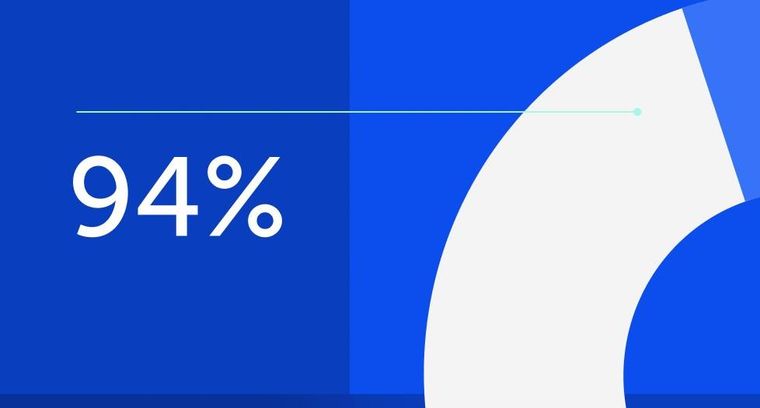
94% of researchers rate our articles as excellent or good
Learn more about the work of our research integrity team to safeguard the quality of each article we publish.
Find out more
ORIGINAL RESEARCH article
Front. Plant Sci., 16 December 2022
Sec. Plant Breeding
Volume 13 - 2022 | https://doi.org/10.3389/fpls.2022.996690
This article is part of the Research TopicAdvances in Genetics and Molecular Breeding of Ornamental PlantsView all 12 articles
Paeonia lactiflora Pall. is an important ornamental plant with high economic and medicinal value, which has considerable development prospects worldwide. The lack of efficient tissue culture techniques and genetic transformation systems has become a master obstacle for P. lactiflora research. The purpose of the present study focuses on obtaining an efficient and stable genetic transformation method using callus as the receptor and exploring an efficient protocol for callus induction and proliferation associated with P. lactiflora. Callus induction and proliferation were performed using MS medium with various concentrations of 2,4-Dichlorophenoxyacetic acid (2,4-D), 1-Naphthaleneacetic acid (NAA), 6-Benzylaminopurine (6-BA) and thidiazuron (TDZ). The sensitivity of callus to kanamycin and cefotaxime was determined. Several parameters such as Agrobacterium cell density, infection time and co-culture duration were studied to optimize transformation efficiency. Agrobacterium strains EHA105 and pBI121 binary vector harboring the β-glucuronidase (GUS) gene were used for transformation. Expression of the GUS reporter gene was detected by GUS assay, polymerase chain reaction (PCR) and Quantitative Real-time PCR (RT-qPCR). The MS medium containing 1.0 mg·L-1 NAA, 0.5 mg·L-1 2,4-D and 0.5 mg·L-1 TDZ was optimal for callus induction and MS medium containing 0.5 mg·L-1 NAA, 1.0 mg·L-1 2,4-D and 0.5 mg·L-1 TDZ was the best for callus proliferation. The concentrations of kanamycin and cefotaxime used for screening positive callus were 125 mg·L-1 and 200 mg·L-1, respectively. Among various combinations analyzed, the best transformation result was obtained via the 25 min of infection of Agrobacterium at 0.6 OD600 and 3 d of co-culture. Overall, this study provided technical support and theoretical guidance for improving the callus induction and proliferation efficiency and the study of gene function in P. lactiflora.
Herbaceous peony (Paeonia lactiflora Pall.) is a perennial herbaceous plant of the genus Paeonia (Zhang et al., 2022). Because of its large, colorful and diverse flowers, herbaceous peony is used as garden, potted, cut and dried flowers in many countries and regions (Zhao et al., 2017; Liu et al., 2022). In addition, it is also used as an important source of crude drugs in traditional Chinese medicine (Hu et al., 2015; Sun et al., 2021). Due to its high ornamental, medicinal and economic value, herbaceous peony is widely cultivated in the world (Xue et al., 2018; Zhao et al., 2019).
Seed and dividing are the traditional propagation methods of herbaceous peony. However, due to the special epicotyl and hypocotyl double dormancy characteristics of herbaceous peony seeds, it usually takes 3-5 years from seed germination to flowering, which makes it difficult for traditional breeding methods to meet the needs of commercial production (Shen et al., 2014). Finding efficient propagation methods of herbaceous peony has become a significant and meaningful research task. In recent years, there is an increasing and new interest to plant tissue culture (Georgiev et al., 2018). Tissue culture can increase the propagation rate, and is an important way to rapidly propagate plants, improve popular plant varieties or select new plant varieties (Osakabe et al., 2018). Although various studies have been studied on vitro culture of herbaceous peony (Jana et al., 2013; Shen et al., 2014; Zhao et al., 2017), many problems continue to be encountered on establishing faster and more efficient methods of tissue culture and its large-scale production. Numerous hurdles related to the tissue culture, such as low efficiency in direct or indirect induction of adventitious shoots, high levels of contamination, browning, poor rooting, and difficulties in transplanting and acclimatization, still need to be solved (Sivanesan et al., 2021). Therefore, it is necessary to establish an efficient and stable method of callus induction and proliferation to alleviate or even solve the above problems, and to establish a basis for the establishment of stable regeneration system of herbaceous peony.
Transgenic plants are commonly used in molecular biology to understand the function of specific genes( Chakraborty et al., 2020). The establishment of an efficient, stable and regenerating receptor system is the basis for genetic transformation. However, there are still so many obstacles on the establishment of the regeneration system associated with herbaceous peony, which not only affect the tissue culture, but also hinder the molecular biology research. Due to the difficulty in regeneration of herbaceous peony during tissue culture, there is no stable genetic transformation system and transgenic plants cannot be obtained, which greatly limits the transgenic work of herbaceous peony (Wang et al., 2021a). Therefore, at present, studies on gene function verification of P. lactiflora are carried out either through heterologous transformation or homologous transient expression (Ni et al., 2018; Bai et al., 2019; Lv et al., 2019). Based on this situation, finding other transgenic receptors to carry out molecular biological research of herbaceous peony has been the focus of scholars (Shen et al., 2012). Gene transformation using callus as receptor has the advantages of high efficiency and short transformation time, and has been widely used in functional analysis of related genes in apple, citrus, grape and other plants (Wu et al., 2017; Xu et al., 2017; Osakabe et al., 2018). Callus of herbaceous peony is easy to obtain, has strong proliferation ability, and can also be used as receptor material for transgene (Guo and Jeong, 2021). At the same time, with the rapid development of plant genetic transformation research, Agrobacterium-mediated transformation has become a convenient tool to transfer favorable traits to plants, and has already successfully applied to many plants (Chetty et al., 2013; Mayavan et al., 2013; Chen et al., 2014; Palla and Pijut, 2014; Liu et al., 2015; Singh et al., 2016; Sedaghati et al., 2018; Sun et al., 2019). However, there are few reports on Agrobacterium-mediated genetic transformation using herbaceous peony callus as receptor. By transferring genes into callus, the function and molecular mechanism of many important genes in P. lactiflora can be determined (An et al., 2018; Qi et al., 2018; Wang et al., 2018; Lu et al., 2019; Zhao et al., 2019b).
