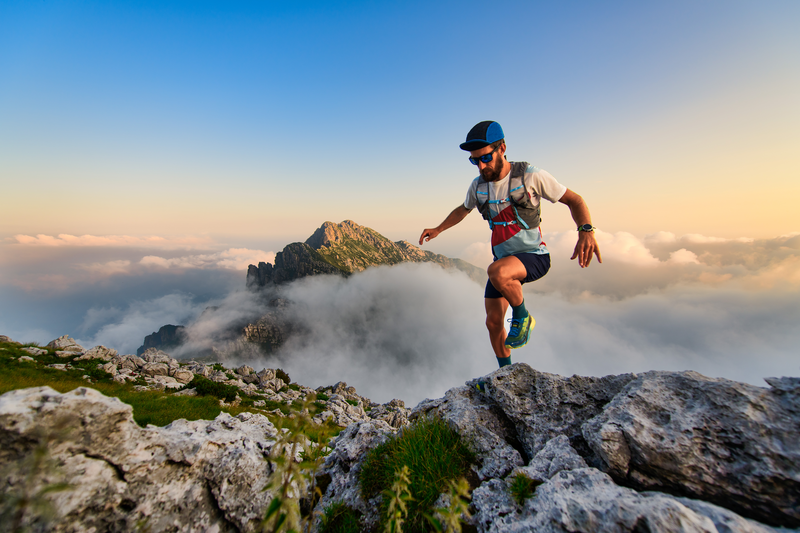
95% of researchers rate our articles as excellent or good
Learn more about the work of our research integrity team to safeguard the quality of each article we publish.
Find out more
ORIGINAL RESEARCH article
Front. Plant Sci. , 10 March 2023
Sec. Plant Abiotic Stress
Volume 14 - 2023 | https://doi.org/10.3389/fpls.2023.1063618
This article is part of the Research Topic Abiotic Stress Alleviation in Plants: Morpho-Physiological and Molecular Aspects View all 31 articles
Using nanofertilizers in certain concentrations can be a novel method to alleviate drought stress effects in plants as a global climate problem. We aimed to determine the impacts of zinc nanoparticles (ZnO-N) and zinc sulfate (ZnSO4) fertilizers on the improvement of drought tolerance in Dracocephalum kotschyi as a medicinal-ornamental plant. Plants were treated with three doses of ZnO-N and ZnSO4 (0, 10, and 20 mg/l) under two levels of drought stress [50% and 100% field capacity (FC)]. Relative water content (RWC), electrolyte conductivity (EC), chlorophyll, sugar, proline, protein, superoxide dismutase (SOD), polyphenol oxidase (PPO) and, guaiacol peroxidase (GPO) were measured. Moreover, the concentration of some elements interacting with Zn was reported using the SEM-EDX method. Results indicated that foliar fertilization of D. kotschyi under drought stress with ZnO-N decreased EC, while ZnSO4 application was less effective. Moreover, sugar and proline content as well as activity of SOD and GPO (and to some extent PPO) in treated plants by 50% FC, increased under the influence of ZnO-N. ZnSO4 application could increase chlorophyll and protein content and PPO activity in this plant under drought stress. Based on the results, ZnO-N and then ZnSO4 improved the drought tolerance of D. kotschyi through their positive effects on physiological and biochemical attributes changing the concentration of Zn, P, Cu, and Fe. Accordingly, due to the increased sugar and proline content and also antioxidant enzyme activity (SOD, GPO, and to some extent PPO) on enhancing drought tolerance in this plant, ZnO-N fertilization is advisable.
According to climate change and changes in global precipitation patterns, predictions and models have shown an increase in the size of deserts and xeric scrublands as the largest terrestrial biome (Guo et al., 2021). Every year, water scarcity and drought cause severe damage to agricultural products, green spaces, forests, and rangelands. In the past few years, these issues have resulted in a global decline in agricultural production (Bodner et al., 2015). In addition, water scarcity and drought contribute to the inhibition of crop growth and negatively impact plant quantitative and qualitative yield, as well as physiology and morphology (Bayat and Moghadam, 2019).
Applying fertilizers and nutritional compounds during the growth state is a crucial strategy to decrease the impact of drought on crops. Several studies have shown that micronutrients mitigate crops drought stress (Adrees et al., 2020; Bashir et al., 2020; Dimkpa et al., 2020). Zinc is a microelement, necessary for many plant activities, which plays a crucial role in protein, enzyme, and chlorophyll synthesis and the improvement of agricultural crop yield (Singh et al., 2018; Dimkpa et al., 2019). Furthermore, studies have confirmed the effect of Zn, in the form of zinc sulfate, zinc chelate, and Zn nanoparticle, on enhanced resistance to environmental stress by improving morphological, physiological, and biochemical factors in different plants (Mahdieh et al., 2018; Farooq et al., 2020; Faizan et al., 2021; Tariverdizadeh et al., 2021).
Nanoparticles (particles that are ≤100 nm in at least one dimension) play an essential role in altering different physiological processes in plants, nutrient absorption, plant growth, and increased plant resistance to abiotic stress (Jordan et al., 2018; Dimkpa et al., 2020). Moreover, nanoparticles are used in the form of micronutrients and fertilizers to improve qualitative and quantitative crop yield. Because of the limited and controlled release of nutrients, nanoparticles can contribute to plant growth and resistance to different types of stress (Adrees et al., 2020; Bashir et al., 2020).
Zn nanoparticle is a new plant fertilizer produced with the technology of nanoparticle synthesis, which has many benefits, such as increased tolerance to abiotic stress in some plants (Cicek and Nadaroglu, 2015; Rossi et al., 2018; Singh et al., 2018; Zhang et al., 2018; Farooq et al., 2020; Taghizadeh et al., 2020; Faizan et al., 2021; Tariverdizadeh et al., 2021). Studies have reported the positive effects of Zn nanoparticles on improved tomato (Faizan et al., 2021) and wheat (Bashir et al., 2021) yield under salinity and drought stress, respectively. In addition, Dimkpa et al. (2019) and Motyka et al. (2019) have approved increased yield of sorghum plants under drought stress and enhanced the situation of bryophytes under oxidative stress with the help of Zn nanoparticles. Moreover, different forms of zinc were applied to the coffee plant, compared to zinc sulfate, Zn nanoparticles could positively affect coffee plant growth and yield (Rossi et al., 2018).
Element interactions in plants can affect plant functions, such as growth, yield, and stress resistance. Studies have suggested that the Zn element has antagonistic and synergistic interactions with Cu, Fe, and P elements in absorption, transfer, and chemical reactions in the plant. It may vary depending on factors such as plant type, soil type, nutrition, and weather conditions (Fageria et al., 2012; Izsáki, 2014; Rietra et al., 2017). The impact of element interactions to nanoparticle form on abiotic stress resistance (e.g., drought) has been reported in some studies (Liu et al., 2020; Ahmed et al., 2022).
Dracocephalum kotschyi belongs to Lamiaceae and is one of the 60 species of the genus Dracocephalum. This species is endemic to Iran and is currently considered an endangered plant. D. kotschyi is known to have several medicinal properties (Muaffarīyān, 1996). This species is traditionally a medicine plant in Iran with several therapeutic effects such as analgesic, antispasmodic, and anticancer (Sharafi et al., 2014). D. kotschyi also was used in the treatment of headaches, congestion, stomach and liver disorders (Dorosti and Jamshidi, 2016). In addition to various pharmacological effects, it is used in industry, medicine, and food as a source of some valuable secondary metabolites. The most important secondary metabolites of this specimen include monoterpene glycosides, trypanocidal terpenoids (Saeidnia et al., 2004), flavonoids, rosmarinic acid (Fattahi et al., 2013), and some essential oils (Saeidnia et al., 2007). This herbaceous, beautiful, and aromatic wild-growing plant with a height of about 10-20 cm is grown in mountainous and highland areas of the central and northern regions of the country (Rechinger, 1986). In addition, D. kotschyi is used as an ornamental plant due to its aesthetic characteristics, such as abundant white and fragrant flowers, plant form, relatively fast growth, long flowering stage, and possible abiotic stress tolerance. Therefore, it can be used as a valuable ornamental plant in sustainable green space design in arid and semi-arid areas.
Climate change, especially consecutive years of drought, has been one of the critical extinctions treat of this valuable medical-ornamental plant. Notably, that most studies performed on this species have focused more on its phytochemical attributes, and few studies are available on strategies to enhance the abiotic stress tolerance of this species which could contribute to the cultivation development and survival of this plant.
