- 1Department of Agronomy, Faculty of Agriculture, Universitas Padjadjaran, Sumedang, Indonesia
- 2Master Program of Agro-Industry Technology, Faculty of Agro-Industrial Technology, Universitas Padjadjaran, Sumedang, Indonesia
- 3Master Program of Agronomy, Faculty of Agriculture, Universitas Padjadjaran, Sumedang, Indonesia
The presence of ethylene during postharvest handling of tomatoes can be the main problem in maintaining fruit shelf-life by accelerating the ripening process and causing several quality changes in fruit. Several researchers have studied the methods for improving the postharvest life of tomato fruit by controlling ethylene response, such as by mutation. New ethylene receptor mutants have been identified, namely Sletr1-1, Sletr1-2, Nr (Never ripe), Sletr4-1, and Sletr5-1. This review identifies the favorable and undesirable effects of several ethylene receptor mutants. Also, the impact of those mutations on the metabolite alteration of tomatoes and the future perspectives of those ethylene receptor mutants. The review data is taken from the primary data of our experiment related to ethylene receptor mutants and the secondary data from numerous publications in Google Scholar and other sources pertaining to ethylene physiology. This review concluded that mutation in the SlETR1 gene was more effective than mutation in NR, SLETR4, and SLETR5 genes in generating a new ethylene mutant. Sletr1-2 mutant is a potential ethylene receptor mutant for developing new tomato cultivars with prolonged fruit-shelf life without any undesirable effect. Therefore, that has many challenges to using the Sletr1-2 mutant for future purposes in breeding programs.
1 Introduction
Tomato (Solanum lycopersicum) is a popular horticulture crop consumed as fresh fruit or raw material for the food industry. Tomato production has increased worldwide every year. Tomato contains high micro and macronutrients such as vitamins, minerals, fiber, and other beneficial compounds for human health. Furthermore, it is a model for studying fruit biology, fruit development, softening, ripening, and fruit metabolism (Brummell and Harpster, 2001; Giovannoni, 2004; Carrari and Fernie, 2006), because it has a small genome size (950 Mb), a relatively short life cycle, and stable genetic transformation (Osorio et al., 2011; Kumar et al., 2012). Tomato belongs to climacteric fruit. Thus postharvest handling is essential during shipment and marketing. In climacteric fruits, ethylene accelerates fruit ripening and softening. Moreover, ethylene affects leaf abscission, stem or root elongation, root hair development, epinasty, and flower fading (Abeles et al., 1992).
In developing countries, the loss of horticultural products during postharvest handling reached 50% due to storage, transportation, and packaging conditions (Kitinoja and Kader, 2015). Moreover, the presence of ethylene directly affects the lost fruit quality. Several methods have been developed to prevent the ethylene effect in reducing postharvest tomato fruit quality, such as inhibiting ethylene biosynthesis and perception by chemical compounds, atmosphere modification, and genetic modification. In climacteric fruits such as tomatoes, the inhibition of ethylene perception is more effective than ethylene biosynthesis due to the limitation in the perception of ethylene to its receptor. 1-Methylcyclopropene (1-MCP) is a non-toxic chemical compound that effectively prevents the binding process of ethylene to the receptor. Therefore, the ethylene effect can be minimized. However, this method can be more laborious and impracticable to apply to the farmers. Recently, the genetic modification approach has been widely used to develop prolonged fruit shelf life by down-regulated the ethylene biosynthesis and perception gene. However, this method needs to be supported and acceptable in some countries. The mutation method would be a practical approach for generating new ethylene-insensitive cultivars. Mutation in the ethylene receptor gene has successfully generated several insensitive tomato mutants, such as Sletr1-1, Sletr1-2, and Sletr4-1 (Okabe et al., 2011; Mubarok et al., 2015; Mubarok et al., 2019). This review discusses the commercial use of the ethylene-insensitive mutants, Nr, Sletr1-1, Sletr1-2, and Sletr4-1, as potential breeding material to generate new prolonged shelf life for cultivated tomatoes. It also highlights the prospect and problems associated with using the mutants.
2 Ethylene biosynthesis and signaling
Fruit ripening is regulated by ethylene. Ethylene biosynthesis and signaling are modulated during the development of plant tissue and are responsible for inducing many biochemical processes (Abeles et al., 1992). Ethylene biosynthesis is subject to both positive and negative feedback regulation (Kende, 1993). Ethylene biosynthesis in higher plants has been well-characterized. 1-aminocyclopropane-1-carboxylic acid (ACC) synthase (ACS) and ACC oxidase (ACO) are enzymes of ethylene biosynthesis that have been recognized as the rate-limiting step (Yang and Hoffman, 1984; Kende, 1993). ACS activity is the critical step in controlling ethylene production, whereas ACO activity is constitutive (Yang and Hoffman, 1984; Theologis et al., 1993). The genes encoding ACS and ACO have been studied in more detail than other enzymes in the ethylene pathway. In higher plants, ACS and ACO are encoded by multigene families. Eight ACS genes (LeACS1A, LeACS1B, and LeACS2-7) (Zarembinski and Theologis, 1994; Oetiker et al., 1997; Shiu et al., 1998) and five ACO genes have been identified in tomatoes (Van-der-Hoeven et al., 2002).
The receptor is the crucial factor for ethylene action. A copper cofactor mediates the binding process of ethylene to the receptor (Rodríguez et al., 2010). The absence of copper cofactor caused less capability to bind ethylene. The binding site for copper could be replaced by any other metal, such as silver, due to a strong affinity issue. Silver is commonly used to inhibit ethylene perception by replacing the site of copper. This situation impedes conformational change that is typically found in the presence of copper cofactor in the receptor site. There were three domains classification of ethylene receptor protein based on its structure, i.e., sensor domain, kinase domain, and response regulator domain (Ciardi and Klee, 2001). Both amino-terminal ethylene-binding and the most highly conserved GAF are reported subdomains of the sensor domain (Aravind and Ponting, 1997).
In tomatoes, at least six ethylene receptor genes (LeETR1–6) were identified, and LeETR3 is denoted as NR (Payton et al., 1996). The expression of each tomato receptor is different in temporal and spatial patterns depending on the development stage and external stimuli (Alexander and Grierson, 2002). LeETR1 and LeETR2 are expressed constitutively in all tissues throughout development, NR is up-regulated at anthesis, and both NR and LeETR4 are up-regulated during ripening, senescence, abscission (Payton et al., 1996; Tieman et al., 2000), and pathogen infection (Ciardi et al., 2000). LeETR5 is expressed in fruit, flowers, and during pathogen infection (Tieman and Klee, 1999).
The binding of ethylene to receptors causes conformational changes in a receptor or inactivates a receptor, resulting in the inactivation of a negative regulator of downstream ethylene signaling such as CTR1 (Kieber et al., 1993). Suppression of CTR1 activates ETHYLENE INSENSITIVE (EIN2) to act as an essential positive regulator of the ethylene signaling pathway (Wang et al., 2002). Genetic epinasty analysis of ethylene response mutants has shown that EIN2 acts downstream of CTR1 and positively signals upstream of EIN3 (Alonso et al., 1999; Wang et al., 2002). EIN3 is both necessary and sufficient for the activation of ethylene-responsive target genes and, in particular, for ERF1 (Solano et al., 1998). ERF1 belongs to a large family of plant-specific transcription factors referred to as ethylene response element-binding proteins (EREBPs) (Carrari and Fernie, 2006). Transcription factor ERF1 and other EREBPs can interact with the GCC box, which causes ethylene responses in plants (Yamamoto et al., 1999).
