- 1College of Resources and Environment, Zhongkai University of Agriculture and Engineering, Guangzhou, China
- 2College of Natural Resources and Environment, South China Agricultural University, Guangzhou, China
- 3School of Agriculture, Food and Wine, The University of Adelaide, Adelaide, SA, Australia
- 4Provincial Key Laboratory of Plant Molecular Breeding, South China Agricultural University, Guangzhou, China
- 5Institute of Biological Sciences, University of Talca, Talca, Chile
- 6Centro de Genómica y Bioinformática, Facultad de Ciencias, Universidad Mayor, Santiago, Chile
- 7College of Plant Science and Technology, Huazhong Agricultural University, Wuhan, China
- 8Department of Soil Science, Faculty of Agriculture and Environmental Sciences, The Islamia University of Bahawalpur, Bahawalpur, Pakistan
- 9Department of Botany and Microbiology, College of Science, King Saud University, Riyadh, Saudi Arabia
- 10Department of Environmental Sciences and Engineering, Government College University, Faisalabad, Pakistan
- 11Department of Biological Sciences and Technology, China Medical University, Taichung, Taiwan
Copper (Cu2+) toxicity can inhibit plant growth and development. It has been shown that silicon (Si) can relieve Cu2+ stress. However, it is unclear how Si-nanoparticles (SiNPs) relieve Cu2+ stress in wheat seedlings. Therefore, the current study was conducted by setting up four treatments: CK, SiNP: (2.5 mM), Cu2+: (500 µM), and SiNP+Cu2+: (2.5 mM SiNP+500 µM Cu2+) to explore whether SiNPs can alleviate Cu2+ toxicity in wheat seedlings. The results showed that Cu2+ stress hampered root and shoot growth and accumulated high Cu2+ concentrations in roots (45.35 mg/kg) and shoots (25.70 mg/kg) of wheat as compared to control treatment. Moreover, Cu2+ treatment inhibited photosynthetic traits and chlorophyll contents as well as disturbed the antioxidant defense system by accumulating malondialdehyde (MDA) and hydrogen peroxidase (H2O2) contents. However, SiNPs treatment increased root length and shoot height by 15.1% and 22%, respectively, under Cu2+ toxicity. Moreover, SiNPs application decreased MDA and H2O2 contents by 31.25% and 19.25%, respectively. SiNPs increased non-enzymatic compounds such as ascorbic acid-glutathione (AsA-GSH) and enhanced superoxide dismutase (SOD), peroxidase (POD), catalase (CAT), and ascorbic peroxidase (APX) activities by 77.5%, 141.7%, 68%, and 80%, respectively. Furthermore, SiNPs decreased Cu2+ concentrations in shoots by 26.2%, as compared to Cu2+ treatment alone. The results concluded that SiNPs could alleviate Cu2+ stress in wheat seedlings. The present investigation may help to increase wheat production in Cu2+ contaminated soils.
Introduction
In agroecosystems, heavy metals are continuously accumulated in the soil and water. The phenomenon can be attributed to several anthropogenic activities. This includes modern farming practices, such as extensively exploiting the resources of the earth and industrialization (Zhang et al., 2019a). Copper (Cu2+), as a micronutrient, is essential for plant growth and development (Droppa and Horváth, 1990) and has a wide range of applications in metal manufacturing and pesticide production, which has attracted much attention in recent years (Saleem et al., 2020). Cu2+ is essential for metabolism, enzymes, and proteins, and maintains structural and catalytic activity during normal plant growth (Emamverdian et al., 2015). In addition, the accumulation of Cu2+ in plants has the potential to pose a serious threat to human health through the food chain. Cu2+ is ranked fourth among the most widely distributed inorganic pollutants according to a report published by the Chinese Ministry of Environment Protection. The assessment of agricultural soil for Cu2+ contamination at 1731 sites nationwide found that 21.02% of the sampling sites surpassed the screening value (50 mg/kg) (Li et al., 2020). In soil, Cu2+ concentrations are in the range of 14–109 mg/kg while 5–30 mg/kg Cu2+ of plant DW is regarded as ideal for crop productivity (Kabata-Pendias, 2000). However, Cu2+ toxicity can negatively affect the cellular and physiological developmental processes of plant metabolism (Peñarrubia et al., 2015). Cu2+ toxicity not only leads to the accumulation of reactive oxygen species (ROS) but also hinders plant growth (Hall, 2002; Adrees et al., 2015a). Cu2+ stress induces oxidative stress (oxidative metabolism) and destroys macromolecules and even causes cell death in severe cases (Noctor et al., 2018). Sulfhydryl groups in membrane proteins can be affected by excessive Cu2+ stress, resulting in the deformations of cell membranes (Emamverdian et al., 2015). The only way for organisms to survive deleterious oxidative stress is through evolving an effective and efficient system of antioxidants, e.g., superoxide dismutase (SOD), catalase (CAT), peroxidases (POD), proline, carotenoids, glutathione (GSH) and ascorbic acid (AsA) (Das and Roychoudhury, 2014; Hasan et al., 2018; Ali et al., 2022). For optimal growth, it is essential to coordinate antioxidants (enzymatic and non-enzymatic) to maintain ROS levels at stable levels, especially under metal contaminations (Noctor et al., 2018). Consequently, the defense system is enhanced in the plant body with the help of exogenous chemicals (Gill and Tuteja, 2010) which is an effective way of fighting unnecessary ROS levels caused by metal contamination (Yi et al., 2018; Zhang et al., 2019b). Therefore, it is necessary to implement remedial measures and/or strategies to reduce Cu2+ accumulation and uptake in plants, thus ensuring crop productivity and reducing possible health risks caused by Cu2+ accumulation.
Silicon (Si) accounts for 28% of the earth’s crust and is found as silica, silicic acid, and silicates (Singer and Munns, 1987). It has been reported that exogenous Si treatment ameliorates heavy metal stress by inducing antioxidative responses in the plant body (Emamverdian et al., 2018; Bhat et al., 2019). Silicon improves the defense system by reducing ROS and oxidative stress (Adrees et al., 2015b; Anwaar et al., 2015; Sabir et al., 2022). Silicon addition increased plant growth, development, and membrane stability under heavy metal stress (Rahman et al., 2017). As a regulator, Si reduces metal uptake and transport by plants and improves physiological adaptability and resource usage under heavy metal stress (Ahmad et al., 2021). Nanotechnology has become a valuable tool for agriculture (Jiang et al., 2021). There is no doubt that it has found widespread application in agriculture due to sustainable agriculture and precise farming (Prasad et al., 2017). Several studies have demonstrated the potential benefits of silicon nanoparticles (SiNPs) in the past few years on plant growth by mitigating abiotic and biotic stresses occurring in plants due to adverse environmental factors (Roychoudhury, 2020). It has been shown that SiNPs can be more effective in reducing heavy metal toxicity in plants in comparison with normal Si (Bhat et al., 2019).
Throughout the world, wheat (Triticum aestivum L.) is the second most cultivated crop and is found in almost all countries. Cu2+ is important in wheat production. However, Cu2+ toxicity can inhibit wheat productivity and Cu2+ can be readily transferred to the grain. In contrast, little information is available on the role of SiNPs in plant tolerance to Cu2+. The mechanism for reducing Cu2+ toxicity in wheat seedlings through SiNPs application remains unknown. Silicon can play a significant role in soil remediation, hence a deeper knowledge of SiNPs and Cu2+ absorption and combined action is needed. Therefore, the objectives of this study were to investigate how SiNPs can play a beneficial role in reducing Cu2+ toxicity by enzymatic (SOD, POD, CAT) and non-enzymatic (AsA-GSH) antioxidant defense systems, and to study how SiNPs can reduce Cu2+ concentrations in plant tissues, which may help to reduce Cu2+ toxicity in wheat seedlings by improving growth parameters. The results of this study may help to increase the yield of wheat in Cu2+ contaminated soil.
Methods and materials
Plant material and experimental procedures
Wheat (Triticum aestivum) “cultivars-97003” was used as test material. The wheat seedlings were grown in hydroponics at South China Agriculture University, China. The seeds were washed with 10% H2O2 for 20 min and then rinsed with distilled water three times. Seeds were spread on wet clothes for germination. After one week of emergence, seedlings were transferred to pots filled with ¼ nutrient strength for a week and then subsequently 1/3 and finally to full strength nutrient solution (mM); “2.0 (NH4)2SO4, 12.0 NaNO3, 1.0 NaH2PO4·2H2O, 3.0 K2SO4·5H2O, 3.0 MgSO4·2H2O, 4.0 CaCl2, (μM), 9.15 MnCl2·4H2O, 0.32 CuSO4·5H2O, 0.77 ZnSO4·7H2O, 0.02 (NH4)6Mo7O24·4H2O, 46.26 H3BO3, and 20.0 FeSO4·7H2O-EDTA”. The 12 seedlings were planted in a 2 litter pot, totaling 16 pots. The following treatments were set up in the experiment, (i) CK: normal nutrient solution; (ii) SiNP: 2.5 mM; (iii) Cu2+: 500 µM (iv) SiNP+Cu2+: 2.5 mM+500 µM. Copper was applied as CuSO4.5H2O. The experiment had 4 treatments and each treatment was replicated four times in a completely randomized design. The experimental treatments were selected based on our trial experiments where we used different concentrations of Cu2+ and SiNP and then finally selected suitable treatments (500 µM Cu2+ and 2.5 mM SiNP) for the current experiment. During the present study, we used silicon nanoparticles (SiNPs), which have been characterized in our previous study (Riaz et al., 2022). The morphology of SiNP was almost spherical and its size was between 12-17 nm. Every three days, the nutrient solution was changed throughout the experiment, pH was maintained at 6.5 daily and samples were taken out after two weeks. In order to clean the roots of the seedling, distilled water was used to wash the surface after taking out the seedlings. The water in the roots of the seedlings was gently blotted with absorbent paper, and the junction of the rhizomes and stems was used as the node to measure the plant height and root length of each wheat plant and recorded in cm. Wheat seedlings were separated from the junction of rhizomes and stems, into the aerial parts and underground parts, and fresh weight (FW) was measured. The samples were stored at -80°C and the remaining samples were oven-dried at 68° C until constant weight.
