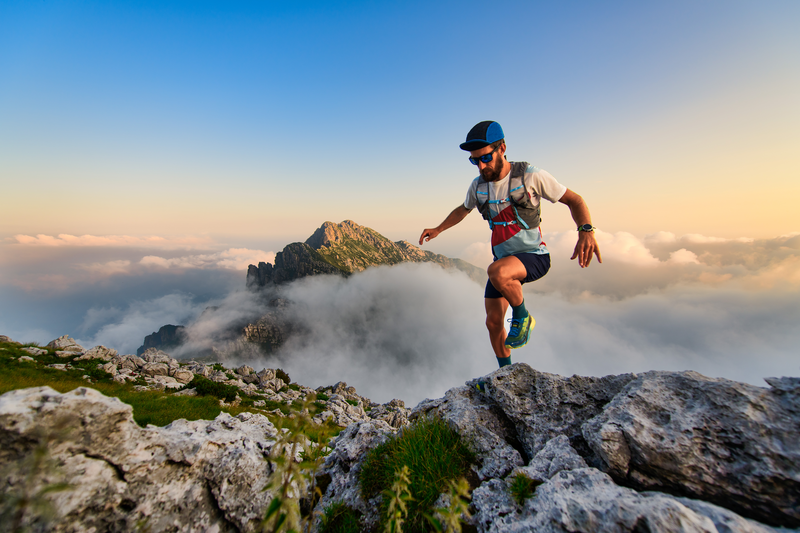
95% of researchers rate our articles as excellent or good
Learn more about the work of our research integrity team to safeguard the quality of each article we publish.
Find out more
ORIGINAL RESEARCH article
Front. Plant Sci. , 26 January 2023
Sec. Plant Development and EvoDevo
Volume 14 - 2023 | https://doi.org/10.3389/fpls.2023.1120820
This article is part of the Research Topic Continuous Flowering: A much needed Ornamental Revolution for Floricultural Crops View all 8 articles
Hybridization is an important evolutionary mechanism ubiquitous to plants. Previous studies have shown that hybrid polyploidization of cultivated chrysanthemum, ‘Zhongshanzigui’, and Leucanthemum paludosum exhibit spring-flowering traits. This study explores the function of the LpFTLs gene via the phenotype of A. thaliana after heterologous transformation of the LpFTLs gene, and analyzes the mechanism ofthe continuous flowering phenotype and heterosis of hybrid offspring. The results suggest that the flowering phenotype of hybrid offspring in spring may be related to the expression of the LpFTLs gene. Ectopic expression of Leucanthemum paludosumLpFTLs in Arabidopsis thaliana resulted in earlier flowering, indicating that the LpFTLs gene also affects the flowering time in L. paludosum. Compound expression of FTLs in C. morifolium × L. paludosum intergeneric hybridization directly leads to serious heterosis in the hybrid offspring. Moreover, continuous flowering appears to be accompanied by hybrid weakness under the balance of vegetative and reproductive growth. Therefore, in future studies on chrysanthemum breeding, a suitable balance point must be established to ensure the target flowering time under normal growth.
Floricultural crops are an integral part of our lives, with uses ranging from aesthetics to food and medicine. Seasonal flowering is an established pattern of floriculture crops, in which flowering occurs in a specific season each year. Chrysanthemum morifolium is one of the four most important ornamental species in cut-flowers worldwide, and its diversity with respect to growth habit, inflorescence form, and color ensures its economic importance, not only for its ornamental value but also for extensive applications in people’s daily lives (Higuchi and Hisamatsu, 2015; Bernardini et al., 2022). C. morifolium is a qualitative short-day flowering plant and there is no spring flowering in the first half of the year under natural production conditions. To maintain annual production, the flowering of C. morifolium should be controlled by photoperiod using lights, which may result in wasting energy (Fukai, 2014; Kang et al., 2019).
