- 1Department of Biotechnology, Graduate School of Engineering, Osaka University, Suita, Osaka, Japan
- 2Industrial Biotechnology Initiative Division, Institute for Open and Transdisciplinary Research Initiatives, Osaka University, Suita, Osaka, Japan
- 3Plant Translational Research Group, Universidad Regional Amazónica IKIAM, Tena, Ecuador
- 4Tsukuba-Plant Innovation Research Center, University of Tsukuba, Tsukuba, Ibaraki, Japan
Oleanolic acid is a pentacyclic triterpenoid found in numerous plant species and is a precursor to several bioactive triterpenoids with commercial potential. However, oleanolic acid accumulates at low levels in plants, and its chemical synthesis is challenging. Here, we established a method for producing oleanolic acid in substantial quantities via heterologous expression of pathway enzymes in Nicotiana benthamiana. The “Tsukuba system” is one of the most efficient agroinfiltration-based transient protein expression systems using the vector pBYR2HS, which contains geminiviral replication machinery and a double terminator for boosting expression. Additionally, the pBYR2HS vector contains an expression cassette for the gene-silencing suppressor p19 protein from tomato bushy stunt virus, which can also contribute to enhancing the expression of target proteins. In this study, we evaluated the applicability of this system to heterologous triterpenoid production in N. benthamiana. Medicago truncatula cytochrome P450 monooxygenase (CYP) 716A12 is the first enzyme to be functionally characterized as β-amyrin C-28 oxidase producing oleanolic acid. A mutant CYP716A12 (D122Q) with improved catalytic activity engineered in our previous study was co-expressed with other enzymes in N. benthamiana leaves. Using pBYR2HS, oleanolic acid yield was increased 13.1-fold compared with that using the conventional binary vector, indicating the advantage of the Tsukuba system. We also demonstrated the efficacy of co-expressing a mutant Arabidopsis thaliana HMGR1 catalytic domain, additional NADPH-cytochrome P450 reductase (CPR) transferring electrons to heterologous CYPs, and application of ascorbic acid for preventing leaf necrosis after agroinfiltration, to improve product yield. As a result, the product yields of both simple (β-amyrin) and oxidized (oleanolic acid and maslinic acid) triterpenoids were significantly improved compared with the previously reported yield in heterologous triterpenoid production in N. benthamiana leaves.
Introduction
Oleanolic acid is a naturally occurring pentacyclic triterpenoid found in more than 200 species of plants. Numerous studies have indicated that it is a precursor of a variety of bioactive triterpenoids of commercial interest, including bardoxolone methyl, a potent activator of the Nrf2 pathway and inhibitor of the NF-κB pathway, currently in a phase 2 clinical trial for the treatment of chronic diseases (Liby and Sporn, 2012). Oleanolic acid is also a precursor of onjisaponin F, which increases nasal anti-influenza virus IgA antibody titers (Li et al., 2016; Peng et al., 2020); platycodin D, which has antitumor and antiviral properties (Jeon et al., 2019; Kim et al., 2021); and maslinic acid, which is currently being studied as a new therapeutic agent with various activities, including anti-inflammatory, hepatoprotective, analgesic, antimicrobial, antimycotic, virostatic, and immunomodulatory activities (Fukumitsu et al., 2016; Reyes-Zurita et al., 2016). However, oleanolic acid accumulates at low levels in plants, and chemical synthesis is difficult due to its complex structure (Dale et al., 2020).
Oleanolic acid is biosynthesized from 2,3-oxidosqualene via cyclization catalyzed by oxidosqualene cyclase (OSC), i.e., β-amyrin synthase (bAS), to produce β-amyrin, followed by three-step oxidation at C-28 of the β-amyrin backbone through erythrodiol (28-hydroxy-β-amyrin) and oleanolic aldehyde as reaction intermediates. This oxidation reaction is catalyzed predominantly by cytochrome P450 monooxygenases (CYPs) belonging to the CYP716A subfamily (Fukushima et al., 2011; Suzuki et al., 2018). The CYP716A subfamily is a member of the CYP716 family of enzymes with highly conserved functionality in triterpenoid biosynthesis; the most common modification of the triterpene backbone catalyzed by CYP716A is three-step site-specific oxidation at the C-28 position of α-amyrin, β-amyrin, and lupeol (Fukushima et al., 2011, 2013; Miettinen et al., 2017). Medicago truncatula CYP716A12 was the first enzyme in the CYP716A subfamily to be functionally characterized as a multifunctional triterpene oxidase capable of producing these C-28-oxidized products (Carelli et al., 2011; Fukushima et al., 2011).
Previously, we identified the key amino acid residues responsible for determining catalytic activity and substrate specificity of CYP716A12 by functional analysis using engineered Saccharomyces cerevisiae producing the β-amyrin backbone (Romsuk et al., 2022). When key amino acid residues in substrate recognition sites were replaced, the product profiles of CYP716A12 were changed. Three mutant CYP716A12s (D122Q, Q358P, D122Q_Q358P) showed improved product yield for oleanolic acid, whereas two others (I212P and I212P_Q358P) predominantly produced erythrodiol.
A synthetic biology technique involving pathway reconstruction in heterologous hosts, such as yeast and Nicotiana benthamiana, has been investigated as an alternative method of triterpenoid production (Fukushima et al., 2011; Buyel et al., 2017; Reed et al., 2017; Suzuki et al., 2018; Dale et al., 2020). Transient protein expression in N. benthamiana leaves via agroinfiltration is a simpler, more flexible, and cost-effective method for studying cell biology and physiology, as well as for producing high levels of recombinant proteins than stable transgenic approaches or traditional cell culture-based systems (Buyel et al., 2017; Suzaki et al., 2019). Transient expression experiments in N. benthamiana have also led to the reconstruction of various natural product pathways. N. benthamiana has recently emerged as a suitable host for the production of terpenes, including triterpenoids (Reed et al., 2017; De La Pena and Sattely, 2021). An efficient and high-level transient expression system should produce a high yield of the desired compound. The Tsukuba system is one of the most efficient agroinfiltration-based transient protein expression systems for plant cells using the high-expression binary vector pBYR2HS (Yamamoto et al., 2018). This vector, which contains geminiviral replication machinery and a double terminator, has been shown to significantly increase transient protein expression efficiency in lettuce, N. benthamiana, tomatoes, eggplant, hot pepper, melon, and orchid. The double terminator enhances the expression level compared to a single terminator by eliminating transcriptional interference, and the geminiviral replication machinery results in a high yield of foreign protein expression (Yamamoto et al., 2018; Suzaki et al., 2019). However, it has not yet been applied to reconstitute plant triterpenoid pathways.
In this study, we evaluated the applicability of the Tsukuba system for triterpenoid production in N. benthamiana through pathway reconstruction. By comparing the pBYR2HS-based expression construct to the conventional binary vector without the geminiviral replication machinery, double terminator, we demonstrated the efficacy of pBYR2HS in increasing oleanolic acid production. We also chose the host based on the performance of two Nicotiana species in producing oleanolic acid. The optimal incubation time and application of ascorbic acid following agroinfiltration were also determined to prevent leaf necrosis and thus increase oleanolic acid production. In addition, we demonstrated the requirement for an additional NADPH-cytochrome P450 reductase (CPR) to optimize the microenvironment of CYPs and increase oleanolic acid production. We selected five mutant CYP716A12s that showed altered product profiles based on a previous study (Romsuk et al., 2022) as well as several wild-type CYP716A enzymes, including Vitis vinifera CYP716A15, Olea europaea CYP716A48, and Beta vulgaris CYP716A49, to compare the potential for high-titer oleanolic acid production using the Tsukuba system. The mutant enzyme CYP716A12_D122Q had the highest potential for heterologous oleanolic acid production. We also optimized the biosynthetic pathway to increase oleanolic acid production by co-introduction of mutant Arabidopsis thaliana HMGR1 catalytic domain (AtHMGR1cd-S577A) to boost the mevalonate pathway. Finally, we demonstrated the adaptability of this expression system for increasing yield in the bioactive triterpenoid pathway by highlighting maslinic acid as a key example.
This study established a general strategy for rapidly producing substantial quantities of triterpenoids and generating valuable and difficult-to-obtain triterpenoids with potent biological activity.
Materials and methods
Chemical authentic standards
All triterpenoid standards (uvaol, β-amyrin, erythrodiol, oleanolic acid, and maslinic acid) were purchased from Extrasynthese (Genay, France).
Plasmid construction
pRI 201-AN (TaKaRa Bio, Shiga, Japan), designed for high-level foreign gene expression in dicotyledonous plants, was used as a conventional binary vector in this study. To construct a gateway-compatible version of pRI 201-AN, a gateway cassette was inserted into pRI 201-AN digested with NdeI and SacI to generate pYS_015 (Srisawat et al., 2019). pRI201-AN was also used as the template vector for cassette amplification to construct pBYR2HS (Yamamoto et al., 2018). The structures of T-DNA regions of pBYR2HS and pRI 201-AN-based expression constructs are shown in Supplementary Figure 1. To construct a gateway-compatible version of pBYR2HS, pBYR2HS-SalI (Yamamoto et al., 2018) was digested with SalI, blunted with KOD polymerase, and ligated with GATEWAY conversion cassette frame A (Invitrogen, Carlsbad, CA, United States).
