- 1Division of Genetics, ICAR-Indian Agricultural Research Institute, New Delhi, India
- 2Division of Entomology, ICAR-Indian Agricultural Research Institute, New Delhi, India
Hybrids have been successfully used to improve crop productivity, including Brassicas. Nucleo-cytoplasmic interactions have been reported to influence the expression of resistance to insect pests in several crops. We studied the effects of Cytoplasmic Male Sterility (CMS) in Brassica juncea carrying alien cytoplasms and their respective maintainer (B) lines on the antibiosis mechanism of resistance, involving development, survival, reproduction potential and population build-up of mustard aphid, Lipaphis erysimi, and the levels of defense phyto-chemicals. Present findings revealed that the numbers of aphids/plant, aphid multiplication rate and aphid resistance index were lower on ber CMS under natural, mori CMS under artificial infestation conditions, and juncea under both the test conditions indicating nucleo-cytoplasmic interactions for aphid reaction. Across cytoplasms, nymphal, reproductive and total developmental periods were significantly longer on SEJ 8, NPJ 161, LES 39, and NPJ 93, while the reproductive potential and survival were lower on PM 30, Pusa Tarak and SEJ 8 as compared to other nuclear backgrounds. Across nuclear backgrounds, nymphal, reproductive and total developmental periods were significantly longer on ber CMS, while reproductive potential and survival were lower on ber and mori CMS as compared to other cytoplasms. Total glucosinolates were significantly greater and myrosinase lower in Pusa Agrani, SEJ 8, LES 39, PM 30, NPJ 112, and Pusa Tarak as compared to the other nuclear backgrounds. Furthermore, total glucosinolates were significantly greater and myrosinase lower in ber CMS and juncea as compared to other cytoplasms. The studies suggest that CMS as well as cytoplasmic and nuclear gene interactions regulate the expression of defense compounds such as glucosinolates and determine the expression of resistance/susceptibility to L. erysimi. These findings shall help in identification of suitable L. erysimi tolerant nucleo-cytoplasmic combinations for their deployment in B. juncea hybrid breeding program.
Introduction
Rapeseed-mustard (Brassica spp.) is the third most important oilseed crop after soybean and oil palm (Palial et al., 2022), and contributes 27.8% to the Indian oilseed industry (Kumrawat and Yadav, 2018). Among the oilseed brassicas, Brassica juncea (L.) Czern & Coss. occupies > 90% acreage in the country (Singh, 2014). The average productivity of mustard in India (1,511 kg/ha) is much lower than the world average (1,980 kg/ha), which is about three-fourths of the world average (Soybean Processors Association of India [SOPA], 2020). Further, the production and productivity of rapeseed-mustard are highly variable due to various biotic and abiotic stresses experienced across crop-growing agro-ecologies of India. Among the biotic stresses, mustard aphid, Lipaphis erysimi (Kaltenbach) is the major yield reducing factor in rapeseed-mustard, causing up to 90% yield loss under severe infestation conditions (Ahuja et al., 2010).
To meet the rising global food demand, hybrids have been successfully used to improve the productivity across the crops, including Brassicas. Cytoplasmic-genetic male sterility has been one of the most efficient hybrid seed production systems deployed for commercial exploitation of heterosis phenomenon in a wide array of field crops (Dhillon et al., 2008). The cytoplasmic male sterility and fertility restorer (CMS-FR) system, comprising of male sterile (A), maintainer (B), and fertility restorer (R) lines, has been extensively used both in cross- and self-pollinated crop species for commercial hybrid seed production (Melonek et al., 2021).
Several male-sterility inducing cytoplasmic sources have been identified among wild or related species of Brassica and nuclear genome of cultivated Brassicas has been transferred through intergeneric or interspecific hybridization into these sterility-inducing cytoplasms such as Raphanus sativus (ogu; Kirti et al., 1995), B. tournefortii (tour; Banga et al., 1995; Pahwa et al., 2004), Moricandia arvensis (mori; Kirti et al., 1998; Prakash et al., 1998), Diplotaxis catholica (cath; Pathania et al., 2003), Enarthrocarpus lyratus (lyr; Banga et al., 2003; Janeja et al., 2003), Diplotaxis erucoides (eru; Malik et al., 1999; Bhat et al., 2006), and Diplotaxis berthautii (ber; Malik et al., 1999; Bhat et al., 2008). Among these, ogu and mori CMS systems are widely used for development of commercial B. juncea hybrids in India (Kaur et al., 2004). Furthermore, the common fertility restorer (Rf) gene from mori restores fertility in mori, eru, and ber cytoplasms (Bhat et al., 2005, 2006, 2008), and offers an opportunity to diversify cytosterility sources without the need of searching for an appropriate restorer gene (Vinu et al., 2017). Good number of test hybrids with eru, ber, and mori cytoplasms have been developed at the Indian Council of Agricultural Research-Indian Agricultural Research Institute (ICAR-IARI), New Delhi (Chamola et al., 2013), and have been evaluated in multilocation trials by the All India Coordinated Research Project on Rapeseed-Mustard (Anonymous, 2021).
Large-scale cultivation of crop hybrids carrying one or a few CMS sources may lead to decrease in genetic diversity, and make them vulnerable to various pests over time and adversely impact sustainable crop production (Dalmacio et al., 1995). Therefore, diversification of cytoplasmic as well as nuclear genome of parental lines is important for sustainable hybrid breeding programs in rapeseed-mustard (Vinu et al., 2017). Furthermore, information available on different CMS systems in cereals revealed that the nuclear background of CMS, cytoplasmic factors, and the interaction effects of cytoplasm and nuclear genomes influence the expression of resistance to insect pests and pathogens (Sharma et al., 2004; Dhillon et al., 2006b; Dhillon et al., 2008). However, information on the effects of sterility inducing cytoplasms on the expression of resistance/susceptibility reaction in rapeseed-mustard against L. erysimi is still missing. Thus, present studies were carried out to decipher the effects of different CMS systems (A lines) and the respective maintainers (B lines) of B. juncea on the development, survival, reproduction potential and population build-up of L. erysimi, and on the levels of major plant defense biochemicals like glucosinolates and myrosinase. For this purpose, a set of isonuclear alloplasmic lines in different B. juncea backgrounds was developed at ICAR-IARI following backcross breeding. The relative performance of these isonuclear alloplasmic lines, carrying cytoplasms from wild species, against L. erysimi shall help in identification of suitable cytoplasmic sources and their deployment in B. juncea hybrid breeding program.
Materials and methods
Plant material
The experimental material consisted of 11 diverse B. juncea maintainer (B) lines and 33 CMS (A) lines carrying three different sterility-inducing cytoplasms viz., D. berthautii (ber), D. erucoides (eru) and M. arvensis (mori) in each maintainer background. Thus, making a set of 33 CMS lines and their 11 B. juncea nuclear donors. The CMS lines and their respective maintainers were sown in 4 row plots of 5 m row length keeping 45 cm row to row and 15 cm plant to plant distance during rabi (winter) 2020–2021 season in the experimental fields of ICAR-IARI, New Delhi. All recommended agronomic practices, except insecticide use, were followed to raise the crop.
Evaluation of A and B lines of Brassica juncea for aphid multiplication rate and resistance index
Natural infestation
To observe the natural infestation of aphids in the A and B lines, five plants were randomly selected from each plot and labeled, thus making five replications in a randomized block design. The observations were recorded on the number of aphids, aphid population index (API on a rating scale of 1–5) and aphid damage index (ADI on a rating scale of 1–5) of all the test genotypes as described by Dhillon et al. (2018). The A and B lines were monitored daily to track mustard aphid infestation, and economic threshold level (ETL; 15 aphids on top 10 cm twig in 10% of plants). After 15 days when L. erysimi reached ETL, the number of aphids on the main branch (upper 10 cm) and aphid resistance index was computed (API + ADI/2) from the labeled plants of each A and B lines as described by Dhillon et al. (2018).
Artificial infestation
Five randomly selected plants of each A and B lines were tagged for artificial infection, thus making five replications in a completely randomized design. At the bud initiation stage, around 20 aphids (nymphs and adults) were inoculated with pieces of infested Brassica twigs pinned on the third shoot from the top of the plant. After aphid inoculation in the buds, the branch was covered with the specially designed twig cage as described by Dhillon et al. (2018). At 15 days after L. erysimi inoculation, observations were recorded on total number of aphids on the caged shoot of each tagged plant. The aphid multiplication rate was obtained by dividing the total number of aphids by 20 for each test plant, and A and B lines separately.
Developmental period, reproductive potential and survival of Lipaphis erysimi on A and B lines of Brassica juncea
The biological performance studies of L. erysimi on different plant parts, viz, leaves, buds, and siliquae of the A and B lines carrying B. juncea nuclear genome, were carried out under laboratory conditions at 17 ± 3o C temperature, 60–70% relative humidity and 12L:12D photoperiod. The L. erysimi were collected from the field and reared on mustard leaves in glass Petri dishes (10 cm h × 2 cm dia.) under laboratory conditions. The moistened filter paper was placed inside the Petri dish. The test plant parts viz., leaf discs, buds and siliquae of each test line were placed on the moist blotting paper to keep them turgid. The newly produced nymphs obtained from the laboratory-reared aphids were transferred to the test plant parts (one nymph each) with the help of a fine moist camel hairbrush. There were 15 replications, for each line and plant part, in a completely randomized design. The respective plant parts were changed daily till the completion of the studies. The observations were recorded on total nymphal period, reproductive period, total developmental period, reproductive potential and survival of L. erysimi. The observations were recorded at 12 h intervals. The total nymphal period was calculated as the period (in hours) between the start of the first instar to the end of the fourth instar stage. The reproductive period of an individual aphid was recorded as the duration (in hours) between it giving birth to the first and the last nymph. The total developmental period was calculated as the time period (in hours) between the birth of the first instar till its death as an adult. The reproductive potential, i.e., number of nymphs produced by each female during its reproductive period was recorded, and expressed as nymphs/female. The observations were recorded till the death of an adult. The total nymphs produced by each female were counted and per cent survival of nymphs was calculated till 48 h, and expressed as per cent survival/female.
