- 1College of Life Sciences, Linyi University, Linyi, China
- 2Department of Agronomy, Abdul Wali Khan University Mardan, Mardan, Pakistan
- 3College of Agriculture, Fujian Agriculture and Forestry University, Fuzhou, China
- 4Division of Biological and Environmental Sciences and Engineering, Smart-Health Initiative and Red Sea Research Center, King Abdullah University of Science and Technology, Thuwal, Saudi Arabia
- 5Agricultural Botany Department, Faculty of Agriculture (Saba Basha), Alexandria University, Alexandria, Egypt
- 6Plant Protection and Biomolecular Diagnosis Department, Arid Lands Cultivation Research Institute, The City of Scientific Research and Technological Applications, New Borg El Arab, Egypt
In recent years, heavy metals-induced soil pollution has increased due to the widespread usage of chromium (Cr) in chemical industries. The release of Cr into the environment has reached its peak causing hazardous environmental pollution. Heavy metal-induced soil pollution is one of the most important abiotic stress affecting the dynamic stages of plant growth and development. In severe cases, it can kill the plants and their derivatives and thereby pose a potential threat to human food safety. The chromium ion effect on plants varies and depends upon its severity range. It mainly impacts the numerous regular activities of the plant's life cycle, by hindering the germination of plant seeds, inhibiting the growth of hypocotyl and epicotyl parts of the plants, as well as damaging the chloroplast cell structures. In this review article, we tried to summarize the possible effects of chromium-induced stress on plant growth, developmental physiology, biochemistry, and molecular regulation and provided the important theoretical basis for selecting remedial plants in chromium-induced contaminated soils, breeding of low toxicity tolerant varieties, and analyzing the mechanism of plant resistance mechanisms in response to heavy metal stress.
Introduction
The accumulation of heavy metals in the environment due to modern industrial development is among the most serious pollutions in China and around the world. At present, heavy metal pollution mainly includes mercury (Hg), cadmium (Cd), lead (Pb), chromium (Cr), and arsenic (As). Numerous studies have reported that high concentrations of Cr6+ compounds contaminate the soil and hinder the germination and growth of many crops as well as economic plant seeds (Cervantes et al., 2001; Wang and Sheng, 2005; Hayat et al., 2012). Chromium (Cr) is the seventh element on earth that has various valence states of oxidation (Iyaka, 2009), including trivalent (iii) and hexavalent nature (iv). Trivalent chromium is present in its most stable and common valence level. Trivalent chromium is an essential trace element for the human body and plays an important role in the regulation of blood sugar (Zhang Y. et al., 2019), and excessive chromium has an adverse effect on plant photosynthesis and nutrient absorption (Cai et al., 2019). In addition, chromium accumulated in plants enters the body through the food chain, causing dermatitis, bronchitis, tuberculosis, and so on. It also increases the risk of cancer in humans. The toxicity of hexavalent chromium is 100 times more than that of trivalent chromium with serious toxicity (Qianqian et al., 2022), and its high chemical activity, which occurs with the rapid development and use of chemicals in a wide range of applications, is more easily absorbed by plants, resulting in its accelerated release into the environment.
Chromium compounds are heavily influenced by mining, paint manufacturing, petroleum refining, leather tanning, wood preservation, textile manufacturing, pulp processing, and biocide development, and large-scale industrial operations involving chromium lead to extreme environmental pollution (Haider et al., 2022). It is estimated that more than 2,000 tons of chromium enter natural water sources through liquid waste from leather factories every year. The chromium concentration in these waste liquids could be up to 2,000 ~ 5,000 mg L−1, which far exceeds the allowable maximum chromium threshold concentration of 2 mg L−1 (Adrees et al., 2015; Wei et al., 2022). In the United States, soil chromium concentrations in chromium alloy production areas have reached 25.9 g·kg−1 (Zayed and Terry, 2003; Ao et al., 2022). The situation of chromium pollution is not optimistic and ~15% of products in the national economy are related to chromium salts. Each ton of chrome salt products emits more than 2.5 tons of chrome slag, and Na2CrO4 and CaCrO4 in chrome slag are the main components that pollute the environment (Chapman, 2012; Liu et al., 2016; Zhang et al., 2017). Currently, more than 20 million hm2 of cultivated land in China is contaminated by heavy metals such as chromium (Batley, 2012; Zhucheng and Lin, 2020).
According to the National Survey Bulletin of the State of Soil Pollution, jointly released by the Department of Environmental Protection and the Department of Land and Resources in 2014, soil in the 14 provinces of the southern China region show higher levels of heavy metal pollution. Pollution from mining, heavy metal processing, and production is particularly severe (Cheng, 2003; Bautista et al., 2013), and it was found that Cd, As, Cr, and other harmful metals account for more than 50 % of the pollution of arable land in an area of 50 km2 in Chengdu district, China. The Cr concentration in Chengdu garbage is 35.39–179.63 mg·kg−1, which exceeds the soil environmental quality standard. It was found that the detection rate of chromium is 100 % and the excess rate of chromium content is 0.9% after analyzing the soil samples from the plains of Chengdu (Cheng, 2003; Ding et al., 2016; Seneviratne et al., 2017; Li et al., 2018). These data suggest that the state of chromium pollution in other provinces and cities should not be underestimated. Soil contaminated with heavy metals such as chromium further degrade the quality of agricultural crops growing on them and pose a potential threat to human food security. Previous research has suggested that excessive chromium levels severely affected crops of sweet potatoes (Ipomoeabatatas) (Gao et al., 2021) and wheat (Triticum aestivum) (Wang et al., 2017), and studies on heavy metals have revealed that the threat of chromium contaminated soil is imminent (Figure 1).
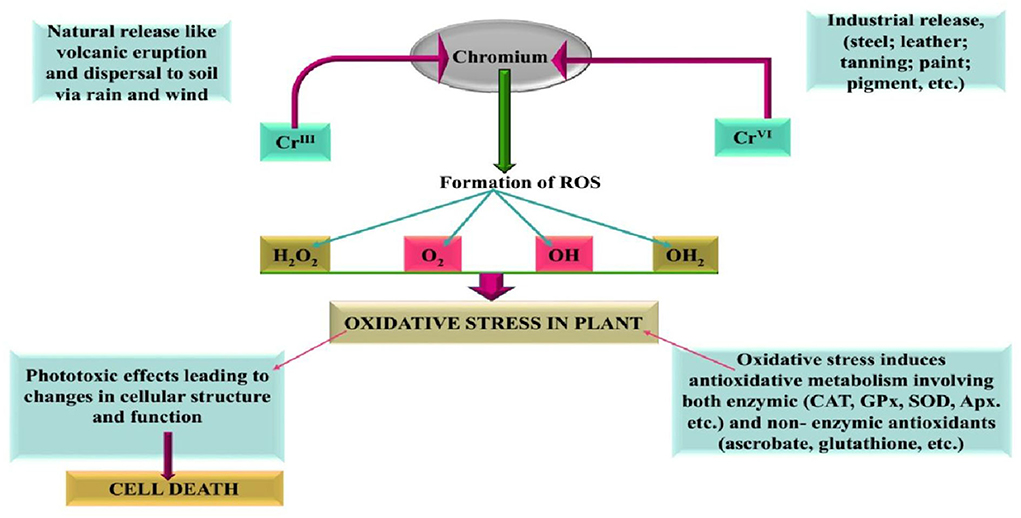
Figure 1. Different sources of chromium and their involvement in including oxidative stress in plants (Panda and Choudhury, 2005).
The remediation of soil contaminated with heavy metals involves two main aspects of consolidation and activation. The earlier remediation methods were mainly based on excavation and landfill, but their sustainability was not viable, especially with the advancement of science and technology. Further, the treatment of heavy metal soil pollution has now evolved into the use of chemical soil stabilization (Hamon et al., 2002), soil leaching (Huang and Keller, 2020), electrodynamic restoration (Zhang M. et al., 2019), and the use of plant ecological restoration (Lian et al., 2018). The earlier methods were relatively expensive and labor intensive; however, the chemical released can cause secondary pollution of the soil environment. Using plants for heavy metal remediation in the soil is relatively inexpensive, and also has good economic and environmental benefits, which is the key direction for future development and exploitation. To select the appropriate equipment for soil heavy metal remediation, we need to understand the effects of heavy metals on plants. The plant's response to external heavy metal stress is a complex process, involving at least heavy metal stress perception, signaling, and initiation of heavy metal stress resistance mechanisms. The generation of Cr heavy metal stress injuries (Hall and Williams, 2003) is more serious and severely affects plant growth and development, product yield, and quality (Arif et al., 2021).
Cr6+ is a typical heavy metal pollutant that can cause numerous diseases. The removal of Cr6+ from the environment or the reduction of the highly toxic Cr6+ to lesser toxic Cr3+ is of great importance in the treatment of chromium exposure (Buchanan-Wollaston and Ainsworth, 1997). Once agricultural surfaces are contaminated with Cr6+, then it becomes difficult to clean them and the cost of soil remediation using physical and chemical methods is extremely high. In addition, its toxic effects in plant cells can destroy the cell membranes and internal components of plants, alter the activity of related enzymes in the main body, and then alter the gene expression in plants as well as regulate the bio-synthesis of certain proteins.
In general, plants can prevent chromium from entering the cell through external rejection, such as organic acids, amino acids, and proteins that combine with heavy metals (Chapman, 2007; Osmolovskaya et al., 2018), effectively alleviating the effect of chromium before it enters the cells structures. At the same time, they can enhance the antioxidant defense in plant body defense mechanisms and reduce the damage to ROS caused by chromium stress (Pandey et al., 2005; Ali and Khan, 2018). Research data on the Web of Science and Scopus indicate that the presence of several common heavy metal elements has increased from 0.074% in 2000 to 0.163% in 2020 around the world (Figure 2). However, much of the research is still industry-focused such as materials science, engineering, and physical chemistry (Pourret, 2018; Pourret and Bollinger, 2018; Pourret and Hursthouse, 2019).
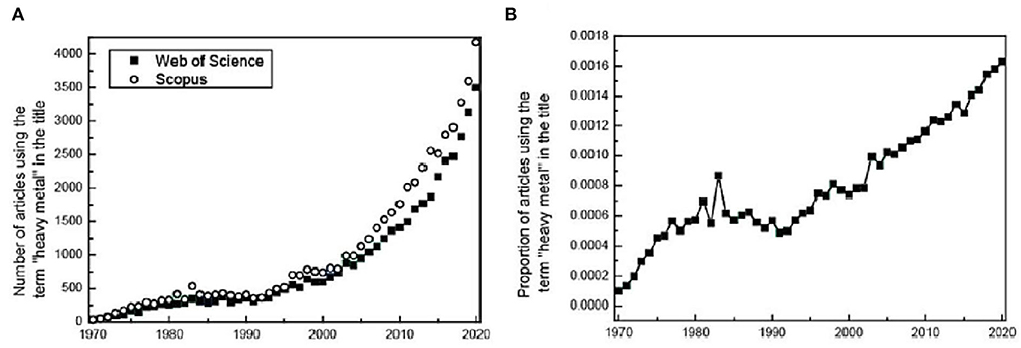
Figure 2. (A) Evolution of the number of publications using the term “heavy metal*” in their titles (Scopus and Web of Science, 24 February 2021). Modified and updated from Pourret and Bollinger (2018) and Pourret and Hursthouse (2019). (B) Evolution of publications using the term “heavy metals” in their titles (number of articles using the term divided by the total number of all articles published in the year) (Scopus data, searched using “heavy metal*”, accessed 24 February 2021).
