- 1Department of Life Sciences, POSTECH, Pohang, South Korea
- 2International Research Centre for Environmental Membrane Biology, Foshan University, Foshan, China
- 3RIKEN Center for Sustainable Resource Science, Yokohama, Japan
- 4Graduate School of Bioagricultural Sciences, Nagoya University, Nagoya, Japan
The Arabidopsis ABC transporter ABCG11 transports lipidic precursors of surface coating polymers at the plasma membrane of epidermal cells. Mutants in ABCG11 exhibit severe developmental defects, suggesting that ABCG11 might also participate in phytohormone-mediated development. Here, we report that ABCG11 is involved in cytokinin-mediated development. The roots of abcg11 mutant seedlings failed to respond to cytokinins and accumulated more cytokinins than wild-type roots. When grown under short-day conditions, abcg11 exhibited longer roots and shorter hypocotyls compared to wild type, similar to abcg14, a knockout mutant in a cytokinin transporter. Treatment with exogenous trans-zeatin, which inhibits primary root elongation in the wild type, enhanced abcg11 primary root elongation. It also increased the expression of cytokinin-responsive Arabidopsis response regulator (ARR) genes, and the signal of the TCS::GFP reporter in abcg11 roots compared to wild-type roots, suggesting that cytokinin signaling was enhanced in abcg11 roots. When we treated only the roots of abcg11 with trans-zeatin, their shoots showed lower ARR induction than the wild type. The abcg14 abcg11 double mutant did not have additional root phenotypes compared to abcg11. Together, these results suggest that ABCG11 is necessary for normal cytokinin-mediated root development, likely because it contributes to cytokinin transport, either directly or indirectly.
Introduction
The ATP-binding cassette (ABC) transporter ABCG11 is required for the transport of cutin and wax components to the extracellular matrix (Panikashvili et al., 2007). A mutant in Arabidopsis (Arabidopsis thaliana) ABCG11 displays diverse developmental defects, including organ fusion, stunted growth, altered petal, and silique morphology, altered root suberin composition, and sterility (Bird et al., 2007; Panikashvili et al., 2007, 2010; McFarlane et al., 2010). ABCG11 is a half-size ABC protein that usually forms dimers to exert its transporter function (Verrier et al., 2008). Indeed, ABCG11 was shown to form homodimers and heterodimers with many other half-size ABCG proteins: ABCG5, ABCG9, ABCG12, and ABCG14 (Do et al., 2021). ABCG11 homodimers and ABCG11–ABCG12 heterodimers export lipidic molecules from epidermal cells to form the protective cuticle (Bird et al., 2007; McFarlane et al., 2010; Panikashvili et al., 2010). ABCG11–ABCG9 heterodimers regulate vascular development (Le Hir et al., 2013), while ABCG11–ABCG5 heterodimers mediate the transport of wax precursors in seedlings (Lee et al., 2020). ABCG11 also interacts with ABCG14 in Arabidopsis protoplasts, based on co-immunoprecipitation (Co-IP) assays (Le Hir et al., 2013). The multiple dimers involving ABCG11 might transport structurally different as well as similar substrates, and a broad substrate range for ABCG11 might explain the pleiotropic and severe phenotypes observed in abcg11 knockout mutants (McFarlane et al., 2010).
Among the many binding partners of ABCG11, ABCG14 is unique in its role in the root-to-shoot translocation of the plant hormone trans-zeatin (tZ)-type cytokinins (Ko et al., 2014; Zhang et al., 2014). Cytokinins are a group of plant hormones critical for regulating plant growth and development (Moubayidin et al., 2010; Tokunaga et al., 2012; Yuan et al., 2016; Mellor et al., 2017; Janečková et al., 2018; Rong et al., 2018; Vatén et al., 2018; Wybouw and De Rybel, 2019). Cytokinins undergo long-distance transport from the root to the shoot via the xylem, or from the shoot to the root via the phloem, to coordinate the growth of both shoot and root (Hirose et al., 2008; Bishopp et al., 2011). The Arabidopsis abcg14 mutant was proposed to be defective in the long-distance transport of tZ-type cytokinins due to its dwarf shoot phenotype and low tZ-type cytokinin levels in shoots but high levels in roots. In addition, xylem exudates from abcg14 contain much less tZ-type cytokinins than the wild type (WT; Ko et al., 2014). Since ABCG14 is also a half-size ABC protein, it should form a homodimer or heterodimer to transport cytokinins, but the identity of its binding partner for the root transport of cytokinins is unknown. Here, we report multiple lines of evidence that support ABCG11 as the partner protein for ABCG14 in mediating cytokinin-dependent development, especially of the root. We show that abcg11 mutant is characterized by an alteration of cytokinin concentrations and cytokinin-related phenotypes. We also discuss how ABCG11 might function to modulate cytokinin-dependent root growth.
Materials and methods
Plant materials and growth conditions
Seeds were surface sterilized with NaOCl and placed in the dark for 2 d at 4°C for stratification before being sown on half-strength Murashige and Skoog (MS) or MGRL medium (Fujiwara et al., 1992) solidified with 0.8% (w/v) phytoagar (Duchefa Biochemie, Netherlands). The medium was supplemented with 20 nM tZ when indicated. Seedlings were grown vertically in a growth chamber (22/18°C; 16 h light/8 h dark or 8 h light/16 h dark).
Two alleles of abcg11, SALK_119873 and SALK_131624, were obtained from the Nottingham Arabidopsis Stock Centre. SALK_119873 was reported to be defective in the secretion of cuticular lipids (Bird et al., 2007), while SALK_131624 was reported previously as cof1-3 (cuticular defect and organ fusion; Ukitsu et al., 2007). The abcg14 mutant line (SK_15918) was previously described (Ko et al., 2014).
To generate Col-0 and abcg11 lines expressing a cytokinin signaling marker system, the TCS::GFP (Two Component signaling Sensor::green fluorescent protein) vector was introduced into Agrobacterium (Agrobacterium tumefaciens) strain GV3101 (Zürcher et al., 2013) for Agrobacterium-mediated floral dip transformation of Col-0 and abcg11/+ plants. Basta was used to select transformants. Seeds were harvested from primary transformants and used for TCS::GFP fluorescence analysis. Homozygous mutant seedlings were identified based on their characteristic organ fusion phenotype.
Quantitative real-time PCR
Total RNA was extracted from the indicated samples using RNAiso Plus (Takara, Japan) according to the manufacturer’s protocol. First-strand cDNAs were synthesized from 2 μg RNA using the Promega reverse transcriptase kit (Promega, United States). Quantitative real-time PCR was performed using TB Green® Premix Ex Taq™ Tli RNase H Plus (TAKARA),1 following the manufacturer’s instructions. The primers used for RT-qPCR are shown in Supplementary Table S1.
Cytokinin quantification
Shoots and roots of 12-d-old seedlings grown on half-strength MS plates were harvested and immediately frozen in liquid nitrogen. Cytokinin contents were quantified from the frozen samples using ultra-performance liquid chromatography–tandem mass spectrometry (AQUITY UPLC System/XEVO-TQS; Waters) with an octadecylsilyl column (AQUITY UPLC HSS T3, 1.8 μm, 2.1 mm × 100 mm; Waters). The detailed method of extraction and quantification was described before (Kojima et al., 2009).
3H-tZ translocation assay
Five 12-d-old seedlings per genotype were transferred to a 48-well plate, with each well containing 900 μl of half-strength MS medium and pH adjusted to pH 5.8 with MES and KOH. Only the roots were submerged in medium. After 30 min of preincubation, 3H-tZ or 3H-H2O was added to the wells to a final concentration of 50 nM or 0.5 μCi/ml, respectively, and the seedlings were incubated for an additional 1 h. The shoots were harvested and their radioactivity was counted with a liquid scintillation counter. The preparation of and quality checks for 3H-tZ were performed, as described previously (Takei et al., 2003).
Results
The abcg11 knockout mutant is altered in the expression of cytokinin-response marker genes
The abcg11 mutant SALK_119873 was shown in previous reports to exhibit extremely severe growth retardation, multiple thin and short inflorescence stems, as well as fusion between shoots and reproductive organs (Bird et al., 2007; Luo et al., 2007; Panikashvili et al., 2007). It has been widely used in ABCG11 research. Here, this mutant is designated abcg11. Since the abcg11 mutant is sterile, we used the progeny of abcg11/+ heterozygous plants for analysis. We easily identified homozygous abcg11 seedlings from their characteristic fusion of shoots relative to the normal appearance of WT Col-0 and abcg11/+ seedlings (Figures 1B,C).
