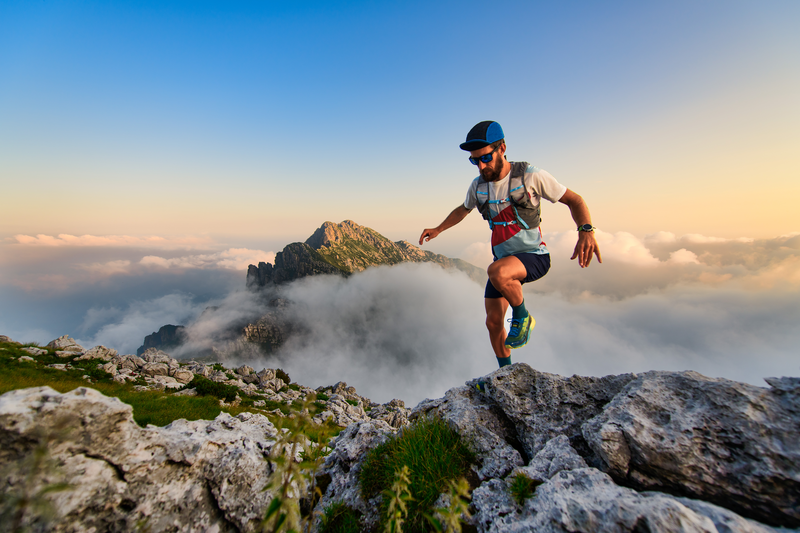
95% of researchers rate our articles as excellent or good
Learn more about the work of our research integrity team to safeguard the quality of each article we publish.
Find out more
ORIGINAL RESEARCH article
Front. Plant Sci. , 29 August 2022
Sec. Crop and Product Physiology
Volume 13 - 2022 | https://doi.org/10.3389/fpls.2022.982373
The application of nitrogen (N) fertilizer combined with nitrification inhibitor is considered to be one of the effective strategies to improve N efficiency and reduce N loss. While the chemical and physical properties of nitrapyrin (CP) in fertilizers have been evaluated to increase N efficiency, a lack of comprehensive evaluation of the effects of adding CP on summer maize yield, environmental benefits and economic income under different fertilization methods. In this study, two fertilization methods were used: split-N application and one-time basal N fertilizer before sowing. The comprehensive effects of N fertilizer with CP on N loss (NH3 volatilization, NO3– leaching, and N2O emissions), N efficiency, yield and profit under two N application methods were explored. Results showed that under the two N application methods, N fertilizer with CP treatment increased the N efficiency and yield (+3.4%∼+5.7%), significantly reduced the soil NO3–-N content and N2O emissions, while increased NH3 volatilization. Especially, the increase amplitude of NH3 was much less than the decrease amplitude of N2O induced by adding CP. Although split-N application could achieve higher yield and N efficiency, N2O emissions and NH3 volatilization also increased. However, the T1 + N (one-time basal N fertilizer before sowing mixed with CP) achieved the same yield level as T2 treatment (split-N application). Taking agronomic, economic and environmental benefits into consideration, one-time basal N fertilizer before sowing mixed with CP could ensure the target yield, increase economic benefits, maintain soil N content, and reduce N losses. Therefore, optimizing N management is essential to the sustainable development of agriculture.
Global food demand requires increased uses of fertilizers, although the use of chemical fertilizer guarantees the high crop yield, it causes low utilization rate, the loss of fertilizer, and a series of environmental pollution problems (Hao et al., 2019). Nitrogen (N) is one of the most restricted nutrients in the formation of maize yield, but excessive and uncoordinated fertilization methods often result in low nitrogen use efficiency (NUE) (Nasielski et al., 2020). Therefore, management practices need to be improved to increase crop productivity and reduce environmental hazards.
In the growing season, split-N application is usually used to meet the N demand of maize to the greatest extent to obtain higher yields (Rahman et al., 2011; Wang et al., 2016). Compared with one-time application of N fertilizer, split-N application could significantly prolong the active period of N accumulation in summer maize and significantly increase the NUE (Lv et al., 2011; Lu et al., 2021). However, the shortage of agricultural workers and higher-cost inputs often limit implementation. Therefore, measures such as one-time base application of urea are usually used in maize production (Lu et al., 2021). After urea is applied to the soil, it is hydrolyzed to ammonium (NH4+) and then quickly converted to nitrate (NO3–). Heavy rainfall often causes a large amount of NO3– leaching into the deep soil layer, which leads to insufficient N to meet the maize demand in the late season (Azad et al., 2019; Fernandez et al., 2020). In order to reduce N loss as much as possible, some technologies, such as the application of controlled-release N fertilizer, improve NUE by controlling the release time of N (Fan et al., 2021). But the positive effects may be limited due to a slowed release of N from controlled-release N fertilizers, especially during early season (Qu et al., 2020). Adding nitrification inhibitors is considered to be one of the effective ways to increase crop yield and NUE. Nitrification inhibitors inhibit soil nitrification, and directly affecting nitrifying bacteria community and nitrification activity, keeping N in the form of NH4+ in soil for a long period, and thereby reducing leaching loss of nitrate N and denitrification of soil (Menendez et al., 2006; Sun et al., 2008; Monge-Muñoz et al., 2021).
