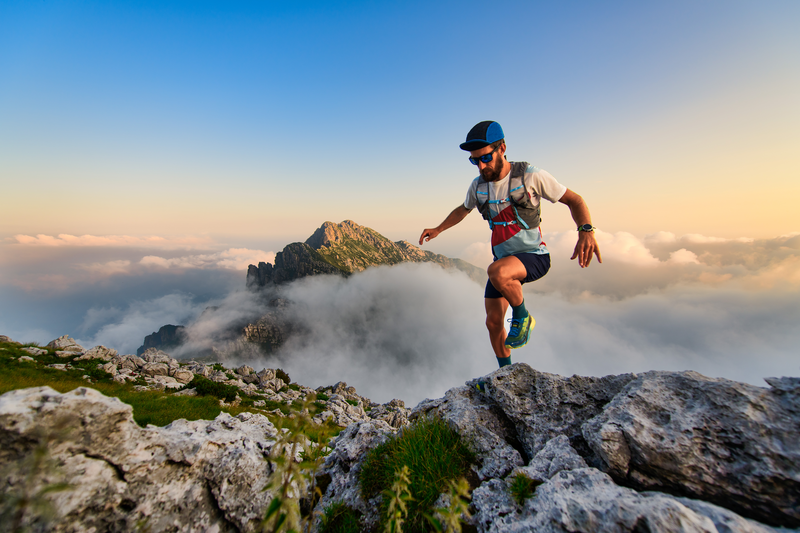
94% of researchers rate our articles as excellent or good
Learn more about the work of our research integrity team to safeguard the quality of each article we publish.
Find out more
ORIGINAL RESEARCH article
Front. Plant Sci. , 14 November 2022
Sec. Plant Systematics and Evolution
Volume 13 - 2022 | https://doi.org/10.3389/fpls.2022.977152
This article is part of the Research Topic Frontiers in the Study of Ancient Plant Remains View all 27 articles
Nut fruits likely played a significant role before and during the origin of agriculture; however, relatively little research conducted on the morphological characteristics and statistical comparisons of nut fruit starch granule hinders the progress of paleodietary analysis of prehistorical society. For better species identification of starch granule remaining on tools discovered at archaeological sites, it is desirable to develop a more abundant morphology database of modern nut fruit starch granules as well as the establishment of relevant identification standards. Therefore, nuts from 40 species in four genera (Quercus, Lithocarpus, Castanea, and Castanopsis) of Fagaceae were collected from South China for statistical measurement and comparative analysis. Starch granules are highly accumulated in 34 species except for 6 species, whose shapes involve oval, subcircular, drop-shaped, rounded triangle, polygonal, spherical caps, and bell-shaped types, or a combination of several types, and the average length is between 10 and 20 μm. According to research on Quercus phylogeny relationships, it was found that the species in the same infragenious section produce similar morphological characteristics of starch granules. The result was applied in the identification of starch granules extracted from stone tools from the 20 to 10 ka cultural layer of Xiaodong Rockshelter, and some starch granules can be recognized to species level, revealing that nuts from Quercus and Lithocarpus were gathered and exploited by ancient people. This expansion of modern starch presentation and comparison of nuts helps to improve the accuracy of the identification of ancient starch and deepen the understanding of plant utilization of ancient humans.
The utilization of plants and animals is the foundation of livelihood and development of ancient human society, learning which can assist us in comprehending the history of human civilization. Domestication and the origin of agriculture furnished a physical foundation in demographic development and dispersal (Liu and Chen, 2012). Currently, the prevailing opinion is that North China is the original region of rain-fed agriculture, with foxtail millet (Setaria italica) and broomcorn millet (Panicum miliaceum) as major crops (Lu et al., 2009; Zhang et al., 2011a; Liu et al., 2011; Yang et al., 2012a; Yang et al., 2012b; Zhao, 2014; Yang et al., 2015; Liu et al., 2015), and the Yangtze River valley is another center of agricultural origin, represented by the cultivation of rice (Bai and Su, 1994; Zhao, 1998; Liu et al., 2007; Jones and Liu, 2009; Huan et al., 2014; Wu et al., 2014; Tao, 2016; Zhao, 2019; Zhao, 2020). Combined with international archaeological and anthropological research data (Anderson, 1980; Mason, 1992; Mason, 1996; Bettinger et al., 1997; Haslam, 2004; Bellwood, 2005), Liu has speculated that acorns may additionally have been a major source of starchy food in many areas prior to the development of China’s prehistory cereal agriculture in the Late and Middle Neolithic (Liu, 2008; Liu, 2010). The main function of Chinese grinding stone tools was to process nuts such as acorns rather than grains (Zhao, 2006; Cui, 2018), and the gathering, processing, and consumption of nuts (especially acorns) contributed to the settlement of human society (Sun et al., 1981; Wang, 2006; Jiang, 2007; Yang and Jiang, 2010), while the manufacturing of cultivated rice and foxtail millet took a secondary position for a long time.
Analyzing the microfossils remaining on the giant quantity of stone tools and pottery excavated from the site permits us to study ancient plant utilization. The plant microfossils primarily include pollen, phytolith, and starch granule. Pollen is solely recognized to the genera level, with an ambiguous indication of human utilization on plant sources; phytoliths are effective in determining particular species such as Poaceae; however, their indicator value for human utilization on woody plants is unclear (Wang and Lü, 1993; Hodson et al., 2005; Ge et al., 2020). In contrast, the richness of starch granule in plant fruits and root tubers, their long-term maintenance in archaeological remains and strata, and the variation in morphological characteristics of different species and genera provide potentially clear indications of human consumption of certain plant resources (Burrell, 2003).
The starch granule morphology varies among different genera (Yang et al., 2006). Starch is a long-chain compound formed by glucose molecules and is stored in organs such as roots, stems, and seeds of plants in the form of starch granule. The formation of starch granule starts at a point called the hilum with additional layers laid down (Tester, 1997). The size of starch granule generally varies from approximately 1 micron to 100 microns (Sivak and Preiss, 1998), and an extinction cross can be viewed with polarized light (Sterling, 1984; Dziezak, 1988; Yiu, 1993). Starch granules are classified as simple, compound, or semi-compound depending on how they are formed in the amyloplast (Banks and Greenwood, 1975). Therefore, according to the morphological characteristics of starch granules, they can be classified to determine plant genera and species.
The method of starch granule analysis has been widely applied to studies on the origin and spread of crops, human utilization on plants, and the functional analysis of stone tools in the Americas, Australia, and East and West Asia (Fullagar, 1998; Piperno and Holst, 1998; Piperno et al., 2000; Pearsall et al., 2004; Piperno et al., 2004; Torrence and Barton, 2006; Perry et al., 2007; Li et al., 2010; Zhang et al., 2011b; Hart, 2014; Lü et al., 2014; Ma et al., 2014; Hardy et al., 2016; García-Granero et al., 2017; Wang et al., 2017; Wang et al., 2019). Starch granule analysis of the grooved basin from the Lingjiatan site, Hanshan County, Anhui Province (Yang et al., 2009b), and of grinding stone tools at the Shangzhai site, Beijing (Sun et al., 2019), revealed that the ancient humans had consciously exploited acorns.
Acorns refer to nuts of the Fagaceae family that can be exploited except for chestnut, which is widely cultivated. Fagaceae plants are mainly found in the tropics and subtropics of the Northern Hemisphere (Hu et al., 2000). The acorn is highly adaptable, with a broad distribution in China, where the annual production is estimated at 6–7 billion kg (Xie and Xie, 2002). Meanwhile, acorns are rich in nutrients, including starch, tannin, protein, oil, amino acids, and minerals (Ao et al., 1998). Acorns are suitable for eating after desiccation and can be used in brewing (Hou and Wang, 1996), textiles, and so on (Du and Li, 1996; He et al., 2003).
