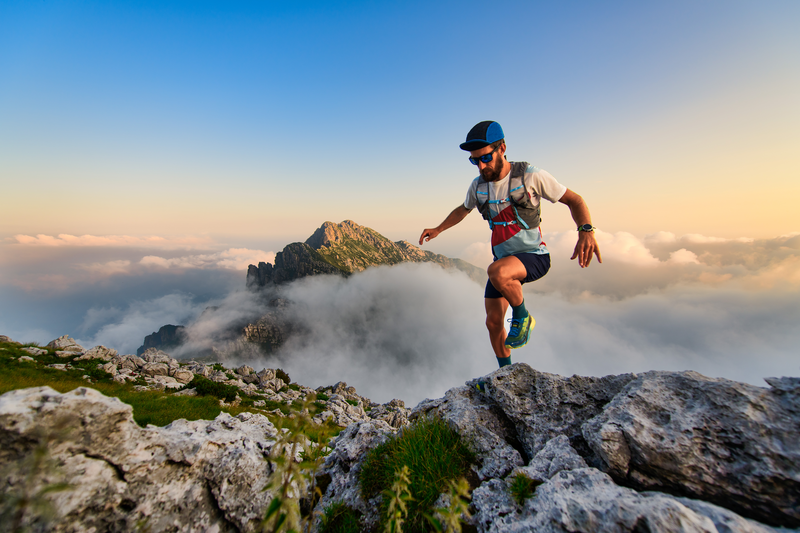
94% of researchers rate our articles as excellent or good
Learn more about the work of our research integrity team to safeguard the quality of each article we publish.
Find out more
ORIGINAL RESEARCH article
Front. Plant Sci. , 10 May 2023
Sec. Plant Breeding
Volume 14 - 2023 | https://doi.org/10.3389/fpls.2023.1128926
Bitter gourd is an economically important vegetable and medicinal crop distinguished by its bitter fruits. Its stigma color is widely used to assess the distinctiveness, uniformity, and stability of bitter gourd varieties. However, limited researches have been dedicated to genetic basis of its stigma color. In this study, we employed bulked segregant analysis (BSA) sequencing to identify a single dominant locus McSTC1 located on pseudochromosome 6 through genetic mapping of an F2 population (n =241) derived from the cross between green and yellow stigma parental lines. An F2-derived F3 segregation population (n = 847) was further adopted for fine mapping, which delimited the McSTC1 locus to a 13.87 kb region containing one predicted gene McAPRR2 (Mc06g1638), a homolog of the Arabidopsis two-component response regulator-like gene AtAPRR2. Sequence alignment analysis of McAPRR2 revealed that a 15 bp insertion at exon 9 results in a truncated GLK domain of its encoded protein, which existed in 19 bitter gourd varieties with yellow stigma. A genome-wide synteny search of the bitter gourd McAPRR2 genes in Cucurbitaceae family revealed its close relationship with other cucurbits APRR2 genes that are corresponding to white or light green fruit skin. Our findings provide insights into the molecular marker-assisted breeding of bitter gourd stigma color and the mechanism of gene regulation for stigma color.
Flower color, especially petal color, is the most noticeable variation of floral features in angiosperms with colors spanning the whole color spectrum perceived by human and pollinators. There is general agreement that the diversity of flower colors in angiosperms is mostly determined by variation in the interactions with pollinators via natural selection, in part because pollinators tend to enhance assortative mating (Gervasi and Schiestl, 2017; Trunschke et al., 2021). In addition to the most visible petal color, other types of flower colors include pollen, gynoecium and inflorescence colors polymorphisms (Carlson and Holsinger, 2010; Koski et al., 2020). The color of stigma may also differ across plant varieties and be distinguishable from the petal background to attract pollinators, such as tulip, melon, and sunflower, which may further enhance the crop production (Miklič et al., 2002; Miller et al., 2011; Lv et al., 2022b). In another instance, the stigma of pistachio (Pistacia vera L.) bears exclusive colors at various developmental stages, which has been utilized as an indicator for the optimum time of artificial pollination (Sharifkhah et al., 2020). Botanically, stigma color is an essential morphological characteristic for differentiating the species or types of plants. The green stigma, for instance, is one of the phenotypic traits used to differentiate the prickly pear cactus Opuntia Bonaerensis from its close relative Opuntia elata (Köhler et al., 2020).
At present, few research have reported the genetic basis of stigma color. Studies on pecan (Carya illinoinensis) and tomato (Solanum lycopersicum) suggest that the green stigma is regulated by a single dominant gene with their green stigma dominating over red and yellow stigma, respectively (Beedanagari et al., 2005; Zhao et al., 2017). In contrast, studies for stigma color for melon (Cucumis melo) and rice (Oryza sativa) propose polygenic model (Meng et al., 2021; Qiao et al., 2021; Lv et al., 2022b). In melon, two quantitative trait loci (QTL) SC2.1 and SC8.1 on chromosomes (Chr) 2 and Chr 8, have been mapped for regulating the green stigma, however their underlying causative genes are remain unclear (Qiao et al., 2021; Lv et al., 2022b). In a rice indica cultivar, its purple apiculi and stigma were genetic regulated by the interaction of two genes involved the anthocyanin biosynthesis pathway: OsC1 (encoding a R2R3-MYB transcription factor) and OsDFR (encoding a dihyroflavonol 4-reductase) (Meng et al., 2021). Recent two transcriptomic studies aimed at revealing the gene network were conducted and showed that the yellow stigma in tomato is very likely due to the accumulation of yellow-colored flavonoid naringenin chalcone, whereas the melon yellow stigma is due to break-down of chloroplast structure interfering chlorophyll biosynthesis (Zhang et al., 2017; Lv et al., 2022a). Despite the crucial functional roles of stigmas play in plant reproduction, there has not been sufficient researches dedicated to the color of stigma.
Bitter gourd (syn. bitter melon; Momordica charantia L.; 2x=2n=22), belongs to the Cucurbitaceae family and the Momordica genus, is an economically important vegetable and medicinal crop marked by its bitter fruits. Bitter gourd is abundantly cultivated in the tropical and subtropical countries or regions, including China, India, Malaysia, Africa, and South America. As the next-generation long-read sequencing method has recently been applied to bitter gourd, the high-quality whole genome assemblies are now available for both bitter gourd cultivars (OHB3-1 and Dali-11) and wild accession (TR), which greatly facilitate the process of addressing fundamental issues and advancing bitter gourd breeding (Urasaki et al., 2017; Cui et al., 2020; Matsumura et al., 2020). In addition, it provides intriguing potential for re-investigating those previously documented horticultural traits. For example, the underlying gene of the black seed coat color of bitter gourd was pinpointed to a 13.2 kb region containing only one candidate gene, MC03g0810, encoding a polyphenol oxidase (PPO) (Kole et al., 2012; Zhong et al., 2022). Other quantitative traits, such as fruit size and yield, were also thoroughly investigated by applying high-density genetic map (Rao et al., 2021).
