- 1Plant Development Lab, Institute of Botany, Hustus-Liebig-University, Giessen, Germany
- 2Laboratory of Medicinal Cell Biology, Kobe Pharmaceutical University, Kobe, Japan
- 3Graduate School of Biostudies, Kyoto University, Kyoto, Japan
- 4Bioorganic Research Institute, Suntory Foundation for Life Science, Kyoto, Japan
- 5Graduate School of Science, Osaka Metropolitan University, Sakai, Japan
California poppy or golden poppy (Eschscholzia californica) is the iconic state flower of California, with native ranges from Northern California to Southwestern Mexico. It grows well as an ornamental plant in Mediterranean climates, but it might be invasive in many parts of the world. California poppy was also highly prized by Native Americans for its medicinal value, mainly due to its various specialized metabolites, especially benzylisoquinoline alkaloids (BIAs). As a member of the Ranunculales, the sister lineage of core eudicots it occupies an interesting phylogenetic position. California poppy has a short-lived life cycle but can be maintained as a perennial. It has a comparatively simple floral and vegetative morphology. Several genetic resources, including options for genetic manipulation and a draft genome sequence have been established already with many more to come. Efficient cell and tissue culture protocols are established to study secondary metabolite biosynthesis and its regulation. Here, we review the use of California poppy as a model organism for plant genetics, with particular emphasis on the evolution of development and BIA biosynthesis. In the future, California poppy may serve as a model organism to combine two formerly separated lines of research: the regulation of morphogenesis and the regulation of secondary metabolism. This can provide insights into how these two integral aspects of plant biology interact with each other.
1 Phylogeny, biogeography and growth conditions
Eschscholzia californica is a member of the Papaveraceae family of the order of Ranunculales, which is sister to the core eudicots (Figure 1A, Hoot et al., 2015; Lane et al., 2018). Sister to all Papaveraceae is the enigmatic Pteridophyllum racemosum, with fern-like leaves and bell-like white flowers in a loose inflorescence. Eschscholzia californica, with common name California poppy, Golden poppy, or Cup of Gold, belongs to the subfamily of Eschscholzioideae, which is the sister group to both, the Papaveroideae (including Papaver somniferum, opium poppy) and the Chelidonioideae (Figure 1B).
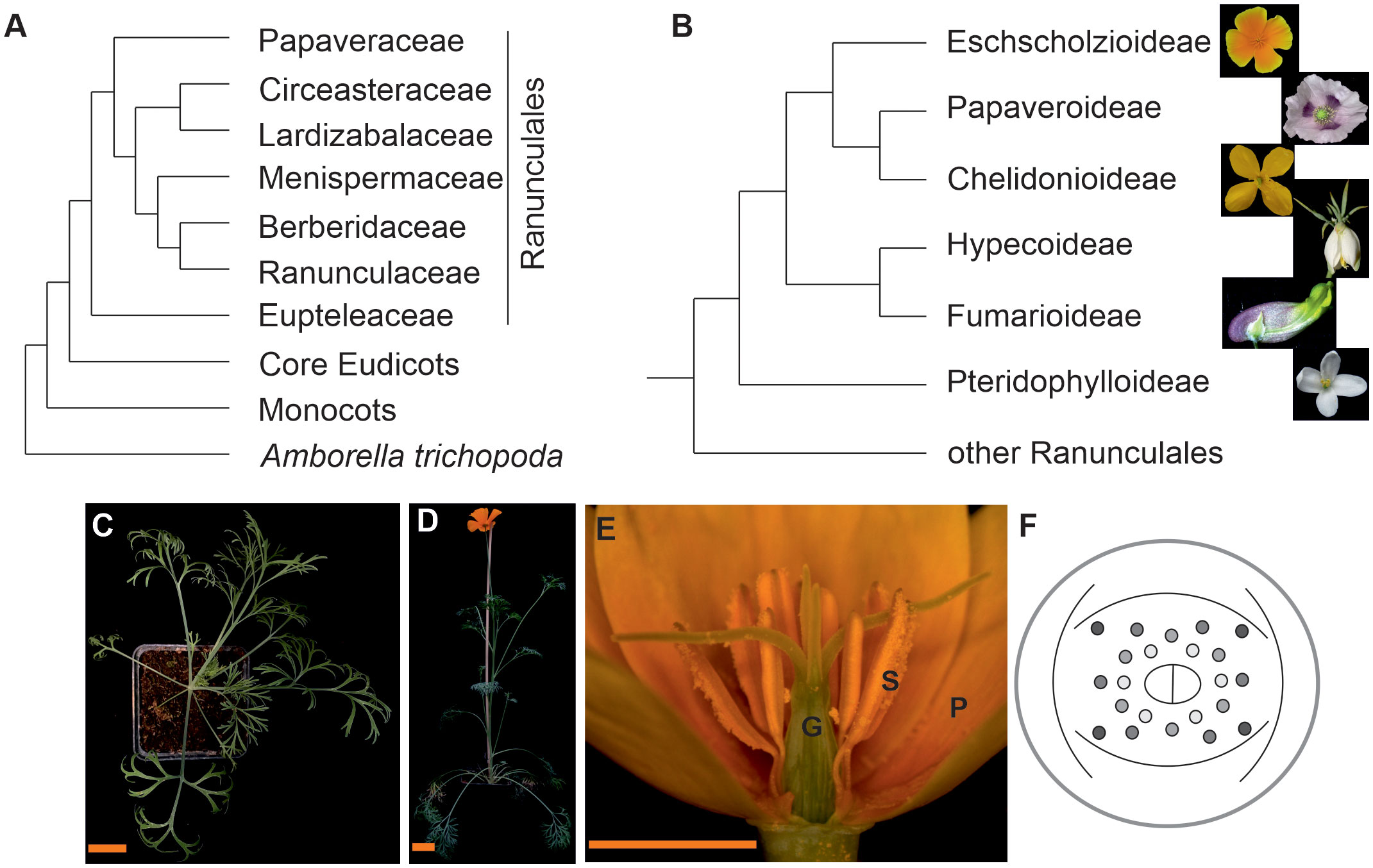
Figure 1 Simplified angiosperm phylogenies focusing on the phylogenetic position and morphology of California poppy. (A) Phylogeny of the Ranunculales [according to Lane et al. (2018) and Hoot et al. (2015)], (B) phylogeny of the Papaveraceae s.l. on subfamily level based on Hoot et al. (2015). Fotos next to the branches show subfamily representatives, from top to bottom California poppy, Papaver somniferum, Chelidonium majus, Hypecoum leptocarpum, Capnoides sempervirens, Pteridophyllum racemosum. (C) top view on California poppy in its vegetative phase, (D) side view of a flowering plant, (E) side view of a California poppy flower (G, gynoecium; P, petal; S, stamens) and (F) floral diagram showing the sepal cap, two whorls of petals, several whorls of stamens and a bicarpellate gynoecium (photos are from Dominik Lotz and Doudou Kong, Gießen, Germany, and Natalia Pabon-Mora, Medellin, Colombia). The size bar in C and D correspond to 3 cm, the size bar in E is 1 cm.
California poppy is growing as an annual or perennial and is native to western North America. Its native range covers the Columbia River in Northern Oregon to Baja California, a peninsula separating the Gulf of California from the Pacific Ocean. It is found from the Pacific Coast to the Great Basin, including the Sierra Nevada and the Mojave Desert (Cook, 1962). In the past 200 years, California poppy was introduced by man to Chile, South Africa, Australia including Tasmania, and New Zealand, where they became naturalized weeds (Cook, 1962; Leger and Rice, 2007). For the Chilean invasive population, the population history was documented in astonishing detail: California poppy was introduced to the Chilean coastal cities as an ornamental plant during the 1890s and spread from there along railway tracks. Within less than 100 years, the species spread 240 km south and 520 km north of their original points of introduction, including all of the Mediterranean climate region of Chile in its range. Furthermore, California poppy habitat covers an enormous altitudinal range between 0 and 2000 m.a.s.l. in Chile (Véliz et al., 2012) with traits differing between coastal and high altitude plants: coastal plants were shorter, required more time to flower, and produced fewer flowers and fewer seeds per fruit, regardless if the plants were collected from California or Chile. After being introduced to Chile, California poppy has improved its abilities to colonize disturbed environments and evolved robust patterns of local adaptations, all in only 150 years (Leger and Rice, 2007).
Owing to its invasive nature and Mediterranean climate natural range, California poppy is easy to grow and a commonly grown ornamental plant. The plants (Becker et al., 2005; Wege et al., 2007), require little space, 42 plants/m² can be grown comfortably, but up to 90 plants/m² are possible, and standard greenhouse conditions (6 cm pots, standard potting mix, 21°C – 25°C day, 15°C-18°C night, natural light supplemented with fluorescent tubes or mercury lamps, 16 h light/8 h darkness). California poppy does not require governmental permits to be raised and does not require CITES documentation as it is not an endangered plant species.
2 California poppy morphology
Cotyledons of California poppy are deeply lobed, and the shoot apical meristem (SAM) gives rise to highly dissected, silvery green leaves forming a rosette. Once the shoot elongates, the SAM converts into an inflorescence meristem, which continues to release leaves. When the uppermost two leaves are formed, the inflorescence meristem converts into a terminal flower meristem. In the leaf axils of the uppermost cauline leaves, new secondary meristems may form. California poppy leaf size and shape depends on the position and age of the leaves: length and degree of dissections increases with age during the vegetative phase, but decreases after transitioning to flowering (Becker et al., 2005).