However, studies of the starch granule morphology of modern nuts lack detailed and clear identification for archaeological research (Xu and Gao, 2004). Chinese scholars have identified starch granule from several genera of Fagaceae in northern China (Yang et al., 2009a), but the starch statistics of edible species from South China, where plant resources are rich, are still desired, which significantly hinders the study of ancient plant utilization in the region. In this paper, nuts of 40 species of Fagaceae from the southern part of China were collected for the presentation and comparison of starch granule morphological characteristics and statistical analysis, providing a reliable identification key for starch granule extracted from archaeological sites and thus revealing the use of plant resources by ancient people in South China.
Forty species specimens of Quercus, Lithocarpus, Castanea, and Castanopsis were primarily collected during the fruit maturation period in September or October in 2020–2021, ranging from Yunnan, Hainan, Guangdong, Guangxi, Jiangxi Province, and Tibet Autonomous Regions, China (Figure 1), involving evergreen broad-leaved forest, tropical rainforest, hillside woodland, and woodland. The detailed collection information is summarized in Supplementary Table S1.
Figure 1 Sampling spots of specimens used in this study (1: Quercus kiukiangensis; 2: Quercus lamellosa; 3: Lithocarpus dealbatus; 4: Quercus schottkyana, Quercus variabilis, Quercus serrata, Quercus aliena, Lithocarpus elizabethiae, Castanea seguinii, Castanopsis orthacantha; 5: Lithocarpus craibianus; 6: Quercus longispica; 7: Quercus rex; 8: Lithocarpus mairei; 9: Quercus franchetii; 10: Quercus cocciferoides; 11: Quercus argyrotricha; 12: Lithocarpus gymnocarpus; 13: Lithocarpus pachylepis; 14: Quercus augustinii, Lithocarpus xylocarpus; 15: Quercus marlipoensis, Lithocarpus balansae, Lithocarpus c.f. annamitorus, Lithocarpus bacgiangensis, Lithocarpus longinux, Lithocarpus sp.1; 16: Quercus patelliformis, Lithocarpus longipedicellatus; 17: Quercus fleuryi, Lithocarpus fenzelianus; 18: Lithocarpus sp.2; 19: Lithocarpus longanoides; 20: Quercus blakei, Quercus phanera, Quercus kouangsiensis; 21: Quercus litseoides, Quercus sessilifolia; 22: Lithocarpus skanianus; 23: Quercus gilva).
Starch granules of acorns were released by the following method: (1) the nut, in a small plastic sealed bag, was broken with a hammer; (2) small pieces of one broken nut were transported to new centrifugal tubes with pure water for 24 h of soaking; (3) after soaking, the samples were crushed with clean glass stirring rods to fully release the starches. Thirty microliters of the suspension was pipetted onto a clean slide and mounted in a 20%/80% glycerin/water solution. The prepared slides were observed under polarized and bright fields using a Leica DM4P microscope, and images were taken using the same system.
For statistical purposes, at least 100 granules of each specimen were recorded. Between 10 and 14 photographs were necessary to produce an adequate sample size when all granules on each photo were measured and characterized. We measured the longest orientable measurement through the hilum of each granule and recorded the following morphological features: granule shape; hilum position (eccentric/centric); the form of the fissure; presence or absence of lamellae; the form of the polarizing cross (cross-shaped/X-shaped) and surface texture (smooth/rough).
The sample was dried at low temperature, tapped with a hammer to make a natural fracture, and attached to the sample desk with the section side down. Specimens were then gold coated, observed, and photographed by scanning electron microscopy (SEM) (Thermo Scientific Quattro S).
Photoshop 2021 software was used to choose individual granules, and their areas were determined. Then, the preprepared images were used for analysis in Image-Pro Plus as follows: granules were colored, and the image was made binary (starch white against black background). Then, each granule was counted, and the size was measured. Statistical analyses and plotting were performed using Origin Pro 2021.
Starch granules of stone tools were extracted by the following method: (1) brushing the stone tool to decontaminate; (2) using an ultrasonic machine with distilled water to remove the residues on the tool surface, the liquid was collected in a centrifuge tube; (3) adding 10 ml of deflocculant and shaking residue samples for 2 h to fully release the starches; and (4) residue samples were processed for starch extraction using the heavy liquid sodium polytungstate in a specific gravity of 1.8. Slides preparation and observation were the same with modern samples.
The morphological characteristics were observed and recorded, and the size of the ancient starch granule was measured. One-to-one comparisons with modern samples from the database were performed to identify the ancient starch grains. Reliable identification of archaeological starch grains is based on size, overall shape, position and form of the hilum, fissure, the presence or absence of lamellae, and the appearance and projection of the Maltese cross under a polarized microscope (Torrence et al., 2004; Wilson et al., 2010; Reichert, 1913).
All 40 samples were observed, and each produced slides with over 100 granules. A detailed description of the morphological characteristics of the starch granule for each species is given in Table 1. We note that for six species, no identifiable starch granules were observed. However, according to the research, Quercus rex, Quercus patelliformis, and Lithocarpus gymnocarpus are rich in starch, particularly L. gymnocarpus, which has been found to have 66.12% starch in the kernel. It is not yet clear why there is no starch in these samples in our study. Further work is required to clarify this issue. Scanning electron micrographs of starch granule in nuts of 34 species are displayed in Figure 2.
Table 1 Summary of starch granule dimensions and morphological features in 34 species from Fagaceae.
Figure 2 Scanning electron micrographs of starch granule in nuts of 34 species from Fagaceae. 1, Quercus blakei; 2, Quercus argyrotricha; 3, Quercus augustinii; 4, Quercus litseoides; 5, Quercus kouangsiensis; 6, Quercus fleuryi; 7, Quercus lamellosa; 8, Quercus serrata; 9, Quercus sessilifolia; 10, Quercus gilva; 11, Lithocarpus elizabethiae; 12, Quercus kiukiangensis; 13, Quercus schottkyana; 14, Lithocarpus pachylepis; 15, Lithocarpus longipedicellatus; 16, Lithocarpus sp.; 17, Lithocarpus xylocarpus; 18, Lithocarpus fenzelianus; 19, Quercus longispica; 20, Quercus marlipoensis; 21, Castanea seguinii; 22, Quercus cocciferoides; 23, Quercus franchetii; 24, Quercus phanera; 25, Quercus aliena; 26, Quercus variabilis; 27, Lithocarpus craibianus; 28, Lithocarpus longanoides; 29, Lithocarpus mairei; 30, Lithocarpus balansae; 31, Castanopsis orthacantha; 32, Lithocarpus skanianus; 33, Lithocarpus dealbatus; 34, Lithocarpus longinux.
The detailed description of starch morphological characteristics from 19 species of Quercus are as follows.
The starch granules from Quercus blakei in our study are divided into two groups according to shape. One is the oval type, which accounts for 61%, with a smooth surface and invisible lamellae, whose mean size is 19.90 ± 3.18 μm. The other is the bell-shaped type, which accounts for 39%, with a smooth surface and invisible lamellae, whose mean size is 12.88 ± 2.25 μm.
The starch granules from Quercus kouangsiensis in our study are divided into two groups according to shape. One is the oval type, which accounts for 78.3%, with some small hollows on the surface and invisible lamellae, whose mean size is 11.48 ± 2.19 μm. The other is the subcircular type, which accounts for 9%, with a smooth surface and invisible lamellae, whose mean size is 10.51 ± 2.19 μm. Meanwhile, compound granule accounts for 12.7%.