The present article effectively summarizes the response mechanisms of plants toward chromium ion stress in multiple aspects, by reviewing the relevant domestic and foreign studies on the effects of chromium stress on plant growth and development, physiology and biochemistry, and molecular regulation. It aims to provide the scientific references and theoretical basis for the development of soil remediation to address heavy metal chromium stress on plants, breeding of low-toxicity plants resistant to heavy metal chromium stress, and discussing the general mechanism of plant heavy metal detoxification. Though the response of plant growth and reaction to chromium stress has been comprehensively understood, there are still some problems to be solved, such as whether there is a difference in chromium tolerance between physiology and biochemistry, and molecular regulation, or the reasons for differences in seed tolerance of different plant varieties. The solution to these problems provides an important theoretical basis for cultivating and screening plant varieties with high resistance to chromium stress.
Effects of chromium-induced stress on plant growth and development
Plant growth and development are the most important life processes and are influenced by various environmental factors. Chromium is a non-essential element for plants, and its high toxicity affects the germination of plant seeds and the growth and development of roots and shoots.
The effect of chromium stress on seed germination
Seed germination is the initial stage which is influenced by the heavy metal chromium as plants rely on seeds for reproduction (Chen et al., 2017), and it is also the primary manifestation reflecting the plant's tolerance level to chromium. Induction of stress through hexavalent chromium in the nutrient solution reduced seed germination. At 300 μM, the germination rate decreased significantly from 95.23% of the control to 75.45 %, which may be due to the decrease in enzyme activity triggered by the accumulation of Cr at the stage of seed germination (Duffus, 2002; Ganesh et al., 2006). The higher ROS generated by the Cr treatment may promote the breakdown of retained nutrients in seeds, leading to changes in cell membrane properties (Shafiq et al., 2008; Appenroth, 2010).
It is widely believed that chromium has an inhibitory effect on seed germination. The germination rate was affected by Cr6+ concentration in plant species as shown in Table 1. For instance, 100 mg·L−1 chromium treated in wheat reduced the germination rate by almost 10%. The same inhibitory effect was observed in Cucumis melo (Akinci and Akinci, 2010). Some studies have shown that low levels of chromium promoted seed germination of green vegetables such as Brassica chinensis, Corchorus spp, and Hibiscus sp (Nikinmaa and Schlenk, 2010; Islam et al., 2014), and then lead to significant inhibition of main root and seed growth with increasing concentrations of chromium in the treatment (Li et al., 2018). In addition, different plant seeds have different tolerance levels to chromium stress concentrations, and different chromium ion concentrations have different effects on plant seed germination. A concentration of 100 M Cr6+ and below on rice seed (Oryza sativa) has no apparent effect on germination potential, and when the chromium treatment concentration is >25 mg L−1, the seeds only germinated without root (Nath et al., 2009). The reason is that chromium sometimes triggers the protease activity resulting in the inhibition of seed germination because the hypocotyl transport is suppressed by the activity of amylase and sugar (Zeid, 2001; Dotaniya et al., 2014b), and in low concentrations, it might promote the α-amylase activity, favoring germination by sugars and thus promoting seed germination (Nayari et al., 1997; Basit et al., 2022a). The low concentration range is mostly between 0 and 10 mg/L.
Different plant varieties have different maximum thresholds of chromium tolerance (Hayat et al., 2012; Haider et al., 2022). Individual plants have a high tolerance to chromium stress and are chromium tolerant plants, such as B. 20 mg/L. The germination of water bamboo leafy greens seeds (Pandey et al., 2005) was promoted under 100 mg/L chromium stress, and the germination rate under 100 mg/L chromium stress was only 2.20% lower than that of the control. A 25 mg/L chromium stress had a promoting effect on the germination of wheat varieties. The germination rate under 100 mg/L chromium stress was only 8.00 and 0.66% lower than the control (Singh et al., 2021) and Casuarina (Lou et al., 2017; Zulfiqar et al., 2020) which may be related to the difference in plant species and the stress level of Cr6+ in the test.
Effect of chromium stress on underground parts of plants
The root system is the site where plants first encounter the soil's heavy metal ions and it is also the key site to absorb heavy metals which suggest that research into plant root growth is extremely important. In some previous studies, chromium stress had a low-promoting and high-inhibitory effect on plant root growth (Table 2). Cr6+-mediated inhibition of root growth may be due to the inhibition of cell division and reduction of cell size in the elongated region (Peralta et al., 2001). It was found that 5 mg·L−1 chromium treatment significantly promoted root growth of Medicago sativa seedlings. However, when the dose was increased to 20 mg·L−1, the promoting effect reversed to inhibit the length of Convolvulus arvensis, which decreased by 77% at the same chromium concentration of 20 mg·L−1. On the other hand, there was no significant difference in Convolvulus arvensis root length after increasing the chromium concentration. Panda and Patra (2000) and Gardea-Torresdey et al. (2004), found that 1 μmol·L−1 chromium with simultaneous nitrogen addition also increases the root length of seedlings. Del Bubba et al. (2013) found that treatment with 50 mg/kg−1 chromium significantly reduced root biomass of wild-type tobacco (Nicotiana langsdorffii), and root accumulation of chromium reached 2,600 μg·g−1 in radish (Brassica campestris) treated with 1 mg L−1 chromium for 21 days and root length was reduced by 80% (Nath et al., 2005; Dotaniya et al., 2014a). Chromium stress also led to a reduction in the number of lateral roots of alfalfa, tall fescue (Festuca arundinacea), and white clover (Trifolium repens), as well as resulted in yellow and necrotic root tips and root cuttings (Qing et al., 2015; Wakeel et al., 2018). For the same treatment concentration, the inhibitory effect of hexavalent chromium on the root length of onions (Allium cepa) was significantly higher than that of trivalent chromium (Liu et al., 1992).
In addition, different plants show different degrees of inhibition at the same treatment concentration. For instance, the root length of Brassica oleracea was reduced by 31% after 7 days of 100 mg. L−1 chromium treatment, while the root length of Sesamum indicum was reduced by more than 86% (Peralta et al., 2001). Wakeel et al. (2018) found that the inhibitory effect of hexavalent chromium on the growth of Arabidopsis thaliana was enhanced by the stimulation auxin resistance caused by AUX1 gene expression increasing auxin accumulation in the root tip and affecting its polar transport. The plant root cell wall is the first barrier for plants, preventing heavy metals from entering the body. It consists of three parts: the intercellular layer, the primary wall, and the secondary wall. It is rich in cellulose, pectin, and lignin, and these substances are also rich in hydroxyl and carboxyl groups. When metal ions enter the cell, they combine with these groups and precipitate, reducing the amount of metal ions entering the protoplasmic layer (Eva et al., 2014), in the root cells of white clover, alfalfa, tall fescue, and rice (Zeng et al., 2011). The main storage locations of chromium are cell walls and cytoplasm where the content of plastids such as mitochondria and chloroplasts are low. Wakeel et al. (2020) also found that the chromium content in the vacuoles of aerial plant parts and root cells of chromium-treated vegetables reached 20 ~ 40%, indicating that plants can also transport it in vacuoles to remove it from active ingredient isolate metabolic sites and avoid toxicity to other organelles.
Effect of chromium stress on above-ground parts of plants
Chromium enters the plants from the root system and is partly transported along with nutrients to the above-ground parts of the plants, which influences the growth of organs such as stems and leaves. The reduction in above-ground biomass after stress is the most intuitive manifestation. Turner and Rust (1971b) and Basit et al. (2022b) found that in hydroponics treated with 0.5 mg L−1· chromium and soil cultures treated with 10 mg·L−1 chromium could significantly reduce the dry matter content in the aerial parts of soybeans (Glycine max). Turner and Rust (1971b) found similar results in subsequent studies with rice (Salvinia minima) and other plants (Prado et al., 2010). Plant height is also significantly affected due to chromium stress. Nath et al. (2005) found that 5 mg L−1 of hexavalent chromium can reduce radish seedlings by almost 50%, and stem elongation of Convolvulus ternata was also greatly inhibited after hexavalent chromium treatment (Gardea-Torresdey et al., 2004; Rhaman et al., 2020); a similar effect was also observed in alfalfa treated with trivalent chromium (Barton et al., 2000). However, no positive correlation was found between plant dry matter content and plant height. Although 80 mg L−1 chromium treatments can greatly inhibit convolvulus stem, elongation did not affect leaf biomass accumulation (Gardea-Torresdey et al., 2004).
It is generally believed that the presence of chromium affects plant photosynthesis and disrupts plant growth. In duckweed (Lemna minor), with increasing treatment concentrations, the color of duckweed leaves gradually turned yellow, and the content of chlorophyll and carotenoids decreased significantly (Sallah-Ud-Din et al., 2017). Studies on Arabidopsis thaliana showed that chloroplast ribosomal recycling factors played an important role in chloroplast biosynthesis, and changes in maize protein under chromium stress suggested that chloroplast ribosomal recycling factors may be involved in the structure of chloroplast under chromium stress through post-translational modifications (Wang et al., 2013; Grabsztunowicz et al., 2019). Most of the identified chromium stress proteins (72%, 42/58) were predicted to be localized in the chloroplast, and these chloroplast-localized chromium stress proteins were associated with chloroplast structure and function, including the participation in photosynthesis electron transport, chloroplast organization, chlorophyll biosynthesis, and chloroplast redox balance. Although the dry matter content of Sophora japonicus continued to decrease with increasing concentration, chromium-treated chlorophyll and carotenoid levels were not affected by chromium. It has been speculated that chromium may also hinder plant growth by interfering with cellular processes other than photosynthesis (Prado et al., 2010). After being treated with 100, 200, and 400 mg/kg−1 chromium, the root dry weight of rice was reduced in tillering, booting, and filling phases but the root-to-shoot ratio increased, indicating that the consumed more heavy metal chromium had an obvious inhibition on plant shoots (Xie et al., 2018).