Although there are a variety of technical improvements, hybridization breeding remains the most effective way to develop new varieties. Hybridization is an important source of genetic variation and functional evolution and is presently recognized as having extensive evolutionary and ecological implications for promoting abundance, distribution, and rapid adaptive evolution (Barton, 2001; Paun et al, 2007). Increasing evidence indicates that the evolution of plant species is an ongoing, dynamic process that originated multiple times through hybridization and polyploidization (Soltis et al, 1993; Soltis and Soltis, 1999). In many species, hybrid vigor of the mature plant biomass or other genetic effects commonly enhances performance relative to their parents, an important factor for increasing the production of major crops such as wheat, maize, rice, soybean, and canola (Chen, 2010). Genetic models explaining the increased yield of hybrids consider the interaction of alleles at many loci, generating altered expression levels and patterns of gene expression (Birchler, 2015). However, there are always exceptions; weakness or disadvantage also appears occasionally in hybrid plant breeding. Hybrid weakness refers to the phenomenon which shows developmental inferiority, such as necrotic leaves, small stature, or feeble growth (Hannah et al., 2007). Furthermore, our understanding of the molecular mechanisms underlying the hybrid weakness is limited. Previous research has established that a gene regulation mechanism is a convincing tool for hybrid weakness (Chen et al., 2014). Gene locus interactions could also cause weakness in specific hybrids, as detrimental (incompatible) genetic interactions between parental alleles, may incur a fitness cost (Zhang et al., 2016; Shiragaki et al., 2019).
Leucanthemum paludosum is a small perennial plant of the Asteraceae family sown in autumn and winter, grows and blooms from early spring to late spring, and can be maintained for two to three months (Wang et al., 2014). As L. paludosum has the potential for early flowering, we aimed to transfer related traits to achieve continuous flowering in C. morifolium. Although intergeneric hybridization is difficult to achieve, in our early studies, we successfully obtained intergeneric hybrids between C. morifolium ‘zhongshanzigui’ and L. paludosum via ovule rescue (Wang et al., 2014). However, the hybrid vigor was low and weak indicating a serious disadvantage.
The switch from vegetative to reproductive growth is a key event in the life cycle of plants which is triggered by a variety of both environmental cues (notably photoperiod and temperature). A change in the environmental stimulates a network of regulatory genes, one of the most important of which is FTL. The FTL gene plays a crucial role in the flowering process of higher plants and is the signal concentration point of the flower development pathway of the reproductive organs of plants. It has a crucial regulatory role in the development of flower organs, as it can integrate the signals of different flowering pathways to regulate the flowering traits and have an impact on the flowering of the composite family, previously demonstrated in studies on the flowering physiology of chrysanthemum (Fukai, 2014; Higuchi and Hisamatsu, 2015; Wang et al., 2020). The chrysanthemum genome harbors three FT-like genes, and FTL3 is a key regulator of photoperiodic flowering (Sun et al., 2017a). The expression of FTL1 and FTL2 was downregulated, whereas that of FTL3 was upregulated under short-day (SD) conditions, and heterologous expression of CmFTL3 in the Arabidopsis thaliana ft mutant could rescue the mutant phenotype, meaning that CmFTL could compensate for the absence of FT, act as a regulator of floral transition, and respond to the photoperiod in which a similar signal network of the genes for flowering is conserved in Arabidopsis and Chrysanthemum (Oda et al., 2012a; Xiang et al., 2012; Sun et al., 2017a). It is reasonable to speculate that the FTL of L. paludosum also plays a very important role in the hybridization of C. morifolium ‘zhongshanzigui’ and L. paludosum. Nevertheless, compared with the relatively clear FTL gene function in chrysanthemum, the number and function of the flowering response in L. paludosum and the hybrid are still unclear. Therefore, in the present study, we aimed to explore the function of the LpFTLs gene via the phenotype of A. thaliana after heterologous transformation of the LpFTLs gene, and analyze the mechanism of the continuous flowering phenotype and heterosis of hybrid offspring, which could provide a reference for the purpose of flowering and the molecular mechanism of distant hybridization breeding.
The conserved plant materials C. morifolium ‘Zhongshanzigui’, L. paludosum, and their F1 hybrids (C. morifolium × L. paludosum) were collected from the Chrysanthemum Germplasm Resource Preserving Center, Nanjing Agricultural University, China. All the plants were propagated from cutting and grown in a greenhouse under natural light. The seedling, flower bud differentiation, flowering, and flowering decay stages of all plant materials were observed and observations were recorded for at least two years. Plant height and leaf area, weak stem thickness, and survival rate (60 days after transplanting) were also collected. Significance testing was performed using SPSS 20.0 (http://www-01.ibm.com/software/cn/analytics/spss/ ).
