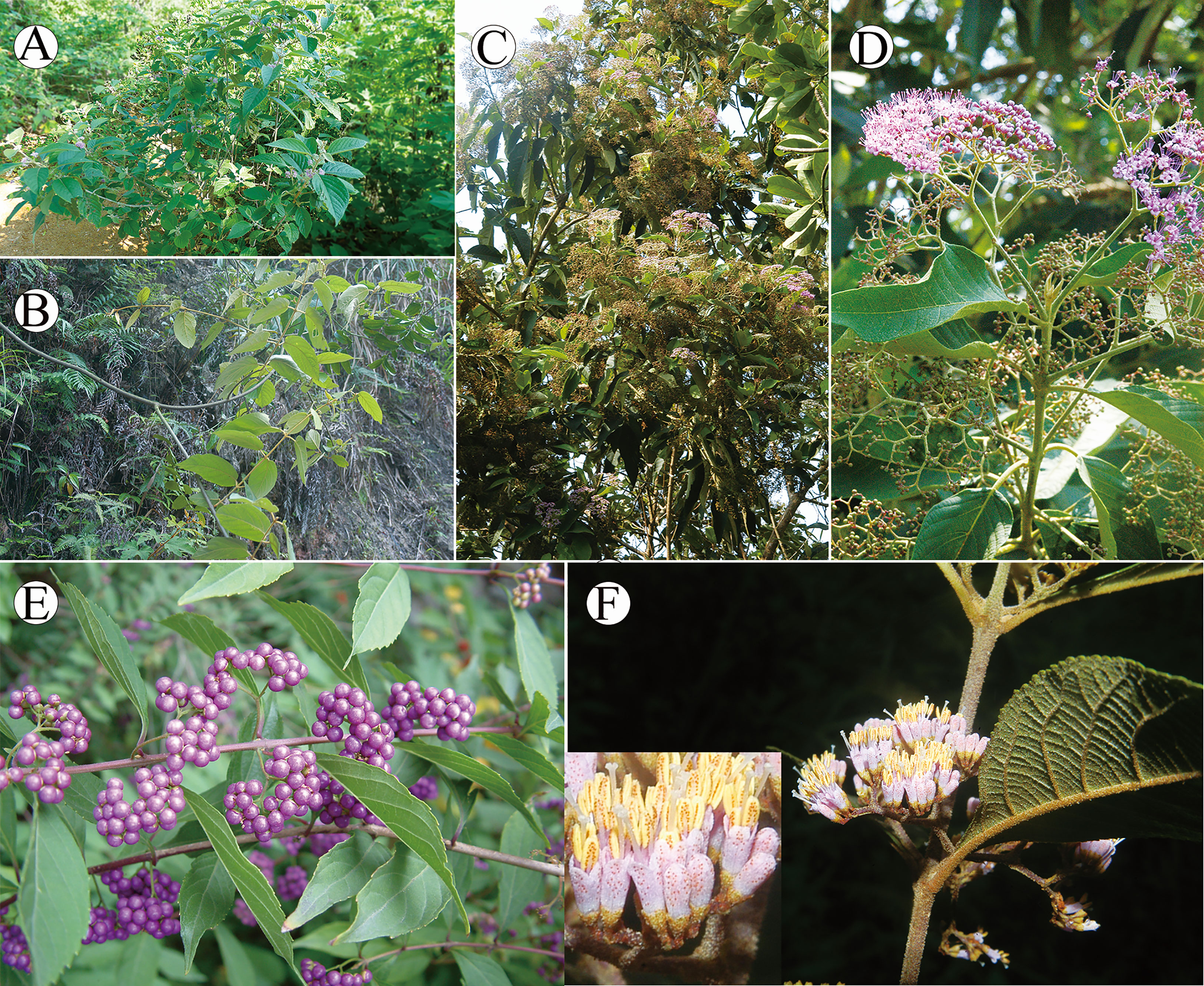
Figure 1 Morphology of Callicarpa. (A) erect shrub showed by C. candicans; (B) woody climber showed by C. integerrima var. chinensis; (C) tree showed by C. nudiflora; (D) Cymose or thyrsoid inflorescence, axillary showed by C. nudiflora; (E) purple fruits showed by C. dichotoma; (F) red glands on the inflorescences showed by C. bodinieri.
To date, no comprehensive molecular phylogenetic study on the infrageneric system of the genus has been presented. Here, we display the first comprehensive molecular investigation of the genus Callicarpa in Asia and discuss the infrageneric phylogenetic relationships. Our samples were mainly collected from China and Southeast Asia, alongside individual species expanding to Korea, New Guinea, Australia, and America. Currently improved phylogenetic underpinning is warranted to identify the factors responsible for shaping the present distribution of Callicarpa and gain new insights into the patterns of diversification of this genus in the World. The reconstruction of ancestral areas on a phylogeny is important for understanding the biogeographical history of a lineage, as it permits the inference of the place of origin and dispersal routes of organisms. In a sense, a better understanding of biogeographic history within Callicarpa could also provide a case for exploring biogeographic patterns in amphi-Pacific tropical disjunctions.
In this study, our objectives are (1) to reconstruct phylogenetic relationships within Callicarpa using eight chloroplast and two nuclear DNA regions, thus with a more comprehensive sampling than in previous studies; (2) reveal the timing of genus and species differentiation; (3) investigate the historical biogeography of the Callicarpa, a pantropical flora.
2 Materials and methods
2.1 Taxon sampling
A total of 145 accessions representing 56 recognized species and several unidentified species ingroups were collected in this study. Among them were four varieties represented by C. bodinieri var. rosthornii, C. integerrima var. chinensis, C. pedunculata var. longifolia and C. longifolia var. lanceolaria, and four forms represented by C. rubella f. angustata, C. rubella f. crenata, C. japonica f. kiiruninsularis, and C. brevipes f. annamensis. We selected Dasymalla teckiana, Dicrastylis parvifolia, Clerodendrum sp., Clerodendrum cyrtophyllum, Gomphostemma Chinense, and Vitex negundo as the outgroups. To estimate molecular divergence times of the Callicarpa group, we expanded our sampling more broadly across Lamiaceae to ensure sufficient representation for assigning appropriate fossil calibrations. Our sampling also encompassed the mainly biogeographic range of Callicarpa, including representatives from Asia, Australia, New Guinea, and America. The original sources of the plant materials used in this study and voucher information are presented in additional files (Table 1). We added fifteen sequences representing five species from Ocimeae, eight species from Mentheae, and two from Elsholtzieae, and the DNA sequence of closely related species were used to substitute for the several species lacking corresponding data (Li et al., 2016). All the data presented in the study are deposited in the NCBI database (https://www.ncbi.nlm.nih.gov/) (Table 2).
2.2 DNA extraction and sequencing
Two nuclear [internal transcribed spacer (ITS) and external transcribed spacer (ETS)] and eight chloroplast [(matK, rpl32-trnL, trnD-trnT, trnH-psbA, psbJ-petA, trnQ-5’rps16, 3’trnV-ndhC, and trnS (GCU)-trnG intergenic spacer] regions were used in this study. Extracting total genomic DNA of the samples with silica dried leaf tissue followed the 2 × CTAB method of Doyle and Doyle (1987), and the DNeasy Plant Mini Kit (Qiagen, Hilden, Germany) was used for herbarium materials following the manufacturer’s instructions. Primer pairs used in polymerase chain reaction (PCR) amplification of the ten regions are listed in Table S1 (White et al., 1990; Sun et al., 1994; Demesure et al., 1995; Sang et al., 1997; Baldwin and Markos, 1998; Beardsley and Olmstead, 2002; Tate and Simpson, 2003; Shaw et al., 2005; Andersson, 2006; Shaw et al., 2007; Dong et al., 2012). PCR products were assessed by electrophoresis on 2% agarose gel. The samples were sequenced on BGI’s sequencing platform both strands of DNA with overlapping regions. The raw sequences were initially edited with Sequencher v5.4.5 (Gene Codes Corporation, Ann Arbor, MI, USA) and then aligned in MAFFT v7.450 (Katoh and Standley, 2013) with manual adjustment where necessary in MEGA v7.0 (Kumar et al., 2016) and BioEdit v7.250 (Hall, 1999).
2.3 Phylogenetic inference
Phylogenetic analyses of the data matrices —two nuclear ribosomal DNA, eight chloroplast DNA and combined ten locus data —were conducted respectively using maximum likelihood (ML) and Bayesian inference (BI) methods. The ML phylogeny was reconstructed in the program Phylosuite v1.2.2 (Zhang et al., 2020a) with the Iqtree (Nguyen et al., 2015). The bootstrap (BS) percentage for each branch was estimated by running 1,000 bootstrap replicates. The BI analysis was conducted with MrBayes (Ronquist et al., 2012) in Phylosuite v1.2.2 (Zhang et al., 2020a) and implemented in CIPRES (http://www.phylo.org/) (Miller et al., 2010). For BI analysis, ModelFinder (Kalyaanamoorthy et al., 2017) was used for the selection of the most appropriate evolutionary model (nucleotide substitution model) (Edge-linked) using BIC criterion. The run with 10,000,000 generations was conducted. Four Markov chains with two runs were implemented and sampled every 1,000 generations, and the first 25% of all trees were regarded as ‘burn-in’. The majority consensus of the remaining trees was generated to show posterior probability (PP) support for clades. Convergence was determined in Tracer v1.7.1 (Rambaut et al., 2018) and was considered to be attained when ESS > 200 or when the average standard deviation of the split frequencies was < 0.01. The best-fit partition model for Iqtree and Mrbayes analysis are listed in Table S2.
2.4 Molecular divergence time estimation
Lineage divergence time provides important information for understanding biogeographic history. As there are no known fossils for Callicarpa, molecular dating in this study relies on fossils from related clades in Lamiaceae. Although Lamiaceae are also not well represented in the fossil record, there are accepted fossils that can be used as calibration points in related studies within the family. We used the following two fossils as calibration points (Drew and Sytsma, 2012; Li et al., 2017). The first one was suggested conservatively to place at the crown of Nepetoideae. It was based on the hexacolpate and three-nucleate pollen fossil from Early Eocene sediments in India, which was identified as Ocimum (Kar, 1996). The second fossil calibration point was the fruit fossil of Melissa from the Early-Middle Oligocene (Martinez-Millan, 2010), which was assigned to constrain the most recent common ancestor (MRCA) of Melissa and Neoeplingia. We used a reduced dataset of 56 samples keeping one accession per species. Divergence times were estimated using BEAST2 (Bouckaert et al., 2014) in CIPRES (http://www.phylo.org/) with the nuclear and plastid concatenated matrix, and before that we got the appropriate XML file by Beauti (part of the BEAST package). A Yule tree prior and the relaxed exponential clock model were selected. The substitution model for each partition was determined within the Akaike information criterion (AIC; Akaike, 1974) as implemented in the program MrMtgui (Nylander, 2004) (Table S3). Two calibration points we all used a lognormal distribution model. Additionally, the first fossil had an offset at 49 million years ago (Ma), a mean of 2.6 Ma, and a standard deviation (SD) of 0.5 Ma while the latter had an offset at 28.4 Ma (mean: 2.6 Ma, SD: 0.5 Ma). Markov Chain Monte Carlo chains were run for 400,000,000 generations with sampling every 40000 generations. Convergence and the adequate effective sample size values (>200) for the BEAST analysis was evaluated in Tracer v1.7 (Rambaut et al., 2018). We ran each dataset four times respectively and combined the tree files in Logcombiner (part of the BEAST package). After burn-in of 20%, the maximum clade credibility (MCC) tree with median branch lengths and 95% highest posterior density (HPD) intervals on nodes was calculated using TreeAnnotator (part of the BEAST package). To get a simple temporal dynamics of diversification of Callicarpa, we generated standard lineage-through-time (LTT) plots for the maximum clade credibility (MCC) tree of sampled species in the R package ‘ape’ (Paradis et al., 2004). To convert stratigraphic ages into absolute ages, we used the geological timescale (Walker et al., 2018).
2.5 Ancestral area reconstruction
For biogeographic reconstruction, we assigned Callicarpa species to eight areas based on their distribution records acquired from the literature, our own fieldwork, and herbarium records: A) East Asia; B) South Asia; C) Southeast Asia; D) Oceania (including Australia, New Guinea, New Zealand; E) Temperate North America; F) Neotropics (Cuba, Columbia, Peru and Bolivia); G) Islands of India Ocean (Mascarene Islands and Reunion Island); H) Pacific Islands. The analysis was implemented by statistical dispersal extinction cladogenesis (S-DEC) in RASP (Yu et al., 2015; Yu et al., 2020). We used the maximum clade credibility (MCC) tree with fossils calibration produced in BEAST to estimate the ancestral geographic ranges of Callicarpa. The trees from BEAST were used as input trees, and other parameters were set to their default.
