- 1Key Laboratory of Grassland Resources and Ecology of Western Arid Region, Ministry of Education, Key Laboratory of Grassland Resources and Ecology of Xinjiang, College of Grassland Science, Xinjiang Agricultural University, Urumqi, China
- 2Institute of Animal Science, Chinese Academy of Agricultural Sciences, Beijing, China
- 3College of Grassland Agriculture, Northwest A&F University, Shanxi, China
Medicago falcata L. is an important legume forage grass with strong drought resistant, which could be utilized as an important gene pool in molecular breed of forage grass. In this study, M. falcata seedlings were treated with 400 mM mannitol to simulate drought stress, and the morphological and physiological changes were investigated, as well as the transcriptome changes of M. falcata seedlings at different treatment time points (0 h, 2 h, 6 h, 12 h, 24 h, 36 h and 48 h). Transcriptome analyses revealed four modules were closely related with drought response in M. falcata by WGCNA analysis, and four ERF transcription factor genes related with drought stress were identified (MfERF053, MfERF9, MfERF034 and MfRAP2.1). Among them, MfERF053 was highly expressed in roots, and MfERF053 protein showed transcriptional activation activity by transient expression in tobacco leaves. Overexpression of MfERF053 in Arabidopsis improved root growth, number of lateral roots and fresh weight under drought, salt stress and exogenous ABA treatments. Transgenic Arabidopsis over-expressing MfERF053 gene grew significantly better than the wild type under both drought stress and salt stress when grown in soil. Taken together, our strategy with transcriptome combined WGCNA analyses identified key transcription factor genes from M. falcata, and the selected MfERF053 gene was verified to be able to enhance drought and salt resistance when over-expressed in Arabidopsis.
Introduction
Drought is one of the most serious abiotic factors that can cause significant damage to both agriculture, human and livestock populations (Gupta et al., 2020). Forage grass are subjected to damage caused by drought stress due to the lack of rainfall and insufficient water supply over a long period of time, which eventually leads to a significant decrease in yield (Ray et al., 2015). Therefore, in order to largely prevent environmental damage, plants themselves have evolved specific regulatory protection mechanisms (Pinhero et al., 1997). During drought stress, dramatic changes occur from biochemical and physiological to gene expression and metabolic processes (Rao et al., 2020; Waititu et al., 2021). Drought stress system promotes the production of metabolites such as proline, initiates the antioxidant defense system internal to scavenge increased reactive oxygen species (ROS), prevents cell damage by scavenging free radicals, reduces the degree of membrane lipid peroxidation, and maintains membrane integrity (Wang et al., 2009; Anjum et al., 2011; Wei et al., 2019). Many drought related genes had been identified and used as candidate genes in genetic engineering, such as EDT1 (Yu et al., 2016), MfNACsa (Duan et al., 2017), CYT75B1 (Rao et al., 2020), MYB30 (Wen et al., 2021), CBF4 (Haake et al., 2002) and ERF172 (Zhao et al., 2020). Among them, many of them are transcription factors, and they play key roles in regulating the expression of downstream targeted genes and metabolic pathway.
AP2/ERF superfamily transcription factors are one of the largest plant-specific transcriptional regulator groups in plants, with a conserved AP2/ERF DNA-binding structural domain of 57-66 amino acids in size (Okamuro et al., 1997). Ethylene responsive factors (ERFs) belong to AP2/ERF superfamily, which participate in plant response to hormone and abiotic stress (Qiang et al., 2010; Gibbs et al., 2015; Jung et al., 2017). In rice, overexpression of JERF3 and OsERF115/AP2/EREBP110 can increase the soluble sugar and proline content of transgenic plants, up-regulated the expression of P5CS gene under drought stress, and improve the tolerance of crops to drought and osmotic stress (Thoenes et al., 2004; Zhang and Huang, 2010). In addition, overexpression of tomato ethylene response factor ERF (TSRF1) in rice can improve permeability and drought resistance through binding GCC box, and up-regulated the expression of MYB, MYC, proline synthesis and photosynthesis-related genes (Quan et al., 2010), activated the expression of the abscisic acid (ABA) synthesis gene SDR, thereby enhanced the sensitivity of transgenic rice to ABA. However, overexpression of OsDERF1 down-regulates ethylene synthesis and negatively affects drought tolerance (Zhai et al., 2012). OsERF71 positively regulates ABA signaling to alter root structure and impart drought tolerance (Dong-Keun et al., 2016; Li et al., 2018). NtERF172 acts as a positive factor in drought stress tolerance, transgenic tobacco showed higher oxidase activity, and lower H2O2 accumulation, in part by regulating the dynamic balance of CAT-mediated H2O2, thereby exhibited greater drought tolerance (Zhao et al., 2020).
M. falcata is widely distributed in northern China, and most of them are wild resources with good drought resistance and good palatability, providing rich nutrients for cattle, sheep and other livestock (Yue and Zhou, 2004). By cross-pollination, M. falcata can be crossed with alfalfa to produce Medicago varia with stronger resistance and higher utilization value, therefore M. falcata is an important gene source for Medicago breeding (Wang et al., 2008; Kang et al., 2011). In M. falcata, some genes have been reported to be associated with abiotic stress response, including MfNACsa (Duan et al., 2017), MfNAC3 (Qu et al., 2016), MfUSP1 (Gou et al., 2020), and galactinol synthase gene 1 (MfGolS1) (Zhuo et al., 2013). At present, the functional study on ERF genes in response to drought are not clear in M. falcata.
In this study, we explored the physiological and molecular responses to drought stress in M. falcata seedlings, and the correlation analysis of the clustered modules with physiological indicators analyzed by WGCNA. Combined with these analysis, an ERF gene was found to be strongly correlated with drought-related module, suggesting a potential role in drought stress. Furthermore, we reported the functional characterization of MfERF053 in conferring multiple resistances to abiotic stresses by over-expression in Arabidopsis.
Materials and methods
Plant materials and sample collection
Seeds of M. falcata were provided by the Key Laboratory of Grassland Resources and Ecology of Western Arid Region, Ministry of Education, College of Grass Industry, Xinjiang Agricultural University. The seedlings were grown at the Institute of Animal Science, Chinese Academy of Agricultural Sciences, Beijing. To ensure the consistency of seed germination, seeds with uniform size and fullness were selected and gently scratched with knife. The seeds were then sterilized with 75% ethanol for 10 min, 5% sodium hypochlorite for 10 min, followed by wash with sterile water for 4-5 times. The seeds were then sow on 1/2 MS medium, and placed at 4 °C for 3 days, and then in an light incubator at 22 °C for germination (16 h light/8 h darkness). Seedlings were transferred into flasks containing different concentrations of mannitol for drought treatment. The concentrations of mannitol were 200 mM, 300 mM, 400 mM, 500 mM, 600 mM, and the treatment without mannitol was set as the control group (CK) in this study.
In this experiment, the treatment with 400 mM mannitol were selected for physiological index determination, and seven different treatment time points were selected with 15-day-old seedlings, these samples were collected at 0 h (CK), 2 h, 6 h, 12 h, 24 h, 36 h and 48 h. For each treatment time point, three biological replicates were performed with 15 plants with whole seedlings for each replicate, samples were quickly collected and frozen in liquid nitrogen, and stored in a -80°C refrigerator.
Measurement of physiological index
The content of MDA, Proline, SOD, CAT and POD in 15-day-old seedlings as for transcriptome sequencing were measured according to the instruction manual as provided at the website (https://www.solarbio.com/), and they were measured by using spectrophotometry methods. Statistical analysis was performed by one-way analysis of variance (ANOVA) and Duncan multiple tests using SPSS 22.0.
RNA extraction and library construction for transcriptome analysis
The Eastep Super Total RNA Extraction Kit was used for RNA extraction (Promega Biotech, Shanghai, China). RNA quality was assessed on an Agilent 2100 Bioanalyzer (Agilent Technologies, Palo Alto, CA, USA) and checked using RNase free agarose gel electrophoresis. Twenty-one cDNA libraries were constructed, then the cDNA fragments were purified with QiaQuick PCR extraction kit (Qiagen, Venlo, The Netherlands), end repaired, poly(A) added, and ligated to Illumina sequencing adapters to sequence. The ligation products were size selected by agarose gel electrophoresis, PCR amplified, and sequenced using Illumina HiSeq2500 by Gene Denovo Biotechnology Co. (Guangzhou, China).
Raw data processing, sequence assembly and functional annotation
Raw read containing adapters or low quality bases will affect the following assembly and analysis, which were uploaded in NCBI SRA (http://www.ncbi.nlm.nih.gov/sra): SRR19146603-SRR19146623. Thus, to get high quality clean reads, reads were further filtered by fastp (Chen et al., 2018) (version 0.18.0). An index of the reference genome was built (Chen et al., 2020), and paired-end clean reads were mapped to the reference genome using HISAT2. 2.4 (Kim et al., 2015) with “-rna-strandness RF” and other parameters set as a default.
Identification of differentially expressed genes and PCA analysis
Analyses on differentially expressed genes were performed by DESeq2 software (Love et al., 2014) between two different groups, and by edgeR (Smyth, 2010) between two samples. The criteria of differentially expressed genes/transcripts (DEGs) screening was set as FDR<0.05 and |log2FC|>1. Principal component analysis (PCA) was performed with R package models (http://www.r-project.org/).
WGCNA analysis
WGCNA (Weighted gene co-expression network analysis) is an analytical method to analyze the gene expression patterns of multiple samples (Zhang and Horvath, 2005), which allows clustering genes with similar expression patterns, and analyzing the association between modules and specific traits or phenotypes. Therefore, in this study, physiological indicator traits were analyzed in association with gene modules, using the R language package (Langfelder and Horvath, 2008). For annotation of the biological functions of the DEGs, GO and KEGG pathway enrichment analyses were performed with agriGO 2.0 (https://systemsbiology.cau.edu.cn/agriGOv2/) and KOBAS 3.0 (https://kobas.cbi.pku.edu.cn/), respectively.
Quantitative real-time PCR
Four MfERF genes were selected for validation using qRT-PCR. Actin gene was amplified as internal standard gene, and AlleleID 6 Tool was used to design the gene-specific primers (Table S1), relative expression level was normalized by comparing with control and calculated using 2-ΔΔCt method (Livak and Schmittgen, 2001), qRT-PCR analysis program was as follows: one cycle at 95°C for 15 min, followed by 40 cycles at 95°C for 10 s, 60°C for 20 s and 72°C for 30 s.
