- Shaanxi Key Laboratory of Economic Plant Resources Development and Utilization, College of Forestry, Northwest A&F University, Yangling, China
The spicy taste and medicinal properties of Zanthoxylum bungeanum are imparted by several alkylamides. Although most studies have focused on their isolation and identification, few have reported their biosynthesis pathways. Among the differentially expressed genes (DEGs) reported in the numerous varieties of Z. bungeanum, some might contribute to alkylamide biosynthesis. However, they are not yet functionally validated. The present study explored the function of two genes, ZbFAD2 and ZbFAD3, in the alkylamide biosynthesis pathway, and their stable and transient expression in Arabidopsis thaliana and Nicotiana benthamiana were also analyzed. As compared with the wild-type (WT), the fatty acid content analysis indicated that ZbFAD2-A. thaliana transgenic seeds had lower oleic acid and higher linoleic acid contents, while the ZbFAD3-A. thaliana transgenic seeds showed lower linoleic acid and higher α-linolenic acid levels. Moreover, hydroxy-α-sanshool, a major alkylamide, was considerably higher in the ZbFAD2-N. benthamiana transgenic plants (0.2167 ± 0.0026 mg/g) than in the WT (0.0875 ± 0.0049 mg/g), while it was lower in the ZbFAD3-N. benthamiana transgenic plants (0.0535 ± 0.0037 mg/g). These results suggest that both ZbFAD2 and ZbFAD3 are vital alkylamide biosynthesis enzymes in Z. bungeanum. Our study not only helps to scale up the alkylamide production, but also establishes the role of the uncharacterized genes.
Introduction
Zanthoxylum bungeanum Maxim. (genus: Zanthoxylum; family Rutaceae) has recently gained significant attention from researchers because of its applications in the pharmaceutical, food, and cosmetic industries (Sun et al., 2019). They are widely distributed in the tropical and subtropical regions, including China, Japan, Korea, India, etc (Sun et al., 2019). To date, over 250 species have been identified globally in this genus, with China harboring at least 45 species (Sun et al., 2019). It contains a variety of health-beneficial bioactive compounds. Numerous studies have focused on the chemical structure and bioactivity of different compounds, including flavonoids, alkaloids, amides, lignans, and coumarins. Among these, alkylamides are known for the pungent flavor and pharmaceutical properties of Z. bungeanum. Twenty-seven alkylamides have been identified and characterized as unsaturated fatty acid amides (Zhao et al., 2013). Alkylamides are formed through conjunction with phenylalanine or valine along with unsaturated fatty acids. These compounds have diverse properties such as antiviral, antifungal, antibacterial, antidiabetic, anti-inflammatory, analgesic, antinociceptive, antioxidant, antithrombotic, antimutagenic, anticancer, antimalarial, antitrypanosomal, psychopharmacological, androgenic, spermatogenic, and insecticidal. They also have valuable immunomodulatory and ethnomedicinal uses and are effective in the treatment of anesthesia, neurodegeneration, and skin disorders (Greger, 1984). Therefore, it is imperative to study the natural origin and production of alkylamides due to their numerous benefits in medicine, food, and cosmetics. To date, most studies have focused on the identification and isolation of these compounds, while very few studies have studied the molecular biology behind their biosynthesis. Deciphering the biosynthesis pathways will not only enhance the understanding of the mechanism of action, but also help to augment their production through metabolic engineering. To achieve this, it is necessary to elucidate the numerous biosynthesis pathways, primary precursors, and genes involved in alkylamide regulation and biosynthesis. Previously, a transcriptome analysis of three varieties of Z. bungeanum was carried out (Wu et al., 2020), and 49 genes were expressed differentially. These genes are speculated to have roles in the alkylamide biosynthesis pathways. Therefore, the present study aims to determine the role of the ZbFAD2 and ZbFAD3 genes in the alkylamide biosynthesis pathway.
Z. bungeanum seeds contain over 90% unsaturated fatty acids (Sun et al., 2016). These are essential fatty acids as they cannot be synthesized by the human body (Stoutjesdijk et al., 2000). Their α- linolenic acid and linoleic acid contents are as high as ~70%. Fatty acid desaturases are extremely important in alkylamide biosynthesis. They desaturate most glycerolipids in plants, either membrane-bound or soluble and are present in the endoplasmic reticulum (ER) and chloroplasts (Bryant and Mezine, 1999). FAD2 and FAD3 mostly desaturate extra-chloroplastic lipids that are found as integral ER membrane-bound proteins (Los and Murata, 1998). In the ER, FAD2 destaurates oleic acid (18:1) into linoleic acid (18:2), while FAD3 destaurates linoleic acid into γ-linolenic acid (C18:3, n6) (Bhunia et al., 2016). During fatty acid desaturation, stearoyl-ACP desaturase first converts stearic acid into oleic acid in advanced plants. Then, the fatty acid dehydrogenase converts the unsaturated bonds into an acyl chain at particular positions (Los and Murata, 1998; Bhunia et al., 2016). The sensation of pungency related to Z. bungeanum is conferred by aliphatic alkylamides with unsaturated fatty acid chains and N-terminal isobutyl structures. Numerous studies on alkylamides have demonstrated the taste and bioactivities of Z. bungeanum (Caterina et al., 1997; Story et al., 2003; Bhunia et al., 2016; Zhang et al., 2019). Hydroxy-ϵ-sanshool, hydroxy-α-sanshool, hydroxy-β-sanshool, hydroxy-γ-sanshool, bungeanool, and isobungeanool are the main sources of alkylamides in Z. bungeanum. However, hydroxy-sanshool has been reported to affect the intensity of pungency in Z. bungeanum (Jordt et al., 2004). The molecular mechanisms of some alkylamide bioactivities have been deciphered, e.g., they display anticancer properties by disrupting the mitochondrion-dependent apoptotic pathway, thereby inhibiting cell survival (Jordt et al., 2004; Sugai et al., 2005). These compounds also improve glucose and lipid metabolism by modulating the A δ mechanosensory nociceptor activity and the pathway of the adenosine monophosphate-activated protein kinase, thereby affecting diabetes (Tsunozaki et al., 2013). Hydroxy-sanshool has been shown to decrease the occurrence of skin wrinkles (Kawai et al., 2013). It can also improve memory and learning by modulating the brain’s neuronal terminals (Sugai et al., 2005; Riera et al., 2009).