3 Results
3.1 Phylogenetic relationships between infrageneric species
Topologies derived from cpDNA (eight regions) and nrDNA (two loci) were broadly congruent (Figures S1-1, S1-2), but better resolution and stronger branch support was achieved by combining the datasets. The phylogenetic trees using BI and ML analyses had nearly the same topology, only differing in the location of C. peichieniana (Figures S2-1, S2-2).
The combined analysis indicated that the genus Callicarpa was monophyletic (Posterior probability, PP=1.00; Bootstrap percentage, BP=100) with respect to the groups considered, and eight well-supported primary clades of the genus were resolved (Figure 2). Clade I (PP=1.00, BP=100) branched off first in the genus and was composed of some tropical species native to Southeast Asia with large anthers and short filaments. Clade II was comprised of tropical species, small trees species of Callicarpa (PP=1.00, BP=73). Callicarpa luteopunctata split from the remaining species and formed a separate branch (Clade III, PP=1.00, BP=100). This species has an extremely narrow geographical distribution and is only found in high altitude mountains of the Yunnan-Guizhou Plateau located in Southwest China. In addition, the species with variable flower parts and initially being of the genus Geunsia Blume formed a strongly supported group Clade IV (PP=1.00, BP=100). Two species from America formed an independent clade V. In BI analyses (Figure S2-1), Clade VI consisted of C. dichotoma, C. integerrima var. chinensis with good support (PP=0.99, BP=97), which represented the rare lianas or slender-climbing species of this genus, and C. peichieniana forming a single branch (PP=0.53), while in the ML tree (Figure S2-2), three species comprised Clade VI (BP=60). It’s a pity that their positions weren’t resolved well. Clade VII was also a well (moderately) supported clade (PP=0.98, BP=97) consisting of two subclades. Subclade VIII consisted of two subtropic species with robust cymes (C. macrophylla and C. nudiflora). Subclade VIIII was characterized by a conspicuous interpetiolar ridge and white baccate drupe, and the tree indicated the paraphyletic status of C. longifolia. Clade VIII was recognized as one broad clade, which was strongly supported as the largest group of Callicarpa investigated in the present study (PP=1.00, BP=100) and comprised some taxa with a broad range of variation in morphological characters. This clade mostly represented small shrubs with simple slender cyme and when more than one accession of a species was applied, they were either exclusive lineages or grouped together with more closely related species in a particular clade or more than one small group (e.g., C. brevipes, C. giraldii, C. bodinieri, C. formosana and C. rubella).
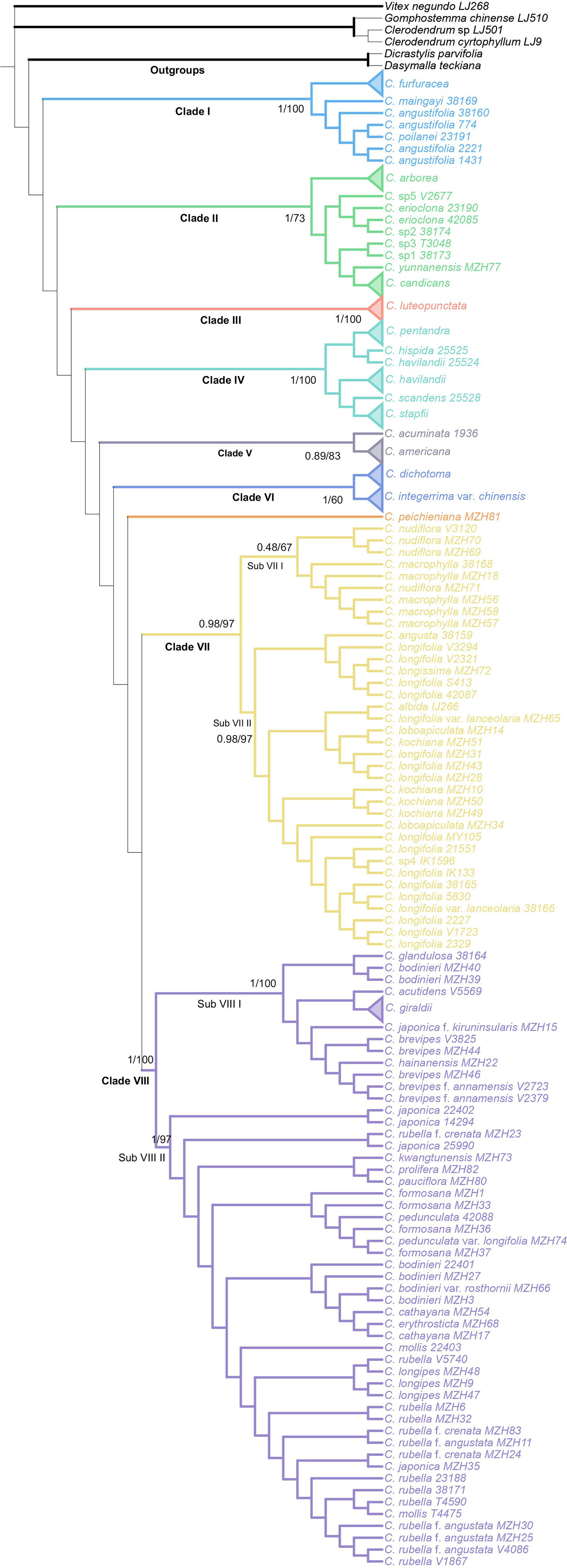
Figure 2 Bayesian consensus tree of Callicarpa based on the combined DNA data (two nuclear and eight chloroplast regions). Maximum likelihood bootstraps and Bayesian posterior probabilities of major clades are shown.
3.2 Divergence time estimation and biogeographical reconstruction
The divergence time estimates based on nrDNA+cpDNA datasets were here reported and used for further biogeographical analysis. The BEAST analysis indicated that the crown group age of Callicarpa was estimated at 36.23 Ma (95% HPD: 18.81–60.39 Ma) around the Late-Eocene and diversification within most clades concentrated in the Miocene and continued to the Pleistocene (Figure 3, node 1). Our dating suggested that C. americana was the first to split from the remaining species in the Middle-Oligocene, ca. 27.9 Ma (Figure 3, node 2). The crown age of the tropical occurring, small trees species of Callicarpa was inferred to be ca. 17.03 Ma (95% HPD: 7.35–28.3 Ma) (Figure 3, node 3). There was an early middle Miocene crown age for several native species in Southeast Asia, ca. 16.51 Ma (95% HPD: 3.42–33.78 Ma) and ca. 16.14 Ma (95% HPD: 6.88–26.98 Ma), respectively (Figure 3, node 4, node 5). The ages of the crown nodes of the two great groups were estimated to be at a similar time, ca. 16.51 Ma (95% HPD: 9.48-25.16 Ma) (Figure 3, node 6) and ca. 13.53 Ma (95% HPD: 6.46-22.37 Ma) (Figure 3, node 7). Furthermore, the divergence time of major species are listed in Table S4. The LTT plot for Callicarpa overall is shown in Figure 3-1. Before about the middle-Miocene (ca. 14 Ma), there was a relatively stable diversification rate and then a subsequently rapid diversification (accelerated lineage accumulation). This upward trend was maintained during the Pliocene and the Pleistocene.
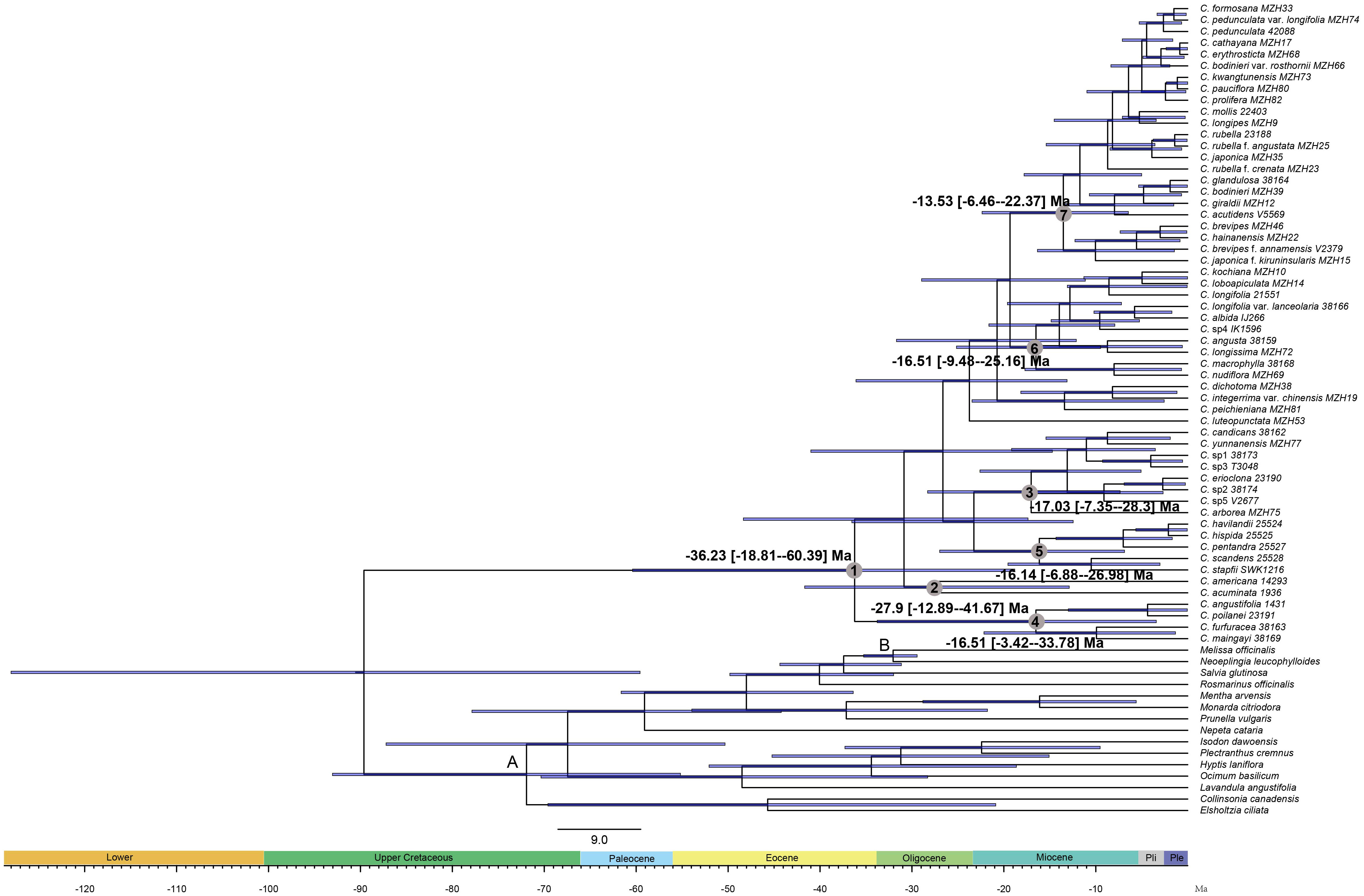
Figure 3 BEAST chronogram of Callicarpa inferred from combined DNA data (two nuclear and eight chloroplast regions) based on two fossil calibrations. Bars representing the 95% highest posterior density intervals. Node A was constrained to 49 Ma and node B was constrained to 28.4 Ma.
The statistical dispersal extinction cladogenesis (S-DEC) model reconstructed a composite area of both East Asia and Southeast Asia as the likely ancestral areas for the recent common ancestor (MRCA) of Callicarpa at approximately 36.23 Ma in the Late-Eocene (Figure 4, node A). There was one dispersal event from East Asia to Southeast Asia and one extinction event in East Asia. The early diversification of a Callicarpa ancestor occurred at 30.86 Ma (95% HPD: 17.37-48.34 Ma) and the first extinction event was inferred to have occurred in East Asia (Figure 4, node B). During this period, this genus may have undergone an eastward dispersal from Asia to Pacific Islands and subsequently there could be dispersal, vicariance, and extinction events resulting in the split between the Old World Callicarpa clade and the New World Callicarpa clade (Figure 4, node C). Subsequently, there was the first dispersal from Southeast Asia to East Asia (Figure 4, node D, ca. 26.63 Ma). During the early-middle Miocene, there were the first dispersal event which led to the successful colonization of the genus in Oceania (Figure 4, node F, ca. 17.03 Ma) and similar dispersal events also occurred after middle Miocene. The dispersal from Asia into the Mascarene Islands and Reunion Island took place at 8.01 Ma (95% HPD: 0.68-17.71 Ma) (Figure 4, node G). After several vicariance and extinction events, some species endemic to China arose. The diversification of C. luteopunctata occurred around 23.75 Ma (95% HPD: 13.12-36.09 Ma). Callicarpa hainanensis, a new species discovered from Hainan, China, diverged at 3 Ma (95% HPD: 0.1-7.35 Ma) with a vicariance event during the boundary period of the Miocene and Pliocene. The RASP analysis suggested that the ancestral area of these species was East Asia and Southeast Asia, and under the influence of these events they were only occurring in China or Southeast Asia. In summary, multiple dispersal and vicariance events have occurred over the evolutionary history of Callicarpa.
