- 1Key Laboratory of Ecohydrology of Inland River Basin, Northwest Institute of Eco-Environment and Resources, Chinese Academy of Sciences, Lanzhou, China
- 2Faculty of Resources and Environment, University of Chinese Academy of Sciences, Beijing, China
- 3Faculty of Resources and Environment, Baotou Teachers’ College, Inner Mongolia University of Science and Technology, Baotou, China
As a desert shrub, Haloxylon ammodendron combines ecological, economic, and social benefits and plays an important role in the ecological conservation of arid desert areas. Understanding its physiological characteristics and its mechanism of light energy utilization is important for the conservation and utilization of H. ammodendron. Therefore, we selected five stands (5-, 11-, 22-, 34-, and 46-year-old) of H. ammodendron as research objects in the study and measured their photosynthetic light response curves by a portable open photosynthesis system (Li-6400) with a red-blue light source (6400-02B). Then, we measured the leaf chlorophyll parameters in the laboratory, calculated the photosynthetic characteristics by using Ye Zipiao’s photosynthetic model, analyzed their variation patterns across stand ages, and explored the relationships between leaf chlorophyll parameters and photosynthetic characteristics. The results showed that leaf chlorophyll parameters and photosynthetic characteristics of H. ammodendron at different stand ages were significantly different. Chl content, Pnmax, and LUEmax of H. ammodendron were V-shaped with the increase of stand age. The 5-year-old H. ammodendron was in the rapid growth period, synthesized more Chl a+b content (8.47 mg g−1) only by using a narrower range of light, and the Pnmax and LUEmax were the highest with values of 36.21 μmol m−2 s−1 and 0.0344, respectively. For the 22-year-old H. ammodendron, due to environmental stress, the values of Chl a+b content, Pnmax, and LUEmax were the smallest and were 2.64 mg g−1, 25.73 μmol m−2 s−1, and 0.0264, respectively. For the older H. ammodendron, its Chl content, Pnmax, and LUEmax were not significantly different and tended to stabilize but were slightly higher than those of the middle-aged H. ammodendron. On the other hand, the other photosynthetic parameters did not show significant variation patterns with stand age, such as Rd, AQE, LSP, LCP, and IL-sat. In addition, we found that the relationships between Chl a+b content and Pnmax and between Chl a+b content and LUEmax were highly correlated, except for the older H. ammodendron. Thus, using leaf chlorophyll content as a proxy for photosynthetic capacity and light use efficiency should be considered with caution. This work will provide a scientific reference for the sustainable management of desert ecosystems and vegetation restoration in sandy areas.
Introduction
Haloxylon ammodendron (C. A. Meyer) Bunge is a multistemmed shrub, which has been widely planted in desert areas for its strong ecological adaptability, windbreak and sand fixation ability, and high economic value. It has played an important role in performing important ecosystem functions, maintaining the structure and function of the desert ecosystem, and maintaining an important ecological security barrier along the northern border of China (Su et al., 2007; Maina and Wang, 2015). However, in recent decades, large-scale decline and death of both nature and cultivated H. ammodendron were widespread in arid desert areas in northwest China, such as the Ganjiahu Haloxylon Forest National Nature Reserve, Gurbantunggut Desert, desert-oasis ecotone of Zhangye and Minqin, Alaska Plateau, and so on (Yu and Wang, 2018; Wang et al., 2021), which affected the sustainability of the ecosystem (Li et al., 2007). The physiological and ecological changes and sensitivity to the environment of H. ammodendron have become one of the key issues that researchers are paying attention to.
Photosynthesis is not only the core component of the carbon cycle in terrestrial ecosystems and the initial driving force of materials and energy cycles on earth but also has a key role in maintaining the carbon–oxygen balance of the atmosphere (Sellers et al., 1997; Zhang et al., 2020; Qian et al., 2021). Leaf chlorophyll (Chl), being the most important and abundant pigment in the plant photosystem (PS), contributes to the conversion of solar radiation into chemical energy (Croft et al., 2020; Qian et al., 2021). Photosynthetic characteristics can reflect the plant’s photosynthetic capacity, physiological and ecological adaptation patterns to different environments, and light utilization strategies (Li et al., 2010). They also may be a diagnostic index of the forest succession and ecological recovery status (Eschenbach et al., 1998; Yang et al., 2008). For trees, the transition from juvenile to adult and then to old may take many years (Merilo et al., 2009). Throughout the growth process, plants have optimized the use of light energy by regulating chlorophyll contents (Chl a, Chl b, and Chl a+b) and ratio (Chl a/b), which allows plants to continuously adapt to their environment and promote plant growth (Li et al., 2018). Chl varies significantly across leaf position, nitrogen supply, growing season, leaf ages, and so on (Yang et al., 2014; Li et al., 2018; Croft et al., 2020; Zhang et al., 2020; Zhang et al., 2021a). Net photosynthesis has a decreased trend with increasing tree age or tree size (Kull and Koppel, 1987; Bond, 2000; Greenwood et al., 2008). Zhang et al. (2021a) found that the younger plants would be more shade-tolerant than the older plants by comparing the photosynthetic response of 1- and 4-year-old savin juniper plants (Zhang et al., 2021b). In the Alaska Plateau, H. ammodendron has been cultivated continuously for more than 40 years. During growth, we suspect that some physiological changes may occur, such as the ability to synthesize photosynthetic pigments and photosynthetic capacity. Perhaps this change is relatively subtle, but it may lead to significant variation in carbon and water exchange in trees of different stand classes (Goulden et al., 1996). Recently, studies on H. ammodendron mainly focused on drought resistance, salt stress tolerance, water utilization, and so on (Xu and Li, 2006; Zhou et al., 2017; Wang et al., 2021; Zhou et al., 2022). Nevertheless, up to now, little is known about how Chl and the photosynthetic characteristic of H. ammodendron vary with stand age.
In addition, light use efficiency (LUE), as a key parameter in estimating gross primary production (GPP), has been widely studied by scholars and measured by different methods (Farquhar et al., 1980; Ahl et al., 2004; Wang et al., 2010; Guan et al., 2021; Wellington et al., 2022). On the LUE-based GPP estimation model, LUEmax was treated as a constant for each biome type (Muramatsu et al., 2014). However, LUEmax varies with plant type, canopy density, age, and nutrient conditions (Turner et al., 2003; Wang et al., 2010; Wellington et al., 2022). Regrettably, the accurate simulation of LUEmax is still an issue that needs to be addressed. Scientists hypothesized a relationship between chlorophyll content and GPP and confirmed that chlorophyll content is a better proxy for photosynthetic capacity than leaf N (Croft et al., 2017; Qian et al., 2021). This idea is innovative, so we ponder whether chlorophyll content can be used as a proxy for LUEmax.
Thus, the objectives of this paper are to address the following: 1) compare the changes of leaf chlorophyll (Chl a, Chl b, Chl a+b, and Chl a/b) and photosynthetic characteristics (Pnmax, LUEmax) for H. ammodendron at five different stand ages (5-, 11-, 22-, 34-, and 46-year-old) and 2) explore the relationship between leaf chlorophyll and photosynthetic characteristics and verify whether leaf chlorophyll content can be used as a proxy for LUEmax. This study helps us to clearly know the photosynthetic change rules of H. ammodendron with growing years and understand the physiological characteristics and the mechanism of light energy utilization, which is important for ecological restoration in extremely arid areas as well as for providing data and useful information for global carbon calculation.
Materials and methods
Site description
The study area is located in Yabulai Town (101°52'E–103°33'E, 39°08'N–40°18'N, mean elevation of 1,585 m), Alxa Right Banner, Alxa League, in Inner Mongolia Autonomous Region of China. It has a typical dry continental climate, the average annual precipitation is 84 mm, and the average annual evaporation is 3,225.7 mm. The region has a large temperature difference between winter and summer, and the average temperature is −9.2°C in January and 25.8°C in July. It has ample sunshine, and the average annual sunshine is 3,000–3,400 h. Due to a worse natural environment, the vegetation cover is low, and the vegetation is mainly composed of super-xerophytic and xerophytic shrubs and subshrubs and xerophytic perennial and annual herbs. After more than 40 years of artificial cultivation, H. ammodendron has become the most important, widely planted and distributed sand-fixing species and plays an important role in restoring the local ecological environment.
Field sampling
The field study was performed on the basis of the actual survey and investigation and used the method of substitution of space for time. The stand sequence of H. ammodendron was represented by five even-aged stands of different ages: 5, 11, 22, 34, and 46 years old (age indicates how many years a tree has grown since it was planted). These stands are located in the southeastern Badain Jaran Desert, and they are very close to each other (Figure 1). They have the same planting density (230 trees per hectare, the most widely used planting density in the study area). They grew on similar soil (sandy soil with loose soil structure) and experience a similar climate. The groundwater level is about 100 m. In addition, H. ammodendron was given manual watering for the first 3 years after being planted, after which there was no other human interference. A 50-cm × 50-cm sample plot was established in each stand. No other shrubs grew in the sample plot and annual herbs were distributed sporadically. Three healthy, well-grown H. ammodendron trees with little variation were selected from the sample plot in each stand separately. We investigated their location (longitude, latitude, and altitude), crown breadth, diameter, and plant height (Table 1). The assimilating branches with better growth and full sunlight were selected from the upper part of each selected H. ammodendron, which were tagged as samples for the photosynthetic light response curves and leaf chlorophyll content measurements.