The present study aimed to determine the effect of different concentrations of zinc in two forms of zinc oxide (ZnO-N) nanoparticles and zinc sulfate (ZnSO4) on the drought tolerance of D. kotschyi plant. It is hypothesized that ZnO-N compared to ZnSO4 might be more effective in alleviating the drought tolerance of D. kotschyi through its positive function on the physiological and biochemical processes of the plant.
Mature seeds of D. kotschyi were collected from its natural habitat in Semirom city (Isfahan province, Iran) with latitude and longitude of 31°49’, 51° 59’ “, respectively, and altitude of 2400 m above sea level. Seeds were grown in growth trays containing 80% cocopeat and 20% perlite. After germination, seedlings in the six-leaf stage were transferred to plastic pots containing sandy loam soil with a low organic matter (1.5%) and a pH of 7.2. Following ten days of seedling transplanting, potted plants were regularly (twice a week) fed with an NPK fertilizer (20-20-20, 2g/l). After three weeks of growth in optimal conditions in the greenhouse, seedlings were divided in two groups: the first group (control) under well-watering (100% field capacity (FC)) and the second one (drought-stressed group) under limited watering (50% field capacity). According to the gravimetric method, soil moisture content was measured (Datta et al., 2009). It was monitored daily during the growth period using a soil moisture meter (EXTECH MO750, USA, probe length: 20 cm probe and max resolution: 0.1%). The pots are watered (when the humidity dropped below a certain level) to keep the moisture content at the desired level (100% and 50% FC).
Foliar fertilization with ZnSO4 and ZnO-N at three concentrations of 0 (control), 10, and 20 mg/l was performed two times; first simultaneously with the start of drought stress and second three weeks after the start of drought stress (Rossi et al., 2018; Azmat et al., 2022). The control plants were watered up to 100% FC and received no fertilizer treatments (ZnSO4 and ZnO-N).
ZnO-N were procured from Iranian Nanomaterials Pioneers Company, NANOSANY (Mashhad, Iran). Its characteristics, such as particle size, and purity, are presented in Figure 1 and Supplementary File 1. ZnO-N were added to deionized water and dispersed using ultra-sonication for 30 minutes. The treated plants were collected 45 days after the start of drought stress treatment, and leaf samples were immediately placed in liquid nitrogen and stored in a -80 freezer for later analysis.
Figure 1 (A) Transmission Electron Micrograph (TEM), (B) SEM (Scanning electron microscope) and (C) X-ray diffraction (XRD) of Zinc oxide nanoparticles, Iranian Nanomaterials Pioneers Company, NANOSANY, 2020.
In order to measure EC, one gram of each leaf was added to 20 ml of distilled water and kept at room temperature (24°C) for 24 h. In addition, its electrical conductivity was measured by an EC meter as the initial EC (EC1). After that, the samples were placed in an autoclave at 120°C for 15 min, and their secondary EC (EC2) was determined. The following equation was exploited to calculate EC.
Fresh weight (FW) of fully mature leaves was measured immediately after collection based on a method by (Ritchie et al., 1990). Afterward, they were placed in distilled water at 4°C for 24 h to determine the turgor weight (TW). In the next stage, samples were dried in an oven at 70°C for 48 hours, and the dry weight (DW) of each sample was recorded. The percentage of RWC was estimated using the equation below:
Leaf samples homogenized with 80% acetone were centrifuged for 5 min at 3000×g to calculate photosynthetic pigments (chlorophyll and carotenoid). Following that, optical absorbance of the supernatant solution was read at wavelengths of 470, 645, and 663 nm using a spectrophotometer (UV-VIS, Optima SP-3000 Plus, Bratislava, Slovakia). In the next stage, total chlorophyll and carotenoids were calculated in mg/g FW (Lichtenthaler, 1987).
Soluble sugar was determined using a phenol-sulfuric acid method based on acid hydrolysis of soluble sugars and furfural mixture creation (Kochert, 1978). After that, 100 µL of ethanol extract from the leaf sample, 300 µL of distilled water, and 1 mL 5% phenol were mixed while vortexed. In the next stage, 1 mL of concentrated sulfuric acid was added to the mixture, and adsorption was read at 485 nm against blanks after 30 min. In addition, the sugar content of the samples was assessed by using standard curves in mg/g FW.
Based on the technique by Bates et al. (1973), 200 µL of ninhydrin and glacial acetic acid reagents was added to 200 µL of extract from homogenized leaf sample and sulfuric acid. Afterward, the samples were placed in a hot water bath at a temperature of 100°C for 30 min. Then, 600 µL of toluene was added to the samples following immediate rapid cooling and vortexed for 30 sec. After 20 minutes, the optical absorbance of the upper solution was read at 520 nm, and the concentration of proline in the solution was calculated in mg/g FW using the proline standard curve.
In order to assay total protein, 1 mL of extraction buffer (PBS) and EDTA with a concentration of 0.1 mM was added to powdered leaves using liquid nitrogen and homogenized. After that, the samples were centrifuged at 12000g for 10 minutes at 4°C. The supernatant was stored in a freezer at -80°C for protein and enzyme activity assay. In the next stage, 2.5 mL of Bradford solution was added to 50 µL of supernatant to determine the protein content of the extract. After 5 min, adsorption was read at a wavelength of 595 nm. Certain concentrations of bovine serum albumin (BSA) standard protein were prepared in order to draw a standard protein diagram (Bradford, 1976).
In this stage, 50 mM of PBS (pH=7), 0.02 M pyrogallol, and 50 µL enzymatic extract were prepared as the reaction mixture to assess the activity of polyphenol oxidase (PPO). Adsorption of all samples was measured based on the purpurogallin content at a wavelength of 420 nm in one min at a 5 second interval., Ultimately, the values obtained were calculated in Umg-1 protein (Raymond et al., 1993).
Superoxide dismutase (SOD) enzyme activity in the reaction mixture was assessed based on the measurement of inhibition of photochemical reduction of nitro blue tetrazolium. Reaction mixture encompasses BPS (50 mM, pH=7.8), methionine (13 mM), nitro blue tetrazolium (75 µM), riboflavin (2 µM), sodium carbonate (50 mM), triton X-100 (0.025%) and 50 µL of enzymatic extract. Sample adsorption was read at a wavelength of 560 nm against the blanks following exposure to light for 15 min. In addition, Blue Formazan production was expressed in Umg-1 protein by measuring the increase in absorption (Giannopolitis and Ries, 1977).
Guaiacol peroxidase (GPO) enzyme activity was assessed using (Hemeda and Klein, 1990) method. The reaction mixture included BPS (20 mM, pH=6), Guaiacol (5 mM), H2O2 (1 mM), and 50 µL of enzymatic extract. In addition, enzyme activity resulting from guaiacol oxidation with increased adsorption at 570 nm was determined. Alternation in adsorption was measured every five seconds for a minute and was expressed in Umg-1 protein.
Following the method by Pathan et al. (2010), intact and mature leaf samples separated from plants were powdered after drying at room temperature without any pretreatment and were used for SEM imaging. In addition, microanalysis of Zn, as well as Cu, Fe, and P elements as the most critical interactive elements with Zn, and elemental mapping was carried out using SEM-EDX (Energy-Dispersive X-ray). It is worth mentioning that all microscopic studies were done at Bu-Ali Research Institute, Mashhad University of Medical Sciences.
In the present study, a factorial experiment was conducted based on the completely randomized design with four replications (each pot represented one replication with two plants). Treatments were drought stress, including 50% FC and 100% FC and two zinc sources, ZnSO4 and ZnO-N, at three concentrations of 0, 10, and 20 mg/l. The distribution normality of data was tested using the Anderson-Darling test prior to analysis. Analysis of variance (ANOVA) and means the comparison of measured attributes was performed using Minitab 16 software and Tukey’s test at 5% probability level, respectively.
It is evident from Table 1 that ZnSO4 and ZnO-N and their interactions with drought stress had significant effects on EC in D. kotschyi (P ≤ 0.05) (Table 1). In this respect, the EC of the samples (22.12%) decreased by 83%, compared to control (40.47%), by increasing the ZnO-N concentration. The lowest EC was observed in samples under drought stress and without drought stress receiving 20 mg of ZnO-N (18.4% and 25.84%). Foliar fertilization with ZnSO4 at a concentration of 10 mg/l had a mitigating effect on the EC, such that the lowest EC was obtained in plants under drought stress and fertilized by 10 mg/l of ZnSO4 (20.62%) (Figure 2, right).
