- 1Department of Plant and Environmental Sciences, Faculty of Science, University of Copenhagen, Frederiksberg, Denmark
- 2Department of Chemistry and Bioscience, Aalborg University, Aalborg, Denmark
- 3CSIRO, Agriculture and Food, Floreat, WA, Australia
The perennial halophytic shrubs Atriplex nummularia and Rhagodia preissii are native to Australia and can be planted on saline land to produce sheep and cattle feed during the autumn. However, an impediment to the adoption of the species on saline land has been the challenges in achieving successful establishment by direct seeding due to a lack of knowledge of the optimal conditions for germination. Therefore, the optimal germination requirements in relation to temperature, salinity level and seed size was assessed for each species to ascertain the optimal conditions for successful establishment by direct seeding on saline land. Seeds of both species showed optimal germination temperature at 10°C. Atriplex nummularia seeds were more tolerant to temperatures above or below 10°C than R. preissii. The germination percentage of A. nummularia was unchanged at 0–200 mM NaCl2. The germination percentage of Rhagodia preissii declined when the NaCl2 content exceeded 50 mM. There was no correlation between seed size, germination and emergence for any of the species. Based on the study, we suggest that sowing operations are performed during the cold winter months in subtropical areas or autumn and spring in temperate areas, to improve the successful establishment of these shrubs by direct seeding.
Introduction
Increasing salinization of agricultural soils constitutes an accelerated problem. Roughly estimated 7% of the world’s agricultural soils are now salt-affected, primarily in the arid and semi-arid regions of the world making them less capable of supporting agricultural production (Wicke et al., 2011; Daliakopoulos et al., 2016). Asia, North Africa, the eastern Mediterranean area, North and South America, and Australia are most severely affected, with the highest proportion of saline lands. They are all facing a considerable loss of agricultural land due to salinity (Masters et al., 2005; Corwin, 2021). The problems with dryland salinity are particularly pronounced in the South West of West Australia (WA) where more than 1 million hectares are salt-affected and with an estimated annual loss of 14.000 hectares (Caccetta et al., 2010). The prospects for the region are further challenged by an expected increase in temperatures and extreme weather events (Furby et al., 2010; Turner et al., 2011).
Development and adoption of perennial halophytic shrubs should be promoted to increase climate resilience and maintain the profitability of otherwise unproductive saline and infertile lands (Norman et al., 2013). The additional forage provided by the shrubs can reduce the cost and reliance on supplemental grains and roughage for hand feeding during the summer/autumn feed gap, which can be a critical production cost within these livestock systems in arid and semi-arid areas (Ben Salem et al., 2010; Norman et al., 2017).
In saline regions, the cultivation of halophytic perennial shrubs has gained interest because they can produce a high-value supplemental feed for livestock on saline soils and increase water use efficacy (Glenn et al., 1999; Norman et al., 2013).
The establishment of Atriplex nummularia, Rhagodia preissii, and other perennial shrubs can play an important role in managing dryland salinity and counteracting erosion and degradation (Louhaichi et al., 2016). A limited number of forage crops tolerate the combined stress of salinity and arid conditions. However, species from the Chenopodiaceae family, especially Atriplex nummularia (Oldman saltbush), have gained attention due to their persistence in dry climates, saline, and infertile soils and their value as a supplemental feed for livestock within these areas (Le Houerou, 1992; Ben Salem et al., 2010; Norman et al., 2013). Likewise, the perennial shrub Rhagodia preissi (Mallee saltbush) is preferred by farmers because it performs well on nutrient-poor sandy soils, is drought tolerant and produces substantial amounts of dry matter (Kotze et al., 2011). As a result of screening potential Australian shrub species for agricultural purposes and revegetation A. nummularia and R. preissii were found to be among the shrubs with the highest biomass production (Revell et al., 2013).
A number of halophytic plants can produce high yields at elevated salinity levels. Aronson et al. (1988) studied 170 halophytes and found seven Atriplex species produced 12.6–20.9 t ha−1 of biomass containing 9.9–19.5% protein on full-strength seawater irrigation. Gallagher (1985) obtained yields of 5.2–9.5 t ha−1 of the saltgrass Distichlis spicata under seawater (30 g l−1) irrigation in Delaware, United States, while Spartina patens yielded 14.4 t ha−1 when harvested in July. Hollington et al. (2001) showed that saltbush (Atriplex spp.), bluebush (Maireana spp.) and other halophytic plants were also highly useful as forage in a wide range of different climatic zones of Faisalabad, Peshawar, Bhawalpur and Karachi in Pakistan. Despite the beneficial attributes of perennial halophytic shrubs in rangeland rehabilitation and livestock feeding, their adoption has been limited because of establishing costs. Commercial nursery production of seedlings was previously initiated, and seedlings were planted by tractor-drawn tree-planting machinery (Barret-Lennard et al., 1991). However, nursery-raised seedlings are expensive due to labor and nursery costs over several months, which restrict the implementation of planting (Barrett-Lennard et al., 2016). These costs are not only a barrier in South West WA but also in rangelands in West Asia and North Africa, where the establishment of these shrubs is highly sought to counter rangeland degradation (Louhaichi et al., 2019). Therefore, research in direct seeding was undertaken with the resultant development of the Mallen niche seeder (Malcolm et al., 1982), creating an optimized seedbed for germination at a much lower cost than mechanical or hand-planting of nursery-raised seedlings.
Direct seeding represents a tradeoff between cost and risk since direct seeding of fruits of A. nummularia only has a successful establishment rate of about 31% compared to 82% when seedlings are planted and has therefore never become a commercial reality (Barrett-Lennard et al., 2016).
The low establishment rate of direct-seeded Atriplex spp. is attributed to the inhibitory effects of the bracteoles surrounding the seeds. Several studies have shown that bracteole removal decreases germination time and increases the germination percentage under laboratory conditions (Stevens et al., 2006; Louhaichi et al., 2019). Therefore, removing the bracteoles is a crucial method to improve field emergence and the speed of seedling development (Ungar, 2001; Louhaichi et al., 2019). Previous studies showed that the optimal germination temperature for A. nummularia was 15°C (Uchiyama, 1981; Malcolm et al., 1982). It was also found that temperatures from 25°C and above reduced the germination by 50% or more and that seeds below 0.5 mg had below 10% germination. In contrast, seeds above 0.5 mg had an 83% or higher germination percentage.
No studies have examined the seed ecology of R. preissii, which is essential to improve direct seeding. A study of the related species Rhagodia baccata (berry saltbush) reported that the viability of the seeds was 63% and that the germination was highest at a temperature regime of 13/26°C compared to 18/33°C (Commander et al., 2009). Germination occurs typically at the highest water availability, and optimum germination temperature also occurs at this time of year (Bell, 1999). This indicates that optimum sowing time should be in autumn/winter, with optimal germination temperatures of 13–18°C for the Mediterranean-type species in the South West WA with winter rainfall (Bell, 1994). Seed germination in arid and semiarid regions usually occurs after the rains when the soil surface salinity has declined caused by the leaching of salts (Khan and Ungar, 1999; Li and Zhang, 2007). Several studies have shown that halophyte species have a high salt tolerance during germination but that the germination is highest in non-saline conditions and decreases with increasing salinity (Gul et al., 2013). Salinity inhibits seed germination if salinity increases above the salinity threshold of the species and causes a delay in seed germination without preventing it when the salinity level is below the species’ salinity threshold (Khan et al., 2002). Seed mass is an essential parameter for germination ability and has been found to be positively correlated with higher germination under saline conditions (Zhang et al., 2015).
Despite the efforts to achieve the successful establishment of these perennial shrubs by direct seeding to bring down the cost of establishment, there is still a lack of knowledge on the germination requirements regarding temperature, salinity tolerance and the importance of seed size. A more thorough understanding of the requirements for optimal germination has the potential to substantially improve the field emergence (Stevens et al., 2006).
This study aimed to estimate the effect of temperature and salinity on the germination of A. nummularia and R. preissii seeds. The optimal seed size for germination and seedling development was also determined to assist plant breeders in selecting appropriate material. We hypothesized that seeds of both species would have optimal germination temperatures at around 10–15°C in non-saline conditions, corresponding to the conditions in the wet winter months in Australia, where these species are naturalized and used in agriculture. We expected that seed weight was positively correlated with the total germination percentage and healthy seedling development.
Materials and methods
Seed collection
Seeds from a population of R. preissii were collected on 18 April 2019 at an experimental plot at CSIRO in Floreat, Western Australia (WA). Fruits were macerated and washed through sieves of different sizes to collect seeds. The seeds were then dried for 3 days at 40°C, and vacuum packed (Figures 1A,B).