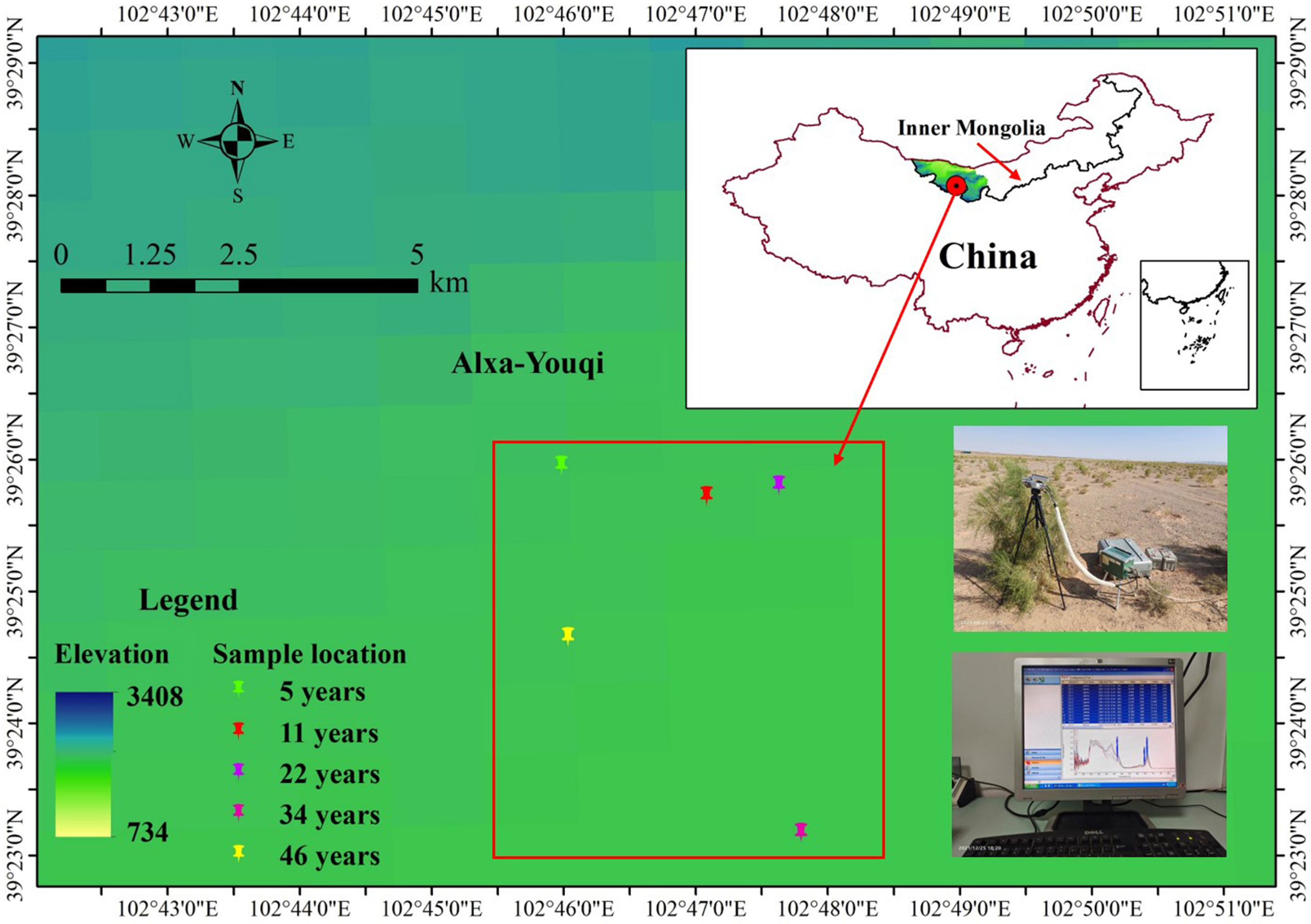
Figure 1 Site locations in Alxa League, China. These were 5, 11, 22, 34, and 46 years of the Haloxylon ammodendron samples, respectively.
Gas exchange measurements
The experiment was conducted in August and September of 2021. Each stand was collected two times, three samples were collected each time, and three replicates were collected for each sample. The photosynthetic light response curves of the leaf photosynthesis of H. ammodendron were measured by a portable open photosynthesis system (Li-6400; Li-Cor, Inc., NE, USA) with a red-blue light source (6400-02B). Measurements were carried out between 10:00 and 14:00 h. Firstly, we selected 6 to 10 assimilating branches and spread them in the leaf chamber. After the assimilating branches were clamped, the surrounding of the leaf chamber was sealed with plasticine to avoid air leakage. A tripod was used to fix the analyzer to ensure that the assimilating branches were in their original growth position. Secondly, adequate light induction of 0.5–1 h was performed on each assimilated branch before the measurement. Finally, the CO2 concentration was held at 400 μmol mol−1 by using a CO2 small cylinder, with a stepwise photosynthetic photon flux density of 2,000, 1,800, 1,500, 1,200, 1,000, 800, 600, 400, 200, 150, 120, 100, 75, 50, 25, and 0 μmol m−2 s−1; leaf temperature of 25°C, flow rate of 500 μmol s−1; and relative humidity kept between 30% and 50%. A complete photosynthetic light response curve approximately 1 h was carried out.
Measurement of photosynthetic area and leaf chlorophyll content
After gas exchange measurements, all assimilating branches enclosed in the leaf chamber were carefully cut off for photosynthetic area measurement and chlorophyll content determination.
The assimilating branches were special and could not fill the whole leaf chamber; thus, the total surface area of the assimilating branches could be regarded as the photosynthetic effective area. The assimilating branch is similar to the cylinder, so the vernier calipers (0.05 mm) were used to determine the diameter, and the total area in the leaf chamber can be calculated according to the formula of calculating the surface area of the cylinder (because the leaf chamber is illuminated on one side, the actual photosynthetic area is one-half of the calculated area) (Chen and Black, 1992). The photosynthetic rate was corrected according to the calculated actual area value.
The leaf area (LA) was calculated by the following formula (1):
Where d is the average diameter of assimilating branches and n is the number of assimilating branches within the leaf chamber.
After measuring the area, the samples were placed in the car refrigerator (0°C) before being transported to the laboratory and stored at −70°C. We measured Chl content by the following steps: 1) 120 ml of distilled water and 480 ml of acetone were mixed thoroughly; 2) fresh leaves were cleaned; 0.1 g was weighed, cut up, and then 1 ml of distilled water was added and powered and transferred to a 10-ml glass test tube; 3) the volume was adjusted to 10 ml using the solution mixed in step (1) and the solution was allowed to stand for 3–6 h in a dark environment; and 4) 1 ml of the solution extract was taken to the cuvette, and the absorbance values at 663 and 645 nm were measured by using a spectrophotometer and recorded as A663 and A645, respectively.
Analytical model
A non-asymptotic model was developed and tested by Ye to better characterize the light response of photosynthesis (Ye, 2007; Ye et al., 2013; Ye et al., 2020). The formula was as follows:
The saturation irradiance (Isat) corresponding to the maximum net photosynthetic rate (Pnmax) can be calculated by formula (7) and formula (8):
α is the initial slope of the light response curve of photosynthesis, β is the photoinhibition coefficient, γ is the saturation coefficient, I is the irradiance, Pn is the photosynthetic rate, and Rd is the dark respiratory rate.
According to the definition of LUE and formula (1), we can derive formula (9):
The saturation irradiance (IL-sat), corresponding to the leaf maximum light energy use efficiency (LUEmax), can be calculated as follows:
Statistical analysis
The light response curves and light energy use efficiency were processed by the photosynthetic model fitting software provided by Ye Zipiao. One-way analysis of variance (ANOVA) with post-hoc Duncan’s multiple comparison was used to test the differences in leaf chlorophyll and photosynthetic parameters for different stand ages. We used Spearman’s correlation analysis to analyze the relationship between leaf chlorophyll and photosynthesis. Then, we used linear regression analyses to explore the relationship changes with stand age. All analyses were conducted by the software SPSS25 (SPSS Inc., Chicago, IL, USA). All figures were produced in OriginPro 2021 (OriginLab Corp., Northampton, MA, USA). The significance level was set at P<0.05.
Results
Distribution and variations in leaf chlorophyll content and ratio
Table 2 illustrates the distribution of leaf chlorophyll parameters, namely, Chl a, Chl b, Chl a+b, and Chl a/b. In the samples, Chl a distribution was in the range of 2.24–7.02 mg g−1, with a mean value of 4.78 mg g−1; Chl b was 0.40–2.19 mg g−1, with a mean value of 1.21 mg g−1; Chl a+b was 2.64–8.47 mg g−1, with a mean value of 5.99 mg g−1; and Chl a/b was more than 2.25-fold and less than 5.65-fold, with an average value about 4.15. Regardless of leaf chlorophyll content and ratio, the coefficients of variation (CVs) were all approximately 0.25 (Table 2).
