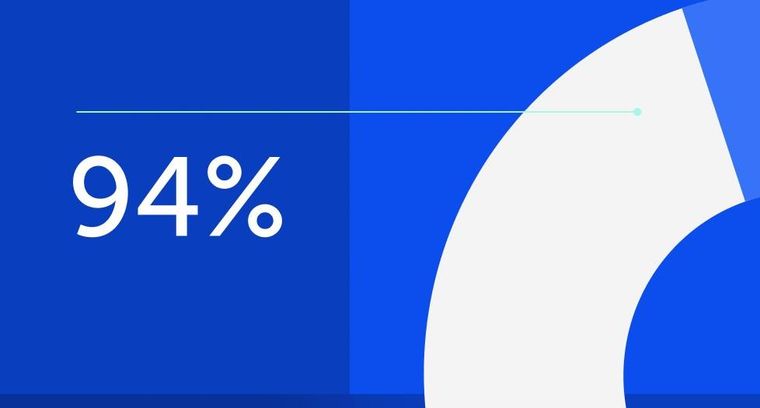
94% of researchers rate our articles as excellent or good
Learn more about the work of our research integrity team to safeguard the quality of each article we publish.
Find out more
ORIGINAL RESEARCH article
Front. Plant Sci., 03 February 2023
Sec. Plant Pathogen Interactions
Volume 14 - 2023 | https://doi.org/10.3389/fpls.2023.1087496
This article is part of the Research TopicThe Interaction of Biotic and Abiotic StressesView all 5 articles
Cucumber angular leaf spot (ALS) disease, caused by Pseudomonas amygdali pv. lachrymans (Pal), is an emerging disease with a high incidence that causes severe damage to cucumber worldwide. Bacterial aerosols play a crucial role in the epidemiology of greenhouse ALS disease. However, little is known about the influence of temperature and relative humidity (RH) on the dynamics of Pal in aerosols. A study was conducted to investigate the relationships between the concentration of Pal aerosols and their dependence on temperature and RH in aerosol chambers and greenhouses. The results demonstrated that temperature and RH are both significant factors influencing the release amount, survival time and infectivity of Pal in aerosols, while RH has a greater influence on particle size than temperature across the range of conditions tested. The release amount and survival time of Pal in aerosols under high RH (95%) and low temperature (≤ 25°C) conditions were significantly higher than those under low RH (35%) and high temperature (35°C) conditions. The highest release amount of Pal aerosol (96 CFU/m3) and highest survival rate (98.41%) were found at 18°C and 95% RH, while the highest disease index (DI = 60.9) caused by Pal aerosol was found at 25°C and 95% RH. In addition, Pal aerosols presented a larger diameter (4.7->7.0 μm) under high RH (95% RH) than under dry conditions (≤ 65% RH). These findings will play a crucial role in elucidating the influence of environmental parameters on the dynamics and transmission of Pal in aerosols. Based on our findings, preliminary recommendations for controlling airborne Pal spread involve controlling air temperature and RH, which will contribute to the effective alleviation and control of cucumber ALS disease.
Cucumber is one of the most important vegetable crops in many countries worldwide. Statistically, China is the largest cucumber producer in the world, with 1.27 Mha of cultivation area and 73.36 million tons of production in 2020 (FAOSTAT, 2020). In the past ten years, cucumber angular leaf spot (ALS) disease, which is caused by Pseudomonas amygdali pv. Lachrymans (Pal), has become one of the most important causes of cucumber loss worldwide (Bhat et al., 2010; Gomila et al., 2017; Arnold and Preston, 2019; Peix et al., 2018; Li et al., 2019). In addition, Pal can attack a wide range of cucurbits, including Citrullus lanatus (watermelon), C. melo (muskmelon), Cucurbita maxima (squash) and C. pepo (pumpkin) (Olczak-Woltman et al., 2009; Bhat et al., 2010; Li et al., 2019). The traditional route of transmission of the globally distributed Pal has been considered to be soil-borne, seed-borne, wind, rain and insect-borne transmission (Leben, 1981; Umekawa et al., 1981; Groth et al., 1995; Hirano et al., 1996; Hansen, 2009). Recently, we showed that Pal aerosols represent an effective mechanism for the spread of ALS disease in greenhouses, and cucumber plant canopies at the early stage of disease development serve as the main source of Pal aerosol (Chai et al., 2020).
Bioaerosols may play an important role in the transmission of pathogens to plants, which can lead to epidemic outbreaks (Allegra et al., 2016; Joung et al., 2017). Bioaerosol can be generated by overhead irrigation in greenhouses, raindrops hitting soil and mechanical crushing of diseased plants in fields (Quinn et al., 1980; Joung et al., 2017). The transmission of pathogenic bacteria by aerosols is potentially influenced by many factors, including the concentration and particle size distribution of bacteria-laden particles, how long airborne bacteria remain infectious under different environmental conditions, which can affect the concentration and viability.
Environmental conditions, including temperature, relative humidity (RH), UV radiation, and air flow, profoundly affect the survival of airborne microorganisms, among which temperature and RH are the major factors affecting bacterial survival in aerosol (Webb, 1961; Moe and Harper, 1983; Ariya and Amyot, 2004; Thompson et al., 2011; Hoeksma et al., 2015; Afsharnia et al., 2018; Islam et al., 2020). Temperature directly affects the metabolism and reproduction of the bacteria, and RH affects the concentration of the ambient bacteria, and these findings are dependent on species and metabolic factors (Burrows et al., 2009; Thompson et al., 2011). For example, the viability of Escherichia coli in aerosols decreases with the decline in RH, and the death rates of E. coli at 30°C were approximately four times faster than those at 15°C at the same RH (Wathes et al., 1986). Various species of bacteria have shown distinctly different adaptations to different levels of temperature and RH. RH have been reported to have lethal effects on the survival of some species in air, e.g., E. coli (Wathes et al., 1986), Legionella pneumophila (Hambleton et al., 1983) and Yersinia pestis (Gut et al., 2016); while the survival of Staphylococcus epidermidis (Thompson et al., 2011) was demonstrated to not be affected by RH. It was reported that the survival of P. syringae in aerosols was greatest at 70%-80% RH and 12°C in a field environment (Marthi et al., 1990). However, little is known about the influence of environmental parameters on the survival of Pal aerosols in greenhouses.
