- Key Laboratory of Sweetpotato Biology and Biotechnology, Ministry of Agriculture and Rural Affairs/ Beijing Key Laboratory of Crop Genetic Improvement/Laboratory of Crop Heterosis and Utilization, Ministry of Education, College of Agronomy & Biotechnology, China Agricultural University, Beijing, China
Small open reading frames (sORFs) can encode functional polypeptides or act as cis-translational regulators in stress responses in eukaryotes. Their number and potential importance have only recently become clear in plants. In this study, we identified a novel sORF gene in sweet potato, IbEGF, which encoded the 83-amino acid polypeptide containing an EGF_CA domain. The expression of IbEGF was induced by PEG6000, H2O2, abscisic acid (ABA), methyl-jasmonate (MeJA) and brassinosteroid (BR). The IbEGF protein was localized to the nucleus and cell membrane. Under drought stress, overexpression of IbEGF enhanced drought tolerance, promoted the accumulation of ABA, MeJA, BR and proline and upregulated the genes encoding superoxide dismutase (SOD), catalase (CAT) and peroxidase (POD) in transgenic sweet potato. The IbEGF protein was found to interact with IbCOP9-5α, a regulator in the phytohormone signalling pathways. These results suggest that IbEGF interacting with IbCOP9-5α enhances drought tolerance by regulating phytohormone signalling pathways, increasing proline accumulation and further activating reactive oxygen species (ROS) scavenging system in transgenic sweet potato.
Introduction
Drought stress is the most complex and devastating factor of abiotic stresses and seriously affects the productivity of agricultural crops in the world (Shinozaki and Yamaguchi-Shinozaki, 2007; Ceccarelli et al., 2010; Zhu, 2016; Peterson et al., 2021). Improving the drought tolerance of agricultural crops has become important for the sake of world food security (Zhu, 2016). The plant hormones, such as abscisic acid (ABA), jasmonic acid (JA), brassinosteroid (BR), ethylene (ETH) and salicylic acid (SA) play important roles in the response of plants to drought stress (Fujita et al., 2005; Vargas et al., 2014; Jin et al., 2016; Xu et al., 2017). The biochemical and genetic studies have revealed that peptides are new signalling molecules in plants (Pearce et al., 2001; Ryan and Pearce, 2001; Lindsey et al., 2002; Ryan et al., 2002). Until the initial discovery of the 18-amino acid (aa) polypeptide defense hormone system in tomato leaves in 1991, polypeptides are thought to be a new class of plant hormones (Pearce et al., 1991).
Small open reading frames (sORFs), which encode polypeptides of less than 100 aa in eukaryotes and 50 aa in prokaryotes, have been historically excluded from genome annotation (Basrai et al., 1997; Frith et al., 2006; Hanada et al., 2013). However, recent studies have revealed thousands of translated sORFs in genomes spanning evolutionary space (Khitun et al., 2019). These sORFs can code for functional polypeptides or act as cis-translational regulators, including stress proteins, energy metabolism-related proteins, mating pheromones, hormones, transcriptional regulators, nucleases, transporters etc. (Basrai et al., 1997; Kastenmayer et al., 2006; Khitun et al., 2019; Kim et al., 2021).
The first systematic investigation of sORFs function was conducted in yeast. It was showed that 299 sORFs existed in the yeast genome, representing approximately 5% of the annotated ORFs, and 22 of them was required for haploid growth, growth in the presence of DNA damage and replication-arrest agents, growth at high temperature or growth in the presence of a nonfermentable carbon source (Kastenmayer et al., 2006). More than 7000 sORFs have been identified in Arabidopsis, and 3241 of them likely belong to novel coding genes in the Arabidopsis genome (Hanada et al., 2007).
A number of specific polypeptides encoded by sORFs have been identified in plants. In tomato, rapid alkalinization factor (RALF), which is a 5-kDa ubiquitous polypeptide, arrested root growth and development (Pearce et al., 2001). The 23-aa peptide AtPep1 increased root development and enhanced resistance to Pythium irregulare in transgenic Arabidopsis (Huffaker et al., 2006; Poncini et al., 2017). In rice, the plants overexpressing OsDT11 encoding an 88-aa peptide displayed reduced water loss and stomatal density and enhanced drought tolerance (Li et al., 2017). The CLAVATA3/EMBRYO-SURROUNDING REGION-RELATED 25 (CLE25) peptide modulated stomatal control in Arabidopsis (Takahashi et al., 2018). The small peptide OsDSSR1 (Oryza sativa L. drought and salt stress response-1) enhanced drought tolerance in transgenic rice (Cui et al., 2018). A C-terminal peptide fragment (AtPep3) increased salt tolerance in transgenic Arabidopsis (Nakaminami et al., 2018). The CLAVATA3/EMBRYO SURROUNDING REGION (CLE) peptides are important ligands that have roles in regulating cell proliferation and differentiation in plant shoots, roots and other tissues (Li et al., 2019; Song et al., 2021). In Arabidopsis, CLE9 induced stomatal closure and enhanced drought tolerance (Zhang et al., 2019). PttCLE47 from Populus promoted cambial development and secondary xylem formation in hybrid aspen (Kucukoglu et al., 2020). The phytosulfokine (PSK) peptide regulated drought-induced flower drop in tomato (Reichardt et al., 2020).
Epidermal growth factor (EGF) is a short peptide with an important role in the migration and proliferation of cells (Cohen, 1983; Plata-Salaman, 1991; Ren et al., 2018). The calcium-binding EGF-like domain (EGF_CA domain) is a sequence of about 40 aa and it is present in numerous extracellular proteins and membrane-bound proteins (Rao et al., 1995). Some cell surface receptors have numerous EGF modules in tandem, and a subset of EGF modules bind one calcium ion (Ca2+) for the biosynthesis of biologically active proteins (Stenflo et al., 2000). The cysteine-rich epidermal patterning factor (EPF) and EPF-like (EPFL) regulate stomatal patterning in Arabidopsis (Hara et al., 2007; Jewaria et al., 2013; Zeng et al., 2020). The epidermal growth factor receptors (EGFR/ErbB) are membrane-anchored tyrosine kinases with a critical role in cell growth and development (Pascarelli et al., 2021). However, the EGF protein family with EGF_CA domain have not been functionally characterized in plants.
Sweetpotato, Ipomoea batatas (L.) Lam., is an important food crop worldwide and its productivity is seriously affected by drought stress (Zhu et al., 2022). Up to now, sORFs have not been identified in sweet potato. In this study, a novel gene coding for the 83-aa polypeptide, IbEGF, was cloned from sweet potato. The EGF protein contained an EGF_CA domain. Functional analysis showed that IbEGF enhanced drought tolerance in transgenic sweet potato. The underlying mechanism of this gene in drought tolerance of sweet potato was also analyzed.
Materials and methods
Plant materials
Sweetpotato line Xushu55-2 with drought tolerance was used for isolation and expression analysis of the IbEGF gene. Sweetpotato cv. Lizixiang was employed to characterize the function of IbEGF. The subcellular localization, bimolecular fluorescence complementation (BiFC) assay and co-immunoprecipitation (co-IP) assay of IbEGF were conducted using Nicotiana benthamiana.
Cloning ands analysis of IbEGF and its promoter
Total RNA from the in vitro-grown Xushu55-2 plants was extracted using the Trozol Up Kit (ET111, Transgen, Beijing, China). The first-strand cDNA was transcribed from the total RNA with the PrimeScript™ RT reagent Kit with gDNA Eraser (PR047A, Takara, Beijing, China). The open-reading frame (ORF) of IbEGF was amplified from the first-strand DNA using the homologous cloning method and the expressed sequence tag (EST) database of Xushu55-2 (Zhu et al., 2018). Amino acid sequence alignment was analyzed using DNAMAN V6 software. The phylogenetic tree was constructed with MEGA 7.0 software with 1000 bootstrap replicates. The molecular weight and theoretical isoelectric point (pI) of IbEGF were calculated with ProtParam tool (https://web.expasy.org/protparam/).
Genomic DNA of the in vitro-grown Xushu55-2 plants was extracted using the cetyltrimethylammonium bromide (CTAB) method (Rogers and Bendich, 1985) for obtaining the genomic sequence and promoter sequence of IbEGF with the homologous cloning method (Supplementary Table S1). The exon-intron and cis-acting regulatory elements in its promoter were analyzed using the Spign tool (http://www.ncbi.nlm.nih.gov/sutils/spign/splign.cgi) and PlantCARE (http://bioinformatics.psb.ugent.be/webtools/plantcare/html/), respectively.
Expression analysis
The transcript levels of IbEGF in leaf, stem and root tissues of the 4-week-old in vitro-grown plants and leaf, stem, hair root, pencil root and storage root tissues of the 80-day-old field-grown plants of Xushu55-2 were analyzed with quantitative real-time PCR (qRT-PCR) (Zhou et al., 2020). Furthermore, the 4-week-old in vitro-grown Xushu55-2 plants were stressed in Hoagland solution with H2O (control), 30% PEG6000, 100 μM H2O2, 100 mM ABA, 100 μM MeJA and 100 nM BR, respectively, and sampled at 0, 1, 3, 6, 12 and 24 h after stresses for analyzing the expression of IbEGF. Ibactin (AY905538) was used to normalize the expression levels in sweet potato (Liu et al., 2014). All the specific primers are showed in Supplementary Table S1.
Subcellular localization
Using primers IbEGF-OS-F/R (Supplementary Table S1), the ORF of IbEGF without stop codon was amplified, and then inserted into pSuper1300 vector to produce green fluorescent protein (GFP) fusion construct pSuper1300-IbEGF. The fusion construct was transformed into the Agrobacterium tumefaciens strain EHA105, and then infiltrated N. benthamiana leaves. The empty pSuper1300 vector was used as a control. After 48 h of infiltration, the fluorescence signal was observed under excitation wavelength 488 nm using a laser scanning confocal microscope (Olympus, Tokyo, Japan). Meanwhile, the pSuper1300-IbEGF and pSuper1300 vectors were transferred into maize protoplasts, respectively. The empty pSuper1300 vector was used as a control. After 16 h of growth, the fluorescence signal was observed under a laser scanning confocal microscope.