There were significant differences in Chl a, Chl b, Chl a+b, and Chl a/b among H. ammodendron at different stand ages (P< 0.05): for Chl a content, 5-year-old > 11-year-old > 34-year-old > 46-year-old > 22-year-old (Figure 4A); for Chl b content, 34-year-old > 5-year-old > 46-year-old > 11-year-old > 22-year-old (Figure 4B); and for Chl a+b content, 5-year-old > 11-year-old > 34-year-old > 46-year-old > 11-year-old (Figure 4C), exactly the same trend as the Chl a content. With increasing planting years, leaf chlorophyll content first decreased, then increased, and finally stabilized, and the values of the 22-year-old stand were the lowest. The Chl a and Chl a+b contents were the highest in the 5-year-old stand, while Chl b content was the highest in the 34-year-old stand. However, Chl a/b did not follow the pattern of leaf chlorophyll content changes. Chl a/b was the lowest in the 34-year-old stand, and there were no significant differences at 5-, 11-, and 22-year-old stands (P< 0.05). This was evidenced where Chl a/b was higher in the younger stand compared to the older stand (Figure 4D).
Variations in photosynthetic characteristics
The model selected in this study well simulated the photosynthetic light response curves of H. ammodendron, the determining coefficients all reaching a significant level, with R2 >0.99 (P< 0.001) (Table 3). When I <250 μmol m−2 s−1, Pn was basically the same at different stand ages, and with the enhancement of I, significant differences in Pn appeared among the different stand ages (Figure 2). Pnmax was the highest in the 5-year-old H. ammodendron, decreased in the 11-year-old H. ammodendron, and reached a valley in the 22-year-old H. ammodendron, and then increased in the 34-year-old H. ammodendron; the differences between the light response curves of the 34- and 46-year-old H. ammodendron were small (Figure 2 and Table 3). There were no significant differences in Pnmax between the 5- and 11-year-old stands and between the 34- and 46-year-old stands (P< 0.05). Above all, the photosynthetic capacity of H. ammodendron was related to the stand age: the younger H. ammodendron > the older H. ammodendron > the middle-aged H. ammodendron.
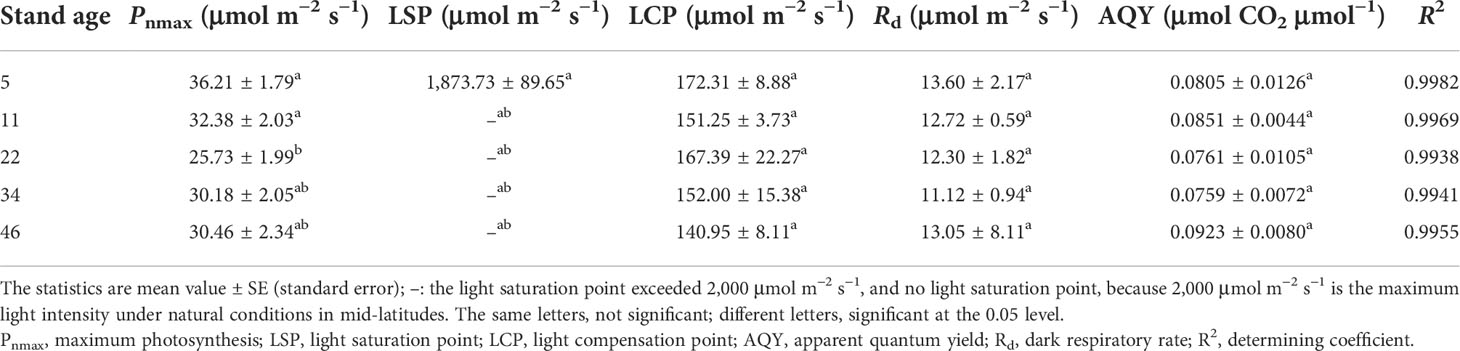
Table 3 Statistics of the photosynthetic characteristics of Haloxylon ammodendron at different stand ages.
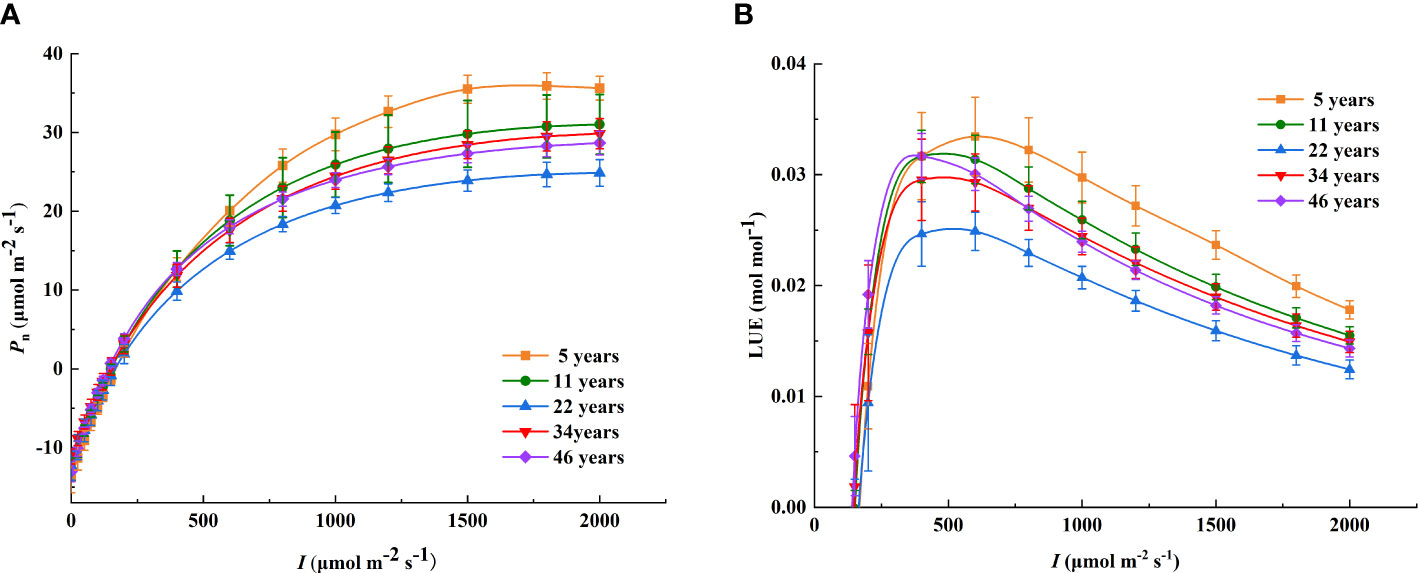
Figure 2 The light response curves of Pn and LUE at different stand ages. (A) The light response curves of Pn; (B) the light response curves of LUE. The different colored lines show Haloxylon ammodendron with different stand ages. I, photosynthetic photon flux density; Pn, net photosynthetic rate; LUE, light use efficiency.
Furthermore, the light saturation point (LSP) of the 5-year-old H. ammodendron was 1,873.73 μmol m−2 s−1, and the others exceeded 2,000 μmol m−2 s−1. There were no significant differences in light compensation point (LCP) among the different stand ages of H. ammodendron; however, the LCP showed a tendency to decrease with the increase of stand age. Similarly, both Rd and apparent quantum yield (AQY) did not have significant differences among all stand ages of H. ammodendron (P< 0.05). Rd tended to decrease with the increase of stand age, while AQY did not show a significant change pattern with the increase of stand age. The minimum AQY was the 34-year-old H. ammodendron with a value of 0.0759, and the maximum AQY was the 46-year-old H. ammodendron with a value of 0.0923.
Variations in LUE
As shown in Figure 2B, under the condition of low light intensity, LUE increased rapidly and peaked and then decreased as I increased. Moreover, the light response curves of light use efficiency were significantly different at different stand ages of H. ammodendron. The light intensity required to reach the maximum LUE was different for different stand ages. Compared to the other age classes of H. ammodendron, the LUEmax of H. ammodendron at age 5 was the highest, and the corresponding IL-sat was also the highest, with values of 0.0344 mol mol−1 and 604.01 μmol m−2 s−1, respectively. The IL-sat corresponding to the LUEmax of the 46-year-old stand was minimal, and the values of IL-sat and LUEmax were 427.62 μmol m−2 s−1 and 0.0320 mol mol−1, respectively. Notably, the LUEmax of the 22-year-old H. ammodendron was 0.0264, which was the smallest among all stand ages. As the stand age increased, LUEmax showed the same trend of decreasing and then increasing similar to Pnmax. However, there was no significant difference (P< 0.05) at different stand ages. The IL-sat of H. ammodendron corresponding to LUEmax ranged from 427.62 to 604.01 μmol m−2 s−1 at each age and tended to decrease with the increase of stand age (Table 4).