To help solve the above problems, in this study, we optimized the callus induction and proliferation protocol of P. lactiflora and further established an efficient Agrobacterium-mediated transgenic method with callus as receptor. We believe that our results provide a theoretical basis and technical support for the establishment of tissue culture regeneration system and further verification of gene function of herbaceous peony.
Herbaceous peony hybrid seeds (‘Fen Yu Nu’×’Xi Shi Fen’) were harvested in the germplasm resource nursery of Shenyang Agricultural University, Liaoning, China. Full and large seeds were chosen and washed under the running tap water for 10 min. After soaking in distilled water for 48 h, the seed coat was removed. Subsequently, they were transferred to a clean bench for further sterilization. The seeds were dipped in 75% (v/v) ethanol for 30 s, and rinsed 2 times with sterile distilled water. Followed by 15 min sterilization with sodium hypochlorite solution (1.5%, w/v), and the seeds were finally rinsed three times in sterile distilled water. After sterilization, the much of endosperm in each seed was removed to leave isolated embryo with a bit of endosperm, which was cultured on Murashige and Skoog (MS) medium supplemented with 0.5 mg·L-1 GA3 (initiation medium) for 45 d to obtain sterile seedlings. All medium and agar used in this article were purchased from Beijing Solarbio Science & Technology Co., LTD. All plant growth regulators (PGRs) were purchased from Shanghai Yien Chemical Technology Co., LTD.
The culture vessels were 100 mL Erlenmeyer flasks with 30 mL of medium. Plants were grown in a tissue culture room at 25 ± 2°C with a 16 h light/8 h dark photoperiod in 50 μmol·m−2·s−1 photosynthetic photon flux density of cool white fluorescent tubes.
The epicotyl stems were cut into 8-10 mm lengths, and both the leaves and cotyledons were cut into 8 mm × 8 mm squares. They were both transferred to MS medium supplemented PGRs, including 0.5 mg·L-1 6-Benzyleaminopurine (6-BA), 1.0 mg·L-1 1-Naphthylacetic acid (NAA) and 1.0 mg·L-1 2,4-dichlorophenoxyacetic acid (2,4-D). Since cotyledons had the best callus induction effect and were easy to obtain, the effects of different PGRs on callus induction were studied by taking sterile cotyledons as explants. According to the results of the preliminary experiment, they were transferred to MS medium containing various concentrations of PGRs alone or in combination, including 6-BA (0.1 mg·L-1, 0.5 mg·L-1, 1.0 mg·L-1), 2,4-D (0.1 mg·L-1, 0.5 mg·L-1, 1.0 mg·L-1), NAA (0.1 mg·L-1, 0.5 mg·L-1, 1.0 mg·L-1) and Thidiazuron (TDZ) (0.5 mg·L-1, 1.0 mg·L-1, 2.0 mg·L-1) (Table 1). Each of the treatments was conducted with at least 30 explants. The callus induction rate was calculated and the callus growth state was observed after 30 d of culture.
Callus from the sterile stem, leave and cotyledon were transferred respectively to MS medium containing 1.0 mg·L-1 TDZ, 1.0 mg·L-1 NAA and 0.5 mg·L-1 2,4-D to observe their proliferation abilities. For the best proliferation effect, the effects of different PGRs on callus proliferation were studied by taking sterile cotyledons as explants. They were transferred to MS medium containing 2,4-D (0.1 mg·L-1, 0.5 mg·L-1, 1.0 mg·L-1), NAA (0.1 mg·L-1, 0.5 mg·L-1, 1.0 mg·L-1) and TDZ (0.5 mg·L-1, 1.0 mg·L-1, 2.0 mg·L-1) alone or in combination (Table 2). There were at least 30 calluses in each treatment. The callus proliferation coefficient and browning rate were calculated after 30 d of culture.
The tolerance limits of cotyledone-induced callus to antibiotic kanamycin (Kan) and cefotaxime (Cef) were assessed prior to Agrobacterium-mediated transformation. The Kan and Cef were sterilized by filter and added to the medium when the medium temperature cooled down to about 40°C. The callus was cultured on a proliferation medium supplemented with different concentrations of Kan (0, 75, 100, 125 and 150 mg·L-1) or Cef (0, 100, 200, 300 and 400 mg·L-1) to determine the concentration of antibiotic needed for the effective selection of transgenic callus. The callus growth state was observed after 14 d of culture. The Kan and Cef were purchased from Beijing Solarbio Science & Technology Co., LTD.
The 20 μL of overnight Agrobacterium tumefaciens EHA105 culture was added to a liquid Luria Bertani (LB) medium containing different concentrations of Cef (0, 100, 200, 300 and 400 mg·L-1) with three biological replicates. After 48 h of incubation, the OD600 value was determined by ultraviolet (UV) spectrophotometer (Qingdao Juchuang Environmental Protection Group Co. LTD, Qingdao, China).
The binary vector pBI121-GUS purchased from Shanghai Maokang Biotechnology Co., LTD. was transformed into the Agrobacterium strain EHA105 purchased from Shanghai Angyu Technology Co., LTD. 1000 ng pBI121-GUS vector plasmid was added to 100 μl of Agrobacterium EHA105 competent cells and gently mixed, then placed in ice, liquid nitrogen and water at 28°C for 5 min, and finally another ice bath for 5 min. 700 μl LB liquid medium was added to the above products and incubated for 3 h in an incubator at 28°C by shaking. After the shock culture, the bacteria were dipped and coated on solid LB medium containing 50 mg·L-1 Kan and incubated upside down for 2 days at 28°C. Single colonies on the above medium were selected for PCR validation to obtain positive strains. Then a positive transformant was picked and inoculated overnight in 2 mL fresh liquid LB medium containing 50 mg·L-1 rifampicin and 50 mg·L-1 Kan at 28 °C in 200×g. The overnight culture liquid was transferred to a 50 ml liquid LB medium at a ratio of 1:50 and then cultured until the Agrobacterium cell density (OD600) reached 0.2-1.0, and then the culture was centrifuged at 5000×g for 10 min. The resuspension solution containing 5% sucrose and 100 μM filter-sterilized acetosyringone was used to resuspend the Agrobacterium as the infection solution. The healthy callus was soaked in the above prepared infection solution for infection, and the callus was gently shaken to make it fully infected. After infection, the callus was transferred to sterile filter paper until almost dry, and then transferred to the co-culture medium (MS medium containing 0.5 mg·L-1 NAA, 1.0 mg·L-1 2,4-D and 0.5 mg·L-1TDZ), dark culture was carried out at 28 °C. After three times of rinse in sterile water containing Cef of 200 mg·L-1 and blotting on sterile filter paper, the calluses were cultured on MS medium containing 0.5 mg·L-1 NAA, 1.0 mg·L-1 2,4-D, 0.5 mg·L-1 TDZ and 200 mg·L-1 Cef. Again, three times of rinse in sterile water containing Cef of 200 mg L-1 and blotting on sterile filter paper, the calluses were transferred to a selection medium (MS medium containing 0.5 mg·L-1 NAA, 1.0 mg·L-1 2,4-D, 0.5 mg·L-1 TDZ and 125 mg·L-1 Kan). After 49 d of culture, part of the resistant callus was taken for GUS staining and Molecular identification. To improve transformation efficiency, the key factors affecting transformation efficiency were optimized, including Agrobacterium cell density (0.2, 0.4, 0.6, 0.8 and 1.0 OD600), infection duration (10, 15, 20, 25 and 30 min) and co-culture duration (0, 1, 2, 3 and 4 d).
