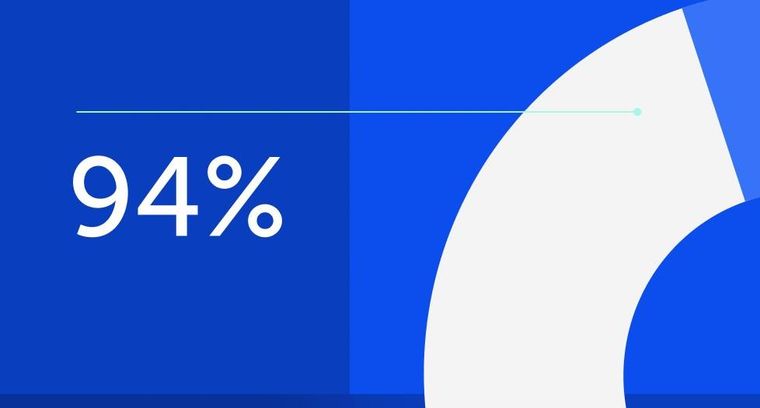
94% of researchers rate our articles as excellent or good
Learn more about the work of our research integrity team to safeguard the quality of each article we publish.
Find out more
ORIGINAL RESEARCH article
Front. Plant Sci., 30 November 2022
Sec. Plant Metabolism and Chemodiversity
Volume 13 - 2022 | https://doi.org/10.3389/fpls.2022.989755
This article is part of the Research TopicPhysiology, biochemistry and molecular biology of fruits, seeds and other sinksView all 5 articles
Ultra-performance liquid chromatography-tandem mass spectrometry (UPLC-MS) and gas chromatography-mass spectrometry (GC-MS) were applied to analyze metabolites in perilla leaves (PLs) during its developmental process. In total, 118 metabolites were identified, including volatile and non-volatile compounds, such as terpenoids, sugars, amino acids, organic acids, fatty acids, phenolic acids, flavonoids, and others. Principal component analysis (PCA) indicated great variations of metabolites during PLs development. Clustering analysis (CA) clarified the dynamic patterns of the metabolites. The heatmap of CA showed that most of the detected metabolites were significantly accumulated at stage 4 which is the pre anthesis period, and declined afterwards. The results of the present study provide a comprehensive overview of the metabolic dynamics of developing PLs which suggested that pre anthesis period is the best harvest time for PLs.
Perilla frutescens (L.) Britt. is an annual herbal plant that belongs to the family of Lamiaceae. It is widely cultivated in Asia counties, such as China, Japan, Korea, Vietnam and other regions (Yu et al., 2017; Zhang et al., 2021). Perilla leaves (PLs) are commonly consumed as kitchen herb in salads, sushi, soups, and as spice, garnish, or food colorant. PLs are also used as traditional Chinese medicine to relieve exterior, dispersing cold, ease stomach pain, reduce phlegm and relieve cough and asthma (Ha et al., 2012; Igarashi and Miyazaki, 2013). Phytochemical studies indicated PLs were rich in essential oils, flavonoids, fatty acids, phenolic compounds, etc (Ahmed, 2018). Compounds of PLs showed various biological activities such as antioxidant, antimicrobial, anti-allergic, antidepressant, anti-inflammatory, and anticancer effects (Banno et al., 2004; Ghimire et al., 2019; Wang et al., 2021; Yang et al., 2021). PLs has been used as a natural herbal medicine for treatment of depression-related disease, asthma, tumors, coughs, allergies, intoxication, fever, chills, headache, stuffy nose, and some intestinal disorders (Ito et al., 2011; Kim et al., 2012; Zhou et al., 2021). Owing to these health benefits, the food and pharmaceutical industries are increasingly interested in PLs.
The pharmacological activities of perilla are closely related to its chemical constituents. Some studies have revealed that great dynamic variation in the nutritional components and phytochemical substances might occur during plant development. Ghimire et al. (Ghimire et al., 2017) compared the total volatile contents of eighteen accessions of PLs and most of them were higher before the flowering time than at the flowering stage. Luo et al. (Luo et al., 2021) invested variation of two phenolic acids and six flavonoids during PLs development and suggested to harvest PLs at different times basing on the targeted metabolites. Peiretti et al. (Peiretti, 2011) evaluated perilla quality according to the content of fatty acid, fiber, crude protein, organic matter and gross energy during the growth cycle of perilla. According to their result, it is better to harvest perilla at around two months after sowing. Though these studies provided a general feature of perilla nutritional contents, a more comprehensive and detailed dynamic profile of developing PLs is still essential for providing more information to determine the harvest time according to different application.
In this study, mass spectrometry (MS) based high throughput metabolomic platforms were applied to ascertain the dynamic trajectory of complex ingredients of PLs during developmental process. In addition, multiple statistical analysis methods, including principal component analysis (PCA) and Clustering analysis (CA) were used to clarify the dynamic patterns of the detected metabolites. These data provide data support for determining the best harvest time of perilla leaves.
HPLC grade methanol (MeOH), acetonitrile (ACN) and formic acid were purchased from Fisher Scientific (Pittsburgh, PA, United States) Ultrapure water was prepared by Synergy water purification system (Millipore, Billerica, United States). The reserpine standards (HPLC grade) and GC grade derivatizing regent MSTFA (N-methyl-N-(trimethylsilyl)trifluoroacetamide), methoxyamine hydrochloride were purchased from Sigma-Aldrich (St. Louis, MO, USA). Chemical reagent n-hexane (GC grade) and Anhydrous pyridine (GC grade) were obtained from Shanghai Aladdin Biochemical Technology Co., Ltd. (Shanghai, China). Salicylic acid, luteolin, apigenin and rosmarinic acid standards were provided by Shanghai Yuanye Bio-Technology Co., Ltd. (Shanghai, China). Reference standards of luteolin-7-O-glucoside, scutellarin, luteolin-7-O-glucuronide, apigenin-7-glucoside and apigenin-7-O-glucuronide were purchased from Shanghai Standard Technology Co., Ltd. (Shanghai, China). The purities of all standards were determined to be higher than 98%. Other chemicals and reagents were analytical grade.