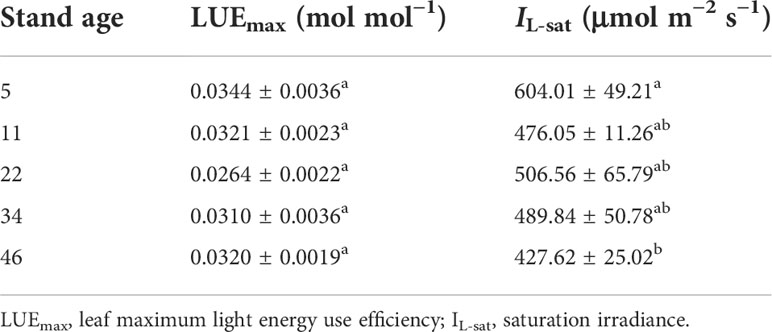
Table 4 The maximum light use efficiency and saturation irradiance for Haloxylon ammodendron at different stand ages.
The relationships between leaf chlorophyll parameters and photosynthetic characteristics
As shown in Figure 3, we found positive relationships between Chl a and Pnmax and between Chl a and LUEmax, and the values of the correlation coefficients were 0.52 and 0.52, respectively. Chl a was weakly correlated with Rd and AQY, did not correlate with IL-sat, and had a weak negative correlation with LSP and LCP (P ≤ 0.05). Chl b was significantly positively correlated with Pnmax, LUEmax, and AQY, and the R2 values were 0.63, 0.68, and 0.39, respectively. Chl b was weakly correlated with LSP, Rd, and AQY and weakly negatively correlated with LCP and IL-sat. Chl a+b was significantly positively correlated with Pnmax and LUEmax, both with R2 values of 0.60 and 0.61, respectively. Chl a+b had weak positive correlations with Rd and AQY, weak negative correlations with LSP and LCP, and no correlation with IL-sat. Chl a/b had an insignificant correlation with photosynthetic characteristics, weak positive correlations with LCP and IL-sat, and negative correlations with Pnmax, LUEmax, LSP, Rd, and AQY. The results indicated that leaf chlorophyll contents (Chl a, Chl b, and Chl a+b) had significant effects on Pnmax and LUEmax of H. ammodendron.
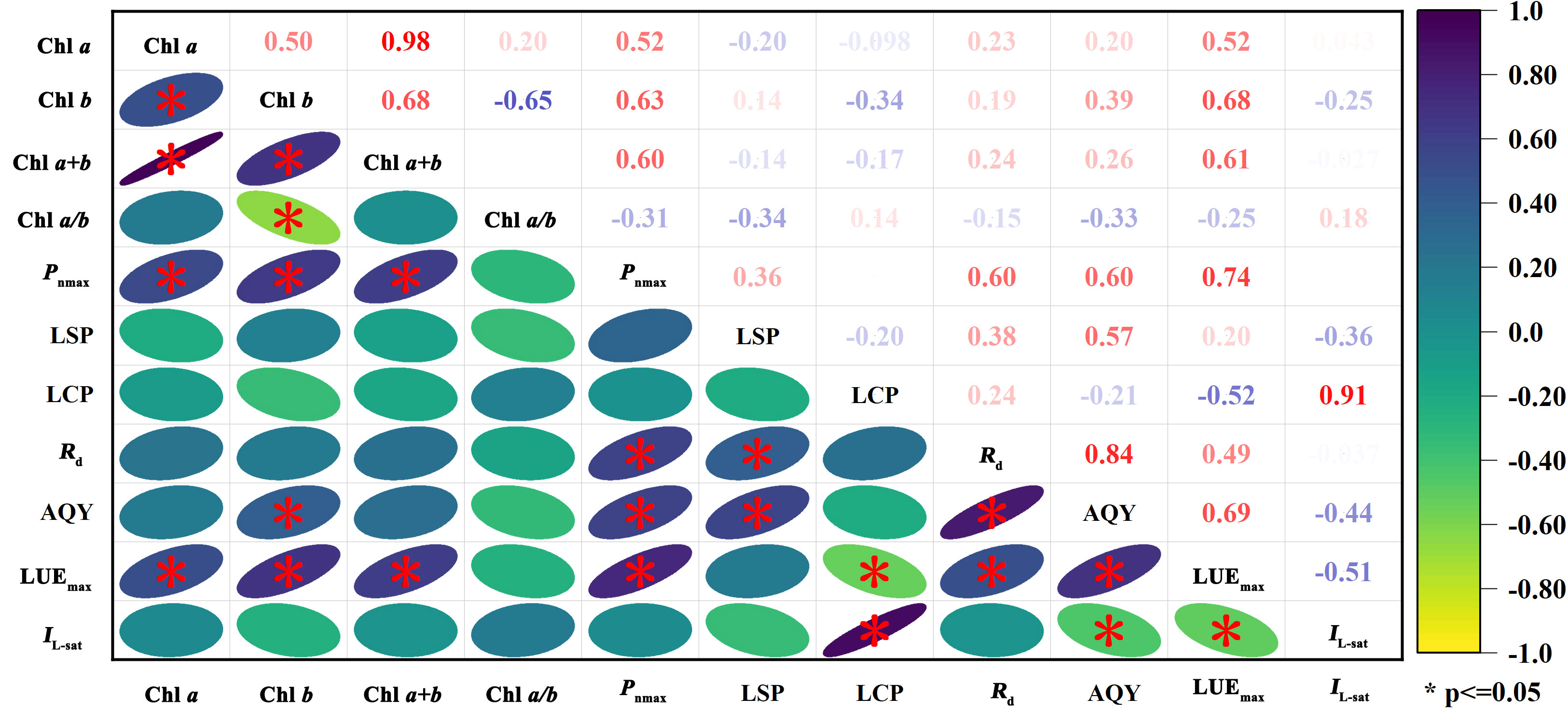
Figure 3 Heatmap of Spearman correlation coefficients between leaf chlorophyll parameters and photosynthetic characteristics (P ≤ 0.05).
In addition, as shown in Figure 6, there were significant positive correlations between Chl a+b content and Pnmax and between Chl a+b content and LUEmax at the stage of younger and middle age. The correlation was weak in the older age stage, and this was even a negative correlation. Therefore, we believed that the stand age of H. ammodendron may have influenced their relationships between Chl a+b content and Pnmax and between Chl a+b content and LUEmax.
Discussion
Impacts of stand age variations in leaf chlorophyll parameters
Leaf chlorophyll content and ratio were analyzed to explore differences among stand ages in light acclimation (Zhang et al., 2021b) and to reflect the use efficiency of direct radiation and adaptability to the environment (Valladares and Niinemets, 2008; Zhang et al., 2020). In our study, the variation of leaf chlorophyll contents (Chl a, Chl b, and Chl a+b) showed a “V” shape with the increase of the stand age (Figure 4). For the 22-year-old H. ammodendron, the leaf chlorophyll content was the lowest and formed an inflection point. This may be due to the fact that the 22-year-old H. ammodendron was subjected to water stress during growth, and water deficiency affected chlorophyll synthesis and promoted chlorophyll decomposition. Chlorophyll is the initiator of plant production. The chlorophyll content of the younger H. ammodendron was higher, which was the basis for its rapid metabolism and growth. Wang et al. (2020) compared the chlorophyll content of five stand ages for H. ammodendron in Minqin, Gansu Province. Although there was no significant difference, the Chl content of the middle-aged H. ammodendron was the lowest and that of the younger H. ammodendron was the highest (Wang et al., 2020). In addition, the Chl content of H. ammodendron in Minqin was significantly lower than that in our study. On the contrary, Wang et al. (2021) found that Chl content increased significantly with the increase of H. ammodendron planted for years in the typical desert–oasis ecotone of the Hexi Corridor (Wang et al., 2021). This may be related to the higher annual rainfall in Hexi Corridor, and H. ammodendron was less affected by water stress.