Similar to other cucurbits, bitter gourd is monoecious with male and female flowers produced on the same plant. In female flowers, the stigmas often present two colors: yellow and green. This characteristic is widely used to assess the distinctiveness, uniformity, and stability of a bitter gourd variety (UPOV: TG/235/1,Guidelines for the conduct of tests for distinctness,uniformity and stability—Bitter gourd, NEQ). The green stigma is easily distinguished from the background of yellow petals of bitter gourd which is suggested be more attractive to the pollinators (such as bees and flies) (Miller et al., 2011; Lv et al., 2022b). The first genetic study for stigma color of bitter gourd was conducted used an F2 population derived from a cross between “Taiwan White” and “CBM12” which had distinct fruit, stigma, and seed colors (Kole et al., 2012). According to Kole et al. (2012), stigma color is regulated by a single dominant locus that is independently inherited and was not found to be related with other fruit traits (e.g., fruit color, fruit surface structure, seed color) (Kole et al., 2012). However, the fine mapping and cloning of the stigma color gene in bitter gourd have not yet been accomplished.
In the present study, we developed a mapping population with 241 F2 plants from the cross of two bitter gourd inbred lines S051 (green stigma) and S093 (yellow stigma), and further applied the bulked-segregant analysis sequencing (BSA-seq) method to identify the inheritance locus/loci. Through map-based cloning using an 847 F3 population, we identified that McAPRR2 as the best candidate gene for stigma color. We further developed a molecular marker linked to the variation presented in McAPRR2. The genome-wide survey of APRR2 genes in Cucurbitaceae revealed that they undergo whole genome duplications (WGDs) event and may have redundant or specific functions in stigma color modulation. This work will allow for the application of marker-assisted selection (MAS) for bitter gourd breeding and further increase our understanding of the molecular mechanism underlying stigma color development.
Two bitter gourd inbred lines, S051 with green stigma and S093 with yellow stigma, were the main subjects in the present study. They were used to generate F2 and F2-derived F3 populations for genetic mapping of stigma color. The F2-derived F3 population was constructed from self-pollination of F2 individuals exhibiting heterozygous genotype in preliminary mapping interval. A total of 241 F2 individuals and 847 F3 individuals were subjected to preliminary mapping using bulk-segregant-analysis (BSA)-seq and fine-mapping for the candidate genes, respectively. All plant materials were grown at QiLin north Campus Teaching & Research Base of South China Agricultural University, Guangzhou, China (23°N, 113°E). The stigma color from mature female flowers was classified using color card of RAL D9 DESIGN CYMPHONY OF COLOURS. The stigma colors close to RAL 110-80-70, 120-70-75, and 100-60-60 were assigned to green, while those are comparable to RAL 100-80-70, 95-80-70, and 90-80-90 were defined as yellow (Figure S1A).
BSA was employed for rapid identification of the stigma color locus. Two DNA bulks, the green stigma and yellow stigma DNA pools, were constructed each mixing equal amounts of genomic DNA from 30 individuals with green and yellow stigmas from F2 population. The two DNA pools were subjected to whole genome resequencing with Illumina GAIIx sequencer (Takagi et al., 2013). Raw reads were filtered by SOAPnuke software for removing adapters contamination and low-quality reads and further filtered by SAMtools with ‘view-q30’ standard parameter for sorting and quality control (Li et al., 2009; Chen et al., 2018). The Variants Calling followed the BWA-GATK workflow using the bitter gourd Dali-11 v1 as reference genome (Li and Durbin, 2009; Cui et al., 2020). The following filtration standards high quality Single Nucleotide Polymorphism (SNP) were applied: Genotype Quality >= 50, removing heterozygous locus between parents and SNP locus lacked in green and yellow pools (McKenna et al., 2010).
The filtered SNPs and insertion-deletion (InDel) were adopted for SNP-index and Euclidean distance (ED) algorithm analysis for BSA (Abe et al., 2012; Hill et al., 2013). The formulas of SNP-index and the Δ(SNP-index) were used as follow: SNP-index = ADr/(ADd+ADr), Δ(SNP-index) = SNP-index (yellow pool)-SNP-index (green pool), where ADr and ADd represent yellow and green stigma allele depth, respectively. An average SNP-index and Δ(SNP-index) of each position were calculated via 200 kb sliding window with 100 kb step, windows out of 95% and 99% confidence intervals of the Δ(SNP-index) were treated as significant and extremely significant windows respectively (Abe et al., 2012). The formula of ED2 was used as follow: ED2 = (Ayellow pool-Agreen pool)2+(Cyellow pool-Cgreen pool)2+(Gyellow pool-Ggreen pool)2+(Tyellow pool-Tgreen pool)2, where A, C, G, T represent the proportion of mutant type and reads respectively (Hill et al., 2013).
The polymorphic variants (SNPs and InDels) between the two parental lines S051 and S093 were further developed as molecular markers using Primer 3 web (https://primer3.ut.ee/) and SnapGene software for Cleaved Amplified Polymorphic Sequences (CAPS). These markers were applied to genotype 241 F2 individuals to construct a linkage map for the candidate region obtained from BSA-seq analysis via JoinMap 4 software. For further narrow down the candidate region, two flanking markers ST5 and ST8 were used to genotype additional 847 F3 individuals to identify recombinants plants. Additional markers were explored in the region defined by flanking markers. Information of all markers and primers used in this study is provided in Table S1.
The candidate genes in the 13.87 kb region of Dali-11 v1, OHB3-1v2, and TR v1 reference genome, and the predicted genes by the FGENESH program (http://www.softberry.com/berry.phtml) were compared and contrast. We then designed three pairs of primers to obtain the full-length CDS of McAPRR2 in both S051 and S093 accordingly (Table S1). The conserved domains were annotated with NCBI Conserved Domain Search (https://www.ncbi.nlm.nih.gov/Structure/cdd).
The expression dynamics of McAPRR2 were examined in different stages of the stigma from the two parental lines, which included 1 to 4 days before flowering (DBF) and the flowering day (FD). The tissue-specific expression of McAPRR2 was examined in the following organs as well: the root, stem, and leaf tissues were collected from 30 days seedlings; the stamen and petals of both male and female flowers were collected at FD; the ovary tissues were harvested from 4DBF to FD, as well as mature fruit skin tissue. All of the tissue samples were collected with three biological replicates, which were frozen temporarily in liquid nitrogen, and then stored at −80 °C for RNA isolation. The reverse transcription was performed using total RNA extracted by Eastep® Super Total RNA Extraction Kit (Promega, Shanghai) as follows: 500 ng RNA template, 2 μL Eastep® RT Master Mix (5×) and additional nuclease-free water to 10 μL reaction system. The qPCR procedure followed the manufacturer’s instruction of Eastep® qPCR Master Mix with the bitter gourd endogenous actin gene Mc01g0724. The fold changes in expression level of stigma and other tissues were relative subjected to stigma at 4DBF of S093 and stigma at FD of S093 using 2-ΔΔCT, respectively.