Table 1 Analysis variance of physiological and biochemical traits in treated D. kotschyi by ZnSO4 and ZnO-N under drought stress.
Figure 2 Impact of ZnSO4 and ZnO-N on RWC (A) and EC (B) in D Kotschyi under Drought Stress. Means followed by the same letters did not differ significantly at p ≤ 0.05, n = 4.
Interaction of foliar fertilization of ZnSO4 and ZnO-N with drought stress had a significant effect on changes in RWC content in D. kotschyi (P ≤ 0.05) (Table 1). At both concentrations of 10 and 20 mg/l, ZnSO4 increased RWC in plants under drought stress by up 10%, compared to plants under drought stress not treated with ZnSO4 (Figure 2, left). This increase was about 3% in plants under drought stress fertilized with ZnO-N at two 10 and 20 mg/l concentrations, although these mitigating changes were not statistically significant. In addition, the highest RWC was related to control plants, which received no treatments, under full irrigation (78.46%) (Figure 2, left).
The highest total chlorophyll content was obtained in plants under drought stress and foliar fertilization with ZnSO4 at concentrations of 10 (1.22 mg/g FW) mg/l and then 20 (1.20 mg/g FW) mg/l. The lowest amount of chlorophyll (1.07 mg/g FW) was observed in plants under drought stress and without zinc treatment. Moreover, the highest level of carotenoids (2 mg/g FW) was obtained in plants under drought stress. The other treatments had no significant effects on these contents (Table 1; Figure 3, left).
Figure 3 Impact of ZnSO4 on total chlorophyll (A) and ZnO-N on sugar (B) in D Kotschyi under Drought Stress. Means followed by the same letters did not differ significantly at p ≤ 0.05, n = 4.
Drought stress and ZnO-N interaction had a significant effect on soluble sugar in leaf samples of D. kotschyi (P ≤ 0.05) (Table 1). In addition, the highest sugar content (32.23 mg/g FW) was observed in plants under full irrigation treated with 10 mg/l of ZnO-N. Meanwhile, no significant difference was observed between these samples and the plants under drought stress treated with 20 mg/l of ZnO-N. In this regard, the lowest sugar content (19.16 mg/l FW) was observed in control samples and plants under full irrigation treated with 20 mg/l of ZnO-N (Figure 3, right).
In this study, drought stress, ZnSO4 and interaction of drought stress with ZnO-N significantly affected proline content in D. kotschyi (P ≤ 0.05) (Table 1). According to the results, proline content increased in plants under drought stress (213.87 µM/g FW) 50% more than plants under non-stressed conditions (142.32 µM/g FW). Furthermore, ZnSO4 fertilization at a concentration of 10 mg/l (233.09 µM/g FW) led to a 136% increase in proline content, compared to plants not treated with this substance (98.67 µM/g FW). According to the results, the highest proline content (230.13 µM/g FW) was observed in plants under drought stress receiving ZnSO4 fertilization at a concentration of 10 mg/l, which had a significant difference with plants under full irrigation (no drought stress) (Figure 4, left).
Figure 4 Impact of ZnSO4 on total protein (A) and ZnO-N on proline (B) and in D Kotschyi under Drought Stress. Means followed by the same letters did not differ significantly at p ≤ 0.05, n = 4.
ZnO-N and also interaction of ZnSO4 with drought stress had a significant effect on the protein content of D. kotschyi (P ≤ 0.05). In this regard, the highest protein content (12.02 mg/g FW) was observed in the treated plants with 10 mg/l ZnSO4 under drought stress. The lowest one was obtained in plants with 10 mg/l ZnSO4 under full irrigation (Figure 4, right).
In this study, the activity levels of SOD, PPO, and GPO enzymes in D. kotschyi under drought stress increased by 20%, 90%, and 75%, respectively, compared to control samples. According to the results, the highest SOD and GPO enzyme activities were observed in plants under drought stress treated with 20 mg/l ZnO-N (Figure 5). Meanwhile, the use of 10 mg/l ZnO-N in plants under drought stress led to the highest activity of the PPO enzyme (Figure 5, right-above) under Drought Stress
Figure 5 Impact of ZnO-N on SOD (A) and GPO (B); ZnSO4 on PPO (C) in D Kotschyi under Drought Stress. Means followed by the same letters did not differ significantly at p ≤ 0.05, n = 4.
SEM-EDX analysis and elemental mapping were conducted to support our findings that Zn-nanoparticles could improve the drought tolerance in D. kotschyi by changing the concentrations of Cu, Fe, and P. As seen in Table 2, Fe and Cu percentages decreased with a decline in the concentration of Zn in plants, while an increase was observed in P percentage. According to Table 3, the concentrations of Zn, Cu, and Fe elements were respectively 5.5%, 5%, and 2.5% higher and the P element was 13% lower in plants treated with ZnO-N and ZnSO4, compared to control plants. In addition, the concentration of Cu, Fe, and Zn elements in ZnSO4 treatment was 1.5% higher, compared to ZnO-N, whereas the difference in P element concentration was 3.5% lower in this regard (Tables 2, 3).
Table 2 Scanning electron microscopy images, Zn maps of leaf surfaces and their EDX results of non-fertilized (control) and foliar fertilized with ZnSO4 and ZnO-N in D. kotschyi under drought stress.
Table 3 Comparison of measured elements percentage with control (non-fertilized) in treated D. kotschyi by ZnSO4 and ZnO-N through SEM-EDX method under drought stress.
The present study aimed to increase the drought tolerance of D. kotschyi using ZnO-N and ZnSO4 treatments. According to the results, ZnO-N reduced EC on the plants under drought stress, while the mitigating effect of ZnSO4 on EC at the same conditions was less than ZnO-N. Meanwhile the highest sugar and proline content as well as the highest activity of SOD and GPO (and to some extent PPO) in D. kotschyi exposed to drought stress were at the same conditions observed by the application of ZnO-N. Using ZnSO4 increased chlorophyll and protein content and also PPO activity in this plant under drought stress.
RWC in plants indicates the amount of water stored in the leaves and the rate of transpiration. Stress (in particular drought stress) usually reduces electrical conductivity in plants, which leads to a decline in RWC (Ahluwalia et al., 2021). In this experiment, there was a significant decrease in RWC in plants under drought stress. Nonetheless, ZnO-N and ZnSO4 treatments increased RWC in plants by 10% and 3%, respectively (although not statistically significant). Studies showed that using ZnO-N increases RWC in Solanum melongena (Semida et al., 2021) and Triticum aestivum (Taran et al., 2017) under drought stress. Similarly, ZnSO4 improved RWC in wheat (Sattar et al., 2022) and triticale (Kheirizadeh Arough et al., 2016) under stress. The Zn element affects water absorption and transport capacity in plants and decreases short-term adverse effects of environmental stresses (Kasim, 2007; Disante et al., 2010). On the other hand, regulation of gene expression affecting tolerance to environmental stress depends on the Zn element (Cakmak, 2000).
Different stresses, such as drought stress, damage cellular membrane in plants and lead to ion leakage, thereby increasing EC in the plant (Luo et al., 2012). Therefore, low EC in plants could be a criterion for resistance to environmental stresses (Blum, 2005). In this experiment, ZnO-N and to some extent ZnSO4 reduced EC in plants under stress, respectively compared to the control plants. According to the results, a decline in EC of plants under stress was due to the use of ZnO-N in wheat (Adrees et al., 2020) and corn (Rizwan et al., 2019).
Results indicated that drought stress led to a decrease in total chlorophyll content in D. kotschyi, and ZnSO4 was able to increase chlorophyll content in the plant under stress conditions. In support of this finding, Cakmak (2000) reported that zinc promotes chlorophyll synthesis by protecting sulfhydryl groups. In addition, it influences pigment content by creating a balance in the concentration and providing other elements involved in chlorophyll synthesis (N and Mg) (Movahhedi et al., 2017). According to various studies, Zn increases chlorophyll content under drought stress (Dimkpa et al., 2020; Faizan et al., 2021; Sun et al., 2021).
While drought treatment did not affect the sugar content of D. kotschyi, ZnO-N at 20 mg/l increased soluble sugar in the plants under drought stress, compared to the control plants. It is notable that Zn plays a role in carbohydrate metabolism and affects the activity of carbonic anhydrase, which regulates the CO2-sensing pathway and improves drought tolerance in the plant (Tewari et al., 2019). The use of Zn in plants under drought stress led to an increase in the accumulation of soluble carbohydrates and increased drought tolerance in Phaseolus vulgaris (Mahdieh et al., 2018) and Sesamum indicum (Movahhedi et al., 2017). In the corn plant, the use of ZnO-N was associated with an improvement in drought tolerance of the plant through the regulation of the activity of key enzymes in carbohydrate metabolism (Sun et al., 2021).