Photosynthetic parameters involved in gas exchange
A portable infrared gas analyzer was used to record the parameters related to gas exchange “Li-6400, Li-Cor, Inc., Lincoln, NE, USA”. We selected only the leaves that were fully expanded. We measured net photosynthetic rates (Pn), transpiration rates (Tr), internal CO2 concentrations (Ci), and stomatal conductance (gs). During measurements, 360 µmol mol-1 was CO2 concentrations and 1000 µmol m-2 was photosynthetic photon flux density s-1 at 25 °C.
Chlorophyll contents in wheat plants
Chlorophyll contents in leaves were determined by the method of Lichtenthaler (1987). As part of the investigation, we determined the contents of chlorophyll in fully expanded leaf tissues. The 200 mg wheat leaf samples after rinsing with deionized water were chopped and treated with 95% alcohol. The mixture was centrifuged at 3000 rpm for 5 min and then chlorophyll contents were measured by a spectrophotometer at 665, 649, and 470 nm wavelengths.
Contents of MDA and H2O2
To determine the MDA content in the samples, the method of De Vos et al. (1991) was used. The 0.5 g of root sample was measured and after homogenizing the samples, 8 mL of 50 mmol/L PBS (PH=7.8) was added and centrifuged at 10000 r/min for 10 min. Next, 2.5 ml of TBA solution was added and plugged with a stopper, placed in boiling water for 20 min at 98 °C, and centrifuged at 4000 r/min for 10 min after cooling. The absorbance values were measured at 532, 600, and 450 nm wavelengths.
For the measurement of H2O2 content, the method reported by Velikova et al. (2000) was used. Briefly, liquid nitrogen was used to crush fresh root samples (0.2 g) and homogenized with a trichloroacetic acid solution of 0.1% (1 mL) and after that homogenate was centrifuged at 12,000 r/min for 20 min (4°C) and the supernatant was collected. In the reaction mixture, 1 mL of BPS (pH 6.8, 100 mM) was added, as well as 0.5 mL of supernatant containing 1 mL of KI (1M). The contents of H2O2 were measured employing a spectrophotometer (UV-VIS 2550, Shimadzu, Japan) at 390 nm wavelength.
The determination of SOD, POD, CAT, and APX activities
The superoxide dismutase (SOD) activity was determined according to the method of Beauchamp and Fridovich (1971). The 0.5 g root sample was homogenized with PBS (PH 7.8) and centrifuged at 10,000 r/min for 10 min at 4°C. Subsequently, supernatant and reagents were mixed and shaken at 4°C and placed under fluorescent light for 25 min. The light-proof test tube was used as a blank, and the absorbance value was measured at 560 nm by a spectrophotometer.
The determination of POD activity refers to the method of Cai et al. (2008). The 0.5 g of root samples were taken into a centrifuge tube and then 10 mL of PBS (PH 7.8) was added and homogenized by a cooled mortar and pestle. The homogenate was centrifuged at 8000 r/min for 10 min at 4°C, and then the supernatant was taken and mixed with the reaction solution. The absorbance was measured at 470 nm by spectrophotometer, and the reading was taken at 30s, 90s, and 150s. CAT activity was measured as described by Aebi (1983).
To determine the activity of APX, the method of Nakano and Asada (1981) was used. For this, 0.5 g of root sample was homogenized in a liquid N2 and taken into a 15 mL centrifuge tube. Then, 10 mL of pre-cooled extract was added with the reagent and centrifuged at 15000 r/min, 4°C for 15 min, and the supernatant was used for the determination of APX activity at 290 nm by spectrophotometer.
Measurement of non-enzymatic antioxidants
Ascorbate (AsA) and contents of dehydroascorbate (DHA) were measured as reported by Gillespie et al. (2007) with some modifications. Briefly, the roots were homogenized by adding 1.6 mL of 6% trichloroacetic acid (TCA). Afterward, the homogenate was centrifuged at 13,000 rpm for 5 min, 4 ◦C. The absorbance was measured at a wavelength of 525 nm. A standard curve was used to estimate the total ascorbate content of the solution, and DHA concentrations were determined based on deducting the reduced ascorbate value from the total ascorbate value. The contents of glutathione (GSH) and oxidized glutathione (GSSG) were assessed, and detection kits were used for this purpose (A061-1) (Nanjing Jiancheng, China).
Measurement of Cu2+ and Si concentrations in roots and shoots
Oven-dried root and shoot samples of wheat were ground to a fine powder and then 0.3 g of corresponding samples were put in a mixture of HNO3:HClO4 (6 mL) (5:1 v/v) overnight and digested on a hotplate at 120 °C until 1 mL liquid was left (Keller et al., 2015). The liquid was cooled, and the final volume was made up to 50 mL by adding deionized water, and Cu2+ and Si concentrations were measured by Atomic Absorption Spectrophotometer (AAS) “AA6300C, Shimadzu, Japan”. Translocation factor of Cu2+ was calculated as “TF = Metal concentration in shoot (mg/kg DW)/Metal concentrations in roots (mg/kg DW)”.
Statistical analysis
Data were analyzed by one-way ANOVA, and the significant differences among different treatments were compared by LSD test (P<0.05), using the protocols given by Steel et al. (1997). Values are mean ± standard deviation (SD) of at least four replicates and graphed using Origin 8.0 software.
Results
Effect of SiNPs on growth traits of wheat seedlings under Cu2+ stress
The growth characteristic of wheat seedlings under Cu2+ stress is shown in Figure 1. As compared to the control treatment, Cu2+ stress alone significantly reduced root length and shoot height by 27.48% and 29.46%, respectively (Figures 1A, B). On the other hand, under SiNPs+Cu2+ treatment, shoot height increased by 22.04%, and root length increased by 15.61%. Cu2+ treatment had a significant negative effect on the fresh biomass of wheat seedlings and decreased the fresh weight of roots and shoots by 38% and 32.7% respectively. However, SiNPs+Cu2+ treatment reduced the inhibition effect of Cu2+ on the growth parameters of wheat seedlings and increased root and shoot fresh weight (Figures 1C, D).
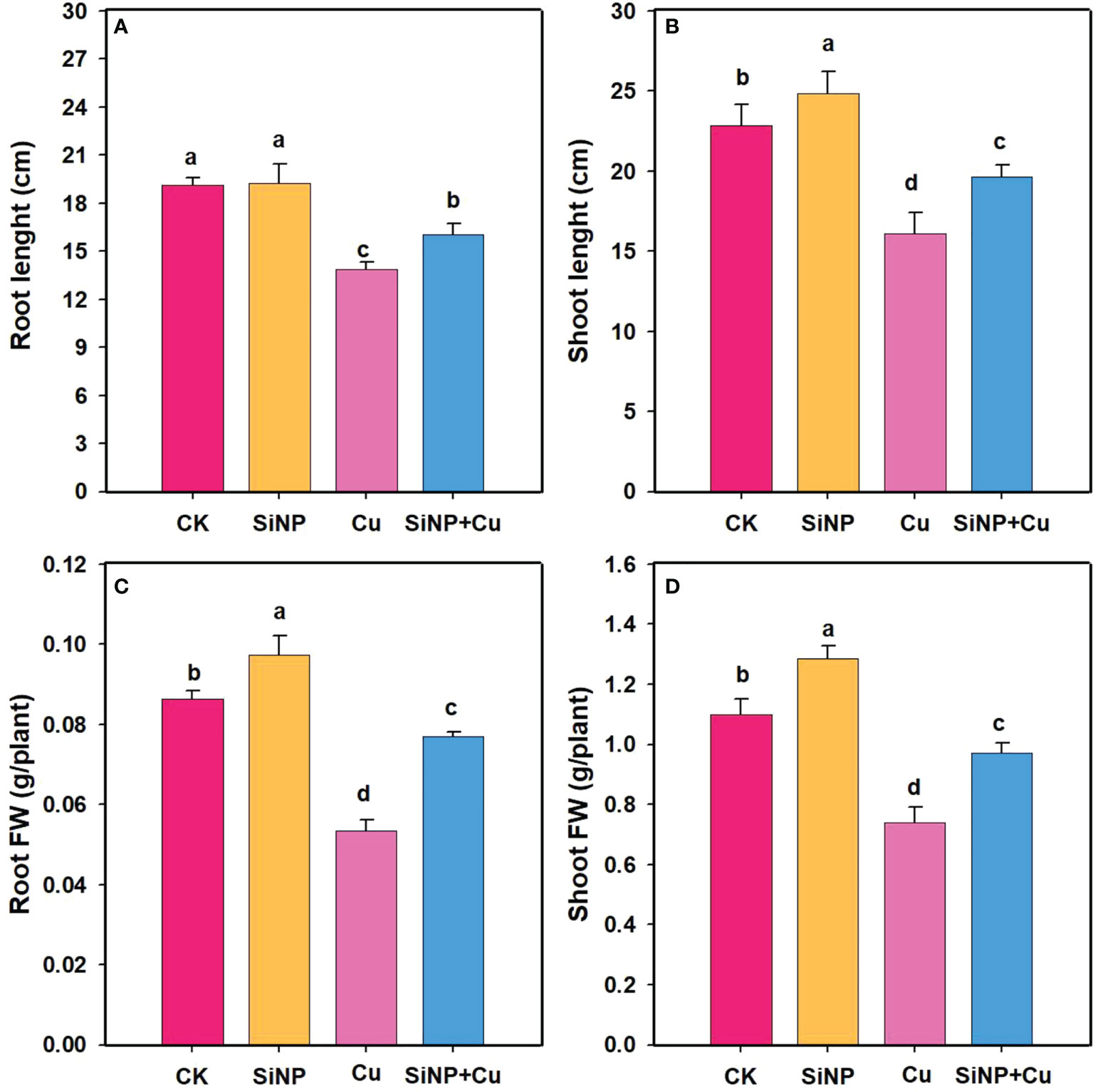
Figure 1 The growth characters of wheat seedlings under Cu2+ stress. Note “Experimental treatments contained: CK (Control); SiNP (2.5 mM SiNPs); Cu2+ (500 µM Cu2+ alone), SiNP+Cu2+ (2.5 mM SiNPs+500 µM Cu2+). The different letters (a, b, c, d) above bars describe the differences in different treatments by LSD test at (P ≤ 0.05)”. (A) root length, (B) shoot length, (C) root FW, (D) shoot FW.