Transactivation activity assay
The full-length of IbEGF was constructed to the yeast expression vector pGBKT7. pGBKT7-IbEGF, pGBKT7-53 (positive control) and pGBKT7 (negative control) were separately transferred into the yeast strain Y2H Gold by the PEG/LiAc method. The transformed yeast strains were cultured on synthetic defined (SD) plates without tryptophan (SD/-trp) for 3 days and then cultured on SD plates containing 5-bromo-4-chioro-3-indoxyl-α-galactopyranoside (X-α-gal) but lacking tryptophan and histidine (SD/-Trp/-His/X-α-gal) for 3 days.
Regeneration of the transgenic sweet potato plants
The overexpression vector pSuper1300-IbEGF was introduced into the A. tumefaciens stain EHA105. Embryogenic suspension cultures of sweet potato cv. Lizixiang were prepared as described by Liu et al. (2001). The transformation and plant regeneration were performed as previously described (Liu et al., 2014). The identification of the transgenic plants was conducted by PCR with specific primers (Supplementary Table S1). The expression levels of IbEGF in the in vitro-grown transgenic and wild type (WT) plants were analyzed using specific primers designed in the non-conserved domain (Supplementary Table S1). The transgenic plants were transferred to pots with soil, vermiculite and humus (1:1:1, v/v/v) in a greenhouse and further to a field for evaluating their drought tolerance.
Drought tolerance assay
The in vitro-grown transgenic and WT plants were cultured on Murashige and Skoog (MS) medium with or without (control) 20% PEG6000 at 27 ± 1°C under 13 h of daylight at 54 μM m–2 s–1. After 4 weeks, the root length and fresh weight were measured (Ren et al., 2020). Furthermore, the 25-cm-long cuttings from the field-grown transgenic and WT plants were treated in Hoagland solution for 4 weeks (control) or in Hoagland solution with 20% PEG6000 for 2 weeks followed by 2 weeks of Hoagland solution in a greenhouse (Ren et al., 2020). The root number and fresh weight were measured.
For further evaluation of drought tolerance, the 25-cm-long cuttings from the field-grown transgenic and WT plants were planted in transplanting boxes with soil, vermiculite and humus (1:1:1, v/v/v) in a greenhouse. After 2 weeks of treatment with Hoagland solution, they were subjected to continuous drought stress for 8 weeks and the plants irrigated with Hoagland solution for 8 weeks were used as a control.
Stomatal aperture assay
The leaves from greenhouse-grown transgenic and WT plants were treated in stomatal opening solution containing 50 mM KCl, 10 mM MES-KOH and 10 mM CaCl2 (pH 6.1) for 3 h and then incubated in stomatal opening solution with 20 μM ABA for 2 h. Randomly selected 80 stomata were measured using a fluorescence microscope (Revolve, Echo, USA).
Measurement of leaf water loss rate
The leaves from greenhouse-grown transgenic and WT plants were placed at the room temperature. These leaves were weighed hourly (0 h-8 h) for calculating the rate of leaf water loss.
Analyses of components and gene expression in response to drought stress
The leaves of the transgenic and WT plants subjected to drought stress for 4 weeks in transplanting boxes were used to analyze the components and gene expression in response to drought stress. The ABA, MeJA and BR contents were analyzed with indirect enzyme linked immune sorbent assay (ELISA) kit (Suzhou Comin Biotechnology Co., Ltd., China). The proline, malondialdehyde (MDA) and H2O2 contents and superoxide dismutase (SOD) activity were measured with Assay Kit (Suzhou Comin Biotechnology Co., Ltd., China). The expression levels of the constitutive photomorphogenesis 9-5α (COP9-5α) gene, a regulator in the phytohormone signalling pathways, and genes encoding SOD, catalase (CAT), and peroxidase (POD) of reactive oxygen species (ROS) scavenging system were analyzed with qRT-PCR (Supplementary Table S1) according to the method of Liu et al. (2014).
Yeast two-hybrid assay
After the transactivation activity assay, the IbEGF without transactivation activity domain was used as the bait to transform Y2H Gold for screening for the sweet potato yeast two-hybrid library following Yeast Protocols Handbook (Clontech). The co-transformed yeast strain was plated onto SD/-Ade/-His/-Leu/-Trp medium containing 3 mM 3-aminotriazole (3-AT) to screen for potential interaction proteins. pGBKT7-53 and pGADT7-T were co-transformed into Y2H Gold as positive control.
BiFC Assay
The coding sequence (CDS) of IbEGF was ligated into pSPYNE-35S vector with the N-terminus of yellow fluorescent protein (nYFP) and the interacted protein gene was constructed into pSPYCE-35S vector with the C-terminus of YFP (cYFP). The two vectors were separately transformed into A. Tumefaciens strain GV3101, and subsequently coinjected into N. benthamiana leaves. After 2 days, the YFP signals were observed (Zhou et al., 2020).
Co-IP assay
The Myc-IbEGF and IbCOP9-5α-GFP vectors were transiently expressed in N. benthamiana leaves. Total proteins were extracted from the leaves with extraction buffer, mixed with Myc magnetic beads (P2118, Beyotime) and then incubated at 4°C for 4 h (Zhang et al., 2020). The agarose was washed at least five times with extraction buffer and boiled in 5× SDS loading buffer for 15 min to separate the proteins from the agarose beads. The proteins were detected using polyclonal anti-Myc (1:10000, BE2011, EASYBIO) and anti-GFP antibodies (1:10000, BE2002, EASYBIO).
Statistical analysis
All experiments were done for three biological replicates. Data were presented as the mean ± SE and analyzed using Student’s t-test (two-tailed analysis). Significance levels at P < 0.05 and P < 0.01 were denoted by ∗ and ∗∗, respectively.
Results
Cloning and sequence analysis of IbEGF and its promoter
The novel IbEGF gene was isolated from sweet potato line Xushu55-2. The 252-bp CDS sequence of IbEGF encoded a polypeptide of 83 aa with a molecular weight of 8.68 kDa and a predicted pI of 10.54. IbEGF consisted of one single exon. The EGF protein contained an EGF_CA domain (Supplementary Figure S1A). This protein showed high sequence identity with EGF proteins from Trema orientale (PON94549.1, 65.06%), Actinidia chiensis var. chinensis (PSS20878.1, 63.10%), Morus natabilis (EXB37025.1, 61.90%), Manihot esculenta (OAY53736.1, 60.92%), Theobroma cacao (EOY30817.1, 60.00%) and Nicotiana attenuate (OIT39415.1, 57.47%) (Supplementary Figure S1B). The 1591-bp promoter region of IbEGF contained several phytohormone or stress-responsive cis-acting regulatory elements, including AuxRE, ABRE, ARE, and LTR (Supplementary Figure S2).
Expression analysis of IbEGF in sweet potato
To study the potential function of IbEGF in sweet potato, its expression in different tissues and treatments of Xushu55-2 was analyzed with qRT-PCR. In the in vitro-grown Xushu55-2 plants, the root tissue showed the highest expression level of IbEGF (Supplementary Figure S3A). For the field-grown Xushu55-2 plants, the expression level of IbEGF was highest in the stem tissue (Supplementary Figure S3B). The expression of IbEGF peaked at 12 h (4.90-, 1.30-, 3.44- and 20.86-fold, respectively) after PEG6000, H2O2, ABA and BR treatments and at 24 h (2.63-fold) after MeJA treatment (Figure 1). These results suggest that IbEGF might be involved in drought, H2O2, ABA, MeJA and BR response pathways.
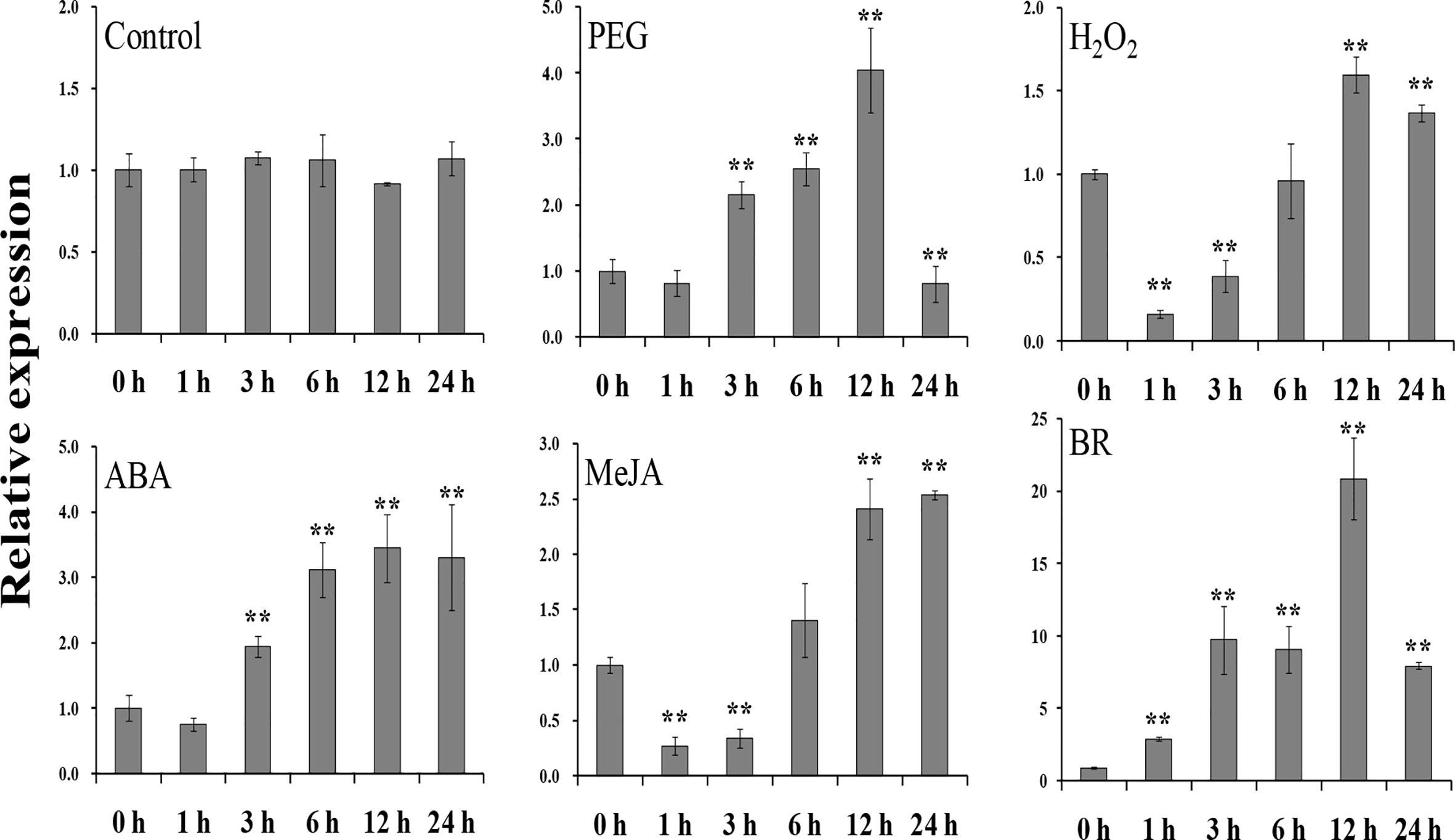
Figure 1 Expression analysis of IbEGF in in vitro-grown Xushu55-2 plants after different time points (h) in response to H2O (control), 30% PEG6000, 100 mM H2O2,100 mM ABA, 100 μM MeJA and 100 nM BR, respectively. The expression level of IbEGF in the plant sampled at 0 h was set to 1. The data are presented as the means ± SEs (n = 3). ** indicates a significant difference from that of the untreated control (0 h) at P<0.01 according to Student’s t-test.