Alkylamides are considered a combination of unsaturated fatty acids, valine, or phenylalanine. However, the alkylamide biosynthesis pathway, critical precursors, and their associated genes in Z. bungeanum are yet to be identified. Although alkylamide compounds have been proven to be the key components of Z. bungeanum, their biosynthesis pathways remain largely unknown. Hydroxyl-α-sanshool is considered an important alkylamide variant. Upon examining the transcriptomes of the three Z. bungeanum varieties with significantly different hydroxyl-α-sanshool levels, it was found that several DEGs were associated with the unsaturated fatty acid, valine, and leucine biosynthesis tended to affect alkylamide synthesis (Rong et al., 2016). Plants are the only organisms capable of de novo fatty acid synthesis in the plastids, which include acetyl-CoA, palmitic acid (16:0), stearic acid (18:0), and oleic acid (18:1△9). These are then further desaturated to form linoleic acid (18:2△9,12) and linolenic acid (18:3△9,12,15) via the prokaryotic pathway. However, the eukaryotic pathway occurs via the ER (Koo et al., 2007). In the ER, FAD2 catalyzes the first step of the polyunsaturated fatty acid biosynthesis, converting oleic acid to linoleic acid and linolenic acid by incorporating a double bond between carbon 12 and 13 at the sn-1 and sn-2 sites in phosphatidylcholine. Additionally, omega-6 FAD2 and omega-3 FAD (FAD3) convert oleic acid (C18:1Δ9) to linoleic acid, linoleic acid, and α-linolenic acid (C18:3Δ9,12,15). FAD3, a linoleate desaturase, regulates the amount of C18:3 in the seed oil by converting the phosphatidylcholine-bound linoleate to linoleate in the ER. However, there are limited studies on the relationship of biosynthetic pathways between polyunsaturated FADs and alkylamides in Z. bungeanum. Furthermore, the whole genome study of Z. bungeanum has shown that ZbFAD2 and ZbFAD3 are crucial genes in sanshool biosynthesis (You et al., 2015).
The present study aimed to functionally validate the ZbFAD2 and ZbFAD3 genes, as well as evaluate the correlation between unsaturated fatty acids and alkylamide compounds. The findings will provide a theoretical basis for the research on the cannabinoids biosynthesis pathway in Z. bungeanum. Furthermore, the molecular research on this pathway in Z. bungeanum provides technical support for the accumulation and directional regulation of the compounds in Z. bungeanum, which has both theoretical significance and economic value.
Materials and methods
Profiling of alkylamide compounds in different varieties of Zanthoxylum bungeanum
Three Z. bungeanum varieties with considerable differences in their alkylamide content, including Hancheng stingless, Fengxian Dahongpao, and Fugu Huajiao (Wu et al., 2020), were used for alkylamide profiling (Figure S1). In a previous study, transcriptome sequencing of all three varieties was conducted to study their gene expression profiles, and then correlated with the biosynthesis of alkylamide compounds (Wu et al., 2020). RT-PCR was used to confirm the expression of both FAD3 (c102796 graph_c0) and FAD2 (c99494 graph_c0) genes. The enzyme classes encoded by the ZbFAD2 and ZbFAD3 genes were identified using homologous sequence comparison (Wu et al., 2020).
Bioinformatics analysis of ZbFAD2 and ZbFAD3
The lengths of the open reading frames (ORF Finder program: https://www.ncbi.nlm.nih.gov/orffinder/) of both FAD genes were determined. ZbFAD2 and ZbFAD3 sequences were subjected to BLAST search using the Uniprot database. Based on the FAD protein sequences from 10 different plants, the phylogenetic tree of the target genes (ZbFAD2 and ZbFAD3) and their homologous sequences were constructed using the MEGA software (Tamura et al., 2011). Phylogenetic trees were constructed from the ZbFAD2 and ZbFAD3 protein sequences. Online analysis tools like PROSITE (http://us.expasy.org/prosite/) and InterPro method (http://www.ebi.ac.uk/interpro/) were used to analyze the domains of the protein sequence of the gene sequence of interest.
Analysis of the functions of ZbFAD2 and ZbFAD3 in Arabidopsis thaliana
The ORF coding regions of ZbFAD3 and ZbFAD2 were amplified from Z. bungeanum by PCR with the following M13-specific primers: forward: 5’-CCCAGTCACGACGTTGTAAAACG-3’; reverse: 5’-CAGGAAACAGCTATGAC-3’. The PCR products were digested with KpnI and BamHI, and then inserted into the 35S-pCAMBIA2300 vector. This construct was transformed into the Agrobacterium tumefaciens GV3101 strain containing the pSoup helper plasmid. This was then transformed into Arabidopsis ecotype Col-0 via Agrobacterium-mediated transformation (Clough and Bent, 1998). T1 seeds were plated on 1/2 Murashige and Skoog (MS) medium containing 1% sucrose and 50 mg/mL kanamycin, for the selection of transformants. Homozygous T3 seeds were sown on 1/2 MS medium and stratified at 4°C for 3 d. T3 seeds were germinated in a growth chamber under a 16-h light/8-h dark cycle at 22°C for an additional 7 d. Seven-day-old plants germinated on 1/2 MS medium were transferred into pots containing a soil mixture (vermiculite: nutrient soil = 4:1). After being grown under favorable moisture conditions for 14 d, the number of surviving plants was recorded. There were three replicated assays. After maturation, the seeds were collected, and the differences in fatty acid composition between WT and transgenic A. thaliana seeds were quantified using gas chromatography (GC). With glyceryl triheptadecanoate (C17:0) as an internal standard (Fan et al., 2019), the proportion of the corresponding fatty acids in the seed was determined based on the peak time for each fatty acid methyl ester in the GC. The significant differences between groups were compared using the t-test (*p < 0.05 and **p < 0.01).
Analysis of the function of ZbFAD2 and ZbFAD3 in Nicotiana benthamiana
The ORFs of ZbFAD3 and ZbFAD2 were amplified from Z. bungeanum by PCR with the M13 specific following primers: forward: 5’-CCCAGTCACGACGTTGTAAAACG-3’; reverse: 5’-CAGGAAACAGCTATGAC-3’. The KpnI and BamHI digested PCR products were inserted into the 35S-pCAMBIA2300 vector. This construct was transformed into the Agrobacterium tumefaciens GV3101 strain containing the pSoup helper plasmid. To further verify the functions of ZbFAD2 and ZbFAD3 in the biosynthesis of alkylamide compounds, 35S-pCAMBIA2300-ZbFAD2 and 35S-pCAMBIA2300-ZbFAD3 were transformed into N. benthamiana via the injection method (Luo et al., 2022). Moreover, high-performance liquid chromatography (HPLC) was conducted to detect the differences in alkylamide levels between the transgenic and WT plants.
RNA extraction and quantitative real-time PCR
Total RNA was isolated using the TransZol UP reagent (Transgen Biotech Co., Ltd., Beijing, China) according to the manufacturer’s instructions. First-strand cDNA was synthesized by reverse transcription of purified total RNA using FastQuant RT Kit (Tiangen, Beijing, China). The CFX96 Touch TM (BioRaD, USA) quantitative PCR instrument was used to carry out the quantitative real-time polymerase chain reaction assay. Reactions comprised a 20-μL volume containing 2 μL diluted cDNA, 1 uL forward primer, 1 uL revise primer, and 25 uL 5x UltraSYBR Mixture. Thermal cycler conditions were: 95°C for 10 min, followed by 40 cycles of 95°C for 10 s, 56°C for 30 s and 72°C for 32 s, and 72°C for 2 min. The specificity of each primer pair was verified by melting curve analysis. The N. benthamiana β-actin gene was used as an internal control. The 2−ΔΔCT method (Livak and Schmittgen, 2001) was used for quantification, and the variation in expression was estimated from three biological replicates. The primer pairs used for qRT-PCR analysis are listed in Supplemental Table 2.