GUS histochemical assay was conducted according to the described procedure (Jefferson, 1987). The resistant callus was divided into two parts, one part was stained with GUS solution, and wild-type callus was used as negative control. The staining results were observed and counted. The other part was used for molecular identification.
Genomic DNA was extracted from the transformed and non-transformed callus using an extraction kit (Aidlab, Beijing, China). The sequence of primers used for GUS gene (1182bp): forward primer(5’-ATACCGAAAGGTTGGGCAGG-3’), and reverse primer(5’-ATAACGGTTCAGGCACAGCA-3’). The PCR procedure were as followed: pre-denaturation at 94°C for 3 min, followed by 35 cycles at 94°C for 30 s, 56°C for 30 s, and 72°C for 1 min, and final extension at 72°C for 5 min. The amplified products were analyzed by electrophoresis in 1.2% (w/v) agarose gels. Total RNAs of callus were extracted by RNAprep pure Plant Kit (TianGen, Beijing, China). The cDNAs were synthesized by PrimeScript™ RT Master Mix kit (Perfect Real Time) (Takara, Beijing, China). The RT-qPCR primers were designed with the Primer Premier 5.0 software based on the GUS gene: forward primer (5’-AAGAGCGATGAGCACGAGTA-3’), and reverse primer (5’-AGAAATCAAACAACCCACGA-3’). PlACTIN (GenBank accession no. JN105299.1) was used as an internal control (Li et al., 2020). The Actin genes were detected using primers as followed: forward primer (5’-GGTCTATTCTTGCTTCCCTC-3’) and reverse primer (5’-CCCTCTGCGTCTACACTTTC-3’). The RT-qPCR was performed with StepOne™ 7500 Real-Time PCR using the TB Green ® Premix Ex Taq™ II (Tli RNaseH Plus) (Takara, Beijing, China). The reactions were accomplished according to the following method, holding stage: 95°C for 30 s; cycling stage: 40 cycles of 95°C for 5 s, 60°C for 30 s; melt curve stage: 95°C for 15 s, 60°C for 1 min, 95°C for 15 s. Each experiment was performed with three biological and technical replicates. The results were analyzed according to the 2−ΔΔCt methods, and exhibited by GraphPad Prism 8.0.
Three biological replicates were performed for all experimental treatments in this article. We used SPSS22.0 software to perform one-way analysis of variance (ANOVA) and Duncan’s method for multiple comparisons of the obtained data to determine whether the differences between the data were significant. The important data involved in the paper are calculated as follows: Induction rate = the number of inducted explants/total explants × 100%, Mortality rate = number of dead explants/total explants × 100%, Proliferation coefficient = weight of fresh proliferated callus/weight of inoculated callus, Browning rate = number of browned explants/total explants × 100%, GUS expression rate = the number of GUS-positive receptors/total number stained × 100%.
The cotyledons are the most likely to produce callus (Figure 1A). Cotyledons became thicker after 3 d of culture, and light green callus was generated after 10 d. It had the highest induction rate (92.33%) and the lowest mortality rate (7.67%) (Figure 2). After 10 d of culture, the stem generated green callus gradually, with induction rate and mortality rate were 55.33% and 44.33%, respectively (Figures 1B and 2). However, leaves were not suitable for inducing callus. Although some leaves could generate calluses after 10 d of culture, most leaves died after 20 d with the highest mortality rate (66.33%) and the lowest induction rate (33.00%) (Figures 1C and 2). Thus, the optimal explant for callus induction is the cotyledon.
Figure 1 Callus induction and proliferation of P. lactiflora. (A) callus from cotyledon, (B) callus from stem, (C) callus from leave, (D) callus induction on MS medium containing 1.0 mg·L-1 2,4-D, 0.5 mg·L-1 NAA and 0.5 mg·L-1 TDZ after culture for 30 days, (E) callus induction on MS medium containing 0.5 mg·L-1 2,4-D, 1.0 mg·L-1 NAA and 0.5 mg·L-1 TDZ after culture for 30 days, (F) proliferation of callus from cotyledon, (G) proliferation of callus from stem, (H) proliferation of callus from leave, (I) waterlogged callus, (J) browned callus.
Figure 2 Effect of different explant of sterile seedling on P. lactiflora callus induction. (A) mortality rate of callus induced by different explants, (B) induction rate of callus induced by different explants, the different letters within a column marked significant differences (p < 0.05).
Auxin had an important influence on the induction rate of callus. After 20 d of culture on MS medium containing 2,4-D, the callus produced and grew slowly. With the increase of 2,4-D concentration, the induction rate increased gradually. The highest value was 57.78% when treated with 1.0 mg·L-1 2,4-D. The calluses in Group 1 were in a better growth state and presented green and full of vitality (Table 1). While on MS medium containing NAA, the cotyledon generated callus only after 7 d of culture. After 20 d, the whole cotyledon became callus. When the concentration of NAA was 0.5 mg·L-1, the callus in Group 2 induction rate reached the maximum (Table 1). However, the callus presented as unhealthy, waterlogged, yellow-white and loose. Some callus stopped growing and even died due to browning. Therefore, 2,4-D was beneficial to callus growth, while NAA could decrease the time required for callus induction.
Cytokinin is also indispensable in the process of inducing callus. Cytokinin promotes cell division and differentiation. An appropriate concentration of cytokinin and auxin will effectively improve the induction efficiency of callus. Based on the results described, 1.0 mg·L-1 2,4-D was added to MS medium. At the same time, different concentrations of 6-BA or TDZ were added. After adding cytokinin, the whole cotyledon became calluses for an average culture of 15 d, and the callus growth state was effectively improved. High or low TDZ concentration was not conducive to the callus induction. The highest rate of callus induction (67.78%) was in Group 3, which was observed from the 1.0 mg·L-1 TDZ (Table 1). The callus was fresh green and loose. Although there was a small amount of browning, it was effectively improved by transferring to a fresh medium. It could be seen from the fourth group that 1.0 mg·L-1 6-BA had the highest induction efficiency (Table 1). The callus presented yellow-green and dense. After 40 d, the callus browning occurred and the browning degree did not decrease after transferring to a fresh medium. Thus, TDZ was more effective on callus induction than 6-BA.
The presence of 2,4-D was important to ensure callus growth state, while NAA was important for the velocity of callus induction. We found that the combination of NAA, 2,4-D and TDZ was more conducive to the callus induction. Meanwhile, the callus presented fresh green, dense and full of vitality (Table 1). On the average culture of 10 d, the whole cotyledon became callus. The highest induction rate (86.67%) was observed on MS medium supplemented with 1.0 mg·L-12,4-D, 0.5 mg·L-1NAA and 0.5 mg·L-1 TDZ or MS medium supplemented with 0.5 mg·L-1 2,4-D, 1.0 mg·L-1 NAA and 0.5 mg·L-1 TDZ. The latter was in a better growth state and presented less browning after culture (Figure 1D and Figure 1E). Therefore, the optimal medium for callus induction was MS medium containing 0.5 mg·L-1 2,4-D, 1.0 mg·L-1 NAA and 0.5 mg·L-1 TDZ.