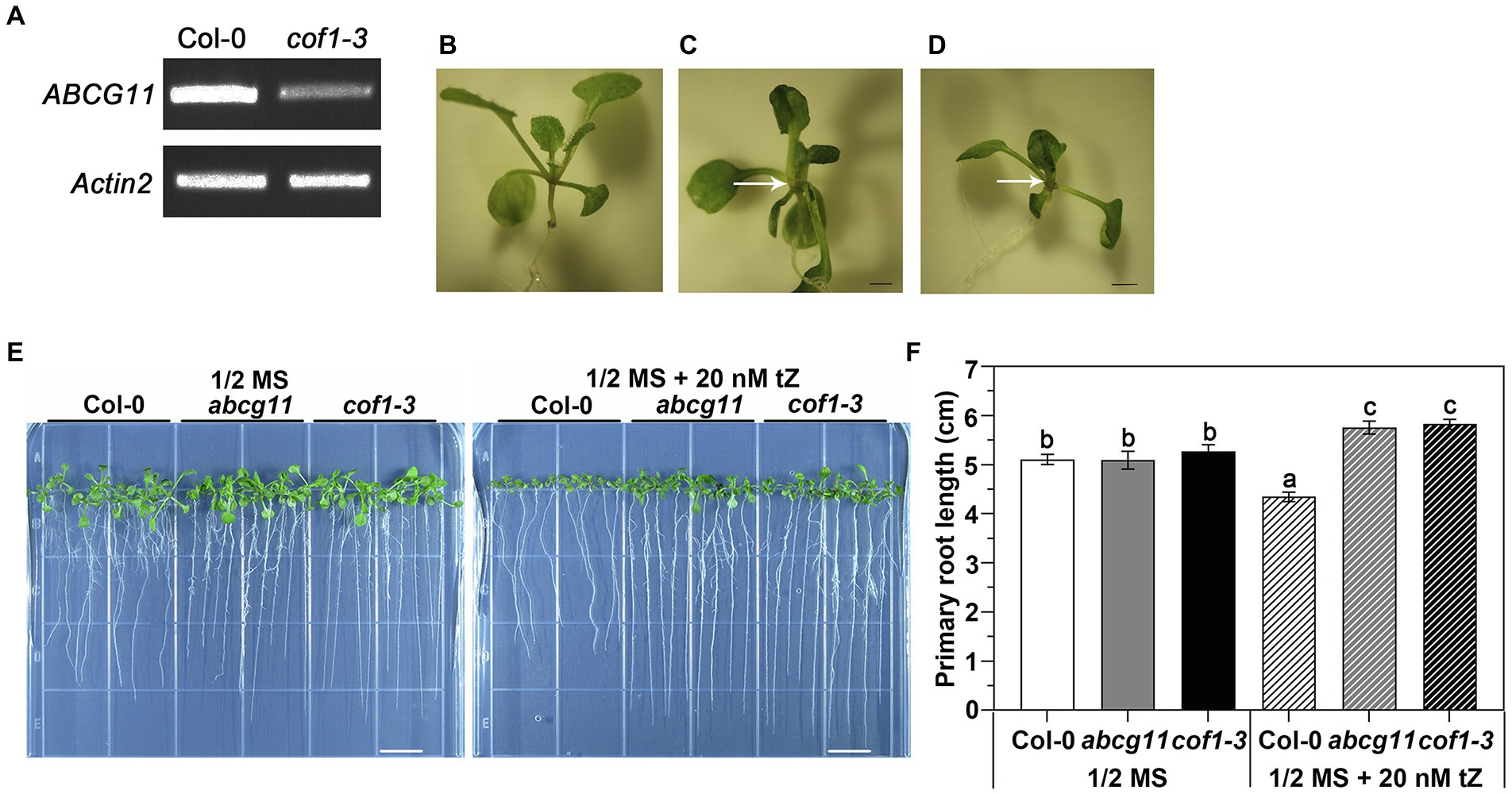
Figure 1. Second allele of abcg11 T-DNA insertion line cof1-3 (SALK_131624) exhibits an altered response to cytokinin, similar to abcg11. (A) cof1-3 is a knockdown mutant of ABCG11. RT-PCR analysis of AtABCG11. Actin2 (At3g18780) was amplified as an internal control. The primers are listed in Supplementary Table S3. (B–D) Photographs of the shoots of 12-day-old seedlings of Col-0, abcg11, and cof1-3. The arrows point the sites of organ fusion. Scale bar = 1.0 mm. (E) The similar responses of cof1-3 and abcg11 roots to 20 nM trans-zeatin (tZ) treatment under long-day conditions (16 h/8 h, day/night). The photographs were taken at 12 days after seed sowing. The photos are representatives of four independent experiments. Scale bars = 1 cm. (F) The average primary root length of the plants shown in (E). The error bars represent the standard error (SE; n = 40). The different letters indicate the significant differences determined by one-way ANOVA (p < 0.05).
To test whether the abcg11 mutant showed any alteration in phytohormone responses, we determined relative transcript levels for marker genes for cytokinins, auxin, and abscisic acid (ABA) in the WT and abcg11 mutant seedlings. The expression of most cytokinin-response marker genes, such as ARR3, CKX3, CKX4, and CKX5, differed between abcg11 and the WT in both roots and shoots (Figures 2A,B). By contrast, only one auxin-response (ARF8) and one ABA-induced gene (ABI5) were expressed to different levels in the two genotypes (Figures 2C–F), prompting us to focus on cytokinins by measuring their concentrations in roots and shoots from the WT and abcg11.
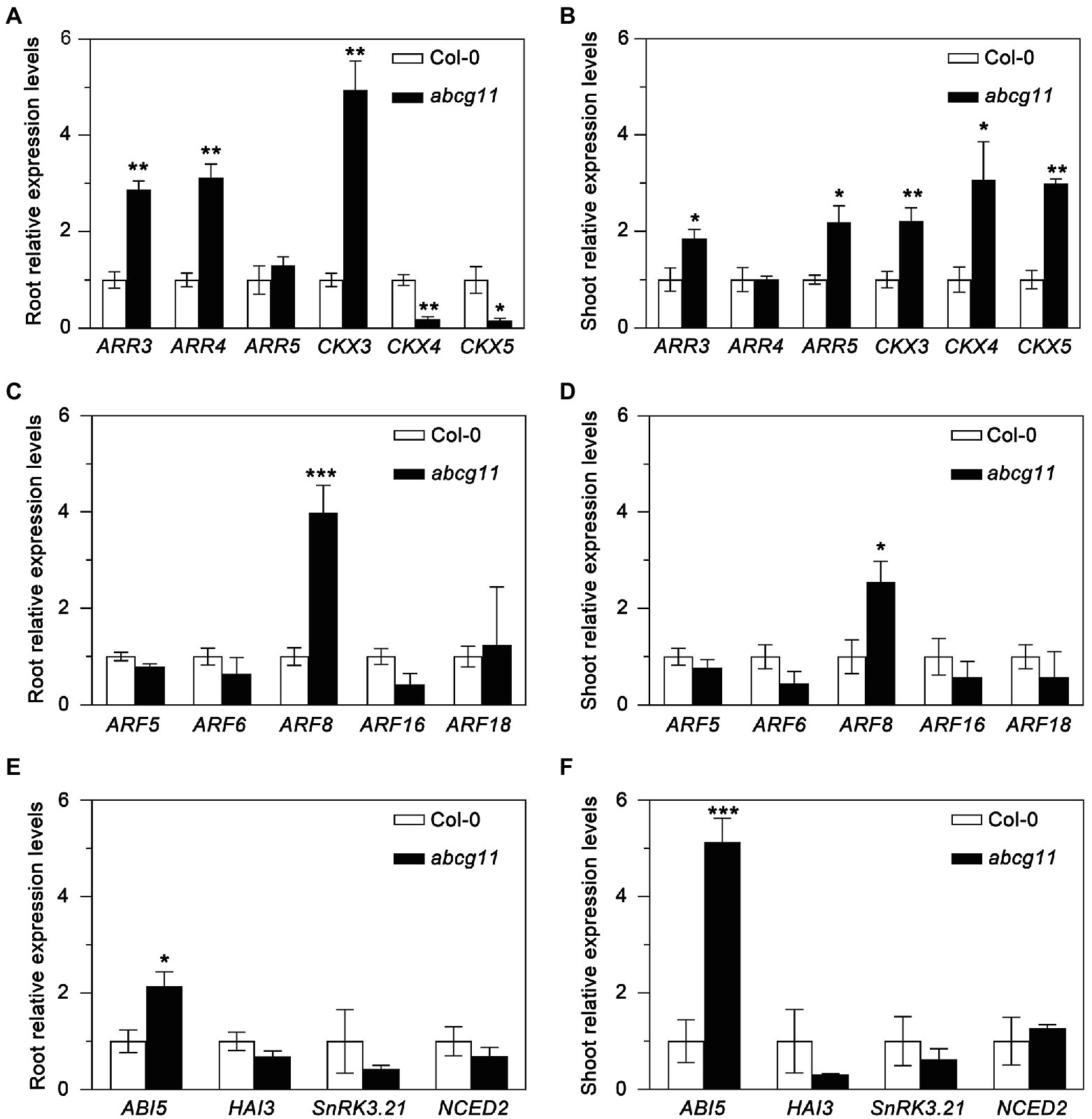
Figure 2. Expression of phytohormone-induced genes in Col-0 and the abcg11 mutant. Relative expression levels of cytokinin- (A,B), auxin- (C,D), and abscisic acid- (E,F) induced genes in roots (A,C,E) and shoots (B,D,E). Seedlings were grown vertically on half-strength MS medium for 12 d (16 h light/8 h dark). TUBULIN8 served as an internal control; values for Col-0 were set to 1. Data are shown as means ± standard error (SE; n = 4). Asterisks represent significant differences by Student’s t-test: *p < 0.05; **p < 0.01; ***p < 0.001.
Concentrations of several cytokinins are higher in abcg11 than in the WT
We measured the contents of the tZ-type cytokinins, isopentyladenine (iP)-type cytokinins, and cis-zeatin (cZ)-type cytokinins in roots and shoots of WT and abcg11 mutant seedlings. In abcg11 roots, the concentrations of the three tZ-types cytokinins tZ, tZ7G, and tZ9G were higher than in WT roots, resulting in a significant (p < 0.05) difference in the total amount of tZ-type cytokinins (Figure 3A; Supplementary Table S4). Concentrations for tZ7G and tZROG were similarly higher in abcg11 shoots, while those of tZOG were lower, relative to WT shoots, resulting in a comparable total sum of tZ-type cytokinins in the two genotypes (Figure 2B; Supplementary Table S4). The concentrations of two iP-types cytokinin, iP7G and iP9G, were higher in abcg11 relative to the WT in both roots and shoots (Figures 3C,D; Supplementary Table S4). While cZ-type cytokinins exhibited much lower levels than those of the other types, they accumulated to higher levels in abcg11 shoots compared to WT shoots, but showed no significant differences in roots, with the exception of cZROG, whose concentrations were very low (Figures 3E,F; Supplementary Table S4). The overall changes in cytokinin concentrations in abcg11 suggested that cytokinin metabolism and/or transport differ in the mutant.