The entry clones of pENTR-CYP716A12, pENTR-selected mutant CYP716A12s, pENTR-CYP716A15, pENTR-CYP716A48, pENTR-CYP716A49, mutant A. thaliana HMGR1 catalytic domain (AtHMGR1cd-S577A), and Lotus japonicus NADPH-cytochrome P450 reductase class II (LjCPR2) were obtained in previous studies (Seki et al., 2008; Fukushima et al., 2011; Robertlee et al., 2018; Suzuki et al., 2018; Istiandari et al., 2021). The amino acid sequences of the enzymes are shown in Supplementary Method 1. The mutant CYP716A12s were generated by site-directed mutagenesis using a PrimeSTAR® Mutagenesis Basal Kit (TaKaRa Bio) in a previous study (Romsuk et al., 2022). We synthesized a complete codon-optimized L. japonicus β-amyrin synthase (LjOSC1) for expression in N. benthamiana (Supplementary Method 2). The coding sequences (CDSs) of the target genes were transferred to gateway-compatible versions of pBYR2HS and pYS_015 using Gateway LR Clonase II Enzyme Mix (Thermo Fisher Scientific, Waltham, MA, United States) to generate plasmids for transient expression in plant cells.
Transient expression in Nicotiana benthamiana
Expression constructs for target genes were introduced into Agrobacterium tumefaciens strain GV3101 (pMP90) by electroporation (MicroPulser™; Bio-Rad, Hercules, CA, United States). Transformants were selected by incubation for 3 days at 28°C on LB selection medium containing 50 mg/l kanamycin, 25 mg/l gentamycin, and 100 mg/l rifampicin. Each selected transformant was cultured in 2 ml LB selection medium at 28°C overnight with shaking at 200 rpm. Aliquots of 75 μl of the overnight cultures were transferred into 5 ml of the same medium and then cultured at 28°C overnight with shaking at 200 rpm. Freshly grown A. tumefaciens cells were resuspended in infiltration buffer (10 mM MgCl2, 10 mM MES, pH 5.6, 100 μM acetosyringone) to adjust OD600 to ~1 as described previously (Yamamoto et al., 2018). Equal volumes of A. tumefaciens harboring target constructs were mixed and infiltrated into the abaxial air spaces of the leaves of 5-week-old N. benthamiana plants using a needleless 1 ml syringe.
To prevent necrosis, we applied 0.3 ml of 200 mM ascorbic acid or 200 mM ascorbic acid sodium salt to each leaf at 2-day intervals (i.e., on days 1, 3, and 5) post-infiltration. The leaves of three plants were harvested for triterpenoid analysis at 7 days post-infiltration.
Metabolite extraction of Nicotiana benthamiana leaves
To quantify triterpenoid production in the N. benthamiana leaves, 20 μl internal standard (uvaol, 100 ppm in methanol) was added to the powdered freeze-dried leaf samples before extraction. The samples (10 mg) were extracted with 1 ml methanol (Wako, Osaka, Japan). Then the mixtures were vortexed and sonicated for 60 min (45% intensity; Sharp, Osaka, Japan). After centrifugation at 12,000 rpm for 5 min, the organic phase was transferred to a fresh tube using a Pasteur pipette. The extracted samples were evaporated using a centrifugal evaporator for 45 min or until they were dry. The remaining powder was resuspended in 1 ml methanol. To remove macromolecules such as sugar and chlorophyll from the extracted sample, saponification was performed by adding 1 ml methanol and 1 ml 4 M HCl. The mixtures were briefly vortexed, heated at 80°C for 1 h, and left to stand at room temperature for 10 min. The final extraction step was performed by adding 2 ml ethyl acetate and hexane (1:1 v/v). After centrifugation at 1500 rpm for 3 min, the organic phase was transferred to a fresh tube using a Pasteur pipette. The extracted samples were evaporated using a centrifugal evaporator for 60 min or until they were dry. The remaining powder was resuspended in 500 μl methanol. The obtained samples were transferred into vials using a Pasteur pipette and stored at 4°C until analysis.
GC–MS analysis of leaf extracts and standards
To quantify triterpenoid production in N. benthamiana, 50 μl sample solution was transferred to vial inserts and then to a centrifugal evaporator for 60 min or until dry. Then the evaporated pellet was derivatized with 50 μl N-methyl-N-(trimethylsilyl) trifluoroacetamide (Sigma-Aldrich, St. Louis, MO, USA) for 30 min at 80°C before GC–MS analysis. Aliquots of 50 μl authentic standard solutions (uvaol, β-amyrin, erythrodiol, oleanolic acid, maslinic acid, 10 ppm in methanol) were applied using the same approach as described above. GC–MS analysis was performed using a 5977A MSD coupled with a 7890B GC system and HP-5MS capillary column (length 30 m, 0.25 mm internal diameter, 0.25 μm film thickness; Agilent Technologies, Santa Clara, CA, United States). The injection component and the MSD transfer line were set to 250°C. The oven temperature was programmed as follows: 80°C for 1 min, followed by an increase to 300°C at a rate of 20°C min−1, and held at 300°C for 18 min. The carrier gas was helium (He) and the flow rate was 1 ml min−1.
Mass spectra were acquired by scanning the 50–750 m/z range. The retention times and mass spectra of the peaks were compared to those of authentic standards. The relative concentrations of triterpenoids in extracted samples were determined by comparing the peak areas of the analyte and internal standard. The concentrations of triterpenoids in the N. benthamiana leaves were determined by comparison with authentic standard curves constructed using β-amyrin, erythrodiol, oleanolic acid, and maslinic acid (Supplementary Method 3; Supplementary Figure 2).
Statistical analysis
The differences in oxidized triterpenoid concentrations between samples extracted from each combination or condition were determined by one-way analysis of variance (ANOVA), and the significance of the means was determined using Tukey’s test. The differences in oxidized triterpenoid levels between the two groups were compared using the unpaired Student’s t-test. Statistical analyses were performed using JASP 0.16 on macOS (JASP Team, University of Amsterdam, Amsterdam, the Netherlands). In all analyses, p < 0.05 was taken to indicate statistical significance.
Results
Establishing a method for improving oleanolic acid production
To compare the yield of oleanolic acid in transiently transfected N. benthamiana leaves harboring pBYR2HS-based expression construct to the conventional pYS_015-based expression construct, leaves were infiltrated with a mixture of A. tumefaciens harboring pBYR2HS expressing LjOSC1 (β-amyrin synthase), LjCPR2, and CYP716A12_D122Q or a combination of pYS_015 vector expressing LjOSC1, LjCPR2, CYP716A12_D122Q, and p19 by infiltration. Given that the pBYR2HS vector contains an expression cassette for p19, we included a separate p19 expression construct in the pYS_015 vector-based infiltration mixture.
As a background control, a combination of pBYR2HS expressing LjOSC1, LjCPR2, and empty vector or pYS_015 vector expressing LjOSC1, LjCPR2, empty vector, and p19 was used. We observed a single major product peak in the gas chromatogram of the extracts of N. benthamiana leaves transiently expressing CYP716A12_D122Q but not in the background control (Figure 1A). This peak was identified as oleanolic acid (3) by comparison with the authentic standard (Figure 1A). We quantified the triterpenoid contents of N. benthamiana leaf extracts to compare the accumulation of oleanolic acid (Figure 1B). When pYS_015 (conventional binary vector) was used, the accumulation of oleanolic acid was determined as 2.1 ± 0.7 mg/g dry weight (dw). Interestingly, the accumulation of oleanolic acid was increased 13.1-fold (27.3 ± 2.6 mg/g dw) when the pBYR2HS (Tsukuba system) vector was used (Figure 1B).