Temperature and RH play important roles in the emission and transmission of bioaerosols as well as in bacterial disease outbreaks (Harrison, 1980; Xin et al., 2016; Huot et al., 2017; Kowalski and Pastuszka, 2018). Plant canopies are considered a source of airborne bacteria (Lindemann et al., 1982; Lindemann and Upper, 1985; Mcinnes et al., 1988). The plant phyllosphere provides an important habitat for microorganisms to colonize and proliferate (Melotto et al., 2008; Zeng et al., 2010; Xin et al., 2016). Some studies have shown that the populations of P. syringae on the leaf surface increased by orders of magnitude after heavy rain, which caused an increasing number of bacterial cells to be transferred to the atmosphere (Hirano and Christen, 2000; Bigg et al., 2015). The dispersal of bacterial aerosols and deposition on healthy plants will accelerate an epidemic of bacterial disease. In addition, phyllosphere bacterial disease outbreaks usually require a period of high RH (Aung et al., 2018), and a suitable temperature promotes the virulence of bacterial pathogens and the interaction between plants and pathogens (Xin et al., 2016; Aguilera et al., 2017). However, studies have not been undertaken to investigate the dynamics of airborne Pal present in cucumber greenhouses and their dependence on temperature and RH.
The size distribution of bioaerosols varies systematically with air temperature and RH, which could affect the behavior of bioaerosols in air. The particle size of bioaerosols has been shown to increase by hygroscopic growth, e.g., coronavirus aerosols presented a larger diameter at 25°C and 79% RH than at 38°C and 24% RH (Liao and Luo, 2005; Pyankov et al., 2018). The particle size as well as the motion of aerosols, such as resuspension, transport by air movement and sedimentation, have been shown to influence the possibility of microbes entering the atmosphere (Liao and Luo, 2005). For example, dust of 30 and 100 μm is most likely to be raised from the surface to the atmosphere (Chamberlain, 1975). In addition, the survivability of a biological particle in the atmosphere will also be affected by its size (Jones and Harrison, 2004). The concentrations of E. coli in PM10, PM2.5 and PM1 are higher in wet aerosols than in dry aerosols (Hoeksma et al., 2015).
Previous studies have shown that the effects of temperature and RH on the release and survival of bacterial aerosols are distinct based on the various species of bacteria. However, little is known about the influence of temperature and RH on the survival, release and pathogenicity of Pal aerosols. In the present study, we extend the findings of our previous study to (i) assess the survival the size distribution of Pal aerosols under different temperature and RH levels; (ii) evaluate the release of Pal aerosols from diseased cucumber in aerosol chambers and greenhouse environments under different temperature and RH levels; and (iii) investigate the infectivity of Pal aerosols to cucumber seedlings at different temperature and RH levels. These data will provide a foundation for the effective alleviation and control of ALS disease by controlling temperature and RH.
A green fluorescence protein gene (GFP)-marked Pal strain (Pal::GFP), which showed consistent fluorescence and was indistinguishable from the wild-type strain in growth rate and pathogenicity, was used in this study (Chai et al., 2020).
The Pal::GFP strain was grown at 28°C for 48 h on nutrient agar (NA) plates (10.0 g of peptone, 3.0 g of beef powder, 5.0 g of NaCl and 15.0 g of agar per liter; pH 7.0; Solarbio Science & Technology Co., Ltd., Beijing, China) containing 50 μg/mL kanamycin (referred to as NK). Then, single colonies were selected and cultured in nutrient broth (NB) medium (containing 10.0 g of peptone, 3.0 g of beef powder and 5.0 g of NaCl per liter; pH 7.0) supplemented with 50 μg/mL kanamycin for 24 h at 28°C with shaking at 200 rpm. The concentration of the Pal::GFP suspension was determined by the optical density at 600 nm (OD600 = 0.8, approximately 1 × 108 CFU/mL).
The experimental cucumber variety ‘Zhongnong no. 6’ (China Vegetable Seed Technology Co., Ltd., Beijing), a typical Huabei-type cucumber of northern China, was used in this study. The healthy cucumber seeds used in this study were first confirmed to be free of Pal by the traditional agar planting method and PCR using the primer pair Pslgap1-F/R as described by Meng et al., 2016.
Environmentally controlled aerosol chambers comprising organic glass with a size of 70 cm × 60 cm × 60 cm (length × width × height) were used in this study (Figure S1). The air temperature and RH in the aerosol chamber were controlled by an air conditioner (Haier, KFRd-27N/PAA12, China) and an air humidifier (Yadu, SC-EB35B, China), respectively, to maintain constant temperature and RH conditions (Chai et al., 2020). The collision nebulizer (BGI, Inc., Waltham, MA) and the Andersen sampler (Thermo-Andersen, Smyrna, GA, USA) were connected to the air inlet and outlet, which were located on the left and right sidewalls of the aerosol chamber, respectively. A fan and a UV light were installed at the top wall for mixing the Pal aerosol well inside the chamber and sterilization, respectively. The door was fixed at the front wall with six screws, and a silicone pad was applied to ensure that the chamber was airtight.
Twenty cucumber seedlings with two true leaves were inoculated with a 108 CFU/mL suspension of Pal::GFP by spraying and incubated at 90% RH and 25°C for 48 h for the development of ALS disease (Figures 1A, B). Then, the infected cucumber seedlings at the early stage of ALS disease were transferred to nine aerosol chambers, which were maintained at three temperatures (18, 25 and 35°C) with three humidity levels (35%, 65% and 95% RH) per temperature. A total of nine temperature-humidity combinations were tested. The release of Pal::GFP aerosol from infected cucumber seedlings was detected at 24 h after incubation at different levels of temperature and RH. The Andersen sampler (Thermo-Andersen, Smyrna, GA, USA) was used to collect Pal::GFP aerosol with a flow of 28.3 L/min for 10 min. For each treatment, the bioaerosol samples were collected onto 90 mm diameter NK plates and then cultured at 28°C for 48 h, and the Pal::GFP colony-forming units (CFU) were counted (Figure S2). The experiments were independently repeated three times.
Figure 1 Effect of temperature and relative humidity (RH) on the release of Pseudomonas amygdali pv. lachrymans (Pal) in aerosols from infected cucumber plants. Twenty cucumber plants at the early stage of the angular leaf spot (ALS) disease, were placed in nine exposure chambers, which maintained at temperatures of 18, 25 and 35°C with humidity levels of 35%, 65% and 95% RH per temperature, respectively. (A), Cucumber plants inoculated with sterile water. (B), Symptoms on cucumber plants at the early stage of the ALS disease, which inoculated with Pal::GFP suspension. (C), The release amount of Pal::GFP in aerosols, which detected at 24 h after incubation under different conditions of temperature and RH levels. The experiments were independently repeated three times. Columns and error bars represent the mean values and standard deviations (SDs) from three replicates. Columns labeled with different letters indicate statistically significant differences (p< 0.05).