The PLs were randomly collected from Perilla frutescens (L.) Britt. cultivated in the plant base of Hebei Academy of Agriculture and Forestry Sciences in Shijiazhuang (China 38°06′41.7′′ N, 114°45′35.8′′E) in mid May 2019, and the samples were collected semimonthly from July 2019 to October 2019. The mean annual temperature was 14.4°C, mean annual humidity was 62%, mean annual precipitation was 422.6 mm, mean annual sunshine hours was 2235.4 hours. Growth process of perilla was performed using manual fertilization, therefore, soil is rich in organic elements. Three biological replicates were collected for each developmental phase (Table 1). The plant was identified by professor Yuguang Zheng (Hebei Chemical and Pharmaceutical College, China), and voucher specimens were deposited in Traditional Chinese Medicine Processing Technology Innovation Center of Hebei Province, Hebei University of Chinese Medicine. The harvested leaves were air-dried in the dark at room temperature for 2 weeks to acquire consistently low water content.
The dried PF samples were pulverized with grinder (FW100, Taisite, Tianjin, China), and screened through 60 mesh sieves. 100 mg of each accurately weighted pulverized sample were thoroughly mixed with 1 mL of n-hexane then sonicated (300 W, 40 kHz) 15 min at room temperature. The extracted solution was centrifuged at 13000 rpm at room temperature for 10 min. The supernatant was injected into the GC-MS for analysis.
The GC-MS analysis was performed with an Agilent 7890B-5977B GC-MS (Agilent, Santa Clara, CA, USA) coupled with a HP-5MS capillary column (30 m × 0.25 mm, 0.25 μm film thickness, Agilent, Santa Clara, CA, USA). Helium (≥ 99.999%) was used as carrier gas at a constant flow rate of 1.0 mL·min-1. 1 μL of the prepared supernatant solution was injected in split-mode with the split ratio set to 2:1 at a temperature of 250°C. The oven temperature program was initially set at 45°C, then increased to 100°C at a rate of 10°C·min-1, and subsequently increased to 280°C at a rate of 4°C·min-1, finally held for 10 min. The electronic ionization voltage of electron-impact (EI) ion source was 70 eV. The mass spectrometer was operated in full scan mode with a scanning range of 50-550 m/z. n-Alkane standard solution (C8-C20, 40 mg·L-1, Sigma-Aldrich, Switzerland) was analyzed under the same condition for retention index (RI) calculation.
An integrative extraction of primary metabolites and secondary metabolites was performed according to a universal extraction protocol (Weckwerth et al., 2010; Mari et al., 2013; Wang et al., 2017) with some modifications. 100 mg of each pulverized samples were extracted with 1 mL of extraction solution (methanol: water: formic acid = 70:28:2) by sonication 15 min. The crude extract was centrifuged at 13,000 rpm at room temperature for 10 min. 50 μL of the supernatant together with 20 μL of salicylic acid (1 mg·mL-1, internal standard) was dried using a SpeedVac (Thermo Scientific, Inc., Bremen, Germany) at 5000 rpm and 40°C for 90 min. Methoxyamination of the carbonyl groups was performed by adding 20 μL of methoxyamine hydrochloride (40 mg·mL-1) in pyridine to each sample followed by incubation in metal bath at 30°C for 90 min. Subsequently, 80 μL of MSTFA (N-Methyl-N-(trimethylsilyl) trifluoroacetamide) was added and the mixtures were incubated at 37°C for 30 min. The derivatized samples were centrifuged at 13,000 rpm at room temperature for 10 min with the supernatants prepared for GC-MS analysis.
Aforementioned GC-MS instrument and column (see 2.3.2) was also applied for analysis of derivatized samples. 1 μL of the derivatized sample was injected using 5:1 split-mode at a temperature of 250°C. The temperature gradient program was as follows: Initial temperature was 80°C, increased to 200°C at a rate of 10°C·min-1; then increased to 250°C at a rate of 6°C·min-1; subsequently increased to 310°C at a rate of 6°C·min-1 and hold at 310°C for 5 min. EI ion source was adjusted to 230°C with electronic energy of 70 eV. The mass spectrometer was determined by the full-scan method ranging from 50 to 550 (m/z). n-Alkane standard solution (C8-C20, 40 mg·L-1, Sigma-Aldrich, Switzerland) was analyzed under the same condition for retention index (RI) calculation.
100 μL of the above mentioned crude extract (see in 2.4.1) was mixed with 100 μL of 5 μg·mL-1 reserpine (internal standard) and diluted with 800 μL of extract solution then centrifuged at 13,000 rpm at room temperature for 10 min with the supernatants prepared for the LC-MS analysis.
The UHPLC-Q/TOF-MS analysis was performed on an Agilent 1290 UHPLC system coupled with an Agilent 6545 quadrupole time-of-flight mass spectrometer system (Agilent, Santa Clara, CA, United States). Chromatographic separation was performed on an Agilent ZORBAX SB C18 column (4.6 × 50 mm, 1.8 μm).
UHPLC chromatographic conditions: the 0.5 μL of prepared samples were loaded on an Agilent 1290 UHPLC system and eluted with 0.1% formic-water (mobile phase A) and acetonitrile (mobile phase B) in the following gradient: 0-2 min, 12% B; 2-26 min, 12%-24% B; 26-35 min, 24%-50% B; 35-38 min, 50%-100% B; 38-45 min, 100% B. The flow rate was maintained at 0.4 mL·min-1, the column temperature was set at 25°C.