3 Strategy to minimize ethylene effect at receptor level
Ethylene has become a central problem in postharvest horticultural products. Several strategies are needed to manipulate the adverse ethylene effects leading to the maintenance of the postharvest quality of the horticultural product, including tomatoes. Developing new cultivars by mutation is one strategy for obtaining tomato mutants with long fruit shelf-life, such as ripening-inhibitor (rin), colorless non-ripening (Cnr), non-ripening (nor), green-ripe (Gr) and Nr.
Targeting induced local lesions in genomes (TILLING) is a general method to identify induced point mutations in the genomes of any organism. This method accelerates identifying the modified function of desired genes and selecting mutants rather than conventional mutation breeding. TILLING method has identified some mutants, for instance, SleIF4E1 of tomato mutant, which showed potyvirus resistance (Piron et al., 2010), CmACO1 of melon mutant, which produced long shelf-life fruit (Dahmani-Mardas et al., 2010), Sletr1-1, Sletr1-2, and Sletr4-1, which show in the reduction of ethylene sensitivity (Okabe et al., 2011; Mubarok et al., 2019).
The expression analysis of related genes of ethylene biosynthesis and perception has been widely investigated in tomato mutants. This analysis showed that each mutant has a different location where the mutation occurred. In the Nr mutant, a mutation occurred in the ethylene-binding domain of the NR ethylene receptor; therefore, ethylene cannot be perceived, and its response cannot be expressed (Lanahan et al., 1994; Wilkinson et al., 1995). In the ripening inhibitor (rin) mutant, the mutation occurred in the RIN transcription factor; therefore, autocatalytic ethylene production does not show, and the ethylene signal downstream cannot be transmitted (Vrebalov et al., 2002).
In the novel ethylene receptor mutants, in the Sletr1-1 and Sletr1-2 tomato mutant of ‘Micro-Tom’, the mutations occurred in the first and second transmembrane domain in the ethylene receptor, respectively. The location of mutation of Sletr1-1 (P51) and Nr (P36) are similar in the first transmembrane domain; however, they have different ethylene sensitivity (Wilkinson et al., 1995; Okabe et al., 2011). In the Sletr4-1, there has an amino acid substitution, G154S, that occurs between the transmembrane and GAF domains, whereas the Sletr5-1 tomato mutant has the amino acid substitution, R278Q, within the GAF domain (Mubarok et al., 2019) (Figure 1).
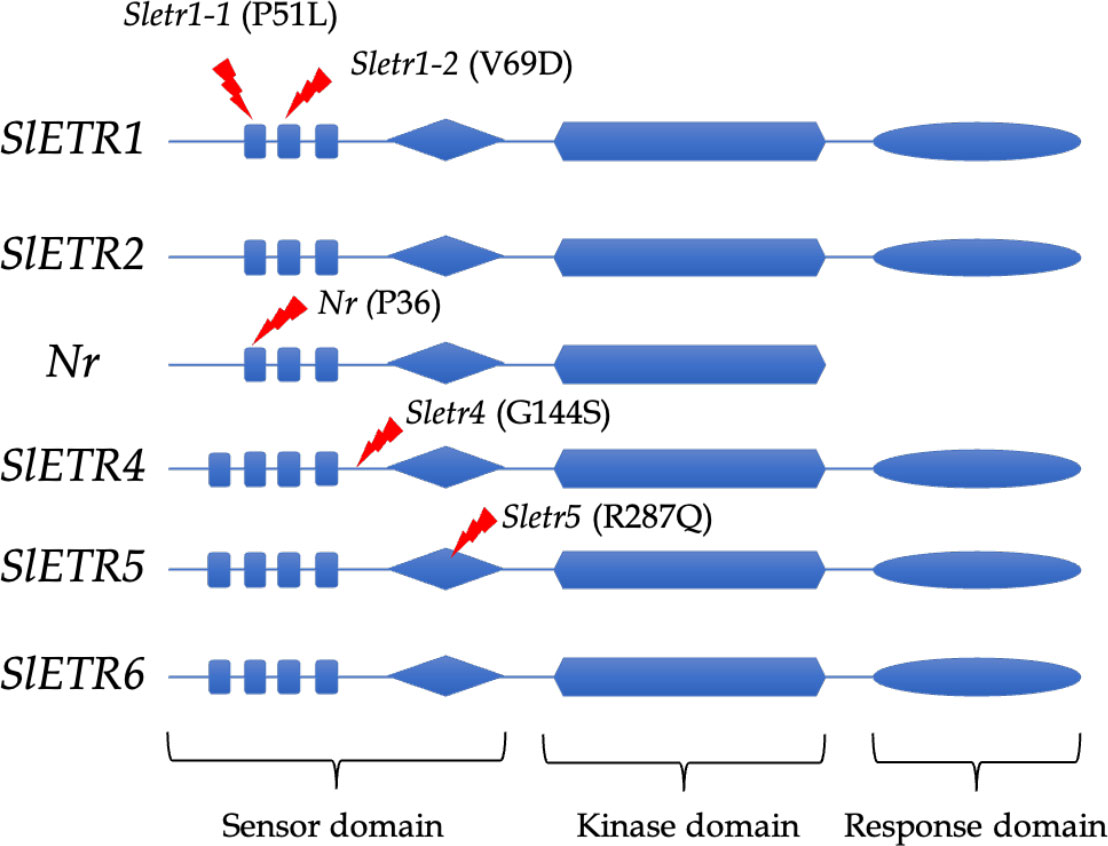
Figure 1 Mutation location of ethylene receptor mutants; Sletr1-1, Sletr1-2, Nr, Sletr4-1 and Sletr5-1.
The ethylene receptor gene plays an important role in ethylene action. Mutation in SlETR1, SlETR4, and SlETR5 results in altered ethylene sensitivity, showing the different changes in ethylene triple response and fruit shelf life. From the seedlings’ ethylene triple response assay, the four new ethylene receptor mutants, Sletr1-1, Sletr1-2, Sletr4-1, and Sletr5-1, exhibited a different ethylene sensitivity. Sletr1-1, Sletr1-2, Sletr4-1, and Sletr5-1 display completely ethylene insensitive, moderate ethylene insensitive, low ethylene sensitivity, and high ethylene sensitivity, respectively (Okabe et al., 2011; Mubarok et al., 2015; Mubarok et al., 2019).
