- 1Departamento de Biología, Área de Botánica, Universidad Autónoma de Madrid, Madrid, Spain
- 2Departmento de Biología y Geología, Física y Química Inorgánica, Universidad Rey Juan Carlos, Móstoles, Spain
- 3Centro de Investigación en Biodiversidad y Cambio Global (CIBC-UAM), Universidad Autónoma de Madrid, Madrid, Spain
- 4Sección Quíımica, Pontificia Universidad Católica del Perú, Lima, Peru
- 5School of Geography and Environment, University of Oxford, Oxfordshire, United Kingdom
- 6Departamento de Ciencias Agrarias, Universidad Científica del Sur, Villa el Salvador, Peru
- 7Departamento de Manejo Forestal, Universidad Nacional Agraria La Molina, Lima, Peru
Elucidating how environmental factors drive plant species distributions and how they affect latitudinal diversity gradients, remain essential questions in ecology and biogeography. In this study we aimed: 1) to investigate the relationships between all three diversity attributes, i.e., taxonomic diversity (TD), functional diversity (FD), and phylogenetic diversity (PD); 2) to quantify the latitudinal variation in these diversity attributes in western Amazonian terra firme forests; and 3) to understand how climatic and edaphic drivers contribute to explaining diversity patterns. We inventoried ca. 15,000 individuals from ca. 1,250 species, and obtained functional trait records for ca. 5,000 woody plant individuals in 50 plots of 0.1 ha located in five terra firme forest sites spread over a latitudinal gradient of 1200 km covering ca. 10°C in latitude in western Amazonia. We calculated all three diversity attributes using Hill numbers: q = 0 (richness), q = 1 (richness weighted by relative abundance), and q = 2 (richness weighted by dominance). Generalized linear mixed models were constructed for each diversity attribute to test the effects of different uncorrelated environmental predictors comprising the temperature seasonality, annual precipitation, soil pH and soil bulk density, as well as accounting for the effect of spatial autocorrelation, i.e., plots aggregated within sites. We confirmed that TD (q = 0, q = 1, and q = 2), FD (q = 0, q = 1, and q = 2), and PD (q = 0) increased monotonically towards the Equator following the latitudinal diversity gradient. The importance of rare species could explain the lack of a pattern for PD (q = 1 and q = 2). Temperature seasonality, which was highly correlated with latitude, and annual precipitation were the main environmental drivers of variations in TD, FD, and PD. All three diversity attributes increased with lower temperature seasonality, higher annual precipitation, and lower soil pH. We confirmed the existence of latitudinal diversity gradients for TD, FD, and PD in hyperdiverse Amazonian terra firme forests. Our results agree well with the predictions of the environmental filtering principle and the favourability hypothesis, even acting in a 10°C latitudinal range within tropical climates.
Introduction
Environmental drivers are key factors that determine plant diversity patterns at different spatial scales (McGill et al., 2006; HilleRisLambers et al., 2012; Arellano et al., 2016), but we still lack a comprehensive understanding of how environmental drivers act in hyperdiverse tropical forests (Wieczynski et al., 2019; Mori et al., 2021; Bañares de Dios et al., 2022). Diversity patterns have been traditionally explored in terms of taxonomic diversity (TD), i.e., species identity (Swenson, 2011; Swenson et al., 2012c). TD provides insights into the distribution of diversity and its underlying mechanisms (Gentry, 1982) but it does not consider two important diversity attributes: functional adaptations and evolutionary history (Swenson, 2011; Dawson et al., 2013; Chao et al., 2014; López et al., 2016). Functional diversity (FD) provides information about phenotypic adaptation to the environment and it is measured through functional traits (Cornelissen et al., 2003; Pérez-Harguindeguy et al., 2013; Córdova-Tapia & Zambrano, 2015). Due to the adaptive information provided, the functional perspective has increased in importance compared with the classic taxonomic approach for answering questions about the interactions between communities and their surrounding environment (Cornelissen et al., 2003; Violle et al., 2007; Swenson, 2012a; Poorter et al., 2017; Poorter et al., 2018). In addition, phylogenetic diversity (PD) is defined as the degree of phylogenetic relatedness among co-occurring species (López et al., 2016; Pellens & Grandcolas, 2016; Massante et al., 2019). The use of PD has grown in popularity in recent decades because the evolutionary history of communities provide insights into species relationships and functional trait evolution (Lean & Maclaurin, 2016; Pellens & Grandcolas, 2016).
Environmental drivers such as climate and edaphic properties contribute to explaining diversity patterns in tropical terra firme forests, i.e., non-flooding. Water availability and soil fertility are abiotic factors with key effects on favouring plant growth, and thus are the main drivers of variations in spatial diversity in lowland tropical forests (Gentry, 1988; Fortunel et al., 2014; Honorio Coronado et al., 2015; Poorter et al., 2017). The available water depends mainly on temperature, precipitation, and soil texture (Sollins, 1998; Clarholm & Skyllberg, 2013; Moles et al., 2014). Crucially, tropical rainforests experience important changes in water availability throughout the year due to precipitation and temperature seasonality (Malhi & Wright, 2004; Moles et al., 2014; Malizia et al., 2020). Therefore, the main climatic constraint on plant growth and survival is usually the severity and duration of the dry season (Malhi & Wright, 2004; Aubry-Kientz et al., 2015; Honorio Coronado et al., 2015), even under tropical climates. This seasonality follows a latitudinal pattern where it intensifies towards higher latitudes. Accordingly, climatic seasonality is the main driver of the global latitudinal diversity gradient, which is one of the most widely accepted diversity patterns on Earth, where TD increases from the poles towards the Equator as a result of climatic favourability (Fischer, 1960; Gentry, 1982; Willig et al., 2003; Weiser et al., 2007; Swenson et al., 2012c; Malizia et al., 2020). FD has also been shown to increase with decreasing latitude (Swenson et al., 2012b; Wieczynski et al., 2019), where the number of functional strategies increases as the climatic conditions become more benign (Fischer, 1960; Swenson et al., 2012b; Moles et al., 2014; Wieczynski et al., 2019). In woody angiosperms, PD also increases towards the Equator (Qian et al., 2013; Kerkhoff et al., 2014; Qian et al., 2017; Massante et al., 2019), partly due to the favourable climatic conditions found at lower latitudes.
Another important aspect of water availability is the soil water content, which depends greatly on the soil texture because it controls aeration, drainage and humidity retention capacity (Sollins, 1998; Rodrigues et al., 2019; Hofhansl et al., 2020). In addition, variability in soil fertility directly affects plant diversity (Sanford & Cuevas, 1996; Garibaldi et al., 2014; Bañares de Dios et al., 2022). Most terra firme tropical soils are oligotrophic but they are rich in nitrogen (Sollins, 1998) with quite acidic pH values (Herrera et al., 1978; Medina & Cuevas, 1989; Sollins, 1998). The fertility of tropical soils depends on pH because it determines the availability of base-metal cations and toxicity of aluminum (Herrera et al., 1978; Sollins, 1998; Nelson & Su, 2010; Bañares de Dios et al., 2022). Nevertheless, species seem to tolerate these low nutrient, acidic, and high aluminum conditions (Herrera et al., 1978; Medina & Cuevas, 1989; Mori et al., 2021). Hence, both the soil nutrient availability and water content play critical roles in environmental filtering (Katabuchi et al., 2012; Rodrigues et al., 2019; Hofhansl et al., 2020), which is mainly dependent on the soil pH and texture (Sollins, 1998; Nelson & Su, 2010). In addition, the soil organic matter content favours moisture retention, cation exchange and nutrient turnover, thereby affecting the soil texture and fertility and thus improving soil quality (Craswell & Lefroy, 2001; Athira et al., 2019; Bañares de Dios et al., 2022). The bulk density is determined by the soil texture and it has an inverse relationship with the soil organic matter content, so it can be used as a proxy for the soil quality (Bauer, 1974; Athira et al., 2019; de la Cruz-Amo et al., 2020).
The main goal of the present study was to investigate the patterns of woody plant diversity in western Amazonian terra firme forests along a 1,200 km latitudinal gradient. Amazonian rainforests, specifically western Amazonian forests, harbour among the most hyperdiverse floras worldwide (Gentry, 1988; Wright, 2002; Honorio Coronado et al., 2015; Brooks, 2018). Amazonian rainforests are characterized by high abundance and diversity of woody angiosperms, where a limited number of species dominate the community (Pitman et al., 2013; Draper et al., 2021). The relatively low number of dominant taxa means that tropical communities usually harbour extremely large numbers of rare species (Ter Steege et al., 2013; Leitão et al., 2016; Draper et al., 2021; Cazzolla Gatti et al., 2022). Many studies have been conducted in Amazonian rainforests over the last century, but none analysed the latitudinal gradients of woody plants within tropical regions by considering all three diversity attributes comprising TD, FD, and PD. In this study we specifically aimed: 1) to investigate the relationship between all three diversity attributes; 2) to quantify the latitudinal variations in TD, FD and PD; and 3) to understand how climatic and edaphic drivers contribute to explaining diversity patterns. We hypothesized that all three diversity attributes would increase towards equatorial latitudes, mainly due to a reduction in seasonality harshness, with differential contributions of climatic drivers and soil properties, in agreement with environmental filtering.
Materials and methods
Study area and taxonomic characterization of plant communities
This study was conducted in western Amazonian forests which comprise several forest types, but the most extended are terra firme forests (Herrera et al., 1978). Terra firme forests are highly diverse and can contain up to 300 tree species per hectare (Gentry, 1988; Valencia et al., 1994). Western Amazonian forests formed on Pliocene and Pleistocene sediments from the Andes, which are fairly young and fertile soils compared with those in central and eastern Amazonia (Pitman et al., 2001; Hoorn et al., 2010; Lamarre et al., 2012; Honorio Coronado et al., 2015; Nobre et al., 2019). These forests have low annual thermal variability, high precipitation, and relatively well-drained fertile soils (Gentry, 1988; Burnham & Johnson, 2004; Brooks, 2018).
The study area encompassed 50 plots sampled throughout five western Amazonian regions in Peru spanning a 1,200 km latitudinal gradient from –2.9496°C to –12.8270°C and covering ca. 10°C in latitude (Figure 1, Table S1). The study regions corresponded to the protected areas of Tambopata National Reserve (Madre de Dios), Buffer Zone of Yanesha Communal Reserve (Pasco), Buffer Zone of Cordillera Azul National Park (Ucayali), Río Abiseo National Park (San Martín), and Maijuna-Kichwa Regional Conservation Area (Loreto). In each region 10 plots of 0.1 ha (50 × 20 m) were established according to Arellano et al. (2016). Plots were located in areas of well conserved terra firme lowland and submontane forests according to the biome classification of Britto (2017), and they were established at least 300 m apart from each other to minimize spatial autocorrelation. All woody plant individuals, including trees, lianas, and hemiepiphytes, rooting inside the plot limits with a diameter at breast height (DBH) ≥ 2.5 cm were included. Plot based studies usually apply an inclusion criterion of DBH ≥ 10 cm, so our study included a high number of juvenile and understory individuals that are rarely considered (Gentry, 1982; Arellano et al., 2016; Draper et al., 2021), which could increase the TD and PD by including typically smaller species, as well as FD by considering more understory individuals that grow in shadier conditions (Niinemets, 2010; Weerasinghe et al., 2014). Up to three individuals from each taxon were sampled in each region to obtain herbarium sheet duplicates and replicates to measure functional traits. Collected vouchers specimens were identified and stored primarily in the USM herbarium, with some duplicates at MOL herbarium. The herbarium acronyms followed Thiers (2021). Taxonomic names were standardized using the ‘Taxostand’ package in R (Cayuela et al., 2012).