A 108 CFU/mL suspension of Pal::GFP was aerosolized by a six-jet Collison nebulizer (BGI, Inc., USA), at a flow rate of 12 L/min for 30 min, to nine aerosol chambers, in which different levels of temperature (18, 25 and 35°C) and RH (35%, 65% and 95% RH) were maintained. Aerosol samples were collected at 0, 5, 15, 30, 45, 60 and 75 min after aerosolization. A six-stage Andersen sampler (Andersen Inc, USA) with a flow rate of 28.3 L/min was used to collect the aerosol samples onto 90 mm diameter NK plates, which were then incubated at 28°C for 48 h, and the Pal::GFP CFUs were counted to evaluate the initial concentration, survival dynamics and particle size distribution of Pal aerosols. The experiments were repeated three times.
The initial concentration of Pal::GFP aerosol at different levels of temperature and RH was determined with aerosol samples collected at 0 min after nebulization. The size distribution of Pal::GFP aerosols at different levels of temperature and RH was assessed by an Andersen sampler based on their inertia-related aerodynamic diameter (Xu et al., 2013). The survival dynamics and decay rate of Pal::GFP in aerosols at different levels of temperature and RH were calculated using the first-order decay model according to Eq. (1) (Clifton et al., 2010). In this model, the decay rate k represents the combined effect of natural biological decay arising from the loss of bacterial viability and the effect of particle deposition on the chamber surfaces. The half-life was calculated according to Eq. (2), and the value of k was obtained from Eq. (1), which was determined by the Pal::GFP aerosol decay experiment. Survival curves for Pal::GFP in aerosols generated in aerosol chambers under different environmental conditions were obtained using GraphPad Prism 7.0 (GraphPad Software, Inc.).
The effects of temperature and RH on the infectivity of Pal::GFP aerosol to cucumber seedlings were examined in nine independent aerosol chambers (Figure S1) in which twenty healthy cucumber seedlings were placed in advance, and the chambers were maintained at temperatures of 18, 25 and 35°C with humidity levels of 35%, 65% and 95% RH. A Pal::GFP suspension (108 CFU/mL) was used to generate aerosol by the six-jet Collison nebulizer at a flow rate of 12 L/min for 2 h. The mixing fan was run at an average velocity of 0.4 m/s during the period of nebulization. The disease index (DI) of cucumber ALS disease were examined 7 days after exposure to Pal::GFP aerosol according to the method described previously (Meng et al., 2016).
The populations of Pal::GFP colonized on symptomatic cucumber leaves were also determined. Briefly, three infected leaves were sampled from each cucumber plant, and four leaf discs (1.0 cm in diameter) were taken using a cork borer. The leaf discs were surface sterilized for 1 min in 75% ethanol, rinsed three times in sterile water (Xin et al., 2016), homogenized in 1 mL sterile water using a mortar and serially diluted and plated on NK plates to determine the CFU (Figure S2). After incubation at 28°C for 48 h, the number of colonies was counted and normalized to the value per cm2 of leaves (Rajendran et al., 2016). The experiments were independently repeated three times.
To determine the effect of temperature and RH on the concentration of Pal aerosol and disease severity in cucumber greenhouses, aerosol samples were collected from nine greenhouses where cucumber ALS disease occurred naturally from 2019 to 2021. The infested greenhouses were located in Wuqing district, Tianjin city (greenhouses I and II); Guantao county, Hebei province (greenhouses III, IV, and V); and Shouguang county, Shandong province (greenhouses VI, VII, VIII, and IX).
A portable sampler (HighBioTrap, Beijing Blue Tech, Inc., Beijing, China) was used to sample aerosol at 1.5 m above the ground in the middle of the greenhouses. The sampler was operated at a flow rate of 1000 L/min, with an impact velocity of approximately 10.2 m/s (S/W = 1.5, T/W = 1) and a cutoff size of ~ 2 μm (Chen and Yao, 2018). For each sampling greenhouse, bioaerosols were collected onto 90 mm (diameter) sterilized aluminum membranes coated with 600 μL of mineral oil. Three independent aerosol samples were collected from 9:00 am to 10:00 am daily for three continuous days and served as three biological replicates. A total of 27 air samples were collected from nine greenhouses. Then, the aerosols were assessed by quantitative PCR (qPCR). Briefly, the mineral oil membrane with aerosols was immediately transferred to a 50 mL centrifuge tube and washed using 3 mL of Tween 20 suspension. Then, the obtained oil-in-water emulsion was centrifuged at 8000 rpm to remove the mineral oil. The pellet remaining in the centrifuge tube was then used for genomic DNA extraction with a TIANamp Bacteria DNA Kit (Tiangen Biotech, Inc., Beijing, China) according to the manufacturer’s guidelines. The concentration of Pal in aerosols was detected by qPCR with the primer pair Pslgap1-F/R according to the protocol described in our previous work (Meng et al., 2016; Chai et al., 2020). The DIs of cucumber ALS disease were also recorded. The temperature and humidity data in greenhouses were obtained using a digital thermohygrometer (DL-WS 210, Gsome Technology Co., Ltd, Hangzhou, China).
Due to the nonnormal distribution of variables describing the concentration of Pal in aerosols under various temperature and RH environments, a nonlinear regression model was assumed and constructed using Origin software (OriginLab, version 8.0). A 3D surface plot (Kowalski and Pastuszka, 2018) was used to determine and visualize the influence of temperature and RH on the concentration levels of Pal in aerosols.
One-way ANOVA and Duncan’s test were used for statistical analysis by SPSS 22.0 software, and p values < 0.05 indicated a statistically significant difference.
Cucumber seedlings at the early stage of ASL disease (Figure 1B) were incubated for 24 h in nine aerosol chambers, which were maintained at temperatures of 18, 25 and 35°C with humidity levels of 35%, 65% and 95% RH, and the concentrations of Pal::GFP aerosol were examined. The results demonstrate that temperature and RH are both significant factors influencing the release of Pal::GFP aerosols from diseased cucumber. High humidity (95% RH) at comparatively low temperature (18°C) is conducive to the release of Pal::GFP aerosols. The highest release amount of Pal::GFP aerosol (96 CFU/m3) from diseased cucumber was found at 18°C and 95% RH, while the lowest concentrations of Pal::GFP aerosol (<10 CFU/m3) were detected at relatively high temperature (25°C and 35°C) and low humidity (35% RH and 65% RH). Under dry conditions (≤ 65% RH), the amount of Pal::GFP aerosols released from cucumber seedlings was significantly lower than that under high RH conditions (95% RH). However, the lower amount of Pal::GFP aerosols released under dry conditions (≤ 65% RH) could be improved by reducing the temperature from high temperature (25°C and 35°C) to low temperature (18°C), with Pal::GFP aerosol concentrations increasing from< 10 CFU/m3 to 84 CFU/m3 (Figure 1C).
