- National Citrus Engineering Research Center, Citrus Research Institute, Southwest University, Chongqing, China
Citrus Huanglongbing (HLB), known as the most economically devastating disease in citrus industry, is mainly caused by phloem-restricted Gram-negative bacterium “Candidatus Liberibacter asiaticus” (CLas). To date, CLas is still unculturable in vitro, which has been dramatically delaying the research on its pathogenesis, and only few Sec-dependent effectors (SDEs) have been identified to elucidate the pathogenesis of CLas. Here, we confirmed that a CLas-secreted Sec-dependent polypeptide, namely SECP8 (CLIBASIA_05330), localized in nucleus, cytoplasm and cytoplasmic membrane, and showed remarkably higher transcript abundance in citrus than in psyllids. Potato virus X (PVX)-mediated transient expression assays indicated that mSECP8 (the mature form of SECP8) suppressed pro-apoptotic mouse protein BAX and Phytophthora infestans elicitin INF1-triggered hypersensitive response (HR) associated phenotypes, including cell death, H2O2 accumulation and callose deposition. Intriguingly, mSECP8 also inhibited SDE1 (CLIBASIA_05315)-induced water-soaked and dwarfing symptoms in Nicotiana benthamiana. In addition, mSECP8 can promote the susceptibility of transgenic Wanjincheng orange (Citrus sinensis) to CLas invasion and further HLB symptom development, and it contributes to the proliferation of Xanthomonas citri subsp. citri (Xcc). Moreover, the expression of ten immunity-related genes were significantly down-regulated in mSECP8 transgenic citrus than those in wide-type (WT) plants. Overall, we propose that mSECP8 may serve as a novel broad-spectrum suppressor of plant immunity, and provide the first evidence counteractive effect among CLas effectors. This study will enrich and provide new evidences for elucidating the pathogenic mechanisms of CLas in citrus host.
Introduction
Huanglongbing (HLB, also referred to as citrus greening), is the most economically destructive disease of citrus (Bové, 2006; Wang, 2019). The causative agents of HLB consist of three species, namely “Candidatus Liberibacter asiaticus (CLas),” “Ca. L. africanus (CLaf),” and “Ca. L. americanus (CLam),” which are the Gram-negative bacteria, belonging to the phloem-limited, alpha-proteobacteria (Jagoueix et al., 1994; Teixeira et al., 2005; Bové, 2006). Among them, CLas transmitted by Asian citrus psyllid (ACP, Diaphorina citri), dominantly threats citrus production because of its wide distribution and strong pathogenicity (Coletta-Filho et al., 2004; Haapalainen, 2014).
In general, host plants respond to pathogen infections through two-branched innate immune systems, namely, pathogen-associated molecular patterns (PAMP)-triggered immunity (PTI) and effector-triggered immunity (ETI), generating a “zig-zag” model in plant immune system (Jones and Dangl, 2006; Boller and Felix, 2009; Yuan et al., 2021). Many extracellular plant bacterial pathogens use a type III secretion system (T3SS) to deliver virulence-associated effectors to suppress the plant innate immunity (Yamane et al., 2004; Jones and Dangl, 2006; Wang et al., 2011; Toruño et al., 2016). However, the insect-transmitted and intracellular Gram-negative bacteria, such as CLas, lack T3SS, but can employ a complete Sec-dependent secretion pathway to secret effectors (Duan et al., 2009; Green and Mecsas, 2016; Wang et al., 2017). When invading into plant host cells, CLas induces a series of immune responses, for instance, induction of pathogenesis-related (PR) genes, callose deposition, reactive oxygen species (ROS) burst and enhanced level of salicylic (SA) (Wang, 2019; Pang et al., 2020; Ibanez et al., 2022).
Previous studies have confirmed that many CLas-associated Sec-dependent effectors (SDEs) as virulence factors can suppress or trigger plant immune responses (Jain et al., 2015, 2018; Pitino et al., 2016, 2018; Clark et al., 2018; Liu et al., 2019; Zhang et al., 2019, 2020; Pang et al., 2020; Du et al., 2021; Du J. et al., 2022; Du M. et al., 2022). For instance, non-classical secretory proteins (ncSecPs) SC2_gp095 and Lasbcp (CLIBASIA_RS00445), down-regulation the expression of RbohB gene associated with H2O2-mediated defense signaling in plants (Jain et al., 2015, 2018). m3875 (CLIBASIA_03875) and m4405 (CLIBASIA_04405) function as two hypersensitive response (HR)-based cell death suppressors in Nicotiana benthamiana (Zhang et al., 2019, 2020). SDE15 (CLIBASIA_04025) inhibits plant immunity extensively through interacting with citrus susceptibility gene CsACD2 (ACCELERATED CELL DEATH 2, ACD2). Intriguingly, ten ncSecPs were identified as cell death suppressors that induce the expression of PR-1, PR-2, and PR-5 in N. benthamiana (Du et al., 2021). Systemic acquired resistance (SAR)-related genes were modulated, and CLas colonization was promoted in transgenic citrus by CaLasSDE115 (CLIBASIA_05115) (Du M. et al., 2022). Only a few CLas secretory proteins trigger HR in plants. SDE1 (CLIBASIA_05315) induces strong callose deposition, cell death and starch accumulation in N. benthamiana, and inhibits the activities of defense genes papain-like cysteine proteases (PLCPs) in citrus (Pitino et al., 2016, 2018; Clark et al., 2018). Prophage-encoded ncSecP AGH17470 triggers PR-associated and salicylic acid (SA)-signaling pathway genes expression and then enhances the resistance of citrus to Xanthomonas citri subsp. citri (Xcc) (Du J. et al., 2022).
Candidatus Liberibacter asiaticus encodes a large number of Sec-dependent proteins with unknown functions, and currently only a few has been characterized. In this study, a Sec-dependent protein SECP8 was identified through bioinformatics analyses and E. coli alkaline phosphatase (PhoA) assay. Its function was characterized by transient expression in N. benthamiana and overexpression in Wanjincheng orange (Citrus sinensis). The results reveal that mSECP8 is a virulence factor by suppressing plant immunity to promote CLas invasion.
Materials and methods
Plant materials, microbial strains, and growth conditions
Wild-type (WT) N. benthamiana plants were grown in a growth chamber maintained at 25 ± 2°C and 16 h light/8 h dark photoperiod. Plants at the five-leaf stage were used for infiltration. The transformant Escherichia coli strains Mach-T1 and Rosetta-gami2 (DE3) were grown on Luria-Bertani (LB) medium at 37°C, and Agrobacterium tumefaciens strains GV3101, GV3101 (pJIC SA_Rep) and EHA105 were cultured on LB medium with 50 μg/mL kanamycin and 20 μg/mL rifampicin at 28°C.
Total RNA extraction, cDNA synthesis, and quantitative PCR analysis
The collected leaf samples were stored in −80°C refrigerator. Total RNA was extracted with RNAiso Plus (Takara) reagent and its concentration was quantified. Then 1 μg total RNA was reverse transcribed with All-in-One 5 × RT MasterMix Reagent Kit (ABM) in a 20 μL reaction. The cDNA was diluted five times and then used for quantitative PCR (qPCR) assay in a 10 μL reaction, consisting of 5 μL of Blastaq™ 2 × qPCR MasterMix (ABM), 3.5 μL of DNase/RNase free water, 0.25 μL of each primer (10 μM), and 1 μL of diluted cDNA template. The PCR cycling consisted of an initial activation step at 95°C for 3 min, followed by 40 cycles of 95°C for 15 s and 60°C for 1 min. The CLas gyrA gene (CLIBASIA_00325) and citrus actin gene (XM_006464503.3) were chosen as internal controls. The relative expression assay of genes was performed with three biological and technical replicates, and calculated using the 2–ΔΔCt method (Livak and Schmittgen, 2001). Primers used were detailed (Supplementary Table 1).
DNA extraction and Candidatus Liberibacter asiaticus detection analysis
For CLas detection, total DNA was extracted from the newly emerged CLas-inoculated leaves using the Biospin Omni Plant Genomic DNA Extraction Kit (BioFlux). PCR was performed with 2 × Taq Master Mix (Novoprotein) in a 12 μL reaction. The qPCR ran in a 10 μL reaction, consisting of 5 μL of Blastaq™ 2 × qPCR MasterMix (ABM), 3.5 μL of DNase/RNase free water, 0.25 μL of each primer (10 μM), and 1 μL of DNA (200 ng/μL). The cycling comprised an initial activation step at 95°C for 3 min, followed by 40 cycles of 95°C for 15 s, 60°C for 15 s and 72°C for 15 s.