Toxic effects of chromium on plant roots
Different parts of the plant have different sensitivities to chromium, and the root is the most susceptible part of the plant to chromium poisoning. The changes in root length and root count are important indexes to measure the effect of chromium on the plant (Saxena et al., 2021; Monga et al., 2022). High concentrations of chromium lead to the withering of root cells and separation of the plasma wall, which induces root apex cells to produce a higher frequency of chromosome distortion, resulting in the inhibition of root cell division and differentiation, and reducing the volume and number of root cells. As the root length shortens, the primary root thickens, does not form lateral roots and root hairs, and even does not form a root. Cr6+ was found to inhibit the growth of young plant roots more than the growth of sprouts and stems, mainly for the following two reasons: First, chromium's first contact is with the plant's roots, and once plant roots are combined with Cr6+ through physical adsorption and chemisorption, chromium could induce roots to produce ethylene stress and transport it to the shoot (Shinwari et al., 2015; Shahid et al., 2017). Stress ethylene causes strong cellular damage, and this damage occurs first in the roots, causing the plant roots to come under chromium stress earlier than the stem and shoot. Secondly, due to the influence of chromium, the structure of the root cell wall of the plant changes along with the content of cellulose. Consequently, the one-OH group and the free carboxyl COO group in the polysaccharide composition increase and bind more Cr6+ to confine it at the site, reducing the amount of translocation to the morphological top. As a result, the root system accumulates more chromium than the stem and the shoot (Ao et al., 2022).
Overall effect of chromium on plant seedling growth
Chromium stress has a tingling effect at low concentrations and inhibits the growth of most plant seedlings at high concentrations. Low chromium concentrations can increase the net rate of photosynthesis and promote plant growth by promoting the electron transport activity of PSII, which can increase the proportion of medulla and outer skin tissue in the root and promote root and root hair growth. A high concentration of chromium hinders water transport, reduces transpiration, affects the uptake of minerals by the root system, and disrupts enzymatic reactions in the plant body, resulting in short stature of the plant, yellowing, and dropping of the leaves, as well as a significant reduction in leaf area and biomass. It was reported that high chromium concentrations could cause permanent plasma wall detachment and water loss in plant tissues (Madrid, 2010; Singh et al., 2021; Srivastava et al., 2021), and irreversible damage to mitochondria, resulting in decreased respiration and even plant cell death. At the same time, it was found that high chromium concentrations caused abnormal stomatal conductivity, reduced intercellular space, and reduced growth and yield of plants (Rath and Das, 2021).
Impacts of chromium stress on plant physiology and biochemistry
The accumulation of chromium in plants inevitably disrupts their homeostasis, damages cell structure, and affects the dynamic balance of the antioxidant enzyme system.
Effects of chromium stress on cell membrane permeability in plants
The selective permeability of the cell membrane can prevent the inflow and outflow of extracellular substances, make the intracellular environment relatively stable, and ensure that the biochemical reactions required for life can proceed in an orderly manner. When plants are poisoned by heavy metals, these harmful metal ions damage cell membranes and their selective permeability, thereby greatly increasing permeability, causing membrane lipid peroxidation, and affecting the normal growth and development of plants. Relative conductivity and malondialdehyde are the two most commonly used indicators to measure cell membrane damage among which, malondialdehyde, as the final product of membrane lipid peroxidation, can indirectly assess the damage of plant cell membranes. Draper and Hadley (1990) found that the relative conductivity of Cyperus malaccensis leaves increased with increasing convulsive concentration, and the relative conductance of malondialdehyde increased from 500 mg L−1 by 255.66 and 185.74% with increasing chromium concentration. The cell membrane structure was significantly destroyed. Pan and Yu (2011) found that the combination of Cr, As, Pb, and Cd could increase the relative conductivity of tea tree leaves (Camellia sinensis) and found that combinations of heavy metals treated differently in chromium concentrations changed relative conductivity. According to the area analysis of the orthogonal experiment, chromium has the strongest effect on membrane permeability.
Damage of plant subcellular structure under chromium stress
When chromium crosses membranes in cells, it inevitably damages the cell's internal structure. Gill et al. (2017) found that rapeseed (Brassica napus) produced large black plastoglobules (PG) and increased the volume and number of starch granules (SG) under chromium stress. The chloroplast ruptured and the thylakoid membrane was also damaged after the dwarf bean (Phaseolus vulgaris) was treated with chromium; the chloroplasts in the first leaf cells showed more basal granules and the number of thylakoids decreased compared to the control. A large central nucleus appeared in the third leaf cells, which were smaller vacuoles (Eleftheriou et al., 2015).
Effects of chromium on the enzymatic anti-oxidant system of plants
Under normal circumstances, the synthesis and decomposition of reactive oxygen species (ROS) in plants would be in dynamic balance; but when exposed to chromium stress, the cellular redox balance is disrupted, and the ROS level in plants increases significantly, which induces hyperoxia affects the production of antioxidant enzymes such as superoxide dismutase (SOD), catalase (CAT), peroxidase (POD), and ascorbate peroxidase (APX), which are used to resist the toxic effects of heavy metals, which in turn affect plant growth. The antioxidant enzyme systems of different plants react differently to chromium stress (Monga et al., 2022). When mustard (Brassica juncea) was treated with different concentrations of chromium, it was found that the activities of SOD, CAT, and APX in roots and leaves first increased and later decreased (Pandey et al., 2005; Hübner et al., 2010), while glutathione transferase (GST) and glutathione reductase (GR) showed an increasing trend. The POD, CAT, and SOD that were treated with hexavalent chromium in duckweed (Lemna minor) (Lopez-Luna et al., 2009; Chen et al., 2015), Arabidopsis plants and Vallisneria spiralis (Xu and Deng, 2012; Elisa and Bartoli, 2013) showed similar expression trends. This is based on the fact that under chromium stress, chromium ions act as a signaling molecule to initiate the plant's antioxidant defense mechanism, and as stress damage increases, antioxidant enzyme activity continues to decrease (Mallick et al., 2010). However, the CAT content in maize (Zea mays) first showed a trend of decreasing (Table 3), then increasing and then decreasing with chromium treatment concentration which may be due to the iron porphyrin contained in the CAT structure, and the interaction between chromium and iron in the metabolic pool resulting a reduction in its activity. It is also possible that the presence of chromium affects the availability of active iron (Sharma et al., 2003).
Impacts of chromium-induced stress on molecular regulation of plant mechanism
With the rapid development of natural sciences, people began to discover that the mechanism of plant resistance is closely related to their own gene expression. The study of the physiological direction cannot solve all scientific problems. Currently, scientists have explored the effects of heavy metal chromium on plants from a molecular biology perspective and found that gene expression, regulation, and protein synthesis in the body change accordingly when plants respond to chromium stress (Table 4). With the development of molecular biology, some genes related to chromium resistance have been reported. In sugarcane (Saccharum spp. hybrid), Jain et al. (2016) found that the expression of the i-gene of the metallothionein (MT) gene was found to increase significantly in stem and leaf under chromium stress. It is consistent with previous reports that MT protein can bind metals and detoxify excess metal ions (Gepstein et al., 2003; Kim et al., 2007). Gill et al. (2017) found that the genes BnaA08g16610D, BnaCnng19320D, and BnaA08g00390D were overexpressed in rapeseed after chromium treatment.
These genes encode nucleic acids, transition metal ion binding proteins kinase activity, phosphotransferase activity, and molecular transporter genes BnaA04g26560D, BnaA02g28130D, and BnaA02g01980D were identified as responsible for transmembrane transport of water molecules in the presence of chromium. The use of transgenic technology to introduce non-plant resistance genes could decrease chromium uptake by plants. Jin et al. (2001) transferred the reductase gene of a heavy metal-reducing bacterium- Pseudomonas aeruginosa into tobacco (Nicotiana tabacum), which could effectively reduce the content of hexavalent chromium in tobacco plants. In addition, when the rat glucocorticoid receptor gene (GR receptor) was transferred to tobacco, the amount of chromium absorbed by the plants was significantly reduced (Fuoco et al., 2013; Lopez-Luna et al., 2016). With the development of high-throughput sequencing technology, it is still necessary to further discuss the mining of important chromium stress response genes and the construction of expression networks among a large number of sequencing results.
The miRNA is a class of exogenous non-coding small RNA that regulates gene expression by inducing cleavage of target mRNA or inhibition of translational at the transcriptional and post-transcriptional levels (Turner et al., 2012). Such small RNA also plays an important role in plant response to heavy metal stress (Zhou et al., 2012). Forty-one conserved miRNA families were identified in chromium-treated tobacco (Bukhari et al., 2015), of which 57 miRNAs from 26 families were up-regulated, 8 miRNAs from the miR166 family were downregulated, and 29 new miRNA families were identified where 14 were differentially expressed under chromium stress. The COG functional class analysis of these miRNAs showed that some predicted miRNA target transcripts were responsive to biological and abiotic stresses, including miR166 target genes derived from the III HD-ZIP family which regulates lateral rooting in Arabidopsis (Bowman, 2004). These results suggest that it may play a similar role in tobacco chromium tolerance.
In addition, chromium stress can also alter the expression of certain proteins in plants. In recent proteomics studies (Sharmin et al., 2012), 36 proteins were found differentially expressed in the roots of Miscanthus sinensis under chromium stress, of which 13 were up-regulated, 21 were downregulated, and 2 new proteins were induced. These proteins turned out to be well-known carbon and nitrogen metabolism-related proteins, molecular chaperone-like heavy metal-inducing proteins, and some enzymes such as inositol mono-phosphatase, nitrate-reducing enzymes (reductase), adenine phosphoribosyl transferase, and other enzyme proteins. In barley (Hordeum vulgare) and grapes (Brassica napus), hexavalent chromium increased chitinase activity (Hewitt, 1953; Jacobsen and Hauschild, 1992), which may be related to the cross-tolerance of plants. In transgenic tobacco overexpressing fungal chitinase, the plant not only increased its resistance to fungal infections but also to salinity and metal ion stress (Hodson, 2004; De Las Mercedes Dana et al., 2006), although the relationship between chitinase and plant metal tolerance is still unclear.
However, the role of metal-specific chitinase may help explain the mechanism of chromium detoxification in plants. Bukhari et al. (2015) found that under chromium stress, dehydrin (DHN), mitochondrial processing peptidase-like (MPP), adenine phosphoribosyl transferase, superoxide dismutase, and mitochondrial malate dehydrogenase (mitochondrial malate dehydrogenase (MDH) in plants were expressed differently. These proteins were further classifications of into four categories based on their function such as related to stress response, energy metabolism, RNA binding, and metabolism. In rice, NADP isocitrate dehydrogenase, heat shock protein 90 (Hsp90), glyoxalase I (glyoxalase I), protein-glycosylated peptides (reversibly the expression of the glycosylated polypeptide (RGP), S-adenosyl-methionine synthetase (SAMS), glutamine synthetase (glutamine synthetase), and other proteins were up-regulated (Zeng et al., 2014). Kaszycki et al. (2018) found that the submerged plant Callitriche cophocarpa expressed a novel NAD(P)H-dependent dehydrogenase FQR1, thought to be a detoxifying protein, after treatment with hexavalent chromium to protect cells from oxidative damage in response to oxidative stress. It exhibited quinone reductase activity in vitro and catalyzed the transfer of two electrons from NAD(P)H to a variety of substrates, including hexavalent chromium. This enzyme is specifically induced by chromate and cannot be produced under salinity and oxidative stress.