The genome-wide syntenic search using McAPRR2 as probe sequence were performed in the synteny module of CuGenDBv2 (http://cucurbitgenomics.org/v2/); their protein of APRR2 in Cucurbitaceae family were downloaded from CuGenDBv2 as well (Yu et al., 2022). The APRR2 genes from apple (LOC103440803), tomato (LOC101245957), pepper (CA06g13040, CA00g25180/Capana01g000809), eggplant (Sme2.5_00446.1_g00003.1) and Arabidopsis (AT4G18020) were downloaded from NCBI or genome database for tree construction as well (Hwang and Sheen, 2001; Jeong et al., 2020; Lim et al., 2021; Fang et al., 2023). The Arabidopsis AtAPRR1 (AT5G61380), AtGLK1 (AT2G20570), and AtGLK2 (AT5G44190) were included for outgroup (Hwang and Sheen, 2001; Susila et al., 2023).
Sequence alignment were performed using DNAMAN V6 software. Bootstrap method with 1000 replications and Poisson model were used as phylogeny test and substitution model, respectively, while constructing the neighbor-joining tree in MEGA 11 software (Tamura et al., 2021). The tree was visualized with R/ggtree package (Yu et al., 2017). The conserved motifs of APRR2 proteins were evaluated via online MEME software (http://meme-suite.org/). The positions of the conserved domains were visualized in R/ggplot2 package.
To reveal the genetic inheritance of the stigma colors of bitter gourd, we employed two bitter gourd lines, S051 and S093, of which stigma displayed distinctive green and yellow colors that were distinguishable as early as four days before flowering (DBF) (Figure 1). Then, they were crossed to develop an F2 population consisting of 241 individuals for genetic study. To prevent any ambiguity in color assessment, we scored the stigma color of F1 plants and the F2 populations using a RAL D9 color card that differentiated a range of green and yellow hues through a series of Hue-Saturation-Lightness (HSL) values (Figure S1). According to the color card, the F1 individuals exhibited a green stigma phenotype implying that the green color of stigma is dominant over yellow stigma (Table S1B). Of the 241 F2 population, 191 plants have green stigma and 50 have yellow stigma, which is consistent with the 3:1 expected segregation ratio (P=0.13 in χ2 test). It demonstrates that the green stigma in bitter gourd is regulated by a single dominant locus, which is designated as McSTC1 (Momordica charantia Stigma Color 1) hereinafter.
Figure 1 Phenotype of stigma color in two parental lines (S051 and S093) from four days before flowering (4DBF) to flowering day (FD). Bars in stigma and female flower represent 1 mm and 1 cm, respectively.
To primarily determine the location of McSTC1, we conducted BSA-seq. Thirty plants with distinctly green and yellow stigma were selected from the 241 F2 population, and their DNA were pooled to create two DNA bulks. The two parental lines together with the two DNA bulks were subjected to whole genome resequencing. A total of average 16.63 Gb clean reads were obtained and the average read depth coverage is 53.83×. After proper filtering, an average of 97.08% reads at Q20 value were mapped to the reference Dali-11 v1 reference genome (Cui et al., 2020; http://cucurbitgenomics.org/v2/) (Table S2). Based on the uniquely mapped reads and proper filtering through GATK pipeline, a total of 392,662 SNPs were identified across 11 chromosomes between the green and yellow pools. These SNPs were adopted to calculate the Δ(SNP-index) and ED2 to determine the associated region with the stigma colors. By plotting against their positions along each pseudochromosome of the Dali-11 v1, a single significant peak with Δ(SNP-index) and ED2 ≥ 0.5 were identified on pseudochromosome 6 at a confidence level higher than 0.99 (Figure 2). The peak covered a physical interval of 4.94 Mb from 21.41 to 26.35 Mb, suggesting this candidate genomic region is the McSTC1 locus and harbors the causative mutation (Table S3).
Figure 2 Bulked segregant analysis (BSA) of the McSTC1 based on SNP-index and ED algorithm. (A) The Manhattan plot of Δ(SNP-index). The black line is the fitted Δ(SNP-index). The pink and blue lines represent the thresholds of top 5% and 1% respectively. (B) The Manhattan plot of ED2 value. The confidence interval of McSTC1 is limited with red dotted lines in 4.94-Mb at pseudochromosome 6.
In order to narrow down the interval of the McSTC1 locus, regional linkage analysis and fine mapping were performed using the called variants obtained from BSA-seq. Based on the mapped reads of the two parental lines and two DNA pools, a total of 3,918 InDels and SNPs were identified in the 4.94 Mb candidate region (Table S3). Among them, we selected 17 InDel variants for polymorphic markers development, approximately evenly dispersed based on their physical positions from 22.67 to 25.41 Mb (Table S1). These markers were first applied to confirm in each F2 individual from two DNA pools. Accordingly, 14 markers (ST1-ST14, spanning from 23.45 to 24.22 Mb) that are closely linked to phenotype in two DNA pools were further utilized to genotype the primary 241 F2 population and to construct a regional linkage map covering a genetic distance of 6.7 cM (Figure 3A). The genetic mapping results showed that two markers, ST6 and ST7, were co-segregated with McSTC1. It then delimited McSTC1 locus into a 117.84 kb region by the two flanking markers, ST5 and ST8 (Figure 3B).
Figure 3 Linkage mapping and fine mapping of the McSTC1 locus. (A) The linkage map developed using 241 F2 population from the cross between S051 and S093. (B) The physical position of each marker according to reference genome Dali-11 v1.0. (C) The fine-mapping of McSTC1 locus based on the recombinants identified from an F3 population consisting of 847 individuals. The black, white, and grey bar indicate the genotypes of S051 homozygotes, S093 homozygotes, and heterozygotes, respectively. The phenotype of G and Y are abbreviations of stigma color Green and Yellow, respectively.
To further pinpoint the candidate gene, we employed additional 5 InDel and 2 CAPS markers (ST15-ST21) in this region. Furthermore, a number of heterozygous F2 plants based on the heterozygous genotypes of ST1 and ST14 were self-pollinated to generate a fine-mapping F3 population. The two flanking markers (ST5 and ST8) were used to genotype a total of 847 F3 seedlings which identified 7 recombinants. Their stigma colors were then investigated in adult stage. By inspection of the genotypic and phenotypic data of the recombinants, the McSTC1 is finally narrowed down into a 13.87 kb region defined by markers ST6 and ST20 (Figure 3C). Within this region, only one protein coded gene was annotated from the Dali-11 v1, namely Mc06g1638 (McAPRR2), which encoded a homolog of Arabidopsis two-component response regulator-like protein (APRR2).