The proliferation of callus from the cotyledon was fast, which presented emerald green, tight and uniform texture (Figure 1F). The highest proliferation coefficient (2.31) was observed on callus from the cotyledon and the browning rate was 13.33% (Figure 3). Callus induced from the stem had the lowest proliferation coefficient (1.12) and poor growth status. Reduced fullness and soft texture were observed during the culture (Figure 1G), with the highest browning rate (26.67%) and a serious browning degree (Figure 3). The proliferation coefficient was 1.63 of leaf callus. Its texture was compact (Figure 1H) and the proliferation rate was slower, while the browning rate was lower (10.00%). Therefore, callus from the cotyledon was the best choice for callus proliferation.
Figure 3 Effect of different explants on P. lactiflora callus proliferation. (A) proliferation coefficient of callus induced by different explants, (B) browning rate of callus induced by different explants, the different letters within a column marked significant differences (p < 0.05).
Considering that the effect of 6-BA on callus was not as good as TDZ, we did not use 6-BA in this experiment. When the concentration of TDZ was constant, 2,4-D and NAA had different effects on callus proliferation. Different concentrations of 2,4-D resulted in no significant difference in proliferation coefficient, while the browning rate increased gradually with the increase of 2,4-D concentration (Table 2, Group 1). When the concentration of TDZ was the constant, high or low concentration of NAA was not conducive to the callus proliferation. The maximum proliferation coefficient (1.68) and lowest browning rate (23.33%) were observed when 0.5 mg·L-1 NAA was applied (Table 2, Group 2). The callus presented yellow-green and loose with the supplement of 2,4-D, while the callus showed different degrees of green, full and loose texture due to the NAA supplement. On these two mediums, the callus will gradually brown from the inside to the outside after a culture of 30 d. Although browning will be effectively alleviated by transfer, the callus aged and even produced white aerial roots. According to this study, 2,4-D was better than NAA on improving callus proliferation coefficient, and the browning rate was lower (Table 2). As for the growth state, NAA had a better effect.
Based on the results of callus proliferation, NAA, 2,4-D and TDZ were combined to improve the callus proliferation and this brought a better effect (Table 2, Group 3). After being transferred to a proliferation medium for 20 d, the callus proliferated vigorously and presented a green, full and loose growth state. However, browning occurred at varying degrees. The callus proliferation coefficient reached the highest value (3.21) on the MS medium containing 0.5 mg·L-1NAA, 1.0 mg·L-12,4-D and 0.5 mg·L-1 TDZ, followed by the 2.80, which was observed on the MS medium containing 1.0 mg·L-1 NAA, 0.5 mg·L-1 2,4-D and 0.5 mg·L-1 TDZ. Their browning rates were 33.33% and 41.11%, respectively. Therefore, the optimal medium for callus proliferation was MS medium containing 0.5 mg·L-1 NAA, 1.0 mg·L-1 2,4-D and 0.5 mg·L-1 TDZ.
In this assay, the growth state of P. lactiflora callus was observed on a proliferation medium containing various concentrations of Kan or Cef (Figure 4). The callus was more sensitive to Kan. 75~150 mg·L-1 of Kan could strongly inhibit the growth of P. lactiflora callus. The higher concentration led to the lower proliferation rate and more serious browning of calluses, and even dead calluses (Table 3). According to the growth state, 125 mg·L-1 Kan was chosen to screen transformed callus of P. lactiflora.
Figure 4 Antibiotic sensitivity assay of P. lactiflora callus. (A-E) kanamycin concentrations were 0, 75, 100, 125 and 150 mg·L-1, respectively. (F-J) cefotaxime concentrations were 0, 100, 200, 300, and 400 mg·L-1, respectively.
Cef could inhibit the growth of P. lactiflora callus. The callus was less sensitive to Cef compared with Kan. Both 100, 200 and 300 mg·L-1 of Cef had an insignificant impact on the growth and proliferation of callus, while 400 mg·L-1 Cef could inhibit the callus obviously. Each callus presented browning. Combining its effect on Agrobacterium growth (Table 4), the optimal concentration of Cef might be 200 mg·L-1.
For exploring the inhibitory effect of cefotaxime on Agrobacterium tumefaciens, we carried out the inhibition test of cefotaxime on Agrobacterium. The results showed that the OD600 value could not be detected when the concentration of cefotaxime was 200, 300 and 400 mg·L-1 (Table 4). Considering the effect of Cef on the callus growth of P. lactiflora, the optimal concentration of Cef was 200 mg·L-1.
In order to establish genetic transformation system and obtain transgenic callus, Agrobacterium strain EHA105 harboring the binary vector pBI121-GUS was used to infect callus. Various combinations of cell densities of Agrobacterium suspension at OD600 (0.2, 0.4, 0.6, 0.8 and 1.0), infection duration (10, 15, 20, 25 and 30 min) and co-culture duration (0, 1, 2, 3 and 4 d) were carried out to select the optimum transgenic system. Then, the GUS staining was used for the identification of transgenic callus after selection culture for 49 d (Figure 5).
Figure 5 Genetic transformation of P. lactiflora callus by Agrobacterium-mediated transformation. (A) callus before Agrobacterium infection, (B) callus after Agrobacterium infection, (C) GUS expression in control callus, (D) GUS expression in transgenic callus.
Calluses induced by cotyledons with good and consistent growth were selected for Agrobacterium transformation in this experiment. Agrobacterium cell densities, infection and co-culture duration are key transformation factors directly affecting genetic transformation. Our results showed that the effects of different Agrobacterium cell densities were quite different (Figure 6). High or low cell densities were not conducive to the genetic transformation of P. lactiflora callus. When OD600 was 0.6, the callus attained the maximum GUS expression rate (53.33%). In the same way, we also found that 25 min of infection and 3 d of co-culture led to the highest GUS expression rate, with 68.33% and 61.67%, respectively. Therefore, OD600 = 0.6, 25 min for infection and co-culture for 3 d were chosen as the optimal Agrobacterium transformation conditions.
Figure 6 Parameters affecting transformation efficiency. (A) GUS expression at different Agrobacterium cell density, (B) GUS expression at different Infection duration, (C) GUS expression at different Co-culture duration, the different letters within a column marked significant differences (p < 0.05).
Further molecular identification of the resistant calluses was confirmed by PCR and RT-qPCR. GUS-positive resistant calluses were selected randomly and confirmed by PCR and RT-qPCR. In PCR validation test, plasmid pBI121 was used as the positive control and non-transgenic callus DNA was used as the negative control. The presence of amplified fragments in GUS-positive resistant calluses samples and pBI121 plasmid confirmed the presence and integration of the GUS gene in the P. lactiflora genome. Non-transgenic callus genomic DNA did not show any amplified fragments (Figure 7). In order to further identify the transgenic callus at the transcriptional level, RT-qPCR was used to detect the expression level of the GUS gene in non-transgenic callus and GUS-positive resistant calluses. The results showed that the GUS gene expression was not detected in the non-transgenic callus, but it was detected in GUS-positive resistant calluses (Figure 8).
Figure 7 PCR analysis of the GUS-positive resistant callus of P. lactiflora. PCR product in 1182 bp was observed in each positive callus except wild-type with GUS gene-specific primer. Lane M, DL2000 DNA marker; lane P, plasmid control; lane WT, wild-type callus; lanes 1-14, different GUS-positive callus.