Subcellular localization
Confocal images taken from the leaf epidermal cells of N. benthamiana and the maize protoplasts exhibited that IbEGF-GFP fluorescence was observed in the nucleus and cell membrane (Figure 2). These results indicated that IbEGF was localized to the nucleus and cell membrane.
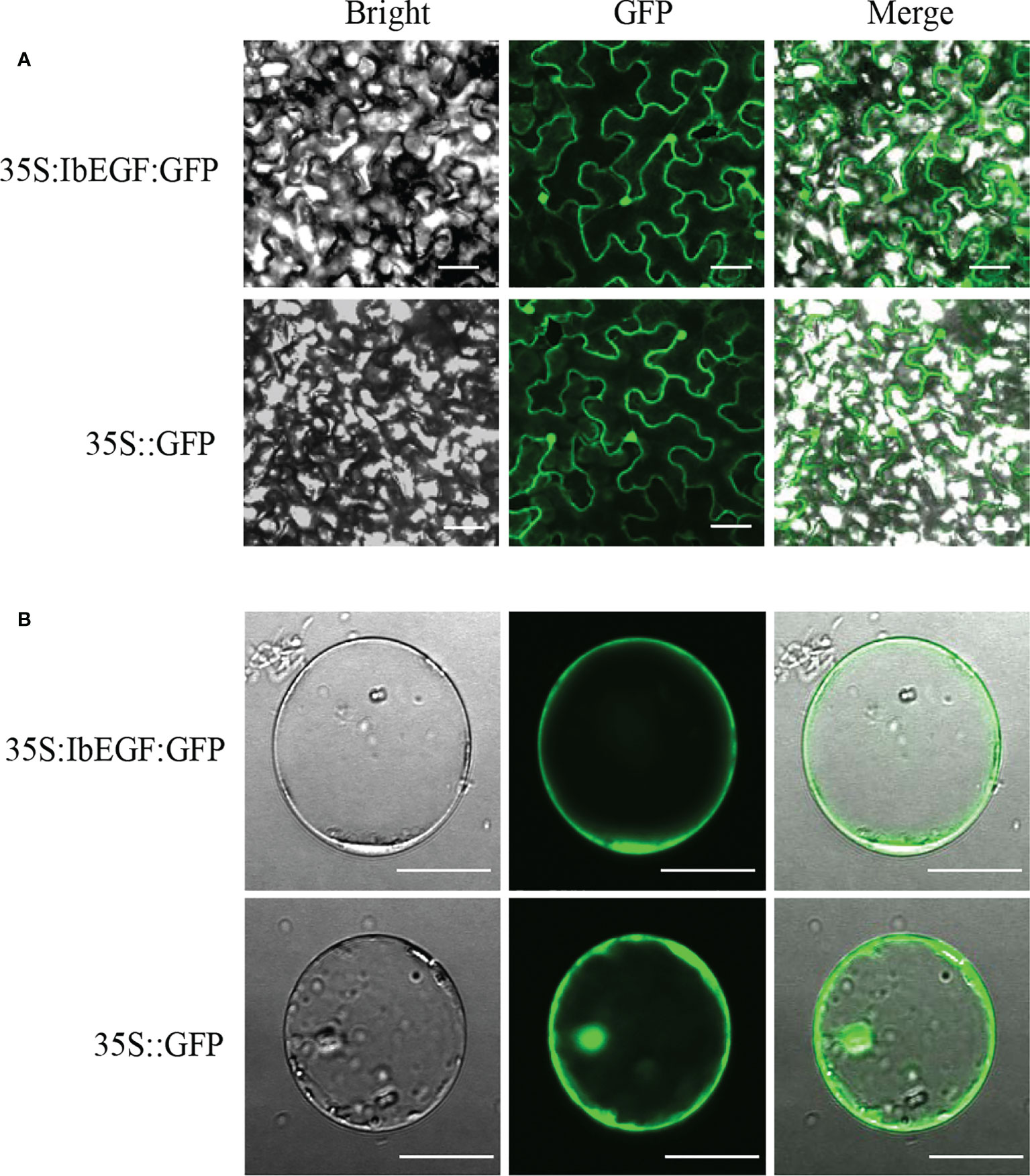
Figure 2 Subcellular localization of IbEGF in tobacco leaf hypodermal cells (A) and maize protoplasts (B). Confocal scanning microscopy images showing the localization of IbEGF-GFP to nucleus and cell membrane. The empty pSuper1300 vector (35S:GFP) was used as a control. Bars = 20 μm.
Transactivation activity
All the yeast cells harbouring pGBKT7-IbEGF, pGBKT7-53 (positive control) or pGBKT7 (negative control) grew well on the SD/-Trp medium (Supplementary Figure S4A). The yeast cells with pGBKT7-IbEGF and negative control did not grow, but the positive control yeast cells showed good growth on medium with X-α-Gal(SD/-Trp/-His/X) (Supplementary Figure S4B). These results demonstrated that IbEGF had no transactivation activity.
Regeneration of the transgenic sweet potato plants
Cell aggregates of Lizixiang (Supplementary Figure S5A) cocultivated with EHA 105 carrying pSuper1300-IbEGF were cultured on the selective MS medium with 2.0 mg L-1 2,4-dichlorophenoxyacetic acid (2,4-D), 100 mg L-1 carbenicillin (Carb) and 10 mg L-1 hygromycin (Hyg). After 4 weeks, the 16 Hyg-resistant embryogenic calluses were obtained from the cocultivated 530 cell aggregates (Supplementary Figure S5B). These Hyg-resistant embryogenic calluses were transferred to MS medium with 1.0 mg L-1 ABA and 100 mg L-1 Carb, and after 4 weeks of transfer, they formed plantlets (Supplementary Figures S5C, D). Forty-four of 95 regenerated plants were proved to be transgenic by PCR analysis, named L1, L2, …, L44, respectively (Supplementary Figure S5E). qRT-PCR analysis revealed that the expression level of IbEGF was significantly increased in most of the transgenic plants compared with that of WT (Supplementary Figure S5F). These transgenic plants were transplanted to pots and showed a survival rate of 100% in a greenhouse (Supplementary Figure S5G).
Enhanced drought tolerance
The three transgenic sweet potato plants, L9, L13 and L26, with the higher expression level of IbEGF, were selected to test their drought tolerance. Under 20% PEG6000 treatment, the transgenic plants exhibited significantly better growth and rooting, and their fresh weight (FW) was significantly increased compared with WT (Figures 3A–C). No difference in growth was observed between the transgenic plants and WT under normal conditions (Figures 3A–C).