Currently, three reference genomes for bitter gourd are available: Dali-11 v1, OHB3-1 v2, and TR v1 (https://cucurbitgenomics.org/v2). All of the three reference genomes and FGENESH annotated one single Mc06g1638 gene in 13.87 kb region, but they show discrepant prediction for its start codon and exon-intron structures. Thus, we designed three pairs of primers to further confirm the full-length cDNA sequences of McAPRR2 in S051 and S093 (Table S1). We showed that the coding sequence of McAPRR2 were 1560 bp and 1152 bp from S051 and S093 that are inconsistent with all reference genomes (CuGenDBv2: Mc06g1638, Moc06g35570 and MS005431.1) (Figure S2). A total of five variants (3 SNPs and 2 InDels) were identified between the two parental lines: a synonymous SNP.G23827556T at the 11th exon; two non-synonymous SNP.A23824615G and SNP.A23825627G at the 6th exon and the 8th exon resulted in D211G and N321D amino acid substitutions, respectively; two insertions in S093 with 39 bp and 15 bp at the 1st exon and the 9th exon resulted in an insertion of 13 amino acid and an introduction of premature stop codon, respectively (Genbank accession numbers OP972606 and OP972607 Figure 4A; Figures S2, S3).
Figure 4 The sequence variance of McAPRR2 and genotypic results of the 15 bp InDel variance of McAPRR2 in 45 inbred lines of bitter gourd. (A) The gene structure, sequence and amino acid variance, and conserved domain analysis of McAPRR2. (B) A total of 19 lines had yellow stigma shared same genotype as S093 with the 15bp insertion. The other 26 lines showed green stigma are same as S051.
The candidate gene McAPRR2 of S051 allele encoded a complete protein of 519 amino acids with three conserved domains including phosphoacceptor receiver (REC) domain superfamily (cl19078) and Golden-2 like superfamily (GLK2, PLN03162) that nested with myb-like DNA-binding domain (myb SHAQKYF). The 39 bp InDel and the two non-synonymous SNPs located at REC domain and GLK2 domain, respectively, whereas the 15 bp InDel leads to a truncated GLK2 domain (Figure 4A).
We noticed that the bitter gourd cultivar Dali-11 has yellow stigma sharing the same variants with S093 including SNP.A23824615G, SNP.A23825627G, and present of the 15 bp insertion, while the wild bitter gourd TR has the green stigma with same variants as S051 (Cui et al., 2020). To confirm the association of these variants with stigma color, we phenotyped and genotyped 45 inbred lines which mainly collected in Southern Asia countries (Table S4). As a result, the 19 inbred lines with yellow stigma have the 15 bp insertion, and 26 inbred lines with green stigma lack it, which were consistent with genotyping results in S051 and S093 (Figure 4B; Table S6). To examine the non-synonymous SNP variants in the 45 lines, we designed a pair of primers covering the two SNPs to amplify the DNA of 45 inbred lines (Table S1). The Sanger sequencing result showed that the SNP.A23825627G is completely consistent with the phenotype while SNP.A23824615G is a bit deviated from it (Table S6). The SNP and InDel markers could be potentially applied for marker-assisted selection (MAS) for stigma color breeding in bitter gourd.
In order to address the expression pattern of McAPRR2, we collected five developmental stages of stigma from 4 DBF to the date it blossoms and other organ tissues including stamen, petal, root, stem, leaf, ovary, and fruit peels from the two parental lines. As shown in Figure 5A, the expression level of the stigma decreases as it grows from immature to blossom stage in both lines. However, regardless of the stigma developmental stages, the green stigma bitter gourd still showed a significant high expression level compared with the yellow stigma gourd. In addition, McAPRR2 shows a higher expression level in S051 than in S093 across all organ tissues (Figure 5B). The expression level of McAPRR2 was relatively low in the petals of both female and male flowers. Combined with the results of fine mapping and expression analysis, it is inferred that McAPRR2 is the best candidate for McSTC1 locus. The interrupting expression of McAPRR2 may result in less accumulation of chlorophyll that result in yellow stigma color in bitter gourd.
Figure 5 The relative expression between the parental lines S051 and S093. (A) The relative developmental expression level of stigma tissues from 4 days before flowering (DBF) to flowering day (FD). (B) The relative tissue-specific expression level in other organ tissues including stamen, petals, root, stem, leaf, ovary from 4DBF to FD, and fruit skin. The data are presented as the average values of three biological replicates (Mean ± SE). * and ** indicate the statistically significant differences of expression level at P < 0.05 and P <0.01 level, respectively, according to student t-test.
It has been revealed that the APRR2 orthologs in the cucurbit plants are associated with plastid number and the chloroplast development (Liu et al., 2016; Jiao et al., 2017; Oren et al., 2019). By conducting a genome-wide survey of the APRR2 gene in the genome of bitter gourd, two APRR2 genes, Mc06g1638/Moc06g35570 (this study) and Mc10g1288/Moc10g28360, are identified in both Dali-11 v1 and OHB3-1 v2 reference genome assembly. Thus, to further understand the functional relationship of APRR2 genes within other cucurbit crops, we performed a genome-wide synteny search of the two bitter gourd McAPRR2 genes with eight crops from Cucurbitaceae family, including cucumber (Cucumis sativus), melon (Cucumis melo), Watermelon (Citrullus lanatus), Sponge gourd (Luffa cylindrica), Bottle gourd (Lagenaria siceraria), Wax gourd (Benincasa hispida), Cucurbita moschata, and Cucurbita maxima (Yu et al., 2022). Similar to bitter gourd, most investigated cucurbit crops here harbored two copies of APRR2 genes, with the exception of cucumber and Cucurbita (Cucurbita moschata and Cucurbita maxima), whose genomes encode a total of three and seven APRR2 genes, respectively (Table S5). The two copies of APRR2 gene in majority of cucurbit crops and the extended APRR2 genes in Cucurbita are probably the result of the first step of whole-genome duplication (WGD) event at the origin of the Cucurbitaceae family and tandem-duplications at Cucurbiteae tribe, respectively (Sun et al., 2017; Guo et al., 2020; Ma et al., 2022).