California poppy has large flowers with four brightly colored petals. The flowers across natural populations are diverse, with petal color in the northern and coastal habitats of its native range being more yellow and the central and southern populations show a larger portion of orange petal color. Stamen numbers range between 16 and 39 with the southern population developing fewer stamens normally (Cook, 1962). California poppy flowers are composed of two sepals fused into a cap-like structure that dehisces when the petals fully elongate. Four petals are 1-3 cm in length and width and are arranged in two concentric whorls (Becker et al., 2005). The petals are not only intensely colored by carotenoids but also have a silky appearance. This effect is caused by a thick, prism-like ridge that runs along enormously elongated petal cells and focuses light to the pigments at the abaxial epidermis cell side (Wilts et al., 2018).
Further inside the flower, the stamens form, with four stamens in the first stamen whorl and all other consecutive whorls forming six stamens. The stamens are composed of a short filament and long, bilobed anthers. The basal part of the anthers is often dark brown with all other parts colored bright yellow. In the center of the flower, the gynoecium is composed of two fused carpels forming a long, superior ovary topped by a short style. Four stigmatic protrusions are covered with papillae that often bend down towards the floral base. A floral tube forms that surrounds the ovary, which is adorned with a torus rim to which the sepals and petals are attached. In the ovary, two rows of ovules are attached to the two placentae (Becker et al., 2005).
After fertilization, the gynoecium develops into a slender capsule of around 5 cm in length at maturity. The capsule dries out and the valves separate from bottom to top from each other releasing the seeds explosively, scattering them up to 1.5 m (Cook, 1962; Becker et al., 2005).
2.1 Molecular regulation of vegetative and reproductive development, examples from functional studies in California poppy
Several studies examining the role of California poppy orthologs of Arabidopsis thaliana developmental regulators in recent years contributed to our understanding of gene function conservation. The focus of these studies was on floral development, with an emphasis on carpel development. The advantage of California poppy in these studies is its comparatively simple floral and fruit morphology allowing direct comparisons with long established model species like Arabidopsis.
Highly conserved developmental regulators, such as MADS-box transcription factors from California poppy show partially similar functions to Arabidopsis, such as specification of floral organ identity. However, their regulation differs from that of Arabidopsis and a higher number of genes in the poppy allows for sub- and possibly neofunctionalization. For example, the AGAMOUS (AG) orthologs of California poppy are required for stamen and carpel organ identity, and the APETALA3 (AP3) and PISTILLATA (PI) orthologs for petal and stamen organ identity (Yellina et al., 2010; Lange et al., 2013). The two AG orthologs and three AP3 orthologs are differentially expressed in the flower. EScaAG1 shows a stronger expression, especially in carpels and fruits. EScaAG2 is expressed mainly in stamens and only very little in other floral tissues. This divergence in expression suggests that EScaAG1 is more important for carpel identify and possibly also for the regulation of floral meristem termination, and EScaAG2 is more important for stamen formation. Expression of the three DEF/AP3-like genes shows organ-level differences, such that EScaDEF3 is most strongly expressed in petals, as is EScaDEF2, but the latter to a lesser extent, and EScaDEF1 is hardly expressed in petals and stamens (Yellina et al., 2010; Lange et al., 2013). In terms of trimeric protein interactions, EScaAG1 participates in complexes of floral homeotic proteins including BBC and BCE class proteins, but EScaAG2 does not, suggesting that the floral homeotic C function may be carried out by EScaAG1. Further, EScaAG2 can form homodimers, while EScaAG1 cannot, suggesting novel, yet unknown functions for EScaAG2. It is also notable that EScaDEF1, the E-function protein Ec-SEP3 and EScaAG1 complexes form, as well as EScaDEF2/Ec-SEP3/EScaAG1, but not EScaDEF3/Ec-SEP3/EScaAG1 (Lange et al., 2013), suggesting subfunctionalization on the level of protein interactions for these floral homeotic proteins.
The regulation of California poppy floral homeotic B and C genes differs from that of A. thaliana: the AG orthologs are activated by the AP3 and PI orthologs while the AG orthologs repress AP3 and PI activity in the carpels, as shown using VIGS experiments and by analysis of the B mutant sei-1 (Yellina et al., 2010; Lange et al., 2013).
California poppy also served to show that the function of INAPERTURATE POLLEN (INP1), a gene required for aperture formation in pollen grains to allow pollen grain germination, is conserved throughout monocots and dicots, even though sequence divergence of orthologs is comparatively high (Mazuecos-Aguilera et al., 2021).
Like in Arabidopsis, the SHOOT MERISTEMLESS (STM) homologs of California poppy are required for floral meristem activity (Scofield et al., 2007) and are, in combination with California poppy CRABS CLAW (CRC) orthologs required for floral meristem maintenance and timely termination. As the carpels are the last organs to be formed in the flower, their presence and number critically depends on floral meristem activity, and when the poppy STM genes are silenced, the floral meristem terminates prematurely leading to failure of carpel formation, as observed in A. thaliana (Scofield et al., 2007; Stammler et al., 2013). Conversely, more carpels are produced when the California poppy CRC ortholog transcription is silenced. This suggests antagonistic functions in floral meristem maintenance for the poppy STM and CRC genes (Orashakova et al., 2009; Stammler et al., 2013). A. thaliana crc mutants show only rarely more carpels, but the gynoecia often fail to fuse at the apex and lack nectaries. Recent work has shown that CRC acts as a repressing transcription factor in floral meristem termination and as a transcriptional activator in carpel fusion and nectary development (Bowman and Smyth, 1999; Groß et al., 2018). Also, in the Phalaenopsis equestris orchid, CRC homologs regulate reproductive development, specifically in the gynostemium, an organ consisting of stamens fused to the gynoecium, suggesting that the regulation of gynoecium development by CRC homologs is conserved between monocots and dicots (Chen et al., 2021).
FRUITFUL (FUL) and NGATHA (NGA) orthologs of poppy were shown to be involved in carpel and fruit development, indicative of deeply conserved gene functions between California poppy genes and their orthologs from Arabidopsis. EcFUL1, EcFUL2 down regulated by VIGS results in shorter fruits that open prematurely and occurrence of leaf-like sepals, suggesting that sepal organ identity is compromised and the lignin deposition pattern in fruits is disturbed (Pabón-Mora et al., 2012). Lignin deposition is also disturbed in the A. thaliana ful mutant, resulting in a failure to form a dehiscence zone leading to fruits that rupture at random positions (Ferrandiz et al., 2000). Outside the eudicots, FUL-like genes are not involved in fruit dehiscence, for example, WAP1 from wheat is required for vernalization and phase transition (Murai et al., 2003), suggesting that the involvement of FUL-like genes in regulation of lignification pattern for dehiscence zone formation is restricted to eudicots.
For NGA-like genes, California poppy VIGS-treated plants provide the only functional data outside the core eudicots, as information on mutants in grasses is lacking so far. Within eudicots, the NGA orthologs of A. thaliana, tobacco and California poppy all share that they are required for style and stigma tissue specification (Fourquin and Ferrándiz, 2014).
CYCLOIDEA/TEOSINTE BRANCHED1-like (CYL/TB1) genes of California poppy regulate plant stature, such that down regulation by VIGS enhances axillary branching, a function conserved throughout dicots and monocots. Further, the CYL/TB1-like genes in California poppy regulate stamen number and petal size. However, the link between stamen number regulation and CYL/TB1-like genes does not seem to be special to Papaveraceae, but floral organ size regulation of CYL/TB1-like genes is in line with a conserved function of these genes in the repeated establishment of zygomorphy within the pentapetalae. In the Papaveraceae, CYL/TB1-like genes do not establish zygomorphy but may regulate the extent of morphological differences between the floral organs by controlling growth (Zhao et al., 2018).
These studies show that knowledge about the conservation of developmental regulator’s gene functions across dicots can be garnered by studying California poppy as genetically tractable representative of the sister lineage to the core eudicots, and the conservation of function between monocots and dicots can be inferred by incorporating California poppy mutants or VIGS-treated plants.
2.2 Floral pigments
California poppy flowers contain unique carotenoids, such as eschscholtzxanthin and retro-carotene-triol (Maoka et al., 2000), which show intense yellow to orange petal pigmentation of this ornamental flower. Whereas orange flowers are more popular, color variations from white to yellow and orange are known. Barrell et al. (2010) analyzed flower color inheritance in diverse variants and showed that all white and yellow variants showed the multiple effects and total of five complementation groups were identified as single recessive loci. Interestingly, all mutations influence both petal and pollen color, suggesting that the same gene controls petal and pollen color.
Zhou et al. (2018) further investigated the carotenoid biosynthetic pathway using a Tobacco Rattle Virus-based virus-induced-gene-silencing (VIGS) approach. VIGS of early (PDS and ZDS) and late (βOH and ZEP) biosynthetic enzymes in carotenoid pathway reduced the transcripts of the target genes in the petals without the effect on other carotenoid biosynthesis gene expressions. Silencing of PDS, ZDS, βOH and ZEP genes reduced total pigment concentration by 75-90% and altered petal color. HPLC and LC-MS measurements suggested that petal color changes were caused by substantially altered pigment profiles and quantity. More recently, Pollack et al. (2019) discovered a single deletion leading to altered splicing and C-terminal truncation of phytoene synthase (PSY), a key enzyme in carotenoid biosynthesis mutated in multiple white petal varieties.
Whereas the key enzyme genes for retro-carotene-triol biosynthesis are still not identified yet, some candidate genes are predicted based on the draft genome sequence and transcriptome analysis for future breeding (Sato et al. unpublished data).
3 Medicinal use
California poppy was highly prized by Native Americans for its medicinal value based on its specialized metabolite biosynthesis, with the most prominent class being the benzylisoquinoline alkaloids (BIAs). Phytochemical analysis revealed that aerial parts and roots accumulate alkaloids, with roots showing a higher concentration of up to 1.6% alkaloids of the dry weight. These alkaloids are mainly BIAs and include benzophenanthridine alkaloids (such as sanguinarine, chelirubine, macarpine, chelerythrine, chelilutine), protopines (protopine, allocryptopine), aporphine alkaloids (magnoflorine, corydine, isoboldine, N-methyllaurotetanine), simple benzylisoquinolines (reticuline), pavine alkaloids (californidine, caryachine, escholtzine), as well as the dihydro-intermediates (Figure 2). Roots contain mainly benzophenanthridine alkaloids and protopines, whereas aerial parts are especially rich in pavine and some aporphine alkaloids (Liscombe et al., 2009; Fedurco et al., 2015; Hori et al., 2018).