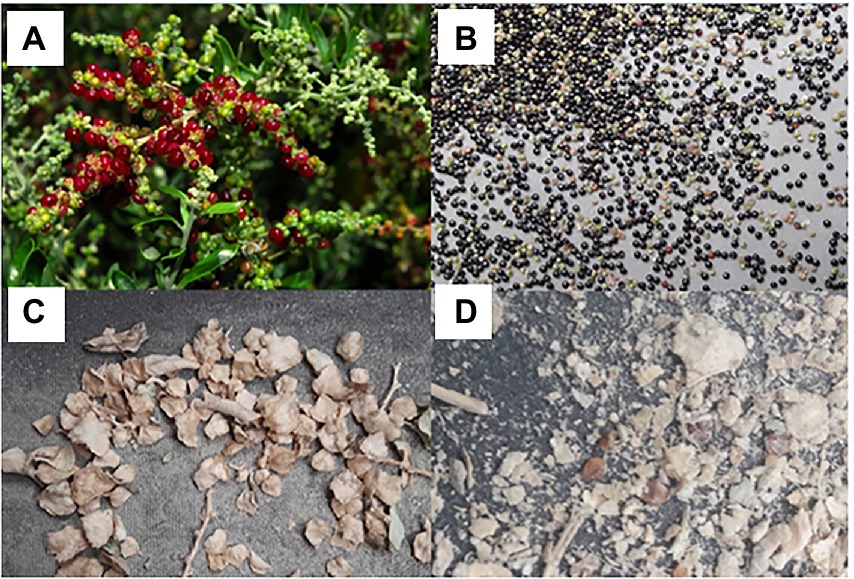
Figure 1. Images showing the fruits and seeds of Rhagodia preissi and Atriplex nummularia. (A) Developing fruits of R. preissii (Muirhead, 2017) that each contain one seed, (B) Seed of R. preissii both mature (black) and immature ones, (C) Fruits of A. nummularia with varying sizes of bracteoles, and (D) Cleaned seeds obtained by gently using corrugated rubber mats.
Seeds from a naturalized population of A. nummularia were collected in January 2019 from a farmer’s field in Cranbrook, WA, Australia and dried for 3 days at 40°C. Pure seeds were obtained by removing the bracteoles which enclosing the seeds by gently rubbing the dried fruits between corrugated rubber matting (Figures 1C,D; Stevens et al., 2006). This is a laborious task but is necessary to obtain clean and undamaged seeds.
Four germination experiments were conducted to estimate the optimal conditions for germination of A. nummularia and R. preissii in relation to 1. temperature, 2. salinity, 3. seed size, and 4. seed weight.
For each treatment, four replicates of 100 randomly selected seeds were germinated in germination trays called the “Jacobsen apparatus” (ISTA, 2011). The apparatus consists of a transparent box with a germination plate upon which filter paper with seeds was placed. The filter paper was kept moist by a wick, extending down into an underlying water bath. Seeds were spaced uniformly and adequately apart on the filter paper. This setup ensured appropriate oxygen and water availability during the experiment (ISTA, 2011). All the germination experiments were conducted in plant growth chambers (Conviron, controlled environment systems) with 12 h of light (6 am–6 pm), and 12 h of darkness. The light source provided white light imitating natural light conditions with a photon flux density of 342 μmol m−2 s−1 of PAR (photosynthetic active radiation, 400–700 nm). Germinated seeds were counted and removed during the light period in all experiments, which lasted for a month.
Experiment 1: Optimal germination temperature
Four replicates of 100 seeds of both species were set to germinate in plant growth chambers under four constant temperature regimes (5, 10, 20, and 30°C) to determine the optimal germination temperature. The selected temperatures are related to the range of temperatures the seeds would experience over the year in WA. Seeds were counted three times a day during the light period, and germinated seeds were removed from the filter paper. The temperature experiment was replicated once to verify the results.
Experiment 2: Germination at different salinity levels
Seeds were placed under five different salinities of sodium chloride solution (0, 50, 100, 200, and 500 mM corresponding to a conductivity of 0, 5, 10, 20, and 50 dS m−1) to assess the salinity tolerance of the seeds during germination and the effect of increasing salinity on the germination percentage. Many arable soils in WA have a salt content belonging to this salinity interval. For each salinity level and species, four replicates of 100 seeds were set to germinate in plant growth chambers at 10°C. Seeds were counted three times a day during the light period. Germinated seeds were removed from the filter paper. The salinity experiment was replicated once to verify the results.
The water level in the germination trays was recorded before the experiment started and after termination. No significant water loss was recorded, indicating that there was no accumulation of salts in the germination media due to evaporation of water.
Experiment 3: The relationship between seed weight, germination and seedling development
The correlation between individual seed weight, hours to germination and the subsequent development of seedlings were examined by weighing 300 individual seeds of A. nummularia and R. preissii on an analytical scale. The weighed seeds were placed in germination trays, and their individual position on the filter paper was noted. Germination trays were placed in a plant growth chamber at 10°C. Germinated seeds were kept in the germination tray to record the number of normal and abnormal seedlings.
Experiment 4: The relationship between seed size and seed vigor
Seeds were sorted using metal hand seed sorters with round holes (0.75, 1, 1.25, 1.5, 1.75, and 2 mm). Seed sorters were placed on top of each other and then shaken with the seed on top for 5 min to divide them into different size fractions. Each seed sorter was thoroughly cleaned to collect all seeds. Seeds of each fraction were then counted, and the 1,000 seed weight was recorded.
Pots with holes in the bottom for drainage were filled with sand (0.4–0.9 mm), and 25 seeds were placed on the surface and covered with 5 mm sand. The pots were then placed in a plant growth chamber at 10°C. The pots were checked once a day for emerging seedlings during the light period, and pots were irrigated from below, ensuring a sufficient water supply. A seedling was characterized as fully developed when the cotyledons have fully emerged. The percentage of seeds developing a seedling was recorded.
Statistical analyses
All experiments were analyzed separately using the open-source program R version 3.5.3.1 Analysis of dose–response curves was described with a three-parameter asymmetric sigmoid Weibull curve using the add-on package drc (Ritz et al., 2005). The seed germination data was modeled using a cumulative distribution model function of the standard log-logistic distribution (Equation 1):
where d is the upper limit parameter that denotes the percentage of seeds that germinated during the experiment out of the initial number of seeds. t50 denotes the time to germination of 50% of the maximum number of germinated seeds. The b-parameter is proportional to the slope of F at time t equal to the parameter t50. The unit of time (hours) applied in the experiment was also applied to t50. The model checking and estimation procedures were performed by dealing with the data as event times by recording the time until germination as the event of interest (Ritz et al., 2013; Andreasen et al., 2014; Jensen et al., 2017). A significance level of 0.05 was applied in all analyses.
Results
Characteristics of the seeds
In general, cleaned seeds had high viability indicating that the cleaning procedure did not damage the seeds. The exact temperatures were logged to verify that the set temperature corresponded to the actual temperatures during the experimental period (Table 1).
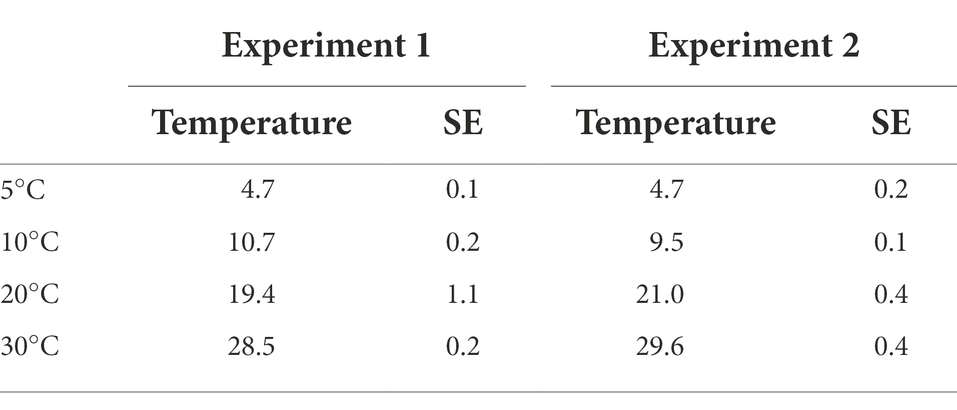
Table 1. Logged temperatures during the experimental period for each of the climate chamber recorded every 5 min’s and averages presented (Standard errors = SE).
The average 1,000 seed weight was 600 mg (SE = 53 mg) for R. preissii and 980 mg (SE = 41 mg) for A. nummularia. The variance in seed weight and size was large for seeds of A. nummularia (Figure 2), while the seeds of R. preissii were more uniform in seed size and weight (Figure 1).
Experiment 1: Optimal germination temperature
The parameter t50 (time to germination of 50% of the maximum number of germinated seeds) was estimated to be the same in both temperature experiments for both species (Tables 2, 3). The maximum germination percentages were also alike (Tables 2, 3). The b parameter (relative slope at t50) is an expression of the speed of germination at t50 (Figure 3).