4 Favorable effects of ethylene receptor mutant on fruit ripening
Tomato fruit development could be divided into three phases, namely (i) the main phase with rapid and active cell division; (ii) the phase with a stable increase in size due to cell expansion, and the fruit ripening phase (Pirrello et al., 2012). During the ripening process, the tomato fruit experienced specific changes in appearance, color, texture, taste, and aroma (Giovannoni, 2004). The ripening process in tomato fruits was further divided into three phases, i.e., mature green, breaker, and red. Tomato fruit discoloration during the ripening process occurred due to the increased lycopene and beta carotene content; and chlorophyll degradation during the transition from chloroplasts to chromoplasts. The mature green stage is the final fruit formation stage, as indicated by fully expanded fruit size. In this stage, seed formation began. In a later phase, the breaker, the fruit starts to rip as characterized by specific metabolites degradation and the initiation of ethylene production spike and respiration as a sign of climacteric characteristics. While in the red phase, the fruit is considered ripe, with optimum metabolite content, and also experiences the beginning of the senescence phase (Fraser et al., 1994; Osei et al., 2017).
The fruit shelf life is one of the essential characteristics of the postharvest quality of horticulture crops. In climacteric fruit such as tomatoes, fruit shelf life is commonly affected by ethylene, which accelerates fruit ripening. Therefore, to improve fruit shelf life, the response of ethylene must be minimized. Mutation in ethylene receptor genes significantly underestimated the ethylene response by extending fruit shelf life. Improving the fruit shelf life of tomatoes by developing a new cultivar is an excellent way to get a significant aim in tomato breeding because it can provide various benefits for both tomato producers and consumers. Besides long fruit shelf life, other traits must be improved in tomatoes, such as fruit performance and fruit nutrient, because it is crucial factors for fruit quality and the human diet.
Several mutant alleles, such as Sletr1-1, Sletr1-2, and Nr, reduced ethylene sensitivity, impacting fruit development and ripening. In both homozygous and heterozygous Sletr1-1 mutants, there is a disturbance in the process of petals withering, while the effect is weaker for Nr in both homozygous and heterozygous conditions. However, this condition is influenced by parental background (Okabe et al., 2011). An earlier study by Okabe et al. (2011) reported that petal flowers of Sletr1-1 mutants still stick to the fruit even up to 60 days after pollination (DAP), while in WT-MT and Sletr1-2, the petal withered at 3 and 5 DAP, respectively.
The delay in petal abortion can be used as one of the indicators related to ethylene sensitivity that further affects fruit development, ripening, and postharvest fruit shelf life. The ripening phenotypes in Sletr1-1 were different between homozygous and heterozygous plants. The homozygous Sletr1-1 displayed yellow and orange color (Okabe et al., 2011) whereas heterozygous Sletr1-1 fruits showed reddish-orange color. Homozygous Sletr1-1 and Nr showed similar fruit ripening phenotypes due to imperfect ripening processes (Lanahan et al., 1994; Okabe et al., 2011). Phenotypic differences in fruit ripening were not detected between homozygous and heterozygous Sletr1-2, where the fruit showed perfect ripening. Crossing commercial tomato cultivars with several mutants, such as Nr, Sletr1-1, and Sletr1-2, might facilitate the development of a commercial F1 hybrid line. However, not all ethylene-insensitive mutants can be used as genetic material in the breeding program of long fruit shelf-life tomatoes, for example, Nr and Sletr1-1. Although both Nr and Sletr1-1 had low sensitivity to ethylene, these two mutants displayed an incomplete maturation phenotype, even though they had an insufficient red color in heterozygous form.
The fruit shelf life of the mutant differs from one another either in homozygote or heterozygous form. In homozygous conditions, the fruits of Sletr1-1 and Sletr1-2 are still intact. They do not show any damage characterized by the absence of a black spot on the fruit surface during the 60 days of storage in a sealed chamber at 25°C, while the WT-MT fruit shows some damage at the age of 20-25 days after harvesting (Okabe et al., 2011). However, the increase in fruit storage resistance is not very strongly shown in the homozygous Sletr4-1 (Mubarok et al., 2019). It was likely that the fruit shelf life could be dramatically extended, and post-harvest fruit damage could be inhibited. The use of Sletr1-2 as breeding material to form a hybrid generation has been carried out. The Sletr1-2 has a strong inheritance pattern in increasing the shelf life of fruits in all commercial parental backgrounds. However, the length of fruit shelf-life resistance is different in each parental background of ‘Aichi First’, ‘Ailsa Craig’, ‘Moneymaker’, and ‘M82’, with an average increase in shelf-life resistance ranging from 4−5 days longer in open room conditions at a storage temperature of 20 ± 2°C and relative humidity of 80% (Mubarok et al., 2015).
5 Undesirable effects of ethylene receptor mutant
Mutations in the SlETR1, SLETR4, and SlETR5 genes do not affect plant external appearance, especially in vegetative organs. However, there is an alteration in plant sensitivity to abiotic stress. The Sletr1-1 is a promising genotype since it has low sensitivity to ethylene and shows dominant inheritance during a breeding program in increasing the shelf life of tomato fruits (Okabe et al., 2011). However, some undesirable characteristics are found in the mutant Sletr1-1, i.e., stress sensitivity response. The F1 generations of Sletr1-1 experience withering and disease attacks during the transplanting process to the NFT system. The wilting plant is caused by root damage when transplanted from the nursery (Mubarok et al., 2015). The inability of the F1 Sletr1-1 to recover the damaged root system and the inhibition of new root formation cause secondary threats, such as the pathogen attack to the root and stem base. In contrast, the F1 Sletr1-2 are not susceptible to biotic and abiotic stress, whereas all hybrid of F1 Sletr1-2 shows similar characteristics to F1 WT-MT, i.e., healthy and white roots without roots rot after transplanting (Mubarok et al., 2015).
Heterozygous Sletr1-1 shows increased susceptibility to infections of diseases caused by Fusarium oxysporum. A similar finding was reported in the Atetr1-1 mutant of Arabidopsis thaliana that showed an increase in disease infection by certain pathogens such as Botrytis cinerea, Fusarium solani, Fusarium oxysporum f. sp. matthiolae, Xanthomonas campestris pv. Campestris, and Pythium spp (O'Donnell et al., 2003; Agarwal et al., 2012). In addition, Nr mutants cannot produce adventitious roots in waterlogging conditions (Visser and Voesenek, 2004; Vidoz et al., 2010) and are susceptible to some pathogens (Francia et al., 2007; Kavroulakis et al., 2007; Cantu et al., 2009).