Chlorophyll contents under Cu2+ stress
Chlorophyll contents in leaves of wheat seedlings under each treatment are shown in Figure 2. Cu2+ treatment decreased chlorophyll a by 15.87%, chlorophyll b by 44.68% (Figures 2A, B), carotenoids by 44%, and total chlorophyll by 23.69% compared to the control treatment (Figures 2C, D). Compared to Cu2+ treatment, SiNP+Cu2+ significantly increased chlorophyll a, b, carotenoids, and total chlorophyll contents by 6.6%, 46.15%, 50%, and 21.9%, respectively.
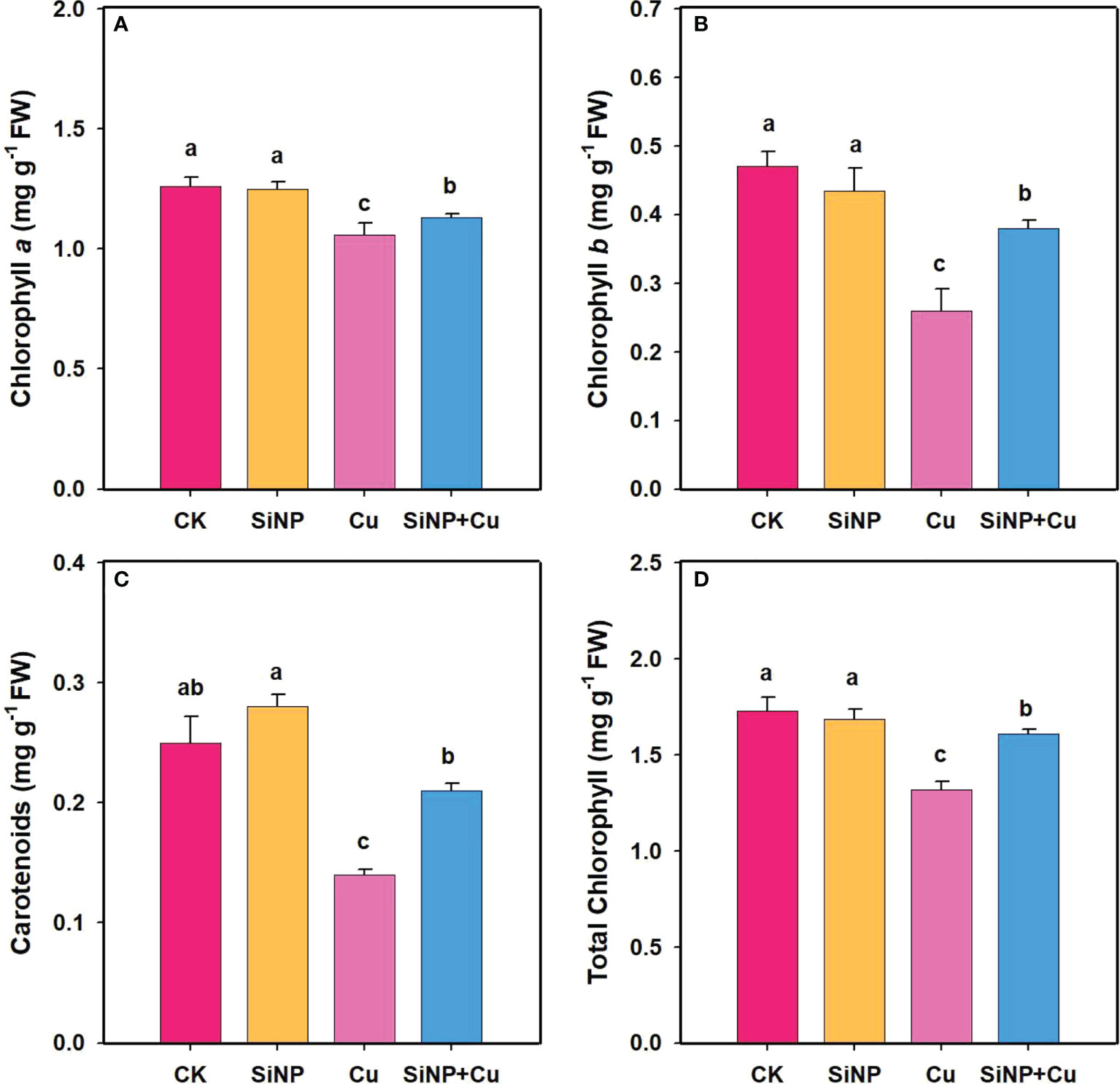
Figure 2 Chlorophyll contents of wheat seedlings under Cu2+ stress. Note “Experimental treatments contained: CK (control); SiNP (2.5 mM SiNPs); Cu (500 µM Cu2+ alone), SiNP+Cu (2.5 mM SiNPs+500 µM Cu2+). The different letters (a, b, c, d) describe the differences in different treatments by LSD test at (P ≤ 0.05)”. (A) chlorophyll a, (B) chlorophyll b, (C) carotenoids, (D) total chlorophyll.
Effects of SiNPs on gas exchange traits of wheat leaves under Cu2+ stress
Compared with the control, Cu2+ treatment alone significantly reduced gas exchange traits of wheat leaves (Figure 3) while SiNP+Cu2+ reversed the effect of Cu2+ on inhibition of gas exchange traits of wheat leaves. SiNPs+Cu2+ treatment increased the Pn (102.63%), gs (71.42%) (Figures 3A, B), and Tr (69.69%) while decreasing Ci (12.33%) of wheat leaves, respectively, compared with Cu2+ alone (Figures 3C, D).
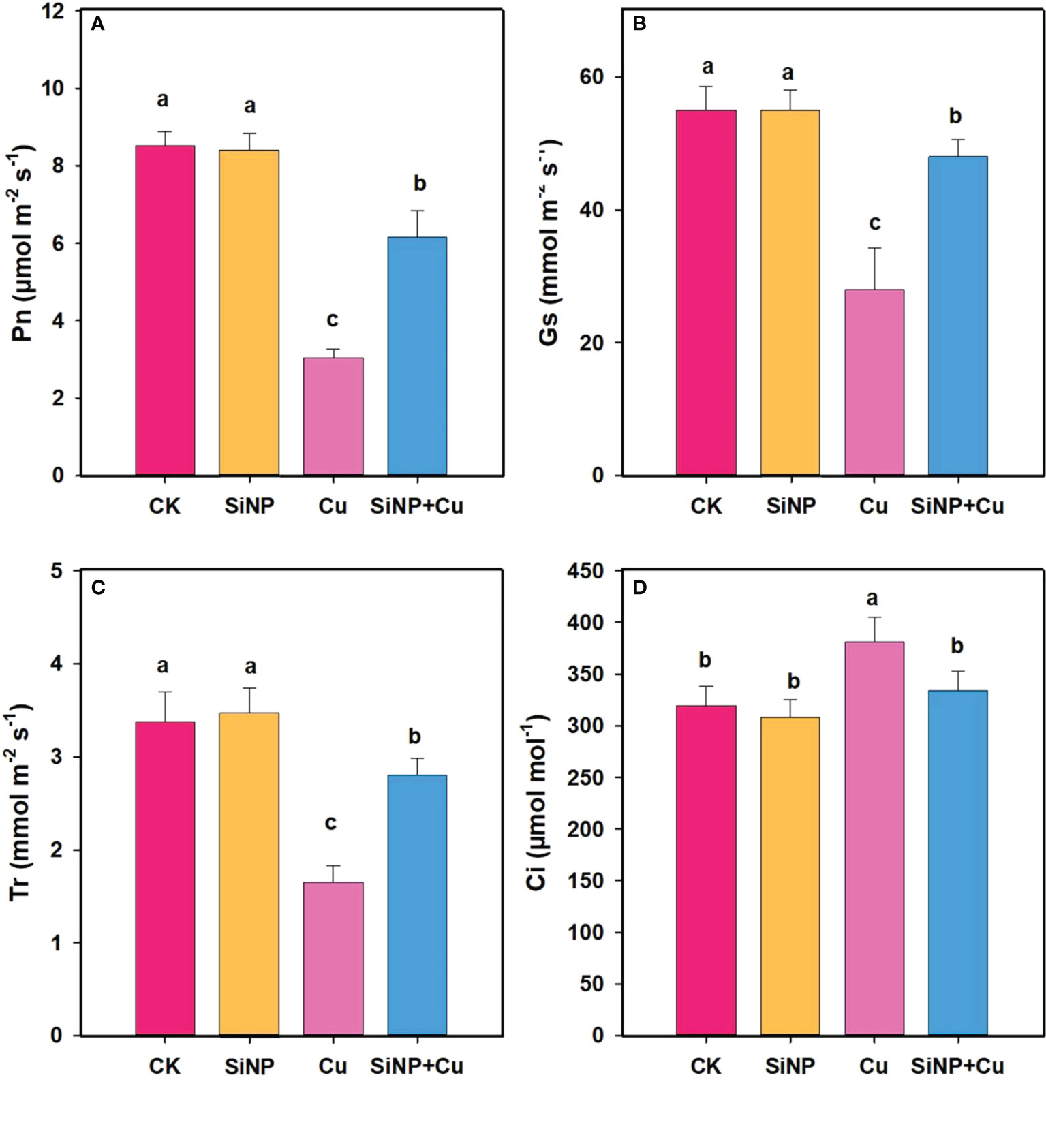
Figure 3 Gas exchange traits under Cu2+ stress. Note “Experimental treatments contained: CK (control); SiNP (2.5 mM SiNPs); Cu (500 µM Cu2+ alone), SiNP+Cu (2.5 mM SiNPs+500 µM Cu2+). The different letters (a, b, c, d) describe the differences in different treatments by LSD test at (P ≤ 0.05)”. (A) Pn, (B) Gs, (C) Tr, (D) Ci.
Effects of SiNPs on MDA and H2O2contents in roots of wheat under Cu2+ stress
Malondialdehyde (MDA) and H2O2 contents in wheat root seedlings were determined, which are presented in Figure 4. The results showed that MDA and H2O2 content were increased by 140.80% and 33.20%, respectively, under Cu2+ treatment alone in comparison to control treatment. However, SiNP+Cu2+ treatment significantly reduced MDA and H2O2 content by 31.25% and 19.25% in the roots of wheat seedlings, respectively, compared with Cu2+ treatment alone, indicating reduced damage to membranes and reduced oxidative stress (Figures 4A, B).