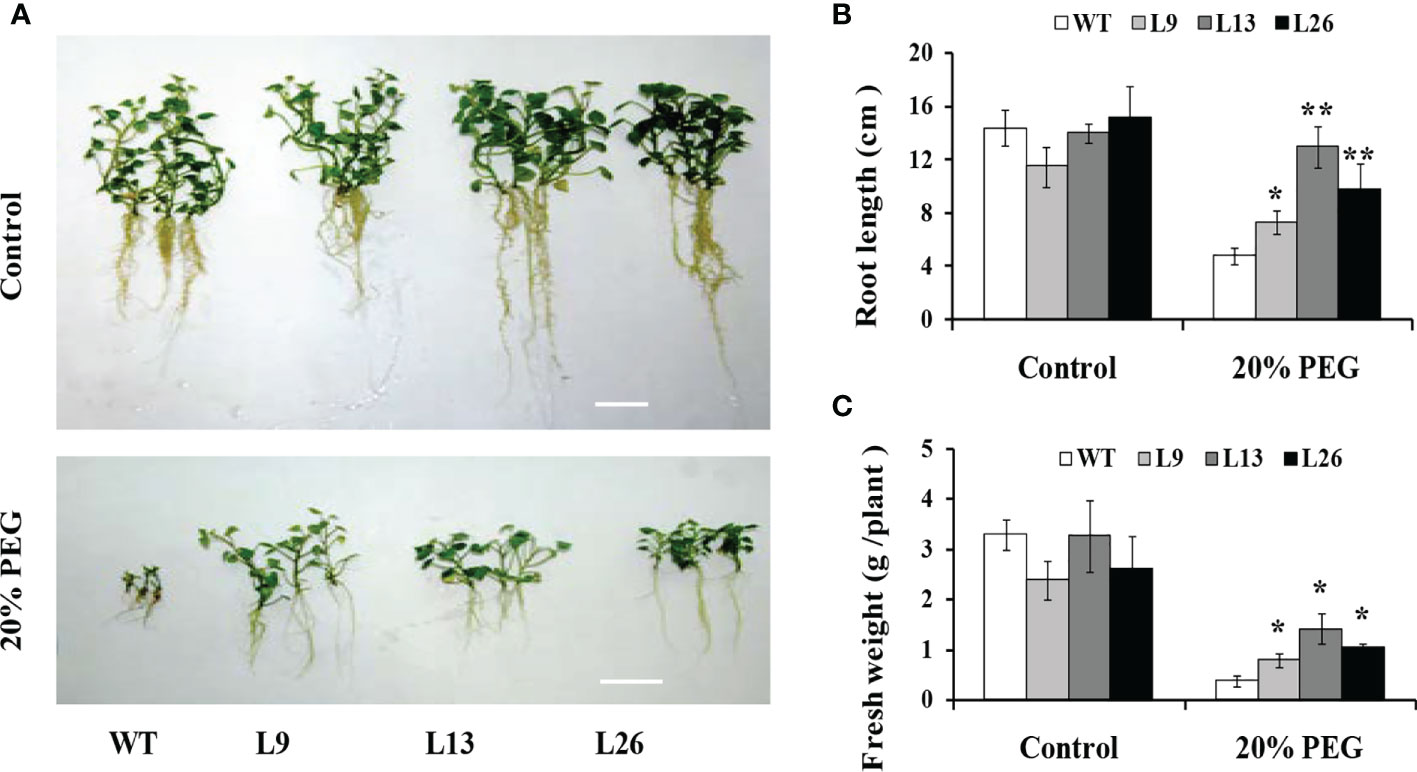
Figure 3 Responses of in vitro-grown transgenic sweet potato plants and WT cultured on MS medium without (control) or with 20% PEG6000 for 4 weeks. (A) Phenotypes. (B) Root length. (C) Fresh weight. The data are presented as the mean ± SEs (n = 3). * and ** indicate significant differences from that of WT at P<0.05 and P<0.01, respectively, according to Student’s t-test.
To further evaluate drought tolerance, the cuttings from field-grown L9, L13, L26 and WT plants were incubated in Hoagland solution with/without 20% PEG6000. As shown in Figures 4A–C, no difference in growth between the transgenic plants and WT was observed in Hoagland solution without PEG6000. However, the growth and rooting of the transgenic plants were significantly better than those of WT in Hoagland solution with PEG6000. Furthermore, the cuttings of the transgenic plants grown in a transplanting box formed new leaves and roots, while WT turned brown to death after drought treatment (Figure 5A). Based on these results, the transgenic sweet potato plants exhibited enhanced drought resistance.
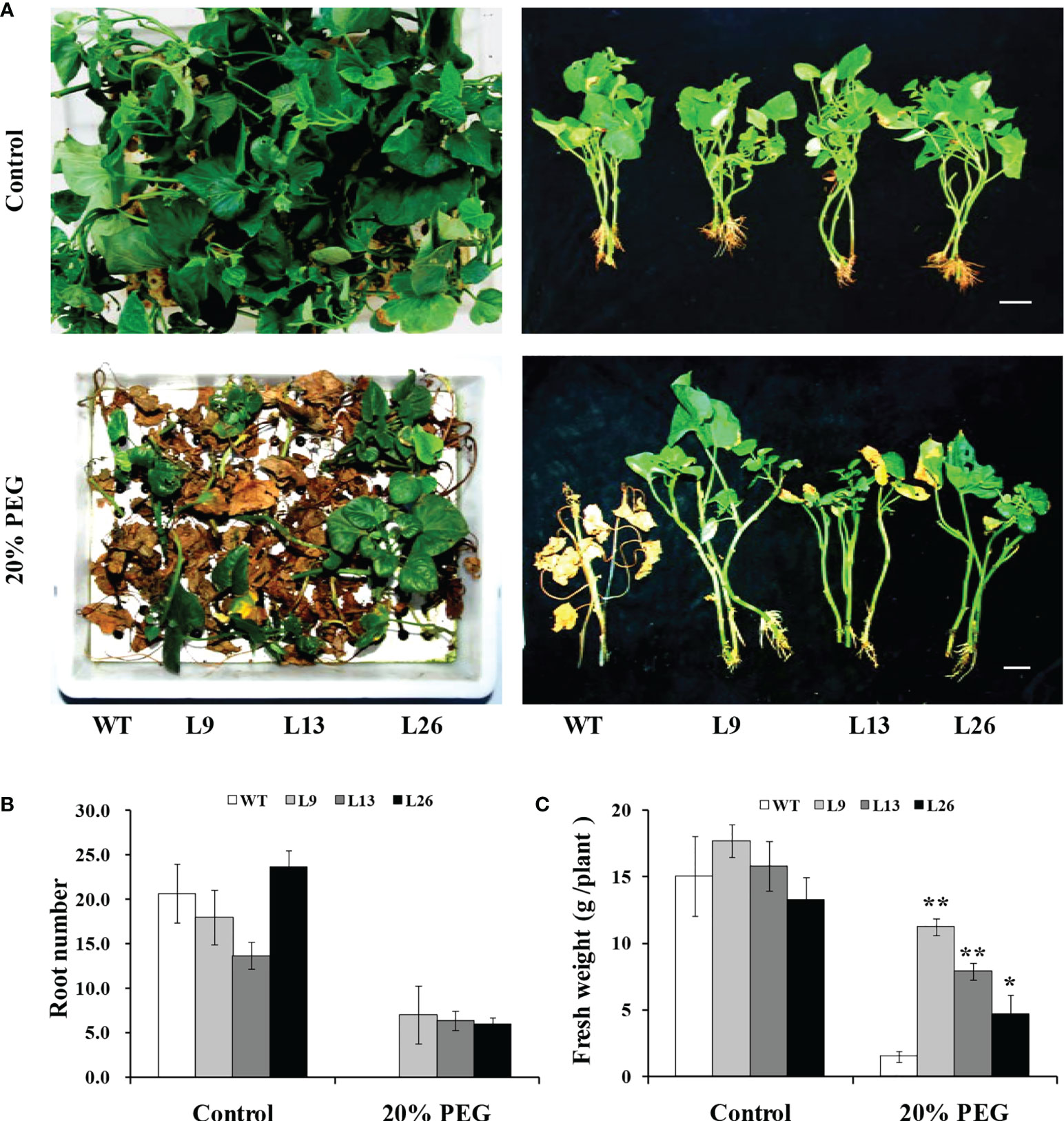
Figure 4 Responses of transgenic sweet potato plants and WT treated in Hoagland solution for 4 weeks (control) or in Hoagland solution with 20% PEG6000 for 2 weeks followed by 2 weeks of Hoagland solution. (A) Phenotypes. (B) Root number. (C) Fresh weight. * and ** indicate significant differences from that of WT at P<0.05 and P<0.01, respectively, according to Student’s t-test.
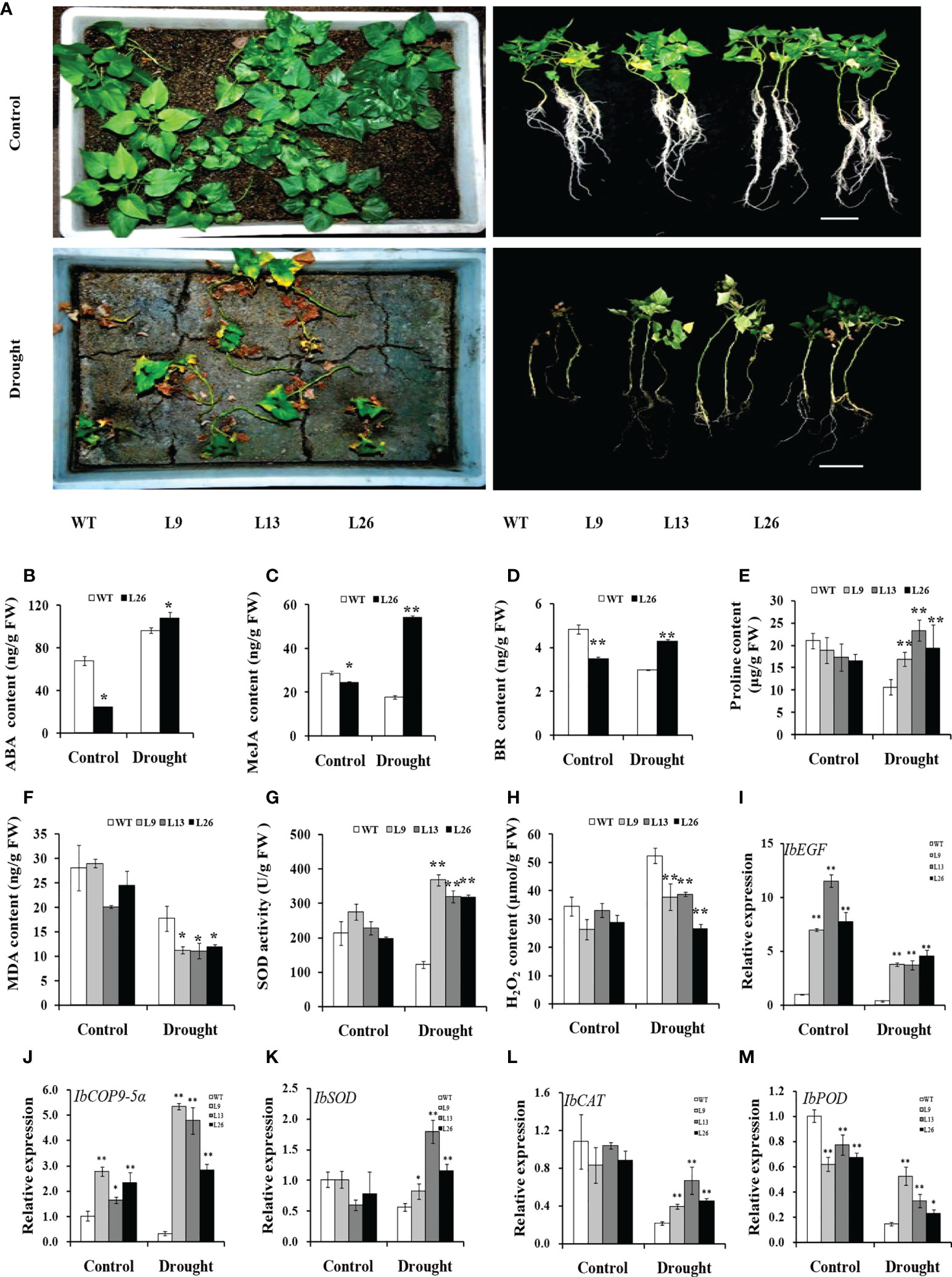
Figure 5 Responses of transgenic sweet potato plants and WT grown in transplanting boxes to drought stress. (A) Phenotypes of plants irrigated with Hoagland solution for 8 weeks (control) or stressed by an 8-week-long drought treatment. (B–H) ABA content, MeJA content, BR content, proline content, MDA content, SOD activity, and H2O2 content in the leaves of plants after 4 weeks of treatment, respectively. (I–M) Transcript levels of IbEGF, IbCOP9-5α, IbSOD, IbCAT, and IbPOD in the leaves of plants after 4 weeks of treatment, respectively. The transcript levels of the genes in WT under normal treatment were set to 1. * and ** indicate significant differences from that of WT at P<0.05 and P<0.01, respectively, according to Student’s t-test.