The MS acquisition parameters were referred to Chang et al. (2021) with minor modifications. The capillary voltage was set to 4000 V; and the collision energy was 20 eV and 35 eV. The analysis was operated in positive mode with the mass range of m/z 50-1000 Da.
For qualitative analysis, the metabolites detected by GC-MS with a similarity more than 80% to the NIST17 standard library were identified using the Agilent MassHunter analysis program (Agilent, Santa Clara, CA, USA). The RI of all the identified compounds were calculated by comparing their corresponding peak retention time to that of n-alkanes (C8–C20) (Chaturvedula and Prakash, 2013; Ma et al., 2014). The identification of detected metabolites in the LC-MS analysis was based on their accurate precursor masses and fragment masses. For quantitative analysis, the integrated peak area was considered to be a variable for analysis and normalized to internal standard. The combined GC-MS and LC-MS dataset was transformed to -1~1 by Min-Max Normalization method. SIMCA P13 software (Umetrics, Umea, Sweden) was used for principal component analysis (PCA). Cluster analysis (CA) and heatmap was performed with Origin Pro 2020 (OriginLab Corporation, USA) software. Duncan’s test was performed with IBM SPSS Statistics 23.0 (IBM, USA) software.
The typical total ion chromatograms (TICs) of GC-MS, pre-column derivatized GC-MS and LC-MS showed metabolomic profiles of PLs (Figures 1A-C). With reference to the NIST17 database, 47 volatile metabolites including aldehydes, ketones, alcohols, fatty acids, steroids and others (Table 2) were identified according to their retention times and mass spectrums. 51 peaks in Figure 1B were identified including sugars, amino acids, organic acids, fatty acids, and phenolic compounds (Table 3). The identification of non-volatile metabolites form LC-MS data were based on their precursor ions and fragmentation patterns. 28 metabolites, mainly flavonoids and anthocyanidins, were identified with their detail information such as retention time, chemical formula, ppm errors and fragment ions were listed in Table 4. Among the putatively identified compounds, eight metabolites (luteoloside (peak C11), scutellarin (peak C16), luteolin-7-O-glucuronide (peak C17), apigenin-7-O-glucoside (peak C18) apigenin-7-O-glucuronide (peak C23), rosmarinic acid (peak C24), luteolin (peak C26), apigenin (peak C27)) were confirmed with reference substances (Figure 1D). The chemical fingerprints showed distinct differences in the chemical composition of PLs at different harvesting (Figure 2).
Figure 1 The typical total ion chromatograms of PLs by GC-MS and LC-MS. (A) TIC of volatile metabolites in pooled samples by GC-MS; (B) TIC of non-volatile metabolites in pooled samples by GC-MS after derivatization; (C) TIC of non-volatile metabolites in pooled samples by LC-MS; (D) TIC of reference substances by LC-MS.
Table 3 Identification of non-volatile metabolites analyzed by pre-column derivatization combining with GC-MS.
Figure 2 The chemical fingerprints of PLs at different harvesting by GC-MS and LC-MS. (A) Fingerprints of volatile metabolites by GC-MS; (B) Fingerprints of non-volatile metabolites by GC-MS after derivatization; (C) Fingerprints of non-volatile metabolites by LC-MS.
PCA was carried out for an overview of the dataset. In the PCA plot, three biological replicates of each stage were compactly gathered together (Figure 3) while samples at different harvest time were clearly separated indicating metabolomic changes during PLs development. PC1 and PC2 explained 77.7% of the total variance. Samples collected at harvest time 4 were completely separated with samples harvested at other periods on PC1 indicating a special and significant meaning of this harvest period. The loading values of all the metabolites are listed in Table 5.
Figure 3 The principal component analysis (PCA) score plots of of PLs samples at different harvesting times.
Table 5 The PCA loading values and Duncan’s test result of metabolites identified in developing PLs.
To observe the dynamic changes of metabolites in different harvest periods in a more intuitive manner, a heatmap of the 118 different metabolites was obtained (Figure 4A).
Figure 4 Metabolome dynamics of developing PLs. (A) Overview of the metabolite dynamics with clustering heat map. (B–G) Present the dynamics of volatile oils, sugars, phytosterols and fatty acids, amino acids, phenolic acids and organic acids, derivatives, flavonoids and anthocyanins.
Volatile oil is a very important and widely studied class of metabolites in perilla. They showed bioactivities such as antibacterial, antiviral, anti-inflammatory, anticarcinogenic, antioxidant, etc (Raut and Karuppayil, 2014). In most flowering plants, the production and emission of volatile metabolites are developmentally regulated and show similar developmental characteristics. Normally, volatile oil accumulates in the early developmental stage when fruits are not mature or before the flowers are ready for pollination. Then a release of volatile components to attract pollinators might cause a decrease of volatile compounds in the early stage of flowering (Dudareva et al., 2000; Dudareva et al., 2013). In the present study, most of volatile oil compounds showed highest level at stage 4 which was pre anthesis period (Figure 4B). Only heptacosane and γ-elemene showed the highest level at stage 6 which was a stage before fruiting period (Figure 4B). The dynamic patterns of volatile compounds indicated their crucial function in plant pollination and reproduction.
During photosynthesis, all kinds of carbon is fixed in the forms of sugars and sugar derivatives (Smeekens and Hellmann, 2014; Sakr et al., 2018) Sugars help plants store energy and play essential roles in signalling pathways of plant growth and development. In this study, the main sugars in PLs are D-fructose, D-glucose and sucrose. They accumulated constantly during the developmental process of PLs and were with highest levels in fruiting phase (Figure 4C). Most of the sugars and sugar derivatives showed similar dynamic patterns as them (Figure 4C). Only five sugar derivatives (xylitol, D-glucitol, myo-inositol, galactinol and galacturonic acid) changed differently, with higher content at early developmental stage and decreased throughout the development process (Figure 4C). Accumulation of sugar content during plant development was also observed in Cichorium spinosum (Petropoulos et al., 2018).