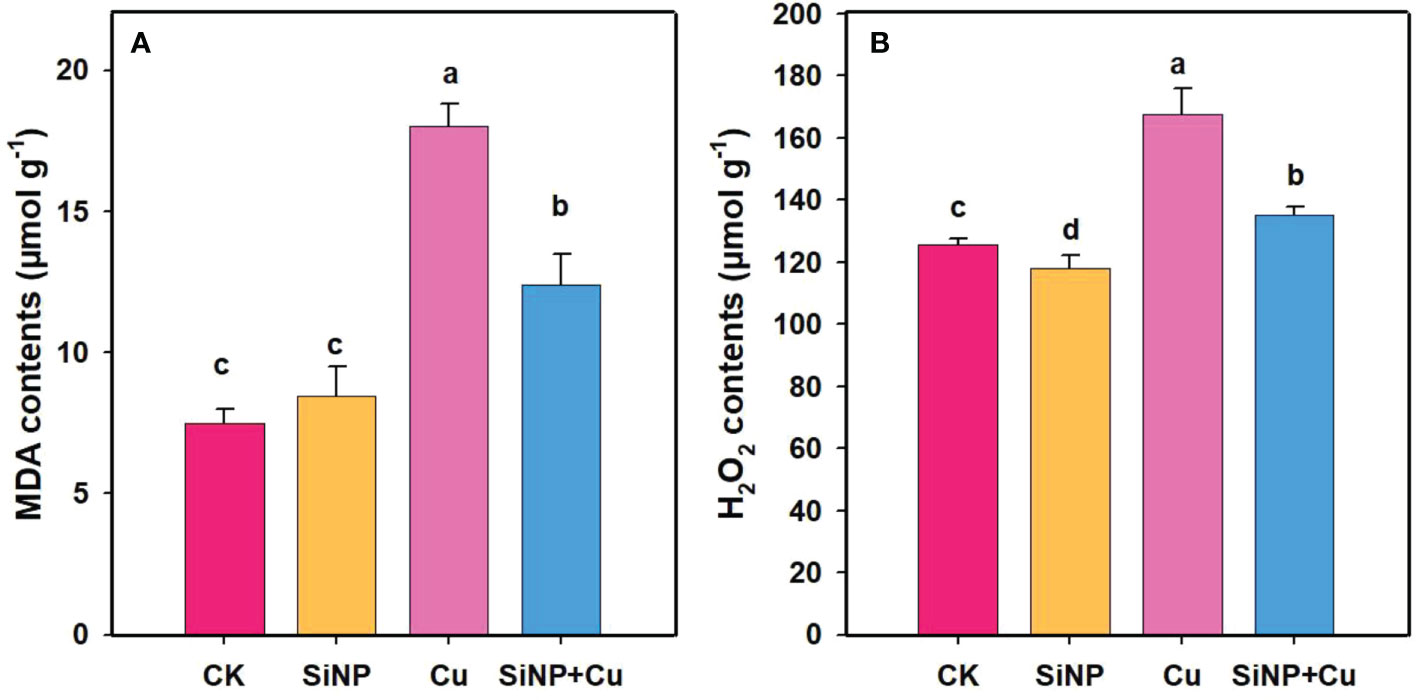
Figure 4 MDA and H2O2 contents of wheat roots under Cu2+ stress. Note “Experimental treatments contained: CK (control); SiNP (2.5 mM SiNPs); Cu (500 µM Cu2+ alone), SiNP+Cu (2.5 mM SiNPs+500 µM Cu2+). The different letters (a, b, c, d) describe the differences in different treatments by LSD test at (P ≤ 0.05)”. (A) MDA contents, (B) H2O2 contents.
Effects of SiNPs on enzymatic activities on roots of wheat under Cu2+ stress
Cu2+ treatment decreased the SOD activity in roots by 22.2% compared to CK (Figure 5). However, SiNP+Cu2+ treatment increased SOD enzyme activity by 77.5% related to Cu2+ treatment alone (Figure 5A). It can be seen from Figure 5 that the POD enzyme activity of wheat roots was inhibited under Cu2+ treatment by 42% while SiNPs+Cu2+ treatment increased POD activity by 141.71% as compared to Cu2+ treatment (Figure 5B). Moreover, SiNP+Cu2+ treatment increased the activity of CAT (Figure 5C) and APX enzymes by 68% and 80% respectively, as compared to Cu2+ treatment alone (Figure 5D).
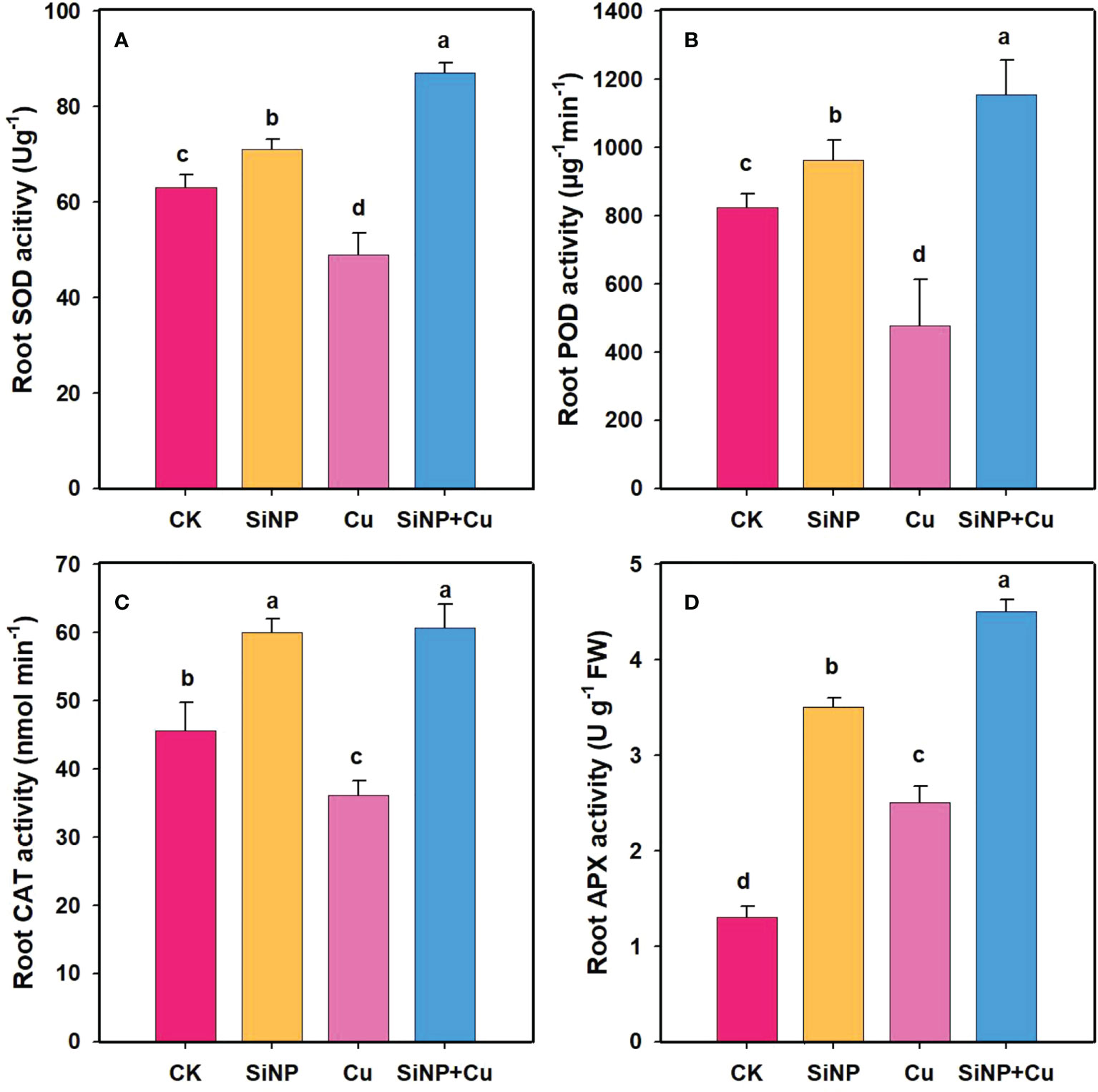
Figure 5 Enzymatic activities under Cu2+ stress. Note “Experimental treatments contained: CK (control); SiNP (2.5 mM SiNPs); Cu (500 µM Cu2+ alone), SiNP+Cu (2.5 mM SiNPs+500 µM Cu2+). The different letters (a, b, c, d) describe the differences in different treatments by LSD test at (P ≤ 0.05)”. (A) SOD, (B) POD, (C) CAT, (D) APX.
Effects of SiNPs on AsA-GSH contents under Cu2+ stress
To explore the role of SiNPs on AsA-GSH contents under Cu2+ stress, the contents of non-enzymatic substances in wheat roots were determined (Figures 7A–D). Compared with control, Cu2+ stress decreased GSH and AsA contents by 9.87% (Figure 6A) and 18.42% (Figure 7A) but increased GSSG and DHA contents by 34.6% and 11.53% in wheat roots. SiNP+Cu2+ treatment significantly increased GSH and AsA contents by 43.39% and 178% and decreased GSSG (Figure 6B) and DHA contents by 24.83% and 50.34%, respectively as compared to Cu2+ treatment alone (Figure 7B).