Estimation of glucosinolates and myrosinase in different plant parts of A and B lines of Brassica juncea
Glucosinolates
For glucosinolate quantification, fresh leaves, buds and siliquae samples from the test genotypes were collected and oven dried at 70°C, then 300 ml of 80% methanol was added to 0.3 g of dried sample, and incubated at 70°C for 5 min. After cooling, 2 mL double distilled water was added to the mixture, and the samples were centrifuged at 15,000 rpm for 15 min, and after that, the supernatants were collected for further analysis. After adding 100 μl of supernatant and 4 ml of 0.2 Mm sodium tetrachloropalladate, the mixture was incubated at room temperature for 1 h. Test samples (200 μl) were dispensed into each well of a 96-well microtiter plate, and absorbance was recorded at a wavelength of 425 nm in an ELISA Reader (SPECTRA max Plus 384, United States). Total glucosinolate content was calculated by using the OD value of each sample in the formula [y = 1.40 + 118.86 × A425] given by Mawlong et al. (2017), and the values thus obtained were expressed as μ moles/g of plant tissue.
Myrosinase
For myrosinase estimation, 50 mg tissue samples of leaves, buds or siliquae were homogenized in 1 ml distilled water and centrifuged at 13,000 rpm for 5 min at 4°C, and the supernatant was collected. The supernatant (15 μl) was mixed with 1,470 μl of 80 mM NaCl (pH 6.5) and 15 μl of 20 mM sinigrin and incubated at 37°C (Piekarska et al., 2013). The myrosinase activity was determined based on measurements of decomposition of sinigrin by following the decrease in absorbance of the reaction mixture at 230 nm using a spectrophotometer (Thermo Fisher Scientific, United States). The total final volume of the reaction mixture was 1.5 mL and the myrosinase activity was calculated using the formula:
Where, “VA” denotes the volume of the reaction mixture, “Vsample” denotes the volume of investigated sample, “A0” denotes the initial absorbance, “At” denotes the absorbance after reaction time, “t” denotes the reaction time corresponding to the initial reaction rate characterized by a linear change in absorption (min), and ‘E’ denotes the molar extinction coefficient, i.e., 7,500 for SIN, 8,870 for GTL (1/mol cm). Final myrosinase activity was expressed as mol of hydrolyzed GLS per min recalculated per 1 g of enzyme prep (U/g prep).
Statistical analysis
The data on population build-up, multiplication rate and resistance index under artificial and natural infestation conditions, L. erysimi biological parameters and the biochemical parameters in different nuclear backgrounds and cytoplasms were subjected to factorial analysis using statistical software SAS® version 9.2. The data on L. erysimi biological and plant biochemical parameters on individual plant parts as well as averaged across plant parts were also subjected to factorial analysis. The significance of differences were tested by F-test, and the treatment means and their interactions were compared using LSD values at P = 0.05.
Results
Population build-up, aphid multiplication rate and aphid resistance index on A and B lines of Brassica juncea
Natural infestation
The numbers of aphids/plant and aphid resistance index on the test cytoplasms and nuclear backgrounds of B. juncea genotypes varied from 159 to 713 and 3.0 to 5.0 under natural infestation conditions (Table 1). There were significant differences in the number of aphids/plant and aphid resistance index among the test B. juncea nuclear background, cytoplasms, and the nuclear background × cytoplasm interactions (Table 1). Across cytoplasms, the numbers of aphids/plant and aphid resistance index were significantly lower on LES 39 and NPJ 93 as compared to other genotypes. Across the nuclear background, the numbers of aphids/plant (Figure 1A) and aphid resistance index (Figure 1B) were significantly lower on ber and juncea as compared to other cytoplasms. Across nuclear background and cytoplasms, the numbers of aphids/plant and aphid resistance index were significantly lower on NPJ 93 in ber and mori, LES 39 in eru, and SEJ 8 in juncea cytoplasmic backgrounds (Table 1).
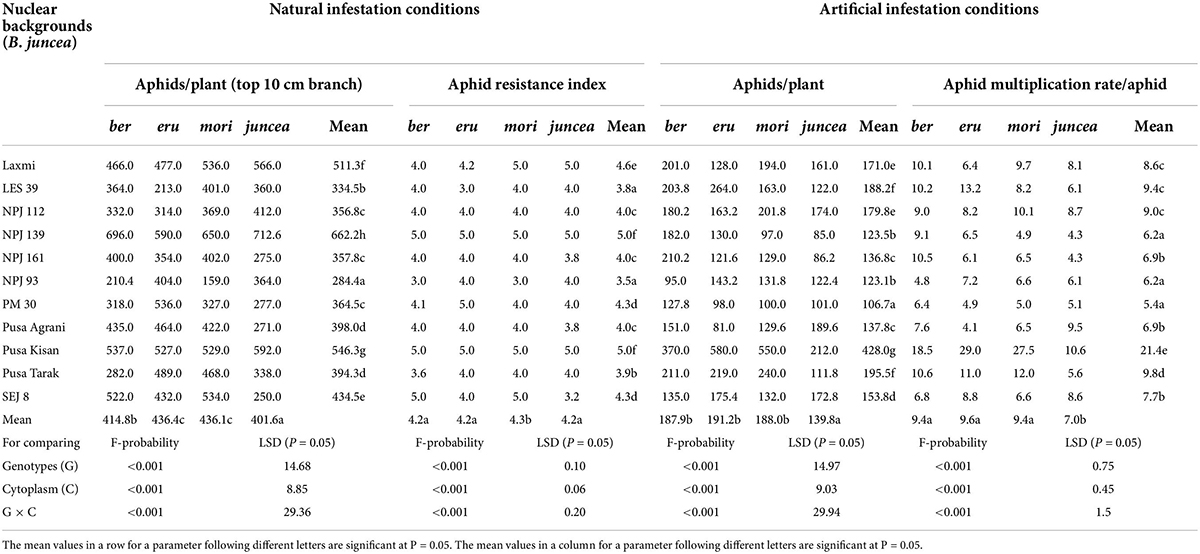
Table 1. Effects of cytoplasms and nuclear backgrounds on aphid resistance index, population build up and multiplication rate of Lipaphis erysimi in auto- and alloplasmic lines of Brassica juncea.
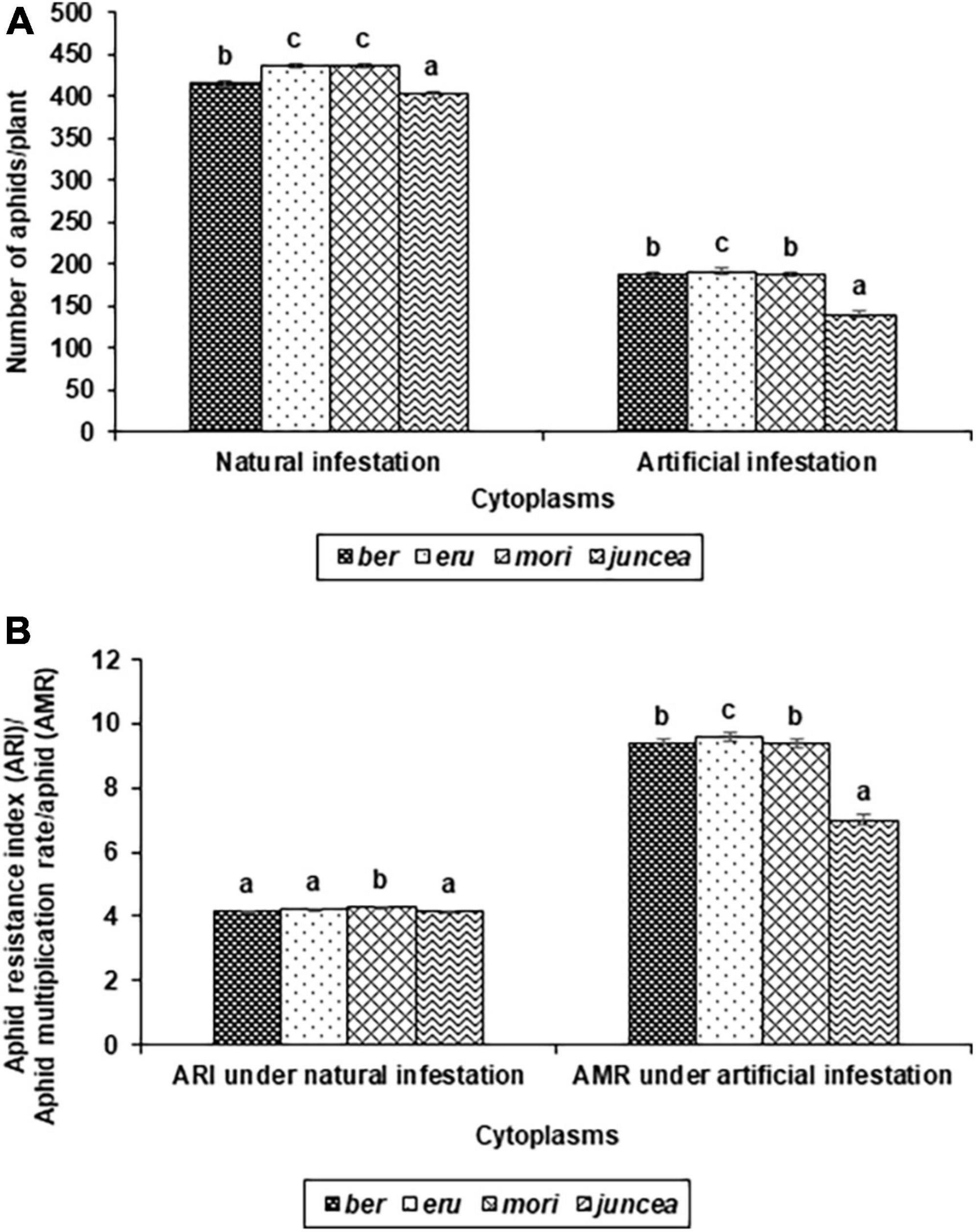
Figure 1. Effects of cytoplasms on population build up (A), and Resistance index and multiplication rate (B) of Lipaphis erysimi under natural and artificial infestation conditions in Brassica juncea.