Plant-chelating peptides (phytochelatin, PCs) are a type of heavy metal chelating proteins that are widely distributed in plants. Catalyzing heavy metal-induced glutathione synthesis (Zenk, 1996; Handa et al., 2018), after chelating with heavy metals, they can be further transported to vacuoles to reduce the intracellular concentration of free heavy metal ions (Brunetti et al., 2015) and they play an important role in heavy metal detoxification. It was found that hexavalent chromium could significantly increase the PC content in the aerial parts of green vegetables, and varieties high in PC content can accumulate more chromium in roots and cell walls, and significantly improve their tolerance (Pan et al., 2011). Glutathione (GSH), which is widely distributed in organelles such as cytoplasm, chloroplasts, and mitochondria, is a direct substrate for the synthesis of PCs. The sulfhydryl group in its chemical structure has the unique property of stabilizing mercapto-metal bonds. This stable binding form combined with higher water solubility allows GSH to better eliminate the toxicity of a variety of heavy metals (Zlobin et al., 2017). After heavy metal stress, the content of GSH in plants decreased due to the increased amount of PC synthesis. Pan et al. (2011) showed that increased PC content is much higher than decreased GSH content, indicating that hexavalent chromium can also stimulate plants to synthesize new GSH. Furthermore, exogenous application of GSH reduced the concentration of free chromium ions and aerial chromium ions in rice roots, indicating that GSH can inhibit chromium ion transport (Zeng et al., 2012), alleviate the damage to plants caused by chromium, and reverse the damage to physiological functions and cellular structure (Hou et al., 2014; Gill et al., 2017).
The uptake of heavy metals in plants affects the normal metabolism of plants. To reduce its toxic effects, plants also excrete organic acids with a high affinity for heavy metals to combine with heavy metal ions to form chelates, which can be divided into external and internal chelation (Oliveira, 2012; Liu et al., 2018). External chelation refers to plants that secrete organic acids through their roots into the rhizosphere that combine with metal ions to alter their mobility and solubility in the medium (Zeng et al., 2008). Increasing and prolonging chromium content in the culture medium treatment time increased the secretion of organic acids such as oxalic acid, citric acid, and malic acid, which promoted the absorption of chromium ions by rice. The exogenous application of citric acid to duckweed (Lemna minor) also promoted the absorption of chromium ions by rice (Sallah-Ud-Din et al., 2017).
The concentrations of citric, formic, lactic, malic, oxalic, and succinic acid in the rhizosphere of Silene vulgaris were significantly increased at 100 mg·kg−1 chromium treatment, but the concentrations varied with different genotypes (García-Gonzalo et al., 2017). Internal chelation mainly refers to the combination of organic acids produced by the plant's own metabolism with heavy metal ions absorbed by the plant, transforming them into non-toxic or low-toxic forms and alleviating their toxic effects on plants (Hall, 2002). Citric acid, malic acid, and oxalic acid are the main organic acids used to chelate metals in plants; however, it is still controversial whether organic acids can detoxify heavy metals in plants. Regardless, in the study by Juneja and Prakash (2005), it was found that citric acid and malic acid were the main complexing agents of trivalent chromium in corn xylem sap, and the complication of trivalent chromium was beneficial to the dissolution and migration of chromium and it was considered that these organic acids may indeed have a detoxification effect on plants.
Summary and future perspectives
In summary, while research on the effects of chromium on plants compared to other heavy metal ions started earlier, it has not been deep enough to study the plants under heavy metal chromium stress response mechanisms and degradation-related genes and proteins or to investigate which is clearly related to the signal transduction network. Further work is required for screening appropriate plants to cultivate on the soil contaminated by heavy metals. There are two approaches to restoring vegetation in heavy metal-contaminated land by using plants for ecological restoration. This involves finding plants with strong qualities of repulsing heavy metal ions or accumulating them underground, thereby effectively utilizing the abandoned and polluted land, but also avoiding the potential risks of using the above-ground part. The use of hyperaccumulators in soil treatments contaminated with heavy metals to restore ecological function is quite common. Hyperaccumulator plants refer to plants whose absorption of heavy metal ions is more than 100 times that of ordinary plants, and whose leaf-to-root ratio of heavy metal content is >1 (Baker et al., 2000). For chromium, the plant absorption threshold should be 300 μg·g−1 (Garcíahernández et al., 1998; Van Der Ent et al., 2013). Current studies have identified that plants such as Leersiahexandra (Zhang et al., 2007), Prosopis laevigata (Buendía-González et al., 2010; Gill et al., 2016), and Spartina argentinensis (Redondo-Gómez et al., 2011) can be hyperaccumulators of chromium. However, existing hyperaccumulator plants have low biomass and economic benefits, so they cannot be used in a variety of practical remediation projects.
Therefore, the researchers believe that further selection and breeding of plants can not only fulfill the ecological restoration function but can also have a higher biomass yield and heavy metal ions can accumulate in the above-ground part, which can effectively and economically control the heavy metal contamination of land. Previous reports of Miscanthus, which are widely distributed in different countries, have rich wild resources, developed root systems, and large biomass, and can absorb organic pollutants, heavy metals, and other solid matter to prevent soil erosion, and promote carbon deposition. They can be used for ecological upgrading of heavy metals as an energy plant, and they also have a very high economic value.
As an industrially widespread heavy metal, chromium is one of the main sources of environmental pollution. Its high toxicity is closely related to its rapid permeability through biofilm and subsequent interaction with proteins and nucleic acids in plants. For some plants, the effects of chromium listed in Tables 3, 4 [from the point of view of independent seed germination, external performance, aboveground and root growth, internal regulatory genes, and the change in the protein (Figure 3)], show that the plant's defense against heavy metal chromium, absorption, resistance, and detoxification mechanism are very complicated and that there are major differences between different plants in their response to chromium stress. In addition, previous studies have shown that chromium also has some effect on the absorption of other heavy metals (Turner and Rust, 1971b; Moral et al., 1996). In general, the existing research has gradually deepened from physiology to molecular direction. Genomics, proteomics, and metabolome research have become an important direction in the study of the mechanism of heavy metal chromium tolerance in plants, and many issues urgently need further research.
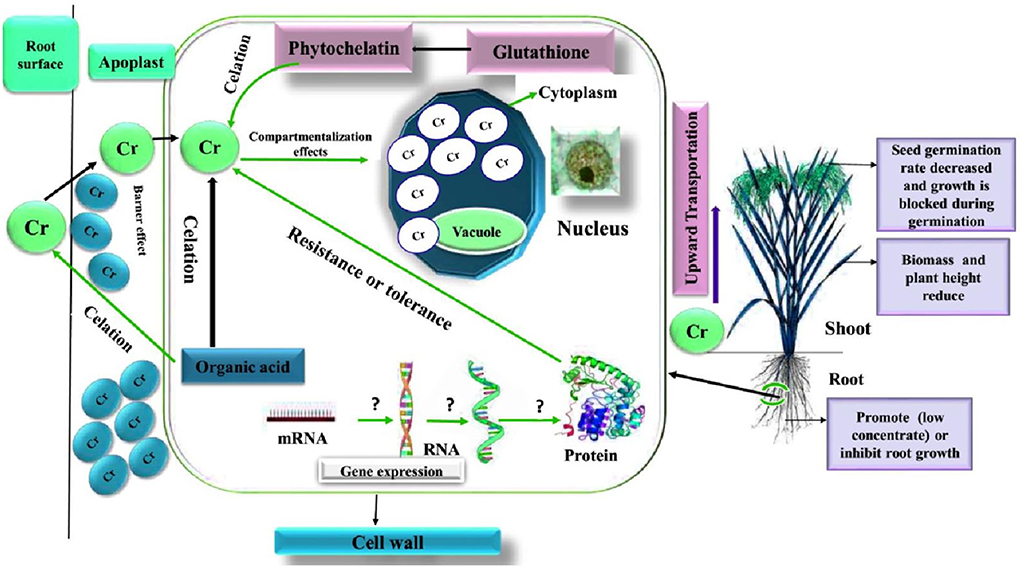
Figure 3. Schematic diagram of the influence of heavy metal chromium on plant growth, physiology, and molecular regulation (Shanker et al., 2005).
Author contributions
SS and DW wrote the initial draft of the manuscript. TJ designed the whole idea of the review article. TJ, MJ, NA, and RG improved the initial draft of the manuscript and also gathered the data. SF undertook the graphical visualization and proofreading of the final version of the manuscript. All authors have read and agreed to the published version of the manuscript.
Funding
This research was supported by the National Natural Science Foundation of China (Projects No. 32001468).
Acknowledgments
The authors would like to acknowledge Alexandria University, Alexandria, Egypt and King Abdullah University of Science and Technology, Saudi Arabia for their financial support.
Conflict of interest
The authors declare that the research was conducted in the absence of any commercial or financial relationships that could be construed as a potential conflict of interest.
Publisher's note
All claims expressed in this article are solely those of the authors and do not necessarily represent those of their affiliated organizations, or those of the publisher, the editors and the reviewers. Any product that may be evaluated in this article, or claim that may be made by its manufacturer, is not guaranteed or endorsed by the publisher.