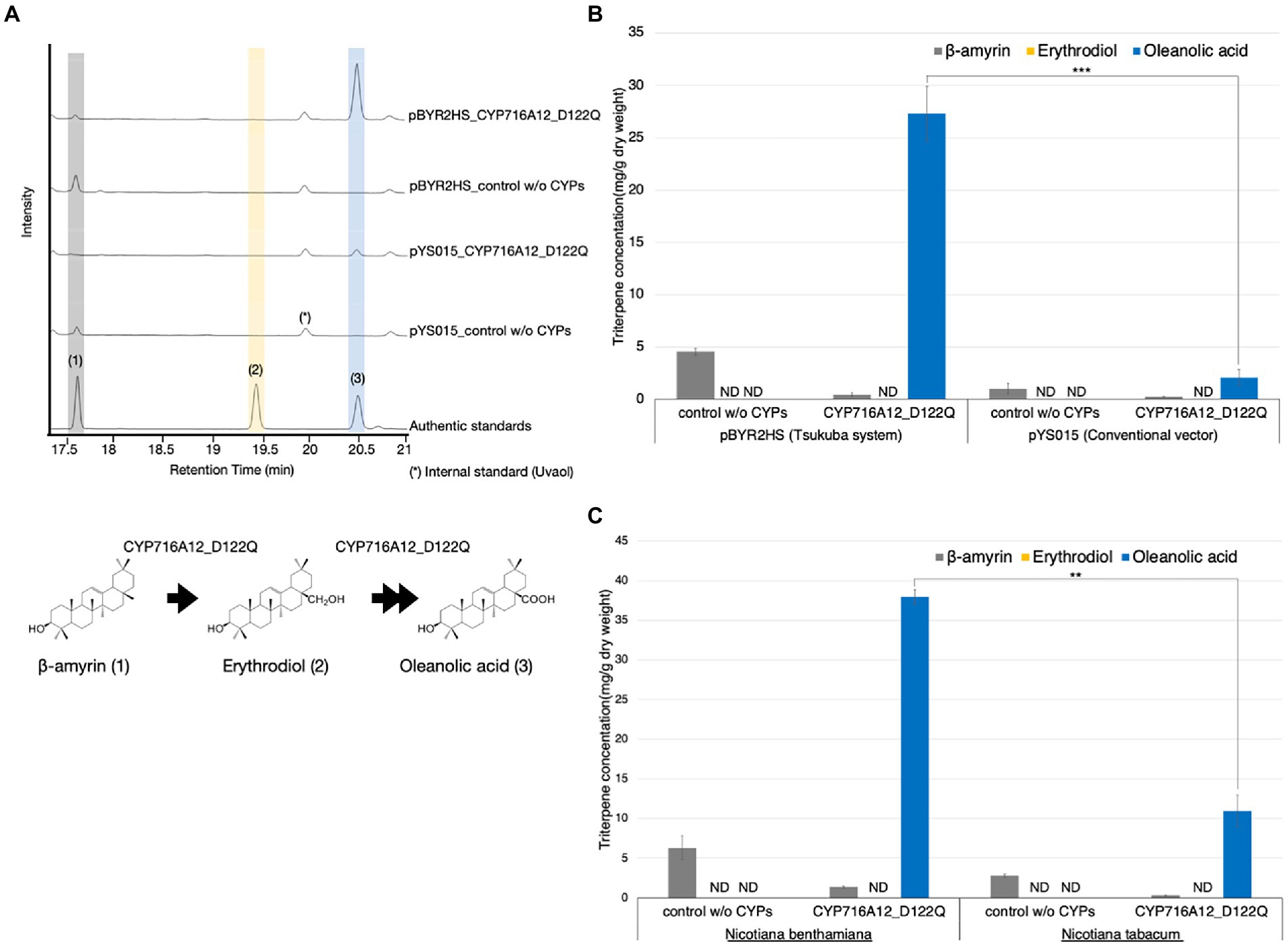
Figure 1. Comparison of the accumulation of oleanolic acid in leaves from different expression systems and Nicotiana species. (A) Total ion chromatograms (TICs) of extracts from Nicotiana benthamiana leaves infiltrated with a mixture of Agrobacterium tumefaciens harboring pBYR2HS expressing LjOSC1, LjCPR2, and CYP716A12_D122Q or a combination of the pYS_015 vector expressing LjOSC, LjCPR2, CYP716A12_D122Q, and p19. As a background control, a combination of pBYR2HS expressing LjOSC, LjCPR2, and empty vector or pYS015 binary vector expressing LjOSC1, LjCPR2, empty vector, and p19 was used. β-Amyrin (1), erythrodiol (2), and oleanolic acid (3) were used as authentic standards. Uvaol (*) was used as an internal standard. (B) Comparison of triterpene concentrations in N. benthamiana leaves infiltrated with different expression vectors. (C) Comparison of the triterpene concentrations in infiltrated leaves of N. benthamiana and N. tabacum. Data are representative of at least three biological replicates (n = 3) and are expressed as the mean ± SEM. Differences in oleanolic acid production were examined using Student’s t-test for each sample. **p < 0.01; ***p < 0.001; ND, not detected.
The transformation of Nicotiana species, specifically N. tabacum and N. benthamiana, with Agrobacterium using leaf disks as the target explant is an extremely useful method for rapid evaluation of transgenes in higher plants (Clemente, 2006). To select the better Nicotiana species for producing oleanolic acid using agroinfiltration-based transient protein expression, equal volumes of A. tumefaciens harboring expression vectors expressing LjOSC1, LjCPR2, and CYP716A12_D122Q were infiltrated into N. benthamiana and N. tabacum.
GC–MS was performed to determine the amounts of oleanolic acid in infiltrated leaves of different Nicotiana species (Supplementary Figure 3). Nicotiana benthamiana leaves yielded 37.9 ± 0.9 mg/g dw oleanolic acid, which was 3.3-fold higher than that in N. tabacum leaves (11.4 ± 1.7 mg/g dw; Figure 1C). Our findings indicate that both N. benthamiana and N. tabacum could be used as heterologous hosts for producing oleanolic acid using the Tsukuba system. However, in terms of product yield per leaf, our observations tend to indicate that of the two species, N. benthamiana would appear to provide a more promising production platform.
Requirement of additional CPR for optimization of the CYP microenvironment to enhance oleanolic acid production
CYPs require electrons transferred by NADPH-CPR for site-specific oxidation. In a heterologous host, native CPRs are likely to be insufficient partners for heterologous CYPs to achieve optimal performance (Zhu et al., 2018; Liu et al., 2019, 2021; Theron et al., 2019; Istiandari et al., 2021). To evaluate the efficacy of co-expression of additional CPR in our system, equal volumes of A. tumefaciens expressing LjOSC1, CYP716A12_D122Q with or without additional LjCPR2 were mixed and infiltrated into N. benthamiana. A mixture of A. tumefaciens harboring pBYR2HS expressing LjOSC1, LjCPR2, and an empty vector was used as a background control. GC–MS analysis and quantification of oleanolic acid in leaf extracts were performed (Figure 2). The N. benthamiana leaves transiently expressing LjOSC1 and CYP716A12_D122Q with LjCPR2 produced 39.3 ± 3.4 mg dw oleanolic acid, which was 2.7-fold higher than that without LjCPR2 co-expression (14.7 ± 0.3 mg/g dw; Figure 2B).
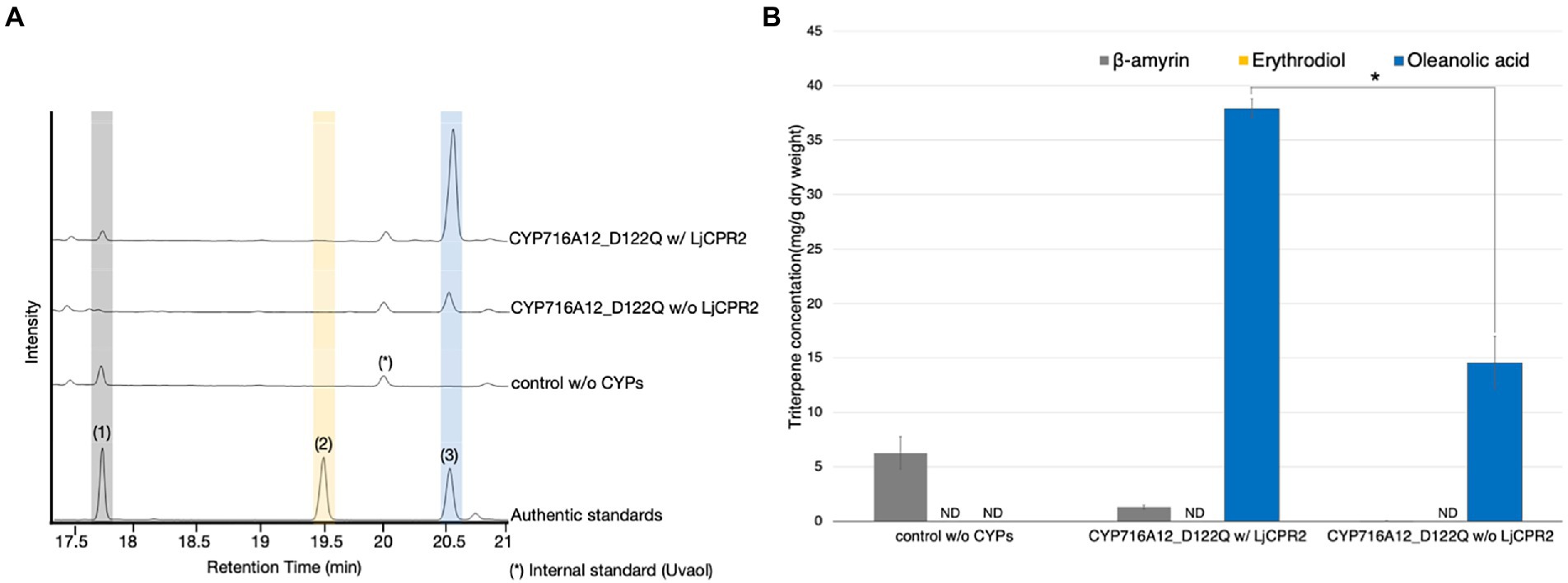
Figure 2. Requirement of additional CPR to optimize the CYP microenvironment for enhancement of oleanolic acid production in Nicotiana benthamiana. (A) TICs of extracts from N. benthamiana leaves transiently expressing a combination of LjOSC, CYP716A12_D122Q with or without LjCPR2 using pBYR2HS. A combination of LjOSC, LjCPR2, and empty vector was used as a background control. β-Amyrin (1), erythrodiol (2), and oleanolic acid (3) were used as authentic standards. Uvaol (*) was used as an internal standard. (B) Quantification of triterpene concentration in N. benthamiana leaf extracts. Data are representative of at least three biological replicates (n = 3) and are expressed as the mean ± SEM. Differences in oleanolic acid production were examined using Student’s t-test for each sample. *p < 0.05; ND, not detected.
These results indicate that additional CPR co-expression is effective to improve the microenvironment and achieve optimal performance of heterologous CYPs in N. benthamiana, leading to increasing oleanolic acid production.