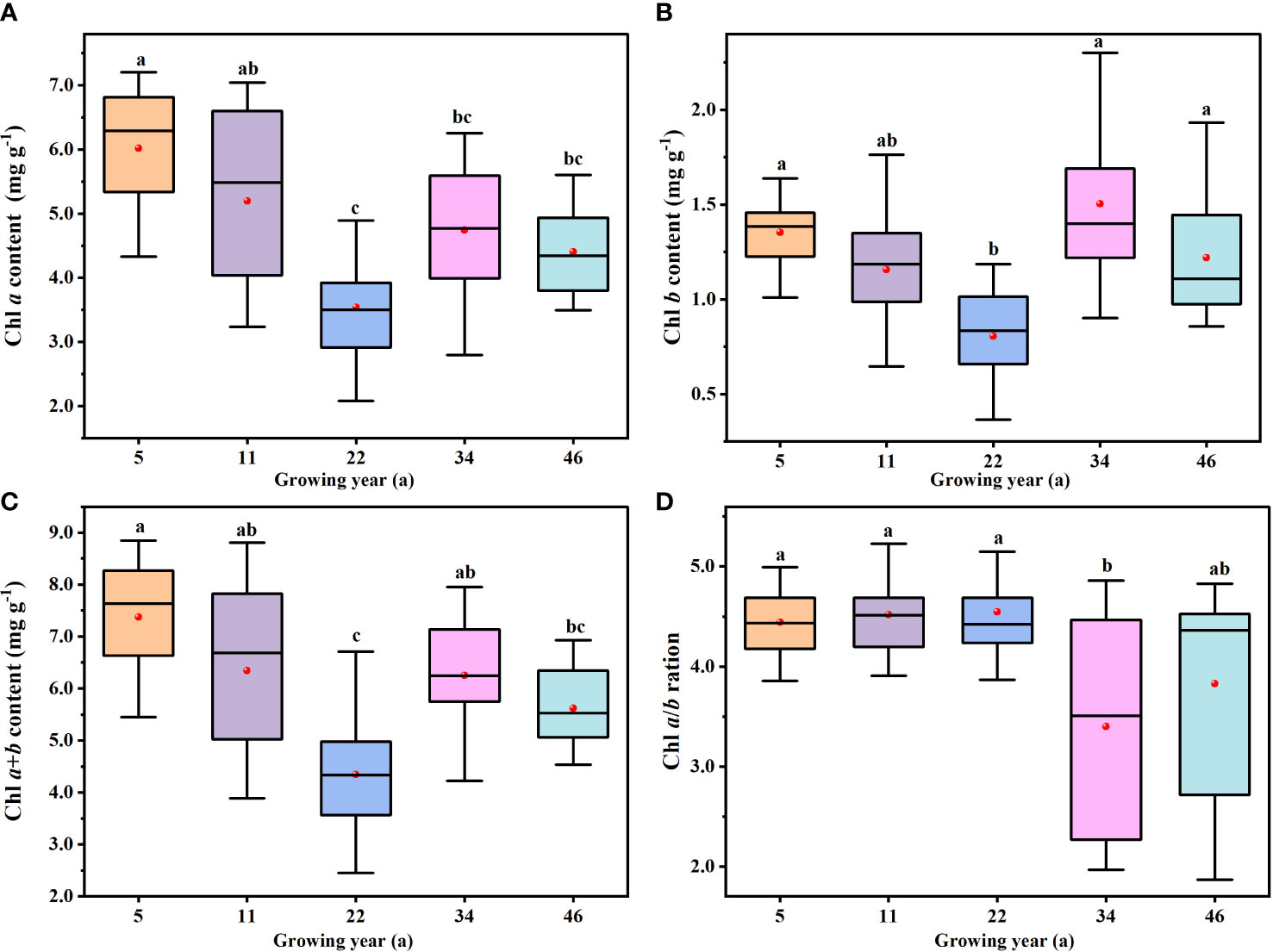
Figure 4 The variations in leaf chlorophyll content and ratio at different stand ages. (A–D) Calculated in Chl a, Chl b, Chl a+b, and Chl a/b, respectively. The black lines across the boxes are median values, and the red points represent the mean values. The same letters denote no significant difference among the different stand ages of Haloxylon ammodendron (P< 0.05).
The older H. ammodendron (34- and 46-year-old stands) had higher Chl b and larger antennae size for better use of diffused light (Grieco et al., 2015). By investigation, we observed that the crown width and plant height of the older H. ammodendron were larger (Table 1), many assimilating branches occluded each other, and more dead branches appeared. Therefore, we suggested that H. ammodendron can improve the utilization rate of low light and achieve light use efficiency by improving the synthesis of Chl b and reducing the ratio of Chl a to Chl b (index of plant tolerance to sun/shade) (Terashima and Evans, 1988; Kitajima and Hogan, 2003). Chl a/b in the younger H. ammodendron was significantly higher than that in the older H. ammodendron (Figure 4D). On the contrary, Avramov et al. (2017); Zhang et al. 2021a and Zhang et al. 2021b found that the younger plants had higher Chl content and Chl a/b than the older plants (Avramov et al., 2017; Zhang et al., 2021b). This may be caused by differences in the study species, ages, growing seasons, or leaf positions (Kitajima and Hogan, 2003; Maina and Wang, 2015; Li et al., 2018; Zhang et al., 2020). In addition, Chl a/b was more stable than Chl content during the growth of H. ammodendron, which shows some phylogenetic signals (Maina and Wang, 2015).
Impacts of stand age variations in Pnmax and LUEmax
Photosynthetic light response curves are an important way to understand plant photochemical efficiency, and the parameters of the curves can reflect the response of plant photosynthetic mechanisms to the environment. Pnmax is the average photosynthetic rate observed when the incident light is above the LSP, which represents the maximum photon utilization capability and the net primary productivity of the plants and reflects biomass accumulation (Yang et al., 2008). LUEmax is an important parameter for estimating GPP or NPP in ecosystems (Yuan et al., 2014). Studying the differences in LUEmax can enhance the accuracy of productivity models and provide a basis for carbon cycle studies at regional and global scales. In our present study, we found that photosynthetic responses of H. ammodendron differed over the growing stages, with both Pnmax and LUEmax showing a “V”-shaped pattern.
The younger H. ammodendron (5- and 11-year-old) were at the peak growth stage, with characteristics such as smaller plants, rapid growth, and well-developed root systems, and the limited precipitation and small soil water content can maintain their growth requirements; thus, they have strong photosynthetic capacity and light use efficiency, which can achieve rapid accumulation of dry matter and thus enhance tolerance to wind and sand flow. At the same time, the Tr and Gs of the younger H. ammodendron varied widely in response to the changes in light intensity (Figure 5), so they have rapid responsiveness to the environmental changes. In addition, the younger H. ammodendron only made use of a less narrow range of I than the other stand ages, and its LSP was lower and its LCP was higher (Table 3), which revealed that using the range of I does not effectively determine Pnmax and LUEmax. Yang et al. (2008) found that Ziziphus jujuba had a higher LUE than Juglans regia, but Z. jujuba only used the less narrow range of I than J. regia (Yang et al., 2008).
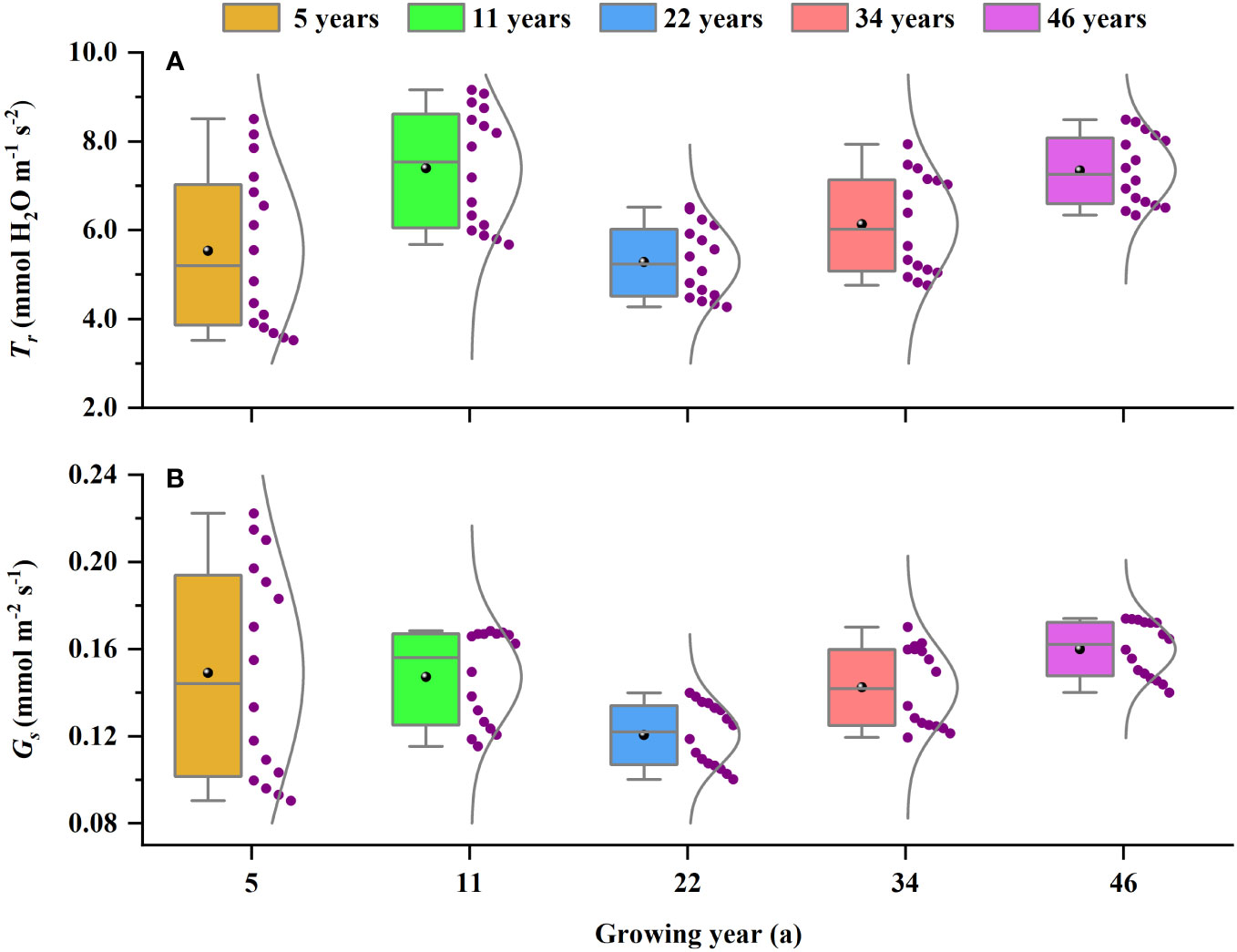
Figure 5 The variations in (A) Tr and (B) Gs at different planting ages. The purple dots represent the values of Tr and Gs under different light intensities. Tr, transpiration; Gs, stomatal conductance.