Fatty acid analysis of Arabidopsis seeds
Ten milligrams of transgenic seeds were put into 2 mL of 5% H2SO4 (v/v) in methanol for methylesterification, and added to each sample 0.25 mL of n-hexane and 0.25 mg of internal standard triglyceride heptadecacarbonate (C17:0). The methylesterification reaction was carried out at 80°C for 2 h. After cooling to room temperature, 1 mL of n-hexane and 2 mL of 0.9% NaCl solution were added. The mixture was then centrifuged at 5000 rpm for 10 min and the supernatant was aspirated for loading. In a gas chromatograph, the fatty acid methylation products were determined using a flame ionization detector (FID) equipped with an AT-FFAP column (0.25 mm × 30 m, 0.25 µm). The GC conditions of the evaporation chamber and FID were set to 260°C and 280°C, respectively. The temperature of the column oven was 160°C/min, then heated to 240°C at 4°C/min, and held at this temperature for 9 min. Using the high-purity helium as the carrier gas, the gas flow in the column was 1.46 mL/min and the split ratio was 20:1 (Fan et al., 2019). Three biological replicates were performed for each sample.
High-performance liquid chromatography assays
Tobacco leaves were ground to powder, 2 mL of chromatographic methanol was added, and ultrasonic extraction was performed at 60°C for 1 h. After passing through a 0.22 um filter head, it was refrigerated at 4°C for use. Three parallel experiments were performed for each sample.
The mobile phase is comprised of water (A) and acetonitrile (B). Hydroxy-α-Sanshool Elution Program 0 - 20 min, B: 25% - 55%; 20 - 40 min, B: 55% - 90%; 40 - 45 min, 90% B kept constant, Hydroxy-α- Sanshool. The retention time of hydroxy-α-sanshool was 20.554 min. The injection volume was 20 μL, the mobile phase flow rate was 0.8 mL/min, and the detection wavelength was 254 nm (Chen et al., 2019).
Results
Profiling of alkylamide compounds in different varieties of Zanthoxylum bungeanum
No significant differences in the several morphological traits were found among the three varieties (Figure S2). All the candidate alkylamide biosynthesis genes were expressed in the three varieties, as shown in Figure 1. RT-PCR expression results revealed similar patterns in the DEG analysis. Among the candidate genes, several FAD genes showed obvious differences at the transcript levels, particularly the c99494 graph_c0 and c102796 graph_c0 sequences (Table S1). Our results are consistent with a previous study (Feng et al., 2021). Through homologous sequence comparison, we analyzed two full-length cDNA sequences in candidate FAD genes and identified them as unsaturated fatty acid dehydrogenases (now designated as ZbFAD2 and ZbFAD3).
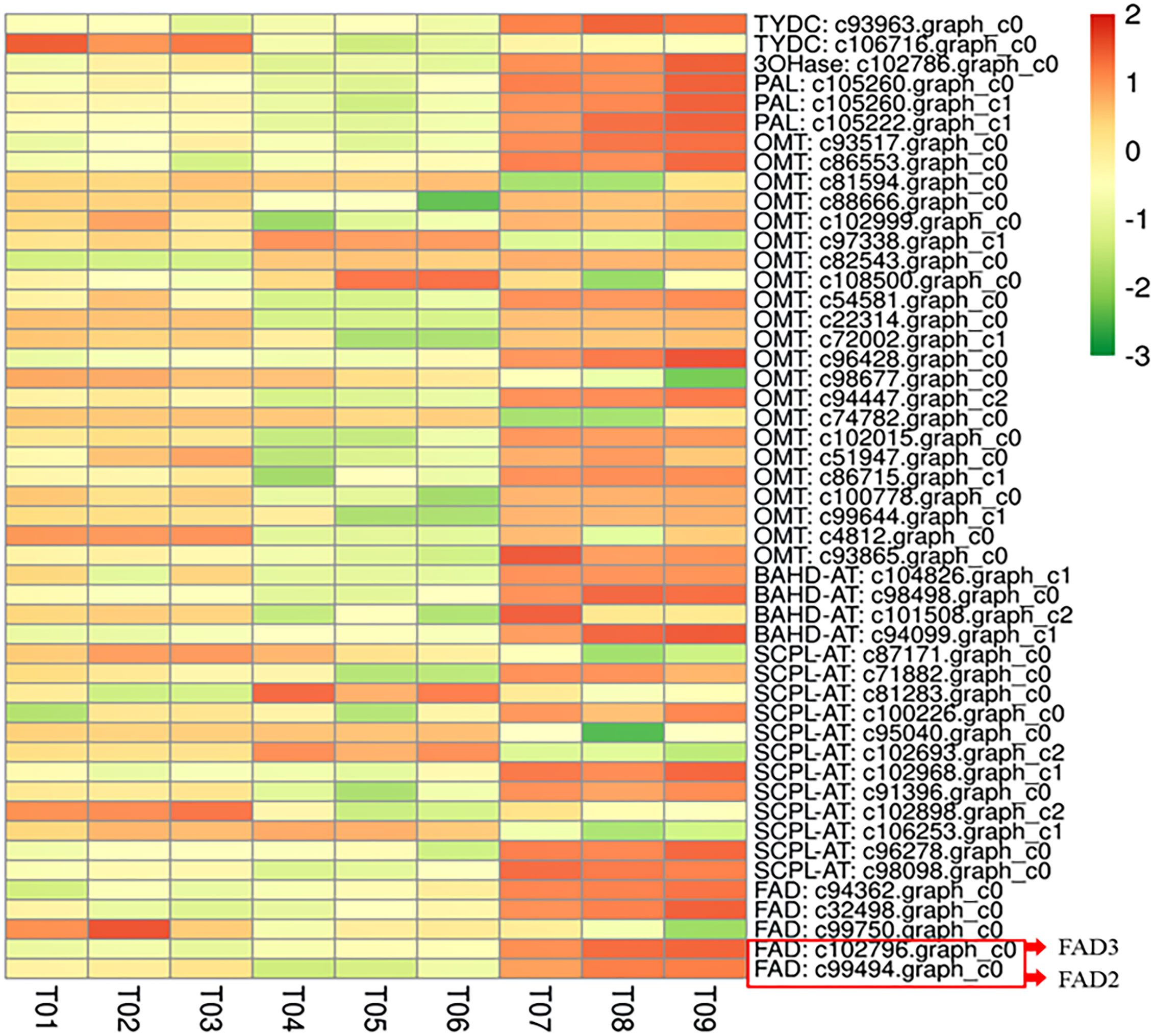
Figure 1 The expression levels of the DEGs related to the alkylamide biosynthesis pathway in Z. bungeanum. The highest expression of these genes was seen in Fugu (T07-T09), followed by Hancheng variety (T01-T03), with the lowest being in Fengxian (T04-T06).
Analysis of ZbFAD2 and ZbFAD3
The ORFs of ZbFAD2 and ZbFAD3 were determined to be 1,167 bp and 1,152 bp long, respectively. The results showed that Z. bungeanum and Citrus were grouped together in the first place, however, farther than that between Quercus and Jute. An amino acid sequence analysis showed the following properties of ZbFAD2 protein: (1) 383 amino acids long, (2) 44.16 kDa molecular weight, (3) isoelectric point of 8.04, (4) grand average of hydropathicity index (GRAVY) of −0.013, and (5) instability index of 37.48. Meanwhile, the properties of ZbFAD3 protein were as follows: (1) 388 amino acids, (2) 44.79 kDa molecular weight, (3) isoelectric point of 8.76, (4) GRAVY of −0.116, and (5) instability index of 33.76 (Figure S3).