In this experiment, proline accumulated in the plants in response to drought stress. Proline acts as an essential osmolyte and an efficient antioxidant in regulating cell osmosis and ROS scavenging in plants under stress. Proline maintains cellular turgidity, stabilizes the structure of enzymes and proteins, and protects the plant under stress. The increase in proline content in plants can be considered an as indicator of improved drought tolerance (Ahluwalia et al., 2021). Fertilization treatment with 10 mg/l ZnO-N increased proline content in plants under drought stress. Results of some previous studies were indicative of increased proline content as a result of ZnO-N (Noohpisheh et al., 2021) and Zn (Zengin, 2006) in Trigonella foenum-graecum and Phaseolus vulgaris under environmental stresses. According to our findings, in some plants under drought stress, the increase in protein concentration was associated with higher RWC (Sandhya et al., 2010; Silva et al., 2020). Moreover, Zn led to the accumulation of proline under drought stress, and proline accumulation resulted in the protection of osmoregulation enzymes and increased drought tolerance in the plant (Hasegawa et al., 2000). The exact mechanism of the role of proline on antioxidant enzymes has not been clarified (Haque et al., 2007). However, under environmental stress such as drought, the increase in the antioxidant enzymes’ activity is usually accompanied by an increase in the proline content (Ghaffari et al., 2019).
The treatments of ZnO-N and ZnSO4 led to increased protein content in plants under drought stress. This could be due to the increased level of photosynthesis, RWC, chlorophyll content, and also enzymes involved in synthesizing sugar in plants (Sun et al., 2021). The decrease in EC and improvement of damages caused by ion leakage in stress conditions could also be another reason for increased protein content in Zn- treated plants (Cakmak, 2000). Sugars and proteins are two key components in regulating plant responses to stresses. On the hand, transcription, translation, protein stability, and activity are the regulatory roles of sugars in plants (Rolland et al., 2006). On the other hand, various enzymes and proteins are involved in the sugar synthesis of plants (Pfister and Zeeman, 2016). Soluble sugars as osmoprotectors, stabilize proteins and cell membranes under environmental stresses lead to different expressions of some proteins related to sugar metabolism. Moreover, sugar signals enhance plant defense responses through some enzymes (like mitogen-activated protein kinase) signaling cascade (Kocal et al., 2008). In a study, a significant positive correlation between soluble proteins and sugars in the growth of two poplar species under drought stress was reported by Yang and Miao (2010). In this respect, our findings are congruent with the reports related to the use of Zn (as a nanoparticle or sulfate) in various plants under drought stress (Patra et al., 2013; Singh et al., 2018; Dimkpa et al., 2019; Motyka et al., 2019; Bashir et al., 2021).
In our study, drought stress significantly increased the activity of SOD, GPO, and PPO antioxidant enzymes in D. kotschyi. Studies show that antioxidant enzyme activities increase to improve plant tolerance in stress conditions. The SOD enzyme plays an important role in the cell’s antioxidant defense system by converting peroxide radicals to hydrogen peroxide (Sharma et al., 2012). On the other hand, the PPO enzyme in the immune system of plants under environmental stress, prevents excessive reduction of electron transfer in photosynthesis (Islam et al., 2020). Meanwhile, GPO can convert H2O2 to water through oxidation (Dianat et al., 2016).
In the current research, ZnO-N increased the activities of SOD, GPO, and some extent PPO enzymes in D. kotschyi under drought stress. Zn is a metal component and cofactor of many enzymes and increases the activity of antioxidant enzymes under drought stress conditions (Kheirizadeh Arough et al., 2016). The use of ZnO-N plays a role in modulating the activity of various enzymes, especially those related to plant adaptation to stress (Verma et al., 2020). In some previous studies, using ZnO-N intensified SOD enzyme activities in Salvinia natan (Hu et al., 2014) and Gossypium hirsutum (Priyanka and Venkatachalam, 2016). Bashir et al. (2021) reported that SOD and PPO activities increased in Triticum aestivum under oxidative stress conditions by using Zn nanoparticles. In addition, iron and Zn nanoparticles increased GPO activities in Oryza sativa (Upadhyaya et al., 2017) and Dracocephalum moldavica (Moradbeygi et al., 2020) under environmental stress.
Some reports are indicating the adverse effects of nanoparticles on biological systems and cellular components. ZnO-N are also shown to have some genotoxic effects on human epidermal cells (Sharma et al., 2009). It has been found that ZnO-N can be toxic for either normal or cancer cells (Ali et al., 2012). Moreover, it can cause oxidative DNA damage in human lung cells (Ng et al., 2017). Despite some reports showing the negative impact of ZnO-N on biological systems, there are other studies indicating that the toxicity of ZnO-N is not significant to human health and the environment. It has been found that ZnO-N are non-toxic up to a certain level but can be dangerous at higher concentrations (Nagara et al., 2022). In this regard, Amuthavalli et al. (2021) showed the moderate side effect of biosynthesized ZnO-N on rats and they reported that ZnO-N could be used as a multipurpose agent in the field of biomedical research (Amuthavalli et al., 2021). ZnO-N synthesized from plants (Melia azedarach) showed less toxicity compared to the one synthesized through the conventional method (Dinga et al., 2022). ZnO-N have a wide range of biomedical applications (Agarwal et al., 2017) and are also used in cosmetic products such as sunscreen creams (Hong et al., 2022). Recent studies have shown that the risks and benefits of zinc oxide nanoparticles depend on various factors including the concentration of ZnO-N, the synthesis method, and the tested organism (Czyżowska and Barbasz, 2022).
Increasing the concentration of Zn in the plant could cause poisoning by disrupting the balance of other nutrients and reducing photosynthesis (Kabata-Pendias, 2010). Meanwhile, nano-fertilizers have a lower chance of creating toxicity in the plant and soil with slower and more appropriate delivery of nutrients to the plant (Solanki et al., 2015). However, nano-fertilizers could be toxic at high concentrations, for instance, ZnO-N at high concentrations could inhibit growth in Fagopyrum esculentum (above 1000 mg/l) (Lee et al., 2013) and Radish (above 20 mg/l) (Lin and Xing, 2007). In this experiment, low concentrations of ZnO-N (10 and 20 mg/l) were applied on D. kotschi with the approach of ornamental/landscape use (not as an edible plant).
According to the findings, there was a significant difference in the concentration of P, Cu, Fe, and Zn elements in treated plants with ZnO-N and ZnSO4, compared to the control plants. However, no significant difference was observed between the treated plants with two fertilizers (ZnO-N and ZnSO4) in terms of the element concentration. Double interactions between P, Fe, and Zn have long been recognized in plants, such that Cu and Fe concentrations increased with an increase in Zn concentration (Fan et al., 2021). Furthermore, studies have approved the antagonistic effect of Zn and Fe with P as a nutrient in some plants (Zheng et al., 2009). Overall, P, Cu, Fe, and Zn play a significant role in drought stress tolerance in plants (Marschner, 2012; Tripathi et al., 2018; Dimkpa et al., 2019).
In a previous study (Akbari et al., 2013), fertilization with Zn reduced drought stress through decreasing H2O2 content and lipid peroxidation, which resulted from increased antioxidant enzymes (CAT, GPX, and SOD). Fe regulates the unfavorable effects of drought, salinity, and heavy elements by controlling cellular redox states and the antioxidant defense mechanism, such as catalase and superoxide dismutase (Tripathi et al., 2018). Moreover, Cu increases drought tolerance by increasing the activity of SOD, ascorbate peroxidase enzymes, and anthocyanin levels (Van Nguyen et al., 2022). In addition, P increases water uptake and maintains cellular turgidity by improving root growth, thereby regulating stomatal conductance and ultimately increasing plant photosynthesis and drought tolerance (Waraich et al., 2011).
Compared to conventional fertilizers, nano fertilizers due to their higher absorption level and slower release rate are more efficient in plant processes, including improving tolerance to stresses (Manjunath et al., 2016; Bashir et al., 2020). In general, foliar spraying by nanoparticles has many paths for absorption in plants due to the small size of the particles. Nanoparticles can enter the leaves through stomata, endocytosis, leaf hydathodes, and direct absorption (Hong et al., 2021). In this experiment, the size of the ZnO-N was not larger than 20 nm, so according to the report of Alshaal and El-Ramady (2017), compared to ZnSO4 it seems to be absorbed to a greater extent, and there was no limitation passing through the cell wall pores. In this regard, Rajemahadik et al. (2018) reported that nanoparticles because of dynamic properties have a high surface area, activity, and catalytic surface. Nanoparticles rapidly react and disperse and can absorb more water. These properties can make nanoparticle fertilizers perform better in plant functions than other forms of fertilizers.