Aside from the increased susceptibility to biotic and abiotic stress, another undesirable characteristic in the ethylene mutant is a change in fruit color as the implication of the pigment reduction, especially lycopene and beta carotene. In tomatoes, the fruit color can be used to estimate maturity level (Table 1). The delay in fruit ripening occurs in the mutant Sletr1-1. This mutant Sletr1-1 undergoes yellow or orange discoloration 7 to 10 days later than WT-MT. Moreover, this mutant mostly does not produce full red fruit color nor does the F1 generation (Okabe et al., 2011; Mubarok et al., 2015). The inability to produce full red color is also observed in the mutant Nr (Lanahan et al., 1994). On the opposite, this phenomenon is not found in the mutant Sletr1-2 or its F1 generation. Sletr1-2 fruits can produce the normal red fruit color as its wild type even in their F1 generation (Okabe et al., 2011; Mubarok et al., 2015). Although the mutants Sletr1-1 and Nr produce fruits with a long shelf life, they have yet to be widely used in breeding programs due to their susceptibility to biotic and abiotic stress, and the imperfection of the fruit ripening process leads to less red color (Francia et al., 2007; Kavroulakis et al., 2007; Cantu et al., 2009)
6 Metabolite alteration of ethylene-insensitive mutants
The change or mutation in related genes in ethylene biosynthesis and action may regulate the gene transcription and ultimately affect the metabolite contents of the tomato fruit. In the rin mutant, the mutation in the RIN gene inhibits carotenoid biosynthesis, aroma, production of flavor compounds, and softening (Herner and Sink, 1973; Tigchelaar et al., 1978; Knapp et al., 1989; Vrebalov et al., 2002; Kumar et al., 2012). The novel insights into the molecular biology of ethylene-mediated ripening regulatory networks in tomato during fruit development has been revealed by analyzing nor, rin, and Nr mutant at transcriptomic, proteomic, and metabolomic levels (Osorio et al., 2011). Recently, a new investigation on the effect of the mutation in rin mutant showed that the RIN mutation results in a profound change in fruit transcriptome during ripening that is similar to other spontaneous mutations, such as Nr, hp-2dg, and cnr (Eriksson et al., 2004; Alba et al., 2005; Osorio et al., 2011; Rohrmann et al., 2011; Kumar et al., 2012; Mubarok et al., 2021).
6.1 Sugar
Ethylene accelerates fruit ripening, which contributes to changes in the nutrient content; however, it also accelerates quality deterioration by shortening the shelf life of the fruit. There is a change in the total sugar content during fruit maturation that can be used to determine the sweetness of tomato fruits (Mubarok et al., 2019). The entire sugar content of the mutant Sletr1-1 and Sletr1-2, both in homozygous and heterozygous form, is lower than WT-MT, except for the F1 Sletr1-2 heterozygous (Mubarok et al., 2016). The fruit of nor mutant has the lowest total sugar content, followed by the fruit of Nr, rin, Sletr1-1, and Sletr1-2 (Osorio et al., 2011; Mubarok et al., 2016; Mubarok et al., 2019; Osorio et al., 2020). The difference in the sensitivity to ethylene can cause the variation in sugar content in these mutants. Mutant tomato plants, namely nor, Nr, and rin, have complete ethylene insensitivity character (Osorio et al., 2011), while the mutant Sletr1-1 and Sletr1-2 have partial ethylene insensitivity character. The variation of ethylene sensitivity may affect the expression of genes that regulate the conversion of starch into sugars (Osorio et al., 2020), thus leading to the difference in sugar content. Baldwin et al. (1998) stated that glucose and fructose are the tomato’s main sugar components contributing to the sweetness level. Under the heterozygous line of the F1 Sletr1-2, the Sletr1-2 mutation did not significantly affect the changes of sucrose, fructose, and glucose under different pure-line cultivar parents. These results contrast with previous studies in the homozygous line of Nr, nor, and rin, demonstrating the reduction in sucrose, glucose, and fructose levels (Hobson, 1980; Osorio et al., 2011).
6.2 Total soluble solid
Sugar content is preliminarily studied as the total soluble solids (TSS) variable, including in the tomato study (Mubarok et al., 2019; Mubarok et al., 2021). The TSS in tomato fruits increased in line with the ripening process (Mubarok et al., 2019), as the impact of the conversion of starch into sugar and the hydrolysis of polysaccharide cell walls to hemicellulose and pectin during the maturation process (Mubarok et al., 2022). In general, the stronger the red color observes, the higher the ripening level of the tomato fruit and the higher the TSS content. Before fully ripe, the starch content in the fruit can reach 20% of the dry weight; then it is degraded into other compounds, such as sugar (Ho, 1996).
Mutant tomatoes, namely Sletr1-1, Sletr1-2, Sletr4-1, Sletr5-1, rin, and nor, have lower TSS content than WT-MT tomatoes on all maturity stadia (Mubarok et al., 2015; Mubarok et al., 2019; Mubarok et al., 2019). The rin and nor mutant have similar TSS, i.e., 4.6°Brix, and this result is still lower than the TSS value of Sletr1-1, Sletr1-2, Sletr4-1, and Sletr5-1 (Mubarok et al., 2015; Mubarok et al., 2019; Mubarok et al., 2019). This phenomenon is associated with the lower expression of genes that regulate the activity of pectinase in rin and nor tomatoes (Osorio et al., 2020), compared to Sletr1-1, Sletr1-2, Sletr4-1, and Sletr5-1. So, the amount of pectin converted by pectinase during storage is also lower (Osorio et al., 2011).
6.3 pH and titratable acidity
Aside from the sugar content indicated by the TSS variable, the alteration in the mutant is also found in terms of acidity level. Fruit pH and titratable acidity (TA) are two common variables used to determine the acidity level of tomato fruit (Mubarok et al., 2019). Along with TSS, acidity variables form the balance of sour and sweet in the fruit taste profile and post-harvest quality in tomato fruits (Grierson and Fray, 1994). An earlier study by Tran et al. (2017) showed that the total acid content increase during fruit formation and enlargement. However, it declines in line with the ripening process due to the degradation of organic acids during ethylene biosynthesis in the respiration stage.
The fruit TA value of insensitive ethylene mutants, namely rin, nor, Sletr1-1, Sletr1-2, Sletr4-1, and Sletr5-1, are higher than WT-MT. Lobit et al. reported that the TA and fruit pH are closely related (Lobit et al., 2002). The increase in TA is accompanied by a decrease in the pH. Therefore, the pH of the insensitive ethylene mutant of rin, nor, Sletr1-1, Sletr1-2, Sletr4-1, and Sletr5-1 is lower than WT-MT (Mubarok et al., 2015; Mubarok et al., 2019; Mubarok et al., 2019).
6.4 Lycopene, beta carotene
In addition to TSS and TA, some phytochemicals, such as lycopene, beta carotene, flavonoid, and polyphenols, are reported to differ in ethylene insensitive mutant compared to its wild type, leading to the variation of antioxidant activity. Lycopene is a carotenoid responsible for reddish color formation on tomato fruits (Rai et al., 2013). The rin and nor have the lowest lycopene content. This finding can be caused by the low expression of genes that play a role in the process of lycopene formation, namely PSY1, PSY2, PDS, ZDS, and CRTISO genes (Kitagawa et al., 2005; Osorio et al., 2020). The Sletr1-1 and Sletr1-2 have a higher lycopene content than rin and nor, but they are still lower than the WT-MT (Mubarok et al., 2015; Mubarok et al., 2019; Mubarok et al., 2019). The findings show that ethylene may associate with the formation of lycopene.
Beta carotene in ethylene insensitive mutant is lower than that in WT-MT. The rin and nor have the lowest beta carotene content. It may be caused by the low activity of the CRTR-b1 gene, which converts γ-carotene into beta-carotene (Osorio et al., 2020). The mutant Sletr1-1 and Sletr1-2 have higher beta carotene than the rin and nor mutant (Mubarok et al., 2015; Mubarok et al., 2019). This phenomenon can be associated with the difference in ethylene insensitivity levels between mutant genotypes.
