- 1National Engineering Laboratory for Applied Technology of Forestry and Ecology in South China, Central South University of Forestry and Technology, Changsha, China
- 2College of Life Science and Technology, Central South University of Forestry and Technology, Changsha, China
- 3Institute of Soil and Water Conservation, Northwest A&F University, Xianyang, Shaanxi, China
Subtropical forests provide diverse ecosystem services to human society. However, how subtropical tree species respond to climate change is still unclear. Using a dendrochronological method, we studied the radial growth patterns and species-specific responses of four main tree species in subtropical China to recent warming and drought. Results showed that the long-term drought caused by global warming and reduced precipitation since 1997 had resulted in the growth decline of Pinus massoniana, Castanea henryi and Castanopsis eyrei but not for Liquidambar formosana. Four species had similar sensitivities to the previous year and the current year, which is probably due to the carryover effect and temporal autocorrelation of climate data. Tree growth was positively correlated with growing season precipitation and relative humidity while negatively correlated with vapor pressure deficit. The negative relationship of tree radial growth with temperatures in the previous and current summer and the positive correlation with precipitation gradually strengthened after 1997. Therefore, we highlighted that drought-induced tree decline in subtropical forests is probably a common phenomenon, and it needed to verify by more tree-ring studies on a large scale. The species-specific responses of tree radial growth to climate change are not obvious, but they still should be considered in regional carbon balance and forest dynamics. Considering future climate change, species that are more drought tolerant should be considered as potential plantation species.
Introduction
Forest ecosystems, an imperative part of the terrestrial ecosystem, account for 30% of the area of the global terrestrial ecosystems (FAO, 2015). Forests act as an important net carbon sink (2.4 ± 0.4 Pg C year−1) and therefore play a key role in the terrestrial carbon cycle (Pan et al., 2011; Gomes et al., 2021). Climate change directly affects forest ecosystems, particularly the capacity of forests to take up carbon (Allen et al., 2015; Zuidema et al., 2022). A variety of climatic and atmospheric changes, including rising temperature, warming-induced atmospheric drought, shifting rainfall patterns, and elevated atmospheric CO2, can have various impacts on tree growth (Panthi et al., 2020).
The impact of global warming on forest ecosystems is uncertain mainly because of the following two reasons. The first is the spatiotemporal heterogeneity of climate change (IPCC, 2021). For example, most studies on tree growth and climate relationships present positive responses to global warming in high latitudes/elevations and negative responses at lower ones (Piao et al., 2019; Zhou et al., 2021). Second, different species have different strategies to cope with climatic changes, and species specificity or forest type is a key factor that defines the growth-climate response patterns (Fan et al., 2009; Rahman et al., 2019). For example, coniferous and broad-leaved species often have different tree growth-climate relationships due to their distinct internal morphological traits, physiological mechanisms, and phonological processes (Dai et al., 2020). Therefore, more work and data on different species or climate conditions are needed to clarify the uncertainty in accessing the effects of climate warming on forest growth (Allen et al., 2015). At the same time, understanding the differences in the responses of different tree species to climate change is of great significance for future forest management and plantations.
Trees are widely distributed and climate sensitive, and their rings can be easily cross-dated (Fritts et al., 1969). As natural archives, tree rings are valuable sources for detecting tree growth-climate relationships, providing accurate proxies for paleoenvironmental studies from local to hemispheric scales (Rahman et al., 2019). Tree ring data have become increasingly valuable in disclosing the long-term climate dynamics and studying the relationship between global warming and forest ecosystems in different regions (Zhu et al., 2021). Hence, quantifying the tree growth-climate relationships using dendrochronological methods will help us understand how trees respond to climate change and hence improve predictions of forest dynamics under future climate conditions.
Subtropical forests are an important part of the world’s forests (Sharma et al., 2022). They are structurally complex, rich in species composition, and essential for the provision of diverse ecosystem services to human society (Isbell et al., 2015; Ferreira et al., 2018). The subtropical forest ecosystem is important for studying the global terrestrial ecosystem carbon cycle (Yu et al., 2014). The East Asian summer monsoon brings a high amount of precipitation to the subtropics of China, home of the world’s largest subtropical evergreen broad-leaved forest, which is known as the ‘oasis’ on the tropic of cancer (Cook et al., 2010). With rich species composition and diverse vegetation types, subtropical forests play a significant role in maintaining local and even global carbon balance and biodiversity conservation. Nevertheless, climate changes have severely impacted the composition and redistribution of species in subtropical forest ecosystems (Zhou et al., 2013). Specifically, substantial mortality of tree species and significant changes in community structure have been evident in subtropical forests in southern China over the past 60 years, which is highly correlated with warming and increased soil dryness (Zhou et al., 2013).
In the last decade, an increasing number of tree-ring studies have been carried out in subtropical regions (Liang et al., 2019; Su et al., 2021; Sharma et al., 2022; Yang et al., 2022a). Many studies conducted in subtropical regions ecosystems suggest that drought stress caused by regional warming has caused vegetation shifts (Allen et al., 2010), increased tree mortality (Allen et al., 2015), increased die-off of some species (Breshears et al., 2005), decreased tree radial growth (Sharma et al., 2022), and decreased carbon sequestration in vegetation (Brienen et al., 2012). These studies suggest that subtropical forests were threatened by their lack of resilience against long-term climate changes (Zhou et al., 2013). Although some dendrochronological studies have been carried out in the subtropical regions of China, dendroecology studies are still relatively incomplete in this area (Luo et al., 2017). Large-scale, multi-species assessments of subtropical China would allow us to better assess how environmental change may control forest growth and the functioning of this key ecosystem. Thus, new tree-ring chronologies of some key and widely distributed species that have not been studied previously from the subtropical zones of China are needed to identify and characterize the impact of climate change on tree growth, such as Castanopsis eyrei, Liquidambar formosana, and Castanea henryi.
Here, we carried out a dendrochronology study on four subtropical tree species (Pinus massoniana, C. eyrei, L. formosana, and C. henryi) growing in the northern Luoxiao Mountain in central China. We aim to (i) determine the growth trends of these tree species, (ii) reveal the differences in the growth-climate relationships of these tree species, and (iii) examine the temporal variation of climate-growth relationships of these tree species. We hypothesized that climate warming caused a decline in tree radial growth in subtropical regions. The growth-climate relationship has changed after climate warming, which differs among tree species.
Materials and methods
Study area and climate
The study was conducted at the Lutou National Station for Scientific Observation and Research of Forest Ecosystems (113°51′52″-113°58′24″E, 28°31′17″-28°38′00″N), Hunan Province, China (Figure 1). The elevation of the sampling sites ranges from 483 to 896 m. The forest communities in this area are mainly subtropical evergreen broad-leaved forests. The forest is mainly composed of P. massoniana, C. eyrei, L. formosana, C. henryi, Rhododendron protist, Cyclocarya paliurus, and Cunninghamia lanceolata. The study area is located in a subtropical humid continental monsoon climate zone with an apparent seasonal variability. Based on the instrument records of Pingjiang Weather Station (113°10′E, 28°43′N, 1097 m a.s.l.), the annual average temperature of the study area is 16.9°C, and the annual precipitation is 1,497 mm. January (1.53°C) and July (33.7°C) are the coldest and warmest months. Precipitation mainly occurs from March to July, accounting for 64% of the yearly rainfall. The frost-free period is about 260 days. The peaks of mean monthly temperature (July–August) and total monthly precipitation (May–June) are not synchronized (Figure 2). The Pettitt test shows that the climate in the region has changed significantly since 1997. The annual mean temperature (k = 0.01, R2 = 0.23, p < 0.001) and vapor pressure deficit (VPD; k = 1.62, R2 = 0.1, p < 0.001) in the study area showed significantly increasing trend. Especially after 1997, the annual mean temperature significantly increased at a rate of 0.03°C per year. The precipitation and VPD slightly decreased (k = −9.9, R2 = 0.03, p = 0.41) and increased (k = 0.048, R2 = 0.04, p = 0.27) after 1997. It is worth mentioning that the continuous high temperature and low precipitation from 1997 to 2019 led to a significant long-term drought in the study area (Figure 2).
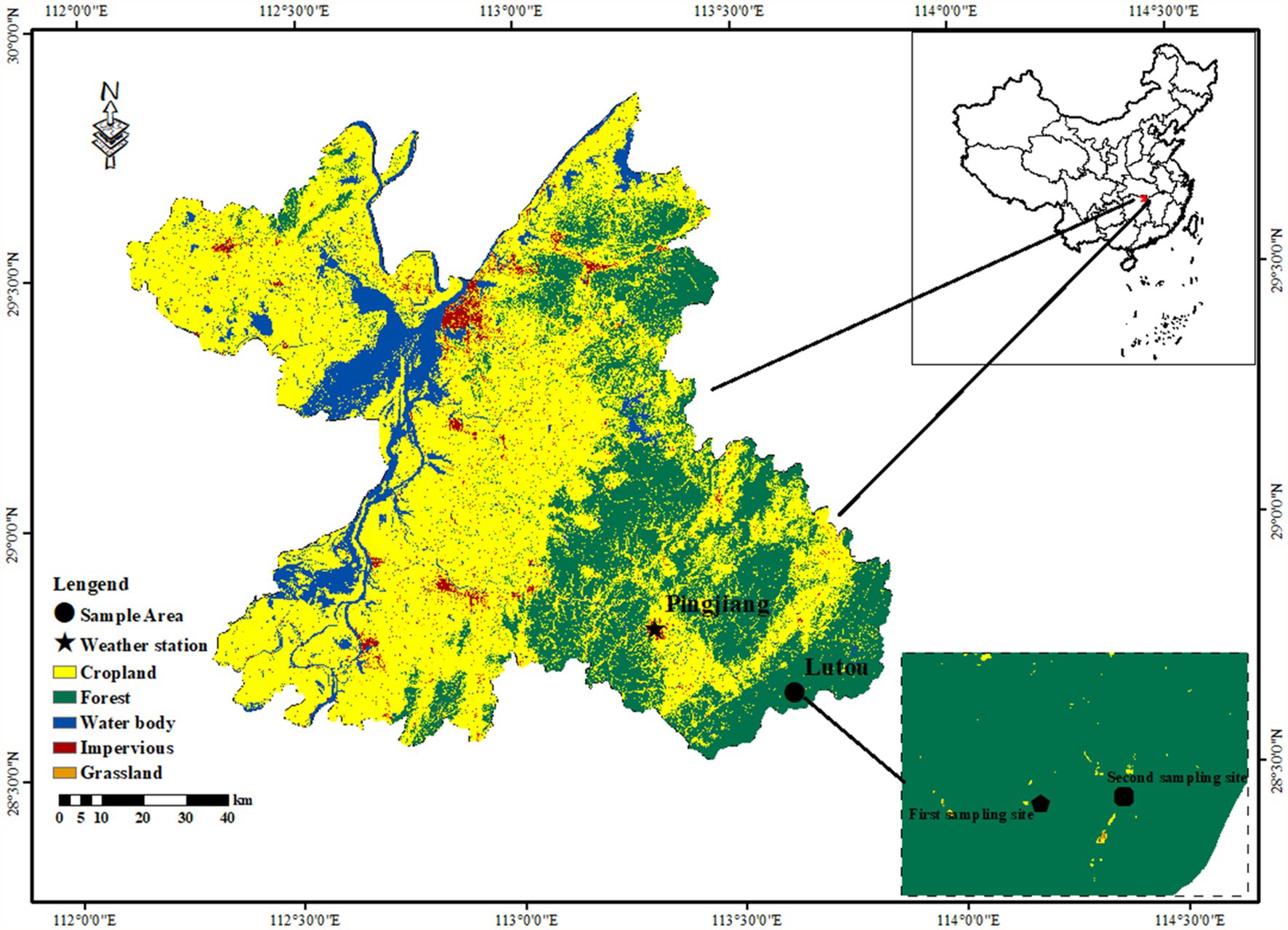
Figure 1. Location of the study area (Lutou) and weather station (Pingjiang) in the northern Luoxiao Mountain. Samples were collected at the first sampling site in September 2020 and at the second sampling site in July 2022.