Bioinformatics analysis
The SECP8 sequence was extracted from the genome of CLas strain Psy62 (GenBank No. CP001677.5) (Duan et al., 2009). Sequences of other CLas strains including AHCA1 (GenBank No. CP029348.1), YNJS7C (GenBank No. QXDO00000000.1), Gxpsy (GenBank No. CP004005.1), A4 (GenBank No. CP010804.1), YCPsy (GenBank No. LIIM00000000.1), JXGC (GenBank No. CP019958.1), and Ishi-1 (GenBank No. AP014595.1) were also downloaded from NCBI1. The signal peptide (SP) and the transmembrane domain were predicted with SignalP 3.0 using neural networks (NN) method (Bendtsen et al., 2004) and TMHMM v2.0 (Krogh et al., 2001), respectively. DNAMAN software was used for sequence alignment.
3,3′-diaminobenzidine trypan-blue and aniline-blue staining assays
For symptoms visualization, the infiltrated leaves were immersed in 95% ethanol (v/v) for 48 h at 37°C until the leaves became clear.
For H2O2 accumulation assay, the infiltrated leaves were sampled and soaked in the 3,3′-Diaminobenzidine (DAB) staining buffer (pH 3.0; 1 mg/mL DAB, 0.05% Tween20 and 10 mM Na2PO4) for 12 h in the dark (Thordal-Christensen et al., 1997; Vanacker et al., 2000). Then the leaves were transferred into boiled bleaching solution (20% acetic acid, 20% glycerin, and 60% ethanol) for discoloration.
For programmed cell death (PCD) observation, the injected leaves were soaked in the trypan-blue staining solution (0.067% w/v trypan-blue, 11.11% w/v phenol, 1 vol of glycerol, 1 vol of lactic acid, 1 vol of double distilled water, 6 vol of 95% ethanol) and boiled for 2 min. Then the samples were transferred into chloral hydrate (1.25 g/mL) and shaken at 50 rpm until leaves were fully de-stained (Heese et al., 2007).
For callose deposition assay, the plant leaves were incubated in solution (acetic/glycerin/ethanol, 1v/1v/3v) overnight until the green color faded away, then washed with 150 mM K2HPO4 for 30 min. Finally, samples were stained with aniline-blue solution (150 mM K2HPO4, 0.05% w/v aniline-blue), and the callose deposition was observed via fluorescence microscope (Sebastian and Adam, 2015).
Agrobacterium-mediated transient expression assay in Nicotiana benthamiana
The coding sequence of mSECP8, SECP8, and SDE1 were amplified with corresponding primers (Supplementary Table 1), and cloned into ClaI/SalI linearized pGR107 vector (a Potato virus X-based expression vector, PVX) to generate experimental recombinant plasmids. These constructs were then transferred into A. tumefaciens GV3101 (pJIC SA_Rep). The PCD suppression assay was performed as described previously (Wang et al., 2011). Briefly, inoculation buffer (10 mM MES, pH 5.6, 10 mM MgCl2, and 100 μM acetosyringone [AS]) or A. tumefaciens cells harboring PVX-SECP8, PVX-mSECP8 and PVX-eGFP (negative control) with OD600 adjusting to 1.0 were firstly infiltrated into the newly expanded leaves of N. benthamiana plants. After 24 h, A. tumefaciens-mediated PVX-BAX, PVX-INF1 or PVX-SDE1 was infiltrated into the same area of leaves. At 48 h post inoculation (hpi), partial infiltrated leaves were used for H2O2 accumulation assay, while the remaining leaves were used for observation of the cell death phenotypes up to 7 days post inoculation (dpi). The suppression effects of SECP8 on SDE1 transient expression plants were evaluated at 15 dpi.
Alkaline phosphatase assay
The signal peptide sequence of SECP8 (SECP8SP) was amplified with the specific primers (Supplementary Table 1) and cloned into pET-mphoA vector. The recombinant plasmids pET-mphoA (negative control), pET-phoA (positive control) and pET-SECP8SP-mphoA were transformed into E. coli Rosetta-gami2 (DE3), then the E. coli cells were grown at 37°C for 12 h on indicator LB solid medium (with 90 μg/mL 5-bromo-4-chloro-3-indolyl phosphate [BCIP], 200 μg/mL isopropyl-β-D-thiogalactopyranoside [IPTG], 75 mM Na2HPO4, and 50 μg/mL Kanamycin) (Liu et al., 2019). Transformants changed to blue were considered with PhoA activity, in contrast to the white transformants without PhoA activity.
Subcellular localization of mSECP8 in Nicotiana benthamiana
The coding sequence of mSECP8 was amplified with specific primers (Supplementary Table 1) and ligated into KpnI/XbaI double-digested pCAMBLIA1300-35S-eGFP vector. The infiltration of the experimental recombinants into N. benthamiana leaves was mediated by A. tumefaciens EHA105, meanwhile, plasma membrane marker pm-rk CD3-1007 (mcherry) (Nelson et al., 2007) and nuclear marker H2B-RFP (Liu et al., 2019) were co-infiltrated. The inoculated leaves were collected after 2 dpi and observed with confocal laser scanning microscopy.
Genetic transformation of Wanjincheng orange (Citrus sinensis)
The coding region of mSECP8 was obtained by PCR with the designated primers (Supplementary Table 1), subsequently inserted into BamHI/EcoRI-digested pCAMBIA-GN-35S-MCS-NOS (pLGN) vector. To obtain the transgenic plants, the epicotyl explants of Wanjincheng orange were used for A. tumefaciens-mediated transformation (Peng et al., 2015; Zou et al., 2017). The successful transgenic lines were identified with both GUS histochemical staining and reverse transcription-PCR (RT-PCR) assays. All transgenic and WT control plants were grafted onto Poncirus trifoliata for following evaluation.
The susceptibility evaluations of transgenic citrus to huanglongbing and canker
Half-year-old transgenic and WT Wanjincheng plants were graft inoculated with CLas positive buds by qPCR verification. The establishment of CLas on transgenic plants was firstly assayed at 40 dpi by both PCR and qPCR (Jagoueix et al., 1996; Li et al., 2006), with the cytochrome oxidase gene of Citrus sinensis as internal control, then detected monthly afterward. All samples were performed with three biological and technical replicates. The primers used were listed in Supplementary Table 1.
To assess the susceptibility of transgenic Wanjincheng plants to Xcc, the in vitro pinprick inoculation was carried out as previously described (Peng et al., 2015, 2017; Zou et al., 2021). Four leaves per transgenic line and WT control were tested, and per leaf contained six infected sites of which each comprised six punctures in 0.5 mm diameter by a needle, 1 μL bacterial Xcc suspension (2.5 × 108 CFU/mL) was subsequently dropped onto each puncture site. Symptom Photographs were taken at 12 dpi, and the lesion sizes were assessed by ImageJ software. For each transgenic lines and WT plants, the disease indexes of 36 punctures per leave were calculated depending on the following rating index: 1, < 0.75 mm2 (lesion size); 2, 0.75–1.25 mm2; 3, 1.25–1.75 mm2; 4, 1.75–2.25 mm2; 5, 2.25–2.75 mm2; 6, 2.75–3.25 mm2; 7, >3.25 mm2. The disease index (DI,%), indicating the level of susceptibility to Xcc, was calculated using the formula (Peng et al., 2017):
At 10 dpi, to count the number of Xcc colonies, the individual disease spots of inoculated leaves were detached using a 5-mm diameter size hole puncher. Three leaf discs were collected together and firstly ground in 200 μL sterile double distilled water (ddH2O), then added another 800 μL ddH2O. The suspension was mixed by 10 times gradient dilution (10–3 to 10–5) and then plated on LB medium. After incubation at 28°C for 48 h, Xcc colonies were counted, and the number of bacterial cells (CFU) per square centimeter of leaf tissue was calculated. Each specific dilution contained three replicates and the test was repeated three times. The bacterial strain Xcc-29-1 used in this examination was provided by Professor Gongyou Chen (School of Agriculture and Biology, Shanghai Jiao Tong University, Shanghai, China).
Results
SECP8 encodes a conserved sec-delivered polypeptide with significantly higher expression level in citrus than in psyllids
In silico analysis of SECP8 (CLIBASIA_05330) indicated that the polypeptide is comprised of 67 amino acids (aa) with an 18-aa predicted signal peptide (namely SECP8SP) in the N-terminal (Figure 1A and Supplementary Figure 1B), and SECP8 was predicted without transmembrane domain (Supplementary Figure 1C). A BLASTn search from the GenBank database revealed that SECP8 encoded a hypothetical protein with 100% similarity to the sequence from all available CLas strains, among them, the SECP8 homologies from eight representative strains were employed for sequence alignments (Supplementary Figure 1A), while the homology of SECP8 could not be found in CLaf and CLam genomes, suggesting SECP8 is unique in CLas strains.