6.5 Polyphenols and flavonoids
The content of polyphenols and flavonoids in the fruit of ethylene-insensitive mutant tomatoes varies in response to genotypic factors. The content of polyphenols and flavonoids in rin and nor mutant is very low (Minoggio et al., 2003). Meanwhile, the polyphenol content in fruits of the Sletr1-1 and Sletr1-2 is not significantly different from WT-MT, whereas the flavonoid content in these mutants is lower than in WT-MT (Mubarok et al., 2019). The rate of polyphenol and flavonoid content, from low to high, can be sorted as follows; rin, nor, Sletr1-1, Sletr1-2, and WT-MT. The lower polyphenols and flavonoids in ethylene-insensitive mutants have ascertained ethylene’s involvement in the biosynthesis of polyphenols and flavonoids (Chaudhary et al., 2018). Meanwhile, the difference in flavonoid and polyphenol content among mutants can be caused by differences in the degree of insensitivity level to ethylene (Osorio et al., 2020).
6.6 Antioxidant activity
The antioxidant activity of ethylene insensitive mutant, namely rin, is the lowest, followed by nor (Minoggio et al., 2003), Sletr1-1, and Sletr1-2. The antioxidant activity of Sletr1-2 is not significantly different from WT-MT (Mubarok et al., 2019), while the antioxidant activity of rin, nor, and Sletr1-1 is lower than WT-MT. This situation is associated with the lower content of lycopene, beta carotene, flavonoids, and polyphenols on rin, nor, and Sletr1-1 rather than Sletr1-2 and the WT-MT (Minoggio et al., 2003; Kitagawa et al., 2005; Mubarok et al., 2019; Osorio et al., 2020). Earlier study reported that both lycopene and beta carotene are potent antioxidant compounds whose content dramatically affects the rate of antioxidant activity of tomato fruit (Mubarok et al., 2019).
6.7 Organic acids
The presence of organic acids correlates with fruit quality that directly affects fruit sourness, such as tomato. Tang et al. (2010) stated that the organic acid content is essential in food nutrition. The primary organic acids in tomato fruit, namely citrate, and malate (Baldwin et al., 1998). Oms-Oliu et al. (2011) stated that the metabolisms of citrate and malate are subjected to ethylene regulation. Several factors affect the levels of organic acids in tomato fruit, and ethylene is one of the influencing factors (Mubarok et al., 2016). The change of organic acid content in fruit is directly affected by the function of ethylene response. Inhibition of the ethylene perception due to a mutation in the ethylene receptor gene significantly increased the total organic acid content (Osorio et al., 2011; Mubarok et al., 2016). Mubarok et al. (2016) stated that the F1 generation of Sletr1-1 and Sletr1-2 mutants have a higher total organic acid, malate, and citrate content than the control. High organic acid content was also detected in the Nr mutant due to a mutation in the ethylene receptor gene (Osorio et al., 2011).
6.8 Amino acids
The ethylene was not directly affecting the change in fruit amino acids. Mubarok et al. (2016) reported that the variation of the amino acids in four F1 generations of Sletr1-2 was dependent on the genetic background. Although the Sletr1-2 mutation did not directly affect the total amino acids, it significantly induced changes in the individual amino acid levels, such as glutamic acid, glutamine, aspartic acid, and GABA (Mubarok et al., 2016). Oms-Oliu et al., 2011 stated that those four amino acids are the primary amino acids in the tomato fruit (Oms-Oliu et al., 2011). Different behaviors in accumulating individual amino acids were also observed in the Nr mutant.56 Regarding fruit taste quality, glutamic acid substantially enhances taste perception or fruitiness intensity that correlates with fruit shelf life (Yilmaz, 2001; Oms-Oliu et al., 2011). Associations between long fruit shelf life and lower levels of glutamic acid have been demonstrated in the Nr mutants. Still, it was not shown in the Sletr1-2 F1 (Pratta et al., 2004; Osorio et al., 2011). The Sletr1-2 F1 hybrid showed no change in the level of glutamic acid compared with the WT-MT F1 hybrid line fruit. Based on this study, we conclude that Sletr1-2 F1 can produce red fruit and glutamic acid that did not influence the postharvest fruit quality (Oms-Oliu et al., 2011).
7 Future perspective
The presence of ethylene hormone can affect the growth, yield, and quality of horticultural commodity yields. In post-harvest handling, the presence of ethylene can have both positive and negative effects depending upon the purpose of its use. For storage and transportation purposes, especially in climacteric fruits such as tomatoes, ethylene accelerates the fruit ripening, leading to shorter fruit shelf life. Mutant with ethylene gene receptor modification can be used as an alternative solution because the negative influence of the ethylene hormone in this genotype can be minimized.
These mutants can be used as elders in plant breeding programs to produce new superior tomato cultivars with longer fruit shelf life (Mubarok et al., 2015; Wiguna et al., 2021). With the production of this shelf-resistant commercial tomato cultivar, the post-harvest problem in tomato fruits can be solved. The use of these mutant tomatoes for breeding programs will be more effective when compared to other ethylene-inhibition methods, such as controlled atmospheric storage with high-cost disadvantages. In the future, these mutants will have a considerable function, especially in plant breeding programs to assemble tomatoes for fresh consumption. With the knowledge of these mutants, it is hoped that the breeding program can run well and that new superior tomato cultivars can be produced, especially for the raw consumed tomatoes such as beef and cherry tomatoes.
8 Conclusions
Ethylene is one of the critical problems in the post-harvest handling of climacteric fruits such as tomatoes. Developing tomato cultivars that are insensitive to ethylene is one of the effective ways to control the negative influence of ethylene in accelerating fruit damage. The TILLING method has successfully obtained mutant tomatoes less sensitive to ethylene, including Nr, Sletr1-1, Sletr1-2, and Sletr4-1. The Sletr1-2 mutant is the most promising genotype for further development among the four mentioned mutant genotypes. The Sletr1-2 mutant is less sensitive to environmental stress and can produce red fruits, unlike the Sletr1-1 mutant, which only has yellow fruits. In its F1 generation, the mutation in the Sletr1-2 allele shows a less significant effect on the nutritional content of the fruit, which is very important for human health. Therefore, the Sletr1-2 mutant is a potential mutant used in breeding programs for assembling new superior tomato cultivars with long fruit shelf life.
Author contributions
All authors contributed to the article and approved the submitted version.
Acknowledgments
We thank all members of our laboratory for helpful discussions throughout the work and to Universitas Padjadjaran for supporting this work through a grant on the scheme of Article Review Writing UNPAD.
Conflict of interest
The authors declare that the research was conducted in the absence of any commercial or financial relationships that could be construed as a potential conflict of interest.
Publisher’s note
All claims expressed in this article are solely those of the authors and do not necessarily represent those of their affiliated organizations, or those of the publisher, the editors and the reviewers. Any product that may be evaluated in this article, or claim that may be made by its manufacturer, is not guaranteed or endorsed by the publisher.
References
Abeles, F. B., Morgan, P. W., Saltveit, M. E. (1992). Ethylene in plant biology. 2nd ed (Sandiego: Academic Press), 83–103.