Nitrapyrin, short for 2-chloro-6-(trichloromethyl) pyridine (CP, N-serve), is a white crystalline solid substance, almost insoluble in water, soluble in organic solvents (Goring, 1962; Borzouei et al., 2021). It is one of the most widely studied and effective nitrification inhibitors. In some regional trials, adding CP significantly increased yield and NUE (Sun et al., 2015). However, a meta-analysis showed that the addition of CP changed the crop yield by –20∼207% (Wolt, 2004). It can be seen that the effectiveness of CP is variable. CP inhibits the activity of soil ammonia oxidizing bacteria and retard the conversion of NH4+ into NO2– (the first step of nitrification), thereby keeping the fertilizer in the form of NH4+. However, higher NH4+ in the soil may increase ammonia volatilization. In a multiyear rice study, the urea with CP increased the NH3 volatilization by 7% compared to the urea treatment without CP (Sun et al., 2015). Conversely, CP targets nitrification and limits the formation of NO3– (the substrate for denitrification), so it has potential to reduce N2O emissions. It is reported that CP reduces greenhouse gas emissions by 21∼70% compared with treatment without CP (Akiyama et al., 2010). The evaluation results of the effects of using nitrification inhibitors on N2O emissions and NH3 volatilization are not consistent.
Most studies have addressed the effect of N management on increasing yield, reducing N2O emissions and NH3 volatilization, but little research has been directed toward how to balance the relationship between yield and N loss, and to resolve the contradiction between economic income and ecological effects. Therefore, it is necessary to reconcile production goals with environmental costs, and choose a reasonable N management strategy. In this study, a comprehensive evaluation of the effects of adding CP on summer maize yield, environmental benefits and economic income under different fertilization methods was conducted. It may provide scientific basis for high-yield and high-efficiency cultivation of summer maize and reduce environmental pollution. We hypothesize that the use of disposable basal application of urea with adding CP can achieve the same or higher yield and NUE as the split-N application, and reduce environmental pollution. Specially, the aims of this study were to: (i) investigate the effects of adding CP on yield and N efficiency of summer maize, (ii) illustrate the relationships among N fertilizer adding CP, N2O emissions, NH3 volatilization, and NO3–-N/NH4+-N accumulation of farmland soil, (iii) explore better N application methods for the environment and economic income.
This study was carried out at the experimental farm (36°10’N, 117°04’E, 151 m a.s.l.) maintained by the State Key Laboratory of Crop Biology of Shandong Agricultural University, China in 2016 and 2017. The region was characterized by brown loam soils and a temperate continental monsoon climate with mean annual temperature of approximately 13°C. The rainfall mainly focused on June, July and August, with an average rainfall of 697 mm in the two maize seasons. Soil physical and chemical properties (0–20 cm) are as listed: (i) pH is 7.2; (ii) organic matter is 10.7 g kg–1; (iii) total N is 0.9 g kg–1; (iv) available phosphorus is 50.7 mg kg–1; and (v) available potassium is 86.2 mg kg–1. Denghai618 (DH618), a commonly grown maize hybrid, was used for this experiment. Maize seeds were sown on 16-June 2016 and 20-June 2017 at a density of 60,000 plants ha–1 and 75,000 plants ha–1, respectively.
The nitrification inhibitor was 2-chloro-6-(trichloromethyl) pyridine (CP), supplied by Dow AgroSciences (Indianapolis, IN, United States). Experimental treatment (length 10 m* width 6 m) was as follow: one-time basal N fertilizer before sowing (T1, 210 kg ha–1 N), one-time basal N fertilizer before sowing mixed with CP (T1 + N), split-N application (T2, 105 kg ha–1 N was applied before sowing, and the other N was applied at the 10th leaf stage), and split-N application mixed with CP (T2 + N). Urea was used as the N source, and the N application rate was 210 kg ha–1 N in all treatments. Both basal and topdressing N fertilizer supplements were applied in bands near the plant row incorporated into the soil via plowing. The rate of CP was 0.24% of the rate of urea-N application, the mixture was obtained by physically mixing it with urea. Each treatment was replicated three times. 84 kg ha–1 P2O5 (calcium superphosphate, 17%) and 168 kg ha–1 K2O (Potassium chloride, 60%) were applied to all plots before sowing. Supplemental irrigation was not applied during the summer maize seasons except for one use after sowing. Micro-sprinkler irrigation was used with a water consumption of about 30 mm. During the growing period of maize, good field management was practiced according to the production habits of local farmers.