Haloxylon ammodendron may consume much soil water and soil nutrients in the early stage of rapid growth, resulting in a stressed growth environment for the middle-aged H. ammodendron (22-year-old). Soil water has an important effect on the activity and quantity of photosynthetic enzymes (Yang et al., 2008); thus, water deficit limited the photosynthetic capacity and LUE (Gao et al., 2016). When subjected to water stress, H. ammodendron regulated the physiological characteristics by reducing stomatal conductance, decreasing transpiration (Figure 5), and reducing respiratory consumption, to realize the optical protection process (Demmig-Adams and Adams, 2000). In this way, organic matter was accumulated through low photosynthesis for defense and adaptation to adversity. At the same time, H. ammodendron was able to expand the range of available light by decreasing LSP and increasing LCP under severe drought conditions (Table 3), which improves its ability to use resources efficiently. This is consistent with the conclusion of Yang et al. (2018). The Pnmax and LUEmax increased in the older H. ammodendron (34- and 46-year-old), and there was a decreasing trend with growth. In response to this phenomenon, we believed that there are three main reasons for this phenomenon: firstly, the growth speed of the belowground part of H. ammodendron was prior than that of the belowground part (Wei et al., 2007), the main depth that H. ammodendron used soil water increased as stand age increased (Zhu and Jia, 2011), and water stress was alleviated by the formation of a more powerful root system in the growth of H. ammodendron (34- and 46-year-old), in order to adapt to the arid environment; secondly, degradation of the older H. ammodendron (34- and 46-year-old) has occurred, and the ratio of the numbers of live to dead branches of individual H. ammodendron decreased, providing opportunities for photosynthesis by live branches which have larger stomatal conductance; thus, the live branches of the older H. ammodendron had higher Pnmax and LUEmax; thirdly, H. ammodendron has developed its own extreme drought tolerance mechanism and photosynthetic regulation strategy in long-term adversity (Gao et al., 2016), and the older H. ammodendron plants are able to use a greater range of light and maintain a higher photosynthetic capacity and light energy use efficiency. However, with the increase of stand age, plants have a tendency to senesce, physiological activity decreases, and photosynthetic capacity gradually decreases (Schoettle, 1994).
Leaf chlorophyll content should be cautiously used as a proxy for LUEmax
Chl is a key factor affecting photosynthetic capacity and vegetation productivity and can be accurately retrieved, so it can be used as a proxy for Vcmax25 at large scales to resolve the uncertainty in Vcmax25 in C cycle modeling (Croft et al., 2013; Croft et al., 2017; Qian et al., 2021). LUEmax is also an important parameter for productivity estimation. The accurate simulation of LUEmax is the basis for quantifying spatial and temporal changes in productivity and the global carbon cycle. Thus, we hope to establish the relationship between LUEmax and Chl content. So, we tested the correlation coefficient between Chl a+b content and LUEmax in different stand ages of H. ammodendron. Contrary to expectations, the R2 tended to decrease with the increase of stand ages (Figure 6) and R2<0.2 in the older H. ammodendron (34- and 46-year-old). We believed that Chl might be used as a proxy for LUEmax in the younger H. ammodendron (5- and 11-year-old) and middle-aged H. ammodendron (22-year-old), but the older H. ammodendron cannot. In the past, scholars found a similar phenomenon when studying the relationship between PRI and LUE. This relationship was affected by leaf age, and the reason was that carotenoid and chlorophyll content had a relatively significant negative correlation on PRI (Penuelas et al., 1995; Gamon et al., 2001; Guo and Trotter, 2004).
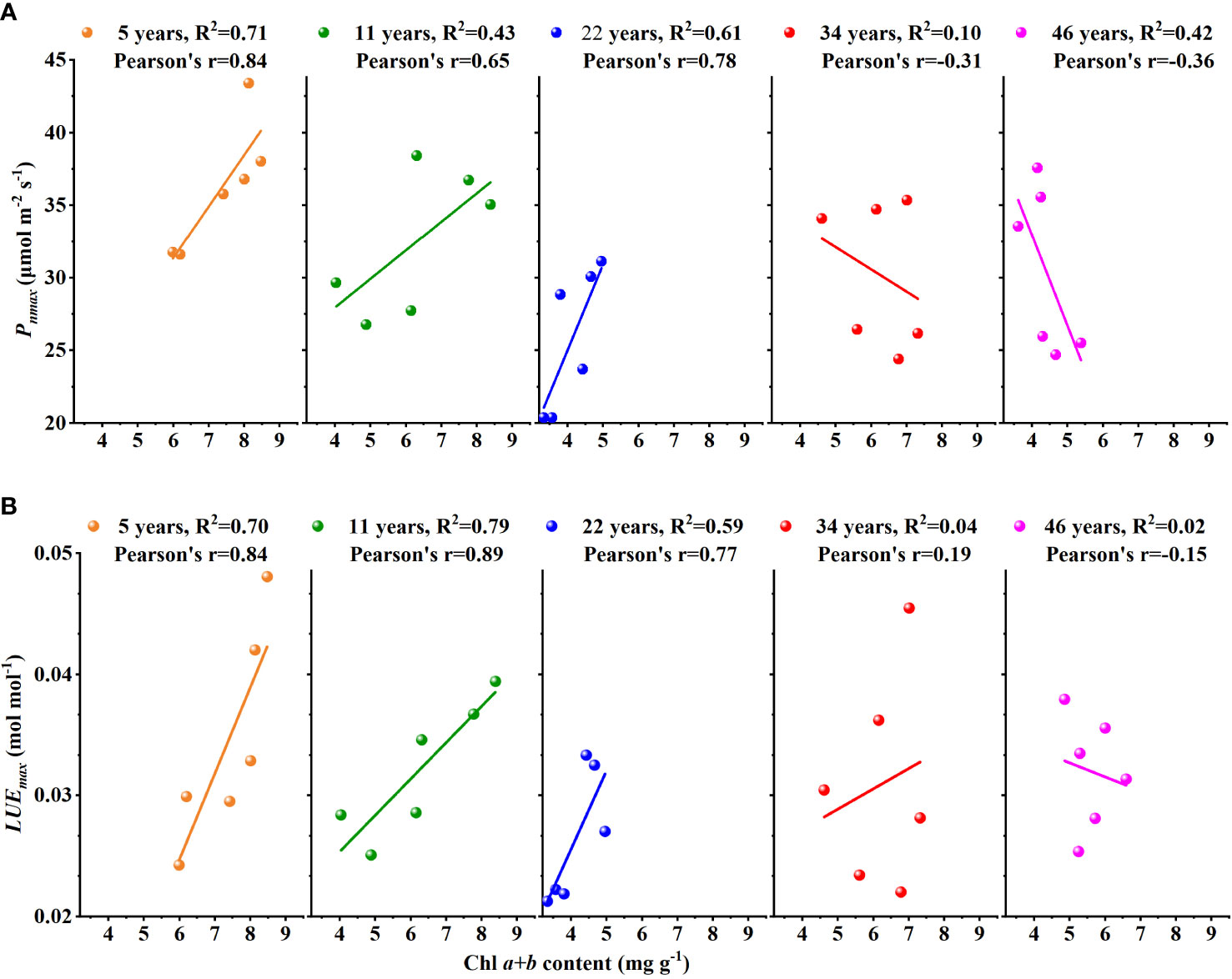
Figure 6 The relationships (A) between Chl a+b content and Pnmax and (B) between Chl a+b content and LUEmax changes with the stand age of Haloxylon ammodendron.
Conclusions
After long-term cultivation, H. ammodendron has become a major windbreak and sand-fixing species in the Alaska Plateau. Understanding the physiological features of H. ammodendron at different stand ages is helpful for protecting and utilizing H. ammodendron, which is important to curb the ecological degradation in arid desert areas. In this study, we reported leaf chlorophyll pigments and photosynthesis variation with stand age. We found that the Chl content, Pnmax, and LUEmax of H. ammodendron were V-shaped with the increase of stand age. The younger H. ammodendron was in the growing stage and had a higher ability of chlorophyll synthesis and photosynthesis; the middle-aged H. ammodendron was affected by environmental stress, and it reduced the synthesis of Chl content and maintained life requirements by reducing photosynthesis, which needs proper human intervention. While the older H. ammodendron can form a stable adaptation mechanism in long-term growth, its Chl content, Pnmax, and LUEmax were not significantly different and tended to stabilize but were slightly higher than those of the middle-aged H. ammodendron. In addition, the relationship between Chl a+b content and LUEmax decreased with the increase of the stand age. Thus, using leaf chlorophyll content as a proxy for photosynthetic capacity and light use efficiency should be considered with caution.