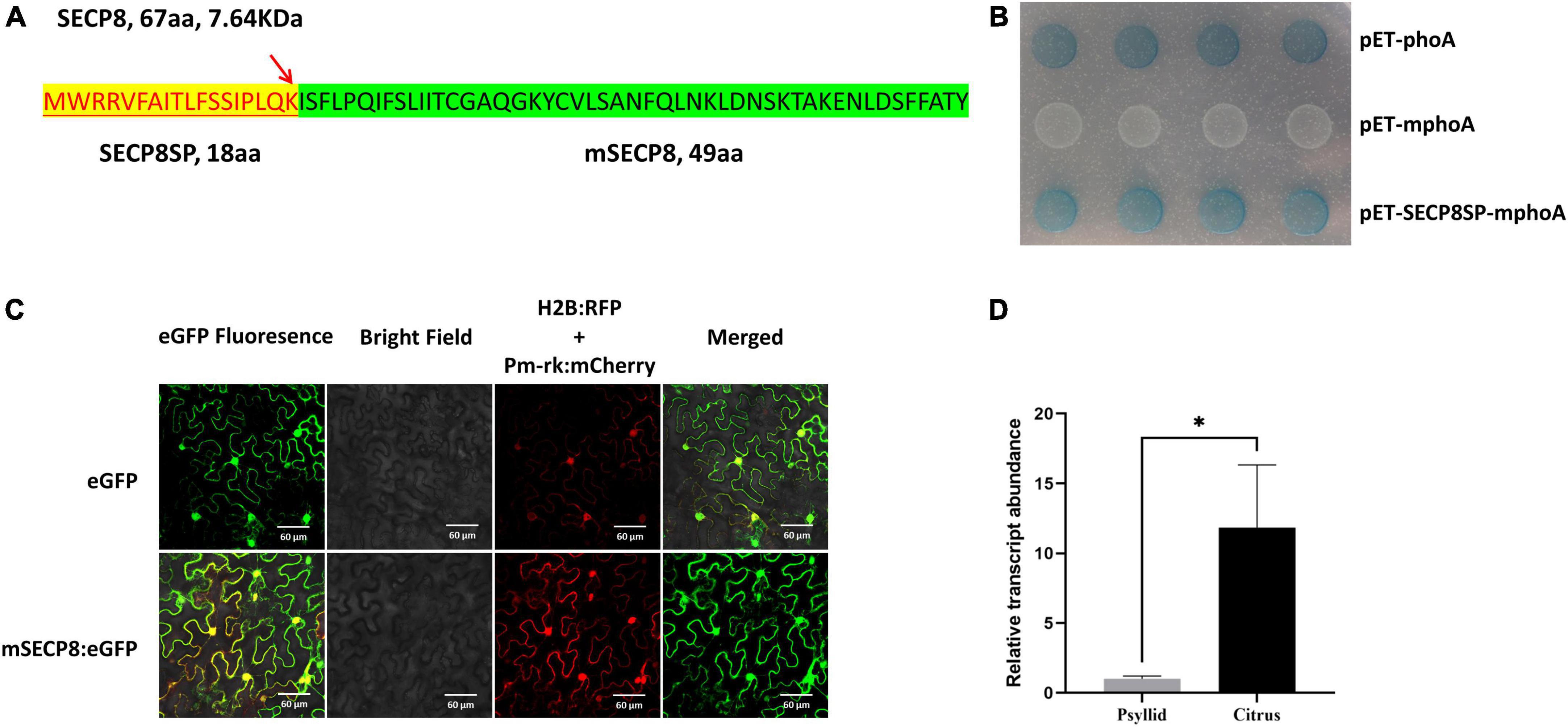
Figure 1. In silico analysis and molecular biological characteristics of SECP8. (A) Analysis of SECP8 amino acid sequence. Amino acid sequence of SECP8 (67 aa, 7.64 KDa) with N-terminal signal peptide (SECP8SP, highlight in yellow), red arrow indicated the cleavage site between 18 and 19 amino acid positions (LQK-IS), SECP8 without the signal peptide SECP8SP was named mSECP8 (49 aa). (B) SECP8 encoded a Sec-delivered protein. PhoA assay was used for validating the secretion of SECP8 in Escherichia coli, E. coli cells harboring the SECP8SP-mPhoA fusion protein turned blue which were similar to PhoA protein (positive control), while negative control mPhoA (without the SP) protein exhibited white on indicator LB medium. It was photographed after 12 h incubation at 37°C. (C) mSECP8 was localized in multiple subcellular compartments of Nicotiana benthamiana. Subcellular localization of mSECP8:eGFP fusion protein was observed via confocal microscopy with the following parameters: eGFP, 488 nm laser wavelength and 510 nm emission wavelength; H2B and Pm-rk, 561 nm laser and 583 nm emission filters. A histone 2B (H2B) fusion with the red fluorescent protein (RFP) and pm-rk CD3-1007 (mcherry) were used as nuclear and plasma membrane location marker, respectively. The samples were collected at 2 dpi. Scale bars represent 60 μm. (D) Relative expression of SECP8 in citrus and psyllids. Transcript abundance of SECP8 was normalized against its expression in Clas-infected Wanjincheng orange and psyllids by RT-qPCR. Bars represented the standard error (SE) of the means, CLasgyrA (CLIBASIA_00325) was used as an internal reference. The asterisks indicate significant difference (*p < 0.05, determined by Student’s t-test). The experiment comprised at least three independent biological replicates and three technical replicates.
Through phoA assay, the E. coli (Rosetta-gami2 DE3 strain) carrying the fusion protein SECP8SP-mphoA turned blue after 12 h of incubation on indicator LB medium as the positive control, while the negative control construct remained white (Figure 1B). Taken together, the phoA assays along with bioinformatics analysis indicated SECP8 as a typical Sec-delivered polypeptide.
To determine the subcellular localization of SECP8, the infiltrated leaves were collected and observed by confocal fluorescence microscopy. Similar to the distribution of eGFP control, the green fluorescence signals of mSECP8 was observed in the nucleus, cytoplasm and cytoplasmic membrane as the membrane and nucleus markers (Figure 1C).
The expression pattern of mSECP8 was further analyzed in CLas-infected citrus and psyllids by reverse transcription-quantitative PCR (RT-qPCR). The transcript level of SECP8 was significantly higher (∼12-fold) in citrus host than in psyllids vectors (Figure 1D), suggesting that SECP8 may play a more important role in planta.
mSECP8 suppresses BAX- and INF1-triggered hypersensitive cell death in Nicotiana benthamiana
The mouse BAX and the P. infestans INF1 are well-known as cell death elicitors and widely applied to identify the PCD suppressor of pathogens (Kamoun et al., 1998; Lacomme and Cruz, 1999). Therefore, the effect of mSECP8 on PCD suppression was evaluated with the strategy. At 7 dpi, both SECP8 and mSECP8 suppressed BAX- and INF1-induced cell death in N. benthamiana, whereas the phenomenon has not been observed in eGFP and buffer infiltration (Figures 2A,B). The result was confirmed by ethanol decolorization and trypan-blue staining assays (Figures 2C,D).