Analysis of the functions of ZbFAD2 and ZbFAD3 in Arabidopsis thaliana
No apparent morphological difference was observed between the WT and transgenic A. thaliana plants grown up to 150 days. As shown in Figure 2, the level of linoleic acid was higher in the ZbFAD2-A. thaliana transgenic seeds (30.47%) than the WT (27.79%), while that of oleic acid was lower in the transgenic seeds (12.83%) than the WT (15.4%). However, the changes in the levels of other fatty acids were not significant. On the contrary, the level of α-linolenic acid was higher in the ZbFAD3-A. thaliana transgenic seeds (19.86%) than in the WT (17.47%), while that of linoleic acid was lower (24.84%) in the transgenic seeds than in the WT (27.79%). However, the changes in the levels of other fatty acids were also not significant in the ZbFAD3-A. thaliana seeds. Therefore, we demonstrated that the recombinant ZbFAD2 and ZbFAD3 proteins had desaturase activity and could catalyze the unsaturated fatty acid dehydrogenation. Thus, both recombinant ZbFAD2 and ZbFAD3 proteins could convert oleic acid into linoleic acid, and linoleic acid into α-linolenic acid, respectively, thereby playing an important role in the unsaturated fatty acid biosynthesis pathway.
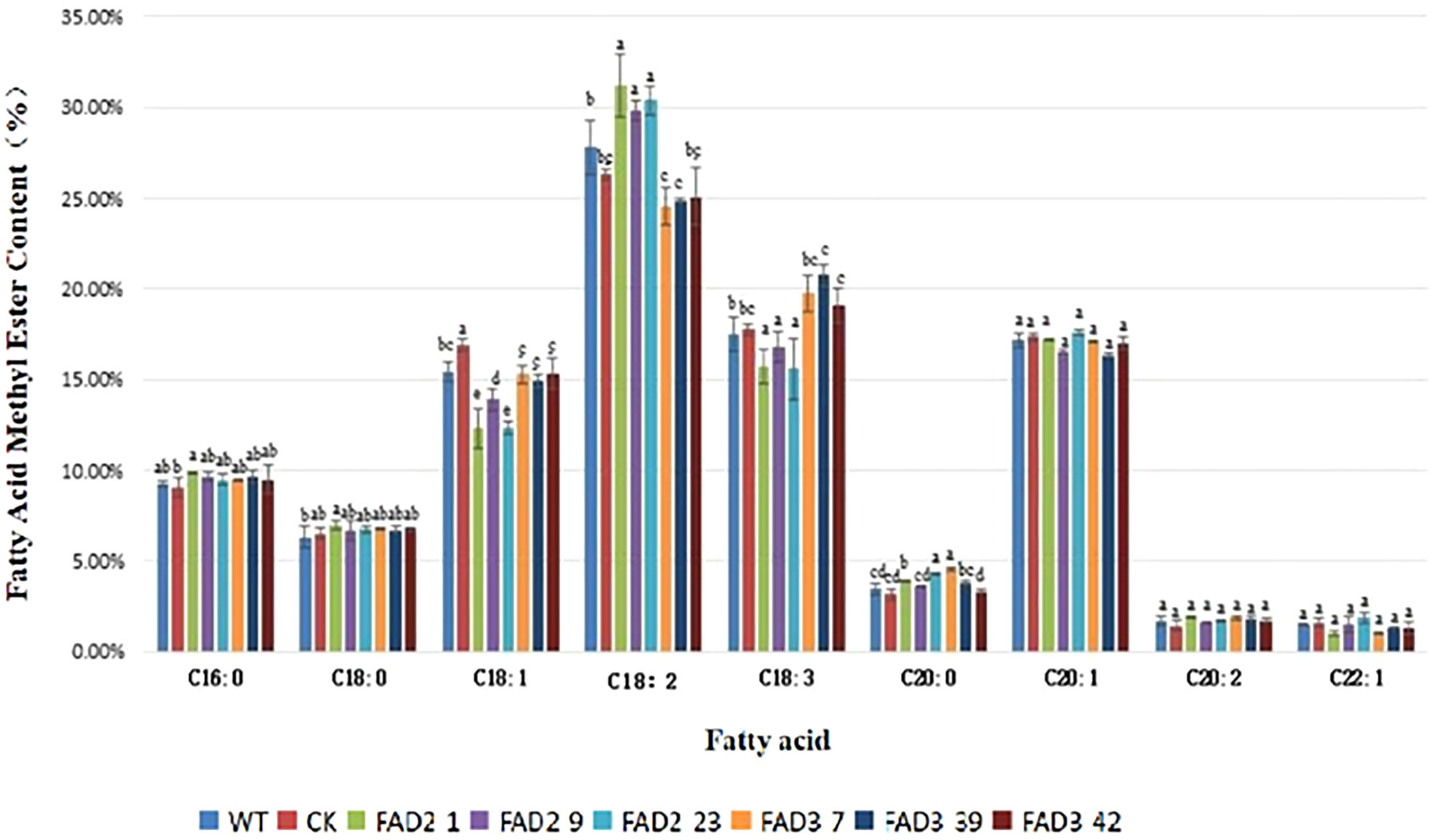
Figure 2 Fatty acid profiles and fatty acid methyl ester levels in the transgenic and WT A. thaliana seeds.
Analysis of the function of ZbFAD2 and ZbFAD3 in Nicotiana benthamiana
We detected an increased expression for both ZbFAD2 and ZbFAD3 (Figure 3) via qRT-PCR assays on ZbFAD3 and ZbFAD2 injection method transgenic tobacco. Using the N benthamiana ZbFAD3 and ZbFAD2 gene as the internal reference gene, the relative expression levels of ZbFAD3 and ZbFAD2 genes in WT and two transgenic plants are shown in Fig 3, the results showed that the relative expression levels of these two genes were 10.69% and 17.45% higher in transgenic plants than WT, respectively. Simultaneously, the ZbFAD3 transgenic plants showed that ZbFAD3 gene expression amplified the expression of ZbFAD2 gene. In contrast, an opposite trend was observed in the ZbFAD2 transgenic plants, where the ZbFAD2 gene expression reduced the expression level of ZbFAD3 gene. Therefore, it shows that these two genes may affect cannabinoid synthesis by affecting the expression of other functional genes in the synthesis pathway.