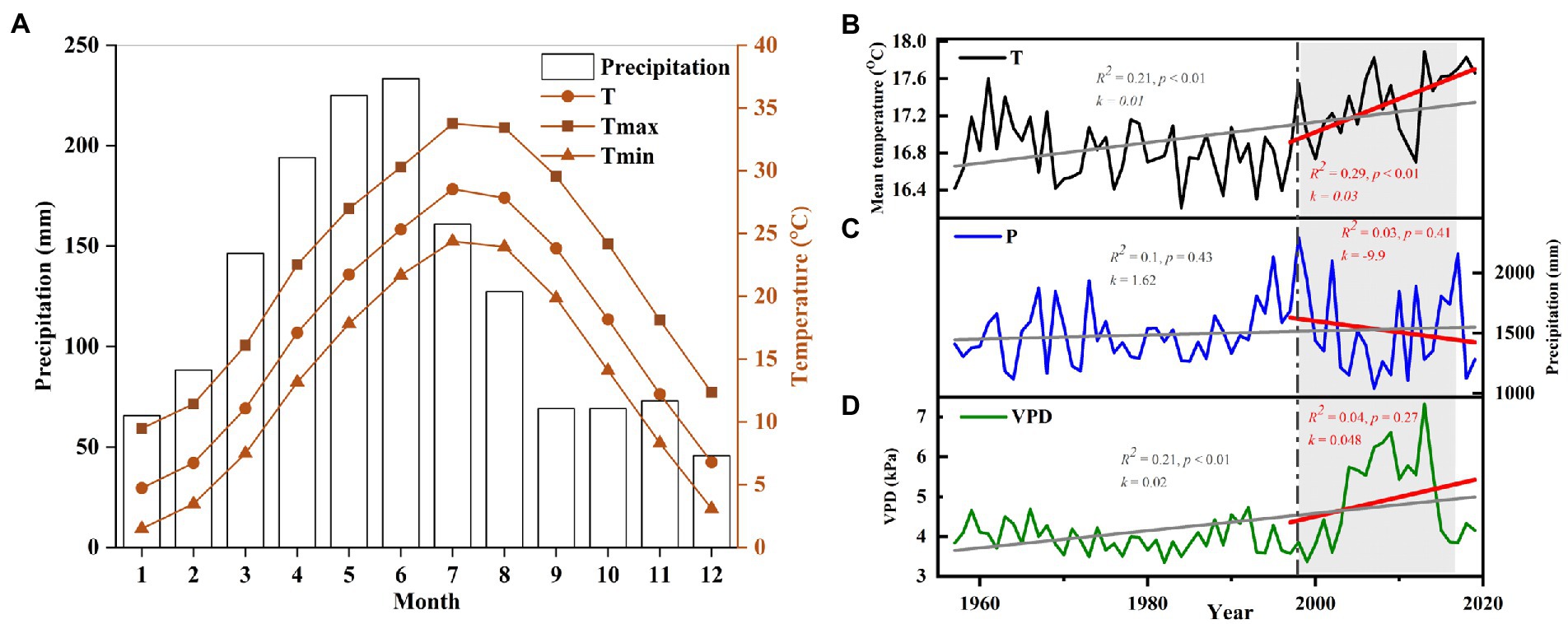
Figure 2. (A) Climograph of the study area showing monthly precipitation and temperatures from Pingjiang station during 1958–2019. Change trends of (B) mean temperature (T), (C) annual total precipitation (P), and (D) vapor pressure deficit (VPD). A linear regression equation fitted the interannual precipitation, temperature, and VPD trends from 1958 to 2019 (grey) and 1997 to 2019 (red). The R-squared, the slope (k), and the value of p of each model are listed in the figure. Severe drought periods are highlighted with a grey box.
Sampling and data measurement
Based on reviewing relevant information and conducting a field survey of the study area, four common species (C. eyrei, C. henryi, P. massoniana, and L. formosana) were selected as target tree species. These species play an imperative role in the forest productivity and succession of forest communities in subtropical regions (Table 1). P. massoniana is a dominant local native tree species in south China. It is a fast-growing, evergreen, sun-loving pioneer conifer species, and a substantial increase in plantations of this species in south China has been witnessed during the past several decades. In contrast, C. eyrei and C. henryi are broad-leaved trees of intermediate shade-tolerance; L. formosana, a dominant deciduous tree species, has strong sprouting power and likes heat but is not drought tolerant. In October 2020 and July 2022, 10–50 mature and healthy individuals were selected as sampling trees for each species. One-to-two cores from each tree were collected at 1.3 m with a 5.15-mm diameter increment borer.
All tree-ring cores were fixed, dried, and polished in the laboratory (Figure 3). Then, they were visually cross-dated using the skeleton plot method under a binocular microscope (Stokes and Smiley, 1968). Tree ring width (TRW) was measured to an accuracy of 0.01 mm using a LINTAB™ 6 measuring system (RINNTECH, Germany). The quality of measurements and cross-dating were statistically tested using COFECHA (Holmes, 1983). The raw tree-ring series were detrended by fitting a negative exponential curve (ModNegExp) or a linear regression function with the “dplR” (Bunn, 2008) package in the R software (R Core Team, 2018). During this process, the conventional negative exponential curve or straight line of a negative slope or horizontal line was firstly applied to each tree-ring series. Then all the dimensionless indexes, computed by dividing the original measurement of each ring by the value of the fitted curve in the corresponding year, were combined into a single standard (STD) chronology (Figure 4) by computing a biweight robust mean (Cook, 1985).
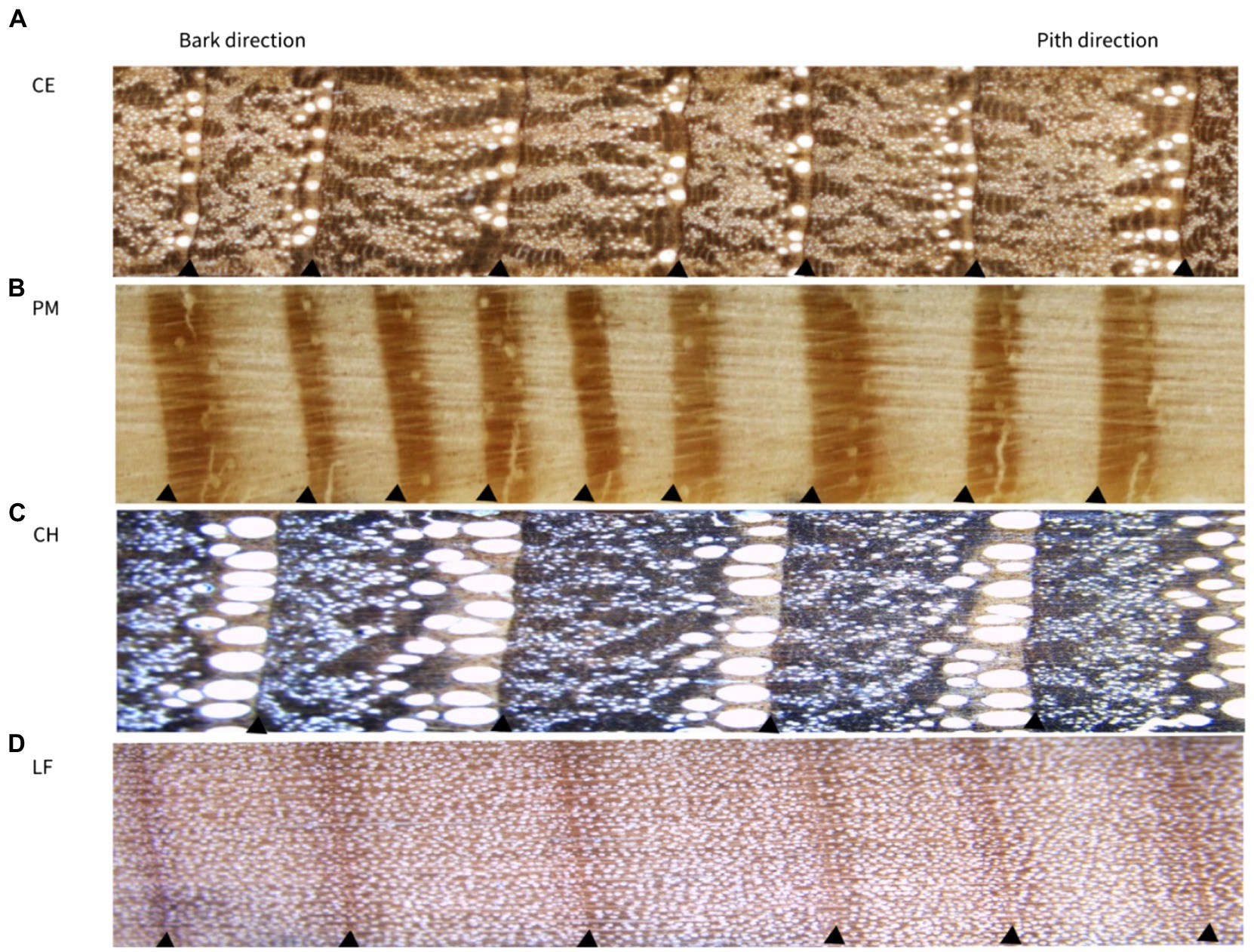
Figure 3. Photographs of the tree rings of the four tree species in the northern Luoxiao Mountain. (A) C. eyrei, (B) P. massoniana, (C) C. henryi and (D) L. formosana. The triangle indicates the ring boundary.