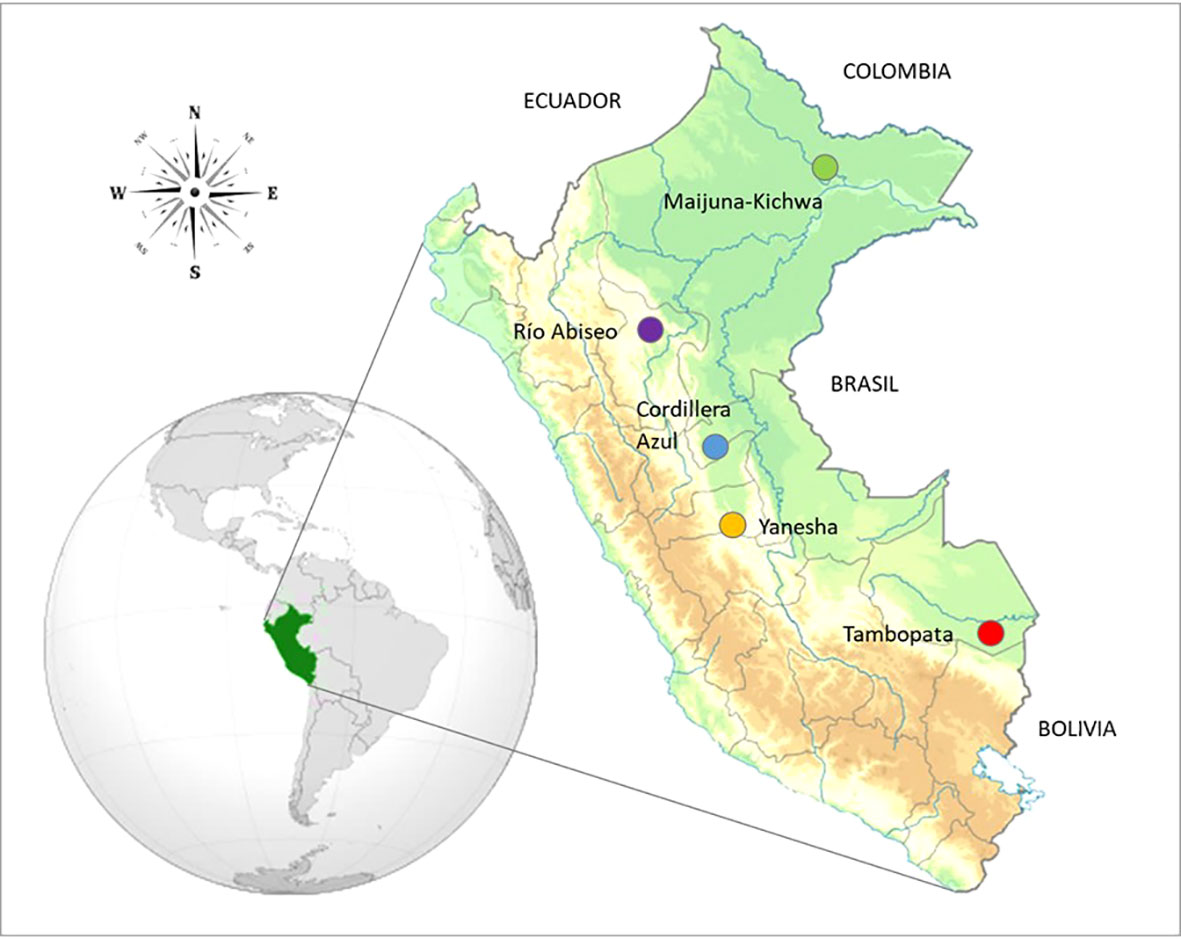
Figure 1 Map of Peru with coloured dots indicating the five regions studied in Western Amazonia. Top to bottom: Maijuna-Kichwa Regional Conservation Area, Río Abiseo National Park, Buffer Zone of Cordillera Azul National Park, Yanesha Comunal Reserve and Tambopata National Reserve.
Climatic and edaphic characterisation of study sites
Climatic data for each plot were retrieved from the CHELSA database (Karger et al., 2019). All 19 CHELSA temperature and precipitation related bioclimatic variables were considered (Table S2).
Five soil subsamples were collected in a zigzag pattern within the plot limits and mixed to obtain one representative sample for each plot. A metallic cylinder was used to extract subsamples of the first 15 cm of the soil layer below plant debris. After mixing and air drying, the soil samples were sieved through a 2 mm mesh to separate the organic fraction comprising organic matter debris, such as leaves and roots, and the coarse fraction. The remaining soil corresponded to the fine fraction which was used for chemical and textural analyses (Table S3). The pH was measured in distilled water as the real acidity and in KCl as the potential acidity. The organic carbon content and total N, S, and C contents were measured using the LECO/Dumas direct combustion method. The available Al contents and those of macronutrients (P, Ca, Mg, Na, and K) and micronutrients (Fe, Co, Cu, Mn, Ni, and Zn) were measured by extraction with the Melich III method followed by an inductively coupled plasma mass spectrometry. Textural analyses were performed using the hydrometer method after adding dispersal solution. Soil mineralogy and clay contents were measured by X-ray diffraction. The soil sampling and characterization analyses were based on the protocols described by Arellano et al. (2016).
To avoid collinearity, we calculated Pearson’s correlation coefficients (r) between all environmental variables (Figure S1), i.e., climate, soil, and latitude. Most of the environmental variables were highly correlated, so we selected two climatic and two edaphic uncorrelated variables based on previous studies. The selected climatic factors comprised the temperature seasonality (°C) and annual precipitation (mm/year) because they reflect climatic limitations on plant growth (Malhi & Wright, 2004; Aubry-Kientz et al., 2015). The selected edaphic factors comprised the pH as an approximation of soil fertility (Sollins, 1998; Nelson & Su, 2010) and the soil bulk density (g/cm3) to consider the soil texture and organic matter content (Bauer, 1974; Motavalli et al., 1995; Athira et al., 2019).
Functional characterization of plant communities
Three functional traits comprising the specific leaf area (SLA), leaf thickness (LT) and wood density (WD) were measured for each individual collected. These traits are used extensively because they are robust indicators of the functional strategies of woody plants in the leaf (Wright et al., 2004) and wood economy spectrum (Chave et al., 2009). Five mature leaves were used to measure the foliar traits. SLA (mm2/mg) was calculated as the ratio between the leaf surface area measured with a CI-202 Portable Laser Leaf Area Meter (CID Bio-Science, WA, USA) and dry mass after drying for 48 h at 80°CC. SLA measurements included all of the leaf structures. LT (mm) was measured with a digital calliper. The branch wood density was used as an approximation for WD (Swenson & Enquist, 2008). For each individual collected, a 10 cm branch section was peeled to remove the bark and its dimensions were measured with a digital calliper to calculate the fresh volume. WD (g/cm3) was calculated as the ratio between the dry mass after drying for 48 h at 80°CC and the fresh volume. All measurements and calculations followed standardized protocols (Cornelissen et al., 2003). Only individuals with records for all three functional traits were included for functional characterization. Mean trait values were calculated for each taxon.
Phylogenetic characterization of plant communities
We obtained a phylogenetic tree using the V.PhyloMaker package in R (Jin & Qian, 2019). This package allowed us to prune a pre-existing mega-tree of vascular plants based on the phylogenies of Smith and Brown (2018) and Zanne et al. (2014) for seed plants and pteridophytes, respectively, with a given list of species. The ‘phylo.maker’ function was used with the arguments nodes=nodes.info.1 and scenarios=S3.
Diversity measurements
We calculated Hill numbers for TD, FD and PD. Hill numbers only differ in terms of the parameter q, which determines the sensitivity to relative abundances (Hill, 1973). For TD (Chao et al., 2014): q = 0 denotes the species richness (i.e., number of species), q = 1 represents the richness considering relative abundance (i.e., Shannon’s diversity), and q = 2 is the richness considering dominance (i.e., inverse of Simpson’s index). Hence, the contribution of rare species to diversity decreases as the value of the parameter q increases. Hill numbers were typically only used for TD but Chao et al. (2010) extended them to PD based on the phylogenetic distances between species, and Chiu and Chao (2014) extended them to FD based on the functional distances between species traits.
By considering the Hill numbers for all three diversity attributes we can obtain a unified framework of attribute diversity, where each component is measured in different units or entities (Chao et al., 2014). TD is measured as the effective number of taxonomic entities, so the attribute value is unity for each taxon. For FD, the attribute value is the functional distance between each pair of taxa based on functional traits, and thus it is measured as the effective number of functional entities. PD is measured as the effective number of phylogenetic entities, where the attribute value is the length of each branch segment. All entities are treated as taxonomically, functionally and phylogenetically equally distinct. Hill numbers for q = 0, q = 1, and q = 2 were obtained for the three diversity attributes using the ‘renyi’ function in the vegan package for TD, with the code provided by Chiu and Chao (2014) for FD, and with the ‘ChaoPD’ function in the entropart package (Chao et al., 2010) for PD. Using different Hill numbers to explore diversity allowed us to examine how rare, abundant, and dominant species responded to the environmental factors and latitude.
Data analyses
First, we explored the relationships between different Hill numbers as well as between Hill numbers and latitude using negative binomial generalized linear models (GLMs). To fit these models, we used the ‘glm.nb’ function in the MASS package (Venables & Ripley, 2002). To account for the variance explained by the models, we calculated Cragg and Uhler’s pseudo-R-squared (pR2) using the ‘pR2’ function in the pscl package (Jackman, 2020). We then constructed negative binomial generalized linear mixed models (GLMMs) to assess the effects of climatic and edaphic variables on the Hill numbers for each diversity attribute by using the ‘glmer.nb’ function in the lme4 package (Bates et al., 2015). Environmental variables comprising the temperature seasonality, annual precipitation, soil pH and soil bulk density were included as fixed effects, whereas region (n = 5) was treated as a random factor to account for potential spatial autocorrelation among plots sampled within the same region. The latitude was not a factor itself but instead it was a surrogate for climatic gradients (Willig et al., 2003), so it was not included in the models. Nevertheless, it was indirectly considered because it was significantly negatively correlated with temperature seasonality. Temperature and seasonality have been shown to reflect the effects of latitudinal variation (Willig et al., 2003; Swenson et al., 2012b), thereby justifying our approach of using temperature seasonality as an accurate proxy for latitude in the models. Interaction terms between predictors were not included. Model selection was conducted based on Akaike’s Information Criterion corrected for small sample size (AICc). When models had a difference in AICc ≥ 2, the model with the highest AICc was considered less than optimal and rejected. When two or more models had a difference in AICc< 2, we selected the most complex to generate model predictions. We calculated two components of the pseudo-R-squared for GLMMs: marginal () and conditional () coefficients, which represent the variance explained by fixed effects and by both random and fixed effects, respectively (Nakagawa & Schielzeth, 2013). We used the ‘r.squaredGLMM’ function in the MuMIn R package (Barton, 2013). All analyses were conducted in R v4.2.1 (R Core Team, 2021).
Results
Environmental and taxonomic overview
The temperature seasonality ranged from 4.16°C in Maijuna to 9.30°C in Tambopata (Table S1) and it had a strong significant correlation with latitude (r = –0.97; Table S4). The maximum annual precipitation was recorded at Cordillera Azul with 3948 mm and the minimum at Río Abiseo with 1756 mm (Table S1), and it had no significant correlation with latitude (r = –0.09; Table S4). Among the edaphic properties, the soil bulk density and pH were not correlated with each other (r = –0.26; Table S4). Both variables were weakly correlated with the annual precipitation (bulk density: r = 0.40; pH: r = –0.29; Table S4), but not with the temperature seasonality (bulk density: r = 0.25; pH: r = –0.18; Table S4) or latitude (bulk density: r = –0.20; pH: r = 0.11; Table S4). The bulk density ranged from 0.61 to 1.00 g/cm3 and it varied fairly heterogeneously among the sites. The pH ranged from 3.78 to 5.46 (Table S1), although all of the pH values above 5.0 were determined in white sand soils in seven plots in Río Abiseo.