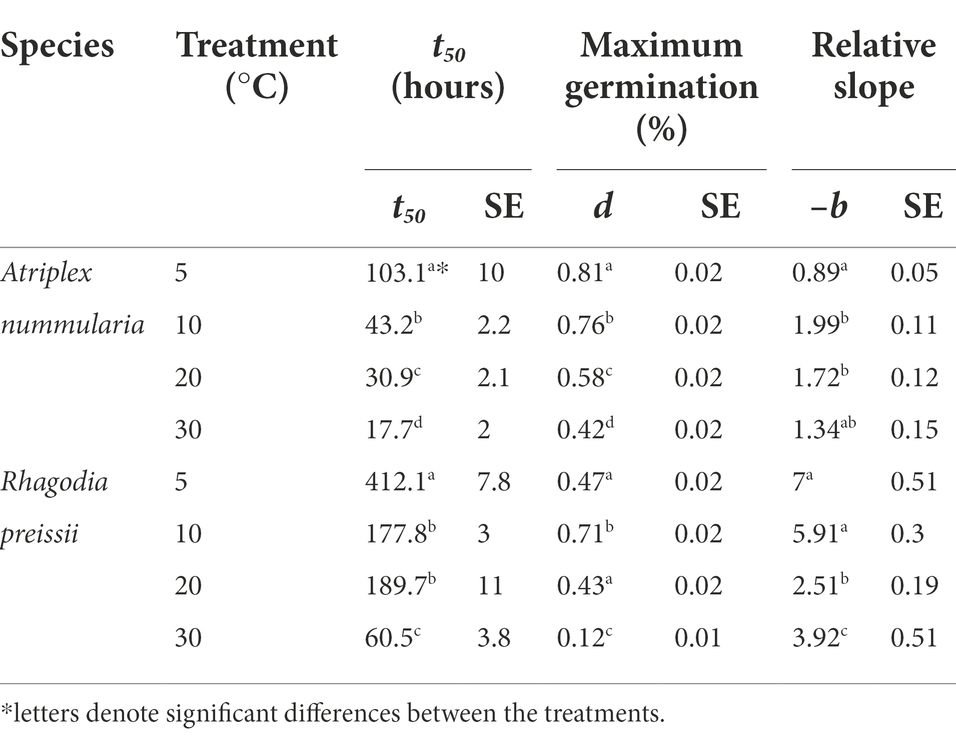
Table 2. Temperature experiment 1A: influence of temperature on seed germination of A. nummularia and R. preissii under four constant temperatures, expressed by the estimated parameters and their associated standard errors (SE) from the log-logistic model (1) applied to the data*.
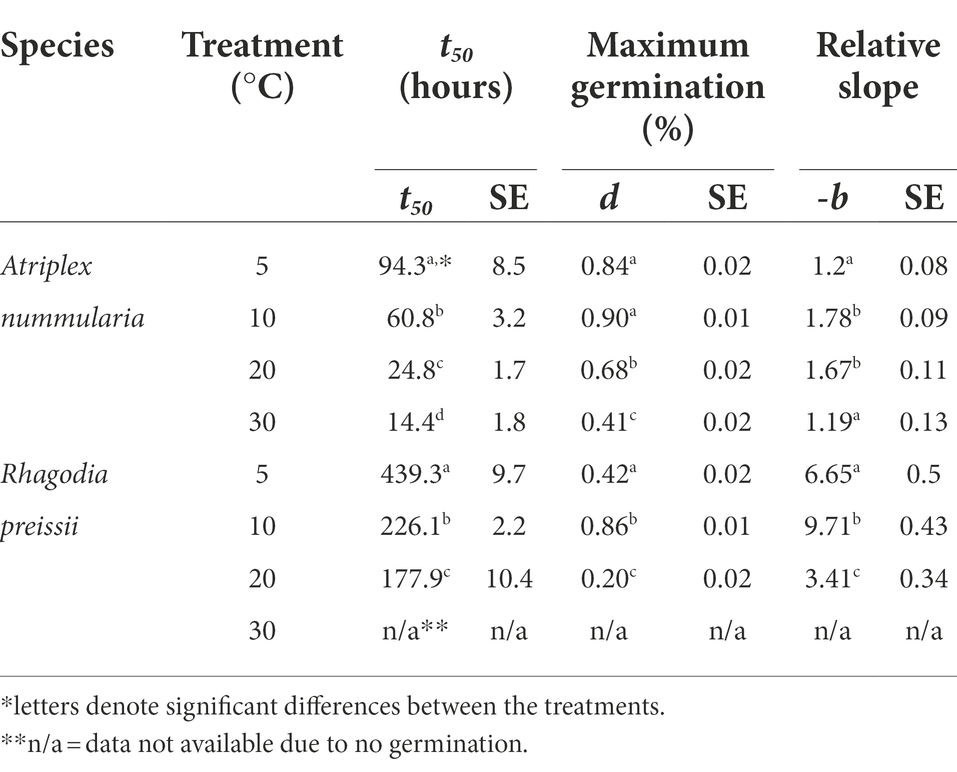
Table 3. Temperature experiment 1B: influence of temperature on seed germination of A. nummularia and R. preissii under four constant temperatures, expressed by the estimated parameters and associated SE from the log-logistic model (1) applied to the data.
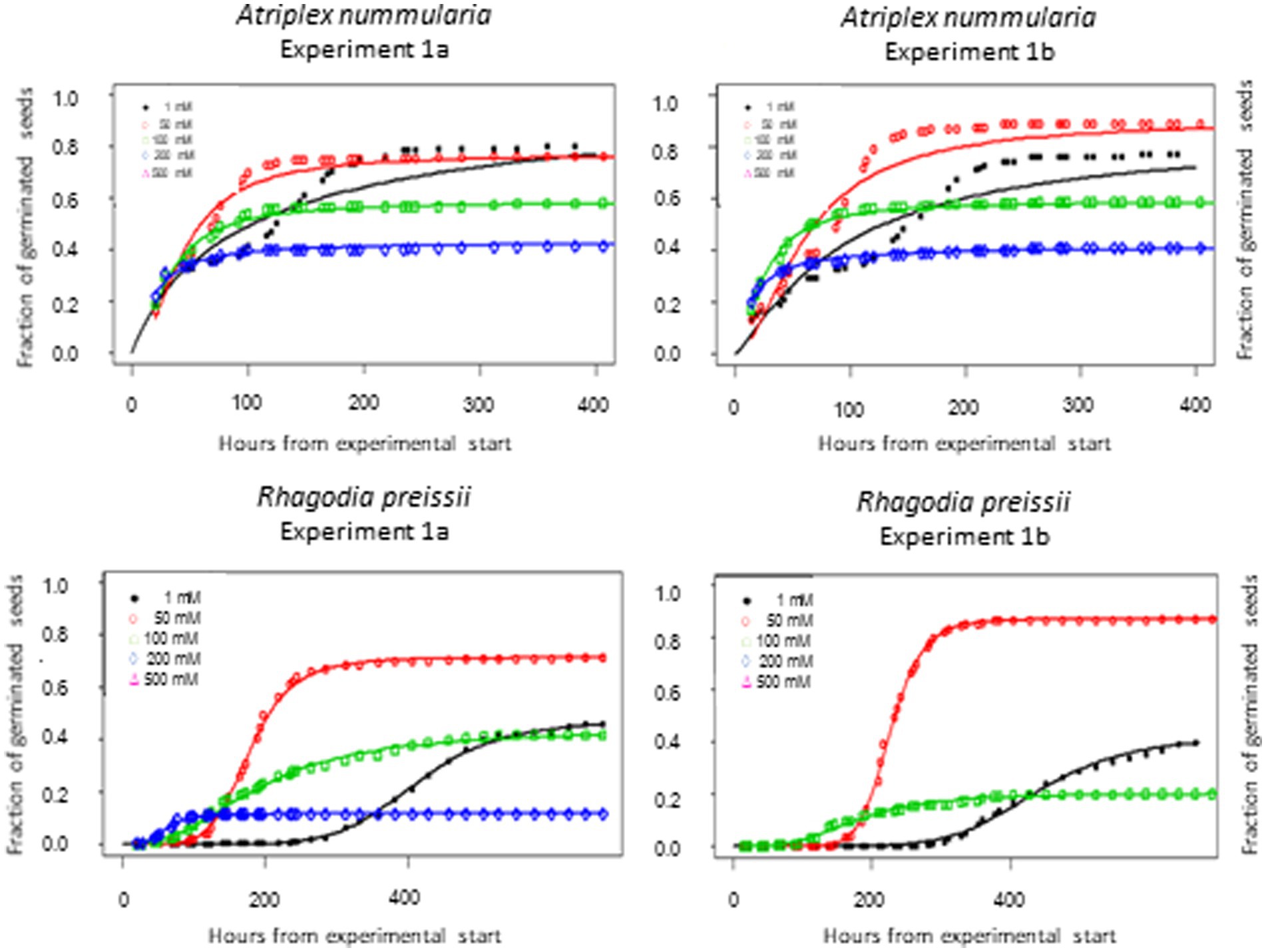
Figure 3. Germination curves for A. nummularia and R. preissii at four constant temperatures. In experiment 1B at 30°C no seeds of R. preissii germinated.
The highest germination percentage occurred at 10°C for both species, with 10°C being significantly higher than the three other temperatures for R. preissii. The germination at 10°C was only significantly higher than at 5°C for A. nummularia in one of the tests, but significantly different from results at 20 and 30°C in both experiments. There was a significant decrease in t50 values for both species at increasing temperatures.
Experiment 2: Germination at different salinity levels
t50 Values and maximum germination percentage were approximately the same in the Experiments 2A and 2B for both species (Tables 4, 5). The highest germination percentage for A. nummularia was reached at 0–200 mM, with no significant differences in Experiment 2A but some significant differences in Experiment 2B for 0–200 mM. 500 mM reduced the maximum germination significantly in both tests.