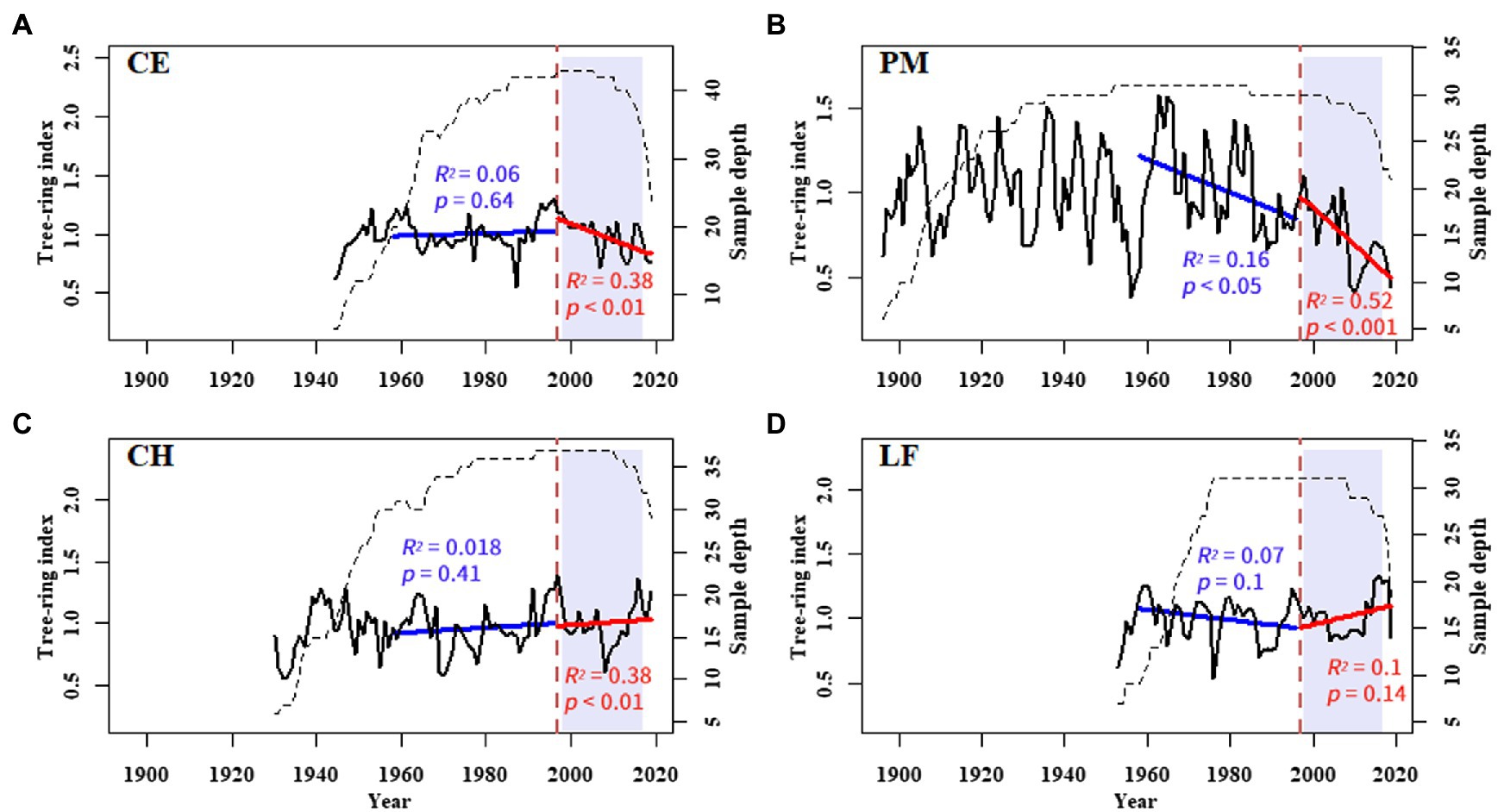
Figure 4. Tree-ring width standard chronologies of (A) C. eyrei, (B) P. massoniana, (C) C. henryi, and (D) L. formosana at the northern Luoxiao Mountain. The interannual growth trends from 1958–1996 (blue), and 1997–2019 (red) are fitted by a linear regression equation. The R-squared and value of p of each model are listed in the figure.
To detect the growth trend of four species, we calculated the basal area increment (BAI) from tree ring width, assuming the increment of each ring is uniform in a circular cross-section of the tree (Peters et al., 2015). BAI is a more informative measure of tree growth trends and has been considered a stable form of growth (Phillips et al., 2009; Sharma et al., 2022). The negative BAI trend strongly indicates a decline in tree growth (LeBlanc, 1990). We sampled the trees to the pith; some cores were not taken perpendicular to the ring borders but with an arc on the cores if possible (Duncan, 1989; Rozas, 2003). In cores without a pith, the number of years missing to pith was estimated by a geometrical method. After dating the pith, we converted the measured tree-ring widths into BAI values using the BIAPlt software. TRW and BAI series were log-transformed and scaled to improve the normality of the data. We also developed regional curve standardization (RCS) chronologies by implementing pith offset. The division of measured values by the values of the age-related growth curve removes the age-related growth trend from measurements, removes the effects of overall differential tree growth rates, and results in a stationary series of tree indices which are in a suitable state to be averaged to form a chronology (Fritts et al., 1969).
Climate data and statistical analysis
Instrumental climate data from the nearest Pingjiang weather station (~35 km) from 1957 to 2019 used in this study were downloaded from China Meteorological Data Service Center.1 Precipitation (P), relative humidity (RH), temperature (T), and extreme minimum (ETmin), extreme maximum (ETmax) temperatures at this station were extracted and used in this study. Atmospheric drought was quantified with the monthly VPD, calculated from the monthly mean temperature and relative humidity. Seasons were defined as follows: winter (December of the previous year to February of the current year), spring (March–May), summer (June–August), and autumn (September–November).
A linear regression model was used to fit the trends in tree growth or climate variations. Pearson correlation analysis was also carried out to determine the radial growth of the four tree species and the main monthly/seasonal climate factors. The abrupt point of climate change was tested using the Pettitt test in python software (Pettitt, 1979). Change-point, or step-trend, detection is an active area of research in statistics because change points may be evidence of natural or anthropogenic changes in climate (Ryberg et al., 2020). The Durbin-Watson test examined the first-order autocorrelation of climate data (Melo, 2014). Considering the lag effect of climate on tree growth, we used the climate variables from March of the previous year to November of the current year for monthly response analysis. Moving correlation analysis with a 25-year window was used to investigate the temporal stability of the relationships between radial growth and the main climate factors. Correlation analysis and moving correlation analysis were performed using the package “treeclim” (Zang and Biondi, 2015) in the R software.
Results
Growth characteristics of the four tree species
The correlation matrix between four species’ chronologies in the common period of 1958–2019 is shown in Table 2. The STD chronology of L. formosana was positively correlated with the STD chronology of C. eyrei (R = 0.297) and C. henryi (R = 0.354). And the STD chronologies of the other three tree species were also positively correlated with each other but not significant (Table 2).
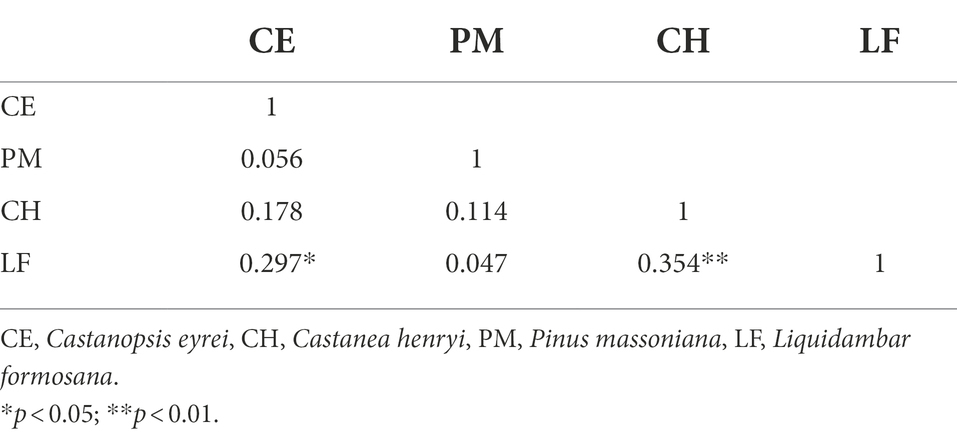
Table 2. Correlation coefficients (R) between four standard tree-ring chronologies during their common period 1958–2019.
The normalized Log BAI and RCS chronologies showed similar trends (Figure 5). C. eyrei and C. henryi showed an increasing trend in 1958–2019 and 1958–1996. After 1997, the growth trend of C. eyrei and C. henryi changed, showing a downward trend. P. massoniana showed a significant downward trend in each growth period. The normalized Log TRW results showed that all tree species except L. formosana showed a decreasing trend in all growth periods. Normalized Log TRW results showed that L. formosana shows a weak growth trend (Figure 5).
