- 1Department of Chemical Engineering, Nadirshaw Eduljee Dinshaw (NED) University of Engineering and Technology, Karachi, Pakistan
- 2Department of Polymer and Petrochemical Engineering, NED University of Engineering and Technology, Karachi, Pakistan
- 3Department of Horticultural Sciences, Faculty of Agriculture and Environment, The Islamia University of Bahawalpur, Bahawalpur, Pakistan
- 4Dr. Muhammad Ajmal Khan Institute of Sustainable Halophyte Utilization, University of Karachi, Karachi, Pakistan
- 5Department of Environmental Sciences, Karakorum International University, Gilgit, Pakistan
- 6Department of Botany, University of Karachi, Karachi, Pakistan
- 7The UWA Institute of Agriculture, The University of Western Australia, Perth, WA, Australia
The ever-increasing demand for agricultural food products, medicine, and other commercial sectors requires new technologies for agricultural practices and promoting the optimum utilization of natural resources. The application of engineered nanomaterials (ENMs) enhance the biomass production and yield of food crop while resisting harmful environmental stresses. Bio-mediated synthesis of ENMs are time-efficient, low-cost, environmentally friendly, green technology. The precedence of using a bio-mediated route over conventional precursors for ENM synthesis is non-toxic and readily available. It possesses many active agents that can facilitate the reduction and stabilization processes during nanoparticle formation. This review presents recent developments in bio-mediated ENMs and green synthesis techniques using plants, algae, fungi, and bacteria, including significant contributions to identifying major ENM applications in agriculture with potential impacts on sustainability, such as the role of different ENMs in agriculture and their impact on different plant species. The review also covers the advantages and disadvantages of different ENMs and potential future research in this field.
Introduction
Growing food scarcity, declining land and water resources, and insufficient investment in sustainable agriculture are major factors limiting agricultural production. Climate change also adversely affects crop yields and is responsible for the deterioration of cultivable land and freshwater resources (Zulfiqar and Hancock, 2020; Hasnain et al., 2022). Therefore, the agriculture sector must focus on satisfying the tradeoff between the increasing demand for food crops and enhanced resource constraints by efficiently using land and water resources. Measures must be taken to prevent or minimize the adverse effects of climate change by adopting modern techniques such as using advanced materials in agricultural practices and promoting the smart utilization of natural resources. Numerous projects and investments are needed to fulfill the growing demand for agricultural products while adopting sustainable approaches (FAO, 2017).
Nanotechnology has many applications in daily life. The food and agriculture industry started adopting nanotechnology in different forms in 2003 (Xing et al., 2021). Research in this field has increased markedly in the last decade (Zulfiqar et al., 2019; Zulfiqar and Ashraf, 2021). Nanotechnology has various advantages, such as improved food quality and safety (Nandini et al., 2017). Nanotechnology enables scientists to engineer particles with unique, adaptive characteristics for various applications ranging from pharmaceuticals to electronics, fuel cells, water and gas treatments, and agriculture (Hochella Michael et al., 2019). Engineered nanomaterials (ENMs) are up to 100 nm in size and designed to have engineered physical and chemical properties with unique properties for various applications, such as sensors, thermoelectric materials, photocatalysts, dye-sensitized solar cells, nutraceuticals, drug delivery, biocidal agents, and nano-fertilizers (Bandala and Berli, 2019).
Some common types of ENMs are metallic oxides (e.g., Au, Ag, Fe3O4, TiO2, and ZnO), polymers, carbon ENMs (CENMs; e.g., fullerenes, carbon nanotubes, and graphene), hybrid particles, and polymer nanocomposites (Dontsova et al., 2019). Particle size and shape are the most important characteristics of ENMs, influencing their reactivity and transport mechanism and regulating the type and distribution of reactive sites of chemical reactions. Due to the high surface area to volume ratio, changes in surface structure impact the extent of nanomaterial reactivity in air, water, soil, or biota (Coman et al., 2019). Environmental factors of ENMs include, dissolution, evaporation, and aggregation (Hochella Michael et al., 2019).
In the last decade, researchers have been exploring advanced technologies, including nanotechnology, to fulfill population demands and address the global challenges in agriculture (FAO, 2017). Nanotechnology could be used to develop fertilizers and pesticides for crops (Ammar, 2018). Encapsulation of ENMs with other materials enhances plant nutrient uptake, consequently decreasing agrochemical waste. For example, nano-encapsulated materials have enhanced stability and solubility; thus, they can be used for efficient and controlled nutrient delivery (Shang et al., 2019). Nanocapsules act as a barrier preventing encapsulated ingredients from leaching into the land. when combined with other biological materials, may enhance plants’ tolerance to abiotic stresses like extreme temperatures and water deficiency Shang et al. (2019) which will be discussed later in detail. There is a clear need to develop ENMs and capitalize on their potential for increasing plant growth, resisting biocides, and minimizing environmental stresses such as flooding, salt, high temperatures, toxic metals, and drought that continue to increase with the increasing food demands and anthropogenic activities (Ali et al., 2017).
Global crop production is becoming insufficient due to lack of adequate nutrients and limitations of traditional agricultural methodologies that can easily be overcome by applying modern cultivation methods and using ENMs (Hao et al., 2018). ENMs are used extensively in modern plant technology and could play a major role in advanced biotechnological applications. Studies have shown that nanomaterials can support the transport of organic molecules into plant cells for nutrient uptake and disease prevention. Reactions such as particle dissolution, ion adsorption, cell interactions, uptake by microorganisms, and many other complex reactions affect the interaction of ENMs with organisms in various environmental media (Schwabe et al., 2014). Nano-agriculture is a branch of nanotechnology that deals with producing ENMs for application in pesticides, agrochemicals, water, soil improvers, and nano fertilizers (Shang et al., 2019). This review elaborates on and highlights the synthesis mechanisms of ENMs to understand the role of ENMs in agricultural systems. Ultimately, this review will help identify the major applications of ENMs in agriculture, potential impacts on sustainability, and the bio-mediated routes and materials for ENM synthesis. This review also highlights the application methodology and limitations of ENMs in the agricultural sector, knowledge gaps, and future trends in ENM development.
Synthesis of engineered nanomaterials
Bio-mediated ENM synthesis is a time-efficient, low-cost, environmentally friendly, green technology. The green synthesis produces more stable ENMs than other means, and it is simple to scale up, and the risk of contamination is also lower. Plant leaves, as the site for photosynthesis, are food factories and can be used to synthesize NP, along with other plant parts, such as stems, seeds, bark, flowers, and roots (Kalpana and Devi Rajeswari, 2018). The precedence of using plant extract over conventional reducing and stabilizing agents such as sodium borohydride, sodium citrate, polyethylene glycol (PEG), polyvinylpyrrolidone (PVP), polyvinyl alcohol (PVA), Bovine serum albumin (BSA), Ethylene diamine tetra acetic acid (EDTA), Chitosan etc. for ENM synthesis is that plants are non-toxic, readily available, and possess many active agents that can facilitate the reduction and stabilization process during NP formation (Shafey, 2020). Most plant extracts contain organic micro- and macromolecules (e.g., hydroquinone, chlorophyll, estragole, allylbenzene, methylxanthine, dimethylxanthine, ascorbic acid, phenols, isoprenoid, poly-carbohydrates, flavonoids, alkaloids, proteins and other enzymes, amino acids, and alcohols), and act as reducing agents and capping ligands. This specific properties of organic micro- and macromolecules provide colloidal stability, prevent agglomeration/coagulation, and stop uncontrolled growth (Saglam et al., 2021). Other bio-mediated routes for ENM synthesis include microorganisms such as algae, yeasts, fungi, and bacteria that provide intra- or extracellular active sites for NP growth. These microreactors are more convenient for synthesizing ENMs in an economical and environmentally friendly manner than the other physical and chemical synthesis (Xu et al., 2021). Figure 1 shows the bio-mediated synthesis routes which will be discussed in this section, including the effect these different synthesis routes will have on the characteristics and applicability of ENMs. Figure 2 summarizes the general procedure of ENM synthesis of ENMs using green routes.
Synthesis of engineered nanomaterials from plants
Plant-mediated engineered nanomaterials (ENMs) synthesis is, low-cost, low-temperature, rapid, green technology using safe solvents (Chung et al., 2016; Munir et al., 2021). Palithya et al. (2021) used leaves of Decaschistia crotonifolia to synthesize silver (Ag) ENMs; combining the first leaf extract with silver nitrate (AgNO3) in a 1:2 ratio changed the solution color from yellowish light green to dark brown. The antimicrobial activity of the synthesized ENMs was analyzed against gram-positive Staphylococcus aureus, Bacillus subtilis, Klebsiella pneumoniae and Escherichia coli. The biosynthesized ENMs exhibited better antibacterial activity against all bacterial species; while moderate efficacy compared to the standard antibiotic Amoxyclavhr. These ENMs had optimum catalytic activity to enhance the dye degradation rate by rapid reduction of nitrophenol to aminophenol. Hence, these ENMs could be used in various pharmaceutical and industrial applications. Hemlata et al. (2020) used leaf extract of Cucumis prophetarum to synthesize silver (Ag) ENMs. The plant extract was mixed in a 1:9 ratio with 0.001 M AgNO3 solution. The mixture was heated at 80°C. The precipitates were then centrifuged and dried to obtain brown-colored silver (Ag) ENMs. The antimicrobial activity of the green-synthesized Ag ENMs and aqueous leaf extract of C. prophetarum was analyzed against Gram-positive Staphylococcus aureus and Gram-negative S. typhi; the ENMs showed greater antibacterial activity against Gram-negative than Gram-positive bacteria. The antiproliferative activity of the green-synthesized Ag-ENMs against cancer cell lines was also tested, with cell viability gradually decreasing with increasing concentration of Ag-ENMs. Figure 3 shows ENM synthesis using Salvia spinosa.
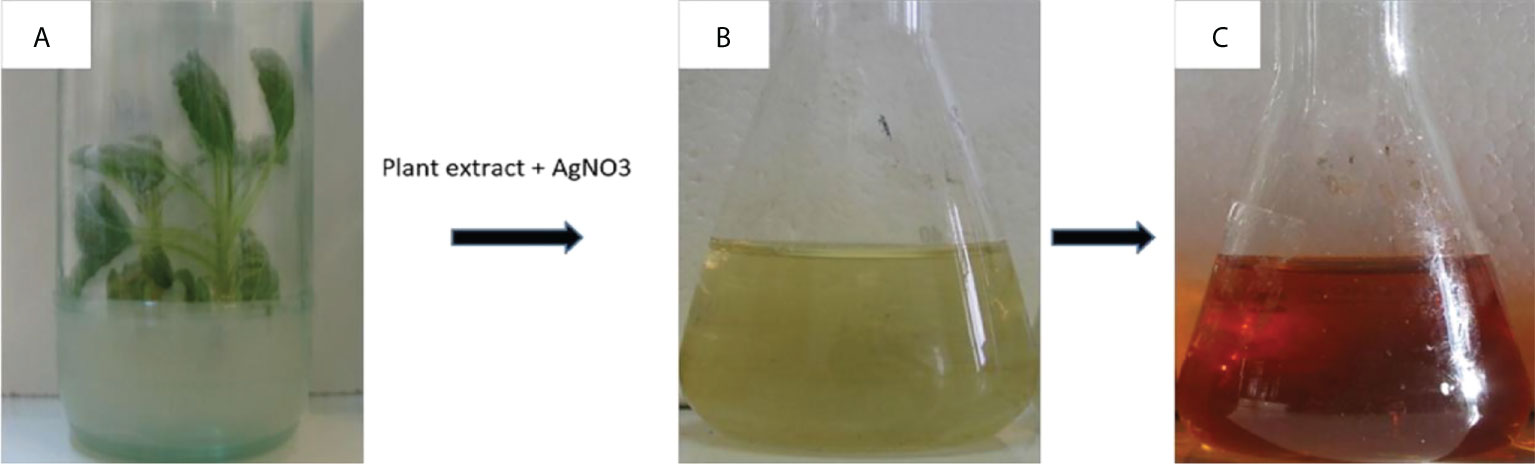
Figure 3 Color changes in the plant extract of Salvia spinosa after adding AgNO3 solution (Pirtarighat et al., 2019). (A) Plant leaves soaked in water. (B) Plant extract without AgNO3. (C) Plant extract with the addition of AgNO3. Copyright 2018.
Khaldari et al. (2021) synthesized copper (II) oxide (CuO) ENMs using lavender and green tea leaves. Copper sulfate (CuSO4.5H2O) and lavender (or green tea) powder were mixed in varying proportions, with the resulting mixture ground into a homogeneous powder, heated at 600°C for 4 hours, and then cooled to room temperature before suspending the residue in ethanol and centrifuging to obtain the required ENMs. Figure 4 shows scanning electron microscopy (SEM) images of the nanomaterials. The X-ray diffraction (XRD) of green synthesized CuOENMs revealed that increasing the plant powder increased the purity of ENMs, and lavender powder produced more pure CuO ENMs than green tea leaf. Compared with chemically synthesized CuO ENMs, the biosynthesized ENMs had a less inhibitory effect on seed germination. Also, green and chemically synthesized CuO ENMs at low concentrations (i.e., 4 μg mL–1) increased root and shoot lengths of lettuce and tomato seedlings (Khaldari et al., 2021).
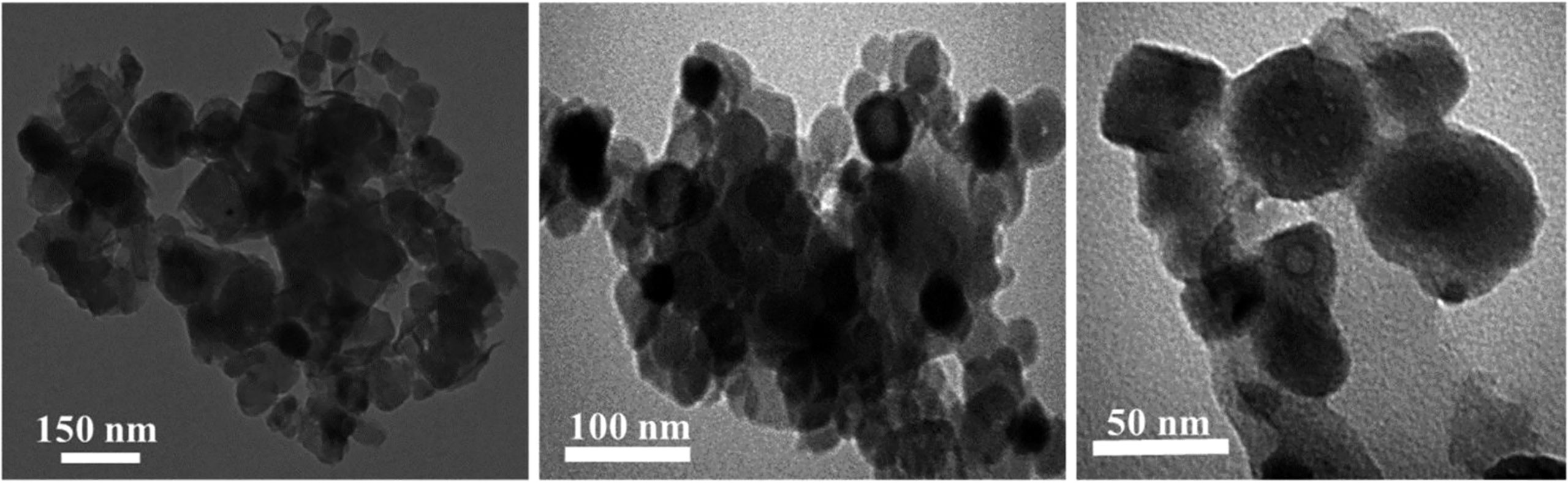
Figure 4 TEM images of copper oxide ENMs synthesized by lavender and green tea leaf powders using the solid-state method (Khaldari et al., 2021). Copyright 2021.