The starch granules from Quercus argyrotricha in our study are divided into two groups according to shape. One is the oval type, which accounts for 53.7%, with some small hollows on the surface and invisible lamellae, whose mean size is 15.99 ± 3.04 μm. The other is the bell-shaped type, which accounts for 43%, with a smooth surface and invisible lamellae, whose mean size is 13.62 ± 2.58 μm. Meanwhile, compound granule accounts for 3.3%.
The starch granules from Quercus gilva in our study are divided into two groups according to shape. One is the subcircular type, which accounts for 31%, with a smooth surface, linear-shaped fissures through the hila, and invisible lamellae, whose mean size is 12.59 ± 1.79 μm. The other is the bell-shaped type, which accounts for 32%, with a smooth surface and invisible lamellae, whose mean size is 12.76 ± 1.80 μm. Meanwhile, compound granule accounts for 37%.
The starch granules from Quercus kiukiangensis in our study are divided into three groups according to shape. The first group is the subcircular type, which accounts for 34.9%, with a smooth surface, linear-shaped fissures through the hila, and invisible lamellae, whose mean size is 13.23 ± 2.71 μm. The second group is the bell-shaped type, which accounts for 37.6%, with a smooth surface and invisible lamellae, whose mean size is 14.92 ± 3.12 μm. The last group is the oval type, which accounts for 27.5%, with a smooth surface, concave toward the distal end, and invisible lamellae, whose mean size is 22.06 ± 2.98 μm.
The starch granules from Quercus phanera in our study is the drop-shaped type with a smooth surface and invisible lamellae, whose mean size is 17.35 ± 5.96 μm.
The starch granules from Quercus schottkyana in our study are divided into three groups according to shape. The first group is the subcircular type, which accounts for 32.9%, with a smooth surface and invisible lamellae, whose mean size is 10.27 ± 2.14 μm. The second group is the bell-shaped type, which accounts for 29.6%, with a smooth surface and invisible lamellae, whose mean size is 10.80 ± 2.56 μm. The last group is the oval type, which accounts for 37.5%, with some small hollows on the surface, concave toward the distal end, and invisible lamellae, whose mean size is 16.94 ± 2.21 μm.
The starch granules from Quercus sessilifolia in our study is the oval type with some small hollows on the surface and invisible lamellae, whose mean size is 17.06 ± 4.46 μm.
The starch granules from Quercus augustinii in our study are divided into two groups according to shape. One is the oval type, which accounts for 84.1%, with some small hollows on the surface and invisible lamellae, whose mean size is 14.37 ± 3.66 μm. The other is the bell-shaped type, which accounts for 15.9%, with smooth surface and invisible lamellae, whose mean size is 12.16 ± 1.83 μm.
The starch granules from Quercus litseoides in our study are divided into two groups according to shape. One is the oval type, which accounts for 80.3%, with some small hollows on the surface and invisible lamellae, whose mean size is 11.85 ± 2.43 μm. The other is the bell-shaped type, which accounts for 15.9%, with a smooth surface and invisible lamellae, whose mean size is 11.69 ± 1.69 μm. Meanwhile, compound granule accounts for 3.8%.
The starch granules from Quercus lamellosa in our study are divided into three groups according to shape. The first group is the subcircular type, which accounts for 47%, with a smooth surface and invisible lamellae, whose mean size is 10.07 ± 3.24 μm. The second group is the polygonal type, which accounts for 5%, with a rough surface and invisible lamellae, whose mean size is 14.83 ± 3.81 μm. The last group is the oval type, which accounts for 48%, with a smooth surface and invisible lamellae, and with very few granules bearing X-shaped fissures through the hila, whose mean size is 12.01 ± 3.78 μm. Meanwhile, compound granule accounts for 2%.
The starch granules from Quercus fleuryi in our study are divided into two groups according to shape. One is the oval type, which accounts for 59%, with a smooth surface and invisible lamellae, whose mean size is 8.79 ± 1.80 μm. The other is the subcircular type, which accounts for 41%, with a smooth surface and invisible lamellae, whose mean size is 7.36 ± 1.27 μm.
The starch granules from Quercus cocciferoides in our study are divided into two groups according to shape. One is the drop-shaped type, which accounts for 78%, with a smooth surface, linear-shaped fissures through the hila, and invisible lamellae, whose mean size is 20.83 ± 3.25 μm. The other is the subcircular type, which accounts for 22%, with a smooth surface and invisible lamellae, whose mean size is 10.89 ± 3.64 μm.
The starch granules from Quercus serrata in our study are divided into two groups according to shape. One is the oval type, which accounts for 57.6%, with a smooth surface, protruding toward the distal end, and invisible lamellae, whose mean size is 12.37 ± 2.31 μm. The other is the rounded triangle type, which accounts for 42.4%, with a smooth surface, linear-shaped fissures through the hila, and invisible lamellae, whose mean size is 9.85 ± 2.39 μm.
The starch granules from Quercus aliena in our study are divided into two groups according to shape. One is the oval type, which accounts for 15%, with some small hollows on the surface and invisible lamellae, whose mean size is 10.99 ± 2.33 μm. The other is the rounded triangle type, which accounts for 85%, with a smooth surface, linear-shaped fissures through the hila, and invisible lamellae, whose mean size is 10.8 ± 2.86 μm.
The starch granules from Quercus variabilis in our study are divided into two groups according to shape. One is the rounded triangle type, which accounts for 64.8%, with a smooth surface, linear-shaped or Y-shaped fissures through the hila, and invisible lamellae, whose mean size is 12.59 ± 4.12 μm. The other is the drop-shaped type, which accounts for 35.2%, with a smooth surface, linear-shaped fissures through the hila, and invisible lamellae, whose mean size is 12.95 ± 4.18 μm.
The starch granules from Quercus franchetii in our study is the drop-shaped type with a smooth surface and invisible lamellae, whose mean size is 13.44 ± 4.00 μm.
The starch granules from Quercus longispica in our study are divided into five groups according to shape. The first group is the subcircular type, which accounts for 13%, with a smooth surface and invisible lamellae, whose mean size is 10.95 ± 2.93 μm. The second group is the polygonal type, which accounts for 15%, with a rough surface and invisible lamellae, whose mean size is 21.74 ± 4.74 μm. The third group is the oval type, which accounts for 23%, with a smooth surface and invisible lamellae, and with very few granules bearing X-shaped or linear-shaped fissures through the hila, whose mean size is 14.38 ± 3.16 μm. The fourth group is the drop-shaped type, which accounts for 35%, with a smooth surface and invisible lamellae, and with very few granules bearing V-shaped fissures through the hila, whose mean size is 18.13 ± 5.68 μm. The last group is the bell-shaped type, which accounts for 13%, with a smooth surface and invisible lamellae, whose mean size is 11.12 ± 3.57 μm. Meanwhile, compound granule accounts for 2%.
The starch granules from Quercus marlipoensis in our study are divided into four groups according to shape. The first group is the subcircular type, which accounts for 17%, with a smooth surface and invisible lamellae, whose mean size is 7.55 ± 1.52 μm. The second group is the polygonal type, which accounts for 6%, with a rough surface and invisible lamellae, whose mean size is 11.89 ± 4.19 μm. The third group is the oval type, which accounts for 32%, with a smooth surface and invisible lamellae, whose mean size is 11.51 ± 4.12 μm. The last group is the drop-shaped type, which accounts for 45%, with a smooth surface and invisible lamellae, whose mean size is 15.32 ± 3.93 μm.
The detailed description of starch morphological characteristics from 13 species of Lithocarpus are as follows.
