- 1Department of Plants and Crops, Faculty of Bioscience Engineering, Ghent University, Ghent, Belgium
- 2Plant Unit, Flanders Research Institute for Agriculture, Fisheries and Food (ILVO), Merelbeke, Belgium
The root-knot nematode, Meloidogyne incognita, is a major pest in tomato production. Paenibacillus polymyxa, which is primarily found in soil and colonizing roots, is considered a successful biocontrol organism against many pathogens. To evaluate the biocontrol capacity of P. polymyxa LMG27872 against M. incognita in tomato, experiments were conducted both in vitro and in vivo. A dose-response effect [30, 50, and 100% (108 CFU/mL)] of bacterial suspensions (BSs) on growth and tomato susceptibility to M. incognita with soil drenching as a mode of application was first evaluated. The results show that the biological efficacy of P. polymyxa LMG27872 against M. incognita parasitism in tomato was dose-dependent. A significantly reduced number of galls, egg-laying females (ELF), and second-stage juveniles (J2) were observed in BS-treated plants, in a dose-dependent manner. The effect of P. polymyxa on tomato growth was also dose-dependent. A high dose of BSs had a negative effect on growth; however, this negative effect was not observed when the BS-treated plants were challenged with M. incognita, indicating tolerance or a defense priming mechanism. In subsequent in vivo experiments, the direct effect of BSs was evaluated on J2 mortality and egg hatching of M. incognita. The effect of BS on J2 mortality was observed from 12 to 24 h, whereby M. incognita J2 was significantly inhibited by the BS treatment. The effect of P. polymyxa on M. incognita egg hatching was also dependent on the BS dose. The results show a potential of P. polymyxa LMG27872 to protect plants from nematode parasitism and its implementation in integrated nematode management suitable for organic productions.
Introduction
Meloidogyne spp. root-knot nematodes (RKNs) are economically important plant-parasitic nematodes (PPNs) with a wide host range (Moens et al., 2009; Jones et al., 2013). They cause gall formation or root knots, disrupting the host plant physiology, thus resulting in crop losses. In some crops, damage caused by RKNs can be extremely high and reach up to 100% (Wesemael et al., 2011). Meloidogyne incognita is one of the most serious belowground pests of tomato, one of the most widely cultivated crops in the world (Seid et al., 2015). In Flanders, RKNs are a major constraint for organic protected fruit vegetable production. While few nematicides are used in the control of this nematode, chemical nematicides are generally non-environmental-friendly and are no option for organic production. Thus, there is a pressing need to promote environment-friendly crop protection products for sustainable agriculture. Microorganisms such as endophytic bacteria that live within a plant at least part of their life cycle may exert beneficial effects on plant growth or health, either directly or indirectly (Vejan et al., 2016). They employ a variety of mechanisms to protect their host plant from biotic and abiotic stress, including the production of antimicrobial metabolites and toxic volatile compounds (VOCs), enhancing tolerance, and activating induced systemic response (ISR) (Gómez-Lama Cabanás et al., 2014; Timmusk et al., 2015; Li and Chen, 2019; Chávez-Ramírez et al., 2020; Mengistu, 2020; Dudeja et al., 2021). They have beneficial effects such as promoting growth via biofertilization, such as fixation of atmospheric nitrogen and converting it to ammonia for plant uptake, phosphate solubilization, or sulfur oxidation (Grayston and Germida, 1991; Turan et al., 2016; Walia et al., 2017; Emami et al., 2020; Langendries and Goormachtig, 2021). Some isolates also can supply or trigger production of phytohormones (Glick et al., 2007; Ek-Ramos et al., 2019; Finkel et al., 2020).
The use of endophytic bacteria has shown promising effects on several crops against PPNs, such as control of M. incognita in tomato (Munif et al., 2013; Li et al., 2019), sponge gourd (Abd El-Aal et al., 2021), cucumber (Hallmann et al., 1998), potato (Hallmann et al., 2001), and coffee (Hoang et al., 2020); M. javanica in tomato (Siddiqui and Ehteshamul-Haque, 2000; Ali Siddiqui and Ehteshamul-Haque, 2001; Siddiqui and Shaukat, 2002a,b) and cotton (Hallmann et al., 1997, 1998, 1999); and control of cyst nematode Globodera pallida in potato (Hasky-Guenther et al., 1998; Reitz et al., 2000). Endophytic bacteria colonize root tissues inter- and/or intracellularly and have a mutualistic interaction with host plants (Schulz and Boyle, 2006; Mandyam and Jumpponen, 2015). They colonize the same niche (root tissues) required by RKNs for survival, leading to competition for space and nutrients in the root, which ultimately results in negative effects on RKNs (Backman and Sikora, 2008; Sikora et al., 2008). Therefore, the association of endophytic bacteria throughout the nematode life cycle makes these bacteria excellent candidates for biocontrol strategies (Siddiqui and Shaukat, 2002a). Endophytic bacteria can affect different stages of the nematode life cycle, such as influencing egg hatching, attraction, and recognition behavior in the rhizosphere, possibly by altering the root exudate patterns (Sikora et al., 2008). They are known to control RKNs at pre-plant and during the vegetative plant growth, by colonizing nematode eggshells or the larval cuticle, or through direct penetration (Hallmann et al., 2009).
In Europe, there are few biological nematicides that are currently in use for the control of PPNs, such as (1) BioAct® or MeloCon® with active ingredient fungus Purpureocillium lilacinum (Kiewnick and Sikora, 2006; Sikora et al., 2008), and (2) Serenade® and VOTiVO® with active ingredient bacteria Bacillus amyloliquefaciens QST713 and B. firmus I-1582 (Terefe et al., 2009; Castillo et al., 2013). However, P. lilacinum effectiveness against Meloidogyne spp. populations was not able to constantly suppress nematode densities under greenhouse conditions (Giné and Sorribas, 2017), and more than one mode of application was necessary for optimum efficacy against M. incognita in tomato (Kiewnick and Sikora, 2004).