Abutilon indicum leaf extract was used to synthesize CuO ENMs using the green combustion method (Ijaz et al., 2017). A homogeneous solution of copper (II) nitrate trihydrate and 0.3 g Abutilon indicum was prepared in 20 mL distilled water, heated at 400°C in a muffle furnace to form CuO ENMs, which were filtered and washed to remove impurities. The XRD results of the synthesized CuO ENMs revealed a highly crystalline structure due to the leaf extract, which controls particle size. Furthermore, the antimicrobial activity of the CuO the green ENMs was analyzed against S. aureus, Klebsiella, and B. subtilis by measuring the radius of inhibition zones, it was observed that the highest inhibition was observed for B. subtilis with 15 mm inhibition zone. When compared with the standard drug ampicillin, the CuO ENMs exhibited strong antimicrobial activity with a minimum inhibitory concentration (MIC) of 0.05 mg mL–1, revealing that the CuO ENMs released copper (Cu) ions which penetrated the bacterial cell membrane, damaging its formation by attaching to negatively charged cell walls.
Ahmad et al. (2021) synthesized iron oxide ENMs using E. hirta leaf. The leaf extract was combined with 0.1 M ferrous sulfate heptahydrate and 0.1 M ferric chloride at constant pH 9 using standard sodium hydroxide (NaOH) solution; brown-colored iron-oxide ENMs formed after 3 h, which were centrifuged to remove impurities. The antimicrobial activities of the engineered ENMs were analyzed by quantifying the size inhibition zone on agar plates and determined to be directly proportional to their concentration. Erci and Cakir-Koc (2021) synthesized iron oxide ENMs (FeO) using T. spicata. The solution was formed by mixing T. spicata in 100 mL water, heated at 60°C for 30 min, and filtered. The extract was mixed with 0.1 M ferrous sulfate (FeSO4.7H2O) to synthesize iron oxide nanomaterials. The antimicrobial activity of the FeO ENMs was analyzed for Gram-positive and Gram-negative bacterial species. B. cereus had MIC and minimum bactericidal concentration (MBC) values of 200 mg mL–1, and S. typhimurium had the lowest MIC of 100 mg mL–1. The inhibition percentage was directly proportional to the fraction of leaf extract used to synthesize ENMs. The cytotoxicity of FeO ENMs on L929 cells was less than that for FeSO4.7H2O. Table 1 lists more examples of ENM synthesis using plant extracts
Synthesis of engineered nanomaterials from algae
Algae are readily available and eco-friendly, with rich source of metabolites and optimum metal uptake capacity for use as reducing agents for nanoparticle synthesis (Abideen et al., 2022; Hasnain et al., 2022). In addition, algae accumulate higher lipids and potential source of biodiesel using saline lands and brackish water. Aboelfetoh et al. (2017) synthesized silver ENMs using Caulerpa serrulata from the Red Sea coast, Egypt. First, the algae were powdered after cleaning and natural drying; 1 g algae powder was cooked in 100 mL water for 10 min at 70°C before mixing 5–25 mL C. serrulata extract with 10−3 M AgNO3 and adjusting the total volume to 100 mL. The formation of ENMs was depicted by yellow to brown color depending on the algal extract used, reaction time, temperature, and pH of the solution. The catalytic activity of the Ag-NP was evaluated in a reducing aqueous solution of Congo Red dye in the presence of sodium borohydride (NaBH4) at room temperature. It was observed that without Ag ENMs no dye degradation was observed even after 3 hours, once biosynthesized Ag ENMs have added dye degradation started within 5 minutes. The antibacterial activity of the Ag-NP increased with increasing concentration of Ag-NP. The highest inhibition tendency occurred for E. coli, and minimum inhibition occurred for S. typhi. Dhas et al. (2013) synthesized silver chloride (AgCl) ENMs from Sargassum plagiophyllum. The seaweed was washed to remove impurities and dried in the shade for 8-10 days before grinding to a powder. A seaweed extract was prepared by mixing 5 g seaweed powder with 50 mL water. To synthesize AgCl ENMs, varying amounts of seaweed extracts (50-500 × 10–6 L) were mixed with 5 mL of 0.001 M AgNO3 solution and kept at room temperature for 1 day. The AgCl ENMs were depicted as yellowish-brown, spherical shapes (18-42 nm) in TEM images. The antibacterial activity of the AgCl ENMs was investigated against E. coli. The inhibitory action proportional to the quantity of AgCl ENMs, as depicted by larger inhibition zones was observed. Abboud et al. (2014) used Bifurcaria bifurcata (brown algae) to synthesize copper ENMs. The algae were washed with water (until it neutralized to pH 7), dried for 12 h at 60°C), and ground to a powder. The powder was boiled in water (1:5 ratio) for ~1 h, mixed with 0.001 M copper sulfate solution (1:10 ratio), and heated at about 100°C under continuous stirring to obtain dark brick red colored precipitates. The formation of spherical copper oxide ENMs (5-45 nm) was depicted in TEM images, with a few elongated ENMs (Figure 5). The antimicrobial activity of the copper oxide ENMs was considerable for Gram-negative and Gram-positive bacterial species (E. aerogenes and S. aureus) compared to the algae extract which had no antimicrobial activity.
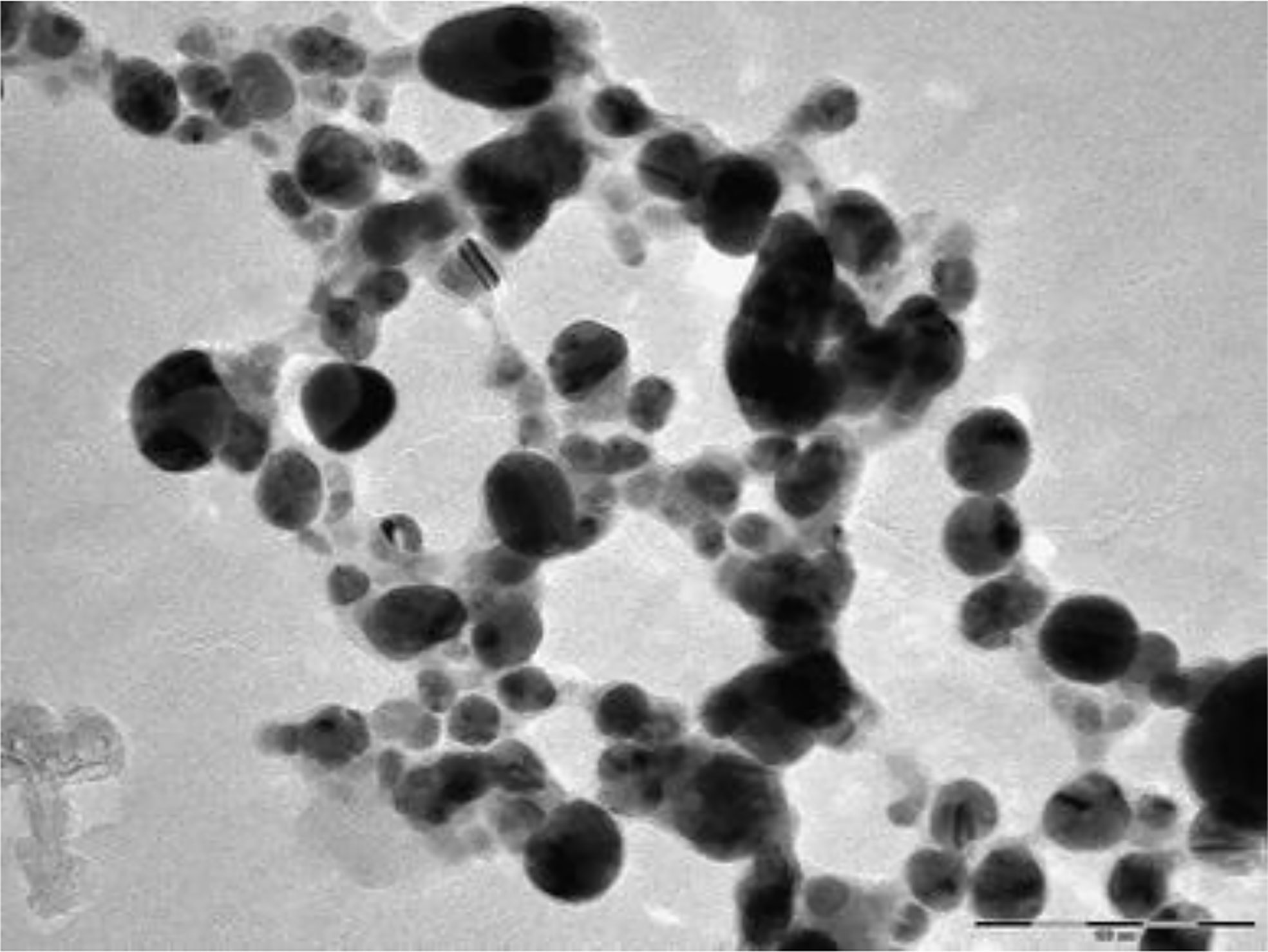
Figure 5 TEM image of copper ENMs (Engineered Nanomaterials) synthesized from algal extract of Bifurcaria bifurcata (Abboud et al., 2014). Copyright 2013.
Eroglu et al. (2013) synthesized spherical palladium nanocrystals from green microalgae (Chlorella vulgaris) using aqueous sodium tetrachloro-palladate Na2 (PdCl4) photosynthetic reactions. The nanocrystals were embedded in electrospun chitosan mats as a catalyst for the Mizoroki–Heck cross-coupling reaction. Cross-linked chitosan mats (3 × 2 cm rectangular shape) developed by electrospinning were dipped into a one-month-old mature microalgal culture in 25 mg L–1 Na2 (PdCl4). The control sample comprised chitosan mats treated with 25 mg L–1 Na2 (PdCl4) solution without microalgae. The efficacy of the biogenic nano palladium-chitosan mats was analyzed as catalyst support in the Mizoroki-Heck reaction. Six dried catalyst mats were placed into a solution containing iodobenzene, butyl acrylate, triethylamine and dimethylformamide (DMF) at 80°C for 16 h; after each reaction, the catalyst was recovered for regenerative studies after washing with DMF under nitrogen gas to prevent oxidation that may lead to the reproduction of Pd (0) ENMs. The catalyst was recyclable for a minimum of four reactions, with reaction yields of 68, 62, 45, and 36% (by weight) for the first to fourth cycles, respectively, much higher than the control at only 5%.
Ishwarya et al. (2018) synthesized zinc oxide (ZnO) ENMs using Ulva lactuca marine seaweeds. The seaweed was washed with water, cut, and dried in the air. A 10% solution of seaweed powder (5 g powder in 50 mL water) was obtained after boiling for 20 min. The extract was filtered and stored for later use when 5 mL extract was mixed with a 95 mL aqueous solution of 1 mM zinc acetate at 70°C with continuous stirring for 3-4 h. After centrifugation at 4,000 rpm for 10 min, yellowish-white precipitates were collected and washed with water before heating at 450°C for 4 h. Agglomerates of sample sponge-like asymmetrical shaped ENMs (10-50 nm) were depicted in TEM images. The inhibition percentage of antibacterial activity of ZnO ENMs against Gram-positive and Gram-negative species was observed between 80 to 95% in dark and visible light; specifically, lactuca-fabricated ZnO ENMs had an 82% reduction rate for B. licheniformis, 84% for B. pumilus, 80% for E. coli, and 79% for P. vulgaris (79%). Furthermore, the highest inhibition percentage of 100% occurred for A. aegypti in 50-μg mL–1 ZnO ENMs at 24 h.
Synthesis of engineered nano materials from fungi
Fungi include many multicellular and unicellular species that used in various processes since early civilization. They play an important role in ecosystems, regulating the never-ending cycle of greenhouse gas emissions. Fungi have positive and negative impacts on our daily lives (Hussin, 2018). They are used in many industrial processes, including manufacturing antibiotics, steroids, and hormones (e.g., penicillin and cephalosporin), fermentation processes, organic acids (e.g., citric acid), and protein and vitamin production. While fungi cause many plant diseases for crops, several species improve soil quality and health, helping plants cope with saline and drought stresses (Ramasamy, 2020). Many fungal species used as protein-rich foods, including some mushrooms, morels, and truffles. Fungi can decompose all materials except plastics. Various fungal species are present in forest soils, withstanding stresses such as changing pH, decomposing cellulose lignin and other organic residues, and importing nutrients through hyphae for plant uptake of nitrogen, phosphorus, micronutrients, and water (Morris, 2004). Due to their rapid growth, simple synthesis, and short life cycle, fungi used in microbiology, biochemistry, and genetic engineering labs to study various metabolisms (e.g., the ‘one gene, one enzyme’ theory in Neurospora for which George W Beadle and Edward L Tatum won the Nobel Prize for Physiology or Medicine in 1958).
Yeasts are single-celled fungi widely used in various fermentation processes. The synthesis of ENMs through yeast simplifies the fermentation process, at ambient temperature; it is also very low cost and environmentally friendly. Moreover, synthesis through yeast has an advantage over other microbial species because yeast is the highest industrially produced microorganism in the world for different fermentation-based products, such as wine and bread. Bao et al. (2010) synthesized cadmium telluride quantum dots using yeast cells grown on a modified Czapek’s medium (450 g sucrose, 15 g sodium nitrate (NaNO3), 5.0 g potassium hydrogen phosphate (K2HPO4), 2.5 g potassium chloride (KCl), 2.5 g magnesium sulfate (MgSO4.7H2O), and 30.0 g sodium citrate tribasic dihydrate dissolved in 5 L deionized water) and incubated for 2 days at 35°C. The yeast culture was combined with 400 mL cadmium chloride (CdCl2) (0.04 mol L–1), and 100 mL sodium tellurite (Na2TeO3) (0.04 mol L–1), 7.5 g MSA, and 5.0 g NaBH4, and incubated in a water bath. The final product comprised well-dispersed crystalline CdTe ENMs in a cubic zinc-blended shape (3.6 nm) after centrifugation dialysis using a dialysis membrane to remove residual chemicals and yeast cells.