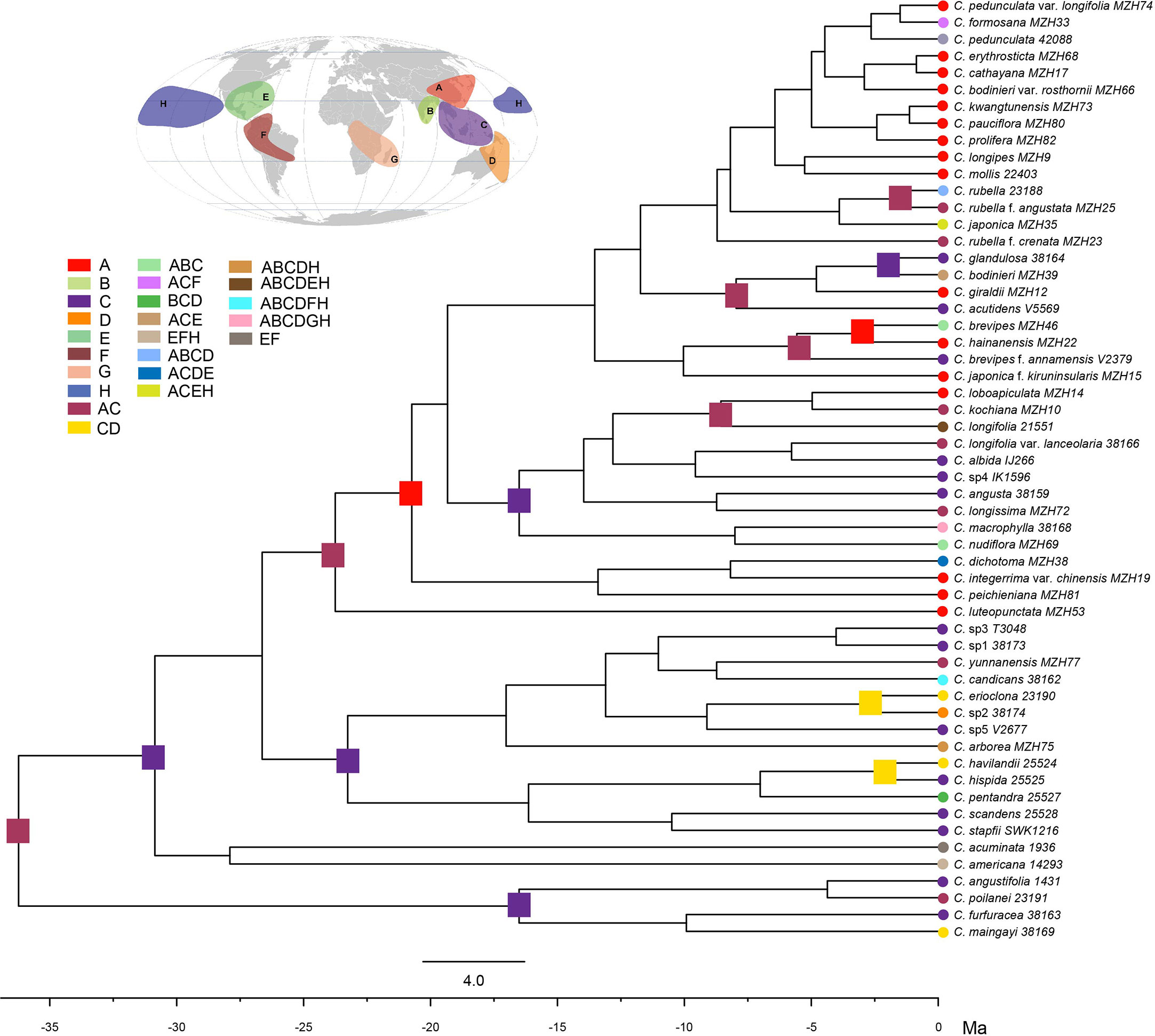
Figure 4 Ancestral range estimation of Callicarpa using the BEAST maximum clade credibility chronogram. Letters a-g indicate nodes of interest. Ancestral range reconstruction was performed by S-DEC in RASP. Map showing eight biogeographical regions in colors as defined in this study.
4 Discussion
4.1 Phylogenetic inference
The combined analysis indicated that Callicarpa was monophyletic (PP=1.00, BP=100) with respect to the groups considered, and within Callicarpa, eight main subgroups were recognized (clade I-VIII) (Figure 2). Clade I, located at the bottom of the phylogenetic tree of Callicarpa, was composed of some Malaysian and Thai species: C. poilanei, C. angustifolia, C. maingayi, and C. furfuracea. After examining and comparing the type of specimens of C. angustifolia and C. poilanei, Leeratiwong et al. (2007) regarded C. poilanei as the synonym of C. angustifolia because they bear a resemblance in having a prominently interpetiolar woody ridge at the stem nodes, grey to brownish-grey hairs on the abaxial surface of leaves, and being glabrous or with sparsely hairy ovary. In the present study, our result supported Leeratiwong’s treatment (PP=1.00, BP=100). Callicarpa furfuracea and C. maingayi formed a robust clade, which suggested their closer relationship. Evidence from morphology (Leeratiwong et al., 2007; Leeratiwong et al., 2009) seemed to favor this clade. Clade II contained several small tree species, with tropical occurrences (Thailand, Vietnam, Indonesia, Malaysia, New Guinea), although C. candicans, C. yunnanensis, and C. arborea also occur in some narrow areas of southern China (south of Hainan Island and Xishuangbanna, Yunnan). In Bramley’s study (Bramley, 2009), C. candicans formed a clade with C. furfuracea, C. maingayi, C. angustifolia, and C. poilanei (assigned into section Clade I in our study) without support rate. However, in the present study, C. candicans exhibited a closer sister relationship with C. erioclona and C. yunnanensis (PP=1.00, BP=71). Morphologically C. candicans is most easily confused with C. erioclona, but the indumentums on the outer surface of the ovary and fruit may be effective morphological markers to distinguish them. In C. erioclona, the ovary and fruit are covered with branched hairs while that of C. candicans is glabrous. In addition, the black fruit (when mature) of C. candicans (Figure 5A) allows it to be distinguished from C. erioclona (maturing purple). Within clade II, C. arborea (Figure 5B) appeared at the top of the clade and as the sister of other tree species. Although C. arborea and C. yunnanensis are extremely similar and share an overlapped distribution, they appeared in different branches in clade II. Geographically, C. arborea is one of the most common species of the genus with a wide distribution (almost everywhere in Southeast Asia, Leeratiwong et al., 2009; Bramley, 2013), while Yunnan (Southwest China) is the edge of its northernmost distribution (Fang, 1982; Chen and Gilbert, 1994). Contrarily, C. yunnanensis is just narrowly distributed in the mixed forests of valleys in southern Yunnan and northern Vietnam (Fang, 1982; Chen and Gilbert, 1994). For palynology, two species can be distinguished from each other by their different types of pollen exine ornamentation: the pollen of C. yunnanensis has an exine sculpture of rugosely reticulate while C. arborea has regulate exine ornamentation (Ma et al., 2016). Callicarpa luteopunctata (Figure 5C) formed an independent branch (Clade III) and this species has an extremely narrow geographical distribution, only found in high altitude mountains of the Yunnan-Guizhou Plateau located in Southwest China. Their branchlets are cylindrical and there are no ridges or hair ring between the two petioles, and the peduncle is usually shorter than the petiole. In particular, both sides of the leaves have densely yellow glades. Clade IV contained several Indonesia-Malaysia-Thailand distributed species originally described as Geunsia (Figure 5D). Our results indicated that the ‘Geunsia’ group formed a well supported clade (PP=1.00, BP=100). The New World group was composed by C. americana (Figure 5E) and C. acuminate, which are ranging in North America and they formed a clade (Clade V, PP=0.89, BP=83). Old World and New World lineages also shows a complex phylogenetic relationship. In the present study, C. integerrima var. chinensis and C. dichotoma formed Clade VI, which represented the few lianas or slender-climbing species of Callicarpa mainly distributed in China (Figures 1B, E). When carrying out a palynology study on Callicarpa in China, Ma et al. (2016) suggested that the distinct coarsely reticulate exine sculpture was found only in certain species – those that represented the climbing shrubs species of Callicarpa native to China (C. integerrima, C. integerrima var. chinensis and C. pilosissima). Based on the distinct, strongly curved hairs on the stem and extremely simple cymes (only 1–3 flowers, or one dichotomous, Figure 5F), Fang (1982) treated C. peichieniana as a monotypic subgenus (Subgen. Peiantha Chun et S. L. Chen) and other species constituted the subgenus Callicarpa. However, our phylogenetic result from ML analyses showed that C. peichieniana was mixed with other species (Subgen. Callicarpa), which does not support Fang’s classification system (Fang, 1982). The traditional subgenus classification system of Callicarpa based on only two characters is unpredictable. Clade VII was further divided into two main subclades: subclade VIII and subclade VIIII. Subclade VIII consisted of C. nudiflora and C. macrophylla (Figure 1D; Figure 5G), and both species share a series of common characteristics. However, inflorescence width and peduncle length are different between the two species, while the most significant difference to distinguish the two species is that the calyx, corolla, and ovary of C. macrophylla are covered by stellate tomentose, while those of C. nudiflora are glabrous. In addition, it is worth bearing in mind that the leaves of C. nudiflora turn black after being dried while those of C. macrophylla do not. Subclade VIIII contained several species characterized by a conspicuous interpetiolar ridge resembling a stipule scar, and a white baccate drupe with an obviously softer fleshy exocarp than other species of Callicarpa. Yet the color and textures of the exocarp have never attracted the attention of previous taxonomic researchers working on Callicarpa. In Subclade VIIII (PP=0.98, BP=97), these species are primarily distributed in China, although C. longifolia is considered as a broadly distributed species (Figure 5H), and C. angusta is endemic in Vietnam. Based on C. kochiana tubular calyx (Figure 5I), Fang (1982) assigned C. kochiana as a monotypic section Tubulosae, and divided other species of the subgenus Callicarpa into Section Callicarpa. In the present study, C. kochiana is embedded in C. longifolia and C. loboapiculata, and together form a clade (PP=0.43, BP=56), which shares a series of synapomorphy: a conspicuous interpetiolar ridge and white baccate drupe. Increasing the number of samples of C. kochiana and more genetic data is all needed to confirm its species status (Chanderbali et al., 2001; Small et al., 2004; Guo and Ge, 2005; Liu et al., 2021). Clade VIII could be recognized as one broader clade which was strongly supported as the largest group of Callicarpa investigated in the present study (PP=1.00, BP=100), and comprised of some taxa with a broad range of morphological variation. The Subclade VIIII was formed with good support (PP=1.00, BP=100). Among them, C. hainanensis and C. brevipes formed a small clade (Figures S2-1, S2-2, PP=0.96, BP=84) characterized by their lanceolate or obovate-lanceolate leaves (Figure 5J), and the two species share a series of typical characters of Chang’s (1951) section Verticirima, such as larger, oblong, apical pore dehiscent anthers and short filaments. However, C. hainanensis is obviously distinguished from the latter by its obovate-lanceolate leaves, long-cup-shaped or subtubular calyx dehisced as the fruits mature, and sharp triangular lobes (Ma and Zhang, 2012). Callicarpa bodinieri is easily distinguished by its red subsessile glands on stem, leaves, and flowers from other species (Figure 5K), and Leeratiwong et al. (2009) found that C. bodinieri and C. glandulosa are conspecific by examining their specimens. Therefore, Leeratiwong et al. (2009) reduced C. glandulosa to be a synonym of C. bodinieri. In this study, they also formed a very close relationship (Figures S2-1, S2-2, PP=1.00, BP=92). Within subclade VIIIII, C. pedunculata, C. formosana, and C. pedunculata var. longifolia clustered into one subclade (Figures S2-1, S2-2, PP=1.00, BP=100). During the taxonomic revision of Philippines Callicarpa, Bramley (2013) treated C. formosana as one of the numerous synonyms of C. pedunculata, a species with an extensive distribution and significant variation in leaf shape. As our molecular results suggest, C. pedunculata from Australia was nested within C. formosana, so we supported reduction of C. formosana to the synonym of C. pedunculata according to the rules of nomenclature. Bramley (2013) indicated that C. pedunculata was most likely to be confused with C. rubella, from which it differed by its typically relatively narrow leaves and lack of glandular hairs but with abaxially stellate tomentose, adaxially minute hispid, and an obtuse or rounded base. Their close relationship was also verified in the present phylogenetic study as two species appearing in close sister clades. It was unexpected that C. kwangtungensis (Figure 5L), designated as a typical species of section Verticirima in the Chang (1951) system, formed a sister clade to C. prolifera and C. pauciflora (Figures S2-1, S2-2, PP=0.95, BP=99). For C. rubella, morphologically, we found in our field investigation that white fruit populations of C. rubella always mix with purple fruit populations in the coinhabiting areas (Figures 5M, N). As a broadly distributed species, C. rubella is variable morphologically, especially in terms of its indumentum and the size and shape of the leaves. The significant difficulty in determining the identity of the complex group of C. rubella based on morphology and phylogenetic placement suggests this group may potentially represent hybrids, although we are unaware of the specific parental origin. It makes sense that identical repeated interspecies hybridization may occur in C. rubella and its infraspecific taxa. In Japanese Callicarpa, Tsukaya et al. (2003) reported the hybridization and introgression occurring between C. japonica and C. mollis in central Japan with molecular data, observing that both species were pollinated by bees (Kawakubo, 1990; Momose et al., 1998; Kato et al., 1999; Kato, 2000). Xu et al. (2013) investigated the cross breeding between C. dichotoma and C. bodinieri in China and their result indicated that there was no crossing barrier between the two species, and they observed a high fruit setting ratio. Callicarpa longipes and the complex group of C. rubella formed a group morphologically well delimited with other species by a cordate leaf base (Figures S2-1, S2-2, PP=0.73, BP=94) (Figure 5O). The complex group of C. rubella has troubled researchers for a long time in terms of distinguishing each species due to their extremely ambiguous morphological circumscription. We have to pay attention to C. japonica which formed a close relationship with C. rubella f. crenata (Figures S2-1, S2-2, BP=100, PP=1.00). In Bramley’s revision of Bornean Callicarpa (Bramley, 2009), the sister clade of C. japonica and C. rubella was also represented.