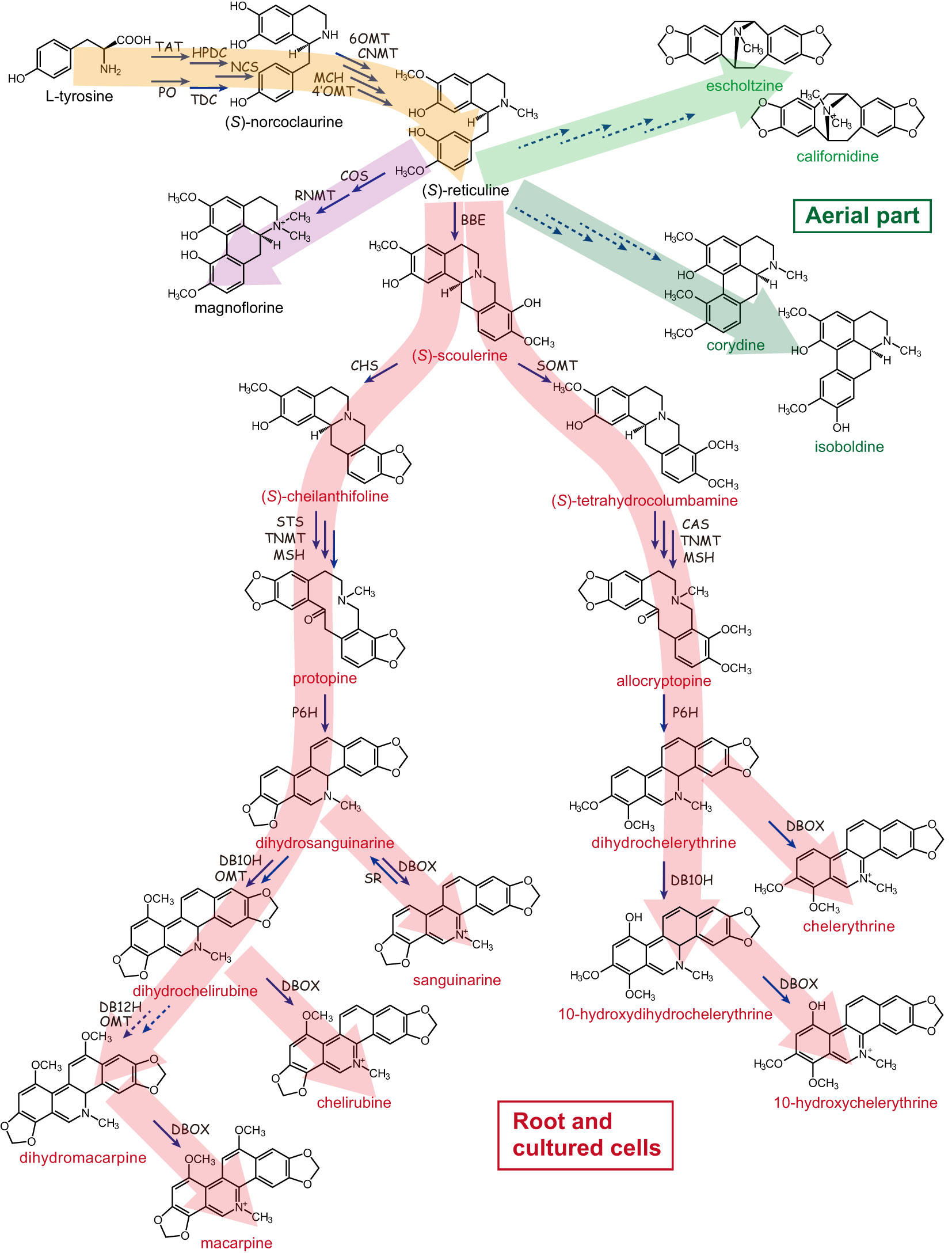
Figure 2 Benzylisoquinoline alkaloid biosynthetic pathway in California poppy. California poppy produces diverse array of benzylisoquinoline type alkaloids (BIAs), which include benzophenanthridine alkaloids (such as sanguinarine, chelirubine, macarpine, chelerythrine, chelilutine), protopines (protopine, allocryptopine), aporphine alkaloids (magnoflorine, corydine, isoboldine), simple benzylisoquinolines (reticuline), pavine alkaloids (californidine, escholtzine), as well as the dihydro-intermediates. The broken lines indicate that the biosynthetic enzyme-encoding genes have not been identified. TAT, tyrosine aminotransferase; HPDC, p-hydroxyphenylpyruvate decarboxylase; PO, phenol oxidase; TDC, tyrosine/DOPA decarboxylase; NCS, (S)-norcoclaurine synthase; 6OMT, (S)-norcoclaurine 6-O-methyltransferase; CNMT, (S)-coclaurine N-methyltransferase; MCH, (S)-N-methylcoclaurine 3’-hydroxylase; 4’OMT, (S)-3’-hydroxy-N-methylcoclaurine 4’-O-methyltransferase; COS, (S)-corytuberine synthase; RNMT, (S)-reticuline N-methyltransferase; BBE, berberine bridge enzyme; SOMT, (S)-scoulerine 9-O-methyltransferase; CHS, (S)-cheilanthifoline synthase; STS, (S)-stylopine synthase; CAS, (S)-canadine synthase; TNMT, (S)-tetrahydroprotoberberine N-methyltransferase; MSH, (S)-N-methylstylopine 14-hydroxylase; P6H, protopine 6-hydroxylase; DBOX, dihydrobenzophenanthridine alkaloid oxidase; SR, sanguinarine reductase; DB10H, dihydrobenzophenanthridine alkaloid 10-hydroxylase; DB12H, dihydrobenzophenanthridine alkaloid 12-hydroxylase. Large arrows in red denote compounds synthesized in root and cultured cells, yellow indicates starting products of BIA synthesis, and green indicates alkaloids found in aerial part.
Pharmacological studies of these BIAs revealed their antifungal, analgesic, anxiolytic, sedative activities (Al-Snafi, 2017). For example, extracts prepared from aerial parts of California poppy as herbal supplement show analgesic, anxiolytic and sedative effects. Rolland et al. (1991) reported that the aqueous extract reduced the behavioral performance in mice with regard to novelty preference, locomotion and rearing in two compartments parameters measured (in a familiar environment test). Further, the California poppy extract treated mice underperformed in the staircase test (non-familiar environment tests). These findings support the traditional use of California poppy herbal extract to induce sleep due to sedative properties. Fedurco et al. (2015) further assigned the depressant properties of aerial California poppy parts to chloride-current modulation at the alpha(3)beta(2)gamma(2) and alpha(5)beta(2)gamma(2) GABA(A) receptors by (S)-reticuline, a minor alkaloid in the herbal extract,
The biological activities of sanguinarine, the main alkaloid in California poppy root and cell cultures, have been investigated in depth and were recently reviewed by Laines-Hidalgo et al. (2022). In brief, sanguinarine shows herbivore deterrent activity as well as antimicrobial effects (Schmeller et al., 1997). These effects may be related to its cytotoxic activity, such as intercalation in nucleic acids, and inhibition of DNA and RNA synthesis. Moreover, sanguinarine can bind to negatively charged membrane surfaces and proteins as heteroaromatic iminium cation (Han et al., 2016) and it inhibits choline acetyltransferase activity in arthropods and vertebrates.
Sanguinarine, chelerythrine, and other BIAs also show antibacterial and antifungal activities (Zhao et al., 2019), whereas their direct effects on these pathogens was not analyzed in planta. Interestingly, antiplaque mouth washes and toothpaste containing sanguinarine were once commercially available, but were later removed from supermarket shelves due to their dramatic side effect: leukoplakia, a pre-malignant condition, of the maxillary vestibule had occurred in some consumers (Laines-Hidalgo et al., 2022).
It is further important to notice that some plant-derived alkaloids, such as protopine and (+)-N-methyllaurotetanine reduced the human cardiac ether-a-go-go-related gene (hERG) expression and poses a potential risk for human hERG toxicity (Schramm et al., 2014).
3.1 Alkaloids
The BIA pathway in California poppy provides a convenient system to study the regulation of biosynthesis and the physiological roles of BIAs (Figure 2). And while Coptis japonica (https://www.sciencedirect.com/topics/biochemistry-genetics-and-molecular-biology/coptis), Thalictrum thalictroides (https://www.sciencedirect.com/topics/pharmacology-toxicology-and-pharmaceutical-science/thalictrum), and Papaver somniferum (https://www.sciencedirect.com/topics/biochemistry-genetics-and-molecular-biology/papaver-somniferum) are also used to study BIAs, California poppy provides practical advantages. The plants are easy and permit-free to cultivate, efficient methods for cell cultures and genetic transformations are available as discussed below (Hagel and Facchini, 2013; Sato, 2013; Lotz et al., 2022).