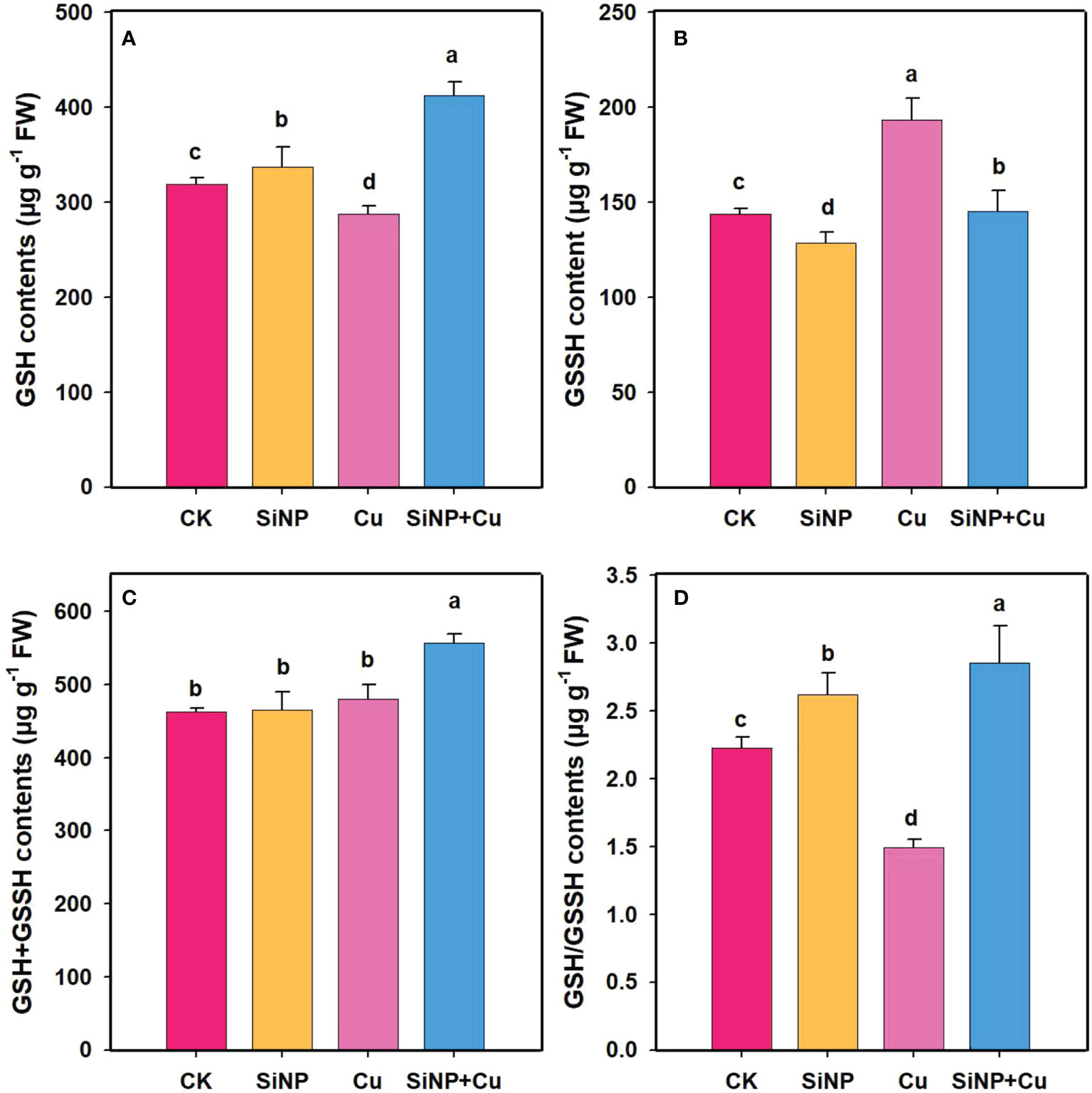
Figure 6 GSH contents under Cu2+ toxicity. Note “Experimental treatments contained: CK (control); SiNP (2.5 mM SiNPs); Cu (500 µM Cu2+ alone), SiNP+Cu (2.5 mM SiNPs+500 µM Cu2+). The different letters (a, b, c, d) describe the differences in different treatments by LSD test at (P ≤ 0.05)”. (A) GSH, (B) GSSH, (C) GSH+GSSH, (D) GSH/GSSH.
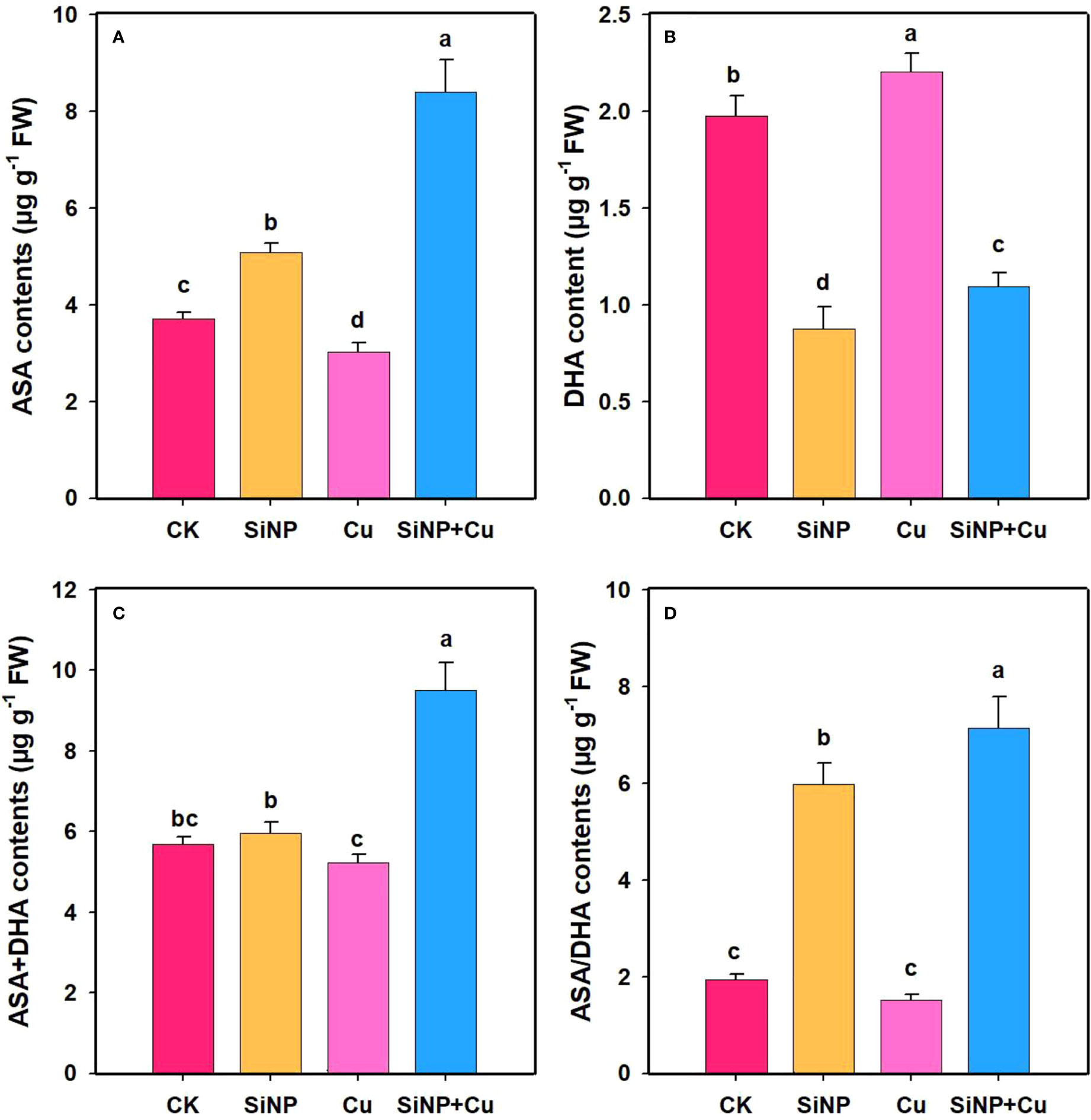
Figure 7 ASA contents under Cu2+ toxicity. Note “Experimental treatments contained: CK (control); SiNP (2.5 mM SiNPs); Cu (500 µM Cu2+ alone), SiNP+Cu (2.5 mM SiNPs+500 µM Cu2+). The different letters (a, b, c, d) describe the differences in different treatments by LSD test at (P ≤ 0.05)”. (A) AsA, (B) DHA, (C) AsA+DHA, (D) AsA/DHA.
Effect of SiNPs on Cu2+ and Si accumulation in various wheat tissues
The contents of Cu2+ in each tissue of wheat seedlings are represented in Figure 8. The results showed that Cu2+ application increased Cu2+ concentration in roots and shoots and the highest concentrations were found in the treatment of Cu2+ (Figures 8A, B). However, the Cu2+ contents in leaves were much lower in SiNP+Cu2+ than that in Cu2+ treatment alone. SiNP+Cu2+ treatment decreased Cu2+ in leaves by 26.29% as compared to Cu2+treatment alone. SiNP+Cu2+ treatment decreased the TF (transfer factor) from 0.56 to 0.31. Moreover, SiNPs addition increased the Si concentrations in both roots and shoots, however, higher concentrations were found in the roots (Figures 8C, D).