The starch granules from Lithocarpus dealbatus in our study are divided into four groups according to shape. The first group is the circular type, which accounts for 24%, with a smooth surface, concave hila, and invisible lamellae, whose mean size is 15.52 ± 3.39 μm. The second group is the polygonal type, which accounts for 21.8%, with a rough surface and invisible lamellae, whose mean size is 20.19 ± 3.55 μm. The third group is the oval type, which accounts for 20.7%, with a smooth surface, concave hila, and invisible lamellae, whose mean size is 19.40 ± 3.79 μm. The last group is the spherical caps type, which accounts for 22.9%, with a smooth surface and invisible lamellae, whose mean size is 14.37 ± 4.73 μm. Meanwhile, compound granule accounts for 10.6%.
The starch granules from Lithocarpus craibianus in our study are divided into two groups according to shape. One is the polygonal type, which accounts for 59.3%, with a rough surface and invisible lamellae, whose mean size is 18.99 ± 3.27 μm. The other is the subcircular type, which accounts for 40.7%, with a smooth surface and invisible lamellae, whose mean size is 15.25 ± 3.19 μm.
The starch granules from Lithocarpus mairei in our study are divided into three groups according to shape. The first group is the subcircular type, which accounts for 7%, with a smooth surface, concave hila, and invisible lamellae, whose mean size is 18.36 ± 4.17 μm. The second group is the polygonal type, which accounts for 82%, with a rough surface, X-shaped or linear-shaped fissures through the hila, and invisible lamellae, whose mean size is 18.53 ± 4.33 μm. The last group is the bell-shaped type, which accounts for 11%, with a smooth surface, concave hila, and invisible lamellae, whose mean size is 16.64 ± 2.98 μm. Meanwhile, compound granule accounts for 2%.
The starch granules from Lithocarpus pachylepis in our study are divided into three groups according to shape. The first group is the subcircular type, which accounts for 68%, with a smooth surface, X-shaped or linear-shaped fissures through the hila, and invisible lamellae, whose mean size is 9.97 ± 2.11 μm. The second group is the polygonal type, which accounts for 19%, with a rough surface, X-shaped fissures through the hila, and invisible lamellae, whose mean size is 11.39 ± 2.57 μm. The last group is the bell-shaped type, which accounts for 4%, with a smooth surface, concave hila, and invisible lamellae, whose mean size is 8.49 ± 1.62 μm. Meanwhile, compound granule accounts for 9%.
The starch granules from Lithocarpus sp. in our study are divided into three groups according to shape. The first group is the subcircular type, which accounts for 70%, with a smooth surface and invisible lamellae, and with very few granules bearing X-shaped fissures through the hila, whose mean size is 11.88 ± 3.37 μm. The second group is the polygonal type, which accounts for 17%, with a rough surface, concave hila, and invisible lamellae, whose mean size is 11.14 ± 5.49 μm. The last group is the bell-shaped type, which accounts for 12%, with a smooth surface, concave hila, and invisible lamellae, whose mean size is 10.09 ± 3.37 μm. Meanwhile, compound granule accounts for 1%.
The starch granules from Lithocarpus elizabethiae in our study are divided into two groups according to shape. One is the subcircular type, which accounts for 93%, with a smooth surface, X-shaped or linear-shaped fissures through the hila, and invisible lamellae, whose mean size is 10.21 ± 2.73 μm. The other is the bell-shaped type, which accounts for 6%, with a smooth surface, X-shaped fissures through the hila, and invisible lamellae, whose mean size is 7.22 ± 10.06 μm. Meanwhile, compound granule accounts for 1%.
The starch granules from Lithocarpus longipedicellatus in our study are divided into three groups according to shape. The first group is the subcircular type, which accounts for 39%, with a smooth surface and invisible lamellae, whose mean size is 13.19 ± 4.46 μm. The second group is the polygonal type, which accounts for 31%, with a rough surface, concave hila, and invisible lamellae, whose mean size is 17.48 ± 5.71 μm. The last group is the bell-shaped type, which accounts for 30%, with a smooth surface and invisible lamellae, whose mean size is 12.83 ± 5.32 μm. Meanwhile, compound granule accounts for 2%.
The starch granules from Lithocarpus fenzelianus in our study is the subcircular type with a smooth surface and invisible lamellae, whose mean size is 8.35 ± 1.94 μm.
The starch granules from Lithocarpus balansae in our study are divided into three groups according to shape. The first group is the subcircular type, which accounts for 12.2%, with a smooth surface and invisible lamellae, whose mean size is 15.99 ± 3.04 μm. The second group is the polygonal type, which accounts for 41.2%, with a rough surface and visible lamellae, whose mean size is 20.79 ± 3.98 μm. The last group is the oval type, which accounts for 39.7%, with some small hollows on the surface and visible lamellae, whose mean size is 18.16 ± 3.71 μm. Meanwhile, compound granule accounts for 6.9%.
The starch granules from Lithocarpus skanianus in our study are divided into three groups according to shape. The first group is the subcircular type, which accounts for 7.3%, with some small hollows on the surface and visible lamellae, whose mean size is 14.9 ± 2.81 μm. The second group is the polygonal type, which accounts for 77.6%, with a rough surface and visible lamellae, whose mean size is 19.93 ± 5.2 μm. The last group is the bell-shaped type, which accounts for 12.7%, with a smooth surface and invisible lamellae, whose mean size is 18.66 ± 3.01 μm. Meanwhile, compound granule accounts for 2.4%.
The starch granules from Lithocarpus longanoides in our study are divided into two groups according to shape. One is the subcircular type, which accounts for 13.5%, with some small hollows on the surface, linear-shaped fissures through the hila, and visible lamellae, whose mean size is 12.63 ± 1.99 μm. The other is the polygonal type, which accounts for 82.5%, with a rough surface, linear-shaped fissures through the hila, and visible lamellae, whose mean size is 15.87 ± 3.34 μm. Meanwhile, compound granule accounts for 4%.
The starch granules from Lithocarpus xylocarpus in our study are divided into two groups according to shape. One is the subcircular type, which accounts for 77.6%, with a smooth surface and invisible lamellae, whose mean size is 12.51 ± 2.95 μm. The other is the oval type, which accounts for 22.4%, with a rough surface and invisible lamellae, whose mean size is 15.76 ± 3.99 μm.
The starch granules from Lithocarpus longinux in our study are divided into two groups according to shape. One is the subcircular type, which accounts for 10.2%, with a smooth surface and invisible lamellae, whose mean size is 7.73 ± 0.88 μm. The other is the bell-shaped type, which accounts for 67%, with a smooth surface and invisible lamellae, whose mean size is 7.69 ± 0.96 μm. Meanwhile, compound granule accounts for 22.8%. The compound granules could be divided into two subtypes: one usually includes two or three small semicircular or fan-shaped single granules, while the other is composed of over four small polygonal single granules.
The starch granules from Castanopsis orthacantha in our study are divided into four groups according to shape. The first group is the subcircular type, which accounts for 17%, with a smooth surface and invisible lamellae, whose mean size is 15.98 ± 3.45 μm. The second group is the polygonal type, which accounts for 65%, with a rough surface and invisible lamellae, whose mean size is 16.67 ± 3.15 μm. The third group is the oval type, which accounts for 8%, with a smooth surface and invisible lamellae, whose mean size is 16.60 ± 2.99 μm. The last group is the bell-shaped type, which accounts for 10%, with a rough surface and invisible lamellae, whose mean size is 12.92 ± 2.16 μm. Meanwhile, compound granule accounts for 2%.
The starch granules from Castanea seguinii in our study are divided into four groups according to shape. The first group is the subcircular type, which accounts for 29%, with a smooth surface and invisible lamellae, whose mean size is 10.34 ± 3.38 μm. The second group is the polygonal type, which accounts for 6%, with a rough surface and invisible lamellae, whose mean size is 14.62 ± 3.25 μm. The third group is the oval type, which accounts for 17%, with a smooth surface and invisible lamellae, whose mean size is 14.65 ± 3.22 μm. The last group is the drop-shaped type, which accounts for 45%, with a smooth surface and invisible lamellae, and with very few granules with concave hila, whose mean size is 12.92 ± 2.16 μm. Meanwhile, compound granule accounts for 3%.