HPLC or LC-MS analyses easily reveal alkaloid composition of California poppy (Liscombe et al., 2009; Hori et al., 2018), whereas the more traditional TLC method is also still in use (Balažová et al., 2020). Kukula-Koch (2017) reported an optimized method for the LC-ESI-Q-TOF-MS analysis for the extracts of Papaveraceae and Berberidaceae families (genera: Papaver, Argemone, Eschscholzia, Chelidonium, Glaucium, and Berberis), providing even more sensitive and precise method for BIA characterization. Based on the identification of alkaloid chemical structures and tracer experiments, the major alkaloid biosynthesis pathways and biosynthetic enzymes of BIAs have been characterized at the molecular level (Hagel and Facchini, 2013; Sato, 2013; Sato, 2020)
BIA biosynthesis commences with the conversion of tyrosine to both dopamine and 4-hydroxyphenylacetaldehyde (4HPAA) by tyrosine/dopa decarboxylase (TDC), or 4-hydroxyphenylpyruvate decarboxylase (HPDC), phenol oxidase (PO), and tyrosine amino transferase (TAT) (Figure 2, brown pathway). Dopamine and 4HPAA are condensed by norcoclaurine synthase (NCS) and yield (S)-norcoclaurine (Samanani and Facchini, 2001). (S)-Norcoclaurine is sequentially converted to (S)-reticuline by norcoclaurine 6-O-methyltransferase (6OMT) (Inui et al., 2007), coclaurine N-methyltransferase (CNMT), N-methylcoclaurine hydroxylase (CYP80B1; MCH) (Pauli and Kutchan, 1998), and 3’-hydroxy N-methylcoclaurine 4’-O-methyltransferase (4’OMT) (Inui et al., 2007).
(S)-Reticuline is converted to (S)-scoulerine by berberine bridge enzyme (BBE), then benzophenanthridine alkaloids (e.g., sanguinarine and macarpine) (Figure 2, red pathway in root and cultured cells) (Dittrich and Kutchan, 1991; Fujii et al., 2007; Ikezawa et al., 2007, 2009; Liscombe et al., 2009; Hagel et al., 2012; Beaudoin and Facchini, 2013; Takemura et al., 2013; Purwanto et al., 2017). Two enzymes unique to California poppy and identified based on draft genome sequence mining are dihydrobenzophenathridine hydroxylase (DB10H) and OMT required for dihydrochelirubine biosynthesis (Hori et al., 2018). Importantly, California poppy cell cultures can self-detoxify exogenously added benzophenanthridines by sanguinarine reductase (SR; Vogel et al., 2010). As shown in Figure 2, the biosynthetic pathway of sanguinarine and related chelerythrine and chelirubine in underground parts and cultured cells of California poppy has been almost completely elucidated.
The characterization of BIA biosynthetic enzymes was the prerequisite to elucidate the mechanisms of their transcriptional regulation (Yamada and Sato, 2021). Firstly, CjWRKY1 and CjbHLH1 were identified as comprehensive transcriptional activators of biosynthetic enzyme genes in BIA biosynthesis of C. japonica cells (Kato et al., 2007; Yamada et al., 2011a). Later, their homologs were isolated from California poppy (EcWRKY and EcbHLH1-1/1-2) allowing for the characterization of the regulation of BIA biosynthesis in California poppy (Yamada et al., 2015; Yamada et al., 2021b). Interestingly, CjbHLH1 and EcbHLH1-1/1-2 are non-MYC2-type bHLH transcription factors and their homologs are only found in BIA-producing plant species (Yamada et al., 2011b). Whereas WRKYs were also identified in California poppy genome, the heterologous expression of CjWRKY1 in California poppy showed limited activation of BIA biosynthetic enzyme genes and only partial increase in BIA production, suggesting that their function is not fully interchangeable (Yamada et al., 2017). Detailed genome-wide analysis of the California poppy WRKY transcription factor family combined with transcriptome analysis suggested that this gene family is involved in the regulation of BIA biosynthesis and is possibly also associated with the accumulation and translocation of BIAs in California poppy (Yamada et al., 2021b).
Further transcriptome analysis and genome mining revealed several transcription factor genes that are strongly upregulated in response to methyl jasmonate (MeJA), such as EcAP2/ERF2, EcAP2/ERF3 and EcAP2/ERF4 (Yamada et al., 2020). MeJA also sequentially induced the expression of bHLH and WRKY genes as well as of BIA biosynthetic enzyme and transporter genes (Yamada and Sato, 2021). This information on additional BIA regulatory transcription factors and MeJA as inducing phytohormone, all obtained in California poppy, can be highly useful to dissect the regulation of BIA biosynthesis in diverse plant species.
Unfortunately, molecular information of the genes encoding the biosynthetic enzymes for pavine-type BIAs in the aerial parts of California poppy, such as californidine and escholtzine (Figure 2, green pathway) is still missing (Hori et al., 2018). Moreover, while pavine-type alkaloids are most prominent in leaves, their physiological role is unknown. And, the important question on why pavine-type alkaloids are found in leaves and benzophenanthridine alkaloids accumulate in roots and how this relates to the defense against different pathogens would be interesting to study in California poppy.
We also note that California poppy has limitations, due to the lack of some commercially important BIAs, such as noscapine and morphinan alkaloids (Hagel and Facchini, 2013; Sato, 2020). The biosynthesis of morphinan alkaloids in opium poppy requires cell-type-specific localization of the biosynthetic enzymes. In situ localization of their transcripts indicated that seven biosynthetic enzymes (6OMT, CNMT, CYP80B, 4’OMT and BBE involved in reticuline biosynthesis, and SAT and COR in the morphine pathway) were localized in sieve elements, whereas proteins were localized in the supporting companion cells, demonstrating a complex spatial organization of morphinan alkaloids (Lee et al., 2013; Ozber et al., 2022). California poppy could serve as preferred host system to reconstruct the morphinan pathway including genes encoding for the biosynthetic enzymes, translocators, and transcription factors genes involved in cell differentiation. This would be a prime example for synthetic biology to recreate a highly complex biosynthetic pathway of a commercially extremely valuable pharmaceutical.
Undoubtedly, the California poppy draft genome provides a useful platform to study the evolution of BIA biosynthesis and its regulation. Phylogenomic approaches using whole genome sequences of five benzylisoquinoline alkaloid (BIA)-producing species from the Ranunculales and Proteales orders including California poppy revealed the sequence and timing of evolutionary events leading to the diversification of BIA alkaloids (Li et al., 2020). 1-Benzylisoquinoline is a pivotal intermediate in the synthesis of many BIAs and phylogenomic analyses revealed parallel evolution in the orders of Ranunculales and Proteales, which diverged -122 million years ago (MYA), with the Ranunculales producing (S)-reticuline and the Proteales the related (R/S)-norcoclaurine. Berberine is present in species across the Ranunculales but lacking in Proteales, and homologs of genes essential for the protoberberine class production were found throughout the Ranunculales. However, benzophenanthridine class is specific to the Papaveraceae family within Ranunculales (Figure 1), and its biosynthetic genes emerged after the Papaveraceae separated from the other Ranunculales, around 110 MYA. Their origin also predates the split of the three Papaveraceae species (opium poppy, California poppy, and Macleaya cordata) at approximately 77 MYA. The phthalideisoquinoline noscapine and morphinan classes of BIAs are exclusive to the opium poppy lineage (Li et al., 2020). In addition, predicted protein-encoding genes and comparative analysis using genome sequences of BIA-producing plants, opium poppy and Aquilegia coerulea, showed many additional candidate genes encoding for biosynthetic enzymes, transcription factors, and transporter genes involved in the BIA pathway (Yamada et al., 2021a).
4 Genetic resources
The species California poppy shows a stunning variation in floral and vegetative traits observed in natural populations and its adaptability suggests high genetic variation. Cytological observations and classical genetic experiments by Ernst and Cook (Ernst, 1958; Cook, 1962) revealed that California poppy has 6 chromosomes, and is self-incompatible to the largest extent, even in the naturalized populations, and genetic barriers to outcrossing were not identified so far. The powdery nature of the pollen allows also for wind pollination, but the flowers are mainly pollinated by insects. While the large flowers do not exudate nectar, they abundantly provide pollen. Beetles, bees and bumblebees contribute significantly to California poppy pollination, but also thrips and hover flies were observed to visit the flowers (Cook, 1962). The open breeding system of this species allows novel adaptive trait combinations and permits differentially adapted population existing close to each other (Cook, 1962) and may be a prerequisite of its invasiveness.
4.1 Sequence resources
Several genetic resources have been developed to facilitate California poppy research with the advantages of relatively small genome size (503.8 Mb): 14 microsatellite markers are available for population genetic analysis that have been utilized to characterize the highly invasive populations in Chile (Véliz et al., 2012). And EST database has been established to facilitate gene discovery (Carlson et al., 2006), and the sequence information garnered from the ESTs was used to generate microarrays for differential gene expression studies (Zahn et al., 2010). In addition to these transcriptomes, RNAseq data for different stages of carpel development obtained by laser microdissection are available from California poppy (Kivivirta et al., 2019).
Meanwhile, a draft genome sequence was published (Hori et al., 2018; Eschscholzia Genome DataBase, http://eschscholzia.kazusa.or.jp) and a reference-quality genome sequencing effort in combination with a transcriptome atlas is about to be completed by the Open Green Genome initiative with the data being deposited in the phytozome database (OGG, https://phytozome-next.jgi.doe.gov/ogg/) for easy access. Further, we are generating a large number of high quality transcriptome data for diverse tissues and these will be available for the research community via a web-based multi-omics platform. This will allow in silico analysis of the genome and digital gene expression analysis along the entire transcriptome and be useful for gene network constructions.
4.2 Resources for genetic manipulation
In addition to sequence information, questions regarding the conservation of gene functions can be addressed by knocking down gene expression of target genes by Virus-Induced Gene Silencing (VIGS). This method allows gene function analysis in a simple and time efficient way by manipulating the plant’s immune reaction towards RNA viruses such that the expression of endogenous genes is reduced (Wege et al., 2007; Rössner et al., 2022). This method was employed several times to elucidate the function of transcription factors involved in vegetative and reproductive development (Orashakova et al., 2009; Yellina et al., 2010; Lange et al., 2013; Stammler et al., 2013; Zhao et al., 2018). While the effect of VIGS is transient and cannot be transferred to subsequent generations, a second, reliable method for stable transformation and regeneration of mature plants is now available (Park and Facchini, 2000; Lotz et al., 2022). Here, Agrobacterium tumefaciens-mediated transformation of cotyledons and subsequent regeneration is works efficiently but is time-consuming in California poppy, requiring at least eight months from the transformation event to mature plants (Park and Facchini, 2000; Lotz et al., 2022).