Total RNA was isolated from young, fully expanded leaves of L. paludosum using the Total RNA Isolation System (Takara Bio) following the manufacturer’s instructions. From 1μL total RNA, first-strand cDNA was synthesized using random primers and SuperScript III Reverse Transcriptase (Invitrogen). PCR amplification was performed using degenerate primers (FT-F: AYACIYTIGTIATGGTIGAYCC; FT-R: CCISWYTCICKYTGRCARTT). The PCR products were cloned using the PMD19 TA cloning kit (Takara, Japan) for confirmation by DNA sequencing. RACE PCR was then used to obtain the full-length cDNA. For the 3′ RACE reaction, the first-strand cDNA was synthesized using an oligo (dT) primer incorporating the sequence of the adaptor primer, followed by nested PCR using gene-specific primer pairs and the adaptor primer. The nested PCR adaptor primer (Abridged Anchor Primer, AAP) and the Abridged Universal Amplification Primer (AUAP) provided with the 5′ RACE System kit v2.0 (Invitrogen) were used to reamplify 5′ RACE and the internal gene-specific primer pairs to isolate full-length cDNAs of LpFTL1. A neighbor-joining tree was generated using MEGA 5 software, applying the Poisson model with gamma-distributed rates and 1000 bootstrap replicates.
Owing to the high conservation of the FTL genes (amino acid sequence identity values> 90%), LpFTL2 and LpFTL3 primer sequences were designed according to CmFTL2 and CmFTL3 (Oda et al., 2012a). The PCR products were cloned using the PMD19 TA cloning kit (Takara) and sequenced. Finally, internal gene-specific primers were designed to distinguish CmFTL2, CmFTL3, LpFTL2, and LpFTL3. The primer sequences and PCR conditions are listed in Table S1.
A total of five day fully expanded fourth and fifth leaves harvested from three plants were collected at the seedling stage (March 5-10), flower bud differentiation (April 5-10), and flower decay (June 5-10) of L. paludosum; the seedling stage (May 5-10), flower bud differentiation (August 5-10) and flower decay (November 5–10) of C. morifolium ‘Zhongshanzigui’; the seedling stage (March 5-10), flower bud differentiation (April 5-10 and August 5-10) and flower decay (November 1-5) of C. morifolium × L. paludosum.
RNA was extracted using the Total RNA Isolation System (Takara) following the manufacturer’s instructions. Before reverse transcription, total RNA was treated with RNase-free DNase I (Takara) at 37°C for 30 min to avoid genomic DNA contamination. For each sample, first-strand cDNA was synthesized using random primers and SuperScript III Reverse Transcriptase (Invitrogen) according to the manufacturer’s instructions. Quantitative real-time PCR was performed using a Mastercycler ep realplex 2 S system (Eppendorf, Germany). The cDNA was amplified using SYBR® Premix Ex TaqTM II (Takara, Japan). PCR conditions were 95°C for 2 min, followed by 40 cycles of 95°C for 30 s, 55-58°C for 30 s, and 72°C for 30 s. Expression levels of genes were calculated relative to EF1α genes using the comparative quantification analysis. The relative transcript abundance was calculated using the 2−△△Ct method. The results are presented as the mean ± SD of three replicates. The primer sequences are listed in Table S1.
The restriction sites of Sal I and Not I were added to the ends of LpFTL1 and LpFTL3, respectively. After double restriction digestion, T4 ligase was used to bind to the pENTR1A entry vector. The pENTR1A-LpFTL1 and pENTR1A-LpFTL3 entry vectors were then recombined with the pMDC43 vector using LR recombination technology to obtain pMDC43-LpFTL1 and pMDC43-LpFTL3 expression vectors.
A. thaliana (Col-0) was transformed using the floral dip method. The seeds of the T1 generation were screened and cultured on 1/2 MS + 25 mg/L Hyg plate medium, the T3 generation was obtained by self-pollination, and the zygosity of the transgene was identified using an RT-PCR assay. Then, the T3 generation seeds and the wild type (Col-0) were cultured for 3–4 weeks in a short-day light incubator (23°C/8 h light, 18°C/16 h dark, 75%-80% relative humidity) and transferred to long-day light with the conditions of 23°C/16 h light, 18°C/8 h dark. The transgenic process was repeated twice (codes C and B) to get sufficient transgenic lines. After bolting, statistical analysis was performed every two days. Photographs were taken when 60% of the transgenic lines bloomed. The data were statistically analyzed using Excel and SPSS software.
The full flowering periods of C. morifolium and L. paludosum occurred from late October to early November and late April to May, respectively. The plants of C. morifolium × L. paludosum bloomed from late April to May and late October to early November under natural conditions without any treatment (Figure S1). This means that the hybrids of C. morifolium × L. paludosum flowered twice a year, from spring to early summer and autumn to winter which shown a superimposition of parental flowering patterns. It is emphasized that the state of C. morifolium (Figure 1A) and L. paludosum (Figure 1B) was natural while only a few flowers can bloom normally in spring for two years but are accompanied by a large number of poorly developed flower buds that cannot open in the later stage (Figures 1C, D). In addition to flowering traits, the ornamental traits of the hybrid were weaker than those of their parents. The main performance criteria consisted of decreased plant height and leaf area, slow growth, weak stem thickness, and low survival rate, which showed strong hybrid weakness (Figure 1E).