To dissect the evolutionary relationship of APRR2 genes, we further performed a phylogenetic analysis using neighbor-joining method for the APRR2 genes from Cucurbitaceae family and other plant species including Arabidopsis, Tomato, Apple, and Pepper (Figure 6A). It clearly shows that the Arabidopsis GLK1/2 and AtAPRR1 clade is distinct from the APRR2 clade of all other plants. All of the APRR2 contained two typical domains: Rec super family and Golden-2 like domain (PLN03162), which corresponded to the myb SHAQKYF domain according to InterproScan and Conserved Domian Databased (CDD) (Figure 6B). In addition, the APRR2 genes from Cucurbits crops were clustered into two groups according to their similarities in amino acid sequence. The genes in Group I and Group II share partial and complete Golden-2 like domain, respectively. The Group I genes include those APRR2 genes that have been shown to correlate to the white or pale green fruit skin such as cucumber CsAPRR2-3, melon CmAPRR2-1, watermelon ClaAPRR2-1, and wax gourd BhAPRR2-1 (Figure 6A; Liu et al., 2016; Oren et al., 2019; Ma et al., 2021). In addition, the Zucchini CpoAPRR2-1 and CpoAPRR2-2 were found associated with light green stem color (Zhu et al., 2022). The bitter gourd McAPRR2 reside in Group I as well (Figure 6A). In particular, the two adjacent genes CmoCh19G004530 and CmoCh19G004540 in Pumpkin reference genome (Cucurbita moschata) were annotated to have the REC and Golden-2 like domain, respectively. We further re-annotated the two genes using FGENESH which indicated that they belong to a single APRR2 gene. Thus, we designated them as CmoAPRR2-7 accordingly (Table S5; Figure 6B).
Figure 6 Phylogenetic analysis of APRR2 from Cucurbitaceae family and other plant species. (A) The neighbor-joining tree of APRR2.(B) The domain demonstration of APRR2. Genes information is provided in Table S5.
In the present study, we conducted BSA-seq of the Stigma color (McSTC1) locus in bitter gourd inbred lines S051 and S093, and delimited it into a 13.87 kb interval at MC06 that contains only one gene, Mc06g01638 (McAPRR2) (Figure 3). The gene expression and allelic diversity analyses supported McAPRR2 as the candidate gene for McSTC1 (Figures 4, 5). McAPRR2 is a homolog of Arabidopsis AtAPRR2 (AT4G18020) that is a plant specific transcription factor belonging to the Pseudo-Response Regulator (PRR) family originated from Authentic Response Regulator (ARRs) (Makino et al., 2000). We further cloned the CDS of McAPRR2 in the two parental lines via sanger sequencing; combined with BSA-seq results, we confirmed the two non-synonymous SNP variants (SNP.A23824615G and SNP.A23825627G) and two InDels (39 bp and 15 bp insertion in S093) (Figure 4A). The 15 bp insertion were designed as InDel markers to genotype 45 bitter gourd inbred lines mainly collected from Southern Asia countries; the present-absent of 15 bp sequence showed a completely agreement with their stigma color with all yellow stigma inbred lines harbor the 15 bp insertion (Figure 4B; Table S6). The two non-synonymous SNP variants were examined through Sanger sequencing: the SNP.A23825627G is consistent with expected phenotypes while SNP.A23824615G showed discrepancies (Table S6). Although the examined collected inbred lines are not abundant, it still suggests a greatly potential of applying the 15 bp InDel marker and SNP markers for bitter gourd MAS breeding.
An early study conducted using an F2 population derived from a cross between bitter gourd inbred lines “Taiwan White” and “CBM12” also revealed a single dominant locus for stigma color StCol which was mapped to LG1 linked with AFLP markers E19M57h and E18M53b; due to the limitation of marker information, its physical position is unknown (Kole et al., 2012). In addition, Kole et al. (2012) reported the independently inherited nature of StCol that is not associated with other investigated fruit traits including fruit skin color, fruit surface structure, and seed color. In contrast to McSTC1, StCol displays a predominance of yellow stigma over green stigma, indicating the existence of other regulatory mechanisms governing the stigma color in bitter gourd. An additional genome-wide association investigation using a widely collected large population may be able to enhance our knowledge of the genetic variability of bitter gourd stigma color (Cui et al., 2022).
In Cucurbit crops, green and yellow stigma are two colors have been observed (Lv et al., 2022b). The green color of stigmas is likely caused by accumulation of chlorophyll, the same pigment that gives plants leaves and fruits their characteristic green appearance. The genetic study for melon (Cucumis melo) stigma color showed that green stigma is dominant over the yellow one; transcriptomic analysis proposed that the yellow color is probably due to the break-down of chloroplast structure interfering with chlorophyll biosynthesis; in contrast, the green stigmas are a functional accumulation of chlorophyll (Lv et al., 2022a; Lv et al., 2022b). This might also be true for the green/yellow stigma of bitter gourd. In the present study, we showed that McAPRR2 is the best candidate gene for McSTC1 locus; particularly the loss-of function allele caused by 15 bp-insertion in S093 resulted in yellow stigma. McAPRR2 is a homolog of Arabidopsis pseudo-response regulator 2 gene (APRR2). A typical PRR2 possesses a receiver-like domain (RLD) and a Golden-2 like (GLK) domain that contains a Myb-like DNA binding domain (Hosoda et al., 2002; Chen et al., 2016). The GLKs transcription factors (TFs) belong to a GARP superfamily TF whose functions are important for the expression of nuclear photosynthesis-related genes and for chloroplast development in both leaves and fruits (Riechmann et al., 2000; Hosoda et al., 2002; Waters et al., 2008; Nguyen et al., 2014). The APRR2 orthologs have been shown to regulate chlorophyll content in a wide range of crops from Solanaceae and Cucurbitaceae including tomato, pepper, eggplants, cucumber, melon, watermelon, wax gourd, and Zucchini (Pan et al., 2013; Liu et al., 2016; Oren et al., 2019; Jeong et al., 2020; Ma et al., 2021; Arrones et al., 2022; Zhu et al., 2022; Fang et al., 2023). These genes are found to associate with increased plastid numbers and chlorophyll accumulation of fruit colors in immature fruit peels, resulted in white or light green. The cucumber CsAPRR2 served as TF contributing to the chloroplast development was reported through the interaction with Class-I KNOTTED 1-like homeobox (KNOX) genes TKN4 and TKN2 (Jiao et al., 2017). Particularly, the KNOX genes play an important role in plant hormone cytokine (CTK) network for its role in regulating the development and activity of chloroplasts (Cortleven and Schmülling, 2015). It is reasonable to proposed that loss of function of McAPRR2 allele in S093 may impair its interaction ability with downstream genes for its regulation roles in the chloroplast development in stigma tissues, resulting in a recessive yellow coloration. In addition, the relative expression level of McAPRR2 in S051 with green stigma is significantly higher than that in S093 with yellow stigma throughout stigma developmental stages, as well as majority of the organ tissue (except for petals). According to the result from BSA-seq, a number of 14 variants at ~2 kb upstream of McAPRR2 were found (highlight in Table S3, L2650-2663). Whether they are responsible for the differential expression between the two parental lines requires further analysis. In addition, the fruits of S093 bear lighter green fruit skin compared with that of S051 line (Figure S4); nevertheless, additional work is required to determine whether the McAPRR2 gene is also responsible for the fruit skin color of bitter gourd.