Data availability statement
The original contributions presented in the study are included in the article/Supplementary Material. Further inquiries can be directed to the corresponding author.
Author contributions
X-HH conceived the ideas, designed the methodology, participated in the field works, and wrote the manuscript. J-HS designed the methodology and organized the manuscript. D-MZ, C-LW, and JQ participated in the field works and indoor experiments. BJ and C-YZ analyzed the data and results. D-MZ, C-LW, JQ, and X-LZ produced the figures and tables. All authors contributed critically to the article and approved the submitted version.
Funding
This study was supported by the Major Science and Technology Project in Inner Mongolia Autonomous region of China (No. Zdzx2018057); the Innovation Cross Team Project of Chinese Academy of Sciences, CAS (No. JCTD-2019-19); Transformation Projects of Scientific and Technological Achievements in Inner Mongolia Autonomous region of China (No. 2021CG0046); Science and Technology Research Project of Colleges and Universities in Inner Mongolia Autonomous Region (NJZY21034); and the National Natural Science Foundation of China (No. 42001038).
Acknowledgments
The authors thank all the members of the Key Laboratory of Eco-Hydrology of Inland River Basin, Northwest Institute of Eco-Environment and Resources, Chinese Academy of Sciences and the Faculty of Resources and Environment, Baotou Teachers’ College, Inner Mongolia University of Science and Technology.
Conflict of interest
The authors declare that the research was conducted in the absence of any commercial or financial relationships that could be construed as a potential conflict of interest.
The reviewer J-LL declared a shared affiliation, with the authors X-HH, J-HS, D-MZ, C-LW, C-YZ, BJ, JQ, X-LZ to the handling editor at the time of the review.
Publisher’s note
All claims expressed in this article are solely those of the authors and do not necessarily represent those of their affiliated organizations, or those of the publisher, the editors and the reviewers. Any product that may be evaluated in this article, or claim that may be made by its manufacturer, is not guaranteed or endorsed by the publisher.
References
Ahl, D. E., Gower, S. T., Mackay, D. S., Burrows, S. N., Norman, J. M., Diak, G. R. (2004). Heterogeneity of light use efficiency in a northern Wisconsin forest: implications for modeling net primary production with remote sensing. Remote Sens Environ. 93, 168–178. doi: 10.1016/j.rse.2004.07.003
Avramov, S., Miljkovic, D., Klisaric, N. B., Zivkovic, U., Tarasjev, A. (2017). Ontogenetic plasticity of anatomical and ecophysiological traits and their correlations in iris pumila plants grown in contrasting light conditions. Plant Species Biol. 32, 392–402. doi: 10.1111/1442-1984.12171
Bond, B. J. (2000). Age-related changes in photosynthesis of woody plants. Trends Plant Sci. 5, 349–353. doi: 10.1016/S1360-1385(00)01691-5
Chen, J. M., Black, T. A. (1992). Defining leaf-area index for non-flat leaves. Plant Cell Environ. 15, 421–429. doi: 10.1111/j.1365-3040.1992.tb00992.x
Croft, H., Chen, J. M., Luo, X. Z., Bartlett, P., Chen, B., Staebler, R. M.. (2017). Leaf chlorophyll content as a proxy for leaf photosynthetic capacity. Glob. Change Biol. 23, 3513–3524. doi: 10.1111/gcb.13599
Croft, H., Chen, J. M., Wang, R., Mo, G., Luo, S., Luo, X., et al. (2020). The global distribution of leaf chlorophyll content. Remote Sens Environ. 236, 111479. doi: 10.1016/j.rse.2019.111479
Croft, H., Chen, J. M., Zhang, Y., Simic, A. (2013). Modelling leaf chlorophyll content in broadleaf and needle leaf canopies from ground, CASI, landsat TM 5 and MERIS reflectance data. Remote Sens Environ. 133, 128–140. doi: 10.1016/j.rse.2013.02.006
Demmig-Adams, B., Adams, W. W. (2000). Photosynthesis - harvesting sunlight safely. Nature 403, 371–373. doi: 10.1038/35000315
Eschenbach, C., Glauner, R., Kleine, M., Kappen, L. (1998). Photosynthesis rates of selected tree species in lowland dipterocarp rainforest of sabah. Malaysia Trees-Struct Funct. 12, 356–365. doi: 10.1007/s004680050162
Farquhar, G. D., Caemmerer, S. V., Berry, J. A. (1980). A biochemical-model of photosynthetic CO2 assimilation in leaves of c-3 species. Planta 149, 78–90. doi: 10.1007/Bf00386231
Gamon, J. A., Field, C. B., Fredeen, A. L., Thayer, S. (2001). Assessing photosynthetic downregulation in sunflower stands with an optically-based model. Photosynthesis Res. 67, 113–125. doi: 10.1023/A:1010677605091
Gao, T. T., Zheng, S. W., Zhou, X. H., Wang, D. X., Lu, X. P. (2016). Photosynthetic physiological characteristics of Gazania rigens l. under drought stress. Iop C Ser. Earth Env. 41, 012027. doi: 10.1088/1755-1315/41/1/012027
Goulden, M. L., Munger, J. W., Fan, S. M., Daube, B. C., Wofsy, S. C. (1996). Exchange of carbon dioxide by a deciduous forest: Response to interannual climate variability. Science 271, 1576–1578. doi: 10.1126/science.271.5255.1576
Greenwood, M. S., Ward, M. H., Day, M. E., Adams, S. L., Bond, B. J. (2008). Age-related trends in red spruce foliar plasticity in relation to declining productivity. Tree Physiol. 28, 225–232. doi: 10.1093/treephys/28.2.225
Grieco, M., Suorsa, M., Jajoo, A., Tikkanen, M., Aro, E. M. (2015). Light-harvesting II antenna trimers connect energetically the entire photosynthetic machinery-including both photosystems II and I. Bba-Bioenergetics 1847, 607–619. doi: 10.1016/j.bbabio.2015.03.004
Guan, X. B., Chen, J. M., Shen, H. F., Xie, X. Y. (2021). A modified two-leaf light use efficiency model for improving the simulation of GPP using a radiation scalar. Agr For. Meteorol. 307, 108546. doi: 10.1016/j.agrformet.2021.108546
Guo, J. M., Trotter, C. M. (2004). Estimating photosynthetic light-use efficiency using the photochemical reflectance index: variations among species. Funct. Plant Biol. 31, 255–265. doi: 10.1071/Fp03185
Kitajima, K., Hogan, K. P. (2003). Increases of chlorophyll a/b ratios during acclimation of tropical woody seedlings to nitrogen limitation and high light. Plant Cell Environ. 26, 857–865. doi: 10.1046/j.1365-3040.2003.01017.x
Kull, O., Koppel, A. (1987). Net photosynthetic response to light intensity of shoots from different crown positions and age in Picea abies (L.) karst. scand. J. For. Res. 2, 157–166. doi: 10.1080/02827588709382454
Li, Y., He, N. P., Hou, J. H., Xu, L., Liu, C. C., Zhang, J. H., et al. (2018). Factors influencing leaf chlorophyll content in natural forests at the biome scale. Front. Ecol. Evol. 6. doi: 10.3389/fevo.2018.00064
Li, X. R., Kong, D. S., Tan, H. J., Wang, X. P. (2007). Changes in soil and vegetation following stabilisation of dunes in the southeastern fringe of the tengger desert, China. Plant Soil 300, 221–231. doi: 10.1007/s11104-007-9407-1
Li, X., Zheng, J. C., Yan, S. H., Ren, C. G., Wang, M., Cong, W., et al. (2010). Dynamics of photosynthesis in eichhornia crassipes solms of jiangsu of China and their influencing factors. Afr J. Biotechnol. 9, 7302–7311. doi: 10.5897/AJB10.1087
Maina, J. N., Wang, Q. (2015). Seasonal response of chlorophyll a/b ratio to stress in a typical desert species: Haloxylon ammodendron. Arid Land Res. Manage 29, 321–334. doi: 10.1080/15324982.2014.980588
Merilo, E., Tulva, I., Räim, O., Kukit, A., Sellin, A., Kull, O. (2009). Changes in needle nitrogen partitioning and photosynthesis during 80 years of tree ontogeny in picea abies. Trees-Struct Funct. 23, 951–958. doi: 10.1007/s00468-009-0337-9
Muramatsu, K., Furumi, S., Soyama, N., Daigo, M. (2014). Estimating the seasonal maximum light use efficiency. Proc. Spie. 9260, 92603R. doi: 10.1117/12.2069142