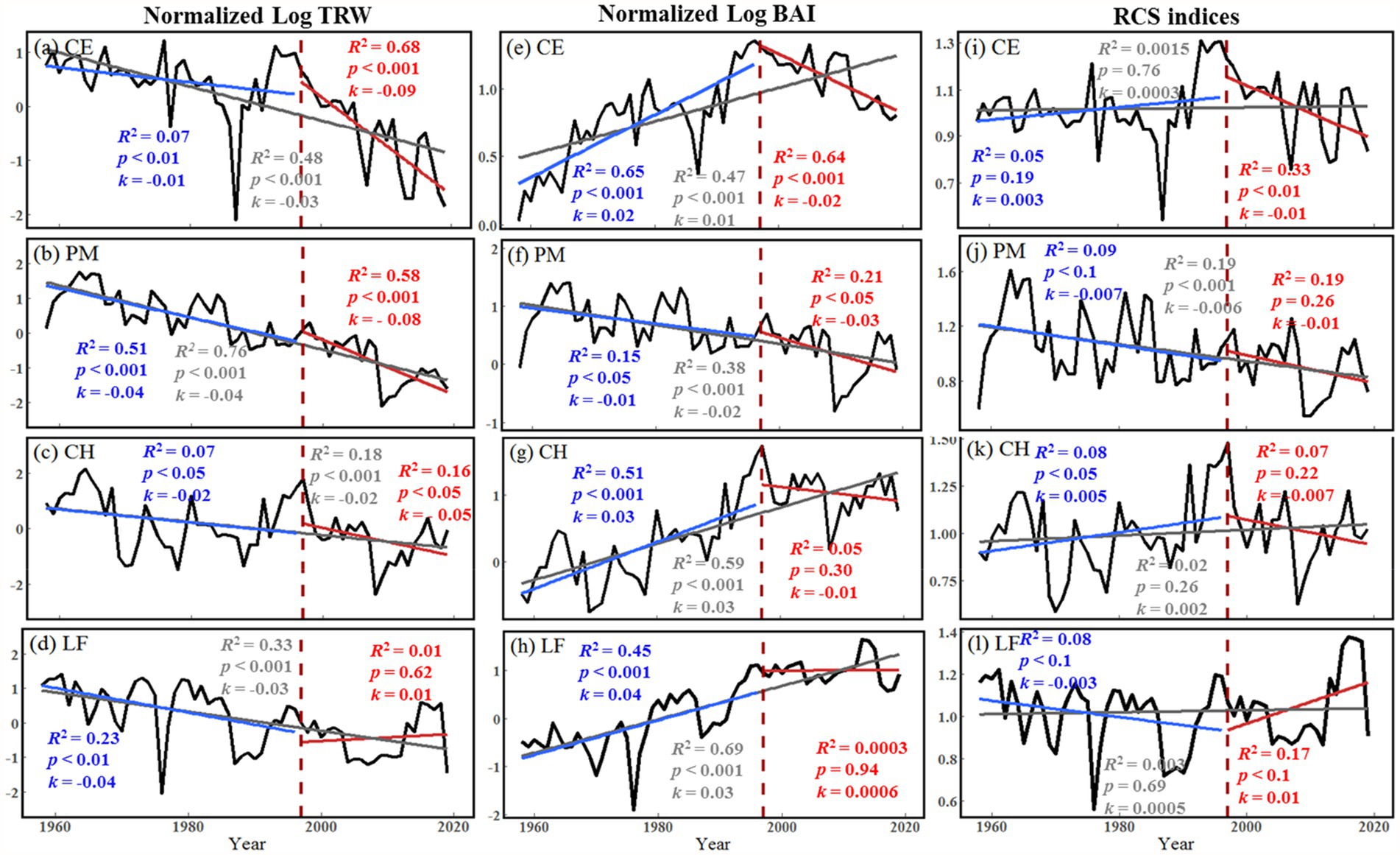
Figure 5. Long-term trends of tree growth (Normalized Log TRW, Normalized Log BAI, and RCS indices) during 1958–1996 (blue), 1997–2019 (red) and 1958–2019 (grey) at the northern Luoxiao Mountain. (a, e, i) CE = C. eyrei, (b, f, j) PM = P. massoniana, (c, g, k) CH = C. henryi and (d, h, l) LF = L. formosana. The R-squared, the slope (k) and value of p of each model are listed in the figure.
Relationships between radial growth and main climatic factors
The relationships between radial growth and climatic variables differed among species (Figures 6, 7). The radial growth of all trees was mostly positively correlated with RH, and mostly negatively correlated with VPD. In the study area, moisture is the main climate factor limiting tree radial growth. ETmax was mostly negatively correlated with the growth of P. massoniana, especially in April–August of the previous year and June–July of the current year. However, ETmin and T in December of the previous year and November of the current year significantly positively affected the growth of C. henryi. P was most positively correlated with the ring-width chronologies of C. eyrei, C. henryi, and L. formosana, and negatively correlated with P. massoniana (Figure 6).
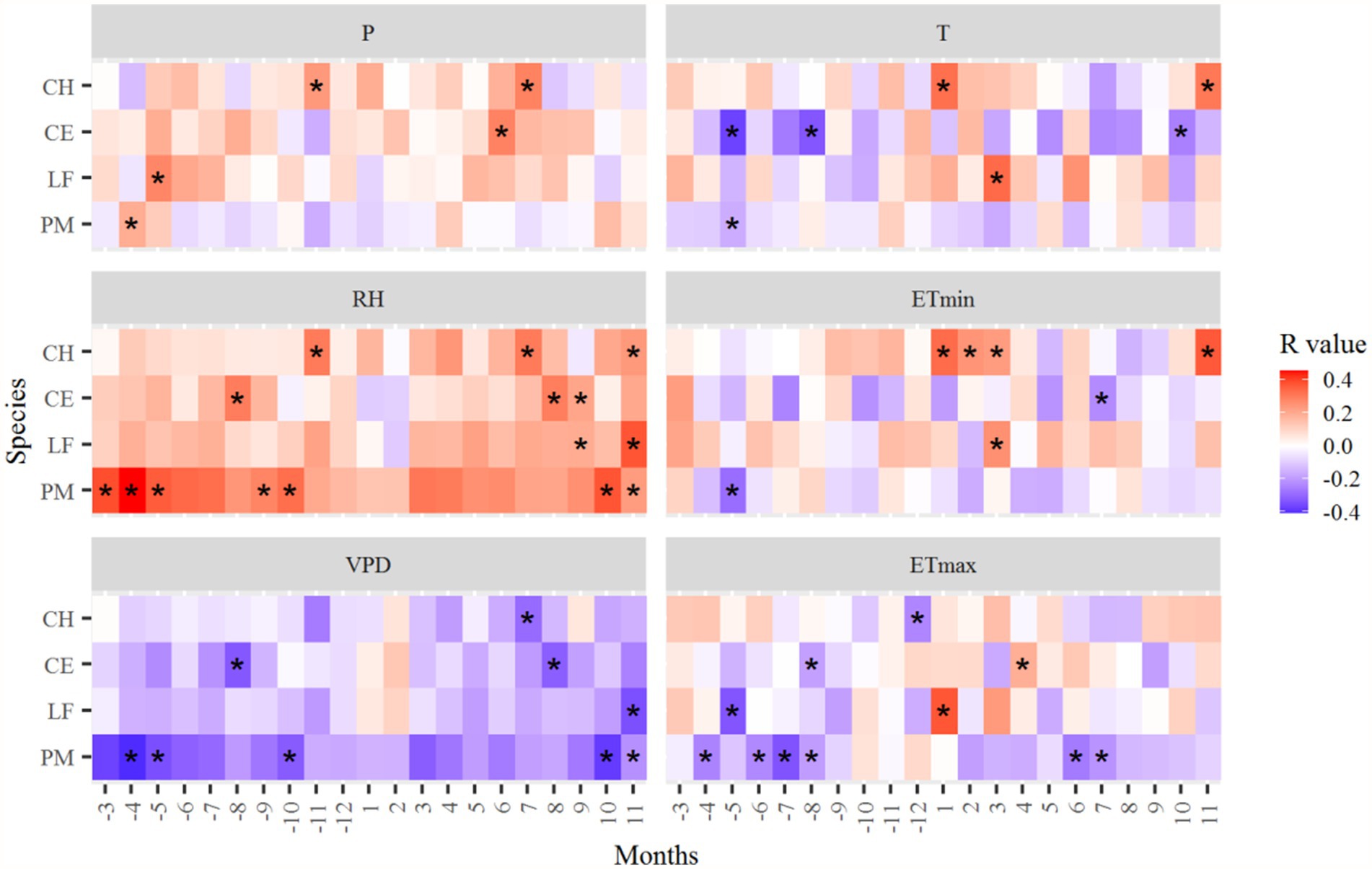
Figure 6. Correlation coefficients of the standard tree-ring chronologies in the northern Luoxiao Mountain with monthly main climate factors. P, precipitation, RH, relative humidity, VPD, vapor pressure deficit, T, mean temperature, ETmin, extreme minimum temperature, ETmax, extreme maximum temperature. Correlation analysis was computed for a 21 months window from previous year March to current year November. *p < 0.05.