Artificial infestation
The numbers of aphids/plant and aphid multiplication rate on the tested cytoplasms across nuclear backgrounds of B. juncea varied from 81 to 580 and 4.1 to 29.0 under artificial infestation conditions (Table 1). There were significant differences for the number of aphids/plant and aphid multiplication rate among the test B. juncea nuclear backgrounds, cytoplasms, and the nuclear background × cytoplasm interactions (Table 1). Across cytoplasms, the numbers of aphids/plant and aphid multiplication rate were significantly lower on PM 30, Pusa Agrani, NPJ 93, NPJ 139, NPJ 161, and SEJ 8 as compared to other genotypes. Across nuclear backgrounds, the numbers of aphids/plant (Figure 1A) and aphid multiplication rate (Figure 1B) were significantly lower on juncea as compared to other (ber, eru and mori) cytoplasms. Among male sterile cytoplasms, it was comparatively lower on ber and mori as compared to eru cytoplasm (Figures 1A,B). Across nuclear backgrounds and cytoplasms, the numbers of aphids/plant and aphid multiplication rate were significantly lower on NPJ 93 in ber, Pusa Agrani in eru, NPJ 139 in mori, and NPJ 161 and NPJ 139 in juncea cytoplasmic backgrounds (Table 1).
Developmental period, reproductive potential and survival of Lipaphis erysimi on A and B lines of Brassica juncea
Nymphal period
The total nymphal period of L. erysimi varied from 75.2 to 104.2 h on the leaves, 83.5 to 112.0 h on buds and 83.0 to 114.6 h on the siliquae, and there were significant differences among the test B. juncea nuclear backgrounds, across cytoplasms, and the nuclear background × cytoplasm interactions (Supplementary Table 1). Across plant parts, the total nymphal period of L. erysimi significantly varied on test nuclear backgrounds (F = 61.93; df = 10,90; P < 0.001), across cytoplasms (F = 12.55; df = 3,90; P < 0.001), and for the nuclear background × cytoplasm interactions (F = 12.28; df = 30,270; P < 0.001). Across cytoplasms, the total nymphal period was significantly longer on SEJ 8, NPJ 161, LES 39, and NPJ 93 as compared to other nuclear backgrounds (Table 2). Across nuclear backgrounds, the total nymphal period was significantly longer on ber as compared to other cytoplasms (Figure 2). Across genotypes and cytoplasms, the total nymphal period was significantly longer on SEJ 8 in ber and juncea, and on LES 39 in eru and mori cytoplasms (Table 2).
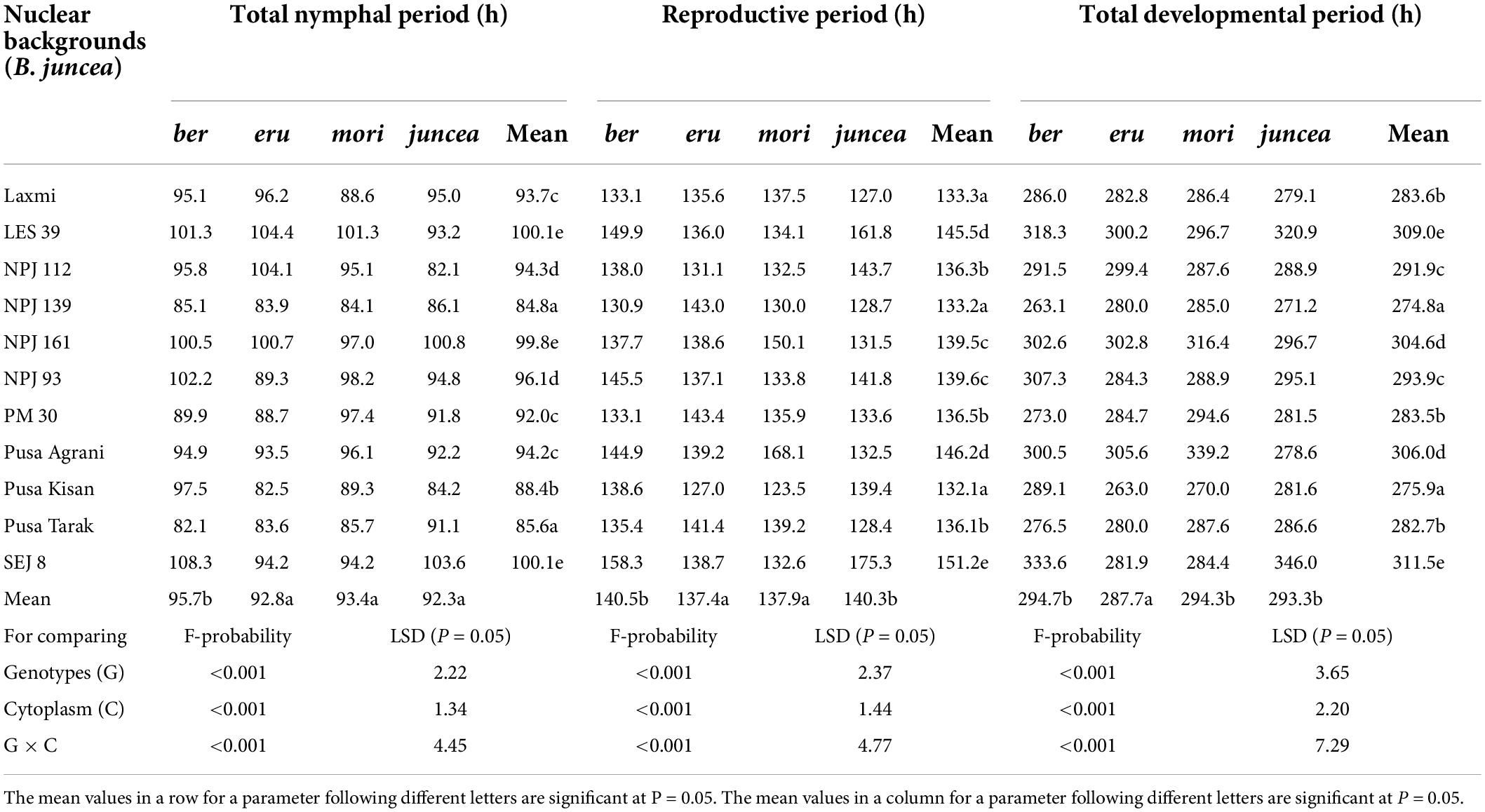
Table 2. Effects of cytoplasms and nuclear backgrounds on developmental and reproductive periods of Lipaphis erysimi in auto- and alloplasmic lines of Brassica juncea.