References
Adams, H. D., Zeppel, M. J. B., Anderegg, W. R. L., Hartmann, H., Landhäusser, S. M., Tissue, D. T., et al. (2017). A multi-species synthesis of physiological mechanisms in drought-induced tree mortality. Nat. Ecol. Evolut. 1, 1285–1291. doi: 10.1038/s41559-017-0248-x
Adrees, M., Ali, S., Iqbal, M., Bharwana, S. A., Siddiqi, Z., Farid, M., et al. (2015). Mannitol alleviates chromium toxicity in wheat plants in relation to growth, yield, stimulation of anti-oxidative enzymes, oxidative stress and Cr uptake in sand and soil media. Ecotoxicol. Environ. Saf. 122, 1–8. doi: 10.1016/j.ecoenv.2015.07.003
Akinci, I. E., and Akinci, S. (2010). Effect of chromium toxicity on germination and early seedling growth in melon (Cucumis melo L.). Afr. J. Biotechnol. 929, 4589–4594. Available online at: http://www.academicjournals.org/AJB
Aldoobie, N., and Beltagi, M. (2013). Physiological, biochemical and molecular responses of common bean (Phaseolus vulgaris L.) plants to heavy metals stress. Afr. J. Biotechnol. 12, 4614–4622. doi: 10.5897/AJB2013.12387
Ali, H., and Khan, E. (2018). What are heavy metals? Long-standing controversy over the scientific use of the term ‘heavy metals'—proposal of a comprehensive definition. Toxicol. Environ. Chem. 100, 6–19. doi: 10.1080/02772248.2017.1413652
Ali, S., Bharwana, S. A., Rizwan, M., Farid, M., Kanwal, S., Ali, Q., et al. (2015). Fulvic acid mediates chromium (Cr) tolerance in wheat (Triticum aestivum L.) through lowering of Cr uptake and improved antioxidant defense system. Environ. Sci. Pollut. Res. 22, 10601–10609. doi: 10.1007/s11356-015-4271-7
Ao, M., Chen, X., Deng, T., Sun, S., Tang, Y., Morel, J. L., et al. (2022). Chromium biogeochemical behaviour in soil-plant systems and remediation strategies: a critical review. J. Hazard. Mater. 424, 127233. doi: 10.1016/j.jhazmat.2021.127233
Appenroth, K.-J. (2010). What are “heavy metals” in plant sciences? Acta Physiol. Plant. 32, 615–619. doi: 10.1007/s11738-009-0455-4
Arif, M. S., Yasmeen, T., Abbas, Z., Ali, S., Rizwan, M., Aljarba, N. H., et al. (2021). Role of exogenous and endogenous hydrogen sulfide (H2S) on functional traits of plants under heavy metal stresses: a recent perspective. Front. Plant Sci. 11, 545453. doi: 10.3389/fpls.2020.545453
Baker, A. J. M., Mcgrath, S. P., Reeves, R. D., and Smith, J. A. C. (2000). “Metal hyperaccumulator plants: a review of the ecology and physiology of a biological resource for phytoremediation of metal-polluted soils,” in Phytoremediation of Contaminated Soil and Water (CRC Press), 85–107. doi: 10.1201/9780367803148-5
Barton, L. L., Johnson, G. V., O'nan, A. G., and Wagener, B. M. (2000). Inhibition of ferric chelate reductase in alfalfa roots by cobalt, nickel, chromium, and copper. J. Plant Nutr. 23, 1833–1845. doi: 10.1080/01904160009382146
Baruah, N., Mondal, S. C., Farooq, M., and Gogoi, N. (2019). Influence of heavy metals on seed germination and seedling growth of wheat, pea, and tomato. Water Air Soil Pollut. 230, 273. doi: 10.1007/s11270-019-4329-0
Basit, F., Bhat, J. A., Dong, Z., Mou, Q., Zhu, X., Wang, Y., et al. (2022a). Chromium toxicity induced oxidative damage in two rice cultivars and its mitigation through external supplementation of brassinosteroids and spermine. Chemosphere 302, 134423. doi: 10.1016/j.chemosphere.2022.134423
Basit, F., Bhat, J. A., Han, J., Guan, Y., Jan, B. L., Shakoor, A., et al. (2022b). Screening of rice cultivars for Cr-stress response by using the parameters of seed germination, morpho-physiological and antioxidant analysis. Saudi J. Biol. Sci. 29, 3918–3928. doi: 10.1016/j.sjbs.2022.02.038
Batley, G. E. (2012). “Heavy metal”—a useful term. Integr. Environ. Assess. Manag. 8, 215–215. doi: 10.1002/ieam.1290
Bautista, O. V., Fischer, G., and Cárdenas, J. F. (2013). Cadmium and chromium effects on seed germination and root elongation in lettuce, spinach and Swiss chard. Agron. Colomb. 31, 48–57. Available online at: http://www.scielo.org.co/scielo.php?script=sci_arttext&pid=S0120-99652013000100006&lng=en&tlng=en
Bowman, J. L. (2004). Roles for class III HD-Zip and KANADI genes in Arabidopsis root development. Plant Physiol. 135, 2261–2270. doi: 10.1104/pp.104.040196
Brunetti, P., Zanella, L., De, P. A., Di Litta, D., Cecchetti, V., Falasca, G., et al. (2015). Cadmium-inducible expression of the ABC-type transporter AtABCC3 increases phytochelatin-mediated cadmium tolerance in Arabidopsis. J. Exp. Bot. 66, 3815. doi: 10.1093/jxb/erv185
Buchanan-Wollaston, V., and Ainsworth, C. (1997). Leaf senescence in Brassica napus: Cloning of senescence-related genes by subtractive hybridization. Plant Mol. Biol. 33, 821–834. doi: 10.1023/A:1005774212410
Buendía-González, L., Orozco-Villafuerte, J., Cruz-Sosa, F., Barrera-Díaz, C. E., and Vernon-Carter, E. J. (2010). Prosopis laevigata a potential chromium (VI) and cadmium (II) hyperaccumulator desert plant. Bioresour. Technol. 101, 5862. doi: 10.1016/j.biortech.2010.03.027
Bukhari, S. A., Shang, S., Zhang, M., Zheng, W. T., Zhang, G. P., Wang, T. Z., et al. (2015). Genome-wide identification of chromium stress-responsive micro RNAs and their target genes in tobacco (Nicotiana tabacum) roots. Environ. Toxicol. Chem. 34, 2573–2582. doi: 10.1002/etc.3097
Cai, M., Hu, C., Wang, X., Zhao, Y., Jia, W., Sun, X., et al. (2019). Selenium induces changes of rhizosphere bacterial characteristics and enzyme activities affecting cr/selenium uptake by pak choi (Brassica campestrisl. ssp. Chinensis makino) in cr contaminated soil. Environ. Pollut. 249, 716–727. doi: 10.1016/j.envpol.2019.03.079
Cervantes, C., Camposgarcía, J., Devars, S., Gutiérrez-Corona, F., Loza-Tavera, H., Torres-Guzmán, J. C., et al. (2001). Interactions of chromium with microorganisms and plants. FEMS Microbiol. Rev. 25, 335–347. doi: 10.1111/j.1574-6976.2001.tb00581.x
Chapman, P. M. (2007). Heavy metal—music, not science. Environ. Sci. Technol. 41, 6C. doi: 10.1021/es072552o
Chapman, P. M. (2012). “Heavy metal”—cacophony, not symphony. Integr. Environ. Assess. Manag. 8, 216. doi: 10.1002/ieam.1289
Chen, G., Ren, L., Zhang, J., Reed, B. M., Zhang, D., and Shen, X. (2015). Cryopreservation affects ROS-induced oxidative stress and antioxidant response in Arabidopsis seedlings. Cryobiology 70, 38–47. doi: 10.1016/j.cryobiol.2014.11.004
Chen, Q., Zhang, X., Liu, Y., Wei, J., Shen, W., Shen, Z., et al. (2017). Hemin-mediated alleviation of zinc, lead and chromium toxicity is associated with elevated photosynthesis, antioxidative capacity; suppressed metal uptake and oxidative stress in rice seedlings. Plant Growth Regul. 81, 253–264. doi: 10.1007/s10725-016-0202-y
Cheng, S. (2003). Heavy metal pollution in China: origin, pattern and control. Environ. Sci. Pollut. Res. Int. 10, 192–198. doi: 10.1065/espr2002.11.141.1
Choudhary, S. P., Kanwar, M., Bhardwaj, R., Yu, J. Q., and Tran, L. S. P. (2012). Chromium stress mitigation by polyamine-brassinosteroid application involves phytohormonal and physiological strategies in Raphanus sativus L. PLoS ONE 7, e33210. doi: 10.1371/journal.pone.0033210
Daud, M. K., Mei, L., Variath, M. T., Ali, S., Li, C., Rafiq, M. T., et al. (2014). Chromium (VI) uptake and tolerance potential in cotton cultivars: effect on their root physiology, ultramorphology, and oxidative metabolism. BioMed Res. Int. 2014, 975946. doi: 10.1155/2014/975946
De Las Mercedes Dana, M., Pintor-Toro, J. A., and Cubero, B. (2006). Transgenic tobacco plants overexpressing chitinases of fungal origin show enhanced resistance to biotic and abiotic stress agents. Plant Physiol. 142, 722–730. doi: 10.1104/pp.106.086140
Del Bubba, M., Ancillotti, C., Checchini, L., Ciofi, L., Fibbi, D., Gonnelli, C., et al. (2013). Chromium accumulation and changes in plant growth, selected phenolics and sugars of wild type and genetically modified Nicotiana langsdorffii. J. Hazard. Mater. 262, 394–403. doi: 10.1016/j.jhazmat.2013.08.073
Ding, Q., Cheng, G., Wang, Y., and Zhuang, D. (2016). Effects of natural factors on the spatial distribution of heavy metals in soils surrounding mining regions. Sci. Total Environ. 578, 577–585. doi: 10.1016/j.scitotenv.2016.11.001
Dotaniya, M. L., Das, H., and Meena, V. D. (2014a). Assessment of chromium efficacy on germination, root elongation, and coleoptile growth of wheat (Triticum aestivum L.) at different growth periods. Environ. Monit. Assess. 186, 2957–2963. doi: 10.1007/s10661-013-3593-5
Dotaniya, M. L., Meena, V. D., and Das, H. (2014b). Chromium toxicity on seed germination, root elongation and coleoptile growth of pigeon pea (Cajanus cajan). Legume Res. 37, 227–229. doi: 10.5958/j.0976-0571.37.2.034
Draper, H. H., and Hadley, M. (1990). Malondialdehyde determination as index of lipid peroxidation. Meth. Enzymol. 186, 421–431. doi: 10.1016/0076-6879(90)86135-I
Duffus, J. H. (2002). “Heavy metals” a meaningless term? (IUPAC Technical Report). Pure Appl. Chem. 74, 793–807. doi: 10.1351/pac200274050793
Duhan, J. S. (2012). Chromium stress on peroxidase, ascorbate peroxidase and acid invertase in pea (Pisum sativum L.) seedling. Int. J. Biotechnol. Mol. Biol. Res. 3, 15–21. doi: 10.5897/IJBMBR11.052
Eleftheriou, E. P., Adamakis, I. D., Panteris, E., and Fatsiou, M. (2015). Chromium-Induced ultrastructural changes and oxidative stress in roots of Arabidopsis thaliana. Int. J. Mol. Sci. 16, 15852–15871. doi: 10.3390/ijms160715852
Elisa, S., and Bartoli, M. (2013). Seasonal variation of radial oxygen loss in Vallisneria spiralis L. An adaptive response to sediment redox. Aquat. Bot. 104, 228–232. doi: 10.1016/j.aquabot.2012.07.007
Eva, M., Ruben, V., Wout, B., and Antoni, M. (2014). The role of the secondary cell wall in plant resistance to pathogens. Front. Plant Sci. 5, 358. doi: 10.3389/fpls.2014.00358
Fuoco, R., Bogani, P., Capodaglio, G., Del Bubba, M., Abollino, O., Giannarelli, S., et al. (2013). Response to metal stress of Nicotiana langsdorffii plants wild-type and transgenic for the rat glucocorticoid receptor gene. J. Plant Physiol. 170, 668–675. doi: 10.1016/j.jplph.2012.12.009
Ganesh, K. S., Sundaramoorthy, P., and Chidambaram, A. L. A. (2006). Chromium toxicity effect on black gram, soybean and paddy. Poll Res. 25, 257–261.