We statistically measured the granule size as a discriminating feature for starch identification. However, the fact is a lot of granule size overlap makes it challenging to adopt granule size as an independent discriminator. Therefore, size should be used in combination with the following morphological features for reliable starch discrimination: overall shape, fissure types, lamella visibility, hilum position, and surface texture. We divided all specimens into seven groups primarily based on the granule shape, namely, oval, subcircular, drop-shaped, rounded triangle, polygonal, spherical caps, and bell-shaped. Starch granule sizes are given in Figure 3.
Group A is mainly composed of ovals with an eccentric hilum and invisible lamellae. Quercus fleuryi has the smallest starch length in this group at mean length (8.21 ± 1.75 μm), while Quercus blakei and Quercus sessilifolia are the largest, 17.11 ± 4.43 μm and 17.06 ± 4.46 μm, respectively. Quercus argyrotricha and Quercus augustinii have similar granule size distributions, as do Quercus litseoides, Quercus kouangsiensis, and Quercus serrata. Group A could be divided into four subtypes based on the type combination. The A1 subtype group contains oval and bell-shaped types; starch granules of Quercus blakei (Figure 4-1) have a smooth surface, while those of Quercus argyrotricha (Figure 4-2), Quercus augustinii (Figure 4-3), and Quercus litseoides (Figure 4-4) have a rough surface. The A2 subtype group is a combination of oval and subcircular types; Quercus kouangsiensis (Figure 4-5) contains 12.7% compound granules with some small hollows on the surface. Quercus fleuryi (Figure 4-6) only contains single granules with a smooth surface. Quercus lamellosa (Figure 4-7) contains several polygonal types with X-shaped fissures. The A3 subtype group contains Quercus serrata (Figure 4-8), in which a small part of the rounded triangle type with linear-shaped fissures is included. The A4 subtype group contains Quercus sessilifolia (Figure 4-9), which only has an oval type with some small hollow surfaces.
Figure 4 Morphological graphs of 34 species from Fagaceae. Scale bar, 20 μm. (a, brightfield light; b, cross-polarized light). 1, Quercus blakei; 2, Quercus argyrotricha; 3, Quercus augustinii; 4, Quercus litseoides; 5, Quercus kouangsiensis; 6, Quercus fleuryi; 7, Quercus lamellosa; 8, Quercus serrata; 9, Quercus sessilifolia; 10, Lithocarpus fenzelianus; 11, Quercus gilva; 12, Lithocarpus elizabethiae; 13, Lithocarpus pachylepis; 14, Lithocarpus sp.; 15, Lithocarpus longipedicellatus; 16, Lithocarpus xylocarpus; 17, Quercus kiukiangensis; 18, Quercus schottkyana; 19, Quercus longispica; 20, Castanea seguinii; 21, Quercus marlipoensis; 22, Quercus cocciferoides; 23, Quercus franchetii; 24, Quercus phanera; 25, Quercus aliena; 26, Quercus variabilis; 27, Lithocarpus craibianus; 28, Lithocarpus longanoides; 29, Lithocarpus mairei; 30, Castanopsis orthacantha; 31, Lithocarpus balansae; 32, Lithocarpus skanianus; 33, Lithocarpus dealbatus; 34, Lithocarpus longinux.
The subcircular type dominates in Group B, which has a visible centric hilum and invisible lamella. Group B could be divided into five subtypes based on the type combination. The B1 subtype group contains Lithocarpus fenzelianus (Figure 4-10), which has the smallest size in Group 2 (8.35 ± 1.94 μm), subcircular type only. The B2 subtype group is a combination of subcircular and bell-shaped types. Quercus gilva (Figure 4-11) contains 37% compound granules, and some of the single granules have linear fissures, while Lithocarpus elizabethiae (Figure 4-12) contains almost single granules, some of which have linear fissures or X-shaped fissures. The B3 subtype group is a combination of subcircular, polygonal with wavy edges or convex surfaces, and bell-shaped types. Lithocarpus pachylepis (Figure 4-13) contains 9% compound granules, and some of the single granules have linear-shaped or X-shaped fissures, while Lithocarpus sp. (Figure 4-14) has a concave hilum. The statistical results revealed that three types in Lithocarpus longipedicellatus (Figure 4-15) have an almost equal percentage. The B4 subtype group contains Lithocarpus xylocarpus (Figure 4-16) with an oval type that has a rough surface. The B5 subtype group contains Quercus kiukiangensis (Figure 4-17) and Quercus schottkyana (Figure 4-18), which include oval (concave toward the distal end) and bell-shaped types. The size of Quercus kiukiangensis (16.3 ± 4.67 μm) is larger than that of Quercus schottkyana (12.93 ± 3.87 μm).
Group C is mainly composed of drop-shaped types with eccentric hila and invisible lamellae. Group C could be divided into three subtypes based on the type combination. The C1 subtype group contains oval, subcircular, and polygonal with wavy edges or convex surfaces. Quercus longispica (Figure 4-19) and Castanea seguinii (Figure 4-20) have some compound granules, while they are not observed in Quercus marlipoensis (Figure 4-21). In addition, the bell-shaped type and oval type, few of which have linear-shaped, V-shaped, and X-shaped fissures, are included in Quercus longispica. The C2 subtype group contains Quercus cocciferoides (Figure 4-22), which includes a considerable number of subcircular types. Some of the granules have linear fissures. The C3 subtype group includes Quercus franchetii (Figure 4-23) and Quercus phanera (Figure 4-24), drop-shaped type only. However, Quercus phanera (17.35 ± 5.96 μm) is larger than Quercus franchetii (13.44 ± 4 μm).
Group D includes Quercus aliena (Figure 4-25) and Quercus variabilis (Figure 4-26), in which the rounded triangle type is dominant, with faint polarizing crosses, linear fissures, and invisible lamellae. Quercus aliena also contains a few oval types, while Quercus variabilis contains several drop-shaped types.
Group E, which is polygonal with wavy edges or convex surfaces, has a mean size larger than 15 μm. Group E could be divided into two subtypes based on the type combination. The E1 subtype group is a combination of polygonal and subcircular types. Lithocarpus craibianus (Figure 4-27) only contains single granules, while Lithocarpus longanoides (Figure 4-28) contains few compound granules, and the single one has linear-shaped fissures. The E2 subtype group included more various, polygonal, oval, subcircular, and bell-shaped types. Lithocarpus mairei (Figure 4-29) and Castanopsis orthacantha (Figure 4-30) have invisible lamellae, while the former has a concave hilum and linear-shaped or X-shaped fissures. The starch granules of Lithocarpus balansae (Figure 4-31) and Lithocarpus skanianus (Figure 4-32) are most likely to have visible lamellae and small hollows on the surface, while the largest size of Lithocarpus skanianus could reach 41.47 μm and that of Lithocarpus balansae can only reach 30.46 μm.
Group F only contains Lithocarpus dealbatus (Figure 4-33), which is a combination of spherical caps and polygonal, oval, and subcircular types, and additionally includes 10.6% compound granules. Some starch granules have a concave hilum.
Group G only contains Lithocarpus longinux (Figure 4-34), which is composed of bell-shaped and subcircular types and contains 22.8% compound granules. The compound granules could be divided into two subtypes as follows: G1 and G2. The former usually includes two or three small semicircular or fan-shaped single granules, while the latter is composed of over four small polygonal single granules. Lithocarpus longinux was the smallest among all the specimens (7.7 ± 0.95 μm).