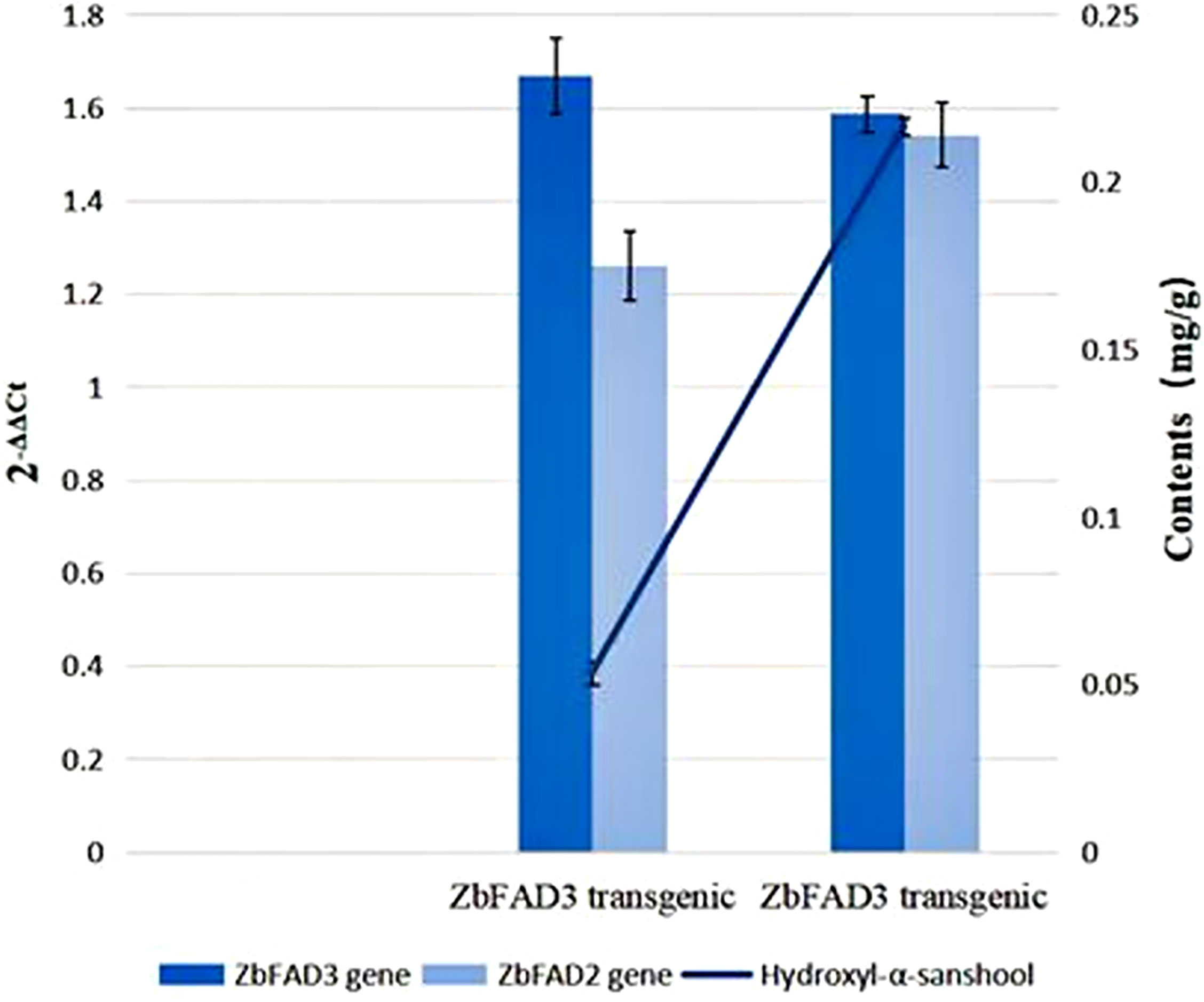
Figure 3 Expression analysis of the ZbFAD3 and ZbFAD2 genes using quantitative real-time PCR, of in the WT and transgenic N benthamiana plants.
The HPLC results indicated significantly higher hydroxy-α-sanshool levels in the ZbFAD2-transgenic N. benthamiana (0.2167 ± 0.0026 mg/g) plants as compared with the WT (0.0875 ± 0.0049 mg/g). Conversely, the level of hydroxy-α-sanshool was lower in the ZbFAD3-transgenic N. benthamiana (0.0535 ± 0.0037 mg/g) plants than in the WT (Figure 4). This is consistent with the previous findings that the expression of ZbFAD3 was down-regulated and ZbFAD2 was up-regulated in the transcriptome sequencing (Wu et al., 2020). Therefore, it can be inferred that these two candidate genes and unsaturated fatty acid biosynthesis are linked to alkylamide biosynthesis.
Discussion
In recent years, Z. bungeanum has increasingly been proven to be a valuable source of bioactive compounds, which endowed it with several human health beneficial properties. Along with the pharmaceutical industry, both the cosmetics and food industries have also benefited. In this regard, numerous classes of compounds, including alkylamides, from Z. bungeanum have been explored and characterized. Alkylamides not only have an extensive range of bioactivities and applications, but also impart a distinct tingling sensation onto mucosal surfaces post application (Tsunozaki et al., 2013). Sanshools are valuable natural alkylamides that cause tingling and paresthesia when applied to the skin or tongue. These belong to a family of polyunsaturated fatty acid amides and constitute an essential flavor and medicinal ingredient in Z. bungeanum, which can be used as metabolic markers to evaluate Z. bungeanum quality. It is important to analyze different plant varieties, their gene expression, and the genetic differences in the Z. bungeanum biosynthetic pathways (Bhatt et al., 2017) for screening and improving its germplasm. Most studies have focused on their biological activities, extraction, and separation, but fewer have studied their secondary metabolism pathway and the synthetic regulation of sanshools. Therefore, the sanshool biosynthesis pathways and the associated active players involved in it still remain obscure (Cortez-Espinosa et al., 2011; Ren et al., 2017; Wu et al., 2020). The transcriptome sequencing of Z. bungeanum helped identify 19 DEGs related to unsaturated fatty acid, isoleucine, leucine, and valine biosynthesis. Keeping this in mind, our study results confirmed the importance of the ZbFAD3 and ZbFAD2 proteins in the alkylamide biosynthesis pathway.
This is the first time that Arabidopsis thaliana has been used to express the ZbFAD2 and ZbFAD3 proteins. Although FAD2 and FAD3, which were previously thought to transform oleic acid into linoleic acid, had been studied in different plants, no reports were found on the cloning and subsequent characterization of these desaturases from Z. bungeanum. The current work is the first report on the isolation of FAD2 and FAD3 from this plant. We performed standard bioinformatics analyses to determine their role in the alkylamide biosynthesis pathways. Through phylogenetic analysis of both desaturases, and the results obtained from gene function verification, we found that these genes were closely associated with PtFAD2 and PeFAD2. This will be helpful in managing the demand pressure of alkylamides in the medicine, cosmetics, and food industry (Xue et al., 2017; Gazave et al., 2020; Feng et al., 2021).