Figure 8 Relative expression level of GUS gene in non-transformed and transgenic callus. WT, non-transformed callus; 1-14, different transgenic callus, the different letters within a column marked significant differences (p < 0.05).
Plant callus is considered to have the potential to be used in rapid mass propagation of plants because of its advantages of easy access, strong ability of proliferation and differentiation (Wang et al., 2021b). However, callus induction and proliferation are distinct in different plant species (Xu et al., 2017). The success of callus induction is strongly related to the choice of the explant. Explant source and growth physiological state will directly affect the process of callus induction (Guo and Jeong, 2021). Seeds, cotyledons, stems, leaves, anthers, short limbs and even young fruits are usually used as explants for callus induction (Singh et al., 2015; Din et al., 2016; Bai et al., 2019). In fact, there have been many studies on callus induction in herbaceous peony, in which explants selection has been generally concerned (Shen et al., 2012). When the underground buds of P. lacactiflora were used as explants, the buds developed well and had strong ability to differentiate and proliferate in vitro (Tian et al., 2010). Orlikowska et al. used petioles, leaves and young stems of Paeonia loniflorica as explants for induction experiments. Their results showed that whether these explants could induce callus successfully depended on their culture conditions in the first 1.5 months, and the young stems were considered to have the most differentiation potential (Orlikowska et al.,1998). For wild herbaceous peony, the callus induction from vegetative organs was not only limited by season, but also complicated and ineffective in sterilization (Shen et al., 2012). In the present study, we further optimized the selection of explants based on the experience of previous studies. We induced callus using different parts of sterile seedlings obtained from cultivated seeds. The reason we did this is that seeds are easy to preserve and obtain, ensuring that explants are available regardless of time and season. In addition, the obtained sterile seedlings don’t need to be sterilized, which could improve the induction efficiency. In our study, the most suitable explant for callus induction was sterile seedling cotyledon (Figure 2), and the order of proliferation ability of callus from different explants was cotyledon > leaf > stem (Figure 3). Therefore, cotyledons obtained from seed culture was most suitable for callus induction and proliferation. The reason for this result, we believe, is that cotyledon emerges at the early stage of growth and development, and the meristem cells are more active (Hu et al., 2015). Just because of this reason, we further speculated that the callus induced by cotyledon had strong growth vigor and high proliferation efficiency. Stem and leaf are at the mid-growth stage. Although their cells are totipotent, the cells of plant organs have already differentiated. So, the dedifferentiation ability of stem and leaf is relatively reduced, which may cause low efficiency in the callus induction and proliferation.
Adding plant growth regulators to the basal medium will make different effects on callus induction and proliferation (Kotb et al., 2020). Many previous studies have proved that PGRs are very important for plant callus induction and proliferation. The combination of auxin and cytokinin could improve the induction rate of callus, and the induction rate was higher when the ratio of cytokinin to auxin was between 1:1.4 and 1:5 (Shen et al., 2012). TDZ and 6-BA are both important cytokinins and play a significant role in the induction and proliferation of plant callus (Roy et al., 2012). TDZ has stronger cytokinin activity, and is superior to 6-BA in both callus and branch proliferation (Zhao et al., 2017). Similarly, our results proved that TDZ was also superior to 6-BA in callus induction of P.lactiflora (Table 1). In this study, on the basis of previous studies, we further optimized the components of the culture medium for callus induction from P. lactiflora.The results showed that two auxins, 2,4-D and NAA, affected P. lactiflora callus induction significantly. On 2,4-D supplemented medium, the induced callus was bright green, dense and high viable, while the induced callus was loose, water-stained, low viable and browning occurred on NAA supplemented medium (Table 1). Therefore, we believe that 2,4-D had a better effect on callus induction than NAA, which was consistent with the previous study (Wang et al., 2010). Our results also show that when combined with TDZ in the process of proliferation, the callus proliferation coefficient was greater in a medium containing 2,4-D than that in a medium containing NAA at the same concentration. The combination of 2,4-D, NAA and TDZ were more effective in both the callus induction and proliferation process, maintaining a higher induction rate and proliferation coefficient as well as a better callus growth state. These results again indicate that 2, 4-D, NAA and TDZ play an important role in callus proliferation.In the course of our experiments, we unexpectedly discovered some preliminary methods that may reduce the browning in P. lactiflora tissue culture. Browning is a common phenomenon in plant tissue culture and has always been a big hurdle in the tissue culture of P. lactiflora (Gao et al., 2020). The explants release brown substances or phenolics into the medium in the course of plant tissue culture, which can inhibit the normal growth and differentiation of cell tissues, and even lead to death (Shen et al., 2012). Plant growth regulators have a certain effect on the browning of callus (Shen et al., 2012). In our present study, in the medium supplemented with 2,4-D, the callus was lightly browned and browning could be effectively suppressed by transferring to a new medium, while callus browning increased in the NAA-added medium. When the ratio of auxin and cytokinin varied from 1:1 to 2:1, the browning degree was lighter. Moreover, frequent transfer could slightly reduce the degree of browning but the callus gradually stopped growing after multiple transfers. Our findings may be helpful in alleviating the browning of P. lactiflora tissue culture, but the novel methods and mechanisms of browning prevention have yet to be obtained.
During genetic transformation, the main role of antibiotics such as Kan and Cef is to screen resistant plants or to inhibit the overgrowth of Agrobacterium. Previous studies have reported the optimal concentration of Kan (5-100 mg·L-1) and Cef (50-400 mg·L-1) in plants of different genera (Matsuda et al., 2005; Freiman et al., 2012; Yang et al., 2017). These studies indicated that different genotypes have different degrees of sensitivity to antibiotics, suggesting the importance of screening antibiotic concentration during transformation. In our study, we found that 125 mg·L-1 Kan could completely inhibit the growth of callus, and 200 mg·L-1 Cef was the optimal concentration to inhibit the growth of Agrobacterium without affecting the growth of callus. (Table 4 and Figure 4). We believe that under the action of these two antibiotics, not only positive transgenic callus can be obtained, but also the growth of Agrobacterium can be completely inhibited, so as to avoid the pollution caused by Agrobacterium attachment during molecular identification.