Gene cloning and analyses on sequences and phylogenetic relationship
The full-length coding DNA sequence (CDS) of MsERF053 was isolated from the roots of Medicago falcata, and cloned into pENTR vector for sequencing. The protein sequence of the homologous gene was selected by blast and multiple sequence comparisons. DNAMAN software was used to perform multiple sequence alignment. The phylogenetic tree was developed using protein sequences from Medicago truncatula, Medicago sativa, Medicago ruthenica, Mucuna pruriens, Arabidopsis thaliana, Glycine sopa, Vigna angularis and Vigna radiata with MEGA 7.0 (http://www.megasoftware.net) and visualize by using Evolview with bootstrap value of 1000 replications.
Transactivation assay
The open reading frame of MfERF053 was also cloned with gene-specific primers by seamless cloning with KOD polymerase. The transactivation construct was generated by inserting the full-length sequence of MfERF053 into the Kpn I and Xba I sites of vector pCAMBIA1300BD. The BDGAL4 plasmid was used as control, and BDGAL4-MfERF053 recombinant plasmid were transformed into A. tumefaciens strain GV3101 and infiltrated into leaves of N. benthamiana as previously reported (Yang et al., 2021). After 48 h, the leave samples were taken separately, the proteins were extracted and the activity was determined.
Phenotypic analysis of transgenic Arabidopsis over-expression MfERF053
MfERF053 gene was cloned into the plant overexpression vector pB2GW7 and transformed into the Agrobacterium tumefaciens strain GV3101 for transformation in Arabidopsis by using floral dipping method. Three over-expression lines (OE19, OE20 and OE33) of T3 generation and wild type Columbia-0 (Col) plants were used for subsequent phenotype analyses. Seedlings were grown in soil at 24°C (16 h light/8 h darkness), 70%-80% relative humidity, and 400 μmol·m-2·s-2 light intensity.
We transferred four-day-old transgenetic and wild type Arabidopsis plants that were germinated on plates containing 1/2 MS medium to plates containing 1/2 MS medium supplied with different concentrations of mannitol (300 mM and 400 mM), NaCl (100 mM, 125 mM, 150 mM and 200 mM) and ABA (50 μM, 100 μM, 150 μM and 200 μM). Root length, lateral root number and fresh weight were measured after 10-day treatment. Each measurement contained 10 seedlings with triplicates. Seedlings grown in soil under normal conditions for 30 days were used for stress treatment. For drought stress, seedlings were grown without water for 15 days, then rewatered for 5 days. For salt stress, transgenic and wild type Arabidopsis plants were treated with 300 mM NaCl for 7 and 12 days, and the seedlings were photographed, respectively.
Results
Analysis of phenotypic and physiological indicators of M. falcata under drought stress
Initially, four-week-old M. falcata seedlings were subjected to mannitol treatment with concentration of 200 mM, 300 mM, 400 mM, 500 mM, and 600 mM. Mannitol treatment inhibited the growth of M. falcata, and the root length gradually decreased and the number of lateral roots significantly decreased with the increase of mannitol concentration (Figure S1A). These seedlings were more severely stressed and showed purplish-red root color and wilted leaves at mannitol concentration of 500 mM and 600 mM (Figure S1A), thus a relatively lower concentration of 400 mM mannitol was selected for subsequent experiments.
Seedlings were subjected to mannitol treatment (400 mM) at different time points (0 h, 2 h, 6 h, 12 h, 24 h, 36 h and 48 h). The seedlings grew normally at 2 h, and the leaves appear slightly wilted at 6 h, and started to lose water at 24 h, and finally the leaves wrinkled and severely wilted at 48 h (Figure S1B). It was clear that leaf wilting became more severe with stress time, and M. falcata responded to mannitol stress in a relatively short period of time.
To study the effect of drought stress on physiology changes in M. falcata, seedlings stressed with 400 mM mannitol at different time points were subjected to determine physiological indicators, including content of MDA, proline, and activity of SOD, POD and CAT (Figure 1). MDA content gradually increased from 2 h to about 55 μmol/g, and then gradually increased from 6 h to 48 h and reached the highest content at 48 h, indicating that the cells were most severely damaged by the treatment (Figure 1A). Drought stress significantly affected proline content in M. falcata plants at later stage (Figure 1B), with 10-fold increase (p<0.01) to 200 μg/g at 24 h, reaching maximum level at 48 h compared to untreated samples (Figure 1C). SOD activity of M. falcata plants increased with treatment time, began to increase significantly (p<0.05) after 2 h, and reached maximum level of approximately 280 U/g at 48 h, an increase of 186% compared to the control (Figure 1C). In terms of POD activity, the greatest increase was observed after 2 h treatment, with a slight increase at 6 h followed by a decrease at 12 h, with no significant differences from 24 h to 48 h (Figure 1D). CAT activity increased from 2 h to 36 h with its maximum value of 4200 U/g-1/min-1 at 12 h (Figure 1E). In conclusion, these physiological indicators of M. falcata responded to mannitol stress at different treatment time points with different degree.
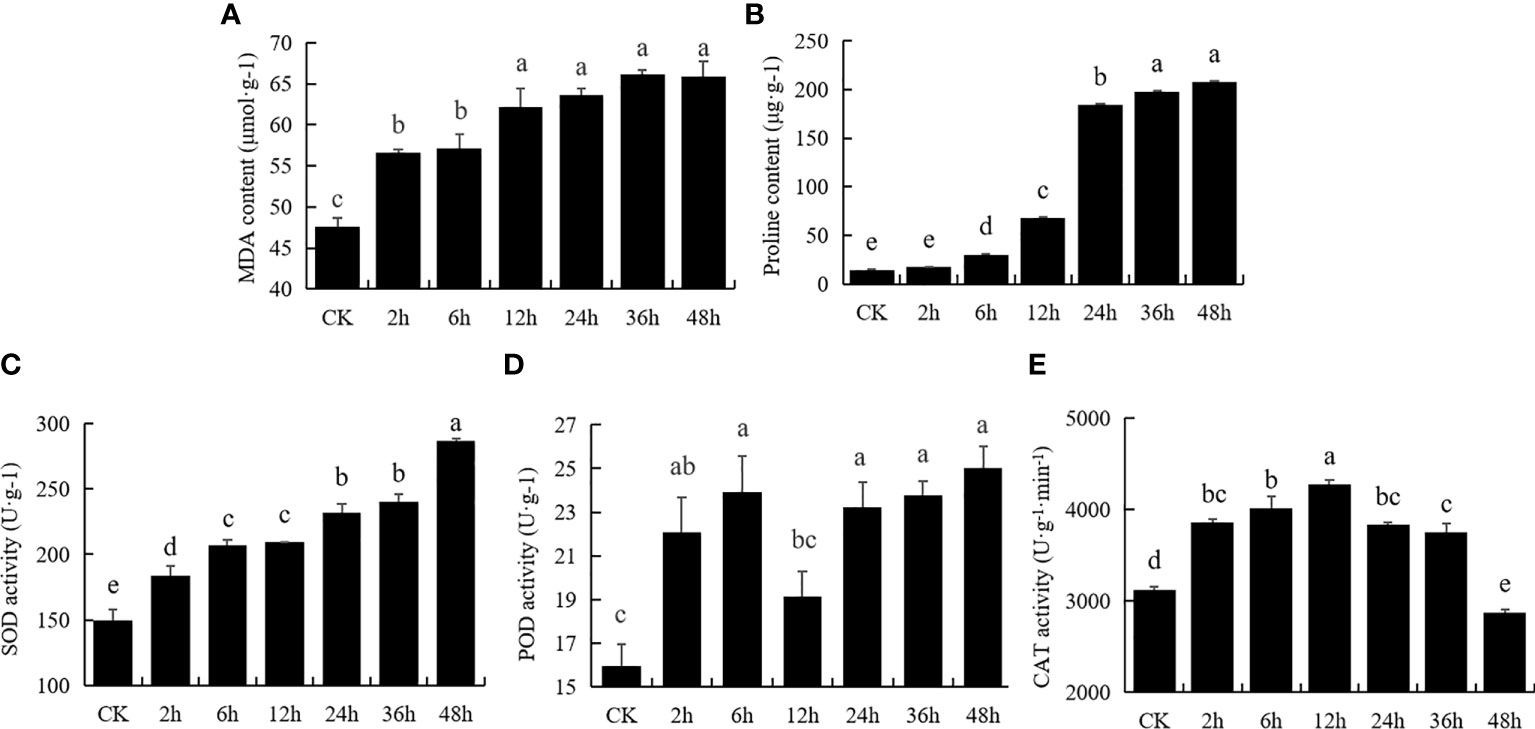
Figure 1 Physiological response of Medicago falcata to 400 mM mannitol treatment. Plant samples were collected under normal conditions and treated with 400 mM mannitol for 2 h, 6 h, 12 h, 24 h, 36 h and 48 h. The values are the average and error of three biological replicates. The same letters mean no significant difference, and different letters mean significant difference. (A), MDA content; (B), Proline content; (C), SOD activity; (D), POD activity; (E), CAT activity.
De novo transcriptome assembly and functional annotation of unigenes
The 21 cDNA libraries yielded 8,624,521,500 bp raw reads (Table S2), the clean data is 8,537,671,848 bp, the Q30 before and after filtration was relatively high (around 94%), and the GC content was around 44% (Table S2), indicating good sequencing quality. In order to assemble the sequencing data, the reference genome of alfalfa ecotype Xinjiang Daye was selected as the reference genome for comparison, and the sequencing results were assembled and annotated as shown in Table S3. For all samples, the unmapped reads were only about 6.92-10.78%, the unique mapped reads were 39.86-42.11%, and the total mapped reads accounted for 89.22-93.68%, thus these data clearly indicated that the genome sequences of M. falcata have a very high matching rate with that of M. sativa, which can be used for subsequent analysis.
Identification and analysis of expression pattern of DEGs
Principal component analysis (PCA) showed that samples from CK were clustered into a separate category and they were separated away from the samples under stress treatments (Figure S2). Samples from 2 h and 6 h treatments were closer than with other treatments, samples from 12 h treatments were clustered into a separate category, and samples from 24 h, 36 h and 48 h with long duration stress treatments were clustered into one category (Figure S2). These data together indicated that samples from different treatments tend to cluster differently.
The FPKM values for different samples were analyzed to investigate the changes in gene expression and to identify critical genes involved in drought stress in M. falcata. The volcano plot can be used to visualize the differentially expressed genes between treatment groups and control group. A total of 16,304 DEGs were obtained from transcriptome of M. falcata at 7 treatment time points. Among them, total 3,426, 3,632, 4,543, 3,944, 2,898, and 3,408 genes were down-regulated at 2 h, 6 h, 12 h, 24 h, 36 h and 48 h when compared with the control group at 0 h, respectively (Figure 2A). Meanwhile, 1,232, 2,165, 3,723, 4,156, 2,932, and 3,916 genes were down-regulated at 2 h, 6 h, 12 h, 24 h, 36 h and 48 h when compared with the control group at 0 h, respectively (Figure 2A).