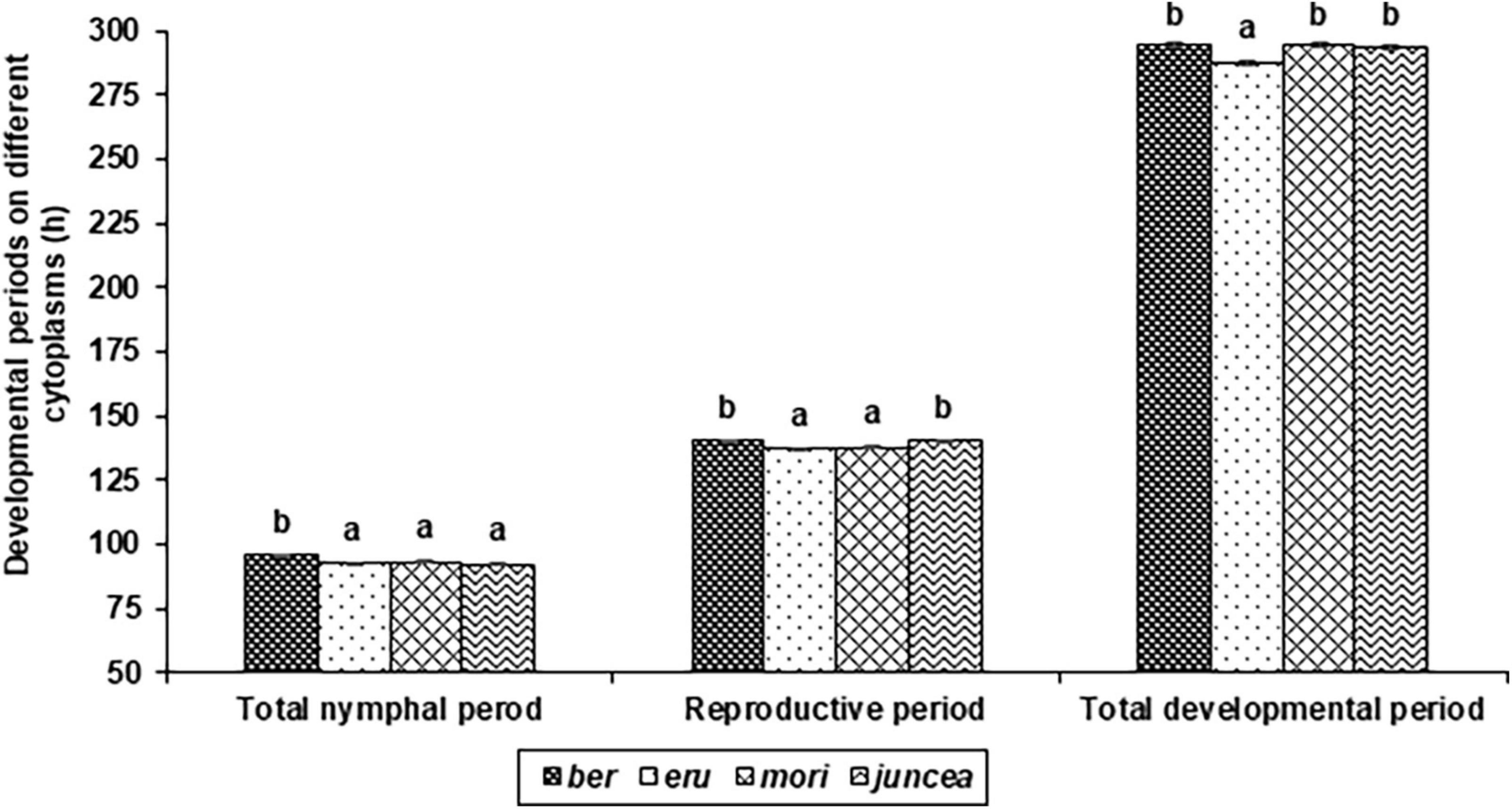
Figure 2. Effects of cytoplasms on developmental and reproductive periods of Lipaphis erysimi in Brassica juncea.
Reproductive period
The reproductive period of L. erysimi varied from 117.6 to 170.5 h on the leaves, 120.6 to174.5 h on buds and 131.4 to 180.9 h on the siliquae, and varied significantly on the test B. juncea nuclear backgrounds, across cytoplasms, and the nuclear backgrounds × cytoplasm interactions (Supplementary Table 2). Across plant parts, the reproductive period of L. erysimi significantly varied on the test genotypes (F = 60.04; df = 10,90; P < 0.001), across cytoplasms (F = 16.33; df = 3,90; P < 0.001), and for the nuclear background × cytoplasm interactions (F = 41.92; df = 30,270; P < 0.001). Across cytoplasms, the reproductive period was significantly longer on SEJ 8, NPJ 161, LES 39, NPJ 93, and Pusa Agrani as compared to other nuclear backgrounds (Table 2). Across nuclear backgrounds, the reproductive period was significantly longer on ber and juncea as compared to other cytoplasms (Figure 2). Across nuclear backgrounds and cytoplasms, the reproductive period was significantly longer on SEJ 8 in ber and juncea, on NPJ 139, PM 30 and Pusa Tarak in eru, and on Pusa Agrani in mori cytoplasmic backgrounds (Table 2).
Total developmental period
The total developmental period of L. erysimi varied from 244.2 to 333.1 h on the leaves, 263.7 to 347.4 h on buds and 280.6 to 363.4 h on the siliquae, and there were significant differences for the B. juncea nuclear backgrounds, across cytoplasms, and the nuclear background × cytoplasm interactions (Supplementary Table 3). Across plant parts, there were significant differences in the total developmental period of L. erysimi on nuclear backgrounds (F = 128.65; df = 10,90; P < 0.001), across cytoplasms (F = 26.54; df = 3,90; P < 0.001), and for the nuclear background × cytoplasm interactions (F = 40.44; df = 30,270; P < 0.001). Across cytoplasms, the total developmental period was significantly longer on SEJ 8, NPJ 161, LES 39, NPJ 93, and Pusa Agrani as compared to other nuclear backgrounds (Table 2). Across nuclear backgrounds, the total developmental period was significantly lower on eru as compared to other cytoplasms (Figure 2). Across nuclear backgrounds and cytoplasms, the total developmental period was significantly greater on SEJ 8 and LES 39 in ber and juncea, and on Pusa Agrani and NPJ 161 in eru and mori cytoplasmic backgrounds (Table 2).
Reproductive potential
The reproductive potential of L. erysimi varied from 43.9 to 74.0 nymphs/female on the leaves, 44.6 to 76.6 nymphs/female on buds and 37.2 to 66.1 nymphs/female on the siliquae, and varied significantly on the test B. juncea nuclear backgrounds, across cytoplasms, and the nuclear background × cytoplasm interactions (Supplementary Table 4). Across plant parts, there was significant variation in the reproductive potential of L. erysimi on the nuclear backgrounds (F = 59.33; df = 10,90; P < 0.001), across cytoplasms (F = 23.67; df = 3,90; P < 0.001), and for the nuclear background × cytoplasm interactions (F = 24.07; df = 30,270; P < 0.001). Across cytoplasms, the reproductive potential was significantly lower on PM 30, Pusa Tarak, SEJ 8, Pusa Kisan, NPJ 93 and Laxmi as compared to other genotypes (Table 3). Across genotypes, the reproductive potential was significantly lower on ber and mori as compared to other cytoplasms (Figure 3). Across nuclear backgrounds and cytoplasms, the reproductive potential was significantly lower on Pusa Agrani, Pusa Tarak and NPJ 93 in ber, PM 30 in eru and mori, and Pusa Tarak in juncea cytoplasmic backgrounds (Table 3).
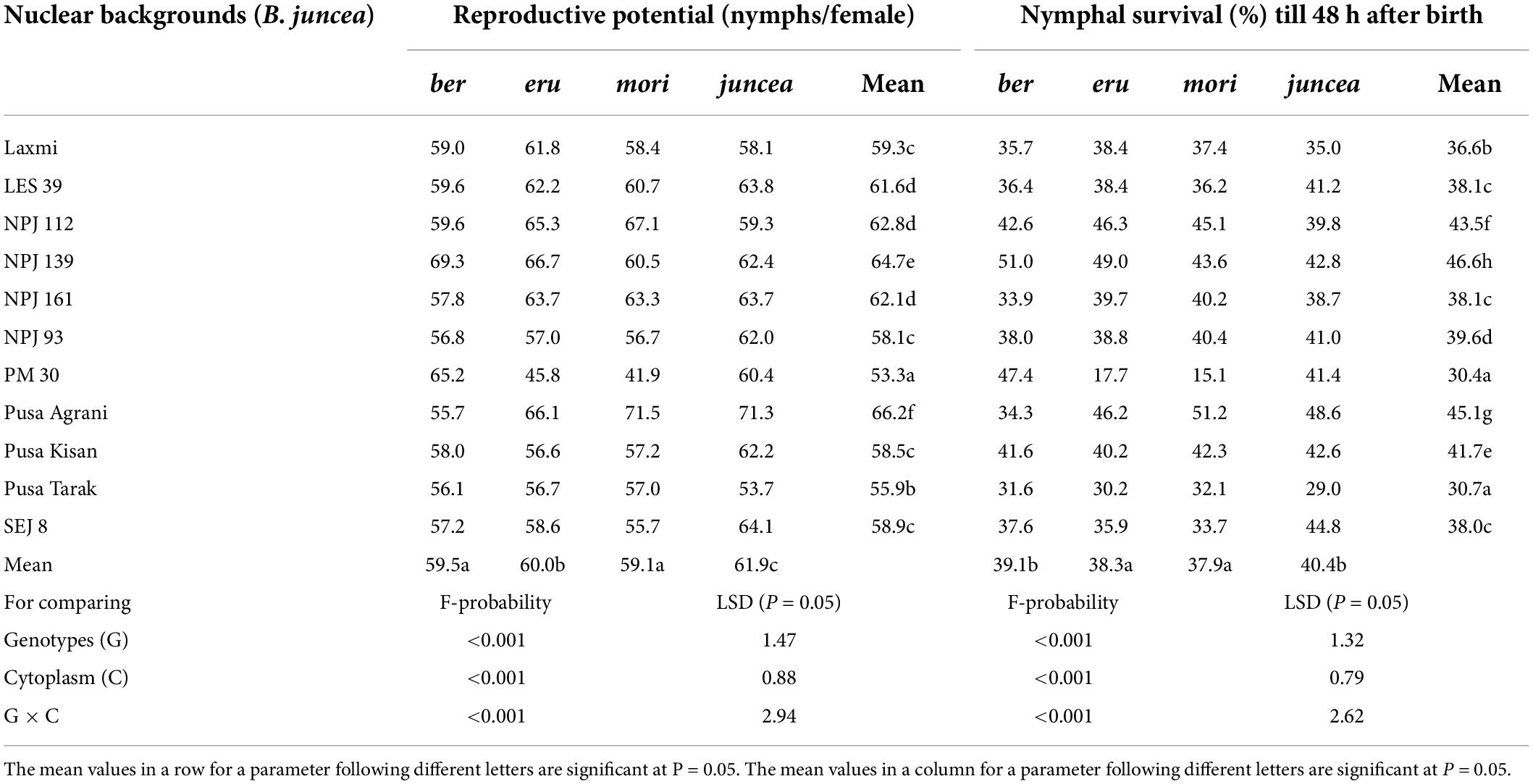
Table 3. Effects of cytoplasms and nuclear backgrounds on reproductive potential and survival of Lipaphis erysimi in auto- and alloplasmic lines of Brassica juncea.