The sterol composition of plants is complex and diverse. The main membrane sterols in higher plants are β-sitosterol, stigmasterol and campesterol (Ruan, 2014). Sterols are not only signal and regulatory molecules involved in plant growth and development, but also play key roles in cell proliferation and differentiation (Guo et al., 1995; Moreau et al., 2018). In this study, all phytosterols were showed the highest level at stage 2, and decreased gradually (γ-sitosterol, β-amyrone, α-amyrin, stigmasterol, campesterol) (Figure 4D). This trend may be due to the vigorous metabolism of cells in the nutritional stage.
Fatty acids and lipids provide structural integrity and energy for various metabolic processes (Lim et al., 2017). The predominant fatty acids detected in PLs were palmitic acid, oleic acid and α-linolenic acid which increased pre anthesis period and declined afterwards (Figure 4D). Oleic acid and α-linolenic acid are essential unsaturated fatty acids (UFAs) and recommended for consumption for their multiple health benefits, such as anti-obesity (Fan et al., 2020), cardioprotection (Russell et al., 2020), anti-diabetes (Canetti et al., 2014), anti-inflammation (Wang et al., 2020), anti-cancer (Schiessel et al., 2015), neuroprotection (Kumari et al., 2019) and so on. Intake of α-linolenic acid rich P. frutescens leaf powder in Japanese adults showed some cardiovascular protective effects (Hashimoto et al., 2020). Considering the health benefits of these unsaturated fatty acids, stage 4, the pre anthesis period would be suitable harvest time for ensuring high content of these UFAs in perilla leaves.
Amino acids are not only important components for plants to complete their life cycle activities (Paulusma et al., 2022), but also essential nutrients for humans and other animals. PLs are rich in amino acids. Amino acids in PLs showed two distinct dynamic patterns during PLs development. Some amino acids were with higher content at early stages and decreased throughout the developmental process, such as L-serine, L-lysine, L-phenylalanine, L-tyrosine, L-glycine (Figure 4E). Other amino acids were showed the highest level at stage 4, and decreased afterwards, such as L-aspartic acid, L-isoleucine, L-threonine, L-leucine, L-glutamine, L-proline, L-valine, L-alanine, etc (Figure 4E). Free amino acids could elicit complex gustatory sensation (Kawai et al., 2012), especially the taste of umami. They can bring fresh and brisk tastes to PLs and participate in the formation of aroma substances (Lee et al., 2019). With the maturity and senescence of leaves, there may be two reasons for the decrease of amino acids. First, amino acids might be involved in the synthesis of storage proteins. Second, the complete oxidation of amino acids produces the energy required to meet the special needs of certain organs, such as stressed leaves or roots. The molecular mechanism of regulation of amino acid catabolism in plants is complex and unclear so far (Hildebrandt et al., 2015). Considering the nutritional value and gustatory sensation of amino acids, it would be appropriate to harvest perilla leaves before the pre anthesis period.
Phenolic acids have various pharmacological activities, such as anti-inflammatory, anti-anxiety, and anti-depressive activities (Tinikul et al., 2018; Deguchi and Ito, 2020). Some of them are connected to the polymer of the cell wall through covalent bonds, which is crucial to the process of plant immune mechanism (Stuper-Szablewska and Perkowski, 2019). The predominant phenolic acids detected in PLs were rosmarinic acid and caffeic acid, which showed highest level at stage 4 (Figure 4F).
Organic acids are the intermediate products of cell metabolic tricarboxylic acid (TCA) cycle (Xiao and Wu, 2014). Many environmental stresses stimulate the biosynthesis and release of organic acids. For example, plants secrete organic acids in root exudates to mobilize phosphorus in deficient soil (Panchal et al., 2021). The main organic acids in PLs are lactic acid, malic acid, tartaric acid and citric acid. They also increased at early stages, showed highest level at around pre anthesis period and decreased afterwards (Figure 4F). Organic acids contribute to the sourness and fruity taste of plants, while inhibit the bitterness taste (Wang et al., 2021). Therefore, considering the high content of these compounds in PLs at the stage 4, alternative uses for food or pharmaceutical can be proposed.
Flavonoids play an important role in plant development and defense, have the ability to scavenge reactive oxygen species (ROS) and protect plants against damage from biotic and abiotic stresses (Iwashina, 2003; Pourcel et al., 2007). During perilla leaves development, the detected flavonoids presented an unanimous changing pattern. All the flavonoids accumulated pre anthesis period and showed the highest level at stage 4 (Figure 4G). Previous studies reported that flavonoids have many biological functions such as anti-inflammatory, anti-oxidative, anti-diabetic, and anti-hypertensive activities (Kawser Hossain et al., 2016; Jiang et al., 2020).
The color of fruits and flowers is crucial in plant ecology, can attract pollinators and seed-dispersal organisms (Grotewold, 2006). The molecular signals that induce pigment biosynthesis during pollination are unclear, but light plays a central role (Farzad et al., 2002). Natural pigments from PLs have exhibited a wide range of bioactive properties including antioxidant effects, anti-inflammatory effects, etc (Chang et al., 2005; Wang and Stoner, 2008; Lila et al., 2016). Natural pigments detected in PLs including shisonin and its derivatives. They showed the highest level at stage 5 (vigorous flowering period) (Figure 4G). According to this result, if the targeted metabolites are these pigments, it is better to harvest PLs during flowering period.
In this study, our results showed the advantages of applying an integrated LC-MS and GC-MS metabolomic platforms the evaluation of optimal harvesting period for plants. We employed metabolomic analysis to clarified the evolutionary trajectories and dynamic changes of volatile oil compounds, sugars, flavonoids, amino acids, organic acids, etc. The results of this study provide a theoretical basis for the development of PLs and offer data support for the optimal harvesting period of PLs. Considering the content of most of the nutrients and bioactive components, pre anthesis period is a suitable harvest time for PLs.