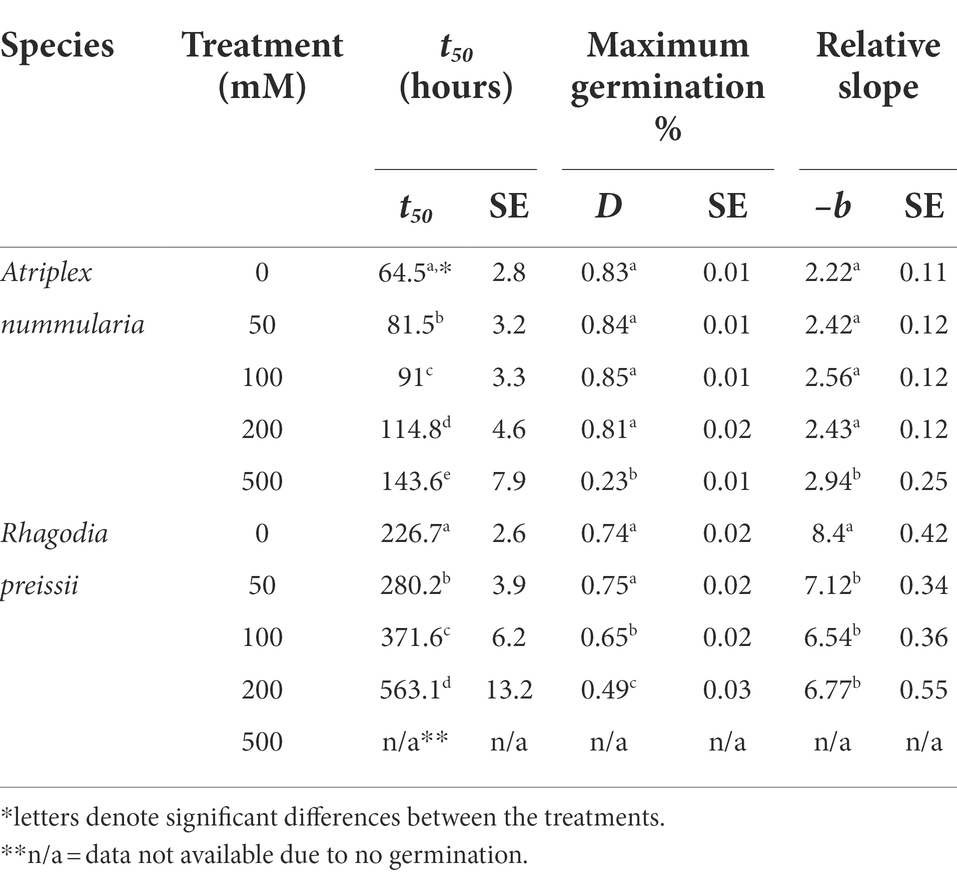
Table 4. Salinity experiment 2B: influence of salinity level on seed germination of A. nummularia and R. preissii at five different salinity levels, expressed by the estimated parameters and associated standard error (SE) from the log-logistic model (1) applied to the data.
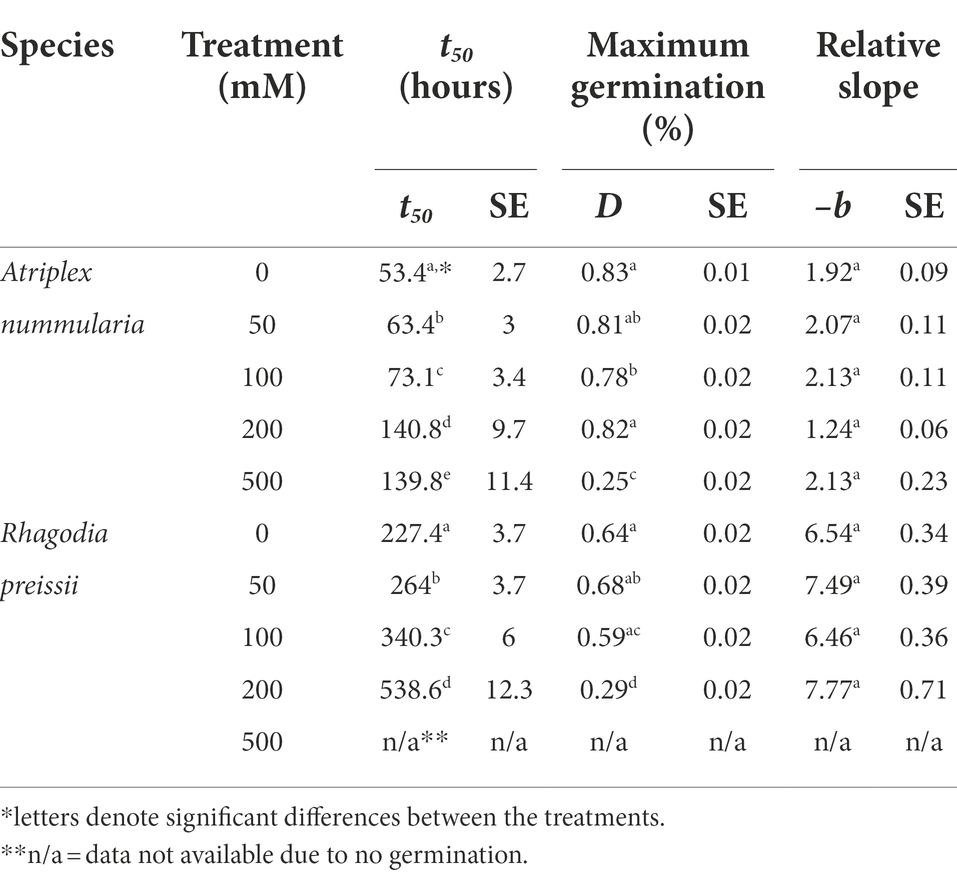
Table 5. Salinity experiment 2A: influence of salinity level on seed germination of A. nummularia and R. preissii at five different salinity levels, expressed by the estimated parameters and associated standard errors (SE) from the log-logistic model (1) applied to the data.
Rhagodia preissii had maximum germination at 0–50 mM. A significant reduction occurred already at 100 mM, and was reduced even more at 200 mM (Figure 4). No R. preissii seeds were able to germinate at 500 mM. There was a significant increase in t50 values with increasing salinity levels for both species.
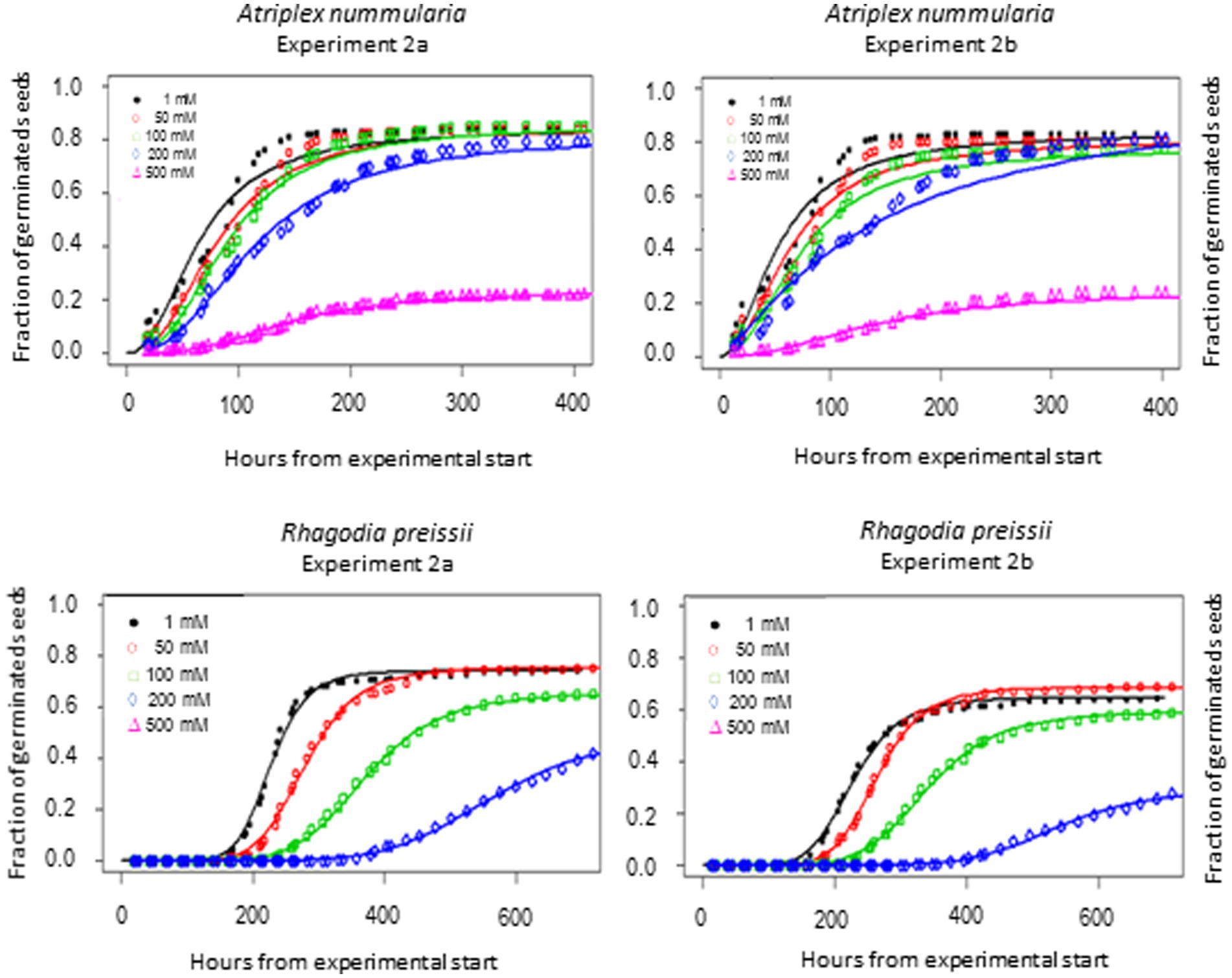
Figure 4. Germination curves for A. nummularia and R. preissii at five different salinity levels. No seeds of R. preissii germinated at the 500 mM.