Fernández et al. (2016) synthesized Ag ENMs using Cryptococcus laurentii and Rhodotorula glutinis incubated at 28°C and 100 rpm for 24 h in Muller–Hinton broth (MHB), containing 2 g beef infusion, 17.5 g acid casein peptone, and 1.5 g corn starch at neutral pH, with 2.0×106 yeast cells in suspension and centrifuged before further use. The Ag ENMs were synthesized by adding 1 mL of 1 mM aqueous silver nitrate solution in 100 mL of yeast culture and stirring at 100 rpm at 28°C for 48 h in the dark. Particle formation (15-220 nm) was validated by the solution changing from yellow to deep brown using TEM images. Many fungi species rot fruits and vegetables and ultimately reduce their shelf life; therefore, the inhibition ability of biosynthesized Ag ENMs was assessed for B. cinerea, P.expansum, A. niger, Alternaria sp., and Rhizopus sp; the synthesized Ag ENMs from R. glutinis had higher antimicrobial activity than from C. laurentii. At 3 ppm, the ENMs synthesized from R. glutinis had antifungal efficacy comparable to antifungal agent iprodione against all fungi species in question (except Rhizopus). Eugenio et al. (2016) synthesized silver and silver chloride using yeasts produced from the gut of ten termites (Cornitermes cumulans). The gastrointestinal matter was placed on Sabouraud agar plates and incubated at 28°C for 48 h. For the incubation of separate clones, the colonies were re-incubated after dilution. Fifteen of these separate clones were used to synthesize AgCl ENMs; each isolated plate was inoculated in a liquid medium containing 4% glucose, 1% peptone, and 1% yeast extract at pH 6.5 at 30°C for 24 h. The media was mixed with 3.5 M silver nitrate solution and further incubated at 30°C in the dark with constant stirring at 150 rpm for 7 days. The silver chloride ENMs were separated from the mixture by centrifugation to obtain circular ENMs (2–10 nm), as confirmed by TEM images. To analyze the cell inhibition efficacy of biosynthesized silver chloride ENMs against S. aureus and K. pneumoniae, the ENMs were suspended in stabilizing solution comprising 1% sodium citrate with a basic pH 8 for 24 h. A dose-dependent inhibition effect was observed against both bacterial species at a maximum loading of 50μg mL–1 (83% and 85% against S. aureus and K. pneumonia, respectively).
Tian et al. (2010) synthesized mesoporous zirconium phosphate using yeast grown in 100 mL of aqueous glucose solution (2 wt.%) at room temperature for 0.5 h before adding 3.2 g zirconyl chloride (ZrOCl2.8H2O). After 12 h, 100 mL of 0.2 M disodium hydrogen phosphate was mixed into the solution, maintaining the pH at 1-3 using 0.05 M HCl. After 2 h of mixing, a white gelatinous texture formed; after 48 h of storage, the mixture was centrifuged and washed vigorously with water and then ethanol. The product was dried at 80°C for 24 h to obtain a wormhole-like mesoporous structure from agglomerates of zirconium phosphate ENMs, which were analyzed for their efficiency as air electrodes for electro-catalytic activity in the oxygen reduction reaction (ORR) in alkaline solution. Due to its open mesoporous structure that can enhance mass transfer and neutralize polarization, this process had superior results to electrolytic manganese dioxide (EMD) air electrodes. These results showed that these mesoporous particles could be used for fuel cell applications.
Synthesis of engineered nanomaterials from bacteria
Kanmani and Lim (2013) synthesized silver ENMs using a lactic acid bacterium that acts as a reducing and stabilizing agent. First, exopolysaccharide (EPS) was produced using bacterium: Lactobacillus rhamnosus GG was incubated in 1 L MRS (De Man, Rogosa, and Sharpe) agar broth at 37°C for 18 h and then heated at 100°C for 15 min to deactivate EPS-degrading enzymes before centrifuging to remove probiotic cells and debris. The supernatant was mixed with ethanol (95%) and cooled at 4°C for 12 h. The EPS precipitates were collected and washed with distilled water before mixing 20 mL EPS with 9 mM AgNO3. The mixture was stored for 60 days in the dark; a 10 h incubation resulted in a yellowish solution, indicating the formation of silver ENMs, with sizes ranging from 2-15 nm and shapes ranging from the spherical, hexagonal, rod, and triangular. The antimicrobial activity of biogenic silver ENMs was dose-dependent against E. coli, L. monocytogenes (food-borne pathogen), and multi-drug resistant pathogens K. pneumonia and P. aeruginosa. P. aeruginosa had the highest inhibition zones, while the growth of L. monocytogenes was only inhibited at high nanoparticle doses (i.e., 2 mg mL–1). Likewise, the efficacy of synthesized ENMs was dose-dependent against fungal pathogens Aspergillus spp. and Penicillium spp., particularly for Aspergillus spp. He et al. (2007) synthesized gold ENMs using the photosynthetic bacterial species Rhodo-pseudomonas capsulate by first maturing in a growth medium comprising pyruvate yeast extract, sodium chloride, ammonium chloride, and potassium hydrogen phosphate at pH 7 at 30°C for 3 days and then centrifuging to remove residual impurities. After washing with water, 1 g of the bacteria was mixed in 20 mL of 1×10−3 M chloroauric acid, neutralizing the pH with 0.1 M NaOH and 0.1 M HCl. As confirmed by TEM images, UV-visible spectra confirmed the nanoparticle synthesis with spherical ENMs (10–20 nm). Hence, synthesis of ENMs using microbial species is a time-efficient, ecofriendly, simple process that produces particles of uniform shape and size. Table 2 summarizes research studies using microbes to synthesize ENMs.
Applications of engineered nanomaterials in agriculture
In recent years, nanomaterials have been studied for various applications ranging from energy generation to food production. For these applications, nanomaterials are engineered to impart application-specific properties. For example, in agriculture, interactions of ENMs with food and non-food crops through various routes have been studied to enhance production and yield. ENMs are mostly applied to plants through roots (as nano-fertilizers) or leaves (by foliar spraying). The physiological mechanisms of leaves and roots are different. Consequently, the absorption mechanisms of ENMs are also different through these organs. Roots release exudates that inhibit the absorption of ENMs, while leaves can isolate ENMs in the cell wall after spraying on leaves. Foliar applied nutrients are transported downwards and further distributed to other parts of the plant (Kannan and Charnel, 1986).
Similarly, ENMs sprayed on the leaves increased ENMs content in the root system, which may help root cells absorb nutrients and improve their disease resistance. Compared with soil fertilization, foliar application of nano fertilizers can compensate for nutrient deficiency in plants more quickly by accumulating the applied nutrient (Alshaal and El-Ramady, 2017). Zinc ENMs were evaluated as nano fertilizers using soil fertilization technique for enhancing the growth of pearl millet. Positive effects were observed on the shoot and root lengths, chlorophyll content, total soluble leaf protein, and consequently grain yield of 37.7% as compared to the control plant i.e. without nano Zinc ENMs (Tarafdar et al., 2014). Foliar application of Zinc ENMs substantially increased plant growth and dry biomass (Najafi Vafa et al., 2015). Also, foliar sprays of TiO2 increased plant total dry matter by enhancing N assimilation, photo-reduction activities of photosystem II and electron transport chain and scavenging the reactive oxygen species (Raliya et al., 2015). The chlorophyll, carotenoids, and anthocyanin contents of maize crops increased significantly after foliar application of TiO2, increasing the yield (Morteza et al., 2013). Foliar application of ENMs, which has many advantages not available in traditional methods, is a research direction with great potential in the agro-industry. Figure 6 elaborates on the ENMs application techniques and impact on enhancing plant productivity.
Seed germination applications
Seed germination and seedling growth are the backbones of crop yield and productivity (Zulfiqar, 2021). Seed priming is a technique used to improve the quality of seeds and their tolerance to environmental stresses. Priming seeds with nanomaterials is a novel method to increase germination rates and decrease seed aging (Itroutwar et al., 2020). The effect of bulk zinc acetate and algal synthesized zinc oxide (ZnO) ENMs on maize seed germination and plant growth. Maize seeds primed with varying concentrations of ZnO ENMs significantly improved the percentage of early seed germination compared to bulk zinc acetate and hydro primed (control) seeds. The 100 mg L–1 ZnO treatment produced the highest germination percentage (87%) after one-week and the highest root and shoot lengths and widths. Panda (2017) soaked rice (Oryza sativa L.) in zinc nanoparticle solutions (5-50 mg L–1) for 1 h under continuous stirring in an incubator. Seeds primed with Zn particles had longer radicles and plumules, higher radical and plumule fresh and dry weights, and higher relative water contents (RWC) of radices and plumules than untreated seeds. Mohammed et al. (2019) observed the effect of silica ENMs (SiO2) at varying concentrations (100-300 mg L–1) on the growth parameters of cucumber seeds. At 200 and 300 mg L–1, silica-primed seeds reached 100% germination in 10 days. Seed priming also decreased the mean germination time from 4.1 days (no priming) to 2.8 days (200 mg L–1). Siddiqui and Al-Whaibi (2014) examined the effect of SiO2 ENMs (0–10 g L–1) on the germination characteristics of tomato seeds (L. esculentum Mill. cv. Super Strain B). Seed germination increased with increasing SiO2 concentration, peaking at 8 g L–1.
Baz et al. (2020) observed the effect of carbon ENMs (CENMs) under saline conditions on the seed germination characteristics of different lettuce species. Lettuce seeds were primed with a 0.3% (wt) solution of CENMs (90–110 nm) for 2–14 h, followed by washing with water and then salt solution before incubating for 9 h. For the salt treatment, seeds were primed with 150-250 mM NaCl for 4 h. The CENMs pretreatment significantly reduced the adverse effects of saline and hot conditions on seed germination. Even 2 h of CENMs priming improved seed resistance to saline, with germination rates reaching 100% compared to only 10% under saline conditions without no CENMs treatment also the germination rates of seeds under thermal stress of 34°C were observed to enhance in seeds primed with CENMs up to 90% as compared to 405 without CENMs. Only a few species had very low germination rates, even after priming for 10 h. Table 3 lists some studies using ENMs for seed priming and germination.
Plant growth, photosynthesis, water uptake, and mineralnutrition applications
Nanomaterials can alter plant growth through nutrient uptake, improving capacity, resistance against biotic and abiotic stresses, promoting nitrogen metabolism, osmotic protection, and plant defense against pathogens. The conventional application of nutrients to the soil has several disadvantages in terms of nutrient availability to plants. Therefore, the foliar application is the most efficient method of compensating for nutrient deficiencies and increasing crop yield and quality. Foliar spraying also reduces environmental contamination and increases nutrient use efficacy by decreasing the amount of fertilizer applied to the soil. Biologically engineered ZnO ENMs have been used as a low-cost biomass-enhancing agent for crop plants (Venkatachalam et al., 2017). The effect of 25-200 mg/L of ZnO ENMs and phosphorous on cotton seeds was analyzed in growth stimulation experiments. Root and shoot lengths, total biomass, and the growth tolerance index were directly proportional to the concentration of (200 mg L–1) zinc oxide ENMs. Rizwan et al. (2019) addressed the effect of zinc and iron ENMs on wheat’s growth characteristics and capacity to accumulate cadmium (Cd) (Triticum aestivum). Wheat seeds were primed for one day with different concentrations of ZnO (25-100 mg L–1) and FeO (5-20 mg L–1). After priming, the seeds were grown in Cd-contaminated soil. Primed seeds produced more healthier plants than unprimed seeds as plant height, shoot and spike lengths, and plant dry weight were directly proportional to the concentrations of Zn and Fe ENMs. Sun et al. (2020) observed growth parameters of tomato plants sprayed with 20-100 mg L–1 ZnO ENMs; the foliar sprayed plants had greater plant heights, root lengths, and chlorophyll contents than the unsprayed plants. In addition, plant mineral and nutrient contents (calcium, magnesium, sucrose, starch and glucose) increased, significantly increasing iron deficiency tolerance.
Song et al. (2012) analyzed the effect of 10-2000 mg/L of Titanium dioxide (TiO2) ENMs and bulk TiO2 on the growth parameters of Lemna minor. For nanoparticle TiO2 concentrations <500 mg L–1, plant growth (root length, fresh weight, and frond number) had a directly proportional relationship with TiO2 concentration; a similar trend occurred for bulk TiO2 but to a lesser degree than the ENMs. The same growth parameters decreased at nanoparticle concentrations ≥500 mg L–1. Daghan (2018) analyzed the effect of TiO2 ENMs (25-100 mg L–1) on the seedling growth of maize irrigated with wastewater. The 25 mg L–1 treatment inhibited the adverse effects of wastewater and reached 100% seed germination, while higher doses had adverse effects on the seed germination rate. Liu and Lal (2014) used hydroxyapatite ENMs on soybean (see Figure 7). All growth parameters (growth rate, plant height, seed yield, aboveground and belowground biomass) increased compared to conventional phosphorus fertilizer.
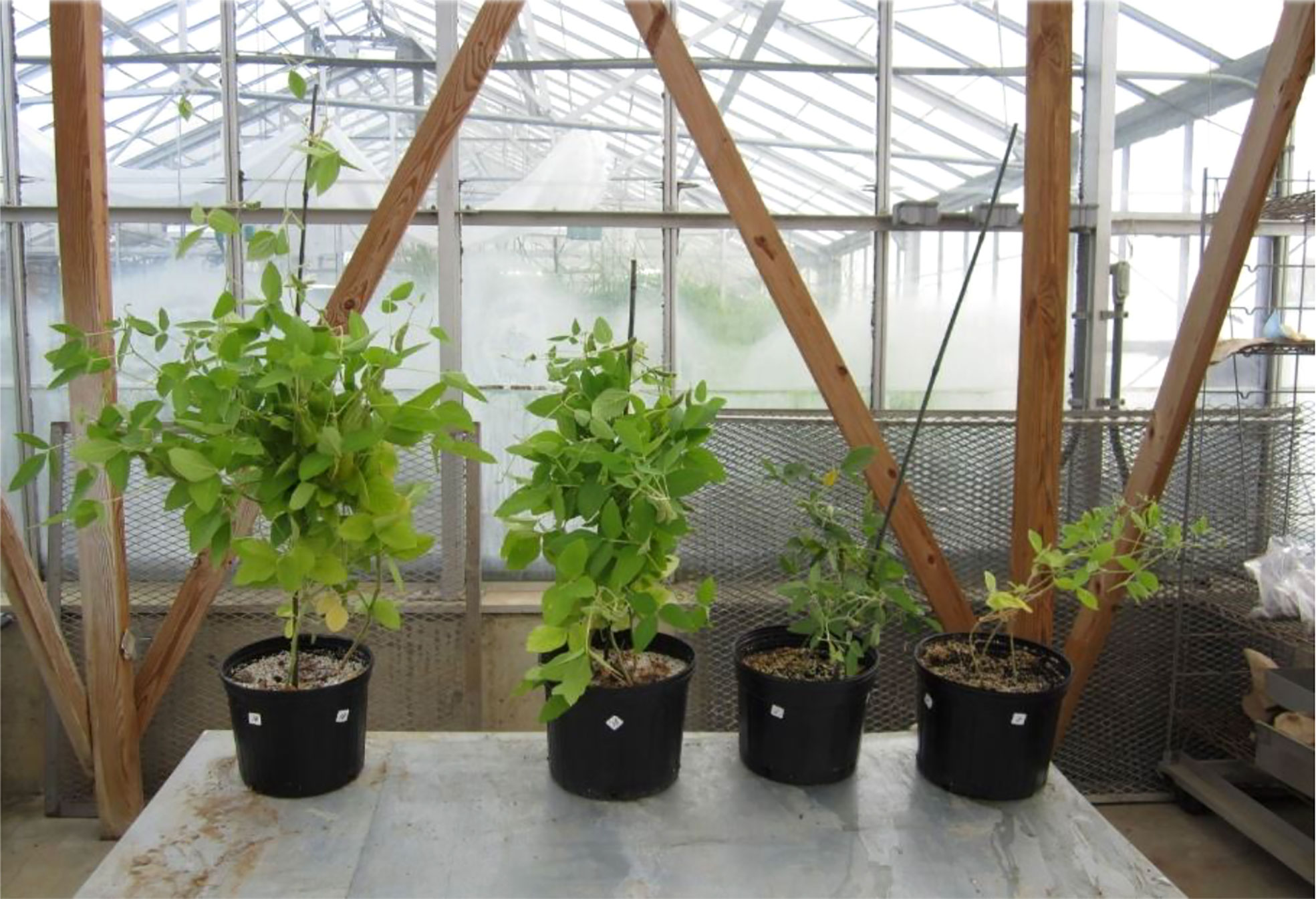
Figure 7 Soybean plant growth 15 weeks after germination under different fertilizer treatments (left to right) Hydroxyapatite ENMs (Engineered Nanomaterials), fertilizer plus normal phosphorous, fertilizer without phosphorous, and water (Liu and Lal, 2014). Copyright 2014.