This study focused on a Gram-positive, rod-shaped bacterium identified as Paenibacillus polymyxa, isolate LMG27872 (formerly known as Bacillus polymyxa), which resides mainly in the soil, rhizosphere, and plant tissue (Lal and Tabacchioni, 2009). Paenibacillus polymyxa is a rod-shaped endospore-forming bacterium and is thermophilic, facultatively anaerobic, neutrophilic, and sporulating with peritrichous flagella for motility and crawling (He et al., 2007; Langendries and Goormachtig, 2021). Paenibacillus polymyxa has been described as a successful biocontrol organism against various plant pathogens, including bacteria, fungi, oomycetes, and PPNs (Khan et al., 2008; Son et al., 2009; Cheng et al., 2017; Chávez-Ramírez et al., 2020, 2021). It is known to produce antibiotic compounds and secondary metabolites, such as non-ribosomally synthesized lipopeptides, polymyxins, fusaricidin, sideromycins, macrolactin D, and other VOCs, with direct effects on pathogens or inducing resistance through elicitation of plant systemic defense (Raza et al., 2009; Zhao et al., 2011; Lee et al., 2012; Raza et al., 2015; Lal et al., 2016; Cheng et al., 2017; Krämer et al., 2020; Langendries and Goormachtig, 2021; Mülner et al., 2021). Paenibacillus polymyxa is known to secrete siderophores, a known secondary metabolite that scavenges iron, protecting the plants from oxidative stress (Raza et al., 2011; Zhou et al., 2016; Kramer et al., 2020). In addition, it enhances growth via production of several plant growth hormones, supplying plants with nitrate/ammonia, and forming biofilms in roots (Timmusk and Wagner, 1999; Timmusk et al., 2005, 2015; Oleńska et al., 2020; Langendries and Goormachtig, 2021). The aim of this study was to evaluate the biocontrol capacity of P. polymyxa LMG27872 against the RKN, M. incognita, in tomato. Experiments were conducted both in vitro and in vivo. This research first evaluated a dose-response effect of P. polymyxa on tomato susceptibility to nematodes and growth, with soil drenching as a mode of application. The effect of P. polymyxa was observed on different stages of nematodes. Furthermore, in vitro tests were performed to evaluate the direct effect of P. polymyxa on M. incognita J2 mortality and hatching.
Materials and methods
Plant material and growth conditions
Tomato, Solanum lycopersicum cv. Marmande, was used in this study. Seeds were germinated on a sterile potting mixture in a glasshouse (20–26°C, 16-h light). At 2 weeks after germination, seedlings were transferred into small pots (200 mL, one seed per pot) containing a heat-sterilized (100°C, 8 h) soil/sand mixture (1:1, v/v). Plants were kept in a glasshouse (20–26°C, 16-h light) and watered upon requirement.
Bacterial culture
The endophytic P. polymyxa LMG27872 isolate was collected from the rhizosphere of tomato plants in the greenhouse of Flanders Research Institute for Agriculture, Fisheries and Food (ILVO), Merelbeke, Belgium. The isolate was streaked from a Microbank vial (Pro-Lab diagnostics) maintained at –70°C onto Pseudomonas agar F (PAF) supplemented with 10 g L–1 sucrose, instead of glycerol (PAF-S, Becton Dickinson), and incubated for 48 h. The bacterium was grown on agar plates and liquid medium, as illustrated in Hoang et al. (2020). To obtain inoculum, a single colony was transferred into brain heart infusion (BHI; OXOID Ltd., Basingstoke, Hampshire, United Kingdom) liquid medium as pre-culture and incubated overnight in an incubator shaker at 28°C continuously shaken at 200 rpm. From this, 1.5 mL of pre-culture bacterium was poured into 500 mL liquid medium and incubated overnight at 28°C and 200 rpm. The suspension of bacteria from the main culture was transferred to sterilized centrifuge bottles and centrifuged at 4°C for 15 min at 4,000 rpm to form pellets. The supernatant was discarded, and sodium phosphate buffer was added to the pellets; it was gently mixed, and this was considered as a stock solution. The density of the stock solution was determined by using an optical density (OD) spectrophotometer (Thermo Fisher Scientific, United Kingdom) at an absorbance of 600 nm, which was confirmed by plating on PAF-S and calculating the colony-forming unit (CFU/mL) (Beal et al., 2020). An OD of 1 represented a concentration of approximately 1 × 108 CFU mL–1, constituting our stock bacterial suspension (BS) (Su et al., 2017). For further use in our experiments, the BS was diluted in PBS at the following concentrations: BS0, BS30, BS50, and BS100%. Fresh BSs were prepared for each experiment.
Chemical preparation
For nematode parasitism assay (pot experiment), β-aminobutyric acid (BABA) was used as a positive control. The RS BABA racemic mixture was supplied by Eastman, Belgium. A measure of 5 mM BABA was dissolved in water and applied as a soil drench. With this dose, anti-nematode effects have previously been observed on tomato (Oka et al., 1999; Oka and Cohen, 2001; Sahebani and Hadavi, 2009; Cohen et al., 2016) with soil drench as a mode of application. For the in vitro assay on J2 mortality (nematicide test) Velum® Prime (Bayer CropScience), a chemical nematicide with active ingredient fluopyram 1.0 μg/mL, was used as positive control.
Effect of Paenibacillus polymyxa LMG27872 on tomato susceptibility to Meloidogyne incognita
For this experiment, 3-week-old tomato seedlings were soil-drenched with bacterial suspension (BS-treated), water (mock-treated), buffer (negative control), and BABA (positive control). The soil was moistened till just below the field capacity and 3 mL of (30, 50 and 100% BS) was applied. After application, the plants were not watered for 24 h to avoid drainage of the BSs. At 4 weeks after germination (1 week after application of BS), each pot was inoculated with 300 freshly hatched J2 of M. incognita. Each treatment was replicated eight times for each observation. To evaluate the number of penetrated J2, developed J3/J4, and galls and egg-laying females (ELF) in the nematode-parasitized root, samples were collected at 2, 7, and 42 days post-inoculation (dpi). Figure 1 shows the nematode parasitism experiment setup and timeline for evaluation of tomato susceptibility. To visualize the nematodes inside the root system, staining as described by Byrd et al. (1983) was followed with slight modifications. First, the soil was gently washed off the roots and subsequently washed in bleach (1% NaOCl solution), followed by rinsing. The nematode-parasitized roots were then boiled for 1 min in 0.013% acid fuchsin and 0.8% acetic acid solution. The roots were washed under running tap water and incubated in acidified glycerol. This treatment stains nematodes with a purple color, while the rest of the root system is gradually destained in the acidified glycerol. Plant susceptibility was determined by counting the number of galls and ELF, J2, and J3/J4 stages with the aid of a binocular microscope. The number of ELF was used as criterion to measure nematode reproduction. The nematode parasitism experiment was repeated two times.