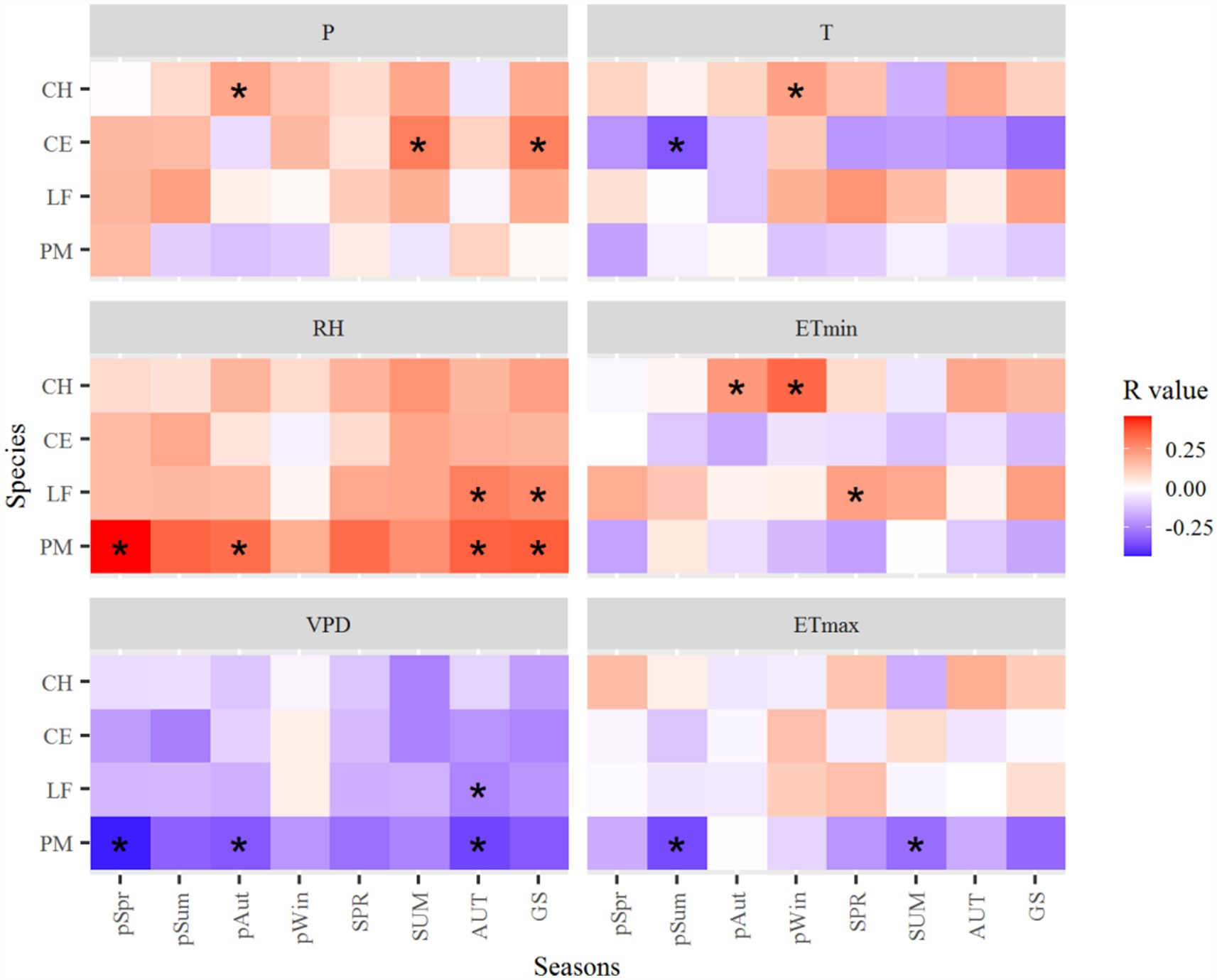
Figure 7. Correlation coefficients of the standard tree-ring chronologies in the northern Luoxiao Mountain with seasonal main climate factors during 1958–2019. P, precipitation, RH, relative humidity, VPD, vapor pressure deficit, T, mean temperature, ETmin, extreme minimum temperature, ETmax, extreme maximum temperature. pSpr, previous year March to May, pSum, previous year June to August, pAut, previous year September to November, pWin, previous year December to current year February, SPR, current year March to current May, SUM, current year June to August, AUT, current year September to November, GS, current year March to November. *p < 0.05.
The growth of C. henryi significantly (p < 0.05) positively correlated with the P in the autumn of the previous year (Figure 7). The growth of L. formosana and P. massoniana significantly positively correlated with the RH in the autumn and growing season (March–November) of the current year. Radial growth of P. massoniana is negatively correlated with temperatures, especially the ETmax. The T in the summer of the previous year significantly negatively affected the growth of C. eyrei. However, the ETmin in the winter of the previous year and the autumn of the previous year positively affected the growth of C. henryi (Figure 7).
Temporal stability in climate sensitivity of study species
The growth responses of almost all tree species to the RH and P in the summer of the previous and current years changed from weak positive or negative correlation to strong positive correlation after 1997 (Figure 8). And the relationships between the radial growth of all species to T, ETmax, ETmin, and VPD in the summer of the previous and current years changed from a positive or weak negative correlation to a strong negative correlation (Figure 8).
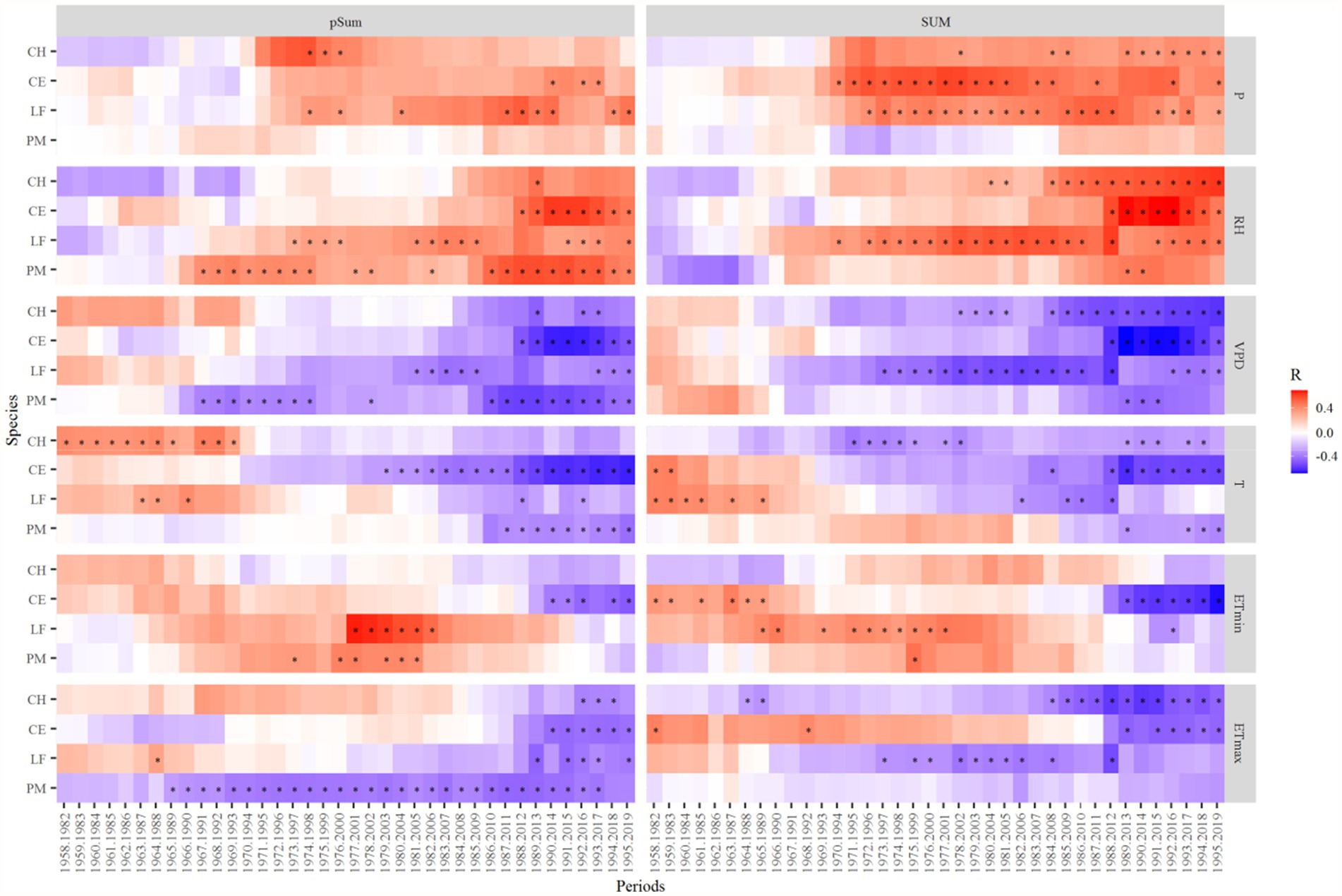
Figure 8. Moving correlation analysis of the standard tree-ring chronologies during 1958–2019. Correlation coefficients were computed for 25-year moving windows. The vertical bars on the right side of the graph represent the scale of correlation coefficients. pSum, previous year June to August, SUM, current year June to August. *p < 0.05.
Discussion
Tree growth decline in subtropical forest
All three analysis methods show the declining trend of radial growth of the three main tree species (Figure 5). Because of some potential bias, using growth rings to detect growth trends is not straightforward and should be considered to make accurate conclusions (Brienen et al., 2012). The “big tree sampling” bias is caused by sampling only the big trees, which usually results in positive growth trends over time. However, the “juvenile selection” effect often causes apparent negative growth trends (Brienen et al., 2012). Therefore, to detect and avoid the possible age/size-related spurious positive/negative trend, we used three different parameters: TRW, BAI, and RCS indices. The analysis results of RCS chronologies and normalized Log BAI showed that the “juvenile selection” did not play a very important role in the decline trend. The decline trend of tree radial growth after 1997 in this area is mainly caused by climate change.
That climate change could directly or indirectly initiate tree decline was first put forward by Hepting (1963). After that, the investigation of climatic influences on radial growth was widely undertaken to better understand the unexpected decline of trees, such as the Pinus koraiensis and Picea jezoensis in northeast Asia (Zhu et al., 2018; Wang et al., 2019), the Nothofagus macrocarpa and Pseudotsuga menziesii in North America (Klesse et al., 2020), and the Abies alba and Fugus sylvatica in Europe (Gazol et al., 2015; Bosela et al., 2018). Analysis of growth trends showed a declining trend in growth for most tree species after 1997 (Figure 5). It contradicts with our common belief that the subtropical forest ecosystems are expected to largely benefit or at least tolerate increasing temperatures (Boisvenue and Running, 2006; Allen et al., 2015). This general growth decline of subtropical trees in our study area is probably related to local climate change.