Agarwal, G., Choudhary, D., Singh, V. P., Arora, A. (2012). Role of ethylene receptors during senescence and ripening in horticultural crops. Plant Signaling Behav. 7 (7), 827–846. doi: 10.4161/psb.20321
Alba, R., Payton, P., Fei, Z., McQuinn, R., Debbie, P., Martin, G. B., et al. (2005). Transcriptome and selected metabolite analyses reveal multiple points of ethylene control during tomato fruit development. Plant Cell 17, 2954–2965. doi: 10.1105/tpc.105.036053
Alexander, L., Grierson, D. (2002). Ethylene biosynthesis and action in tomato: a model for climacteric fruit ripening. J. Exp. Bot. 53, 2039–2055. doi: 10.1093/jxb/erf072
Alonso, J. M., Hirayama, T., Roman, G., Nourizadeh, S., Ecker, J. R. (1999). EIN2, a bi-functional transducer of ethylene and stress responses in arabidopsis. Science 284, 2148–2152. doi: 10.1126/science.284.5423.2148
Aravind, L., Ponting, C. P. (1997). The GAF domain: an evolutionary link between diverse phototransducing proteins. Trends Biochem. Sci. 12 (22), 458–459. doi: 10.1016/S0968-0004(97)01148-1
Baldwin, E. A., Scott, J. W., Einstein, M. A., Malundo, T. M. M., Carr, B. T., Shewfelt, R. L., et al. (1998). Relationship between sensory and instrumental analysis for tomato flavor. J. Am. Soc Hortic. Sci. 123, 906–915. doi: 10.21273/JASHS.123.5.906
Brummell, D. A., Harpster, M. H. (2001). Cell wall metabolism in fruit softening and quality and its manipulation in transgenic plants. Plant Mol. Biol. 47, 311–340. doi: 10.1023/A:1010656104304
Cantu, D., Blanco-Ulate, B., Yang, L., Labavitch, J. M. (2009). Ripening- regulated susceptibility of tomato fruit to botrytis cinerea requires NOR but not RIN or ethylene. Plant Physiol. 150, 1434–1449. doi: 10.1104/pp.109.138701
Carrari, F., Fernie, A. R. (2006). Metabolic regulation underlying tomato fruit development. J. Exp. Bot. 57, 1883–1897. doi: 10.1093/jxb/erj020
Chaudhary, P., Sharma, A., Singh, B., Nagpal, A. K. (2018). Bioactivities of phytochemicals present in tomato. J. Food Sci. Technol. 55 (8), 2833–2849. doi: 10.1007/s13197-018-3221-z
Ciardi, J., Klee, H. (2001). Regulation of ethylene-mediated responses at the level of the receptor. Ann. Bot. 88, 813–822. doi: 10.1006/anbo.2001.1523
Ciardi, J. A., Tieman, D. M., Lund, S. T., Jones, J. B., Stall, R. E., Klee, H. J. (2000). Response to xanthomonas campestris pv. vesicatoria in tomato involves regulation of ethylene receptor gene expression. Plant Physiol. 123 (1), 81–92. doi: https://doi.org/10.1104/pp.123.1.81
Dahmani-Mardas, F. C., Troades, A., Boualam, S., Leveque, A. A., Alsadon, A. A., Aldoss, C., et al. (2010). Engineering melon plants with improved fruit shelf life using the TILLING approach. PloS One 5, e15776. doi: 10.1371/journal.pone.0015776
Eriksson, E. M., Bovy, A., Manning, K., Harrison, L., Andrews, J., De Silva., J., et al. (2004). Effect of the colorless nonripening mutation on cell wall biochemistry and gene expression during tomato fruit development and ripening. Plant Physiol. 136, 4184–4197. doi: 10.1104/pp.104.045765
Francia, D., Demaria, D., Calderini, O., Ferraris, L., Valentino, D., Arcioni, S., et al. (2007). Wounding induces resistance to pathogens with different lifestyles in tomato: role of ethylene in cross-protection. Plant Cell Environ. 30, 1357–1365. doi: 10.1111/j.1365-3040.2007.01709.x
Fraser, P. D., Truesdale, M. R., Bird, C. R., Schuch, W., Bramley, P. M. (1994). Carotenoid biosynthesis during tomato fruit development (evidence for tissue-specific gene expression). Plant Physiol. 105 (1), 405–413. doi: 10.1104/pp.105.1.405
Giovannoni, J. J. (2004). Genetic regulation of fruit development and ripening. Plant Cell (Suppl) 16, S170–S180. doi: 10.1105/tpc.019158
Grierson, D., Fray, R. (1994). Control of ripening in transgenic tomatoes. Euphytica 79, 251–263. doi: 10.1007/BF00022526
Herner, R. C., Sink, K. C. (1973). Ethylene production and respiratory behavior of the rin tomato mutant. Plant Physiol. 52, 38–42. doi: 10.1104/pp.52.1.38
Ho, L. C. (1996). The mechanism of assimilate partitioning and carbohydrate compartmentation in fruit in relation to the quality and yield of tomato. J. Exp. Bot. 47, 1239–1243. doi: 10.1093/jxb/47.Special_Issue.1239
Hobson, G. E. (1980). Effect of the introduction of non-ripening mutant genes on the composition and enzyme content of tomato fruit. J. Sci. Food Agric. 31, 578–584. doi: 10.1002/jsfa.2740310610
Kavroulakis, N., Ntougias, S., Zervakis, G. I., Ehaliotis, C., Haralampidis, K., Papadopoulou, K. K. (2007). Role of ethylene in the protection of tomato plants against soil-borne fungal pathogens conferred by an endophytic fusarium solani strain. J. Exp. Boot 58, 3853–3864. doi: 10.1093/jxb/erm230
Kende, H. (1993). Ethylene biosynthesis. Annu. Rev. Plant Physiol. Plant Mol. Biol. 44, 283–307. doi: 10.1146/annurev.pp.44.060193.001435
Kieber, J. J., Rothenberg, M., Roman, G., Feldmann, K. A., Ecker, J. R. (1993). CTR1, a negative regulator of the ethylene response pathway in arabidopsis, encodes a member of the raf family of protein kinases. Cell 72, 427–441. doi: 10.1016/0092-8674(93)90119-B
Kitagawa, M., Ito, H., Shiina, T., Nakamura, N., Inakuma, T., Kasumi, T., et al. (2005). Characterization of tomato fruit ripening and analysis of gene expression in F1 hybrids of the ripening inhibitor (rin) mutant. Physiol. Plant 123, 331–338. doi: 10.1111/j.1399-3054.2005.00460.x
Kitinoja, L., Kader, A. A. (2015). Measuring postharvest losses of fresh fruits and vegetables in developing countries. PEF white pap. 15, 26.