Overall, 14,681 individuals were inventoried in five regions belonging to 2,211 species (See Table S5 for the list of species). A total of 302 taxa were identified in Tambopata, 467 taxa in Yanesha, 511 taxa in Cordillera Azul, 154 taxa in Río Abiseo and 542 taxa in Maijuna (Table S6). Among the functional data, 274 taxa had records for all functional traits in Tambopata, 442 taxa in Yanesha, 466 taxa in Cordillera Azul, 269 taxa in Río Abiseo and 450 taxa in Maijuna (Table S6). The generated phylogenetic tree included 302 taxa present in Tambopata, 467 taxa in Yanesha, 511 taxa in Cordillera Azul, 292 taxa in Río Abiseo and 542 taxa in Maijuna (Table S6).
Correlation between diversity attributes
When all sites were considered together in the analysis, TD and FD were positively and significantly correlated with each other for all three Hill numbers q = 0 (pR2 = 0.71), q = 1 (pR2 = 0.66), and q = 2 (pR2 = 0.50) (Figure 2A; Table 1). However, when PD was considered with either TD or FD, the only Hill numbers with positive and significant correlations were q = 0 (with TD: pR2 = 0.90; with FD: pR2 = 0.81) and q = 1 (with TD: pR2 = 0.51; with FD: pR2 = 0.53) (Figures 2B, C; Table 1).
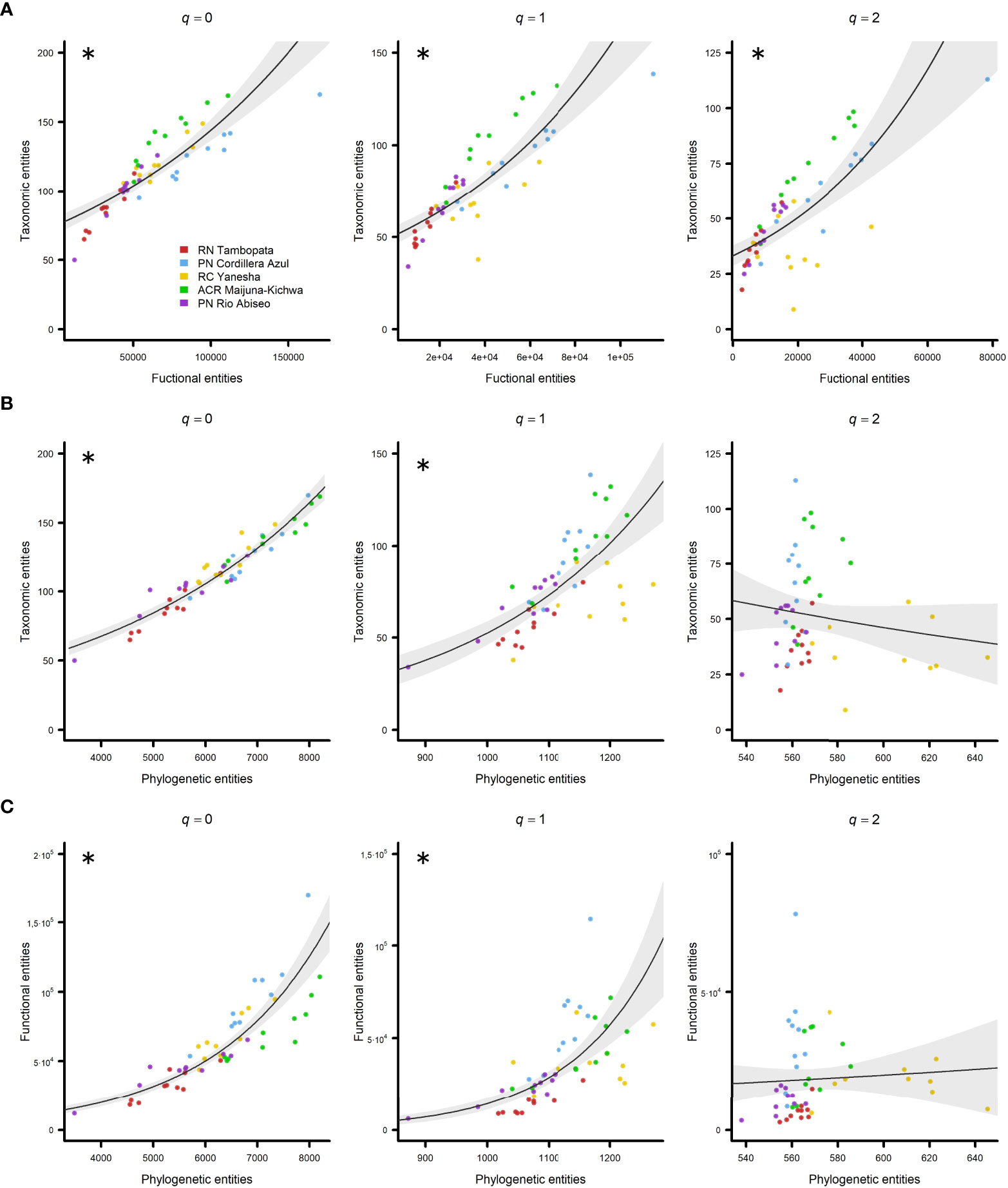
Figure 2 Relationship between diversity attributes in all five regions studied in Western Amazonia represented by negative binomial generalized linear models (GLMs) with 95% confidence intervals. (A) Taxonomic vs. functional entities, (B) taxonomic vs. phylogenetic entities, and (C) functional vs. phylogenetic entities. Hill numbers were considered as diversity indexes: q = 0 (left column), q = 1 (central column), and q = 2 (right column). Regions are represented by different colours (see the legend). Significant pseudo R-squared (pR2) values are marked with asterisks.
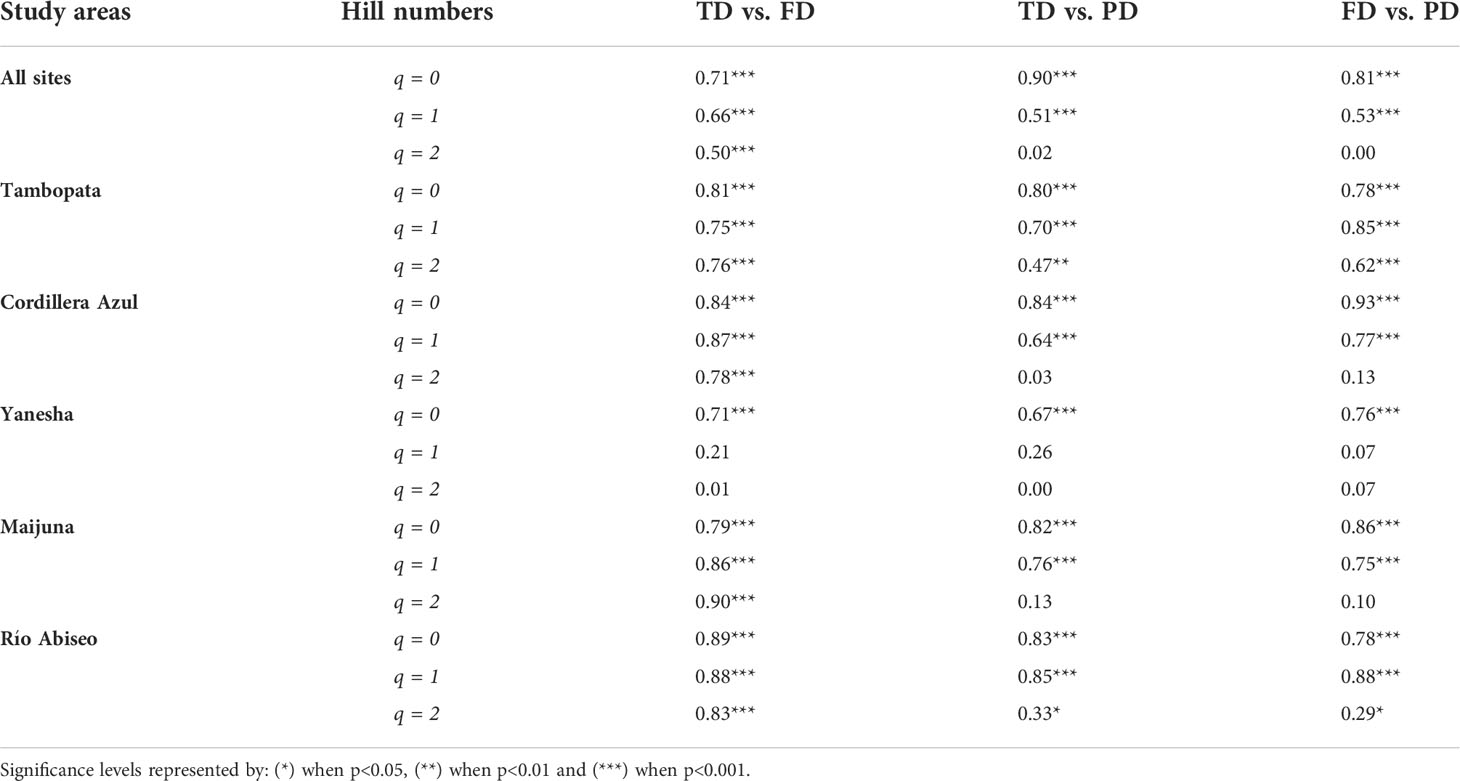
Table 1 Pseudo R-squared (pR2) values for negative binomial generalized linear models (GLMs) between diversity attributes for different Hill numbers: taxonomic diversity (TD), functional diversity (FD), and phylogenetic diversity (PD).
Latitudinal patterns
When the TD patterns were explored across latitude, significant positive relationships were found for all three Hill numbers: q = 0 (pR2 = 0.27), q = 1 (pR2 = 0.34), and q = 2 (pR2 = 0.28) (Figure 3A; Table 2), thereby indicating that diversity increases towards the Equator. For FD, all of the Hill numbers exhibited significant positive trends, but latitude explained a lower proportion of the variability in FD: q = 0 (pR2 = 0.10), q = 1 (pR2 = 0.12), and q = 2 (pR2 = 0.10) (Figure 3B; Table 2). The only Hill number for PD that had a significant positive latitudinal trend was q = 0 (pR2 = 0.27) (Figure 3C; Table 2).