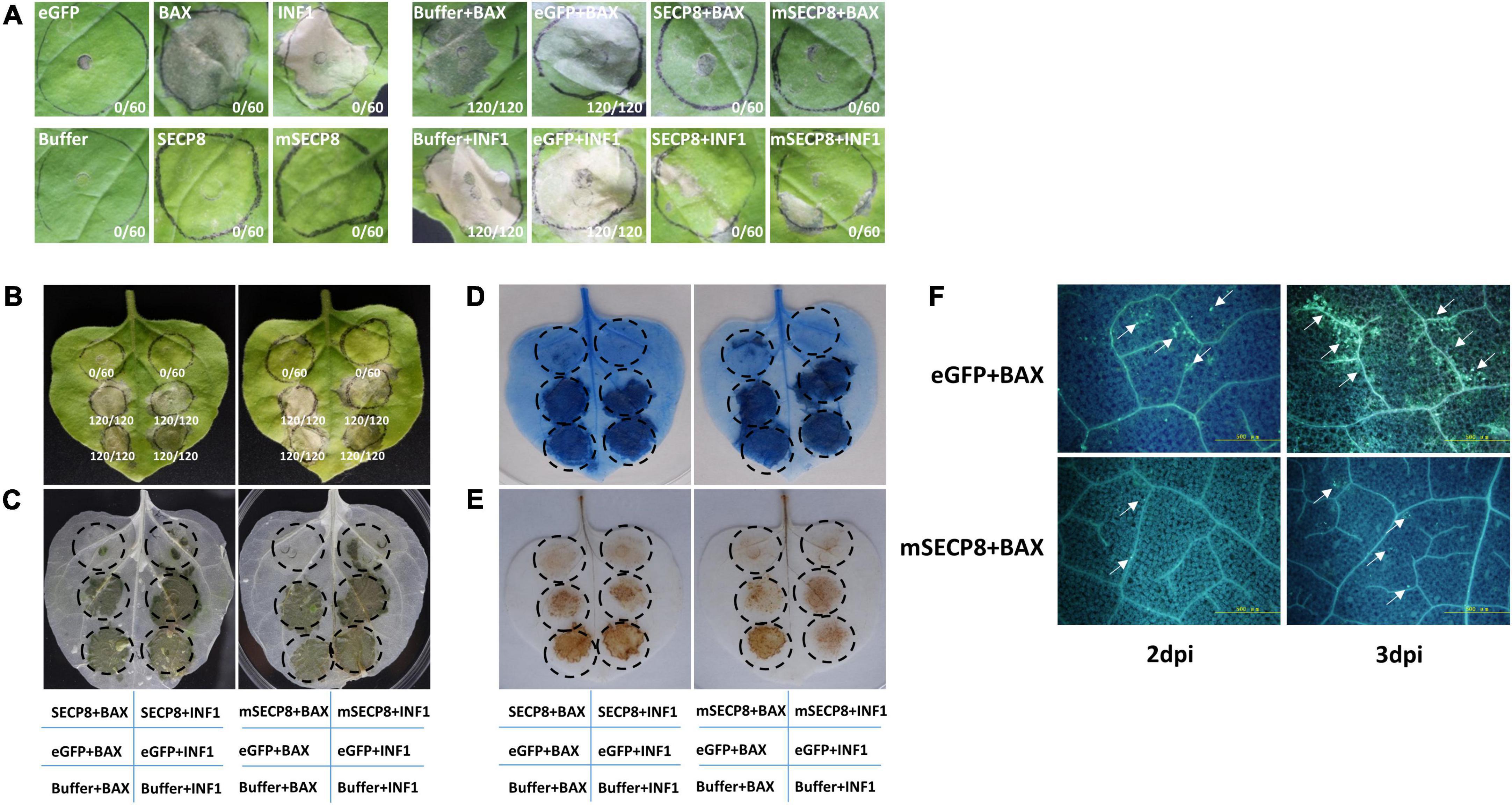
Figure 2. Cell death, H2O2 accumulation and callose deposition suppressed by mSECP8. (A,B) The SECP8 or mSECP8 suppressed the hypersensitive cell death triggered by BAX and INF1 in Nicotiana benthamiana. The Agrobacterium GV3101 (pJIC SA_Rep) strain harboring SECP8, mSECP8, eGFP, BAX, and INF1, or inoculation buffer were infiltrated into the leaves of N. benthamiana, either alone or followed 24 h later with GV3101 carrying BAX or INF1 within the regions marked by the dashed circle. Numbers in the photos, for instance 0/60, indicated that no infiltration spot exhibited cell death out of 60 infiltration spots. The leaves were harvested at 7 days after infiltration BAX and INF1, followed by (C) decolorization with ethanol and (D) trypan-blue staining (blue-stained tissues indicated dead cells). (E) SECP8 or mSECP8 inhibited burst of H2O2 triggered by BAX and INF1. Samples were detached at 2 days after transient expression of BAX and INF1 for 3,3′-diaminobenzidine (DAB) staining. (F) SECP8 or mSECP8 suppressed accumulation of callose caused by BAX. Leaves inoculated with BAX at 2 and 3 days later were sampled for aniline-blue staining. Scale bars represent 500 μm.
To further explore the role of mSECP8 on cell death suppression, the accumulation of H2O2 as a marker of plant cell death was assayed in N. benthamiana leaves by DAB staining. We found that mSECP8 significantly inhibited H2O2 burst triggered by both BAX- and INF1 (Figure 2E). In contrast, neither eGFP nor the infiltration buffer interfered with H2O2 accumulation.
Callose deposition as another indicator during plant immunity responses was examined. At 2∼3 dpi, mSECP8 dramatically reduced BAX-triggered callose deposition compared to eGFP control, particularly at 3 dpi (Figure 2F). Herein, these results clearly demonstrate that SECP8 is a PCD suppressor.
mSECP8 inhibits SDE1 induced water-soaked necrosis and plant dwarfing in Nicotiana benthamiana
Previously, SDE1 (CLIBASIA_05315) was identified as a CLas Sec-dependent effector and induced water-soaked necrosis in N. benthamiana at 4 dpi (Pitino et al., 2016, 2018). Then we examined whether the PCD suppressor SECP8 interferes SDE1 function. We observed that SDE1 induced water-soaked necrosis was present at injection areas at 4 dpi (Figure 3C) and the plants even displayed severe necrosis, dwarfing, leaf curling and shrinking phenotypes at 15 dpi (Figures 3A,B). Similar symptoms were also observed in N. benthamiana infected with eGFP + SDE1 and buffer + SDE1. However, SDE1 induced phenotypes were not observed in plants by co-infiltrated with mSECP8 (Figures 3A,B,D). Additionally, no SDE1-associated phenotypes appeared on eGFP or mSECP8 single infiltration plants (Supplementary Figures 2A,B). Therefore, these results indicate that PCD suppressor mSECP8 inhibits SDE1-mediated necrosis and dwarfing in N. benthamiana.
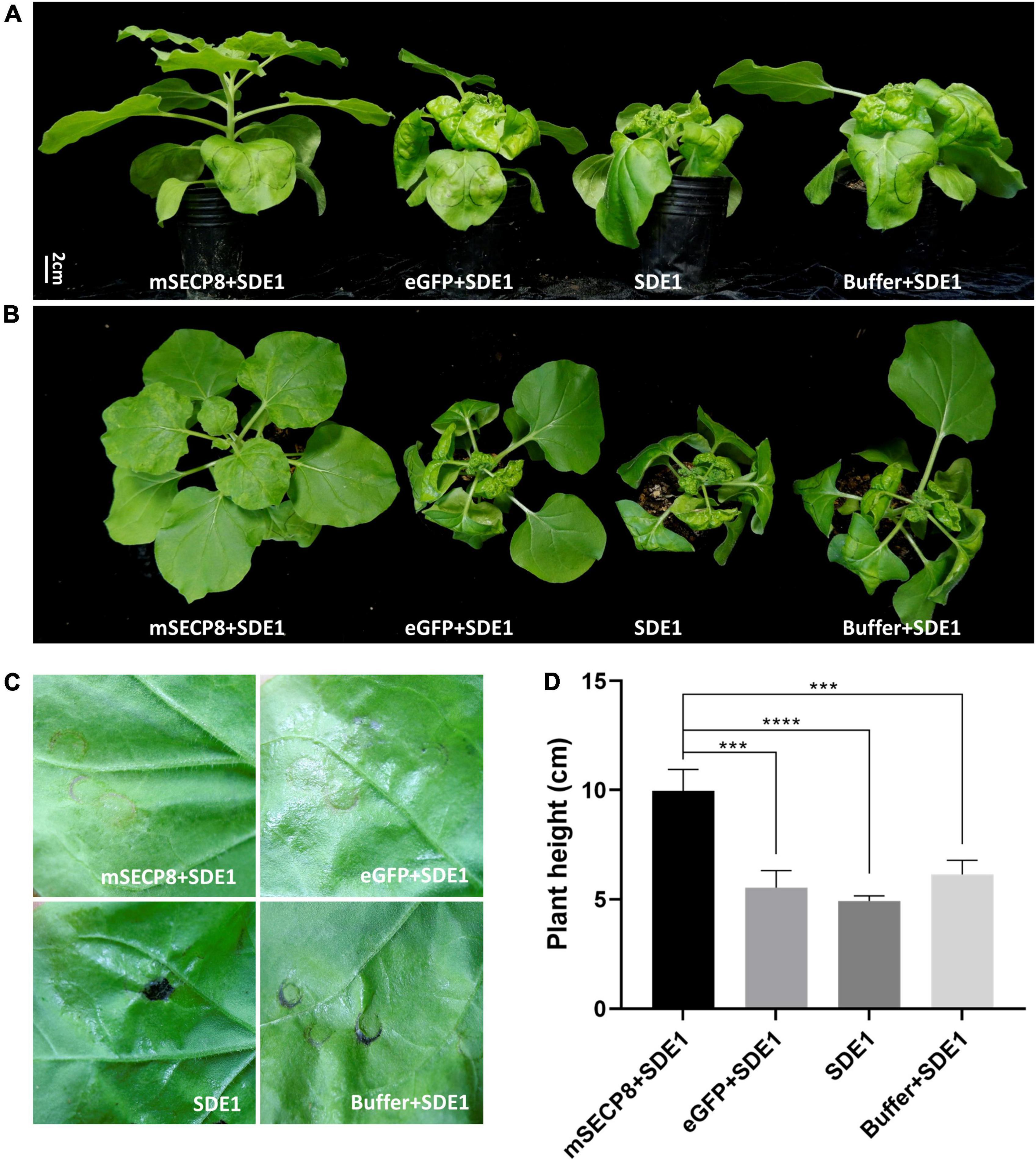
Figure 3. mSECP8 inhibited water-soaked necrosis and dwarfing phenotype induced by SDE1. Nicotiana benthamiana leaves were infiltrated with A. tumefaciens harboring mSECP8, eGFP or with buffer followed 24 h later by Agrobacterium tumefaciens carrying SDE1. (A,B) Photos were taken at 15 days for plant phenotypes and (C) 4 days for inoculated-leaves in N. benthamiana, respectively. (D) The height of N. benthamiana-inoculated plants at 15 dpi. The asterisks indicate significant difference (***p < 0.001, ****p < 0.0001, determined by one-way ANOVA with Dunnett’s test). Scale bar represents 2 cm in (A). The experiment contains at least three independent biological replicates.