Pal::GFP was aerosolized to nine aerosol chambers, which were maintained at 18, 25 and 35°C with three humidity levels of 35%, 65% and 95% RH, and Pal::GFP concentrations were examined from 0 to 75 min. The initial concentrations of Pal::GFP in aerosols, which were examined 0 min after nebulization, were largely affected by RH, showing a downtrend with the decrease in humidity levels at the same temperature (Figure 2). The highest initial concentrations of Pal::GFP in aerosols were detected at 95% RH, with 4040 ± 590 CFU/m3 at 18°C and 4338 ± 260 CFU/m3 at 35°C. The lowest initial concentrations of Pal::GFP aerosol were detected at 35% RH, with 1584 ± 291 CFU/m3 at 18°C and 634 ± 305 CFU/m3 at 35°C. Inexplicably, the initial concentrations of Pal::GFP in aerosols generated at 25°C were always lower than those in aerosols generated at 18°C and 35°C at the same RH levels.
Figure 2 Effect of temperature and relative humidity (RH) on the initial concentration of Pseudomonas amygdali pv. lachrymans (Pal) in aerosols after aerosolization. Pal::GFP suspension (108 CFU/mL) was aerosolized to nine aerosol chambers, which maintained at 18, 25 and 35°C with three humidity levels of 35%, 65% and 95% RH per temperature, respectively, and the initial concentrations of Pal::GFP in aerosols were examined 0 min after nebulization. The experiments were independently repeated three times. Columns and error bars represent the mean values and standard deviations (SDs) of three replicates. Columns labeled with different letters indicate statistically significant differences (p< 0.05).
Generally, the decay rate of Pal::GFP in aerosols showed a decreasing trend with rising temperature and falling RH (Figure 3). Pal::GFP in aerosols decayed exponentially with a calculated decay rate of 0.0881-0.1192 min-1, corresponding to a half-life of 1.98-6.79 min (Table 1). The fastest decay rate (0.0881 min-1) of Pal::GFP in aerosols was detected at 35°C and 35% RH, corresponding to a half-life of 1.98 min (Table 1, Figure 3C). The slowest decay rate of Pal::GFP in aerosols was detected at 95% RH, corresponding to half-lives of 6.27 min at 35°C and 6.79 min at 25°C, respectively (Table 1, Figure 3A).
Figure 3 Effect of temperature and relative humidity (RH) on the survival rate of Pseudomonas amygdali pv. lachrymans (Pal) in the aerosol chambers. (A) 95% RH, (B) 65% RH and (C) 35% RH. The survival rates of Pal::GFP aerosol were determined at 0, 5, 15, 30, 45, 60 and 75 min after aerosolization, respectively. Error bars represent the standard deviations (SDs) of three replicates.
Table 1 Effect of temperature and relative humidity (RH) on Pseudomonas amygdali pv. lachrymans (Pal) survival in aerosols.
The survival rate of Pal::GFP in aerosols decreased significantly during the first 15 min after aerosolization, and few Pal::GFP cells remained at 75 min after aerosolization. Temperature was found to be a crucial factor that influenced the survival dynamics of Pal in aerosols. A significant decrease (p < 0.05) in the Pal survival rate was observed as the temperature increased from 18°C to 35°C at the same RH levels. At 95% RH conditions, a larger decay was observed within 5 min after aerosolization at 35°C, with a survival rate of 1.33%, which was significantly lower (p < 0.05) than that at 18°C (98.41%) and 25°C (27.42%). A similar trend was also obtained at 65% RH and 35% RH; as the temperature increased from 18°C to 35°C at 5 min, the survival rate decreased from 22.88% to 3.15% and 35.04% to 5.46%, respectively (Figure 3). In addition, the decay rate of Pal::GFP increased as the RH decreased.
The size distributions of Pal::GFP aerosols under different temperature (18, 25 and 35°C) and humidity (35%, 65% and 95% RH) conditions were analyzed according to the inertia-related aerodynamic diameter of the six-stage Andersen sampler. Generally, RH played a critical role in the particle size distribution. Pal::GFP aerosols presented a smaller diameter under low humidity than under high humidity conditions. The proportions of Pal::GFP aerosols detected in stage 5 (1.1-2.1 μm) at 35% RH (30.87%-42.59%) were significantly higher than those at 65% RH (21.72%-34.85%) and 95% RH (14.72%-7.82%) (Figure 4). However, no significant differences were observed in the size distribution of Pal::GFP aerosols among different levels of temperature.
Figure 4 Effect of temperature and relative humidity (RH) on the size distribution of Pseudomonas amygdali pv. lachrymans (Pal) particles in the aerosol chambers. The size distribution of Pal::GFP aerosol were detected immediately after aerosolizing Pal::GFP suspension (108 CFU/mL) for 30 min at the flowrate of 12 L/min by a six -jet Collision nebulizer. Error bars represent the standard deviations (SDs) of three replicates.
The symptoms and severity of cucumber ALS disease caused by Pal aerosol were influenced by physiological or environmental factors, especially temperature and RH. The optimum conditions for cucumber ALS disease development were 25°C and 95% RH, with a DI of 60.9; however, the disease developed slightly at 18°C and 35°C, with DI values lower than 10.0. Meanwhile, the DI decreased with the decline in RH. At 25°C, when cucumber seedlings were exposed to Pal::GFP aerosols at 65% RH, the DI decreased to 4.86. No visible symptoms were observed when cucumber was exposed to Pal::GFP aerosols at 35% RH (Figure 5A).
Figure 5 Effect of temperature and relative humidity (RH) on the pathogenicity and colonization of Pseudomonas amygdali pv. lachrymans (Pal) in aerosols on cucumber leaves. Healthy cucumber plant were exposed to Pal::GFP aerosol, generated by a six-jet Collison nebulizer for 30 min at the flow rate of 12 L/min, in chambers at different levels of temperature and RH. (A) The disease index and (B) colonization of Pal::GFP in cucumbers leaves were measured at 7 days after exposed to Pal::GFP aerosols at different levels of temperature and RH. The experiments were independently repeated three times. Columns and error bars represent the mean values and standard deviations (SDs) of three replicates. Columns labeled with different letters indicate statistically significant differences (p< 0.05).
In addition, the populations of Pal::GFP colonized on cucumber leaves were also evaluated. Concomitantly, the highest level of Pal::GFP multiplication was observed at 25°C and 95% RH, with Pal::GFP concentrations of 107.58 CFU/cm2 (Figure 5B). The population of Pal::GFP decreased with increasing temperature and decreasing RH, and low Pal::GFP concentrations were detected on cucumber leaves at 35°C and RH ≤ 65% (Figure 5B).