Gao, J., Zhang, D., Proshad, R., Uwiringiyimana, E., and Wang, Z. (2021). Assessment of the pollution levels of potential toxic elements in urban vegetable gardens in southwest China. Sci. Rep. 11, 22824. doi: 10.1038/s41598-021-02069-6
García-Gonzalo, P., Del Real, A. E. P., Lobo, M. C., and Pérez-Sanz, A. (2017). Different genotypes of Silene vulgaris (Moench) Garcke grown on chromium-contaminated soils influence root organic acid composition and rhizosphere bacterial communities. Environ. Sci. Pollut. Res. 24, 1–12. doi: 10.1007/s11356-016-6667-4
Garcíahernández, M., Murphy, A., and Taiz, L. (1998). Metallothioneins 1 and 2 have distinct but overlapping expression patterns in Arabidopsis. Plant Physiol. 118, 387–397. doi: 10.1104/pp.118.2.387
Gardea-Torresdey, J. L., Peralta-Videa, J. R., Montes, M., De La Rosa, G., and Corral-Diaz, B. (2004). Bioaccumulation of cadmium, chromium and copper by Convolvulus arvensis L.: impact on plant growth and uptake of nutritional elements. Bioresour. Technol. 92, 229–235. doi: 10.1016/j.biortech.2003.10.002
Gepstein, S., Sabehi, G., Carp, M. J., Hajouj, T., Nesher, M. F., Yariv, I., et al. (2003). Large-scale identification of leaf senescence-associated genes. Plant J. 36, 629–642. doi: 10.1046/j.1365-313X.2003.01908.x
Gill, R. A., Ali, B., Yang, S., Tong, C., Islam, F., Gill, M. B., et al. (2017). Reduced glutathione mediates pheno-ultrastructure, kinome and transportome in chromium-induced Brassica napus L. Front. Plant Sci. 8, 2037. doi: 10.3389/fpls.2017.02037
Gill, R. A., Zhang, N., Ali, B., Farooq, M. A., Xu, J., Gill, M. B., et al. (2016). Role of exogenous salicylic acid in regulating physio-morphic and molecular changes under chromium toxicity in black- and yellowseeded Brassica napus L. Environ. Sci. Pollut. Res. 23, 20483–20496. doi: 10.1007/s11356-016-7167-2
Grabsztunowicz, M., Mulo, P., Baymann, F., Mutoh, R., Kurisu, G., Sétif, P., et al. (2019). Electron transport pathways in isolated chromoplasts from Narcissus pseudonarcissus L. Plant J. 99, 245–256. doi: 10.1111/tpj.14319
Haider, F. U., Wang, X., Farooq, M., Hussain, S., Cheema, S. A., Ain, N. U., et al. (2022). Biochar application for the remediation of trace metals in contaminated soils: Implications for stress tolerance and crop production. Ecotoxicol. Environ. Saf. 230, 113165. doi: 10.1016/j.ecoenv.2022.113165
Hall, J. L. (2002). Cellular mechanisms for heavy metal detoxification and tolerance. J. Exp. Bot. 53, 1–11. doi: 10.1093/jexbot/53.366.1
Hall, L., and Williams, L. E. (2003). Transition metal transporters in plants. J. Exp. Bot. 54, 2601–2613. doi: 10.1093/jxb/erg303
Hamon, R. E., Mclaughlin, M. L., and Cozens, G. (2002). Mechanisms of attenuation of metal availability in in situ remediation treatments. Environ. Sci. Technol. 36, 3991–3996. doi: 10.1021/es025558g
Handa, N., Kohli, S. K., Thukral, A. K., Bhardwaj, R., Alyemeni, M. N., Wijaya, L., et al. (2018). Protective role of selenium against chromium stress involving metabolites and essential elements in Brassica juncea L. seedlings. 3 Biotech 8, 66. doi: 10.1007/s13205-018-1087-4
Hayat, S., Khalique, G., Irfan, M., Wani, A. S., and Tripathi, B. N. (2012). Physiological changes induced by chromium stress in plants: an overview. Protoplasma 249, 599–611. doi: 10.1007/s00709-011-0331-0
Hewitt, E. J. (1953). Effects of some metal toxicities on sugar beet, tomato, oat, potato and marrow stem kale grown in sand culture. J. Exp. Bot. 4, 59–64. doi: 10.1093/jxb/4.1.59
Hodson, M. E. (2004). Heavy metals - Geochemical bogey men? Environ. Pollut. 129, 341–343. doi: 10.1016/j.envpol.2003.11.003
Hou, J., Liu, G. N., Xue, W., Fu, W. J., Liang, B. C., and Liu, X. H. (2014). Seed germination, root elongation, root-tip mitosis, and micronucleus induction of five crop plants exposed to chromium in fluvo-aquic soil. Environ. Toxicol. Chem. 33, 671–676. doi: 10.1002/etc.2489
Huang, Y., and Keller, A. A. (2020). Remediation of heavy metal contamination of sediments and soils using ligand-coated dense nanoparticles. PLoS ONE 15, e0239137. doi: 10.1371/journal.pone.0239137
Hübner, R., Astin, K. B., and Herbert, R. J. H. (2010). 'Heavy metal'-time to move on from semantics to pragmatics? J. Environ. Monit. 12, 1511–1514. doi: 10.1039/c0em00056f
Islam, M. K., Alam, I., Khanam, M. S., Lee, S. Y., Waghmode, T. R., and Huh, M. R. (2014). Accumulation and tolerance characteristics of chromium in nine jute varieties (Corchorus spp. and Hibiscus spp). Plant Omics 7, 392–402.
Iyaka, Y. A. (2009). Chromium in soils: a review of its distribution and impacts. Cont. J. Environ. Sci. 3, 13–18. doi: 10.4314/afrrev.v3i3.47511
Jacobsen, S., and Hauschild, M. Z. (1992). Rasmussen U. Induction by chromium ions of chitinases and polyamines in barley (Hordeum vulgare L.) and rape (Brassica napus L. ssp. oleifera). Plant Sci. 84, 119–128. doi: 10.1016/0168-9452(92)90125-6
Jain, R., Singh, S. P., Singh, A., Singh, S., Tripathi, P., Chandra, A., et al (2016). Study on physio-biochemical attributes and metallothionein gene expression affected by chromium (VI) in sugarcane (Saccharum spp. hybrid). J. Environ. Biol. 37, 375–382. Available online at: http://www.jeb.co.in/.../paper_08
Jin, T. E., Kim, I. G., and Kim, W. S. (2001). Expression of chromium (VI) reductase gene of heavy metal reducing bacteria in tobacco plants. J. Plant Biotechnol. 3, 13–17. Available online at: https://koreascience.kr/article/JAKO200111921597038.pdf
Joutey, N. T., Bahafid, W., Sayel, H., and El Ghachtouli, N. (2013). Phytotoxic effect of hexavalent chromium on germination and seedling growth of seeds of different plant species. Int. J. Agric. Technol. 9, 293–304. Available online at: http://www.ijat-aatsea.com/pdf/v9_n2
Juneja, S., and Prakash, S. (2005). The chemical form of trivalent chromium in xylem sap of maize (Zea mays L.). Chem. Spec. Bioavail. 17, 161–169. doi: 10.3184/095422906783438820
Kaszycki, P., Dubicka-Lisowska, A., Augustynowicz, J., Piwowarczyk, B., and Wesołowski, B. (2018). Callitriche cophocarpa (water starwort) proteome under chromate stress: evidence for induction of a quinone reductase. Environ. Sci. Pollut. Res. 25, 8928–8942. doi: 10.1007/s11356-017-1067-y
Kim, S. H., Lee, H. S., Song, W. Y., Choi, K. S., and Hur, Y. (2007). Chloroplast-targeted BrMT1 (Brassica rapa type-1 metallothionein) enhances resistance to cadmium and ros in transgenicatabidopsis plants. J. Plant Biol. 50, 1–7. doi: 10.1007/BF03030592
Kováčik, J., Babula, P., Klejdus, B. I., and Hedbavny, J. (2013). Chromium uptake and consequences for metabolism and oxidative stress in chamomile plants. J. Agric. Food Chem. 61, 7864–7873. doi: 10.1021/jf401575a
Li, X., Zhang, X., Wu, Y., Li, B., and Yang, Y. (2018). Physiological and biochemical analysis of mechanisms underlying cadmium tolerance and accumulation in turnip. Plant Diver. 40, 19–27. doi: 10.1016/j.pld.2017.12.005
Lian, X., Piao, S., Huntingford, C., Li, Y., Zeng, Z., Wang, X., et al. (2018). Partitioning global land evapotranspiration using CMIP5 models constrained by observations. Nat. Clim. Chang. 8, 640–646. doi: 10.1038/s41558-018-0207-9
Liu, D., Jiang, W., and Li, M. (1992). Effects of trivalent and hexavalent chromium on root growth and cell division of Allium cepa. Hereditas 117, 23–29. doi: 10.1111/j.1601-5223.1992.tb00003.x
Liu, H., Yang, H., and Yi, F. (2016). Experimental study of the complex resistivity and dielectric constant of chrome-contaminated soil. J. Appl. Geophys. 131,109–116. doi: 10.1016/j.jappgeo.2016.05.001
Liu, L., Li, W., Song, W., and Guo, M. (2018). Remediation techniques for heavy metal-contaminated soils: principles and applicability. Sci. Total Environ. 633, 206–219. doi: 10.1016/j.scitotenv.2018.03.161
Lopez-Luna, J., Gonzalez-Chavez, M. C., Esparza-Garcia, F. J., and Rodriguez-Vazquez, R. (2009). Toxicity assessment of soil amended with tannery sludge, trivalent chromium and hexavalent chromium, using wheat, oat and sorghum plants. J. Hazard. Mater. 163, 829–834. doi: 10.1016/j.jhazmat.2008.07.034
Lopez-Luna, J., Silva-Silva, M. J., Martinez-Vargas, S., Mijangos-Ricardez of Gonzalez-Chavez M. C., Solis-Dominguez, F. A., and Cuevas-Diaz, M. C. (2016). Magnetite nanoparticle (NP) uptake by wheat plants and its effect on cadmium and chromium toxicological behavior. Sci. Total Environ. 565, 941–950. doi: 10.1016/j.scitotenv.2016.01.029
Lou, Y., Zhao, P., Wang, D., Amombo, E., Sun, X., Wang, H., et al. (2017). Germination, physiological responses and gene expression of tall fescue (Festuca arundinacea Schreb.) growing under Pb and Cd. PLoS ONE 12, e0169495. doi: 10.1371/journal.pone.0169495
Ma, J., Lv, C., Xu, M., Chen, G., Lv, C., and Gao, Z. (2016). Photosynthesis performance, antioxidant enzymes, and ultrastructural analyses of rice seedlings under chromium stress. Environ. Sci. Pollut. Res. 23, 1768–1778. doi: 10.1007/s11356-015-5439-x
Madrid, L. (2010). “Heavy metals”: Reminding a long-standing and sometimes forgotten controversy. Geoderma 155, 128–129. doi: 10.1016/j.geoderma.2009.11.031
Maiti, S., Ghosh, N., Mandal, C., Das, K., Dey, N., and Adak, M. K. (2012). Responses of the maize plant to chromium stress with reference to antioxidation activity. Braz. J. Plant. Physiol. 24, 203–212. doi: 10.1590/S1677-04202012000300007
Mallick, S., Sinam, G., Mishra, R. K., and Sinha, S. (2010). Interactive effects of Cr and Fe treatments on plants growth, nutrition and oxidative status in Zea mays L. Ecotoxicol. Environ. Saf. 73, 987–995. doi: 10.1016/j.ecoenv.2010.03.004
Monga, A., Fulke, A. B., and Dasgupta, D. (2022). Recent developments in essentiality of trivalent chromium and toxicity of hexavalent chromium: implications on human health and remediation strategies. J. Hazard. Mater. Adv. 7, 100113. doi: 10.1016/j.hazadv.2022.100113
Moral, R., Gómez, I., Pedreno, J. N., and Mataix, J. (1996). Absorption of Cr and effects on micronutrient content in tomato plant (Lycopersicum esculentum M.). Agrochimica 40, 132–138.