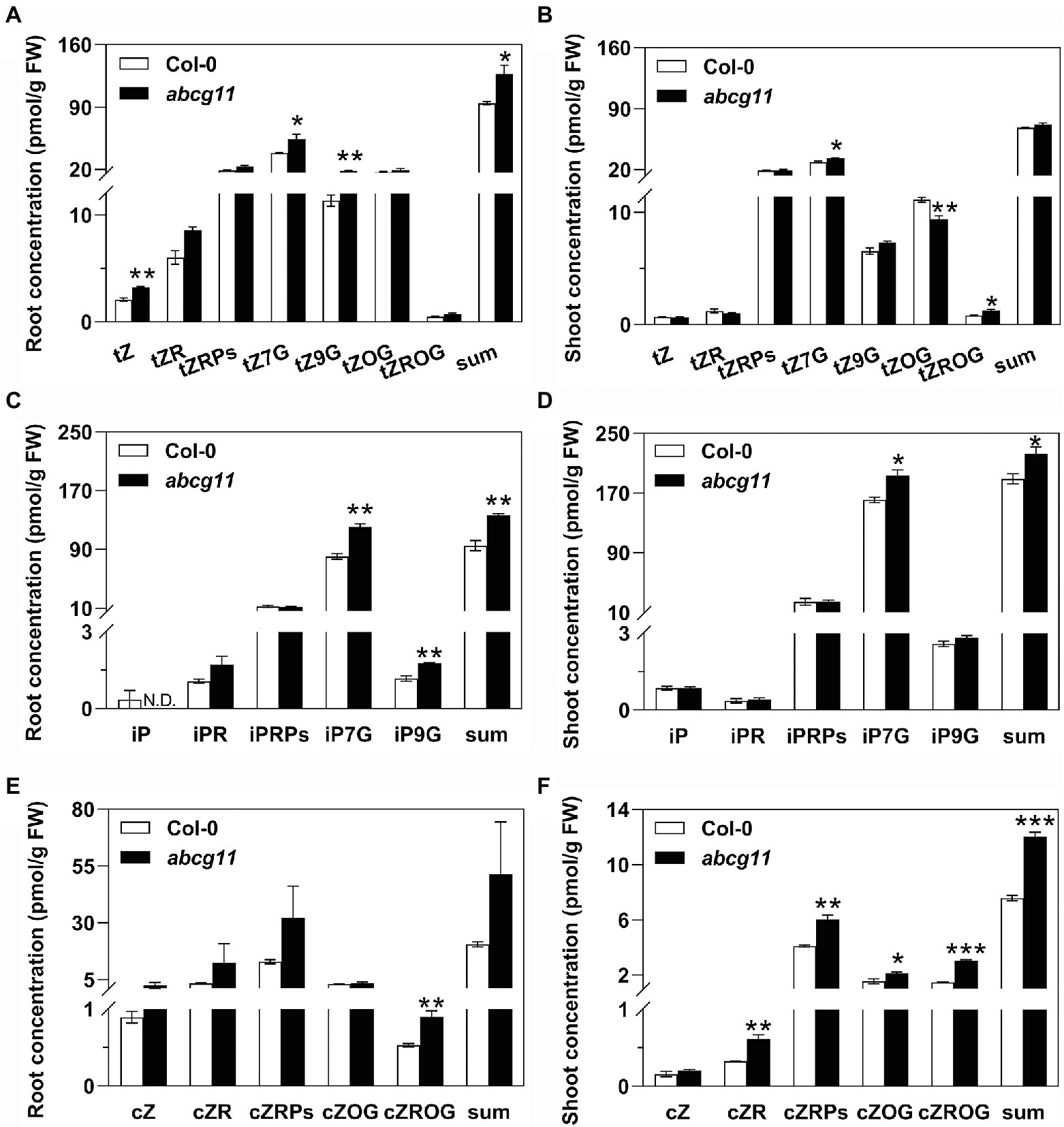
Figure 3. The concentration of several cytokinin types is higher in abcg11 than in the wild type. Concentrations for the trans-zeatin (tZ)-type (A,B), isopentyladenine (iP)-type (C,D), and cis-zeatin (cZ)-type (E,F) cytokinins in roots (A,C,E) and shoots (B,D,F) of Col-0 and abcg11. Seedlings were grown on half-strength MS medium for 12 d under long-day conditions (16 h light/8 h dark). Data are shown as means ± SE (n = 4). Asterisks represent significant differences by Student’s t-test: *p < 0.05; **p < 0.01.
abcg11 exhibits root phenotypes similar to those of abcg14, a cytokinin transporter knockout mutant
To assess the potential role of ABCG11 in cytokinin transport, we compared the phenotype of the abcg11 mutant with that of the previously reported cytokinin transporter mutant abcg14 (Ko et al., 2014; Zhang et al., 2014). In previous research, abcg14 seedlings were grown on MGRL medium under short-day conditions (Ko et al., 2014). When the seeds derived from abcg11/+ plants were sown and grown under the same conditions, the seedlings exhibited two different growth patterns; some seedlings had longer roots and shorter hypocotyls than others (Figure 4A). Under the same growth conditions, the abcg14 mutant also exhibited longer roots and shorter hypocotyls than its WT background Col-4 (Figures 4A,C). Importantly, all progenies with longer roots and shorter hypocotyls from abcg11/+ plants were homozygous for the T-DNA insertion, while the remaining seedlings either harbored the WT or abcg11/+ genotype (Figure 4B). In addition, quantified results also revealed that the primary root length of both abcg11 and abcg14 mutants had significantly longer roots compared to their respective WT (p < 0.01, Figure 4C). These results suggested that ABCG11 and ABCG14 may function similarly in regulating root and hypocotyl growth.
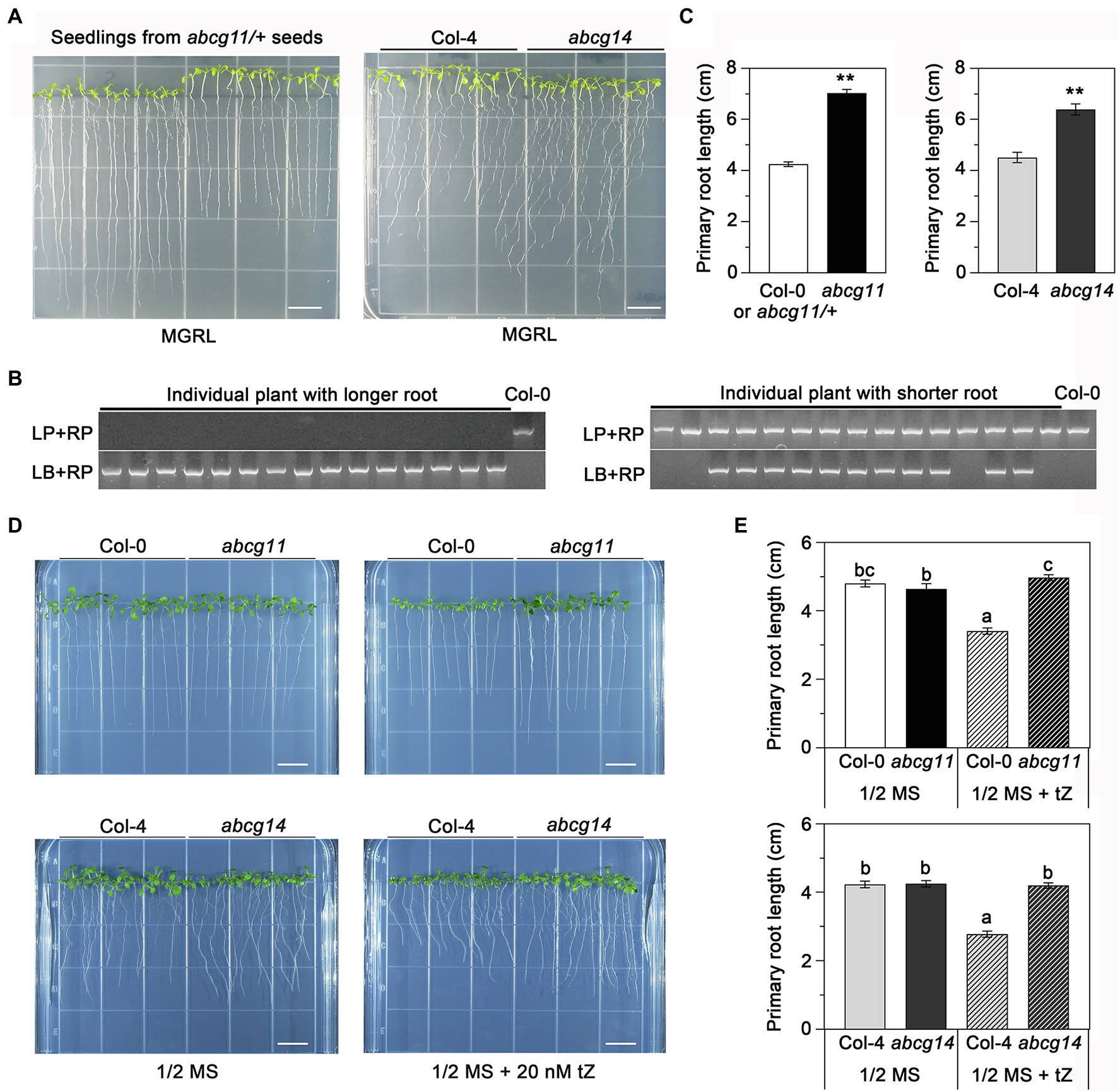
Figure 4. abcg11 behaves similarly as abcg14 in root growth on MGRL medium and in response to exogenous cytokinins. (A, left panel) The progeny of abcg11/+ plants exhibit two distinct phenotypes. The seeds were sown on MGRL medium and grown vertically for 12 days under short-day conditions (8 h light/16 h dark). Note that seedlings were rearranged according to their primary root length before taking the picture. (A, right panel) Phenotype of abcg14 under the same conditions. Scale bars, 1 cm. (B) Genotyping of the individual seedlings shown in (A), left panel. Note how the seedlings with longer roots are abcg11 homozygous mutants. The primers for genotyping are listed in Supplementary Table S2. (C) Primary root length of seedlings in (A). Data are shown as means ± SE (n = 15). Asterisks represent significant differences by Student’s t-test: **p < 0.01. (D) Phenotype of seedlings grown with or without 20 nM trans-zeatin (tZ) for 12 days under long-day conditions (16 h light/8 h dark) on half-strength MS medium. The photographs are representative of three independent experiments. Scale bars, 1 cm. (E) Primary root length of the seedlings in (D). Data are shown as means ± SE (n = 40). Different letters indicate significant differences, as determined by one-way ANOVA (p < 0.05).