Both desaturases (FAD2 and FAD3) are part of a larger family (FAD) of the membrane FADS-like superfamily. Five transmembrane helices, composed of a random coil, beta-turn, and alpha helix, were found in the secondary structures of FAD2 and FAD3. According to the transcriptomic study of Z. bungeanum, FAD5, FAD6, FAD7, and FAD8 were also involved in the biosynthesis of fatty acids. FAD2 and FAD6 converted oleic acid into linoleic acid via desaturation, whereas FAD3, FAD7, and FAD8 converted linoleic acid into α-linolenic acid through desaturation. This highlights the potential of using Z. bungeanum for synthesizing linoleic acid (Chen et al., 2014; Busch et al., 2020). Increasing linoleic acid content in plants is mainly achieved through their trait-directed breeding. The expression of FAD2 in Brassica napus and Brassica juncea increased their oleic acid content to 89% and 73%, respectively (Horiguchi et al., 2000). FAD3 gene has been successfully cloned in Arabidopsis (Arondel et al., 1992), tobacco (Shimada et al., 2000; Orlova et al., 2003), wheat (Horiguchi et al., 2000), flax (Vrinten et al., 2005), rapeseed and other plants. It has been found that inhibiting the expression of FAD3 in Arabidopsis thaliana or other plants, that is, inactivating and mutating it, will cause the content of 18:3 in the transgenic lines to decrease and the content of 18:2 to increase (Rahman et al., 1996). On the contrary, overexpression of FAD3 gene will increase the 18:3 content in transgenic lines (Shimada et al., 2000; Orlova et al., 2003; Shah and Xin, 1997).
Ectopic expression of FAD2 and FAD3 genes in the Arachis hypogaea, Sesamum indicum, Helianthusannuus, etc different plants have shown that these genes could convert oleic acid into linoleic acid and generate hexadecadienoic acid (16:2 Δ9,12), using palmitoleic acid (16:1Δ9) as a catalyzed substrate. This may be attributed to some specific substrate preference or varied transmembrane topologies found in the FAD2s and FAD3s (Huang et al., 2010; Fan et al., 2019).
Three Z. bungeanum varieties were subjected to transcriptomic analysis in a previous study (Wu et al., 2020). Based on the obtained data, we found that all alkylamide biosynthesis-related genes were expressed in the three varieties, with varying degrees of expression. However, some FAD genes exhibited differences in transcript levels, which were consistent with previous findings. Upon performing a homologous sequence comparison based on the cDNA sequences of these FAD genes, we identified them as belonging to the unsaturated fatty acid dehydrogenase family and subsequently designated them as ZbFAD2 and ZbFAD3. The lengths of the ORFs of these enzymes were determined to be 1,167 bp and 1,152 bp, respectively. By comparing the expression pattern and linoleic content, we found that the expression levels of ZbFAD2 and ZbFAD3 were positively correlated with the linoleic acid concentration of Z. bungeanum. The findings demonstrate that ZbFAD2 and ZbFAD3 significantly affect the accumulation and regulation of polyunsaturated fatty acids (PUFAs), by converting oleic acid to linoleic acid at different growth stages of Z. bungeanum.
Based on the results of bioinformatics analysis and prokaryotic expression, we found that ZbFAD2 and ZbFAD3 encoded ER-located-like FAD2 and FAD3 enzymes. The functional characterization of desaturases in A. thaliana tested the ability of ZbFAD2 and ZbFAD3 proteins in catalyzing linoleic acid synthesis. Our study provides proteomic data for the molecular regulation and coordination in Z. bungeanum with high linoleic acid content. Further characterization of ZbFAD2 and ZbFAD3 and their functional relationship may provide more information on the mechanisms controlling the fatty acid composition of Z. bungeanum, which may help in trait improvement via both genetic engineering and breeding.
The molecular weight of both purified proteins was very close to the bioinformatically-predicted value. Our findings showed that ZbFAD2 reacted with oleic acid (C18:1△9) to produce linoleic acid (C18:2△9,12), while ZbFAD3 potentially reacted with linoleic acid to produce α-linolenic acid (C18:3△9,12,15) (Figure 4). The homologous sequence analysis revealed that ZbFAD2 and ZbFAD3 could catalyze the conversion from oleic acid to linoleic acid, and the conversion from linoleic acid to α-linolenic acid, respectively. Therefore, both unsaturated fatty acid dehydrogenases function by introducing a second and third double bond to the fatty acid chain.
It was observed that the linoleic acid and oleic acid contents in the ZbFAD2-A. thaliana transgenic seeds were higher and lower than in the WT, respectively. However, the levels of α-linolenic acid and linoleic acid in the ZbFAD3-A. thaliana transgenic seeds were higher and lower than in the WT, respectively. Our results indicated that the recombinant ZbFAD2 and ZbFAD3 proteins functioned as desaturase enzymes and could catalyze unsaturated fatty acid dehydrogenation; which agreed with the prokaryotic expression analysis results, thereby confirming their roles in the unsaturated fatty acid biosynthesis pathway.
At present, both FAD2 and FAD3 genes have been successfully cloned in perilla, tobacco, Arabidopsis, wheat, flax, etc. The content of 18:3 in rice bran oil and roots increased significantly after the soybean FAD2 and FAD3 genes were transformed into rice, with the α-linolenic acid content in rice bran oil increasing significantly. These results show that overexpression of FAD2 and FAD3 genes can increase the α-linolenic acid content in plants. Therefore, we speculate that both ZbFAD2 and ZbFAD3 genes have an important role in the alkylamide biosynthesis pathway.
Conclusion
To sum up, the prokaryotic and eukaryotic expression vectors of ZbFAD2 and ZbFAD3 genes were constructed, and their functions were evaluated via substrate reactions. Furthermore, expressing them in A. thaliana and N. benthamiana could identify their functional roles in the alkylamide biosynthesis pathway. The difference in the levels of unsaturated fatty acids and hydroxy-α-sanshool indicated that the two target genes were involved in the alkylamide biosynthesis pathway, and fatty acid synthesis was correlated with alkylamide synthesis. Our transient assays on N. benthamiana leaves and stable transformation on A. thaliana demonstrated that the expression of ZbFAD2 and ZbFAD3 genes induced the expression of some key genes for the biosynthesis of hydroxy-α-sanshool. It was observed that Z. bungeanum possessed an updated pathway for hydroxyl-α-sanshool biosynthesis (Figure S4). Therefore, ZbFAD2 and ZbFAD3 are the crucial enzymes for regulating the alkylamide biosynthesis pathway in Z. bungeanum. These would lay the groundwork for further research of the sanshools in the plants. Nevertheless, future studies should investigate the relationship between the biosynthesis of fatty acids and alkylamide compounds in Z. bungeanum to gain further insights into the alkylamide biosynthesis pathway.
Data availability statement
The original contributions presented in the study are included in the article/Supplementary Materials. Further inquiries can be directed to the corresponding author.
Author contributions
JZ performed the statistical analysis and drafted the manuscript. ZW and NH participated in the statistical analysis and the writing of the manuscript. DW conceived the research, participated in the research design and coordination, and provided suggestions on the writing of the manuscript. All authors contributed to the article and approved the submitted version.
Funding
This work was supported by the National Natural Science Foundation of China (31872706), and the National Key Research and Development Program of China (2019YFD1000603).
Conflict of interest
The authors declare that the research was conducted in the absence of any commercial or financial relationships that could be construed as a potential conflict of interest.
Publisher’s note
All claims expressed in this article are solely those of the authors and do not necessarily represent those of their affiliated organizations, or those of the publisher, the editors and the reviewers. Any product that may be evaluated in this article, or claim that may be made by its manufacturer, is not guaranteed or endorsed by the publisher.