Khan et al. (2021) analyzed the efficiency of silver ENMs (10-30 mM) for enhancing the growth of pearl millet under saline conditions (up to 150 mM NaCl). Adding ENMs under saline conditions significantly increased plant growth, including plant height, root, shoot, and dry grain weights, chlorophyll content, and photosynthetic rate. Suciaty et al. (2018) analyzed the effect of nano-silica (1.25-3.75 mL L–1) and rice hull ash (1-3 t ha–1) on the growth characteristics of Grobogan, a soybean cultivar. Rice hull ash increased plant height, but silica ENMs had no effect; silica ENMs and rice hull ash increased dry seed weight after applied individually. Table 4 lists other studies using ENMs for plant growth applications.
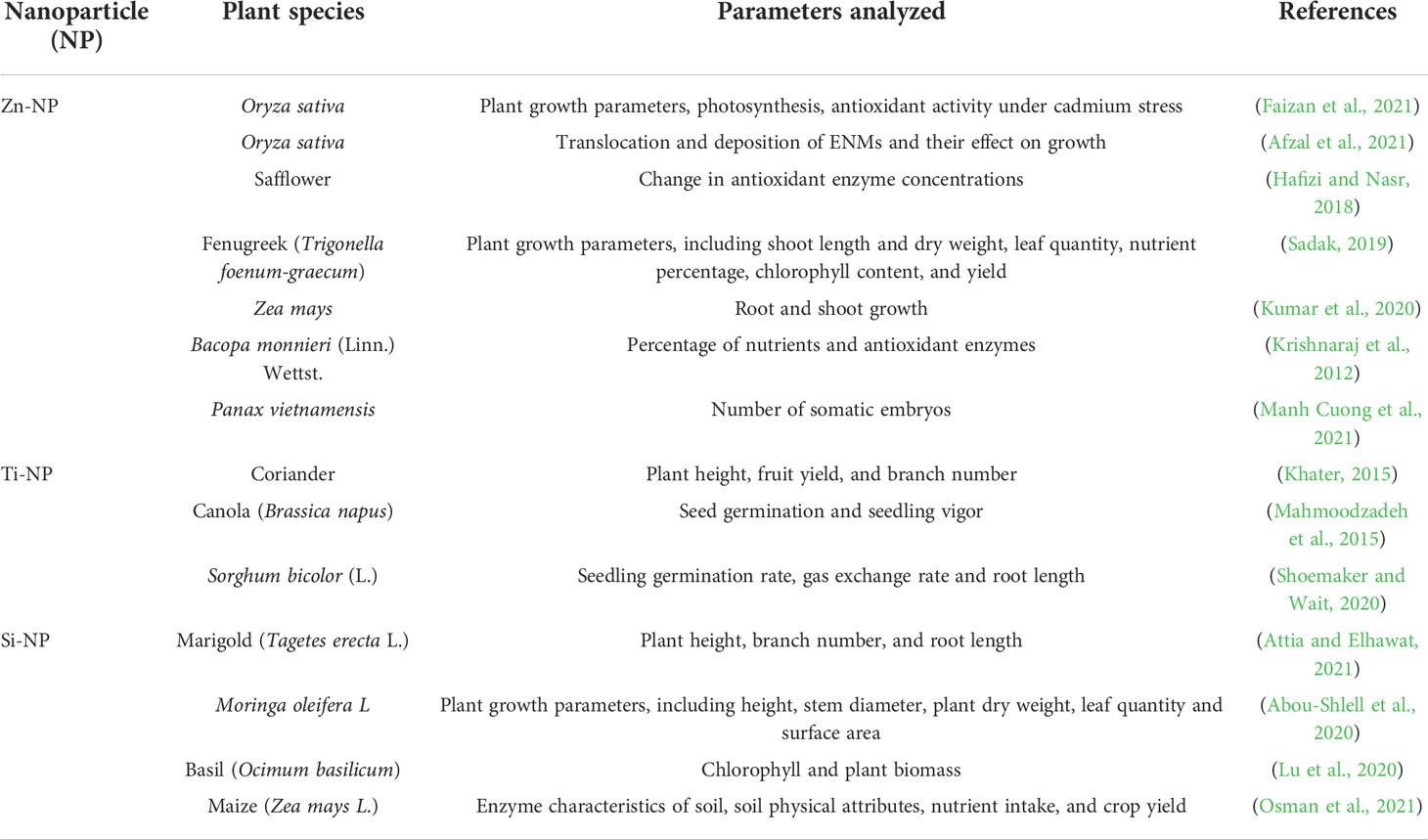
Table 4 Application of engineered nano materials to enhance plant growth and other eco- physiological parameters. .
Plant disease control and fruit yield applications
Nanomaterials extensively used to alleviate plant diseases, as many nanomaterials have inhibitory effects against disease-causing microorganisms. Nanomaterials can also increase the yield of fruit-bearing plants by fulfilling their nutrient requirements or catalyzing photosynthesis. Hernández-Hernández et al. (2017) evaluated the effect of copper ENMs (0.02-10 mg) embedded in chitosan-polyvinyl alcohol (Cs-PVA) hydrogels on the growth, productivity, and fruit quality of tomato plants. Growth parameters (stem diameter, stem, root, and leaf weights, flower bunches per plant, leaf number per plant, and yield) increased with nanoparticle treatment. (Xing et al. 2021) observed dose-dependent antimicrobial activity of oleoyl-chitosan (O-chitosan) ENMs against several plant pathogenic fungi. Four fungal species (N. sphaerica, B. dothidea, N. oryzae, and A. tenuissima) had significant growth inhibition at 2 mg mL–1 O-chitosan. Sathiyabama and Manikandan (2018) Analyzed the efficacy of copper-chitosan ENMs (seed priming or foliar spray) on finger millet. The combined treatment i.e. seed priming and foliar spray, increased plant yield, chlorophyll content, and leaf quantity and decreased spore growth of Pyricularia grisea, which causes blast disease in millet. Hao et al. (2019) analyzed the efficacy of four engineered nanomaterials [multi-walled carbon nanotubes (MWCNTs), reduced graphene oxide (RGO), titanium dioxide (TiO2), and copper oxide (CuO)] for fungal disease control in rose plants. The ENMs were suspended in water (50 or 200 mg L–1) and sprayed on the leaves of rose plants infected with Podosphaera pannosa (P. pannosa), which causes powdery mildew disease (see Figure 8). After 19 days of 200 mg L–1 treatment, RGO, MWCNTs, and TiO2 ENMs had decreased the size of the infected area by 46%, 60%, and 70%, respectively. At 50 mg L–1, no inhibitory affect was observed for these three materials. For CuO ENMs, the 50 and 200 mg L–1 treatments decreased the infected area by 73% and 99%, respectively. Hao et al. (2018) analyzed the efficacy of four ENMs (TiO2, Ferric oxide (Fe2O3), MWCNT, and fullerene) for antiviral activity and growth of tobacco (Nicotiana benthamiana) infected with turnip mosaic virus. A 50 or 200 mg L–1 of each nanoparticle-water suspension was sprayed on tobacco leaves for 21 days. For the metallic ENMs, the maximum antiviral effect occurred at 50 mg L–1, while MWCNT had an inhibitory effect at both doses, while Fullerene (C6) had no antiviral activity. Similarly, the metallic ENMs increased fresh biomass by 56% at 50 mg L–1, with no change at 200 mg L–1. The 50 and 200 mg L–1 treatment of MWCNTs and C60 increased fresh biomass by 55%. Zahedi et al. (2019b) sprayed selenium ENMs embedded in sodium metal (Na2SeO4; 1-2 μM) on pomegranate leaves (Punica granatum L. cv. Malase Saveh) to evaluate their effect on growth characteristics and fruit quality. Plants sprayed with selenium ENMs had higher leaf surface area, chlorophyll content, and fruit numbers than unsprayed plants; the 2 μM treatment increased fruit yield/plant by 35%, peel thickness by 33.6%, fruit weight by 5.2%, fruit length by 30%, and fruit diameter by 11%, and decreased the fruit cracking percentage by 54%. Nandini et al. (2017) analyzed the efficacy of a foliar spray of selenium (mediated with Trichoderma) ENMs against downy mildew disease (Sclerospora graminicola) in pearl millet. Different species of Trichoderma were used; T. asperelleum combined with Se ENMs had the best results against downy mildew disease, with a MIC of 150 ppm. Similarly, seed priming with T. asperelleum-mediated Se ENMs improved plant height, tiller number per seedling, dry weight, and chlorophyll content. Joshi et al. (2021) used myogenic selenium ENMs to prime tomato seeds and analyzed their efficacy against late blight disease; the Se-primed seeds inhibited the disease by 73%, increased plant height by 51% and fruit weight by 83%, and expedited flower emergence from 70 days (unprimed) to 61 days.
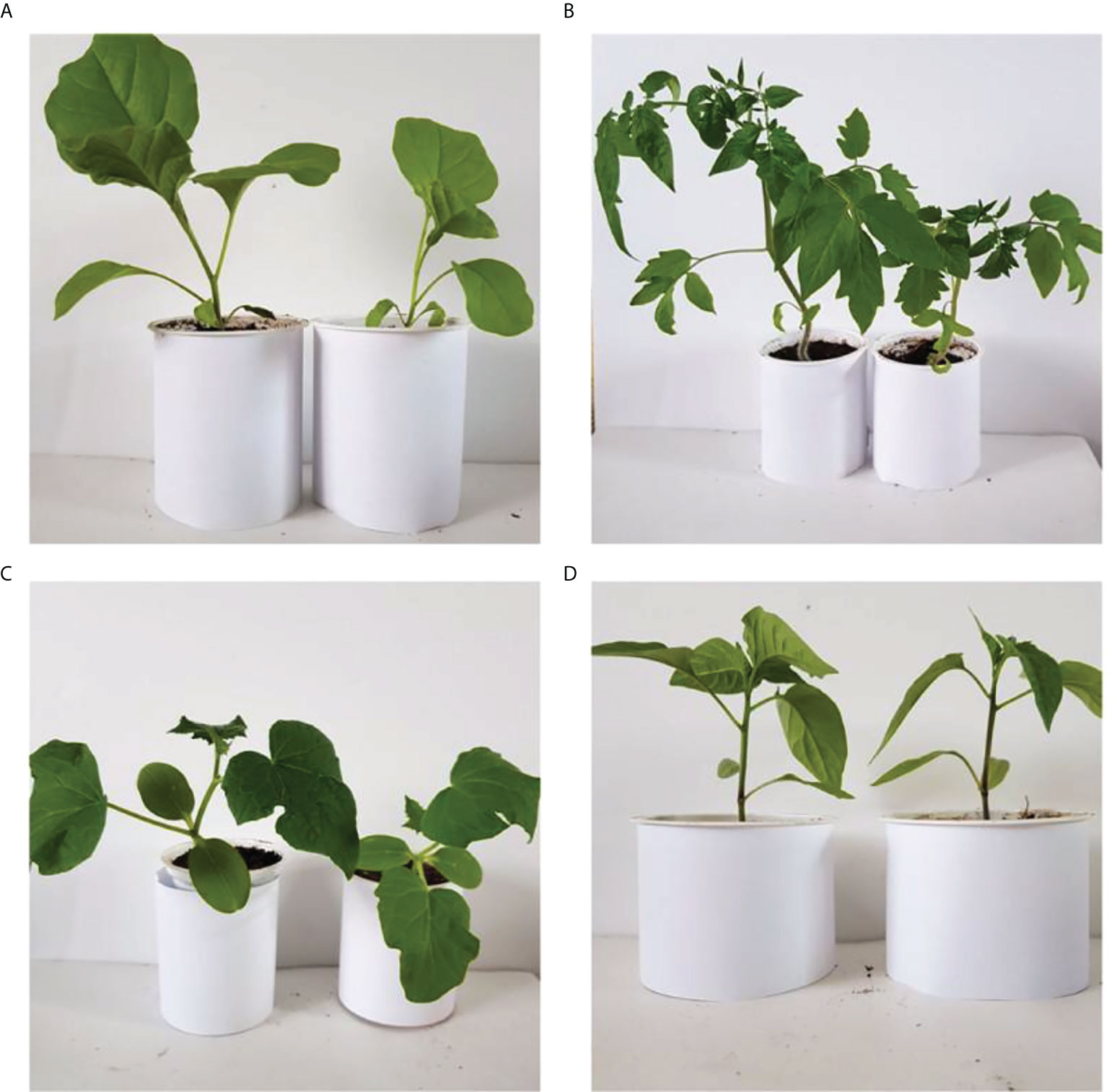
Figure 8 Effect of Se nano-fertilizers on (A) eggplant (B) cucumber (C) tomato, and (D) chili pepper (Gudkov et al., 2020). Copyright 2020.
Kumari et al. (2017) applied green-synthesized silver ENMs (5 μg mL–1) to tomato as an antidote for early blight disease (Alternaria solani). Three days of treatment eliminated the spore count. The silver ENMs treatment increased fresh and dry weights of leaves by 32 and 23%, respectively, Chlorophyll a by 14%, Chlorophyll b by 50%, total chlorophyll by 23%, and carotenoid content by 20%. Quiterio-Gutiérrez et al. (2019) sprayed selenium and copper ENMs on tomato leaves infected with Alternaria solani, which increased the number of fruit clusters per plant by 9%. Table 5 lists other ENM applications for plant disease inhibition and fruit yield enhancement.