The concentration of Pal cells was specifically detected in bioaerosol samples collected from naturally infested greenhouses by qPCR (Table S1). The highest levels of Pal cells (825-955 cells/m3) were found in aerosol samples collected from greenhouses I, V and VI located in Tianjin city, Hebei and Shandong provinces, with temperatures of 19.0-23.1°C and RHs of 91.5%-95.1%. Relatively high levels of Pal cells (173-215 cells/m3) were obtained from greenhouse IV located in Hebei province, with temperatures of 16.7-18.8°C, and RHs of 69.8%-75.9%. The lowest levels of Pal cells (27-61 cells/m3) were obtained from greenhouses II and IX located in Tianjin city and Shandong province, with temperatures of 29.3-31.4°C and RHs of 30.2%-40.9%.
The surface response of the concentration levels of Pal aerosol to the combined effects of temperature and RH was obtained by the equation Z = Z0 + ax + by + cxy + dx2 + fy2 (Table 2). A lower temperature and high RH caused a larger amount of Pal release in greenhouses, and the most suitable conditions for Pal aerosol release were approximately 22°C-23°C and 100% RH (Figure 6).
Table 2 Parameter estimates of the non-liner regression model describing the co-influence of temperature and relative humidity (RH) on the concentration levels of Pseudomonas amygdali pv. lachrymans (Pal) in aerosols.
Figure 6 The 3D surface plot of the co-influence of temperature and relative humidity (RH) on concentration levels of Pseudomonas amygdali pv. lachrymans (Pal) in aerosols in naturally infested cucumber greenhouses. Bioaerosol samples were collected from nine naturally infested greenhouses, which located in Tianjin City (n=2), Hebei (n=3) and Shandong (n=4) Provinces, from 2019 to 2021. The concentration of Pal in aerosols was detected by qPCR. Temperature and RH in greenhouses were obtained using a digital thermo-hygrometer. The co-influence of temperature and RH on Pal aerosol concentration presented as a six-parameter model: Z = Z0 + ax + by + cxy + dx2 + fy2. In which, Z = Pal aerosols concentration, x = temperature, y = RH.
Plant canopies are considered a source of airborne bacteria, and the amount of bacterial aerosol released is different under various environments (Lindemann et al., 1982; Lindemann and Upper, 1985; Mcinnes et al., 1988). Bacteria on plant surfaces can generally be regarded as being inertly released into the atmosphere due to raindrops or shaking (Robertson and Alexander, 1994; Jones and Harrison, 2004). However, our previous study suggested that Pal aerosols could be emitted to the atmosphere in cases without rain or other external forces. Such emissions of Pal aerosol to the atmosphere may play a critical role in the dispersal of cucumber ALS disease in greenhouses (Chai et al., 2020). To estimate the potential extent of Pal aerosol emissions under different temperature and RH, we performed experiments both in aerosol chambers and in infested cucumber greenhouses located in different areas. Our experimental measurements revealed that during the release of Pal aerosol from infected cucumber plants, Pal in aerosols can reach the highest concentrations under conditions of 95% RH at a low temperature (≤ 25°C), with approximately 100 CFU/m3 in the aerosol chamber detected by an Andersen sampler (Figure 1C) and 1000 cells/m3 in the greenhouse detected by a portable sampler (Figure 5B). The concentration of Pal in aerosols under high temperature (35°C) and low RH (35% RH) was less than 50 CFU/m3. In addition, the decay rate of Pal in aerosols also accelerated (0.0881 min-1) under high temperature and low RH. Taken together, the inability of Pal in aerosols to cause cucumber ALS disease under high temperature and low RH could be explained by the release amount of Pal in aerosols being lower than the infection threshold value of Pal in aerosols (84-179 CFU/m3), the shorter survival time and the more rapid decay rate (Chai et al., 2020). Previous studies have demonstrated that the optimal conditions for the development of cucumber ALS disease were 25°C and more than 90% RH (Umekawa and Watanabe, 1980; Umekawa and Watanabe, 1982).
The initial concentrations of airborne bacteria during aerosolization and airborne suspension have been shown to be significantly different at various temperature and RH levels (Clifton et al., 2010; Ng et al., 2018). The initial concentration and survival rate of E. coli in aerosols at high RHs of 60%-80% was shown to be much higher than those at lower RH of 40% (Ng et al., 2018). In this study, the initial concentrations of Pal::GFP after aerosolization showed a downward trend with the decrease in RH levels at the same temperature, which was similar to the results for E. coli in aerosols. It has been suggested that the different damage levels of membrane integrity and fluidity index (FI) of Pal in aerosols are produced during aerosolization under different temperatures and RHs, which may lead to the difference in the initial concentration of Pal::GFP in aerosols (Ng et al., 2018).
The effect of temperature and RH on the survival of bacteria in aerosols varies based on the bacterial species. For example, the decay rate of E. coli in aerosols was significantly greater at low RH (<50% RH) at 15°C or 30°C than at high RH (Wathes et al., 1986). The death rate of Pasteurella tularensis in aerosols increased with increasing environmental temperature (Ehrlich and Miller, 1973). However, the decay rates of B. subtilis cells were not significantly influenced by the environmental temperature. In this study, we showed that high temperature (35°C) and lower RH (35%) have a synergistic effect on the decay of Pal in aerosols, with a half-life of 1.98 min; meanwhile, lower temperatures (25°C) and high RH (95%) support prolonged survival of Pal in aerosols, corresponding to a half-life of 6.79 min. This result suggested that high RH and low temperature favor the survival of Pal in aerosols.
There is strong evidence that temperature and RH affect the size of airborne particles, e.g., the size distributions of B. subtilis aerosols but not P. fluorescens aerosols were affected by RH (Johnson, 1999; Jones and Harrison, 2004; Pyankov et al., 2018). In this study, Pal aerosols with sizes of 0.65-2.1 μm were also profoundly affected by RH, and the concentration of Pal in aerosols in the 0.65-2.1 μm size range was relatively higher even under dry conditions. Our result was consistent with previous research showing that bacteria in smaller-sized aerosols (0.56-1 μm) have a higher viability (50%-70%) (Zhang et al., 2019). In addition, the distribution of Pal aerosols at 35°C and 65% RH was mainly 2.1-3.3 μm, which was similar to the P. glyinea aerosols collected in infected soybean plots during rainstorms and sprinkler irrigation (Venette and Kennedy, 1975). The stomatal aperture of healthy cucumber leaves is 4-5 μm (Wang et al., 2012), and the particle size of Pal aerosols released by cucumber plants predominantly ranged from 1.1 to 4.7 μm (accounting for 72.06%), which might be suitable for Pal aerosols to enter the cucumber plant and cause ALS disease (Chai et al., 2020). In this study, the particle size of Pal aerosols ranging from 2.1 to 4.7 μm was highest at 25°C and 95% RH. Interestingly, it is also the optimal condition for cucumber ALS disease development. Therefore, Pal aerosols distributed in the 2.1-4.7 μm range may play an important role in infecting cucumber plants.