Experiment 3: The relationship between seed weight, germination and seedling development
The correlation between seed weight, germination ability and the consequent development of healthy seedlings is essential for sowing operations. The germination percentage increased with increasing seed weight above a minimum weight. Atriplex nummularia seeds above 0.75 mg and R. preissii seeds above 0.5 mg had the highest germination percentage (Table 6). For both species, there were no significant differences or trends in hours to germination with increasing seed weight. While seeds of A. nummularia readily germinated, seeds of R. preissii started germinating 6 days after.
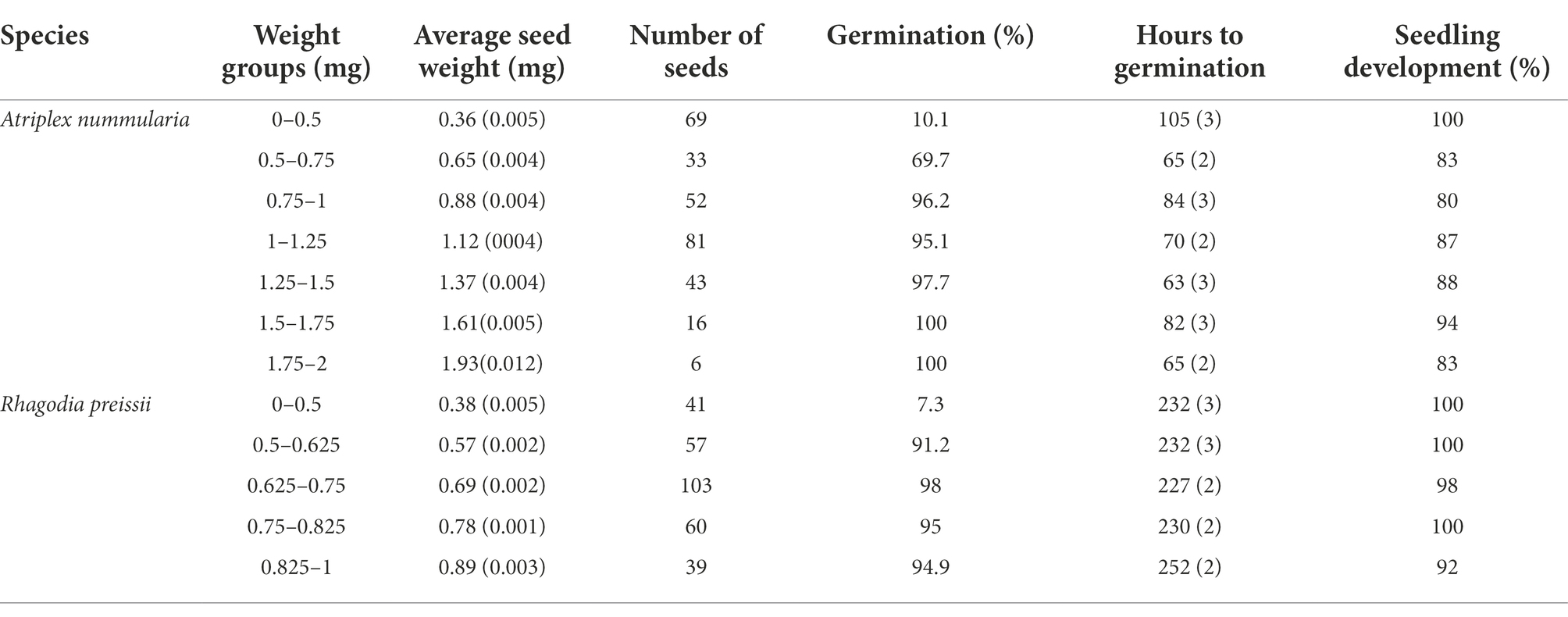
Table 6. Germination percentage of individually weighed seeds sorted into weight groups, and the groups average time (hours) to germination and seedling development with standard errors (SE).
The uniformity in hours to germination for different seed weights was primarily caused by a high variation within each weight group, as visualized in Figure 5. On average, the variation in hours to germination within all size groups was 45 h for A. nummularia and 42 h for R. preissii.
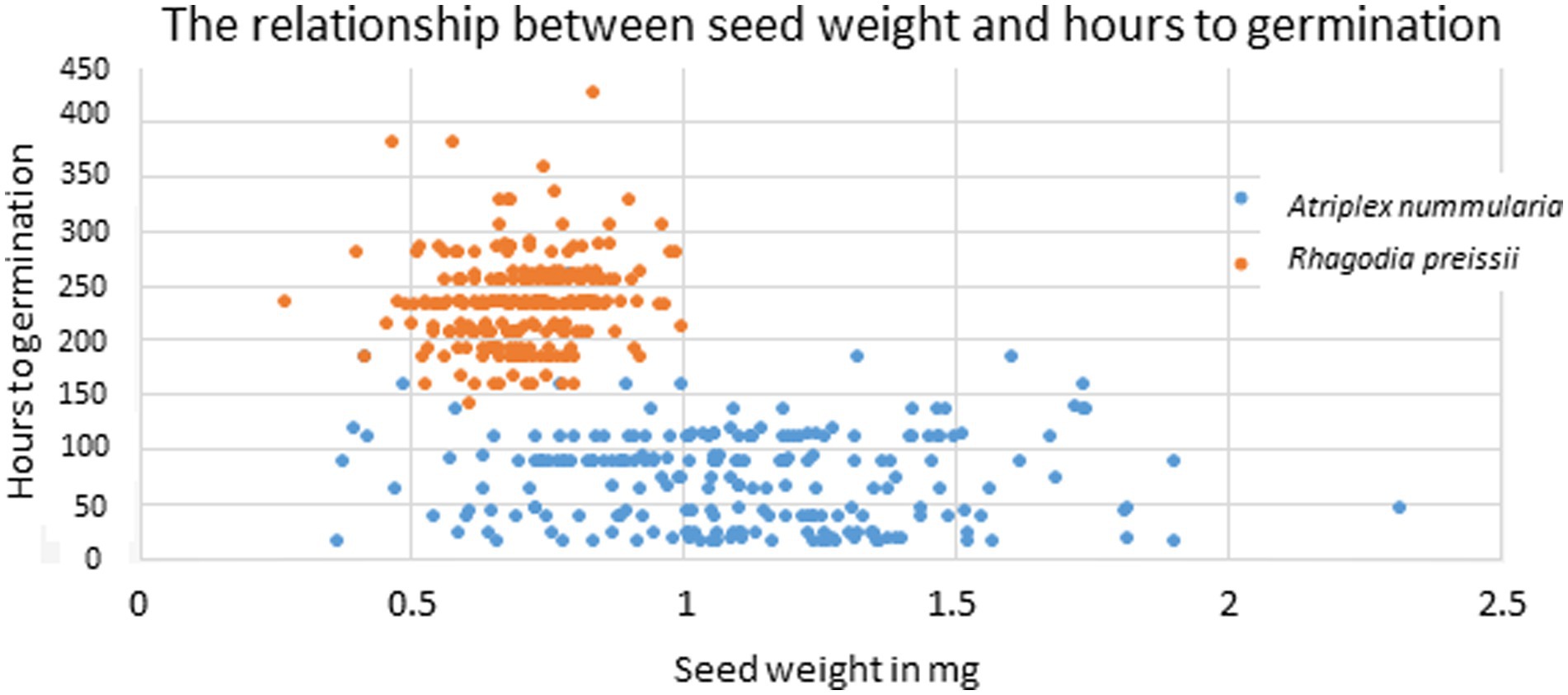
Figure 5. Scatter plot showing the correlation between individual seed weight and the number of hours to germination for A. nummularia (blue) and R. preissii (orange).
Experiment 4: The relationship between seed size and seed vigor
All seeds of A. nummularia passed through the 2 mm hand seed sorter, and roughly 90% of the seeds were between 1.25 and 1.75 mm (Table 7). All R. preissii seeds passed through the 2 mm, 1.75 mm, and 1.5 mm hand seed sorters. Approximately 75% of the seeds were between 0.75 and 1.25 mm. There was a correlation between seed size and 1,000 seed weight for A. nummularia, while this correlation was not significant for R. preissii, which had a more uniform seed weight and shape.
Seeds of A. nummularia emerged faster than R. preissii seeds, and consequently, the seedlings of A. nummularia were considerably larger at the end of the experiment than the R. preissii seedlings. There was a significant increase in t50 values with increasing seed size up to 1.5 mm for A. nummularia, although no correlation was found between increasing size and maximum emergence. However, only seeds < 1.5 mm and seeds < 1.75 mm performed significantly differently (Table 8).