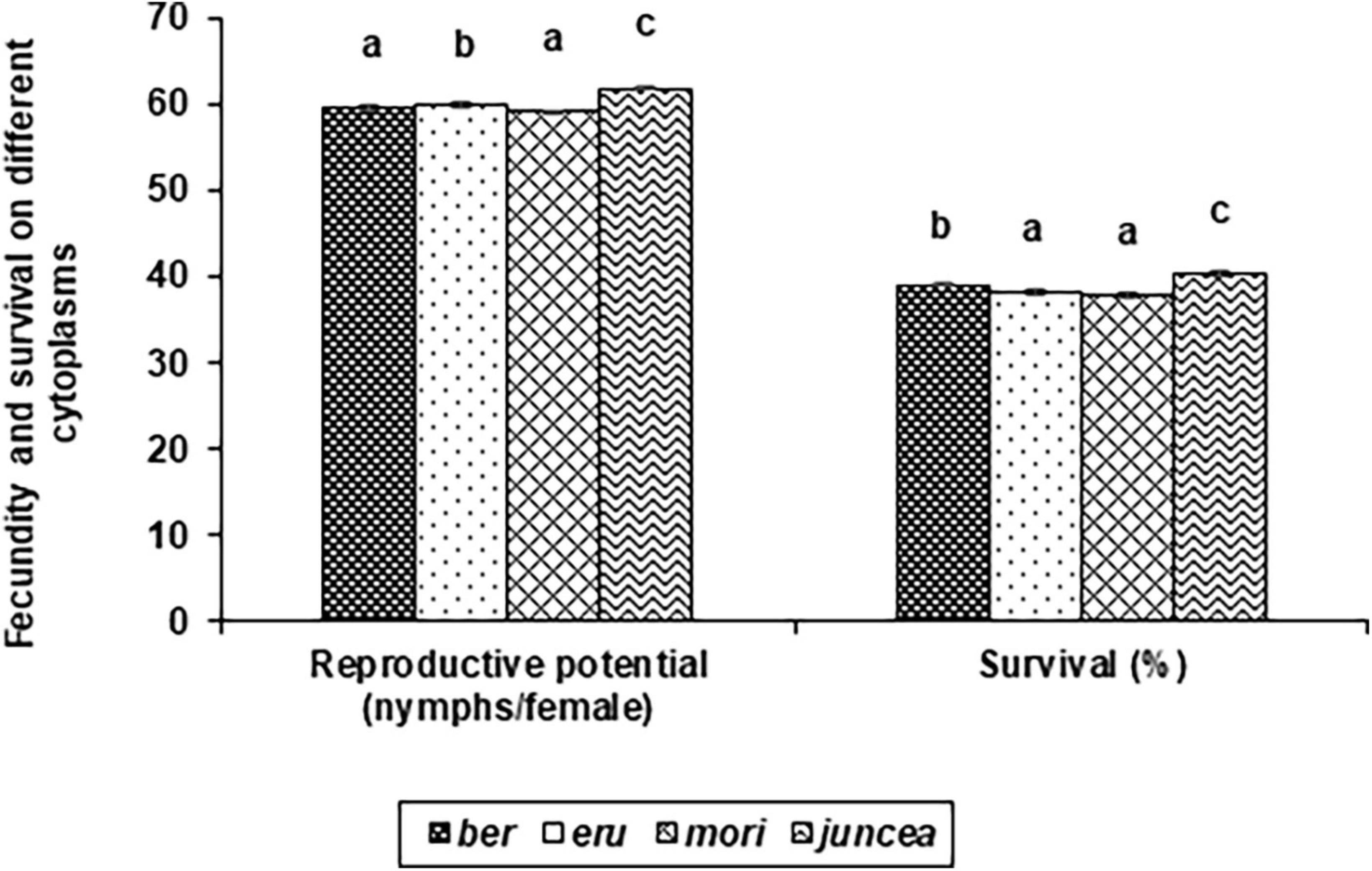
Figure 3. Effects of cytoplasms on reproductive potential and survival Lipaphis erysimi in Brassica juncea.
Survival
The survival of L. erysimi varied from 17.9 to 60.0% on the leaves, 17.5 to 60.0% on buds and 9.9 to 41.0% on the siliquae, and there were significant differences for the test B. juncea nuclear backgrounds, across cytoplasms, and the nuclear background × cytoplasm interactions (Supplementary Table 5). Across plant parts, there were significant differences in the survival of L. erysimi on test nuclear backgrounds (F = 135.66; df = 10,90; P < 0.001), across cytoplasms (F = 30.47; df = 3,90; P < 0.001), and for the nuclear background × cytoplasm interactions (F = 46.14; df = 30,270; P < 0.001). Across cytoplasms, the L. erysimi survival was significantly lower on PM 30, Pusa Tarak and SEJ 8 as compared to other nuclear backgrounds (Table 3). Across nuclear backgrounds, the L. erysimi survival was significantly lower on ber and mori as compared to other cytoplasms (Figure 3). Across nuclear backgrounds and cytoplasms, the L. erysimi survival was significantly lower on Pusa Tarak in ber and juncea, and PM 30 in eru and mori cytoplasmic backgrounds (Table 3).
Glucosinolates and myrosinase content in different plant parts of A and B lines of Brassica juncea
Glucosinolates
The total glucosinolates varied from 29.5 to 77.5 mg/g in the leaves, 66.8 to 109.6 mg/g in buds and 84.6 to 127.4 mg/g in the siliquae, and significantly varied for the test B. juncea nuclear backgrounds, across cytoplasms, and the nuclear background × cytoplasm interactions (Supplementary Table 6). Across plant parts, the total glucosinolates significantly varied in the test nuclear backgrounds (F = 90.41; df = 10,90; P < 0.001), across cytoplasms (F = 69.59; df = 3,90; P < 0.001), and for the nuclear background × cytoplasm interactions (F = 35.83; df = 30,270; P < 0.001). Across cytoplasms, the total glucosinolates were significantly higher in Pusa Agrani, SEJ 8, LES 39, PM 30, and NPJ 112 as compared to other nuclear backgrounds (Table 4). Across nuclear backgrounds, the total glucosinolates were significantly higher in ber and juncea as compared to other cytoplasms (Figure 4). Across genotypes and cytoplasms, the total glucosinolates were significantly higher in SEJ 8, PM 30 and NPJ 112 in ber, LES 39, Pusa Agrani and NPJ 112 in eru and mori, and NPJ 161 and Pusa Agrani in juncea cytoplasmic backgrounds (Table 4).
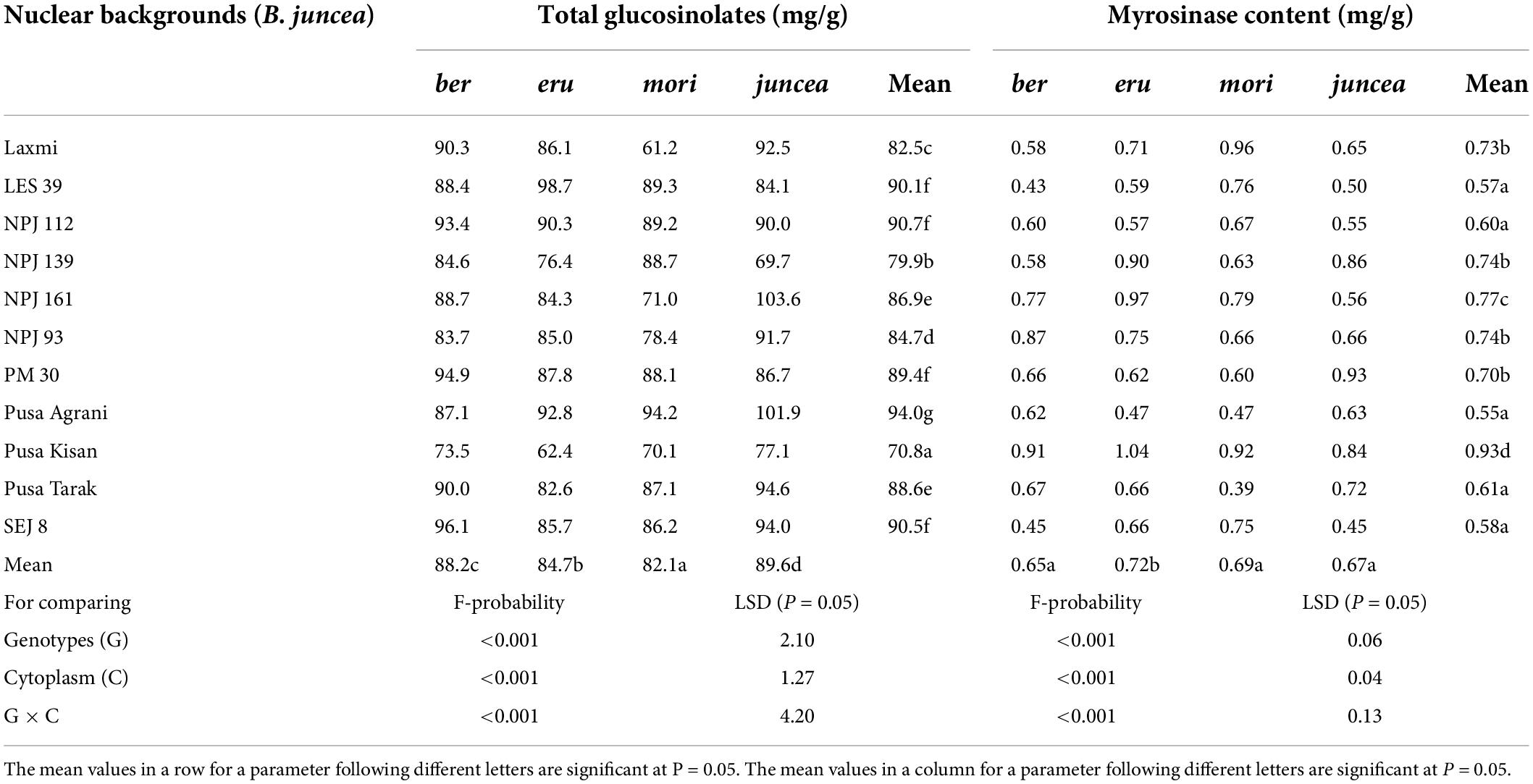
Table 4. Effects of cytoplasms and nuclear backgrounds on total glucosinolate and myrosinase contents in auto- and alloplasmic lines of Brassica juncea.