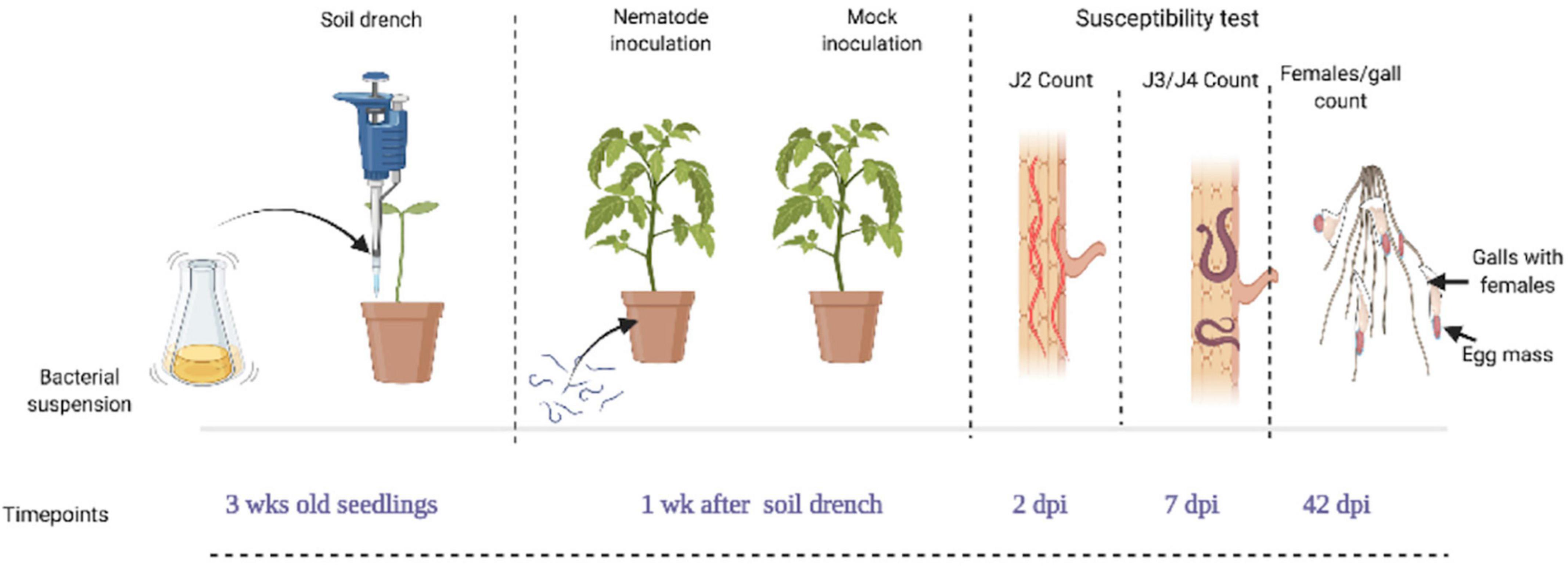
Figure 1. Diagram showing the nematode parasitism experimental setup and timeline for susceptibility test on seedlings. Tomato seedlings of 3 weeks old were soil-drenched with bacterial suspension (BS) at doses of 30, 50, and 100%, while control plants were mock- and buffer-treated (Ctrl and BF) with the positive control, β-aminobutyric acid (BABA). Four weeks after germination (1 week after application of the bacteria), plants were inoculated with 300 M. incognita J2 per plant and were analyzed for the total number of galls and egg laying females (ELF) at 42 days post-inoculation (dpi), the number of J2 and J3/J4 stages at 2 and 7 dpi. This figure was created with www.BioRender.com.
Effect of Paenibacillus polymyxa LMG27872 on tomato growth
To evaluate if P. polymyxa LMG27872 has an effect on plant phenotype, BS-treated plants inoculated with nematodes (parasitized group), from the previous experiment, were measured for their shoot height (SH), root length (RL), shoot weight (SW), and root weight (RW) at 2 dpi corresponding to 9 days posttreatment (dpt), 7 dpi/14 dpt, and 42 dai/49 dpt, respectively. For each dose and time of evaluation, a set of eight additional plants was grown without nematode inoculation but BS-treated; their growth was evaluated at 9, 14, and 49 dpt. SH and RL were measured using a ruler and Fiji software, an image processing package based on ImageJ (Schindelin et al., 2012). Fresh weight was measured, whereby root and shoot tissues were separated. Tissues were blotted dry and weighed using a digital balance (A&D Weighing BM-200 Ion Analytical Balance). The phenotypic effect of applying P. polymyxa LMG27872 on tomato seedlings was also evaluated with an in vitro assay according to Khaliluev et al. (2022) with slight modifications. Seeds were surface-sterilized in NaCl and rinsed in water. A total of 10 seeds were germinated in a moist tissue paper in a sterile Petri dish, and 3 days later, 1 mL of a different dose of BS was applied, by immersing the seedling roots in BS for 30 min. The seedlings were then transferred to MS agar media and kept in an incubator at 24°C. Control groups were mock-treated or with buffer. At 5 days posttreatment, the seedlings were evaluated for their SH, RL, SW, and RW.
Effect of Paenibacillus polymyxa LMG27872 on hatching of Meloidogyne incognita (in vitro test)
The effect of different doses of BS (30, 50, and 100%) on hatching of M. incognita eggs was tested, as described in Wesemael et al. (2006), with slight modifications. In the control treatments, the BS was replaced by distilled water (mock-treated), buffer was the negative control, and Velum® Prime was the positive control. Egg masses were excised from 6-week-old parasitized tomato plants. Eggs were extracted by cutting heavily galled roots into 1-cm pieces and then vigorously shaken in a 1% NaOCl water solution for 3 min. Single eggs were freed from the egg mass, and approximately 100 individual eggs per replicate were exposed to the different BS doses in embryo dishes. The eggs were kept in the dark in an incubator at 25°C. Hatching was monitored with the aid of a binocular microscope after 1, 3, 7, and 14 days. Each treatment was replicated eight times. The number of hatched J2 was counted for each time point, and the relative suppression rate of M. incognita egg hatch was calculated over the time course of 14 days, according to Yang et al. (2016). The relative suppression rate was calculated as follows: Relative suppression rate (%) = (No. of J2 in control – No. J2 in treatments)/No. J2 in control × 100.
Effect of Paenibacillus polymyxa LMG27872 on J2 mortality
The direct effect of P. polymyxa LMG27872 on J2 mortality was evaluated using 100 freshly hatched J2 that were added into cavity blocks with 1-mL high dose (100%) of BS, with a mock-treated and a positive control (Velum® Prime 1.0 μg/mL). The cavity blocks were placed in the dark at 23°C. The J2 motility was monitored by viewing J2 under a dissecting microscope at 6, 12, and 24 h post-incubation. During observation, the immobile J2 were probed with a picking needle; if they were not moving, they were considered dead. After observation, the nematodes were transferred into distilled water to check for recovery. Dead J2 were observed, and mortality was calculated as% of total at respective time point of exposure.