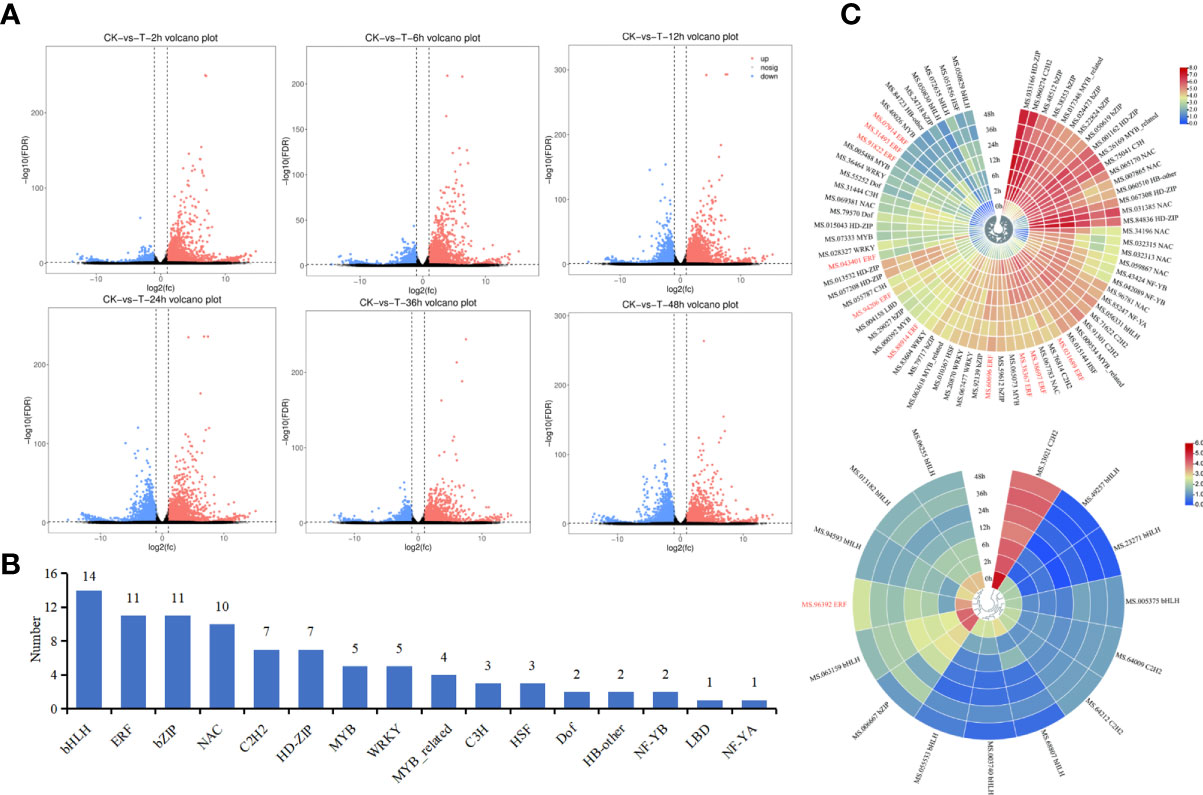
Figure 2 Analysis of differentially expressed genes at each time point under mannitol treatment. (A), Volcano of difference among treatments. The horizontal coordinate indicates the log2(FC) of the difference between two groups, and the vertical coordinate indicates the negative log10 value of the FDR of the difference between the two groups. Red (up-regulated expression of group_2 relative to group_1) and blue (down-regulated expression) points indicate difference in gene expression (judged by FDR < 0.05, and more than two-fold difference), and black points indicate no difference. (B), Statistic analysis of the number of different types of transcription factors. (C), Heat map clustering of transcription factor expression. Red represents high expression and blue represents low expression. MfERF genes were highlighted in red.
Among these DEGs, 88 of them were found to be transcription factor genes belonging to 16 TF families (Figure 2B), including 14 bHLH genes, 11 ERF genes, 11 bZIP genes, and 10 NAC genes. Heat maps for the expression profiles of these 88 transcription factor genes under drought treatment showed that 73 of them were up-regulated compared with the control group (0 h) (Figure 2C, top), whereas 15 of them were down-regulated (Figure 2C, bottom). Among them, 10 out of 11 ERF genes were up-regulated (Figure 2C), indicating they may play leading roles in drought resistance in M. falcata.
WGCNA of common DEGs in drought stress
In order to further investigate potential key genes involved in drought response in M. falcata, the weighted gene co-expression network was constructed using WGCNA, resulting in eight modules (Figure S3). In order to explore the correlation of these clustered modules with the above-mentioned physiological indicators, correlation analysis was performed between the module eigenvalues with activity of POD, CAT, SOD, content of Pro and MDA. Heat map was used to display the top correlation coefficient (Figure 3). The correlation coefficient between the black module and three indicators (MDA, SOD and Pro) were relatively high with values of 0.83, 0.88 and 0.92 (significance of 4e-06, 2e-07 and 3e-09), respectively (Figure 3). Grey60 module was significantly correlated with SOD activity and Pro content with correlations coefficient of 0.72 and 0.88, respectively (Figure 3). Both the lightcyan and darkgreen modules showed highly significant positive correlations with CAT activity, with correlation coefficients of 0.75 and 0.64 (significance of 1e-04 and 0.002), respectively (Figure 3). These data clearly indicated that four modules, namely black module, Grey60 module, lightcyan module and darkgreen module, were likely contain genes involved in drought responsive in M. falcata.
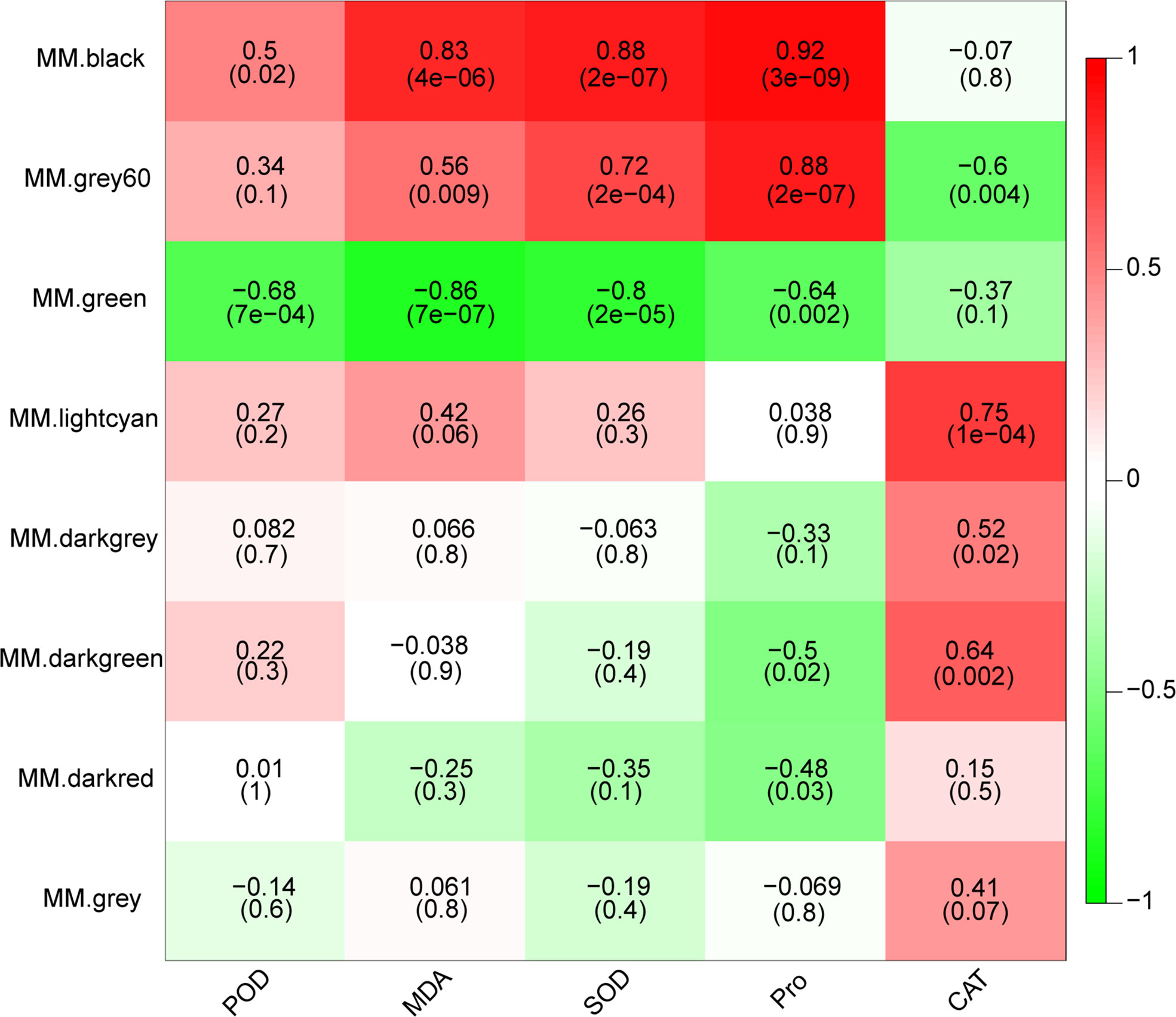
Figure 3 Character correlation diagram of physiological indicators. The horizontal coordinates indicate the character and the vertical coordinates indicate the module, which is plotted with Pearson correlation coefficient. Red represents positive correlation and green represents negative correlation. The darker the color, the stronger the correlation. The number in the brackets below represents significance P-value. The smaller the value, the stronger the significance.
Screening of candidate gene among DEGs
The four modules (black, Grey60, lightcyan and darkgreen) were up-regulated modules, but they differed in expression patterns, which was consistent with the expected expression pattern of concern. The darkgreen module was up-regulated in early stage (2 h and 6 h) but down-regulated in the late stage (12 h, 36 h and 48 h, Figure 4A). The lightcyan module was up-regulated in the early and middle stage (2 h, 6 h, 12 h) and down-regulated in the late stage (36 h and 48 h, Figure 4B). The black and grey60 modules showed a higher expression in the late stage (36 h and 48 h) than the earlier time points (Figure 4C, D). Furthermore, venn diagram with these four modules and ERF genes showed that 6 ERF genes were expressed in two out of four modules (Figure 4E). Among these 6 genes, both MS.gene31493 and MS.gene043401 from the darkgreen modules were two different transcripts for the same gene MfERF053, and MS.gene38367 and MS.gene38697 were for MfRAP2-1 gene (Table S4), therefore, the expression of these four genes, namely MfERF053, MfERF9, MfRAP2-1 and MfERF034 were further verified by qRT-PCR. It was revealed that the expression of these four genes were up-regulated to different levels under drought treatment at different time points (Figure 4F), which is consistent the transcriptome sequencing data as verified by correlation analyses (Figure S4). Among these four genes, MfERF053 showed the highest expression level, which was thus selected for further investigation.