The nitrous oxide (N2O) was collected and analyzed by closed-chamber gas chromatography. Three chambers with a volume of 35 cm length × 35 cm width × 20 cm height were set per for each treatment and placed before each sampling. The closed chamber was enclosed with plastic sheets (sponge material and aluminum foil on the outside) and an air vent was installed in the middle of chamber. A pedestal was placed under the chamber and sealed with water. The gas samples (50 mL) were collected once every one days within a week after fertilization, and then every 7–10 days (1–2 times a week, then every 7–10 days after fertilization in 2017) for collection at 8:00–11:00 a.m. using glass syringes from chamber headspace 0, 10, 20, and 30 min after soil sample was covered. The gas samples were analyzed for N2O concentration within 36 h after sampling by an Agilent GC7890 gas chromatograph (Agilent, Santa Clara, CA, United States) equipped with an electron capture detector (ECD). The N2O emissions flux was calculated as follow (Yu et al., 2022):
where J is N2O emissions flux (mg m–2 h–1), dc/dt is the change in gas concentration per unit time (mg m–3 h–1). M is the molar mass of the gas (mg mol–1), P is atmospheric pressure (KPa), T is the absolute temperature (K), H is the height of the chamber (m), V0 is the gas molar volume under standard conditions (m3 mol–1), T0 is the absolute air temperature under standard conditions (K), P0 is the atmospheric pressure under standard conditions (KPa).
Five vented-chamber devices for NH3 volatilization measurements were placed for each plot (Zhao et al., 2013). The vented chamber consisted of a gray round polyvinyl chloride (PVC) tubing (15 cm internal diameter and 10 cm high) and two round sponges (16 cm in diameter and 2 cm in thickness). The sponges were presoaked with a 15 mL phosphate-glycerol solution (50 mL analytical phosphate and 40 mL glycerol diluted to 1,000 mL with pure water) and then inserted into each chamber.
In summer maize-growing season, the sponges were replaced between 8:00 a.m. and 11:00 a.m. daily after fertilization during the first week and every 2–3 days during the second and third week. Ammonia in the phosphate solution in each sponge inside the vented chamber was extracted with 300 mL of 1 M KCl after 1 h of oscillation. Ammonium quantities were measured using the micro-Kjeldahl method (CN61M/KDY-9820, Beijing, China). NH3 volatilization from the soil was estimated by the following formula (Li et al., 2007):
where NV is the NH3 volatilization (kg N ha–1 d–1), M is the NH3 captured by the vented chamber during each sampling (mg N), A is the cross-section area of the round chamber (m2), D is the duration of each sampling (days).
In each plot, three replicate soil samples were collected every 7 days from the start of basal N fertilizer supplement to the six-leaf stage (V6), topdressing N fertilizer supplement to the tasseling stage (VT), and then every 14 days until the maturity stage (R6) in 2016. And soil samples were taken at V6, VT, and R6 stage in 2017. The samples were divided into three layers (60 cm length by 20 cm depth). Soil NH4+-N and NO3–-N were extracted with 1 M KCl and measured by AA3 Continuous Flow Analytical System.
Five plant samples were obtained from each plot at R6 stage. All samples were separated into stem, leaf, and ear, dried at 60°C in a force-draft oven (DHG-9420A, Bilon Instruments Co. Ltd., Shanghai, China) to a constant weight. Total N was measured using the Kjeldahl method. Partial factor productivity from applied nitrogen (PFPN, kg kg–1), agronomic efficiency of applied nitrogen (AEN, kg kg–1), nitrogen recovery efficiency (REN, kg kg–1) and soil nitrogen dependency rate (SNDR, %) were calculated as follow (Wu et al., 2008):
where YF is the grain yield in the fertilized plot (kg), YC is the grain yield in the control plot (kg), NA is the amount of applied N (kg), TC is the total N uptake by plant in the control plot (kg), TN is the total N uptake by plant in the fertilized plot (kg).
Grain yield and ear traits were analyzed by harvesting the three central rows of each plot in 2016–2017. AT R6 stage, 30 consecutive plants per row were harvested to measure summer maize yield (moisture content is approximately 14%, GB/T 29890-2013).
Environmental cost (EC, $ kg–1) was the cost of N emissions pollution caused by N fertilization, following the calculation of Yin et al. (2019):
where EC refer to the environmental damage costs caused by soil acidification and climate warming caused by N2O and eutrophication caused by NH3 volatilization. The pollution cost values of N2O-N and NH3-N are $11.2 and $2.11 kg–1, respectively.
Net income (NI, $ ha–1) was calculated as follows (Zhang et al., 2018):
where GI is the grain income (determined by grain yield multiplying the grain price), AC is the agronomic cost.
The statistical analyses were performed using SPSS 17.0 (SPSS Inc., Chicago, IL, United States). All data were subjected to one-way analyses of variance (ANOVA) followed by mean comparisons using the least significant difference (LSD, P ≤ 0.05). All the figures were produced using Sigma Plot 14.0 (Systat Software Inc., CA, United States).