High RH has a strong influence on the development of phyllosphere bacterial disease (Xin et al., 2016), and temperature impacts plant defense and bacterial virulence (Huot et al., 2017). In this study, the infectivity of Pal in aerosols to cucumber was significantly influenced by temperature and RH, and the highest infectivity of Pal in aerosols to cucumber was also shown at 25°C and 95% RH, in which the half-life of Pal in aerosols was much longer; this is similar to the findings of previous studies (Umekawa and Watanabe, 1980; Umekawa and Watanabe, 1982). Therefore, we consider 25°C and 95% RH as the optimal conditions for Pal to be infective to cucumber. The infectivity of Pal in aerosols is the lowest at 35% RH, which might be an extreme condition for Pal to infect cucumber. It is important to note that, however, the difference in the infectivity of Pal in aerosols to cucumber under various conditions was also affected by the concentration, survival and Pal in aerosols and the size of these aerosols, which needs more study to confirm.
This study investigated the effect of temperature and RH on the survival, release and infectivity of airborne Pal in aerosols. The release amount and survival time of Pal in aerosols under high RH (95%) and low temperature (≤ 25°C) conditions were significantly higher than those under low RH (35%) and high temperature (35°C) conditions. The highest release amount (96 CFU/m3) of Pal aerosol from infested cucumber and the survival rate (98.41%) were found at 18°C and 95% RH, while the highest disease index caused by Pal aerosol was found at 25°C and 95% RH. Additionally, the lowest amount of Pal released in aerosols and the fastest decay rate were detected at high temperature (35°C) and low RH (35%), and Pal aerosols in this condition resulted in the lowest disease index. These data provide valuable insight into the outbreaks of cucumber ALS disease and will aid further research into plant bacterial disease epidemiology by aerosol transmission. Our findings further suggest a novel means of preventing and controlling cucumber ALS disease by controlling the temperature and RH in greenhouses.
The original contributions presented in the study are included in the article/Supplementary Material. Further inquiries can be directed to the corresponding author.
AC: Supervision, Funding acquisition, Methodology, Writing - review & editing. LY: Writing - original draft, Methodology, Resources. XL: Validation. LL: Formal analysis. YS: Data curation. XX: Investigation. BL: Conceptualization, Project administration. All authors contributed to the article and approved the submitted version.
This work was carried out with the support of the National Natural Science Foundation of China (31872159, 32172653), the Modern Agro-industry Technology Research System (CARS-23), the Science and Technology Innovation Program, Chinese Academy of Agricultural Sciences (CAAS-ASTIP-IVFCAAS), and the Key Laboratory of Biology and Genetic Improvement of Horticultural Crops, Ministry of Agriculture and Rural Affairs, China (IVF2019).
The authors declare that the research was conducted in the absence of any commercial or financial relationships that could be construed as a potential conflict of interest.
All claims expressed in this article are solely those of the authors and do not necessarily represent those of their affiliated organizations, or those of the publisher, the editors and the reviewers. Any product that may be evaluated in this article, or claim that may be made by its manufacturer, is not guaranteed or endorsed by the publisher.
The Supplementary Material for this article can be found online at: https://www.frontiersin.org/articles/10.3389/fpls.2023.1087496/full#supplementary-material
Afsharnia, M., Biglari, H., Mohammadzadeh, A., Shakeri, H. (2018). Identifying and determining dispersion boundary bio-aerosols of bacterial and fungal pathogens from municipal waste collection containers. Arch. Hyg. Sci. 7, 39–46. doi: 10.29252/ArchHygSci.7.1.39
Aguilera, S., Alvarez-Morales, A., Murillo, A., Hernandez-Flores, J. L., Bravo, J., Torre-Zavala, S. D. (2017). Temperature-mediated biosynthesis of the phytotoxin phaseolotoxin by Pseudomonas syringae pv. phaseolicola depends on the autoregulated expression of the phtABC genes. PloS One 12 (6), e0178441. doi: 10.1371/journal.pone.0178441
Allegra, S., Leclerc, L., Massard, P. A., Girardot, F., Riffard, S., Pourchez, J. (2016). Characterization of aerosols containing Legionella generated upon nebulization. Sci. Rep. 6, 33998. doi: 10.1038/srep33998
Ariya, P. A., Amyot, M. (2004). New directions: the role of bioaerosols in atmospheric chemistry and physics. Atmos. Environ. 38, 1231–1232. doi: 10.1016/j.atmosenv.2003.12.006
Arnold, D. L., Preston, G. M. (2019). Pseudomonas syringae: enterprising epiphyte and stealthy parasite. Microbiology 165 (3), 251. doi: 10.1099/mic.0.000715
Aung, K., Jiang, Y., He, S. Y. (2018). The role of water in plant-microbe interactions. Plant J. 93, 771–780. doi: 10.1111/tpj.13795
Bhat, N. A., Bhat, K. A., Zargar, M. Y., Teli, M. A., Nazir, M., Zargar, S. M. (2010). Current status of angular leaf spot (Pseudomonas syringae pv. lachrmans) of cucumber: a review. J. Curr. Res. 8, 1–11.
Bigg, E. K., Soubeyrand, S., Morris, C. E. (2015). Persistent after-effects of heavy rain on concentrations of ice nuclei and rainfall suggest a biological cause. Atmos. Chem. Phys. 15, 2313–2326. doi: 10.5194/acp-15-2313-2015
Burrows, S., Butler, T., Jöckel, P., Tost, H., Kerkweg, A., Pöschl, U., et al. (2009). Bacteria in the global atmosphere - part 2: Modeling of emissions and transport between different ecosystems. Atmos. Chem. Phys. 9, 9281–9297. doi: 10.5194/acp-9-9281-2009
Chai, A., Yuan, L., Li, L., Shi, Y., Xie, X., Wang, Q., et al. (2020). Aerosol transmission of Pseudomonas amygdali pv. lachrymans in greenhouses. Sci. Total. Environ. 748, 141433. doi: 10.1016/j.scitotenv.2020.141433
Chamberlain, A. C. (1975). “The movement of particles in plant communities,” in Vegitation and the atmosphere, vol. 1 . Ed. Monteith, J. L. (London: Academic Press), 155–203.