Throughout the plant evolutionary history, the Cucurbitaceae plants experienced at least four whole-genome duplication (WGD) events (Guo et al., 2020). The first large-scale duplication event is the cucurbit-common tetraploidization (CCT) occurred at the origin of Cucurbit crops (115-130 Mya) (Wang et al., 2018; Guo et al., 2020; Ma et al., 2022). The CCT event likely contribute to the presence of two copies of APRR2 in bitter gourd and other cucurbits crops, as disclosed by a genome-wide syntenic search for Cucurbitaceae family. Additionally, a cluster of APRR2 genes from Cucurbita were showed in the phylogenetic tree (CmoCh02G016320-350 and CmaCh02G015890-930), that likely evolved in response to a lineage-specific recent WGD event (Sun et al., 2017; Ma et al., 2022).
The APRR2 genes from cucurbit crops fall into two groups (I and II) accordingly and both groups have conserved REC superfamily and GLK2 superfamily domains. The genes in Group I and Group II are mainly distinguished by the presence of partial and complete Golden-2 like (GLK) domain, respectively (Figure 6B). The Group I clade includes the CsAPRR2 in cucumber, CmAPRR2 in melon, ClaAPRR2 in watermelon, BhAPRR2 in wax gourd which have been shown to correlate with white or light green fruit skin and CpoAPRR2 in Zucchini is associated with stem color (Table S5, Figure 6A; Liu et al., 2016; Oren et al., 2019; Ma et al., 2021; Zhu et al., 2022). McAPRR2, the candidate gene in regulating green stigma color of bitter gourd, is clustered with the genes in Group I as well (Figure 6A). It is reasonable to speculate that McAPRR2 may also play a role in chloroplast development for fruit skin color, since the line S093 bears lighter green fruit skin of bitter gourd compared with line S051(Figure S4). A further investigation of the fruit skin color using the same population might aid in dissolving the involvement of McAPRR2. In addition, Further studies are needed to determine if the two APRR2 copies act redundantly or specifically in coordinating the control of chloroplast development throughout all fruit tissues and developmental stages.
The datasets presented in this study can be found in online repositories. The names of the repository/repositories and accession number(s) can be found in the article/Supplementary material.
JYZ and JZ performed majority of the experiments. YW participated the data analysis. KH and JC conceive and supervised the study. YW wrote the manuscript with JYZ. All authors contributed to the article and approved the submitted version.
This study was supported by a grant from the Key Project of Basic and Applied Research for University in Guangdong Province (2018KZDXM016), the Science and Technology Planning Project of Guangdong Province (2018B020202007), the Guangzhou Science and Technology Plan Projects (202002020086, 202102020800, and 202206010170), and the Science and Technology Plan Projects of Guangdong Province (2019A050520002). YW was supported by the Nanjing Agricultural University Startup Fund (130804131).
The authors would like to thank Jiayue Jin, Jiali Liu, and Mingwei Zhuang for their assistance throughout the field management.
The authors declare that the research was conducted in the absence of any commercial or financial relationships that could be construed as a potential conflict of interest.
All claims expressed in this article are solely those of the authors and do not necessarily represent those of their affiliated organizations, or those of the publisher, the editors and the reviewers. Any product that may be evaluated in this article, or claim that may be made by its manufacturer, is not guaranteed or endorsed by the publisher.
The Supplementary Material for this article can be found online at: https://www.frontiersin.org/articles/10.3389/fpls.2023.1128926/full#supplementary-material
Abe, A., Kosugi, S., Yoshida, K., Natsume, S., Takagi, H., Kanzaki, H., et al. (2012). Genome sequencing reveals agronomically important loci in rice using MutMap. Nat. Biotechnol. 30, 174–178. doi: 10.1038/nbt.2095
Arrones, A., Mangino, G., Alonso, D., Plazas, M., Prohens, J., Portis, E., et al. (2022). Mutations in the SmAPRR2 transcription factor suppressing chlorophyll pigmentation in the eggplant fruit peel are key drivers of a diversified colour palette. Front. Plant Sci. 13. doi: 10.3389/fpls.2022.1025951
Beedanagari, S. R., Dove, S. K., Wood, B. W., Conner, P. J. (2005). A first linkage map of pecan cultivars based on RAPD and AFLP markers. Theor. Appl. Genet. 110, 1127–1137. doi: 10.1007/s00122-005-1944-5
Carlson, J. E., Holsinger, K. E. (2010). Natural selection on inflorescence color polymorphisms in wild protea populations: the role of pollinators, seed predators, and intertrait correlations. Am. J. Bot. 97, 934–944. doi: 10.3732/ajb.0900348
Chen, Y., Chen, Y., Shi, C., Huang, Z., Zhang, Y., Li, S., et al. (2018). SOAPnuke: a MapReduce acceleration-supported software for integrated quality control and preprocessing of high-throughput sequencing data. Gigascience 7, 1–6. doi: 10.1093/gigascience/gix120
Chen, M., Ji, M., Wen, B., Liu, L., Li, S., Chen, X., et al. (2016). GOLDEN 2-LIKE transcription factors of plants. Front. Plant Sci. 7. doi: 10.3389/fpls.2016.01509
Cortleven, A., Schmülling, T. (2015). Regulation of chloroplast development and function by cytokinin. J. Exp. Bot. 66, 4999–5013. doi: 10.1093/jxb/erv132
Cui, J., Yang, Y., Luo, S., Wang, L., Huang, R., Wen, Q., et al. (2020). Whole-genome sequencing provides insights into the genetic diversity and domestication of bitter gourd (Momordica spp.). Hortic. Res. 7, 1–11. doi: 10.1038/s41438-020-0305-5