The original contributions presented in the study are included in the article/supplementary material. Further inquiries can be directed to the corresponding author.
LW and YZ conceived and designed the experiments. JC performed the experiments. JC, GY, and AY analyzed the data. JC wrote the manuscript. LW, YZ and LG revised and edited the manuscript. All authors have read and agreed to the published version of the manuscript.
This research was funded by Natural Science Foundation of Hebei Province (C2020423047); Research Foundation of Hebei Provincial Administration of Traditional Chinese Medicine (2019083); The Innovation Team of Hebei Province Modern Agricultural Industry Technology System (HBCT2018060205).
We would like to thank Prof. Chunxiu Wen and her team for providing us the plant materials. We would like to thank the gardeners for their great maintenance of the perilla. We would like to thank all the members in Traditional Chinese Medicine Processing Technology Innovation Center of Hebei Province for fruitful discussions.
The authors declare that the research was conducted in the absence of any commercial or financial relationships that could be construed as a potential conflict of interest.
All claims expressed in this article are solely those of the authors and do not necessarily represent those of their affiliated organizations, or those of the publisher, the editors and the reviewers. Any product that may be evaluated in this article, or claim that may be made by its manufacturer, is not guaranteed or endorsed by the publisher.
Ahmed, H. M. (2018). Ethnomedicinal, phytochemical and pharmacological investigations of perilla frutescens (L.) britt. Mol. (Basel. Switzerland). 24 (1), 102. doi: 10.3390/molecules24010102
Banno, N., Akihisa, T., Tokuda, H., Yasukawa, K., Higashihara, H., Ukiya, M., et al. (2004). Triterpene acids from the leaves of perilla frutescens and their anti-inflammatory and antitumor-promoting effects. Biosci. Biotechnol. Biochem. 68 (1), 85–90. doi: 10.1271/bbb.68.85
Canetti, L., Werner, H., Leikin-Frenkel, A. (2014). Linoleic and alpha linolenic acids ameliorate streptozotocin-induced diabetes in mice. Arch. Physiol. Biochem. 120 (1), 34–39. doi: 10.3109/13813455.2013.868002
Chang, Y. C., Huang, H. P., Hsu, J. D., Yang, S. F., Wang, C. J. (2005). Hibiscus anthocyanins rich extract-induced apoptotic cell death in human promyelocytic leukemia cells. Toxicol. Appl. Pharmacol. 205 (3), 201–212. doi: 10.1016/j.taap.2004.10.014
Chang, Y., Zhang, D., Yang, G., Zheng, Y., Guo, L. (2021). Screening of anti-lipase components of artemisia argyi leaves based on spectrum-effect relationships and HPLC-MS/MS. Front. Pharmacol. 12. doi: 10.3389/fphar.2021.675396
Chaturvedula, V., Prakash, I. (2013). Isolation and structure elucidation of two triterpene acids from the leaves of perilla frutescens. J. Pharmacogn. Phytochem. 1 (6), 49–53. doi: 10.3969/j.issn.2095-0837.1998.04.002
Deguchi, Y., Ito, M. (2020). Caffeic acid and rosmarinic acid contents in genus perilla. J. Natural Medicines 74 (4), 834–839. doi: 10.1007/s11418-020-01418-5
Dudareva, N., Klempien, A., Muhlemann, J. K., Kaplan, I. (2013). Biosynthesis, function and metabolic engineering of plant volatile organic compounds. New Phytol. 198 (1), 16–32. doi: 10.1111/nph.12145
Dudareva, N., Murfitt, L. M., Mann, C. J., Gorenstein, N., Kolosova, N., Kish, C. M., et al. (2000). Developmental regulation of methyl benzoate biosynthesis and emission in snapdragon flowers. Plant Cell 12 (6), 949–961. doi: 10.1105/tpc.12.6.949
Fan, R., Kim, J., You, M., Giraud, D., Toney, A. M., Shin, S. H., et al. (2020). α-linolenic acid-enriched butter attenuated high fat diet-induced insulin resistance and inflammation by promoting bioconversion of n-3 PUFA and subsequent oxylipin formation. J. Nutr. Biochem. 76, 108285. doi: 10.1016/j.jnutbio.2019.108285
Farzad, M., Griesbach, R., Weiss, M. R. (2002). Floral color change in viola cornuta l. (violaceae): a model system to study regulation of anthocyanin production. Plant Sci. 162 (2), 225–231. doi: 10.1016/S0168-9452(01)00557-X
Ghimire, B. K., Ji, H. Y., Chang, Y. Y., Kim, S. H., Chung, I. M. (2019). Profiling volatile and phenolic compound composition and characterization of the morphological and biological activities of perilla frutescence britton var. Jpn. Accessions. Acta Physiol. Plant. 41, 108. doi: 10.1007/s11738-019-2890-1
Ghimire, B. K., Yoo, J. H., Yu, C. Y., Chung, I. M. (2017). GC-MS analysis of volatile compounds of perilla frutescens britton var. japonica accessions: Morphological and seasonal variability. Asian Pac. J. Trop. Med. 10 (7), 643–651. doi: 10.1016/j.apjtm.2017.07.004
Grotewold, E. (2006). The genetics and biochemistry of floral pigments. Annu. Rev. Plant Biol. 57, 761–780. doi: 10.1146/annurev.arplant.57.032905.105248