We also tested how abcg11 and abcg14 roots responded to exogenous cytokinins when grown on half-strength MS medium. The addition of 20 nM tZ to the medium reduced the growth of primary roots of the WT Col-0 and Col-4 by about 33% but had no effect on abcg11 or abcg14 (Figures 4D,E), revealing that abcg11 responds similarly as abcg14 to exogenous cytokinin treatment. These results indicated that ABCG11 and ABCG14 have similar functions in root development.
We obtained another T-DNA allele in ABCG11 (SALK_131624), which was previously designated cof1-3 (Ukitsu et al., 2007) and tested its root response to tZ. Homozygous cof1-3 plants are sterile, necessitating the use of segregating progeny, as with abcg11. Seedlings germinated from the seeds collected from cof1-3/+ plants exhibited two distinct growth patterns, as observed with the progeny from abcg11/+: with some seedlings experiencing organ fusion (Figure 1D). Seedlings with the organ fusion phenotype were homozygous for cof1-3 and only decrease but did not abrogate transcription of ABCG11, making cof1-3 a knockdown mutant (Figure 1A). Homozygous cof1-3 seedling roots displayed a similar response to exogenous cytokinins as abcg11 (Figure 1E), with a modest root lengthening in both mutants relative to untreated seedlings, in contrast to the shorter roots seen in their WT upon cytokinin treatment (Figure 1F). That two independent mutant alleles of ABCG11 exhibited a similar alteration in root growth response to cytokinin treatment, thus supporting a role for ABCG11 in cytokinin responses.
Cytokinin signaling is enhanced in abcg11 mutant roots upon cytokinin treatment
We tested whether the levels of the cytokinin signal itself were different between the WT and abcg11. When treated with tZ, the root expression of type-A ARRs (Arabidopsis response regulators), which is induced by cytokinin (Rashotte et al., 2003; Argyros et al., 2008), was induced 9 to 20-fold in abcg11 mutant roots, but only 3 to 10-fold in WT roots, indicating that the induction levels in abcg11 were much higher than in the WT (Figure 5A). We also introduced the TCS::GFP reporter construct, a marker of the transcriptional output of cytokinin signaling (Zürcher et al., 2013), into abcg11/+ plants and determined the fluorescence intensity from the green fluorescent protein (GFP) in the transgenic progeny, subjected or not to 20 nM tZ treatment. We isolated abcg11 knockout seedlings harboring the TCS::GFP transgene based on their leaf or stem organ fusion phenotypes as above. When treated with 20 nM tZ, TCS::GFP fluorescence intensity increased more than two-fold in abcg11 mutant roots (Figures 5B,C) but did not change significantly in WT roots, indicating that cytokinin signaling is enhanced in abcg11 roots.
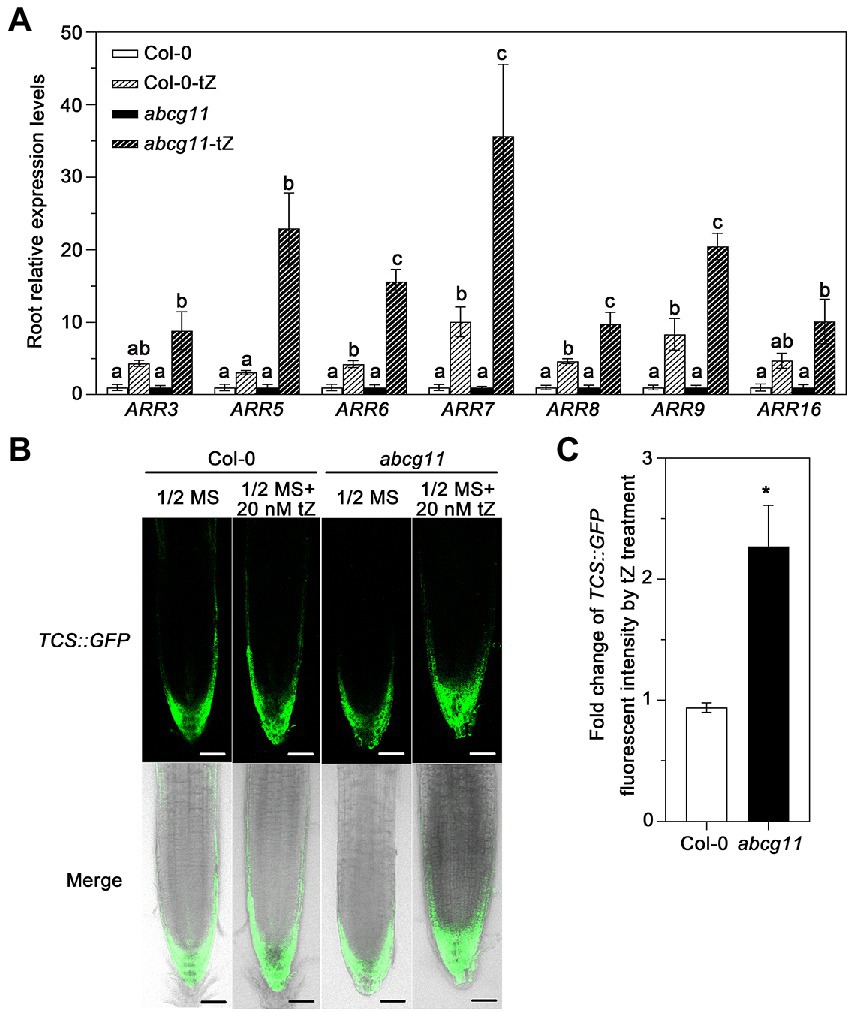
Figure 5. Cytokinin signaling is enhanced in abcg11 seedlings upon tZ treatment. (A) Relative expression levels of ARRs in Col-0 and abcg11 roots, as determined by RT-qPCR. Seedlings were grown vertically on half-strength MS medium with or without 20 nM trans-zeatin (tZ) plate for 12 days under long-day conditions (16 h light/8 h dark). TUBULIN8 served as an internal control; values in Col-0 and abcg11 under control conditions were set to 1. Data are shown as means ± SE (n = 4). The different letters indicate the significant difference in each gene expression levels determined by one-way ANOVA (p < 0.05). (B) Fluorescence intensity of TCS::GFP in the roots of Col-0 and abcg11. Scale bars, 50 mm. (C) Fold-change in TCS::GFP fluorescence intensity induced by tZ treatment in the root tips of WT or abcg11 transgenic seedlings expressing TCS::GFP. Fluorescence intensity was determined using ImageJ. Data are shown as means ± SE from two independent experiments (total 10 biological replicates). Asterisks represent significant differences by Student’s t-test: *p < 0.05.
abcg11 and abcg14 mutants exhibit a similar decrease in root-to-shoot transfer of cytokinin signals
To test whether ABCG11 affects the long-distance transport of cytokinins from the root to the shoot as does ABCG14, we treated abcg11 and abcg14 roots with exogenous cytokinins and determined the relative transcript levels of type-A ARR genes in the shoot. After a 1-h treatment with 1 μM tZ, the expression levels of all ARR genes tested rose in WT shoots over untreated control seedlings. However, the same treatment failed to induce the expression of ARR3, ARR7, ARR8, ARR9, and ARR16 in the shoots of the abcg11 and abcg14 mutants (Figures 6A,B). While ARR5 and ARR6 expression levels increased in the shoots of abcg11 upon the same tZ treatment, the magnitude of the response was weaker than in WT shoots (Figure 6A).