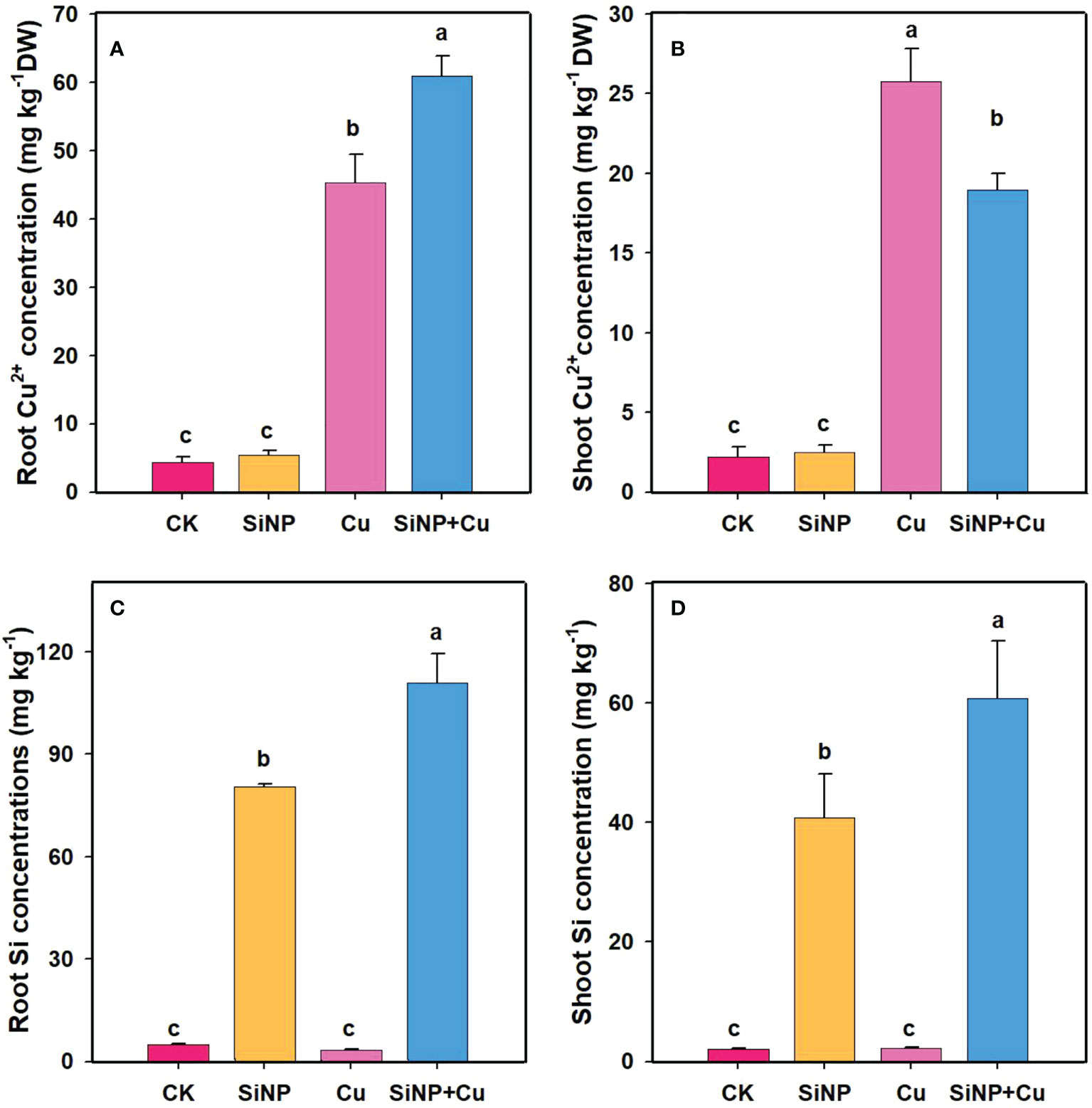
Figure 8 Cu2+ and Si contents of wheat seedlings under Cu2+ stress. Note “Experimental treatments contained: CK (control); SiNP (2.5 mM SiNPs); Cu (500 µM Cu2+ alone), SiNP+Cu (2.5 mM SiNPs+500 µM Cu2+). The different letters (a, b, c, d) describe the differences in different treatments by LSD test at (P ≤ 0.05)”. (A) root Cu2+, (B) shoot Cu2+, (C) Root Si, (D) shoot Si.
Discussion
Heavy metal pollution, particularly Cu2+, adversely affects plant growth and productivity (Keller et al., 2015; Huang et al., 2021). The results of our study showed that Cu2+ stress resulted in a significant decrease in the chlorophyll contents as well as inhibition of root growth compared with the control group. It has been reported that photosynthesis is impaired due to the accumulation of Cu2+ ions and the production of reactive oxygen species (ROS) (Zeng et al., 2021). These reactive oxygen species disrupt the structure of chloroplasts and reduce the activity of key photosynthetic enzymes. Cu2+ toxicity in our study greatly reduced photosynthetic traits including Pn, Tr, and gs. Similar findings were reported by Ashraf and Harris (2013), who observed that photosynthesis was inhibited due to damage caused by heavy metals to photosynthetic pigments. Consistent with their findings, our study found that chlorophyll a and chlorophyll b contents in wheat leaves were significantly reduced under Cu2+ stress. It has been well established that excessive ROS production has been shown to lead to chlorophyll degradation (Tamaka and Tanaka, 2006; Malik et al., 2022). Heavy metal may cause deficiency of essential nutrients and Fe and Mg deficiencies resulting from heavy metal stress (necessary for chlorophyll synthesis) may affect the synthesis of chloroplast in leaves, which may also lead to a decrease in pigment concentration (Seregin and Kozhevnikova, 2006; Zeng et al., 2021). Our results are consistent with the findings of Vieira Filho and Monteiro (2020) which indicated that Si addition increased chlorophyll content under Cu2+ toxicity in Panicum maximum cv. Tanzania. A previous study showed that Si has a significant role in the alleviation of abiotic stress in plants (Zhang et al., 2021). In our study, as a result of exogenous SiNPs, wheat seedlings were able to grow more rapidly and had increased chlorophyll contents. Plants under Cu2+ stress showed a significant decrease in root and shoot FW, plant height, and root length (Figure 1). However, SiNP+Cu2+ treatment increased the FW of roots and shoots as well as shoot height and root length of wheat seedlings (Figure 1). The reason for this increase in plant biomass might be due to decreased uptake and translocation of Cu2+ to shoot of wheat plants. Furthermore, decreased concentration of Cu2+ in wheat tissues also indicates an alleviating effect of SiNPs on Cu2+ toxicity.
It has been shown that heavy metals such as Cu2+ can interfere with the normal metabolism of plants, which can lead to the accumulation of ROS such as and H2O2 (Gill and Tuteja, 2010). High accumulation of ROS can cause lipid peroxidation (MDA) and degradation of biological macromolecules as well as induce a large amount of unsaturated fatty acid production, which eventually leads to cellular oxidative damage (Berni et al., 2019). There have been several mechanisms developed by plants for scavenging ROS accumulation, including antioxidant defense systems such as SOD, POD, and CAT which can maintain cellular homeostasis (Asada, 1992; Li et al., 2011; Antoniou et al., 2017). In addition, it has been shown that abiotic stress can be alleviated by enzymatic and non-enzymatic antioxidants involved in the AsA-GSH cycle (Ahmad et al., 2018). The results of our study demonstrated that Cu2+ toxicity increased membrane lipid peroxidation (MDA) and oxidative stress. Similar results were also reported by Zeng et al. (2021) in wheat under Cu2+ toxicity. In our current study, SiNPs treatment significantly reduced the production of ROS in wheat seedlings which are indicated by decreased MDA and H2O2 contents. The reason for this decrease in MDA and H2O2 contents could be related to the improved antioxidant system which maintained a balance between the generation of ROS and the scavenger of free radicals in the plant during growth and development under heavy metal stress (Singh et al., 2016; Morkunas et al., 2018; Berni et al., 2019). Our results are in line with the study of Kim et al. (2014) who reported that Si improved the antioxidant defense system and reduced membrane peroxidation in rice plants under heavy metal stress.
The activity of SOD is considered one of the most important first-line defenses in plants by dismutating superoxide radicals into hydrogen peroxide (Saleem et al., 2020; Kamran et al., 2021). SiNPs increased the activity of SOD under Cu2+ toxicity. A previous study also reported similar results that Si application under Cu2+ toxicity in Arabidopsis and cotton (Khandekar and Leisner, 2011; Ali et al., 2016). The same trend was also evident in our results with regard to the increase in SOD and CAT activity which could break down H2O2 into water and oxygen (Das and Roychoudhury, 2014). Our results showed that SiNP+Cu2+ increased the activities of CAT, POD, and reduced contents of H2O2 indicating actively converting H2O2 to H2O, hence reducing the oxidative stress caused by Cu2+. In addition, Pirooz et al. (2021) exposed salvia to Cu2+ and found that 1 mmol L- 1 Si application had a positive response in activating CAT and SOD activities. Our results are consistent with the study of Vieira-Filho and Monteiro (2022) that Cu2+ toxicity decreased the antioxidant defense system while the application of Si increased the antioxidant defense system in Tanzania Guinea (Panicum maximum cv. Tanzania) grass. Gilabel et al. (2014) exposed Tanzania guinea grass plants to Cu2+ up to 1000 μmol L−1 which resulted in a drastic decrease in biomass production due to oxidative stress, identified by the increase in the contents of MDA and H2O2. Similar results were reported in rice plants exposed to 100 μmol L−1 Cu2+ (Kim et al., 2014).
It is crucial to maintain an adequate pool of ascorbate (AsA)-glutathione (GSH) under stress conditions (Nahar et al., 2016). AsA is a non-enzymatic and water-soluble antioxidant that interacts directly with ROS in a cell and can reduce the accumulation of ROS in the cell (Al Mahmud et al., 2018). GSH is one of the most important non-antioxidants in the plant body and serves as a stress indicator. In our study, it is interesting to note that a significant increase in SOD, POD, and CAT activities was associated with the increase in AsA-GSH contents under SiNPs (Figure 6). The increase in GSH contents might be related to the complexation and detoxification of Cu2+ in the vacuoles which are also evident in the decreased concentrations of Cu2+ in the upper part of plant tissues. It is interesting to note that concentrations of Cu2+ in the roots of the plant in the treatment of SiNP+Cu2+ were higher than in the treatment of Cu2+ which could be due to the plant tolerance mechanism. Plants retain a high concentration of heavy metals in the roots, therefore, resist their movement and translocation to the upper parts of plants. The non-enzymatic antioxidant glutathione (GSH) produces thiol compounds with relatively low molecular weights (Berni et al., 2019) which not only decrease ROS within the body (Szalai et al., 2009) but are also involved in the formation of phytochelatins (PCs), and complexes with toxic metals in the cytosol. These cytosolic toxins are transported into vacuoles for detoxification, thus reducing the toxicity (Lin et al., 2012; Seth et al., 2012).
Conclusion
The results of the current study showed that Cu2+ stress inhibited root length and shoot height as well as corresponding fresh weights. Moreover, Cu2+ stress caused membrane peroxidation (MDA) and high contents of H2O2 in root tissues which are correlated with high Cu2+concentrations in wheat tissues. However, SiNPs application improved plant growth parameters and also reduced the accumulation of MDA and H2O2 contents by regulating activities of the antioxidant defense system. The improved defense system and reduced Cu2+ concentrations might have led to the increasing growth of wheat seedlings under Cu2+ stress by SiNPs, suggesting a mitigating effect of SiNPs on Cu2+ toxicity. This study provides a valuable reference for the application of exogenous SiNPs to mitigate the Cu2+ toxicity in Cu2+ contaminated soil. Future research should be focused on the natural environment and molecular mechanisms involved in the SiNP-induced alleviation of Cu2+ toxicity should be considered.
Data availability statement
The original contributions presented in the study are included in the article/supplementary material. Further inquiries can be directed to the corresponding authors.
Author contributions
MR and SZ designed and supervised this study; MR and MK conducted the experiments; MR, NUR, AP, and MS performed data interpretation; MR, SZ, and MK drafted the manuscript; FA-H, CM, AA-G, ME, and NUR helped in data validation. MR, FM-P, SA, and SZ critically reviewed the final manuscript. FA-H, SA, SZ, ME, and FM-P supervised the research project. SZ, AA-G, CM, and ME got financial support. All authors read and approved the final manuscript.