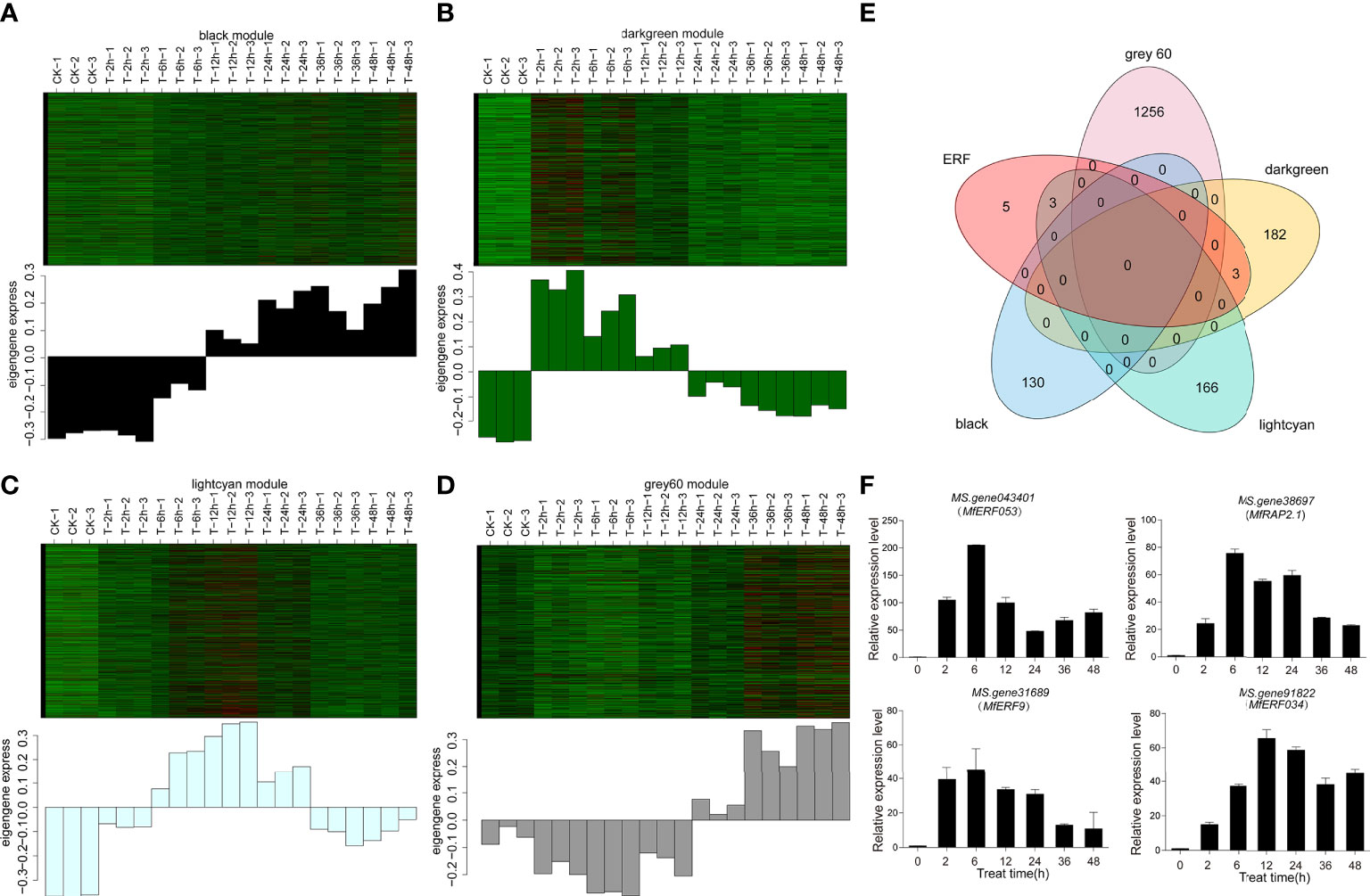
Figure 4 Differential candidate gene analysis. (A–D), Heat map of gene expression pattern of each module. The above figure showed the expression level map of genes in modules in different samples; The following figure shows the characteristic values of modules in different samples. (A–D) represent darkgreen, lightcyan, black and gray60 module, respectively. (E), The number of transcription factors differentially expressed in the five candidate combinations were screened by Venn map. Black, lightcyan, darkgreen and grey60 represent the three candidate modules screened by WGCNA, and ERF represents the number of ERF genes common shared by all treatments and controls. (F), The expression level of four MfERF genes under mannitol treatment at different time point as detected by qRT-PCR.
Cloning, multiple sequence alignment and evolutionary tree analysis
The full-length open reading frame of MfERF053 was cloned and the sequence was submitted to the National Center for Biotechnology Information (NCBI) under GenBank accession number of OM970125. Multiple sequence alignment of MfERF053 with ERF053 from other plant species showed that MfERF053 shared 95%, 56%, 54% similarity with its homology genes from M. truncatula, Vigna angularis and Glycine soja at amino acid level (Figure 5A). All proteins from M. truncatula, G. soja, V. angularis, Arabidopsis thaliana, Vigna radiata var. radiata, M. sativa, Medicago ruthenica shared the characteristics features of DREB proteins, with conserved YRG, WLG, and RAYD motifs (Figure 5A).
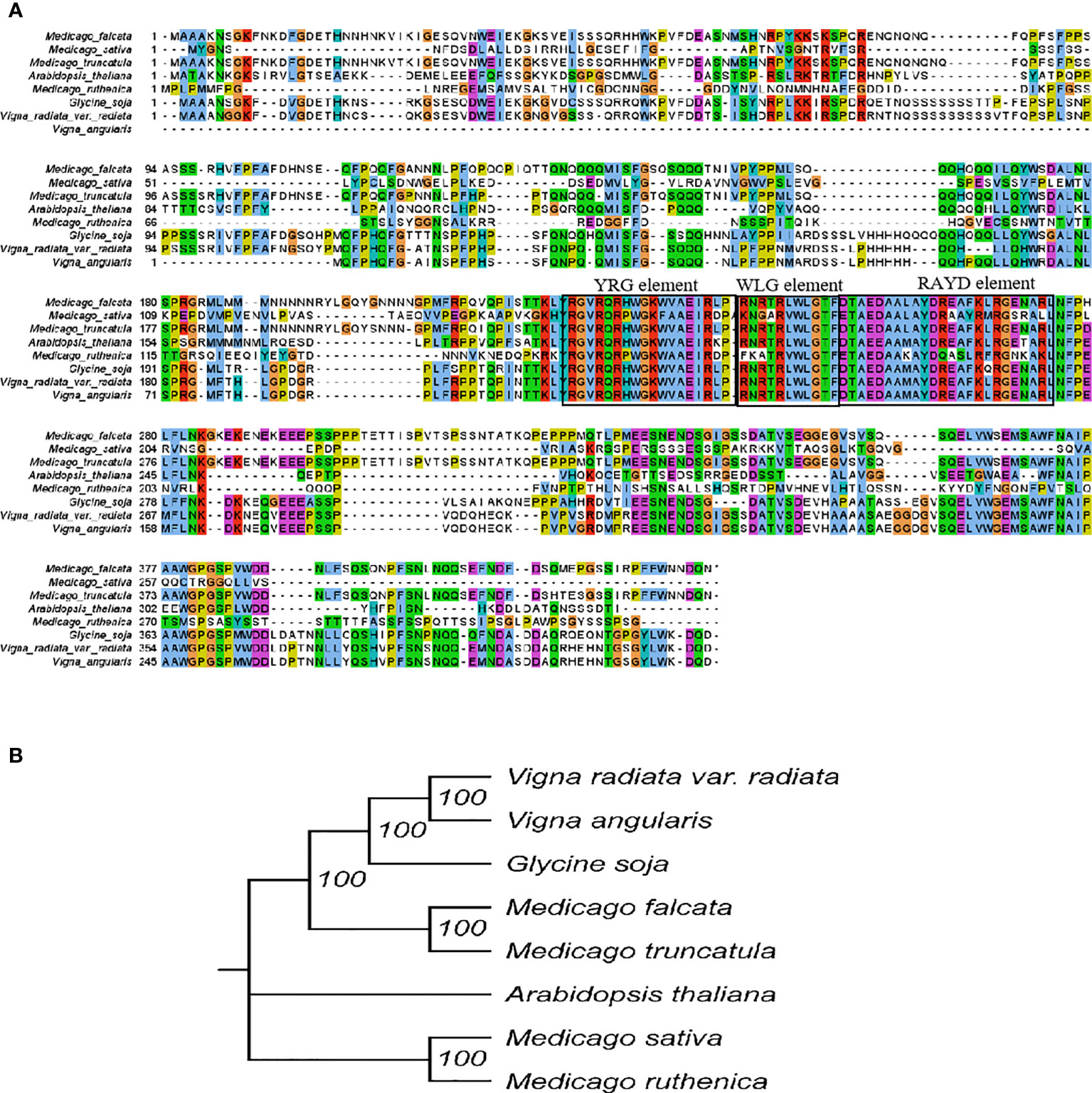
Figure 5 Sequence analysis of plant ERF genes. (A), Multiple sequence alignment. The alignment were constructed by MEGA-X and visualized by Jalview. Residues with more than 50% similarity were shaded. Conserved regions (YRG element, WLG element and RAYD element) were indicated at the top. (B), Phylogenetic analysis ERF053 genes from different plant species.
Phylogenetic analyses revealed that MfERF053 was clustered with ERF053 from M. truncatula and G. soja, which was separated from those of A. thaliana, M. sativa, M. ruthenica with relatively low sequence similarity (51%, 49% and 48%, respectively, Figure 5B).
Expression profile and transcriptional activity of MfERF053
We analyzed relative expression level of MfERF053 gene by qRT-PCR in roots, stems, leaves, flowers, branches and inflorescences of seedlings of M. falacata under normal growth condition. It was found that MfERF053 was most highly expressed in roots, followed by in leaves and stems (Figure 6A). This finding suggests that MfERF053 may be involved in mediating drought stress signaling through roots.