Compared with one-time base application of N fertilizer, the split-N application treatment significantly increased the grain yield. The grain yield of T2 was 2.5% higher than that of T1, and the T2 + N treatment was 4.7% higher than T1 + N. Furthermore, the fertilized treatments with the CP exhibited higher grain yield than treatments without the CP under the two fertilization methods. The yield increase of CP treatment was mainly to the improvement of grain number per spike and 1000-grain weight of summer maize by 2.2% and 1.4% on average, compared to those of no CP treatment, while the differences were not significant. In particular, compared with the split-N application treatment (T2), the one-time base application of N fertilizer with CP (T1 + N) increased the grain yield (the grain yield of T1 + N treatment was higher than that of T2 for 1 year, while there was no significant difference in 2016) (Table 1).
Table 1. Effect of nitrogen with CP on grain yield and yield components of summer maize under different fertilization methods.
As shown in Figure 1, N2O emission rates peaked within 10–20 days after applying the base fertilizer and topdressing, and then decreased. The first peak value of the split-N application treatment was significantly lower than the one-time base application of N fertilizer, but the second increased significantly. In addition, the cumulative N2O emission fluxes of split-N application treatment in two maize seasons were significantly higher than the one-time base application of N fertilizer. Obviously, under the two N application methods, the N2O emission peak value of the CP treatment was significantly reduced. Compared with T1, the peak value of T1 + N treatment decreased by 48.6 and 20.2% in 2016 and 2017, respectively; while the first peak value of T2 + N treatment was reduced by 49.5 and 19.4%, respectively, compared with T2, and the second was reduced by 59.2 and 10.8%. Similarly, the cumulative N2O emission fluxes of the fertilized treatments with the CP were also significantly decreased. In addition, the cumulative N2O emission fluxes of the one-time base application of N fertilizer with the CP was significantly lower than other treatments.
Figure 1. Dynamics of N2O volatilization emission rates. (A,B) T1, one-time basal N fertilizer before sowing; T1 + N, one-time basal N fertilizer before sowing mixed with CP; (C,D) T2, split-N application; T2 + N, split-N application mixed with CP; BF, basal N fertilizer supplement; TF, topdressing N fertilizer supplement. The downward arrow indicates fertilization.
Split-N application significantly increased NH3 volatilization rate, especially after top dressing. Under the two N application methods, on CP addition, the peak value and duration of NH3 volatilization increased, and the accumulation of NH3 volatilization was also higher than the single N application treatment. The accumulation of NH3 volatilization for T1 + N was 15.6% greater than T1 treatment, and the T2 + N treatment was increased by 12.9%, compared to T2. However, the cumulative NH3 volatilization of the one-time base application of N fertilizer with CP treatment (T1 + N) was lower than that of the split-N application treatment (T2) (Figure 2).
Figure 2. Dynamics of NH3 volatilization emission rates and cumulative NH3 volatilization. T1, one-time basal N fertilizer before sowing; T1 + N, one-time basal N fertilizer before sowing mixed with CP; T2, split-N application; T2 + N, split-N application mixed with CP.
The soil NO3–-N content of the one-time base application of N fertilizer treatment increased first and then decreased, while the split-N application treatment had two obvious peak value. Under the two N application methods, on CP addition, the NO3–-N content in the different soil layers was lower than the single N application treatment. The content of soil NO3–-N for T1 + N was decreased by 13.1, 15.1, and 13.4% in 0–20, 20–40, and 40–60 cm, compared to those of T1, respectively. And the T2 + N treatment was decreased by 15.6, 11.0, and 17.0%, compared to those of T2, respectively. The results of different stages of maize in 2017 showed that the adding CP treatment significantly reduced NO3–-N content in the 0–20 cm soil layer at V6 and VT, but there was no significant difference in the 40–60 cm soil layer at R6 stage, compared to the single N application treatment (Figure 3).
Figure 3. Dynamics of soil NO3–-N content. T1, one-time basal N fertilizer before sowing; T1 + N, one-time basal N fertilizer before sowing mixed with CP; T2, split-N application; T2 + N, split-N application mixed with CP; V6, six-leaf stage (12/7/2017); VT, tasseling stage (5/8/2017); R6, maturity stage (3/10/2017).
After fertilization, the content of soil NH4+-N increased firstly and then decreased. The spatial and temporal distribution of NH4+-N in soil was changed by split-N application, and the retention time of NH4+-N in soil was effectively improved, compared to that of one-time base application of N fertilizer. In addition, the content of NH4+-N in the 0–40 cm soil layer increased significantly when the CP was added, but there was no significant difference in the 40–60 cm soil layer. The content of soil NH4+-N for T1 + N was increased by 34.2, 12.4, and 7.6% in 0–20, 20–40, and 40–60 cm, compared to those of T1, respectively. However, the content of soil NH4+-N for T2 + N was increased by 45.4, 17.7, and 4.1% in 0–20, 20–40, and 40–60 cm, compared to those of T2, respectively (Figure 4).