Underlying mechanism of IbEGF in drought tolerance
To investigate the underlying mechanism of IbEGF in drought tolerance, the components related to drought tolerance were measured. The results showed that the ABA, MeJA, BR and proline contents and SOD activity of the transgenic plants were significantly increased, while their MDA and H2O2 contents were significantly decreased compared with those of WT under drought stress (Figures 5B–H). Further analysis indicated that the expression of IbCOP9-5α, IbSOD, IbCAT and IbPOD was upregulated in the transgenic plants (Figures 5I–M). The transgenic sweet potato plants exhibited reduced ABA-induced stomatal apertures (Figures 6A, B) and reduced leaf water loss rate compared with WT (Figure 6C). Furthermore, Y2H assays showed that IbCOP9-5α was the potential interacting protein with IbEGF (Figure 7A). To further investigate interactions between IbEGF and IbCOP9-5α in plants, BiFC and co-IP assays were performed. The YFP signals were observed in the nucleus of N. benthamiana leaf hypodermal cells (Figures 7B, C). In a co-IP assay, Myc-IbEGF was co-precipitated by anti-Myc antibody using total proteins extracted from N. benthamiana leaves co-expressing IbCOP9-5α-GFP and Myc-IbEGF, but not using total proteins extracted from control leaves expressing IbCOP9-5α-GFP alone (Figure 7C). All the results indicated that IbEGF interacted with IbCOP9-5α.
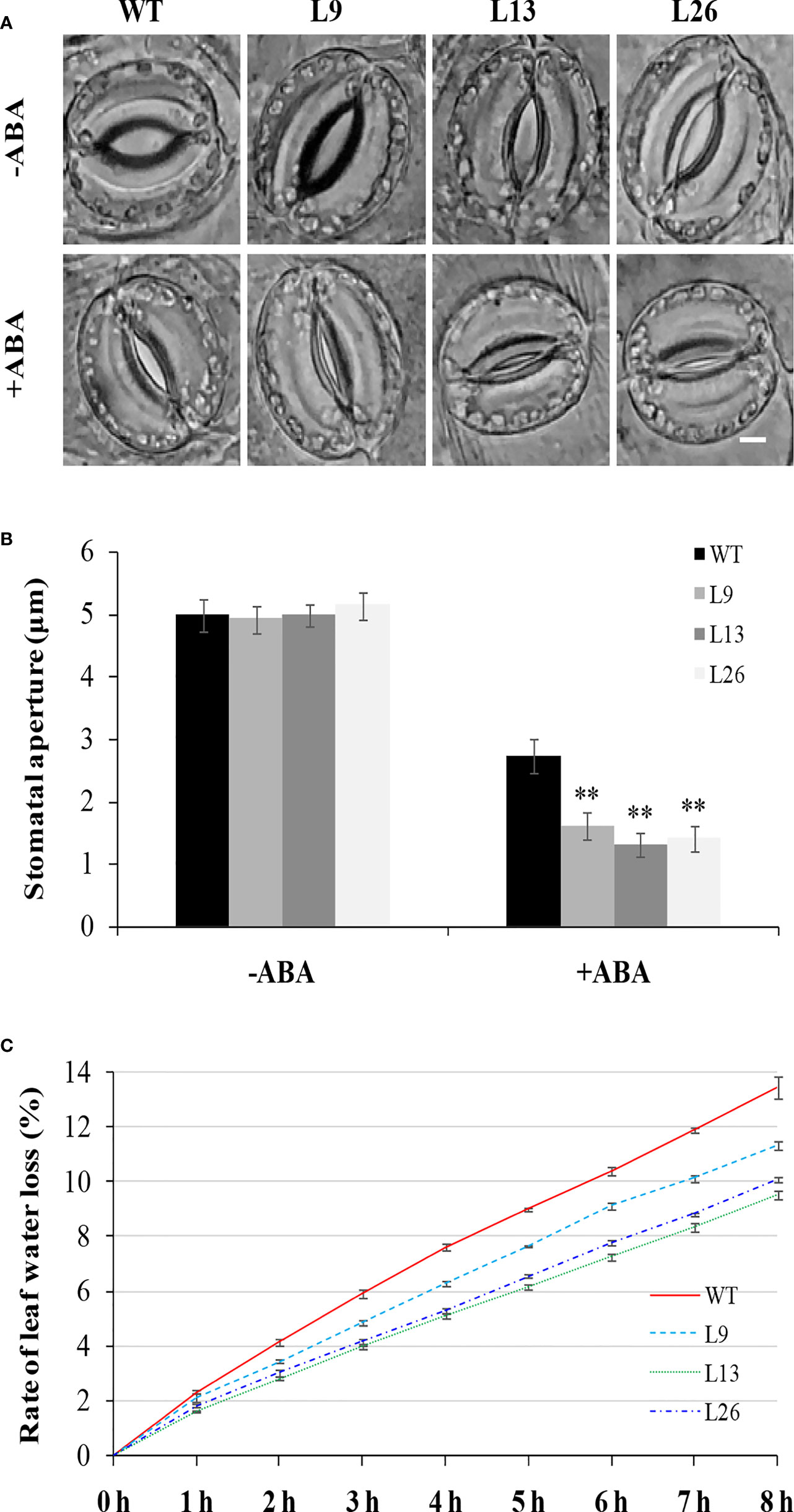
Figure 6 Stomatal aperture and leaf water loss rate of transgenic sweet potato plants and WT. (A, B) Stomatal aperture of greenhouse-grown plants under normal condition (-ABA) and treated with 20 μM ABA for 2 h. Bar = 5 μm. Data are presented as the means ± SD (n = 80). ** indicates significant difference from that of WT at P<0.01, according to Student’s t-test. (C) Leaf water loss rate of greenhouse-grown plants.
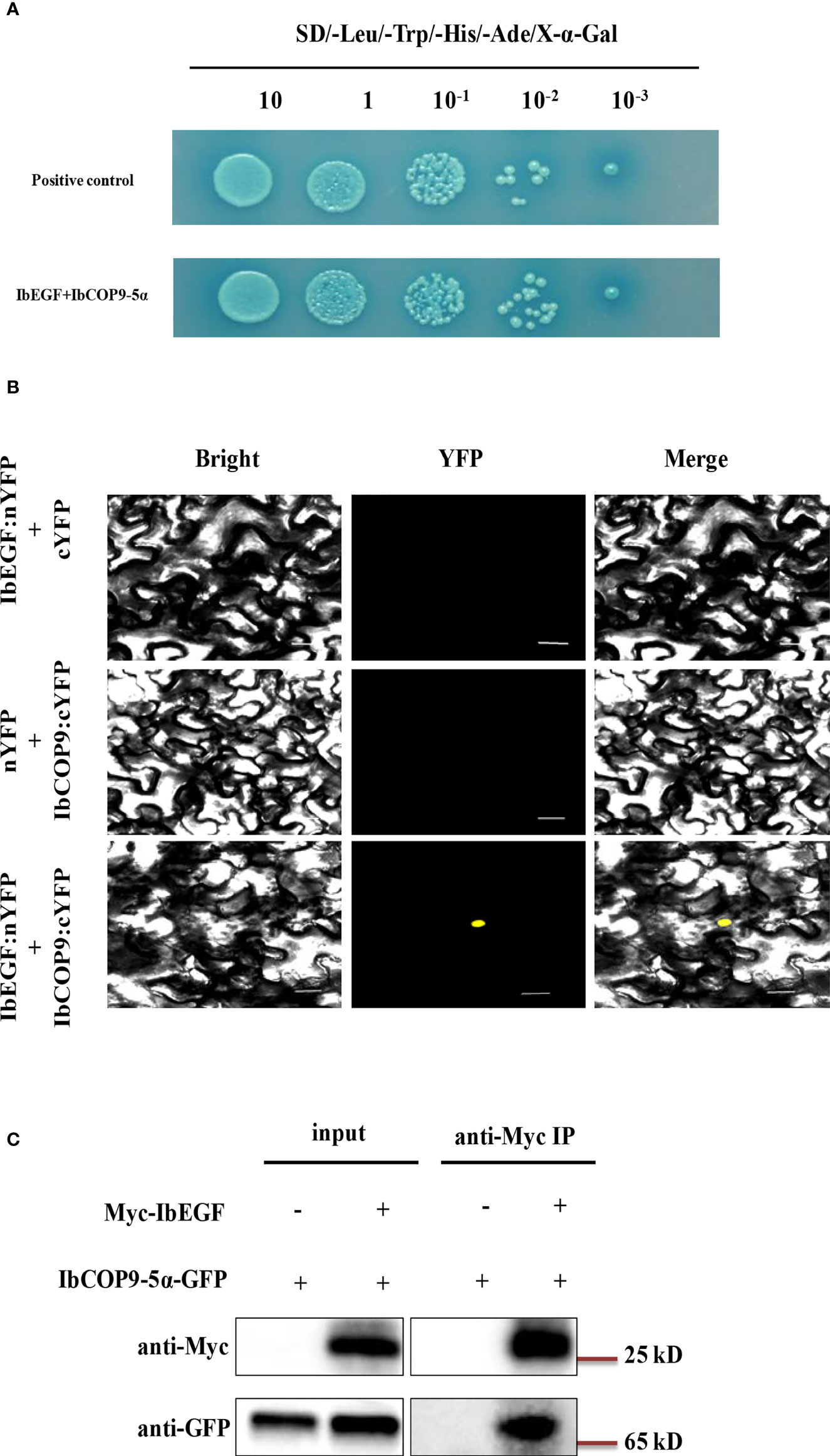
Figure 7 In vivo interaction between IbEGF and IbCOP9-5α. (A) IbEGF/IbCOP9-5α interaction in Y2H Gold cells by Y2H assay. (B) IbEGF/IbCOP9-5α interaction in the tobacco nucleus by BiFC assay. The yellow fluorescent protein (YFP) signals were predominantly localized in the nucleus. Bars = 20 μm. (C) IbEGF/IbCOP9-5α interaction by the co-IP assay. Total proteins from N. benthamiana leaf cells expressing Myc-IbEGF and IbCOP9-5α-GFP were extracted and incubated with anti-Myc magnetic beads. Proteins before (input) and after IP were detected with anti-Myc and anti-GFP antibodies.