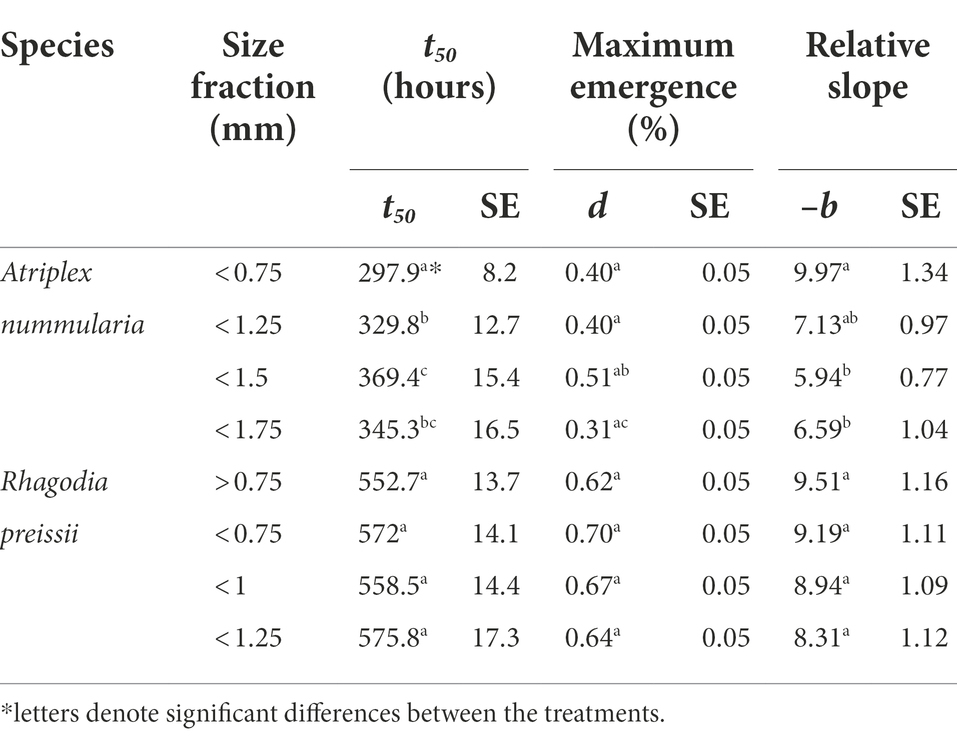
Table 8. The influence of seed size on the germination of A. nummularia and R. preissii expressed by the estimated parameters and their associated standard errors (SE) for each size fraction.
The seeds of R. preissii had a slower emergence rate and smaller seedlings and were more uniform with no significant differences in t50 values or total emergence percentage (Figure 6). The total emergence percentage was on average for all four size fractions 65.8% for R. preissii and 40.5% for A. nummularia.
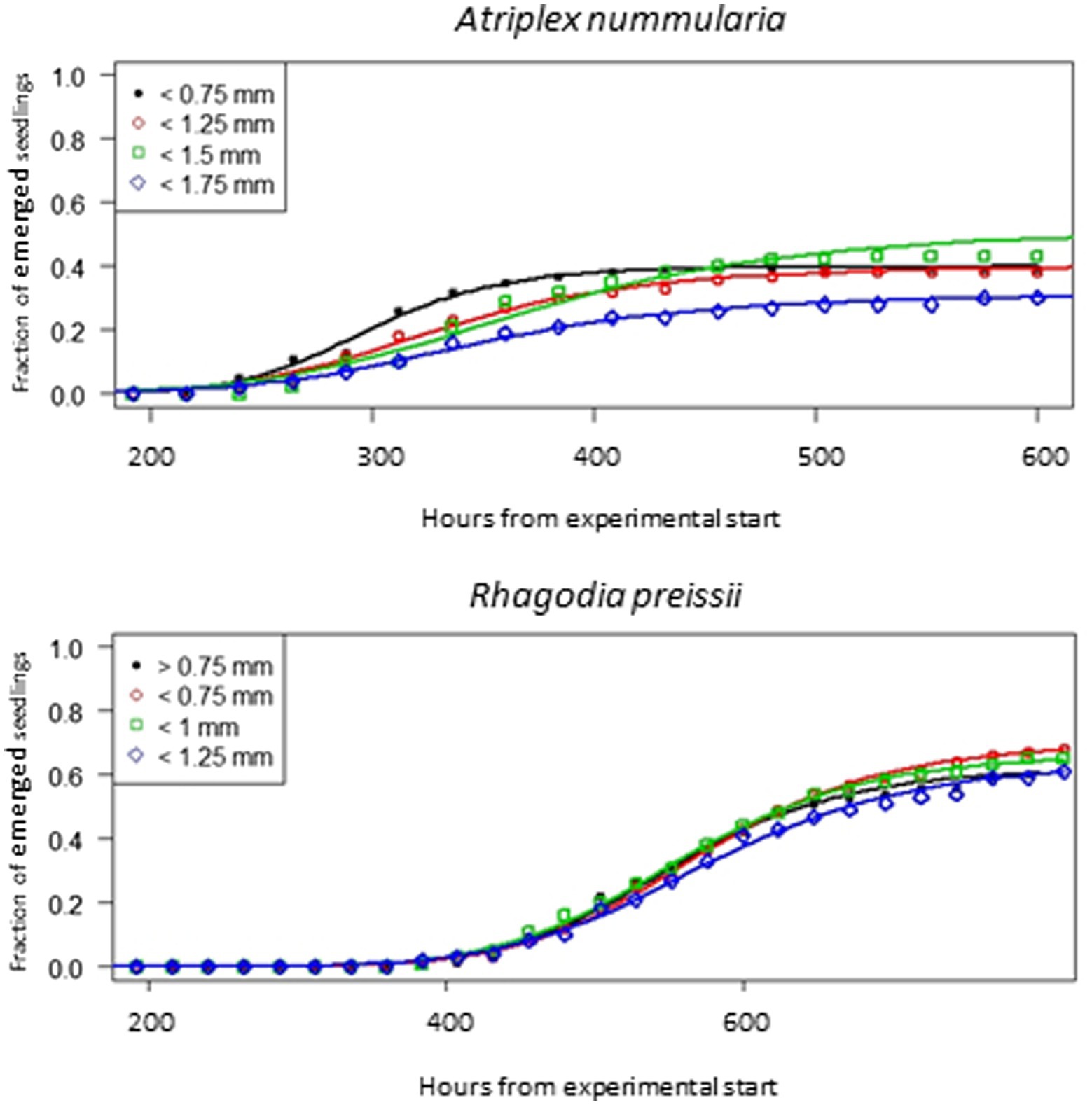
Figure 6. Emergence curves for four different seed size fractions of A. nummularia and R. preissii sown in 5 mm depth in sand.
Discussion
Temperature requirement
Generally, A. nummularia germinated faster than R. preissii, with an average t50 of 52 h (2.1 days) at 10°C compared with a t50 value of 202 h (8.4 days) for R. preissii. There was a significant decrease in t50 values with increasing temperatures, indicating that the seeds germinate faster at higher temperatures. Fast germination and seedling development are required to utilize the available moisture in the soil after a rainfall event, ensuring root development and persistence through potential dry periods after germination. Although rapid germination is desired, higher temperatures come at the cost of reduced germination percentage above 10°C. Atriplex nummularia had similar maximum germination at 5 and 10°C (82.5 and 83%), and only 63% germination at 20°C and 41.5% at 30°C.
Rhagodia preissii was more sensitive to high temperatures. None or a few seeds germinated at 30°C, and only roughly 40% of the seeds germinated at 5 and 20°C compared with, on average, 78.5% germination at 10°C. The experiments were conducted at constant temperatures. However, the temperature usually fluctuates day and night, and testing different thermo-periods would probably improve the estimation of the optimal sowing time. However, it is degree days that determine the germination speed.
The tradeoff between maximum germination and germination time should be considered in relation to the risk of water stress and drying of the topsoil during germination. The tradeoff is less critical for A. nummularia than R. preissii, as R. preissii is more sensitive to temperatures above or below 10°C. The water availability is higher, and the temperature lower during winter, creating ideal conditions for germination. The 10-degree temperature optimum corresponds to temperatures in June to August in WA, the months with the most rainfall, indicating that the two species have naturally adapted to germinate during the winter months when the temperature is low, and the water availability is higher. In the spring, the temperatures are higher, and periods between rainfall events are greater, increasing the risk of drying out of germinating seeds before seedlings have been established.
One factor that could impair the sowing of seeds in winter at the onset of the rains could be a high soil salinity level, inhibiting and delaying germination and thereby making it more favorable to delay sowing until more salt has leached. Direct seeding is only recommended for sandy soils due to the faster leaching of salts from these soils after the onset of rains in May.
Salinity tolerance
Experiments 2A and 2B showed that A. nummularia had a very high salinity threshold with no reductions in maximum germination at 200 mM, but t50 increased significantly, illustrating the delay in seed germination also observed for many other species (Gul et al., 2013). At 500 mM, about 27% of A. nummularia seeds still germinated, a significantly higher percentage than the 10% previously reported (Uchiyama, 1987). The seeds used in this study were collected from a saline field, which may explain the difference. It has been documented that A. nummularia seeds collected from plants growing in a high salinity medium had a higher salt tolerance at the germination stage than seeds originating from plants grown in a low salinity medium (Uchiyama, 1987). Therefore, seeds should be collected from plants growing on saline soils for plant production on saline soils.