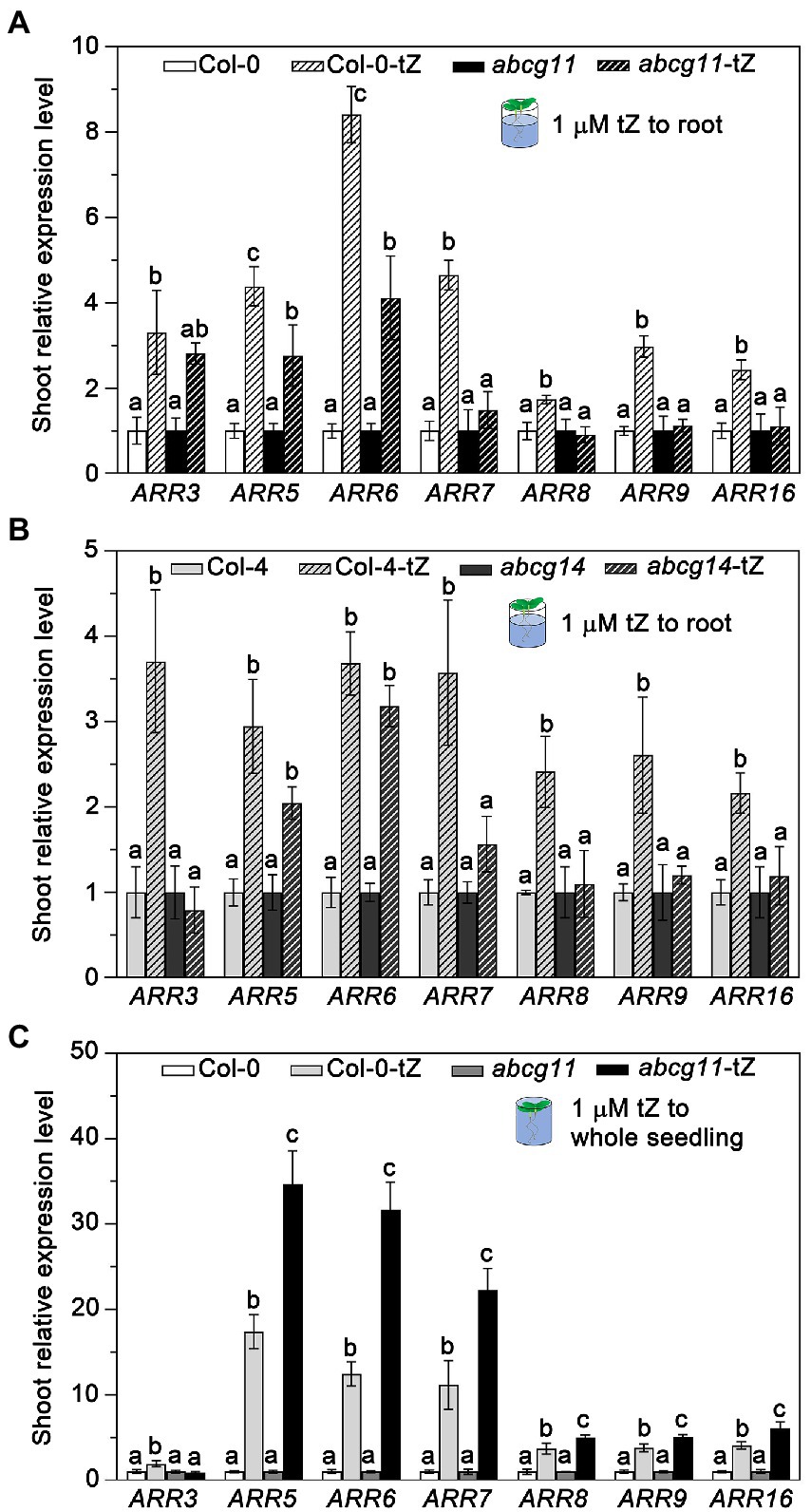
Figure 6. The abcg11 and abcg14 mutants exhibit lower root-to-shoot transfer of cytokinin signaling. (A,B) Relative expression levels of type-A ARRs in the shoots of Col-0 and abcg11 (A), and Col-4 and abcg14 (B). Only the roots of 12-d-old seedlings were soaked in half-strength MS medium or half-strength MS medium containing 1 μM trans-zeatin (tZ) for 1 h. The shoots were harvested for analysis of ARR expression levels. (C) Relative expression levels of ARRs of Col-0 and abcg11 after tZ treatment of whole seedlings. The 12-d-old seedlings were submerged in 1 μM tZ for 30 min. TUBULIN8 served as an internal control; values in Col-0 and abcg11 under control conditions were set to 1. Data are shown as means ± SE (n = 4). Different letters in (A), (B), and (C) indicate significant differences in gene expression levels, as determined by one-way ANOVA (p < 0.05).
To test whether the reduced ARR gene expression response in abcg11 shoots was due to developmental problems in the shoot, we exposed entire seedlings to 1 μM tZ and measured ARR expression levels in shoots. After 1 h of submergence, abcg11 shoots induced the expression of most ARR genes to higher levels than those of WT shoots (Figure 6C), indicating that abcg11 shoots can perceive and respond to cytokinin signals.
To distinguish between a specific defect in cytokinin transport from the root to the shoot or a general shoot developmental defect leading to an indirect effect on phytohormone movement, we examined the root-to-shoot transfer signal of the other phytohormone, ABA. Accordingly, we incubated WT and abcg11 roots with 100 μM ABA for 1 h and measured the relative transcript levels of ABA-induced genes in the shoots. We selected 100 μM ABA for the analysis of short-term gene expression responses as a commonly used ABA concentration (Anderson et al., 2004; Li et al., 2016). Importantly, ABA-induced genes appeared to be expressed to largely similar levels in WT and abcg11 shoots (Supplementary Figure S1). These results suggested that the root-to-shoot transfer of cytokinins is specifically disrupted in abcg11, as is the case in abcg14.
We also directly assayed cytokinin translocation from the root to the shoot of WT (Col-0), abcg11, and abcg14 plants. To this end, we specifically exposed roots to 3H-labeled tZ or tritiated water (T2O) for 1 h and measured the resulting radioactivity in the shoots. When incubated with 3H-tZ, we detected less radioactivity in the shoots of the abcg11 (~69% of WT) and abcg14 (~27% of WT) mutants than in the WT (Figure 7A). We observed no differences in T2O radioactivity between Col-0 and abcg14, while abcg11 was characterized only 20% T2O radioactivity relative to the WT (Figure 7B), indicating that water transpiration is defective in abcg11. As we were unable to devise conditions that would bring transpiration rates in abcg11 close to those of the WT, the possibility remains that the drop in cytokinin translocation from the root to the shoot is an indirect consequence of reduced transpiration.
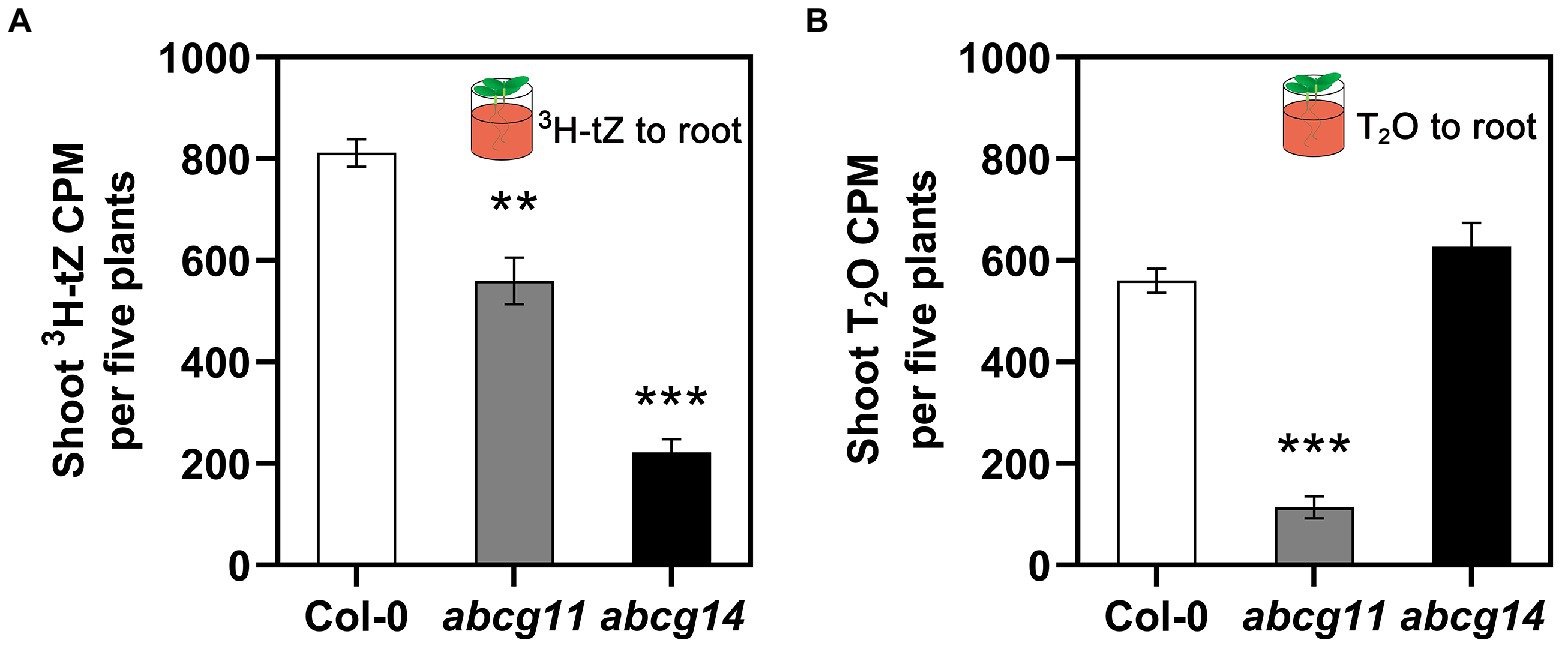
Figure 7. The root-to-shoot transfer of [3H]tZ and T2O. Only the roots of 12-day-old seedlings were treated with 50 nM 3H-tZ or 0.5 mCi/ml T2O for 1 h, and then the shoots were harvested for the assay of the transferred 3H-tZ or T2O. The error bars represent the standard deviation (SE; n = 6–8). The asterisks represent the significant differences between mutants and wild type by Student’s t-test: **p < 0.01, ***p < 0.001.
The abcg14 abcg11 double knockout shows root phenotypes similar to those of abcg11
To further test the functional relationship between ABCG11 and ABCG14, we attempted to generate the abcg14 abcg11 double mutant. As abcg11 is sterile, we collected seeds from abcg14 abcg11/+ plants and used them for growth analyses. We visually differentiated putative abcg14 abcg11 seedlings from putative abcg14 abcg11/+ seedlings based on their very different growth characteristics (Figure 8A) and confirmed the genotype of individual plants by PCR (Figure 8D), as performed earlier for the selection of abcg11 homozygous seedlings (Figure 4B). The abcg14 abcg11 shoots exhibited the stem and leaf organ fusion phenotypes similar to abcg11, and were as small as those from abcg14 (Figures 8F,G). When grown on MGRL medium under short-day conditions, the root length of abcg14 abcg11 homozygous seedlings was similar to that of the abcg11 single mutant, but longer than that of abcg14 abcg11/+ or abcg14 single mutant seedlings (Figures 8A–E). These results suggested that abcg14 abcg11 double knockout does not exacerbate the root developmental phenotypes of the abcg11 single mutant. We also checked the response of abcg14 abcg11 to exogenous cytokinins by root elongation assays. However, the primary root growth of abcg14 abcg11 seedlings was insensitive to exogenous cytokinin treatment, similar to that observed for abcg14 and abcg11 (Figures 9A,B), but in contrast to their corresponding WTs (Figures 9A,B). These results indicated that the abcg14 abcg11 double mutant does not have additional root phenotypes compared to abcg11.