Discussion
IbEGF enhances drought tolerance
The sORF-encoded polypeptides play crucial roles in plant defense, growth and development, fertilization and environmental stresses including drought, salt, mechanical wounding, pathogen infection and nutrient imbalance (Lindsey et al., 2002; Ryan et al., 2002; Matsuzaki et al., 2010; Nakaminami et al., 2018; Takahashi et al., 2018; Chen et al., 2020). OsDT11 and OsDSSR1 in rice and CLE25 and CLE9 in Arabidopsis positively regulate responses to drought stress (Li et al., 2017; Cui et al., 2018; Takahashi et al., 2018; Zhang et al., 2019). However, the functions of the EGF protein family with EGF_CA domain in plants are still unclear.
In the present study, the IbEGF gene was isolated from sweet potato. Its ORF encoded a polypeptide of 83 aa (Supplementary Figure S1). This is the first reported sORF with the EGF_CA domain in sweet potato. The expression of IbEGF was significantly upregulated under PEG stress (Figure 1). Its overexpression significantly enhanced drought tolerance of the transgenic sweet potato plants (Figures 3–5). It is thought that IbEGF is a novel sORF gene involved in drought tolerance of sweet potato.
IbEGF positively regulates ABA, JA and BR signalling pathways
Drought causes oxidative stress and metabolic and osmotic damage and inhibits plant growth (Fàbregas and Fernie, 2019). Plants evolve complex regulatory hormonal signalling networks for adapting to drought conditions (Miller et al., 2010). Drought triggers the accumulation of ABA, JA and BR in plant tissues, which leads to drought tolerance (Rohwer and Erwin, 2008; Daszkowska-Golec, 2016; Zhou et al., 2020). ABA emerges as a crucial regulator of the drought response (Li et al., 2021). JA plays an important role as a signal molecule that induces tolerance mechanisms under drought stress (Rohwer and Erwin, 2008). BR regulates adaptations to cope with drought stress (Planas-Riverola et al., 2019).
As a new class of plant hormones, the sORF-encoded polypeptides play an essential role in responses to changes in the environments by modulating other plant hormones (Ryan and Pearce, 2001; Chen et al., 2020; Maurel et al., 2021). It was reported that OsDT11 in rice and CLE25 and CLE9 in Arabidopsis reduced stomatal density or aperture and enhanced drought tolerance by ABA signalling (Li et al., 2017; Takahashi et al., 2018; Zhang et al., 2019). There was crosstalk between peptide hormone BoPep4 and ABA signalling pathways under salinity stress (Wang et al., 2022a). The serine rich endogenous peptide (SCOOP) increased defense against a generalist herbivore by modulating the JA pathway in Arabidopsis (Stahl et al., 2022).
COP9 signalosome is a highly conserved transcriptional regulator (Chamovitz, 2009; Jin et al., 2014). Several findings have showed that COP9 signalosome regulates plant response to abiotic stresses such as oxidative stress, salt stress and high-temperature stress through the ABA, JA, gibberellic acid (GA), auxin and ROS signalling pathways (Nahlik et al., 2010; Singh and Chamovitz, 2019; Wang et al., 2022b). COP9-5α (CNS5A) is an essential player in the regulation of plant development and stress (Singh et al., 2021). In Arabidopsis, CSN5A regulated seed germination and salt tolerance by ABA signalling (Jin et al., 2018; Zhou et al., 2021). IbGATA24 interacting with IbCOP9-5α positively regulated drought and salt resistance by ABA and ROS signalling in transgenic Arabidopsis (Zhu et al., 2022).
Our results showed that the IbEGF gene was upregulated after treatments of ABA, MeJA and BR, respectively (Figure 1). The IbEGF-overexpressing sweet potato plants exhibited better growth and more accumulation of ABA, MeJA and BR under drought stress (Figures 3–5). Further analysis indicated that the expression level of IbCOP9-5α was increased in the transgenic sweet potato plants under drought stress (Figure 5). Y2H, BiFC and co-IP assays demonstrated that IbEGF interacted with COP9-5α (Figures 7). Our previous study indicated that IbCOP9-5α is a positive regulator of the response to drought stress and the IbCOP9-5α-overexpressing Arabidopsis plants exhibited significantly enhanced drought tolerance (Zhu et al., 2022). Therefore, it is thought that IbEGF with the help of COP9-5α could positively regulate the ABA, JA and BR signalling pathways under drought stress, which results in enhanced drought tolerance (Figure 8).
IbEGF Positively Modulates Proline Accumulation and ROS-Scavenging System
The overproduction of ROS in plants causes damage to proteins, lipids, carbohydrates and DNA under drought, salinity and heat stresses (Gill and Tuteja, 2010; Choudhury et al., 2017; Waszczak et al., 2018). More proline accumulation can protect plants from drought stress and ROS damage (Phang et al., 2010; Liu et al., 2014; Adamipour et al., 2020). In rice, OsDSSR1 was induced by drought, salinity, ABA and H2O2 treatments and its overexpression enhanced drought tolerance by increasing the accumulation of free proline and soluble sugars and further promoting OsSodCc2 and OscAPX expression and SOD and ascorbate peroxidase (APX) activities (Cui et al., 2018).
In our study, overexpression of IbEGF increased the proline content and SOD activity and reduced the H2O2 level in transgenic sweet potato under drought stress (Figure 5). IbSOD, IbCAT and IbPOD were also upregulated (Figure 5). It is suggested that overexpression of IbEGF enhances drought tolerance by positively modulating proline accumulation and ROS-scavenging system in transgenic sweet potato (Figure 8). Based on all the above results, we propose that IbEGF interacting with IbCOP9-5α positively regulates the hormone signalling pathways and proline biosynthesis and further activates the ROS scavenging system, which lead to enhanced drought tolerance in transgenic sweet potato (Figure 8).
Conclusion
A novel sORF gene, IbEGF, was isolated and characterized from sweet potato. Its overexpression in sweet potato enhanced tolerance to drought stress, increased contents of ABA, MeJA, BR and proline and activity of SOD, decreased levels of MDA and H2O2 and upregulated expression of IbCOP9-5α, IbSOD, IbCAT and IbPOD. It is suggested that IbEGF interacting with IbCOP9-5α enhances drought tolerance by positively regulating the hormone signalling pathways, increasing proline accumulation and further activating the ROS scavenging system in transgenic sweet potato.
Data availability statement
The original contributions presented in the study are included in the article/Supplementary Material. Further inquiries can be directed to the corresponding author.
Author contributions
QL and YZ conceived and designed the experiments. YZ performed the experiments. YZ and HoZ analyzed the data. QL, SX, ZW, SH, HuZ, SG and NZ contributed reagents, materials and analysis tools. QL and YZ wrote the paper. All authors contributed to the article and approved the submitted version.
Funding
This study was supported by the National Key R&D Program of China (grant number 2018YFD1000704/2018YFD1000700) and the earmarked fund for CARS-10-Sweetpotato.
Conflict of interest
The authors declare that the research was conducted in the absence of any commercial or financial relationships that could be construed as a potential conflict of interest.
Publisher’s note
All claims expressed in this article are solely those of the authors and do not necessarily represent those of their affiliated organizations, or those of the publisher, the editors and the reviewers. Any product that may be evaluated in this article, or claim that may be made by its manufacturer, is not guaranteed or endorsed by the publisher.