mSECP8 enhances the susceptibility of transgenic citrus to both Candidatus Liberibacter asiaticus and Xanthomonas citri subsp. citri
To evaluate the role of mSECP8 on CLas colonization, transgenic citrus plants were generated and six lines (OE-1, OE-4, OE-8, OE-15, OE-17, and OE-25) were verified by both RT-PCR and GUS histochemical staining assay (Figures 4A,B). By comparing to line OE-8, the three lines (OE-1, OE-4, and OE-17) with greater transcript abundance were graft inoculated with CLas for further susceptibility evaluation (Figure 4C). CLas titers in OE-1, OE-4, and OE-17 lines were higher than that of WT plants at 40 dpi, especially at 70 dpi (Figure 4D). Nevertheless, CLas titers in WT plants got close to that in transgenic plants at 100 dpi (Figure 4D). From 70 to 145 dpi, CLas associated chlorosis or mottled yellow symptoms were observed on the leaves of transgenic plants, and these transgenic lines were significantly dwarfing compared with WT controls (Figures 4E–G), while transgenic treatments and WT control showed no phenotype differences without CLas inoculation (Supplementary Figures 3A–C). These results suggest that mSECP8 contributes to CLas early proliferation and symptom development in transgenic citrus plants.
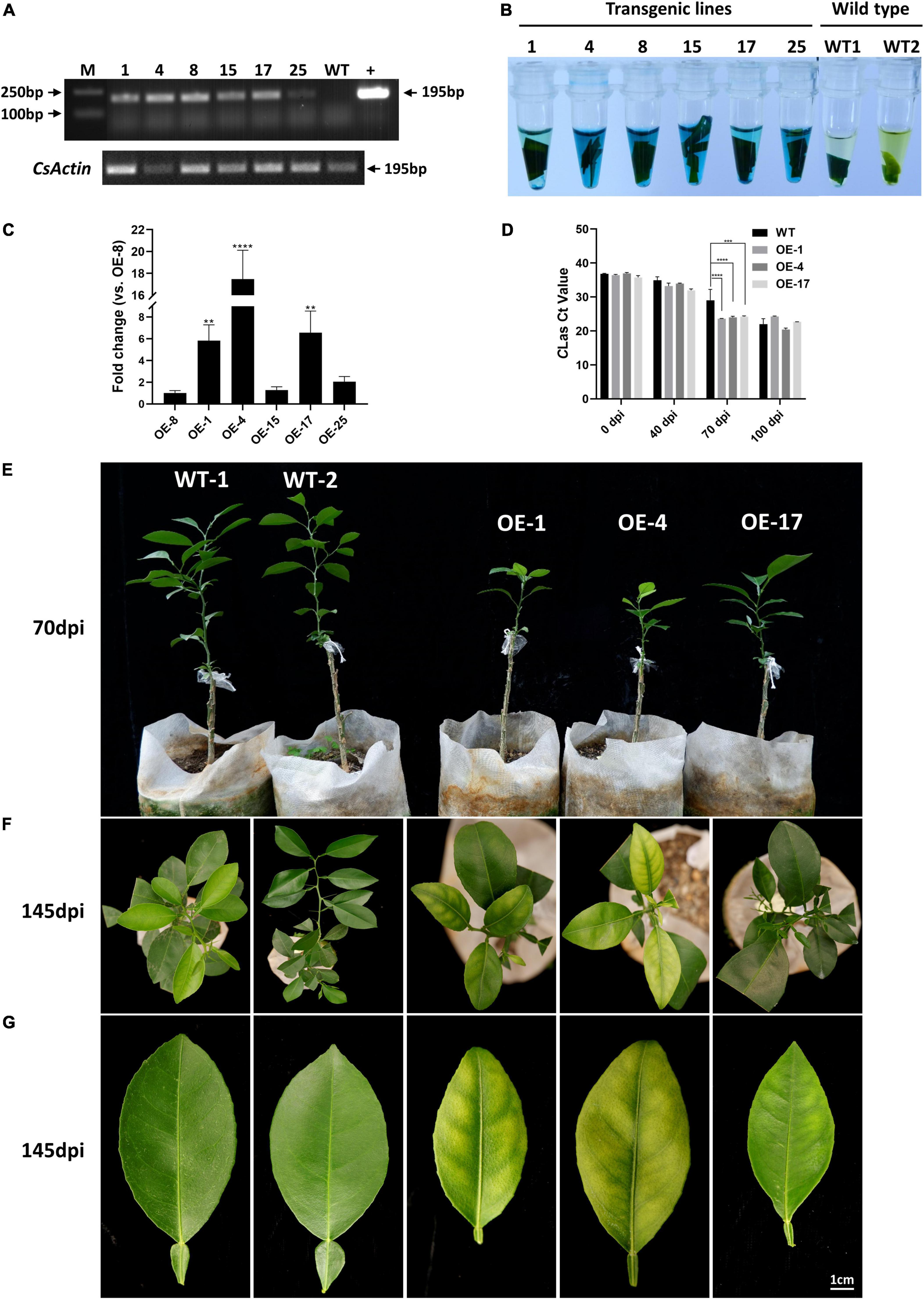
Figure 4. mSECP8-transgenic Wanjincheng orange plants were more susceptible to CLas. (A–C) Identification of mSECP8-transgenic plants. M, DL 2,000 DNA marker; 1, 4, 8, 15, 17, and 25 represented different SECP8-transgenic lines; WT1 and WT2, wild type of Wanjincheng plants; + , pLGN-mSECP8 plasmid. (A) A 195 bp fragment was detected by RT-PCR using pLGN-mSECP8-F/R primer pairs (Supplementary Table 1), the actin gene of citrus sinensis (CsActin) was used as internal reference. (B) Leaves of transgenic plants were used for GUS staining, and the blue-stained indicated positive plants. (C) SECP8 relative expression level in OE-1, OE-4, and OE-15, OE-17, and OE-25 compared with that in OE-8 was determined by RT-qPCR, the CsActin gene was used as internal reference. The asterisks indicate significant difference (**p < 0.01, ****p < 0.0001, determined by one-way ANOVA with Dunnett’s test). (D) The Clas titers in the mSECP8-transgenic citrus and WT control citrus were determined by qPCR at 0, 40, 70, and 100 dpi with CLas, the cytochrome oxidase gene of citrus sinensis (CsCOX) was chosen as internal control. Each Ct value was represented by means ± SE (nOE–mSECP8 = 3, nWT = 3). Asterisks represent significant differences in the Clas titer of 0, 40, 70, and 100 dpi between mSECP8-transgenic citrus and WT control by two-way ANOVA with Tukey’s test (***P < 0.001, and ****P < 0.0001). (E) Plants phenotype obtained at 70 dpi with Clas via branch and budding grafting. (F) Top view of CLas-infected WT and transgenic Wanjincheng plants at 145 dpi, and (G) symptoms of single leave detached from the contemporaneous plants. Scale bar represents 1 cm.
To observe whether mSECP8 enhanced susceptibility of transgenic plants to other pathogens, transgenic citrus leaves were inoculated using Xcc. Compared to WT plant, the lesion size (LZ) increased by 147.3∼289.6% (Supplementary Figure 4A), and the disease index (DI) increased from 22.9 to 53.2∼81.8% (Supplementary Figure 4B), and significantly more colony-forming units (CFUs) were observed in transgenic plants (Supplementary Figure 4C). Furthermore, leaves of Xcc-inoculated mSECP8-overexpressing plants exhibited remarkably earlier and larger pustule eruptions than those of control plants (Supplementary Figure 4D).
The expression level of the defense-related genes are remarkably decreased in mSECP8-transgenic citrus
To further investigate how mSECP8 interferes with plant defense responses, the expression of 10 immunity-related genes were analyzed. The results showed that the expression level of all the SAR-related (CsPR1, CsPR2, CsPR3, and CsPR5) and PTI marker (CsFRK1, CsGST1, CsWRKY22, and CsWRKY29) genes were significantly reduced in transgenic plants (Figure 5). After challenging with CLas, similar results were exhibited in OE-mSECP8 plants (Supplementary Figure 5), indicating that mSECP8 may suppress the plants defense responses through interrupting SAR- and PTI-mediated pathways.