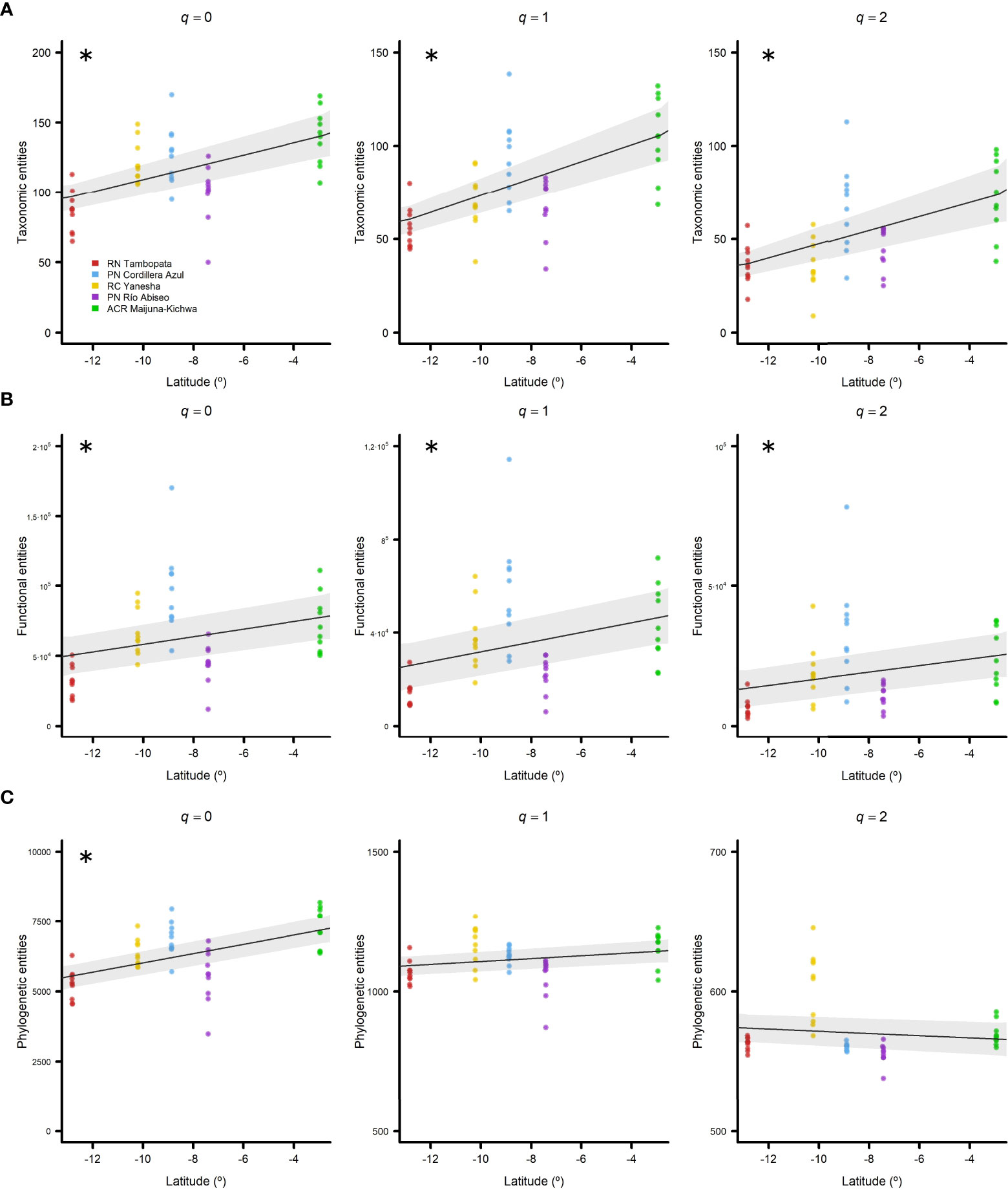
Figure 3 Diversity latitudinal trends for all five regions studied in Western Amazonia represented by negative binomial generalized linear models (GLMs) with 95% confidence intervals. (A) Taxonomic, (B) functional, and (C) phylogenetic entities found against latitude in decimal degrees. Hill numbers were considered as diversity indexes: q = 0 (left column), q = 1 (central column), and q = 2 (right column). Regions are represented by different colours (see the legend). Significant pseudo R-squared (pR2) values are marked with asterisks.
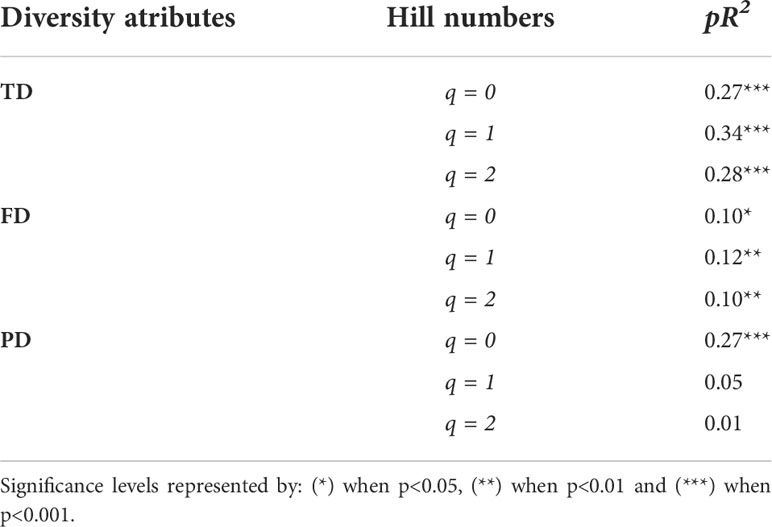
Table 2 Pseudo R-squared (pR2) values for negative binomial generalized linear models (GLMs) between latitude and diversity attributes for different Hill numbers: taxonomic diversity (TD), functional diversity (FD) and phylogenetic diversity (PD).
Effects of environmental variables on TD, FD and PD
Our results indicated monotonic increases in all diversity attributes towards lower latitudes, except when we considered relative abundances (q = 1) and dominance (q = 2) for PD (Figure 3B). Analysis of the contributions of environmental variables to diversity indicated that climatic factors seemed to be responsible for the latitudinal diversity pattern, whereas the edaphic properties appeared to have different effects on common and rare species (Figure 4). The effect of temperature seasonality was significant for all diversity attributes irrespective of whether relative abundances and dominance were considered (Figure 4).
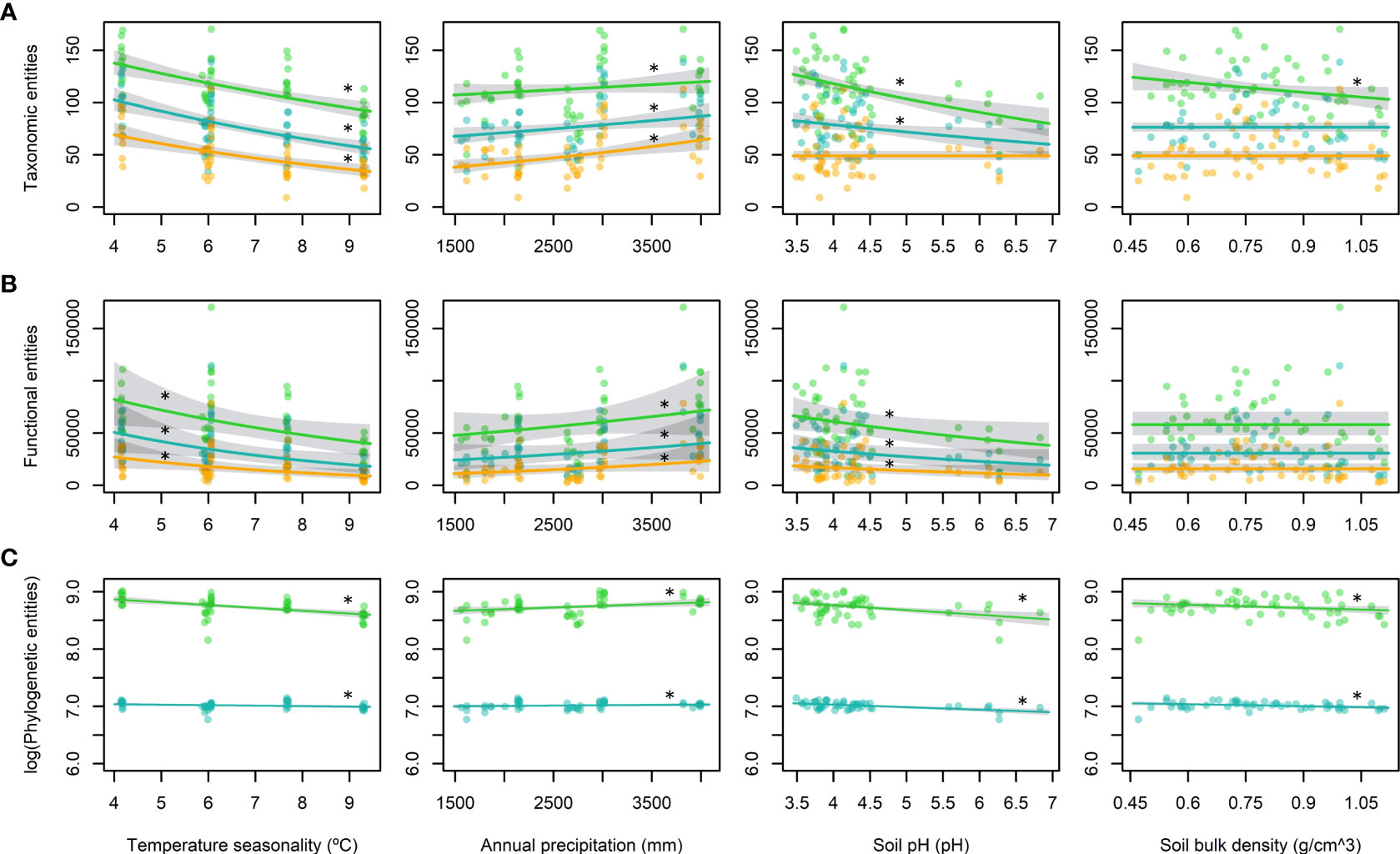
Figure 4 Predictions of the best-fit generalized linear mixed models (GLMMs) with 95% confidence intervals for each diversity attribute: (A) taxonomic entities, (B) functional entities, and (C) phylogenetic entities. Hill numbers are shown in different colours: q = 0 in green, q = 1 in blue, and q = 2 in yellow. Each column corresponds to one of the terms included in the models: temperature seasonality (left column), annual precipitation (central left column), soil pH (central right column), and soil bulk density (right column). Terms included in the best-fit model are marked with asterisks.
The best-fit model was selected for each diversity attribute and each Hill number based on the AICc values. All of the best-fit models for TD, FD, and PD included temperature seasonality, which was highly correlated with latitude (r = –0.97; Table S4), and annual precipitation as fixed terms (Figure 4; Table 3). Both the soil pH and density appeared in the best-fit models for TD with q = 0, and PD with q = 0 and q = 1 (Figure 4; Table 3). In addition, pH was also present in TD with q = 1 and all three best-fit models for FD (Figure 4; Table 3). The best-fit model for PD with q = 2 (Table 3) had no explanatory variables except for the random factor, i.e., null model.