Evaluation of Paenibacillus polymyxa colonization in tomato roots
To evaluate P. polymyxa LMG27872 colonization of the treated plants, an assay was performed, as described in Zhao et al. (2015) and Rat et al. (2021), with slight modifications. Then, 3-week-old tomato seedlings were soil-drenched with high-dose 100% BS (since this is where we observed the most efficient control of nematodes), while control plants were mock-treated. Plant roots were investigated for colonization at three different time points after BS treatment, 1, 3, and 7 days. Segments of roots from five plants per treatment were pooled together and were first cleaned with water and then sterile water to remove remaining soil particles and attached epiphytic bacteria. A paint brush was used to gently remove soil particles without creating any wound. The roots were cut into 1–2-cm sections. These sections were further surface-sterilized by sequential immersion in 70% ethanol for 5 min and then rinsed again for 3 min, followed by further sterilization with a solution of 1.4% of NaOCl for 30 min. The root samples were given a final rinse in sterile water for 10 min and then immersed in 2% Na2SO3 for 10 min to neutralize the effect of bleach. The sterilized roots were placed into a sterile metal mortar, ground to slurry with 0.85% sterile saline, and shaken with a vortex for 1 min. The supernatant was collected for OD measurement. Subsequently, 50 μL of each processed sample suspension was plated (with three replicates per sample) on PAF-S media. The supernatant from the control group (mock-treated) and that from pure stock solution were also plated as negative and positive controls, respectively. All procedures were performed in a laminar flow cabinet. The cultures were incubated at 28°C for 2 d, and the number of colonies was counted. The OD of the supernatant was measured, as described earlier.
Data analysis
Data were analyzed using IBM SPSS (Statistical Package for the Social Sciences), version 17.0 data editor software (SPSS, Chicago, IL, United States). The normality of data was checked by using the Kolmogorov–Smirnov test of composite normality (α = 0.05). Homogeneity of variance was checked by using the Levene test (α = 0.05). If test assumptions were not met, data were taken square root or log-transformed. One-way analysis of variance (ANOVA) and multiple comparisons of differences between treatments were then performed by Tukey’s post hoc multiple range (α = 0.05). Different lowercase letters indicate significant differences between treatments (p < 0.05).
Results
Biological efficacy of Paenibacillus polymyxa LMG27872 on Meloidogyne incognita parasitism in tomato is dose-dependent
To evaluate the biological efficacy of P. polymyxa LMG27872 on M. incognita parasitism in tomato, 3-week-old plants were treated with BS via soil drenching, while control plants were mock- and buffer-treated (Ctrl and BF, respectively). The number of galls on the root system was counted at 42 days post-inoculation (dpi). In comparison with the control group (mock-treated), significantly lower numbers of gall, with 25, 35, and 48% reduction, were observed in plants treated with 30, 50, and 100% BS, respectively (Figure 2A). Plants treated with a high dose (100% BS) exhibited a much stronger effect (25% more effective) than those treated with a low dose (30% BS) (Figure 2A). In addition, smaller galls were observed in plants treated with 100% BS, while mostly large galls were observed in mock-/buffer-treated control groups (Figures 2B,C). To evaluate the effect of P. polymyxa on M. incognita reproduction, the number of total females (TF) and ELF was counted. In comparison with the control group (mock-treated), a significantly low number of TF was observed, with 29, 47, and 55% reduction in plants treated with 30, 50, and 100% BS, respectively (Figure 2D). A notably much stronger and a dose-dependent effect on ELF was observed with a 55, 66, and 83% reduction (Figure 2D). The effect of 50 and 100% BS was at least two- to three-fold stronger in reducing the number of ELF than the 30% BS. Since P. polymyxa LMG27872 had an effect on nematode susceptibility (galls and egg mass at 42 dpi), in the subsequent experiment, the effect of BS dose was evaluated on the J2 stage (effect on penetration) and J3/J4 (developmental stages) at 2 and 7 dpi, respectively. In comparison with the control (mock-treated), the number of J2 was significantly reduced by 21 and 32% in plants treated with 50 and 100% BS (Figure 2E). The ELF is embedded inside the gall and penetrated J2 inside the roots are shown in Figure 2F. At 7 dpi, the number of J3/J4 decreased with increasing doses of BS, but this was not significant (Supplementary Figure 1). Furthermore, at all-time points, significantly lower numbers of nematodes were observed in the positive control (BABA) than in the mock-treated plants (Figures 2A,D,E), validating the parasitism experiments performed using P. polymyxa LMG27872. Furthermore, to evaluate if P. polymyxa LMG27872 colonized the treated plants, an assay was performed with non-parasitized plants at 1, 3, and 7 days after BS treatment. As shown in Supplementary Figure 2, bacterial colonies were detected in PAF-S plates of tomato roots after 3 and 7 days of BS treatment, with supernatant ODs of 0.3 and 0.5, respectively. No colonization was observed at 1 day after BS treatment.