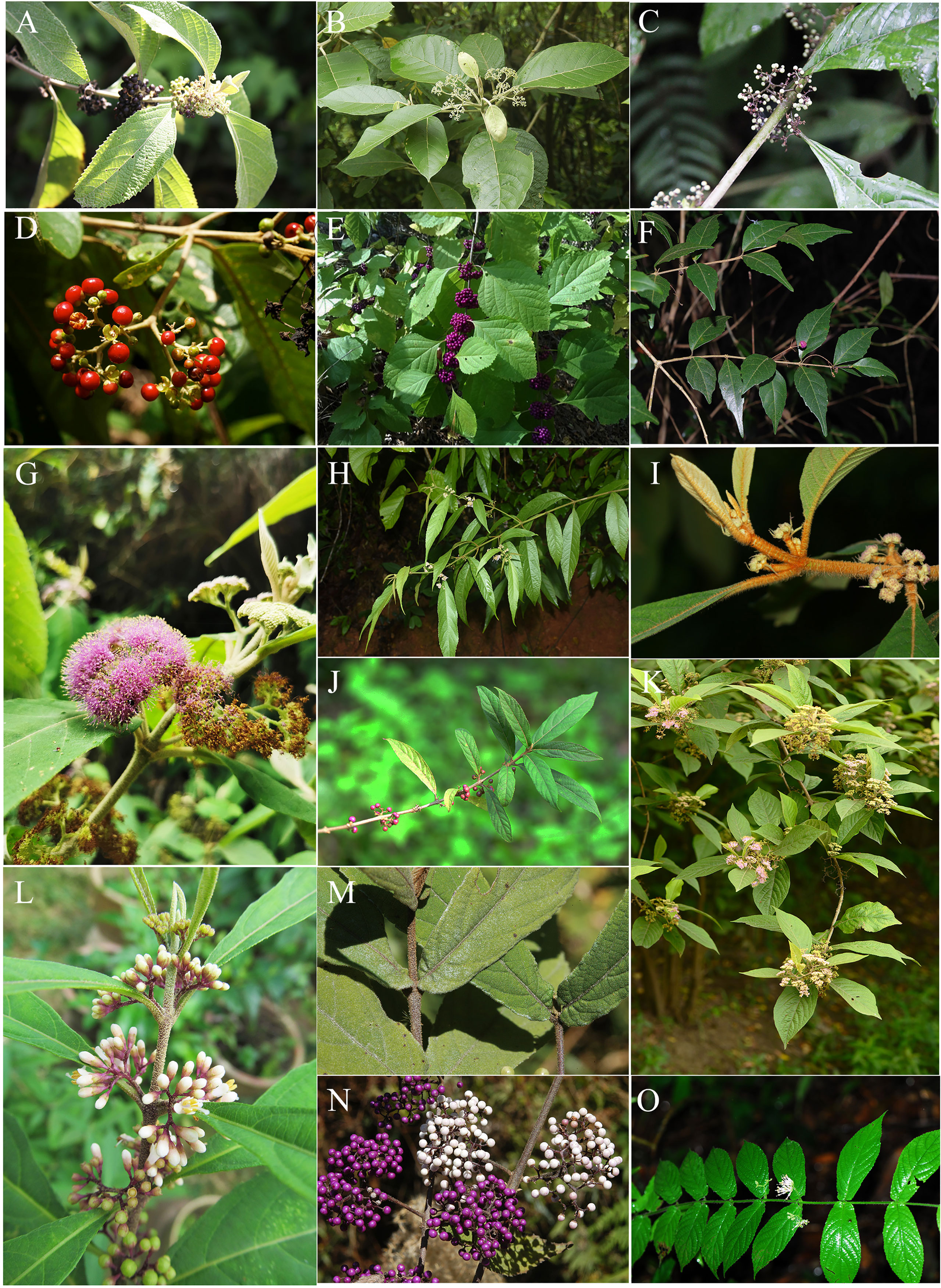
Figure 5 Morphological characteristics of Callicarpa for phylogenic discussion. (A) C. candicans; (B) C. arborea; (C) C. luteopunctata; (D) C. pentandra; (E) C. americana; (F) C. peichieniana; (G) C. macrophylla; (H) C. longifolia; (I) C. kochiana; (J) C. brevipes; (K) C. bodinieri; (L) C. kwangtungensis; (M, N) C. rubella; (O) C. longipe.
4.2 East Asia and Southeast Asia as the ancestral area and Callicarpa’s lineage diversification within Asia
The ancestral range reconstruction analyses indicated that Callicarpa most likely originated in East Asia and Southeast Asia (Figure 4, node A). With our time estimates, the S-DEC model inferred that the main diversification events in Callicarpa were dated to the Middle-Oligocene, mainly concentrated to the Miocene (Figure 4). Multiple dispersal events were likely responsible for the current biogeographic patterns of the genus. This biome began to rise in the early Miocene (ca. 20 Ma) and further diversified in the late Miocene, driven probably by the intensifying East Asian summer monsoon during these two periods (Guo et al., 2002; Sun and Wang, 2005). In later branching lineages the genus might have experienced more rapid diversification in the Middle-Miocene (Figure 3-1, LTT plot). It probably corresponded with one of the major uplifts of the QTP and subsequent aridification events (Yu et al., 2014). Before about 14 Ma, there was a relatively stable diversification rate and subsequently an accelerated lineage accumulation. This upward trend was maintained during the Pliocene and the Pleistocene, which had been attributed to topographic and climatic circumstances (Qian and Ricklefs, 2000). Since the middle-late Oligocene to the Miocene, the coverage of Antarctic glaciers shrunk owing to global warming, and there was a high point of temperature in the middle-Miocene (Gao et al., 2020). Donoghue and Smith (2004) once proposed that the Miocene was one of the active periods of species diversification. The occurrence of the monsoon was characterized by changes in the prevailing wind direction and severe precipitation. It was often accompanied by the rapid strengthening of atmospheric-energy and the water cycle, which directly affected the global hydrothermal cycle and heat distribution, and regulated global climate change (Webster et al., 1998; An et al., 2000; Jiang et al., 2017). Numerous studies have suggested that Southeast Asia is a ‘museum’ of early angiosperms, harboring tropical rain forests with the most pronounced monsoon climate and acting as an ‘evolutionary front’ for some tropical taxa, given that it has the largest archipelagos and probably has the most complex geological history in the world (Van Welzen et al., 2011; Tan et al., 2020). The extremely rich biodiversity in Southeast Asia hints not only to one of the birthplaces and refuges of early angiosperms (Buerki et al., 2014), but also to a meeting point for the long-distance spread of species (Gunasekara, 2004; Lohman et al., 2011), which profoundly affects the formation and evolution of global flora. The Sunxdaland (Malay Peninsula; Borneo; Sumatra) and the Philippines are two acknowledged biodiversity hotspots in Southeast Asia (Myers et al., 2000) and there appear to be two major centers of diversity in terms of numbers of Malesian species for Callicarpa: Borneo and the Philippines (Bramley, 2013). Moreover, our findings likewise suggested that East Asia and Southeast Asia are main sources of biodiversity of Callicarpa.
Around 23.75 Ma (95%HPD: 13.12-36.09 Ma), there occurred the third extinction event in Southeast Asia resulting in Callicarpa luteopunctata splitting from the remaining species (Figure 4, node E). This species has an extremely narrow geographical distribution and is found only in high altitude mountains of the Yunnan-Guizhou Plateau located in Southwest China. Coincidentally, C. kinabaluensis and C. clemensorum were also reported to be narrowly distributed at high altitude (1600–2500 m) on the peaks surrounding Mount Kinabalu in Malesia (Bramley, 2011). A set of common features shared by C. kinabaluensis and C. clemensorum was speculated to be an adaptation to the frigid environment: so dense hairs and an interesting inflorescence structure with distinct peduncles and almost globose cymes. In addition, their twigs have scattered warty growth and the lamina surface occurred vesiculose (Bramley, 2009). However, C. luteopunctata has the indumentum on the petioles and cymes obviously sparser than that of C. kinabaluensis and C. clemensorum. It is a pity that this study failed to contain the two Malaysian species and we still lack an understanding of the unambiguous mechanism of these specific high altitude species in the genus Callicarpa of typical near tropics originated (Bramley, 2009; Bramley, 2013; Ma et al., 2016). Further studies on how the mechanism developed are urgently needed in the future. However, the onset of the Asian monsoon around the Oligocene-Miocene transition created a connection between forests from the low to high latitudes of East Asia (Sun and Wang, 2005; Ji et al., 2019), which might provide some insights. Subsequently, more frequent exchanges took place in the Asian interior among species we investigated in the present study. At 36.23 Ma (95%HPD: 18.81-60.39 Ma) there was the first dispersal from East Asia to Southeast Asia (Figure 4, node A; Figure 6, line 1) and subsequently, after the Oligocene, several similar dispersals (from East Asia to Southeast Asia) happened again with higher probability in Callicarpa. At the Oligocene-Miocene boundary, the Tibetan Plateau experienced a rapid uplift and previous geological evidence indicated that different areas of the Plateau have experienced different degrees of uplift at different times (Neogene and Quatemary) (Royden et al., 2008; Wang, 2017). These uplifts since the early Miocene have created high mountains and deep valleys within the plateau, which could have accelerated the production of new allopatric species, and been partly responsible for the high local and regional species richness (Liu et al., 2006) and induced extreme drying and desertification in the Asian interior, strengthening the Asian monsoons with a shift occurring from arid/semi-arid in the Asian continental interior (Guo et al., 2002; Sun and Wang, 2005). These factors probably effected the expansion of Callicarpa species within Asia regions later on (Figure 6, line 3, 5). Our study indicated that at 26.63 Ma (95%HPD: 14.72-41 Ma), there was first migration from Southeast Asia to East Asia (Figure 4, node D Figure 6, line 3) and during the Miocene-Pliocene boundary, two vicariance events occurred between Southeast Asia and East Asia, which influenced C. brevipes f. kiruninsularis, C. hainanensis, and C. giraldii all endemic to China. Under the influence of tectonic motion and climate fluctuation, dispersal and vicariant events occurred alternately, and habitats periodically isolated and merged, which may accelerate species differentiation in this process (Thomas et al., 2012). In Asia, East Asian monsoons, South Asian monsoons, and Northwest Pacific monsoons prevailed in summer (Jiang et al., 2017), which might have promoted the rapid differentiation and spread of Callicarpa and facilitate tropical species to spread northward (mainly to the area now China) by regulating rainfall. Apparently, the climate of Asia was controlled mainly by the monsoon system due to intense land-ocean thermal contrast, and the dynamics and thermal effects of the Tibet Plateau (Sun and Wang, 2005). The monsoon climate intensified from the late-Miocene, simultaneously bringing an East Asian subtropical humid climate and promoting the floristic expansion of Asia (Ji et al., 2019). We speculated that climatic change and global cooling since the mid-Miocene might have played a crucial role in the inferred onset of diversification of Callicarpa in Asia. During this time, several independent vicariance events between East Asia and Southeast Asia occurred, which accelerated some endemic species to form in China. Also, the S-DEC model revealed the divergence of C. dichotoma and C. japonica, two species distributed in Japan. The divergence times of them were 8.19 Ma (Miocene) and 3.9 Ma (Pliocene), and our results speculated that the opening of the Japan Sea corresponded roughly to their spread from continental East Asia to the Japan islands (Santosh and Senshu, 2011; Ji et al., 2019). Sea levels fluctuating alternately enabled alternate conditions for population fragmentation and admixture of temperate biota in this East China-Japan-Korea region (Qiu et al., 2011). It has long been recognized that the climatic changes of the Quaternary caused repeated shifts in the distribution of plants and animals presently found throughout the SJFR (Sino-Japanese Floristic Region). The RASP analysis also suggested the last ancestral area of C. giraldii was East Asia and Southeast Asia, and under the influence of one vicariance event, the species occurred only in China. C. giraldii seems able to tolerate a relatively cool climate and is widely distributed from subtropical Southeast China (Jiangxi, Fujian, Hunan) to temperate areas (Henan, Shanxi). Morphologically, they are small shrubs with cabined, tight cyme.