The Efficiency of ZnO-N compared to ZnSO4 (as two Zn fertilizers) on the growth of Zea mays was confirmed in the study of Subbaiah et al. (2016). Also, Rossi et al. (2018) by foliar spraying of ZnO-N and ZnSO4 on coffee plants reported that ZnO-N significantly increased the photosynthesis rate and growth traits. Moreover, there are some studies on the positive effect of Zn-nanoparticles on enhancing tolerance to abiotic stresses (in particular drought) in Solanum melongena (Semida et al., 2021), Triticum aestivum (Taran et al., 2017), wheat (Adrees et al., 2020), corn (Rizwan et al., 2019), Trigonella foenum-graecum (Noohpisheh et al., 2021) and Phaseolus vulgaris (Zengin, 2006).
Moreover, given the equal leaf Zn level in the treated plants with ZnO-N and ZnSO4 at the end of the experiment (EDX analysis), the toxicity of ZnO-N was not significant than ZnSO4. In other words, it can be said that due to the use of low concentrations of ZnO-N (10 and 20 mg/l) in this experiment, no toxicity was observed using this fertilizer. Moreover, D. kotschyi can be considered as a model organism to present reduced toxicity effects of ZnO-N through supplementary experiments related to toxicity assessments (Supplementary File 2 and Supplementary File 3).
Considering the drought problem, using compounds that could increase drought tolerance in plants plays an essential role in water management in agriculture and urban green space. This research has demonstrated that zinc nanoparticles (ZnO-N) improved drought tolerance in Dracocephalum kotschyi (a medicinal-ornamental endangered plant) under drought stress. Based on our findings, ZnO-N positively affected most of biochemical (sugar and proline) and physiological (electrolyte conductivity and to some extent relative water content) factors in drought conditions. Moreover, ZnO-N significantly increased the activity of antioxidant enzymes (SOD, GPO, and some extent PPO) in D. kotschyi exposed to drought stress. The positive effect of ZnSO4 application on this plant under drought stress was achieved through increased EC, RWC, chlorophyll and protein content, and also POP activity. In addition, no significant difference was observed in the concentration of P, Cu, Fe, and Zn elements in plants treated with ZnO-N and ZnSO4. Given the significant role of sugar and proline and also antioxidant enzymes (SOD, GPO, and to some extent PPO) in plants’ defense systems and tolerance to environmental stresses, ZnO-N seems to be more efficient compared to ZnSO4 in improving drought tolerance in D. kotschyi. Since there are some reports regarding the toxicity of ZnO-N at higher concentrations, it is recommended to use ZnO-N at a low level (less than 1000 mg/l).
The original contributions presented in the study are included in the article/Supplementary Material. Further inquiries can be directed to the corresponding author.
All authors contributed to the study conception and design. Material preparation, experimental works and analysis were performed by ZK. The first draft of the manuscript was written by ZK. LS commented on preliminary versions of the manuscript. All authors contributed to the article and approved the submitted version.
This work was supported by a grant from Ferdowsi University of Mashhad-Iran [Grant number: 50779].
The authors declare that the research was conducted in the absence of any commercial or financial relationships that could be construed as a potential conflict of interest.
All claims expressed in this article are solely those of the authors and do not necessarily represent those of their affiliated organizations, or those of the publisher, the editors and the reviewers. Any product that may be evaluated in this article, or claim that may be made by its manufacturer, is not guaranteed or endorsed by the publisher.
The Supplementary Material for this article can be found online at: https://www.frontiersin.org/articles/10.3389/fpls.2023.1063618/full#supplementary-material
Adrees, M., Khan, Z. S., Ali, S., Hafeez, M., Khalid, S., Rehman, M. Z., et al. (2020). Simultaneous mitigation of cadmium and drought stress in wheat by soil application of iron nanoparticles. Chemosphere 238, 124681. doi: 10.1016/j.chemosphere.2019.124681
Agarwal, H., Venkat Kumar, S., Rajeshkumar, S. (2017). A review on green synthesis of zinc oxide nanoparticles–an eco-friendly approach. Res. Eff. Tech. 3, 406–413. doi: 10.1016/j.reffit.2017.03.002
Ahluwalia, O., Singh, P. C., Bhatia, R. (2021). A review on drought stress in plants: Implications, mitigation and the role of plant growth promoting rhizobacteria. Res. Envir. Sustai. 5, 100032. doi: 10.1016/j.resenv.2021.100032
Ahmed, T., Noman, M., Rizwan, M., Ali, S., Ijaz, U., Nazir, M. M., et al. (2022). Green molybdenum nanoparticles-mediated bio-stimulation of bacillus sp. strain ZH16 improved the wheat growth by managing in planta nutrients supply, ionic homeostasis and arsenic accumulation. J. Hazard. Mater. 423, 127024. doi: 10.1016/j.jhazmat.2021.127024
Akbari, G. A., Amirinejad, M., Baghizadeh, A., Allahdadi, I., Shahbazi, M. (2013). Effect of zn and fe foliar application on yield, yield components and some physiological traits of cumin (Cuminum cyminum) in dry farming. Int. J. Agro. Plant Prod. 4, 3231–3237.
Ali, S. M. U., Fakhar-e-Alam, M., Wazir, Z., Kashif, M., Atif, M., Willander, M., et al. (2012). Cytotoxic effects of zinc oxide nanoflakes (ZNO NFS) in human muscle arcinoma. Int. J. Med. Med. Sci. 2, 053–058.
Alshaal, T., El-Ramady, H. (2017). Foliar application: from plant nutrition to biofortification. EBSS 1, 71–83. doi: 10.21608/jenvbs.2017.1089.1006
Amuthavalli, P., Hwang, J. S., Dahms, H. U., Wang, L., Anitha, J., Vasanthakumaran, M., et al. (2021). Zinc oxide nanoparticles using plant lawsonia inermis and their mosquitocidal, antimicrobial, anticancer applications showing moderate side effects. Sci. Rep. 11, 8837. doi: 10.1038/s41598-021-88164-0
Azmat, A., Tanveer, Y., Yasmin, H., Hassan, M. N., Shahzad, A., Reddy, M., et al. (2022). Coactive role of zinc oxide nanoparticles and plant growth promoting rhizobacteria for mitigation of synchronized effects of heat and drought stress in wheat plants. Chemosphere 297, 133982. doi: 10.1016/j.chemosphere.2022.133982
Bashir, A., Rehman, M. Z., Hussaini, K. M., Adrees, M., Qayyum, M. F., Sayal, A. U., et al. (2021). Combined use of zinc nanoparticles and co-composted biochar enhanced wheat growth and decreased cd concentration in grains under cd and drought stress: A field study. Environ. Technol. Innov. 23, 101518. doi: 10.1016/j.eti.2021.101518
Bashir, M. A., Silvestri, C., Ahmad, T., Hafiz, I. A., Abbasi, N. A., Manzoor, A., et al. (2020). Osmotin: A cationic protein leads to improve biotic and abiotic stress tolerance in plants. Plants (Basel) 9, 992. doi: 10.3390/plants9080992
Bates, L. S., Waldren, R. P., Teare, I. D. (1973). Rapid determination of free proline for water-stress studies. Plant Soil 39, 205–207. doi: 10.1007/BF00018060
Bayat, H., Moghadam, A. N. (2019). Drought effects on growth, water status, proline content and antioxidant system in three Salvia nemorosa l. cultivars. Acta Physiol. Plant 41, 1–8. doi: 10.1007/s11738-019-2942-6
Blum, A. (2005). Drought resistance, water-use efficiency, and yield potential–are they compatible, dissonant, or mutually exclusive? Aust. J. Agric. Res. 56, 1159–1168. doi: 10.1071/AR05069
Bodner, G., Nakhforoosh, A., Kaul, H. P. (2015). Management of crop water under drought: a review. Agron. Sustain. Dev. 35, 401–442. doi: 10.1007/s13593-015-0283-4