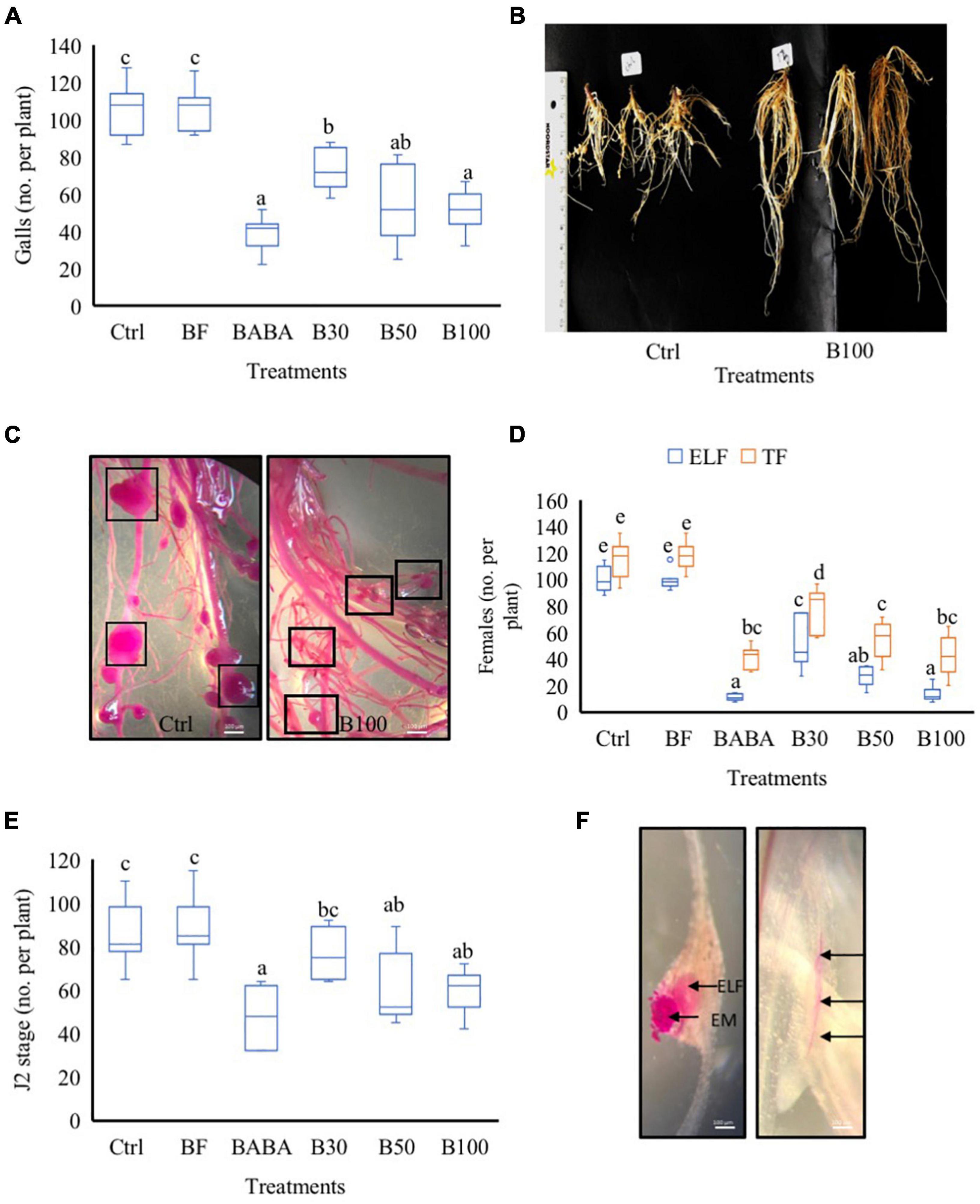
Figure 2. Effect of P. polymyxa LMG27872 strain on M. incognita parasitism in tomato. (A) Total number of galls at 42 dpi. (B) Nematode-parasitized roots of the control group and B100-treated plants at 42 dpi. (C) Acid fuchsin-stained roots showing gall sizes in the control group vs. B100-treated plants at 42 dpi. (D) Number of total females (TF) and the number of egg-laying females (ELF) at 42 dpi. (E) Total number J2 at 2 dpi and (F) acid fuchsin-stained roots of ELF embedded inside the gall, egg mass (EM) on the surface, and J2 inside the roots (depicted with black arrows). Data were analyzed by one-way ANOVA, followed by Tukey’s post-hoc test, and data are shown by box plots with median, upper quartile, and lower quartile. Different letters indicate statistically different means at 95% confidence. Each treatment was replicated eight times (n = 8), and each experiment was repeated at least two times. Bars = 100 μm.
Paenibacillus polymyxa LMG27872 effect on tomato growth is dose-dependent
Treatment with different doses of BS, with or without nematodes, did not cause any significant increase in SH, SW, RL, and RW in comparison with mock-treated plants at 2 dpi/9 dpt (data not shown). At 7 dpi or 14 dpt, a significantly reduced RL (25% reduction) was observed in non-parasitized plants treated with 100% BS when compared to the mock-treated (control not parasitized) tomato plants (Figure 3A). When these (100% BS) treated plants were challenged with nematodes, no negative effect (reduced RL) was observed in comparison with the control parasitized group (Figure 3A). In addition, significantly increased RW was observed in 100% BS-treated + parasitized plants in comparison with ctrl plants (Figure 3B). No effect of 30 and 50% BS was observed on RL and RW for both non-parasitized and parasitized plants. At 7 dpi/14 dpt, no significant changes were observed in SH and SW of plants treated with 30, 50, and 100% BS when compared to control parasitized and non-parasitized groups, respectively (data not shown). At later time points (42 dpi/49 dpt), a dose-dependent effect on growth parameters was observed in BS-treated + parasitized plants. An increase of 10 and 14% on SH (Figure 3C) and 19 and 25% increase in SW (Figure 3D) was observed in 50 and 100% BS-treated + parasitized plants, respectively, when compared with parasitized control plants. No effect of 30% BS was observed on SH and SW for both non-parasitized and parasitized groups (Figures 3C,D). A significant reduced RW (33% reduction) was observed in plants treated with 100% BS when compared to mock-treated (ctrl) non-parasitized plants (Figure 3E). However, when the (100% BS) treated plants were challenged with nematodes, the negative effect (reduced root weight) was not observed in comparison with the parasitized control plants (Figure 3E).