Penuelas, J., Filella, I., Gamon, J. A. (1995). Assessment of photosynthetic radiation-use efficiency with spectral reflectance. New Phytol. 131, 291–296. doi: 10.1111/j.1469-8137.1995.tb03064.x
Qian, X., Liu, L., Croft, H., Chen, J. (2021). Relationship between leaf maximum carboxylation rate and chlorophyll content preserved across 13 species. J. Geophys Res-Biogeo 126, e2020JG006076. doi: 10.1029/2020JG006076
Schoettle, A. W. (1994). Influence of tree size on shoot structure and physiology of pinus-contorta and pinus-aristata. Tree Physiol. 14, 1055–1068. doi: 10.1093/treephys/14.7-8-9.1055
Sellers, P. J., Dickinson, R. E., Randall, D. A., Betts, A. K., Hall, F. G., Berry, J. A., et al. (1997). Modeling the exchanges of energy, water, and carbon between continents and the atmosphere. Science 275, 502–509. doi: 10.1126/science.275.5299.502
Su, P. X., Cheng, G. D., Yan, Q. D., Liu, X. M. (2007). Photosynthetic regulation of c-4 desert plant Haloxylon ammodendron under drought stress. Plant Growth Regul. 51, 139–147. doi: 10.1007/s10725-006-9156-9
Terashima, I., Evans, J. R. (1988). Effects of light and nitrogen nutrition on the organization of the photosynthetic apparatus in spinach. Plant Cell Physiol. 29, 143–155. doi: 10.1093/oxfordjournals.pcp.a077461
Turner, D. P., Urbanski, S., Bremer, D., Wofsy, S. C., Meyers, T., Gower, S. T., et al. (2003). A cross-biome comparison of daily light use efficiency for gross primary production. Glob. Change Biol. 9, 383–395. doi: 10.1046/j.1365-2486.2003.00573.x
Valladares, F., Niinemets, U. (2008). Shade tolerance, a key plant feature of complex nature and consequences. Annu. Rev. Ecol. Evol. S 39, 237–257. doi: 10.1146/annurev.ecolsys.39.110707.173506
Wang, G. H., Chen, Y. L., Gou, Q. Q. (2021). Responses of Haloxylon ammodendron with different plantation ages to changes of soil moisture in a desert-oasis ecotone. Acta Ecol Sin. 41, 5658–5668. doi: 10.5846/stxb202007151859
Wang, F., Guo, S. J., Fan, B. L., Zhang, W. X., Wang, F. L., Han, F. G., et al. (2020). Variation in leaf functional traits of different-aged Haloxylon ammodendron communities, and the relationship with soil factors. Pratacult Sci. 37 (12), 2486–2496. doi: 10.11829/j.issn.1001-0629.2020-0266
Wang, H. S., Jia, G. S., Fu, C. B., Feng, J. M., Zhao, T. B., Ma, Z. G. (2010). Deriving maximal light use efficiency from coordinated flux measurements and satellite data for regional gross primary production modeling. Remote Sens Environ. 114, 2248–2258. doi: 10.1016/j.rse.2010.05.001
Wei, J., Zhang, X. M., Shan, L. S., Yan, H. L., Liang, S. M. (2007). Seedling growth dynamic of Haloxylon ammodendron and its adaptation strategy to habitat condition in hinterland of desert. Sci. China Ser. D: Earth Sci. 50, 107–114. doi: 10.1007/s11430-007-5006-5
Wellington, M. J., Kuhnert, P., Renzullo, L. J., Lawes, R. (2022). Modelling within-season variation in light use efficiency enhances productivity estimates for cropland. Remote Sens-Basel 14 (6), 1495. doi: 10.33390/rs14061495
Xu, H., Li, Y. (2006). Water-use strategy of three central Asian desert shrubs and their responses to rain pulse events. Plant Soil 285, 5–17. doi: 10.1007/s11104-005-5108-9
Yang, H. B., An, S. Q., Sun, J. X., Shi, Z. M., She, X. S., Sun, Q. Y., et al. (2008). Seasonal variation and correlation with environmental factors of photosynthesis and water use efficiency of juglans regia and ziziphus jujuba. J. Integr. Plant Biol. 50, 210–220. doi: 10.1111/j.1744-7909.2007.00391.x
Yang, S. R., Fan, J. W., Sun, Y. Q., Li, C. J., Xu, X. W. (2018). Photosynthetic characteristics and response of Haloxylon ammodendron to drought stress in hinterland of the lop nur. Arid Zone Res. 35 (2), 379–286. doi: 10.13866/j.azr.2018.02.16
Yang, H., Li, J. W., Yang, J. P., Wang, H., Zou, J. L., He, J. J. (2014). Effects of nitrogen application rate and leaf age on the distribution pattern of leaf SPAD readings in the rice canopy. PloS One 9 (2), e88421. doi: 10.1371/journal.pone.0088421
Ye, Z. P. (2007). A new model for relationship between irradiance and the rate of photosynthesis in Oryza sativa. Photosynthetica 45, 637–640. doi: 10.1007/s11099-007-0110-5
Ye, Z. P., Ling, Y., Yu, Q., Duan, H. L., Kang, H. J., Huang, G. M., et al. (2020). Quantifying light response of leaf-scale water-use efficiency and its interrelationships with photosynthesis and stomatal conductance in c-3 and c-4 species. Front. Plant Sci. 11. doi: 10.3389/fpls.2020.00374
Ye, Z. P., Suggett, D. J., Robakowski, P., Kang, H. J. (2013). A mechanistic model for the photosynthesis-light response based on the photosynthetic electron transport of photosystem II in C3 and C4 species. New Phytol. 199, 110–120. doi: 10.1111/nph.12242
Yuan, W. P., Cai, W. W., Xia, J. Z., Chen, J. Q., Liu, S. G., Dong, W. J., et al. (2014). Global comparison of light use efficiency models for simulating terrestrial vegetation gross primary production based on the la thuile database. Agr For. Meteorol 192, 108–120. doi: 10.1016/j.agrformet.2014.03.007
Yu, K. L., Wang, G. H. (2018). Long-term impacts of shrub plantations in a desert-oasis ecotone: accumulation of soil nutrients, salinity, and development of herbaceour layer. Land Degrad Dev. 29 (8), 2681–2693. doi: 10.1002/ldr.3009
Zhang, Y., Li, Y., Wang, R. M., Xu, L., Li, M. X., Zhao, G. L., et al. (2020). Spatial variation of leaf chlorophyll in northern hemisphere grasslands. Front. Plant Sci. 11. doi: 10.3389/fpls.2020.01244
Zhang, J. L., Li, X. L., Xu, X. H., Chen, H. P., Li, Y. L., Guy, R. D. (2021b). Leaf morphology, photosynthesis and pigments change with age and light regime in savin juniper. Plant Biol. 23, 1097–1108. doi: 10.1111/plb.13256
Zhang, J. F., Wan, L., Lgathinathane, C., Zhang, Z., Guo, Y., Sun, D. W., et al. (2021a). Spatiotemporal heterogeneity of chlorophyll content and fluorescence response within rice (Oryza sativa l.) canopies under different nitrogen treatments. Front. Plant Sci. 12. doi: 10.3389/fpls.2021.645977
Zhou, D. M., Si, J. H., He, X. H., Jia, B., Zhao, C. Y., Wang, C. L., et al. (2022). The process of soil desiccation under Haloxylon ammodendron plantations: A case study of the alxa legue desert, China. Plants 11, 235. doi: 10.3390/plants11030235
Zhou, H., Zhao, W. Z., Zhang, G. F. (2017). Varying water utilization of Haloxylon ammodendron plantations in a desert-oasis ecotone. Hydrol Process 31 (4), 825–883. doi: 10.1002/hyp.11060
Keywords: Haloxylon ammodendron, leaf chlorophyll parameters, maximal net photosynthetic rate (Pnmax), maximum light energy use efficiency (LUEmax), stand age
Citation: He X-h, Si J-h, Zhou D-m, Wang C-l, Zhao C-y, Jia B, Qin J and Zhu X-l (2022) Leaf chlorophyll parameters and photosynthetic characteristic variations with stand age in a typical desert species (Haloxylon ammodendron). Front. Plant Sci. 13:967849. doi: 10.3389/fpls.2022.967849
Received: 13 June 2022; Accepted: 13 September 2022;
Published: 06 October 2022.
Edited by:
Yuanrun Zheng, Institute of Botany (CAS), ChinaReviewed by:
Ji-Liang Liu, Northwest Institute of Eco-Environment and Resources (CAS), ChinaFeng Zhang, Gansu Agricultural University, China
Shengkui Cao, Qinghai Normal University, China
Copyright © 2022 He, Si, Zhou, Wang, Zhao, Jia, Qin and Zhu. This is an open-access article distributed under the terms of the Creative Commons Attribution License (CC BY). The use, distribution or reproduction in other forums is permitted, provided the original author(s) and the copyright owner(s) are credited and that the original publication in this journal is cited, in accordance with accepted academic practice. No use, distribution or reproduction is permitted which does not comply with these terms.
*Correspondence: Jian-hua Si, amlhbmh1YXNAbHpiLmFjLmNu