Bradford, M. M. (1976). A rapid and sensitive method for the quantitation of microgram quantities of protein utilizing the principle of protein-dye binding. Anal. Biochem. 72, 248–254. doi: 10.1016/0003-2697(76)90527-3
Cakmak, I. (2000). Tansley review no. 111: Possible roles of zinc in protecting plant cells from damage by reactive oxygen species. New Phytol. 146, 185–205. doi: 10.1046/j.1469-8137.2000.00630.x
Cicek, S., Nadaroglu, H. (2015). The use of nanotechnology in the agriculture. Adv. Nano. Res. 3, 207–223. doi: 10.12989/anr.2015.3.4.207
Czyżowska, A., Barbasz, A. (2022). A review: zinc oxide nanoparticles – friends or enemies? Int. J. Environ. Health Res. 32, 885–901. doi: 10.1080/09603123.2020.1805415
Datta, A., Sindel, B. M., Kristiansen, P., Jessop, R. S., Felton, W. L. (2009). The effects of temperature and soil moisture on chickpea (Cicer arietinum l.) genotype sensitivity to isoxaflutole. J. Agron. Crop Sci. 195, 178–185. doi: 10.1111/j.1439-037X.2009.00362.x
Dianat, M., Saharkhiz, M. J., Tavassolian, I. (2016). Salicylic acid mitigates drought stress in Lippia citriodora l.: Effects on biochemical traits and essential oil yield. Biocatal. Agric. Biotechnol. 8, 286–293. doi: 10.1016/j.bcab.2016.10.010
Dimkpa, C. O., Andrews, J., Sanabria, J., Bindraban, P. S., Singh, U., Elmer, W. H., et al. (2020). Interactive effects of drought, organic fertilizer, and zinc oxide nanoscale and bulk particles on wheat performance and grain nutrient accumulation. Sci. Total. Environ. 722, 137808. doi: 10.1016/j.scitotenv.2020.137808
Dimkpa, C. O., Singh, U., Bindraban, P. S., Elmer, W. H., Gardea-Torresdey, J. L., White, J. C. (2019). Zinc oxide nanoparticles alleviate drought-induced alterations in sorghum performance, nutrient acquisition, and grain fortification. Sci. Total. Environ. 688, 926–934. doi: 10.1016/j.scitotenv.2019.06.392
Dinga, E., Mthiyane, M. N., Marume, U., Botha, T. L., Horn, S., Pieters, R., et al. (2022). Biosynthesis of ZnO nanoparticles using melia azedarach seed extract: Evaluation of the cytotoxic and antimicrobial potency. OpenNano 8, 100068. doi: 10.1016/j.onano.2022.100068
Disante, K. B., Fuentes, D., Cortina, J. (2010). Sensitivity to zinc of Mediterranean woody species important for restoration. Sci. Total. Environ. 408, 2216–2225. doi: 10.1016/j.scitotenv.2009.12.045
Dorosti, N., Jamshidi, F. (2016). Plant-mediated gold nanoparticles by dracocephalum kotschyi as anticholinesterase agent: synthesis, characterization, and evaluation of anticancer and antibacterial activity. J. Appl. Biomed. 14 (3), 235–245. doi: 10.1016/j.jab.2016.03.001
Fageria, N. K., Moreira, A., Coelho, A. M. (2012). Nutrient uptake in dry bean genotypes. Commun. Soil Sci. Plant Anal. 43, 2289–2302. doi: 10.1080/00103624.2012.701689
Faizan, M., Bhat, J. A., Chen, C., Alyemeni, M. N., Wijaya, L., Ahmad, P., et al. (2021). Zinc oxide nanoparticles (ZnO-NPs) induce salt tolerance by improving the antioxidant system and photosynthetic machinery in tomato. Plant Physiol. Biochem. 161, 122–130. doi: 10.1016/j.plaphy.2021.02.002
Fan, X., Zhou, X., Chen, H., Tang, M., Xie, X. (2021). Cross-talks between macro-and micronutrient uptake and signaling in plants. Front. Plant 12. doi: 10.3389/fpls.2021.663477
Farooq, M., Romdhane, L., Al Sulti, M. K. R. A., Rehman, A., Al-Busaidi, W. M., Lee, D. J. (2020). Morphological, physiological and biochemical aspects of osmopriming-induced drought tolerance in lentil. J. Agron. Crop Sci. 206, 176–186. doi: 10.1111/jac.12384
Fattahi, M., Nazeri, V., Torras-Claveria, L., Sefidkon, F., Cusido, R. M., Zamani, Z., et al. (2013). Identification and quantification of leaf surface flavonoids in wild-growing populations of Dracocephalum kotschyi by LC-DAD-ESI-MS. Food Chem. 141, 139–146. doi: 10.1016/j.foodchem.2013.03.019
Ghaffari, H., Tadayon, M. R., Nadeem, M., Cheema, M., Razmjoo, J. (2019). Proline-mediated changes in antioxidant enzymatic activities and the physiology of sugar beet under drought stress. Acta Physiol. Plant 41, 23. doi: 10.1007/s11738-019-2815-z
Giannopolitis, C. N., Ries, S. K. (1977). Superoxide dismutases: II. purification and quantitative relationship with water-soluble protein in seedlings. Plant Physiol. 59, 315–318. doi: 10.1104/pp.59.2.315
Guo, X., Chen, R., Thomas, D. S. G., Li, Q., Xia, Z., Pan, Z. (2021). Divergent processes and trends of desertification in inner Mongolia and Mongolia. Land Degrad. Dev. 32, 3684–3697. doi: 10.1002/ldr.3825
Haque, A. K., Yamaoka, N., Nishiguchi, M. (2007). Cytosine methylation is associated with RNA silencing in silenced plants but not with systemic and transitive RNA silencing through grafting. Gene 396, 321–331. doi: 10.1016/j.gene.2007.04.003
Hasegawa, P. M., Bressan, R. A., Zhu, J. K., Bohnert, H. J. (2000). Plant cellular and molecular responses to high salinity. Annu. Rev. Plant Biol. 51, 463–499. doi: 10.1146/annurev.arplant.51.1.463
Hemeda, H. M., Klein, B. P. (1990). Effects of naturally occurring antioxidants on peroxidase activity of vegetable extracts. J. Food Sci. 55, 184–185. doi: 10.1111/j.1365-2621.1990.tb06048.x
Hong, H., Liu, Z., Li, S., Wua, D., Jiang, L., Li, P., et al. (2022). Zinc oxide nanoparticles (ZnO-NPs) exhibit immune toxicity to crucian carp (Carassius carassius) by neutrophil extracellular traps (NETs) release and oxidative stress. Fish Shellfish Immunol. 129, 22–29. doi: 10.1016/j.fsi.2022.07.025
Hong, J., Wang, C., Wagner, D. C., Gardea-Torresdey, J. L., He, F., Rico, C. M. (2021). Foliar application of nanoparticles: mechanisms of absorption, transfer, and multiple impacts. J. Rico Environ. Sci. Nano. 8, 1196–1210. doi: 10.1039/D0EN01129K
Hu, C., Liu, X., Li, X., Zhao, Y. (2014). Evaluation of growth and biochemical indicators of Salvinia natans exposed to zinc oxide nanoparticles and zinc accumulation in plants. Environ. Sci. pollut. Res. 21, 732–739. doi: 10.1007/s11356-013-1970-9
Islam, M., Kim, J., Begum, M., Abu, T., Sohel, M. A., Lim, Y. S. (2020). Physiological and biochemical changes in sugar beet seedlings to confer stress adaptability under drought condition. Plants 9, 1511. doi: 10.3390/plants9111511
Izsáki, Z. (2014). Effects of phosphorus supplies on the nutritional status of maize (Zea mays l.). Commun. Soil. Sci. Plant Anal. 45, 516–529. doi: 10.1080/00103624.2013.863910
Jordan, J., Singh, K., Cañas-Carrell, J. (2018). Carbon-based nanomaterials elicit changes in physiology, gene expression, and epigenetics in exposed plants: A review. Curr. Opin. Environ. Sci. Health 6, 29–35. doi: 10.1016/j.coesh.2018.07.007
Kasim, W. A. (2007). Physiological consequences of structural and ultra-structural changes induced by zn stress in Phaseolus vulgaris. i. growth and photosynthetic apparatus. Int. J. Bot. 3, 15–22. doi: 10.3923/ijb.2007.15.22
Kheirizadeh Arough, Y., Seyed Sharifi, R., Seyed Sharifi, R. (2016). Bio fertilizers and zinc effects on some physiological parameters of triticale under water-limitation condition. J. Plant Interact. 11, 167–177. doi: 10.1080/17429145.2016.1262914
Kocal, N., Sonnewald, U., Sonnewald, S. (2008). Cell wall-bound invertase limits sucrose export and is involved in symptom development and inhibition of photosynthesis during compatible interaction between tomato and xanthomonas campestris pv vesicatoria. Plant Physiol. 148, 1523–1536. doi: 10.1104/pp.108.127977
Kochert, G. (1978). “Carbohydrate determination by the phenol-sulfuric acid method,” in Handbook of physiological methods. handb phycol methods, phycol biochem methods. Eds. Helebust, J. A., Craig, J. S. (Cambridge: Cambridge University Press), 95–97.