Figure 1 Abnormal spring flowering phenomenon and hybrid disadvantage of (C) morifolium × L. paludosum. Florescence state of (C) morifolium (A), L. paludosum (B) and (C) morifolium × L. paludosum bloomed from April to May (C) and late October to early November (D) under natural conditions without any treatment. Plant height and leaf area, weak stem thickness, and survival rate of hybrids (E). Values are mean ± SD. The dotted line denotes the mid-parent value. Bar=5cm.
Three FT-like genes have been successfully cloned. The full-length sequences of LpFTL1, LpFTL2, and LpFTL3 cDNA contained a total of 522 nucleotides. LpFTL1, LpFTL2, and LpFTL3 are predicted to encode 174 polypeptide residues. The predicted LpFTL1, LpFTL2, and LpFTL3 protein had calculated molecular mass of 19.76 kDa, 19.79 kDa, and 19.62 kDa, and theoretical pI of 9.14, 8.07, and 7.44, respectively.
The sequences of several other FT orthologs were used to construct a phylogenetic tree (maximum likelihood method) for the FT group genes (Figure 2A). The phylogenetic tree was grouped into three major clades: FT, BFT, and TFL1. As expected, LpFTL1, LpFTL2, and LpFTL3 were classified in the FT clade. It was more closely related to CmFTL3/CsFTL3 than to the others. As shown in Figure 2B, these three sequences encoded residues for Y85 (shown in red box) and Q140 (shown in black box), which are required for the formation of an external loop, and the conserved segments (indicated by red and green lines)
Figure 2 The sequences of LpFTL1, LpFTL2, and LpFTL3. (A) Phylogenetic tree of the deduced amino acid sequences of LpFTLs and other plant species FT sequences. (B) Comparison of the predicted amino acid sequences of L. paludosum FTLs and related proteins. Y85 (shown in red box) and Q140 (shown in black box) are essential for the formation of an external loop. The red and green lines highlight the conserved segments, required for FT-like activity.
qRT-PCR was used to examine the expression patterns of FTLs. The LpFTL1 and LpFTL3 transcripts mainly accumulated during the flower bud differentiation (April), were 15.5 and 22.1 times of the seedling stage (March), respectively, and showed a sudden decrease at the flower decay stage (June) (Figure 3A). The expression levels of CmFTL1, CmFTL2, and CmFTL3 in ‘Zhongshanzigui’ are shown in Figure 3B. Three CmFTLs were expressed at low levels in the seedling (May) and flower decay (November) stages. The CmFTL3 was strongly transcribed with a 29.2 value at the key flowering gene than the leaf collected at seedling and flower bud differentiation stages (August).
Figure 3 qRT-PCR verification of FTL genes. qRT-PCR analysis of LpFTL1, LpFTL2, and LpFTL3 in L. paludosum (A), CmFTL1, CmFTL2, and CmFTL3 in C. morifolium (B) and six FTLs in C. morifolium × L. paludosum (C).
Although hybridization may affect gene expression to a certain extent, the expression of all FTLs tended to be silent during the seedling stage (March). During flower bud differentiation (April and August), the potential key flowering genes, LpFTL1/LpFTL3 and CmFTL3 transcripts, significantly increased in April and August, respectively, showing an overlay of expression modes in C. morifolium × L. paludosum (Figure 3C).
In the present study, a total of 26 and 30 independent T3 Arabidopsis lines constitutively expressing LpFTL1 and LpFTL3 were produced, and two representative Ox lines of each gene were selected from the T3 generation seeds of transgenic A. thaliana to observe for expression of phenotypic effect in LpFTL1 (Figure 4A) and LpFTL3 (Figure 4B) for Arabidopsis. Among these, FTL1-C4, FTL1-B2 Ox, FTL3-C16, and FTL3-B9 in multiple independent transgenic Arabidopsis calls showed earlier bolting and flowering than WT (Col-0) plants. The most intuitive reason is the timing of transgenic lines showing bolting or even flowering; WT Arabidopsis was still in vegetative growth and the phyllotaxy at bolting remained the same. While the number of rosette leaves was not different at flowering stage (WT VS. transgenic lines), ranging from 12 to 15 (Figure 4C). This result shows that LpFTL1 andLpFTL3 are involved in controlling flowering in Arabidopsis.