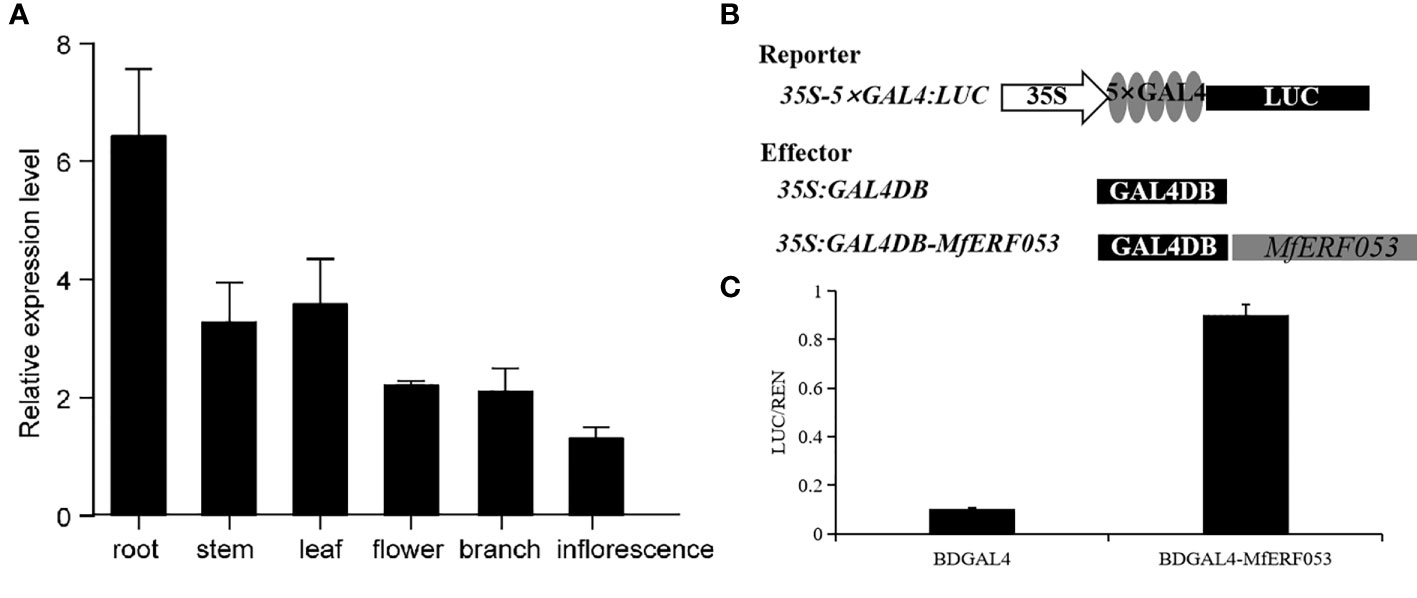
Figure 6 Characteristic of MfERF053 gene. (A), Validation of expression pattern of M. falcata in various tissues by qRT-PCR. (B), Schematic diagram of reporter and effector for MfERF053 gene for transactivation assay. (C), MfERF053 transcriptional activity analysis.
To investigate whether MfERF053 possesses transactivation activity, we generated a transactivation construct (35S::GAL4DB- MfERF053) and expressed it in tobacco epidermal cells by Agrobacterium-medicated transformation, using GAL4DB as a negative control (Figure 6B). It was revealed that MfERF053 could activate the GAL4-responsive expression of the LUC reporter protein, and the relative luciferase activity for MfERF053 were about 9 times higher than the control (GALDB), indicating that MfERF053 acts as a transcription activator (Figure 6C).
Over-expression of MfERF053 in Arabidopsis enhanced resistance under plate culture condition
qRT-PCRs were performed to measure the expression levels of MfERF053 in leaves of the transgenic Arabidopsis of the T3 generation. Among twenty-five lines that were detected, three independent transgenic lines with relatively high expression level were selected for further analyses (lines OE19, OE 20, and OE 33). No significant difference were observed between the transgenic line and the wild type control under normal plate culture condition (Figure S5). Both the transgenic lines and the wild-type Arabidopsis seedlings were grown on plates supplied with mannitol of different concentrations (300 mM and 400 mM), NaCl (100 mM, 125 mM, 150 mM, and 200 mM) and ABA (50 μM, 100 μM, 150 μM and 200 μM) (Figure 7, Figure S6, Figure S7, Figure S8).
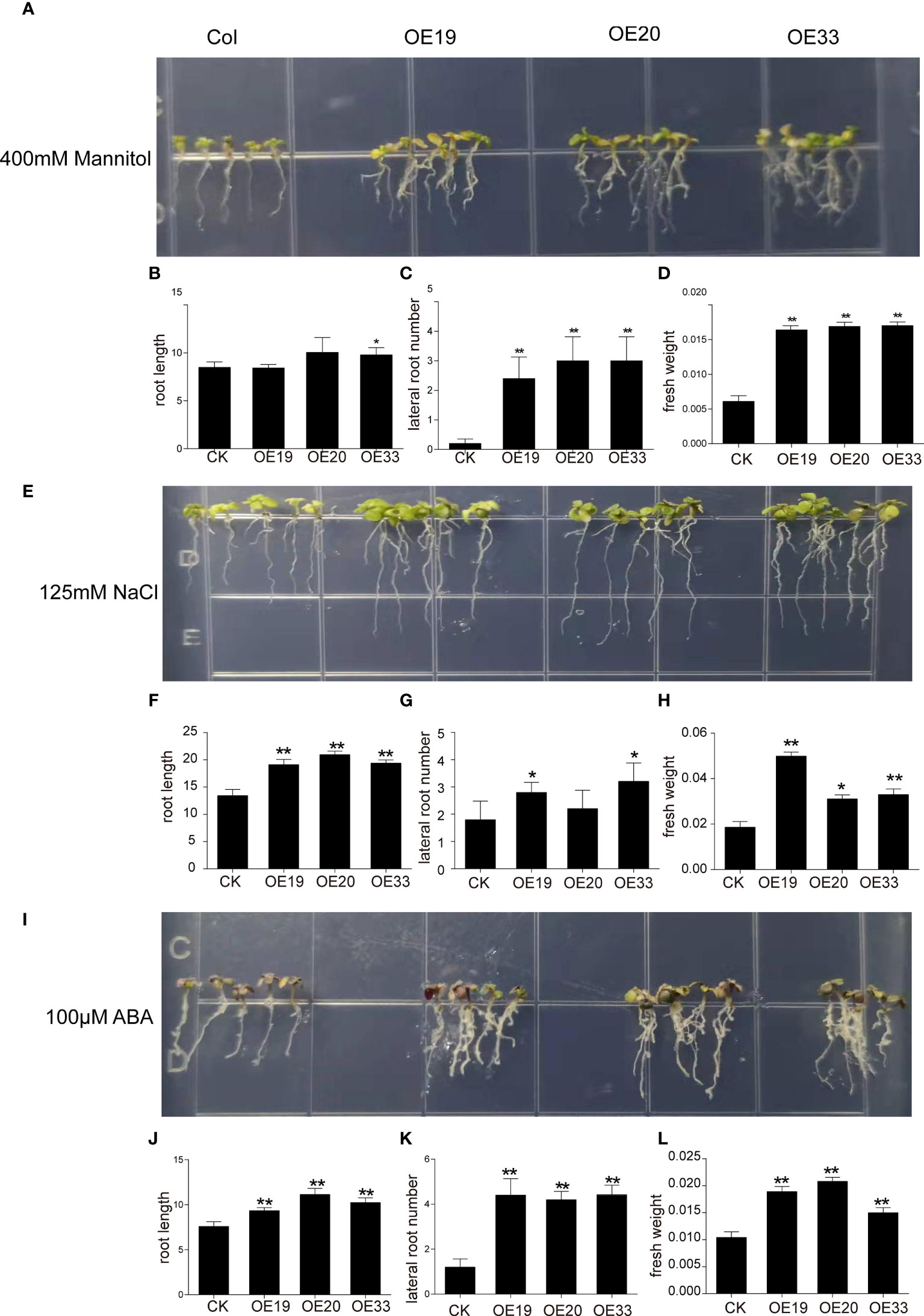
Figure 7 Evaluation of different resistance of MfERF053 transgenic Arabidopsis. (A, E, I), Plants overexpression MfERF053 were treated with 400 mM mannitol, 100 mM NaCl and 100 μM ABA with wide type, respectively. (B, F, J), Root length of different plant lines. (C, G, K), Lateral root number of different plant lines. (D, H, L), Fresh weight of different plant lines. Three replicates per treatment, 6 plants per replicate, * p < 0.05, ** p < 0.01, Duncan’s t-test.
When treated with 300 mM mannitol and 400 mM mannitol for 10 days, the root length of the overexpression lines did not show any difference from the control (Figure 7A, Figure S6), but the number of lateral roots and fresh weight increased significantly compared with the control (p < 0.05). Moreover, the increase in both lateral root number and fresh weight under 400 mM mannitol treatment were greater than those with 300 mM mannitol treatment (Figures 7B–D, Figure S6).
After 10 days of treatment with 100 mM NaCl, overexpression lines grew significantly better than the wild type, showing longer root length and increased number of lateral roots (Figure S7). After 10 days of treatment with 125 mM NaCl stress, the chlorophyll content of the leaves in the wild-type plants decreased with yellow leaves, while the overexpression lines showed green leaves and increased in root length, number of lateral roots and fresh weight (Figures 7E–H). After 10 days of treatment with 150 mM NaCl stress, the wild-type plants turned white, but the overexpression lines remained green, with significantly more green leaves and fibrous root than the wild-type (Figure S7). After 10 days of treatment with 200 mM NaCl stress, both the wild-type and overexpression lines turned white, and their growth were severely inhibited, but leave color of the overexpression lines changed to light purple and some leaves turned white (Figure S7).
In addition, the wild-type and overexpression Arabidopsis were grown on plates supplied with different concentrations of ABA treatment for 10 d (Fig 7I, Fig S7). It was found that the leaves of the overexpression lines showed darker green and the number of lateral root increased after ABA stress with low concentration (50 and 100 μM) for 10 d (Figure 7I, Figure S8). However, high ABA concentration inhibited the growth of Arabidopsis roots, but the transgenic lines grew better than the wild-type (Figure S8). The root length, lateral root number and fresh weight of the overexpression lines were significantly increased compared with the wild type under ABA treatment (p < 0.01), indicating that overexpression of MfERF053 gene could improve the sensitivity to ABA on root growth in Arabidopsis under certain concentration (Figures 7J–L, Figure S8).