The Agrobacterium-mediated genetic transformation is the most widely used transformation method (Liu et al., 2021). However, there were few applications on P. lactiflora. Transformation receptor and condition are key factors affecting genetic transformation (Shen et al., 2012). Plant callus is very suitable as transformation receptor (Liu et al., 2021). It is generally believed that the pre-culture and wound of explants before transformation can improve the transformation rate of exogenous genes (Wu et al., 2014; Yan et al., 2019). Nevertheless, callus can be used as acceptor material without considering these steps (Sedaghati et al., 2018). Agrobacterium cell density, infection time and co-culture time have important effects on genetic transformation efficiency, and optimal parameters vary depending on the plant materials and/or acceptor types (Sedaghati et al., 2018; Yan et al., 2019). Low cell density may not provide sufficient bacteria and short periods are not conducive to establish contact between the acceptor material and Agrobacterium. High cell density or long co-culture time will easily cause contamination (Yan et al., 2019). Extreme cell density and infection time affect the transformation efficiency because of the Agrobacterium toxicosis or incomplete contact with the surface of callus (Ahmed et al., 2015). Short co-culture time may lead to incomplete T-DNA transfer process. Conversely, long co-culture time will lead to the excessive multiplication of Agrobacterium, which poisons the callus (Wu et al., 2015). In order to determine the optimal transformation conditions for Agrobacterium-mediated genetic transformation and whether the target gene can be effectively transferred into peony callus, we used GUS staining experiment to observe the transformation efficiency of the target gene. From the results, we can also see that different amounts of GUS chromogenesis were observed under various transformation conditions designed by us. Agrobacterium cell density at 0.6 OD600, 25 min of infection and 3 d of co-culture showed high GUS staining positive rate during the screening process (Figure 6). PCR and RT-qPCR are commonly used to detect transgenic plants (Rajeevkumar et al., 2015; Wu et al., 2019; Wang et al., 2021b). As can be seen from PCR results, clear and bright amplified fragments were observed in 14 GUS-positive samples randomly selected, and the length of the fragments was consistent with the target gene (Figure 7). This indicates that our method can successfully transfer the target gene into the callus of P. lactiflora. RT-qPCR results also showed that GUS gene expression was detected in all GUS-positive resistant calluses compared with the non-transgenic callus, although GUS gene expression was slightly different in different GUS-positive resistant calluses (Figure 8). However, from the results, the relative expression levels of target genes in most samples are relatively stable, which indicates that our method is feasible.
In conclusion, this study optimized and established an efficient method for callus induction and proliferation of P. lactiflora. Based on this method, cotyledons were used as explants to induce and proliferate high-quality callus, and then an efficient transgenic method using callus of P. lactiflora was established through Agrobacterium mediated genetic transformation. Our results provide theoretical basis and technical support for the establishment of a complete and stable genetic transformation system of herbaceous peony and further understanding of the molecular and genetic mechanisms related to herbaceous peony.
The datasets presented in this study can be found in online repositories. The names of the repository/repositories and accession number(s) can be found in the article/Supplementary Material.
XS, SD and XL designed the project. WC, QP, XL, SD and RF completed the callus induction and proliferation experiment. SD, RX and XL completed Agrobacterium-mediated transformation conditions screening experiment. XL and RF were involved in GUS staining experiment. This article was written by SD, XL and SG. All authors contributed to the article and approved the submitted version.
This work was supported by the National Natural Science Foundation of China (32071814 and 31470696).
The authors declare that the research was conducted in the absence of any commercial or financial relationships that could be construed as a potential conflict of interest.
All claims expressed in this article are solely those of the authors and do not necessarily represent those of their affiliated organizations, or those of the publisher, the editors and the reviewers. Any product that may be evaluated in this article, or claim that may be made by its manufacturer, is not guaranteed or endorsed by the publisher.
Ahmed, M., Khan, N., Hafiz, I. A., Abbasi, N. A., Anjum, M. A. (2015). Effect of various factors on the efficiency of Agrobacterium-mediated transformation of grape (Vitis vinifera l.). Vegetos 28, 171. doi: 10.5958/2229-4473.2015.00024.5
An, J. P., Li, R., Qu, F. J., You, C. X., Wang, X. F., Hao, Y. J. (2018). An apple NAC transcription factor negatively regulates cold tolerance via CBF-dependent pathway. J. Plant Physiol. 221, 74–80. doi: 10.1016/j.jplph.2017.12.009
Bai, S. L., Tao, R. W., Tang, Y. X., Yin, L., Ma, Y. J., Ni, J. B., et al. (2019). BBX16, a b-box protein, positively regulates light-induced anthocyanin accumulation by activating MYB10 in red pear. Plant Biotechnol. J. 17, 1985–1997. doi: 10.1111/pbi.13114
Bai, S. L., Tao, R. Y., Yin, L., Ni, J. B., Yang, Q. S., Yan, X. H., et al. (2019). Two b-box proteins, PpBBX18 and PpBBX21, antagonistically regulate anthocyanin biosynthesis via competitive association with Pyrus pyrifolia elongated hypocotyl 5 in the peel of pear fruit. Plant J. 100, 1208–1233. doi: 10.1111/tpj.14510
Chakraborty, N., Chakraborty, P., Sen, M., Bandopadhyay, R. (2020). Choice of explant for plant genetic transformation. Methods Mol. Biol. 2124, 107–123. doi: 10.1007/978-1-0716-0356-7_5. Springer Press.
Chen, Y., Rivlin, A., Lange, A., Ye, X. D., Vaghchhipawala, Z., Eisinger, E., et al. (2014). High throughput Agrobacterium tumefaciens-mediated germline transformation of mechanically isolated meristem explants of cotton (Gossypium hirsutum l.). Plant Cell Rep. 33, 153–164. doi: 10.1007/s00299-013-1519-x
Chetty, V. J., Ceballos, N., Garcia, D., Narvaez-Vasquez, J., Lopez, W., Orozco-Cardenas, M. L. (2013). Evaluation of four Agrobacterium tumefaciens strains for the genetic transformation of tomato (Solanum lycopersicum l.) cultivar micro-tom. Plant Cell Rep. 32, 239–247. doi: 10.1007/s00299-012-1358-1