Figure 4. Dynamics of soil NH4+-N content. T1, one-time basal N fertilizer before sowing; T1 + N, one-time basal N fertilizer before sowing mixed with CP; T2, split-N application; T2 + N, split-N application mixed with CP; V6, six-leaf stage (12/7/2017); VT, tasseling stage (5/8/2017); R6, maturity stage (3/10/2017).
Split-N application significantly increased the nitrogen accumulation (NA), nitrogen partial factor productivity (PFPN), nitrogen agronomic efficiency (AEN), and nitrogen recovery efficiency (REN) of summer maize. For all the N application treatments, the addition of CP obtained higher N efficiency. The PFPN, AEN, and REN of T1 + N were increased by 3.4, 20.2 and 162.7%, respectively, compared with T1. Compared with T2, the T2 + N treatment were increased by 5.7, 30.5, and 46.0%, respectively. However, the soil nitrogen dependency rate (SNDR) of CP treatment was decreased significantly, with an average decrease of 13.1%, compared with that of no CP treatment. Furthermore, compared with T2, the NA and REN of TI + N treatment increased by 8.8 and 36.8% respectively in 2016, while there was no significant difference in 2017 (Table 2).
Table 2. Effect of nitrogen with CP on nitrogen efficiency of summer maize under different fertilization methods.
Compared with the one-time base application of N fertilizer treatment, split-N application significantly increased the yield value, while also increasing labor input and environmental costs. Under the two fertilization methods, the net income of the treatment with CP was significantly higher than the single N application treatment. Compared with T1, the net income of T1 + N increased by 4.1%, and the T2 + N treatment enhanced by 7.1% compared with T2. Overall, the net income of T2 + N was significantly higher than that of other treatments, while there was no significant difference between T1 and T2 treatments (Table 3).
Reasonable N application is considered to be one of the most effective management strategies to increase crop yield and N efficiency. It is necessary to consider the appropriate N source, application rate and time during crop growth to increase yield and N efficiency, and reduce production costs and environmental pollution (Abbasi et al., 2012; Schulte-Uebbing and Vries, 2021). Therefore, it is usually recommended to apply fertilizer in batches as a way to increase output and reduce N loss in production (Xu et al., 2021). Compared with single N application before sowing, split N application could increase grain yield and NUE (Sangoi et al., 2007). In this study, compared with the one-time basal application before planting, the grain yield of summer maize increased by divided fertilization, especially the 1,000-grain weight increased (Table 1), which was similar to the previous study (Amanullah and Shah, 2010; Guo et al., 2016). The increase in yield caused by the split-N application may be due to the acquisition of more effective N in the late growth period of summer maize. However, the output was enhanced under the two fertilization measures after adding CP. Obviously, there was no significant difference between the one-time base application of urea with CP added before sowing and the split-N application treatment. This may be related to the increase in NUE after adding CP. Sun et al. (2015) reported that adding CP to rice could save 60 kg N ha–1. Similarly, the results of N efficiency also showed that N accumulation, AEN, REN, and PFPN were significantly improved after adding CP (Table 2).
In crop production, the loss of N is difficult to effectively avoid. Although it has been found in most studies that adding a nitrification inhibitor would minimize N loss. However, there were also opposite research results, especially the results of N2O emissions and ammonia volatilization were not the same. Nitrapyrin mainly acted on nitrite oxidizing bacteria (Nitrobacter) to participate in the oxidation of NO2– to NO3– process, which was the second step in nitrification, but as long as one step of the reaction was inhibited, the whole nitrification was inhibited (Wu et al., 2008). Previous studies showed that nitrification inhibitors could slow down soil nitrification rate by acting on soil microorganisms, reduce the accumulation of NO2–-N, and ultimately achieved the goal of reducing N2O emissions (Ju et al., 2011; Hu et al., 2013).
Nitrification inhibitors reduced soil N2O emissions, while increased soil NH3 volatilization at the same time (Freney et al., 1995; Parkin and Hatfield, 2010; Rex and Tony, 2013). Our studies showed that NH4+-N concentrations after applying CP were higher in 0–20 and 20–40 cm soil layers compared to concentrations in no CP treatment, whereas NO3–-N concentrations in all soil layers (0–20, 20–40, and 40–60 cm) were lower in CP treatment (Figures 3, 4). This result might be due to the presence of nitrification inhibitors, which prevented the transformation of NH4+-N into NO3–-N, and maintaining N in the form of NH4+-N in soil for a longer time period. Since N was retained in the form of less mobile NH4+-N, the rate of leaching was significantly slowed, and thus reduced N leaching and increased NUE (Rahmatullah et al., 2010). Nitrate leaching losses is mainly determined by the concentration of NO3–-N in the soil solution and the amount of soil leachate (Vibart et al., 2016). Similarly, split fertilization also slowed down the leaching rate of NO3–-N in shallow soils and prolonged the effectiveness of N. This also explained the increase in grain yield, although it also caused an increase in N2O emissions (Figure 1).