The radial growth of most subtropical trees was negatively correlated with VPD and positively correlated with relative humidity (Figures 6, 7). It suggested that water availability limits the radial growth of trees in subtropical regions. In the study area, there was a drying trend after 1997 due to significantly increased temperature and decreased precipitation (Figure 2). Hence, warming and increasing atmospheric drought at our study sites can lead to a decline in tree growth. Our results are consistent with studies by Su et al. (2021) and Huang et al. (2021), where drought contributed to the decline of forests in subtropical regions. Firstly, drought stress reduces leaf size, stem extension, and root proliferation, disturbs plant water relations, and reduces water-use efficiency (Farooq et al., 2009; Allen et al., 2015). Moreover, warming-induced high evapotranspiration reduces soil water content, vegetation photosynthesis, and carbohydrate reserves. At the same time, it will increase the atmospheric demand for water vapor, which deteriorates the water status in leaf and stem tissues (Wang et al., 2019).
In fact, the adverse effects of high temperatures and summer droughts on tree growth are widespread worldwide (Schwab et al., 2018; Harvey et al., 2020). Prolonged water deficits have caused increased tree mortality rates, severe forest decline, and vegetation shifts on dry sites and at the edge of species distribution ranges (Rigling et al., 2002; Galiano et al., 2010). For example, forest mortality has risen rapidly in temperate and boreal regions of southern Europe (Bosela et al., 2021; Martinez del Castillo et al., 2022) and western North America (Bigler et al., 2006; Bréda et al., 2006; Keen et al., 2022), and widespread death of many tree species in multiple forest types has affected well over 10 million ha since 1997 (Raffa et al., 2008). Our results indicate that further increases in forest decline and even tree mortality could be expected if climate warming continues, potentially driving the eventual regional loss of current subtropical trees or forests (Liu et al., 2020). A reduction in tree radial growth caused by warming-mediated drought stress has been found in many regions of China, such as tropical (Sharma et al., 2022), subtropical (Yang et al., 2022b), and Tibetan Plateau (Liang et al., 2016). The warming of the climate and the rise of atmospheric carbon dioxide increase the respiration of plants, leading to the reduction of carbon assimilation and inhibiting the radial growth of trees (Yang et al., 2022b). In addition, there is an urgent need for more studies at a regional scale to test the declining trends in subtropical forest growth and the mechanism behind it.
The effect of climate change on the response stability of different tree species
Recent rapid warming in subtropical China has significantly affected tree radial growth. Moving correlation analysis showed that the negative correlation of tree growth with temperature and the positive correlation with precipitation gradually strengthened after 1997 (Figure 8). It is probably related to rapid warming in the study area. High summer temperatures can likely accelerate soil water evaporation and increase the VPD, thereby reducing soil moisture availability to trees (Keyimu et al., 2020). And higher temperatures exacerbate drought stress and increase the risk of drought-induced cavitation (Kannenberg et al., 2019). This suggests that the role of moisture availability in the growing season is becoming increasingly important for growth. Negative impacts due to increased temperature and moisture limitation will be more severe if climate warming intensifies. In the context of global warming, the effects of warm-induced drought stress on tree growth may be more severe, possibly leading to a large-scale tree decline in subtropical forests.
Climate change has been widely observed as a key factor that affects tree species’ sensitivity to climate (Kannenberg et al., 2019). Our results showed that tree growth-climate sensitivity is relatively consistent in this region, and tree species specificity is not obvious (Figure 8). This differs from other studies in subtropical regions. For example, Fan et al. (2009) pointed out that fir (Abies georgei) and spruce (Picea brachytyla) trees show a different climate-response behavior, both seasonally and in magnitude. The study of Yang et al. (2022b) in Southwest China showed that the two pine tree species (Pinus kesiya and Pinus yunnanensis) had different growth climate sensitivity. Growth-climate sensitivity of tree species varies with site-specific environmental conditions. The tree growth-climate sensitivity is not obvious among species in this study. It is probably because the precipitation in this area is relatively abundant compared with that in Southwest China. Therefore, it may be subject to less drought stress compared with other regions.
Our results show that all four species revealed almost similar climate sensitivity (although they differed in magnitude) for both previous year and current year climate (Figures 6–8). There are two possible reasons: The first one could be the carryover effect of reserve carbohydrates (in leaves) from previous years or the legacy effect of climate. The second one is the temporal autocorrelation in climate data and collinearity among climate variables. The main reason for the similar sensitivities in the previous year and the current year in T, P, ETmin, and ETmax is the legacy effects (Supplementary Figure S1; Table 3). Evidence for a lagged climate-growth response is commonly found in dendrochronological studies across the tropical and subtropical regions (Dünisch et al., 2003; Brienen and Zuidema, 2005). Water balance condition of the current year is critical to tree stem radial growth, and the pre-growing season droughts could profoundly affect later radial growth. Both the onset timing and severity of drought strengthen its “legacy effects,” which reduce trees’ resilience and make it harder for them to recover completely from drought (Gao et al., 2018). However, the DW test results showed that RH and VPD have significant first-order autocorrelation (Table 3). And the first-order difference data analysis results also proved this conclusion (Supplementary Figure S1). Therefore, except for the “legacy effects,” similar climate sensitivities in previous and current year RH and VPD may also be caused by the temporal autocorrelation of climate data (Supplementary Figure S1; Table 3). In tree rings, the physiology of trees serves as a basis for growth resources from previous years to be carried over a number of forthcoming years, resulting in tree-ring values that are depending on temporally adjacent values (Fritts et al., 1969). The main component of this autocorrelation comes from climate, which shows persistence through fluctuations and trends (Karl, 1988). Data autocorrelation may have an impact on long-term climate reconstruction, which should be considered in future research on paleoclimate reconstruction in subtropical regions.
In this study, we found that L. formosana still showed a higher growth trend after climate change (Figure 5). The species-specific tree growth patterns and tolerance to drought are closely related to these tree species’ physiological structures and traits because each wood type (e.g., ring-porous, diffuse-porous, and coniferous) differs in the structure, size, and spatial distribution of xylem conduits and the scaling of hydraulic properties with stem diameter (Meinzer et al., 2013). Previous studies have reported that the ability of trees to tolerate a dry environment is more important than the rapid transport of water (Lemoine et al., 2001). Our study of different tree species in subtropical forests also supports this view. Coniferous trees with a tight stomatal regulation such as pines (Klein et al., 2018) might display a lower resistance with increasing drought intensity. In contrast, vulnerability to drought-induced cavitation tends to be higher in ring-porous species (C. henryi and C. eyrei), because large-vessel cavitation yields a considerable loss in hydraulic conductivity (Hacke et al., 2006). These hydraulic properties depending on xylem anatomical features are linked to downstream stomatal regulation of transpiration (Lemoine et al., 2001; Bush et al., 2008). It is well established that some deciduous angiosperm species depend on sugar reserves to start secondary growth (Michelot et al., 2012). The later cambial activity of diffuse-porous (L. formosana) enables them to avoid using their stored carbohydrates for leaf flush. The stem improved the water balance of L. formosana (Köcher et al., 2013).
A significant reduction in tree growth during drought years, as found in this study and other studies across the subtropical region, may have important implications for the global carbon cycle. If climate warming intensifies, it could lead to a decline or death of drought-intolerant species. Reforestation, restoration, and a large-scale plantation establishment (afforestation) around the subtropics have been accepted as mitigation strategies of ongoing climate change impacts (Stocker et al., 2014; Rahman et al., 2019). Species selection is important for plantation design and depends on management objectives. Survival of different tree species will develop in a changing climate to compose an optimal species portfolio, leading to changes in forest composition, structure, and productivity. Considering future climate change, L. formosana should be selected as the main afforestation tree species. Additionally, the results highlight that it is important to protect relatively humid habitats, choose drought-tolerant tree species, implement water and soil protection measures during forest restoration, and take other management measures to maintain forest productivity in our study area.
Conclusion
To examine the potential effects of climate change on forest growth of major tree species in subtropical forests, we investigated the response of radial growth of four major tree species in subtropical forests to climate change. Our results suggested that warming-related drought stress contributes to the decline of most tree species in subtropical forests. The radial growth of most subtropical trees was negatively correlated with vapor pressure deficit and positively correlated with relative humidity. The results of the moving correlation analysis showed that the negative correlation of tree growth with temperature and the positive correlation with precipitation and relative humidity gradually strengthened. Drought stress caused by rapid warming may be the main factor affecting the radial growth of trees in subtropical regions. And due to the influence of the legacy effect and the temporal autocorrelation in climate data, the four tree species in the study area have a similar growth-climate relationship. If climate warming continues, it will potentially drive the eventual regional loss of current subtropical trees or forests. Determining which tree species are more tolerant of climate change should be considered in future forest management.
Data availability statement
All data used in the study can be found in the Supplementary material.
Author contributions
All authors listed have made a substantial, direct, and intellectual contribution to the work and approved it for publication.
Funding
The study was supported by the National Natural Science Foundation of China (42107476 and 31901241), the China Postdoctoral Science Foundation (2020M682600), the Research Foundation of the Bureau of Education in Hunan Province (20B627), and the Start-up Scientific Research Foundation for the Introduction of Talents in Central South University of Forestry and Technology (2020YJ012).
Conflict of interest
The authors declare that the research was conducted in the absence of any commercial or financial relationships that could be construed as a potential conflict of interest.
Publisher’s note
All claims expressed in this article are solely those of the authors and do not necessarily represent those of their affiliated organizations, or those of the publisher, the editors and the reviewers. Any product that may be evaluated in this article, or claim that may be made by its manufacturer, is not guaranteed or endorsed by the publisher.