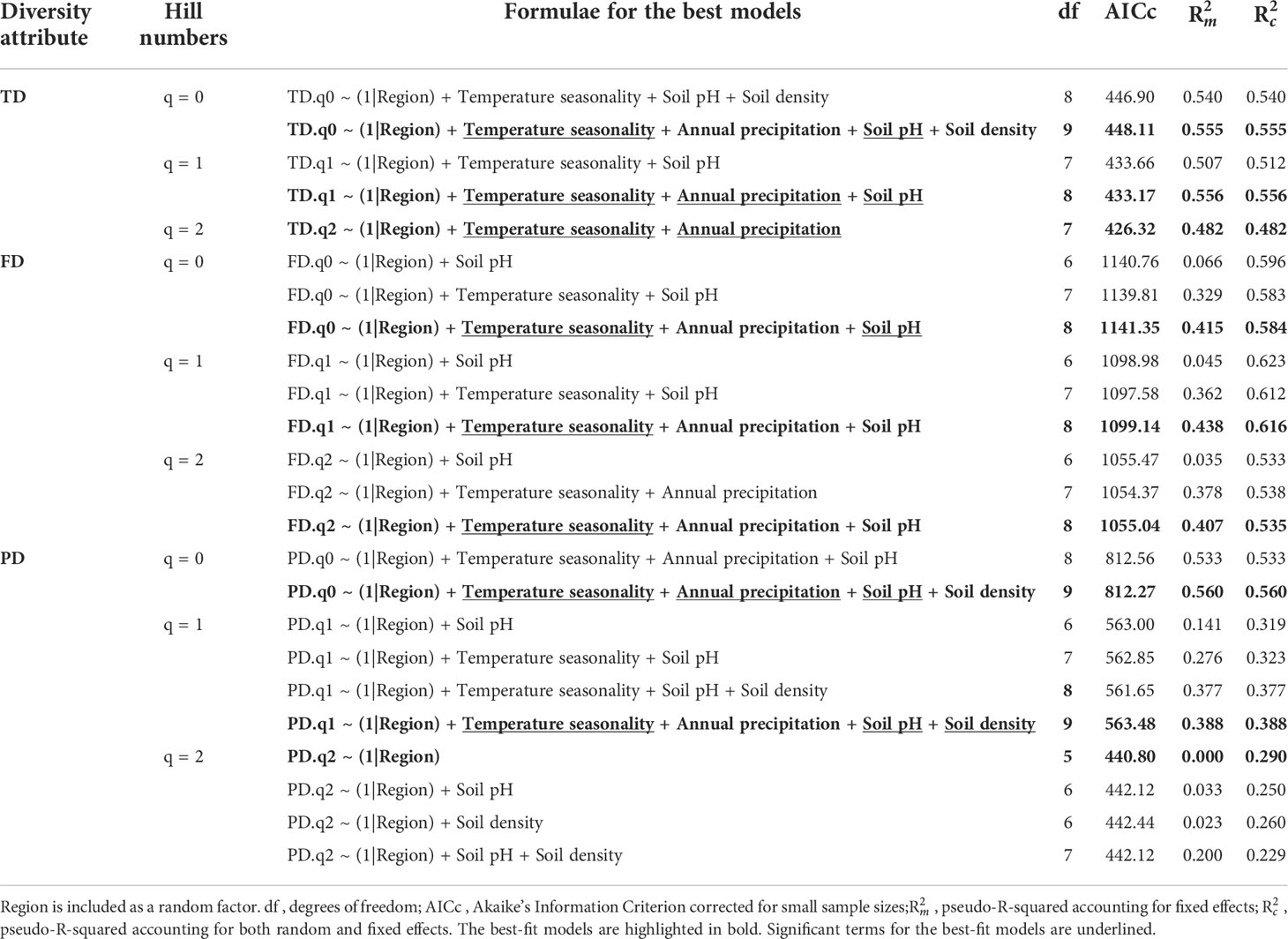
Table 3 Comparison of the best alternative models for Hill numbers of taxonomic diversity (TD), functional diversity (FD), and phylogenetic diversity (PD) as functions of temperature seasonality, annual precipitation, soil pH, and soil bulk density.
Discussion
Correlations between diversity attributes
The relationship between TD and FD is clear because, a larger potential range of functional strategies can be found when more species are present (Kooyman et al., 2012). We found that TD and FD were positively and significantly correlated (Figure 2A) even when more weight was given to abundant and dominant taxa (q = 1 and especially q = 2), thereby suggesting that although dominant species exhibited a narrower spectrum of functional strategies compared with rare species, they were not limited to the same suite of functional strategies, and thus FD increased as the number of dominant species increased (Figure 2A, q = 2). The relationship between TD and FD was in agreement with previous studies conducted at a global scale (Li et al., 2018), in the New World (Swenson et al., 2012b; Lamanna et al., 2014), and North America (Swenson & Weiser, 2014).
In addition, TD is closely related to PD, and thus they should be strongly positively correlated (Honorio Coronado et al., 2015). However, local speciation or extinction events could lead to the adaptive radiation of a few lineages and the opposite trend, i.e., high TD and low PD (Losos, 2008; Massante et al., 2019). Our results showed that TD and PD increased together (Figure 2B, q = 0 and q = 1), so there seemed to be no indication of high diversification by a few lineages as shown in a previous study (Giehl & Jarenkow, 2012). However, this was not the case when we considered dominance (Figure 2B, q = 2) because a few clades appeared to account for many of the dominant species (Draper et al., 2021).
Finally, phylogenetic relatedness involves some degree of evolutionary preservation of the adaptive strategies reflected in functional traits (Swenson et al., 2012c; López et al., 2016; Coelho de Souza et al., 2019). Thus, if FD is strongly influenced by a shared evolutionary history, there should be a positive correlation between phylogenetic proximity and ecological similarity (Webb, 2000; Webb et al., 2008; Kooyman et al., 2012). However, not all functional traits are necessarily phylogenetically conserved (Cavender-Bares et al., 2004; Swenson & Enquist, 2009; López et al., 2016), and strong adaptation of related lineages to a heterogeneous environment leads to high FD and low PD, thereby inverting the trend (Losos, 2008; López et al., 2016). The positive relationship between FD and PD (Figure 2C, q = 0 and q = 1) suggested that the functional traits in our study were phylogenetically conserved. However, when dominance was considered (q = 2), the dominant clades probably had a limited set of functional adaptations and/or these traits were not phylogenetically conserved. The absence of significant relationships between TD–PD and FD–PD when considering dominance (Figures 2B, C, q = 2) were probably due to seven plots in Yanesha with surprisingly high PD but relatively low TD, i.e., a few species from distant lineages dominated the community, and FD, i.e., a few functional strategies that appeared in distant lineages dominated the community.
Regardless of the causes, the positive relationships between the three diversity attributes showed that if we take action to preserve and conserve communities with high TD, we are also indirectly preserving FD and PD, at least in western Amazonian terra firme forests.
Latitudinal patterns
Overall, our results indicated that the three diversity attributes increased monotonically towards the Equator in western Amazonian terra firme forests (Figure 3), where they consistently followed the latitudinal diversity gradient. These findings agree well with the predictions of the environmental filtering principle, which states that communities are shaped by abiotic deterministic factors (Götzenberger et al., 2012; López et al., 2016; Poorter et al., 2017), and the favourability hypothesis, which maintains that the effect of environmental filtering becomes more restrictive when the environmental conditions are less favourable (Swenson et al., 2012b; Lamanna et al., 2014; Wieczynski et al., 2019), and thus they forecast that TD, FD, and PD will increase towards lower latitudes due to climatic favourability (Fischer, 1960; Weiser et al., 2007; Swenson et al., 2012c; Qian et al., 2013; Qian et al., 2017; Kubota et al., 2018; Massante et al., 2019; Wieczynski et al., 2019). In addition, our results showed that the latitudinal decrease in all three diversity attributes was noticeable within tropical areas, even in a 10°C latitudinal gradient. Thus, although tropical environments have benign climatic conditions in global terms, environmental filtering and climatic favourability are still important for these species-rich communities (Fortunel et al., 2014). In fact, several studies found that environmental filtering had a stronger effect in species-rich communities, such as tropical forests, compared with species-poor communities (Lamanna et al., 2014; Swenson & Weiser, 2014; Li et al., 2018)
We found a monotonic increase in TD with decreasing latitude, which was consistent for all Hill numbers (Figure 3A), thereby indicating that this pattern remained regardless of whether the relative abundances (q = 1) and dominance (q = 2) of taxa were considered. Our results showed that TD increased towards the Equator, which is a widely accepted trend (Fischer, 1960; Gentry, 1982; Willig et al., 2003; Weiser et al., 2007). Some recent studies of latitudinal gradients obtained similar results in terms of the species richness in North America (Qian et al., 2013) and South America (Cazzolla Gatti et al., 2022).
Our results indicated a monotonic increase in FD with decreasing latitude (Figure 3B), which is in agreement with previous studies of woody plants in the New World (Swenson et al., 2012b; Lamanna et al., 2014). This finding was consistent for all Hill numbers, thereby indicating that this pattern remained regardless of whether relative abundances (q = 1) and dominance (q = 2) were considered. The reduction in functional entities when abundance and dominance were considered could be explained by the absence of rare species with rare ecological strategies, i.e., uncommon combinations of functional traits, which were greatly responsible for the FD of the community (Mouillot et al., 2013; Leitão et al., 2016).
Finally, we found a monotonic increase in PD of woody plants with decreasing latitude only for the Hill number of q = 0 (Figure 3C). This pattern is consistent with recent studies of woody angiosperms in the New World (Kerkhoff et al., 2014), North America (Qian et al., 2013), and even at a global scale (Qian et al., 2017). The results obtained by Massante et al. (2019) appear to contradict ours because they found a worldwide increase in PD of woody plants towards higher latitudes. However, they also considered gymnosperms in their analyses, which usually have greater tolerance of harsher conditions at high latitudes, and the trend inverted when they were excluded. In addition, no significant trend was found between PD and latitude when relative abundances and dominance were considered (Figure 3C, q = 1 and q = 2, respectively), which remained fairly constant along the latitudinal gradient. The decrease in phylogenetic entities and loss of the latitudinal pattern can be explained by the high contribution of rare species to diversity in species-rich tropical communities (Leitão et al., 2016; Lamanna et al., 2017; Cazzolla Gatti et al., 2022).
The presence and persistence of rare species is inversely correlated with latitude in tropical forests worldwide (Lamanna et al., 2017). In addition, the diversity of all species increases towards lower latitudes, but the diversity of rare species appears to increase more steeply than those of common or dominant species when approaching the Equator (Stevens & Willig, 2002; Lamanna et al., 2017). Thus, the loss of a significant latitudinal pattern for PD (q = 1 and q = 2) in the present study suggests important contributions of rare species to the maintenance of PD in western Amazonian terra firme forests (Leitão et al., 2016).
Effects of environmental variables on TD, FD, and PD
The overall diversity tended to increase as the temperature seasonality decreased, annual precipitation increased, soil pH decreased, and soil bulk density decreased, although to a lesser extent for the latter (Figure 4). Temperature seasonality seemed to be the most important environmental driver for shaping diversity trends because it was included as a significant factor in all of the best-fit models for all three diversity attributes, where the only exception was PD with q = 2 (Table 3). Annual precipitation was also included in all of the models but it was a significant factor only for TD with q = 1 and q = 2, and PD with q = 0 (Table 3), thereby suggesting that seasonal temperature variation was more important for shaping diversity than total water discharge. Similarly, previous studies consistently found that temperature variables had greater power for explaining diversity patterns than precipitation variables (Giehl & Jarenkow, 2012; Moles et al., 2014; Chen et al., 2015). Our findings are also consistent with environmental filtering and the favourability hypothesis for all diversity attributes because diversity increases with lower temperature seasonality and higher precipitations (Fischer, 1960; Swenson et al., 2012b; Qian et al., 2013; Asefa et al., 2017; Wieczynski et al., 2019).
Among the edaphic factors, the soil pH was present in all of the best-fit models, except for TD with q = 2, and it was a significant fixed factor in the best-fit models for TD with q = 0 and q = 1, FD with q = 0, and PD with q = 0 and q = 1 (Table 3). Our results showed that the diversity was greater when the soil was more acidic, which suggests that these communities are adapted to high soil acidity conditions (pH 3.5–4.5), although soils with a pH under 5.3 are characterized by low availability of base metal cation and toxic aluminium concentrations (Sollins, 1998; Bañares de Dios et al., 2022). In addition, the soil bulk density only appeared in the best-fit models for TD with q = 0, and PD with q = 0 and q = 1 (Table 3). Diversity increased as the bulk density decreased and the soils had lower bulk density values of 0.47 to 1.11 g/cm3 than average ones for fully mineral soils, i.e., clay = 1.0 to 1.6 g/cm3 and sand = 1.2 to 1.8 g/cm3 (Athira et al., 2019), which suggests that the organic matter content increased as the bulk density decreased (Bauer, 1974; Athira et al., 2019; de la Cruz-Amo et al., 2020). Therefore, the soil organic matter content may have facilitated the adaptation of tropical plants to soils with low nutrient contents, high aluminium concentrations and low pH. Tropical soils are regarded as depleted of organic matter but large amounts of organic material are continuously supplied by decaying vegetation and its breakdown usually occurs very fast in the tropics, with rapid mineralization and availability for uptake (Herrera et al., 1978; Craswell & Lefroy, 2001; de la Cruz-Amo et al., 2020).