Supplementary material
The Supplementary Material for this article can be found online at: https://www.frontiersin.org/articles/10.3389/fpls.2022.991882/full#supplementary-material
References
Arondel, V., Lemieux, B., Hwang, I., Gibson, S., Goodman, H. M., Somerville, C. R. (1992). Map-based cloning of a gene controlling omega-3 fatty acid desaturation in arabidopsis. Science 258 (5086), 1353–1355. doi: 10.1126/science.1455229
Bhatt, V., Sharma, S., Kumar, N., Sharma, U., Singh, B. (2017). Simultaneous quantification and identification of flavonoids, lignans, coumarin and amides in leaves of zanthoxylum armatum using UPLC-DAD-ESI-QTOF–MS/MS. J. Pharm. Biomed. Anal. 132, 46–55. doi: 10.1016/j.jpba.2016.09.035
Bhunia, R. K., Kaur, R., Maiti, M. K. (2016). Metabolic engineering of fatty acid biosynthetic pathway in sesame (Sesamum indicum l.): assembling tools to develop nutritionally desirable sesame seed oil. Phytochem. Rev. 15 (5), 799–811.
Bryant, B. P., Mezine, I. (1999). Alkylamides that produce tingling paresthesia activate tactile and thermal trigeminal neurons. Brain Res. 842 (2), 452–460. doi: 10.1016/S0006-8993(99)01878-8
Busch, F. A., Tominaga, J., Muroya, M., Shirakami, N., Takahashi, S., Yamori, W., et al. (2020). Overexpression of BUNDLE SHEATH DEFECTIVE 2 improves the efficiency of photosynthesis and growth in arabidopsis. Plant J. 102 (1), 129–137. doi: 10.1111/tpj.14617
Caterina, M. J., Schumacher, M. A., Tominaga, M., Rosen, T. A., Levine, J. D., Julius, D. (1997). The capsaicin receptor: a heat-activated ion channel in the pain pathway. Nature 389 (6653), 816–824.
Chen, G., Qu, S., Wang, Q., Bian, F., Peng, Z., Zhang, Y., et al. (2014). Transgenic expression of delta-6 and delta-15 fatty acid desaturases enhances omega-3 polyunsaturated fatty acid accumulation in synechocysti ssp. PCC6803. Biotechnol. Biofuels 7 (1), 32–35. doi: 10.1186/1754-6834-7-32
Chen, X., Wang, W., Wang, C., Liu, Z., Sun, Q., Wang, D. (2019). Quality evaluation and chemometric discrimination of zanthoxylum bungeanum maxim leaves based on flavonoids profiles, bioactivity and HPLC-fingerprint in a common garden experiment. Industr. Crops Prod 134, 225–233. doi: 10.1016/j.indcrop.2019.04.017
Clough, S. J., Bent, A. F. (1998). Floral dip: a simplified method for agrobacterium mediated transformation of arabidopsis thaliana. Plant J. 16 (6), 735–743. doi: 10.1046/j.1365-313x.1998.00343.x
Cortez-Espinosa, N., Aviña-Verduzco, J. A., Ramírez-Chávez, E., Molina-Torres, J., Ríos-Chávez, P. (2011). Valine and phenylalanine as precursors in the biosynthesis of alkamides in acmella radicans. Nat. Prod. Commun. 6 (6), 857–861. doi: 10.1177/1934578X1100600625
Fan, R., Li, L., Cai, G., Ye, J., Liu, M., Wang, S., et al. (2019). Molecular cloning and function analysis of FAD2 gene in idesia polycarpa. Phytochemistry 168, 112114. doi: 10.1016/j.phytochem.2019.112114
Feng, S., Liu, Z., Cheng, J., Li, Z., Tian, L., Liu, M., et al. (2021). Zanthoxylum-specific whole genome duplication and recent activity of transposable elements in the highly repetitive paleotetraploid z. bungeanum genome. Horticult. Res. 8, 205. doi: 10.1038/s41438-021-00665-1
Gazave, E., Tassone, E. E., Baseggio, M., Cryder, M., Byriel, K., Oblath, E., et al. (2020). Genome-wide association study identifies acyl-lipid metabolism candidate genes involved in the genetic control of natural variation for seed fatty acid traits in brassica napus l. Ind. Crops Prod 145, 112080. doi: 10.1016/j.indcrop.2019.112080
Greger, H. (1984). Alkamides: Structural relationships, distribution and biological Activity1. Planta Med. 50 (05), 366–375. doi: 10.1055/s-2007-969741
Horiguchi, G., Fuse, T., Kawakami, N., Kodama, H., Iba, K. (2000). Temperature-dependent translational regulation of the ER ω-3 fatty acid desaturase gene in wheat root tips. Plant J. 24 (6), 805–813. doi: 10.1046/j.1365-313x.2000.00925.x
Huang, F. C., Studart-Witkowski, C., Schwab, W. (2010). Overexpression of hydroperoxide lyase gene in nicotiana benthamiana using a viral vector system. Plant Biotechnol. J. 8 (7), 783–795. doi: 10.1111/j.1467-7652.2010.00508.x
Jordt, S. E., Bautista, D. M., Chuang, H. H., McKemy, D. D., Zygmunt, P. M., Högestätt, E. D., et al. (2004). Mustard oils and cannabinoids excite sensory nerve fibres through the TRP channel ANKTM1. Nature 427 (6971), 260–265.
Kawai, K., Kawai, H., Tomoda, Y., Matsusaki, K., Hagura, Y. (2013). Production of high value added starchy food by pre-dehydration. Cryobiol. Cryotechnol. 59 (1), 71–74. doi: 10.20585/cryobolcryotechnol.59.1_71
Koo, J. Y., Jang, Y., Cho, H., Lee, C. H., Jang, K. H., Chang, Y. H., et al. (2007). Hydroxy-α-sanshool activates TRPV1 and TRPA1 in sensory neurons. Eur. J. Neurosci. 26 (5), 1139–1147. doi: 10.1111/j.1460-9568.2007.05743.x
Livak, K. J., Schmittgen, T. D. (2001). Analysis of relative gene expression data using real-time quantitative PCR and the 2–ΔΔCT method. Methods 25 (4), 402–408.
Los, D. A., Murata, N. (1998). Structure and expression of fatty acid desaturases. Biochim. Biophys. Acta Lipids Lipid Metab. 1394 (1), 3–15. doi: 10.1016/S0005-2760(98)00091-5
Luo, P., Chen, Y., Rong, K., Lu, Y., Wang, N., Xu, Z., et al. (2022). ZmSNAC13, a maize NAC transcription factor conferring enhanced resistance to multiple abiotic stresses in transgenic arabidopsis. Plant Physiol. Biochem. 170, 160–170. doi: 10.1016/j.plaphy.2021.11.032
Orlova, I. V., Serebriiskaya, T. S., Popov, V., Merkulova, N., Nosov, A. M., Trunova, T. I., et al. (2003). Transformation of tobacco with a gene for the thermophilic acyl-lipid desaturase enhances the chilling tolerance of plants. Plant Cell Physiol. 44 (4), 447–450. doi: 10.1093/pcp/pcg047
Rahman, S. M., Takagi, Y., Kumamaru, T. (1996). Low linolenate sources at the fan locus in soybean lines m-5 and IL-8. Breed Sci. 46, 155–158.