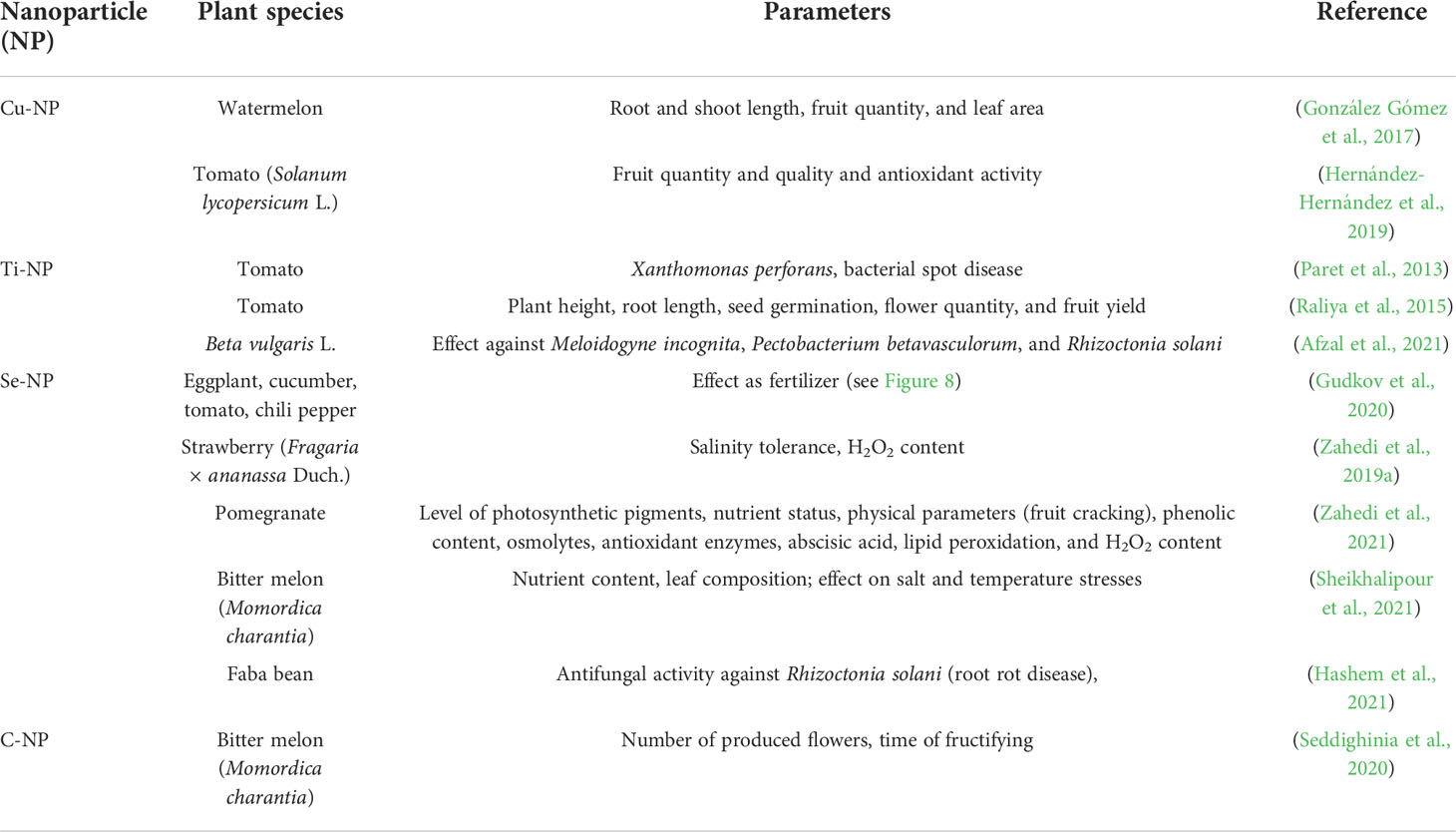
Table 5 Application of engineered nano-materials to enhance fruit yield and control disease in plants.
Limitations of using engineered nano materials
Despite many positive effects of using ENMs to enhance crop production, long-term exposure and accumulation of nanomaterials need to be addressed (Rajput et al., 2020). The effect of nanomaterials on plants largely depends on exposure intensity, concentration, size and shape of ENMs, and mode of contact (Corredor et al., 2009). Studies have shown that using ENMs in agriculture can affect plant metabolism, nutrient transport, and root morphology various cellular functions (Sunghyun et al., 2013). ENMs alter the protein, carbohydrate, and antioxidant patterns in some plants. Once ENMs enter cells. Adverse effects of ENMs include binding ENM ions to sulfhydryl groups of proteins and replacing essential cations with specific binding sites, leading to enzyme inactivation and production of reactive oxygen species, with consequent oxidative damage to lipids, proteins, and nucleic acids. ENMs can also trigger oxidative stress by depleting enzymes and major thiols containing antioxidant cells, disrupting the electron transport chain or inducing lipid peroxidation. Zhao et al. (2014) reported that CeO2 ENMs (800 mg kg–1) significantly reduced the phenolic content in cucumber, an important characteristic as it may reduce the risk of cancer. Parameters like the type of materials, synthesis method, Particle size and shape, functional groups stabilization agent used and application technique also affect the interactions and the outcome and add more complexity in cellular outputs (Bhardwaj et al., 2022). Detailed toxicological studies are required to analyze their safety for field use. An important concern regarding the use of nano-fertilizers is the buildup of ENMs in plant tissues without transformation and assimilation or even physical entrapment on plant surfaces. Stampoulis et al. (2009) investigated the effect of MWCNT, Ag, Cu, ZnO, and Si ENMs and their bulk materials (0, 1.0, 10, 50, 100, 500, and 1000 mg L–1) on the growth parameters of Cucurbita pepo (zucchini). The application of Cu ENMs at 1000 mgL-1 decreased root elongation by 77% compared to the control. At 1000 mg L–1, MWCNT, Ag, Cu, and ZnO ENMs decreased plant biomass by 60, 75, 90, and 90%, respectively, compared to control. Similar studies have reported potential adverse impacts of ENMs on plant growth, crop yield, shoot and root lengths, seed germination, water content, and relative chlorophyll (Table 6).
Conclusion and future prospectus
This review summarized eco-friendly and sustainable methods for synthesizing novel materials in agricultural applications and to exploit the chemical and physical properties of naturally occurring plant extract. Nanomaterials can be useful for specific applications using various natural extracts—easing the synthesis process by stabilizing and reducing agents—and in specific sizes and shapes. There are increasing concerns in the agricultural sector about enhancing crop production and yield while resisting harmful environmental stresses. Engineered nanomaterials (ENMs) for specific applications in the agricultural sector can be used as nano-fertilizers to provide essential nutrients for plant growth. They can also be used in seed priming and foliar spraying to mitigate environmental stresses and plant diseases. The increasing demand for food, medicine, and other crops and the changing climate requires new technologies to reduce the demand and supply gap. Several challenges are associated with the synthesis and application of ENMs, including determining the complete life cycle of ENMs in the food chain to identifying the potential toxicity mechanisms such as the uptake and accumulation of ENMs developing methodologies for green synthesis of ENMs at an industrial scale, exploiting new multifunctional bio-mediated ENMs with improved plant growth and control over environmental stresses, and identifying the effect of ENMs on physiological parameters and nutritional quality of different edible and non-edible plant species. Addressing these challenges will help decrease the reluctance of the agricultural sector to adopt new technologies.
Author contributions
All authors contributed to the study’s conception and design. Material preparation, search, and collection of relevant articles and reviews were performed by KB, SH and FZ, ZA, HA. ZA, ZS and KHMS thoroughly checked the first draft and critically improved the manuscript. All authors contributed to the article and approved the submitted version.
Conflict of interest
The authors declare that the research was conducted in the absence of any commercial or financial relationships that could be construed as a potential conflict of interest.
Publisher’s note
All claims expressed in this article are solely those of the authors and do not necessarily represent those of their affiliated organizations, or those of the publisher, the editors and the reviewers. Any product that may be evaluated in this article, or claim that may be made by its manufacturer, is not guaranteed or endorsed by the publisher.
Abbreviations
Ag, Silver; AgCl, Silver chloride; AgNO3, Silver nitrate; Cd, Cadmium; CdCl2, Cadmium chloride; CdTe, Cadmium telluride; CENMs, Carbon nanoparticles; Cu, Copper; CuO, Copper (II) oxide; CuSO4, Copper sulfate; DMF, Dimethylformamide; EMD, Electrolytic manganese dioxide; ENMs, Engineered nanomaterials; EPS, Exopolysaccharide; FeSO4, Ferrous sulfate; FeO, Iron oxide; Fe3O4, Iron (II,III) oxide; HCl, Hydrochloric acid; KCl, Potassium chloride; K2HPO4, Potassium hydrogen phosphate; MgSO4, Magnesium sulfate; MIC, Minimum inhibition concentration; MBC, Minimum bactericidal concentration; MHB, Muller-Hinton Broth; MRS, De Man, Rogosa, and Sharpe agar; MSA, Mercaptosuccinic acid; MWCNTs, Multiwalled carbon nanotubes; NaBH4, Sodium borohydride; NaCl, Sodium chloride; NaOH, Sodium hydroxide; Na2(PdCl4), Sodium tetrachloropalladate; Na2TeO3, Sodium tellurite; NPs, Nanoparticles; ORR, Oxygen reduction reaction; RGO, Reduced graphene oxide (RGO); RWC, Relative water content; Se, Selenium; SEM, Scanning electron microscopy; TEM, Transmission electron microscopy; TiO2, Titanium dioxide; XRD, X-ray diffraction; ZnO, Zinc oxide; ZrOCl2, Zirconyl chloride.
References
Abboud, Y., Saffaj, T., Chagraoui, A., El Bouari, A., Brouzi, K., Tanane, O., et al. (2014). Biosynthesis, characterization and antimicrobial activity of copper oxide nanoparticles (CONPs) produced using brown alga extract (Bifurcaria bifurcata). Appl. Nanosc. 4, 571–576. doi: 10.1007/s13204-013-0233-x
Abdel-Raouf, N., Al-Enazi, N. M., Ibraheem, I. B. M., Alharbi, R. M., Alkhulaifi, M. M. (2019). Biosynthesis of silver nanoparticles by using of the marine brown alga Padina pavonia and their characterization. Saudi. J. Biol. Sci. 26, 1207–1215. doi: 10.1016/j.sjbs.2018.01.007
Abideen, Z., Waqif, H., Munir, N., El-Keblawy, A., Hasnain, M., Radicetti, E., et al. (2022). Algal-mediated nanoparticles, phycochar, and biofertilizers for mitigating abiotic stresses in plants: A review. Agronomy. 12 (8), 1788. doi: 10.3390/agronomy12081788
Aboelfetoh, E. F., El-Shenody, R. A., Ghobara, M. M. (2017). Eco-friendly synthesis of silver nanoparticles using green algae (Caulerpa serrulata): Reaction optimization, catalytic and antibacterial activities. Environ. Monit. Assess. 189 (7), 349. doi: 10.1007/s10661-017-6033-0
Abou-Shlell, M. K., El-Emary, F. A., Khalifa, A. A. (2020). Effect of nanoparticle on growth, biochemical and anatomical characteristics of moringa plant (Moringa oleifera l.) under salinity stress condition. Arch. Agric. Sci. J. 3, 186–213. doi: 10.21608/AASJ.2020.47687.1044
Afzal, S., Aftab, T., Singh, N. (2021). Impact of zinc oxide and iron oxide nanoparticles on uptake, translocation, and physiological effects in Oryza sativa l. J. Plant Growth Regul. 41, 1445–1461. doi: 10.1007/s00344-021-10388-1
Agarwal, H., Nakara, A., Menon, S., Shanmugam, V. (2019). Eco-friendly synthesis of zinc oxide nanoparticles using Cinnamomum tamala leaf extract and its promising effect towards the antibacterial activity. J. Drug Deliv. Sci. Technol. 53, 101212. doi: 10.1016/j.jddst.2019.101212
Ahmad, W., Kumar Jaiswal, K., Amjad, M. (2021). Euphorbia herita leaf extract as a reducing agent in a facile green synthesis of iron oxide nanoparticles and antimicrobial activity evaluation. Inorg. Nano-Met. Chem. 51 (9), 1147–1154. doi: 10.1080/24701556.2020.1815062
Ahmad, N., Sharma, S., Alam, M. K., Singh, V. N., Shamsi, S. F., Mehta, B. R., et al. (2010). Rapid synthesis of silver nanoparticles using dried medicinal plant of basil. Colloids. Surf. B. Biointerfaces. 81 (1), 81–86. doi: 10.1016/j.colsurfb.2010.06.029
Ahmed, S., Saifullah, Ahmad, M., Swami, B. L., Ikram, S. (2016). Green synthesis of silver nanoparticles using azadirachta indica aqueous leaf extract. J. Radiat. Res. Appl. Sci. 9, 1–7. doi: 10.1016/j.jrras.2015.06.006
Ahmed, B., Shahid, M., Khan, M. S., Musarrat, J. (2018). Chromosomal aberrations, cell suppression and oxidative stress generation induced by metal oxide nanoparticles in onion (Allium cepa) bulb. Metallomics. 10 (9), 1315–1327. doi: 10.1039/c8mt00093j
Alijani, H. Q., Pourseyedi, S., Torkzadeh Mahani, M., Khatami, M. (2019). Green synthesis of zinc sulfide (ZnS) nanoparticles using Stevia rebaudiana bertoni and evaluation of its cytotoxic properties. J. Mol. Struct. 1175, 214–218. doi: 10.1016/j.molstruc.2018.07.103
Ali, S., Liu, Y., Ishaq, M., Shah, T., Abdullah Ilyas, A., Din, I. U. (2017). Climate change and its impact on the yield of major food crops: Evidence from pakistan. Foods 6, 39. doi: 10.3390/foods6060039
Alshaal, T., El-Ramady, H. (2017). Foliar application: From plant nutrition to biofortification. Env. Biodivers. Soil Secur. 1, 71–83. doi: 10.21608/JENVBS.2017.1089.1006
Altikatoglu, M., Attar, A., Erci, F., Cristache, C., Isildak, I. (2017). Green synthesis of copper oxide nanoparticles using Ocimum basilicum extract and their antibacterial activity. Fresenius. Environ. Bull. 26, 7832–7837.