Overall, we found that TD increased as the temperature seasonality decreased, precipitation increased, and soil acidity increased (Figure 4A). Previous studies have demonstrated that the species richness of woody plants is negatively correlated with temperature seasonality, and positively correlated with the mean annual temperature and soil water content (Hofhansl et al., 2020). When we considered dominance (q = 2), only climatic factors were included in the best-fit model. This suggests that dominant species with wider tolerances and more widespread distributions were more dependent on the climate than the soils compared with rarer or less abundant species, which seemed more related to the local edaphic conditions (Pitman et al., 2013; Arellano et al., 2014).
According to our results, FD was affected by the temperature seasonality, annual precipitation and soil pH (Figure 4B), even when relative abundances and dominance were considered (q = 1 and q = 2, respectively). These results confirm that functional strategies are a consequence of environmental filtering because abiotic factors affect FD of both rare and common species in a similar manner. Therefore, although tropical climatic conditions are benign, they still strongly condition the successful functional strategies (Fortunel et al., 2014; Lamanna et al., 2014), thereby agreeing with the predictions of the favourability hypothesis in tropical environments. Recent studies obtained similar results for climatic and edaphic drivers (Swenson, 2012a; Molina-Venegas et al., 2018; Hofhansl et al., 2020).
We found that PD increased as the temperature seasonality decreased, precipitation increased, soil acidity increased, and bulk density decreased (Figure 4C). When traits are phylogenetically conserved, PD increases with decreasing environmental harshness, i.e., increasing temperature or precipitation (Qian et al., 2013; Satdichanh et al., 2015; Qian et al., 2017). Therefore, environmental filtering and the favourability hypothesis are consistent with our results for PD with q = 0 and q = 1. Recent studies also showed that climatic filtering and soil fertility shaped PD of woody angiosperms (Honorio Coronado et al., 2015; Kubota et al., 2018). The lack of a best-fit model other than the null model for PD with q = 2 could be explained by the fact that dominant clades probably appeared and expanded under conditioning by their dispersion abilities and historical factors which are not reflected in the actual environmental conditions (Pitman et al., 2001; Arellano et al., 2016).
Conclusions
The latitudinal diversity gradient is explained by the environmental filtering principle and favourability hypothesis through climatic factors. Increases in temperature and precipitation seasonality are found in tropical regions towards higher latitudes (Fortunel et al., 2014), thereby leading to a noticeable latitudinal diversity gradient on a global scale but also within narrower latitudinal ranges such as the tropics. We found that temperature seasonality was still a relevant driver of all diversity attributes comprising TD, FD, and PD within a 10°C latitudinal gradient in the Neotropics. Therefore, our results support environmental filtering and the favourability hypothesis within hyperdiverse tropical regions.
In addition, the positive relationships found between the three diversity attributes indicate that if we take measures to protect and conserve TD, we also indirectly protect FD and PD, at least in western Amazonian terra firme forests. Furthermore, several studies found effects of TD, FD, and PD on productivity, and thus preserving forests with multiple functional strategies and a great evolutionary legacy will also protect their vital ecosystem functions (Balvanera et al., 2006; Gamfeldt et al., 2008; Cadotte, 2013; Coelho de Souza et al., 2019). Future studies should aim to understand diversity from this holistic perspective.
Data availability statement
The raw data supporting the conclusions of this article will be made available by the authors, without undue reservation.
Author contributions
CB, GB, MM and LC conceived the ideas. CB, JA and GB collected the data. NS and MTC provided bureaucratic, logistic and field support. CB and GB analyzed the data. CS led the writing. GB, JA, LM-G, LC, and MM gave continuous input to the manuscript writing and statistical analyses. All authors contributed to the article and approved the submitted version.
Funding
The appropriate permits were obtained to collect material in the field: Área de Conservación Regional Maijuna-Kichwa No. 003-2019-GLR-GGR-ARA, Parque Nacional Río Abiseo No. 001-2016-SERNANP-PNRA-JEF, Zona de Amortiguamientó Parque Nacional Cordillera Azul No. 315-2017-SERFOR-DGGSPFFS, Zona de Amortiguamiento Reserva Comunal Yanesha No. 401-2018-MINAGRISERFOR-DGGSPFFS, Reserva Nacional Tambopata No. 35-2017-SERNANP-DGANP.
Acknowledgments
We would like to express our most sincere gratitude to the native communities of Nueva Vida, Leoncio Prado, San Carlos, Yamino and Infierno for receiving us so kindly in their homes and forests, and for their crucial assistance in the field. We would also like to thank the Peruvian local authorities of Servicio Nacional de Áreas Naturales Protegidas por el Estado (SERNANP), Servicio Nacional Forestal (SERFOR), and Autoridad Regional Ambiental del Gobierno Regional de Loreto (ARA-GOREL) for assistance and support that made the expeditions possible in the sampled protected areas. We offer our most sincere gratitude to Victoria Cala, who performed the edaphic analyses, for her much appreciated contribution. We show our special appreciation to Luis Torres Montenegro for its valuable taxonomic and logistic work; Mara Paneghel for her crucial and most valuable help; Manuel Marca for his assistance in the field; Camino Monsalve for her contribution to the functional traits; and Iñigo Gómez, Maaike Pyck and Silvia Aguado for their much appreciated volunteer work.
Conflict of interest
The authors declare that the research was conducted in the absence of any commercial or financial relationships that could be construed as a potential conflict of interest.
Publisher’s note
All claims expressed in this article are solely those of the authors and do not necessarily represent those of their affiliated organizations, or those of the publisher, the editors and the reviewers. Any product that may be evaluated in this article, or claim that may be made by its manufacturer, is not guaranteed or endorsed by the publisher.
Supplementary material
The Supplementary Material for this article can be found online at: https://www.frontiersin.org/articles/10.3389/fpls.2022.978299/full#supplementary-material
References
Arellano, G., Cala, V., Fuentes, A., Cayola, L., Jørgensen, P. M., Macía, M. J. (2016). A standard protocol for woody plant inventories and soil characterisation using temporary 0.1-ha plots in tropical forests. J. Trop. For. Sci. 28 (4), 508–516.
Arellano, G., Cala, V., Macía, M. J. (2014). Niche breadth of oligarchic species in Amazonian and Andean rain forests. J. Veg. Sci. 25 (6), 1355–1366. doi: 10.1111/jvs.12180
Arellano, G., Jørgensen, P. M., Fuentes, A. F., Loza, M. I., Torrez, V., Macía, M. J. (2016). Oligarchic patterns in tropical forests: Role of the spatial extent, environmental heterogeneity and diversity. J. Biogeogr. 43 (3), 616–626. doi: 10.1111/jbi.12653
Arellano, G., Tello, J. S., Jørgensen, P. M., Fuentes, A. F., Loza, M. I., Torrez, V., et al. (2016). Disentangling environmental and spatial processes of community assembly in tropical forests from local to regional scales. Oikos 125 (3), 326–335. doi: 10.1111/oik.02426
Asefa, M., Cao, M., Zhang, G., Ci, X., Li, J., Yang, J. (2017). Environmental filtering structures tree functional traits combination and lineages across space in tropical tree assemblages. Sci. Rep. 7 (1), 1–11. doi: 10.1038/s41598-017-00166-z
Athira, M., Jagadeeswaran, R., Kumaraperumal, R. (2019). Influence of soil organic matter on bulk density in coimbatore soils. Int. J. Chem. Stud. 7 (3), 3520–3523.
Aubry-Kientz, M., Rossi, V., Wagner, F. (2015). Identifying climatic drivers of tropical forest dynamics. Biogeosciences 12 (19), 5583–5596. doi: 10.5194/bgd-12-1-2015
Balvanera, P., Pfisterer, A. B., Buchmann, N., He, J. S., Nakashizuka, T., Raffaelli, D., et al. (2006). Quantifying the evidence for biodiversity effects on ecosystem functioning and services. Ecol. Lett. 9 (10), 1146–1156. doi: 10.1111/j.1461-0248.2006.00963.x
Bañares-de-Dios, G., Macía, M. J., de Carvalho, G. M., Arellano, G., Cayuela, L. (2022). Soil and climate drive floristic composition in tropical Forests : A literature review. Front. Ecol. Evol. 10, 866905. doi: 10.3389/fevo.2022.866905
Barton, K. (2013). MuMIn: Multi-model inference. R package version1.43.17. Available at: https://CRAN.Rproject.org/package=MuMIn.
Bates, D., Mächler, M., Ben, B., Walker, S. (2015). Fitting linear mixed-effects models using lme4. J. Stat. Softw. 67 (1), 1–48. doi: 10.18637/jss.v067.i01.Corresponding
Bauer, A. (1974). Influence of soil organic matter on bulk density and available water capacity of soils. Farm Res. 31 (5), 44–52.
Britto, B. (2017). Actualización de las ecorregiones terrestres de perú propuestas en el libro rojo de plantas endémicas del perú. Gayana Botánica 74 (1), 15–29. doi: 10.4067/s0717-66432017005000318
Brooks, D. (2018). Tropical and subtropical moist broadleaf forests. World Wildlife Fund (WWF), Central South America: Bolivia and Argentina. Available at: https://www.worldwildlife.org/ecoregions/nt0165.