After adding CP, soil nitrification was inhibited, the content of NH4+-N in the soil was at a high level, resulting in the loss of NH4+-N in the form of NH3 volatilization (+12.9%∼+15.6%) (Figure 2). However, the increase amplitude of NH3 was much less than that of N2O (–29.9∼-34.8%) induced by applying CP. But it did not reduce environmental costs (Table 3). The reason for this may be that we did not consider other costs such as NO3–-N leaching in the soil. In addition, the net income obtained by adding CP treatment increased significantly. Therefore, adding CP in actual production may have lower environmental costs and higher benefits. Especially, our results also showed that compared with the split-N application treatment, the net benefit of the one-time base application of N fertilizer with CP increased significantly. It can be seen that optimizing N fertilizer management would significantly improve crop production.
Optimizing N fertilizer management is essential to increase agricultural productivity and economic benefits, improve environmental pollution and human health. Our research aggregated N application methods, N losses, output value and benefits, which may provide an effective method for balancing the relationship between agronomic, economic, and environmental benefits. In this study, although split-N application could achieve higher yield, it also caused higher N2O emissions and NH3 volatilization. Nitrapyrin was conducive to alleviate N2O emission and N leaching loss, improving N efficiency, and increasing grain yield of summer maize. One-time basic application of N fertilizer with nitrapyrin was an efficient fertilization method, which achieved the same or even higher yield level as that of split-N application, reduced environmental pollution and obtained better economic value.
The original contributions presented in this study are included in the article/supplementary material, further inquiries can be directed to the corresponding author.
BR: data curation, writing—original draft, visualization, and investigation. ZM: writing—review and editing. PL and BZ: supervision. JZ: conceptualization, writing—review and editing, and funding acquisition. All authors contributed to the article and approved the submitted version.
We are grateful for grants from the University Youth Innovation Science and Technology Support Program of Shandong Province (2021KJ073), National Key Research and Development Program of China (2017YFD0300304 and 2018YFD0300603), National Modern Agricultural Technology & Industry System (CARS-02-18), and Major Scientific and Technological Innovation Project of Shandong Province (2019JZZY010716).
The authors declare that the research was conducted in the absence of any commercial or financial relationships that could be construed as a potential conflict of interest.
All claims expressed in this article are solely those of the authors and do not necessarily represent those of their affiliated organizations, or those of the publisher, the editors and the reviewers. Any product that may be evaluated in this article, or claim that may be made by its manufacturer, is not guaranteed or endorsed by the publisher.
Abbasi, M. K., Tahir, M. M., Sadiq, A., Iqbal, M., and Zafar, M. (2012). Yield and nitrogen use efficiency of rainfed maize response to splitting and nitrogen rates in Kashmir, Pakistan. Agron. J. 104, 448–457. doi: 10.2134/agronj2011.0267
Akiyama, H., Yan, X., and Yagi, K. (2010). Evaluation of effectiveness of enhanced-efficiency fertilizers as mitigation options for N2O and NO emissions from agricultural soils: Meta-analysis. Glob. Change Biol. 16, 1837–1846. doi: 10.1111/j.1365-2486.2009.02031.x
Amanullah Shah, P. (2010). Timing and rate of nitrogen application influence grain quality and yield in maize planted at high and low densities. J. Sci. Food Agric. 90, 21–29. doi: 10.1002/jsfa.3769
Azad, N., Behmanesh, J., Rezaverdinejad, V., Abbasi, F., and Navabian, M. (2019). Evaluation of fertigation management impacts of surface drip irrigation on reducing nitrate leaching using numerical modeling. Environ. Sci. Pollut. Res. 26, 36499–36514. doi: 10.1007/s11356-019-06699-2
Borzouei, A., Mander, U., Teemusk, A., Sanz-Cobena, A., Zaman, M., Kim, D. G., et al. (2021). Effects of the nitrification inhibitor nitrapyrin and tillage practices on yield-scaled nitrous oxide emission from a maize field in Iran. Pedosphere 31, 314–322. doi: 10.1007/s11356-021-16768-0
Fan, Z., Chen, J., Zhai, S., Ding, X., Zhang, H., Sun, S., et al. (2021). Optimal blends of controlled-release urea and conventional urea improved nitrogen use efficiency in wheat and maize with reduced nitrogen application. J. Soil Sci. Plant Nutr. 21, 1103–1111. doi: 10.1007/s42729-021-00425-z
Fernandez, J. A., Debruin, J., Messina, C. D., and Ciampitti, I. A. (2020). Late-season nitrogen fertilization on maize yield: A meta-analysis. Field Crops Res. 247:107586. doi: 10.1016/j.fcr.2019.107586