Rhagodia preissii seeds had a lower salinity threshold than A. nummularia seeds, with significant reductions in maximum germination starting to occur at 100 mM and significant for both salinity tests at 200 mM. The delay in germination was also higher for R. preissii, increasing the t50 by 324 h (14 days) on average when the salinity increased from 0 to 200 mM, compared with 69 h (3 days) for A. nummularia. The t50 increased by 242% for R. preissii and 221% for A. nummularia. Hence, the effect on t50 is similar. Still, in practice, the longer delay and reduction in maximum germination can make it more difficult to successfully establish R. preissii, especially in soils with high salinity levels, as a concentration of 500 mM ultimately hampered the germination. Seeds of R. preissii were collected from a non-saline area. Therefore, it should be studied whether seeds from plants growing on more saline soils tolerate a higher salt concentration during germination.
For many halophyte species, seeds inhibited by a salinity level above the species threshold, will resume germination when the salinity level is lowered (Khan and Gul, 2006). This mechanism ensures that the seeds persist in the seed bank despite high salinity stress under high evaporative conditions. This mechanism may also count for R. preissii. It has been shown that A. nummularia seeds resume germination when the salinity level is lowered (Uchiyama, 1987). If seeds are sowed too early in the winter when the salinity is high, they will eventually start germinating when the level falls below the threshold for seed germination. However, it remains unknown how large a fraction of the seeds remain viable after enduring extreme salinities in the soil solution. The low salinity threshold and sensitivity to warmer temperatures above 10°C make the window for the sowing of R. preissii narrower than for A. nummularia.
The relationship between seed weight, germination and seedling development
The percentage of healthy developed seedlings was highest for R. preissii seeds. On average, of all the germinated seeds, 98 ± 3.5% of the R. preissii seeds developed into a healthy seedling while 87.8 ± 7% of A. nummularia seeds did. Only a few germinated seeds did not develop seedlings and died after germination or developed abnormally.
For both species, there was no correlation between seed weight and the number of hours to germination. There was a considerable variation in time to germination within each weight group. Atriplex nummularia is an outcrossing species producing genetically different seeds, which may explain the large variation in germination time. The reproductive physiology of R. preissii remains unknown.
The seed weight of cleaned seeds was not essential to achieving optimal germination and seedling development. Our results indicate that if seed weight is above 0.75 mg for A. nummularia and 0.5 mg for R. preissii, most seeds are viable and able to develop into healthy seedlings, given that optimal conditions are present. Therefore, there is no reason to collect and sort only the heaviest seeds but avoid the lightest.
The relationship between seed size on seed vigor
Despite the high percentage of healthy developed seedlings, the vigor test showed a low emergence percentage of A. nummularia seeds (40.5%) in the sand at a constant water level of 10°C. Stevens et al. (2006) found that the emergence percentage was considerably higher when seeds were sown at 8 mm depth in a temperature regime during the day and night of 19.4–4.4°C compared with only 5% emergence in field trials (Stevens et al., 2006). However, their field experiment was performed in September, when the temperatures are less optimal with fewer rainfalls than in the winter months.
Despite having similar germination at 10°C the emergence percentage was significantly higher for R. preissii (65.8% of seedlings emerged). At 10°C, there were approximately 200 h (8 days) from the first seed germinated until the first seedling emerges. The time to emergence was roughly the same for both species and each seed size fraction.
An explanation why clean seeds do not establish seedlings when they are sown in the field could be that the tiny seeds start germinating when rainfall occurs but dry out before the next rainfall. The small seed size and the consequent requirement for a low seeding depth makes the seeds prone to water stress in the early developmental stages. Our results show that despite providing optimal temperature and water availability for germination of A. nummularia the emerged seedling only represents 50% of the viable seeds, which germinated at 10°C in the controlled environment. The seeding depth might be responsible for the reduction in emergence percentage of A. nummularia. Light only increases A. nummularia germination by approximately 12%. A related species, A. amnicola, was not negatively affected by increasing the sowing depth from 5 to 10 mm (Stevens et al., 2006).
Recommendations for direct seeding based on germination requirements
Farmers should initiate sowing operations in autumn to improve the success of direct seeding by utilizing the winter rains ensuring root development and establishment before the next dry period occurs. Sowing in the autumn should occur after the first rains have washed out most salts from the topsoil. For species growing in arid and semi-arid regions, germination usually occurs after the rain periods when the surface soil salinity has declined by the leaching of salts (Khan and Ungar, 1999). Especially A. nummularia can be sown successfully in soils with relatively high soil salinity (up 200 mM NaCl) without reducing the germination. Rhagodia preissii, on the other hand, has a narrower window for optimal germination due to lower salinity tolerance and low tolerance of temperatures above 10°C. Hence, it might be challenging to successfully establish R. preissii by direct seeding on clay soils because the salinity level of the soil solution may not be low enough before temperatures are too high for optimal germination.
However, earlier sowing also exposed the seeds and young plants to colder temperatures and occasional night frost during winter, compared to sowing in late winter or the spring. Both species are known to be frost tolerant, but the growth and survival rate of seedlings grown from sown seeds compared with 4 months old nursery seedlings remains to be clarified. Seeds adapted to germinate at a temperature optimum of 10°C, which occurs during winter, should also be able to persist and grow during this season. Experiments with seedlings of A. nummularia have indeed shown that seedling growth was less affected by high salinity levels at cold temperatures (Uchiyama, 1987).
Sowing during the autumn may be constrained by competing priorities like the sowing of annual crops. If the successful establishment of these shrubs through direct seeding are to be achieved, the importance of the timing of sowing operations needs to be communicated to farmers, extension staff and researchers intending to establish these shrubs.
Conclusion
The seeds of both species had high viability of roughly 80% when optimal conditions were present. Seeds of A. nummularia and R. preissii had optimal germination temperatures at 10°C under non-saline conditions, confirming the proposed hypothesis. Seeds of A. nummularia were found to have a high salinity threshold only with significant reductions in germination at 500 mM NaCl. Rhagodia preissii germination was more sensitive to temperatures above or below 10°C and at salinities above 50 mM than A. nummularia. Experiments combining temperature and salinity levels are needed to estimate the combined effect of the two factors.
In contrast to expectations, no correlation was found between seed weight and total germination and healthy seedling development for both species when the seed weight was above 0.75 mg for A. nummularia and 0.5 mg for R. preissii. Likewise, there was no correlation between seed size and emergence for seeds sown in the sand at a depth of 5 mm. Instead of selecting only the largest and heaviest seeds, the smallest and lightest should be avoided for sowing. The emergence percentage of R. preissii corresponded to the number of viable seeds, but only 50% of the viable seeds of A. nummularia emerged due to unknown factors. Based on the required temperatures for optimal germination and the salinity tolerance of the seeds of the two species, sowing operations are recommended to be performed early during the winter months of June and July in WA. Sowing of R. preissii would be recommended to be performed on soils with less salinity or later in the winter.
Data availability statement
The original contributions presented in the study are included in the article/supplementary material, further inquiries can be directed to AC, aslakheuser@gmail.com.
Author contributions
AC did the experimental work and the statistics and wrote the first draft for the article. HN and CA supervised the work during the project and improved the manuscripts. All authors contributed to the article and approved the submitted version.
Funding
The research was partially funded by University of Copenhagen and by scholarships from William Demant Foundation, Knud Højgaards Foundation, and Ingeniør Svend G. Fiedler og Hustrus foundation to Promote Botanic Research. The saltbush and rhagodia improvement project is supported by Meat and Livestock Australia and Australian Wool Innovation.
Acknowledgments
We thank Ed Barrett-Lennard (DPIRD), Ian Walsh (producer, Cranbrook) and Kingsley Dixon and Simone Pedrini (Curtin University) for insights. Principal Research Scientist HN who facilitated AC’s stay at the Commonwealth Scientific and Industrial Research Organization (CSIRO) in Floreat, WA made this work possible.
Conflict of interest
The authors declare that the research was conducted in the absence of any commercial or financial relationships that could be construed as a potential conflict of interest.
Publisher’s note
All claims expressed in this article are solely those of the authors and do not necessarily represent those of their affiliated organizations, or those of the publisher, the editors and the reviewers. Any product that may be evaluated in this article, or claim that may be made by its manufacturer, is not guaranteed or endorsed by the publisher.
Footnotes
References
Andreasen, C., Kemezys, A. H., and Muller, R. (2014). The effect of fertilizer level and foliar-applied calcium on seed production and germination of Gerbera Hybrida. Am. Soc. Hortic. Sci. 49, 538–543. doi: 10.21273/HORTSCI.49.5.538
Aronson, J. A., Pasternak, D., and Danon, A. (1988). “Introduction and first evaluation of 120 halophytes under seawater irrigation,” in Arid Lands Today and Tomorrow: Proceedings of an International Research and Development Conference. eds. E. E. Whitehead, C. F. Hutchinson, B. N. Timmerman, and R. G. Varady (Boulder, CO: Westview Press), 737–746.
Barret-Lennard, E. G., Frost, F., Vlahos, S., and Richards, N. (1991). Revegetating salt-affected land with shrubs. J. Depart. Agriculture, Western Australia 32, 124–129.