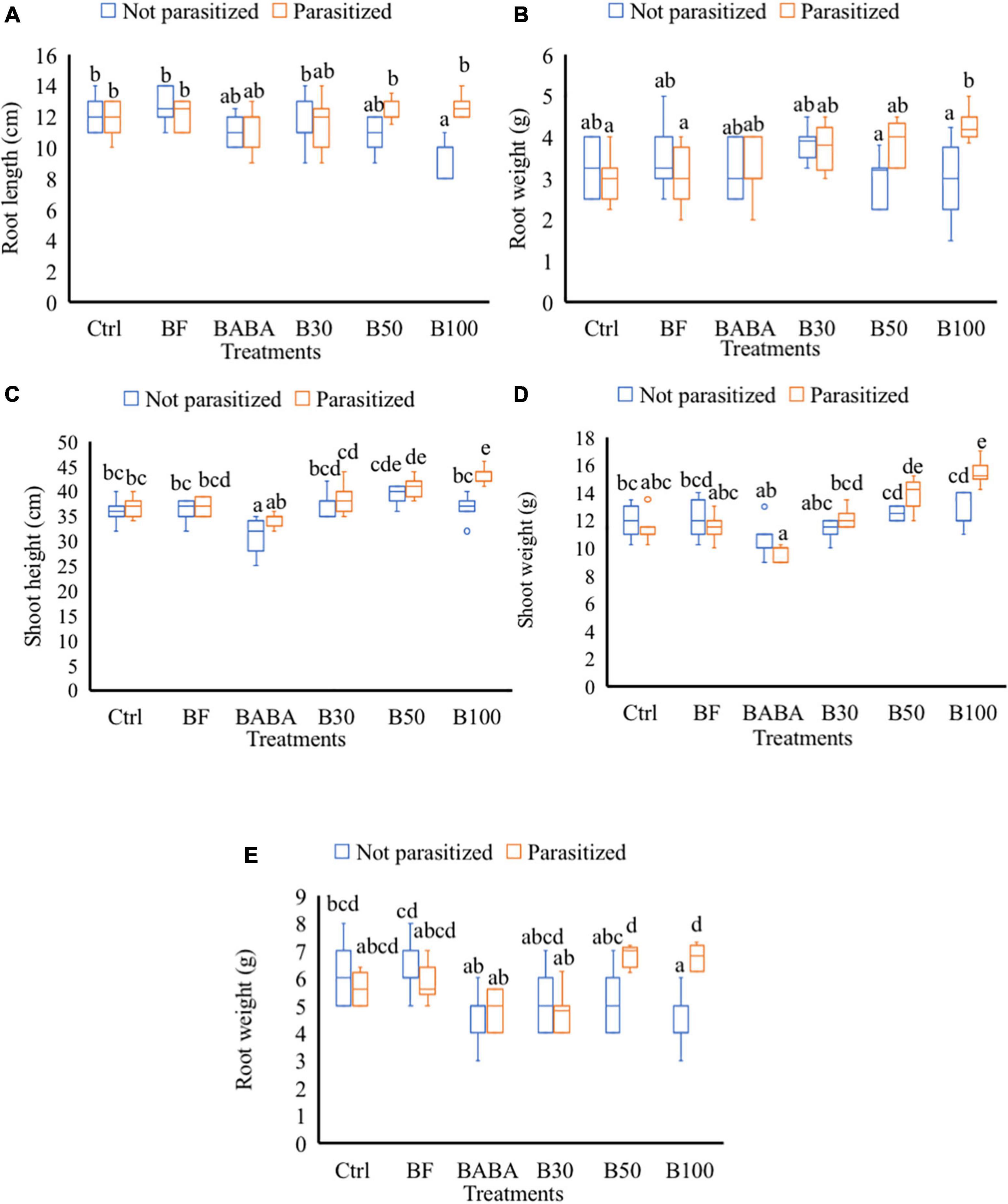
Figure 3. Effect of P. polymyxa strain LMG27872 on tomato growth. A subset of plants (8) from each treatment were inoculated with c. 300 second-stage juveniles of M. incognita (parasitized groups) and a subset of plants were mock-inoculated (not parasitized groups). Data show the effect on plant growth, (A) root length, and (B) fresh root weight at 2 days post-inoculation (dpi) corresponding to 9 days posttreatment (dpt), (C) SH, (D) fresh shoot weight, and (E) fresh root weight at 42 dpi/49 dpt, respectively. Data were analyzed by one-way ANOVA, followed by Tukey’s post-hoc test, and data are shown by box plots with median, upper quartile, and lower quartile. Different letters indicate statistically different means at 95% confidence.
To test whether P. polymyxa affects plant growth, an in vitro experiment was set up to evaluate the effect of P. polymyxa LMG27872 on plant growth. A significant increase of 30 and 42% in the SH (Figures 4A,E) and 23 and 25% in SW (Figures 4B,E) was observed in seedlings treated with 30 and 50% BS, respectively, at 5 dpt when compared to the control plants. On the other hand, a significant decrease of 48, 50, and 50% in the SW (Figures 4B,E), RL (Figures 4C,E), and RW (Figures 4D,E) was observed in seedlings treated with 100% BS, respectively, at 5 dpt compared to control plants (Figure 4A). The effect of P. polymyxa LMG27872 on tomato growth was dose-dependent. While a high dose of bacteria alone affected plant growth, this negative effect was not observed when these bacterium-treated plants were challenged with nematodes. The inhibitory or stimulating effect of the bacteria on tomato growth in vitro also depended on the dose of BS used.
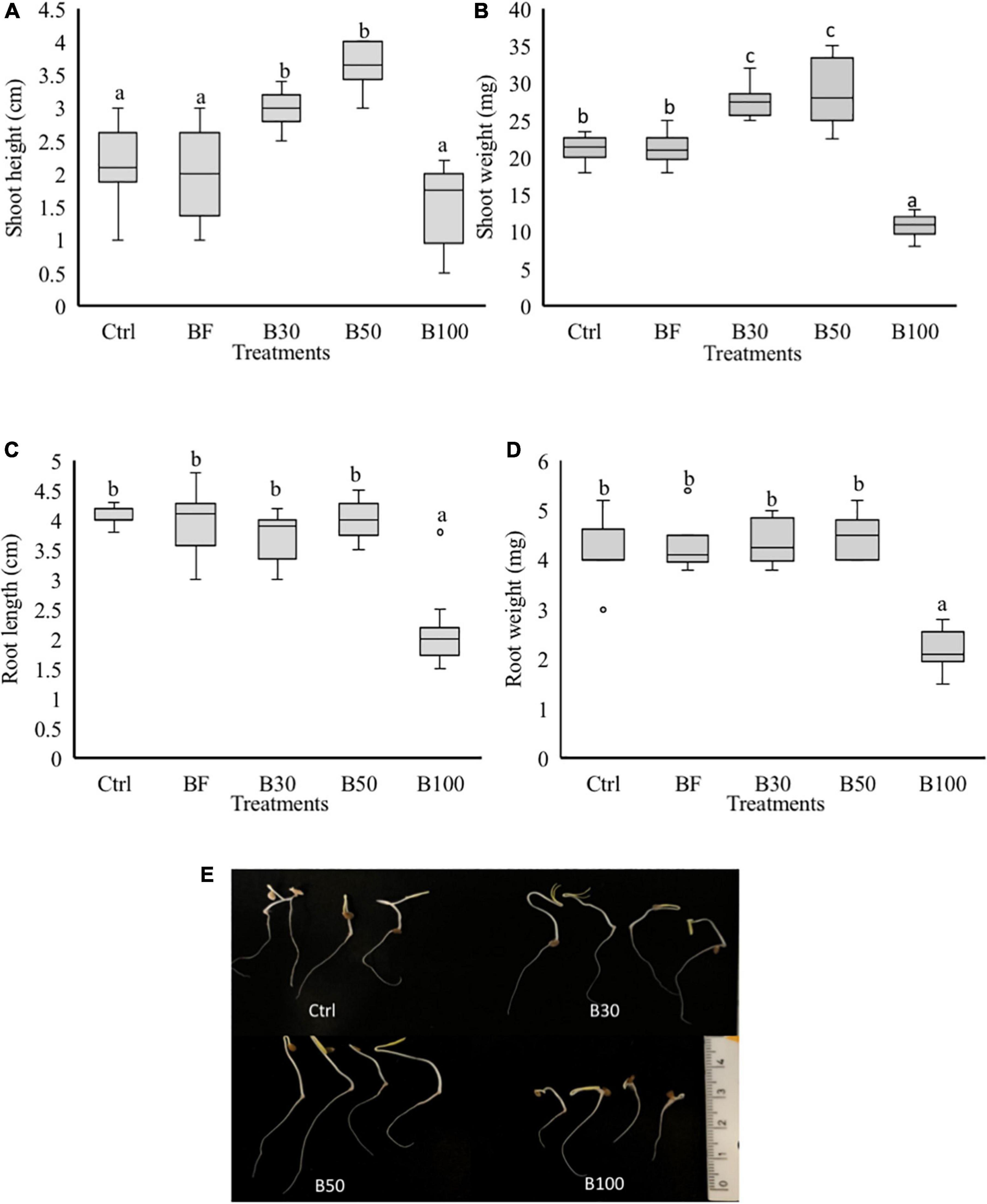
Figure 4. Phenotypic effect of P. polymyxa LMG27872 on tomato seedlings. Treatment of 3-day-old seedlings with 1 mL of bacterial inoculum at different bacterial suspension doses of 30, 50, and 100% was performed, while control plants were mock- and buffer-treated. The (A) SH, (B) SW, (C) RL, and (D) RW measured at 5 days after application. (E) Shoot and root growth of 5-day-old seedlings treated with 30, 50, and 100% BS. Data analyzed by one-way ANOVA, followed by Tukey’s post-hoc test, and data are shown by box plots with median, upper, and lower quartiles. Different letters indicate statistically different means at 95% confidence.