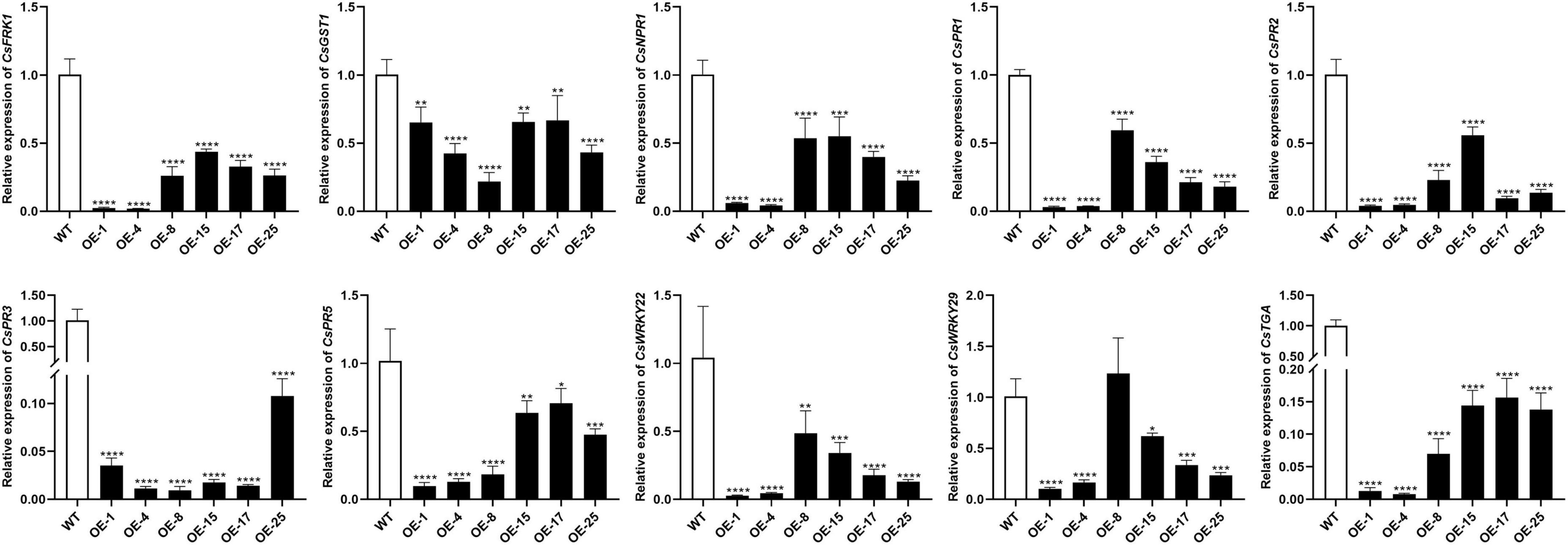
Figure 5. mSECP8 down-regulated the expression of defense-related genes in transgenic citrus. Transcript level of CsFRK1, CsGST1, CsNPR1, CsPR1, CsPR2, CsPR3, CsPR5, CsWRKY22, CsWRKY29, and CsTGA were significantly lower in mSECP8-transgenic than that in WT Wanjincheng plants. The CsActin gene was used as an endogenous control. The asterisks indicate significant difference (*P < 0.05, **p < 0.01, ***P < 0.001 and ****P < 0.0001, one-way ANOVA with Dunnett’s test). The experiment comprised at least three independent biological replicates and three technical replicates.
Discussion
In the host-pathogen arms race, on one hand, to prevent the spread of pathogen, host plants defense against pathogens by PTI and ETI with HR-associated PCD; on the other hand, to invade the host plants successfully, pathogens including bacteria, fungi and oomycetes evolve various effectors as virulence factors to suppress PTI or even ETI (Nimchuk et al., 2003; Jones and Dangl, 2006; Coll et al., 2011; Nguyen et al., 2021; Yuan et al., 2021). HR suppression of plant immune system plays a leading role in pathogenic virulence strategy. As is well known, numerous pathogens including bacteria, fungi, and oomycetes colonize extracellularly and suppress host HR by T3SS-secreted or Sec pathway-dependent effectors (Boller and Felix, 2009; Segers and Anné, 2011; Rocafort et al., 2020; Yuan et al., 2021).
PCD plays critical roles in plant immunity upon pathogen infection at early stage (Coll et al., 2011). By contrast, the pathogens secrete effectors to suppress cell death to facilitate their successful infection. CLas encodes a large number of Sec-dependent secretory proteins with unknown functions and a few have been characterized, but with only three, SDE1, m460, and AGH17470 identified as PCD elicitors, while others functioning as suppressors (Pitino et al., 2016, 2018; Liu et al., 2019; Du J. et al., 2022). In our study, mSECP8 was also identified as a suppressor by suppressing BAX- and INF-triggered PCD syndromes. It is noteworthy that mSECP8 interfered with SDE1-mediated water-soaked necrosis, the plant dwarfing and flowering delay (data not shown) in N. benthamiana. Previously, CLas effector SDE15 was verified as a broad-spectrum suppressor to inhibit PCD elicited by XccAw and Xanthomonas vesicatoria effector AvrBsT (Pang et al., 2020). Both SDE1 and mSECP8 play critical roles in citrus host and potentially target multiple host proteins due to their higher mRNA transcriptional levels in citrus than in psyllids with diverse subcellular localization. SDE1 is systematically distributed and has been demonstrated to interact with host PLCP proteins in reducing target activity to promote CLas infection (Clark et al., 2018). Therefore, mSECP8 is speculated as a potential broad-spectrum suppressor of plant immunity, and potentially interacting with CLas elicitor SDE1 to co-manipulate plant immune responses. The counteractive effect of the two effectors could assist CLas escaping from host immunity, resulting in long latency and slowly developed symptoms of HLB. However, the molecular mechanisms of how mSECP8 interfering with SDE1 remains to be determined.
Systemic acquired resistance is highly associated with plant defense against bacterial pathogens (Durrant and Dong, 2004). In CLas-citrus interaction system, the expression of SAR-related genes (CsPR1, CsPR2, CsPR3, and CsPR5) and PTI marker genes (CsFRK1, CsGST1, CsWRKY22, and CsWRKY29) were remarkably down-regulated in transgenic plants expressing SDE15 by challenged with XccAw. Similarly, the transcript abundance of WRKY and PR genes was reduced in mSDE115-overexpressing citrus (Pang et al., 2020; Du M. et al., 2022). Whereas the expression of PR- and SA-related genes were significantly induced in AGH17470-transgenic citrus (Du J. et al., 2022). Intriguingly, 10 non-classic SecPs triggered the overexpression of NbPR1, NbPR2, and NbPR5 genes (Du et al., 2021). Herein, mSECP8 potentially promoted CLas infection via repression of SAR- and PTI-mediated defenses, but the detailed mechanisms of how mSECP8 suppress plant immunity remains to be addressed through finding its host interacting targets.
Conclusively, we identified the CLas-secreted Sec-dependent effector SECP8 as a broad-spectrum suppressor by inhibiting elicitor-triggered PCD in N. benthamiana and SDE1-induced phenotypes, and thereby provide the first evidence of counteractive effect of CLas suppressors and elicitors. Through susceptibility assessment of transgenic plants to CLas and Xcc, it revealed that mSECP8 may be involved in suppression of the plant immunity through manipulating SAR- and PTI-mediated defenses to facilitate pathogen infection. The present findings illustrate the role of SECP8 in pathogenicity of CLas and may shed new insight in CLas-citrus interactions.
Data availability statement
The datasets presented in this study can be found in online repositories. The names of the repository/repositories and accession number(s) can be found in the article/Supplementary material.
Author contributions
PS, XW, and CZ designed the experiments. PS and XL performed the experiments. PS analyzed the data and wrote the manuscript. SF, XW, and CZ revised and polished the manuscript. All authors contributed to the article and approved the submitted version.
Funding
This work was supported by the grants from the National Key Research and Development Program of China (2021YFD1400800), the National Natural Sciences Foundation of China (31871925), and Innovation Research 2035 Pilot Plan of Southwest University (SWU-5331000008).
Acknowledgments
We thank Professor Weimin Li (College of Bioscience and Resources Environment, Beijing University of Agriculture, Beijing, China) for providing pET-mphoA, pET-phoA, PVX plasmids together with A. tumefaciens cells harboring PVX-BAX and PVX-INF1 constructs, and Professor Gongyou Chen (School of Agriculture and Biology, Shanghai Jiao Tong University, Shanghai, China) for providing Xcc-29-1 bacterial strain.
Conflict of interest
The authors declare that the research was conducted in the absence of any commercial or financial relationships that could be construed as a potential conflict of interest.
Publisher’s note
All claims expressed in this article are solely those of the authors and do not necessarily represent those of their affiliated organizations, or those of the publisher, the editors and the reviewers. Any product that may be evaluated in this article, or claim that may be made by its manufacturer, is not guaranteed or endorsed by the publisher.