Din, A. J. M., Ahmad, F. I., Wagiran, A., Abd Samad, A., Rahmat, Z., Sarmidi, M. R. (2016). Improvement of efficient in vitro regeneration potential of mature callus induced from Malaysian upland rice seed (Oryza sativa cv. panderas). Saudi J. Biol. Sci. 23, S69–S77. doi: 10.1016/j.sjbs.2015.10.022
Freiman, A., Shlizerman, L., Golobovitch, S., Yablovitz, Z., Korchinsky, R., Cohen, Y., et al. (2012). Development of a transgenic early flowering pear (Pyrus communis l.) genotype by RNAi silencing of PcTFL1-1 and PcTFL1-2. Planta 235, 1239–1251. doi: 10.1007/s00425-011-1571-0
Gao, J., Xue, J. Q., Xue, Y. Q., Liu, R., Ren, X. X., Wang, S. L., et al. (2020). Transcriptome sequencing and identification of key callus browning-related genes from petiole callus of tree peony (Paeonia suffruticosa cv. kao) cultured on media with three browning inhibitors. Plant Physiol. Biochem. 149, 36–49. doi: 10.1016/j.plaphy.2020.01.029
Georgiev, V., Slavov, A., Vasileva, I., Pavlov, A. (2018). Plant cell culture as emerging technology for production of active cosmetic ingredients. Eng. Life Sci. 18, 779–798. doi: 10.1002/elsc.201800066
Guo, G., Jeong, B.R. (2021). Explant, medium, and plant growth regulator (PGR) affect induction and proliferation of callus in Abies koreana. Forests 12, 10. doi: 10.3390/f12101388
Hu, S., Ma, Y. Y., Jiang, H. L., Feng, D. J., Yu, W., Dai, D. M., et al. (2015). Production of paeoniflorin and albiflorin by callus tissue culture of Paeonia lactiflora pall. Chin. J. Chem. Eng. 23, 451–455. doi: 10.1016/j.cjche.2014.06.036
Jana, S., Sivanesan, I., Lim, M. Y., Jeong, B. R. (2013). In vitro zygotic embryo germination and somatic embryogenesis through cotyledonary explants of Paeonia lactiflora pall. Flower Res. J. 21, 17–22. doi: 10.11623/frj.2013.21.1.2
Jefferson, R. A. (1987). Assaying chimeric genes in plants: The GUS gene fusion system. Plant Mol. Biol. Rep. 5, 387–405. doi: 10.1007/bf02667740
Kotb, O. M., Abd El-Latif, F. M., Atawia, A. R., Saleh, S. S., El-Gioushy, S. F. (2020). In vitro propagation and callus induction of pear (Pyrus communis) cv. ‘Le-conte’. Asian J. Biotechnol. Genet. Eng. 3, 1–10. doi: 10.3390/agronomy12102531
Li, X. T., Fei, R. W., Chen, Z. J., Fan, C. Z., Sun, X. M. (2020). Plant hormonal changes and differential expression profiling reveal seed dormancy removal process in double dormant plant-herbaceous peony. PLoS One 15, e0231117. doi: 10.1371/journal.pone.0231117
Liu, Y. R., Cen, H. F., Yan, J. P., Zhang, Y. W., Zhang, W. J. (2015). Inside out: high-efficiency plant regeneration and Agrobacterium-mediated transformation of upland and lowland switchgrass cultivars. Plant Cell Rep. 34, 1099–1108. doi: 10.1007/s00299-015-1769-x
Liu, C. Y., Jiao, S. Q., Zhang, Q., Yao, P. Q., Xie, L. H., Wang, Z., et al. (2022). Transcriptome profiling provides preliminary molecular insights into adventitious bud formation in herbaceous peony (Paeonia x ‘Coral charm’). J. Horticult. Sci. Biotechnol. 97, 476–486. doi: 10.1080/14620316.2021.2025156
Liu, G. Q., Yuan, Y., Jiang, H., Bao, Y., Ning, G. G., Zhao, L. J., et al. (2021). Agrobacterium tumefaciens-mediated transformation of modern rose (Rosa hybrida) using leaf-derived embryogenic callus. Hortic. Plant J. 7, 359–366. doi: 10.1016/j.hpj.2021.02.001
Lu, J., Sun, M. H., Ma, Q. J., Kang, H., Liu, Y. J., Hao, Y. J., et al. (2019). MdSWEET17, a sugar transporter in apple, enhances drought tolerance in tomato. J. Integr. Agric. 18, 2041–2051. doi: 10.1016/s2095-3119(19)62695-x
Lv, Y. D., Zhang, M. L., Wu, T., Wu, T. L., Zhong, Y. (2019). The infiltration efficiency of Agrobacterium-mediated transient transformation in four apple cultivars. Sci. Hortic. 256, 108597. doi: 10.1016/j.scienta.2019.108597
Matsuda, N., Gao, M., Kanji, I., Tadashi, T., Nishimura, K. (2005). Development of an Agrobacterium-mediated transformation method for pear (Pyrus communis l.) with leaf-section and axillary shoot-meristem explants. Plant Cell Rep. 24, 45–51. doi: 10.1007/s00299-005-0924-1
Mayavan, S., Subramanyam, K., Arun, M., Rajesh, M., Dev, G., Sivanandhan, G., et al. (2013). Agrobacterium tumefaciens-mediated in planta seed transformation strategy in sugarcane. Plant Cell Rep. 32, 1557–1574. doi: 10.1007/s00299-013-1467-5
Ni, J. B., Bai, S. L., Zhao, Y., Qian, M. J., Tao, R. Y., Yin, L., et al. (2018). Ethylene response factors Pp4ERF24 and Pp12ERF96 regulate blue light-induced anthocyanin biosynthesis in ‘Red zaosu’ pear fruits by interacting with MYB114. Plant Mol.Biol. 99, 67–78. doi: 10.1007/s11103-018-0802-1
Orlikowska, T., Marasek, A., Kucharska, D. (1998). Regeneration of Paeonia mloksewitschii lom. and Paeonia tenuifolia lom. in vitro from different explants. Acta Soc Bot. Pol. 67, 223–227. doi: 10.5586/asbp.1998.026
Osakabe, Y., Liang, Z., Ren, C., Nishitani, C., Osakabe, K., Wada, M., et al. (2018). CRISPR-Cas9-mediated genome editing in apple and grapevine. Nat. Protoc. 13, 2844–2863. doi: 10.1038/s41596-018-0067-9
Palla, K. J., Pijut, P. M. (2014). Agrobacterium-mediated genetic transformation of Fraxinus americana hypocotyls. Plant Cell Tissue Organ Cult. 120, 631–641. doi: 10.1007/s11240-014-0630-1
Qi, C. H., Zhao, X. Y., Jiang, H., Zheng, P. F., Liu, H. T., Li, Y. Y., et al. (2018). Isolation and functional identification of an apple MdCER1 gene. Plant Cell Tissue Organ Cult. 136, 1–13. doi: 10.1007/s11240-018-1504-8
Rajeevkumar, S., Anunanthini, P., Sathishkumar, R. (2015). Epigenetic silencing in transgenic plants. Front. Plant Sci. 6. doi: 10.3389/fpls.2015.00693
Roy, A. R., Deka, B. C., Sajeev, S., Pattanayak, A. (2012). TDZ induced micropropagation in Cymbidium giganteum wall. ex lindl. and assessment of genetic variation in the regenerated plants. Plant Growth Regul. 68, 435–445. doi: 10.1007/s10725-012-9732-0
Sedaghati, B., Haddad, R., Bandehpour, M. (2018). Efficient plant regeneration and Agrobacterium-mediated transformation via somatic embryogenesis in purslane (Portulaca oleracea l.): an important medicinal plant. Plant Cell Tissue Organ Cult. 136, 231–245. doi: 10.1007/s11240-018-1509-3
Shen, M. M., Tang, Z. J., Teixeira, da Silva, J. A., Yu, X. N. (2014). Induction and proliferation of axillary shoots from in vitro culture of Paeonia lactiflora pall. mature zygotic embryos. N. Z. J. Crop Hortic. Sci. 43, 42–52. doi: 10.1080/01140671.2014.944548
Shen, M. M., Wang, Q., Yu, X. N., da Silva, J. A. T. (2012). Micropropagation of herbaceous peony (Paeonia lactiflora pall.). Sci. Hortic. 148, 30–38. doi: 10.1016/j.scienta.2012.09.017
Singh, P., Khan, S., Kumar, S., Rahman, L. (2016). Establishment of an efficient Agrobacterium-mediated genetic transformation system in Pelargonium graveolens: an important aromatic plant. Plant Cell Tissue Organ Cult. 129, 35–44. doi: 10.1007/s11240-016-1153-8