Ren, T., Zhu, Y., Kan, J. (2017). Zanthoxylum alkylamides activate phosphorylated AMPK and ameliorate glycolipid metabolism in the streptozotocin-induced diabetic rats. Clin. Exp. Hypertens. 39 (4), 330–338. doi: 10.1080/10641963.2016.1259332
Riera, C., Menozzi-Smarrito, C., Affolter, M., Michlig, S., Munari, C., Robert, F., et al. (2009). Compounds from sichuan and melegueta peppers activate, covalently and non-covalently, TRPA1 and TRPV1 channels. Brit. J. Pharmacol. 157 (8), 1398–1409. doi: 10.1111/j.1476-5381.2009.00307.x
Rong, R., Cui, M. Y., Zhang, Q. L., Zhang, M. Y., Yu, Y. M., Zhou, X. Y., et al. (2016). Anesthetic constituents of zanthoxylum bungeanum maxim.: A pharmacokinetic study. J. Separat. Sci. 39 (14), 2728–2735. doi: 10.1002/jssc.201600295
Shah, S., Xin, Z. (1997). Overexpression of the FAD3 desaturase gene in a mutant of arabidopsis. Plant Physiol. 114 (4), 1533–1539. doi: 10.1104/pp.114.4.1533
Shimada, T., Wakita, Y., Otani, M., Iba, K. (2000). Modification of fatty acid composition in rice plants by transformation with a tobacco microsomal ω-3 fatty acid desaturase gene (Nt FAD3). Plant Biotechnol. 17 (1), 43–48. doi: 10.5511/plantbiotechnology.17.43
Story, G. M., Peier, A. M., Reeve, A. J., Eid, S. R., Mosbacher, J., Hricik, T. R., et al. (2003). ANKTM1, a TRP-like channel expressed in nociceptive neurons, is activated by cold temperatures. Cell 112 (6), 819–829. doi: 10.1016/S0092-8674(03)00158-2
Stoutjesdijk, P., Hurlestone, C., Singh, S. P. (2000). High-oleic acid australian brassica napua and b. juncea varieties produced by co-suppression of endogenous deltal2-desaturases. Biochem. Soc Trans. 28 (6), 938–940. doi: 10.1042/BST0280938
Sugai, E., Morimitsu, Y., Iwasaki, Y., Morita, A., Watanabe, T., Kubota, K. (2005). Pungent qualities of sanshool-related compounds evaluated by a sensory test and activation of rat TRPV1. Biosci. Biotechnol. Biochem. 69 (10), 1951–1957. doi: 10.1271/bbb.69.1951
Sun, W., Liang, L., Meng, X., Li, Y., Gao, F., Liu, X., et al. (2016). Biochemical and molecular characterization of a flavonoid 3-o-glycosyltransferase responsible for anthocyanins and flavonols biosynthesis in freesia hybrida. Front. Plant Sci. 7, 410. doi: 10.3389/fpls.2016.00410
Sun, L., Yu, D., Wu, Z., Wang, C., Wei, A., Wang, D. (2019). Comparative transcriptome analysis and expression of genes reveal the biosynthesis and accumulation patterns of key flavonoids in different varieties of zanthoxylum bungeanum leaves. J. Agric. Food Chem. 67 (48), 13258–13268. doi: 10.1021/acs.jafc.9b05732
Tamura, K., Peterson, D., Peterson, N., Stecher, G., Nei, M., Kumar, S. (2011). MEGA5: molecular evolutionary genetics analysis using maximum likelihood, evolutionary distance, and maximum parsimony methods. Mol. Biol. Evol. 28 (10), 2731–2739. doi: 10.1093/molbev/msr121
Tsunozaki, M., Lennertz, R. C., Vilceanu, D., Katta, S., Stucky, C. L., Bautista, D. M. (2013). A ‘toothache tree’ alkylamide inhibits aδ mechanonociceptors to alleviate mechanical pain. J. Physiol. 591 (13), 3325–3340. doi: 10.1113/jphysiol.2013.252106
Vrinten, P., Hu, Z., Munchinsky, M. A., Rowland, G., Qiu, X. (2005). Two FAD3 desaturase genes control the level of linolenic acid in flax seed. Plant Physiol. 139 (1), 79–87. doi: 10.1104/pp.105.064451
Wu, Z., Wang, W., Sun, L., Wei, A., Wang, D. (2020). Accumulation and biosynthesis of hydroxyl-α-sanshool in varieties of zanthoxylum bungeanum maxim. by HPLC-fingerprint and transcriptome analyses. Ind. Crops Prod 145, 111998. doi: 10.1016/j.indcrop.2019.111998
Xue, Y., Yin, N., Chen, B., Liao, F., Win, A. N., Jiang, J., et al. (2017). Molecular cloning and expression analysis of two FAD2 genes from chia (Salvia hispanica). Acta Physiol. Plant 39 (4), 95. doi: 10.1007/s11738-017-2390-0
You, Y., Zhou, M., Lu, H., Shirima, G. G., Cheng, Y., Liu, X. (2015). Sanshool from zanthoxylum l. induces apoptosis in human hepatocarcinoma HepG2 cells. Food Sci. Biotechnol. 24 (6), 2169–2175. doi: 10.1007/s10068-015-0289-3
Zhang, L. L., Zhao, L., Wang, H. Y., Shi, B. L., Liu, L. Y., Chen, Z. X. (2019). The relationship between alkylamide compound content and pungency intensity of zanthoxylum bungeanum based on sensory evaluation and ultra-performance liquid chromatography-mass spectrometry/mass spectrometry (UPLC-MS/MS) analysis. J. Sci. Food Agric. 99 (4), 1475–1483. doi: 10.1002/jsfa.9319
Keywords: zanthoxylum bungeanum maxim, alkylamides, biosynthesis, ZbFAD2 and ZbFAD3, functional validation, fatty acid dehydrogenase
Citation: Zhang J, Wu Z, Han N and Wang D (2022) Functional validation of ZbFAD2 and ZbFAD3 in the alkylamide biosynthesis pathway from Zanthoxylum bungeanum Maxim. Front. Plant Sci. 13:991882. doi: 10.3389/fpls.2022.991882
Received: 12 July 2022; Accepted: 06 September 2022;
Published: 23 September 2022.
Edited by:
Yunpeng Cao, Chinese Academy of Sciences (CAS), ChinaReviewed by:
Chaobo Tong, Key Laboratory of Biology and Genetics Improvement of Oil Crops, Oil Crops Research Institute of Chinese Academy of Agricultural Sciences, Ministry of Agriculture and Rural Affairs, ChinaDi-an Ni, Shanghai Institute of Technology, China
Noelle Anglin, International Potato Center, Peru
Copyright © 2022 Zhang, Wu, Han and Wang. This is an open-access article distributed under the terms of the Creative Commons Attribution License (CC BY). The use, distribution or reproduction in other forums is permitted, provided the original author(s) and the copyright owner(s) are credited and that the original publication in this journal is cited, in accordance with accepted academic practice. No use, distribution or reproduction is permitted which does not comply with these terms.
*Correspondence: Dongmei Wang, ZG13bGlAMTYzLmNvbQ==