Supplementary material
The Supplementary Material for this article can be found online at: https://www.frontiersin.org/articles/10.3389/fpls.2022.997825/full#supplementary-material
Supplementary Figure 1 | Bioinformatics features of SECP8. (A) The nucleotide sequence alignments among SECP8 (CLIBASIA_05330) and the homologies of other CLas typical strains from United States, China, and Japan. (B) SP and (C) transmembrane domain prediction of SECP8.
Supplementary Figure 2 | No plant phenotype differences were induced by eGFP or mSECP8. (A,B) Photos were taken at 15 dpi, and scale bar represents 2 cm in (A).
Supplementary Figure 3 | Phenotype comparison between WT and overexpressing (OE)-mSECP8 citrus plants. (A–C) One-year old plants and corresponding detached leaves were exhibited. Scale bar represents 1 cm in (C).
Supplementary Figure 4 | mSECP8-transgenic Wanjincheng orange plants were more susceptible to Xcc. (A–C) The susceptibility evaluation of mSECP8-transgenic plants to Xcc. (A) Lesion size (mm2) was analyzed using imageJ software, followed by the calculation of (B) disease index (DI,%) and (C) colony numbers (CFU/cm2). Each value was represented by means ± SE (nOE–mSECP8 = 3, nWT = 3). Asterisks represent significant differences between mSECP8-transgenic lines and WT control by one-way ANOVA with Dunnett’s test (****P < 0.0001). CFU, colony-forming units. (D) At 12 dpi, disease spots were exhibited after inoculation with Xcc cells at a concentration of 2.5×108 CFU/mL using pinprick inoculation method, scale bar represents 1 cm. The experiment comprised at least three independent biological replicates and three technical replicates.
Supplementary Figure 5 | mSECP8 reduced the transcript abundance of immunity-associated genes in CLas-infected transgenic citrus. Expression level of CsFRK1, CsGST1, CsPR1, CsPR2, CsPR3, CsPR5, CsWRKY22, CsWRKY29, and CsTGA were significantly decreased in mSECP8-overexpressing plants. The CsActin was chosen as an endogenous reference. The asterisks indicate significant difference (*P < 0.05, **p < 0.01, one-way ANOVA with Dunnett’s test). The experiment comprised at least three independent biological replicates and three technical replicates.
Supplementary Table 1 | Primers used in this study.
Footnotes
References
Bendtsen, J. D., Nielsen, H., von Heijne, G., and Brunak, S. (2004). Improved prediction of signal peptides: SignalP 3.0. J. Mol. Biol. 340, 783–795. doi: 10.1016/j.jmb.2004.05.028
Boller, T., and Felix, G. (2009). A renaissance of elicitors: Perception of microbe-associated molecular patterns and danger signals by pattern-recognition receptors. Annu. Rev. Plant Biol. 60, 379–406. doi: 10.1146/annurev.arplant.57.032905.105346
Bové, J. M. (2006). Huanglongbing: A destructive, newly-emerging, century-old disease of citrus. J. Plant Pathol. 88, 7–37. doi: 10.4454/jpp.v88i1.828
Clark, K., Franco, J. Y., Schwizer, S., Pang, Z., Hawara, E., Liebrand, T. W. H., et al. (2018). An effector from the huanglongbing-associated pathogen targets citrus proteases. Nat. Commun. 9:1718. doi: 10.1038/s41467-018-04140-9
Coletta-Filho, H. D., Targon, M. L. P. N., Takita, M. A., De Negri, J. D., Pompeu, J., Machado, M. A., et al. (2004). First report of the causal agent of huanglongbing (“candidatus liberibacter asiaticus”) in Brazil. Plant Dis. 88, 1382–1382. doi: 10.1094/Pdis.2004.88.12.1382c
Coll, N. S., Epple, P., and Dangl, J. L. (2011). Programmed cell death in the plant immune system. Cell Death Differ. 18, 1247–1256. doi: 10.1038/cdd.2011.37
Du, J., Wang, Q., Zeng, C., Zhou, C., and Wang, X. (2022). A prophage-encoded nonclassical secretory protein of “Candidatus Liberibacter asiaticus” induces a strong immune response in Nicotiana benthamiana and citrus. Mol. Plant Pathol. 23, 1022–1034. doi: 10.1111/mpp.13206
Du, M., Wang, S., Dong, L., Qu, R., Zheng, L., He, Y., et al. (2022). Overexpression of a “Candidatus Liberibacter Asiaticus” effector gene CaLasSDE115 contributes to early colonization in Citrus sinensis. Front. Microbiol. 12:797841. doi: 10.3389/fmicb.2021.797841
Du, P., Zhang, C., Zou, X., Zhu, Z., Yan, H., Wuriyanghan, H., et al. (2021). “Candidatus Liberibacter asiaticus” secretes nonclassically secreted proteins that suppress host hypersensitive cell death and induce expression of plant pathogenesis-related proteins. Appl. Environ. Microbiol. 87:e00019–e00021. doi: 10.1128/AEM.00019-21
Duan, Y., Zhou, L., Hall, D. G., Li, W., Doddapaneni, H., Lin, H., et al. (2009). Complete genome sequence of citrus huanglongbing bacterium, ‘Candidatus Liberibacter asiaticus’ obtained through metagenomics. Mol. Plant Microbe Interact. 22, 1011–1020. doi: 10.1094/MPMI-22-8-1011
Durrant, W. E., and Dong, X. (2004). Systemic acquired resistance. Annu. Rev. Phytopathol. 42, 185–209. doi: 10.1146/annurev.phyto.42.040803.140421
Green, E. R., and Mecsas, J. (2016). Bacterial secretion systems - an overview. Microbiol. Spectr. 4, 4.4.13. doi: 10.1128/microbiolspec.VMBF-0012-2015
Haapalainen, M. (2014). Biology and epidemics of Candidatus Liberibacter species, psyllid-transmitted plant-pathogenic bacteria. Ann. Appl. Biol. 165, 172–198. doi: 10.1111/aab.12149
Heese, A., Hann, D. R., Gimenez-Ibanez, S., Jones, A. M. E., He, K., Li, J., et al. (2007). The receptor-like kinase SERK3/BAK1 is a central regulator of innate immunity in plants. Proc. Natl. Acad. Sci. U.S.A. 104, 12217–12222. doi: 10.1073/pnas.0705306104
Ibanez, F., Suh, J. H., Wang, Y., Rivera, M., Setamou, M., and Stelinski, L. L. (2022). Salicylic acid mediated immune response of Citrus sinensis to varying frequencies of herbivory and pathogen inoculation. BMC Plant Biol. 22:7. doi: 10.1186/s12870-021-03389-5
Jagoueix, S., Bove, J. M., and Garnier, M. (1994). The phloem-limited bacterium of greening disease of citrus is a member of the alpha-subdivision of the Proteobacteria. Int. J. Syst. Bacteriol. 44, 379–386. doi: 10.1099/00207713-44-3-379
Jagoueix, S., Bové, J. M., and Garnier, M. (1996). PCR detection of the two ‘Candidatus’ liberobacter species associated with greening disease of citrus. Mol. Cell. Probes 10, 43–50. doi: 10.1006/mcpr.1996.0006
Jain, M., Fleites, L. A., and Gabriel, D. W. (2015). Prophage-encoded peroxidase in ‘Candidatus Liberibacter asiaticus’ is a secreted effector that suppresses plant defenses. Mol. Plant Microbe Interact. 28, 1330–1337. doi: 10.1094/Mpmi-07-15-0145-R
Jain, M., Munoz-Bodnar, A., Zhang, S., and Gabriel, D. W. (2018). A secreted ‘Candidatus Liberibacter asiaticus’ peroxiredoxin simultaneously suppresses both localized and systemic innate immune responses in planta. Mol. Plant Microbe Interact. 31, 1312–1322. doi: 10.1094/Mpmi-03-18-0068-R
Jones, J. D. G., and Dangl, J. L. (2006). The plant immune system. Nature 444, 323–329. doi: 10.1038/nature05286
Kamoun, S., West, P. V., Vleeshouwers, V. G. A. A., Groot, K. E. D., and Govers, F. (1998). Resistance of Nicotiana benthamiana to Phytophthora infestans is mediated by the recognition of the elicitor protein INF1. Plant Cell 10, 1413–1425. doi: 10.1105/tpc.10.9.1413
Krogh, A., Larsson, B., Heijne, G. V., and Sonnhammer, E. L. L. (2001). Predicting transmembrane protein topology with a Hidden Markov Model: Application to complete genomes. J. Mol. Biol. 305, 567–580. doi: 10.1006/jmbi.2000.4315
Lacomme, C., and Cruz, S. S. (1999). Bax-induced cell death in tobacco is similar to the hypersensitive response. Proc. Natl. Acad. Sci. U.S.A. 96, 7956–7961. doi: 10.1073/pnas.96.14.7956