Myrosinase
The myrosinase content varied from 0.31 to 0.92 mg/g in the leaves, 0.44 to 1.45 mg/g in buds and 0.38 to 0.99 mg/g in the siliquae, and there were significant differences for the B. juncea nuclear backgrounds, across cytoplasms, and the nuclear backgrounds × cytoplasm interactions (Supplementary Table 7). Across plant parts, the myrosinase content significantly varied in the test B. juncea nuclear backgrounds (F = 39.07; df = 10,90; P < 0.001), across cytoplasms (F = 7.36; df = 3,90; P < 0.001), and for the nuclear backgrounds × cytoplasm interactions (F = 10.39; df = 30,270; P < 0.001). Across cytoplasms, the myrosinase content was significantly lower in Pusa Agrani, LES 39, SEJ 8, NPJ 112, and Pusa Tarak as compared to other nuclear backgrounds (Table 4). Across genotypes, the myrosinase content was significantly lower in ber and juncea as compared to other cytoplasms (Figure 4). Across genotypes and cytoplasms, the myrosinase content was significantly lower in LES 39 and SEJ 8 in ber and juncea, Pusa Agrani, LES 39 and NPJ 112 in eru, and Pusa Agrani and Pusa Tarak in mori cytoplasmic backgrounds (Table 4).
Discussion
The parthenogenetic viviparity, high fecundity, and host specificity support L. erysimi to multiply faster on Brassica plants. The interaction between olfactory cues like plant volatiles and visual stimuli including photosynthetic pigments are crucial for host plant finding by the aphids (Hardie, 1980; Dilawari and Atwal, 1989; Samal et al., 2022). Due to non-availability of aphid resistance sources in the primary gene pool of brassicas (Rana, 2005), the only effective and easily available strategy to control this pest is through systemic insecticides (El-Wakeil et al., 2013). The efforts are going on to increase the yield potential and productivity using hybrid technology in B. juncea through different male sterility-inducing cytoplasmic sources derived from the wild brassicas (Chamola et al., 2013). Earlier studies have found that the genetic background of CMS, cytoplasmic factors, and their interaction with nuclear genes influence the morphological, agronomic and physiological traits, and expression of resistance to insect pests and diseases (Dhillon et al., 2008). Present findings revealed that the numbers of aphids/plant, aphid multiplication rate and aphid resistance index were significantly lower on the native cytoplasm of B. juncea as compared to male sterile cytoplasms both under natural and artificial infestation conditions. Earlier studies also reported lower oviposition and damage on the maintainer than the CMS lines of sorghum by Atherigona soccata (Rondani), Stenodiplosis sorghicola (Coquillett), Peregrinus maidis Ashmead and Melanaphis sacchari Zehnter (Sharma et al., 2004; Dhillon et al., 2006b). Among the male sterile cytoplasms, numbers of aphids/plant, aphid multiplication rate and aphid resistance index were lower for ber under natural and for mori under artificial infestation conditions as compared to other cytoplasms, indicating that the ber and mori male sterile cytoplasms have a similar reaction against L. erysimi. Varying levels of damage and antixenosis by A. soccata in sorghum genotypes under different CMS backgrounds have also been reported earlier, being lower in A4G1 and A4M as compared to other male sterile cytoplasms (Dhillon et al., 2005, 2006a). Furthermore, the numbers of aphids/plant, aphid multiplication rate and aphid resistance index were significantly lower on NPJ 93 in ber, on LES 39 and Pusa Agrani in eru, and on NPJ 139, PM 30 and NPJ 93 in mori cytoplasmic backgrounds under different testing conditions. These findings suggest that not only the CMS source but the cytoplasmic and nuclear genome interactions also play an important role in expressing reaction against L. erysimi.
Prolonged developmental periods, and reduced reproduction and survival are important components of the antibiosis mechanism of resistance against L. erysimi in B. juncea (Samal et al., 2021). Earlier studies reported greater deleterious effects of maintainer cytoplasm on the developmental biology of several insects such as A. soccata, S. sorghicola, P. maidis, and M. sacchari (Sharma et al., 1994; Sharma, 2001; Dhillon et al., 2005, 2006a,2008) and diseases (Xu and Song, 1997; Xu et al., 1998) as compared to the male sterile cytoplasms, which could be because of interaction between cytoplasmic and nuclear genes of particular CMS line or incomplete genome recovery of the maintainer into CMS lines. Moreover, as a consequence of natural selection pressure, plant species evolved for resisting insect pest’s establishment and multiplication to improve their fitness and adaptability. It was observed in the present study that across cytoplasms, total nymphal, reproductive and total developmental periods were significantly longer on SEJ 8, NPJ 161, LES 39, and NPJ 93, while reproductive potential and survival were lower on PM 30, Pusa Tarak and SEJ 8 as compared to other nuclear backgrounds. Whereas, total nymphal, reproductive and total developmental periods across nuclear backgrounds were significantly longer on ber, while reproductive potential and survival were lower on ber and mori as compared to other cytoplasms, suggesting that the use of these male-sterile cytoplasms in the development of B. juncea hybrids could be useful for reducing losses caused by L. erysimi in addition to genetic superiority for productivity traits. Earlier studies have also found significant detrimental effects of male sterile cytoplasms like A4M and A4VzM on the development and survival of A. soccata in sorghum (Dhillon et al., 2005, 2006a). Further, the total nymphal, reproductive and total developmental periods were significantly longer on SEJ 8 and reproductive potential and survival lower on Pusa Tarak in ber and juncea cytoplasmic backgrounds as compared to other cytoplasms. The total nymphal period, on the other hand, was significantly higher on LES 39 and total developmental period on Pusa Agrani and NPJ 161 in eru and mori, while the reproductive period was higher on NPJ 139, PM 30 and Pusa Tarak in eru, and Pusa Agrani in mori cytoplasmic backgrounds. The reproductive potential and survival were significantly lower on PM 30 in eru and mori cytoplasmic backgrounds. These findings further reiterate that the CMS as well as cytoplasmic and nuclear gene interactions determine reaction against L. erysimi. Earlier studies also found the influence of cytoplasmic factors on the expression of midge and shoot fly resistance in sorghum because of interaction of cytoplasmic and nuclear genes (Sharma et al., 1996; Dhillon et al., 2006c).
Similar to insect reactions, CMS has also been reported to influence the synthesis and metabolism of certain proteins, amino acids, nucleic acid and carbohydrates resulting in altered pollen development and physiology in several crops (Fukasawa, 1957; Erickson, 1967; Savchenko et al., 1968; Alam and Sandal, 1969; Mian et al., 1974; Chen and Qin, 1989; Shi et al., 1996; Murty et al., 1997). Present studies found that across cytoplasms, the total glucosinolates were significantly greater and myrosinase content lower in Pusa Agrani, SEJ 8, LES 39, PM 30, NPJ 112, and Pusa Tarak as compared to other genotypes. Across nuclear backgrounds, the total glucosinolates were significantly higher and myrosinase content lower in ber and juncea as compared to other cytoplasms. It is already established through earlier studies that the activity of several enzymes like cytochrome oxidase, peroxidase, esterase, ribulose-bisphosphate carboxylase and adenosine triphosphatase is being altered in the anthers of CMS as compared to maintainer lines in several crops (Anonymous, 1977; Watson et al., 1977; Senthil and Manickam, 1995; Murty et al., 1997). Furthermore, it has also been reported that the glucosinolates, a group of isothiocyanates present in the Brassicas play a key role in regulating the infestation, establishment and reproduction of aphids (Fahey et al., 2001; Brader et al., 2006; Kumar and Sangha, 2017). In response to herbivore attack, the enzyme myrosinase comes in contact with its glucosinolate substrates, resulting in the production of bioactive compounds (Andreasson et al., 2001). In the present studies, the total glucosinolates were significantly higher and myrosinase content lower in SEJ 8 in ber and B. juncea, and LES 39 and NPJ 112 in eru and mori cytoplasmic backgrounds; suggesting that not only the cytoplasmic or nuclear genes but their interaction also regulate the expression of glucosinolates and associated enzymes. These findings will be helpful in the identification of suitable L. erysimi tolerant nucleo-cytoplasmic combinations for their deployment in B. juncea hybrid breeding program.