Figure 4 Appearance of 36-day-old wild-type WT, FTL1-C4, FTL1-B2 (A) and FTL3-C16, FTL3-B9 (B). Florescence state of transgenic Arabidopsis line (C). Bar=3cm.
Statistical analysis showed that the average flowering days from the sowing of LpFTL1 ox were 37.2 d and 38.9 d and those from the sowing of LpFTL3 ox were 37.4 d and 37.4 d, while that of control WT Arabidopsis was 41.50 d; the difference was more than 2.5 d, above the significance level of 0.05. The mean flowering days of the transgenic plants were significantly different from those of the control WT Arabidopsis (Figure 5).
Figure 5 Flowering time statistics of FTL1-C4, FTL1-B2, FTL3-C16, FTL3-B9. Different superscripts indicate significant differences between means at P<0.01 according to Tukey’s test. Values are mean ± SD (from three biological replications).
Hybridization is an important mechanism of genetic variation and functional evolution (Richards, 2003; Kearney, 2005). New combinations of favorable genes come from different parents, potentially resulting in heterosis. In addition to the parent phenotypes, nascent hybrids often show novel traits that do not exist in the contributing parents, such as increasing levels of heat, cold, drought tolerance, pest resistance, flowering time change, and organs allowing their offspring to thrive in harsh environments (McClintock, 1983; Paun et al., 2006; Birchler, 2015; Botet and Keurentjes, 2020). In Arabidopsis allotetraploids, the expression of A. thaliana and A. arenosa FLC loci is additive, leading to a late-flowering phenotype (Wang et al., 2006). The flowering time directly affects plant reproduction and adaptation. In our study, the natural flowering of C. morifolium was in autumn, while L. paludosum was in the spring. Intergeneric hybrids are powerful, and the only way for Chrysanthemums to put forth florescence. Although hybrids between different genera are difficult to obtain, particularly between two species with relatively distant classifications, we obtained hybrids between C. morifolium and L. paludosum. After two years of observation, we ensured that all hybrids exhibited continuous flowering phenotype, and their flower bud differentiation in April and August, which led to flowering under natural conditions without any treatment. These results were consistent with our breeding objectives.
In the analysis of FTLs gene expression in hybrids, the results clearly showed that the FTLs gene is involved in the opening of C. morifolium × L. paludosum. In the flowering process, of L. paludosum and C. morifolium hybrids, it can be tentatively concluded that the first spring flowering is mainly affected by the LpFTL1 and LpFTL3 genes from L. paludosum, whereas the second flowering in autumn is determined by the CmFTLs gene from C. morifolium. Therefore, we speculate that the introduction of LpFTLs also promotes early flowering. From the results, it can be concluded that the LpFTLs gene from L. paludosum regulates flowering and the flowering period of A. thaliana, successfully converting LpFTL1 and LpFTL3 and was 3–5 days earlier than that of WT. Some studies on the verification of the transgenic function of A. thaliana cloned from C. morifolium showed that function was super-expressed by significantly promoting the early flowering of A. thaliana (Oda et al., 2012a; Higuchi and Hisamatsu, 2015; Bernardini et al., 2022). These results are in accordance with our study and prove that the FTL gene prevalent in a variety of plants plays a significant role in regulating flowering and this gene acting on reproductive growth is also similar (Storchova et al., 2015).
After the FTL protein was identified as an anthocyanin, a large number of researchers have isolated, identified, and functionally studied FTL homologous genes from different varieties of crops such as rice, wheat, fruit (apples), vegetables (tomatoes, potatoes), and ornamental crops (orchids, orange stalks, and chrysanthemums), which have a large number of early or late flower expression (Trankner et al., 2011; Li et al., 2014; Higuchi and Hisamatsu, 2015). Among the many varieties, the rational use of interspecific hybridization, intergeneral hybridization, and transgenics will create a broader possibility of flowering regulation (Chen et al., 2014; Qi et al., 2018; Mitrofanova et al., 2020). Most ornamental chrysanthemums are short-day plants, and if the LpFTLs gene is overexpressed in a chrysanthemum, it is likely to change its original flowering characteristics, such as the phenotype of continuous flowering in offspring. However, the exogenous FTL gene and the endogenous FTL gene may have a mutual influence. In an experiment to transfer the cotton FTL gene to tobacco, data suggested that adequate levels of transgenic cotton FTL might interfere with the balance of endogenous FTL inducers and inhibitors, leading to phenotypic changes in transgenic tobacco (Faure et al., 2007). Therefore, to apply the FTL gene to the breeding of continuous flowering, it is also necessary to study species as target plants in a more detailed and clearer model.