Chen, H., Yao, M. (2018). A high-flow portable biological aerosol trap (HighBioTrap) for rapid microbial detection. J. Aerosol. Sci. 117, 212–223. doi: 10.1016/j.jaerosci.2017.11.012
Clifton, I. J., Fletcher, L. A., Beggs, C. B., Denton, M. S., Conway, P. D., Peckham, G. (2010). An aerobiological model of aerosol survival of different strains of Pseudomonas aeruginosa isolated from people with cystic fibrosis. J. Cyst. Fibros. 9, 64–68. doi: 10.1016/j.jcf.2009.11.004
Ehrlich, R., Miller, S. (1973). Survival of airborne Pasteurella tularensis at different atmospheric temperatures. Appl. Microbiol. 25, 369–372. doi: 10.1128/am.25.3.369-372.1973
FAOSTAT. (2020). Retrieved from http://faostat3.fao.org/.
Gomila, M., Busquest, A., Mulet, M., García-Valdés, E., Lalucat, J. (2017). Clarification of taxonomic status within the Pseudomonas syringae species group based on a phylogenomic analysis. Front. Microbiol. 8, 2422. doi: 10.3389/fmicb.2017.02422
Groth, E. M., Hirano, S. S., Baker, L. S., Upper, C. D. (1995). Insect-mediated dispersal of Pseudomonas syringae in a bean field. Phytopathology 85, 1188.
Gut, I. M., Bartlett, R. A., Yeager, J. J., Leroux, B., Ratnesar-Shumate, S., Dabisch, et al. (2016). Extraction of aerosol-deposited Yersinia pestis from indoor surfaces to determine bacterial environmental decay. Appl. Environ. Microbio. 82, 2809. doi: 10.1128/AEM.03989-15
Hambleton, P., Broster, M. G., Dennis, P. J., Henstridge, R., Fitzgeorge, R., Conlan., J. W. (1983). Survival of virulent Legionella pneumophila in aerosols. J. Hyg. Camb. 90, 451–460. doi: 10.1017/S0022172400029090
Harrison, M. D. (1980). Aerosol dissemination of bacterial plant pathogens. ann. the NY. Acad. Sci. 353, 94–104. doi: 10.1111/j.1749-6632.1980.tb18911.x
Hirano, S. S., Baker, L. S., Upper, C. D. (1996). Raindrop momentum triggers growth of leaf-associated populations of Pseudomonas syringae on field-grown snap bean plants. Appl. Environ. Microbiol. 62, 2560–2566. doi: 10.1128/aem.62.7.2560-2566.1996
Hirano, S. S., Christen, D. U. (2000). Bacteria in the leaf ecosystem with emphasis on Pseudomonas syringae-a pathogen, ice nucleus, and epiphyte. Microbiol. Mol. Biol. R. 64, 624–653. doi: 10.1128/MMBR.64.3.624-653.2000
Hoeksma, P., Aarnink, A., Ogink, N. (2015). Effect of temperature and relative humidity on the survival of airborne bacteria Vol. 864 (Wageningen: Wageningen UR (University & Research centre) Livestock Research).
Huot, B., Castroverde, C. D. M., Velásquez, A. C., Hubbard, E., Pulman, J. A., Yao, J., et al. (2017). Dual impact of elevated temperature on plant defence and bacterial virulence in Arabidopsis. Nat. Commun. 8, 1808. doi: 10.1038/s41467-017-01674-2
Islam, M. A., Ikeguchi, A., Naide, T. (2020). Influence of temperature and humidity on the dynamics of aerosol numbers and airborne bacteria in a dairy calf house. Biosyst. Eng. 194, 213–226. doi: 10.1016/j.biosystemseng.2020.04.003
Johnson, D. L. (1999). The effect of phosphate buffer on aerosol size distribution of nebulized bacillus subtilis and Pseudomonas fluorescens bacteria. Aerosol. Sci. Tech. 30, 202–210. doi: 10.1080/027868299304787
Jones, A. M., Harrison, R. M. (2004). The effects of meteorological factors on atmospheric bioaerosol concentrations - a review. Sci. Total. Environ. 326, 151–180. doi: 10.1016/j.scitotenv.2003.11.021
Joung, Y. S., Ge, Z., Buie, C. R. (2017). Bioaerosol generation by raindrops on soil. Nat. Commun. 8, 14668. doi: 10.1038/ncomms14668
Kowalski, M., Pastuszka, J. (2018). Effect of ambient air temperature and solar radiation on changes in bacterial and fungal aerosols concentration in the urban environment. Ann. Agr. Env. Med. 25, 259–261. doi: 10.26444/aaem/75877
Leben, C. (1981). Survival of Pseudomonas syringae pv. lachrymans with cucumber seed. Can. J. Plant Pathol. 3, 247–249. doi: 10.1080/07060668109501359
Liao, C. M., Luo, W. C. (2005). Use of temporal/seasonal- and size-dependent bioaerosol data to characterize the contribution of outdoor fungi to residential exposures. Sci. Total. Environ. 347, 78–97. doi: 10.1016/j.scitotenv.2004.12.036
Lindemann, J., Constantinidiou, H. A., Barchet, W. R., Upper, C. D. (1982). Plants as source of airborne bacteria, including ice nucleation-active bacteria. Appl. Environ. Microb. 44, 1059–1063. doi: 10.1128/aem.44.5.1059-1063.1982
Lindemann, J., Upper, C. D. (1985). Aerial dispersal of epiphytic bacteria over bean plants. Appl. Environ. Microb. 50, 1229–1232. doi: 10.1128/aem.50.5.1229-1232.1985
Li, L., Yuan, L., Shi, Y., Xie, X., Chai, A., Wang, Q., et al. (2019). Comparative genomic analysis of Pseudomonas amygdali pv. lachrymans NM002: insights into its potential virulence genes and putative invasion determinants. Genomics 111, 1493–1503. doi: 10.1016/j.ygeno.2018.10.004
Marthi, B., Fieland, V. P., Walter, M., Seidler, R. J. (1990). Survival of bacteria during aerosolization. Appl. Environ. Microb. 56, 3463–3467. doi: 10.1128/aem.56.11.3463-3467.1990
Mcinnes, T. B., Gitaitis, R. D., Mccarter, S. M., Jaworski, C. A., Phatak, S. C. (1988). Airborne dispersal of bacteria in tomato and pepper transplant fields. Plant Dis. 72, 575–579. doi: 10.1094/PD-72-0575
Melotto, M., Underwood, W., He, S. Y. (2008). Role of stomata in plant innate immunity and foliar bacterial diseases. Annu. Rev. Phytopathol. 46, 101–122. doi: 10.1146/annurev.phyto.121107.104959
Meng, X., Chai, A., Chen, L., Shi, Y., Xie, X., Ma, Z., et al. (2016). Rapid detection and quantification of viable Pseudomonas syringae pv. lachrymans cells in contaminated cucumber seeds using propidium monoazide and a real-time PCR assay. Can. J. Plant Pathol. 38, 296–306. doi: 10.1080/07060661.2016.1216897