Funding
Researchers Supporting Project number (RSP2022R483), King Saud University, Riyadh, Saudi Arabia. This project is financially supported by Science and Technology Program of Guangzhou, China, under the grant number: 202102020229.
Acknowledgments
The authors extend their appreciation to the Researchers Supporting Project number (RSP2022R483), King Saud University, Riyadh, Saudi Arabia, for providing help to publish this research work.
Conflict of interest
The authors declare that the research was conducted in the absence of any commercial or financial relationships that could be construed as a potential conflict of interest.
Publisher’s note
All claims expressed in this article are solely those of the authors and do not necessarily represent those of their affiliated organizations, or those of the publisher, the editors and the reviewers. Any product that may be evaluated in this article, or claim that may be made by its manufacturer, is not guaranteed or endorsed by the publisher.
References
Adrees, M., Ali, S., Rizwan, M., Ibrahim, M., Abbas, F., Farid, M., et al. (2015a). The effect of excess copper on growth and physiology of important food crops: A review. Environ. Sci. Pollut. Res. 22, 8148–8162. doi: 10.1007/s11356-015-4496-5
Adrees, M., Ali, S., Rizwan, M., Zia-Ur-Rehman, M., Ibrahim, M., Abbas, F., et al. (2015b). Mechanisms of silicon-mediated alleviation of heavy metal toxicity in plants: A review. Ecotoxicol. Environ. Saf. 119, 186–197. doi: 10.1016/j.ecoenv.2015.05.011
Aebi, H. (1983). Catalase in Vitro. Methods in Enzymology 105, 121–126. doi: 10.1016/S0076-6879(84)05016-3
Ahmad, P., Ahanger, M. A., Alyemeni, M. N., Wijaya, L., Alam, P. (2018). Exogenous application of nitric oxide modulates osmolyte metabolism, antioxidants, enzymes of ascorbate-glutathione cycle and promotes growth under cadmium stress in tomato. Protoplasma 255, 79–93. doi: 10.1007/s00709-017-1132-x
Ahmad, A., Yasin, N. A., Khan, W. U., Akram, W., Wang, R., Shah, A. A., et al. (2021). Silicon assisted ameliorative effects of iron nanoparticles against cadmium stress: Attaining new equilibrium among physiochemical parameters, antioxidative machinery, and osmoregulators of phaseolus lunatus. Plant Physiol. Biochem. 166, 874–886. doi: 10.1016/j.plaphy.2021.06.016
Ali, M., Parveen, A., Malik, Z., Kamran, M., Saleem, M. H., Abbasi, G. H., et al. (2022). Zn alleviated salt toxicity in solanum lycopersicum l. seedlings by reducing na+ transfer, improving gas exchange, defense system and zn contents. Plant Physiol. Biochem. 186, 52–63. doi: 10.1016/j.plaphy.2022.06.028
Ali, S., Rizwan, M., Ullah, N., Bharwana, S. A., Waseem, M., Farooq, M. A., et al. (2016). Physiological and biochemical mechanisms of silicon-induced copper stress tolerance in cotton (Gossypium hirsutum l.). Acta Physiologiae Plantarum 38, 1–11. doi: 10.1007/s11738-016-2279-3
Al Mahmud, J., Hasanuzzaman, M., Nahar, K., Bhuyan, M. B., Fujita, M. (2018). Insights into citric acid-induced cadmium tolerance and phytoremediation in brassica juncea l.: Coordinated functions of metal chelation, antioxidant defense and glyoxalase systems. Ecotoxicol. Environ. Saf. 147, 990–1001. doi: 10.1016/j.ecoenv.2017.09.045
Antoniou, C., Chatzimichail, G., Xenofontos, R., Pavlou, J. J., Panagiotou, E., Christou, A., et al. (2017). Melatonin systemically ameliorates drought stress-induced damage in m edicago sativa plants by modulating nitro-oxidative homeostasis and proline metabolism. J. Pineal Res. 62, e12401. doi: 10.1111/jpi.12401
Anwaar, S. A., Ali, S., Ali, S., Ishaque, W., Farid, M., Farooq, M. A., et al. (2015). Silicon (Si) alleviates cotton (Gossypium hirsutum l.) from zinc (Zn) toxicity stress by limiting zn uptake and oxidative damage. Environ. Sci. Pollut. Res. 22, 3441–3450. doi: 10.1007/s11356-014-3938-9
Asada, K. (1992). Ascorbate peroxidase–a hydrogen peroxide-scavenging enzyme in plants. Physiologia Plantarum 85, 235–241. doi: 10.1111/j.1399-3054.1992.tb04728.x
Ashraf, M., Harris, P. J. C. (2013). Photosynthesis under stressful environments: An overview. Photosynthetica 51, 163–190. doi: 10.1007/s11099-013-0021-6
Beauchamp, C., Fridovich, I. (1971). Superoxide dismutase: Improved assays and an assay applicable to acrylamide gels. Anal. Biochem. 44, 276–287. doi: 10.1016/0003-2697(71)90370-8
Berni, R., Luyckx, M., Xu, X., Legay, S., Sergeant, K., Hausman, J.-F., et al. (2019). Reactive oxygen species and heavy metal stress in plants: Impact on the cell wall and secondary metabolism. Environ. Exp. Bot. 161, 98–106. doi: 10.1016/j.envexpbot.2018.10.017
Bhat, J. A., Shivaraj, S., Singh, P., Navadagi, D. B., Tripathi, D. K., Dash, P. K., et al. (2019). Role of silicon in mitigation of heavy metal stresses in crop plants. Plants 8, 71. doi: 10.3390/plants8030071
Cai, K., Gao, D., Luo, S., Zeng, R., Yang, J., Zhu, X. (2008). Physiological and cytological mechanisms of silicon-induced resistance in rice against blast disease. Physiologia Plantarum 134, 324–333. doi: 10.1111/j.1399-3054.2008.01140.x
Das, K., Roychoudhury, A. (2014). Reactive oxygen species (ROS) and response of antioxidants as ROS-scavengers during environmental stress in plants. Front. Environ. Sci. 2, 53. doi: 10.3389/fenvs.2014.00053
De Vos, C., Schat, H., De Waal, M., Vooijs, R., Ernst, W. (1991). Increased resistance to copper-induced damage of the root cell plasmalemma in copper tolerant silene cucubalus. Physiologia Plantarum 82, 523–528. doi: 10.1111/j.1399-3054.1991.tb02942.x
Droppa, M., Horváth, G. (1990). The role of copper in photosynthesis. Crit. Rev. Plant Sci. 9 (2), 111–123. doi: 10.1080/07352689009382284
Emamverdian, A., Ding, Y., Mokhberdoran, F., Xie, Y. (2015). Heavy metal stress and some mechanisms of plant defense response. Sci. World J. 2015:756120. doi: 10.1155/2015/756120
Emamverdian, A., Ding, Y., Xie, Y. (2018). Effects of silicon in the amelioration of zn toxicity on antioxidant enzyme activities. Toxicol. Environ. Health Sci. 10, 90–96. doi: 10.1007/s13530-018-0351-7
Gilabel, A. P., Nogueirol, R. C., Garbo, A. I., Monteiro, F. A. (2014). The role of sulfur in increasing guinea grass tolerance of copper phytotoxicity. Water Air Soil Pollut. 225, 1–10. doi: 10.1007/s11270-013-1806-8
Gillespie, K. M., Chae, J. M., Ainsworth, E. A. (2007). Rapid measurement of total antioxidant capacity in plants. Nat. Protoc. 2, 867–870. doi: 10.1038/nprot.2007.100
Gill, S. S., Tuteja, N. (2010). Reactive oxygen species and antioxidant machinery in abiotic stress tolerance in crop plants. Plant Physiol. Biochem. 48, 909–930. doi: 10.1016/j.plaphy.2010.08.016
Hall, J.Á. (2002). Cellular mechanisms for heavy metal detoxification and tolerance. J. Exp. Bot. 53, 1–11. doi: 10.1093/jexbot/53.366.1
Hasan, M., Liu, C.-X., Pan, Y.-T., Ahammed, G. J., Qi, Z.-Y., Zhou, J. (2018). Melatonin alleviates low-sulfur stress by promoting sulfur homeostasis in tomato plants. Sci. Rep. 8, 1–12. doi: 10.1038/s41598-018-28561-0
Huang, G., Ding, C., Guo, N., Ding, M., Zhang, H., Kamran, M., et al. (2021). Polymer-coated manganese fertilizer and its combination with lime reduces cadmium accumulation in brown rice (Oryza satival.). J. Hazard. Mater. 415, 125597. doi: 10.1016/j.jhazmat.2021.125597
Jiang, M., Song, Y., Kanwar, M. K., Ahammed, G. J., Shao, S., Zhou, J. (2021). Phytonanotechnology applications in modern agriculture. J. Nanobiotechnol. 19, 1–20. doi: 10.1186/s12951-021-01176-w
Kabata-Pendias, A. (2000). Trace elements in soils and plants (Boca Raton:CRC press). doi: 10.1201/9781420039900
Kamran, M., Danish, M., Saleem, M. H., Malik, Z., Parveen, A., Abbasi, G. H., et al. (2021). Application of abscisic acid and 6-benzylaminopurine modulated morpho-physiological and antioxidative defense responses of tomato (Solanum lycopersicum l.) by minimizing cobalt uptake. Chemosphere 263, 128169. doi: 10.1016/j.chemosphere.2020.128169
Keller, C., Rizwan, M., Davidian, J. C., Pokrovsky, O. S., Bovet, N., Chaurand, P., et al. (2015). Effect of silicon on wheat seedlings (Triticum turgidum l.) grown in hydroponics and exposed to 0 to 30 µM Cu. Planta 241 (4), 847–860. doi: 10.1007/s11356-015-5351-4
Khandekar, S., Leisner, S. (2011). Soluble silicon modulates expression of arabidopsis thaliana genes involved in copper stress. J. Plant Physiol. 168, 699–705. doi: 10.1016/j.jplph.2010.09.009
Kim, Y.-H., Khan, A. L., Kim, D.-H., Lee, S.-Y., Kim, K.-M., Waqas, M., et al. (2014). Silicon mitigates heavy metal stress by regulating p-type heavy metal ATPases, Oryza sativa low silicon genes, and endogenous phytohormones. BMC Plant Biol. 14, 1–13. doi: 10.1186/1471-2229-14-13