Burnham, R. J., Johnson, K. R. (2004). South American palaeobotany and the origins of neotropical rainforests. Philos. Trans. R. Soc. B: Biol. Sci. 359 (1450), 1595–1610. doi: 10.1098/rstb.2004.1531
Cadotte, M. W. (2013). Experimental evidence that evolutionarily diverse assemblages result in higher productivity. Proc. Natl. Acad. Sci. United States America 110 (22), 8996–9000. doi: 10.1073/pnas.1301685110
Cavender-Bares, J., Ackerly, D. D., Baum, D. A., Bazzaz, F. A. (2004). Phylogenetic overdispersion in Floridian oak communities. Am. Nat. 163 (6), 823–843. doi: 10.1086/386375
Cayuela, L., Granzow-de la Cerda, Í., Albuquerque, F. S., Golicher, D. J. (2012). Taxonstand: An r package for species names standardisation in vegetation databases. Methods Ecol. Evol. 3 (6), 1078–1083. doi: 10.1111/j.2041-210X.2012.00232.x
Cazzolla Gatti, R., Reich, P. B., Gamarra, J. G., Crowther, T., Hui, C., Morera, A., et al. (2022). The number of tree species on earth. Proc. Natl. Acad. Sci. 119 (6), 1–11. doi: 10.1073/pnas.2115329119/-/DCSupplemental.Published
Chao, A., Chiu, C. H., Jost, L. (2010). Phylogenetic diversity measures based on hill numbers. Philos. Trans. R. Soc. B: Biol. Sci. 365 (1558), 3599–3609. doi: 10.1098/rstb.2010.0272
Chao, A., Chiu, C. H., Jost, L. (2014). Unifying species diversity, phylogenetic diversity, functional diversity, and related similarity and differentiation measures through hill numbers. Annu. Rev. Ecol. Evol. Syst. 45, 297–324. doi: 10.1146/annurev-ecolsys-120213-091540
Chave, J., Coomes, D., Jansen, S., Lewis, S. L., Swenson, N. G., Zanne, A. E. (2009). Towards a worldwide wood economics spectrum. Ecol. Lett. 12 (4), 351–366. doi: 10.1111/j.1461-0248.2009.01285.x
Chen, S.B., Ferry Slik, J. W., Gao, J., Mao, L. F., Bi, M. J., Shen, M. W., et al. (2015). Latitudinal diversity gradients in bryophytes and woody plants: Roles of temperature and water availability. J. Syst. Evol. 53 (6), 535–545. doi: 10.1111/jse.12158
Chiu, C. H., Chao, A. (2014). Distance-based functional diversity measures and their decomposition: A framework based on hill numbers. PloS One 9 (7), 1–17. doi: 10.1371/journal.pone.0100014
Clarholm, M., Skyllberg, U. (2013). Translocation of metals by trees and fungi regulates pH, soil organic matter turnover and nitrogen availability in acidic forest soils. Soil Biol Biochem 63 (2013), 142–153. doi: 10.1016/j.soilbio.2013.03.019
Coelho de Souza, F., Dexter, K. G., Phillips, O. L., Pennington, R. T., Neves, D., Sullivan, M. J. P., et al. (2019). Evolutionary diversity is associated with wood productivity in Amazonian forests. Nat. Ecol. Evol. 3 (12), 1754–1761. doi: 10.1038/s41559-019-1007-y
Córdova-Tapia, F., Zambrano, L. (2015). La diversidad funcional en la ecología de comunidades. Ecosistemas 24 (3), 78–87. doi: 10.7818/ecos.2015.24-3.10
Cornelissen, J. H. C., Lavorel, S., Garnier, E., Díaz, S., Buchmann, N., Gurvich, D. E., et al. (2003). A handbook of protocols for standardised and easy measurement of plant functional traits worldwide. Aust. J. Bot. 51 (4), 335–380. doi: 10.1071/BT02124
Craswell, E. T., Lefroy, R. D. B. (2001). The role and function of organic matter in tropical soils. NIn Managing organic matter in tropical soils: Scope and limitations. Dordrecht: Springer pp. 7–18. doi: 1978-1-4613-1163-8_10/978-94-017-2172-1_2
Dawson, M. N., Algar, A. C., Antonelli, A., Dávalos, L. M., Davis, E., Early, R., et al. (2013). An horizon scan of biogeography. Front. Biogeogr. 5 (2), 130–157. doi: 10.21425/f5fbg18854
de la Cruz-Amo, L., Bañares-de-Dios, G., Cala, V., Granzow-de la Cerda, Í., Espinosa, C. I., Ledo, A., et al. (2020). Trade-offs among aboveground, belowground, and soil organic carbon stocks along altitudinal gradients in Andean tropical montane forests. Front. Plant Sci. 11. doi: 10.3389/fpls.2020.00106
Draper, F. C., Costa, F. R. C., Arellano, G., Phillips, O. L., Duque, A., Macía, M. J., et al. (2021). Amazon Tree dominance across forest strata. Nat. Ecol. Evol. 5 (6), 757–767. doi: 10.1038/s41559-021-01418-y
Fischer, A. G. (1960). Latitudinal variations in organic diversity. Evolution 14 (1), 64–81. doi: 10.2307/2405923
Fortunel, C., Paine, C. E. T., Fine, P. V. A., Kraft, N. J. B., Baraloto, C. (2014). Environmental factors predict community functional composition in Amazonian forests. J. Ecol. 102 (1), 145–155. doi: 10.1111/1365-2745.12160
Gamfeldt, L., Hillebrand, H., Jonsson, P. R. (2008). Multiple functions increase the importance of biodiversity for overall ecosystem functioning. Ecology 89 (5), 1223–1231. doi: 10.1890/06-2091.1
Garibaldi, C., Nieto-Ariza, B., Macía, M. J., Cayuela, L. (2014). Soil and geographic distance as determinants of floristic composition in the azuero peninsula (Panama). Biotropica 46 (6), 687–695. doi: 10.1111/btp.12174
Gentry, A. H. (1982). Patterns of Neotropical plant species diversity. Evol. Biol., 1–84. Springer, Boston, MA. doi: 1978-1-4613-1163-8_10/978-1-4615-6968-8_1
Gentry, A. H. (1988). Tree species richness of upper Amazonian forests. Proc. Natl. Acad. Sci. 85 (1), 156–159. doi: 10.1073/pnas.85.1.156
Giehl, E. L. H., Jarenkow, J. A. (2012). Niche conservatism and the differences in species richness at the transition of tropical and subtropical climates in south America. Ecography 35 (10), 933–943. doi: 10.1111/j.1600-0587.2011.07430.x
Götzenberger, L., de Bello, F., Bråthen, K. A., Davison, J., Dubuis, A., Guisan, A., et al. (2012). Ecological assembly rules in plant communities-approaches, patterns and prospects. Biol. Rev. 87 (1), 111–127. doi: 10.1111/j.1469-185X.2011.00187.x
Herrera, R., Klinge, H., Medina, E. (1978). Amazon Ecosystems: their structure and functioning with particular emphasis on nutrients. Interciencia 3 (4), 223–231.
Hill, M. O. (1973). Diversity and Evenness : A unifying notation and its consequences. Ecology 54(2), 427–432. doi: 10.2307/1934352
HilleRisLambers, J., Adler, P. B., Harpole, W. S., Levine, J. M., Mayfield, M. M. (2012). Rethinking community assembly through the lens of coexistence theory. Annu. Rev. Ecol. Evol. Syst. 43 (1), 227–248. doi: 10.1146/annurev-ecolsys-110411-160411
Hofhansl, F., Chacón-Madrigal, E., Fuchslueger, L., Jenking, D., Morera-Beita, A., Plutzar, C., et al. (2020). Climatic and edaphic controls over tropical forest diversity and vegetation carbon storage. Sci. Rep. 10 (1), 1–11. doi: 10.1038/s41598-020-61868-5
Honorio Coronado, E. N., Dexter, K. G., Pennington, R. T., Chave, J., Lewis, S. L., Alexiades, M. N., et al (2015) Phylogenetic diversity of Amazonian tree communities Diversity Distrib. 21(11), 1295–1307. doi: 10.1111/ddi.12357
Hoorn, C., Wesselingh, F. P., ter Steege, H., Bermudez, M. A., Mora, A., Sevink, J., et al. (2010). Amazonia Through Time : Andean uplift, climate change, landscape evolution, and biodiversity. Science 330, 927–931. doi: 10.1126/science.1194585
Jackman, S. (2020). pscl: Classes and methods for R. Department of Political Science (Stanford, CA: Stanford University).
Jin, Y., Qian, H. (2019). V.PhyloMaker: an r package that can generate very large phylogenies for vascular plants. Ecography 42 (8), 1353–1359. doi: 10.1111/ecog.04434
Karger, D. N., Conrad, O., Böhner, J., Kawohl, T., Kreft, H., Soria-Auza, R. W., et al. (2019). Climatologies at high resolution for the earth land surface areas. CHELSA V1. 2: Technical specification. Swiss Federal Research Institute WSL, Switzerland. doi: 10.1594/WDCC/CHELSA
Katabuchi, M., Kurokawa, H., Davies, S. J., Tan, S., Nakashizuka, T. (2012). Soil resource availability shapes community trait structure in a species-rich dipterocarp forest. J. Ecol. 100 (3), 643–651. doi: 10.1111/j.1365-2745.2011.01937.x
Kerkhoff, A. J., Moriarty, P. E., Weiser, M. D. (2014). The latitudinal species richness gradient in new world woody angiosperms is consistent with the tropical conservatism hypothesis. Proc. Natl. Acad. Sci. United States America 111 (22), 8125–8130. doi: 10.1073/pnas.1308932111
Kooyman, R., Rossetto, M., Allen, C., Cornwell, W. (2012). Australian Tropical and subtropical rain forest community assembly: Phylogeny, functional biogeography, and environmental gradients. Biotropica 44 (5), 668–679. doi: 10.1111/j.1744-7429.2012.00861.x
Kubota, Y., Kusumoto, B., Shiono, T., Ulrich, W. (2018). Environmental filters shaping angiosperm tree assembly along climatic and geographic gradients. J. Veg. Sci. 29 (4), 607–618. doi: 10.1111/jvs.12648
Lamanna, C., Blonder, B., Violle, C., Kraft, N. J. B., Sandel, B., Šímová, I., et al. (2014). Functional trait space and the latitudinal diversity gradient. Proc. Natl. Acad. Sci. U. S. A. 111 (38), 13745–13750. doi: 10.1073/pnas.1317722111
Lamanna, J. A., Mangan, S. A., Alonso, A., Bourg, N. A., Brockelman, W. Y., Bunyavejchewin, S., et al. (2017). Plant diversity increases with the strength of negative density dependence at the global scale. Science 356 (6345), 1389–1392. doi: 10.1126/science.aam5678
Lamarre, G. P. A., Baraloto, C., Fortunel, C., Dávila, N., Mesones, I., Rios, J. G., et al. (2012). Herbivory, growth rates, and habitat specialization in tropical tree lineages: Implications for Amazonian beta-diversity. Ecology 93 (sp8), 195–210. doi: 10.1890/11-0397.1
Lean, C., Maclaurin, J. (2016). The value of phylogenetic diversity. In Biodiversity conservation and phylogenetic systematics. Cham: Springer. (pp. 19–37).
Leitão, R. P., Zuanon, J., Villéger, S., Williams, S. E., Baraloto, C., Fortune, C., et al. (2016). Rare species contribute disproportionately to the functional structure of species assemblages. Proc. R. Soc. B: Biol. Sci. 283 (1828), 1–9. doi: 10.1098/rspb.2016.0084
Li, Y., Shipley, B., Price, J. N., Dantas, V., de, L., Tamme, R., et al. (2018). Habitat filtering determines the functional niche occupancy of plant communities worldwide. J. Ecol. 106 (3), 1001–1009. doi: 10.1111/1365-2745.12802
López, B. E., Burgio, K. R., Carlucci, M. B., Palmquist, K. A., Parada, A., Weinberger, V. P., et al. (2016). A new framework for inferring community assembly processes using phylogenetic information, relevant traits and environmental gradients. One Ecosyst. 1, 1–24. doi: 10.3897/oneeco.1.e9501
Losos, J. B. (2008). Phylogenetic niche conservatism, phylogenetic signal and the relationship between phylogenetic relatedness and ecological similarity among species. Ecol. Lett. 11 (10), 995–1003. doi: 10.1111/j.1461-0248.2008.01229.x
Malhi, Y., Wright, J. (2004). Spatial patterns and recent trends in the climate of tropical rainforest regions. Philos. Trans. R. Soc. B: Biol. Sci. 359 (1443), 311–329. doi: 10.1098/rstb.2003.1433
Malizia, A., Blundo, C., Carilla, J., Acosta, O. O., Cuesta, F., Duque, A., et al. (2020). Elevation and latitude drives structure and tree species composition in Andean forests: Results from a large-scale plot network. PloS One 15 (4), 1–18. doi: 10.1371/journal.pone.0231553
Massante, J. C., Götzenberger, L., Takkis, K., Hallikma, T., Kaasik, A., Laanisto, L., et al. (2019). Contrasting latitudinal patterns in phylogenetic diversity between woody and herbaceous communities. Sci. Rep. 9 (1), 1–10. doi: 10.1038/s41598-019-42827-1
McGill, B. J., Enquist, B. J., Weiher, E., Westoby, M. (2006). Rebuilding community ecology from functional traits. Trends Ecol. Evol. 21 (4), 178–185. doi: 10.1016/j.tree.2006.02.002
Medina, E., Cuevas, E. (1989). Patterns of nutrient accumulation and release in Amazonian forests of the upper Rio Negro basin In Mineral nutrients in tropical forest and savanna ecosystems. Melbourne: Blackwell Scientific Publications. 217–240.