Supplementary material
The Supplementary Material for this article can be found online at: https://www.frontiersin.org/articles/10.3389/fpls.2022.965069/full#supplementary-material
References
Adamipour, N., Khosh-Khui, M., Salehi, H., Razi, H., Karami, A., Moghadam, A. (2020). Metabolic and genes expression analyses involved in proline metabolism of two rose species under drought stress. Plant Physiol. Biochem. 155, 105–113. doi: 10.1016/j.plaphy.2020.07.028
Basrai, M. A., Hieter, P., Boeke, J. D. (1997). Small open reading frames: beautiful needles in the haystack. Genome Res. 7, 768–771. doi: 10.1101/gr.7.8.768
Ceccarelli, S., Grando, S., Maatougui, M., Michael, M., Slash, M., Haghparast, R., et al. (2010). Plant breeding and climate changes. J. Agric. Sci. 148, 627–637. doi: 10.1017/S0021859610000651
Chamovitz, D. A. (2009). Revisiting the COP9 signalosome as a transcriptional regulator. EMBO Rep. 10, 352–358. doi: 10.1038/embor.2009.33
Chen, Y. L., Fan, K. T., Hung, S. C., Chen, Y. R. (2020). The role of peptides cleaved from protein precursors in eliciting plant stress reactions. New Phytol. 225, 2267–2282. doi: 10.1111/nph.16241
Choudhury, F. K., Rivero, R. M., Blumwald, E., Mittler, R. (2017). Reactive oxygen species, abiotic stress and stress combination. Plant J. 90, 856–867. doi: 10.1111/tpj.13299
Cohen, S. (1983). The epidermal growth factor (EGF). Cancer 51, 1787–1791. doi: 10.1002/1097-0142(19830515)51:10<1787::aid-cncr2820511004>3.0.co;2-a
Cui, Y., Li, M., Yin, X., Song, S., Xu, G., Wang, M., et al. (2018). OsDSSR1, a novel small peptide, enhances drought tolerance in transgenic rice. Plant Sci. 270, 85–96. doi: 10.1016/j.plantsci.2018.02.015
Daszkowska-Golec, A. (2016). The role of abscisic acid in drought stress: how ABA helps plants to cope with drought stress. Drought Stress Tolerance Plants 2, 123–151. doi: 10.1007/978-3-319-32423-4_5
Fàbregas, N., Fernie, A. R. (2019). The metabolic response to drought. J. Exp. Bot. 70, 1077–1085. doi: 10.1093/jxb/ery437
Frith, M. C., Forrest, A. R., Nourbakhsh, E., Pang, K. C., Kai, C., Kawai, J., et al. (2006). The abundance of short proteins in the mammalian proteome. PloS Genet. 2, e52. doi: 10.1371/journal.pgen.0020052
Fujita, Y., Fujita, M., Satoh, R., Maruyama, K., Parvez, M. M., Seki, M., et al. (2005). AREB1 is a transcription activator of novel ABRE-dependent ABA signaling that enhances drought stress tolerance in Arabidopsis. Plant Cell 17, 3470–3488. doi: 10.1105/tpc.105.035659
Gill, S. S., Tuteja, N. (2010). Reactive oxygen species and antioxidant machinery in abiotic stress tolerance in crop plants. Plant Physiol. Biochem. 48, 909–930. doi: 10.1016/j.plaphy.2010.08.016
Hanada, K., Higuchi-Takeuchi, M., Okamoto, M., Yoshizumi, T., Shimizu, M., Nakaminami, K., et al. (2013). Small open reading frames associated with morphogenesis are hidden in plant genomes. Proc. Natl. Acad. Sci. U.S.A. 110, 2395–2400. doi: 10.1073/pnas.1213958110
Hanada, K., Zhang, X., Borevitz, J. O., Li, W. H., Shiu, S. H. (2007). A large number of novel coding small open reading frames in the intergenic regions of the Arabidopsis thaliana genome are transcribed and/or under purifying selection. Genome Res. 17, 632–640. doi: 10.1101/gr.5836207
Hara, K., Kajita, R., Torii, K. U., Bergmann, D. C., Kakimoto, T. (2007). The secretory peptide gene EPF1 enforces the stomatal one-cell-spacing rule. Genes Dev. 21, 1720–1725. doi: 10.1101/gad.1550707
Huffaker, A., Pearce, G., Ryan, C. A. (2006). An endogenous peptide signal in Arabidopsis activates components of the innate immune response. Proc. Natl. Acad. Sci. U.S.A. 103, 10098–10103. doi: 10.1073/pnas.0603727103
Jewaria, P. K., Hara, T., Tanaka, H., Kondo, T., Betsuyaku, S., Sawa, S., et al. (2013). Differential effects of the peptides stomagen, EPF1 and EPF2 on activation of MAP kinase MPK6 and the SPCH protein level. Plant Cell Physiol. 54, 1253–1262. doi: 10.1093/pcp/pct076
Jin, D., Li, B., Deng, X. W., Wei, N. (2014). Plant COP9 signalosome subunit 5, CSN5. Plant Sci. 224, 54–61. doi: 10.1016/j.plantsci.2014.04.001
Jin, D., Wu, M., Li, B., Bucker, B., Keil, P., Zhang, S., et al. (2018). The COP9 signalosome regulates seed germination by facilitating protein degradation of RGL2 and ABI5. PloS Genet. 14, e1007237. doi: 10.1371/journal.pgen.1007237
Jin, Y., Zheng, H. Y., Jin, X. J., Zhang, Y. X., Ren, C. Y. (2016). Effect of ABA, SA and JA on soybean growth under drought stress and re-watering. Soybean Sci. 35, 958–963. doi: 10.11861/j.issn.1000-9841
Kastenmayer, J. P., Ni, L., Chu, A., Kitchen, L. E., Au, W. C., Yang, H., et al. (2006). Functional genomics of genes with small open reading frames (sORFs) in. S. cerevisiae. Genome Res. 16, 365–373. doi: 10.1101/gr.4355406
Kawamoto, N., Carpio, D. P. D., Hofmann, A., Mizuta, Y., Kurihara, D., Higashiyama, T., et al. (2020). A peptide pair coordinates regular ovule initiation patterns with seed number and fruit size. Curr. Biol. 30, 4352–4361. doi: 10.1016/j.cub.2020.08.050
Khitun, A., Ness, T. J., Slavoff, S. A. (2019). Small open reading frames and cellular stress responses. Mol. Omics 15, 108–116. doi: 10.1039/c8mo00283e
Kim, M. J., Jeon, B. W., Oh, E., Seo, P. J., Kim, J. (2021). Peptide pignaling during plant reproduction. Trends Plant Sci. 26, 822–835. doi: 10.1016/j.tplants.2021.02.008
Kucukoglu, M., Chaabouni, S., Zheng, B., Mahonen, A. P., Helariutta, Y., Nilsson, O. (2020). Peptide encoding populus CLV3/ESR-RELATED 47 (PttCLE47) promotes cambial development and secondary xylem formation in hybrid aspen. New Phytol. 226, 75–85. doi: 10.1111/nph.16331
Li, X., Han, H., Chen, M., Yang, W., Liu, L., Li, N., et al. (2017). Overexpression of OsDT11, which encodes a novel cysteine-rich peptide, enhances drought tolerance and increases ABA concentration in rice. Plant Mol. Biol. 93, 21–34. doi: 10.1007/s11103-016-0544-x
Li, Z., Liu, D., Xia, Y., Li, Z., Niu, N., Ma, S., et al. (2019). Identification and functional analysis of the CLAVATA3/EMBRYO SURROUNDING REGION (CLE) gene family in wheat. Int. J. Mol. Sci. 20, 4319. doi: 10.3390/ijms20174319
Lindsey, K., Casson, S., Chilley, P. (2002). Peptides: new signalling molecules in plants. Trends Plant Sci. 7, 78–83. doi: 10.1016/s1360-1385(01)02194-x
Liu, D. G., He, S. Z., Zhai, H., Wang, L. J., Zhao, Y., Wang, B., et al. (2014). Overexpression of IbP5CR enhances salt tolerance in transgenic sweetpotato. Plant Cell Tiss. Organ Cult. 117, 1–16. doi: 10.1007/s11240-013-0415-y
Liu, Q. C., Zhai, H., Wang, Y., Zhang, D. P. (2001). Efficient plant regeneration from embryogenic suspension cultures of sweetpotato. In vitro cell. dev. biol. Plant 37, 564–567. doi: 10.1007/s11627-001-0098-7
Li, C., Yan, C., Sun, Q., Wang, J., Yuan, C., Mou, Y., et al. (2021). The bHLH transcription factor AhbHLH112 improves the drought tolerance of peanut. BMC Plant Biol. 21, 1–12. doi: 10.1186/s12870-021-03318-6
Matsuzaki, Y., Ogawa-Ohnishi, M., Mori, A., Matsubayashi, Y. (2010). Secreted peptide signals required for maintenance of root stem cell niche in Arabidopsis. Science 329, 1065–1067. doi: 10.1126/science.1191132
Maurel, C., Tournaire-Roux, C., Verdoucq, L., Santoni, V. (2021). Hormonal and environmental signaling pathways target membrane water transport. Plant Physiol. 187, 2056–2070. doi: 10.1093/plphys/kiab373
Miller, G., Suzuki, N., Ciftci-Yilmaz, S., Mittler, R. (2010). Reactive oxygen species homeostasis and signalling during drought and salinity stresses. Plant Cell Environ. 33, 453–467. doi: 10.1111/j.1365-3040.2009.02041.x
Nahlik, K., Dumkow, M., Bayram, O., Helmstaedt, K., Busch, S., Valerius, O., et al. (2010). The COP9 signalosome mediates transcriptional and metabolic response to hormones, oxidative stress protection and cell wall rearrangement during fungal development. Mol. Microbiol. 78, 964–979. doi: 10.1111/j.1365-2958.2010.07384.x
Nakaminami, K., Okamoto, M., Higuchi-Takeuchi, M., Yoshizumi, T., Yamaguchi, Y., Fukao, Y., et al. (2018). AtPep3 is a hormone-like peptide that plays a role in the salinity stress tolerance of plants. Proc. Natl. Acad. Sci. U.S.A. 115, 5810–5815. doi: 10.1073/pnas.1719491115
Pascarelli, S., Merzhakupova, D., Uechi, G. I., Laurino, P. (2021). Binding of single-mutant epidermal growth factor (EGF) ligands alters the stability of the EGF receptor dimer and promotes growth signaling. J. Biol. Chem. 297, 100872. doi: 10.1016/j.jbc.2021.100872