Application of ascorbic acids for higher rates of oleanolic acid production
Agrobacterium-induced leaf necrosis, referring to the death of leaves, can occur in N. benthamiana leaves after infiltration (Nosaki et al., 2021). To prevent necrosis after agroinfiltration, leaves were sprayed with ascorbic acid, and optimal incubation times for improving oleanolic acid production were determined. The leaves were infiltrated with a mixture of equal volumes of A. tumefaciens harboring pBYR2HS expressing LjOSC1, LjCPR2, and CYP716A12_D122Q. A. tumefaciens harboring pBYR2HS expressing LjOSC1, LjCPR2, and empty vector was used as the background control. Leaves were sprayed with 200 mM ascorbic acid or 200 mM ascorbic acid sodium salt (sodium L-ascorbate) every 2 days (i.e., days 1, 3, 5, and 7) post-infiltration. Leaves that were not sprayed with ascorbic acid were used as negative controls. The leaves of three plants were harvested for triterpenoid analyses at 3, 5, 7, and 9 days post-infiltration. Extracts of leaves were subjected to GC–MS analysis and triterpenoid quantification (Figure 3; Supplementary Figure 4). Oleanolic acid production was significantly increased by spraying leaves with 200 mM ascorbic acid (30.3 ± 3.3 mg/g dw) or 200 mM sodium L-ascorbate (24.7 ± 2.8 mg/g dw) after 7 days of agroinfiltration compared with without spraying ascorbic acid (15.3 ± 1.7 mg/g dw). No significant difference was observed in the yield detected at 7 and 9 days after infiltration (Figure 3).
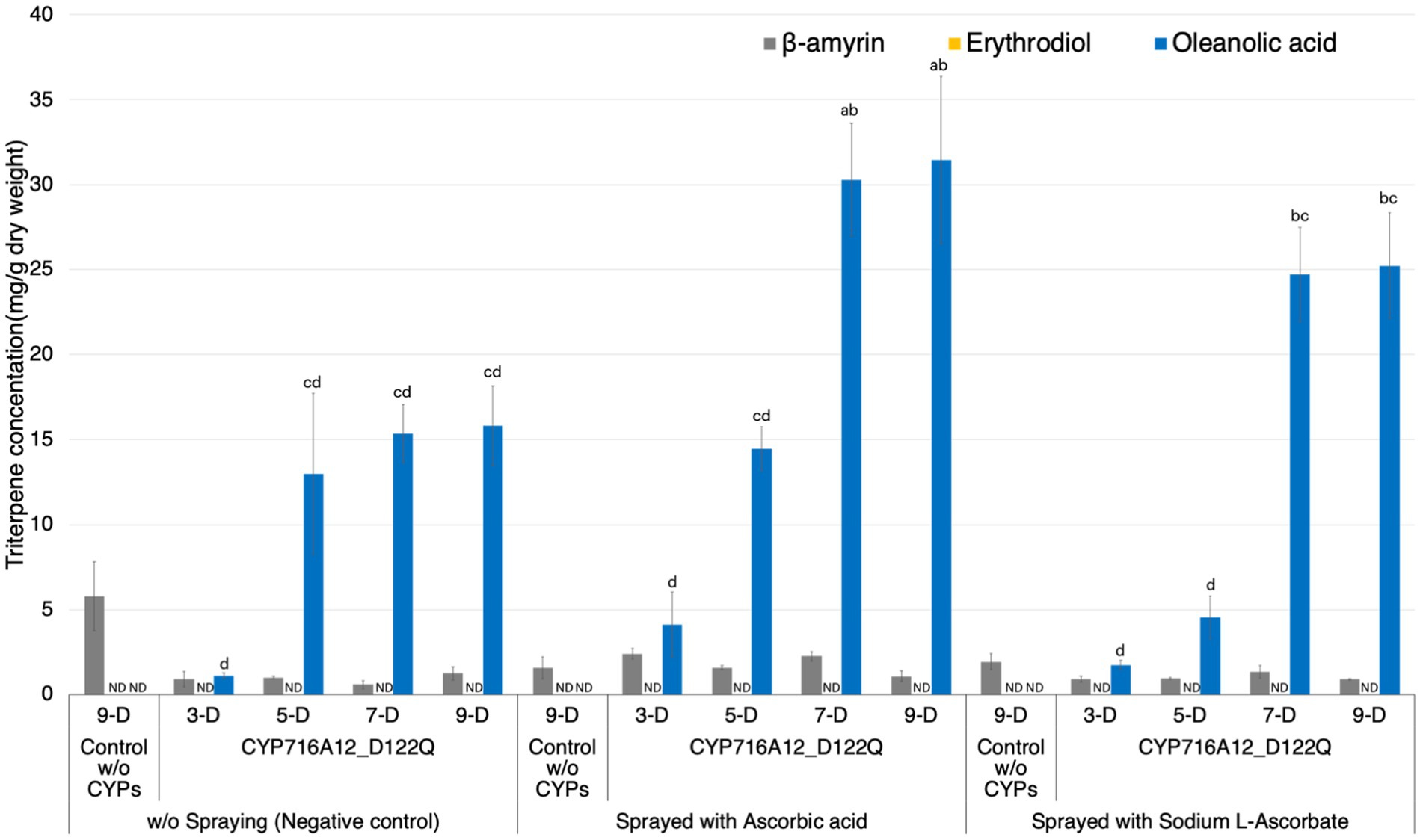
Figure 3. Improving production of oleanolic acid in Nicotiana benthamiana leaves by application of ascorbic acid. Quantification of triterpene concentration in N. benthamiana leaf extracts transiently expressing a combination of LjOSC, LjCPR2, and CYP716A12_D122Q or pBYR2HS empty vector (control) with or without foliar application of 200 mM ascorbic acid or ascorbic acid sodium salt (sodium L-ascorbate). Metabolites were extracted from leaves at 3, 5, 7, or 9 days after agroinfiltration. A combination of pBYR2HS expressing LjOSC, LjCPR2, and empty vector was used as a background control (9 days). Values and error bars represent the mean and standard deviation and the data are representative of at least three biological replicates (n = 3). Letters indicate significant differences in the levels of oleanolic acid (a–d) among samples (one-way ANOVA with Tukey’s post-hoc test, p < 0.05). ND, not detected.
As a result, foliar spraying with 200 mM ascorbic acid and incubation for 7 days after agroinfiltration yielded the highest oleanolic acid production titer, so these conditions were used in further experiments.
Identification of CYP716A12 mutants with the highest potential for oleanolic acid production
In a previous study, we identified critical amino acid residues for improving the catalytic activity and substrate specificity of CYP716A for triterpenoid production in S. cerevisiae (Romsuk et al., 2022). We chose five mutant CYP716A12s with altered product profiles when critical amino acid residues in the substrate recognition site were replaced, i.e., D122Q, I212P, Q358P, D122Q_Q358P, and I212P_Q358P. We also compared the catalytic activities of wild-type CYP716A12, CYP716A15, CYP716A48, and CYP716A49 in oleanolic acid biosynthesis to selected mutant CYP716A12s. In this experiment, all candidate genes were cloned into the pBYR2HS vector to identify the gene with the highest potential for oleanolic acid production in N. benthamiana using the Tsukuba system. Equal volumes of A. tumefaciens harboring a pBYR2HS expressing LjOSC1, LjCPR2, and candidate CYP716A were mixed and infiltrated into N. benthamiana. As a background control, leaves were infiltrated with a mixture of A. tumefaciens harboring pBYR2HS expressing LjOSC1, LjCPR2, and empty vector. To determine the triterpenoid profile of N. benthamiana leaf extract, metabolites were analyzed by GC–MS. The metabolite profiles of N. benthamiana leaves transiently expressing almost all candidates, but not the background control, showed a single major peak of oxidized triterpenoid. The major peak was identified as oleanolic acid (3) based on a comparison with the authentic standard (Figure 4A). Only when CYP716A12_I212P or CYP716A12_I212P_Q358P was transiently expressed, erythrodiol (2) was detected as a minor oxidized triterpenoid peak (Figure 4A). The concentrations of triterpenoids were examined in N. benthamiana leaf extracts transiently expressing each of the five mutant CYP716A12s or wild-type CYP716A12, CYP716A15, CYP716A48, or CYP716A49. The highest oleanolic acid concentration was detected in N. benthamiana leaves transiently expressing CYP716A12_D122Q (36.9 ± 1.6 mg/g dw; Figure 4B).