Freney, J. R., Keerthisinghe, D. G., Phongpan, S., Chaiwanakupt, P., and Harrington, K. J. (1995). Effect of urease, nitrification, algal inhibitors on ammonia loss and grain yield of flooded rice in Thailand. Fertil. Res. 40, 225–233. doi: 10.1007/BF00750469
Goring, C. A. I. (1962). Control of nitrification by 2-chloro-6-(trichloromethyl) pyridine. Soil Sci. 93, 211–218. doi: 10.1097/00010694-196203000-00010
Guo, J., Wang, Y., Fan, T., Chen, X., and Cui, Z. (2016). Designing corn management strategies for high yield and high nitrogen use efficiency. Agron. J. 108, 922–929. doi: 10.2134/agronj2015.0435
Hao, T., Zhu, Q., Zeng, M., Shen, J., Shi, X., Liu, X., et al. (2019). Quantification of the contribution of nitrogen fertilization and crop harvesting to soil acidification in a wheat-maize double cropping system. Plant Soil 434, 167–184. doi: 10.1007/s11104-018-3760-0
Hu, X. K., Su, F., Ju, X. T., Gao, B., Oenema, O., Christie, P., et al. (2013). Greenhouse gas emissions from a wheat-maize double cropping system with different nitrogen fertilization regimes. Environ. Pollut. 176, 198–207. doi: 10.1016/j.envpol.2013.01.040
Ju, X. T., Lu, X., Gao, Z. L., Chen, X., Su, F., Kogge, M., et al. (2011). Processes and factors controlling N2O production in an intensively managed low carbon calcareous soil under sub-humid monsoon conditions. Environ. Pollut. 159, 1007–1016. doi: 10.1016/j.envpol.2010.10.040
Li, Z. X., Dong, S. T., Wang, K. J., Zhang, J. W., Liu, P., Wang, Q. C., et al. (2007). In situ study on influence of different fertilization strategies for summer maize on soil nitrogen leaching and volatilization. Plant Nutr. Fertil. Sci. 13, 998–1005.
Lu, J., Hu, T., Zhang, B., Wang, L., Yang, S., Fan, J., et al. (2021). Nitrogen fertilizer management effects on soil nitrate leaching, grain yield and economic benefit of summer maize in Northwest China. Agric. Water Manag. 247:106739. doi: 10.1016/j.agwat.2021.106739
Lv, P., Zhang, J. W., Liu, W., Yang, J. S., Su, K., Liu, P., et al. (2011). Effects of nitrogen application on yield and nitrogen use efficiency of summer maize under super-high yield conditions. Plant Nutr. Fertil. Sci. 17, 852–860.
Menendez, S., Merino, P., Pinto, M., González-Murua, C., and Estavillo, J. M. (2006). 3,4-dimethylpyrazol phosphate effect on nitrous oxide, nitric oxide, ammonia and carbon dioxide emissions from grasslands. J. Environ. Qual. 35, 973l–998l. doi: 10.2134/jeq2005.0320
Monge-Muñoz, M., Urquiaga, S., Müller, C., Cambronero-Heinrichs, J. C., Zaman, M., Chinchilla-Soto, C., et al. (2021). Nitrapyrin effectiveness in reducing nitrous oxide emissions decreases at low doses of urea in an Andosol. Pedosphere 31, 303–313. doi: 10.1016/S1002-0160(20)60072-8
Nasielski, J., Earl, H., and Deen, B. (2020). Which plant traits are most strongly related to post-silking nitrogen uptake in maize under water and/or nitrogen stress? J. Plant Physiol. 244:153059. doi: 10.1016/j.jplph.2019.153059
Parkin, T. B., and Hatfield, J. L. (2010). Influence of nitrapyrin on N2O losses from soil receiving fall-applied anhydrous ammonia. Agric. Ecosyst. Environ. 136, 81–86. doi: 10.1016/j.agee.2009.11.014
Qu, Z. M., Qi, X. C., Shi, R., Zhao, Y., Hu, Z., Chen, Q., et al. (2020). Reduced N fertilizer application with optimal blend of controlled-release urea and urea improves tomato yield and quality in greenhouse production system. J. Soil Sci. Plant Nutr. 20, 1741–1750. doi: 10.1007/s42729-020-00244-8
Rahman, M. A., Sarker, M., Amin, M., Jahan, A., and Akhter, M. (2011). Yield response and nitrogen use efficiency of wheat under different doses and split application of nitrogen fertilizer. Bangladesh J. Agric. Res. 36, 231–240. doi: 10.3329/bjar.v36i2.9249
Rahmatullah Gill, M. A., Wissemeier, A. H., and Steffens, D. (2010). Phosphate availability from phosphate rock as related to nitrogen form and the nitrification inhibitor DMPP. J. Plant Nutr. Soil Sci. 169, 675–678. doi: 10.1002/jpln.200520577
Rex, A. O., and Tony, J. V. (2013). Nitrification Kinetics and Nitrous Oxide Emissions when Nitrapyrin is Coapplied with Urea–Ammonium Nitrate. Agron. J. 105, 1475–1486. doi: 10.2134/agronj2013.0184
Sangoi, L., Ernani, P. R., and Silva, P. R. F. D. (2007). Maize response to nitrogen fertilization timing in two tillage systems in a soil with high organic matter content. Rev. Bras. Cienc. Solo 31, 507–517. doi: 10.1590/S0100-06832007000300011
Schulte-Uebbing, L., and Vries, W. D. (2021). Reconciling food production and environmental boundaries for nitrogen in the European union. Sci. Total Environ. 786:147427. doi: 10.1016/j.scitotenv.2021.147427
Sun, H., Zhang, H., Powlson, D., Ju, M., and Shi, W. (2015). Rice production, nitrous oxide emission and ammonia volatilization as impacted by the nitrification inhibitor 2-chloro-6-(trichloromethyl)-pyridine. Field Crops Res. 173, 1–7. doi: 10.1016/j.fcr.2014.12.012
Sun, Z. M., Wu, Z. J., Chen, L. J., and Jia, L. B. (2008). Regulation of soil nitrification with nitrification inhibitors and related mechanisms. Chin. J. Appl. Ecol. 19, 1389–1395.