Lichtenthaler, H. K. (1987). Chlorophylls and carotenoids: Pigments of photosynthetic biomembranes. Method Enzymol. 148, 350–382. doi: 10.1016/0076-6879(87)48036-1
Lin, L., Zhou, W., Dai, H., Cao, F., Zhang, G., Wu, F. (2012). Selenium reduces cadmium uptake and mitigates cadmium toxicity in rice. J. hazard. mater. 235, 343–351. doi: 10.1016/j.jhazmat.2012.08.012
Li, Q., Yu, B., Gao, Y., Dai, A.-H., Bai, J.-G. (2011). Cinnamic acid pretreatment mitigates chilling stress of cucumber leaves through altering antioxidant enzyme activity. J. Plant Physiol. 168, 927–934. doi: 10.1016/j.jplph.2010.11.025
Li, X., Zhang, J., Gong, Y., Liu, Q., Yang, S., Ma, J., et al. (2020). Status of copper accumulation in agricultural soils across chin–2016). Chemosphere 244, 125516. doi: 10.1016/j.chemosphere.2019.125516
Malik, Z., Afzal, S., Dawood, M., Abbasi, G. H., Khan, M. I., Kamran, M., et al. (2022). Exogenous melatonin mitigates chromium toxicity in maize seedlings by modulating antioxidant system and suppresses chromium uptake and oxidative stress. Environ. Geochem. Health 44 (5), 1451–1469. doi: 10.1007/s10653-021-00908-z
Morkunas, I., Woźniak, A., Mai, V. C., Rucińska-Sobkowiak, R., Jeandet, P. (2018). The role of heavy metals in plant response to biotic stress. Molecules 23, 2320. doi: 10.3390/molecules23092320
Nahar, K., Hasanuzzaman, M., Alam, M. M., Rahman, A., Suzuki, T., Fujita, M. (2016). Polyamine and nitric oxide crosstalk: Antagonistic effects on cadmium toxicity in mung bean plants through upregulating the metal detoxification, antioxidant defense and methylglyoxal detoxification systems. Ecotoxicol. Environ. Saf. 126, 245–255. doi: 10.1016/j.ecoenv.2015.12.026
Nakano, Y., Asada, K. (1981). Hydrogen peroxide is scavenged by ascorbate-specific peroxidase in spinach chloroplasts. Plant Cell Physiol. 22, 867–880. doi: 10.1093/oxfordjournals.pcp.a076232
Noctor, G., Reichheld, J.-P., Foyer, C. H. (2018). “ROS-related redox regulation and signaling in plants,” in Seminars in cell & developmental biology (Elsevier), 80, 3–12. doi: 10.1016/j.semcdb.2017.07.013
Peñarrubia, L., Romero, P., Carrió-Seguí, A., Andrés-Bordería, A., Moreno, J., Sanz, A. (2015). Temporal aspects of copper homeostasis and its crosstalk with hormones. Front. Plant Sci. 6, 255. doi: 10.3389/fpls.2015.00255
Pirooz, P., Amooaghaie, R., Ahadi, A., Sharififar, F. (2021). Silicon-induced nitric oxide burst modulates systemic defensive responses of salvia officinalis under copper toxicity. Plant Physiol. Biochem. 162, 752–761. doi: 10.1016/j.plaphy.2021.02.048
Prasad, R., Bhattacharyya, A., Nguyen, Q. D. (2017). Nanotechnology in sustainable agriculture: Recent developments, challenges, and perspectives. Front. Microbiol. 8, 1014. doi: 10.3389/fmicb.2017.01014
Rahman, M. F., Ghosal, A., Alam, M. F., Kabir, A. H. (2017). Remediation of cadmium toxicity in field peas (Pisum sativum l.) through exogenous silicon. Ecotoxicol. Environ. Saf. 135, 165–172. doi: 10.1016/j.ecoenv.2016.09.019
Riaz, M., Kamran, M., Fahad, S., Wang, X. (2022). Nano-silicon mediated alleviation of cd toxicity by cell wall adsorption and antioxidant defense system in rice seedlings. Plant Soil 1-15. doi: 10.1007/s11104-022-05588-x
Roychoudhury, A. (2020). Silicon-nanoparticles in crop improvement and agriculture. Int. J. Recent Adv. Biotechnol. Nanotechnol. 3(1).
Sabir, F., Noreen, S., Malik, Z., Kamran, M., Riaz, M., Dawood, M., et al. (2022). “Silicon improves salinity tolerance in crop plants: Insights into photosynthesis, defense system, and production of phytohormones,” in Silicon and nano-silicon in environmental stress management and crop quality improvement (Academic Press), 91–103.
Saleem, M. H., Kamran, M., Zhou, Y., Parveen, A., Rehman, M., Ahmar, S., et al. (2020). Appraising growth, oxidative stress and copper phytoextraction potential of flax (Linum usitatissimum l.) grown in soil differentially spiked with copper. J. Environ. Manage. 257, 109994. doi: 10.1016/j.jenvman.2019.109994
Seregin, I. V., Kozhevnikova, A. D. (2006). Physiological role of nickel and its toxic 21 effects on higher plants. Russ. J. Plant Physiol. 53 (2), 257–277. doi: 10.1134/S1021443706020178
Seth, C. S., Remans, T., Keunen, E., Jozefczak, M., Gielen, H., Opdenakker, K., et al. (2012). Phytoextraction of toxic metals: A central role for glutathione. Plant Cell Environ. 35, 334–346. doi: 10.1111/j.1365-3040.2011.02338.x
Singer, M. J., Munns, D. (1987). Soils: An introduction (MacMillan, New York: McMillan Publishing Company).
Singh, S., Parihar, P., Singh, R., Singh, V. P., Prasad, S. M. (2016). Heavy metal tolerance in plants: Role of transcriptomics, proteomics, metabolomics, and ionomics. Front. Plant Sci. 61143. doi: 10.3389/fpls.2015.01143
Steel, R. G. D., Torrie, J. H., Dickey, D. (1997). Principles and procedure of statistics (New York: A biometrical approach 3rd Ed. McGraw HillBookCo. Inc.), 352–358.
Szalai, G., Kellős, T., Galiba, G., Kocsy, G. (2009). Glutathione as an antioxidant and regulatory molecule in plants under abiotic stress conditions. J. Plant Growth Regul. 28, 66–80. doi: 10.1007/s00344-008-9075-2
Tamaka, A., Tanaka, R. (2006). Chlorophyll metabolism. Curr. Opin. Plant Biol. 9 (3), 248–255. doi: 10.1016/j.pbi.2006.03.011
Velikova, V., Yordanov, I., Edreva, A. (2000). Oxidative stress and some antioxidant systems in acid rain-treated bean plants: Protective role of exogenous polyamines. Plant Sci. 151, 59–66. doi: 10.1016/S0168-9452(99)00197-1
Vieira Filho, L. O., Monteiro, F. A. (2020). Silicon modulates copper absorption and increases yield of Tanzania guinea grass under copper toxicity. Environ. Sci. pollut. Res. 27, 31221–31232. doi: 10.1007/s11356-020-09337-4
Vieira-Filho, L. O., Monteiro, F. A. (2022). Silicon improves photosynthetic activity and induces antioxidant enzyme activity in Tanzania Guinea grass under copper toxicity. Plant Stress 3, 100045. doi: 10.1016/j.stress.2021.100045
Yi, Z., Li, S., Liang, Y., Zhao, H., Hou, L., Yu, S., et al. (2018). Effects of exogenous spermidine and elevated CO2 on physiological and biochemical changes in tomato plants under iso-osmotic salt stress. J. Plant Growth Regul. 37, 1222–1234. doi: 10.1007/s00344-018-9856-1
Zeng, J., Tang, J., Zhang, F., Wang, Y., Kang, H., Chen, G., et al. (2021). Ammonium regulates redox homeostasis and photosynthetic ability to mitigate copper toxicity in wheat seedlings. Ecotoxicol. Environ. Saf. 226, 112825. doi: 10.1016/j.ecoenv.2021.112825
Zhang, Y., Chen, H., Liang, Y., Lu, T., Liu, Z., Jin, X., et al. (2021). Comparative transcriptomic and metabolomic analyses reveal the protective effects of silicon against low phosphorus stress in tomato plants. Plant Physiol. Biochem. 166, 78–87. doi: 10.1016/j.plaphy.2021.05.043
Zhang, Y., Liang, Y., Zhao, X., Jin, X., Hou, L., Shi, Y., et al. (2019b). Silicon compensates phosphorus deficit-induced growth inhibition by improving photosynthetic capacity, antioxidant potential, and nutrient homeostasis in tomato. Agronomy 9, 733. doi: 10.3390/agronomy9110733
Keywords: wheat, silicon, oxidative stress, AsA-GSH, copper toxicity
Citation: Riaz M, Zhao S, Kamran M, Ur Rehman N, Mora-Poblete F, Maldonado C, Hamzah Saleem M, Parveen A, Ahmed Al-Ghamdi A, Al-Hemaid FM, Ali S and Elshikh MS (2022) Effect of nano-silicon on the regulation of ascorbate-glutathione contents, antioxidant defense system and growth of copper stressed wheat (Triticum aestivum L.) seedlings. Front. Plant Sci. 13:986991. doi: 10.3389/fpls.2022.986991
Received: 05 July 2022; Accepted: 20 September 2022;
Published: 13 October 2022.
Edited by:
Heba Mahmoud Mohammad Abdel-Aziz, Mansoura University, EgyptReviewed by:
Golam Jalal Ahammed, Henan University of Science and Technology, ChinaBadr A. Mohamed, Cairo University, Egypt
Copyright © 2022 Riaz, Zhao, Kamran, Ur Rehman, Mora-Poblete, Maldonado, Hamzah Saleem, Parveen, Ahmed Al-Ghamdi, Al-Hemaid, Ali and Elshikh. This is an open-access article distributed under the terms of the Creative Commons Attribution License (CC BY). The use, distribution or reproduction in other forums is permitted, provided the original author(s) and the copyright owner(s) are credited and that the original publication in this journal is cited, in accordance with accepted academic practice. No use, distribution or reproduction is permitted which does not comply with these terms.
*Correspondence: Shaopeng Zhao, emhhb3NoYW9wZW5nQHpoa3UuZWR1LmNu; Carlos Maldonado, Y21hbGRvMTc4MkBnbWFpbC5jb20=