Elucidating the molecular basis of the hybrid phenotype will contribute to our understanding of evolution and enhance crop breeding. In the present study, all hybrids exhibited a continuous-flowering phenotype. As chrysanthemum is produced by vegetative propagation, it can be cloned using cuttings, which could keep early flowering characteristics. However, success is temporary, and the growth characteristics show serious hybrid weakness compared to their parents. Therefore, in our later study, it is reasonable to expect a backcross between the hybrid and paternal ‘Zhongshanzigui’ for better ornamental traits.
A prevailing model explains how hybrid phenotypes in which deleterious parental alleles are compensated in hybrid progeny by cumulative enhancement of gene expression (Chen et al., 2014; Tikhenko et al., 2015). Although this matches with our aim to get continuous flowering as an improvement of ornamental traits, it was found in our research center that this is caused by compound expression leading to shorter vegetative growth, especially after the appearance of the first flowers in less than two months. This directly leads to the weakness of heterosis in hybrid offspring. Compared with other crops, continuous flowering is a fascinating goal for ornamental plants; however, strong growth and higher biomass are often ignored. Nevertheless, an intuitive model of continuous flowering observed in our present study appears to be accompanied by hybrid weakness under the balance of vegetative and reproductive growth. Therefore, in future chrysanthemum breeding programs, a suitable balance point must be confirmed to ensure the target flowering time is under normal growth (Figure 6).
Figure 6 Breeding target model for continuous flowering and hybrid weakness balancing vegetative and reproductive growth. A suitable balance point is to be confirmed to ensure the target flowering time under the normal growth state.
A previous study in maize documented cases of positive associations between targeted gene expression and superior phenotypes in hybrids, which is inclusive of the occurrence of hybrid weakness because the actual results can be condition-dependent (Romagnoli et al., 1990). Meanwhile, some slow growth may be due to the haploid level of each parental genome (Sun et al, 2017b). Although the model discussed above appears to be a simple genetic model, the molecular basis of hybrid weakness is usually complex and often involves incremental effects and interactions of genes at multiple loci, most of which have not been characterized, particularly in ornamental plants (Tikhenko et al., 2015). Thus, breeding for continuous flowering either through introgression or transgenics should simultaneously target multiple loci to achieve a desired level of equilibrium (Alcazar et al., 2009). This suggests the need for further identification and molecular characterization of the weakness of hybrid loci. Such findings could facilitate the development of ornamental plants to efficiently utilize hybridization.
The original contributions presented in the study are included in the article/Supplementary Material. Further inquiries can be directed to the corresponding author.
HW and FC contributed to conception and design of the study. HW, JZ, YL and ZW organized the data. HW, WF, SC, ZW, HZ and JJ performed the statistical analysis. HW and XW wrote the first draft of the manuscript. CM and ZL wrote sections of the manuscript. KZ, HW, SC, ZL, JJ, WF, and FC contributed to writing-review and editing. All authors contributed to the article and approved the submitted version.
This work was supported by Natural Science Foundation of Jiangsu Province (BK20211215), the Fundamental Research Funds for the Central Universities (KYCXJC2022001) and the interdiscipline project of College of Horticulture, Nanjing Agricultural University (YYJC202102).
The authors declare that the research was conducted in the absence of any commercial or financial relationships that could be construed as a potential conflict of interest.
All claims expressed in this article are solely those of the authors and do not necessarily represent those of their affiliated organizations, or those of the publisher, the editors and the reviewers. Any product that may be evaluated in this article, or claim that may be made by its manufacturer, is not guaranteed or endorsed by the publisher.
The Supplementary Material for this article can be found online at: https://www.frontiersin.org/articles/10.3389/fpls.2023.1120820/full#supplementary-material
Supplementary Figure 1 | Flowering time of the hybrid and their parents.
Alcazar, R., Garcia, A. V., Parker, J. E. (2009). Incremental steps toward incompatibility revealed by arabidopsis epistatic interactions modulating salicylic acid pathway activation. Proc. Natl. Acad. Sci. United States America 106, 334–339.
Bernardini, C., Santi, S., Mian, G., Levy, A., Buoso, S., Suh, J. H., et al. (2022). Increased susceptibility to chrysanthemum yellows phytoplasma infection in Atcals7ko plants is accompanied by enhanced expression of carbohydrate transporters. Planta 43, 256.