Whereas prolonged modulation of gene expression with A. tumefaciens mediated stable transformation, or VIGS/virus-based expression is useful for the functional characterization of biosynthetic enzyme or developmental genes, transient assays using protoplasts are faster to characterize certain gene functions such as transcription factor genes regulating BIA biosynthesis. Protoplasts are prepared from plant cells after the digestion of cell walls with cellulase, pectinase and other cell wall digesting enzymes. Protoplasts take up DNA, RNA, or proteins easily when treated with polyethylene glycol (PEG), or electric stimulus. For example, the efficacy of double-stranded (ds) RNAs prepared against candidate transcription factor encoding genes or over-expression plasmids for transcription factor encoding genes were examined in protoplasts of C. japonica, a relevant model for BIA-producing plants using PEG-mediated transformation. Suppression effects of TFs on biosynthetic enzyme genes were successfully monitored by quantitative reverse transcription (RT)-polymerase chain reaction (PCR) in C. japonica (Dubouzet et al., 2005). Since mesophyll protoplasts are rather easily isolated from California poppy leaves (data not shown), similar system will be applicable, for example to characterize the pavine-biosynthesis in leaf tissue.
4.3 Cell culture systems
California poppy cell cultures have been intensively used to study BIA pathway, since cell cultures produce the major BIAs and provides sufficient materials for biochemical and molecular genetics characterization (Sato, 2013). Cell culture systems are also useful to modify biosynthetic pathways using genetically transformed cultures (Sato et al., 2001; Fujii et al., 2007; Inui et al., 2007), and to test the effect of chemicals such as elicitors from pathogens or MeJA as BIA pathway activator (Tanahashi and Zenk, 1990; Färber et al., 2003; Ikezawa et al., 2009).
In fact, introduction of the C. japonica scoulerine 9-O-methyltransferase (CjSMT) gene into BIA biosynthesis in a California poppy cell culture system shifted the metabolic flow from the sanguinarine type to chelerythrine type (Sato et al., 2001, Figure 2). Whereas both introduced CjSMT and endogenous cheilanthifoline synthase (EcCYP719A2/A3) accept scoulerine as substrate, the highly reactive CjSMT dominated the pathway when compared to the endogenous EcCYP719A2/A3. Similarly, when the C. japonica (S)-tetrahydroberberine oxidase (CjTHBO) was introduced to California poppy cells, CjTHBO hijacked the intermediates in benzophenanthridine biosynthesis to convert them into protoberberine type products (Matsushima et al., 2012).
The physiological role of down-regulation using antisense RNA, co-suppression or gene-knockout with CRISPR/Cas 9 is also effectively monitored in cell culture systems. For example, the effects of RNA-interference (RNAi) of berberine bridge enzyme (BBE) gene in BIA pathway can be detected as the accumulation of the key intermediate reticuline with substantial production of 7-O-methylated derivative of reticuline, laudanine, which indicates the dynamics of metabolism (Fujii et al., 2007).
Sterilized California poppy seedlings grown on 1% agar medium containing Murashige–Skoog inorganic salts under continuous light (100 μE/m2/s) at 25°C are preferable materials for cell culture or transformation with Agrobacterium tumefaciens. Calli generally form after 2 months (about three successive selection cultures) on culture medium containing appropriate plant growth factors such as auxin, cytokinin, and antibiotics for selecting the transgenics. Transformation efficiency, regeneration/callus formation efficiency, and secondary metabolite productivity can vary considerably and require seed variety comparisons as preliminary experiments. For the establishment of an embryogenic culture, juvenile tissues such as shoot meristem and immature seed are often preferred materials (Takemura et al., 2010). Whereas Cauliflower Mosaic Virus 35S is a commonly used promoter sequence to over-express desired genes constitutively in host plant cells, gene expression in specific tissues or developmental stages requires carefully selected promoters and even enhancers. Thus, for alkaloid engineering, more research on the regulation of metabolic pathway and specific gene expression profiles is needed. One example for a comprehensive characterization of biosynthetic enzymes was done with the genes encoding for the two C. japonica enzymes norcoclaurine 6-O methyltransferase (Cj6OMT) and 3’-hydroxy-N-methylcoclaurine 4’-O-methyltransferase (Cj4’OMT). They were over-expressed in California poppy cell cultures and showed different effects. Over-expression of Cj6OMT increased the alkaloid content to 7.5 times greater than that of the wild type, whereas the over-expression of Cj4’OMT had only a marginal effect (Inui et al., 2007).
Cell culture systems proved also useful to dissect BIA induction and its role in the molecular mechanism of phytopathogen defense. Whereas jasmonate treatment is commonly used to activate BIA biosynthesis (Färber et al., 2003; Ikezawa et al., 2009), Balažová et al. (2020) examined salicylic acid (SA), and simultaneous or sequential treatment of SA and L-tyrosine in cell cultures to enhance production of macarpine, a BIA specific to few Papaveraceae species only. Angelova et al. (2010) used root-derived cell cultures to characterize the elicitation mechanism, initiated by a short contact to low concentrations of a yeast glycoprotein elicitor, which led to the transient acidification of the cytoplasm. In contrast to low concentration treatment, high elicitor concentration signal increased jasmonate concentration and triggered hypersensitive cell death, resulting in massive mRNA decay.
Taken together, transient and stable genetic transformation methods have been established for California poppy to interrogate gene functions in different contexts. For metabolic engineering and the analysis of metabolite biosynthesis regulation, stable genetic transformation of cell culture systems have been used extensively. For the analysis of developmental regulators, fully grown plants are required and VIGS was used efficiently to unravel their function and regulatory circuits, even though this method is transient. The novel method for stable transformation and regeneration of California poppy will provide even more possibilities, especially for targeted, heritable mutagenesis by CRISPR-Cas.
5 Outlook
California poppy’s high level of genetic diversity comes with the cost of being an obligate outcrossing plant. This suggests that the level of heterozygosity is high and the isogenic and even near-isogenic lines production is very challenging. However, homozygous mutants can be created by sibling crossing. Moreover, CRISPR-Cas guided genome editing introduced by Agrobacterium-mediated transformation and regeneration provides an efficient means to elucidate gene function in homozygous knock-out mutants. Further, VIGS can be combined with CRISPR-Cas such that the guide RNAs may be delivered by VIGS to a plant carrying a CRISPR-Cas expressing transgene. These future developments of the California poppy toolkit will enhance the potential of this already established model organism to study, for example, BIA biosynthesis and its regulation in fully grown plants and link this with developmental genetics analyses. Availability of many high quality transcriptome datasets allows the calculation of gene networks based on genes’ co-expression to identify whole modules of putatively interacting genes. This type of analysis is independent of candidate genes associated to biological processes in other species, such as Arabidopsis, and is thus bias-free.
Additionally to California poppy genome and transcriptome datasets being generated, these datasets are becoming available also for other Ranunculales, allowing comparative analyses. Several Ranunculales genomes have been published recently, some even at chromosome-level, including M. cordata (Liu et al., 2017), Coptis chinensis (Liu et al., 2021), P. somniferum (Guo et al., 2018), Papaver rhoeas, Papaver setigerum (Yang et al., 2021), and A. coerulea (Filiault et al., 2018), Aquilegia oxysepala (Xie et al., 2020), Thalictrum thalictroides (Arias et al., 2021), Kingdonia uniflora (Sun et al., 2020), Akebia trifoliata (Huang et al., 2021), and Corydalis tomentella (Xu et al., 2022).
While genome sequencing requires the extraction of a single sample of high molecular weight DNA, transcriptome analysis requires the collection of several biological replicates for many tissues, stages, and/or treatments, rendering this method more time consuming and laborious. While for Nigella damascena, A. coerulea, and T. thalictroides more extensive transcriptomes datasets have already been published facilitating gene identification in Ranunculales and gene expression analysis to a limited extent (Meaders et al., 2020; Zhang et al., 2020; Arias et al., 2021). However, the datasets often comprise only few tissues and an insufficient number of replicates to allow for digital gene expression analysis. Furthermore, gene expression comparison of orthologous genes is challenging at the present state, if possible at all. Comparable datasets including representatives covering all Ranunculales subfamilies is desirable for cross-species comparison of gene expression data. Sufficient expression data for each representative species is further required for co-expressed gene network calculations and to compare these networks between species to learn about novel network nodes that may correspond to morphological or metabolic novelties in the Ranunculales.
Furthermore, California poppy allows the fusion of two formerly separated fields in plant biology: developmental genetics and regulation of metabolism: floral homeotic genes specify floral organ identity and any anomaly in the structure or expression of these genes apparently may result in morphological variations of the flower/capsule and consequently in the alkaloid yield. One such recessive mutation, aco (androcarpel organ), has been described in the opium poppy (Prajapati, 2002), in which androcarpels are formed in place of stamens in the mutant flowers. The androcarpel walls synthesized and accumulated alkaloids similar to the main carpel walls and thus provided a means for increasing the carpel wall husk mass and alkaloid yield. Singh et al. (2017) also reported the presence of major BIAs in the carpeloid stamens of the floral homeotic mutant OM, unlike in wild type stamens, indicating functional similarities between the carpeloid stamen and the capsule wall in their capacity to synthesize BIAs. Whereas California poppy does not synthesize morphinan alkaloids, it also accumulates BIAs in floral organs and thus allows the study of the interplay of regulatory genes in flower morphogenesis and specialized metabolism, i.e., carotenoid and BIA biosynthesis for crop improvement in future breeding programs of Papaveraceae species.