When compound granules are milled, they can break up into the separate subgranules. Meanwhile, we noted that the species that produced the bell-shaped type almost produced compound granules, and the compound granules are mainly composed of two single bell-shaped granules, which means that the bell-shaped type may originate from the segregative compound granules. There are many folds on the surface of almost all polygonal starch granules. Transverse fissures are present in most Quercus kiukiangensis, Quercus gilva, Quercus variabilis, Quercus serrata, Quercus aliena, Quercus cocciferoides, Quercus longispica, Lithocarpus mairei, Lithocarpus pachylepis, Lithocarpus elizabethiae, and Lithocarpus longanoides starch granules. Most starch granules of Lithocarpus longanoides, Lithocarpus skanianus, and Lithocarpus balansae featured lamellae.
Combining the previous work of phylogenetic relationships (Deng et al., 2018; Hipp et al., 2020) with the species tested in this paper, the phylogenetic relationships are given in Table 2, and starch granule sizes are given in Figure 5.
Figure 5 Box plot of the granule sizes of plant materials used for morphological analysis based on phylogeny in this study.
Starch granule morphology is largely dependent on the genetic composition of the plant, but size and shape can be modified by both the internal and external environments of the plant (Nikuni, 1978; Oliveira et al., 1994; Haase and Plate, 1996). Regardless of environmental factors, the shape and size of the starch granule are often characteristic of the plant taxon. We noticed that all samples in the STB lineage in section Cyclobalnaopsis produced oval-type starch granules, which was not observed in the others. Both sections Ilex and Cerris contain drop-shaped types, which is reasonable because these two sections are close in their phylogenetic relationships. It is a combination of rounded triangle and oval types in two samples of section Quercus.
The modern starch presentation and comparison of nuts was applied in the identification of starch granules extracted from stone tools from the 20 to 10 ka cultural layer of Xiaodong Rockshelter, which is located in Southwestern Yunnan and the earliest Hoabinhian site discovered so far (Ji et al., 2016). A starch granule extracted from a chopper, which is polygonal with a rough surface and linear-shaped fissures with a size of 18.18 μm, is consistent with Lithocarpus mairei in both size and morphology (Figure 6A). Another starch granule extracted from a sumatralith, which is rounded triangle with a smooth surface and an X-shaped polarizing cross with a size of 10.7 μm, is consistent with Quercus serrata in both size and morphology (Figure 6B). Moreover, one starch granule extracted from another sumatralith is consistent with Quercus variabilis and Quercus aliena in both size and morphology, which is rounded triangle with a smooth surface and linear-shaped fissures with a size of 12.99 μm (Figure 6C). Based on the starch granule analysis, it can be inferred that nuts from Quercus and Lithocarpus were gathered and exploited by ancient people in this region. More starch granules extracted from stone tools in this site show the morphological characteristics of Fagaceae species.
Figure 6 Morphological graphs of starch granules found in the Xiaodong site. Scale bar, 20 μm (a, brightfield light; b, cross-polarized light). (A), ancient starch consistent with Lithocarpus mairei; (B), ancient starch consistent with Quercus serrata; (C), ancient starch consistent with Quercus variabilis and Quercus aliena.
Although our statistical analysis of granule size overlaps among some species, it still evaluates distribution differences, and combining it with morphological features can help species discrimination. Morphological analysis on a phylogenetic basis shows the discrimination among different groups of phylogeny of Quercus section. Different groups tend to produce the same shape starch granules. An application case in archaeology shows that some starch granules from Paleolithic stone tools can be recognized to species level, suggesting that the identification of starches from Fagaceae in our study sample from South China is reliable. Therefore, we confirm the potential for starch granule analysis in archaeology research, which helps to improve the accuracy of the identification of ancient starch and deepen the understanding of plant utilization of ancient humans.
The original contributions presented in the study are included in the article/Supplementary Material. Further inquiries can be directed to the corresponding author.
Conceived and designed the experiments: QY, HZ, and XJ. Performed the experiments: TY, NC and WY. Analyzed the data: TY, NC and MD. Wrote the paper: TY, QY and NC. Collected the samples in fieldwork and species identification: MD and KY. All authors contributed to the article and approved the submitted version.
This work was jointly supported by the National Natural Science Foundation of China (41991323, U1902208, and 41672344), the Strategic Priority Research Program of Chinese Academy of Sciences (XDB26020301), the Second Tibetan Plateau Scientific Expedition and Research (STEP) (2019QZKK0704), and Yunnan Leading Talent Project (202005AB160008).
The authors declare that the research was conducted in the absence of any commercial or financial relationships that could be construed as a potential conflict of interest.
All claims expressed in this article are solely those of the authors and do not necessarily represent those of their affiliated organizations, or those of the publisher, the editors and the reviewers. Any product that may be evaluated in this article, or claim that may be made by its manufacturer, is not guaranteed or endorsed by the publisher.
The Supplementary Material for this article can be found online at: https://www.frontiersin.org/articles/10.3389/fpls.2022.977152/full#supplementary-material
Supplementary Table 1 | Plant materials used in this study and their collection information.
Supplementary Table 2 | Dichotomous key for starch granule of Fagaceae.
Anderson, P. C. (1980). A testimony of prehistoric tasks: diagnostic residues on stone tool working edges. World Archaeol. 12, 181–194. doi: 10.1080/00438243.1980.9979791
Ao, T., Yang, Q., Mi, L., Zhao, Z. H., Shi, H. P. (1998). Studies on nutrient contents in arcorn of quercus Mongolia fisch. J. Inner Mongolia Institute Agric. Anim. Husbandry 19, 72–76.
Banks, W., Greenwood, C. T. (1975). Starch and Its Components. Edinburgh: Edinburgh University Press.
Bellwood, P. (2005). First farmers: The origins of agricultural societies (Oxford: Blackwell Publishing).
Bettinger, R., Malhi, R., McCarthy, H. (1997). Central place models of acorn and mussell processing. J. Archaeol Sci. 24, 887–899. doi: 10.1006/jasc.1996.0168
Burrell, M. M. (2003). Starch: The need for improved quality or quantity - an overview. J. Exp. Bot. 54, 451–456. doi: 10.1093/jxb/erg049
Cui, Q. L. (2018). The study of lithic assemblage at Jiahu site, Henan province. (Anhui: University of science and technology of China).
Deng, M., Hipp, A. L., Song, Y. G., Li, Q. S., Coombes, A., Cotton, A. (2014). Leaf epidermal features of Quercus subgenus Cyclobalanopsis (Fagaceae) and their systematic significance. Botanical Journal of the Linnean Society. 176, 224–259. doi: 10.1111/boj.12207
Deng, M., Jiang, X. L., Hipp, A. L., Paul, S. M., Marlene, H. (2018). Phylogeny and biogeography of East Asian evergreen oaks (Quercus section Cyclobalanopsis; fagaceae): Insights into the Cenozoic history of evergreen broad-leaved forests in subtropical Asia. Mol. Phylogenet. Evol. 119, 170–181. doi: 10.1016/j.ympev.2017.11.003
Du, L. Q., Li, X. Y. (1996). An exploration of acorns. China For. Sci. Technol. 1, 28–29. doi: 10.13360/j.issn.1000-8101.1996.01.015
Fullagar, R. (1998). A closer look: recent Australian studies of stone tools. Sydney: Archaeological Computing Laboratory, University of Sydney.