Supplementary material
The Supplementary material for this article can be found online at: https://www.frontiersin.org/articles/10.3389/fpls.2022.964400/full#supplementary-material
Footnotes
References
Allen, C. D., Breshears, D. D., and McDowell, N. G. (2015). On underestimation of global vulnerability to tree mortality and forest die-off from hotter drought in the Anthropocene. Ecosphere 6:129. doi: 10.1890/ES15-00203.1
Allen, C. D., Macalady, A. K., Chenchouni, H., Bachelet, D., McDowell, N., Vennetier, M., et al. (2010). A global overview of drought and heat-induced tree mortality reveals emerging climate change risks for forests. For. Ecol. Manag. 259, 660–684. doi: 10.1016/j.foreco.2009.09.001
Bigler, C., Bräker, O. U., Bugmann, H., Dobbertin, M., and Rigling, A. (2006). Drought as an inciting mortality factor in scots pine stands of the Valais, Switzerland. Ecosystems 9, 330–343. doi: 10.1007/s10021-005-0126-2
Boisvenue, C., and Running, S. W. (2006). Impacts of climate change on natural forest productivity–evidence since the middle of the 20th century. Glob. Chang. Biol. 12, 862–882. doi: 10.1111/j.1365-2486.2006.01134.x
Bosela, M., Lukac, M., Castagneri, D., Sedmák, R., Biber, P., Carrer, M., et al. (2018). Contrasting effects of environmental change on the radial growth of co-occurring beech and fir trees across Europe. Sci. Total Environ. 615, 1460–1469. doi: 10.1016/j.scitotenv.2017.09.092
Bosela, M., Tumajer, J., Cienciala, E., Dobor, L., Kulla, L., Marčiš, P., et al. (2021). Climate warming induced synchronous growth decline in Norway spruce populations across biogeographical gradients since 2000. Sci. Total Environ. 752:141794. doi: 10.1016/j.scitotenv.2020.141794
Bréda, N., Huc, R., Granier, A., and Dreyer, E. (2006). Temperate forest trees and stands under severe drought: a review of ecophysiological responses, adaptation processes and long-term consequences. Ann. For. Sci. 63, 625–644. doi: 10.1051/forest:2006042
Breshears, D. D., Cobb, N. S., Rich, P. M., Price, K. P., Allen, C. D., Balice, R. G., et al. (2005). Regional vegetation die-off in response to global-change-type drought. Proc. Natl. Acad. Sci. 102, 15144–15148. doi: 10.1073/pnas.0505734102
Brienen, R. J. W., Gloor, E., and Zuidema, P. A. (2012). Detecting evidence for CO2 fertilization from tree ring studies: the potential role of sampling biases. Glob. Biogeochem. Cycles 26:GB1025. doi: 10.1029/2011GB004143
Brienen, R. J. W., and Zuidema, P. A. (2005). Relating tree growth to rainfall in Bolivian rain forests: a test for six species using tree ring analysis. Oecologia 146, 1–12. doi: 10.1007/s00442-005-0160-y
Bunn, A. G. (2008). A dendrochronology program library in R (dplR). Dendrochronologia 26, 115–124. doi: 10.1016/j.dendro.2008.01.002
Bush, S. E., Pataki, D. E., Hultine, K. R., West, A. G., Sperry, J. S., and Ehleringer, J. R. (2008). Wood anatomy constrains stomatal responses to atmospheric vapor pressure deficit in irrigated, urban trees. Oecologia 156, 13–20. doi: 10.1007/s00442-008-0966-5
Cook, E. R. (1985). A Time Series Analysis Approach to Tree Ring Standardization. Arizona: University of Arizona Tucson.
Cook, E. R., Anchukaitis, K. J., Buckley, B. M., D’Arrigo, R. D., Jacoby, G. C., and Wright, W. E. (2010). Asian monsoon failure and megadrought during the last millennium. Science 328, 486–489. doi: 10.1126/science.1185188
Dai, Y., Lin, W., and Wan, X. (2020). Frost fatigue and its spring recovery of xylem conduits in ring-porous, diffuse-porous, and coniferous species in situ. Plant Physiol. Biochem. 146, 177–186. doi: 10.1016/j.plaphy.2019.11.014
Duncan, R. P. (1989). An evaluation of errors in tree age estimates based on increment cores in kahikatea (Dacrycarpus dacrydioides). NZ Nat. Sci. 16, 31–37.
Dünisch, O., Montóia, V. R., and Bauch, J. (2003). Dendroecological investigations on Swietenia macrophylla king and Cedrela odorata L.(Meliaceae) in the Central Amazon. Trees 17, 244–250. doi: 10.1007/s00468-002-0230-2
Fan, Z.-X., Bräuning, A., Cao, K.-F., and Zhu, S.-D. (2009). Growth–climate responses of high-elevation conifers in the central Hengduan Mountains, southwestern China. For. Ecol. Manag. 258, 306–313. doi: 10.1016/j.foreco.2009.04.017
Farooq, M., Wahid, A., Kobayashi, N., Fujita, D., and Basra, S. M. A. (2009). Plant drought stress: effects, mechanisms and management. Agron. Sustain. Dev. 29, 185–212. doi: 10.1051/agro:2008021
Ferreira, J., Lennox, G. D., Gardner, T. A., Thomson, J. R., Berenguer, E., Lees, A. C., et al. (2018). Carbon-focused conservation may fail to protect the most biodiverse tropical forests. Nat. Clim. Chang. 8, 744–749. doi: 10.1038/s41558-018-0225-7
Fritts, H. C., Mosimann, J. E., and Bottorff, C. P. (1969). A revised computer program for standardizing tree-ring series. Tree-Ring Bulletin 29, 15–20.
Galiano, L., Martínez-Vilalta, J., and Lloret, F. (2010). Drought-induced multifactor decline of scots pine in the Pyrenees and potential vegetation change by the expansion of co-occurring oak species. Ecosystems 13, 978–991. doi: 10.1007/s10021-010-9368-8
Gao, S., Liu, R., Zhou, T., Fang, W., Yi, C., Lu, R., et al. (2018). Dynamic responses of tree-ring growth to multiple dimensions of drought. Glob. Chang. Biol. 24, 5380–5390. doi: 10.1111/gcb.14367
Gazol, A., Camarero, J. J., Gutiérrez, E., Popa, I., Andreu-Hayles, L., Motta, R., et al. (2015). Distinct effects of climate warming on populations of silver fir (Abies alba) across Europe. J. Biogeogr. 42, 1150–1162. doi: 10.1111/jbi.12512
Gomes, E., Inácio, M., Bogdzevič, K., Kalinauskas, M., Karnauskaitė, D., and Pereira, P. (2021). Future land-use changes and its impacts on terrestrial ecosystem services: a review. Sci. Total Environ. 781:146716. doi: 10.1016/j.scitotenv.2021.146716
Hacke, U. G., Sperry, J. S., Wheeler, J. K., and Castro, L. (2006). Scaling of angiosperm xylem structure with safety and efficiency. Tree Physiol. 26, 689–701. doi: 10.1093/treephys/26.6.689
Harvey, J. E., Smiljanić, M., Scharnweber, T., Buras, A., Cedro, A., Cruz-García, R., et al. (2020). Tree growth influenced by warming winter climate and summer moisture availability in northern temperate forests. Glob. Chang. Biol. 26, 2505–2518. doi: 10.1111/gcb.14966
Hepting, G. H. (1963). Climate and Forest diseases. Annu. Rev. Phytopathol. 1, 31–50. doi: 10.1146/annurev.py.01.090163.000335
Holmes, R. L. (1983). Computer-assisted quality control in tree-ring dating and measurement. Tree-Ring Bull. 43, 51–67.
Huang, X., Dai, D., Xiang, Y., Yan, Z., Teng, M., Wang, P., et al. (2021). Radial growth of Pinus massoniana is influenced by temperature, precipitation, and site conditions on the regional scale: a meta-analysis based on tree-ring width index. Ecol. Indic. 126:107659. doi: 10.1016/j.ecolind.2021.107659
IPCC, C. C. (2021). The Physical Science Basis. Contribution of Working Group I To The Sixth Assessment Report Of The Intergovernmental Panel on Climate Change. Cambridge and New York, NY: Cambridge University Press.
Isbell, F., Craven, D., Connolly, J., Loreau, M., Schmid, B., Beierkuhnlein, C., et al. (2015). Biodiversity increases the resistance of ecosystem productivity to climate extremes. Nature 526, 574–577. doi: 10.1038/nature15374
Kannenberg, S. A., Novick, K. A., Alexander, M. R., Maxwell, J. T., Moore, D. J. P., Phillips, R. P., et al. (2019). Linking drought legacy effects across scales: from leaves to tree rings to ecosystems. Glob. Chang. Biol. 25, 2978–2992. doi: 10.1111/gcb.14710
Karl, T. R. (1988). Multi-year fluctuations of temperature and precipitation: the gray area of climate change. Clim. Chang. 12, 179–197. doi: 10.1007/BF00138938
Keen, R. M., Voelker, S. L., Wang, S. Y. S., Bentz, B. J., Goulden, M. L., Dangerfield, C. R., et al. (2022). Changes in tree drought sensitivity provided early warning signals to the California drought and forest mortality event. Glob. Chang. Biol. 28, 1119–1132. doi: 10.1111/gcb.15973
Keyimu, M., Li, Z., Wu, X., Fu, B., Liu, G., Shi, S., et al. (2020). Recent decline of high altitude coniferous growth due to thermo-hydraulic constrains: evidence from the Miyaluo Forest reserve, Western Sichuan Plateau of China. Dendrochronologia 63:125751. doi: 10.1016/j.dendro.2020.125751
Klein, T., Zeppel, M. J. B., Anderegg, W. R. L., Bloemen, J., De Kauwe, M. G., Hudson, P., et al. (2018). Xylem embolism refilling and resilience against drought-induced mortality in woody plants: processes and trade-offs. Ecol. Res. 33, 839–855. doi: 10.1007/s11284-018-1588-y
Klesse, S., DeRose, R. J., Babst, F., Black, B. A., Anderegg, L. D. L., Axelson, J., et al. (2020). Continental-scale tree-ring-based projection of Douglas-fir growth: testing the limits of space-for-time substitution. Glob. Chang. Biol. 26, 5146–5163. doi: 10.1111/gcb.15170
Köcher, P., Horna, V., and Leuschner, C. (2013). Stem water storage in five coexisting temperate broad-leaved tree species: significance, temporal dynamics and dependence on tree functional traits. Tree Physiol. 33, 817–832. doi: 10.1093/treephys/tpt055
LeBlanc, D. C. (1990). Relationships between breast-height and whole-stem growth indices for red spruce on Whiteface Mountain, New York. Can. J. For. Res. 20, 1399–1407. doi: 10.1139/x90-185