Over-expression of MfERF053 in Arabidopsis enhanced resistance grown in soil
Three transgenic Arabidopsis strains (OE19, OE20 and OE33) showed no significant difference with the wild type control when plants grew in soil under natural watering condition (Figure 8A). But after 12 days of drought stress, wild-type Arabidopsis was more severely stressed than the transgenic lines (Figure 8B). The wild type plants turned significantly purple, whereas some of the older leaves of the transgenic lines were still green or green-yellow (Figure 8B). When drought stress was continued for 15 d, the wild-type Arabidopsis plants withered and could not grow, while the transgenic Arabidopsis continued to grow and flowered, and the leaves remained green (Figure 8C). After rehydration for 5 days, the wild-type Arabidopsis plants did not recovered and dried completely, while the overexpression Arabidopsis plants grew well and the leaves became green and the inflorescences could develop into pods normally, indicating that the transgenic Arabidopsis plants over-expressing MfERF053 have conferred drought resistance (Figure 8D).
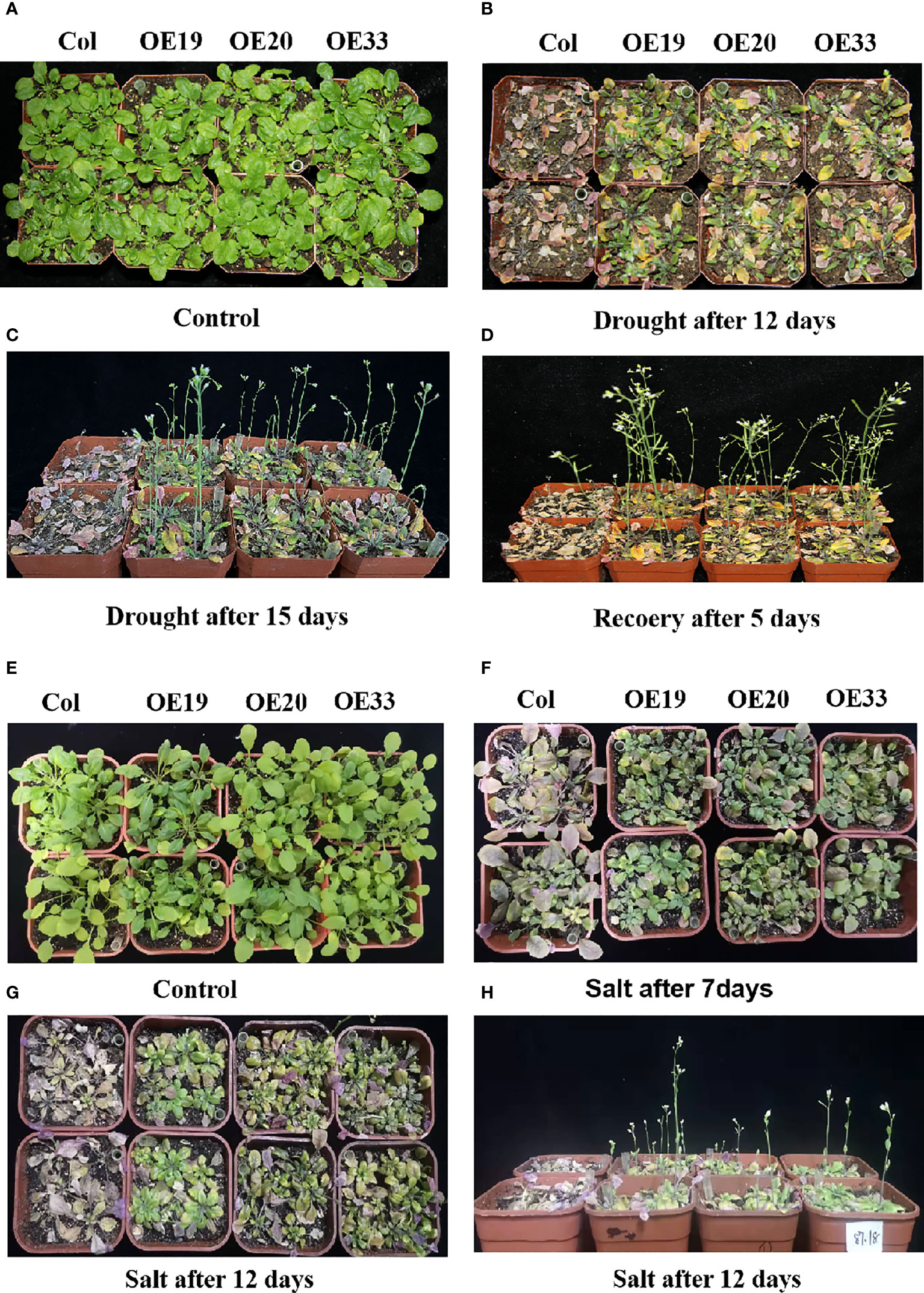
Figure 8 Phenotype of MfERF053 transgenic overexpression Arabidopsis under drought NaCl and treatment in soil. (A-D), The phenotype of wide type and transgenic of Arabidopsis under normal (A), drought after 12 days (B), drought after 15 days (C) and recover after 5 days (D), respectively. (E-H): The phenotype of wide type and transgenic of different strains of Arabidopsis under normal (E), salt after 7 days (F), salt after 12 days (H) and salt after 12 days (I), respectively.
Both the transgenic and the wild type Arabidopsis were treated with 300 mM NaCl in the soil (Figure 8E). After 7 d of treatment, the leaves of the wild-type plants turned purple with significantly reduced chlorophyll, while the transgenic Arabidopsis remained green with few leaves turning purple (Figure 8F). After continuous stress for 12 days (Figure 8G), the wild-type Arabidopsis withered and died, while the transgenic Arabidopsis remained green with some old leaves turning purple, and they could still flower normally and grow (Figure 8H), indicating that the transgenic Arabidopsis conferred salt resistance when compared with the wild-type.
Discussion
Among various environmental stress, drought is one of most serious stresses affecting the growth and development of plant. Drought stress triggers a series of responses from morphology to physiology, and to gene level. With the increase of drought stress, the antioxidant system of plants is destroyed, and the free radicals produced are greater than those cleared, resulting in excessive accumulation of ROS and membrane damage (Puyang et al., 2015; Nahar et al., 2017). In this study, the detailed information of physiological and transcriptome data of M. falcata under drought stress was provided. Under drought stress, the activities of SOD, POD and CAT increased (Figure 1), and the contents of proline and MDA increased, indicating that M. falcata has better ROS scavenging ability toward drought. It can be proven that the increase of these enzyme activities can eliminate stress-induced ROS and peroxides, inhibit plasma membrane peroxidation, and protect cells from damage (Zhang et al., 2004; Anjum et al., 2011; Koh et al., 2015; Quan et al., 2015; Xiong et al., 2022). The results of physiological indexes showed that M. falcata is capable of reducing the accumulation of harmful substances by regulating the activity of defense enzymes.
M. falcata is one of the candidate models to study abiotic stress response mechanism in legumes (Miao et al., 2015). The transcriptome analysis of drought provides a new biochemical and molecular mechanism for abiotic stress adaptation. Taking the genome of M. sativa cv ‘Xinjiang Daye’ as the reference genome, the tetraploid M. falcata transcriptome was sequenced, assembled and annotated, resulting in a total of 172,892 genes, with a comparison rate of more than 75% with the reference genome. The annotation of most genes is more similar to the annotation information of M. truncatula, indicating that the M. falcata transcriptome has good homologous sequence coverage, and it will also provide evidence for the expression of predicted genes in the genome of M. truncatula (Miao et al., 2015). Compared with the other transcriptome data for alfalfa with similar treatment (Luo et al., 2019), this study seems more complete. The number of genes obtained in each library in this study is higher than that produced in transcriptome of alfalfa under abiotic stress, which may be due to different samples used for sequencing. The sample for alfalfa is the roots, while the sample of this study is the whole seedlings. Therefore, the genes obtained for the transcriptome of alfalfa may be the genes specifically expressed in the roots, while in this study the obtained genes may be widely expressed in various parts.
In plants, some studies have screened stress resistance related genes through transcriptome sequencing (Gao et al., 2021; Li et al., 2022). In this study, we used M. sativa cv Xinjiang Daye as the reference genome, screened candidate genes through RNA-seq technology, and used expression patterns analysis and WGCNA analysis to mine hub ERF genes in response to dehydration of M. falcata (Figures 3, 4). The biological functions of these genes need to be further explored and verified, but at least qRT-PCR for these four candidate genes were consistent with the transcriptome data, which proves the reliability of WGCNA co-expression network analysis method. In conclusion, this strategy of screening functional genes related to drought stress is of great significance to the study of stress resistance in M. falcata as in other studies (Qin et al., 2020).
A drought responsive MfERF053 gene in M. falcata was screened in this study by transcriptome sequencing (Figure 4). Previous studies have reported that ERF transcription factors act as both activators and repressors of transcriptional functions (Yant et al., 2010). MfERF053 was shown to have transcriptional activation activity based on transcriptional activity assays (Figure 6B), which is similar as that of the SlERF3 gene (Pan et al., 2010). The activation activity of MfERF053 is consistent with its lack of an EAR (ERF-associated amphiphilic repression) inhibitory element, therefore, it is reasonable that MfERF053 is involved in different biological processes mainly in the form of activator.
Compared with the wild type, overexpression lines with higher expression level of MfERF053 showed significant changes in root length and lateral roots as well as fresh weight (Figures 7, 8), indicating that overexpression of MfERF053 had a significant effect on root growth in Arabidopsis. It has been shown that overexpression of the apple MdERF11 and MdERF106 genes (Han et al., 2020) can significantly improve the growth characteristics of plants to withstand abiotic stresses. Initial studies found that higher expression of AtERF53 showed no significant difference in dehydration tolerance from wild type, it is speculated that the AtERF53 protein requires or may require post-translational modifications controlled by another mechanism (Hsieh et al., 2013). In addition, other studies showed that the E3 ligase RGLG1 E3 can promote the degradation of PP2CA through an ABA dependent pathway, and the RING E3 ligase RGLG2 interacts with AtERF53 to negatively regulate drought stress response in Arabidopsis (Cheng et al., 2012; Belda-Palazon et al., 2019). GmERF113 can enhance the drought resistance through activating the expression of PR10-1 by binding to the GCC-box in soybean plants (Fang et al., 2022). Overexpression of the GmERF75 gene in soybean hairy roots showed stronger growth than wild type under 100 μmol/L-1 ABA and 120 mM NaCl treatment, indicating that overexpression of GmERF75 improved soybean tolerance to salinity and exogenous ABA (Zhao et al., 2019). All these results suggested that ERF genes have a conserved role in response to abiotic stresses in different plant species. In this study, overexpression of MfERF053 improved the resistance of Arabidopsis to osmotic stress through the ABA transduction pathway. Nevertheless, the regulation mechanism of MfERF053 on drought and salt resistance in both M. falcata and in transgenic Arabidopsis requires further investigation in the near future.