Nath, K., Saini, S., and Sharma, Y. K. (2005). Chromium in tannery industry effluent and its effect on plant metabolism and growth. J. Environ. Biol. 26, 197–204.
Nath, K., Singh, D., Shyam, S., and Sharma, Y. K. (2009). Phytotoxic effects of chromium and tannery effluent on growth and metabolism of Phaseolus mungo Roxb. J. Environ. Biol. 30, 227–234.
Nayari, H. F., Szalai, T., Kadar, I., and Csatho, P. (1997). Germination characteristics of pea seeds originating from a field trial treated with different level of harmful elements. Acta Agronom. Hungar. 45, 147–154.
Nikinmaa, M., and Schlenk, D. (2010). Uses of phrases. Aquat. Toxicol. 97, 1–2. doi: 10.1016/j.aquatox.2010.02.015
Oliveira, H. (2012). Chromium as an environmental pollutant: Insights on induced plant toxicity. J. Bot. 2012, 1–8. doi: 10.1155/2012/375843
Osmolovskaya, N., Dung, V. V., and Kuchaeva, L. (2018). The role of organic acids in heavy metal tolerance in plants. Bio. Comm. 63, 9–16. doi: 10.21638/spbu03.2018.103
Paiva, L. B., Correa, S. F., Santa Catarina, C., Floh, E. I. S., Silva, M. G. D., and Vitória, A. P. (2014). Ecophysiological and biochemical parameters for assessing Cr+6 stress conditions in Pterogyne nitens Tul.: new and usual methods for the management and restoration of degraded areas. Environ. Eng. Manag. J. 13, 3073–3081. doi: 10.30638/eemj.2014.346
Pan, J., and Yu, L. (2011). Effects of Cd or/and Pb on soil enzyme activities and microbial community structure. Ecol. Eng. 37, 1889–1894. doi: 10.1016/j.ecoleng.2011.07.002
Pan, Y., Birdsey, R. A., Fang, J., Houghton, R., Kauppi, P. E., Kurz, W. A., et al. (2011). A large and persistent carbon sink in the World's forests. Science 333, 988–993. doi: 10.1126/science.1201609
Panda, S. K., and Choudhury, S. (2005). Chromium stress in plants. Braz. J. Plant Physiol. 17, 95–102. doi: 10.1590/S1677-04202005000100008
Panda, S. K., and Patra, H. K. (2000). Nitrate and ammonium ions effect on the chromium toxicity in developing wheat seedlings. Proc. Natl. Acad. Sci. India 70, 75–80.
Pandey, V., Dixit, V., and Shyam, R. (2005). Antioxidative responses in relation to growth of mustard (Brassica juncea cv. Pusa Jaikisan) plants exposed to hexavalent chromium. Chemosphere 61, 40–47. doi: 10.1016/j.chemosphere.2005.03.026
Peralta, J. R., Gardea-Torresdey, J. L., Tiemann, K. J., Gomez, E., Arteaga, S., Rascon, E., et al. (2001). Uptake and effects of five heavy metals on seed germination and plant growth in alfalfa (Medicago sativa L.). Bull. Environ. Contam. Toxicol. 66, 727–734. doi: 10.1007/s001280069
Pourret, O. (2018). On the necessity of banning the term “heavy metal” from the scientific literature. Sustainability 10, 2879. doi: 10.3390/su10082879
Pourret, O., and Bollinger, J. C. (2018). “Heavy metal” - what to do now: to use or not to use? Sci. Total Environ. 610–611, 419–420. doi: 10.1016/j.scitotenv.2017.08.043
Pourret, O., and Hursthouse, A. (2019). It's time to replace the term “heavy metals” with “potentially toxic elements” when reporting environmental research. Int. J. Environ. Res. Public Health 16, 4446. doi: 10.3390/ijerph16224446
Prado, C., Rodríguez-Montelongo, L., González, J. A., Pagano, E. A., Hilal, M., and Prado, F. E. (2010). Uptake of chromium by Salvinia minima: effect on plant growth, leaf respiration and carbohydrate metabolism. J. Hazard. Mater. 177, 546–553. doi: 10.1016/j.jhazmat.2009.12.067
Qianqian, M., Haider, F. U., Farooq, M., Adeel, M., Shakoor, N., Jun, W., et al. (2022). Selenium treated foliage and biochar treated soil for improved lettuce (Lactuca Sativa L.) growth in Cd-polluted soil. J. Clean. Prod. 335, 130267. doi: 10.1016/j.jclepro.2021.130267
Qing, X., Zhao, X., Hu, C., Wang, P., Zhang, Y., Zhang, X., et al. (2015). Selenium alleviates chromium toxicity by preventing oxidative stress in cabbage (Brassica campestris L. ssp Pekinensis) leaves. Ecotoxicol. Environ. Saf. 114, 179–189. doi: 10.1016/j.ecoenv.2015.01.026
Rahman, M. M., Rahman, M. M., Islam, K. S., and Chongling, Y. (2010). Effect of chromium stress on antioxidative enzymes and malondialdehyde content activities in leaves and roots of mangrove seedlings Kandelia candel (L.) druce. J. Environ. Sci. 26, 171–179.
Rai, V., Vajpayee, P., Singh, S. N., and Mehrotra, S. (2004). Effect of chromium accumulation on photosynthetic pigments, oxidative stress defense system, nitrate reduction, proline level and eugenol content of Ocimum tenuiflorum L. Plant Sci. 167, 1159–1169. doi: 10.1016/j.plantsci.2004.06.016
Rath, A., and Das, A. B. (2021). Chromium stress induced oxidative burst in Vigna mungo (L.) hepper: physio-molecular and antioxidative enzymes regulation in cellular homeostasis. Physiol. Mol. Biol. Plants 27, 265–279. doi: 10.1007/s12298-021-00941-3
Redondo-Gómez, S., Mateos-Naranjo, E., Vecino-Bueno, I., and Feldman, S. R. (2011). Accumulation and tolerance characteristics of chromium in a cordgrass Cr-hyperaccumulator, Spartina argentinensis. J. Hazard. Mater. 185, 962–869. doi: 10.1016/j.jhazmat.2010.09.101
Rhaman, M. S., Imran, S., Rauf, F., Khatun, M., Baskin, C. C., Murata, Y., et al. (2020). Seed Priming with phytohormones: an effective approach for the mitigation of abiotic stress. Plants 10, 37. doi: 10.3390/plants10010037
Sallah-Ud-Din, R., Farid, M., Saeed, R., Ali, S., Rizwan, M., Tauqeer, H. M., et al. (2017). Citric acid enhanced the antioxidant defense system and chromium uptake by Lemna minor L. grown in hydroponics under Cr stress. Environ. Sci. Pollut. Res. 24, 1–10. doi: 10.1007/s11356-017-9290-0
Samantaray, S., Rout, G. R., and Das, P. (2001). Induction, selection and characterization of Cr and Ni-tolerant cell lines of Echinochloa colona (L.) Link in vitro. J. Plant Physiol. 158, 1281–1290. doi: 10.1078/0176-1617-00533
Saxena, G., Kumar, V., and Shah, M. P. (2021). Advances in bioremediation of hexavalent chromium: cytotoxicity, genotoxicity, and microbial alleviation strategies for environmental safety. Bioremed. Environ. Sustain. 2021, 55–72. doi: 10.1016/B978-0-12-820524-2.00003-1
Seneviratne, M., Rajakaruna, N., Rizwan, M., Madawala, H. M. S. P., Ok, Y. S., and Vithanage, M. (2017). Heavy metal-induced oxidative stress on seed germination and seedling development: a critical review. Environ. Geochem. Health 41, 1813–1831. doi: 10.1007/s10653-017-0005-8
Shafiq, M., Iqbal, M. Z., and Mohammad, A. (2008). Effect of lead and cadmium on germination and seedling growth of Leucaena leucocephala. J. Appl. Sci. Environ. Manag. 12, 61–66. doi: 10.4314/jasem.v12i3.55497
Shahid, M., Shamshad, S., Rafiq, M., Khalid, S., Bibi, I., Niazi, N. K., et al. (2017). Chromium speciation, bioavailability, uptake, toxicity and detoxification in soil-plant system: a review. Chemosphere 178, 513–533. doi: 10.1016/j.chemosphere.2017.03.074
Shanker, A., Cervantes, C., Lozatavera, H., and Avudainayagam, S. (2005). Chromium toxicity in plants. Environ. Int. 31, 739–753.doi: 10.1016/j.envint.2005.02.003
Shanker, A. K., Djanaguiraman, M., Sudhagar, R., Chandrashekar, C., and Pathmanabhan, G. (2004). Differential antioxidative response of ascorbate glutathione pathway enzymes and metabolites to chromium speciation stress in green gram [Vigna radiata (L.) R. Wilczek. cv CO4] roots. Plant Sci. 166, 1035–1043. doi: 10.1016/j.plantsci.2003.12.015
Sharma, D. C., Sharma, C. P., and Tripathi, R. D. (2003). Phytotoxic lesions of chromium in maize. Chemosphere 51, 63–68. doi: 10.1016/S0045-6535(01)00325-3
Sharmin, S. A., Alam, I., Kim, K. H., Kin, Y. G., Kim, P. J., Bahk, J. D., et al. (2012). Chromium-induced physiological and proteomic alterations in roots of Miscanthus sinensis. Plant Sci. 187, 113–126. doi: 10.1016/j.plantsci.2012.02.002
Shinwari, K. I., Jan, M., Shah, G., Khattak, S. R., Urehman, S., Daud, M. K., et al. (2015). Seed priming with salicylic acid induces tolerance against chromium (VI) toxicity in rice (Oryza sativa L.). Pak. J. Bot. 47, 161–170.