Lemoine, D., Peltier, J.-P., and Marigo, G. (2001). Comparative studies of the water relations and the hydraulic characteristics in Fraxinus excelsior, Acer pseudoplatanus and A. opalus trees under soil water contrasted conditions. Ann. For. Sci. 58, 723–731. doi: 10.1051/forest:2001159
Liang, H., Huang, J.-G., Ma, Q., Li, J., Wang, Z., Guo, X., et al. (2019). Contributions of competition and climate on radial growth of Pinus massoniana in subtropics of China. Agric. For. Meteorol. 274, 7–17. doi: 10.1016/j.agrformet.2019.04.014
Liang, E., Leuschner, C., Dulamsuren, C., Wagner, B., and Hauck, M. (2016). Global warming-related tree growth decline and mortality on the north-eastern Tibetan plateau. Clim. Chang. 134, 163–176. doi: 10.1007/s10584-015-1531-y
Liu, T., Wu, X., Li, H., Alharbi, H., and Yan, W. (2020). Soil organic matter, nitrogen and pH driven change in bacterial community following forest conversion. For. Ecol. Manag. 477:118473. doi: 10.1016/j.foreco.2020.118473
Luo, D., Huang, J.-G., Jiang, X., Ma, Q., Liang, H., Guo, X., et al. (2017). Effect of climate and competition on radial growth of Pinus massoniana and Schima superba in China’s subtropical monsoon mixed forest. Dendrochronologia 46, 24–34. doi: 10.1016/j.dendro.2017.08.001
Martinez del Castillo, E., Zang, C. S., Buras, A., Hacket-Pain, A., Esper, J., Serrano-Notivoli, R., et al. (2022). Climate-change-driven growth decline of European beech forests. Commun. Biol. 5, 1–9. doi: 10.1038/s42003-022-03107-3
Meinzer, F. C., Woodruff, D. R., Eissenstat, D. M., Lin, H. S., Adams, T. S., and McCulloh, K. A. (2013). Above-and belowground controls on water use by trees of different wood types in an eastern US deciduous forest. Tree Physiol. 33, 345–356. doi: 10.1093/treephys/tpt012
Melo, S. P. (2014). Statistical Analysis of Meteorological Data. FIU Electronic Theses and Dissertations. 1527. doi: 10.25148/etd.FI14071147
Michelot, A., Simard, S., Rathgeber, C., Dufrêne, E., and Damesin, C. (2012). Comparing the intra-annual wood formation of three European species (Fagus sylvatica, Quercus petraea and Pinus sylvestris) as related to leaf phenology and non-structural carbohydrate dynamics. Tree Physiol. 32, 1033–1045. doi: 10.1093/treephys/tps052
Pan, Y., Birdsey, R. A., Fang, J., Houghton, R., Kauppi, P. E., Kurz, W. A., et al. (2011). A large and persistent carbon sink in the world’s forests. Science 333, 988–993. doi: 10.1126/science.1201609
Panthi, S., Fan, Z. X., van der Sleen, P., and Zuidema, P. A. (2020). Long-term physiological and growth responses of Himalayan fir to environmental change are mediated by mean climate. Glob. Chang. Biol. 26, 1778–1794. doi: 10.1111/gcb.14910
Peters, R. L., Groenendijk, P., Vlam, M., and Zuidema, P. A. (2015). Detecting long-term growth trends using tree rings: a critical evaluation of methods. Glob. Chang. Biol. 21, 2040–2054. doi: 10.1111/gcb.12826
Pettitt, A. N. (1979). A non-parametric approach to the change-point problem. J R Stat. Soc. Ser. C 28, 126–135. doi: 10.2307/2346729
Phillips, O. L., Aragão, L. E. O. C., Lewis, S. L., Fisher, J. B., Lloyd, J., López-González, G., et al. (2009). Drought sensitivity of the Amazon rainforest. Science 323, 1344–1347. doi: 10.1126/science.1164033
Piao, S., Wang, X., Park, T., Chen, C., Lian, X., He, Y., et al. (2019). Characteristics, drivers and feedbacks of global greening. Nat. Rev. Earth Environ. 1, 1–14. doi: 10.1038/s43017-019-0001-x
R Core Team (2018). R: A Language and Environment for Statistical Computing. R Foundation for Statistical Computing, Vienna.
Raffa, K. F., Aukema, B. H., Bentz, B. J., Carroll, A. L., Hicke, J. A., Turner, M. G., et al. (2008). Cross-scale drivers of natural disturbances prone to anthropogenic amplification: the dynamics of bark beetle eruptions. Bioscience 58, 501–517. doi: 10.1641/B580607
Rahman, M., Islam, M., and Bräuning, A. (2019). Species-specific growth resilience to drought in a mixed semi-deciduous tropical moist forest in South Asia. For. Ecol. Manag. 433, 487–496. doi: 10.1016/j.foreco.2018.11.034
Rigling, A., Bräker, O., Schneiter, G., and Schweingruber, F. (2002). Intra-annual tree-ring parameters indicating differences in drought stress of Pinus sylvestris forests within the Erico-pinion in the Valais (Switzerland). Plant Ecol. 163, 105–121. doi: 10.1023/A:1020355407821
Rozas, V. (2003). Tree age estimates in Fagus sylvatica and Quercus robur: testing previous and improved methods. Plant Ecol. 167, 193–212. doi: 10.1023/A:1023969822044
Ryberg, K. R., Hodgkins, G. A., and Dudley, R. W. (2020). Change points in annual peak streamflows: method comparisons and historical change points in the United States. J. Hydrol. 583:124307. doi: 10.1016/j.jhydrol.2019.124307
Schwab, N., Kaczka, R. J., Janecka, K., Böhner, J., Chaudhary, R. P., Scholten, T., et al. (2018). Climate change-induced shift of tree growth sensitivity at a central Himalayan treeline ecotone. Forests 9:267. doi: 10.3390/f9050267
Sharma, B., Fan, Z.-X., Panthi, S., Gaire, N. P., Fu, P.-L., and Zaw, Z. (2022). Warming induced tree-growth decline of Toona ciliata in (sub-) tropical southwestern China. Dendrochronologia 73:125954. doi: 10.1016/j.dendro.2022.125954
Stocker, T. F., Qin, D., Plattner, G. K., Tignor, M. M. B., Allen, S. K., Boschung, J., et al. (2014). Climate Change 2013: the Physical Science Basis. Contribution of Working Group I to the Fifth Assessment Report of IPCC the Intergovernmental Panel on Climate Change. Cambridge: Cambridge University Press.
Stokes, M. A., and Smiley, T. L. (1968). An Introduction to Tree-ring Dating. Tucson, AZ: University of Arizona Press.
Su, J., Gou, X., HilleRisLambers, J., Deng, Y., Fan, H., Zheng, W., et al. (2021). Increasing climate sensitivity of subtropical conifers along an aridity gradient. For. Ecol. Manag. 482:118841. doi: 10.1016/j.foreco.2020.118841
Wang, X., Pederson, N., Chen, Z., Lawton, K., Zhu, C., and Han, S. (2019). Recent rising temperatures drive younger and southern Korean pine growth decline. Sci. Total Environ. 649, 1105–1116. doi: 10.1016/j.scitotenv.2018.08.393
Yang, R.-Q., Fu, P.-L., Fan, Z.-X., Panthi, S., Gao, J., Niu, Y., et al. (2022a). Growth-climate sensitivity of two pine species shows species-specific changes along temperature and moisture gradients in Southwest China. Agric. For. Meteorol. 318:108907. doi: 10.1016/j.agrformet.2022.108907
Yang, R.-Q., Zhao, F., Fan, Z.-X., Panthi, S., Fu, P.-L., Bräuning, A., et al. (2022b). Long-term growth trends of Abies delavayi and its physiological responses to a warming climate in the Cangshan Mountains, southwestern China. For. Ecol. Manag. 505:119943. doi: 10.1016/j.foreco.2021.119943
Yu, G., Chen, Z., Piao, S., and Zhu, X. (2014). High carbon dioxide uptake by subtropical forest ecosystems in the east Asian monsoon region. Proc. Natl. Acad. Sci. 111, 4910–4915. doi: 10.1073/pnas.1317065111
Zang, C., and Biondi, F. (2015). Treeclim: an R package for the numerical calibration of proxy-climate relationships. Ecography 38, 431–436. doi: 10.1111/ecog.01335
Zhou, P., Huang, J.-G., Liang, H., Rossi, S., Bergeron, Y., Shishov, V. V., et al. (2021). Radial growth of Larix sibirica was more sensitive to climate at low than high altitudes in the Altai Mountains, China. Agric. For. Meteorol. 304–305:108392. doi: 10.1016/j.agrformet.2021.108392
Zhou, G., Peng, C., and Li, Y. (2013). A climate change-induced threat to the ecological resilience of a subtropical monsoon evergreen broad-leaved forest in southern China. Glob. Chang. Biol. 19, 1197–1210. doi: 10.1111/gcb.12128
Zhu, L., Cooper, D. J., Han, S., Yang, J., Zhang, Y., Li, Z., et al. (2021). Influence of the Atlantic multidecadal oscillation on drought in northern Daxing’an mountains, Northeast China. CATENA 198:105017. doi: 10.1016/j.catena.2020.105017
Zhu, L., Cooper, D. J., Yang, J., Zhang, X., and Wang, X. (2018). Rapid warming induces the contrasting growth of Yezo spruce (Picea jezoensis var. microsperma) at two elevation gradient sites of Northeast China. Dendrochronologia 50, 52–63. doi: 10.1016/j.dendro.2018.05.002
Keywords: tree rings, subtropical forests, rapid warming, tree growth decline, forest management
Citation: Jing M, Zhu L, Liu S, Cao Y, Zhu Y and Yan W (2022) Warming-induced drought leads to tree growth decline in subtropics: Evidence from tree rings in central China. Front. Plant Sci. 13:964400. doi: 10.3389/fpls.2022.964400
Edited by:
Ze-Xin Fan, Xishuangbanna Tropical Botanical Garden (CAS), ChinaReviewed by:
Luca Vitale, National Research Council (CNR), ItalyShankar Panthi, Xishuangbanna Tropical Botanical Garden (CAS), China
Copyright © 2022 Jing, Zhu, Liu, Cao, Zhu and Yan. This is an open-access article distributed under the terms of the Creative Commons Attribution License (CC BY). The use, distribution or reproduction in other forums is permitted, provided the original author(s) and the copyright owner(s) are credited and that the original publication in this journal is cited, in accordance with accepted academic practice. No use, distribution or reproduction is permitted which does not comply with these terms.
*Correspondence: Liangjun Zhu, liangjun_zhu@126.com; Shuguang Liu, shuguang.liu@yahoo.com