Author contributions
AB, YY, and FS wrote the draft and final version of the manuscript. All authors contributed to the article and approved the submitted version.
Funding
Research on California poppy in AB’s group has been funded continuously by the German Research Foundation (DFG, grants BE2547/3-1; 6-1; 6-2; 7-2; 14-1; 24-1, 27-1). California poppy research in YY’s group was funded by the Ministry of Education, Culture, Sports, Science and Technology of Japan (MEXT, Grant-in-Aid for Young Scientists 21K14830) and partially by JSPS, PRESTO (Grant Number JPMJPR21DA), Japan. Work in FS’s lab on California poppy was funded by MEXT (Grant-in-Aid for Scientific Research (S) 26221201).
Conflict of interest
The authors declare that the research was conducted in the absence of any commercial or financial relationships that could be construed as a potential conflict of interest.
Publisher’s note
All claims expressed in this article are solely those of the authors and do not necessarily represent those of their affiliated organizations, or those of the publisher, the editors and the reviewers. Any product that may be evaluated in this article, or claim that may be made by its manufacturer, is not guaranteed or endorsed by the publisher.
References
Al-Snafi, A. E. (2017). Eschscholzia californica: A phytochemical and pharmacological - review. doi: 10.5281/zenodo.344931
Angelova, S., Buchheim, M., Frowitter, D., Schierhorn, A., Roos, W. (2010). Overproduction of alkaloid phytoalexins in California poppy cells is associated with the co-expression of biosynthetic and stress-protective enzymes. Mol. Plant 3, 927–939. doi: 10.1093/mp/ssq043
Arias, T., Riaño-Pachón, D. M., Di Stilio, V. S. (2021). Genomic and transcriptomic resources for candidate gene discovery in the ranunculids. Appl. Plant Sci. 9, e11407. doi: 10.1002/aps3.11407
Balažová, A., Urdová, J., Forman, V., Mučaji, P. (2020). Enhancement of macarpine production in Eschscholzia californica suspension cultures under salicylic acid elicitation and precursor supplementation. Molecules 25, 1261. doi: 10.3390/molecules25061261
Barrell, P. J., Wakelin, A. M., Gatehouse, M. L., Lister, C. E., Conner, A. J. (2010). Inheritance and epistasis of loci influencing carotenoid content in petal and pollen color variants of california poppy (Eschscholzia californica cham.). J. Hered 101, 750–756. doi: 10.1093/jhered/esq079
Beaudoin, G. A. W., Facchini, P. J. (2013). Isolation and characterization of a cDNA encoding (S)-cis-N-methylstylopine 14-hydroxylase from opium poppy, a key enzyme in sanguinarine biosynthesis. Biochem. Biophys. Res. Commun. 431, 597–603. doi: 10.1016/j.bbrc.2012.12.129
Becker, A., Gleissberg, S., Smyth, D. R. (2005). Floral and vegetative morphogenesis in California poppy (Eschscholzia californica cham.). Int. J. Plant Sci. 166, 537–555. doi: 10.1086/429866
Bowman, J. L., Smyth, D. R. (1999). CRABS CLAW, a gene that regulates carpel and nectary development in Arabidopsis, encodes a novel protein with zinc finger and helix-loop-helix domains. Development 126, 2387–2396. doi: 10.1242/dev.126.11.2387
Carlson, J. E., Leebens-Mack, J. H., Wall, P. K., Zahn, L. M., Mueller, L. A., Landherr, L. L., et al. (2006). EST database for early flower development in California poppy (Eschscholzia californica cham., Papaveraceae) tags over 6,000 genes from a basal eudicot. Plant Mol. Biol. 62, 351–369. doi: 10.1007/s11103-006-9025-y
Chen, Y.-Y., Hsiao, Y.-Y., Li, C.-I., Yeh, C.-M., Mitsuda, N., Yang, H.-X., et al. (2021). The ancestral duplicated DL/CRC orthologs, PeDL1 and PeDL2, function in orchid reproductive organ innovation. J. Exp. Bot. 72, 5442–5461. doi: 10.1093/jxb/erab195
Cook, S. A. (1962). Genetic system, variation, and adaptation in Eschscholzia californica. Evolution 16, 278. doi: 10.2307/2406277
Dittrich, H., Kutchan, T. M. (1991). Molecular cloning, expression, and induction of berberine bridge enzyme, an enzyme essential to the formation of benzophenanthridine alkaloids in the response of plants to pathogenic attack. Proc. Natl. Acad. Sci. U.S.A. 88, 9969–9973. doi: 10.1073/pnas.88.22.9969
Dubouzet, J. G., Morishige, T., Fujii, N., An, C.-I., Fukusaki, E., Ifuku, K., et al. (2005). Transient RNA silencing of scoulerine 9-O-methyltransferase expression by double stranded RNA in Coptis japonica protoplasts. Biosci. Biotechnol. Biochem. 69, 63–70. doi: 10.1271/bbb.69.63
Ernst, W. R. (1958). Chromosome numbers of some western Papaveraceae. Contr. Dudley Herb. 5, 109–115.
Färber, K., Schumann, B., Miersch, O., Roos, W. (2003). Selective desensitization of jasmonate- and pH-dependent signaling in the induction of benzophenanthridine biosynthesis in cells of Eschscholzia californica. Phytochemistry 62, 491–500. doi: 10.1016/S0031-9422(02)00562-9
Fedurco, M., Gregorová, J., Šebrlová, K., Kantorová, J., Peš, O., Baur, R., et al. (2015). Modulatory effects of Eschscholzia californica alkaloids on recombinant GABAA receptors. Biochem. Res. Int. 2015, 617620. doi: 10.1155/2015/617620
Ferrandiz, C., Liljegren, S. H., Yanofsky, M. (2000). Negative regulation of the SHATTERPROOF genes by FRUITFULL during Arabidopsis fruit development. Science 289, 436–438. doi: 10.1126/science.289.5478.436
Filiault, D. L., Ballerini, E. S., Mandáková, T., Aköz, G., Derieg, N. J., Schmutz, J., et al. (2018). The Aquilegia genome provides insight into adaptive radiation and reveals an extraordinarily polymorphic chromosome with a unique history. Elife 7, e36426. doi: 10.7554/eLife.36426
Fourquin, C., Ferrándiz, C. (2014). The essential role of NGATHA genes in style and stigma specification is widely conserved across eudicots. New Phytol. 202, 1001–1013. doi: 10.1111/nph.12703
Fujii, N., Inui, T., Iwasa, K., Morishige, T., Sato, F. (2007). Knockdown of berberine bridge enzyme by RNAi accumulates (S)-reticuline and activates a silent pathway in cultured California poppy cells. Transgenic Res. 16, 363–375. doi: 10.1007/s11248-006-9040-4
Groß, T., Broholm, S., Becker, A. (2018). CRABS CLAW acts as a bifunctional transcription factor in flower development. Front. Plant Sci. 9, 835. doi: 10.1093/pcp/pct020
Guo, L., Winzer, T., Yang, X., Li, Y., Ning, Z., He, Z., et al. (2018). The opium poppy genome and morphinan production. Science 362, 343–347. doi: 10.1126/science.aat4096
Hagel, J. M., Beaudoin, G. A. W., Fossati, E., Ekins, A., Martin, V. J. J., Facchini, P. J. (2012). Characterization of a flavoprotein oxidase from opium poppy catalyzing the final steps in sanguinarine and papaverine biosynthesis. J. Biol. Chem. 287 (51), 42972–42983. doi: 10.1074/jbc.M112.420414
Hagel, J. M., Facchini, P. J. (2013). Benzylisoquinoline Alkaloid Metabolism: A Century of Discovery and a Brave New World. Plant Cell Physiol. 54, 647–672. doi: 10.1093/pcp/pct020
Han, N., Yang, Z., Liu, Z., Liu, H., Yin, J. (2016). Research progress on natural benzophenanthridine alkaloids and their pharmacological functions: A review. Nat. Prod. Commun. 11, 1181–1188. doi: 10.1177/1934578X1601100838
Hoot, S. B., Wefferling, K. M., Wulff, J. A. (2015). Phylogeny and character evolution of Papaveraceae s. l. (Ranunculales). Syst. Bot. 40, 474–488. doi: 10.1600/036364415X688718
Hori, K., Yamada, Y., Purwanto, R., Minakuchi, Y., Toyoda, A., Hirakawa, H., et al. (2018). Mining of the uncharacterized cytochrome P450 genes involved in alkaloid biosynthesis in California poppy using a draft genome sequence. Plant Cell Physiol. 59, 222–233. doi: 10.1093/pcp/pcx210