Guan, Z., Li, S., Lin, Z., Yang, R., Zhao, Y., Liu, J., et al. (2014). Identification and quantitation of phenolic compounds from the seed and pomace of perilla frutescens using HPLC/PDA and HPLC-ESI/QTOF/MS/MS. Phytochem. Anal. 25 (6), 508–513. doi: 10.1002/pca.2521
Guo, D. A., Venkatramesh, M., Nes, W. D. (1995). Developmental regulation of sterol biosynthesis in zea mays. Lipids 30 (3), 203–219. doi: 10.1007/BF02537823
Ha, T. J., Lee, J. H., Lee, M. H., Lee, B. W., Kwon, H. S., Park, C. H., et al. (2012). Isolation and identification of phenolic compounds from the seeds of perilla frutescens (L.) and their inhibitory activities against α-glucosidase and aldose reductase. Food Chem. 135 (3), 1397–1403. doi: 10.1016/j.foodchem.2012.05.104
Hashimoto, M., Tanabe, Y., Hossain, S., Matsuzaki, K., Ohno, M., Kato, S., et al. (2020). Intake of alpha-linolenic acid-rich perilla frutescens leaf powder decreases home blood pressure and serum oxidized low-density lipoprotein in Japanese adults. Mol. (Basel. Switzerland). 25 (9), 2099. doi: 10.3390/molecules25092099
He, Y. K., Yao, Y. Y., Chang, Y. N. (2015). Characterization of anthocyanins in perilla frutescens var. acuta extract by advanced UPLC-ESI-IT-TOF-MSn method and their anticancer bioactivity. Mol. (Basel. Switzerland). 20 (5), 9155–9169. doi: 10.3390/molecules20059155
Hildebrandt, T. M., Nunes Nesi, A., Araújo, W. L., Braun, H. P. (2015). Amino acid catabolism in plants. Mol. Plant 8 (11), 1563–1579. doi: 10.1016/j.molp.2015.09.005
Igarashi, M., Miyazaki, Y. (2013). A review on bioactivities of perilla: progress in research on the functions of perilla as medicine and food. Evidence-Based Complement. Altern. Med. 2013, 925342. doi: 10.1155/2013/925342
Ito, N., Nagai, T., Oikawa, T., Yamada, H., Hanawa, T. (2011). Antidepressant-like effect of l-perillaldehyde in stress-induced depression-like model mice through regulation of the olfactory nervous system. Evidence-Based Complement. Altern. Med. 2011, 512697. doi: 10.1093/ecam/nen045
Iwashina, T. (2003). Flavonoid function and activity to plants and other organisms. Uchu. Seibutsu. Kagaku 17 (1), 24–44. doi: 10.2187/bss.17.24
Jiang, T., Guo, K., Liu, L., Tian, W., Xie, X., Wen, S., et al. (2020). Integrated transcriptomic and metabolomic data reveal the flavonoid biosynthesis metabolic pathway in perilla frutescens (L.) leaves. Sci. Rep. 10 (1), 16207. doi: 10.1038/s41598-020-73274-y
Kaufmann, C. M., Grassmann, J., Letzel, T. (2016). HPLC method development for the online-coupling of chromatographic perilla frutescens extract separation with xanthine oxidase enzymatic assay. J. Pharm. Biomed. Anal. 124, 347–357. doi: 10.1016/j.jpba.2016.03.011
Kawai, M., Sekine-Hayakawa, Y., Okiyama, A., Ninomiya, Y. (2012). Gustatory sensation of (L)- and (D)-amino acids in humans. Amino Acids 43 (6), 2349–2358. doi: 10.1007/s00726-012-1315-x
Kawser Hossain, M., Abdal Dayem, A., Han, J., Yin, Y., Kim, K., Kumar Saha, S., et al. (2016). Molecular mechanisms of the anti-obesity and anti-diabetic properties of flavonoids. Int. J. Mol. Sci. 17 (4), 569. doi: 10.3390/ijms17040569
Kim, J. K., Park, S. Y., Na, J. K., Seong, E. S., Yu, C. Y. (2012). Metabolite profiling based on lipophilic compounds for quality assessment of perilla (Perilla frutescens) cultivars. J. Agric. Food Chem. 60 (9), 2257–2263. doi: 10.1021/jf204977x
Kumari, S., Mazumder, A. G., Bhardwaj, A., Singh, D. (2019). Early α-linolenic acid exposure to embryo reduces pentylenetetrazol-induced seizures in zebrafish larva. Prostaglandins. Leukotrienes. Essential. Fatty Acids 143, 15–20. doi: 10.1016/j.plefa.2019.02.002
Lee, J., Kim, D. S., Cho, J., Hong, S. J., Pan, J. H., Kim, J. K., et al. (2019). Perilla frutescens britton: A comprehensive study on flavor/taste and chemical properties during the roasting process. Mol. (Basel. Switzerland). 24 (7), 1374. doi: 10.3390/molecules24071374
Lee, J. H., Park, K. H., Lee, M. H., Kim, H. T., Seo, W. D., Kim, J. Y., et al. (2013). Identification, characterization, and quantification of phenolic compounds in the antioxidant activity-containing fraction from the seeds of Korean perilla (Perilla frutescens) cultivars. Food Chem. 136 (2), 843–852. doi: 10.1016/j.foodchem.2012.08.057
Lila, M. A., Burton-Freeman, B., Grace, M., Kalt, W. (2016). Unraveling anthocyanin bioavailability for human health. Annu. Rev. Food Sci. Technol. 7, 375–393. doi: 10.1146/annurev-food-041715-033346
Lim, G. H., Singhal, R., Kachroo, A., Kachroo, P. (2017). Fatty acid- and lipid-mediated signaling in plant defense. Annu. Rev. Phytopathol. 55, 505–536. doi: 10.1146/annurev-phyto-080516-035406
Luo, Y. J., Guo, J. Q., Li, W. P., Yao, Y., Wen, C. M., Guo, B. L. (2021). Contents determination of eight phenolic compounds in perilla frutescens leaves of different cultivation years and harvesting periods. Zhongguo. Zhong. Yao. Za. Zhi. 46 (3), 567–574. doi: 10.19540/j.cnki.cjcmm.20201114.101