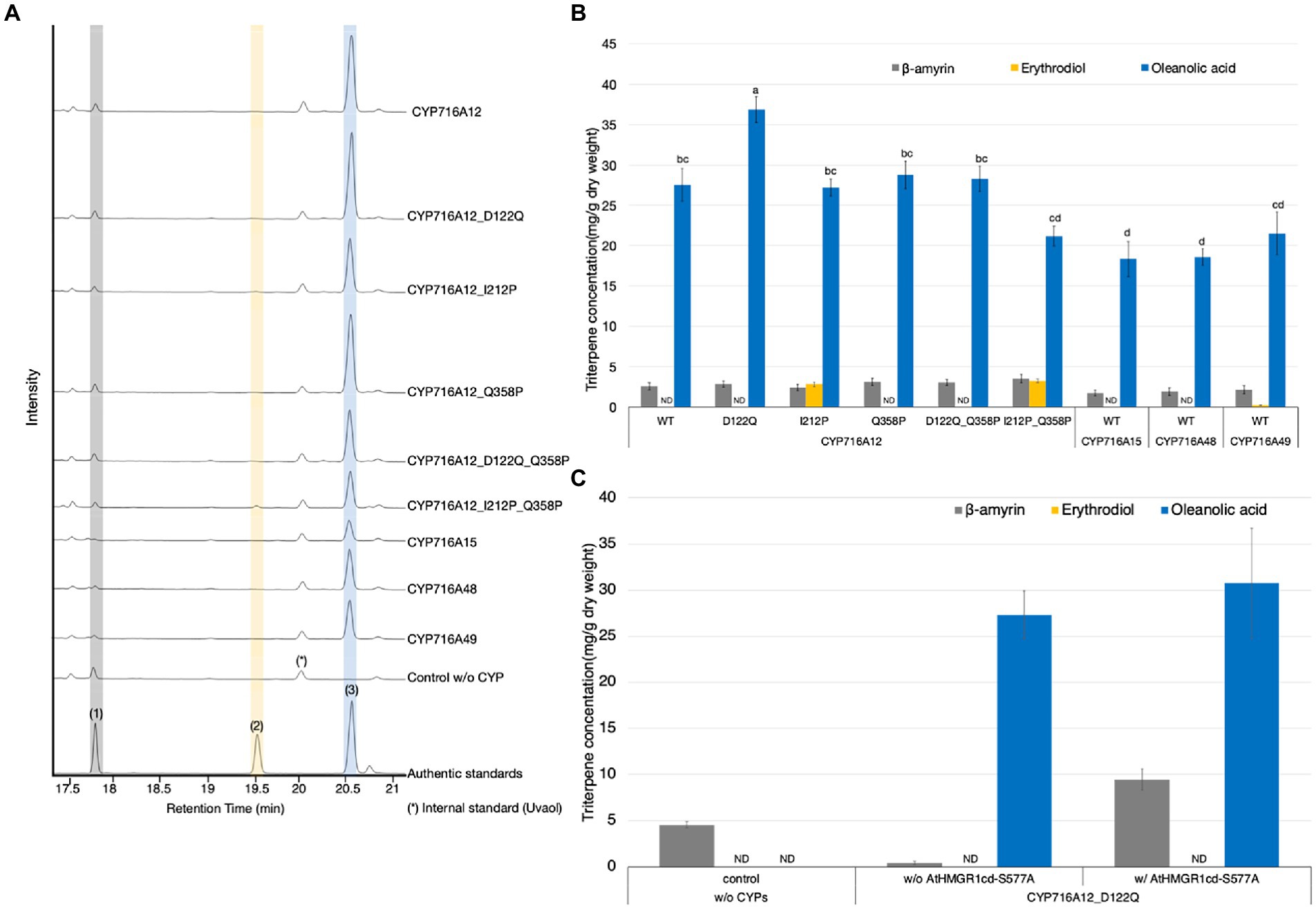
Figure 4. Identification of CYP716A with the highest potential for high-level oleanolic acid production in Nicotiana benthamiana using the Tsukuba system. (A) TICs of extracts from N. benthamiana leaves transiently expressing a combination of LjOSC, LjCPR2, and mutant CYP716A12s (D122Q, I212P, Q358P, D122Q Q358P, and I212P Q358P), wild-type CYP716As (CYP716A12, CYP716A15, CYP716A48, and CYP716A49), or empty vector as a control. β-Amyrin (1), erythrodiol (2), and oleanolic acid (3) were used as authentic standards. Uvaol (*) was used as an internal standard. (B) Quantification of triterpene concentration in N. benthamiana leaf extracts analyzed in (A). (C) The accumulation of triterpenes in N. benthamiana leaves transiently expressing a combination of LjOSC1, LjCPR2, and CYP716A12_D122Q with or without AtHMGR1cd-S577A. Values and error bars represent the mean and standard deviation, and the data are representative of at least three biological replicates with similar results from three independent experiments (n = 9). Letters indicate significant differences in the levels of oleanolic acid (a–d) among samples (one-way ANOVA with Tukey’s post-hoc test, p < 0.05). ND, not detected.
In our previous studies, co-expression of the A. thaliana HMGR1 catalytic domain with the S577A mutation (AtHMGR1cd-S577A, lacking an inactivating phosphorylation site and exhibiting better activity in vitro compared with AtHMGR1cd) increased triterpenoid production in agroinfiltrated N. benthamiana leaves (Robertlee et al., 2018; Srisawat et al., 2019). Therefore, we used this strategy to increase oleanolic acid production using the Tsukuba system. Leaves were infiltrated with a mixture of A. tumefaciens harboring pBYR2HS expressing LjOSC1, LjCPR2, and CYP716A12_D122Q with or without AtHMGR1cd-S577A. Leaves infiltrated with a combination of A. tumefaciens lacking CYPs were used as background controls.
The leaves were harvested at 7 days post-agroinfiltration and processed for metabolite extraction, GC–MS analysis, and quantification of oleanolic acid (Figure 4C; Supplementary Figure 5). As a result, the accumulation of β-amyrin was increased nearly 21-fold after co-expression of AtHMGR1cd-S577A (Figure 4C). The accumulation of oleanolic acid also tended to increase after co-expression of AtHMGR1cd-S577A (30.8 ± 6.0 mg/g dw; Figure 4C).
Combinatorial biosynthesis of maslinic acid in Nicotiana benthamiana
We hypothesized that our transient expression platform using the Tsukuba system would be suitable for high-titer production of oleanolic acid as a precursor of other bioactive triterpenoids. There are numerous natural sources of maslinic acid, a pentacyclic triterpene that has been used in traditional medicine (Lozano-Mena et al., 2014; Reyes-Zurita et al., 2016). We previously identified CYP716C53 from gray mangrove (Avicennia marina) showed that it can catalyze the C-2α hydroxylation of oleanolic acid to produce maslinic acid in engineered yeast (Nakamura et al., 2018). To produce maslinic acid in N. benthamiana leaves using the Tsukuba system, leaves were infiltrated with A. tumefaciens harboring pBYR2HS expressing LjOSC1, LjCPR2, CYP716A12_D122Q, and CYP716C53 with or without AtHMGR1cd-S577A. The leaves were harvested 7 days after agroinfiltration and processed for metabolite extraction. We performed GC–MS analysis and quantification of maslinic acid in leaf extracts (Figure 5). Maslinic acid (4) was detected, based on comparison with the authentic standard, as a major peak of oxidized triterpenoid in N. benthamiana leaves, only when CYP716C53 was transiently expressed. Three new minor peaks (peaks 5, 6, and 7) were also found when CYP716C53 was expressed, although the chemical structures of these peaks are unknown. The mass spectrometry data suggested that peaks 5 and 7 are likely isomers of maslinic acid differing in the position of the hydroxyl group introduced by CYP716C53 from C-2. Peak 6 was presumed to be a mono-hydroxylated product of β-amyrin (Figure 5A; Supplementary Figure 6).