Huang, H., Liang, J., Tan, Q., Ou, L., Li, X., Zhong, C., et al. (2021). Insights into triterpene synthesis and unsaturated fatty-acid accumulation provided by chromosomal-level genome analysis of Akebia trifoliata subsp. australis. Hortic. Res. 8, 33. doi: 10.1038/s41438-020-00458-y
Ikezawa, N., Iwasa, K., Sato, F. (2007). Molecular cloning and characterization of methylenedioxy bridge-forming enzymes involved in stylopine biosynthesis in Eschscholzia californica. FEBS J. 274, 1019–1035. doi: 10.1111/j.1742-4658.2007.05652.x
Ikezawa, N., Iwasa, K., Sato, F. (2009). CYP719A subfamily of cytochrome P450 oxygenases and isoquinoline alkaloid biosynthesis in Eschscholzia californica. Plant Cell Rep. 28, 123–133. doi: 10.1007/s00299-008-0624-8
Inui, T., Tamura, K.-I., Fujii, N., Morishige, T., Sato, F. (2007). Overexpression of Coptis japonica norcoclaurine 6-O-methyltransferase overcomes the rate-limiting step in benzylisoquinoline alkaloid biosynthesis in cultured Eschscholzia californica. Plant Cell Physiol. 48, 252–262. doi: 10.1093/pcp/pcl062
Kato, N., Dubouzet, E., Kokabu, Y., Yoshida, S., Taniguchi, Y., Dubouzet, J. G., et al. (2007). Identification of a WRKY protein as a transcriptional regulator of benzylisoquinoline alkaloid biosynthesis in Coptis japonica. Plant Cell Physiol. 48, 8–18. doi: 10.1093/pcp/pcl041
Kivivirta, K., Herbert, D., Lange, M., Beuerlein, K., Altmüller, J., Becker, A. (2019). A protocol for laser microdissection (LMD) followed by transcriptome analysis of plant reproductive tissue in phylogenetically distant angiosperms. Plant Methods 15, 151. doi: 10.1186/s13007-019-0536-3
Kukula-Koch, W. (2017). The elevation of LC-ESI-Q-TOF-MS response in the analysis of isoquinoline alkaloids from some Papaveraceae and Berberidaceae representatives. J. Anal. Methods Chem. 2017, 8384107. doi: 10.1155/2017/8384107
Laines-Hidalgo, J. I., Muñoz-Sánchez, J. A., Loza-Müller, L., Vázquez-Flota, F. (2022). An update of the sanguinarine and benzophenanthridine alkaloids' biosynthesis and their applications. Molecules 27, 1378. doi: 10.3390/molecules27041378
Lane, A. K., Augustin, M. M., Ayyampalayam, S., Plant, A., Gleissberg, S., Di Stilio, V. S., et al. (2018). Phylogenomic analysis of Ranunculales resolves branching events across the order. Bot. J. Linn. Soc. 187, 157–166. doi: 10.1093/botlinnean/boy015
Lange, M., Orashakova, S., Lange, S., Melzer, R., Theißen, G., Smyth, D. R., et al. (2013). The seirena b class floral homeotic mutant of California poppy (Eschscholzia californica) reveals a function of the enigmatic PI motif in the formation of specific multimeric MADS domain protein complexes. Plant Cell 25, 438–453. doi: 10.1105/tpc.112.105809
Lee, E.-J., Hagel, J. M., Facchini, P. J. (2013). Role of the phloem in the biochemistry and ecophysiology of benzylisoquinoline alkaloid metabolism. Front. Plant Sci. 4. doi: 10.3389/fpls.2013.00182
Leger, E. A., Rice, K. J. (2007). Assessing the speed and predictability of local adaptation in invasive California poppies (Eschscholzia californica). J. Evol. Biol. 20, 1090–1103. doi: 10.1111/j.1420-9101.2006.01292.x
Li, Y., Winzer, T., He, Z., Graham, I. A. (2020). Over 100 million years of enzyme evolution underpinning the production of morphine in the Papaveraceae family of flowering plants. Plant Commun. 1, 100029. doi: 10.1016/j.xplc.2020.100029
Liscombe, D. K., Ziegler, J., Schmidt, J., Ammer, C., Facchini, P. J. (2009). Targeted metabolite and transcript profiling for elucidating enzyme function: isolation of novel N-methyltransferases from three benzylisoquinoline alkaloid-producing species. Plant J. 60, 729–743. doi: 10.1111/j.1365-313X.2009.03980.x
Liu, X., Liu, Y., Huang, P., Ma, Y., Qing, Z., Tang, Q., et al. (2017). The genome of medicinal plant Macleaya cordata provides new insights into benzylisoquinoline alkaloids metabolism. Mol. Plant 10, 975–989. doi: 10.1016/j.molp.2017.05.007
Liu, Y., Wang, B., Shu, S., Li, Z., Song, C., Liu, D., et al. (2021). Analysis of the Coptis chinensis genome reveals the diversification of protoberberine-type alkaloids. Nat. Commun. 12, 3276. doi: 10.1038/s41467-021-23611-0
Lotz, D., Imani, J., Ehlers, K., Becker, A. (2022). Towards a genetic model organism: an efficient method for stable genetic transformation of Eschscholzia californica (Ranunculales). Plant Cell Tiss Organ Cult. 149, 823–832. doi: 10.1007/s11240-021-02223-y
Maoka, T., Fujiwara, Y., Hashimoto, K., Takeda, S., Takaragaki, S., Ida, K. (2000). A new retro-carotenoid from the petals of the Californian yellow poppy Eschscholzia californica. J. Nat. Prod. 63, 1288–1289. doi: 10.1021/np0000670
Matsushima, Y., Minami, H., Hori, K., Sato, F. (2012). Pathway engineering of benzylisoquinoline alkaloid biosynthesis in transgenic California poppy cells with ectopic expression of tetrahydroberberine oxidase from Coptis japonica. Plant Biotechnol. 29, 473–481. doi: 10.5511/plantbiotechnology.12.1101a
Mazuecos-Aguilera, I., Romero-García, A. T., Klodová, B., Honys, D., Fernández-Fernández, M. C., Ben-Menni Schuler, S., et al. (2021). The role of INAPERTURATE POLLEN1 as a pollen aperture factor is conserved in the basal eudicot Eschscholzia californica (Papaveraceae). Front. Plant Sci. 12. doi: 10.3389/fpls.2021.701286
Meaders, C., Min, Y., Freedberg, K. J., Kramer, E. (2020). Developmental and molecular characterization of novel staminodes in Auilegia. Ann. Bot. 126, 231–243. doi: 10.1093/aob/mcaa029
Murai, K., Miyamae, M., Kato, H., Takumi, S., Ogihara, Y. (2003). WAP1, a wheat APETALA1 homolog, plays a central role in the phase transition from vegetative to reproductive growth. Plant Cell Phys. 44, 1255–1265. doi: 10.1093/pcp/pcg171
Orashakova, S., Lange, M., Lange, S., Wege, S., Becker, A. (2009). The CRABS CLAW ortholog from California poppy (Eschscholzia californica, Papaveraceae), EcCRC, is involved in floral meristem termination, gynoecium differentiation and ovule initiation. Plant J. 58, 682–693. doi: 10.1111/j.1365-313X.2009.03807.x
Ozber, N., Carr, S. C., Morris, J. S., Liang, S., Watkins, J. L, Caldo, K. M., et al (2022). Alkaloid binding to opium poppy major latex proteins triggers structural modification and functional aggregation. Nat. Commun. 13, 6768. doi: 10.1038/s41467-022-34313-6
Pabón-Mora, N., Ambrose, B. A., Litt, A. (2012). Poppy APETALA1/FRUITFULL orthologs control flowering time, branching, perianth identity, and fruit development. Plant Physiol. 158, 1685–1704. doi: 10.1104/pp.111.192104
Park, S.-U., Facchini, P. J. (2000). Agrobacterium-mediated genetic transformation of California poppy, Eschscholzia californica cham., via somatic embryogenesis. Plant Cell Rep. 19, 1006–1012. doi: 10.1007/s002990000213
Pauli, H. H., Kutchan, T. M. (1998). Molecular cloning and functional heterologous expression of two alleles encoding (S)-N-methylcoclaurine 3'-hydroxylase (CYP80B1), a new methyl jasmonate-inducible cytochrome P-450-dependent mono-oxygenase of benzylisoquinoline alkaloid biosynthesis. Plant J. 13, 793–801. doi: 10.1046/j.1365-313x.1998.00085.x
Pollack, A. J., Gong, X., Pollack, J. R. (2019). A common phytoene synthase mutation underlies white petal varieties of the California poppy. Sci. Rep. 9, 11615. doi: 10.1038/s41598-019-48122-3
Prajapati, S., Bajpai, S., Gupta, M.M. (2001). The floral androcarpel organ (ACO) mutation permits high alkaloid yields in opium poppy, Papaver somniferum. Curr. Sci. 81, 1109–1112.
Prajapati, S. (2002). The floral androcarpel (ACO) permits high alkaloid yields in opium poppy papaver somiferum. Curr. Sci., 1109–1112.