Data availability statement
The datasets presented in this study can be found in online repositories. The names of the repository/repositories and accession number(s) can be found in the article/Supplementary Material.
Author contributions
QL and WJ designed this experiment, performed the experiments, and drafted the manuscript. ZJ, WD, JS, ZQ analyzed experimental data, visualized and perform experiment. BZ, YW and YP revised the manuscript and directed the study. All authors contributed to the article and approved the submitted version.
Funding
This research was funded by National Nature Fund (31860675 and U1906201) and China Agriculture Research System of MOF and MARA (CARS-34).
Conflict of interest
The authors declare that the research was conducted in the absence of any commercial or financial relationships that could be construed as a potential conflict of interest.
Publisher’s note
All claims expressed in this article are solely those of the authors and do not necessarily represent those of their affiliated organizations, or those of the publisher, the editors and the reviewers. Any product that may be evaluated in this article, or claim that may be made by its manufacturer, is not guaranteed or endorsed by the publisher.
Supplementary material
The Supplementary Material for this article can be found online at: https://www.frontiersin.org/articles/10.3389/fpls.2022.995754/full#supplementary-material
Supplementary Table 1 | Primers used in this experiment.
Supplementary Table 2 | Statistical information on transcriptome sequencing data.
Supplementary Table 3 | Transcriptome alignment statistics.
Supplementary Table 4 | Details of candidate genes.
Supplementary Figure 1 | Phenotypic changes of M. falcata under mannitol treatment. (A), Morphological response of M. falcata to different concentrations of mannitol. (B), Effect of 400 mM mannitol drought stress at different times on leaf morphology of M. falcata.
Supplementary Figure 2 | Principle component analysis of M. falcata at different times of 400 mM mannitol.
Supplementary Figure 3 | Gene clustering map by WGCNA analysis. Left, Sample Hierarchical Clustering Tree. Right, Module level clustering diagram.
Supplementary Figure 4 | Correlation analysis between qRNA-seq and RT-PCR data for the four candidate MfERF genes.
Supplementary Figure 5 | Phenotype of MfERF053 overexpression plants under normal plate growth condition. Col, the wide type control; Three over-expression lines: OE19, OE20 and OE33.
Supplementary Figure 6 | Evaluation of overexpression plants MfERF053 were treated with 300 mM mannitol. (A), Overexpression of Arabidopsis 10-day phenotype under 300mM mannitol treatments. (B), Root length of different plant lines. (C), Lateral root number of different plant lines. (D), Fresh weight of different plant lines.
Supplementary Figure 7 | Evaluation of overexpression plants MfERF053 were treated with different salt concentrations. (A, E, I), Overexpression of Arabidopsis 10-day phenotype under 100 mM, 150 mM and 200 mM mannitol treatments, respectively. (B, F, J), Root length of different plant lines. (C, G, K), Lateral root number of different plant lines. (D, H, L), Fresh weight of different plant lines.
Supplementary Figure 8 | Evaluation of overexpression plants MfERF053 were treated with different ABA concentrations. (A, E, I), Overexpression of Arabidopsis thaliana 10-day phenotype under 100 μM, 150 μM and 200 μM ABA treatments, respectively. (B, F, J), Root length of different plant lines. (C, G, K), Lateral root number of different plant lines. (D, H, L), Fresh weight of different plant lines.
References
Anjum, S. A., Wang, L., Farooq, M., Khan, I., Xue, L. (2011). Methyl jasmonate-induced alteration in lipid peroxidation, antioxidative defence system and yield in soybean under drought. J. Agron. Crop Sci. 197 (4), 296–301. doi: 10.1111/j.1439-037X.2011.00468.x
Belda-Palazon, B., Julian, J., Coego, A., Wu, Q., Zhang, X., Batistic, O., et al. (2019). ABA inhibits myristoylation and induces shuttling of the RGLG1 E3 ligase to promote nuclear degradation of PP2CA. Plant J. 98, 813–825. doi: 10.1111/tpj.14274
Cheng, M. C., Hsieh, E. J., Chen, J. H., Chen, H. Y., Lin, T. P. (2012). Arabidopsis RGLG2, functioning as a RING E3 ligase, interacts with AtERF53 and negatively regulates the plant drought stress response. Plant Physiol. 158, 363–375. doi: 10.1104/pp.111.189738
Chen, H. T., Zeng, Y., Yang, Y. Z., Huang, L. L., Tang, B. L., Zhang, H., et al. (2020). Allele-aware chromosome-level genome assembly and efficient transgene-free genome editing for the autotetraploid cultivated alfalfa. Nat. Commun. 11 (1), 2494–2505. doi: 10.1038/s41467-020-16338-x
Chen, S. F., Zhou, Y. Q., Chen, Y. R., Gu, J. (2018). Fastp: an ultra-fast all-in-one FASTQ preprocessor. Bioinformatics 34 (17), 884–890. doi: 10.1093/bioinformatics/bty560
Dong-Keun, L., Suin, Y., Youn, S., Hyunyong, K. (2016). Rice OsERF71-mediated root modification affects shoot drought tolerance. Plant Signal Behav. 12 (1), e1268311. doi: 10.1080/15592324.2016.1268311
Duan, M., Zhang, R., Zhu, F., Zhang, Z., Gou, L., Wen, J., et al. (2017). A lipid-anchored NAC transcription factor is translocated into the nucleus and activates glyoxalase I expression during drought stress. Plant Cell 29 (7), 1748–1772. doi: 10.1105/tpc.17.00044
Fang, X., Ma, J., Guo, F., Qi, D., Zhao, M., Zhang, C., et al. (2022). The AP2/ERF GmERF113 positively regulates the drought response by activating GmPR10-1 in soybean. Int. J. Mol. Sci. 23 (15), 8159–8181. doi: 10.3390/ijms23158159
Gao, G., Lv, Z., Zhang, G., Li, J., Zhang, J., He, C. (2021). An ABA-flavonoid relationship contributes to the differences in drought resistance between different sea buckthorn subspecies. Tree Physiol. 41 (5), 744–755. doi: 10.1093/treephys/tpaa155
Gibbs, D., Conde, J. V., Berckhan, S., Mendiondo, G. M., Prasad, G., Holdsworth, M. J. (2015). Group VII ethylene response factors coordinate oxygen and nitric oxide signal transduction and stress responses in plants. Plant Physiol. 169 (1), 23–31. doi: 10.1104/pp.15.00338
Gou, L., Zhuo, C., Lu, S., Guo, Z. (2020). A universal stress protein from Medicago falcata (MfUSP1) confers multiple stress tolerance by regulating antioxidant defense and proline accumulation. Environ. Exp. Bot. 178, 104168–104179. doi: 10.1016/j.envexpbot.2020.104168
Gupta, A., Rico-Medina, A., Cao-Delgado, A. I. (2020). The physiology of plant responses to drought. Science 368 (6488), 266–269. doi: 10.1126/science.aaz7614
Haake, V., Cook, D., Riechmann, J. L., Pineda, O., Thomashow, M. F., Zhang, J. Z. (2002). Transcription factor CBF4 is a regulator of drought adaptation in arabidopsis. Plant Physiol. 130 (2), 639–648. doi: 10.1104/pp.006478
Han, D., Han, J., Yang, G., Wang, S., Xu, T., Li, W. (2020). An ERF transcription factor gene from Malus baccata l. borkh, MbERF11, affects cold and salt stress tolerance in Arabidopsis. Forests 11 (5), 514–529. doi: 10.3390/f11050514
Hsieh, E. J., Cheng, M. C., Lin, T. P. (2013). Functional characterization of an abiotic stress-inducible transcription factor AtERF53 in Arabidopsis thaliana. Plant Mol. Biol. 82 (3), 223–237. doi: 10.1007/s11103-013-0054-z
Jung, H., Chung, P. J., Park, S. H., Redillas, M., Kim, Y. S., Suh, J. W., et al. (2017). Overexpression of OsERF48 causes regulation of OsCML16, a calmodulin-like protein gene that enhances root growth and drought tolerance. Plant Biotechnol. J. 15 (10), 1295–1308. doi: 10.1111/pbi.12716
Kang, Y., Han, Y., Torres-Jerez, I., Wang, M., Tang, Y., Monteros, M., et al. (2011). System responses to long-term drought and re-watering of two contrasting alfalfa varieties. Plant J. 68 (5), 871–889. doi: 10.1111/j.1365-313X.2011.04738.x
Kim, D., Landmead, B., Salzberg, S. L. (2015). HISAT: a fast spliced aligner with low memory requirements. Nat. Methods 12 (4), 357–U121. doi: 10.1038/Nmeth.3317
Koh, J., Chen, G., Yoo, M. J., Zhu, N., Dufresne, D., Erickson, J. E., et al. (2015). Comparative proteomic analysis of Brassica napus in response to drought stress. J. Proteome Res. 14 (8), 3068–3081. doi: 10.1021/pr501323d
Langfelder, P., Horvath, S. (2008). WGCNA: an r package for weighted correlation network analysis. BMC Bioinf. 9 (1), 559. doi: 10.1186/1471-2105-9-559
Li, J., Guo, X., Zhang, M., Wang, X., Zhao, Y., Yin, Z., et al. (2018). OsERF71 confers drought tolerance via modulating ABA signaling and proline biosynthesis. Plant Sci. 270, 131–139. doi: 10.1016/j.plantsci.2018.01.017
Li, P., Lin, P., Zhao, Z., Li, Z., Liu, Y., Huang, C., et al. (2022). Gene co-expression analysis reveals transcriptome divergence between wild and cultivated sugarcane under drought stress. Int. J. Mol. Sci. 23 (1), 569–592. doi: 10.3390/ijms23010569
Livak, K. J., Schmittgen, T. (2001). Analysis of relative gene expression data using real-time quantitative PCR and the 2-△△Ct method. Methods 25 (4), 402–408. doi: 10.1006/meth.2001.1262
Love, M. I., Huber, W., Anders, S. (2014). Moderated estimation of fold change and dispersion for RNA-seq data with DESeq2. Genome Biol. 15 (12), 550. doi: 10.1186/s13059-014-0550-8