Lee, S., Kim, S., Kim, S., Lee, I. (2013). Assessment of phytotoxicity of ZnO NPs on a medicinal plant, Fagopyrum esculentum. environ. Sci. pollut. Res. 20, 848–854. doi: 10.1007/s11356-012-1069-8
Lichtenthaler, H. K. (1987). Chlorophyll fluorescence signatures of leaves during the autumnal chlorophyll breakdown. J. Plant Physiol. 131, 101–110. doi: 10.1016/S0176-1617(87)80271-7
Lin, D., Xing, B. (2007). Phytotoxicity of nanoparticles: inhibition of seed germination and root growth. Environ. pollut. 150, 243–250. doi: 10.1016/j.envpol.2007.01.016
Liu, Z., Liu, T., Liang, L., Li, Z., Hassan, M. J., Peng, Y., et al. (2020). Enhanced photosynthesis, carbohydrates, and energy metabolism associated with chitosan-induced drought tolerance in creeping bentgrass. Crop Sci. 60, 1064–1076. doi: 10.1002/csc2.20026
Luo, X., Bai, X., Zhu, D., Li, Y., Ji, W., Cai, H., et al. (2012). GsZFP1, a new Cys2/His2-type zinc-finger protein, is a positive regulator of plant tolerance to cold and drought stress. Planta 235, 1141–1155. doi: 10.1007/s00425-011-1563-0
Mahdieh, M., Sangi, M. R., Bamdad, F., Ghanem, A. (2018). Effect of seed and foliar application of nano-zinc oxide, zinc chelate, and zinc sulphate rates on yield and growth of pinto bean (Phaseolus vulgaris) cultivars. J. Plant Nutr. 41, 2401–2412. doi: 10.1080/01904167.2018.1510517
Manjunath, S. B., Biradar, D. P., Aladakatti, Y. R. (2016). Nanotechnology and its applications in agriculture: A review. J. Farm. Sci. 29 (1), 1–13. doi: 10.18782/2582-2845.8405
Marschner, P. (2012). Marschner's mineral nutrition of higher plants (Amsterdam, Netherlands: Elsevier/Academic Press), 684. doi: 10.1016/C2009-0-63043-9
Moradbeygi, H., Jamei., R., Heidari, R., Darvishzadeh, R. (2020). Investigating the enzymatic and non-enzymatic antioxidant defense by applying iron oxide nanoparticles in Dracocephalum moldavica l. plant under salinity stress. Sci. Hortic. 272. doi: 10.1016/j.scienta.2020.109537
Motyka, O., Strbova, K., Olšovská, E., Seidlerová, J. (2019). Influence of nano-ZnO exposure to plants on l-ascorbic acid levels: Indication of nanoparticle-induced oxidative stress. J. Nanosci. Nanotechnol. 19, 3019–3023. doi: 10.1166/jnn.2019.15862
Movahhedi, M. D., Misagh, M., Yadavi., A., Merajipoor., M. (2017). Physiological responses of sesame (Sesamum indicum l.) to foliar application of boron and zinc under drought stress conditions. J. Plant Proc. Func. 6, 27–36.
Nagara, V., Singh, T., Tiwari, Y., Aseri, V., Pandit, P. P., Chopade, R. L., et al. (2022). ZnO nanoparticles: Exposure, toxicity mechanism and assessment. Mater. Today: Proc. Press 69, 56–63. doi: 10.1016/j.matpr.2022.09.001
Ng, C. T., Yong, L. Q., Hande, M. P., Ong, C. N., Yu, L. E., Bay, B. H., et al. (2017). Zinc oxide nanoparticles exhibit cytotoxicity and genotoxicity through oxidative stress responses in human lung fibroblasts and drosophila melanogaster. Int. J. Nanomed. 12, 1621–1637. doi: 10.2147/IJN.S124403.eCollection2017
Noohpisheh, Z., Amiri, H., Mohammadi, A., Farhadi, S. (2021). Effect of the foliar application of zinc oxide nanoparticles on some biochemical and physiological parameters of Trigonella foenum-graecum under salinity stress. Plant Biosyst. 155, 267–280. doi: 10.1080/11263504.2020.1739160
Pathan, A. K., Bond, J., Gaskin, R. E. (2010). Sample preparation for SEM of plant surfaces. Mater 12, 32–43. doi: 10.1016/S1369-7021(10)70143-7
Patra, P., Choudhury, S. R., Mandal, S., Basu, A., Goswami, A., Gogoi, R., et al. (2013). “Effect sulfur and ZnO nanoparticles on stress physiology and plant (Vigna radiata) nutrition,” in Advanced nanomaterials and nanotechnology (Springer), 301–309. doi: 10.1007/978-3-642-34216-5_31
Pfister, B., Zeeman, S. C. (2016). Formation of starch in plant cells. Cell. Mol. Life. Sci. 73 (14), 2781–2807. doi: 10.1007/s00018-016-2250-x
Priyanka, N., Venkatachalam, P. (2016). Biofabricated zinc oxide nanoparticles coated with phycomolecules as novel micronutrient catalysts for stimulating plant growth of cotton. Adv. Natural sci.: J. Nanosci. Nanotechnol. 7, 45018. doi: 10.1088/2043-6262/7/4/045018
Rajemahadik, V. A., Chavan, S. A., More, V. G., Chavan, V. G., Chavan, A. P., Shetye, V. N. (2018). Nanotechnology: Innovative approach in crop nutrition management. Int. J. Agric. Sci. 10 (8), 5826–5582.
Raymond, J., Rakariyatham, N., Azanza, J. L. (1993). Purification and some properties of polyphenoloxidase from sunflower seeds. Phytochemistry 34, 927–931. doi: 10.1016/S0031-9422(00)90689-7
Rechinger, H. (1986). Lora iranica, labiatae Vol. 150 (Graz, Austria: Akademische Druck- und Verlagsanstalt).