Singh, R., Rai, M. K., Kumari, N. (2015). Somatic embryogenesis and plant regeneration in Sapindus mukorossi gaertn. from leaf-derived callus induced with 6-benzylaminopurine. Appl. Biochem. Biotechnol. 177, 498–510. doi: 10.1007/s12010-015-1758-0
Sivanesan, I., Muthu, M., Gopal, J., Tasneem, S., Kim, D. H., Oh, J. W. (2021). A fumigation-based surface sterilization approach for plant tissue culture. Int. J. Environ. Res. Public Health 18, 5. doi: 10.3390/ijerph18052282
Sun, Z. L., Li, X., Zhou, W., Yan, J., Gao, Y., Li, X. W., et al. (2019). Agrobacterium-mediated genetic transformation of Chinese chestnut (Castanea mollissima blume). Plant Cell Tissue Organ Cult. 140, 95–103. doi: 10.1007/s11240-019-01713-4
Sun, M., Wang, Y. Z., Yang, Y., Lv, M. W., Li, S. S., da Silva, J. A. T., et al. (2021). Analysis of chemical components in the roots of eight intersubgeneric hybrids of Paeonia. Chem. Biodivers. 18, 2. doi: 10.1002/cbdv.202000848
Tian, D., Tilt, K. M., Dane, F., Woods, F. M., Sibley, J. L. (2010). Comparison of shoot induction ability of different explants in herbaceous peony (Paeonia lactiflora pall.). Sci. Hortic. 123, 385–389. doi: 10.1016/j.scienta.2009.10.007
Wang, J. F., Li, Q., Meng, H. (2010). Induction and regeneration of callus tissues in five peony cultivars. J. Beijing Forestry Univ. 32, 213–216. doi: 10.13332/j.1000-1522.2010.03.009
Wang, F. P., Wang, X. F., Zhang, J., Ma, F., Hao, Y. J. (2018). MdMYB58 modulates fe homeostasis by directly binding to the MdMATE43 promoter in plants. Plant Cell Physiol. 59, 2476–2489. doi: 10.1093/pcp/pcy168
Wang, Q., Fan, Y., da Silva, J. A. T., Yu, X. N. (2021a). Transcriptomics analysis of Paeonia lactiflora pall. in response to drought stress by high-throughput sequencing. J. Horticult. Sci. Biotechnol. 96, 479–493. doi: 10.1080/14620316.2021.1886000
Wang, X. H., Zhou, F. L., Liu, J. L., Liu, W. Q., Zhang, S. L., Li, D. L. (2021b). Establishment of efficient callus genetic transformation system for Pyrus armeniacaefolia. Sci. Hortic. 289, 110429. doi: 10.1016/j.scienta.2021.110429
Wu, J. D., Jiang, Y. L., Liang, Y. N., Chen, L., Chen, W. J., Cheng, B. J. (2019). Expression of the maize MYB transcription factor ZmMYB3R enhances drought and salt stress tolerance in transgenic plants. Plant Physiol. Biochem. 137, 179–188. doi: 10.1016/j.plaphy.2019.02.010
Wu, J., Liu, C., Seng, S. S., Khan, M., Sui, J. J., Gong, B. H., et al. (2015). Somatic embryogenesis and agrobacterium-mediated transformation of Gladiolus hybridus cv. ‘Advance red’. Plant Cell Tissue Organ Cult. 120, 717–728. doi: 10.1007/s11240-014-0639-5
Wu, R. G., Wang, Y., Wu, T., Xu, X. F., Han, Z. H. (2017). Functional characterisation of MdMYB44 as a negative regulator in the response to cold and salt stress in apple calli. j. pomol. J. Horticult. Sci. Biotechnol. 93, 347–355. doi: 10.1080/14620316.2017.1373038
Xue, J. Q., Tang, Y., Wang, S. L., Yang, R. W., Xue, Y. Q., Wu, C. H., et al. (2018). Assessment of vase quality and transcriptional regulation of sucrose transporter and invertase genes in cut peony (Paeonia lactiflora ‘Yang fei chu yu’) treated by exogenous sucrose. Postharvest Biol. Technol. 143, 92–101. doi: 10.1016/j.postharvbio.2018.04.014
Xu, J. D., Wang, ,. X., Cao, H., Xu, H. D., Xu, Q., Deng, X. X. (2017). Dynamic changes in methylome and transcriptome patterns in response to methyltransferase inhibitor 5-azacytidine treatment in citrus. DNA Res. 24, 509–522. doi: 10.1093/dnares/dsx021
Xu, K., Zhang, X. M., Fan, C. M., Chen, F. L., Zhu, J. L., Zhang, S. L., et al. (2017). A callus transformation system for gene functional studies in soybean. J. Integr. Agric. 16, 1913–1922. doi: 10.1016/S2095-3119(16)61621-0
Yang, Y. J., Wang, D. F., Wang, C. S., Wang, X. H., Li, J. N., Wang, R. (2017). Construction of high efficiency regeneration and transformation systems of Pyrus ussuriensis maxim. Plant Cell Tissue Organ Cult. 131, 139–150. doi: 10.1007/s11240-017-1271-y
Yan, R., Wang, Z. P., Ren, Y. M., Li, H. Y., Liu, N., Sun, H. M. (2019). Establishment of efficient genetic transformation systems and application of CRISPR/Cas9 genome editing technology in Lilium pumilum DC. fisch. and Lilium longiflorum white heaven. Int. J. Mol. Sci. 20, 12. doi: 10.3390/ijms20122920
Zhang, R. L., Wang, X. B., Shi, X. H., Shao, L. M., Xu, T., Xia, Y. P., et al. (2021). Chilling requirement validation and physiological and molecular responses of the bud endodormancy release in Paeonia lactiflora ‘Meiju’. Int. J. Mol. Sci. 22, 16. doi: 10.3390/ijms22168382
Zhao, D. Q., Xia, X., Tang, Y., Tao, J. (2019a). Microstructural and lignin characteristics in herbaceous peony cultivars with different stem strengths. Postharvest Biol. Technol. 159, 111043. doi: 10.1016/j.postharvbio.2019.111043
Zhao, X. Y., Qi, C. H., Jiang, H., Zheng, P. F., Zhong, M. S., Zhao, Q., et al. (2019b). Functional identification of apple on MdHIR4 in biotic stress. Plant Sci. 283, 396–406. doi: 10.1016/j.plantsci.2018.10.023
Keywords: herbaceous peony, callus, induction, proliferation, Agrobacterium, genetic transformation, GUS expression
Citation: Duan S, Xin R, Guan S, Li X, Fei R, Cheng W, Pan Q and Sun X (2022) Optimization of callus induction and proliferation of Paeonia lactiflora Pall. and Agrobacterium-mediated genetic transformation. Front. Plant Sci. 13:996690. doi: 10.3389/fpls.2022.996690
Received: 18 July 2022; Accepted: 30 November 2022;
Published: 16 December 2022.
Edited by:
Shunli Wang, Chinese Academy of Agricultural Sciences (CAAS), ChinaReviewed by:
Xiaonan Yu, Beijing Forestry University, ChinaCopyright © 2022 Duan, Xin, Guan, Li, Fei, Cheng, Pan and Sun. This is an open-access article distributed under the terms of the Creative Commons Attribution License (CC BY). The use, distribution or reproduction in other forums is permitted, provided the original author(s) and the copyright owner(s) are credited and that the original publication in this journal is cited, in accordance with accepted academic practice. No use, distribution or reproduction is permitted which does not comply with these terms.
*Correspondence: Xiaomei Sun, c3htNzI4MEBzeWF1LmVkdS5jbg==
†These authors have contributed equally to this work
Disclaimer: All claims expressed in this article are solely those of the authors and do not necessarily represent those of their affiliated organizations, or those of the publisher, the editors and the reviewers. Any product that may be evaluated in this article or claim that may be made by its manufacturer is not guaranteed or endorsed by the publisher.
Research integrity at Frontiers
Learn more about the work of our research integrity team to safeguard the quality of each article we publish.