Luo, D., Zhou, Q., Wu, Y., Chai, X., Liu, W., Wang, Y., et al. (2019). Full-length transcript sequencing and comparative transcriptomic analysis to evaluate the contribution of osmotic and ionic stress components towards salinity tolerance in the roots of cultivated alfalfa (Medicago sativa L.). BMC Plant Biol. 19 (1), 32. doi: 10.1186/s12870-019-1630-4
Miao, Z., Xu, W., Li, D., Hu, X., Liu, J., Zhang, R., et al. (2015). Denovo transcriptome analysis of Medicago falcata reveals novel insights about the mechanisms underlying abiotic stress-responsive pathway. BMC Genomics 16, 818. doi: 10.1186/s12864-015-2019-x
Nahar, K., Hasanuzzaman, M., Alam, M. M., Rahman, A., Mahmud, J. A., Suzuki, T., et al. (2017). Insights into spermine-induced combined high temperature and drought tolerance in mung bean: osmoregulation and roles of antioxidant and glyoxalase system. Protoplasma 254 (1), 445–460. doi: 10.1007/s00709-016-0965-z
Okamuro, J. K., Caster, B., Villarroel, R., Van, M. M., Jofuku, K. D. (1997). The AP2 domain of APETALA2 defines a large new family of DNA binding proteins in arabidopsis. PNAS 94 (13), 7076–7081. doi: 10.1073/pnas.94.13.7076
Pan, I. C., Li, C. W., Su, R. C., Cheng, C. P., Lin, C. S., Chan, M. T. (2010). Ectopic expression of an EAR motif deletion mutant of SlERF3 enhances tolerance to salt stress and ralstonia solanacearum in tomato. Planta 232 (5), 1075–1086. doi: 10.1007/s00425-010-1235-5
Pinhero, R. G., Rao, M. V., Paliyath, G., Murr, D. P., Fletcher, R. A. (1997). Changes in activities of antioxidant enzymes and their relationship to genetic and paclobutrazol-induced chilling tolerance of maize seedlings. Plant Physiol. 114 (2), 695–704. doi: 10.1104/pp.114.2.695
Puyang, X., An, M., Han, L., Zhang, X. (2015). Protective effect of spermidine on salt stress induced oxidative damage in two Kentucky bluegrass (Poa pratensis L.) cultivars. Ecotoxicol. Environ. Saf. 117, 96–106. doi: 10.1016/j.ecoenv.2015.03.023
Qiang, Z., Zhang, J., Gao, X., Tong, J., Xiao, L., Li, W., et al. (2010). The arabidopsis AP2/ERF transcription factor RAP2.6 participates in ABA, salt and osmotic stress responses. Gene 457 (1-2), 1–12. doi: 10.1016/j.gene.2010.02.011
Qin, T. Y., Chao, S., Zhen-Zhen, B., Wen-Jun, L., Peng-Cheng, L., Jun-Lian, Z., et al. (2020). Identification of drought-related co-expression modules and hub genes in potato roots based on WGCNA. Acta Agron. Sin. 46 (7), 19. doi: 10.3724/SP.J.1006.2020.94130
Quan, R., Hu, S., Zhang, Z., Zhang, H., Zhang, Z., Huang, R. (2010). Overexpression of an ERF transcription factor TSRF1 improves rice drought tolerance. Plant Biotechnol. J. 8 (4), 476–488. doi: 10.1111/j.1467-7652.2009.00492.x
Quan, W., Liu, X., Wang, H., Chan, Z. (2015). Comparative physiological and transcriptional analyses of two contrasting drought tolerant alfalfa varieties. Front. Plant Sci. 6 1256. doi: 10.3389/fpls.2015.01256
Qu, Y., Duan, M., Zhang, Z., Dong, J., Wang, T. (2016). Overexpression of the Medicago falcata NAC transcription factor MfNAC3 enhances cold tolerance in Medicago truncatula. Environ. Exp. Bot. 129, 67–76. doi: 10.1016/j.envexpbot.2015.12.012
Rao, M. J., Xu, Y., Tang, X., Huang, Y., Liu, J., Deng, X., et al. (2020). CsCYT75B1, a citrus CYTOCHROME P450 gene, is involved in accumulation of antioxidant flavonoids and induces drought tolerance in transgenic arabidopsis. Antioxid. (Basel) 9 (2), 161–182. doi: 10.3390/antiox9020161
Ray, I. M., Han, Y. H., E, L., Meenach, C. D., Santantonio, N., Sledge, M. K., et al. (2015). Identification of quantitative trait loci for alfalfa forage biomass productivity during drought stress. Crop Sci. 55 (5), 2012–2033. doi: 10.2135/cropsci2014.12.0840
Smyth, G. K. (2010). edgeR: a bioconductor package for differential expression analysis of digital gene expression data. Bioinformatics 26 (1), 139. doi: 10.1093/bioinformatics/btp616
Thoenes, E., Dixit, S., Pereira, A., Aharoni, A., Rooy, G. V., Jetter, R. (2004). The SHINE clade of AP2 domain transcription factors activates wax biosynthesis, alters cuticle properties, and confers drought tolerance when overexpressed in Arabidopsis. Plant Cell 16 (9), 2463–2480. doi: 10.1105/TPC.104.022897
Waititu, J. K., Zhang, X., Chen, T., Zhang, C., Zhao, Y., Wang, H. (2021). Transcriptome analysis of tolerant and susceptible maize genotypes reveals novel insights about the molecular mechanisms underlying drought responses in leaves. Int. J. Mol. Sci. 22 (13), 6980–7011. doi: 10.3390/ijms22136980
Wang, W. B., Kim, Y. H., Lee, H. S., Kim, K. Y., Deng, X. P., Kwak, S. S. (2009). Analysis of antioxidant enzyme activity during germination of alfalfa under salt and drought stresses. Plant Physiol. Bioch. 47 (7), 570–577. doi: 10.1016/j.plaphy.2009.02.009
Wang, J. J., Yun, J. F., Shi-Jie, L. V. (2008). Characteristic and utilizing values on the germplasm of Medicago falcata. J. Inner Mongolia Agric. Univ. (Natural Sci. Edition) 29 (1), 216–220. doi: 10.3901/JME.2008.09.177
Wei, T., Wang, Y., Xie, Z., Guo, D., Chen, C., Fan, Q., et al. (2019). Enhanced ROS scavenging and sugar accumulation contribute to drought tolerance of naturally occurring autotetraploids in poncirus trifoliata. Plant Biotechnol. J. 17 (7), 1394–1407. doi: 10.1111/pbi.13064
Wen, X., Geng, F., Cheng, Y., Wang, J. (2021). Ectopic expression of CsMYB30 from citrus sinensis enhances salt and drought tolerance by regulating wax synthesis in Arabidopsis thaliana. Plant Physiol. Biochem. 166, 777–788. doi: 10.1016/j.plaphy.2021.06.045
Xiong, Y., Fan, X. H., Wang, Q., Yin, Z. G., Sheng, X. W., Chen, J., et al. (2022). Genomic analysis of soybean PP2A-b family and its effects on drought and salt tolerance. Front. Plant Sci. 2 (12), 784038. doi: 10.3389/fpls.2021.784038
Yang, J., Li, Q., Du, W., Yao, Y., Shen, G., Jiang, W., et al. (2021). Genome-wide analysis of glycoside hydrolase family 35 genes and their potential roles in cell wall development in Medicago truncatula. Plants (Basel) 10 (8), 1639–1655. doi: 10.3390/plants10081639
Yant, L., Mathieu, J., Dinh, T. T., Ott, F., Lanz, C., Wollmann, H., et al. (2010). Orchestration of the floral transition and floral development in arabidopsis by the bifunctional transcription factor APETALA2. Plant Cell 22 (7), 2156–2170. doi: 10.1105/tpc.110.075606
Yue, X. Q., Zhou, D. W. (2004). Good characteristics and its utilization of Medicago falcata. Jilin J. Anim. Husb. Vet. Med. 8, 26–28. doi: 10.3969/j.issn.1672-2078.2004.08.011
Yu, L. H., Wu, S. J., Peng, Y. S., Liu, R. N., Chen, X., Zhao, P., et al. (2016). Arabidopsis EDT1/HDG11 improves drought and salt tolerance in cotton and poplar and increases cotton yield in the field. Plant Biotechnol. J. 14 (1), 72–84. doi: 10.1111/pbi.12358
Zhai, Y., Li, J. W., Li, X. W., Lei, T. T., Wang, Q. Y. (2012). Isolation and characterization of a novel transcriptional repressor GmERF6 from soybean. Chem. Eng. J. 57 (1), 624–629. doi: 10.1007/s10535-012-0146-7
Zhang, B., Horvath, S. (2005). A general framework for weighted gene co-expression network analysis. Stat. Appl. Genet. Mol. 4 (1), 1–40. doi: 10.2202/1544-6115-1128
Zhang, Z., Huang, R. (2010). Enhanced tolerance to freezing in tobacco and tomato overexpressing transcription factor TERF2/LeERF2 is modulated by ethylene biosynthesis. Plant Mol. Biol. 73 (3), 241–249. doi: 10.1007/s11103-010-9609-4
Zhang, H., Huang, Z., Xie, B., Chen, Q., Tian, X., Zhang, X., et al. (2004). The ethylene, jasmonate, abscisic acid and NaCl-responsive tomato transcription factor JERF1 modulates expression of GCC box-containing genes and salt tolerance in tobacco. Planta 220 (2), 262–270. doi: 10.1007/s00425-004-1347-x
Zhao, Q., Hu, R. S., Liu, D., Liu, X., Wang, J., Xiang, X. H., et al. (2020). The AP2 transcription factor NtERF172 confers drought resistance by modifying NtCAT. Plant Biotechnol. J. 18 (12), 2444–2455. doi: 10.1111/pbi.13419
Zhao, M. J., Yin, L. J., Liu, Y., Ma, J., Zheng, J. C., Lan, J. H., et al. (2019). The ABA-induced soybean ERF transcription factor gene GmERF75 plays a role in enhancing osmotic stress tolerance in Arabidopsis and soybean. BMC Plant Biol. 19 (1), 506. doi: 10.1186/s12870-019-2066-6
Keywords: Medicago falcata, Drought, RNA-Seq, WGCNA, MfERF053
Citation: Li Q, Jiang W, Jiang Z, Du W, Song J, Qiang Z, Zhang B, Pang Y and Wang Y (2022) Transcriptome and functional analyses reveal ERF053 from Medicago falcata as key regulator in drought resistances. Front. Plant Sci. 13:995754. doi: 10.3389/fpls.2022.995754
Received: 16 July 2022; Accepted: 01 September 2022;
Published: 11 October 2022.
Edited by:
Linkai Huang, Sichuan Agricultural University, ChinaCopyright © 2022 Li, Jiang, Jiang, Du, Song, Qiang, Zhang, Pang and Wang. This is an open-access article distributed under the terms of the Creative Commons Attribution License (CC BY). The use, distribution or reproduction in other forums is permitted, provided the original author(s) and the copyright owner(s) are credited and that the original publication in this journal is cited, in accordance with accepted academic practice. No use, distribution or reproduction is permitted which does not comply with these terms.
*Correspondence: Yuxiang Wang, wyx9868@163.com; Yongzhen Pang, pangyongzhen@caas.cn
†These authors have contributed equally to this work