Knapp, J., Moureau, P., Schuch, W., Grierson, D. (1989). Organisation and expression of polygalacturonase and other ripening genes in ailsa Craig ‘Neverripe’ and ‘ripening inhibitor’ tomato mutants. Plant Mol. Biol. 12, 105–116. doi: 10.1007/BF00017453
Kumar, R., Sharma, M. K., Kapoor, S., Tyagi, A. K., Sharma, A. K. (2012). Transcriptome analysis of rin mutant fruit and in silico analysis of promoters of differentially regulated genes provides insight into LeMADS-RIN-regulated ethylene-dependent as well as ethylene-independent aspects of ripening in tomato. Mol. Genet. Genomics 287, 189–203. doi: 10.1007/s00438-011-0671-7
Lanahan, M. B., Yen, H. C., Giovannoni, J. J., Klee, H. J. (1994). The never ripe mutation blocks ethylene perception in tomato. Plant Cell 6, 521–530. doi: 10.1105/tpc.6.4.521
Lobit, P., Soing, P., Génard, M., Habib, R. (2002). Theoretical analysis of relationships between composition, pH, and titratable acidity of peach fruit. J. Plant Nutr. 25 (12), 2775–2792. doi: 10.1081/PLN-120015538
Minoggio, M., Bramati, L., Simonetti, P., Gardana, C., Iemoli, L., Santangelo, E., et al. (2003). Polyphenol pattern and antioxidant activity of different tomato lines and cultivars. Ann. Nutr. Metab. 47 (2), 64–69. doi: 10.1159/000069277
Mubarok, S., Dahlania, S., Suwali, N. (2019). Dataset on the change of postharvest quality of physalis peruviana l. as an effect of ethylene inhibitor. Data Brief 24, 103849. doi: 10.1104/pp.123.1.81
Mubarok, S., Ezura, H., Qonit, M. A. H., Prayudha, E., Suwali, N., Kurnia, D. (2019). Alteration of nutritional and antioxidant level of ethylene receptor tomato mutants, Sletr1-1 and Sletr1-2. Sci. Hortic. 256, 108546. doi: 10.1016/j.scienta.2019.108546
Mubarok, S., Ezura, H., Rostini, N., Suminar, E., Wiguna, G. (2019). Impacts of Sletr1-1 and Sletr1-2 mutations on the hybrid seed quality of tomatoes. J. Integr. Agric. 18 (5), 1170–1176. doi: https://doi.org/10.1016/j.dib.2019.103849
Mubarok, S., Hoshikawa, K., Okabe, Y., Yano, R., Tri, M. D., Ariizumi, T., et al. (2019). Evidence of the functional role of the ethylene receptor genes SlETR4 and SlETR5 in ethylene signal transduction in tomato. Mol. Genet. Genomics 294 (2), 301–313. doi: 10.1007/s00438-018-1505-7
Mubarok, S., Maulida Rahman, I., Kamaluddin, N. N., Solihin, E. (2022). Impact of 1-methylcyclopropene combined with chitosan on postharvest quality of tropical banana ‘Lady finger’. Int. J. Food Prop. 25 (1), 1171–1185. doi: 10.1080/10942912.2022.2074028
Mubarok, S., Okabe, Y., Fukuda, N., Ariizumi, T., Ezura, H. (2015). Potential use of a weak ethylene receptor mutant, Sletr1-2, as breeding material to extend fruit shelf life of tomato. J. Agric. Food Chem. 63 (36), 7995–8007. doi: 10.1021/acs.jafc.5b02742
Mubarok, S., Okabe, Y., Fukuda, N., Ariizumi, T., Ezura, H. (2016). Favorable effects of the weak ethylene receptor mutation Sletr1-2 on postharvest fruit quality changes in tomatoes. Postharvest Biol. Technol. 120, 1–9. doi: 10.1016/j.postharvbio.2016.04.022
Mubarok, S., Wicaksono, F. Y., Budiarto, R., Rahmat, B. P. N., Khoerunnisa, S. A. (2021). Metabolite correlation with antioxidant activity in different fruit maturation stages of physalis peruviana. Biodiversitas J. Biol. Diversity 22 (5), 2743–2749. doi: 10.13057/biodiv/d220536
O'Donnell, P. J., Schmelz, E. A., Moussatche, P., Lund, S. T., Jones, J. B., Klee, H. J. (2003). Susceptible to intolerance a range of hormonal actions in a susceptible arabidopsis pathogen response. Plant J. 33, 245–257. doi: 10.1046/j.1365-313X.2003.01619.x
Oetiker, J. H., Olson, D. C., Shiu, O. Y., Yang, S. F. (1997). Differential induction of seven 1-aminocyclopropane-1-carboxylate synthase genes by elicitor in suspension cultures of tomato (Lycopersicon esculentum). Plant Mol. Biol. 34, 275–286. doi: 10.1023/A:1005800511372
Okabe, Y., Asamizu, E., Saito, T., Matsukura, C., Ariizumi, T., Bres, C., et al. (2011). Tomato TILLING technology: development of a reverse genetic tool for the efficient isolation of mutants from micro-tom mutant libraries. Plant Cell Physiol. 52, 1994–2005. doi: 10.1093/pcp/pcr134
Oms-Oliu, G., Hertog, M.L.A.T.M., Van de Poel, B., Ampofo-Asiama, J., Geeraerd, A. H., Nicolaiü, B. M. (2011). Metabolic characterization of tomato fruit during preharvest development, ripening, and postharvest shelf-life. Postharvest Biol. Technol. 62, 7–16. doi: 10.1016/j.postharvbio.2011.04.010
Osei, M. K., Danquah, A., Blay, E. T., Danquah, E., Adu-Dapaah, H. (2017). An overview of tomato fruit-ripening mutants and their use in increasing shelf life of tomato fruits. Afr. J. Agric. Res. 12, 3520–3528. doi: 10.5897/AJAR2017.12756
Osorio, S., Alba, R., Damasceno, C. M. B., Lopez-Casado, G., Lohse, M., Zanor, M. I., et al. (2011). Systems biology of tomato fruit development: Combined transcript, protein, and metabolite analysis of tomato transcription factor (nor, rin) and ethylene receptor (Nr) mutants reveals novel regulatory interactions. Plant Physiol. 157, 405–425. doi: 10.1104/pp.111.175463
Osorio, S., Carneiro, R. T., Lytovchenko, A., McQuinn, R., Sørensen, I., Vallarino, J. G., et al. (2020). Genetic and metabolic effects of ripening mutations and vine detachment on tomato fruit quality. Plant Biotechnol. J. 18 (1), 106–118. doi: 10.1111/pbi.13176
Payton, S., Fray, R. G., Brown, S., Grierson, D. (1996). Ethylene receptor expression is regulated during fruit ripening, fower senescence and abscission. Plant Mol. Biol. 31, 1227–1231. doi: 10.1007/BF00040839
Piron, F., Nicolai, M., Minoia, S., Piednoir, E., Moretti, A., Salgues, A., et al. (2010). An induced mutation in tomato eIF4E leads to immunity to two potyviruses. PloS One 5, e11313. doi: 10.1371/journal.pone.0011313
Pirrello, J., Prasad, B. C., Zhang, W., Chen, K., Mila, I., Zouine, M., et al. (2012). Functional analysis and binding affinity of tomato ethylene response factors provide insight on the molecular bases of plant differential responses to ethylene. BMC Plant Biol. 12 (1), 1–15. doi: 10.1186/1471-2229-12-190
Pratta, G., Zorzoli, R., Boggio, S. B., Picardi, L. A., Valle, E. M. (2004). Glutamine and glutamate levels and related metabolizing enzymes in tomato fruit with different shelf-life. Sci. Hortic. 100, 341–347. doi: 10.1016/j.scienta.2003.08.004
Rai, A. C., Singh, M., Shah, K. (2013). Engineering drought tolerant tomato plants over-expressing BcZAT12 gene encoding a C2H2 zinc finger transcription factor. Phytochemistry 85, 44–50. doi: 10.1016/j.phytochem.2012.09.007
Rodríguez, G. R., Pratta, G. R., Liberatti, D. R., Zorzoli, R., Picardi, L. A. (2010). Inheritance of shelf life and other quality traits of tomato fruit estimated from F1’s, F2’s and backcross generatios derived from standard cultivar, nor homozygote and wild cherry tomato. Euphytica 176, 137–147. doi: 10.1007/s10681-010-0241-9
Rohrmann, J., Tohge, T., Alba, R., Osorio, S., Caldana, C., McQuinn, R., et al. (2011). Combined transcription factor profiling, microarray analysis and metabolite profiling reveals the transcriptional control of metabolic shifts occurring during tomato fruit development. Plant J. 68, 999–1013. doi: 10.1111/j.1365-313X.2011.04750.x
Shiu, O. Y., Oetiker, J. H., Yip, W. K., Yang, S. F. (1998). “The promoter of LE-ACS7, an early ¯ooding-induced 1- aminocyclopropane-1- carboxylate synthase gene of the tomato, is tagged by a Sol3transposon,” in Proceedings of the National Academy of Sciences, 95(17), 10334–10339.