Pearce, G., Moura, D. S., Stratmann, J., Ryan, C. J. (2001). RALF, a 5-kDa ubiquitous polypeptide in plants, arrests root growth and development. Proc. Natl. Acad. Sci. U.S.A. 98, 12843–12847. doi: 10.1073/pnas.201416998
Pearce, G., Strydom, D., Johnson, S., Ryan, C. A. (1991). A polypeptide from tomato leaves induces wound-inducible proteinase inhibitor proteins. Science 253, 895–897. doi: 10.1126/science.253.5022.895
Peterson, T. J., Saft, M., Peel, M. C., John, A. (2021). Watersheds may not recover from drought. Science 372, 745–749. doi: 10.1126/science.abd5085
Phang, J. M., Liu, W., Zabirnyk, O. (2010). Proline metabolism and microenvironmental stress. Annu. Rev. Nutr. 30, 441–463. doi: 10.1146/annurev.nutr.012809.104638
Planas-Riverola, A., Gupta, A., Betegon-Putze, I., Bosch, N., Ibanes, M., CanoDelgado, A. I. (2019). Brassinosteroid signaling in plant development and adaptation to stress. Development 146, dev151894. doi: 10.1242/dev.151894
Plata-Salaman, C. R. (1991). Epidermal growth factor and the nervous system. Peptides 12, 653–663. doi: 10.1016/0196-9781(91)90115-6
Poncini, L., Wyrsch, I., Denervaud, T. V., Vorley, T., Boller, T., Geldner, N., et al. (2017). In roots of Arabidopsis thaliana, the damage-associated molecular pattern AtPep1 is a stronger elicitor of immune signalling than flg22 or the chitin heptamer. PloS One 12, e185808. doi: 10.1371/journal.pone.0185808
Rao, Z., Handford, P. A., Knott, V., Mayhew, M., Brownlee, G. G., Stuart, D. (1995). Crystallization of a calcium-binding EGF-like domain. Acta Crystallogr. D. Biol. Crystallogr. 51, 402–403. doi: 10.1107/S0907444994009881
Reichardt, S., Piepho, H. P., Stintzi, A., Schaller, A. (2020). Peptide signaling for drought-induced tomato flower drop. Science 367, 1482–1485. doi: 10.1126/science.aaz5641
Ren, Z. T., He, S. Z., Zhou, Y. Y., Zhao, N., Jiang, T., Zhai, H., et al. (2020). A sucrose non-fermenting-1-related protein kinase-1 gene, IbSnRK1, confers salt, drought and cold tolerance in sweet potato. Crop J. 8, 905–917. doi: 10.1016/j.cj.2020.04.010
Ren, Y. X., Ma, J. X., Zhao, F., An, J. B., Geng, Y. X., Liu, L. Y. (2018). Effects of curcumin on epidermal growth factor in proliferative vitreoretinopathy. Cell Physiol. Biochem. 5, 2136–2146. doi: 10.1159/000491525
Rogers, S. O., Bendich, A. J. (1985). Extraction of DNA from milligram amounts of fresh, herbarium and mummified plant tissues. Plant Mol. Biol. 5, 69–76. doi: 10.1007/BF00020088
Rohwer, C. L., Erwin, J. E. (2008). Horticultural applications of jasmonates. J. Hortic. Sci. Biotechnol. 83, 283–304. doi: 10.1016/j.biotechadv.2013.09.009
Ryan, C. A., Pearce, G. (2001). Polypeptide hormones. Plant Physiol. 125, 65–68. doi: 10.1104/pp.125.1.65
Ryan, C. A., Pearce, G., Scheer, J., Moura, D. S. (2002). Polypeptide hormones. Plant Cell 14 Suppl, S251–S264. doi: 10.1105/tpc.010484
Shinozaki, K., Yamaguchi-Shinozaki, K. (2007). Gene networks involved in drought stress response and tolerance. J. Exp. Biol. 58, 221–227. doi: 10.1093/jxb/erl164
Singh, A. K., Chamovitz, D. A. (2019). Role of COP9 signalosome subunits in the environmental and hormonal balance of plant. Biomolecules 9, 224. doi: 10.3390/biom9060224
Singh, A. K., Dhanapal, S., Finkelshtein, A., Chamovitz, D. A. (2021). CSN5A subunit of COP9 signalosome is required for resetting transcriptional stress memory after recurrent heat stress in Arabidopsis. Biomolecules 11, 668. doi: 10.3390/biom11050668
Song, X. F., Hou, X. L., Liu, C. M. (2021). CLE peptides: critical regulators for stem cell maintenance in plants. Planta 255, 5. doi: 10.1007/s00425-021-03791-1
Stahl, E., Fernandez, M. A., Glauser, G., Guillou, M. C., Aubourg, S., Renou, J. P., et al. (2022). The MIK2/SCOOP signaling system contributes to Arabidopsis resistance against herbivory by modulating jasmonate and indole glucosinolate biosynthesis. Front. Plant Sci. 13. doi: 10.3389/fpls.2022.852808
Stenflo, J., Stenberg, Y., Muranyi, A. (2000). Calcium-binding EGF-like modules in coagulation proteinases: Function of the calcium ion in module interactions. Biochim. Biophys. Acta 1477, 51–63. doi: 10.1016/s0167-4838(99)00262-9
Takahashi, F., Suzuki, T., Osakabe, Y., Betsuyaku, S., Kondo, Y., Dohmae, N., et al. (2018). A small peptide modulates stomatal control via abscisic acid in long-distance signalling. Nature 556, 235–238. doi: 10.1038/s41586-018-0009-2
Vargas, L., Santa, B. A., Mota, F. J., de Carvalho, T. G., Rojas, C. A., Vaneechoutte, D., et al. (2014). Drought tolerance conferred to sugarcane by association with Gluconacetobacter diazotrophicus: a transcriptomic view of hormone pathways. PloS One 9, e114744. doi: 10.1371/journal.pone.0114744
Wang, A., Guo, J., Wang, S., Zhang, Y., Lu, F., Duan, J., et al. (2022a). BoPEP4, a c-terminally encoded plant elicitor peptide from broccoli, plays a role in salinity stress tolerance. Int. J. Mol. Sci. 23, 3090. doi: 10.3390/ijms23063090
Wang, D., Musazade, E., Wang, H., Liu, J., Zhang, C., Liu, J., et al. (2022b). Regulatory mechanism of the constitutive photomorphogenesis 9 signalosome complex in response to abiotic stress in plants. J. Agric. Food Chem. 70, 2777–2788. doi: 10.1021/acs.jafc.1c07224
Waszczak, C., Carmody, M., Kangasjarvi, J. (2018). Reactive oxygen species in plant signaling. Annu. Rev. Plant Biol. 69, 209–236. doi: 10.1146/annurev-arplant-042817-040322
Xu, Y., Burgess, P., Huang, B. (2017). Transcriptional regulation of hormone-synthesis and signaling pathways by overexpressing cytokinin-synthesis contributes to improved drought tolerance in creeping bentgrass. Physiol. Plant 161, 235–256. doi: 10.1111/ppl.12588
Zeng, S. M., Lo, E., Hazelton, B. J., Morales, M. F., Torii, K. U. (2020). Effective range of non-cell autonomous activator and inhibitor peptides specifying plant stomatal patterning. Development 147, 192237. doi: 10.1242/dev.192237
Zhang, L., Shi, X., Zhang, Y., Wang, J., Yang, J., Ishida, T., et al. (2019). CLE9 peptide-induced stomatal closure is mediated by abscisic acid, hydrogen peroxide, and nitric oxide in Arabidopsis thaliana. Plant Cell Environ. 42, 1033–1044. doi: 10.1111/pce.13475
Zhang, H., Zhang, Q., Zhai, H., Gao, S. P., Yang, L., Wang, Z., et al. (2020). IbBBX24 promotes the jasmonic acid pathway and enhances fusarium wilt resistance in sweet potato. Plant Cell 32, 1102–1123. doi: 10.1105/tpc.19.00641
Zhou, Y., Li, X. H., Guo, Q. H., Liu, P., Li, Y., Wu, C. A. (2021). Salt responsive alternative splicing of a RING finger E3 ligase modulates the salt stress tolerance by fine-tuning the balance of COP9 signalosome subunit 5A. PloS Genet. 17, e1009898. doi: 10.1371/journal.pgen.1009898
Zhou, Y. Y., Zhai, H., He, S. Z., Zhu, H., Gao, S. P., Xing, S. H., et al. (2020). The sweetpotato BTB-TAZ protein gene, IbBT4, enhances drought tolerance in transgenic Arabidopsis. Front. Plant Sci. 11. doi: 10.3389/fpls.2020.00877
Zhu, J. K. (2016). Abiotic stress signaling and responses in plants. Cell 167, 313–324. doi: 10.1016/j.cell.2016.08.029
Zhu, H., Zhai, H., He, S. Z., Zhang, H., Gao, S. P., Zhao, N., et al. (2022). A novel sweetpotato GATA transcription factor, IbGATA24, interacting with IbCOP9-5a positively regulates drought and salt tolerance. Environ. Exp. Bot. 194, 104735. doi: 10.1016/j.envexpbot.2021.104735
Keywords: sweet potato, sORF, IbEGF, drought tolerance, phytohormone
Citation: Zhou Y, Zhai H, Xing S, Wei Z, He S, Zhang H, Gao S, Zhao N and Liu Q (2022) A novel small open reading frame gene, IbEGF, enhances drought tolerance in transgenic sweet potato. Front. Plant Sci. 13:965069. doi: 10.3389/fpls.2022.965069
Received: 09 June 2022; Accepted: 10 October 2022;
Published: 31 October 2022.
Edited by:
Bhumi Nath Tripathi, Indira Gandhi National Tribal University, IndiaCopyright © 2022 Zhou, Zhai, Xing, Wei, He, Zhang, Gao, Zhao and Liu. This is an open-access article distributed under the terms of the Creative Commons Attribution License (CC BY). The use, distribution or reproduction in other forums is permitted, provided the original author(s) and the copyright owner(s) are credited and that the original publication in this journal is cited, in accordance with accepted academic practice. No use, distribution or reproduction is permitted which does not comply with these terms.
*Correspondence: Qingchang Liu, bGl1cWNAY2F1LmVkdS5jbg==