García-Granero, J. J., Lancelotti, C., Madella, M. (2017). A methodological approach to the study of microbotanical remains from grinding stones: a case study in northern gujarat (india). Vegetation History Archaeobot. 26, 43–57. doi: 10.1007/s00334-016-0557-z
Ge, Y., Lu, H., Wang, C., Gao, X. (2020). Phytoliths in selected broad-leaved trees in china. Sci. Rep. 10, 15577. doi: 10.1038/s41598-020-72547-w
Haase, N. U., Plate, J. (1996). Properties of potato starch in relation to varieties and environmental factors. Starch/Stärke 48, 167–171. doi: 10.1002/star.19960480503
Hardy, K., Radini, A., Buckley, S., Sarig, R., Copeland, L., Gopher, A., et al. (2016). Dental calculus reveals potential respiratory irritants and ingestion of essential plant-based nutrients at lower palaeolithic Qesem cave Israel. Quaternary International 398, 129–135. doi: 10.1016/j.quaint.2015.04.033
Hart, T. C. (2014). Analysis of starch granule produced in select taxa encountered in southwest asia. Ethnobiol. Lett. 5, 135. doi: 10.14237/ebl.5.2014.251
Haslam, M. (2004). The decomposition of starch granule in soils: implications for archaeological residue analyses. J. Archaeol Sci. 31, 1715–1734. doi: 10.1016/j.jas.2004.05.006
He, G., Xie, T., Xie, B. (2003). Study on granule properties of quercus variabilis starch. J. Mountain Agric. Biol. 3, 226–229.
Hipp, A. L., Manos, P. S., Hahn, M., Avishai, M., Bodénès, C., Cavender-Bares, J., et al. (2020). Genomic landscape of the global oak phylogeny. New Phytol. 226, 1198–1212. doi: 10.1111/nph.16162
Hodson, M. J., White, P. J., Mead, A., Broadley, M. R. (2005). Phylogenetic variation in the silicon composition of plants. Ann. Bot. 96, 1027–1046. doi: 10.1093/aob/mci255
Hou, Y., Wang, J. (1996). Acorn as a raw material for the production of health white wine. Brewing 4, 13.
Huan, X. J., Li, Q., Ma, Z. K., Yang, X. Y. (2014). Fan-shaped phytoliths reveal the process of rice domestication at shangshan site, zhejiang province. Quaternary Sci. 34 (1), 106–113. doi: 10.3969/j.issn.1001-7410.2014.01.13 (in Chinese)
Hu, F., Li, J., Li, R. (2000). Oak plant resources in hunan with reference to its integrated utilization. J. Cent. South For. Univ. 20, 41–45. doi: 10.14067/j.cnki.1673-923x.2000.04.009
Jiang, Y. P. (2007). Excavation on the shangshan site in pujiang county, zhejiang. Archaeology 9, 97–98.
Ji, X. P., Kuman, K., Clarke, R. J., Forestier, H., Li, Y. H., Ma, J., et al. (2016). The oldest hoabinhian technocomplex in Asia (43.5 ka) at xiaodong rockshelter, yunnan province, southwest China. Quaternary Int. 400, 166–174. doi: 10.1016/j.quaint.2015.09.080
Jones, M. K., Liu, X. (2009). Origins of agriculture in East Asia. Science 324, 730–731. doi: 10.1126/science.1172082
Liu, L. (2008). “Prehistoric grinding stone tools, nut collection, settlement and agricultural origin in China,” in Collection of essays for celebrating the nineties birthday of he bingdi (San Qin Publishing House), 105–127.
Liu, L., Chen, X. (2012). The archaeology of china: from the late paleolithic to the early bronze age. Cambridge: Cambridge University Press. doi: 10.1017/CBO9781139015301
Liu, L., Duncan, N. A., Chen, X. C., Liu, G. X., Zhao, H. (2015). Plant domestication, cultivation and foraging by the first farmers in early neolothic northeast China: Evidence from microbotanical remains. Holocene 25, 12. doi: 10.1177/0959683615596830
Liu, L., Field, J., Weisskopf, A., Webb, J., Jiang, L. P., Wang, H. M., et al. (2010). The exploitation of acorn and rice in early Holocene Lower Yangzi River, China. Acta Anthropol. Sinica 29, 317–336. doi: 10.1017/S0004972710001772
Liu, L., Ge, W., Bestel, S., Jones, D., Shi, J. M., Song, Y. H., et al. (2011). Plant exploitation of the last foragers at shizitan in the middle yellow river valley China: evidence from grinding stones. J. Archaeol Sci. 38 (12), 3524–3532. doi: 10.1016/j.jas.2011.08.015
Liu, L., Lee, G. A., Jiang, L. P., Zhang, J. Z. (2007). Evidence for the early beginning (c. 9000 cal. BP) of rice domestication in China: a response. Holocene 17, 1059–1068. doi: 10.1177/0959683607085121
Li, M. Q., Yang, X. Y., Wang, H., Wang, Q., Jia, X., Ge, Q. S. (2010). Starch granule from dental calculus reveal ancient plant foodstuffs at chenqimogou site, gansu province. Sci. China Earth Sci. 40, 486–492. doi: 10.1007/s11430-010-0052-9
Lü, H. Y., Li, Y. M., Zhang., J. P., Yang, X. Y., Ye, M. L., Li, Q., et al. (2014). Component and simulation of the 4000-year-old noodles excavated from the archaeological site of lajia in qinghai, China. Chin. Sci. Bull. 59, 5136–5152. doi: 10.1007/s11434-014-0663-1
Lu, H. Y., Zhang, J. P., Wu, N. Q., Liu, K., Xu, D. K., Li, Q. (2009). Phytoliths analysis for the discrimination of foxtail millet(Setaria italica)and common millet(Panicum miliaceum). PloS One 4, 1–15. doi: 10.1371/journal.pone.0004448
Ma, Z. K., Li, Q., Huan, X. J., Yang, X. Y., Zheng, J. Y., Ye, M. L. (2014). Plant microremains provide direct evidence for the functions of stone knives from the lajia site, northwestern China. Chin. Sci. Bull. 59, 1151–1158. doi: 10.1007/s11434-014-0174-0
Mason, S. L. R. (1996). “Acornutopia? Determining the role of acorns in past human subsistence,” in Food in Antiquity (Exeter: University of Exeter Press), 12–24.
Nikuni, Z. (1978). Studies on starch granules. Starch/Stärke 30, 105–111. doi: 10.1002/star.19780300402
Oliveira, A. B., Rasnusson, D. C., Fulcher, R. G. (1994). Genetic aspects of starch granule traits in barley. Crop Sci. 34, 1176–1180. doi: 10.2135/cropsci1994.0011183X003400050006x
Pearsall, D. M., Chandler-Ezell, K., Zeidler, J. A. (2004). Maize in ancient Ecuador: results of residue analysis of stone tools from the real alto site. J. Archaeol Sci. 31, 423–442. doi: 10.1016/j.jas.2003.09.010
Perry, L., Dickau, R., Zarrillo, S., Holst, I., Pearsall, D. M., Piperno, D. R., et al. (2007). Starch fossils and the domestication and dispersal of chili peppers (Capsicum spp. L.) in the Americas. Science 315, 986–988. doi: 10.1126/science.1136914
Piperno, D. R., Holst, I. (1998). The presence of starch granule on prehistoric stone tools from the humid neotropics: Indications of early tuber use and agriculture in Panama. J. Archaeol Sci. 25, 765–776. doi: 10.1006/jasc.1997.0258
Piperno, D. R., Ranere, A. J., Holst, I., Hansell, P. (2000). Starch granule reveal early root crop horticulture in the Panamanian tropical forest. Nature 407, 894–897. doi: 10.1038/35038055
Piperno, D. R., Weiss, E., Holst, I., Nadel, D. (2004). Processing of wild cereal granules in the upper Palaeolithic revealed by starch granule analysis. Nature 430, 670–673. doi: 10.1038/nature02734
Reichert, E. T. (1913). The differentiation and specificity of starches in relation to genera, species, etc. (in two parts) (Washington D.C: Carnegie Institution of Washington).
Sivak, M. N., Preiss, J. (1998). Physiochemical structure of the starch granule. Starch: Basic Sci. Biotechnol., 13–32.