Botet, R., Keurentjes, J. J. B. (2020). The role of transcriptional regulation in hybrid vigor. Front. Plant Sci. 11, 410.
Chen, Z. J. (2010). Molecular mechanisms of polyploidy and hybrid vigor. Trends Plant Sci. 15, 57–71.
Chen, C., Chen, H., Lin, Y. S., Shen, J. B., Shan, J. X., Qi, P., et al. (2014). A two-locus interaction causes interspecific hybrid weakness in rice. Nat. Commun. 5, 3357.
Faure, S., Higgins, J., Turner, A., Laurie, D. A. (2007). The FLOWERING LOCUS T-like gene family in barley (Hordeum vulgare). Genetics 176, 599–609.
Fukai, S. (2014). Flowering physiology of chrysanthemum. Int. Symposium Orchids Ornamental Plants 1025, 135–142.
Hannah, M. A., Kramer, K. M., Geffroy, V., Kopka, J., Blair, M. W., Erban, A., et al. (2007). Hybrid weakness controlled by the dosage-dependent lethal (DL) gene system in common bean (Phaseolus vulgaris) is caused by a shoot-derived inhibitory signal leading to salicylic acid-associated root death. New Phytol. 176, 537–549.
Higuchi, Y., Hisamatsu, T. (2015). CsTFL1, a constitutive local repressor of flowering, modulates floral initiation by antagonising florigen complex activity in chrysanthemum. Plant Sci. 237, 1–7.
Kang, D. R., Dai, S. L., Gao, K., Zhang, F., Luo, H. (2019). Morphological variation of five cut chrysanthemum cultivars induced by 5-azacytidine treatment. Hortscience 54, 1208–1216.
Kearney, M. (2005). Hybridization, glaciation and geographical parthenogenesis. Trends Ecol. Evol. 20, 495–502.
Li, C. Q., Luo, L., Fu, Q. T., Niu, L. J., Xu, Z. F. (2014). Isolation and functional characterization of JcFT, a FLOWERING LOCUS T (FT) homologous gene from the biofuel plant jatropha curcas. BMC Plant Biol. 14, 125.
McClintock, B. (1983). The significance of responses of the genome to challenge. hysiology Or Med. Literature Peace Economic Sci. 226, 792-801.
Mitrofanova, O. V., Lesnikova-Sedoshenko, N. P., Ivanova, N. N., Smykova, N. V., Mitrofanova, I. V. (2020). In vitro propagation and preservation of promising chrysanthemum cultivars and hybrid forms. Acta Horticulturae. 1285, 139–145.
Oda, A., Narumi, T., Li, T., Kando, T., Higuchi, Y., Sumitomo, K., et al. (2012a). CsFTL3, a chrysanthemum FLOWERING LOCUS T-like gene, is a key regulator of photoperiodic flowering in chrysanthemums. J. Exp. Bot. 63, 1461–1477.
Paun, O., Fay, M. F., Soltis, D. E. (2007). Genetic and epigenetic alterations after hybridization and genome doubling. Taxon 56, 649–656.
Paun, O., Stuessy, T. F., Horandl, E. (2006). The role of hybridization, polyploidization and glaciation in the origin and evolution of the apomictic ranunculus cassubicus complex. New Phytol. 171, 223–236.
Qi, X. Y., Wang, H. B., Song, A. P., Jiang, J. F., Chen, S. M., Chen, F. D. (2018). Genomic and transcriptomic alterations following intergeneric hybridization and polyploidization in the chrysanthemum nankingenseXTanacetum vulgare hybrid and allopolyploid (Asteraceae). Horticulture Res. 5, 5.
Richards, A. J. (2003). Apomixis in flowering plants: an overview. Philos. Trans. R Soc. Lond B Biol. Sci. 358, 1085–1093.
Romagnoli, S., Maddaloni, M., Livini, C., Motto, M. (1990). Relationship between gene-expression and hybrid vigor in primary root-tips of young maize (Zea-mays l) plantlets. Theor. Appl. Genet. 80, 769–775.
Shiragaki, K., Iizuka, T., Ichitani, K., Kuboyama, T., Morikawa, T., Oda, M., et al. (2019). HWA1- and HWA2-mediated hybrid weakness in rice involves cell death, reactive oxygen species accumulation, and disease resistance-related gene upregulation. Plants-Basel 8, 450.
Soltis, D. E., Soltis, P. S. (1999). Polyploidy: recurrent formation and genome evolution. Trends Ecol. Evol. 14, 348–352.