Ma, X. Q., Leung, A. K., Chan, C. L., Su, T., Li, W. D., Li, S. M., et al. (2014). UHPLC UHD q-TOF MS/MS analysis of the impact of sulfur fumigation on the chemical profile of codonopsis radix (Dangshen). Analyst. 139 (2), 505–516. doi: 10.1039/c3an01561k
Mari, A., Lyon, D., Fragner, L., Montoro, P., Piacente, S., Wienkoop, S., et al. (2013). Phytochemical composition of potentilla anserina l. analyzed by an integrative GC-MS and LC-MS metabolomics platform. Metabolomics 9 (3), 599–607. doi: 10.1007/s11306-012-0473-x
Meng, L., Lozano, Y. F., Gaydou, E. M., Li, B. (2008). Antioxidant activities of polyphenols extracted from perilla frutescens varieties. Mol. (Basel. Switzerland). 14 (1), 133–140. doi: 10.3390/molecules14010133
Moreau, R. A., Nyström, L., Whitaker, B. D., Winkler-Moser, J. K., Baer, D. J., Gebauer, S. K., et al. (2018). Phytosterols and their derivatives: Structural diversity, distribution, metabolism, analysis, and health-promoting uses. Prog. Lipid Res. 70, 35–61. doi: 10.1016/j.plipres.2018.04.001
Panchal, P., Miller, A. J., Giri, J. (2021). Organic acids: versatile stress-response roles in plants. J. Exp. Bot. 72 (11), 4038–4052. doi: 10.1093/jxb/erab019
Paulusma, C. C., Lamers, W. H., Broer, S., van de Graaf, S. (2022). Amino acid metabolism, transport and signalling in the liver revisited. Biochem. Pharmacol. 201, 115074. doi: 10.1016/j.bcp.2022.115074
Peiretti, P. G. (2011). Fatty acid content and chemical composition of vegetative parts of perilla (perilla frutescens l.) after different growth lengths. Res. J. Med. Plant 5 (1), 72–78. doi: 10.3923/rjmp.2011.72.78
Pereira, O. R., Silva, A., Domingues, M., Cardoso, S. M. (2012). Identification of phenolic constituents of cytisus multiflorus. Food Chem. 131 (2), 652–659. doi: 10.1016/j.foodchem.2011.09.045
Petropoulos, S. A., Fernandes, Â., Vasileios, A., Ntatsi, G., Barros, L., Ferreira, I. (2018). Chemical composition and antioxidant activity of cichorium spinosum l. leaves in relation to developmental stage. Food. Chem. 239, 946–952. doi: 10.1016/j.foodchem.2017.07.043
Pourcel, L., Routaboul, J. M., Cheynier, V., Lepiniec, L., Debeaujon, I. (2007). Flavonoid oxidation in plants: from biochemical properties to physiological functions. Trends Plant Sci. 12 (1), 29–36. doi: 10.1038/s41598-020-73274-y
Quirantes-Piné, R., Arráez-Román, D., Segura-Carretero, A., Fernández-Gutiérrez, A. (2010). Characterization of phenolic and other polar compounds in a lemon verbena extract by capillary electrophoresis-electrospray ionization-mass spectrometry. J. Sep. Sci. 33 (17-18), 2818–2827. doi: 10.1002/jssc.201000228
Raut, J. S., Karuppayil, S. M. (2014). A status review on the medicinal properties of essential oils. Ind. Crops Products. 62 (62), 250–264. doi: 10.1016/j.indcrop.2014.05.055
Ruan, Y. L. (2014). Sucrose metabolism: gateway to diverse carbon use and sugar signaling. Annu. Rev. Plant Biol. 65, 33–67. doi: 10.1146/annurev-arplant-050213-040251
Russell, J. S., Griffith, T. A., Naghipour, S., Vider, J., Du Toit, E. F., Patel, H. H., et al. (2020). Dietary α-linolenic acid counters cardioprotective dysfunction in diabetic mice: Unconventional PUFA protection. Nutrients 12 (9), 2679. doi: 10.3390/nu12092679
Sakr, S., Wang, M., Dédaldéchamp, F., Perez-Garcia, M. D., Ogé, L., Hamama, L., et al. (2018). The sugar-signaling hub: Overview of regulators and interaction with the hormonal and metabolic network. Int. J. Mol. Sci. 19 (9), 2506. doi: 10.3390/ijms19092506
Schiessel, D. L., Yamazaki, R. K., Kryczyk, M., Coelho, I., Yamaguchi, A. A., Pequito, D. C., et al. (2015). α-linolenic fatty acid supplementation decreases tumor growth and cachexia parameters in walker 256 tumor-bearing rats. Nutr. Cancer 67 (5), 839–846. doi: 10.1080/01635581.2015.1043021
Smeekens, S., Hellmann, H. A. (2014). Sugar sensing and signaling in plants. Front. Plant Sci. 5. doi: 10.3389/fpls.2014.00113
Stuper-Szablewska, K., Perkowski, J. (2019). Phenolic acids in cereal grain: Occurrence, biosynthesis, metabolism and role in living organisms. Crit. Rev. Food Sci. Nutr. 59 (4), 664–675. doi: 10.1080/10408398.2017.1387096
Tinikul, R., Chenprakhon, P., Maenpuen, S., Chaiyen, P. (2018). Biotransformation of plant-derived phenolic acids. Biotechnol. J. 13 (6), e1700632. doi: 10.1002/biot.201700632
Wang, H., Hua, J., Yu, Q., Li, J., Wang, J., Deng, Y., et al. (2021). Widely targeted metabolomic analysis reveals dynamic changes in non-volatile and volatile metabolites during green tea processing. Food Chem. 363, 130131. doi: 10.1016/j.foodchem.2021.130131
Wang, T., Sha, L., Li, Y., Zhu, L., Wang, Z., Li, K., et al. (2020). Dietary α-linolenic acid-rich flaxseed oil exerts beneficial effects on polycystic ovary syndrome through sex steroid hormones-Microbiota-Inflammation axis in rats. Front. Endocrinol. 11. doi: 10.3389/fendo.2020.00284