Barrett-Lennard, E. G., Norman, H. C., and Dixon, K. (2016). Improving saltland revegetation through understanding the “recruitment niche”: potential lessons for ecological restoration in extreme environments. Restor. Ecol. 24, S91–S97. doi: 10.1111/rec.12345
Bell, D. T. (1994). Interaction of fire, temperature and light in the germination response of 16 species from the Eucalyptus marginata forest of South-Western Western Australia. Austr. J. Bot. 42, 501–509. doi: 10.1071/BT9940501
Bell, D. T. (1999). The process of germination in Australian species. Austr. J. Bot. 47, 475–517. doi: 10.1071/bt98007
Ben Salem, H., Norman, H. C., Nefzaoui, A., Mayberry, D. E., Pearce, K. L., and Revell, D. K. (2010). Potential use of oldman saltbush (Atriplex nummularia Lindl.) in sheep and goat feeding. Small Ruminant Res. 91, 13–28. doi: 10.1016/j.smallrumres.2009.10.017
Caccetta, P., Dunne, R., George, R., and Mcfarlane, D. (2010). A methodology to estimate the future extent of dryland salinity in the southwest of Western Australia. J. Environ. Qual. 39, 26–34. doi: 10.2134/jeg2009.0037
Commander, L. E., Merritt, D. J., Rokich, D. P., and Dixon, K. W. (2009). Seed biology of Australian arid zone species: germination of 18 species used for rehabilitation. J. Arid Environ. 73, 617–625. doi: 10.1016/j.jaridenv.2009.01.007
Corwin, D. L. (2021). Climate change impacts on soil salinity in agricultural areas. Eur. J. Soil Sci. 72, 842–862. doi: 10.1111/ejss.13010862CORWIN
Daliakopoulos, I. N., Tsanis, I. K., Koutroulis, A. G., Kourgialas, N., Varouchakis, E. A., Karatzas, G. P., et al. (2016). The threat of soil salinity: a European scale review. Sci. Total Environ. 573, 727–739. doi: 10.1016/j.scitotenv.2016.08.177
Furby, S., Caccetta, P., and Wallace, J. (2010). Salinity monitoring in Western Australia using remotely sensed and other spatial data. Environ. Qual. 39, 16–25. doi: 10.2134/jeq2009.0036
Gallagher, J. L. (1985). Halophytic crops for cultivation at seawater salinity. PLSOA 89, 323–336. doi: 10.1007/978-94-009-5111-2_22
Glenn, E. P., Brown, J. J., Salt, B. E., and Tolerance and Crop Potential of Halophytes (1999). Salt tolerance and crop potential of halophytes. Crit. Rev. Plant Sci. 18, 227–255. doi: 10.1080/07352689991309207
Gul, B., Ansari, R., Flowers, T. J., and Khan, M. A. (2013). Germination strategies of halophyte seeds under salinity. Environ. Exp. Bot. 92, 4–18. doi: 10.1016/j.envexpbot.2012.11.006
Hollington, P. A., Hussain, Z., Kahlown, M. A., and Abdullah, M. (2001). Success stories in saline agriculture in Pakistan: from research to production and development. In BAC Saline Agriculture Conference, March, 19–21, 2001.
Jensen, S. M., Andreasen, C., Streibig, J. C., Keshkar, E., and Ritz, C. (2017). A note on the analysis of germination data from complex experimental designs. Seed Sci. Res. 27, 321–327. doi: 10.1017/S0960258517000228
Khan, M., and Gul, B. (2006). “Halophyte seed germination,” in Ecophysiology of High Salinity Tolerant Plants. Tasks for Vegetation Science. eds. M. Khan and D. Weber (Dordrecht: Springer).
Khan, M. A., Gul, B., and Weber, D. J. (2002). Seed germination in the Great Basin halophyte Salsola iberica. Can. J. Bot. 80, 650–655. doi: 10.1139/b02-046
Khan, M. A., and Ungar, I. A. (1999). Effect of salinity on seed germination of Triglochin maritima under various temperature regimes. Great Basin Nat. 59, 144–150.
Kotze, A. C., Zadow, E. N., Vercoe, P. E., Phillips, N., Toovey, A., Williams, A., et al. (2011). Animal grazing selectivity and plant chemistry issues impact on the potential of Rhagodia preissii as an anthelmintic shrub. Parasitology 138, 628–637. doi: 10.1017/S0031182010001769
Le Houerou, H. N. (1992). The role of saltbushes (Atriplex spp.) in arid land rehabilitation i n the Mediterranean Basin: a review. Agrofor. Syst. 18, 107–148. doi: 10.1007/BF00115408
Li, L., and Zhang, X. (2007). Germination strategies of two halophytes in the salt desert of northwestern China. Sci. China Ser. D Earth Sci. 50, 115–121. doi: 10.1007/s11430-007-5004-7
Louhaichi, M., Hassan, S., Missaoui, A. M., Ates, S., Petersen, S. L., Niane, A. A., et al. (2019). Impacts of bracteole removal and seeding rate on seedling emergence of halophyte shrubs: implications for rangeland rehabilitation in arid environments. Rangeland J. 41:33. doi: 10.1071/RJ18064
Louhaichi, M., Yigezu, Y. A., Werner, J., Dashtseren, L., El-Shater, T., and Ahmed, M. (2016). Financial incentives: possible options for sustainable rangeland management? J. Environ. Manag. 180, 493–503. doi: 10.1016/j.jenvman.2016.05.077
Malcolm, C. V., Hillman, B. J., Swaan, T. C., Denby, C., Carlson, D., and D'antuono, M. (1982). Black paint soil amendment and mulch effects on chenopod establishment in a saline soil. J. Arid Environ. 5, 179–189. doi: 10.1016/S0140-1963(18)31548-9
Masters, D. G., Norman, H. C., and Barret-Lennard, E. G. (2005). Agricultural systems for saline soil: the potential role of livestock. Asian-Austr. J. Animal Sci. 18, 296–300. doi: 10.5713/ajas.2005.296
Muirhead, D. (2017). Rhagodia [Online]. Available at: https://www.inaturalist.org/photos/13558521: https://www.inaturalist.org/ (Accessed July 05, 2022).
Norman, H. C., Masters, D. G., and Barrett-Lennard, E. G. (2013). Halophytes as forages in saline landscapes: interactions between plant genotype and environment change their feeding value to ruminants. Environ. Exp. Bot. 92, 96–109. doi: 10.1016/j.envexpbot.2012.07.003
Norman, H. C., Wilmot, M. G., Hulm, E., and Young, P. (2017). Developing perennial shrubs to fill feed gaps on marginal soils in Australia. In 19th Symposium of the European Grassland Federation; May 7−10, 2017; Alghero, Sardinia, Italy, 79–81.
Revell, D. K., Norman, H. C., Vercoe, P. E., Phillips, N., Toovey, A., Bickell, S., et al. (2013). Australian perennial shrub species add value to the feed base of grazing livestock in low- to medium-rainfall zones. Anim. Prod. Sci. 53, 1221–1230.
Ritz, C., Pipper, C. B., and Streibig, J. C. (2013). Analysis of germination data from agricultural experiments. European J. Agron. 45, 1–6. doi: 10.1016/j.eja.2012.10.003
Ritz, C., and Streibig, J. C. (2005). Bioassay analysis using R. J. Stat. Softw. 12, 1–22. doi: 10.18637/jss.v012.i05
Stevens, J. C., Barrett-Lennard, E. G., and Dixon, K. W. (2006). Enhancing the germination of three fodder shrubs (Atriplex amnicola, A. nummularia, A. undulata; Chenopodiaceae): implications for the optimisation of field establishment. Aust. J. Agricul. Res 57:1279. doi: 10.1071/AR06031
Turner, C. N., Molyneux, N., Yang, S., Xiong, Y., and Siddique, K. H. M. (2011). Climate change in south-West Australia and north-West China: challenges and opportunities for crop production. Crop Pasture Sci. 62, 445–456. doi: 10.1071/CP10372
Uchiyama, Y. (1981). Studies on the germination of saltbushed. 1. The relationship between temperature and germination of Atriplex nummularia lindl. Japanese J. Tropical Agri. 25, 62–67. doi: 10.11248/JSTA1957.25.62
Uchiyama, Y. (1987). Salt tolerance of Atriplex nummularia. Tech. Bull. Trop. Agric. Res. Center 22, 1–69.
Ungar, I. (2001). Effect of bracteoles on seed germination and dispersal of two species of Atriplex. Annals Bot. 87, 233–239. doi: 10.1006/anbo.2000.1321
Wicke, B., Smeets, E., Dornburg, V., Vashev, B., Gaiser, T., Turkenburg, W., et al. (2011). The global technical and economic potential of bioenergy from salt-affected soils. Energy Environ. Sci. 4, 2669–2681. doi: 10.1039/c1ee01029h
Keywords: dryland crops, dryland salinity, infertile land, salinity threshold, saltbush
Citation: Christiansen AHC, Norman HC and Andreasen C (2022) Utilization of the halophytic shrubs Atriplex nummularia Lindl and Rhagodia preissii Moq as crops in salt-affected semi-arid regions: How temperature, salinity, seed weight and size affect seed germination. Front. Plant Sci. 13:989562. doi: 10.3389/fpls.2022.989562
Edited by:
Dorin Gupta, The University of Melbourne, AustraliaReviewed by:
Pedro José Correia, University of Algarve, PortugalValeria Cavallaro, National Research Council (CNR), Italy
Copyright © 2022 Christiansen, Norman and Andreasen. This is an open-access article distributed under the terms of the Creative Commons Attribution License (CC BY). The use, distribution or reproduction in other forums is permitted, provided the original author(s) and the copyright owner(s) are credited and that the original publication in this journal is cited, in accordance with accepted academic practice. No use, distribution or reproduction is permitted which does not comply with these terms.
*Correspondence: Christian Andreasen, can@plen.ku.dk