Singh, D., Sharma, N. L., Singh, C. K., Yerramilli, V., Narayan, R., Sarkar, S. K., et al. (2021). Chromium (VI)-induced alterations in physio-chemical parameters, yield, and yield characteristics in two cultivars of mungbean (Vigna radiata L.). Front. Plant Sci. 12, 735129. doi: 10.3389/fpls.2021.735129
Srivastava, D., Tiwari, M., Dutta, P., Singh, P., Chawda, K., Kumari, M., et al. (2021). Chromium stress in plants: toxicity, tolerance and phytoremediation. Sustainability 13, 4629. doi: 10.3390/su13094629
Tang, M., Mao, D., Xu, L., Li, D., Song, S., and Chen, C. (2014). Integrated analysis of miRNA and mRNA expression profiles in response to Cd exposure in rice seedlings. BMC Genom. 15, 835. doi: 10.1186/1471-2164-15-835
Trinh, N. N., Huang, T. L., Chi, W. C., Fu, S. F., Chen, C. C., and Huang, H. J. (2014). Chromium stress response effect on signal transduction and expression of signaling genes in rice. Physiol. Plant 150, 205–224. doi: 10.1111/ppl.12088
Turner, M., Yu, O., and Subramanian, S. (2012). Genome organization and characteristics of soybean microRNAs. BMC Genomics 13, 169. doi: 10.1186/1471-2164-13-169
Turner, M. A., and Rust, R. H. (1971a). Effects of chromium on growth and mineral nutrition of soybeans1. Soc. Sci. Am. J. 35, 755. doi: 10.2136/sssaj1971.0361599500
Turner, M. A., and Rust, R. H. (1971b). Effects of chromium on growth and mineral nutrition of soybeans. Soil Sci. Soc. Am. J. 35, 755–758. doi: 10.2136/sssaj1971.03615995003500050035x
Van Der Ent, A., Baker, A. J. M., Reeves, R. D., Pollard, A. J., and Schat, H. (2013). Hyperaccumulators of metal and metalloid trace elements: facts and fiction. Plant Soil 362, 319–334. doi: 10.1007/s11104-012-1287-3
Vázquez, M. D., Poschenrieder, C., and Barceló, J. (1987). Chromium VI induced structural and ultrastructural changes in Bush Bean plants (Phaseolus vulgaris L.). Ann. Bot. 59, 427–438.
Wakeel, A., Ali, I., Upreti, S., Azizullah, A., Liu, B., Khan, A. R., et al. (2018). Ethylene mediates dichromate-induced inhibition of primary root growth by altering AUX1 expression and auxin accumulation in Arabidopsis thaliana. Plant Cell Environ. 41, 1453–1467. doi: 10.1111/pce.13174
Wakeel, A., Xu, M., and Gan, Y. (2020). Chromium-Induced reactive oxygen species accumulation by altering the enzymatic antioxidant system and associated cytotoxic, genotoxic, ultrastructural, and photosynthetic changes in plants. Int. J. Mol. Sci. 21, 728. doi: 10.3390/ijms21030728
Wang, H. B., and Sheng, S. W. (2005). Ecology for heavy metal pollution: recent advances and future prospects. Acta Ecol. Sin. 25, 596–605.
Wang, S., Wu, W., Liu, F., Liao, R., and Hu, Y. (2017). Accumulation of heavy metals in soil-crop systems: a review for wheat and corn. Environ. Sci. Pollut. Res. 24, 15209–15225. doi: 10.1007/s11356-017-8909-5
Wang, W., Ciais, P., Nemani, R. R., Canadell, J. G., Piao, S., Sitch, S., et al. (2013). Variations in atmospheric CO2 growth rates coupled with tropical temperature. Proc. Nat. Acad. Sci. 110, 13061–13066. doi: 10.1073/pnas.1219683110
Wei, Y., Usman, M., Farooq, M., Adeel, M., Haider, F. U., Pan, Z., et al. (2022). Removing hexavalent chromium by nano zero-valent iron loaded on attapulgite. Water Air Soil Pollut. 233, 48. doi: 10.1007/s11270-022-05513-z
Xie, L., Hao, P., Cheng, Y., Ahmed, I. M., and Cao, F. (2018). Effect of combined application of lead, cadmium, chromium and copper on grain, leaf and stem heavy metal contents at different growth stages in rice. Ecotoxicol. Environ. Saf. 162, 71–76. doi: 10.1016/j.ecoenv.2018.06.072
Xu, F., and Deng, J. (2012). Effects of salicylic acid on germination of soybean seed under chromium stress. Soybean Sci. 31, 852–854.
Xu, L., Han, L., and Huang, B. (2011). Membrane fatty acid composition and saturation levels associated with leaf dehydration tolerance and post-drought rehydration in kentucky bluegrass. Crop Sci. 51, 273–281. doi: 10.2135/cropsci2010.06.0368
Zayed, A. M., and Terry, N. (2003). Chromium in the environment: factors affecting biological remediation. Plant Soil 249, 139–156. doi: 10.1023/A:1022504826342
Zeid, I. M. (2001). Responses of Phaseolus vulgaris to chromium and cobalt treatments. Biol. Plant. 44, 111–115. doi: 10.1023/A:1017934708402
Zeng, F., Chen, S., Miao, Y., Wu, F., and Zhang, G. (2008). Changes of organic acid exudation and rhizosphere pH in rice plants under chromium stress. Environ. Pollut. 155, 284. doi: 10.1016/j.envpol.2007.11.019
Zeng, F., Qiu, B., Wu, X., Niu, S., Wu, F., and Zhang, G. (2012). Glutathione-mediated alleviation of chromium toxicity in rice plant. Biol. Trace Elem. Res. 148, 255–263. doi: 10.1007/s12011-012-9362-4
Zeng, F., Wu, X., Qiu, B., Wu, F., Jiang, L., and Zhang, G. (2014). Physiological and proteomic alterations in rice (Oryza sativa L.) seedlings under hexavalent chromium stress. Planta 240, 291–308. doi: 10.1007/s00425-014-2077-3
Zeng, F., Zhou, W., Qiu, B., Ali, S., Wu, F., and Zhang, G. (2011). Subcellular distribution and chemical forms of chromium in rice plants suffering from different levels of chromium toxicity. J. Plant Nutr. Soil Sci. 174, 249–256. doi: 10.1002/jpln.200900309
Zenk, M. H. (1996). Heavy metal detoxification in higher plants: a review. Gene 179, 21–30. doi: 10.1016/S0378-1119(96)00422-2
Zhang, M., Xiangchun, W., Long, Y., and Yangyang, C. (2019). Research on progress in combined remediation technologies of heavy metal polluted sediment. Int. J. Environ. Res. Public Health 16, 5098. doi: 10.3390/ijerph16245098
Zhang, Q., Chang, G., Gao, T., Sun, H., Chen, Y., Yue, B., et al. (2017). “Review of chromium residue and chromium-containing waste water treatment,” in 6th International Conference on Energy and Environmental Protection (ICEEP 2017) (Advances in Engineering Research), 143. doi: 10.2991/iceep-17.2017.75
Zhang, X., Zhang, S., Xu, X., Li, T., Gong, G., Jia, Y., et al. (2010). Tolerance and accumulation characteristics of cadmium in Amaranthus hybridus L. J. Hazard. Mater. 180, 303–308. doi: 10.1016/j.jhazmat.2010.04.031
Zhang, X. H., Liu, J., Huang, H. T., Chen, J., Zhu, Y. N., and Wang, D. Q. (2007). Chromium accumulation by the hyperaccumulator plant Leersia hexandra Swartz. Chemosphere 67, 1138–1143. doi: 10.1016/j.chemosphere.2006.11.014
Zhang, Y., Snow, T., Smith, A. J., Holmes, G., and Prabakar, S. (2019). A guide to high-efficiency chromium (III)-collagen cross-linking: synchrotron SAXS and DSC study. Int. J. Biol. Macromol. 126,123–129. doi: 10.1016/j.ijbiomac.2018.12.187
Zhou, Z. S., Song, J. B., and Yang, Z. M. (2012). Genome-wide identification of Brassica napus microRNAs and their targets in response to cadmium. J. Exp. Bot. 63, 4597–4613. doi: 10.1093/jxb/ers136
Zhucheng, W., and Lin, W. (2020). Research progress on remediation of heavy metal pollution in soil by garden plants. Int. J. New Dev. Eng. Soc. 1, 117–125. doi: 10.25236/IJNDES.040116
Zlobin, I. E., Kartashov, A. V., and Shpakovski, G. V. (2017). Different roles of glutathione in copper and zinc chelation in Brassica napus roots. Plant Physiol. Biochem. 118, 333–341. doi: 10.1016/j.plaphy.2017.06.029
Keywords: heavy metals, chromium, oxidative stress, molecular regulation, physiology and biochemistry
Citation: Saud S, Wang D, Fahad S, Javed T, Jaremko M, Abdelsalam NR and Ghareeb RY (2022) The impact of chromium ion stress on plant growth, developmental physiology, and molecular regulation. Front. Plant Sci. 13:994785. doi: 10.3389/fpls.2022.994785
Received: 15 July 2022; Accepted: 10 August 2022;
Published: 28 October 2022.
Edited by:
Sikandar Amanullah, Northeast Agricultural University, ChinaReviewed by:
Kamel Shoueir, Université de Strasbourg, FranceAwais Shakoor, Teagasc Environment Research Centre, Ireland
Copyright © 2022 Saud, Wang, Fahad, Javed, Jaremko, Abdelsalam and Ghareeb. This is an open-access article distributed under the terms of the Creative Commons Attribution License (CC BY). The use, distribution or reproduction in other forums is permitted, provided the original author(s) and the copyright owner(s) are credited and that the original publication in this journal is cited, in accordance with accepted academic practice. No use, distribution or reproduction is permitted which does not comply with these terms.
*Correspondence: Depeng Wang, dwyandywang@163.com; Shah Fahad, shah_fahad80@yahoo.com; Mariusz Jaremko, mariusz.jaremko@kaust.edu.sa