Cui, J., Zhou, Y., Zhong, J., Feng, C., Hong, Y., Hu, K., et al. (2022). Genetic diversity among a collection of bitter gourd (Momordica charantia l.) cultivars. Genet. Resour. Crop Evol. 69, 729–735. doi: 10.1007/s10722-021-01258-6
Fang, H., Wang, P., Wang, W., Peng, J., Zheng, J., Zhu, G., et al. (2023). Fine mapping and identification of SmAPRR2 regulating rind color in eggplant (Solanum melongena l.). Int. J. Mol. Sci. 24, 1–15. doi: 10.3390/ijms24043059
Gervasi, D. D. L., Schiestl, F. P. (2017). Real-time divergent evolution in plants driven by pollinators. Nat. Commun. 8, 1–8. doi: 10.1038/ncomms14691
Guo, J., Xu, W., Hu, Y., Huang, J., Zhao, Y., Zhang, L., et al. (2020). Phylotranscriptomics in cucurbitaceae reveal multiple whole-genome duplications and key morphological and molecular innovations. Mol. Plant 13, 1117–1133. doi: 10.1016/j.molp.2020.05.011
Hill, J. T., Demarest, B. L., Bisgrove, B. W., Gorsi, B., Su, Y. C., Yost, H. J. (2013). MMAPPR: mutation mapping analysis pipeline for pooled RNA-seq. Genome Res. 23, 687–697. doi: 10.1101/gr.146936.112
Hosoda, K., Imamura, A., Katoh, E., Hatta, T., Tachiki, M., Yamada, H., et al. (2002). Molecular structure of the GARP family of plant myb-related DNA binding motifs of the arabidopsis response regulators. Plant Cell 14, 2015–2029. doi: 10.1105/tpc.002733
Hwang, I., Sheen, J. (2001). Two-component circuitry in arabidopsis cytokinin signal transduction. Nature 413, 383–389. doi: 10.1038/35096500
Jeong, H. B., Jang, S. J., Kang, M. Y., Kim, S., Kwon, J. K., Kang, B. C. (2020). Candidate gene analysis reveals that the fruit color locus C1 corresponds to PRR2 in pepper (Capsicum frutescens). Front. Plant Sci. 11. doi: 10.3389/fpls.2020.00399
Jiao, J., Liu, H., Liu, J., Cui, M., Xu, J., Meng, H., et al. (2017). Identification and functional characterization of APRR2 controlling green immature fruit color in cucumber (Cucumis sativus l.). Plant Growth Regul. 83, 233–243. doi: 10.1007/s10725-017-0304-1
Köhler, M., Esser, L. F., Font, F., Souza-Chies, T. T., Majure, L. C. (2020). Beyond endemism, expanding conservation efforts: what can new distribution records reveal? Perspect. Plant Ecol. Evol. Syst. 45, 125543. doi: 10.1016/j.ppees.2020.125543
Kole, C., Olukolu, B. A., Kole, P., Rao, V. K., Bajpai, A., Backiyarani, S., et al. (2012). The first genetic map and positions of major fruit trait loci of bitter melon (Momordica charantia). J. Plant Sci. Mol. Breed. 1, 1. doi: 10.7243/2050-2389-1-1
Koski, M. H., Berardi, A. E., Galloway, L. F. (2020). Pollen colour morphs take different paths to fitness. J. Evol. Biol. 33, 388–400. doi: 10.1111/jeb.13599
Li, H., Durbin, R. (2009). Fast and accurate short read alignment with burrows-wheeler transform. Bioinformatics 25, 1754–1760. doi: 10.1093/bioinformatics/btp324
Li, H., Handsaker, B., Wysoker, A., Fennell, T., Ruan, J., Homer, N., et al. (2009). The sequence Alignment/Map format and SAMtools. Bioinformatics 25, 2078–2079. doi: 10.1093/bioinformatics/btp352
Lim, J., Lim, C. W., Lee, S. C. (2021). Pepper novel pseudo response regulator protein CaPRR2 modulates drought and high salt tolerance. Front. Plant Sci. 12. doi: 10.3389/fpls.2021.736421
Liu, H., Jiao, J., Liang, X., Liu, J., Meng, H., Chen, S., et al. (2016). Map-based cloning, identification and characterization of the w gene controlling white immature fruit color in cucumber (Cucumis sativus l.). Theor. Appl. Genet. 129, 1247–1256. doi: 10.1007/s00122-016-2700-8
Lv, Y., Amanullah, S., Liu, S., Zhang, C., Liu, H., Zhu, Z., et al. (2022a). Comparative transcriptome analysis identified key pathways and genes regulating differentiated stigma color in melon (Cucumis melo l.). Int. J. Mol. Sci. 23, 1–17. doi: 10.3390/ijms23126721
Lv, Y., Gao, P., Liu, S., Fang, X., Zhang, T., Liu, T., et al. (2022b). Genetic mapping and QTL analysis of stigma color in melon (Cucumis melo l.). Front. Plant Sci. 13. doi: 10.3389/fpls.2022.865082
Ma, L., Liu, Z., Cheng, Z., Gou, J., Chen, J., Yu, W., et al. (2021). Identification and application of BhAPRR2 controlling peel colour in wax gourd (Benincasa hispida). Front. Plant Sci. 12. doi: 10.3389/fpls.2021.716772
Ma, L., Wang, Q., Zheng, Y., Guo, J., Yuan, S., Fu, A., et al. (2022). Cucurbitaceae genome evolution, gene function, and molecular breeding. Hortic. Res. 9, 1–24. doi: 10.1093/hr/uhab057
Makino, S., Kiba, T., Imamura, A., Hanaki, N., Nakamura, A., Suzuki, T., et al. (2000). Genes encoding pseudo-response regulators: insight into his-to-Asp phosphorelay and circadian rhythm in arabidopsis thaliana. Plant Cell Physiol. 41, 791–803. doi: 10.1093/pcp/41.6.791
Matsumura, H., Hsiao, M. C., Lin, Y. P., Toyoda, A., Taniai, N., Tarora, K., et al. (2020). Long-read bitter gourd (Momordica charantia) genome and the genomic architecture of nonclassic domestication. Proc. Natl. Acad. Sci. U. S. A. 117, 14543–14551. doi: 10.1073/pnas.1921016117
McKenna, A., Hanna, M., Banks, E., Sivachenko, A., Cibulskis, K., Kernytsky, A., et al. (2010). The genome analysis toolkit: a MapReduce framework for analyzing next-generation DNA sequencing data. Genome Res. 20, 1297–1303. doi: 10.1101/gr.107524.110
Meng, L., Qi, C., Wang, C., Wang, S., Zhou, C., Ren, Y., et al. (2021). Determinant factors and regulatory systems for anthocyanin biosynthesis in rice apiculi and stigmas. Rice 14, 1–18. doi: 10.1186/s12284-021-00480-1
Miklič, V., Sakač, Z. O., Dušanić, N. Z., Atlagić, J. D., Joksimović, J. P., Vasić, D. M. (2002). Effects of genotype, fertilization and microclimate on visitation by pollinators and yield of sunflower. Zbornik radova Instituta za ratarstvo i povrtarstvo. (36), 179–188.