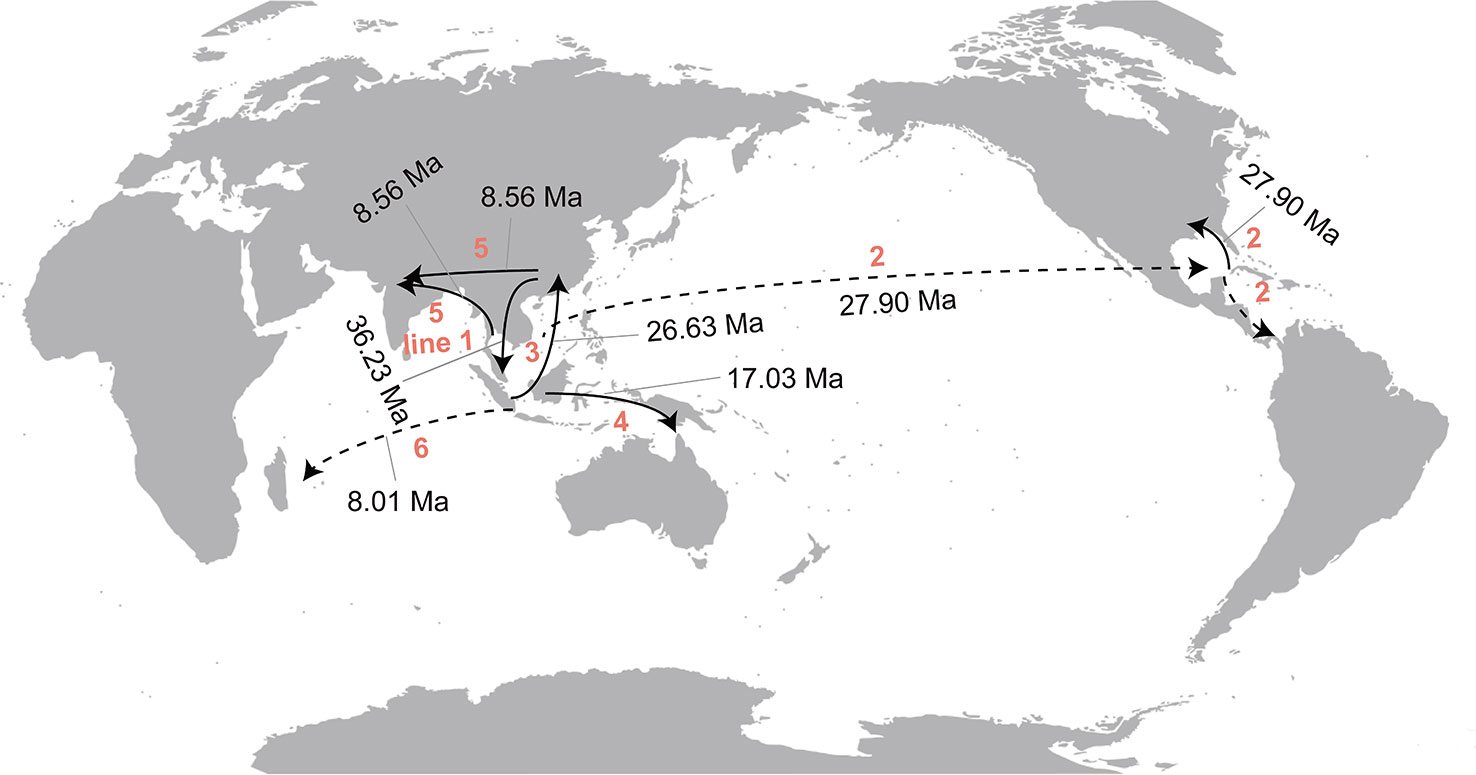
Figure 6 Historical migrations in Callicarpa estimated from the ancestral area reconstruction. The estimated times for the first migration among areas are shown. The dotted line represents the guessed route according to the distribution of the genus.
4.3 The disjunct pattern between the Paleotropics and Neotropics in Callicarpa
Callicarpa also presents an amphi-Pacific distribution pattern. Our results revealed that during the Middle-Oligocene, floristic exchange in Callicarpa occurred between Southeast Asia and the Temperate North America-Neotropical region (Figure 4, node C). Nevertheless, our inferred date for migrations of Callicarpa from the Paleotropics to Neotropics is too young to have been caused by the breakup of Gondwana (Lomolino, 2010; Jin et al., 2020). The oft-stated view is that the North Atlantic Land Bridge (NALB) or Bering Land Bridge (BLB) has played a major role in this process (Wolfe, 1975; Tiffney, 1985; Tiffney and Manchester, 2001; Chen et al., 2020; Zhang et al., 2020b). The NALB generally functioned as a famous migration route for thermophilous plant taxa between Europe and eastern North America (Tiffney and Manchester, 2001; Ian, 2006; Jin et al., 2020). Tiffney and Manchester (2001) summarized biogeographic continuity across the North Atlantic Ocean during the Tertiary and pointed out that the bridge was broken by late Eocene. According to the lack of records of the extant or fossil species of Callicarpa from Europe, the route (by the NALB) seems infrequent for this originally tropical genus and it is also believable that the NALB was no longer available at the time for the dispersal of Callicarpa. Likewise, the way via Beringian connections seems unlikely owing to the mostly tropical affinities of Callicarpa (Chen et al., 2020; Li et al., 2020a). To explain this amphi-Pacific tropical and subtropical disjunction occurring in Callicarpa, the ‘Long-distance migration across the Pacific Ocean’ route was speculated herein, combined with our S-DEC inferences. Yang et al. (2018) reported that Kingsboroughia alba (Sabiaceae), with an amphi-Pacific tropical disjunct distribution, migrated from Central America to tropical Asia via long-distance dispersal during the time of the Neogene and Quaternary boundary. Similar transoceanic biogeographical patterns have been found in other plant groups, such as Nettles (Urticeae, Urticaceae) (Huang et al., 2019), Lardizabalaceae (Wang et al., 2020), and polystichoid ferns (Dryopteridaceae) (Le Pechon et al., 2016). In our results, owing to the taxa discovered from Western Pacific Islands and Hawaiian islands, a hypothesis could be proposed that during the Middle-Oligocene the ancestor of C. americana and C. acuminate dispersed for the first time from Southeast Asia to the Neotropics by trans-ocean routes (Figure 6, line 2) and subsequently further migrated northward to temperate North America and southward to South America (Figure 6, line 2), resulting in the amphi-Pacific disjunction pattern of the genus Callicarpa. The achievement of plant migration between North America and South America was also partly related to taking advantage of island stepping-stones (island hopping) and stochastic long-distance dispersals (Gentry, 1982; Bacon et al., 2015). In late Tertiary, the colonization of Callicarpa in Pacific Islands, Temperate North America, and the Neotropics occurred again. Physical connections between affected areas provide inference about the biogeographic history. The uplift of the northern Andes and the rising of volcanic islands may have provided the conditions responsible for the exchange of Callicarpa between Central and South America (Li and Wen, 2013), and these islands eventually coalesced into today’s lower Central America with a substantial land connection across the Isthmus of Panama (Gentry, 1982). The Panama Isthmus as the narrow strip of land connected North and South America and offered a channel for migration in the Pliocene after it closed (Keigwin, 1978). In the New World, 33 species of Callicarpa were recognized, particularly on the Caribbean Islands (24 species currently recognized in Cuba) (Bramley, 2013). Cuba possibly has broadly similar climates and floristic components to Southeast Asia (they are at similar latitudes) (Milne and Abbott, 2002) and Cuba appears to be another center of diversification of Callicarpa. In sum, a trans-Pacific dispersal between two regions is plausible (Wang et al., 2020) to explain the establishment of a New World distribution of Callicarpa, and this hypothesis requires further analysis and testing. Callicarpa successfully reached the Hawaii Islands, other oceanic islands and the Neotropics, to some extent reflecting efficient seed dispersal by birds, usually attracted by small and brightly colorful fruits (especially purple fruits) (Popp et al., 2011). Interestingly, in the progress of tracking the extraordinary migratory journeys and broad-scale habitat use of sooty shearwaters, Shaffer et al. (2006) found that this small seabird can fly right across the Pacific Ocean. Biotic seed dispersal is possibly correlated to large species range sizes and most biotically dispersed species with colorful berries (fleshy fruit) and endozoochorous seeds embedded in juicy pericarp were regarded more suitable for frugivorous birds to spread over long-distance (Loiselle and Blake, 1999; Kessler-Rios and Kattan, 2012; Tan et al., 2020). When the mode of dispersal of Cornus was studied, Lindelof et al. (2020) reported that ‘Island hopping’ was a possible mode of dispersal in bird-dispersed genera (producing fleshy, nutritious fruits). However, what kinds of birds are involved in the spreading of Callicarpa is still unknown, and further studies about dispersal agents and other driving factors are required to gain more insights into the patterns of diversification of Callicarpa.
4.4 Migrations between Southeast Asia and oceanic regions or islands of the Indian ocean
During the early middle-Miocene, population exchanges of Callicarpa among Southeast Asia, Papua New Guinea, and Northern Australia may have occurred (Figure 6, line 4). Between the regions of East and Southeast Asia and Australasia multiple migrations of Callicarpa occurred during different geological periods (the Neogene and Quatemary). The Australian plate started to move northward at the beginning of the Paleogene, ca. 45 Ma and Northern Australia reached the tropics by the beginning of the Miocene, progressively moving northwards each year subsequently (Li and Powell, 2001; Yuan et al., 2005), colliding with the Southeast Asian plate in the middle-Miocene (Lee and Lawver, 1995; Tan et al., 2020), which quite possibly facilitated the flora exchanges among these regions. Furthermore, the onset of the New Guinean highland orogenesis in the late Miocene (Zachos et al., 2001) and putative island chain connections between Asia and Australia during mid to late Miocene (Baldwin et al., 2012; Wu et al., 2019; Li et al., 2020b) made migrations possible. The emergence of the land masses of the eastern Wallace’s Line including Wallacea, Sulawesi, New Guinea, and a series of volcanic islands along the Sunda Arc, the Banda Arc, and the Halmahera Arc connecting these regions from the late Miocene onwards, probably offered a potential channel for dispersals between the two regions (Hall, 2009). Whereafter, the declining sea level during the maximum glacial period (Hantoro et al., 1995; Tan et al., 2020) undoubtedly provided more chances for the exchange of Callicarpa, including its spread westward —the opposite dispersal from northern Australia and the island of New Guinea to Southeast Asia. The last common ancestor of C. macrophylla has experienced one westward dispersal to the Mascarene Islands and Reunion Islands (Figure 6, line 6). Molecular dating frames for the African-Asian disjunction ranged from the Cretaceous (Conti et al., 2002) to the Pleistocene (Li et al., 2009). Our divergence time estimates for Callicarpa appear too young to be explained by Indian rafting (Cretaceous). Researchers have built a strong argument that many tropical taxa could have migrated between Africa and Asia through Arabia (Zhou et al., 2012; Jin et al., 2020). However, this overland migration does not seem likely because there are no records of extant species of Callicarpa from Arabia. The best candidate for explaining the dispersal from Southeast Asia to Madagascar might be through transoceanic long distance dispersal (Les et al., 2003; Yuan et al., 2005; Yao et al., 2016).