Effect of Paenibacillus polymyxa LMG27872 on hatching and J2 mortality of Meloidogyne incognita
Exposure to P. polymyxa LMG27872 100% BS significantly inhibited M. incognita egg hatching (55% reduced J2) in comparison to the control at day 1 (Figure 5A). At day 3, P. polymyxa 100% BS significantly inhibited M. incognita egg hatching (59% reduced J2) in comparison to control at days 7 days (70% reduced J2) and 14 (72% reduced J2). Although a slight effect of 30 and 50% BS was observed on hatching, it was not significantly different from that of the control (Figure 5A). The relative suppression rate of M. incognita egg hatch was calculated over the time course of 14 days, and the relative suppression rate was 5, 13, and 63% in 30, 50, and 100% BS, respectively. The high dose of 100% BS was 12- and 5-fold more effective than 30 and 50% BS, respectively (Figure 5B). The high dose of 100% BS was selected and tested for its effect on M. incognita J2 mortality. Within 6 h, there was no effect of 100% BS on J2 mortality, while positive control significantly increased the M. incognita J2 mortality, with 18% dead juveniles (Table 1). An effect of 100% BS on J2 mortality was observed at 12- and 24-h exposure time, with mortality of 14 and 60% when compared to the control at respective time points. In general, the effect of BS was visible mostly at 24 h, whereby M. incognita J2 were significantly inhibited by P. polymyxa LMG27872.
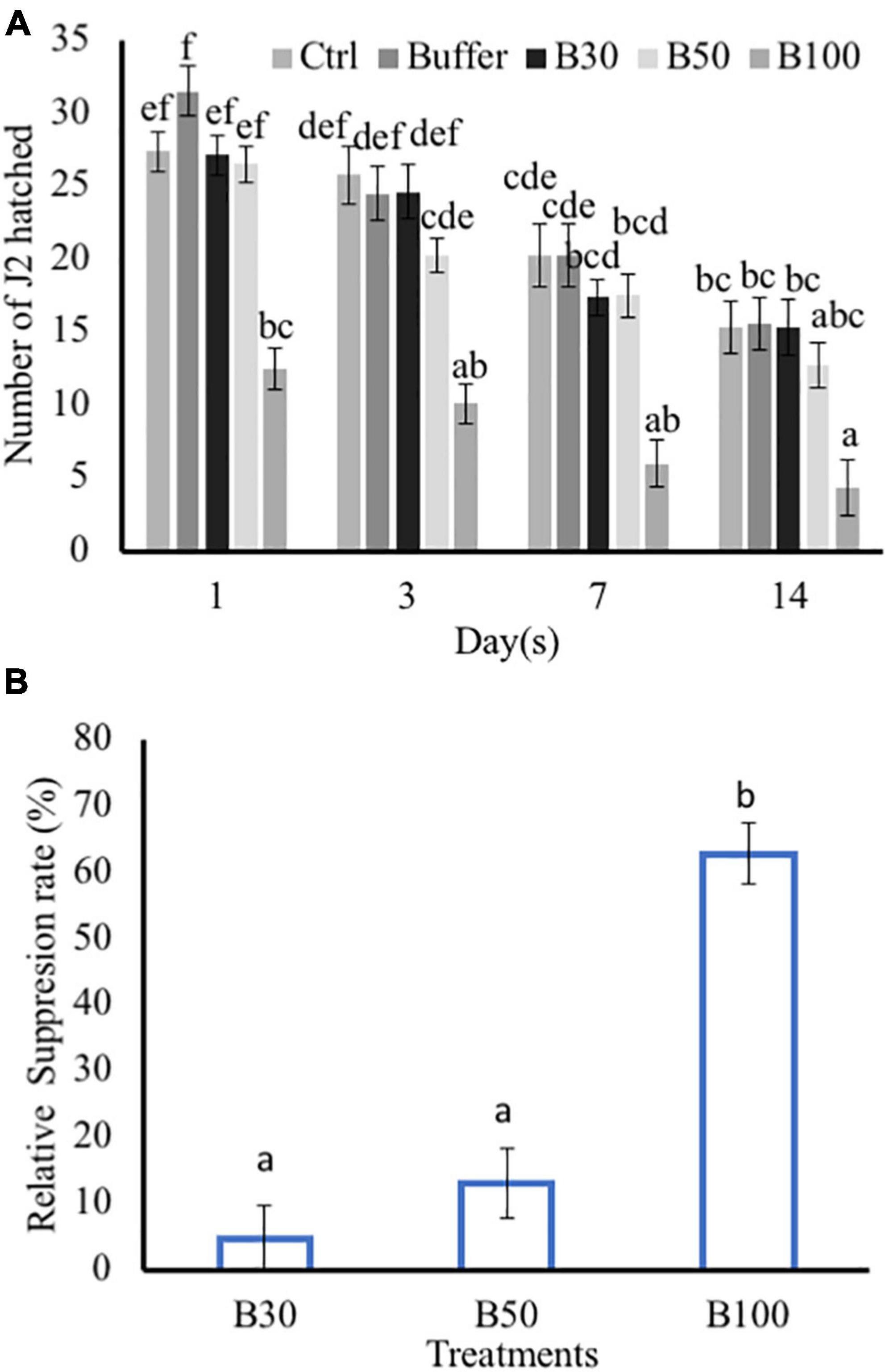
Figure 5. Effect of P. polymyxa strain LMG27872 on hatching of M. incognita in vitro. The effect of different bacterial suspension doses (30, 50, and 100%) on hatching of M. incognita eggs. (A) Number of J2 hatched counted at respective time points and (B) relative suppression rate of M. incognita egg hatching. Data were analyzed by one-way ANOVA, followed by Tukey’s post-hoc test; data are shown by box plots with median, and upper and lower quartiles. Different letters indicate statistically different means at (95% confidence).