Moles, A. T., Perkins, S. E., Laffan, S. W., Flores-Moreno, H., Awasthy, M., Tindall, M. L., et al. (2014). Which is a better predictor of plant traits: Temperature or precipitation? J. Veg. Sci. 25 (5), 1167–1180. doi: 10.1111/jvs.12190
Molina-Venegas, R., Aparicio, A., Lavergne, S., Arroyo, J. (2018). Soil conditions drive changes in a key leaf functional trait through environmental filtering and facilitative interactions. Acta Oecologica 86, 1–8. doi: 10.1016/j.actao.2017.11.008
Mori, G. B., Poorter, L., Schietti, J., Piedade, M. T. F. (2021). Edaphic characteristics drive functional traits distribution in Amazonian floodplain forests. Plant Ecol. 222 (3), 349–360. doi: 1978-1-4613-1163-8_10/s11258-020-01110-4
Motavalli, P. P., Palm, C. A., Parton, W. J., Elliott, E. T., Frey, S. D. (1995). Soil pH and organic c dynamics in tropical forest soils: Evidence from laboratory and simulation studies. Soil Biol. Biochem. 27 (12), 1589–1599. doi: 10.1016/0038-0717(95)00082-P
Mouillot, D., Bellwood, D. R., Baraloto, C., Chave, J., Galzin, R., Harmelin-Vivien, M., et al. (2013). Rare species support vulnerable functions in high-diversity ecosystems. PloS Biol. 11 (5), 1–11. doi: 10.1371/journal.pbio.1001569
Nakagawa, S., Schielzeth, H. (2013). A general and simple method for obtaining R2 from generalized linear mixed-effects models. Methods Ecol. Evol. 4 (2), 133–142. doi: 10.1111/j.2041-210x.2012.00261.x
Nelson, P. N., Su, N. (2010). Soil pH buffering capacity: A descriptive function and its application to some acidic tropical soils. Aust. J. Soil Res. 48 (3), 201–207. doi: 10.1071/SR09150
Niinemets, Ü. (2010). A review of light interception in plant stands from leaf to canopy in different plant functional types and in species with varying shade tolerance. Ecol. Res. 25 (4), 693–714. doi: 1978-1-4613-1163-8_10/s11284-010-0712-4
Nobre, C. A., Berenguer, E., Brando, P. M., Poveda, G. (2019). Scientific framework to save the amazon. doi: 10.13140/RG.2.2.24053.12009
Pellens, R., Grandcolas, P. (2016). Phylogenetics and conservation biology: Drawing a path into the diversity of life. In Biodivers. Conserv. Phylogenet. Syst. 14, 1–15. doi: 1978-1-4613-1163-8_10/978-3-319-22461-9
Pérez-Harguindeguy, N., Díaz, S., Garnier, E., Lavorel, S., Poorter, H., Jaureguiberry, P., et al. (2013). New handbook for standardised measurement of plant functional traits worldwide. Aust. J. Bot. 61 (3), 167–234. doi: 10.1071/BT12225
Pitman, N. C. A., Silman, M. R., Terborgh, J. W. (2013). Oligarchies in Amazonian tree communities: A ten-year review. Ecography 36 (2), 114–123. doi: 10.1111/j.1600-0587.2012.00083.x
Pitman, N. C. A., Terborgh, J. W., Silman, M. R., Núñez, P. V., Neill, D. A., Cerón, C. E., et al. (2001). Dominance and distribution of tree species in upper Amazonian terra firme forests. Ecology 82 (8), 2101–2117. doi: 10.1890/0012-9658(2001)082[2101:DADOTS]2.0.CO;2
Poorter, L., Castilho, C. V., Schietti, J., Oliveira, R. S., Costa, F. R. C. (2018). Can traits predict individual growth performance? a test in a hyperdiverse tropical forest. New Phytol. 219 (1), 109–121. doi: 10.1111/nph.15206
Poorter, L., van der Sande, M. T., Arets, E. J. M. M., Ascarrunz, N., Enquist, B., Finegan, B., et al. (2017). Biodiversity and climate determine the functioning of Neotropical forests. Global Ecol. Biogeogr. 26 (12), 1423–1434. doi: 10.1111/geb.12668
Qian, H., Jin, Y., Ricklefs, R. E. (2017). Patterns of phylogenetic relatedness of angiosperm woody plants across biomes and life-history stages. J. Biogeogr. 44 (6), 1383–1392. doi: 10.1111/jbi.12936
Qian, H., Zhang, Y., Zhang, J., Wang, X. (2013). Latitudinal gradients in phylogenetic relatedness of angiosperm trees in north America. Global Ecol. Biogeogr. 22 (11), 1183–1191. doi: 10.1111/geb.12069
R Core Team (2021). R: A language and environment for statistical computing (R Foundation for Statistical Computing, Vienna, Austria). Available at: http://www.R-project.org/
Rodrigues, P. M. S., Silva, J. O., Schaefer, C. E. G. R. (2019). Edaphic properties as key drivers for woody species distributions in tropical savannic and forest habitats. Aust. J. Bot. 67 (1), 70–80. doi: 10.1071/BT17241
Sanford, R. L., Cuevas, E. (1996). Root growth and rhizosphere interactions in tropical forests. In Tropical forest plant ecophysiology. Boston, MA: Springer. (pp. 268–300) doi: 1978-1-4613-1163-8_10/978-1-4613-1163-8_10
Satdichanh, M., Millet, J., Heinimann, A., Nanthavong, K., Harrison, R. D. (2015). Using plant functional traits and phylogenies to understand patterns of plant community assembly in a seasonal tropical forest in. PloS One 10 (6), 1–15. doi: 10.1371/journal.pone.0130151
Smith, S. A., Brown, J. W. (2018). Constructing a broadly inclusive seed plant phylogeny. Am. J. Bot. 105 (3), 302–314. doi: 10.1002/ajb2.1019
Sollins, P. (1998). Factors influencing species composition in tropical lowland rain forest: Does soil matter? Ecology 79 (1), 23–30. doi: 10.1890/0012-9658(1998)079[0023:FISCIT]2.0.CO;2
Stevens, R. D., Willig, M. R. (2002). Geographical ecology at the community level: Perspectives on the diversity of new world bats. Ecology 83 (2), 545–560. doi: 10.1890/0012-9658(2002)083[0545:GEATCL]2.0.CO;2
Swenson, N. G. (2011). The role of evolutionary processes in producing biodiversity patterns, and the interrelationships between taxonomic, functional and phylogenetic biodiversity. Am. J. Bot. 98 (3), 472–480. doi: 10.3732/ajb.1000289
Swenson, N. G. (2012a). The functional ecology and diversity of tropical tree assemblages through space and time: From local to regional and from traits to transcriptomes. ISRN forestry. 2012, 1–16. doi: 10.5402/2012/743617
Swenson, N. G., Enquist, B. J. (2008). The relationship between stem and branch wood specific gravity and the ability of each measure to predict leaf area. Am. J. Bot. 95 (4), 516–519. doi: 10.3732/ajb.95.4.516
Swenson, N. G., Enquist, B. J. (2009). Opposing assembly mechanisms in a Neotropical dry forest: Implications for phylogenetic and functional community ecology. Ecology 90 (8), 2161–2170. doi: 10.1890/08-1025.1
Swenson, N. G., Enquist, B. J., Pither, J., Kerkhoff, A. J., Boyle, B., Weiser, M. D., et al. (2012b). The biogeography and filtering of woody plant functional diversity in north and south America. Global Ecol. Biogeogr. 21 (8), 798–808. doi: 10.1111/j.1466-8238.2011.00727.x
Swenson, N. G., Erickson, D. L., Mi, X., Bourg, N. A., Forero-Montana, J., Ge, X., et al. (2012c). Phylogenetic and functional alpha and beta diversity in temperate and tropical tree communities. Ecology 93, 112–125. doi: 10.1890/11-0402.1
Swenson, N. G., Weiser, M. D. (2014). On the packing and filling of functional space in eastern north American tree assemblages. Ecography 37 (11), 1056–1062. doi: 10.1111/ecog.00763
Ter Steege, H., Pitman, N. C. A., Sabatier, D., Baraloto, C., Salomão, R. P., Guevara, J. E., et al. (2013). Hyperdominance in the Amazonian tree flora. Science 342 (6156), 1–43. doi: 10.1126/science.1243092
Thiers, B. (2021). Index herbariorum: A global directory of public herbaria and associated staff. New York, USA: New York Botanical Garden’s Virtual Herbarium.
Valencia, R., Balslev, H., Paz Y Miño C, G. (1994). High tree alpha-diversity in Amazonian Ecuador. Biodivers. Conserv. 3 (1), 21–28. doi: 10.1007/BF00115330
Venables, W., Ripley, B. (2002). Modern applied statistics with s (Springer US, New York, NY). doi: 10.1007/978-0-387-21706-2_10
Violle, C., Navas, M.-L., Vile, D., Kazakou, E., Fortunel, C., Hummel, I., et al. (2007). Let the concept of trait be functional! Oikos 116 (5), 882–892. doi: 10.1111/j.2007.0030-1299.15559.x
Webb, C. O. (2000). Exploring the phylogenetic structure of ecological communities: An example for rain forest trees. Am. Nat. 156 (2), 145–155. doi: 10.1086/303378
Webb, C. O., Cannon, C. H., Davies, S. J. (2008). Ecological organization, biogeography, and the phylogenetic structure of tropical forest tree communities. tropical forest community ecology. In Tropical forest community ecology, Blackwell Publishing Ltd. West Sussex (UK): Blackwell Publishing Ltd. 79–97
Weerasinghe, L. K., Creek, D., Crous, K. Y., Xiang, S., Liddell, M. J., Turnbull, M. H., et al. (2014). Canopy position affects the relationships between leaf respiration and associated traits in a tropical rainforest in far north Queensland. Tree Physiol. 34 (6), 564–584. doi: 10.1093/treephys/tpu016
Weiser, M. D., Enquist, B. J., Boyle, B., Killeen, T. J., Jørgensen, P. M., Fonseca, G., et al. (2007). Latitudinal patterns of range size and species richness of new world woody plants. Global Ecol. Biogeogr. 16 (5), 679–688. doi: 10.1111/j.1466-8238.2007.00323.x
Wieczynski, D. J., Boyle, B., Buzzard, V., Duran, S. M., Henderson, A. N., Hulshof, C. M., et al. (2019). Climate shapes and shifts functional biodiversity in forests worldwide. Proc. Natl. Acad. Sci. United States America 116 (2), 587–592. doi: 10.1073/pnas.1813723116
Willig, M. R., Kaufman, D. M., Stevens, R. D. (2003). Latitudinal gradients of biodiversity: Pattern, process, scale, and synthesis. Annu. Rev. Ecol. Evol. Syst. 34, 273–309. doi: 10.1146/annurev.ecolsys.34.012103.144032
Wright, S. J. (2002). Plant diversity in tropical forests: A review of mechanisms of species coexistence. Oecologia 130 (1), 1–14. doi: 10.1007/s004420100809
Wright, I. J., Reich, P. B., Westoby, M., Ackerly, D. D., Baruch, Z., Bongers, F., et al. (2004). The worldwide leaf economics spectrum. Nature 428 (6985), 821–827. doi: 10.1038/nature02403
Keywords: latitudinal diversity gradient, taxonomic diversity (TD), functional diversity (FD), phylogenetic diversity (PD), environmental filtering, favourability hypothesis, tropical terra firme forest, woody plant
Citation: Ben Saadi C, Cayuela L, Bañares de Dios G, de Aledo JG, Matas-Granados L, Salinas N, La Torre Cuadros MdlÁ and Macía MJ (2022) Latitudinal patterns and environmental drivers of taxonomic, functional, and phylogenetic diversity of woody plants in western Amazonian terra firme forests. Front. Plant Sci. 13:978299. doi: 10.3389/fpls.2022.978299
Received: 25 June 2022; Accepted: 15 September 2022;
Published: 07 October 2022.
Edited by:
Boris Rewald, University of Natural Resources and Life Sciences Vienna, AustriaCopyright © 2022 Ben Saadi, Cayuela, Bañares de Dios, de Aledo, Matas-Granados, Salinas, La Torre Cuadros and Macía. This is an open-access article distributed under the terms of the Creative Commons Attribution License (CC BY). The use, distribution or reproduction in other forums is permitted, provided the original author(s) and the copyright owner(s) are credited and that the original publication in this journal is cited, in accordance with accepted academic practice. No use, distribution or reproduction is permitted which does not comply with these terms.
*Correspondence: Celina Ben Saadi, celina.bensaadi@gmail.com