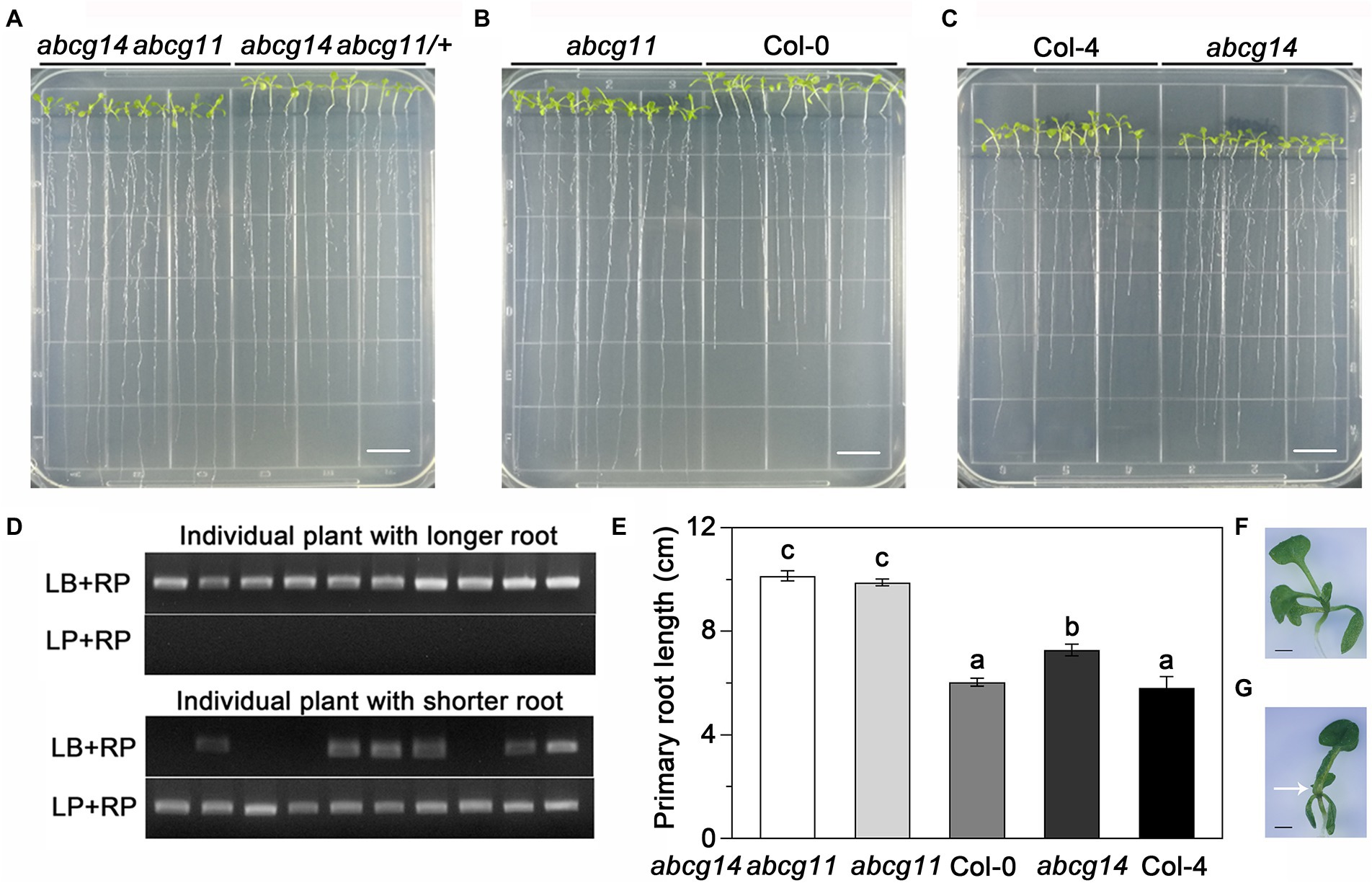
Figure 8. The abcg14 abcg11 double knockout mutant exhibits a similar root length phenotype as the abcg11 single mutant. (A–C) Photographs of seedlings grown vertically on MGRL medium for 12 d under short-day conditions (8 h light/16 h dark). Scale bars, 1 cm. Note that seedlings in (A) were rearranged according to their primary root length prior to the photograph. (D) Genotyping results of abcg14 abcg11/+ and abcg14 abcg11 seedlings shown in (A). (E) Primary root length of the seedlings shown in (A–C). Data are shown as means ± SE (n = 30). Different letters represent significant differences, as determined by one-way ANOVA (p < 0.05). (F,G) Photographs of abcg14 (F) and abcg14 abcg11 (G) seedlings. The arrow points to a site of organ fusion. Scale bars, 1.0 mm.
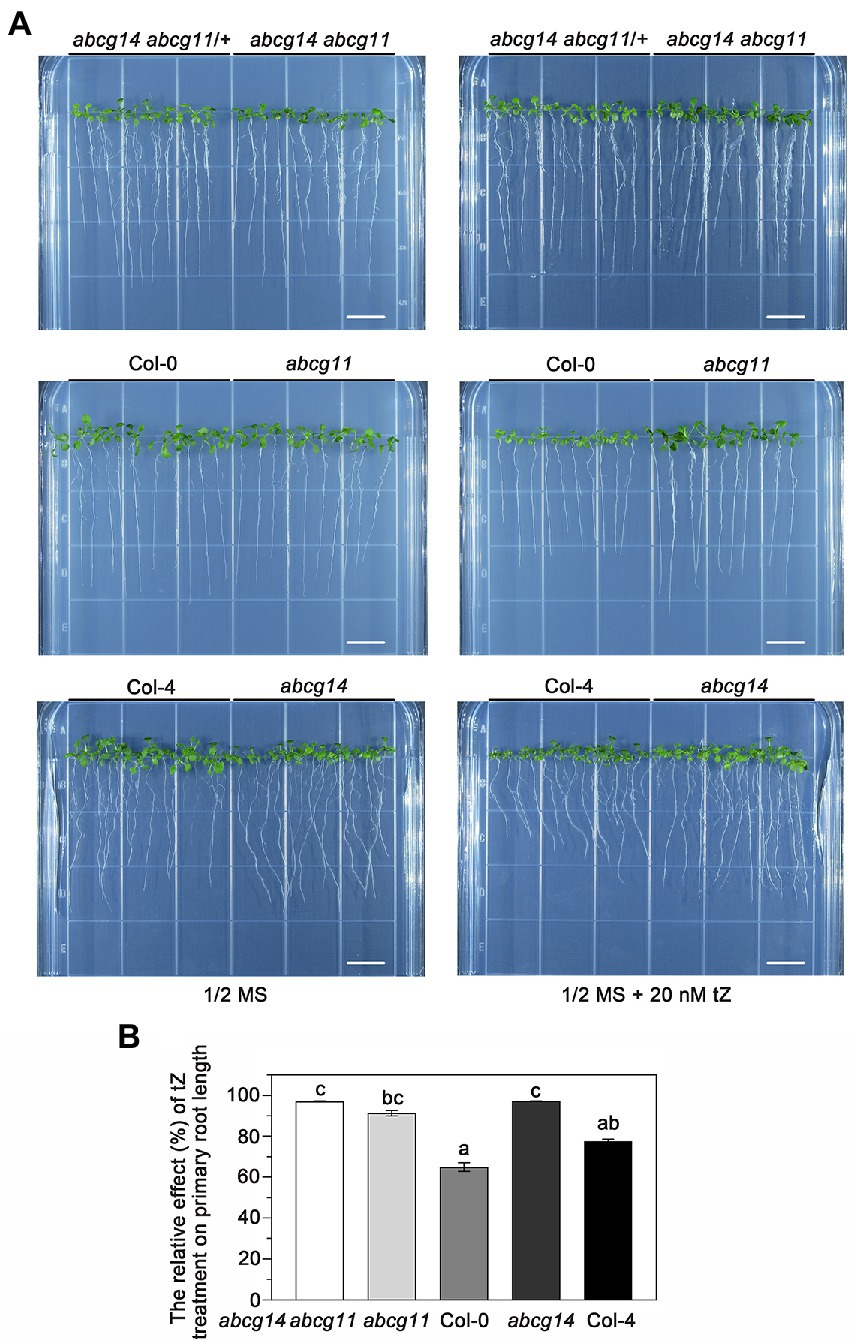
Figure 9. The abcg14 abcg11 double knockout mutant is similar to abcg11 and abcg14 single mutants in root growth in response to cytokinins. (A) Seedlings grown with or without 20 nM trans-zeatin (tZ) treatment for 12 days under long-day conditions (16 h light/8 h dark). The photographs are representatives of four independent experiments. Scale bars, 1 cm. (B) Relative effect of tZ treatment on primary root growth. The relative effect was calculated as primary root length under tZ treatment normalized by that of the control treatment. Data are shown as means ± SE from four independent experiments. Different letters represent significant differences, as determined by one-way ANOVA (p < 0.05).
Discussion
ABCG11 has previously been established as an efflux transporter critical for the formation of surface coating layers such as wax and cutin (Bird et al., 2007; Luo et al., 2007; Panikashvili et al., 2007, 2010; Ukitsu et al., 2007). Since abcg11 knockout plants exhibit very dramatic developmental defects and sterility, and because ABCG11 forms dimers with multiple partner ABCGs (McFarlane et al., 2010; Le Hir et al., 2013), we suspected that it might also participate in phytohormone transport to regulate plant development. We presented here four lines of evidence, demonstrating that ABCG11 is involved in cytokinin responses. First, the expression of many cytokinin-induced genes was different in abcg11 compared to the WT (Figures 2A,B). Second, abcg11 accumulated more cytokinins than the WT (Figure 3). Third, the growth of abcg11 mutant roots was insensitive to exogenous cytokinins (Figures 4D,E, 1E,F). Fourth, the strength of the cytokinin signal was stronger in abcg11 roots than in WT roots upon treatment with exogenous cytokinins (Figure 5). Together, these data support the idea that ABCG11 is involved in the regulation of cytokinin-mediated development.