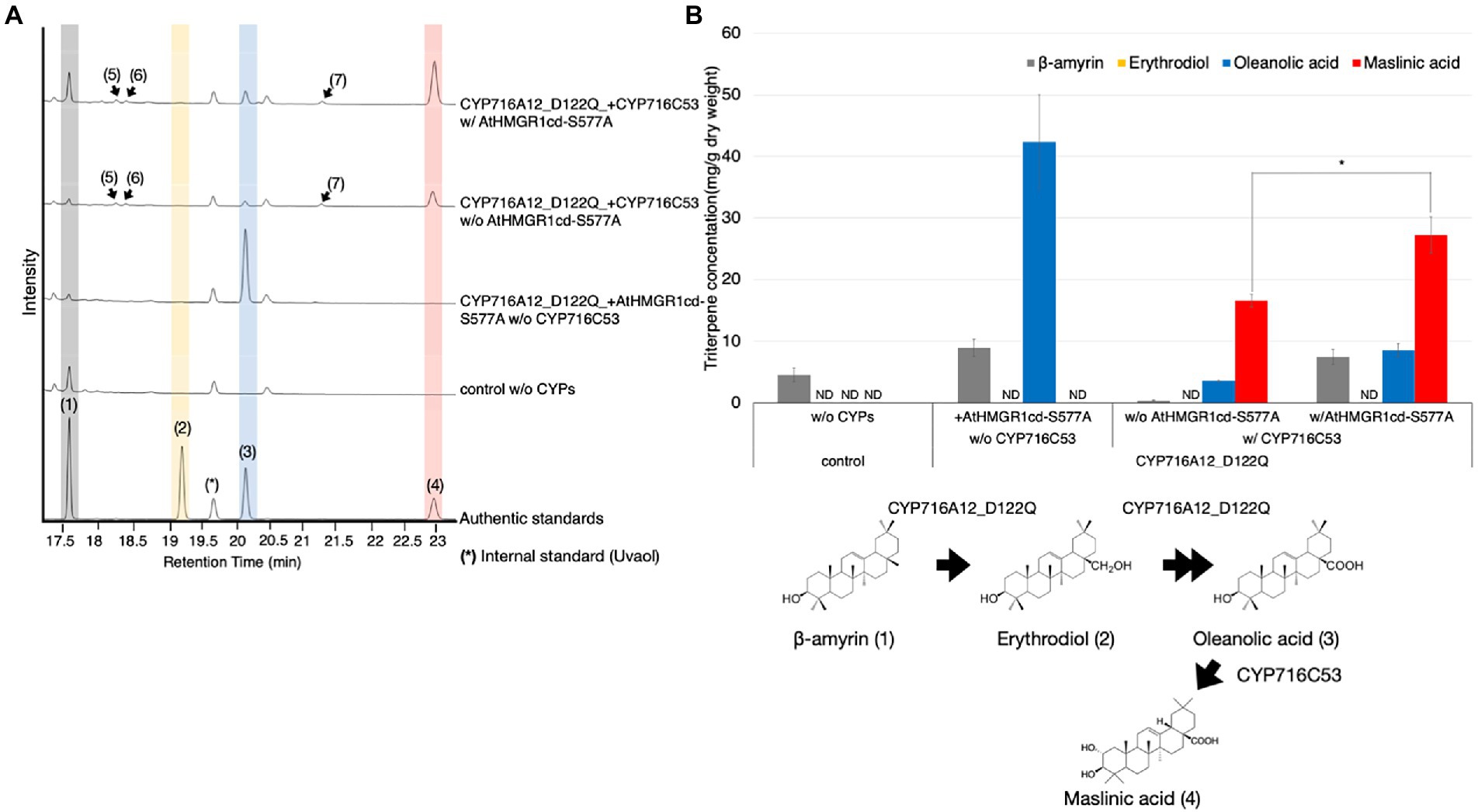
Figure 5. Production of maslinic acid in Nicotiana benthamiana leaves using the Tsukuba system. (A) TICs of extracts from N. benthamiana leaves transiently expressing a combination of LjOSC, LjCPR2, CYP716A12_D122Q, and CYP716C53 with or without AtHMGR1cd-S577A. A combination of pBYR2HS expressing LjOSC, LjCPR2, and empty vector was used as a background control. β-Amyrin (1), erythrodiol (2), oleanolic acid (3), and maslinic acid (4) were used as authentic standards. Uvaol (*) was used as an internal standard. (B) Quantification of maslinic acid concentration in N. benthamiana leaf extract. Data are representative of at least three biological replicates (n = 3) and are expressed as the mean ± SEM. Differences in maslinic acid concentration were compared using Student’s t-test for each sample. *p < 0.05; ND, not detected.
Maslinic acid production was quantified to allow comparison of the condition with versus without AtHMGR1cd-S577A (Figure 5B). Nicotiana benthamiana leaves transiently expressing LjOSC1, LjCPR2, CYP716A12_D122Q, and CYP716C53 without AtHMGR1cd-S577A showed maslinic acid production of 16.6 ± 1.0 mg/g dw, which was increased 1.6-fold (27.2 ± 3.0 mg/g dw) after co-expressing AtHMGR1cd-S577A (Figure 5B).
Discussion
Plants are abundant sources of the bioactive pentacyclic triterpenoids. For example, fruits and leaves of the olive tree (O. europaea) are particularly rich sources of oleanolic and maslinic acids. Both oleanolic and maslinic acids are concentrated on the surface of olive leaves to form a physical barrier that is involved in plant defense against water loss and pathogens (Chen et al., 2005; Jager et al., 2009; Romero et al., 2010; Gudoityte et al., 2021). Several recent studies have demonstrated that oleanolic acid has a diverse range of biological activities, including antitumor, antidiabetic, antioxidant, cardioprotective, neuroprotective, antiparasitic, and growth-stimulating properties. Moreover, oleanolic acid is a precursor of several commercially important bioactive triterpenoids (Liu, 1995; Jeon et al., 2019; Kim et al., 2021). CYP716A12 catalyzes the three-step oxidation of C-28 of β-amyrin to produce oleanolic acid via erythrodiol (28-hydroxy-β-amyrin) and oleanolic aldehyde intermediates (Fukushima et al., 2011; Suzuki et al., 2018). In a previous study using yeast engineered to produce β-amyrin, we found that the product profile of CYP716A12 was changed by substituting key amino acid residues on substrate recognition sites; three mutant CYP716A12s (D122Q, Q358P, D122Q_Q358P) had potential for high-level oleanolic acid production, and two mutant CYP716A12s (I212P, I212P_Q358P) had potential for high-level erythrodiol production in yeast-producing β-amyrin (Romsuk et al., 2022).
Significant progress in triterpenoid production has recently been reported using microorganisms, particularly S. cerevisiae, as alternative hosts (for a review, see Yao et al., 2020). However, when using such microbial systems, extensive metabolic engineering to maximize upstream carbon flux flow from acetyl-CoA to 2,3-oxidosqualene and improving enzyme activities by codon optimization are generally required to achieve high-yield production. Compared with microbial expression systems, Nicotiana species provide an optimal environment for plant-derived biosynthetic enzymes. A plant chassis is a rich source of precursor molecules, intermediates from primary metabolism, and enzyme cofactors required to facilitate the functioning of biosynthetic pathways. Moreover, expression of multiple biosynthetic enzymes can be achieved simply by coinfiltration of multiple A. tumefaciens strains, each containing a unique construct, without the need to generate multi-gene constructs (Christ et al., 2019; De La Pena and Sattely, 2021).
Using N. benthamiana as a heterologous expression host for rapid, increased production and reconstruction of plant triterpenoids pathways is a translational synthetic biology platform because it is susceptible to the Agrobacterium-based transient expression system, which is scalable and flexible (Geisler et al., 2013; Reed et al., 2017; Reed and Osbourn, 2018). The Tsukuba system is one of the most powerful transient protein expression systems for plant cells, utilizing agroinfiltration to deliver desired genes into plant cells for rapid recombinant protein production based on the use of a geminiviral replication machinery (Yamamoto et al., 2018; Suzaki et al., 2019). Heterologous expression in N. benthamiana utilizing the Tsukuba system showed that mutant CYP716A12 had the potential for high-level oleanolic acid production. The highest oleanolic acid production titer was found with the mutant CYP716A12 where aspartic acid 122 in substrate recognition site (SRS) I was substituted with glutamine (D122Q). Using this expression system, we demonstrate that oleanolic acid production was increased 13.1-fold (27.3 ± 2.6 mg/g dw) compared with the conventional binary vector (2.1 ± 0.7 mg/g dw; Figure 1B).
As mentioned above, CYP716A12_D122Q showed the highest production in N. benthamiana, although CYP716A12_D122Q_Q358P showed the highest production in yeast-producing β-amyrin, followed by CYP716A12_D122Q. This might be due to differences in the expression systems, which may affect the catalytic activity (Czarnotta et al., 2017), resulting in different oxidized product yields. Erythrodiol was detected as a minor product only when mutant CYP716A12s, in which the key amino acid residue isoleucine 212 (SRSII) was substituted with proline (I212P or I212P_Q358P). Isoleucine 212 and the nearest-neighbor residues in SRSII of CYP716A12 significantly influenced the efficiency of C-28 oxidation of triterpene backbones. By substituting these residues in the ligand-binding pocket, it is possible to alter the production of triterpene-oxidized products in N. benthamiana.
In this study, we also compared N. benthamiana and N. tabacum as hosts for the production of oleanolic acid. Although there were no significant differences in the production of β-amyrin between N. tabacum and N. benthamiana leaves (Figure 1C), the levels of oleanolic acid production were 3.3-fold higher in the leaves of N. benthamiana than in those of N. tabacum. These observations would thus tend to imply that in terms of product yield per leaf, N. benthamiana might be a more appropriate host for producing oleanolic acid. Biological stress caused by Agrobacterium infection causes necrosis and/or dehydration, reducing the transformation and expression efficiency of recombinant proteins (Herlache et al., 2001; Gils et al., 2005; Magdum, 2013). Spraying leaves after agroinfiltration with sodium L-ascorbate reduced the necrosis of leaves and increased the accumulation of human recombinant proteins transiently expressed in N. benthamiana (Nosaki et al., 2021). Our results indicate that spraying with 200 mM ascorbic acid after agroinfiltration led to an almost two-fold increase in oleanolic acid accumulation at 7–9 days after agroinfiltration (Figure 3).
Triterpenoids are structurally diverse because of modification of the triterpene scaffold by CYPs. These enzymes require electrons transferred by NADPH-CPR to catalyze site-specific oxidation (Istiandari et al., 2021). Triterpenoid production can be enhanced when CYPs are in a microenvironment that supports their optimal performance (Zhu et al., 2018; Liu et al., 2019, 2021; Theron et al., 2019; Istiandari et al., 2021). In this study, we observed that oleanolic acid production increased 2.70-fold after co-expression with LjCPR2 (Figure 2B). Our previous research also showed that the co-expression of CPRs derived from certain plant species may or may not be the best partners for CYPs in yeast (Istiandari et al., 2021). Therefore, the proper pairing of CYPs and CPRs would be critical for high-yield triterpenoid production in N. benthamiana. In addition, to achieve optimal CYP activity, future studies must determine the best CPR:CYP ratio.