Moe, K., Harper, G. J. (1983). The effect of relative humidity and temperature on the survival of bovine rotavirus in aerosol. Arch. Virol. 76, 211–216. doi: 10.1007/BF01311105
Ng, T. W., Chan, W. L., Lai, K. M. (2018). Influence of membrane fatty acid composition and fluidity on airborne survival of Escherichia coli. appl. Microbiol. Biot. 102, 3327–3336. doi: 10.1007/s00253-018-8826-7
Olczak-Woltman, H., Schollenberger, M., Niemirowicz-Szczytt, K. (2009). Genetic background of host-pathogen interaction between Cucumis sativus l. and Pseudomonas syringae pv. lachrymans. J. Appl. Genet. 50, 1–7. doi: 10.1007/BF03195645
Peix, A., Ramírez-Bahena, M. H., Velázquez, E. (2018). Status on the taxonomy of pseudomonas revisited: an the current update. Infect. Genet. Evol. 57, 106–116. doi: 10.1016/j.meegid.2017.10.026
Pyankov, O. V., Bodnev, S. A., Pyankova, O. G., Agranovski, I. E. (2018). Survival of aerosolized coronavirus in the ambient air. J. Aerosol. Sci. 115, 158–163. doi: 10.1016/j.jaerosci.2017.09.009
Quinn, C. E., Sells, I. A., Graham, D. C. (1980). Soft rot Erwinia bacteria in the atmospheric bacterial aerosol. J. Appl. Microbiol. 49, 175–181. doi: 10.1111/J.1365-2672.1980.TB01055.X
Rajendran, D. K., Park, E., Nagendran, R., Hung, N. B., Cho, B. K., Kim, K. H. (2016). Visual analysis for detection and quantification of Pseudomonas cichorii disease severity in tomato plants. Plant Pathol. J. 32, 300–310. doi: 10.5423/PPJ.OA.01.2016.0032
Robertson, B. K., Alexander, M. (1994). Mode of dispersal of the stem-nodulating bacterium, azorhizobium. Soil Biol. Biochem. 126, 1535–11540. doi: 10.1016/0038-0717(94)90095-7
Thompson, K. A., Bennett, A. M., Walker, J. T. (2011). Aerosol survival of Staphylococcus epidermidis. J. Hosp. Infect. 78, 216–220. doi: 10.1016/j.jhin.2010.12.009
Umekawa, M., Watanabe, Y. (1980). Control of angular leaf spot of cucumber by suppressing the relative humidity in the plastic house. Ann. Rept. Plant Prot. North Jpn. 31, 69–70.
Umekawa, M., Watanabe, Y. (1982). Relation of temperature and humidity to the occurrence of angular leaf spot of cucumber grown under plastic house. Ann. Phytopath. Soc Japan 48, 301–307. doi: 10.3186/jjphytopath.48.301
Umekawa, M., Watanabe, Y., Inomata, Y. (1981). Facilitative effect of rainfall on the transmission of the pathogen and the development of angular leaf spot of cucumber. Ann. Phytopath. Soc Japan 47, 346–351. doi: 10.3186/jjphytopath.47.346
Venette, J. R., Kennedy, B. W. (1975). Naturally produced aerosols of Pseudomonas glycinea. Phytopathol. note. 65, 737–741. doi: 10.1094/Phyto-65-737
Wang, M., Ling, N., Dong, X., Zhu, Y., Shen, Q., Guo, S. (2012). Thermographic visualization of leaf response in cucumber plants infected with the soil-borne pathogen Fusarium oxysporum f. sp. cucumerinum. Plant Physiol. Bioch. 61, 153–161. doi: 10.1016/j.plaphy.2012.09.015
Wathes, C. M., Howard, K., Webster, A. J. F. (1986). The survival of Escherichia coli in an aerosol at air temperatures of 15 and 30°C and a range of humidities. Epidemiol. Infect. 97, 489–496. doi: 10.1017/s0022172400063671
Webb, S. J. (1961). Factors affecting the viability of airborne bacteria. v. the effect of desiccation on some metabolic systems of Escherichia coli. Can. J. Microbiol. 7, 621–632. doi: 10.1139/m61-071
Xin, X. F., Nomura, K., Aung, K., Velásquez, A. C., Yao, J., Boutrot, F., et al. (2016). Bacteria establish an aqueous living space in plants crucial for virulence. Nature 539, 524–529. doi: 10.1038/nature20166
Xu, Z. Q., Wen, Y., Wu, Y., Shen, F. X., Chen, Q., Li, M. Z., et al (2013). Enhancing bioaerosol sampling by Andersen impactors using mineral-oil-spread agar plate. PLoS One 8, e56896.
Zeng, W., Melotto, M., He, S. Y. (2010). Plant stomata: a checkpoint of host immunity and pathogen virulence. Curr. Opin. Biotechnol. 21, 599–603. doi: 10.1016/j.copbio.2010.05.006
Keywords: temperature, relative humidity, bioaerosol, Pseudomonas amygdali pv. lachrymans, cucumber angular leaf spot disease
Citation: Chai A, Yuan L, Li X, Li L, Shi Y, Xie X and Li B (2023) Effect of temperature and humidity on dynamics and transmission of Pseudomonas amygdali pv. lachrymans aerosols. Front. Plant Sci. 14:1087496. doi: 10.3389/fpls.2023.1087496
Received: 02 November 2022; Accepted: 05 January 2023;
Published: 03 February 2023.
Edited by:
Prachi Pandey, National Institute of Plant Genome Research (NIPGR), IndiaReviewed by:
Collin M. Timm, Applied Physics Laboratory, Johns Hopkins University, United StatesCopyright © 2023 Chai, Yuan, Li, Li, Shi, Xie and Li. This is an open-access article distributed under the terms of the Creative Commons Attribution License (CC BY). The use, distribution or reproduction in other forums is permitted, provided the original author(s) and the copyright owner(s) are credited and that the original publication in this journal is cited, in accordance with accepted academic practice. No use, distribution or reproduction is permitted which does not comply with these terms.
*Correspondence: Baoju Li, bGliYW9qdWl2ZkAxNjMuY29t
†These authors have contributed equally to this work
Disclaimer: All claims expressed in this article are solely those of the authors and do not necessarily represent those of their affiliated organizations, or those of the publisher, the editors and the reviewers. Any product that may be evaluated in this article or claim that may be made by its manufacturer is not guaranteed or endorsed by the publisher.
Research integrity at Frontiers
Learn more about the work of our research integrity team to safeguard the quality of each article we publish.