Despite the results shown here, we did not pinpoint how ABCG11 modulates cytokinin-mediated development. ABCG11 might transport cytokinins to modulate their concentration and distribution in the root. This possibility is supported by three sets of evidences. First, abcg11 shares many phenotypes with abcg14 (Figure 4); ABCG14 is important for root-to-shoot cytokinin transport (Ko et al., 2014; Zhang et al., 2014) and cytokinin redistribution in shoots (Zhao et al., 2021). The similar phenotypes of abcg11 and abcg14 suggest that ABCG11 might collaborate with ABCG14 in transporting cytokinins. Second, the lower induction of type-A ARR expression in abcg11 shoots upon root exposure to cytokinins established that the mutant has a reduced root-to-shoot translocation of cytokinin signaling, as does abcg14 (Figure 6). Third, ABCG11 formed a heterodimer with ABCG14 (Le Hir et al., 2013). If ABCG11 is indeed involved in cytokinin transport, it exists as two distinct ABCG11-containing protein complexes (ABCG11 homodimers and ABCG11/ABCG14 heterodimers) and joins ABCG14 homodimers, which might also transport cytokinin. However, homodimers of either ABCG11 or ABCG14 alone are unlikely to be sufficient for normal cytokinin transport to the root, as the single mutants abcg11 (with functional ABCG14) and abcg14 (with functional ABCG11) exhibited altered responses to cytokinins in roots (Figures 4D,E) and showed altered root-to-shoot translocation of cytokinin signaling (Figure 6). The two homodimers may therefore cooperate for full normal cytokinin transport from roots to shoots, or this role is reserved for the ABCG11/ABCG14 heterodimer. In addition, it is possible that the heterodimer of ABCG11 and ABCG14 may have stronger transport activity of cytokinin, while homodimers have lower activity for cytokinin transportation. Notably, the abcg14 abcg11 double mutant did not exhibit any additive root phenotypes relative to the abcg11 knockout but produced longer roots than the abcg14 single knockout (Figure 8). We suspect that this might reflect the involvement of ABCG11 in diverse functions, whereas ABCG14 is more specific. It would be interesting to further study the cytokinin transport activity of ABCG11 and ABCG14 by structure analysis and protein transport assay.
Importantly, the measured cytokinin contents did not fully support a function for ABCG11 in cytokinin transport, as cytokinin levels in both the shoot and root were higher in the abcg11 mutant than in the WT (Figure 3). We speculate that the role of ABCG11 in the long-distance transport of cytokinin is more complex than that of ABCG14, including both direct effects as a component of cytokinin transporter and indirect effects via transport of its other substrates, such as the cutin/wax components. We attempted cytokinin transport assays in abcg11 and abcg14 seedlings and by expressing ABCG11 and/or ABCG14 in yeast (Saccharomyces cerevisiae), but did not reach a clear conclusion due to many technical problems. Further technological advances will be required to reliably track the routes of cytokinin transport in roots and the involvement of ABCG11 in this process.
An alternative possibility for ABCG11 in cytokinin transport would rely on the transport of very-long-chain fatty acids (VLCFAs). ABCG11 has been shown to be involved in transporting components of wax, cutin, and suberin, including VLCFAs (Luo et al., 2007; Panikashvili et al., 2007, 2010). Multiple VLCFA derivatives are essential components of the wax and cutin layer (Kunst and Samuels, 2003). VLCFAs were shown to alter the development of the shoot apical meristem by suppressing cytokinin biosynthesis (Nobusawa et al., 2013). VLCFAs in epidermal cells inhibit cytokinin biosynthesis in the vasculature, while a decrease in VLCFA contents raised the expression of the cytokinin biosynthesis gene ADENYLATE ISOPENTENYLTRANS FERASE3 (IPT3; Nobusawa et al., 2013). If a similar mechanism exists in the root, a defect in the export of VLCFAs in abcg11 roots may be responsible for the observed change of cytokinin signaling in abcg11 roots. This possibility needs to be tested empirically.
Finally, we should consider the possibility that the severe developmental defects in abcg11 might have indirectly contributed to its abnormal cytokinin responses or to affecting cytokinin concentrations via feedback control mechanisms. The lower water transpiration rate seen in abcg11 (Figure 7) was in line with this possibility, but the normal transfer of ABA signaling from roots to shoots in the abcg11 mutant (Supplementary Figure S1) disagreed with this hypothesis. Additional studies are necessary to dissect the many functions of ABCG11 and to determine the direct and indirect effects resulting from its loss of function in transport and development.
It should be noted that the root phenotypes of abcg11 and abcg14 mutants differ depending on the growth conditions (Figure 4). Indeed, we used a specific growth medium and photoperiod conditions to observe the phenotypes described here. Such diverse root responses indicate that plants have highly flexible mechanisms to employ cytokinins and ABC proteins to optimize root growth and adapt to their environment, which we do not fully understand. The de-coupling between cytokinin signaling and the physiological responses of abcg11 roots also reflected the complexity of cytokinin signaling; the mutant roots were less sensitive to exogenous cytokinins (Figure 4), but cytokinin signaling was higher at the root apical meristem (Figure 5). A similar case of de-coupling was reported in plants defective in another cytokinin transporter. A knockdown in PURINE PERMEASE14 (PUP14), encoding a cytokinin importer from the apoplast to the cytosol, has shorter primary roots and fewer lateral roots, which are typical phenotypes associated with cytokinin accumulation (Zürcher et al., 2016). However, pup14 knockdown plants did not exhibit altered expression of TCSn::GFP in roots (Zürcher et al., 2016). There is therefore precedent for a defect in cytokinin transport activity that de-couples the intensity of cytokinin signaling markers, especially that of TCSn::GFP, from visible plant phenotypes. We suspect that the dual localization of cytokinin receptors to the endoplasmic reticulum and at the plasma membrane (Kubiasová et al., 2020) and the different activities between extracellular cytokinin pools and cytoplasmic cytokinin pools (Romanov and Schmülling, 2021) might contribute to the de-coupling, but this remains a speculation.
In conclusion, our results indicate that ABCG11 plays an important role in cytokinin-mediated root development in Arabidopsis, either directly as a cytokinin transporter or indirectly by transporting molecules that modulate cytokinin biosynthesis or transport. We favor the former, as ABCG11 binds with ABCG14, which is a well-known cytokinin transporter (Le Hir et al., 2013). Together with previous reports, our results suggest that ABCG11 may be involved in the transport of diverse substrates, by forming distinct dimers with diverse half-size ABCG proteins. Our findings also reveal the importance of cytokinin transport in normal root development and how much is yet to be learned about the complex mechanism of cytokinin movement and function in the root.
Data availability statement
The original contributions presented in the study are included in the article/Supplementary material, further inquiries can be directed to the corresponding author.
Author contributions
QY and YL conceived the research. QY and JZ conducted the experiments. MK, YT, TU, and TK conducted the cytokinin measurements and isotope transport. QY, JZ, TK, HS, and YL wrote the manuscript. All authors contributed to the article and approved the submitted version.
Funding
This research was supported by a National Research Foundation of Korea (NRF) grant (2021R1A2B5B03001711) awarded to YL, TK, and HS were supported by Grants-in-Aid from the Ministry of Education, Culture, Sports, Science and Technology, Japan (17H06473).
Acknowledgments
We thank Bruno Müller for the kind gift of the TCS::GFP vector.
Conflict of interest
The authors declare that the research was conducted in the absence of any commercial or financial relationships that could be construed as a potential conflict of interest.
Publisher’s note
All claims expressed in this article are solely those of the authors and do not necessarily represent those of their affiliated organizations, or those of the publisher, the editors and the reviewers. Any product that may be evaluated in this article, or claim that may be made by its manufacturer, is not guaranteed or endorsed by the publisher.