As a consequence, this diversity is not evenly distributed and fairly closely follows the distribution of certain topographic and climatic conditions. Major biogeographic lines were crossed frequently and multiple past colonization events had left traces.
5 Conclusions
Our phylogenetic results indicated that Callicarpa is monophyletic with respect to the groups considered, and eight primary clades were well supported. We supported a two-species treatment of certain synonyms, regarding C. poilanei as the synonym of C. angustifolia and C. formosana as the synonym of C. pedunculata. Our biogeographic analyses suggested that the probable ancestor of the Callicarpa crown clade originated in the tropical regions of East Asia and Southeast Asia around the Late-Eocene. The early diversification of a Callicarpa ancestor occurred at 30.86 Ma and during that period this genus may have undergone an eastward dispersal from Asia to Pacific Islands by trans-ocean long-distance dispersal. Subsequently, there could be dispersal, vicariance, and extinction events resulting in the split between the Old World Callicarpa clade and the New World Callicarpa clade. Subsequently, Asian summer monsoons probably contributed to the diversification of the Callicarpa lineage within Asia. Around the early middle-Miocene, the onset of the New Guinean highland orogenesis and putative island chain connections made migrations to Oceania possible. The dispersal from Southeast Asia to Madagascar might have been achieved through transoceanic long distance dispersal.
Data availability statement
The datasets presented in this study can be found in online repositories. The names of the repository/repositories and accession number(s) can be found in the article/Supplementary Material.
Author contributions
ZM and HC designed the study. HC analyzed the data and wrote the manuscript. XL collected the geographical data of species and WW performed the experiments. ZM, BL and GB collected plant material and provided valuable advices for the manuscript. All authors revised the manuscript and approved the submitted version.
Funding
This work was funded by National Natural Science Foundation of China under Grant [31760045, 31970220 and 32260047] and Natural Science Foundation of Guangxi Province under Grant [2018GXNSFAA281132], and Foundation of Guangxi Key Laboratory of Sugarcane Biology [GXKLSCB-202004].
Acknowledgments
We immensely appreciated the precious samples from DZ (South China Botanical Garden, CAS, China) and GB (The Royal Botanic Gardens, Kew, UK) and advices for the manuscript. We are also incredibly grateful to Pengcheng Fu (Luoyang Normal University, China) and Yongjia Zhan (Capital Normal University, China) for guiding and providing valuable advices for data analysis.
Conflict of interest
The authors declare that the research was conducted in the absence of any commercial or financial relationships that could be construed as a potential conflict of interest.
Publisher’s note
All claims expressed in this article are solely those of the authors and do not necessarily represent those of their affiliated organizations, or those of the publisher, the editors and the reviewers. Any product that may be evaluated in this article, or claim that may be made by its manufacturer, is not guaranteed or endorsed by the publisher.
Supplementary material
The Supplementary Material for this article can be found online at: https://www.frontiersin.org/articles/10.3389/fpls.2023.1133157/full#supplementary-material
References
An, Z. S., Porter, S. C., Kutzbach, J. E., Wu, X. H., Wang, S. M., Liu, X. D., et al. (2000). Asynchronous Holocene optimum of the East Asian monsoon. Quaternary Sci. Rev. 19, 743–762. doi: 10.1016/S0277-3791(99)00031-1
Andersson, S. (2006). On the phylogeny of the genus Calceolaria (Calceolariaceae) as inferred from ITS and plastid matK sequences. Taxon 55, 125–137. doi: 10.2307/25065534
Bacon, C. D., Silvestro, D., Jaramillo, C., Smith, B. T., Chakrabarty, P., Antonelli, A. (2015). Biological evidence supports an early and complex emergence of the isthmus of Panama. Proc. Natl. Acad. Sci. United States America 112, E3631–E3631. doi: 10.1073/pnas.1423853112
Baldwin, S. L., Fitzgerald, P. G., Webb, L. E. (2012). Tectonics of the new Guinea region. Annu. Rev. Earth Planetary Sci. 40, 495–520. doi: 10.1146/annurev-earth-040809-152540
Baldwin, B. G., Markos, S. (1998). Phylogenetic utility of the external transcribed spacer (ETS) of 18S-26S rDNA: congruence of ETS and ITS trees of Calycadenia (Compositae). Mol. Phylogenet. Evol. 10, 449–463. doi: 10.1006/mpev.1998.0545
Beardsley, P. M., Olmstead, R. (2002). Redefining phrymaceae: the placement of Mimulus, tribe mimuleae, and Phryma. Am. J. Bot. 89, 1093–1102. doi: 10.3732/ajb.89.7.1093
Bouckaert, R., Heled, J., Kuhnert, D., Vaughan, T., Wu, C. H., Xie, D., et al. (2014). BEAST 2: a software platform for bayesian evolutionary analysis. PloS Comput. Biol. 10, 6. doi: 10.1371/journal.pcbi.1003537
Bramley, G. L. C. (2009). The genus Callicarpa (Lamiaceae) on Borneo. Botanical J. Linn. Soc. 159, 416–455. doi: 10.1111/j.1095-8339.2009.00907.x
Bramley, G. L. C. (2011). Distribution patterns in malesian Callicarpa (Lamiaceae). Gardens' Bull. Singapore 63, 287–298. Available at: http://biostor.org/reference/140290
Bramley, G. L. C. (2013). The genus Callicarpa (Lamiaceae) in the Philippines. Kew Bull. 68, 369–418. doi: 10.1007/s12225-013-9456-y
Buerki, S., Forest, F., Alvarez, N. (2014). Proto-South-East Asia as a trigger of early angiosperm diversification. Botanical J. Linn. Soc. 174, 326–333. doi: 10.1111/boj.12129
Chanderbali, A. S., van der Werff, H., Renner, S. S. (2001). Phylogeny and historical biogeography of lauraceae: evidence from the chloroplast and nuclear genomes. Ann. Missouri Botanical Garden 88, 104–134. doi: 10.2307/2666133
Chen, X. H., Xiang, K. L., Lian, L., Peng, H. W., Erst, A. S., Xiang, X. G., et al. (2020). Biogeographic diversification of Mahonia (Berberidaceae): implications for the origin and evolution of East Asian subtropical evergreen broadleaved forests. Mol. Phylogenet. Evol. 151, 11. doi: 10.1016/j.ympev.2020.106910
Conti, E., Eriksson, T., Schonenberger, J., Sytsma, K. J., Baum, D. A. (2002). Early tertiary out-of-India dispersal of crypteroniaceae: evidence from phylogeny and molecular dating. Evolution 56, 1931–1942. doi: 10.1111/j.0014-3820.2002.tb00119.x
Demesure, B., Sodzi, N., Petit, R. J. (1995). A set of universal primers for amplification of polymorphic non-coding regions of mitochondrial and chloroplast DNA in plants. Mol. Ecol. 4, 129–134. doi: 10.1111/j.1365-294X.1995.tb00201.x
Dong, W., Liu, J., Yu, J., Wang, L., Zhou, S. (2012). Highly variable chloroplast markers for evaluating plant phylogeny at low taxonomic levels and for DNA barcoding. PloS One 7, e35071. doi: 10.1371/journal.pone.0035071
Donoghue, M. J., Smith, S. A. (2004). Patterns in the assembly of temperate forests around the northern hemisphere. Philos. Trans. R. Soc. B-Biological Sci. 359, 1633–1644. doi: 10.1098/rstb.2004.1538
Doyle, J. J., Doyle, J. L. (1987). A rapid DNA isolation procedure for small quantities of fresh leaf tissue. Phytochemical Bull. 19, 11–15.
Drew, B. T., Sytsma, K. J. (2012). Phylogenetics, biogeography and staminal evolution in the tribe mentheae (Lamiaceae). Am. J. Bot. 99, 933–953. doi: 10.3732/ajb.1100549
Fritsch, P. W., Manchester, S. R., Stone, R. D., Cruz, B. C., Almeda, F. (2015). Northern hemisphere origins of the amphi-pacific tropical plant family symplocaceae. J. Biogeography 42, 891–901. doi: 10.1111/jbi.12442
Gao, J., Yu, T., Li, J. (2020). Phylogenetic and biogeographic study of Acer (Sapindaceae) based on three chloroplast DNA fragment sequences. Acta Ecologica Sin. 40, 5992–6000. doi: 10.1038/s41598-020-78145-0
Gaynor, M. L., Fu, C. N., Gao, L. M., Lu, L. M., Soltis, D. E., Soltis, P. S. (2020). Biogeography and ecological niche evolution in diapensiaceae inferred from phylogenetic analysis. J. Systematics Evol. 58, 646–662. doi: 10.1111/jse.12646
Gentry, A. H. (1982). Neotropical Floristic diversity: phytogeographical connections between central and south America, pleistocene climatic fluctuations, or an accident of the Andean orogeny? Ann. Missouri Botanical garden 69, 557–593. doi: 10.2307/2399084
Gunasekara, N. (2004). Phylogenetic and molecular dating analyses of the tropical tree family dipterocarpaceae based on chloroplast matK nucleotide sequence data. Masters thesis. (Montreal: Concordia University).
Guo, Y. L., Ge, S. (2005). Molecular phylogeny of oryzeae (Poaceae) based on DNA sequences from chloroplast, mitochondrial, and nuclear genomes. Am. J. Bot. 92, 1548–1558. doi: 10.3732/ajb.92.9.1548
Guo, Z. T., Ruddiman, W. F., Hao, Q. Z., Wu, H. B., Qiao, Y. S., Zhu, R. X., et al. (2002). Onset of Asian desertification by 22 myr ago inferred from loess deposits in China. Nature 416, 159–163. doi: 10.1038/416159a
Hall, T. A. (1999). BioEdit: a user-friendly biological sequence alignment editor and analysis program for windows 95/98/NT. Nucleic Acids Symposium Ser. 41, 95–98.
Hall, R. (2009). Southeast asia's changing palaeogeography. Blumea 54, 148–161. doi: 10.3767/000651909X475941
Hantoro, W., Faure, H., Djuwansah, R., Faure-Denard, L., Pirazzoli, P. J. (1995). The sunda and sahul continental platform: lost land of the last glacial continent in SE Asia. Quaternary Int. 29, 129–134. doi: 10.1016/1040-6182(95)00015-B
Higgins, S. I., Nathan, R., Cain, M. L. (2003). Are long-distance dispersal events in plants usually caused by nonstandard means of dispersal? Ecology 84, 1945–1956. doi: 10.1890/01-0616
Huang, X. H., Deng, T., Moore, M. J., Wang, H. C., Li, Z. M., Lin, N., et al. (2019). Tropical Asian origin, boreotropical migration and long-distance dispersal in nettles (Urticeae, urticaceae). Mol. Phylogenet. Evol. 137, 190–199. doi: 10.1016/j.ympev.2019.05.007
Ian, M. R. (2006). Northern hemisphere plant disjunctions: a window on tertiary land bridges and climate change? Ann. Bot. 98, 465–472. doi: 10.1093/aob/mcl148
Ji, Y. H., Yang, L. F., Chase, M. W., Liu, C. K., Yang, Z. Y., Yang, J., et al. (2019). Plastome phylogenomics, biogeography, and clade diversification of Paris (Melanthiaceae). BMC Plant Biol. 19, 14. doi: 10.1186/s12870-019-2147-6
Jiang, C., Tan, K., Ren, M. (2017). Effects of monsoon on distribution patterns of tropical plants in Asia. Chin. J. Plant Ecol. 41, 1103–1112. doi: 10.17521/cjpe.2017.0070
Jin, J. J., Yang, M. Q., Fritsch, P. W., Van Velzen, R., Li, D. Z., Yi, T. S. (2020). Born migrators: historical biogeography of the cosmopolitan family cannabaceae. J. Systematics Evol. 58, 461–473. doi: 10.1111/jse.12552
Kalyaanamoorthy, S., Minh, B. Q., Wong, T. K. F., Von Haeseler, A., Jermiin, L. S. (2017). ModelFinder: fast model selection for accurate phylogenetic estimates. Nat. Methods 14, 587–589. doi: 10.1038/nmeth.4285
Kar, R. (1996). On the Indian origin of Ocimum (Lamiaceae): a palynological approach. Palaeobotanist 43, 43–50.