Miller, R., Owens, S. J., Rørslett, B. (2011). Plants and colour: flowers and pollination. Opt. Laser Technol. 43, 282–294. doi: 10.1016/j.optlastec.2008.12.018
Nguyen, C. V., Vrebalov, J. T., Gapper, N. E., Zheng, Y., Zhong, S., Fei, Z., et al. (2014). Tomato GOLDEN2-LIKE transcription factors reveal molecular gradients that function during fruit development and ripening. Plant Cell 26, 585–601. doi: 10.1105/tpc.113.118794
Oren, E., Tzuri, G., Vexler, L., Dafna, A., Meir, A., Faigenboim, A., et al. (2019). The multi-allelic APRR2 gene is associated with fruit pigment accumulation in melon and watermelon. J. Exp. Bot. 70, 3781–3794. doi: 10.1093/jxb/erz182
Pan, Y., Bradley, G., Pyke, K., Ball, G., Lu, C., Fray, R., et al. (2013). Network inference analysis identifies an APRR2-like gene linked to pigment accumulation in tomato and pepper fruits. Plant Physiol. 161, 1476–1485. doi: 10.1104/pp.112.212654
Qiao, A., Fang, X., Liu, S., Liu, H., Gao, P., Luan, F. (2021). QTL-seq identifies major quantitative trait loci of stigma color in melon. Hortic. Plant J. 7, 318–326. doi: 10.1016/j.hpj.2021.01.004
Rao, P. G., Behera, T. K., Gaikwad, A. B., Munshi, A. D., Srivastava, A., Boopalakrishnan, G., et al. (2021). Genetic analysis and QTL mapping of yield and fruit traits in bitter gourd (Momordica charantia l.). Sci. Rep. 11, 1–10. doi: 10.1038/s41598-021-83548-8
Riechmann, J. L., Heard, J., Martin, G., Reuber, L., Jiang, C. Z., Keddie, J., et al. (2000). Arabidopsis transcription factors: genome-wide comparative analysis among eukaryotes. Sci. 290, 2105–2110. doi: 10.1126/science.290.5499.2105
Sharifkhah, M., Bakhshi, D., Pourghayoumi, M., Abdi, S., Hokmabadi, H. (2020). Effect of pollination time on yield and antioxidant properties of some pistachio cultivars. Int. J. Hortic. Sci. Technol. 7, 51–58. doi: 10.22059/ijhst.2020.285527.306
Sun, H., Wu, S., Zhang, G., Jiao, C., Guo, S., Ren, Y., et al. (2017). Karyotype stability and unbiased fractionation in the paleo-allotetraploid cucurbita genomes. Mol. Plant 10, 1293–1306. doi: 10.1016/j.molp.2017.09.003
Susila, H., Nasim, Z., Gawarecka, K., Jung, J.-Y., Jin, S., Youn, G., et al. (2023). Chloroplasts prevent precocious flowering through a GOLDEN2-LIKE–B-BOX DOMAIN PROTEIN module. Plant Commun. 4, 100515. doi: 10.1016/j.xplc.2023.100515
Takagi, H., Abe, A., Yoshida, K., Kosugi, S., Natsume, S., Mitsuoka, C., et al. (2013). QTL-seq: rapid mapping of quantitative trait loci in rice by whole genome resequencing of DNA from two bulked populations. Plant J. 74, 174–183. doi: 10.1111/tpj.12105
Tamura, K., Stecher, G., Kumar, S. (2021). MEGA11: molecular evolutionary genetics analysis version 11. Mol. Biol. Evol. 38, 3022–3027. doi: 10.1093/molbev/msab120
Trunschke, J., Lunau, K., Pyke, G. H., Ren, Z. X., Wang, H. (2021). Flower color evolution and the evidence of pollinator-mediated selection. Front. Plant Sci. 12. doi: 10.3389/fpls.2021.617851
Urasaki, N., Takagi, H., Natsume, S., Uemura, A., Taniai, N., Miyagi, N., et al. (2017). Draft genome sequence of bitter gourd (Momordica charantia), a vegetable and medicinal plant in tropical and subtropical regions. DNA Res. 24, 51–58. doi: 10.1093/dnares/dsw047
Wang, J., Sun, P., Li, Y., Liu, Y., Yang, N., Yu, J., et al. (2018). An overlooked paleotetraploidization in cucurbitaceae. Mol. Biol. Evol. 35, 16–26. doi: 10.1093/molbev/msx242
Waters, M. T., Moylan, E. C., Langdale, J. A. (2008). GLK transcription factors regulate chloroplast development in a cell-autonomous manner. Plant J. 56, 432–444. doi: 10.1111/j.1365-313X.2008.03616.x
Yu, G., Smith, D. K., Zhu, H., Guan, Y., Lam, T. T. Y. (2017). GGTREE: an r package for visualization and annotation of phylogenetic trees with their covariates and other associated data. Methods Ecol. Evol. 8, 28–36. doi: 10.1111/2041-210X.12628
Yu, J., Wu, S., Sun, H., Wang, X., Tang, X., Guo, S., et al. (2022). CuGenDBv2: an updated database for cucurbit genomics. Nucleic Acids Res. 51, D1457–D1464. doi: 10.1093/nar/gkac921
Zhang, Y., Zhao, G., Li, Y., Zhang, J., Shi, M., Muhammad, T., et al. (2017). Transcriptome profiling of tomato uncovers an involvement of cytochrome P450s and peroxidases in stigma color formation. Front. Plant Sci. 8. doi: 10.3389/fpls.2017.00897
Zhao, G., Lei, Q., Muhammad, T., Zhang, Y., Zhang, Y., Liang, Y. (2017). Inheritance and phenotypic analysis of yellow stigma(ys)Mutant of tomato. Acta Hortic. Sin. 44, 2109–2116. doi: 10.16420/j.issn.0513-353x.2017-0170
Zhong, J., Cheng, J., Cui, J., Hu, F., Dong, J., Liu, J., et al. (2022). MC03g0810, an important candidate gene controlling black seed coat color in bitter gourd (Momordica spp.). Front. Plant Sci. 13. doi: 10.3389/fpls.2022.875631
Keywords: bitter gourd, stigma color, BSA-seq, McAPRR2, fine mapping
Citation: Zhan J, Zhong J, Cheng J, Wang Y and Hu K (2023) Map-based cloning of the APRR2 gene controlling green stigma in bitter gourd (Momordica charantia). Front. Plant Sci. 14:1128926. doi: 10.3389/fpls.2023.1128926
Received: 21 December 2022; Accepted: 20 March 2023;
Published: 10 May 2023.
Edited by:
Byoung-Cheorl Kang, Seoul National University, Republic of KoreaReviewed by:
Je Min Lee, Kyungpook National University, Republic of KoreaCopyright © 2023 Zhan, Zhong, Cheng, Wang and Hu. This is an open-access article distributed under the terms of the Creative Commons Attribution License (CC BY). The use, distribution or reproduction in other forums is permitted, provided the original author(s) and the copyright owner(s) are credited and that the original publication in this journal is cited, in accordance with accepted academic practice. No use, distribution or reproduction is permitted which does not comply with these terms.
*Correspondence: Yuhui Wang, eXVodWlfd2FuZ0BuamF1LmVkdS5jbg==; Kailin Hu, aHVrYWlsaW5Ac2NhdS5lZHUuY24=
†These authors have contributed equally to this work
Disclaimer: All claims expressed in this article are solely those of the authors and do not necessarily represent those of their affiliated organizations, or those of the publisher, the editors and the reviewers. Any product that may be evaluated in this article or claim that may be made by its manufacturer is not guaranteed or endorsed by the publisher.
Research integrity at Frontiers
Learn more about the work of our research integrity team to safeguard the quality of each article we publish.