In a previous report, the triterpenoid 12,13-epoxy,16-hydroxy-β-amyrin was obtained at a yield of 1.18 mg/g dw from leaves following transient expression in N. benthamiana (Geisler et al., 2013; Reed et al., 2017). Moreover, co-expression of the HMGR catalytic domain increased triterpenoid production; the levels of simple (β-amyrin) and oxidized triterpenoid (i.e., 12,13-epoxy,16-hydroxy-β-amyrin) production reached 3.3 and 3.9 mg/g dw, respectively (Reed et al., 2017; Reed and Osbourn, 2018). In our previous studies using a conventional binary vector, we also found enhanced α-amyrin production in N. benthamiana after transient co-expression of AtHMGR1cd with the S577A mutation (AtHMGR1cd-S577A) lacking the inactivating phosphorylation site (Robertlee et al., 2018; Srisawat et al., 2019). In the present study, we demonstrated that transient AtHMGR1cd-S577A expression increased triterpenoid production in N. benthamiana using the Tsukuba system. After transient co-expression of AtHMGR1cd-S577A, the amounts of both simple and oxidized triterpenoids were increased, yielding β-amyrin (9.4 mg/g dw, Figure 4C), oleanolic acid (30.8 mg/g dw, Figure 4C), and maslinic acid (27.2 mg/g dw, Figure 5B). Therefore, our transient expression system in N. benthamiana showed improved triterpenoid production compared with the previously reported yield.
Maslinic acid, a pentacyclic triterpene that has been used in traditional medicine for centuries, has antitumor, antidiabetic, antioxidant, cardioprotective, antiparasitic, and growth-stimulating properties (Jager et al., 2009; Romero et al., 2010; Reyes-Zurita et al., 2016). In our previous in vivo functional analysis of CYP716C53 in engineered yeast, maslinic acid was produced as a minor product when co-expressed with bAS, CPR, and CYP716A259 (β-amyrin C-28 oxidase of A. marina; Nakamura et al., 2018). In comparison, maslinic acid was detected as a major oxidized product when CYP716C53 was expressed in N. benthamiana leaves using the Tsukuba system (Figure 5). Maslinic acid is found in various natural sources, including olives, which are a rich source of maslinic acid (Romero et al., 2010; Lozano-Mena et al., 2014). The accumulation of triterpenoids in raw plant materials may also depend on the age of the plant (Jager et al., 2009; Romero et al., 2010; Reyes-Zurita et al., 2016). In this study, maslinic acid was produced in N. benthamiana leaves, yielding 27.2 ± 3.0 mg/g dw at 7 days after agroinfiltration (Figure 5B). This concentration is 20.7 times higher than that in olive fruit (cv. Kalamata), which have been reported to contain high maslinic acid concentration (1.3 mg/g dw; Romero et al., 2010; Lozano-Mena et al., 2014).
In conclusion, this study established a framework for optimizing oleanolic acid production as a precursor of bioactive triterpenoids via Agrobacterium-mediated transient expression in N. benthamiana using the Tsukuba system. We confirmed the utility of this approach by producing substantial amounts of maslinic acid, as an example of a pharmaceutically active plant-derived triterpenoid. This research will contribute to the development of a more efficient platform for synthesizing and accessing previously unavailable natural products and analogs, as well as the possibility of revitalizing drug discovery pipelines.
Data availability statement
The original contributions presented in the study are included in the article/Supplementary material, further inquiries can be directed to the corresponding author.
Author contributions
JR, SY, TM, and HS conceived the study. JR, KM, and HS designed the experiment. SY, EF, TM, and HS supervised the study. JR performed the experiments and wrote the manuscript. JR and HS analyzed the data. KM and HS made manuscript revisions. All authors contributed to the article and approved the submitted version.
Funding
This work was supported by JSPS KAKENHI Grant Numbers JP19H04657, JP20H02913, and JP21K19082 awarded to HS, Osaka University’s International Joint Research Promotion Program (Type B) awarded to EF and TM, and by the Monbukagakusho Scholarship from MEXT awarded to JR.
Acknowledgments
We thank the Leaf Tobacco Research Center, Japan Tobacco Inc., for providing Nicotiana benthamiana seeds. This study will be included in a dissertation submitted by JR to Osaka University in partial fulfillment of the requirement for his doctoral degree.
Conflict of interest
The authors declare that the research was conducted in the absence of any commercial or financial relationships that could be construed as a potential conflict of interest.
Publisher’s note
All claims expressed in this article are solely those of the authors and do not necessarily represent those of their affiliated organizations, or those of the publisher, the editors and the reviewers. Any product that may be evaluated in this article, or claim that may be made by its manufacturer, is not guaranteed or endorsed by the publisher.
Supplementary material
The Supplementary material for this article can be found online at: https://www.frontiersin.org/articles/10.3389/fpls.2022.991909/full#supplementary-material
References
Buyel, J. F., Twyman, R. M., and Fischer, R. (2017). Very-large-scale production of antibodies in plants: The biologization of manufacturing. Biotechnol. Adv. 35, 458–465. doi: 10.1016/j.biotechadv.2017.03.011
Carelli, M., Biazzi, E., Panara, F., Tava, A., Scaramelli, L., Porceddu, A., et al. (2011). Medicago truncatula CYP716A12 is a multifunctional oxidase involved in the biosynthesis of hemolytic saponins. Plant Cell 23, 3070–3081. doi: 10.1105/tpc.111.087312
Chen, Y., Liu, J., Yang, X., Zhao, X., and Xu, H. (2005). Oleanolic acid nanosuspensions: preparation, in-vitro characterization and enhanced hepatoprotective effect. J. Pharm. Pharmacol. 57, 259–264. doi: 10.1211/0022357055407
Christ, B., Xu, C., Xu, M., Li, F. S., Wada, N., Mitchell, A. J., et al. (2019). Repeated evolution of cytochrome P450-mediated spiroketal steroid biosynthesis in plants. Nat. Commun. 10:3206. doi: 10.1038/s41467-019-11286-7
Clemente, T. (2006). Nicotiana (Nicotiana tobaccum, Nicotiana benthamiana). Methods Mol. Biol. 343, 143–154. doi: 10.1385/1-59745-130-4:143
Czarnotta, E., Dianat, M., Korf, M., Granica, F., Merz, J., Maury, J., et al. (2017). Fermentation and purification strategies for the production of betulinic acid and its Lupane-type precursors in Saccharomyces cerevisiae. Biotechnol. Bioeng. 114, 2528–2538. doi: 10.1002/bit.26377
Dale, M. P., Moses, T., Johnston, E. J., and Rosser, S. J. (2020). A systematic comparison of triterpenoid biosynthetic enzymes for the production of oleanolic acid in Saccharomyces cerevisiae. PLoS One 15:e0231980. doi: 10.1371/journal.pone.0231980
De La Pena, R., and Sattely, E. S. (2021). Rerouting plant terpene biosynthesis enables momilactone pathway elucidation. Nat. Chem. Biol. 17, 205–212. doi: 10.1038/s41589-020-00669-3
Fukumitsu, S., Villareal, M. O., Aida, K., Hino, A., Hori, N., Isoda, H., et al. (2016). Maslinic acid in olive fruit alleviates mild knee joint pain and improves quality of life by promoting weight loss in the elderly. J. Clin. Biochem. Nutr. 59, 220–225. doi: 10.3164/jcbn.16-40
Fukushima, E. O., Seki, H., Ohyama, K., Ono, E., Umemoto, N., Mizutani, M., et al. (2011). CYP716A subfamily members are multifunctional oxidases in triterpenoid biosynthesis. Plant Cell Physiol. 52, 2050–2061. doi: 10.1093/pcp/pcr146
Fukushima, E. O., Seki, H., Sawai, S., Suzuki, M., Ohyama, K., Saito, K., et al. (2013). Combinatorial biosynthesis of legume natural and rare triterpenoids in engineered yeast. Plant Cell Physiol. 54, 740–749. doi: 10.1093/pcp/pct015
Geisler, K., Hughes, R. K., Sainsbury, F., Lomonossoff, G. P., Rejzek, M., Fairhurst, S., et al. (2013). Biochemical analysis of a multifunctional cytochrome P450 (CYP51) enzyme required for synthesis of antimicrobial triterpenes in plants. Proc. Natl. Acad. Sci. U. S. A. 110, E3360–E3367. doi: 10.1073/pnas.1309157110
Gils, M., Kandzia, R., Marillonnet, S., Klimyuk, V., and Gleba, Y. (2005). High-yield production of authentic human growth hormone using a plant virus-based expression system. Plant Biotechnol. J. 3, 613–620. doi: 10.1111/j.1467-7652.2005.00154.x
Gudoityte, E., Arandarcikaite, O., Mazeikiene, I., Bendokas, V., and Liobikas, J. (2021). Ursolic and Oleanolic acids: plant metabolites with Neuroprotective potential. Int. J. Mol. Sci. 22:4599. doi: 10.3390/ijms22094599
Herlache, T. C., Zhang, H. S., Ried, C. L., Carle, S. A., Zheng, D., Basaran, P., et al. (2001). Mutations that affect agrobacterium vitis-induced grape necrosis also Alter its ability to cause a hypersensitive response on tobacco. Phytopathology 91, 966–972. doi: 10.1094/PHYTO.2001.91.10.966
Istiandari, P., Yasumoto, S., Srisawat, P., Tamura, K., Chikugo, A., Suzuki, H., et al. (2021). Comparative analysis of NADPH-cytochrome P450 Reductases From legumes for heterologous production of Triterpenoids in transgenic Saccharomyces cerevisiae. Front. Plant Sci. 12:762546. doi: 10.3389/fpls.2021.762546
Jager, S., Trojan, H., Kopp, T., Laszczyk, M. N., and Scheffler, A. (2009). Pentacyclic triterpene distribution in various plants - rich sources for a new group of multi-potent plant extracts. Molecules 14, 2016–2031. doi: 10.3390/molecules14062016
Jeon, D., Kim, S. W., and Kim, H. S. (2019). Platycodin D, a bioactive component of Platycodon grandiflorum, induces cancer cell death associated with extreme vacuolation. Anim Cells Syst. 23, 118–127. doi: 10.1080/19768354.2019.1588163
Kim, T. Y., Jeon, S., Jang, Y., Gotina, L., Won, J., Ju, Y. H., et al. (2021). Platycodin D, a natural component of Platycodon grandiflorum, prevents both lysosome-and TMPRSS2-driven SARS-CoV-2 infection by hindering membrane fusion. Exp. Mol. Med. 53, 956–972. doi: 10.1038/s12276-021-00624-9
Li, X., Cui, J., Yu, Y., Li, W., Hou, Y., Wang, X., et al. (2016). Traditional Chinese Nootropic medicine radix Polygalae and its active constituent Onjisaponin B reduce beta-amyloid production and improve cognitive impairments. PLoS One 11:e0151147. doi: 10.1371/journal.pone.0151147
Liby, K. T., and Sporn, M. B. (2012). Synthetic oleanane triterpenoids: multifunctional drugs with a broad range of applications for prevention and treatment of chronic disease. Pharmacol. Rev. 64, 972–1003. doi: 10.1124/pr.111.004846
Liu, J. (1995). Pharmacology of oleanolic acid and ursolic acid. J. Ethnopharmacol. 49, 57–68. doi: 10.1016/0378-8741(95)90032-2
Liu, T., Huang, Y., Jiang, L., Dong, C., Gou, Y., and Lian, J. (2021). Efficient production of vindoline from tabersonine by metabolically engineered Saccharomyces cerevisiae. Commun. Biol. 4:1089. doi: 10.1038/s42003-021-02617-w
Liu, J., Zhang, C., and Lu, W. (2019). Biosynthesis of long-chain omega-Hydroxy fatty acids by engineered Saccharomyces cerevisiae. J. Agric. Food Chem. 67, 4545–4552. doi: 10.1021/acs.jafc.9b00109
Lozano-Mena, G., Sanchez-Gonzalez, M., Juan, M. E., and Planas, J. M. (2014). Maslinic acid, a natural phytoalexin-type triterpene from olives--a promising nutraceutical? Molecules 19, 11538–11559. doi: 10.3390/molecules190811538
Magdum, S. S. (2013). Effect of agrobacterium induced necrosis, antibiotic induced Phytotoxicity and other factors in successful plant. J. Stress Physiol. Biochem. 9, 98–112.