Li, W., Hartung, J. S., and Levy, L. (2006). Quantitative real-time PCR for detection and identification of Candidatus Liberibacter species associated with citrus huanglongbing. J. Microbiol. Methods 66, 104–115. doi: 10.1016/j.mimet.2005.10.018
Liu, X., Fan, Y., Zhang, C., Dai, M., Wang, X., and Li, W. (2019). Nuclear import of a secreted “Candidatus Liberibacter asiaticus” protein is temperature dependent and contributes to pathogenicity in Nicotiana benthamiana. Front. Microbiol. 10:1684. doi: 10.3389/fmicb.2019.01684
Livak, K. J., and Schmittgen, T. D. (2001). Analysis of relative gene expression data using real-time quantitative PCR and the 2(T)(-Delta Delta C) method. Methods 25, 402–408. doi: 10.1006/meth.2001.1262
Nelson, B. K., Cai, X., and Nebenführ, A. (2007). A multicolored set of in vivo organelle markers for co-localization studies in Arabidopsis and other plants. Plant J. 51, 1126–1136. doi: 10.1111/j.1365-313X.2007.03212.x
Nguyen, Q.-M., Iswanto, A. B. B., Son, G. H., and Kim, S. H. (2021). Recent advances in effector-triggered immunity in plants: New pieces in the puzzle create a different paradigm. Int. J. Mol. Sci. 22:4709. doi: 10.3390/ijms22094709
Nimchuk, Z., Eulgem, T., Holt, B. F. III., and Dangl, J. L. (2003). Recognition and response in the plant immune system. Annu. Rev. Genet. 37, 579–609. doi: 10.1146/annurev.genet.37.110801.142628
Pang, Z., Zhang, L., Coaker, G., Ma, W., He, S. Y., and Wang, N. (2020). Citrus CsACD2 Is a target of Candidatus Liberibacter Asiaticus in huanglongbing disease. Plant Physiol. 184, 792–805. doi: 10.1104/pp.20.00348
Peng, A., Chen, S., Lei, T., Xu, L., He, Y., Wu, L., et al. (2017). Engineering canker-resistant plants through CRISPR/Cas9-targeted editing of the susceptibility gene CsLOB1 promoter in citrus. Plant Biotechnol. J. 15, 1509–1519. doi: 10.1111/pbi.12733
Peng, A., Xu, L., He, Y., Lei, T., Yao, L., Chen, S., et al. (2015). Efficient production of marker-free transgenic ‘Tarocco’ blood orange (Citrus sinensis Osbeck) with enhanced resistance to citrus canker using a Cre/loxP site-recombination system. Plant Cell Tissue Organ Cult. 123, 1–13. doi: 10.1007/s11240-015-0799-y
Pitino, M., Allen, V., and Duan, Y. (2018). Las delta 5315 effector induces extreme starch accumulation and chlorosis as Ca. Liberibacter asiaticus infection in Nicotiana benthamiana. Front. Plant Sci. 9:113. doi: 10.3389/fpls.2018.00113
Pitino, M., Armstrong, C. M., Cano, L. M., and Duan, Y. (2016). Transient expression of Candidatus Liberibacter Asiaticus effector induces cell death in Nicotiana benthamiana. Front. Plant Sci. 7:982. doi: 10.3389/fpls.2016.00982
Rocafort, M., Fudal, I., and Mesarich, C. H. (2020). Apoplastic effector proteins of plant-associated fungi and oomycetes. Curr. Opin. Plant Biol. 56, 9–19. doi: 10.1016/j.pbi.2020.02.004
Sebastian, T. S., and Adam, S. (2015). Staining of callose depositions in root and leaf tissues. Bio Protocol. 5:e1429. doi: 10.21769/BioProtoc.1429
Segers, K., and Anné, J. (2011). Traffic jam at the bacterial sec translocase: Targeting the SecA nanomotor by small-molecule inhibitors. Chem. Biol. 18, 685–698. doi: 10.1016/j.chembiol.2011.04.007
Teixeira, D. D. C., Saillard, C., Eveillard, S., Danet, J. L., Costa, P. I. D., Ayres, A. J., et al. (2005). ‘Candidatus Liberibacter americanus’, associated with citrus huanglongbing (greening disease) in São Paulo State, Brazil. Int. J. Syst. Evol. Microbiol. 55, 1857–1862. doi: 10.1099/ijs.0.63677-0
Thordal-Christensen, H., Zhang, Z., Wei, Y., and Collinge, D. B. (1997). Subcellular localization of H2O2 in plants. H2O2 accumulation in papillae and hypersensitive response during the barley-powdery mildew interaction. Plant J. 11, 1187–1194. doi: 10.1046/j.1365-313X.1997.11061187.x
Toruño, T. Y., Stergiopoulos, I., and Coaker, G. (2016). Plant-pathogen effectors: Cellular probes interfering with plant defenses in spatial and temporal manners. Annu. Rev. Phytopathol. 54, 419–441. doi: 10.1146/annurev-phyto-080615-100204
Vanacker, H., Carver, T. L. W., and Foyer, C. H. (2000). Early H2O2 accumulation in mesophyll cells leads to induction of glutathione during the hyper-sensitive response in the barley-powdery mildew interaction. Plant Physiol. 123, 1289–1300. doi: 10.1104/pp.123.4.1289
Wang, N. (2019). The citrus huanglongbing crisis and potential solutions. Mol. Plant 12, 607–609. doi: 10.1016/j.molp.2019.03.008
Wang, N., Pierson, E. A., Setubal, J. C., Xu, J., Levy, J. G., Zhang, Y., et al. (2017). The Candidatus Liberibacter-host interface: Insights into pathogenesis mechanisms and disease control. Annu. Rev. Phytopathol. 55, 451–482. doi: 10.1146/annurev-phyto-080516-035513
Wang, Q., Han, C., Ferreira, A. O., Yu, X., Ye, W., Tripathy, S., et al. (2011). Transcriptional programming and functional interactions within the Phytophthora sojae RXLR effector repertoire. Plant Cell. 23, 2064–2086. doi: 10.1105/tpc.111.086082
Yamane, K., Bunai, K., and Kakeshita, H. (2004). Protein traffic for secretion and related machinery of Bacillus subtilis. Biosci. Biotechnol. Biochem. 68, 2007–2023. doi: 10.1271/bbb.68.2007
Yuan, M., Ngou, B. P. M., Ding, P., and Xin, X. F. (2021). PTI-ETI crosstalk: An integrative view of plant immunity. Curr. Opin. Plant Biol. 62:102030. doi: 10.1016/j.pbi.2021.102030
Zhang, C., Du, P., Yan, H., Zhu, Z., Wang, X., and Li, W. (2020). A Sec-dependent secretory protein of the huanglongbing-associated pathogen suppresses hypersensitive cell death in Nicotiana benthamiana. Front. Microbiol. 11:594669. doi: 10.3389/fmicb.2020.594669
Zhang, C., Wang, X., Liu, X., Fan, Y., Zhang, Y., Zhou, X., et al. (2019). A novel ‘Candidatus Liberibacter asiaticus’-encoded Sec-dependent secretory protein suppresses programmed cell death in Nicotiana benthamiana. Int. J. Mol. Sci. 20:5802. doi: 10.3390/ijms20225802
Zou, X., Du, M., Liu, Y., Wu, L., Xu, L., Long, Q., et al. (2021). CsLOB1 regulates susceptibility to citrus canker through promoting cell proliferation in citrus. Plant J. 106, 1039–1057. doi: 10.1111/tpj.15217
Keywords: huanglongbing (HLB), CLas, PCD suppressor, effector, HR, plant immunity, SDE1 (CLIBASIA_05315)
Citation: Shen P, Li X, Fu S, Zhou C and Wang X (2022) A “Candidatus Liberibacter asiaticus”-secreted polypeptide suppresses plant immune responses in Nicotiana benthamiana and Citrus sinensis. Front. Plant Sci. 13:997825. doi: 10.3389/fpls.2022.997825
Received: 19 July 2022; Accepted: 23 August 2022;
Published: 24 October 2022.
Edited by:
Wei Qian, Institute of Microbiology (CAS), ChinaReviewed by:
Lukasz Lech Stelinski, University of Florida, Gainesville, United StatesZhiqian Pang, University of Florida, Gainesville, United States
Lulu Cai, University of Florida, Gainesville, United States
Copyright © 2022 Shen, Li, Fu, Zhou and Wang. This is an open-access article distributed under the terms of the Creative Commons Attribution License (CC BY). The use, distribution or reproduction in other forums is permitted, provided the original author(s) and the copyright owner(s) are credited and that the original publication in this journal is cited, in accordance with accepted academic practice. No use, distribution or reproduction is permitted which does not comply with these terms.
*Correspondence: Changyong Zhou, emhvdWN5QGNyaWMuY24=; Xuefeng Wang, d2FuZ3h1ZWZlbmdAY3JpYy5jbg==