Ammar, A. S. (2018). Nanotechnologies associated to floral resources in agri-food sector. Acta Agronómica. 67, 146–159. doi: 10.1016/j.heliyon.2021.e08539
Anjali, K. P., Sangeetha, B. M., Raghunathan, R., Devi, G., Dutta, S. (2021). Seaweed mediated fabrication of zinc oxide nanoparticles and their antibacterial, antifungal and anticancer applications. Chem. Select. 6, 647–656. doi: 10.1002/slct.202003517
Anjum, N. A., Singh, N., Singh, M. K., Sayeed, I., Duarte, A. C., Pereira, E., et al. (2014). Single-bilayer graphene oxide sheet impacts and underlying potential mechanism assessment in germinating faba bean (Vicia faba l.). Sci. Total. Environ. 472, 834–841. doi: 10.1016/j.scitotenv.2013.11.018
Araya-Castro, K., Chao, T.-C., Durán-Vinet, B., Cisternas, C., Ciudad, G., Rubilar, O. (2021). Green synthesis of copper oxide nanoparticles using protein fractions from an aqueous extract of brown algae Macrocystis pyrifera. Processes. 9 (1), 78. doi: 10.3390/pr9010078
Aritonang, H. F., Koleangan, H., Wuntu, A. D. (2019). Synthesis of silver nanoparticles using aqueous extract of medicinal plants’ (>Impatiens balsamina and Lantana camara) fresh leaves and analysis of antimicrobial activity. Int. J. Microbiol. 8642303. doi: 10.1155/2019/8642303
Arya, A., Gupta, K., Chundawat, T. S., Vaya, D. (2018). Biogenic synthesis of copper and silver nanoparticles using green alga Botryococcus braunii and its antimicrobial activity. Bioinorg. Chem. Appl. 2018, 7879403. doi: 10.1155/2019/8642303
Attia, E. A., Elhawat, N. (2021). Combined foliar and soil application of silica nanoparticles enhances the growth, flowering period and flower characteristics of marigold (Tagetes erecta l.). Sci. Hortic. 282, 110015. doi: 10.1016/j.scienta.2021.110015
Bandala, E. R., Berli, M. (2019). Engineered nanomaterials (ENMs) and their role at the nexus of food, energy, and water. Mater. Sci. Technol. 2, 29–40. doi: 10.1016/j.mset.2018.09.004
Bao, H., Hao, N., Yang, Y., Zhao, D. (2010). Biosynthesis of biocompatible cadmium telluride quantum dots using yeast cells. Nano. Res. 3, 481–489. doi: 10.1007/s12274-010-0008-6
Baz, H., Creech, M., Chen, J., Gong, H., Bradford, K., Huo, H. (2020). Water-soluble carbon nanoparticles improve seed germination and post-germination growth of lettuce under salinity stress. Agronomy. 10, 1192. doi: 10.3390/agronomy10081192
Begum, P., Ikhtiari, R., Fugetsu, B. (2011). Graphene phytotoxicity in the seedling stage of cabbage, tomato, red spinach, and lettuce. Carbon. 49, 3907–3919. doi: 10.1016/j.carbon.2011.05.029
Bhardwaj, A. K., Arya, G., Kumar, R., Hamed, L., Pirasteh-Anosheh, H., Jasrotia, P., et al. (2022). Switching to nanonutrients for sustaining agroecosystems and environment: The challenges and benefits in moving up from ionic to particle feeding. J. Nanobiotechnol. 20 (1), 19. doi: 10.1186/s12951-021-01177-9
Bhavyasree, P. G., Xavier, T. S. (2020). Green synthesis of copper Oxide/Carbon nanocomposites using the leaf extract of Adhatoda vasica nees, their characterization and antimicrobial activity. Heliyon. 6 (2), e03323. doi: 10.1016/j.heliyon.2020.e03323
Bhuiyan, M. S. H., Miah, M. Y., Paul, S. C., Aka, T. D., Saha, O., Rahaman, M. M., et al. (2020). Green synthesis of iron oxide nanoparticle using carica papaya leaf extract: Application for photocatalytic degradation of remazol yellow RR dye and antibacterial activity. Heliyon. 6, e04603. doi: 10.1016/j.heliyon.2020.e03323
Burke, D. J., Pietrasiak, N., Situ, S. F., Abenojar, E. C., Porche, M., Kraj, P., et al. (2015). Iron oxide and titanium dioxide nanoparticle effects on plant performance and root associated microbes. Int. J. Mol. Sci. 16 (10), 23630–23650. doi: 10.3390/ijms161023630
Burman, U., Saini, M., Kumar, P. (2013). Effect of zinc oxide nanoparticles on growth and antioxidant system of chickpea seedlings. Environ. Toxicol. Chem. 95, 605–612. doi: 10.1080/02772248.2013.803796
Chung, I.-M., Park, I., Seung-Hyun, K., Thiruvengadam, M., Rajakumar, G. (2016). Plant-mediated synthesis of silver nanoparticles: Their characteristic properties and therapeutic applications. Nanoscale Res. Lett. 11, 40. doi: 10.1186/s11671-016-1257-4
Coman, V., Oprea, I., Leopold, L. F., Vodnar, D. C., Coman, C. (2019). Soybean interaction with engineered nanomaterials: A literature review of recent data. Nanomaterials. 9, 1248. doi: 10.3390/nano9091248
Corredor, E., Testillano, P. S., Coronado, M.-J., González-Melendi, P., Fernández-Pacheco, R., Marquina, C., et al. (2009). Nanoparticle penetration and transport in living pumpkin plants: In situsubcellular identification. BMC Plant Biol. 9, 45. doi: 10.1186/1471-2229-9-45
Crişan, M., Grozav, M., Bertea, C. (2009). Arabidopsis thaliana seed germination and early seedling growth are inhibited by monoethanolamine salts of para-halogenated benzoic acids. J. Plant Interact. 4 (4), 271–277. doi: 10.1080/17429140903063072
Daghan, H. (2018). Effects of TiO2 nanoparticles on maize (Zea mays l.) growth, chlorophyll content and nutrient uptake. Appl. Ecol. Environ. Res. 16, 6873–6883. doi: 10.15666/aeer/1605_68736883
de Aragão, A. P., De Oliveira, T. M., Quelemes, P. V., Perfeito, M. L. G., Araújo, M. C., Santiago, J. D. A. S., et al. (2019). Green synthesis of silver nanoparticles using the seaweed Gracilaria birdiae and their antibacterial activity. Arab. J. Chem. 12, 4182–4188. doi: 10.1016/j.arabjc.2016.04.014
Devi, H. S., Boda, M. A., Shah, M. A., Parveen, S., Wani, A. H. (2019). Green synthesis of iron oxide nanoparticles using platanus orientalis leaf extract for antifungal activity. Green Process. Synth. 8, 38–45. doi: 10.1515/gps-2017-0145
Dhas, T. S., Kumar, V. G., Karthick, V., Angel, K. J., Govindaraju, K. (2013). Facile synthesis of silver chloride nanoparticles using marine alga and its antibacterial efficacy. Spectrochim. Acta A. Mol. Biomol. 120, 416–420. doi: 10.1016/j.saa.2013.10.044
Dontsova, T. A., Nahirniak, S. V., Astrelin, I. M. (2019). Metaloxide nanomaterials and nanocomposites of ecological purpose. J. Nanomater. 2020, 5942194. doi: 10.1155/2019/5942194
Dulta, K., Koşarsoy Ağçeli, G., Chauhan, P., Jasrotia, R., Chauhan, P. K. (2021). Ecofriendly synthesis of zinc oxide nanoparticles by Carica papaya leaf extract and their applications. J. Clust. Sci. 33, 603–617. doi: 10.1007/s10876-020-01962-w
Erci, F., Cakir-Koc, R. (2021). Rapid green synthesis of noncytotoxic iron oxide nanoparticles using aqueous leaf extract of Thymbra spicata and evaluation of their antibacterial, antibiofilm, and antioxidant activity. Inorg. Nano-Met. Chem. 51, 683–692. doi: 10.1080/24701556.2020.1802754
Eroglu, E., Chen, X., Bradshaw, M., Agarwal, V., Zou, J., Stewart, S. G., et al. (2013). Biogenic production of palladium nanocrystals using microalgae and their immobilization on chitosan nanofibers for catalytic applications. RSC Advances. 3, 1009–1012. doi: 10.1039/C2RA22402J
Eugenio, M., Müller, N., Frasés, S., Almeida-Paes, R., Lima, L. M. T. R., Lemgruber, L., et al. (2016). Yeast-derived biosynthesis of silver/silver chloride nanoparticles and their antiproliferative activity against bacteria. RSC Advances. 6, 9893–9904. doi: 10.1039/C5RA22727E
Faizan, M., Bhat, J. A., Hessini, K., Yu, F., Ahmad, P. (2021). Zinc oxide nanoparticles alleviates the adverse effects of cadmium stress on Oryza sativa via modulation of the photosynthesis and antioxidant defense system. Ecotoxicol. Environ. Saf. 220, 112401. doi: 10.1016/j.ecoenv.2021.112401
FAO (2017). The future of food and agriculture: Trends and challenges (Rome, Italy: The Food and Agriculture Organization (FAO)) 296, 1–180.
Fernández, J. G., Fernández-Baldo, M. A., Berni, E., Camí, G., Durán, N., Raba, J., et al. (2016). Production of silver nanoparticles using yeasts and evaluation of their antifungal activity against phytopathogenic fungi. Process Biochem. 51, 1306–1313. doi: 10.1016/j.procbio.2016.05.021
González Gómez, H., Ramírez Godina, F., Ortega Ortiz, H., Benavides Mendoza, A., Robledo Torres, V., Cabrera de la Fuente, M. (2017). Use of chitosan-PVA hydrogels with copper nanoparticles to improve the growth of grafted watermelon. Molecules. 22 (7), 1031. doi: 10.3390/molecules22071031
Gudkov, S. V., Shafeev, G. A., Glinushkin, A. P., Shkirin, A. V., Barmina, E. V., Rakov, I. I., et al. (2020). Production and use of selenium nanoparticles as fertilizers. ACS Omega. 5, 17767–17774. doi: 10.1021/acsomega.0c02448
Hafizi, Z., Nasr, N. (2018). The effect of zinc oxide nanoparticles on safflower plant growth and physiology. Eng. Appl. Sci. Res. 8, 2508–2513. doi: 10.48084/etasr.1571
Haghighi, M., Teixeira Da Silva, J. A. (2014). The effect of carbon nanotubes on the seed germination and seedling growth of four vegetable species. J. Crop Sci. Biotechnol. 17, 201–208. doi: 10.1007/s12892-014-0057-6
Hamouda, R. A., Hussein, M. H., Abo-Elmagd, R. A., Bawazir, S. S. (2019). Synthesis and biological characterization of silver nanoparticles derived from the cyanobacterium oscillatoria limnetica. Sci. Rep. 9, 13071. doi: 10.1038/s41598-019-49444-y
Hao, Y., Fang, P., Ma, C., White, J. C., Xiang, Z., Wang, H., et al. (2019). Engineered nanomaterials inhibit Podosphaera pannosa infection on rose leaves by regulating phytohormones. Environ. Res. 170, 1–6. doi: 10.1016/j.envres.2018.12.008
Hao, R., Li, D., Zhang, J., Jiao, T. (2021). Green synthesis of iron nanoparticles using green tea and its removal of hexavalent chromium. Nanomaterials. 11 (3), 650. doi: 10.3390/nano11030650
Hao, Y., Yuan, W., Ma, C., White, J. C., Zhang, Z., Adeel, M., et al. (2018). Engineered nanomaterials suppress turnip mosaic virus infection in tobacco (Nicotiana benthamiana). Environ. Sci. Nano. 5, 1685–1693. doi: 10.1039/C8EN00014J
Hashem, A. H., Abdelaziz, A. M., Askar, A. A., Fouda, H. M., Khalil, A. M. A., Abd-Elsalam, K. A., et al. (2021). Bacillus megaterium-mediated synthesis of selenium nanoparticles and their antifungal activity against Rhizoctonia solani in faba bean plants. J. Fungus. 7, 195. doi: 10.3390/jof7030195
Hasheminya, S.-M., Dehghannya, J. (2020). Green synthesis and characterization of copper nanoparticles using Eryngium caucasicum trautv aqueous extracts and its antioxidant and antimicrobial properties. Part. Sci. Technol. 38, 1019–1026. doi: 10.1080/02726351.2019.1658664
Hasnain, M., Munir, N., Siddiqui, Z. S., Ali, F., El-Keblawy, A., Abideen, Z. (2022). Integral approach for the evaluation of sugar cane bio-waste molasses and effects on algal lipids and biodiesel production. Waste Biomass Valori. 1, 20. doi: 10.1007/s12649-022-01864-0
He, S., Guo, Z., Zhang, Y., Zhang, S., Wang, J., Gu, N. (2007). Biosynthesis of gold nanoparticles using the bacteria Rhodopseudomonas capsulata. Mater. Lett. 61, 3984–3987. doi: 10.1016/j.matlet.2007.01.018
Hemlata, Meena, P. R., Singh, A. P., Tejavath, K. K. (2020). Biosynthesis of silver nanoparticles using Cucumis prophetarum aqueous leaf extract and their antibacterial and antiproliferative activity against cancer cell lines. ACS Omega. 5, 5520–5528. doi: 10.1021/acsomega.0c00155
Hernández-Hernández, H., Benavides-Mendoza, A., Ortega-Ortiz, H., Hernández-Fuentes, A., Juárez Maldonado, A. (2017). Cu Nanoparticles in chitosan-PVA hydrogels as promoters of growth, productivity and fruit quality in tomato. Emir. J. Food Agric. 29 (8), 573–580. doi: 10.9755/ejfa.2016-08-1127
Hernández-Hernández, H., Quiterio-Gutiérrez, T., Cadenas-Pliego, G., Ortega-Ortiz, H., Hernández-Fuentes, A. D., Cabrera de la Fuente, M., et al. (2019). Impact of selenium and copper nanoparticles on yield, antioxidant system, and fruit quality of tomato plants. Plants (Basel). 8 (10), 355. doi: 10.3390/plants8100355
Hochella Michael, F., Mogk David, W., Ranville, J., Allen Irving, C., Luther George, W., Marr Linsey, C., et al. (2019). Natural, incidental, and engineered nanomaterials and their impacts on the earth system. Science. 363, eaau8299. doi: 10.1126/science.aau8299
Hojjat, S., Hojjat, H. (2016). Effects of silver nanoparticle exposure on germination of lentil (Lens culinaris medik.). Int. J. Farm Alli. Sci. 5 (3), 248–252.