Vibart, R. E., Vogeler, I., Dodd, M., and Koolaard, J. (2016). Simple versus diverse temperate pastures: Aspects of soil-plant-animal interrelationships central to nitrogen leaching losses. Agron. J. 108, 2174–2188. doi: 10.2134/agronj2016.04.0193
Wang, S., Luo, S., Yue, S., Shen, Y., and Li, S. (2016). Fate of 15N fertilizer under different nitrogen split applications to plastic mulched maize in semiarid farmland. Nutr. Cycl. Agroecosyst. 105, 129–140. doi: 10.1007/s10705-016-9780-3
Wolt, J. D. (2004). A meta-evaluation of nitrapyrin agronomic and environmental effectiveness with emphasis on corn production in the midwestern USA. Nutr. Cycl. Agroecosyst. 69, 23–41. doi: 10.1023/B:FRES.0000025287.52565.99
Wu, Z. J., Shi, Y. F., and Chen, L. J. (2008). Progress of research on nitration inhibitory mechanism. Chin. J. Soil Sci. 39, 962–970.
Xu, D., Zhu, Y., Zhu, H., Hu, Q., Liu, G., Wei, H., et al. (2021). Effects of a one-time application of controlled-release nitrogen fertilizer on yield and nitrogen accumulation and utilization of late japonica rice in China. Agriculture 11:1041. doi: 10.3390/agriculture11111041
Yin, Y., Ying, H., Xue, Y., Zheng, H., Zhang, Q., and Cui, Z. (2019). Calculating socially optimal nitrogen (N) fertilization rates for sustainable N management in China. Sci. Total Environ. 688, 1162–1171. doi: 10.1016/j.scitotenv.2019.06.398
Yu, Y. Z., Jiao, Y., Yang, W. Z., Song, C. N., Zhang, J., and Liu, Y. B. (2022). Mechanisms underlying nitrous oxide emissions and nitrogen leaching from potato fields under drip irrigation and furrow irrigation. Agric. Water Manag. 260:107270. doi: 10.1016/j.agwat.2021.107270
Zhang, Y. T., Wang, H. Y., Lei, Q. L., Luo, J. F., Lindsey, S., Zhang, J. Z., et al. (2018). Optimizing the nitrogen application rate for maize and wheat based on yield and environment on the Northern China Plain. Sci. Total Environ. 618, 1173–1183. doi: 10.1016/j.scitotenv.2017.09.183
Keywords: fertilization method, nitrapyrin, ammonia volatilization, N2O emission, nitrogen leaching, grain yield
Citation: Ren B, Ma Z, Zhao B, Liu P and Zhang J (2022) Influences of split application and nitrification inhibitor on nitrogen losses, grain yield, and net income for summer maize production. Front. Plant Sci. 13:982373. doi: 10.3389/fpls.2022.982373
Received: 30 June 2022; Accepted: 12 August 2022;
Published: 29 August 2022.
Edited by:
Peng Hou, Chinese Academy of Agricultural Sciences (CAAS), ChinaReviewed by:
Yunshan Yang, Shihezi University, ChinaCopyright © 2022 Ren, Ma, Zhao, Liu and Zhang. This is an open-access article distributed under the terms of the Creative Commons Attribution License (CC BY). The use, distribution or reproduction in other forums is permitted, provided the original author(s) and the copyright owner(s) are credited and that the original publication in this journal is cited, in accordance with accepted academic practice. No use, distribution or reproduction is permitted which does not comply with these terms.
*Correspondence: Jiwang Zhang, and6aGFuZ0BzZGF1LmVkdS5jbg==
†These authors have contributed equally to this work
Disclaimer: All claims expressed in this article are solely those of the authors and do not necessarily represent those of their affiliated organizations, or those of the publisher, the editors and the reviewers. Any product that may be evaluated in this article or claim that may be made by its manufacturer is not guaranteed or endorsed by the publisher.
Research integrity at Frontiers
Learn more about the work of our research integrity team to safeguard the quality of each article we publish.