Sterling, C. (1984). “The light microscope in food analysis,” in Food analysis: Principles and techniques. Eds. Gruenwedel, W. D., Whitaker, J. R., 175–201. New York: Marcel Dekker.
Sun, D. H., Liu, Y., Chen, G. T. (1981). The cishan site in wu’an, hebei province. Acta Archaeol. Sinica 3, 407–414.
Sun, Q. L., Shuo, Z., Wu, Y., Yang, Y. M. (2019). Starch granule analysis of the grooved basin from the lingjiatan site, Hanshan county, Anhui Province. Acta Anthropol. Sinica 38, 16. doi: 10.16359/j.cnki.cn11-1963/q.2018.0036
Tao, D. (2016). Research of archaeobotanical and origin of agriculture in China. J. Zhengzhou Univ. 49, 109–112.
Tester, R. F. (1997). “Starch: The polysaccharide fractions,” in Starch: Structure and functionality. Eds. Frazier, P. J., Richmond, P., Donald, A. M. (London: Royal Society of Chemistry, Special Publications No. 205), 163–171.
Torrence, R., Wright, R., Conway, R. (2004). Identification of starch granules using imageanalysis and multivariate techniques. J. Archaeol Sci. 31, 519–532. doi: 10.1016/j.jas.2003.09.014
Wang, X. (2006). Xiaohuangshan hill: An archaeological examination. J. Shaoxing Coll. Arts Sci. 2, 6–10.
Wang, C. X., Cheng, J. T., Cao, J. N., Ta, L., Xiong, Z. L., Guan, Y. (2017). Diet and related issues revealed by starch granule analysis on pottery unearthed from the Weijiawopu site, Inner Mongolia. Acta Anthropol. Sinica 36, 406–414. doi: 10.16359/j.cnki.cn11-1963/q.2017.0055
Wang, Y. J., Lü, H. Y. (1993). A study on plant opal and its use (Beijing: China ocean press), 1–267.
Wang, J. B., Zhao, X. Y., Wang, H., Liu, L. (2019). Plant exploitation of the first farmers in northwest china: microbotanical evidence from dadiwan. Quaternary Int. 529, 3–9. doi: 10.1016/j.quaint.2018.10.019
Wilson, J., Hardy, K., Allen, R., Copeland, L., Wrangham, R., Collins, M. (2010). Automated classification of starch granules using supervised pattern recognition of morphological properties. J. Archaeol Sci. 37, 594–604. doi: 10.1016/j.jas.2009.10.024
Wu, Y., Jiang, L. P., Zheng, Y. F., Wang, C. S., Zhao, Z. J. (2014). Morphological trend analysis of rice phytolith during the early Neolithic in the Lower Yangtze. J. Archaeol Sci. 49, 326–331. doi: 10.1016/j.jas.2014.06.001
Xie, B. X., Xie, T. (2002). Exploitation study of acorn resources in China. J. Cent. South Univ. For. Technol. 1, 94–103.
Yang, X. Y., Jiang, L. P. (2010). Starch grain analysis reveals ancient diet at Kuahuqiao site, Zhejiang Province. Chin. Sci. Bull. 55, 600–606. doi: 10.1007/s11434-009-0545-0
Yang, X. Y., Kong, Z., Liu, C., Zhang, Y., Ge, Q. (2009a). Characteristics of starch granule from main nuts in north China. Quaternary Sci. 29, 153–158.
Yang, X. Y., Lü, H. Y., Cui, C. (2009b). Starch grain analysis reveals function of grinding stone tools at Shangzhai site, Beijing. Science in China Series D: Earth Sciences 39, 1266–1273. doi: 10.1007/s11430-009-0089-9
Yang, X. Y., Lü, H. Y., Xia, Z. (2006). Starch analysis method and practice in Archaeology relics. Archaeol. Cult. Relics, 3–87-91. doi: 10.3969/j.issn.1000-7830.2006.03.015
Yang, X. Y., Ma, Z. K., Li, J., Yu, J. C., Stevens, C., Zhuang, Y. J. (2015). Comparing subsistence strategies in different landscapes of north China 10000 years ago. Holocene 25 (12). doi: 10.1177/0959683615596833
Yang, X. Y., Wan, Z. W., Perry, L., Lu, H. Y., Wang, Q., Zhao, C. H., et al. (2012a). Early millet use in northern China. Proc. Natl. Acad. Sci. United States America 109, 10. doi: 10.1073/pnas.1115430109
Yang, X. Y., Zhang, J. P., Perry, L., Ma, Z. K., Wan, Z. W., Li, M. Q., et al. (2012b). From the modern to the archaeological: starch grains from millets and their wild relatives in China. J. Archaeol Sci. 39. doi: 10.1016/j.jas.2011.09.001
Yiu, S. H. (1993). Food microscopy and the nutritional quality of cereal foods. Food Struct. 12, 123–133.
Zhang, J. P., Lu, H. Y., Wu, N. Q., Yang, X. Y., Diao, X. M. (2011a). Phytolith analysis for differentiating be- tween foxtail Millet (Setaria italica) and green foxtail (Setaria viridis). PloS One 6, 5. doi: 10.1371/journal.pone.0019726
Zhang, Y. H., Weng, Y., Yao, L., Zhang, J. Z., Zhou, Y. J., Fang, F., et al. (2011b). Identification and analysis of starch granules on the surface of the slabs from the Peiligang Site. Quaternary Sci. 5, 891–899. doi: 10.3969/j.issn.1001-7410.2011.05.14
Zhao, Z. (1998). The middle Yangtze region in China is one place where rice was domesticated: Phytolith evidence from the diaotonghuan cave, northern Jiangxi. Antiquity 72, 885–897. doi: 10.1017/S0003598X00087524
Zhao, C. H. (2006). Prehistoric donghulin site in mentougou District,Beijing city. Archaeology 7, 3–8.
Zhao, Z. (2014). The process of origin of agriculture in China: agriculture evidence from flotation results. Quaternary Sci. 34, 73–84. doi: 10.3969/j.issn.1001-7410.2014.10
Zhao, Z. (2019). Introduction of the origin of agriculture in China. Res. Heritages Preserv. 4, 1–7.
Keywords: South China, nut fruits, Quercus, morphological characteristics, starch granule
Citation: Yu T, Yang Q, Deng M, Cheng N, Yao K, Yang W, Ji X and Zheng H (2022) Morphological characteristics of seed starch granules of Fagaceae in South China and their implication in paleodiet. Front. Plant Sci. 13:977152. doi: 10.3389/fpls.2022.977152
Received: 24 June 2022; Accepted: 14 October 2022;
Published: 14 November 2022.
Edited by:
Ying Guan, Institute of Vertebrate Paleontology and Paleoanthropology (CAS), ChinaReviewed by:
Xiujia Huan, Institute of Geology and Geophysics (CAS), ChinaCopyright © 2022 Yu, Yang, Deng, Cheng, Yao, Yang, Ji and Zheng. This is an open-access article distributed under the terms of the Creative Commons Attribution License (CC BY). The use, distribution or reproduction in other forums is permitted, provided the original author(s) and the copyright owner(s) are credited and that the original publication in this journal is cited, in accordance with accepted academic practice. No use, distribution or reproduction is permitted which does not comply with these terms.
*Correspondence: Qing Yang, eWFuZ3FpbmdAeW51LmVkdS5jbg==
Disclaimer: All claims expressed in this article are solely those of the authors and do not necessarily represent those of their affiliated organizations, or those of the publisher, the editors and the reviewers. Any product that may be evaluated in this article or claim that may be made by its manufacturer is not guaranteed or endorsed by the publisher.
Research integrity at Frontiers
Learn more about the work of our research integrity team to safeguard the quality of each article we publish.