Hojjat, S. S., Kamyab, M. (2017). The effect of silver nanoparticle on Fenugreek seed germination under salinity levels. Russ. Agric. Sci. 43, 61–65. doi: 10.3103/S1068367417010189
Hou, Y., Hou, Y., Ren, Y., Shi, Y., Jin, X., Dong, Y., et al. (2020). C. aromaticus leaf extract mediated synthesis of zinc oxide nanoparticles and their antimicrobial activity towards clinically multidrug-resistant bacteria isolated from pneumonia patients in nursing care. Mater. Res. Express. 7, 095015. doi: 10.1155/2020/7459042
Ijaz, F., Shahid, S., Khan, S. A., Ahmad, W., Zaman, S. (2017). Green synthesis of copper oxide nanoparticles using abutilon indicum leaf extract: Antimicrobial, antioxidant and photocatalytic dye degradation activities. Trop. J. Pharm. Res. 16 (4), 743. doi: 10.4314/tjpr.v16i4.2
Ishwarya, R., Vaseeharan, B., Kalyani, S., Banumathi, B., Govindarajan, M., Alharbi, N. S., et al. (2018). Facile green synthesis of zinc oxide nanoparticles using Ulva lactuca seaweed extract and evaluation of their photocatalytic, antibiofilm and insecticidal activity. J. Photochem. Photobiol. B. 178, 249–258. doi: 10.1016/j.jphotobiol.2017.11.006
Itroutwar, P. D., Kasivelu, G., Raguraman, V., Malaichamy, K., Sevathapandian, S. K. (2020). Effects of biogenic zinc oxide nanoparticles on seed germination and seedling vigor of maize (Zea mays). Biocatal. Agric. Biotechnol. 29, 101778. doi: 10.1016/j.bcab.2020.101778
Joshi, S. M., De Britto, S., Jogaiah, S. (2021). Myco-engineered selenium nanoparticles elicit resistance against tomato late blight disease by regulating differential expression of cellular, biochemical and defense responsive genes. J. Biotechnol. 325, 196–206. doi: 10.1016/j.jbiotec.2020.10.023
Kalpana, V. N., Devi Rajeswari, V. (2018). A review on green synthesis, biomedical applications, and toxicity studies of ZnO NPs. Bioinorg. Chem. Appl. 2018, 3569758. doi: 10.1155/2018/3569758
Kanmani, P., Lim, S. T. (2013). Synthesis and structural characterization of silver nanoparticles using bacterial exopolysaccharide and its antimicrobial activity against food and multidrug resistant pathogens. Process Biochem. 48, 1099–1106. doi: 10.1016/j.procbio.2013.05.011
Kannan, S., Charnel, A. (1986). Foliar absorption and transport of inorganic nutrients. CRC Crit. Rev. Plant Sci. 4, 341–375. doi: 10.1080/07352688609382231
Katata-Seru, L., Moremedi, T., Aremu, O. S., Bahadur, I. (2018). Green synthesis of iron nanoparticles using moringa oleifera extracts and their applications: Removal of nitrate from water and antibacterial activity against escherichia coli. J. Mol. Liq. 256, 296–304. doi: 10.1016/j.molliq.2017.11.093
Kathiraven, T., Sundaramanickam, A., Shanmugam, N., Balasubramanian, T. (2015). Green synthesis of silver nanoparticles using marine algae Caulerpa racemosa and their antibacterial activity against some human pathogens. Appl. Nanosci. 5, 499–504. doi: 10.1007/s13204-014-0341-2
Khalaki, M., Ghorbani, A., Moameri, M. (2016). Effects of silica and silver nanoparticles on seed germination traits of Thymus kotschyanus in laboratory conditions. J. Rangel. Sci. 6, 221–231. doi: 20.1001.1.20089996.2016.6.3.4.5
Khaldari, I., Naghavi, M. R., Motamedi, E. (2021). Synthesis of green and pure copper oxide nanoparticles using two plant resources via solid-state route and their phytotoxicity assessment. RSC Advances. 11, 3346–3353. doi: 10.1039/D0RA09924D
Khan, Z., Ansari, M. Y. K. (2018). Impact of engineered Si nanoparticles on seed germination, vigour index and genotoxicity assessment via DNA damage of root tip cells in lens culinaris. J. Plant Biochem. Physiol. 6, 219. doi: 10.4172/2329-9029.1000218
Khan, I. A.-O., Awan, S. A., Raza, M. A., Rizwan, M., Tariq, R., Ali, S., et al. (2021). Silver nanoparticles improved the plant growth and reduced the sodium and chlorine accumulation in pearl millet: a life cycle study. Environ. Sci. pollut. Res. Int. 28 (11), 13712–13724. doi: 10.1007/s11356-020-11612-3
Khater, M. (2015). Effect of titanium nanoparticles (TiO2) on growth, yield and chemical constituents of coriander plants. Arab. J. Nucl. Sci. Appl. 48, 187–194.
Khedkar, H. J. A., Khedkar, D. D. (2020). Effects of silver nanoparticles synthesized from rare medicinal plant Radermacheraxylocarpa(Roxb.) K schumandchlorophyll content on seed germination in brassica juncea and Vignaradiata. Zenodo. Biosci. Biotech. Res. Asia. 7 (2), 89–103. doi: 10.5281/zenodo.4308462
Khodakovskaya, M. V., De Silva, K., Nedosekin, D. A., Dervishi, E., Biris, A. S., Shashkov, E. V., et al. (2010). Complex genetic, photothermal, and photoacoustic analysis of nanoparticle-plant interactions. Proc. Natl. Acad. Sci. 108 (3), 1028–1033. doi: 10.1073/pnas.1008856108
Kim, E., Kim, S.-H., Kim, H.-C., Lee, S. G., Lee, S. J., Jeong, S. W. (2011). Growth inhibition of aquatic plant caused by silver and titanium oxide nanoparticles. Toxicol. Environ. Health Sci. 3, 1–6. doi: 10.1007/s13530-011-0071-8
Kim, S., Lee, S., Lee, I. (2012). Alteration of phytotoxicity and oxidant stress potential by metal oxide nanoparticles in Cucumis sativus. Water Air Soil pollut. 223, 2799–2806. doi: 10.1007/s11270-011-1067-3
Krishnaraj, C., Jagan, E. G., Ramachandran, R., Abirami, S. M., Mohan, N., Kalaichelvan, P. T. (2012). Effect of biologically synthesized silver nanoparticles on bacopa monnieri (Linn.) wettst. plant growth metabolism. Process Biochem. 47 (4), 651–658. doi: 10.1016/j.procbio.2012.01.006
Kumari, M., Pandey, S., Bhattacharya, A., Mishra, A., Nautiyal, C. S. (2017). Protective role of biosynthesized silver nanoparticles against early blight disease in solanum lycopersicum. Plant Physiol. Biochem. 121, 216–225. doi: 10.1016/j.plaphy.2017.11.004
Kumar, P., Pahal, V., Gupta, A., Vadhan, R., Chandra, H., Dubey, R. C. (2020). Effect of silver nanoparticles and bacillus cereus LPR2 on the growth of Zea mays. Sci. Rep. 10, 20409. doi: 10.1038/s41598-020-77460-w
Lakshminarayanan, S., Shereen, M. F., Niraimathi, K. L., Brindha, P., Arumugam, A. (2021). One-pot green synthesis of iron oxide nanoparticles from Bauhinia tomentosa: Characterization and application towards synthesis of 1, 3 diolein. Sci. Rep. 11 (1), 8643. doi: 10.1038/s41598-021-87960-y
Lawre, S., Raskar, S. (2014). Influence of zinc oxide nanoparticles on growth, flowering and seed productivity in onion. Int. J. Curr. Microbiol. 3, 874–881.
Liu, R., Lal, R. (2014). Synthetic apatite nanoparticles as a phosphorus fertilizer for soybean (Glycine max). Sci. Rep. 4, 5686. doi: 10.1038/srep05686
Liu, Q., Zhang, X., Zhao, Y., Lin, J., Shu, C., Wang, C., et al. (2013). Fullerene-induced increase of glycosyl residue on living plant cell wall. Environ. Sci. Technol. 47 (13), 7490–7498. doi: 10.1021/es401022
Lu, X., Sun, D., Zhang, X., Hu, H., Kong, L., Rookes, J. E., et al. (2020). Stimulation of photosynthesis and enhancement of growth and yield in Arabidopsis thaliana treated with amine-functionalized mesoporous silica nanoparticles. Plant Physiol. Biochem. 156, 566–577. doi: 10.1016/j.plaphy.2020.09.036
Mahmoodzadeh, H., Nabavi, M., Kashefi, H. (2015). Effect of nanoscale titanium dioxide particles on the germination and growth of canola (Brassica napus). J. Hortic. Sci. Ornam. Plants. 3, 25–32.
Mandal, S., Marpu, S. B., Hughes, R., Omary, M. A., Shi, S. Q. (2021). Green synthesis of silver nanoparticles using Cannabis sativa extracts and their anti-bacterial activity. Green Sustain. Chem. 11, 38–48. doi: 10.4236/gsc.2021.111004
Manh Cuong, D., Cong Du, P., Tung, H. T., Ngan, H. T. M., Luan, V. Q., Phong, T. H., et al. (2021). Silver nanoparticles as an effective stimulant in micropropagation of Panax vietnamensis–a valuable medicinal plant. Plant Cell Tiss. Organ Cult. 146, 577–588. doi: 10.1007/s11240-021-02095-2
Moghaddasi, S., Fotovat, A., Khoshgoftarmanesh, A. H., Karimzadeh, F., Khazaei, H. R., Khorassani, R. (2017). Bioavailability of coated and uncoated ZnO nanoparticles to cucumber in soil with or without organic matter. Ecotoxicol. Environ. Saf. 144, 543–551. doi: 10.1016/j.ecoenv.2017.06.074
Mohammed, M., Al-Saeedi, A., Elgarawany, M., El-Ramady, H., Alshaal, T., Al-Otaibi, A. (2019). Application of silica nanoparticles induces seed germination and growth of cucumber (Cucumis sativus). Met. Env. Arid. Land. Agric. Sci. 28 (1), 57–68. doi: 10.4197/Met.28-1.6
Mondal, A., Basu, R., Das, S., Nandy, P. (2011). Beneficial role of carbon nanotubes on mustard plant growth: an agricultural prospect. J. Nanopar. Res. 13, 4519. doi: 10.1007/s11051-011-0406-z
Morris, L. A. (2004). Soil biology and tree growth | soil organic matter forms and functions. In Burley, J. (Ed.) Encyclopedia of Forest Sciences. (Oxford: Elsevier) 1201–07.
Morteza, E., Moaveni, P., Farahani, H. A., Kiyani, M. (2013). Study of photosynthetic pigments changes of maize (Zea mays l.) under nano TiO2 spraying at various growth stages. Springer Plus. 2, 247. doi: 10.1186/2193-1801-2-247
Munir, N., Hanif, M., Dias, D. A., Abideen, Z. (2021). The role of halophytic nanoparticles towards the remediation of degraded and saline agricultural lands. Environ. Sci. pollut. Res. 28 (43), 60383–60405. doi: 10.1007/s11356-021-16139-9
Murthy, H. C. A., Desalegn, T., Kassa, M., Abebe, B., Assefa, T. (2020). Synthesis of green copper nanoparticles using medicinal plant Hagenia abyssinica (Brace) JF. gmel. leaf extract: Antimicrobial properties. J. Nanomater., 3924081. doi: 10.1155/2020/3924081
Naika, H. R., Lingaraju, K., Manjunath, K., Kumar, D., Nagaraju, G., Suresh, D., et al. (2015). Green synthesis of CuO nanoparticles using Gloriosa superba l. extract and their antibacterial activity. J. Taibah Univ. Sci. 9, 7–12. doi: 10.1016/j.jtusci.2014.04.006
Najafi Vafa, Z., Sirousmehr, A., Ghanbari, A., Khammari, I., Falahi, N. (2015). Effects of nano zinc and humic acid on quantitative and qualitative characteristics of savory (Satureja hortensis l.). J. Biosci. Biotechnol. Discv. 6 (3), 124–136. doi: 10.12692/ijb/6.3.124-136
Nandini, B., Hariprasad, P., Prakash, H. S., Shetty, H. S., Geetha, N. (2017). Trichogenic-selenium nanoparticles enhance disease suppressive ability of trichoderma against downy mildew disease caused by Sclerospora graminicola in pearl millet. Sci. Rep. 7, 2612–2612. doi: 10.1038/s41598-017-02737-6
Narendra Kumar, H. K., Chandra Mohana, N., Nuthan, B. R., Ramesha, K. P., Rakshith, D., Geetha, N., et al. (2019). Phyto-mediated synthesis of zinc oxide nanoparticles using aqueous plant extract of ocimum americanum and evaluation of its bioactivity. SN. Appl. Sci. 1 (6), 1–9.
Nejatzadeh, F. (2021). Effect of silver nanoparticles on salt tolerance of Satureja hortensis i. during in vitro and in vivo germination tests. Heliyon. 7 (2), e05981. doi: 10.1016/j.heliyon.2021.e05981
Ogunyemi, S. O., Abdallah, Y., Zhang, M., Fouad, H., Hong, X., Ibrahim, E., et al. (2019). Green synthesis of zinc oxide nanoparticles using different plant extracts and their antibacterial activity against xanthomonas oryzae pv. oryzae. Artif. Cells Nanomed. Biotechnol. 47 (1), 341–352. doi: 10.1080/21691401.2018.1557671
Osman, H., Gowayed, S., Okasha, S., Omara, A. E.-D., Sami, R., El-Monem, A., et al. (2021). Minimizing the adversely impacts of water deficit and soil salinity on maize growth and productivity in response to the application of plant growth-promoting rhizobacteria and silica nanoparticles. Agronomy. 11 (4), 676. doi: 10.3390/agronomy11040676
Palithya, S., Gaddam, S. A., Kotakadi, V. S., Penchalaneni, J., Challagundla, V. N. (2021). Biosynthesis of silver nanoparticles using leaf extract of Decaschistia crotonifolia and its antibacterial, antioxidant, and catalytic applications. Green Chem. Lett. Rev. 14 (1), 137–152. doi: 10.1080/17518253.2021.1876172
Panda, S. (2017). Physiological impact of zinc nanoparticle on germination of rice (Oryza sativa l) seed. J. Plant Sci. Phytopathol. 1, 062–070. doi: 10.29328/journal.jpsp.1001008
Pan, Z., Lin, Y., Sarkar, B., Owens, G., Chen, Z. (2020). Green synthesis of iron nanoparticles using red peanut skin extract: Synthesis mechanism, characterization and effect of conditions on chromium removal. J. Colloid. Interface Sci. 558, 106–114. doi: 10.1016/j.jcis.2019.09.106
Paret, M. L., Vallad Ge Fau - Averett, D. R., Averett Dr Fau - Jones, J. B., Jones Jb Fau - Olson, S. M., Olson, S. M. (2013). Photocatalysis: effect of light-activated nanoscale formulations of TiO2 on Xanthomonas perforans and control of bacterial spot of tomato. Phytopathology. 103 (3), 228–236. doi: 10.1094/PHYTO-08-12-0183-R
Perveen, R., Shujaat, S., Qureshi, Z., Nawaz, S., Khan, M. I., Iqbal, M. (2020). Green versus sol-gel synthesis of ZnO nanoparticles and antimicrobial activity evaluation against panel of pathogens. J. Mater. Res. Technol. 9 (4), 7817–7827. doi: 10.1016/j.jmrt.2020.05.004
Pirtarighat, S., Ghannadnia, M., Baghshahi, S. (2019). Green synthesis of silver nanoparticles using the plant extract of salvia spinosa grown in vitro and their antibacterial activity assessment. J. Nanostruct. Chem. 9, 1–9. doi: 10.1007/s40097-018-0291-4
Pourkhaloee, A., Haghighi, M., Saharkhiz, M., Jouzi, H., Mahdi, M. (2011). Carbon nanotubes can promote seed germination via seed coat penetration. Seed. Technol. 33 (2), 155–169.