Supplementary material
The Supplementary Material for this article can be found online at: https://www.frontiersin.org/articles/10.3389/fpls.2022.976267/full#supplementary-material
Footnotes
References
Anderson, J. P., Badruzsaufari, E., Schenk, P. M., Manners, J. M., Desmond, O. J., Ehlert, C., et al. (2004). Antagonistic interaction between abscisic acid and jasmonate-ethylene signaling pathways modulates defense gene expression and disease resistance in Arabidopsis. Plant Cell 16, 3460–3479. doi: 10.1105/tpc.104.025833
Argyros, R. D., Mathews, D. E., Chiang, Y.-H., Palmer, C. M., Thibault, D. M., Etheridge, N., et al. (2008). Type B response regulators of Arabidopsis play key roles in cytokinin signaling and plant development. Plant Cell 20, 2102–2116. doi: 10.1105/tpc.108.059584
Bird, D., Beisson, F., Brigham, A., Shin, J., Greer, S., Jetter, R., et al. (2007). Characterization of Arabidopsis ABCG11/WBC11, an ATP binding cassette (ABC) transporter that is required for cuticular lipid secretion. Plant J. 52, 485–498. doi: 10.1111/j.1365-313x.2007.03252.x
Bishopp, A., Lehesranta, S., Vatén, A., Help, H., El-Showk, S., Scheres, B., et al. (2011). Phloem-transported cytokinin regulates polar auxin transport and maintains vascular pattern in the root meristem. Curr. Biol. 21, 927–932. doi: 10.1016/j.cub.2011.04.049
Do, T. H., Martinoia, E., Lee, Y., and Hwang, J.-U. (2021). 2021 update on ATP-binding cassette (ABC) transporters: how they meet the needs of plants. Plant Physiol. 187, 1876–1892. doi: 10.1093/plphys/kiab193
Fujiwara, T., Hirai, M. Y., Chino, M., Komeda, Y., and Naito, S. (1992). Effects of sulfur nutrition on expression of the soybean seed storage protein genes in transgenic Petunia. Plant Physiol. 99, 263–268. doi: 10.1104/pp.99.1.263
Hirose, N., Takei, K., Kuroha, T., Kamada-Nobusada, T., Hayashi, H., and Sakakibara, H. (2008). Regulation of cytokinin biosynthesis, compartmentalization and translocation. J. Exp. Bot. 59, 75–83. doi: 10.1093/jxb/erm157
Janečková, H., Husičková, A., Ferretti, U., Prčina, M., Pilařová, E., Plačková, L., et al. (2018). The interplay between cytokinins and light during senescence in detached Arabidopsis leaves. Plant Cell Environ. 41, 1870–1885. doi: 10.1111/pce.13329
Ko, D., Kang, J., Kiba, T., Park, J., Kojima, M., Do, J., et al. (2014). Arabidopsis ABCG14 is essential for the root-to-shoot translocation of cytokinin. Proc. Natl. Acad. Sci. U. S. A. 111, 7150–7155. doi: 10.1073/pnas.1321519111
Kojima, M., Kamada-Nobusada, T., Komatsu, H., Takei, K., Kuroha, T., Mizutani, M., et al. (2009). Highly sensitive and high-throughput analysis of plant hormones using MS-probe modification and liquid chromatography-tandem mass spectrometry: an application for hormone profiling in Oryza sativa. Plant Cell Physiol. 50, 1201–1214. doi: 10.1093/pcp/pcp057
Kubiasová, K., Montesinos, J. C., Šamajová, O., Nisler, J., Mik, V., Semerádová, H., et al. (2020). Cytokinin fluoroprobe reveals multiple sites of cytokinin perception at plasma membrane and endoplasmic reticulum. Nat. Commun. 11, 4285. doi: 10.1038/s41467-020-17949-0
Kunst, L., and Samuels, A. L. (2003). Biosynthesis and secretion of plant cuticular wax. Prog. Lipid Res. 42, 51–80. doi: 10.1016/s0163-7827(02)00045-0
Le Hir, R., Sorin, C., Chakraborti, D., Moritz, T., Schaller, H., Tellier, F., et al. (2013). ABCG9, ABCG11 and ABCG14 ABC transporters are required for vascular development in Arabidopsis. Plant J. 76, 811–824. doi: 10.1111/tpj.12334
Lee, E. J., Kim, K. Y., Zhang, J., Yamaoka, Y., Gao, P., Kim, H., et al. (2020). Arabidopsis seedling establishment under waterlogging requires ABCG5-mediated formation of a dense cuticle layer. New Phytol. 229, 156–172. doi: 10.1111/nph.16816
Li, X., Li, X., Li, M., Yan, Y., Liu, X., and Li, L. (2016). Dual function of NAC072 in ABF3-mediated ABA-responsive gene regulation in Arabidopsis. Front. Plant Sci. 7:1075. doi: 10.3389/fpls.2016.01075
Luo, B., Xue, X.-Y., Hu, W.-L., Wang, L.-J., and Chen, X.-Y. (2007). An ABC transporter gene of Arabidopsis thaliana, AtWBC11, is involved in cuticle development and prevention of organ fusion. Plant Cell Physiol. 48, 1790–1802. doi: 10.1093/pcp/pcm152
McFarlane, H. E., Shin, J. J. H., Bird, D. A., and Samuels, A. L. (2010). Arabidopsis ABCG transporters, which are required for export of diverse cuticular lipids, dimerize in different combinations. Plant Cell 22, 3066–3075. doi: 10.1105/tpc.110.077974
Mellor, N., Adibi, M., El-Showk, S., De Rybel, B., King, J., Mähönen, A. P., et al. (2017). Theoretical approaches to understanding root vascular patterning: a consensus between recent models. J. Exp. Bot. 68, 5–16. doi: 10.1093/jxb/erw410
Moubayidin, L., Perilli, S., Dello Ioio, R., Di Mambro, R., Costantino, P., and Sabatini, S. (2010). The rate of cell differentiation controls the Arabidopsis root meristem growth phase. Curr. Biol. 20, 1138–1143. doi: 10.1016/j.cub.2010.05.035
Nobusawa, T., Okushima, Y., Nagata, N., Kojima, M., Sakakibara, H., and Umeda, M. (2013). Synthesis of very-long-chain fatty acids in the epidermis controls plant organ growth by restricting cell proliferation. PLoS Biol. 11:e1001531. doi: 10.1371/journal.pbio.1001531
Panikashvili, D., Savaldi-Goldstein, S., Mandel, T., Yifhar, T., Franke, R. B., René, H., et al. (2007). The Arabidopsis DESPERADO/AtWBC11 transporter is required for cutin and wax secretion. Plant Physiol. 145, 1345–1360. doi: 10.1104/pp.107.105676
Panikashvili, D., Shi, J. X., Bocobza, S., Franke, R. B., Schreiber, L., and Aharoni, A. (2010). The Arabidopsis DSO/ABCG11 transporter affects cutin metabolism in reproductive organs and suberin in roots. Mol. Plant 3, 563–575. doi: 10.1093/mp/ssp103
Rashotte, A. M., Carson, S. D. B., To, J. P. C., and Kieber, J. J. (2003). Expression profiling of cytokinin action in Arabidopsis. Plant Physiol. 132, 1998–2011. doi: 10.1104/pp.103.021436
Romanov, G. A., and Schmülling, T. (2021). Opening doors for cytokinin trafficking at the ER membrane. Trends Plant Sci. 26, 305–308. doi: 10.1016/j.tplants.2021.02.006
Rong, X. F., Sang, Y. L., Wang, L., Meng, W. J., Zou, C. H., Dong, Y. X., et al. (2018). Type-B ARRs control carpel regeneration through mediating AGAMOUS expression in Arabidopsis. Plant Cell Physiol. 59, 761–769. doi: 10.1093/pcp/pcx187
Takei, K., Dekishima, Y., Eguchi, T., Yamaya, T., and Sakakibara, H. (2003). A new method for enzymatic preparation of isopentenyladenine-type and trans-zeatin-type cytokinins with radioisotope-labeling. J. Plant Res. 116, 259–263. doi: 10.1007/s10265-003-0098-2
Tokunaga, H., Kojima, M., Kuroha, T., Ishida, T., Sugimoto, K., Kiba, T., et al. (2012). Arabidopsis lonely guy (LOG) multiple mutants reveal a central role of the LOG-dependent pathway in cytokinin activation. Plant J. 69, 355–365. doi: 10.1111/j.1365-313x.2011.04795.x
Ukitsu, H., Kuromori, T., Toyooka, K., Goto, Y., Matsuoka, K., Sakuradani, E., et al. (2007). Cytological and biochemical analysis of COF1, an Arabidopsis mutant of an ABC transporter gene. Plant Cell Physiol. 48, 1524–1533. doi: 10.1093/pcp/pcm139
Vatén, A., Soyars, C. L., Tarr, P. T., Nimchuk, Z. L., and Bergmann, D. C. (2018). Modulation of asymmetric division diversity through cytokinin and SPEECHLESS regulatory interactions in the Arabidopsis stomatal lineage. Dev. Cell 47, 53.e5–66.e5. doi: 10.1016/j.devcel.2018.08.007
Verrier, P. J., Bird, D., Burla, B., Dassa, E., Forestier, C., Geisler, M., et al. (2008). Plant ABC proteins – a unified nomenclature and updated inventory. Trends Plant Sci. 13, 151–159. doi: 10.1016/j.tplants.2008.02.001
Wybouw, B., and De Rybel, B. (2019). Cytokinin – a developing story. Trends Plant Sci. 24, 177–185. doi: 10.1016/j.tplants.2018.10.012
Yuan, L., Liu, Z., Song, X., Johnson, C., Yu, X., and Sundaresan, V. (2016). The CKI1 histidine kinase specifies the female gametic precursor of the endosperm. Dev. Cell 37, 34–46. doi: 10.1016/j.devcel.2016.03.009
Zhang, K., Novak, O., Wei, Z., Gou, M., Zhang, X., Yu, Y., et al. (2014). Arabidopsis ABCG14 protein controls the acropetal translocation of root-synthesized cytokinins. Nat. Commun. 5, 3274–3212. doi: 10.1038/ncomms4274
Zhao, J., Ding, B., Zhu, E., Deng, X., Zhang, M., Zhang, P., et al. (2021). Phloem unloading via the apoplastic pathway is essential for shoot distribution of root-synthesized cytokinins. Plant Physiol. 186, 2111–2123. doi: 10.1093/plphys/kiab188
Zürcher, E., Liu, J., di Donato, M., Geisler, M., and Müller, B. (2016). Plant development regulated by cytokinin sinks. Science 353, 1027–1030. doi: 10.1126/science.aaf7254
Keywords: ABC transporters, ABCG11, ABCG14, heterodimer, phytohormone, root, signaling, transport
Citation: Yang Q, Zhang J, Kojima M, Takebayashi Y, Uragami T, Kiba T, Sakakibara H and Lee Y (2022) ABCG11 modulates cytokinin responses in Arabidopsis thaliana. Front. Plant Sci. 13:976267. doi: 10.3389/fpls.2022.976267
Edited by:
Ming Luo, South China Botanical Garden (CAS), ChinaReviewed by:
Caiji Gao, South China Normal University, ChinaYanru Hu, Xishuangbanna Tropical Botanical Garden (CAS), China
Copyright © 2022 Yang, Zhang, Kojima, Takebayashi, Uragami, Kiba, Sakakibara and Lee. This is an open-access article distributed under the terms of the Creative Commons Attribution License (CC BY). The use, distribution or reproduction in other forums is permitted, provided the original author(s) and the copyright owner(s) are credited and that the original publication in this journal is cited, in accordance with accepted academic practice. No use, distribution or reproduction is permitted which does not comply with these terms.
*Correspondence: Youngsook Lee, ylee@postech.ac.kr
†These authors have contributed equally to this work and share first authorship