Kato, M. (2000). Anthophilous insect community and plant-pollinator interactions on amami islands in the Ryukyu archipelago, Japan. Kyoto Univ. 29, 157–254. Available at: http://hdl.handle.net/2433/156116
Kato, M., Shibata, A., Yasui, T., Nagamasu, H. (1999). Impact of introduced honeybees, Apis mellifera, upon native bee communities in the bonin (Ogasawara) islands. Res. Population Ecol. 41, 217–228. doi: 10.1007/s101440050025
Katoh, K., Standley, D. M. (2013). MAFFT multiple sequence alignment software version 7: improvements in performance and usability. Mol. Biol. Evol. 30, 772–780. doi: 10.1093/molbev/mst010
Kawakubo, N. (1990). Dioecism of the genus Callicarpa (Verbenaceae) in the bonin (Ogasawara) islands. botanical magazine= Shokubutsu-gaku-zasshi 103, 57–66. doi: 10.1007/BF02488411
Keigwin, L. D. (1978). Pliocene closing of the isthmus of Panama, based on biostratigraphic evidence from nearby pacific ocean and Caribbean Sea cores. Geology 6, 630–634. doi: 10.1130/0091-7613(1978)6<630:PCOTIO>2.0.CO;2
Kessler-Rios, M. M., Kattan, G. H. (2012). Fruits of melastomataceae: phenology in Andean forest and role as a food resource for birds. J. Trop. Ecol. 28, 11–21. doi: 10.1017/S0266467411000642
Kumar, S., Stecher, G., Tamura, K. (2016). MEGA7: molecular evolutionary genetics analysis version 7.0 for bigger datasets. Mol. Biol. Evol. 33, 1870–1874. doi: 10.1093/molbev/msw054
Latham, R. E., Ricklefs, R. E. (1993). Continental comparisons of temperate-zone tree species diversity. Species Diversity Ecol. communities: historical geographical Perspect II, 294–314.
Lavin, M., Luckow, M. (1993). Origins and relationships of tropical north America in the context of the boreotropics hypothesis. Am. J. Bot. 80, 1–14. doi: 10.1002/j.1537-2197.1993.tb13761.x
Lee, T. Y., Lawver, L. A. (1995). Cenozoic Plate reconstruction of southeast Asia. Tectonophysics 251, 85–138. doi: 10.1016/0040-1951(95)00023-2
Leeratiwong, C., Chantaranothai, P., Paton, A. (2007). Notes on the genus Callicarpa (Lamiaceae) in Thailand. Thai For. Bull. 37, 73–79. Available at: https://li01.tci-thaijo.org/index.php/ThaiForestBulletin/article/view/24203
Leeratiwong, C., Chantaranothai, P., Paton, A. J. (2009). A synopsis of the genus Callicarpa L.(Lamiaceae) in Thailand. Thai For. Bull. 35, 36–58. Available at: https://li01.tcithaijo.org/index.php/ThaiForestBulletin/article/view/24334
Le Pechon, T., Zhang, L., He, H., Zhou, X. M., Bytebier, B., Gao, X. F., et al. (2016). A well-sampled phylogenetic analysis of the polystichoid ferns (Dryopteridaceae) suggests a complex biogeographical history involving both boreotropical migrations and recent transoceanic dispersals. Mol. Phylogenet. Evol. 98, 324–336. doi: 10.1016/j.ympev.2016.02.018
Les, D. H., Crawford, D. J., Kimball, R. T., Moody, M. L., Landolt, E. (2003). Biogeography of discontinuously distributed hydrophytes: a molecular appraisal of intercontinental disjunctions. Int. J. Plant Sci. 164, 917–932. doi: 10.1086/378650
Li, B., Cantino, P. D., Olmstead, R. G., Bramley, G. L., Xiang, C. L., Ma, Z. H., et al. (2016). A large-scale chloroplast phylogeny of the lamiaceae sheds new light on its subfamilial classification. Sci. Rep. 6, 34343. doi: 10.1038/srep34343
Li, Y. Q., Dressler, S., Zhang, D. X., Renner, S. S. (2009). More Miocene dispersal between Africa and Asia-the case of Bridelia (Phyllanthaceae). Systematic Bot. 34, 521–529. doi: 10.1600/036364409789271263
Li, Z. Z., Lehtonen, S., Martins, K., Gichira, A. W., Wu, S., Li, W., et al. (2020b). Phylogenomics of the aquatic plant genus Ottelia (Hydrocharitaceae): implications for historical biogeography. Mol. Phylogenet. Evol. 152, 106939. doi: 10.1016/j.ympev.2020.106939
Li, H. W., Liu, B., Davis, C. C., Yang, Y. (2020a). Plastome phylogenomics, systematics, and divergence time estimation of the Beilschmiedia group (Lauraceae). Mol. Phylogenet. Evol. 151, 13. doi: 10.1016/j.ympev.2020.106901
Li, Z. X., Powell, C. (2001). An outline of the palaeogeographic evolution of the Australasian region since the beginning of the neoproterozoic. Earth-Science Rev. 53, 237–277. doi: 10.1016/S0012-8252(00)00021-0
Li, P., Qi, Z. C., Liu, L. X., Ohi-Toma, T., Lee, J., Hsieh, T. H., et al. (2017). Molecular phylogenetics and biogeography of the mint tribe elsholtzieae (Nepetoideae, lamiaceae), with an emphasis on its diversification in East Asia. Sci. Rep. 7, 12. doi: 10.1038/s41598-017-02157-6
Li, R., Wen, J. (2013). Phylogeny and biogeography of Dendropanax (Araliaceae), an amphi-pacific disjunct genus between tropical/subtropical Asia and the neotropics. Systematic Bot. 38, 536–551. doi: 10.1600/036364413X666606
Lian, L., Ortiz, R. D. C., Jabbour, F., Zhang, C. F., Xiang, X. G., Erst, A. S., et al. (2020). Phylogeny and biogeography of pachygoneae (Menispermaceae), with consideration of the boreotropical flora hypothesis and resurrection of the genera Cebatha and Nephroia. Mol. Phylogenet. Evol. 148, 106825. doi: 10.1016/j.ympev.2020.106825
Lindelof, K., Lindo, J. A., Zhou, W. B., Ji, X., Xiang, Q. Y. (2020). Phylogenomics, biogeography, and evolution of the blue- or white-fruited dogwoods (Cornus)-insights into morphological and ecological niche divergence following intercontinental geographic isolation. J. Systematics Evol. 58, 604–645. doi: 10.1111/jse.12676
Liu, J. Q., Wang, Y. J., Wang, A. L., Hideaki, O., Abbott, R. J. (2006). Radiation and diversification within the Ligularia-Cremanthodium-Parasenecio complex (Asteraceae) triggered by uplift of the qinghai-Tibetan plateau. Mol. Phylogenet. Evol. 38, 31–49. doi: 10.1016/j.ympev.2005.09.010
Liu, C., Yang, J., Jin, L., Wang, S., Yang, Z., Ji, Y. (2021). Plastome phylogenomics of the East Asian endemic genus Dobinea. Plant Diversity 43, 35–42. doi: 10.1016/j.pld.2020.05.002
Lohman, D. J., De Bruyn, M., Page, T., Von Rintelen, K., Hal, R., Ng, P. K. L., et al. (2011). Biogeography of the indo-Australian archipelago. Annu. Rev. Ecology Evolution Systematics 42, 205–226. doi: 10.1146/annurev-ecolsys-102710-145001
Loiselle, B. A., Blake, J. G. (1999). Dispersal of melastome seeds by fruit-eating birds of tropical forest understory. Ecology 80, 330–336. doi: 10.1890/0012-9658(1999)080[0330:DOMSBF]2.0.CO;2
Lomolino, M. V. (2010). Four Darwinian themes on the origin, evolution and preservation of island life. J. Biogeography 37, 985–994. doi: 10.1111/j.1365-2699.2009.02247.x
Ma, Z., Bramley, G. L. C., Zhang, D. (2016). Pollen morphology of Callicarpa l. (Lamiaceae) from China and its systematic implications. Plant Systematics Evol. 302, 67–88. doi: 10.1007/s00606-015-1244-8
Ma, W. J., Su, Z. W., Ma, Z. H. (2022). Chemical constituents of Callicarpa integerrima. Guihaia 42, 1–11. doi: 10.11931/guihaia.gxzw202202008
Ma, Z. H., Zhang, D. X. (2012). Callicarpa hainanensis: a new species of Callicarpa from hainan, China. J. Systematics Evol. 50, 573–573. doi: 10.1111/j.1759-6831.2012.00229_1.x
Manos, P. S., Donoghue, M. J. (2001). Progress in northern hemisphere phytogeography: an introduction. Int. J. Plant Sci. 162, S1–S2. doi: 10.1086/324421
Martinez-Millan, M. (2010). Fossil record and age of the asteridae. Botanical Rev. 76, 83–135. doi: 10.1007/s12229-010-9040-1
Miller, M., Pfeiffer, W., Schwartz, T. (2010). Creating the CIPRES science gateway for inference of large phylogenetic trees in proceedings of the gateway. New Orleans: Computing Environments Workshop (GCE) 1–8. doi: 10.1109/GCE.2010.5676129
Milne, R. I., Abbott, R. J. (2002). The origin and evolution of tertiary relict floras. Adv. Botanical Res. 38, 281–314. doi: 10.1016/S0065-2296(02)38033-9
Momose, K., Yumoto, T., Nagamitsu, T., Kato, M., Nagamasu, H., Sakai, S., et al. (1998). Pollination biology in a lowland dipterocarp forest in Sarawak, Malaysia. I. Characteristics of the plant-pollinator community in a lowland dipterocarp forest. Am. J. Bot. 85, 1477–1501. doi: 10.2307/2446404
Myers, N., Mittermeier, R. A., Mittermeier, C. G., Da Fonseca, G., Kent, J. (2000). Biodiversity hotspots for conservation priorities. Nature 403, 853–858. doi: 10.1038/35002501
Nguyen, L. T., Schmidt, H. A., von Haeseler, A., Minh, B. Q. (2015). IQ-TREE: a fast and effective stochastic algorithm for estimating maximum-likelihood phylogenies. Mol. Biol. Evol. 32, 268–274. doi: 10.1093/molbev/msu300
Paradis, E., Claude, J., Strimmer, K. (2004). APE: analyses of phylogenetics and evolution in r language. Bioinformatics 20, 289–290. doi: 10.1093/bioinformatics/btg412
Popp, M., Mirre, V., Brochmann, C. (2011). A single mid-pleistocene long-distance dispersal by a bird can explain the extreme bipolar disjunction in crowberries (Empetrum). Proc. Natl. Acad. Sci. United States America 108, 6520–6525. doi: 10.1073/pnas.1012249108
Qian, H., Ricklefs, R. E. (2000). Large-Scale processes and the Asian bias in species diversity of temperate plants. Nature 407, 180–182. doi: 10.1038/35025052
Qiu, Y. X., Fu, C. X., Comes, H. P. (2011). Plant molecular phylogeography in China and adjacent regions: tracing the genetic imprints of quaternary climate and environmental change in the world's most diverse temperate flora. Mol. Phylogenet. Evol. 59, 225–244. doi: 10.1016/j.ympev.2011.01.012
Rambaut, A., Drummond, A. J., Xie, D., Baele, G., Suchard, M. A. (2018). Posterior summarization in bayesian phylogenetics using tracer 1.7. Systematic Biol. 67, 901. doi: 10.1093/sysbio/syy032
Ronquist, F., Teslenko, M., van der Mark, P., Ayres, D. L., Darling, A., Hohna, S., et al. (2012). MrBayes 3.2: efficient bayesian phylogenetic inference and model choice across a large model space. Systematic Biol. 61, 539–542. doi: 10.1093/sysbio/sys029
Royden, L. H., Burchfiel, B. C., van der Hilst, R. D. (2008). The geological evolution of the Tibetan plateau. Science 321, 1054–1058. doi: 10.1126/science.1155371
Sang, T., Crawford, D. J., Stuessy, T. F. (1997). Chloroplast DNA phylogeny, reticulate evolution, and biogeography of Paeonia (Paeoniaceae). Am. J. Bot. 84, 1120–1136. doi: 10.2307/2446155
Santosh, M., Senshu, H. (2011). History of supercontinents and its relation to the origin of Japanese islands. J. geography-chigaku zasshi 120, 100–114. doi: 10.5026/jgeography.120.100
Shaffer, S. A., Tremblay, Y., Weimerskirch, H., Scott, D., Thompson, D. R., Sagar, P. M., et al. (2006). Migratory shearwaters integrate oceanic resources across the pacific ocean in an endless summer. Proc. Natl. Acad. Sci. United States America 103, 12799–12802. doi: 10.1073/pnas.060371510
Shaw, J., Lickey, E. B., Beck, J. T., Farmer, S. B., Liu, W. S., Miller, J., et al. (2005). The tortoise and the hare II: relative utility of 21 noncoding chloroplast DNA sequences for phylogenetic analysis. Am. J. Bot. 92, 142–166. doi: 10.3732/ajb.92.1.142