Prasad, T. N. V. K. V., Sudhakar, P., Sreenivasulu, Y., Latha, P., Munaswamy, V., Reddy, K. R., et al. (2012). Effect of nanoscale zinc oxide particles on the germination, growth and yield of peanut. J. Plant Nutr. 35 (6), 905–927. doi: 10.1080/01904167.2012.663443
Quiterio-Gutiérrez, T., Ortega-Ortiz, H., Cadenas-Pliego, G., Hernández-Fuentes, A. D., Sandoval-Rangel, A., Benavides-Mendoza, A., et al. (2019). The application of selenium and copper nanoparticles modifies the biochemical responses of tomato plants under stress by alternaria solani. Int. J. Mol. Sci. 20 (8), 1950. doi: 10.3390/ijms20081950
Rajput, V., Minkina, T., Mazarji, M., Shende, S., Sushkova, S., Mandzhieva, S., et al. (2020). Accumulation of nanoparticles in the soil-plant systems and their effects on human health. Ann. Agric. Sci. 65 (2), 137–143. doi: 10.1016/j.aoas.2020.08.001
Raliya, R., Nair, R., Chavalmane, S., Wang, W.-N., Biswas, P. (2015). Mechanistic evaluation of translocation and physiological impact of titanium dioxide and zinc oxide nanoparticles on the tomato (Solanum lycopersicum l.) plant. Metallomics. 7 (12), 1584–1594. doi: 10.1039/C5MT00168D
Ramasamy, M. Y. A. M. (2020). Role of fungi in agriculture, biostimulants in plant science. Intech. Open 000.
Rawashdeh, R. Y., Harb, A. M., Alhasan, A. M. (2020). Biological interaction levels of zinc oxide nanoparticles; lettuce seeds as case study. Heliyon. 6 (5), e03983. doi: 10.1016/j.heliyon.2020.e03983
Rizwan, M., Ali, S., Ali, B., Adrees, M., Arshad, M., Hussain, A., et al. (2019). Zinc and iron oxide nanoparticles improved the plant growth and reduced the oxidative stress and cadmium concentration in wheat. Chemosphere. 214, 269–277. doi: 10.1016/j.chemosphere.2018.09.120
Roohizadeh, G., Majd, A., Arbabian, S. (2015). The effect of sodium silicate and silica nanoparticles on seed germination and growth in the vicia faba l. Trop. Plant Res. 2, 85–89.
Sadak, M. S. (2019). Impact of silver nanoparticles on plant growth, some biochemical aspects, and yield of fenugreek plant (Trigonella foenum-graecum). Bull. Natl. Res. Cent. 43 (1), 38. doi: 10.1186/s42269-019-0077-y
Saglam, N., Korkusuz, F., Prasad, R. (2021). Nanotechnology applications in health and environmental sciences. Nurse Educ. Today. 107 (2021), 105098. doi: 10.1007/978-3-030-64410-9
Sathishkumar, R. S., Sundaramanickam, A., Srinath, R., Ramesh, T., Saranya, K., Meena, M., et al. (2019). Green synthesis of silver nanoparticles by bloom forming marine microalgae trichodesmium erythraeum and its applications in antioxidant, drug-resistant bacteria, and cytotoxicity activity. J. Saudi. Chem. Soc 23, 1180–1191. doi: 10.1016/j.jscs.2019.07.008
Sathiyabama, M. A.-O., Manikandan, A. (2018). Application of copper-chitosan nanoparticles stimulate growth and induce resistance in finger millet (Eleusine coracana gaertn.) plants against blast disease. J. Agric. Food Chem. 66 (8), 1784–1790. doi: 10.1021/acs.jafc.7b05921
Sayadi, M. H., Salmani, N., Heidari, A., Rezaei, M. (2018). Bio-synthesis of palladium nanoparticle using Spirulina platensis alga extract and its application as adsorbent. Surf. Interfaces. 10, 136–143. doi: 10.1016/j.surfin.2018.01.002
Schwabe, F., Schulin, R., Rupper, P., Rotzetter, A., Stark, W., Nowack, B. (2014). Dissolution and transformation of cerium oxide nanoparticles in plant growth media. J. Nanopart. Res. 16, 2668. doi: 10.1007/s11051-014-2668-8
Seddighinia, F. S., Iranbakhsh, A., Oraghi Ardebili, Z., Nejad Satari, T., Soleimanpour, S. (2020). Seed priming with cold plasma and multi-walled carbon nanotubes modified growth, tissue differentiation, anatomy, and yield in bitter melon (Momordica charantia). J. Plant Growth Regul. 39, 87–98. doi: 10.1007/s00344-019-09965-2
Shafey, A. M. E. (2020). Green synthesis of metal and metal oxide nanoparticles from plant leaf extracts and their applications: A review. Green Process. Synth. 9 (1), 304–339. doi: 10.1515/gps-2020-0031
Shang, Y., Hasan, M. K., Ahammed, G. J., Li, M., Yin, H., Zhou, J. (2019). Applications of nanotechnology in plant growth and crop protection: A review. Molecules. 24 (14), 2558. doi: 10.3390/molecules24142558
Sheikhalipour, M., Esmaielpour, B., Behnamian, M., Gohari, G. A.-O., Giglou, M. A.-O., Vachova, P. A.-O., et al. (2021). Chitosan-selenium nanoparticle (Cs-Se NP) foliar spray alleviates salt stress in bitter melon. Nanomaterials. 11 (3), 684. doi: 10.3390/nano11030684
Shoemaker, A. G., Wait, D. A. (2020). The effects of titanium dioxide nanoparticles on the growth and development of Sorghum bicolor (L.). moenech. Adv. Agric. Horti. Ento. 2020, AAHE–A132. doi: 10.37722/AAHAE.202052
Siddiqi, K. S., Husen, A. (2016). Green synthesis, characterization and uses of Palladium/Platinum nanoparticles. Nanoscale. Res. Lett. 11 (1), 482. doi: 10.1186/s11671-016-1695-z
Siddiqui, M. H., Al-Whaibi, M. H. (2014). Role of nano-SiO2 in germination of tomato (Lycopersicum esculentum seeds mill.). Saudi. J. Biol. Sci. 21 (1), 13–17. doi: 10.1016/j.sjbs.2013.04.005
Sohail, M. F., Rehman, M., Hussain, S. Z., Huma, Z.-E., Shahnaz, G., Qureshi, O. S., et al. (2020). Green synthesis of zinc oxide nanoparticles by neem extract as multi-facet therapeutic agents. J. Drug Deliv. Sci. Technol. 59, 101911. doi: 10.1016/j.jddst.2020.101911
Song, G., Gao Y Fau - Wu, H., Wu H Fau - Hou, W., Hou W Fau - Zhang, C., Zhang C Fau - Ma, H., Ma, H. (2012). Physiological effect of anatase TiO2 nanoparticles on lemna minor. Environ. Toxicol. Chem. 31 (9), 2147–2152. doi: 10.1002/etc.1933
Stampoulis, D., Sinha, S. K., White, J. C. (2009). Assay-dependent phytotoxicity of nanoparticles to plants. Environ. Sci. Technol. 43 (24), 9473–9479. doi: 10.1021/es901695c
Suciaty, T., Purnomo, D., Sakya, A. T. (2018). The effect of nano-silica fertilizer concentration and rice hull ash doses on soybean (Glycine max (L.) merrill) growth and yield. Earth Environ. Sci. 129, 12009. doi: 10.1088/1755-1315/129/1/012009
Sukumar, S., Rudrasenan, A., Padmanabhan Nambiar, D. (2020). Green-synthesized rice-shaped copper oxide nanoparticles using Caesalpinia bonducella seed extract and their applications. ACS Omega. 5 (2), 1040–1051. doi: 10.1021/acsomega.9b02857
Sunghyun, K., Hyunjoo, S., Sooyeon, L. (2013). Influence of metal oxide particles on soil enzyme activity and bioaccumulation of two plants. J. Microbiol. Biotechnol. 23 (9), 1279–1286. doi: 10.4014/jmb.1304.04084
Sun, L., Wang, Y., Wang, R., Wang, R., Zhang, P., Ju, Q., et al. (2020). Physiological, transcriptomic, and metabolomic analyses reveal zinc oxide nanoparticles modulate plant growth in tomato. Environ. Sci. Nano. 7, 3587–3604. doi: 10.1039/D0EN00723D
Tarafdar, J., Raliya, R., Mahawar, H. (2014). Development of zinc nanofertilizer to enhance crop production in pearl millet (Pennisetum americanum). Agric. Res. 3, 257–262. doi: 10.1007/s40003-014-0113-y
Tian, X., He, W., Cui, J., Zhang, X., Zhou, W., Yan, S., et al. (2010). Mesoporous zirconium phosphate from yeast biotemplate. J. Colloid. Interface Sci. 343 (1), 344–349. doi: 10.1016/j.jcis.2009.11.037
Trujillo-Reyes, J., Majumdar, S., Botez, C. E., Peralta-Videa, J. R., Gardea-Torresdey, J. L. (2014). Exposure studies of core–shell Fe/Fe3O4 and Cu/CuO NPs to lettuce (Lactuca sativa) plants: Are they a potential physiological and nutritional hazard? J. Hazard. Mater. 267, 255–263. doi: 10.1016/j.jhazmat.2013.11.067
Tymoszuk, A., Wojnarowicz, J. (2020). Zinc oxide and zinc oxide nanoparticles impact on In vitro germination and seedling growth in allium cepa l. Materials 13 (12), 2784. doi: 10.3390/ma13122784
Veisi, H., Karmakar, B., Tamoradi, T., Hemmati, S., Hekmati, M., Hamelian, M. (2021). Biosynthesis of CuO nanoparticles using aqueous extract of herbal tea (Stachys lavandulifolia) flowers and evaluation of its catalytic activity. Sci. Rep. 11, 1983. doi: 10.1038/s41598-021-81320-6
Venkatachalam, P., Priyanka, N., Manikandan, K., Ganeshbabu, I., Indiraarulselvi, P., Geetha, N., et al. (2017). Enhanced plant growth promoting role of phycomolecules coated zinc oxide nanoparticles with p supplementation in cotton (Gossypium hirsutum l.). Plant Physiol. Biochem. 110, 118–127. doi: 10.1016/j.plaphy.2016.09.004
Xing, K., Shen, X., Zhu, X., Ju, X., Miao, X., Tian, J., et al. (2021). Synthesis and in vitro antifungal efficacy of oleoyl-chitosan nanoparticles against plant pathogenic fungi. Int. J. Biol. Macromol. 82, 830–836. doi: 10.1016/j.ijbiomac.2015.09.074
Xu, L., Zhu, Z., Sun, D.-W. (2021). Bioinspired nanomodification strategies: Moving from chemical-based agrosystems to sustainable agriculture. ACS Nano. 15 (8), 12655–12686. doi: 10.1021/acsnano.1c03948
Yousefi, S., Kartoolinejad, D., Naghdi, R. (2017). Effects of priming with multi-walled carbon nanotubes on seed physiological characteristics of hopbush (Dodonaeaviscosa l.) under drought stress. J. Environ. Sci. 74 (4), 528–539. doi: 10.1080/00207233.2017.1325627
Zahedi, S. M., Abdelrahman, M., Hosseini, M. S., Hoveizeh, N. F., Tran, L.-S. P. (2019a). Alleviation of the effect of salinity on growth and yield of strawberry by foliar spray of selenium-nanoparticles. Environ. pollut. 253, 246–258. doi: 10.1016/j.envpol.2019.04.078
Zahedi, S., Hosseini, M., Daneshvar Hakimi Meybodi, N., Peijnenburg, W. (2021). Mitigation of the effect of drought on growth and yield of pomegranates by foliar spraying of different sizes of selenium nanoparticles. J. Sci. Food Agric. 101, 5202–5213. doi: 10.1002/jsfa.11167
Zahedi, S. M., Hosseini, M. S., Daneshvar Hakimi Meybodi, N., Teixeira Da Silva, J. A. (2019b). Foliar application of selenium and nano-selenium affects pomegranate (Punica granatum cv. Malase saveh) fruit yield and quality. S. Afr. J. Bot. 24, 350–358. doi: 10.1016/j.sajb.2019.05.019
Zhao, L., Peralta-Videa, Jr, Rico, C. M., Hernandez-Viezcas, J. A., Sun, Y., Niu, G., et al. (2014). CeO₂ and ZnO nanoparticles change the nutritional qualities of cucumber (Cucumis sativus). Agric. Food Chem. 62 (13), 2752–2759. doi: 10.1021/jf405476u
Zheng, L., Hong F Fau - Lu, S., Lu S Fau - Liu, C., Liu, C. (2005). Effect of nano-TiO2 on strength of naturally aged seeds and growth of spinach. Biol. Trace Elem. Res. 104 (1), 83–91. doi: 10.1385/BTER:104:1:083
Zulfiqar, F. (2021). Effect of seed priming on horticultural crops. Scientia. Hortic. 286, 110197. doi: 10.1016/j.scienta.2021.110197
Zulfiqar, F., Ashraf, M. (2021). Nanoparticles potentially mediate salt stress tolerance in plants. Plant Physiol. Biochem. 160, 257–268. doi: 10.1016/j.plaphy.2021.01.028
Zulfiqar, F., Hancock, J. T. (2020). Hydrogen sulfide in horticulture: emerging roles in the era of climate change. Plant Physiol. Biochem. 155, 667–675. doi: 10.1016/j.plaphy.2020.08.010
Zulfiqar, F., Navarro, M., Ashraf, M., Akram, N. A., Munné-Bosch, S. (2019). Nanofertilizer use for sustainable agriculture: Advantages and limitations. Plant Sci. 289, 110270. doi: 10.1016/j.plantsci.2019.110270
Keywords: bio-mediated, engineered nanomaterials (ENMs), agriculture, seed germination, plant growth, sustainable
Citation: Bora KA, Hashmi S, Zulfiqar F, Abideen Z, Ali H, Siddiqui ZS and Siddique KHM (2022) Recent progress in bio-mediated synthesis and applications of engineered nanomaterials for sustainable agriculture. Front. Plant Sci. 13:999505. doi: 10.3389/fpls.2022.999505
Received: 21 July 2022; Accepted: 12 September 2022;
Published: 03 October 2022.
Edited by:
Tasir Sharief Per, Aligarh Muslim University, IndiaReviewed by:
Temoor Ahmed, Zhejiang University, ChinaDalia Gamil Aseel, Arid Lands Cultivation Research Institute (ALCRI), Egypt
Copyright © 2022 Bora, Hashmi, Zulfiqar, Abideen, Ali, Siddiqui and Siddique. This is an open-access article distributed under the terms of the Creative Commons Attribution License (CC BY). The use, distribution or reproduction in other forums is permitted, provided the original author(s) and the copyright owner(s) are credited and that the original publication in this journal is cited, in accordance with accepted academic practice. No use, distribution or reproduction is permitted which does not comply with these terms.
*Correspondence: Faisal Zulfiqar, ch.faisal.zulfiqar@gmail.com; Kadambot H. M. Siddique, kadambot.siddique@uwa.edu.au