Soltis, D., Soltis, P., Rieseberg, D. L. H. (1993). Molecular data and the dynamic nature of polyploidy. Crit. Rev. Plant Sci. 12, 243–273.
Storchova, H., Drabesova, J., Chab, D., Kolar, J., Jellen, E. N. (2015). The introns in FLOWERING LOCUS T-LIKE (FTL) genes are useful markers for tracking paternity in tetraploid chenopodium quinoa willd. Genet. Resour. Crop Evol. 62, 913–925.
Sun, J., Wang, H., Ren, L., Chen, S., Chen, F., Jiang, J. (2017a). CmFTL2 is involved in the photoperiod- and sucrose-mediated control of flowering time in chrysanthemum. Horticulture Res. 4, 17001.
Sun, S., Wu, Y., Lin, X. Y., Wang, J., Yu, J. M., Sun, Y., et al. (2017b). Hybrid weakness in a rice interspecific hybrid is nitrogen-dependent, and accompanied by changes in gene expression at both total transcript level and parental allele partitioning. PloS One 12, 72919.
Tikhenko, N., Rutten, T., Tsvetkova, N., Voylokov, A., Borner, A. (2015). Hybrid dwarfness in crosses between wheat (Triticum aestivum l.) and rye (Secale cereale l.): a new look at an old phenomenon. Plant Biol. 17, 320–326.
Trankner, C., Lehmann, S., Hoenicka, H., Hanke, M. V., Fladung, M., Lenhardt, D., et al. (2011). Note added in proof to: Over-expression of an FT-homologous gene of apple induces early flowering in annual and perennial plants. Planta 233, 217–218.
Wang, H. B., Jiang, J. F., Chen, S. M., Qi, X. Y., Fang, W. M., Guan, Z. Y., et al. (2014). Rapid genetic and epigenetic alterations under intergeneric genomic shock in newly synthesized chrysanthemum morifolium x leucanthemum paludosum hybrids (Asteraceae). Genome Biol. Evol. 6, 247–259.
Wang, L. J., Sun, J., Ren, L. P., Zhou, M., Han, X. Y., Ding, L., et al. (2020). CmBBX8 accelerates flowering by targeting CmFTL1 directly in summer chrysanthemum. Plant Biotechnol. J. 18, 1562–1572.
Wang, J., Tian, L., Lee, H. S. (2006). Nonadditive regulation of FRI and FLC loci mediates flowering-time variation in arabidopsis allopolyploids. Genetics 173, 965–974.
Xiang, L., Li, X., Qin, D., Guo, F., Wu, C., Miao, L., et al. (2012). Functional analysis of FLOWERING LOCUS T orthologs from spring orchid (Cymbidium goeringii rchb. f.) that regulates the vegetative to reproductive transition. Plant Physiol. Biochem. 58, 98–105.
Keywords: hybridization, chrysanthemum, FTLs, hybrid weakness, continuous flowering
Citation: Li Z, Mao C, Wu X, Zhou H, Zhao K, Jiang J, Chen S, Fang W, Guan Z, Zhang J, Liao Y, Wang Z, Chen F and Wang H (2023) Hybrid weakness and continuous flowering caused by compound expression of FTLs in Chrysanthemum morifolium × Leucanthemum paludosum intergeneric hybridization. Front. Plant Sci. 14:1120820. doi: 10.3389/fpls.2023.1120820
Received: 10 December 2022; Accepted: 12 January 2023;
Published: 26 January 2023.
Edited by:
Tangchun Zheng, Beijing Forestry University, ChinaReviewed by:
Xiang Gao, Northeast Normal University, ChinaCopyright © 2023 Li, Mao, Wu, Zhou, Zhao, Jiang, Chen, Fang, Guan, Zhang, Liao, Wang, Chen and Wang. This is an open-access article distributed under the terms of the Creative Commons Attribution License (CC BY). The use, distribution or reproduction in other forums is permitted, provided the original author(s) and the copyright owner(s) are credited and that the original publication in this journal is cited, in accordance with accepted academic practice. No use, distribution or reproduction is permitted which does not comply with these terms.
*Correspondence: Haibin Wang, aGJAbmphdS5lZHUuY24=
†These authors have contributed equally to this work
Disclaimer: All claims expressed in this article are solely those of the authors and do not necessarily represent those of their affiliated organizations, or those of the publisher, the editors and the reviewers. Any product that may be evaluated in this article or claim that may be made by its manufacturer is not guaranteed or endorsed by the publisher.
Research integrity at Frontiers
Learn more about the work of our research integrity team to safeguard the quality of each article we publish.