Shaw, J., Lickey, E. B., Schilling, E. E., Small, R. L. (2007). Comparison of whole chloroplast genome sequences to choose noncoding regions for phylogenetic studies in angiosperms: the tortoise and the hare III. Am. J. Bot. 94, 275–288. doi: 10.3732/ajb.94.3.275
Small, R. L., Cronn, R. C., Wendel, J. F. (2004). Use of nuclear genes for phylogeny reconstruction in plants. Aust. Systematic Bot. 17, 145–170. doi: 10.1071/SB03015
Smith, A. B., Peterson, K. J. (2002). Dating the time of origin of major clades: molecular clocks and the fossil record. Annu. Rev. Earth Planetary Sci. 30, 65–88. doi: 10.1146/annurev.earth.30.091201.140057
Sun, Y., Skinner, D. Z., Liang, G. H., Hulbert, S. H. (1994). Phylogenetic analysis of Sorghum and related taxa using internal transcribed spacers of nuclear ribosomal DNA. Theor. Appl. Genet. 89, 26–32. doi: 10.1007/BF00226978
Sun, X., Wang, P. (2005). How old is the Asian monsoon system? —palaeobotanical records from China. Palaeogeography Palaeoclimatology Palaeoecol. 222, 181–222. doi: 10.1016/j.palaeo.2005.03.005
Tan, K., Malabrigo Pastor, L., Ren, M. (2020). Origin and evolution of biodiversity hotspots in southeast Asia. Acta Ecologica Sin. 40, 3866–3877. doi: 10.5846/stxb201904160762
Tate, J. A., Simpson, B. B. (2003). Paraphyly of Tarasa (Malvaceae) and diverse origins of the polyploid species. Systematic Bot. 28, 723–737. doi: 10.1043/02-64.1
Thomas, D. C., Hughes, M., Phutthai, T., Ardi, W. H., Rajbhandary, S., Rubite, R., et al. (2012). West To east dispersal and subsequent rapid diversification of the mega-diverse genus Begonia (Begoniaceae) in the malesian archipelago. J. Biogeography 39, 98–113. doi: 10.1111/j.1365-2699.2011.02596.x
Tiffney, B. (1985). The Eocene north Atlantic land bridge: its importance in tertiary and modern phytogeography of the northern hemisphere. J. Arnold Arbor. 66, 243–273. doi: 10.5962/bhl.part.13183
Tiffney, B. H., Manchester, S. R. (2001). The use of geological and paleontological evidence in evaluating plant phylogeographic hypotheses in the northern hemisphere tertiary. Int. J. Plant Sci. 162, S3–S17. doi: 10.1086/323880
Toussaint, E. F. A., Hendrich, L., Hajek, J., Michat, M. C., Panjaitan, R., Short, A. E. Z., et al. (2017). Evolution of pacific rim diving beetles sheds light on amphi-pacific biogeography. Ecography 40, 500–510. doi: 10.1111/ecog.02195
Tsukaya, H., Fukuda, T., Yokoyama, J. (2003). Hybridization and introgression between Callicarpa japonica and C. mollis (Verbenaceae) in central Japan, as inferred from nuclear and chloroplast DNA sequences. Mol. Ecol. 12, 3003–3011. doi: 10.1046/j.1365-294X.2003.01961.x
Tu, Y. H., Sun, L. N., Guo, M. L., Chen, W. S. (2013). The medicinal uses of Callicarpa l. @ in traditional Chinese medicine: an ethnopharmacological, phytochemical and pharmacological review. J. Ethnopharmacology 146, 465–481. doi: 10.1016/j.jep.2012.12.051
Van Damme, K., Sinev, A. Y. (2013). Tropical amphi-pacific disjunctions in the cladocera (Crustacea: branchiopoda). J. Limnology 72, 209–244. doi: 10.4081/jlimnol.2013.s2.e11
Van Der Pijl, L. (1982). “Principles of dispersal in higher plants,” in Springer, vol. 214. (Berlin: Verlag).
Van Welzen, P. C., Parnell, J., Slik, J. W. F. (2011). Wallace's line and plant distributions: two or three phytogeographical areas and where to group Java? Biol. J. Linn. Soc. 103, 531–545. doi: 10.1111/j.1095-8312.2011.01647.x
Walker, J., Geissman, J., Bowring, S., Babcock, L. (2018). Geologic time scale v. 5.0. Geological Soc. America. Available at: https://www.geosociety.org/.
Wang, Z. (2017). Geological features, the formation and the evolution of the qinghai-Tibetan plateau. Sci. Technol. Rev. 35, 51–58. doi: 10.3981/j.issn.1000-7857.2017.06.005
Wang, W., Xiang, X. G., Xiang, K. L., Ortiz, R. D. C., Jabbour, F., Chen, Z. D. (2020). A dated phylogeny of lardizabalaceae reveals an unusual long-distance dispersal across the pacific ocean and the rapid rise of East Asian subtropical evergreen broadleaved forests in the late Miocene. Cladistics 36, 447–457. doi: 10.1111/cla.12414
Webster, P. J., Magana, V. O., Palmer, T. N., Shukla, J., Tomas, R. A., Yanai, M., et al. (1998). Monsoons: processes, predictability, and the prospects for prediction. J. Geophysical Research-Oceans 103, 14451–14510. doi: 10.1029/97JC02719
Wei, R., Xiang, Q. P., Schneider, H., Sundue, M. A., Kessler, M., Kamau, P. W., et al. (2015). Eurasian Origin, boreotropical migration and transoceanic dispersal in the pantropical fern genus Diplazium (Athyriaceae). J. Biogeography 42, 1809–1819. doi: 10.1111/jbi.12551
Wen, J. (1999). Evolution of eastern Asian and eastern north American disjunct distributions in flowering plants. Annu. Rev. Ecol. Systematics 30, 421–455. doi: 10.1146/annurev.ecolsys.30.1.421
Wen, J. (2001). Evolution of eastern Asian-Eastern north American biogeographic disjunctions: a few additional issues. Int. J. Plant Sci. 162, S117–S122. doi: 10.1086/322940
Wen, J., Nie, Z. L., Ickert-Bond, S. M. (2016). Intercontinental disjunctions between eastern Asia and western north America in vascular plants highlight the biogeographic importance of the Bering land bridge from late Cretaceous to neogene. J. Systematics Evol. 54, 469–490. doi: 10.1111/jse.12222
White, T. J., Bruns, T., Lee, S., Talyor, J. (1990). Amplification and direct sequencing of fungal ribosomal RNA genes for phylogenetics. PCR protocols: guide to Methods Appl. 18, 315–322. doi: 10.1016/B978-0-12-372180-8.50042-1
Wolfe, J. (1975). Some aspects of plant geography of the northern hemisphere during the late Cretaceous and tertiary. Ann. Missouri Botanical Garden 62, 264–279. doi: 10.2307/2395198
Wu, Z., Chen, J., Xu, F., Wang, H. (2018). Research progress on Callicarpa plants. J. Guangdong Pharm. Univ. 34, 808–813. doi: 10.16809/j.cnki.2096-3653.2018102101
Wu, X. K., Liu, X. Y., Kodrul, T., Quan, C., Jin, J. H. (2019). Dacrycarpus pattern shedding new light on the early floristic exchange between Asia and Australia. Natl. Sci. Rev. 6, 1086–1090. doi: 10.1093/nsr/nwz060
Wu, S., Wu, Z. (1996). A proposal for a new floristic kingdom (realm): the Asiatic kingdom its delineationand characteristics. Floristic Characteristics, 3–42.
Xu, L., Chen, F., Xie, Y. (2013). Cross-breeding between two species of Callicarpa. J. Huazhong Agric. Univ. 32, 23–27. doi: 10.3969/j.issn.1000-2421.2013.04.005
Yang, Y., Li, Z. Y., Shao, J. J., Wang, G., Wen, R., Tian, J. Z., et al. (2021). Callicarpa nudiflora hook. & am.: a comprehensive review of its phytochemistry and pharmacology. J. Ethnopharmacology 264, 113123. doi: 10.1016/j.jep.2020.113123
Yang, M. Q., Li, D. Z., Wen, J., Yi, T. S. (2017). Phylogeny and biogeography of the amphi-pacific genus Aphananthe. PloS One 12, e0171405. doi: 10.1371/journal.pone.0171405
Yang, T., Lu, L. M., Wang, W., Li, J. H., Manchester, S. R., Wen, J., et al. (2018). Boreotropical range expansion and long-distance dispersal explain two amphi-pacific tropical disjunctions in sabiaceae. Mol. Phylogenet. Evol. 124, 181–191. doi: 10.1016/j.ympev.2018.03.005
Yao, G., Drew, B. T., Yi, T. S., Yan, H. F., Yuan, Y. M., Ge, X. J. (2016). Phylogenetic relationships, character evolution and biogeographic diversification of Pogostemon s.l. (Lamiaceae). Mol. Phylogenet. Evol. 98, 184–200. doi: 10.1016/j.ympev.2016.01.020
Yu, Y., Blair, C., He, X. (2020). RASP 4: ancestral state reconstruction tool for multiple genes and characters. Mol. Biol. Evol. 37, 604–606. doi: 10.1093/molbev/msz257
Yu, Y., Harris, A. J., Blair, C., He, X. J. (2015). RASP (Reconstruct ancestral state in phylogenies): a tool for historical biogeography. Mol. Phylogenet. Evol. 87, 46–49. doi: 10.1016/j.ympev.2015.03.008
Yu, X. Q., Maki, M., Drew, B. T., Paton, A. J., Li, H. W., Zhao, J. L., et al. (2014). Phylogeny and historical biogeography of Isodon (Lamiaceae): rapid radiation in south-west China and Miocene overland dispersal into Africa. Mol. Phylogenet. Evol. 77, 183–194. doi: 10.1016/j.ympev.2014.04.017
Yuan, Y. M., Wohlhauser, S., Möller, M., Klackenberg, J., Callmander, M. W., Küpfer, P. (2005). Phylogeny and biogeography of Exacum (Gentianaceae): a disjunctive distribution in the Indian ocean basin resulted from long distance dispersal and extensive radiation. Syst. Biol. 54, 21–34. doi: 10.1080/10635150590905867
Zachos, J., Pagani, M., Sloan, L., Thomas, E., Billups, K. (2001). Trends, rhythms, and aberrations in global climate 65 ma to present. Science 292, 686–693. doi: 10.1126/science.1059412
Zhang, D., Gao, F. L., Jakovlic, I., Zou, H., Zhang, J., Li, W. X., et al. (2020a). PhyloSuite: an integrated and scalable desktop platform for streamlined molecular sequence data management and evolutionary phylogenetics studies. Mol. Ecol. Resour. 20, 348–355. doi: 10.1111/1755-0998.13096
Zhang, M. H., Wang, C. Y., Zhang, C., Zhang, D. G., Li, K. G., Nie, Z. L., et al. (2020b). Phylogenetic relationships and biogeographic history of the unique Saxifraga sect. Irregulares (Saxifragaceae) from eastern Asia. J. Systematics Evol. 58, 958–971. doi: 10.1111/jse.12547
Zhou, L. L., Su, Y. C. F., Thomas, D. C., Saunders, R. M. K. (2012). Out-of-Africa' dispersal of tropical floras during the Miocene climatic optimum: evidence from Uvaria (Annonaceae). J. Biogeography 39, 322–335. doi: 10.1111/j.1365-2699.2011.02598.x
Keywords: Callicarpa, phylogeny, historical biogeography, amphi-Pacific tropical disjunction, long-distance dispersal
Citation: Cai H, Liu X, Wang W, Ma Z, Li B, Bramley GLC and Zhang D (2023) Phylogenetic relationships and biogeography of Asia Callicarpa (Lamiaceae), with consideration of a long-distance dispersal across the Pacific Ocean —insights into divergence modes of pantropical flora. Front. Plant Sci. 14:1133157. doi: 10.3389/fpls.2023.1133157
Received: 28 December 2022; Accepted: 20 April 2023;
Published: 15 May 2023.
Edited by:
Roman A. Volkov, Chernivtsi University, UkraineReviewed by:
Wen-Bin Yu, Chinese Academy of Sciences (CAS), ChinaFloris C. Breman, Wageningen University and Research, Netherlands
Copyright © 2023 Cai, Liu, Wang, Ma, Li, Bramley and Zhang. This is an open-access article distributed under the terms of the Creative Commons Attribution License (CC BY). The use, distribution or reproduction in other forums is permitted, provided the original author(s) and the copyright owner(s) are credited and that the original publication in this journal is cited, in accordance with accepted academic practice. No use, distribution or reproduction is permitted which does not comply with these terms.
*Correspondence: Zhonghui Ma, bWF6aG9uZ2h1aUBneHUuZWR1LmNu