Data availability statement
The original contributions presented in the study are included in the article/Supplementary material, further inquiries can be directed to the corresponding author/s.
Author contributions
NS and MD conceptualized and designed the study. Both authors performed the experimental setup, data collection, analysis, and wrote and approved the final manuscript.
Acknowledgments
We are thankful to S. R. Bhat for providing the cytoplasmic male sterility sources for development of B. juncea A lines and guiding the research. We also thankful to ICAR-Indian Agricultural Research Institute, New Delhi for providing necessary facilities for conducting this study.
Conflict of interest
The authors declare that the research was conducted in the absence of any commercial or financial relationships that could be construed as a potential conflict of interest.
Publisher’s note
All claims expressed in this article are solely those of the authors and do not necessarily represent those of their affiliated organizations, or those of the publisher, the editors and the reviewers. Any product that may be evaluated in this article, or claim that may be made by its manufacturer, is not guaranteed or endorsed by the publisher.
Supplementary material
The Supplementary Material for this article can be found online at: https://www.frontiersin.org/articles/10.3389/fpls.2022.971606/full#supplementary-material
References
Ahuja, I., Rohloff, J., and Bones, A. M. (2010). Defence mechanisms of Brassicaceae: Implications for plant-insect interactions and potential for integrated pest management-A review. Agron. Sustain. Dev. 30, 311–348. doi: 10.1051/agro/2009025
Alam, S., and Sandal, P. C. (1969). Electrophoretic analysis of anther proteins from male fertile and male sterile sudangrass Sorghum vulgare var. sudanensis (Piper). Crop Sci. 9, 157–159. doi: 10.2135/cropsci1969.0011183X000900020013x
Andreasson, E., Jorgensen, L. B., Hoglund, A. S., Rask, L., and Meijer, J. (2001). Different myrosinase and idioblast distribution in Arabidopsis and Brassica napus. Plant Physiol. 127, 1750–1763. doi: 10.1104/pp.010334
Anonymous. (1977). The relationship between male sterility of rice and activities of some enzymes and rate of respiration in pollen. Acta Bot. Sin. 20, 150–153.
Anonymous. (2021). Annual progress report 2021. All india coordinated research project on rapeseed-mustard. Sewar, AK: ICAR-Directorate of Rapeseed-Mustard Research, 321303
Banga, S. S., Banga, S. K., and Sandha, G. S. (1995). Development and characterization of tournefortii CMS system in Brassica napus L. Proceedings of the 9th International Rapeseed Congress. Cambridge, UK: Organising Committee 1, 55–57.
Banga, S. S., Deol, J. S., and Banga, S. K. (2003). Alloplasmic male-sterile Brassica juncea with Enarthrocarpus lyratus cytoplasm and the introgression of gene(s) for fertility restoration from cytoplasm donor species. Theor. Appl. Genet. 106, 1390–1395. doi: 10.1007/s00122-002-1180-1
Bhat, S. R., Kumar, P., and Prakash, S. (2008). An improved cytoplasmic male sterile (Diplotaxis berthautii) Brassica juncea: Identification of restorer and molecular characterization. Euphytica 159, 145–152. doi: 10.1007/s10681-007-9467-6
Bhat, S. R., Prakash, S., Kirti, P. B., Dinesh Kumar, V., and Chopra, V. L. (2005). A unique introgression from Moricandia arvensis confers male fertility upon two different cytoplasmic male-sterile lines of Brassica juncea. Plant Breed. 124, 117–120. doi: 10.1111/j.1439-0523.2005.01054.x
Bhat, S. R., Priya, V., Ashutosh Dwivedi, K. K., and Prakash, S. (2006). Diplotaxis erucoides induced cytoplasmic male sterility in Brassica juncea is rescued by the Moricandia arvensis restorer: Genetic and molecular analyses. Plant Breed. 125, 150–155. doi: 10.1111/j.1439-0523.2006.01184.x
Brader, G., Mikkelsen, M. D., Halkier, B. A., and Palva, E. T. (2006). Altering glucosinolate profiles modulates disease resistance in plants. Plant J. 46, 758–767. doi: 10.1111/j.1365-313X.2006.02743.x
Chamola, R., Balyan, H. S., and Bhat, S. R. (2013). Effect of alien cytoplasm and fertility restorer genes on agronomic and physiological traits of Brassica juncea (L) Czern. Plant Breed. 132, 681–687. doi: 10.1111/pbr.12080
Chen, J. M., and Qin, T. C. (1989). Anther nucleic acid content at various developmental stages of male sterile wheat (Triticum aestivum L.). Jiangsu J. Agric. Sci. 5, 14–19.
Dalmacio, R., Brar, D. S., Ishii, T., Sitch, L. A., Virmani, S. S., and Khush, G. S. (1995). Identification and transfer of a new cytoplasmic male sterility source from Oryza perennis into indica rice (O. sativa). Euphytica 82, 221–225. doi: 10.1007/BF00029564
Dhillon, M. K., Sharma, H. C., Naresh, J. S., Singh, R., and Pampapathy, G. (2006b). Influence of cytoplasmic male sterility on expression of different mechanisms of resistance in sorghum to Atherigona soccata (Diptera: Muscidae). J. Econ. Entomol. 99, 1452–1461. doi: 10.1093/jee/99.4.1452
Dhillon, M. K., Sharma, H. C., Pampapathy, G., and Reddy, B. V. S. (2006a). Cytoplasmic male-sterility affects expression of resistance to shoot bug, Peregrinus maidis, sugarcane Aphid, Melanaphis sacchari, and spotted stem borer, Chilo partellus in sorghum. SAT eJ 2, 66–68.
Dhillon, M. K., Sharma, H. C., Reddy, B. V. S., and Ram Singh Naresh, J. S. (2006c). Inheritance of resistance to sorghum shoot fly. Atherigona soccata. Crop Sci. 46, 1377–1383. doi: 10.2135/cropsci2005.06-0123
Dhillon, M. K., Sharma, H. C., Reddy, B. V. S., Singh, R., Naresh, J. S., and Kai, Z. (2005). Relative susceptibility of different male-sterile cytoplasms in sorghum to shoot fly. Atherigona soccata. Euphytica 144, 275–283. doi: 10.1007/s10681-005-7246-9
Dhillon, M. K., Sharma, H. C., and Smith, C. M. (2008). Implications of cytoplasmic male-sterility systems for development and deployment of pest resistant hybrids in cereals. CAB Rev: Pers. Agric. Veter. Sci. Nutr. Nat. Resour. 3, 1–16. doi: 10.1079/PAVSNNR20083068
Dhillon, M. K., Singh, N., Tanwar, A. K., Yadava, D. K., and Vasudeva, S. (2018). Standardization of screening techniques for resistance to Lipaphis erysimi (Kalt.) in rapeseed-mustard under field conditions. Indian J. Exp. Biol. 56, 674–685.
Dilawari, V. K., and Atwal, A. S. (1989). Response of mustard aphid Lipaphis erysimi (Kalt.) to allylisothiocyanate. J. Insect Sci. 2, 103–108.
El-Wakeil, N., Gaafar, N., Sallam, A., and Volkmar, C. (2013). “Side effects of insecticides on natural enemies and possibility of their integration in plant protection strategies,” in Insecticides: Development of safer and more effective technologies, ed. S. Trdan (Ljubljana: IntechOpen), doi: 10.5772/54199
Erickson, J. R. (1967). Biochemical investigations of cytoplasmic male sterility in spring wheat (Triticum aestivum L.). Agron. Abstr 8–9.
Fahey, J. W., Zalcmann, A. P., and Talalay, P. (2001). The chemical diversity and distribution of glucosinolates and isothiocyanates among plants. Phytochem. 56, 50–51. doi: 10.1016/S0031-9422(00)00316-2
Fukasawa, H. (1957). Studies on restoration and substitution of nucleus (genome) in Aegilotricum I. Appearance of male sterile durum in substitution crosses. Cytologia 18, 167–175. doi: 10.1508/cytologia.18.167
Hardie, J. (1980). Reproductive, morphological and behavioural affinities between the alate gynopara and virginopara of the aphid, Aphis fabae. Physiol. Entomol. 5, 385–396. doi: 10.1111/j.1365-3032.1980.tb00247.x
Janeja, H. S., Banga, S. K., Bhasker, P. B., and Banga, S. S. (2003). Alloplasmic male sterile Brassica napus with Enarthrocarpus lyratus cytoplasm: Introgression and molecular mapping of an E. lyratus chromosome segment carrying a fertility restoring gene. Genome 46, 792–797. doi: 10.1139/g03-055
Kaur, G., Banga, S. K., Gogna, K. P. S., Joshi, S., and Banga, S. S. (2004). Moricandia arvensis cytoplasm based system of cytoplasmic male sterility in Brassica juncea: Reappraisal of fertility restoration and agronomic potential. Euphytica 138, 271–276. doi: 10.1023/B:EUPH.0000047098.33909.b7
Kirti, P. B., Mohapatra, T., Prakash, S., and Chopra, V. L. (1995). Development of a stable cytoplasmic male sterile line of Brassica juncea from somatic hybrid Trachystoma balli + Brassica juncea. Plant Breed. 114, 434–438. doi: 10.1111/j.1439-0523.1995.tb00826.x
Kirti, P. B., Prakash, S., Gaikwad, K., Bhat, S. R., Dinesh Kumar, V., and Chopra, V. L. (1998). Chloroplast substitution overcomes leaf chlorosis in a Moricandia arvensis-based cytoplasmic male sterile Brassica juncea. Theor. Appl. Genet. 97, 1179–1182. doi: 10.1007/s001220051007
Kumar, S., and Sangha, M. K. (2017). Biochemical mechanism of resistance in some Brassica genotypes against Lipaphis erysimi (Kaltenbach) (Homoptera: Aphidiae). Vegetos 26, 387–395. doi: 10.5958/j.2229-4473.26.2.103
Kumrawat, M., and Yadav, M. (2018). Trends in area, production, and yield of mustard crop in Bharatpur region of Rajasthan. Inter. J. Eng. Dev. Res. 6, 315–321.