Miettinen, K., Pollier, J., Buyst, D., Arendt, P., Csuk, R., Sommerwerk, S., et al. (2017). The ancient CYP716 family is a major contributor to the diversification of eudicot triterpenoid biosynthesis. Nat. Commun. 8:14153. doi: 10.1038/ncomms14153
Nakamura, M., Linh, T. M., Lien, L. Q., Suzuki, H., Mai, N. C., Giang, V. H., et al. (2018). Transcriptome sequencing and identification of cytochrome P450 monooxygenases involved in the biosynthesis of maslinic acid and corosolic acid in Avicennia marina. Plant Biotechnol. 35, 341–348. doi: 10.5511/plantbiotechnology.18.0810a
Nosaki, S., Kaneko, M. K., Tsuruta, F., Yoshida, H., Kato, Y., and Miura, K. (2021). Prevention of necrosis caused by transient expression in Nicotiana benthamiana by application of ascorbic acid. Plant Physiol. 186, 832–835. doi: 10.1093/plphys/kiab102
Peng, F., Lu, L., Wei, F., Wu, D., Wang, K., and Tang, J. (2020). The onjisaponin B metabolite tenuifolin ameliorates dopaminergic neurodegeneration in a mouse model of Parkinson's disease. Neuroreport 31, 456–465. doi: 10.1097/WNR.0000000000001428
Reed, J., and Osbourn, A. (2018). Engineering terpenoid production through transient expression in Nicotiana benthamiana. Plant Cell Rep. 37, 1431–1441. doi: 10.1007/s00299-018-2296-3
Reed, J., Stephenson, M. J., Miettinen, K., Brouwer, B., Leveau, A., Brett, P., et al. (2017). A translational synthetic biology platform for rapid access to gram-scale quantities of novel drug-like molecules. Metab. Eng. 42, 185–193. doi: 10.1016/j.ymben.2017.06.012
Reyes-Zurita, F. J., Rufino-Palomares, E. E., Garcia-Salguero, L., Peragon, J., Medina, P. P., Parra, A., et al. (2016). Maslinic acid, a natural Triterpene, induces a death receptor-mediated apoptotic mechanism in Caco-2 p 53-deficient colon adenocarcinoma cells. PLoS One 11:e0146178. doi: 10.1371/journal.pone.0146178
Robertlee, J., Kobayashi, K., Tang, J., Suzuki, M., and Muranaka, T. (2018). Evidence that the Arabidopsis thaliana 3-hydroxy-3-methylglutaryl-CoA reductase 1 is phosphorylated at Ser577 in planta. Plant Biotechnol. 35, 1–7. doi: 10.5511/plantbiotechnology.17.1208a
Romero, C., Garcia, A., Medina, E., Ruiz-Mendez, M. V., de Castro, A., and Brenes, M. (2010). Triterpenic acids in table olives. Food Chem. 118, 670–674. doi: 10.1016/j.foodchem.2009.05.037
Romsuk, J., Yasumoto, S., Seki, H., Fukushima, E. O., and Muranaka, T. (2022). Identification of key amino acid residues toward improving the catalytic activity and substrate specificity of plant-derived cytochrome P450 monooxygenases CYP716A subfamily enzyme for triterpenoid production in Saccharomyces cerevisiae. Front. Bioeng. Biotechnol. 10:955650. doi: 10.3389/fbioe.2022.955650
Seki, H., Ohyama, K., Sawai, S., Mizutani, M., Ohnishi, T., Sudo, H., et al. (2008). Licorice beta-amyrin 11-oxidase, a cytochrome P450 with a key role in the biosynthesis of the triterpene sweetener glycyrrhizin. Proc. Natl. Acad. Sci. U. S. A. 105, 14204–14209. doi: 10.1073/pnas.0803876105
Srisawat, P., Fukushima, E. O., Yasumoto, S., Robertlee, J., Suzuki, H., Seki, H., et al. (2019). Identification of oxidosqualene cyclases from the medicinal legume tree Bauhinia forficata: a step toward discovering preponderant alpha-amyrin-producing activity. New Phytol. 224, 352–366. doi: 10.1111/nph.16013
Suzaki, T., Tsuda, M., Ezura, H., Day, B., and Miura, K. (2019). Agroinfiltration-based efficient transient protein expression in leguminous plants. Plant Biotechnol. 36, 119–123. doi: 10.5511/plantbiotechnology.19.0220b
Suzuki, H., Fukushima, E. O., Umemoto, N., Ohyama, K., Seki, H., and Muranaka, T. (2018). Comparative analysis of CYP716A subfamily enzymes for the heterologous production of C-28 oxidized triterpenoids in transgenic yeast. Plant Biotechnol. 35, 131–139. doi: 10.5511/plantbiotechnology.18.0416a
Theron, C. W., Labuschagne, M., Albertyn, J., and Smit, M. S. (2019). Heterologous coexpression of the benzoate-Para-hydroxylase CYP53B1 with different cytochrome P450 reductases in various yeasts. Microb. Biotechnol. 12, 1126–1138. doi: 10.1111/1751-7915.13321
Yamamoto, T., Hoshikawa, K., Ezura, K., Okazawa, R., Fujita, S., Takaoka, M., et al. (2018). Improvement of the transient expression system for production of recombinant proteins in plants. Sci. Rep. 8:4755. doi: 10.1038/s41598-018-23024-y
Yao, L., Wang, J., He, J., Huang, L., and Gao, W. (2020). Endophytes, biotransforming microorganisms, and engineering microbial factories for triterpenoid saponins production. Crit. Rev. Biotechnol. 41, 249–272. doi: 10.1080/07388551.2020.1869691
Keywords: plant-specialized metabolites, oleanolic acid, transient protein expression, CYP716A12, Nicotiana benthamiana, triterpenoids
Citation: Romsuk J, Yasumoto S, Fukushima EO, Miura K, Muranaka T and Seki H (2022) High-yield bioactive triterpenoid production by heterologous expression in Nicotiana benthamiana using the Tsukuba system. Front. Plant Sci. 13:991909. doi: 10.3389/fpls.2022.991909
Edited by:
Eiicihro Ono, Suntory, JapanCopyright © 2022 Romsuk, Yasumoto, Fukushima, Miura, Muranaka and Seki. This is an open-access article distributed under the terms of the Creative Commons Attribution License (CC BY). The use, distribution or reproduction in other forums is permitted, provided the original author(s) and the copyright owner(s) are credited and that the original publication in this journal is cited, in accordance with accepted academic practice. No use, distribution or reproduction is permitted which does not comply with these terms.
*Correspondence: Hikaru Seki, hseki@bio.eng.osaka-u.ac.jp