Solano, R., Stepanova, A., Chao, Q., Ecker, J. R. (1998). Nuclear events in ethylene signaling: A transcriptional cascade mediated by ETHYLENE-INSENSITIVE3 and ETHYLENE-RESPONSE-FACTOR1. Genes Dev. 12, 3703–3714. doi: 10.1101/gad.12.23.3703
Tang, M., Bie, Z. L., Wu, M. Z., Yi, H. P., Feng, J. X. (2010). Changes in organic acids and acid metabolism enzymes in melon fruit during development. Sci. Hortic. 123, 360–365. doi: 10.1016/j.scienta.2009.11.001
Theologis, A., Oeller, P. W., Wong, L. M., Rottmann, W. H., Gantz, D. M. (1993). Use of a tomato mutant constructed with reverse genetics to study fruit ripening, a complex developmental process. Dev. Genet. 14, 282–295. doi: 10.1002/dvg.1020140406
Tieman, D. M., Klee, H. J. (1999). Differential expression of two novel members of the tomato ethylene-receptor family. Plant Physiol. 120, 165–172. doi: 10.1104/pp.120.1.165
Tieman, D. M., Taylor, M. G., Ciardi, J. A., Klee, H. J. (2000). The tomato ethylene receptors NR and LeETR4 are negative regulators of ethylene response and exhibit functional compensation within a multigene family. Proc. Natl. Acad. Sci. U.S.A. 97, 5663–5668. doi: 10.1073/pnas.090550597
Tigchelaar, E. C., Mcglasson, W. B., Franklin, M. J. (1978). Natural and ethephon-stimulated ripening of F1 hybrids of the ripening inhibitor (rin) and non-ripening (nor) mutants of tomato (Lycopersicon esculentum mill.). Aust. J. Plant Physiol. 5, 449–456. doi: 10.1071/PP9780449
Tran, D. T., Tran, T. L. H., Hertog, M., Picha, D., Nicolaï, B. (2017). Quality changes of tomato during fruit development and climacteric ripening. Eur. J. Hortic. Sci. 82 (3), 319–325. doi: 10.17660/eJHS.2017/82.3.1
Van-der-Hoeven, R., Ronning, C., Giovannoni, J. J., Martin, G., Tanksley, S. (2002). Deductions about the number, organization, and evolution of genes in the tomato genome based on analysis of a large expressed sequence tag collection and selective genomic sequencing. Plant Cell 14, 1441–1456. doi: 10.1105/tpc.010478
Vidoz, M. L., Loreti, E., Mensuali, A., Alpi, A., Perata, P. (2010). Hormonal interplay during adventitious root formation in flooded tomato plants. Plant J. 63, 551–562. doi: 10.1111/j.1365-313X.2010.04262.x
Visser, E. J. W., Voesenek, L. A. C. J. (2004). Acclimation to soil flooding – sensing and signal-transduction. Root Physiol. Gene Funct. 254, 197–214. doi: 10.1007/s11104-004-1650-0
Vrebalov, J., Ruezinsky, D., Padmanabhan, V., White, R., Medrano, D., Drake, R., et al. (2002). MADS-box gene necessary for fruit ripening at tomato ripening-inhibitor (Rin) locus. Science 296, 343–346. doi: 10.1126/science.1068181
Wang, K. L. C., Li, H., Ecker, J. R. (2002). Ethylene biosynthesis and signaling networks. Plant Cell, 14, S131–S151. doi: 10.1105/tpc.001768
Wiguna, G., Damayanti, F., Mubarok, S., Ezura, H., Anas, A. (2021). Genetic control of fruit shelf life and yield in crossbreeding of Sletr1-2 mutant with Indonesian tropical tomatoes: Combining ability of Sletr1-2 mutant. Biodiversitas J. Biol. Diversity 22 (10), 4671–4675. doi: 10.13057/biodiv/d221060
Wilkinson, J., Lanahan, M., Yen, H., Giovannoni, J., Klee, H. (1995). An ethylene-inducible component of signal transduction encoded by never-ripe. Science 270, 1807–1809. doi: 10.1126/science.270.5243.1807
Yamamoto, S., Suzuki, K., Shinshi, H. (1999). Elicitor responsive, ethylene-independent activation of GCC box–mediated transcription that is regulated by both protein phosphorylation and dephosphorylation in cultured tobacco cells. Plant J. 20, 571–579. doi: 10.1046/j.1365-313X.1999.00634.x
Yang, S. F., Hoffman, N. E. (1984). Ethylene biosysnthesis and its regulation in higher plants. Annu. Rev. Plant Physiol. 35, 155–189. doi: 10.1146/annurev.pp.35.060184.001103
Keywords: ethylene, mutant, postharvest, tomato, receptor
Citation: Mubarok S, Qonit MAH, Rahmat BPN, Budiarto R, Suminar E and Nuraini A (2023) An overview of ethylene insensitive tomato mutants: Advantages and disadvantages for postharvest fruit shelf-life and future perspective. Front. Plant Sci. 14:1079052. doi: 10.3389/fpls.2023.1079052
Received: 24 October 2022; Accepted: 13 January 2023;
Published: 27 January 2023.
Edited by:
Sangram K. Lenka, Gujarat Biotechnology University, IndiaReviewed by:
Qinggang Zhu, Northwest A&F University, ChinaIrene Romero, Spanish National Research Council (CSIC), Spain
Yuanyue Shen, Beijing University of Agriculture, China
Copyright © 2023 Mubarok, Qonit, Rahmat, Budiarto, Suminar and Nuraini. This is an open-access article distributed under the terms of the Creative Commons Attribution License (CC BY). The use, distribution or reproduction in other forums is permitted, provided the original author(s) and the copyright owner(s) are credited and that the original publication in this journal is cited, in accordance with accepted academic practice. No use, distribution or reproduction is permitted which does not comply with these terms.
*Correspondence: Syariful Mubarok, c3lhcmlmdWwubXViYXJva0B1bnBhZC5hYy5pZA==