Malik, M., Vyas, P., Rangaswamy, N. S., and Shivanna, K. R. (1999). Development of two new cytoplasmic male sterile lines of Brassica juncea through wide hybridization. Plant Breed. 118, 75–78. doi: 10.1046/j.1439-0523.1999.118001075.x
Mawlong, I., Sujith Kumar, M. S., Gurung, B., Singh, K. H., and Singh, D. (2017). A simple spectrophotometric method for estimating total glucosinolates in mustard de-oiled cake. Inter. J. Food Prop. 20, 3274–3281. doi: 10.1080/10942912.2017.1286353
Melonek, J., Duarte, J., Martin, J., Beuf, L., Murigneux, A., and Murigneux, A. (2021). The genetic basis of cytoplasmic male sterility and fertility restoration in wheat. Nat. Commun. 12:1036. doi: 10.1038/s41467-021-21225-0
Mian, H. R., Kuspira, J., Walker, G. W. R., and Muntagewerff, N. (1974). Histological and cytological studies on five genetic male sterile lines of barley (Hordeum vulgare). Can. J. Genet. Cyto. 16, 355–379. doi: 10.1139/g74-040
Murty, B. R., Thakur, R. P., Mehta, S. L., and Srivastava, K. N. (1997). Physiological analysis of cytoplasmic differentiation in pearl millet. Proc. Indian Nat. Sci. Acad. 63B, 333–348.
Pahwa, R. S., Banga, S. K., Gogna, K. P. S., and Banga, S. S. (2004). Tournefortii male sterile system in Brassica napus: Identification expression and genetic characterization of male sterile restorers. Plant Breed. 123, 444–448. doi: 10.1111/j.1439-0523.2004.00960.x
Palial, S., Kumar, S., Atri, C., Sharma, S., and Banga, S. S. (2022). Antixenosis and antibiosis mechanisms of resistance to turnip aphid, Lipaphis erysimi (Kaltenbach) in Brassica juncea-fruticulosa introgression lines. J. Pest Sci. 95, 749–760. doi: 10.1007/s10340-021-01418-8
Pathania, A., Bhat, S. R., Dinesh Kumar, V., Kirti, P. B., Ashutosh Prakash, S., et al. (2003). Cytoplasmic male sterility in alloplasmic Brassica juncea carrying Diplotaxis catholica cytoplasm: Molecular characterization and genetics of fertility restoration. Theor. Appl. Genet. 107, 455–461. doi: 10.1007/s00122-003-1266-4
Piekarska, A., Kusznierewicz, B., Meller, M., Dziedziul, K., Namieśnik, J., and Bartoszek, A. (2013). Myrosinase activity in different plant samples; Optimisation of measurement conditions for spectrophotometric and pH-stat methods. Ind. Crops. Prod. 50, 58–67. doi: 10.1016/j.indcrop.2013.06.048
Prakash, S., Kirti, P. B., Bhat, S. R., Gaikwad, K., Kumar, V. D., and Chopra, V. L. (1998). A Moricandia arvensis based cytoplasmic male sterility and fertility restoration system in Brassica juncea. Theor. Appl. Genet. 97, 488–492. doi: 10.1007/s001220050921
Rana, J. S. (2005). Performance of Lipaphis erysimi (Homoptera: Aphididae) on different Brassica species in a tropical environment. J. Pest Sci. 78, 155–160. doi: 10.1007/s10340-005-0088-3
Samal, I., Dhillon, M. K., and Singh, N. (2021). Biological performance and biochemical interactions of mustard aphid (Lipaphis erysimi) in Brassica juncea. Indian J. Agric. Sci. 91, 1347–1352.
Samal, I., Singh, N., Bhoi, T. K., and Dhillon, M. K. (2022). Elucidating effect of different photosynthetic pigments on Lipaphis erysimi preference and population build-up on diverse Brassica juncea genotypes. Ann. Appl. Biol. doi: 10.1111/aab.12774
Savchenko, N. I., Belons, V. E., and Scraya, L. V. (1968). Biochemical peculiarities of winter lines with cytoplasmic male sterility. Tistol Genet. Kiev USSR 2, 226–231.
Senthil, N., and Manickam, A. (1995). Peroxidase activity in sorghum anthers. Ann. Agril. Res. 16, 104–105.
Sharma, H. C. (2001). Cytoplasmic male-sterility and source of pollen influence the expression of resistance to sorghum midge. Stenodiplosis sorghicola. Euphytica 122, 391–395. doi: 10.1023/A:1012995409298
Sharma, H. C., Abraham, C. V., Vidyasagar, P., and Stenhouse, J. W. (1996). Gene action for resistance in sorghum to midge, Contarinia sorghicola. Crop Sci. 36, 259–265. doi: 10.2135/cropsci1996.0011183X003600020008x
Sharma, H. C., Dhillon, M. K., Naresh, J. S., Singh, R., Pampapathy, G., and Reddy, B. V. S. (2004). “Influence of cytoplasmic male-sterility on the expression of resistance to insects in sorghum,” in Proceeding of the fourth international crop science congress, 25 September–1 October 2004, eds T. Fisher, N. Turner, J. Angus, L. McIntyre, M. Robertson, A. Borrell, et al. (Brisbane).
Sharma, H. C., Vidyasagar, P., Abraham, C. V., and Nwanze, K. F. (1994). Effect of cytoplasmic male-sterility in sorghum on host plant interaction with sorghum midge, Contarinia sorghicola. Euphytica 74, 35–39. doi: 10.1007/BF00033764
Shi, C. H., Xue, J. M., Yu, Y. G., Yang, X. E., and Zhu, J. (1996). Analysis of genetic effects on nutrient quality traits in indica rice. Theor. Appl. Genet. 92, 1099–1102. doi: 10.1007/BF00224055
Singh, D. (2014). “Genetic enhancement of mustard for seed yield and its sustainability,” in Proceeding of the national conference on brassicas for addressing edible oil and nutritional security. Society for rapeseed mustard research, eds V. Kumar, P. D. Meena, D. Singh, S. Banga, V. Sardana, and S. S. Banga (Ludhiana: Bharatpur at Punjab Agricultural University), 18.
Soybean Processors Association of India [SOPA] (2020). India oilseeds-area, production and productivity. The soybean processors association of India. Available online at: http://www.sopa.org/india-oilseeds-area-production-and-productivity (accessed April 5, 2022).
Vinu, V., Singh, N., Yadava, D. K., Sujata, V., Saini, N., Bhat, S. R., et al. (2017). Effect of cytoplasms and genetic backgrounds of parental lines on fertility restoration in Brassica juncea hybrids. Indian J. Agric. Sci. 87, 197–202.
Watson, C. V., Nath, J., and Nanda, D. (1977). Possible mitochondrial involvement in mechanism of cytoplasmic male sterility in maize (Zea mays). Biochem. Genet. 15, 1113–1124. doi: 10.1007/BF00484501
Xu, Z. G., and Song, H. M. (1997). Resistance to bacterial leaf blight in some CMS lines of rice. Acta Agric. Bor. Sin. 12, 46–50.
Keywords: Brassica juncea, Lipaphis erysimi, cytoplasmic male sterility, host plant resistance, mustard aphid, resistance mechanisms
Citation: Singh N and Dhillon MK (2022) Nucleo-cytoplasmic interactions affecting biological performance of Lipaphis erysimi in Brassica juncea. Front. Plant Sci. 13:971606. doi: 10.3389/fpls.2022.971606
Received: 17 June 2022; Accepted: 01 August 2022;
Published: 18 August 2022.
Edited by:
Prem Lal Kashyap, Indian Institute of Wheat and Barley Research (ICAR), IndiaReviewed by:
Preetinder Singh Sarao, Punjab Agricultural University, IndiaHari C. Sharma, Dr. Yashwant Singh Parmar University of Horticulture and Forestry, India
Copyright © 2022 Singh and Dhillon. This is an open-access article distributed under the terms of the Creative Commons Attribution License (CC BY). The use, distribution or reproduction in other forums is permitted, provided the original author(s) and the copyright owner(s) are credited and that the original publication in this journal is cited, in accordance with accepted academic practice. No use, distribution or reproduction is permitted which does not comply with these terms.
*Correspondence: Mukesh K. Dhillon, bXVrZXNoZGhpbGxvbkByZWRpZmZtYWlsLmNvbQ==
†ORCID: Mukesh K. Dhillon, https://orcid.org/0000-0001-6781-9211