Rietra, R. P. J. J., Heinen, M., Dimkpa, C. O., Bindraban, P. S. (2017). Effects of nutrient antagonism and synergism on yield and fertilizer use efficiency. Commun. Soil Sci. Plant Anal. 48, 1895–1920. doi: 10.1080/00103624.2017.1407429
Ritchie, S. W., Nguyen, H. T., Holaday, A. S. (1990). Leaf water content and gas-exchange parameters of two wheat genotypes differing in drought resistance. Crop Sci. 30, 105–111. doi: 10.2135/cropsci1990.0011183X003000010025x
Rizwan, M., Ali, S., Rehman, M. Z., Adrees, M., Arshad, M., Qayyum, M. F., et al. (2019). Alleviation of cadmium accumulation in maize (Zea mays l.) by foliar spray of zinc oxide nanoparticles and biochar to contaminated soil. Environ. pollut. 248, 358–367. doi: 10.1016/j.envpol.2019.02.031
Rolland, F., Baena-Gonzalez, E., Sheen, J. (2006). Sugar sensing and signaling in plants: conserved and novel mechanisms. Annu. Rev. Plant Biol. 57, 675–709. doi: 10.1146/annurev.arplant.57.032905.105441
Rossi, L., Fedenia, L., Sharifan, H., Ma, X., Lombardini, L. (2018). Effects of foliar application of zinc sulfate and zinc nanoparticles in coffee (Coffea arabica l.) plants. Plant Physiol. Biochem. 135, 160–166. doi: 10.1016/j.plaphy.2018.12.005
Saeidnia, S., Gohari, A., Hadjiakhoondi, A. (2007). Bioactive compounds of the volatile oil of Dracocephalum kotschyi. Z. Naturforsch. C. J. Biosci. 62, 793–796. doi: 10.1515/znc-2007-11-1203
Saeidnia, S., Gohari, A., Uchiyama, N., Ito, M., Honda, G., Kiuchi, F. (2004). Two new monoterpene glycosides and trypanocidal terpenoids from Dracocephalum kotschyi. Chem. Pharm. Bull. 52, 1249–1250. doi: 10.1248/cpb.52.1249
Sandhya, V., Ali, S. Z., Grover, M., Reddy, G., Venkateswarlu, B. (2010). Effect of plant growth promoting pseudomonas spp. on compatible solutes, antioxidant status and plant growth of maize under drought stress. Plant Growth Regul. 62, 21–30. doi: 10.1007/s10725-010-9479-4
Sattar, A., Wang, X., Ul-Allah, S., Sher, A., Ijaz, M., Irfan, M., et al. (2022). Foliar application of zinc improves morpho-physiological and antioxidant defense mechanisms, and agronomic grain biofortification of wheat (Triticum aestivum l.) under water stress. Saudi Sci. J. Biol. Sci. 29, 1699–1706. doi: 10.1016/j.sjbs.2021.10.061
Semida, W. M., Abdelkhalik, A., Mohamed, G., El-Mageed, A., Taia, A., El-Mageed, A., et al. (2021). Foliar application of zinc oxide nanoparticles promotes drought stress tolerance in eggplant (Solanum melongena l.). Plants 10, 421. doi: 10.3390/plants10020421
Sharafi, A., Sohi, H. H., Azadi, P., Sharafi, A. A. (2014). Hairy root induction and plant regeneration of medicinal plant Dracocephalum kotschyi. Physiol. Mol. Biol. Plants 20, 257–262. doi: 10.1007/s12298-013-0217-z
Sharma, P., Jha, A., Dubey, R., Pessarakli, M. (2012). Reactive oxygen species, oxidative damage, and antioxidative defense mechanism in plants under stressful conditions. J. Bot. 2012. doi: 10.1155/2012/217037
Sharma, V., Shukla, R. K., Saxena, N., Parmar, D., Das, M., Dhawan, A. (2009). DNA Damaging potential of zinc oxide nanoparticles in human epidermal cells. Toxicol. Lett. 185, 211–218. doi: 10.1016/j.toxlet.2009.01.008
Silva, V., Singh, R. K., Gomes, N., Soares, B. G., Silva, A., Falco, V., et al. (2020). Comparative insight upon chitosan solution and chitosan nanoparticles application on the phenolic content, antioxidant and antimicrobial activities of individual grape components of sousão variety. Antioxidants 9, 178. doi: 10.3390/antiox9020178
Singh, A., Singh, N. B., Afzal, S., Singh, T., Hussain, I. (2018). Zinc oxide nanoparticles: a review of their biological synthesis, antimicrobial activity, uptake, translocation and biotransformation in plants. J. Mater. Sci. 53, 185–201. doi: 10.1007/s10853-017-1544-1
Solanki, P., Bhargava, A., Chhipa, H., Jain, N., Panwar, J. (2015). “Nano-fertilizers and their smart delivery system,” in Nanotechnologies in food and agriculture (Springer Cham), 81–101. doi: 10.1007/978-3-319-14024-7_4
Subbaiah, L. V., Prasad, T. N., Krishna, T. G., et al. (2016). Novel effects of nanoparticulate delivery of zinc on growth, productivity, and zinc biofortification in maize (Zea mays l.). J. Agric. Food. Chem. 64 (19), 3778–3788. doi: 10.1021/acs.jafc.6b00838
Sun, L., Song, F., Zhu, X., Liu, S., Liu, F., Wang, Y., et al. (2021). Nano-ZnO alleviates drought stress via modulating the plant water use and carbohydrate metabolism in maize. Arch. Agron. Soil Sci. 67, 245–259. doi: 10.1080/03650340.2020.1723003
Taghizadeh, M., Nasibi, F., Kalantari, K. M., Benakashani, F. (2020). Callogenesis optimization and cell suspension culture establishment of Dracocephalum polychaetum bornm. and Dracocephalum kotschyi boiss.: An in vitro approach for secondary metabolite production. S Afr. J. Bot. 132, 79–86. doi: 10.1016/j.sajb.2020.04.015
Taran, N., Storozhenko, V., Svietlova, N., Batsmanova, L., Shvartau, V., Kovalenko, M. (2017). Effect of zinc and copper nanoparticles on drought resistance of wheat seedlings. Nanoscale Res. Lett. 12, 1–6. doi: 10.1186/s11671-017-1839-9
Tariverdizadeh, N., Mohebodini, M., Chamani, E., Ebadi, A. (2021). Iron and zinc oxide nanoparticles: An efficient elicitor to enhance trigonelline alkaloid production in hairy roots of fenugreek. Ind. Crops Prod. 162, 113240. doi: 10.1016/j.indcrop.2021.113240
Tewari, R. K., Kumar, P., Sharma, P. N. (2019). An effective antioxidant defense provides protection against zinc deficiency-induced oxidative stress in zn-efficient maize plants. J. Plant Nutr. Soil Sci. 182, 701–707. doi: 10.1002/jpln.201800622
Tripathi, D. K., Singh, S., Gaur, S., Singh, S., Yadav, V., Liu, S., et al. (2018). Acquisition and homeostasis of iron in higher plants and their probable role in abiotic stress tolerance. Front. Environ. Sci. 5. doi: 10.3389/fenvs.2017.00086
Upadhyaya, H., Roy, H., Shome, S., Tewari, S., Bhattacharya, M. K., Panda, S. K. (2017). Physiological impact of zinc nanoparticle on germination of rice (Oryza sativa l) seed. J. Plant Sci. Phytopathol. 1, 062–070. doi: 10.29328/journal.jpsp.1001008
Van Nguyen, D., Nguyen, H. M., Le, N. T., Nguyen, K. H., Nguyen, H. T., Le, H. M., et al. (2022). Copper nanoparticle application enhances plant growth and grain yield in maize under drought stress conditions. J. Plant Growth Regul. 41, 364–375. doi: 10.1007/s00344-021-10301-w
Verma, K. K., Song, X. P., Zeng, Y., Li, D. M., Guo, D. J., Rajput, V. D., et al. (2020). Characteristics of leaf stomata and their relationship with photosynthesis in Saccharum officinarum under drought and silicon application. ACS omega 5, 24145–24153. doi: 10.1021/acsomega.0c03820
Waraich, E. A., Ahmad, R., Ashraf, M. Y. (2011). Role of mineral nutrition in alleviation of drought stress in plants. Aust. J. Crop Sci. 5, 764–777.
Yang, F., Miao, L. F. (2010). Adaptive responses to progressive drought stress in two poplar species originating from different altitudes. Silva Fennica 44, 23–37. doi: 10.14214/sf.160
Zengin, F. K. (2006). The effects of Co2+ and Zn2+ on the contents of protein, abscisic acid, proline and chlorophyll in bean (Phaseolus vulgaris cv. strike) seedlings. J. Environ. Biol. 27, 441–448.
Zhang, Z., Szota, C., Fletcher, T. D., Williams, N. S. G., Werdin, J., Farrell, C. (2018). Influence of plant composition and water use strategies on green roof stormwater retention. Sci. Total Environ. 625, 775–781. doi: 10.1016/j.scitotenv.2017.12.231
Keywords: antioxidant enzyme activity, drought stress, nanoparticles, zinc fertilizers, elemental composition
Citation: Karimian Z and Samiei L (2023) ZnO nanoparticles efficiently enhance drought tolerance in Dracocephalum kotschyi through altering physiological, biochemical and elemental contents. Front. Plant Sci. 14:1063618. doi: 10.3389/fpls.2023.1063618
Received: 07 October 2022; Accepted: 14 February 2023;
Published: 10 March 2023.
Edited by:
Diaa Abd El Moneim, Arish University, EgyptReviewed by:
Shixian Sun, Southwest Forestry University, ChinaCopyright © 2023 Karimian and Samiei. This is an open-access article distributed under the terms of the Creative Commons Attribution License (CC BY). The use, distribution or reproduction in other forums is permitted, provided the original author(s) and the copyright owner(s) are credited and that the original publication in this journal is cited, in accordance with accepted academic practice. No use, distribution or reproduction is permitted which does not comply with these terms.
*Correspondence: Zahra Karimian, emthcmltaWFuQHVtLmFjLmly
Disclaimer: All claims expressed in this article are solely those of the authors and do not necessarily represent those of their affiliated organizations, or those of the publisher, the editors and the reviewers. Any product that may be evaluated in this article or claim that may be made by its manufacturer is not guaranteed or endorsed by the publisher.
Research integrity at Frontiers
Learn more about the work of our research integrity team to safeguard the quality of each article we publish.