Wang, L. S., Stoner, G. D. (2008). Anthocyanins and their role in cancer prevention. Cancer Lett. 269 (2), 281–290. doi: 10.1016/j.canlet.2008.05.020
Wang, L., Sun, X., Jakob, W., Wolfram, W. (2017). System-level and granger network analysis of integrated proteomic and metabolomic dynamics identifies key points of grape berry development at the interface of primary and secondary metabolism. Front. Plant 8. doi: 10.3389/fpls.2017.01066
Wang, Z., Tu, Z., Xie, X., Cui, H., Kong, K. W., Zhang, L. (2021). Perilla frutescens leaf extract and fractions: polyphenol composition, antioxidant, enzymes (α-glucosidase, acetylcholinesterase, and tyrosinase) inhibitory, anticancer, and antidiabetic activities. Foods. (Basel. Switzerland). 10 (2), 315. doi: 10.3390/foods10020315
Weckwerth, W., Wenzel, K., Fiehn, O. (2010). Process for the integrated extraction, identification and quantification of metabolites, proteins and RNA to reveal their co-regulation in biochemical networks. Proteomics 4 (1), 78–83. doi: 10.1002/pmic.200200500
Wei, C. L., Zhang, C. W., Guo, B. L., Li, W. P., Gao, Z. X., Zhang, F., et al. (2017). Research on effects of chemotype and components of perilla frutescens leaf volatile oil I: different phenological periods. Zhong. guo. Zhong. Yao. Za. Zhi. 42 (4), 712–718. doi: 10.19540/j.cnki.cjcmm
Xiao, M., Wu, F. (2014). A review of environmental characteristics and effects of low-molecular weight organic acids in the surface ecosystem. J. Environ. Sci. (China). 26 (5), 935–954. doi: 10.1016/S1001-0742(13)60570-7
Yamazaki, M., Nakajima, J., Yamanashi, M., Sugiyama, M., Makita, Y., Springob, K., et al. (2003). Metabolomics and differential gene expression in anthocyanin chemo-varietal forms of perilla frutescens. Phytochemistry 62 (6), 987–995. doi: 10.1016/s0031-9422(02)00721-5
Yang, H., Sun, W., Fan, Y. N., Li, S. Y., Yuan, J. Q., Zhang, Z. Q., et al. (2021). Perilla leaf extract attenuates asthma airway inflammation by blocking the syk pathway. Mediators Inflammation 2021, 6611219. doi: 10.1155/2021/6611219
Yu, H., Qiu, J. F., Ma, L. J., Hu, Y. J., Li, P., Wan, J. B. (2017). Phytochemical and phytopharmacological review of perilla frutescens l. (Labiatae), a traditional edible-medicinal herb in China. Food Chem. Toxicol. 108 (Pt B), 375–391. doi: 10.1016/j.fct.2016.11.023
Zhang, Y., Shen, Q., Leng, L., Zhang, D., Chen, S., Shi, Y., et al. (2021). Incipient diploidization of the medicinal plant perilla within 10,000 years. Nat. Commun. 12 (1), 5508. doi: 10.1038/s41467-021-25681-6
Zheng, Y. F., Li, D. Y., Sun, J., Cheng, J. M., Chai, C., Zhang, L., et al. (2020). Comprehensive comparison of two color varieties of perillae folium using rapid resolution liquid chromatography coupled with quadruple-time-of-flight mass spectrometry (RRLC-Q/TOF-MS)-based metabolic profile and in vivo/in vitro anti-oxidative activity. J. Agric. Food Chem. 68 (49), 14684–14697. doi: 10.1021/acs.jafc.0c05407
Zhou, X. J., Yan, L. L., Yin, P. P., Shi, L. L., Zhang, J. H., Liu, Y. J., et al. (2014). Structural characterisation and antioxidant activity evaluation of phenolic compounds from cold-pressed perilla frutescens var. arguta seed flour. Food Chem. 164, 150–157. doi: 10.1016/j.foodchem.2014.05.062
Keywords: Perilla leaf, mass spectrometry, metabolomic dynamics, harvest time, multivariate statistical analysis
Citation: Chen J, Guo L, Yang G, Yang A, Zheng Y and Wang L (2022) Metabolomic profiling of developing perilla leaves reveals the best harvest time. Front. Plant Sci. 13:989755. doi: 10.3389/fpls.2022.989755
Received: 08 July 2022; Accepted: 10 November 2022;
Published: 30 November 2022.
Edited by:
Claudio Bonghi, University of Padua, ItalyReviewed by:
Kamel Msaada, Center of Biotechnology of Borj Cedria (CBBC), TunisiaCopyright © 2022 Chen, Guo, Yang, Yang, Zheng and Wang. This is an open-access article distributed under the terms of the Creative Commons Attribution License (CC BY). The use, distribution or reproduction in other forums is permitted, provided the original author(s) and the copyright owner(s) are credited and that the original publication in this journal is cited, in accordance with accepted academic practice. No use, distribution or reproduction is permitted which does not comply with these terms.
*Correspondence: Yuguang Zheng, enlnMzE0QDE2My5jb20=; Lei Wang, d2FuZ2xlaTEwMzFAMTI2LmNvbQ==
†These authors have contributed equally to this work
Disclaimer: All claims expressed in this article are solely those of the authors and do not necessarily represent those of their affiliated organizations, or those of the publisher, the editors and the reviewers. Any product that may be evaluated in this article or claim that may be made by its manufacturer is not guaranteed or endorsed by the publisher.
Research integrity at Frontiers
Learn more about the work of our research integrity team to safeguard the quality of each article we publish.