Purwanto, R., Hori, K., Yamada, Y., Sato, F. (2017). Unraveling additional O-methylation steps in benzylisoquinoline alkaloid biosynthesis in California poppy (Eschscholzia californica). Plant Cell Physiol. 58, 1528–1540. doi: 10.1093/pcp/pcx093
Rolland, A., Fleurentin, J., Lanhers, M. C., Younos, C., Misslin, R., Mortier, F., et al. (1991). Behavioural effects of the American traditional plant Eschscholzia californica: sedative and anxiolytic properties. Planta Med. 57, 212–216. doi: 10.1055/s-2006-960076
Rössner, C., Lotz, D., Becker, A. (2022). VIGS goes viral: How VIGS transforms our understanding of plant science. Annu. Rev. Plant Biol. 73, 703–728. doi: 10.1146/annurev-arplant-102820-020542
Samanani, N., Facchini, P. J. (2001). Isolation and partial characterization of norcoclaurine synthase, the first committed step in benzylisoquinoline alkaloid biosynthesis, from opium poppy. Planta 213, 898–906. doi: 10.1007/s004250100581
Sato, F. (2013). Characterization of plant functions using cultured plant cells, and biotechnological applications. Biosci. Biotechnol. Biochem. 77, 1–9. doi: 10.1271/bbb.120759
Sato, F. (2020). “Plant alkaloid engineering,” in Comprehensive natural products III (Oxford, UK: Elsevier), 700–755. doi: 10.1016/B978-0-12-409547-2.14696-7
Sato, F., Hashimoto, T., Hachiya, A., Tamura, K., Choi, K. B., Morishige, T., et al. (2001). Metabolic engineering of plant alkaloid biosynthesis. Proc. Natl. Acad. Sci. U.S.A. 98, 367–372. doi: 10.1073/pnas.98.1.367
Schmeller, T., Latz-Brüning, B., Wink, M. (1997). Biochemical activities of berberine, palmatine and sanguinarine mediating chemical defense against microorganisms and herbivores. Phytochemistry 44, 257–266. doi: 10.1016/S0031-9422(96)00545-6
Schramm, A., Saxena, P., Chlebek, J., Cahlíková, L., Baburin, I., Hering, S., et al. (2014). Natural products as potential human ether-a-go-go-related gene channel inhibitors - screening of plant-derived alkaloids. Planta Med. 80, 740–746. doi: 10.1055/s-0034-1368590
Scofield, S., Dewitte, W., Murray, J. A. H. (2007). The KNOX gene SHOOT MERISTEMLESS is required for the development of reproductive meristematic tissues in Arabidopsis. Plant J. 50, 767–781. doi: 10.1111/j.1365-313X.2007.03095.x
Singh, S. K., Gupta, S., Ahmad, N., Shukla, A. K., Shasany, A. K., Lal, R. K., et al. (2017). Variability and heritability studies in floral homeotic mutants of Papaver somniferum l. Ind. Crops Prod. 95, 276–285. doi: 10.1016/j.indcrop.2016.10.032
Stammler, A., Meyer, S. S., Plant, A. R., Townsley, B. T., Becker, A., Gleissberg, S. (2013). Duplicated STM-like KNOX I genes act in floral meristem activity in Eschscholzia californica (Papaveraceae). Dev. Genes Evol. 223, 289–301. doi: 10.1007/s00427-013-0446-8
Sun, Y., Deng, T., Zhang, A., Moore, M. J., Landis, J. B., Lin, N., et al. (2020). Genome sequencing of the endangered Kingdonia uniflora (Circaeasteraceae, Ranunculales) reveals potential mechanisms of evolutionary specialization. iScience 23, 101124. doi: 10.1016/j.isci.2020.101124
Takemura, T., Chow, Y., Todokoro, T., Okamoto, T., Sato, F. (2010). Over-expression of rate-limiting enzymes to improve alkaloid productivity. Methods Mol. Biol. 643, 95–109. doi: 10.1007/978-1-60761-723-5_7
Takemura, T., Ikezawa, N., Iwasa, K., Sato, F. (2013). Molecular cloning and characterization of a cytochrome P450 in sanguinarine biosynthesis from Eschscholzia californica cells. Phytochemistry 91, 100–108. doi: 10.1016/j.phytochem.2012.02.013
Tanahashi, T., Zenk, M. H. (1990). Elicitor induction and characterization of microsomal protopine-6-hydroxylase, the central enzyme in benzophenanthridine alkaloid biosynthesis. Phytochemistry 29, 1113–1122. doi: 10.1016/0031-9422(90)85414-B
Véliz, D., Gauci, R., Bustamante, R. O. (2012). Characterization of novel microsatellite markers for Eschscholzia californica (Papaveraceae), an invasive species in central Chile. Am. J. Bot. 99, e366–e368. doi: 10.3732/ajb.1200076
Vogel, M., Lawson, M., Sippl, W., Conarad, U., Roos, W. (2010). Structure and mechanism of sanguinarine reductase, an enzyme of alkaloid detoxification. J. Biol. Chem. 285, 18397–18406. doi: 10.1074/jbc.M109.088989
Wege, S., Scholz, A., Gleissberg, S., Becker, A. (2007). Highly efficient virus-induced gene silencing (VIGS) in California poppy (Eschscholzia californica): an evaluation of VIGS as a strategy to obtain functional data from non-model plants. Ann. Bot. 100, 641–649. doi: 10.1093/aob/mcm118
Wilts, B. D., Rudall, P. J., Moyroud, E., Gregory, T., Ogawa, Y., Vignolini, S., et al. (2018). Ultrastructure and optics of the prism-like petal epidermal cells of Eschscholzia californica (California poppy). New Phytol. 219, 1124–1133. doi: 10.1111/nph.15229
Xie, J., Zhao, H., Li, K., Zhang, R., Jiang, Y., Wang, M., et al. (2020). A chromosome-scale reference genome of Aquilegia oxysepala var. kansuensis. Hortic. Res. 7, 113. doi: 10.1038/s41438-020-0328-y
Xu, Z., Li, Z., Ren, F., Gao, R., Wang, Z., Zhang, J., et al. (2022). The genome of Corydalis reveals the evolution of benzylisoquinoline alkaloid biosynthesis in Ranunculales. Plant J. 111, 217–230. doi: 10.1111/tpj.15788
Yamada, Y., Hirakawa, H., Hori, K., Minakuchi, Y., Toyoda, A., Shitan, N., et al. (2021a). Comparative analysis using the draft genome sequence of California poppy (Eschscholzia californica) for exploring the candidate genes involved in benzylisoquinoline alkaloid biosynthesis. Biosci. Biotechnol. Biochem. 85, 851–859. doi: 10.1093/bbb/zbaa091
Yamada, Y., Kokabu, Y., Chaki, K., Yoshimoto, T., Ohgaki, M., Yoshida, S., et al. (2011a). Isoquinoline alkaloid biosynthesis is regulated by a unique bHLH-type transcription factor in Coptis japonica. Plant Cell Physiol. 52, 1131–1141. doi: 10.1093/pcp/pcr062
Yamada, Y., Koyama, T., Sato, F. (2011b). Basic helix-loop-helix transcription factors and regulation of alkaloid biosynthesis. Plant Signal Behav. 6, 1627–1630. doi: 10.4161/psb.6.11.17599
Yamada, Y., Motomura, Y., Sato, F. (2015). CjbHLH1 homologs regulate sanguinarine biosynthesis in Eschscholzia californica cells. Plant Cell Physiol. 56, 1019–1030. doi: 10.1093/pcp/pcv027
Yamada, Y., Nishida, S., Shitan, N., Sato, F. (2020). Genome-wide identification of AP2/ERF transcription factor-encoding genes in California poppy (Eschscholzia californica) and their expression profiles in response to methyl jasmonate. Sci. Rep. 10, 18066. doi: 10.1038/s41598-020-75069-7
Yamada, Y., Nishida, S., Shitan, N., Sato, F. (2021b). Genome-wide profiling of WRKY genes involved in benzylisoquinoline alkaloid biosynthesis in California poppy (Eschscholzia californica). Front. Plant Sci. 12. doi: 10.3389/fpls.2021.699326
Yamada, Y., Sato, F. (2021). Transcription factors in alkaloid engineering. Biomolecules 11, 1719. doi: 10.3390/biom11111719
Yamada, Y., Shimada, T., Motomura, Y., Sato, F. (2017). Modulation of benzylisoquinoline alkaloid biosynthesis by heterologous expression of CjWRKY1 in Eschscholzia californica cells. PloS One 12, e0186953. doi: 10.1371/journal.pone.0186953
Yang, X., Gao, S., Guo, L., Wang, B., Jia, Y., Zhou, J., et al. (2021). Three chromosome-scale Papaver genomes reveal punctuated patchwork evolution of the morphinan and noscapine biosynthesis pathway. Nat. Commun. 12, 6030. doi: 10.1038/s41467-021-26330-8
Yellina, A. L., Orashakova, S., Lange, S., Erdmann, R., Leebens-Mack, J., Becker, A. (2010). Floral homeotic c function genes repress specific b function genes in the carpel whorl of the basal eudicot California poppy (Eschscholzia californica). Evodevo 1, 13. doi: 10.1186/2041-9139-1-13
Zahn, L. M., Ma, X., Altman, N. S., Zhang, Q., Wall, P. K., Tian, D., et al. (2010). Comparative transcriptomics among floral organs of the basal eudicot Eschscholzia californica as reference for floral evolutionary developmental studies. Genome Biol. 11, R101. doi: 10.1186/gb-2010-11-10-r101
Zhang, R., Fu, X., Zhao, C., Cheng, J., Liao, H., Wang, P., et al. (2020). Identification of the key regulatory genes involved in elaborate petal development and specialized character formation in Nigella damascena (Ranunculaceae). Plant Cell 32, 3095–3112. doi: 10.1105/tpc.20.00330
Zhao, Y., Pfannebecker, K., Dommes, A. B., Hidalgo, O., Becker, A., Elomaa, P. (2018). Evolutionary diversification of CYC/TB1-like TCP homologs and their recruitment for the control of branching and floral morphology in Papaveraceae (basal eudicots). New Phytol. 220, 317–331. doi: 10.1111/nph.15289
Zhao, Z. M., Shang, X. F., Lawoe, R. K., Liu, Y. Q., Zhou, R., Sun, Y. F., et al. (2019). Anti-phytopathogenic activity and the possible mechanisms of action of isoquinoline alkaloid sanguinarine. Pest Biochem. Physiol. 159, 51–58. doi: 10.1016/j.pestbp.2019.05.015
Keywords: flower development, benzylisoquinoline alkaloid, Ranunculales, evo devo, VIGS (virus-induced gene silencing)
Citation: Becker A, Yamada Y and Sato F (2023) California poppy (Eschscholzia californica), the Papaveraceae golden girl model organism for evodevo and specialized metabolism. Front. Plant Sci. 14:1084358. doi: 10.3389/fpls.2023.1084358
Received: 30 October 2022; Accepted: 16 February 2023;
Published: 02 March 2023.
Edited by:
Verónica S. Di Stilio, University of Washington, United StatesReviewed by:
Zhichao Xu, Northeast Forestry University, ChinaRainer Melzer, University College Dublin, Ireland
Copyright © 2023 Becker, Yamada and Sato. This is an open-access article distributed under the terms of the Creative Commons Attribution License (CC BY). The use, distribution or reproduction in other forums is permitted, provided the original author(s) and the copyright owner(s) are credited and that the original publication in this journal is cited, in accordance with accepted academic practice. No use, distribution or reproduction is permitted which does not comply with these terms.
*Correspondence: Annette Becker, annette.becker@bot1.bio.uni-giessen.de; Fumihiko Sato, fsato@lif.kyoto-u.ac.jp