Discussion
RKNs are the most damaging PPN group. The pot experiments showed that P. polymyxa LMG27872 inhibited M. incognita parasitism in tomato in a dose-dependent manner. Reduced numbers of galls, egg masses, and J2s were observed in all BS-treated plants, with 100% BS-treated plants exhibiting a much stronger effect on nematode penetration, development, and reproduction. Similarly, application of a BS of P. polymyxa GBR-1 (Khan et al., 2008) and KM2501-1 (Cheng et al., 2017) into potting soil reduced the M. incognita root galls and the number of nematodes in a dose-dependent manner in tomato. The biological efficacy of P. polymyxa was also observed in other crops. A greenhouse experiment on carrots with P. alvei T30 resulted in significantly reduced galls and the egg mass index of M. incognita (Viljoen et al., 2019). The reduced susceptibility observed with P. polymyxa LMG27872 was comparable with positive control RS BABA. RS BABA-induced resistance has previously been described to be effective in tomato against M. incognita (Devran and Baysal, 2018) and M. javanica (Oka et al., 1999). Paenibacillus polymyxa displayed antimicrobial activity against many other plant pathogens, such as hairy root disease, caused by rhizogenic Agrobacterium in tomato (Vanlommel et al., 2020; Vargas et al., 2021a,b, and antifungal activity in peanuts (Arachis villosa) (Costa et al., 2021) and hemp (Cannabis sativa) (Mahmoud and Jabaji, 2021). Other P. polymyxa varieties such as NMA1017 have shown to exert biocontrol activity against Rhizoctonia solani on maize (Zea mays) in vitro, while pot experiments with bean (Phaseolus vulgaris) exhibited the growth of fungal pathogens such as R. solani and Pythium ultimum (Chávez-Ramírez et al., 2020).
A beneficial effect was also reported against Phytophthora tropicalis, on cacao (Theobroma cacao) (Chávez-Ramírez et al., 2021). In contrast to the findings obtained in our research, P. polymyxa LMG27872 showed limited biocontrol activity against the fungal pathogen Botrytis cinerea on strawberry (Fragaria ananassa) and against Rhizoctonia solani on maize (Langendries, 2022). The type of assays, dose, host plants, and pathogens can affect the biological efficacy of this endophytic bacterium. Nevertheless, several in vitro and in vivo studies have demonstrated the potential of several bacteria and fungi as microbial control agents for PPNs (Kumar and Dara, 2021).
Endophytic bacteria are known to produce antimicrobial metabolites, toxic VOCs which can have direct effects on nematodes. P. polymyxa is known to produce an array of compounds and traits with effects on plant growth, biofertilization, biocontrol, and protection against abiotic stresses (Langendries and Goormachtig, 2021). Results from the in vitro assays showed direct effects of P. polymyxa on J2 mortality, comparable with the commercial nematicides used as a control. This is in line with previous research whereby in vitro experiments showed M. incognita (Khan et al., 2008; Viljoen et al., 2019) and M. graminicola (Bui et al., 2020) J2 mortality when exposed to Paenibacillus species.
Endophytic bacteria can directly attack, kill, immobilize, or repel PPNs as they find their host, compete for space, and produce secondary metabolites and other toxic VOCs, such as flavonoids, peptides, quinones, alkaloids, steroids, phenols, terpenoids, and polyketones, or lytic enzymes such as chitinases, cellulases, hemicellulases, and 1,3-glucanases (Gamalero and Glick, 2020; Kumar and Dara, 2021). The differences in the observed phenotypes with different doses of BSs could be due to dilution of already low concentration of the compound/substance present in the suspension. Only further investigations of present compounds in different dilutions can elucidate this. Some endophytic bacteria are also known to produce hydrogen cyanide, suppressing RKNs such as M. incognita by inhibiting mitochondrial respiration (Abd El-Rahman et al., 2019). On the other hand, endophytic bacteria can suppress PPNs indirectly through competition for nutrients, inducing systemic resistance (ISR) and triggering accumulation of hydrogen peroxide and ascorbic acid (Hasky-Guenther et al., 1998; Oka et al., 1999; Reitz et al., 2000; Siddiqui and Shaukat, 2002a; Choudhary et al., 2007; Lee et al., 2012; Vos et al., 2012; Li and Chen, 2019; Gamalero and Glick, 2020). Further investigation on the mode of action of P. polymyxa suppressing M. incognita can shed more light on our findings. Genomic analyses of P. polymyxa LMG27872 showed genes associated with metabolites, such as rhizomide genes encoding chitinases (Langendries, 2022). These metabolites are known to have antimicrobial properties and could explain its biological efficacy. Paenibacillus polymyxa produces avermectin, polymyxin, fusaricidin, and polyketide, which protect plants against pathogens and are involved in activation of ISR (Abd El Daim et al., 2015; Rybakova et al., 2017; Park et al., 2018; Li and Chen, 2019; Chávez-Ramírez et al., 2020). Pot experiments performed here also showed reduced numbers of J2 in P. polymyxa-treated plants in a dose-dependent manner. Less J2 in the roots could be explained by the direct effect of P. polymyxa on the soil by means of VOCs. Also, the formation of biofilms on roots could hamper penetration. Paenibacillus polymyxa is known to form tight biofilms around the root, which produce exopolysaccharides, protecting from pathogenic bacteria and fungi (Haggag and Timmusk, 2008; Timmusk et al., 2009, 2019; Abd El Daim et al., 2015; Luo et al., 2018; Yi et al., 2019). It is also known to repel M. incognita J2 (Hu et al., 2017) when P. polymyxa attaches to the cuticle of M. hapla and activates PAMP-triggered immunity (PTI)-responsive genes in tomato roots, reducing the J2 establishment, confirming an
ISR (Topalović et al., 2019, 2020). In vitro tests showed that P. polymyxa had an inhibitory effect on hatching of M. incognita in a dose-dependent manner. Similarly, exposure of M. incognita to P. polymyxa GBR-1 under in vitro conditions significantly reduced egg hatching in a dose-dependent manner (Khan et al., 2008). Endobacteria are known to inhibit PPNs through direct antagonism with synthesis of lytic enzymes (Gamalero and Glick, 2020). Chitinase produced by P. illinoisensis KJA-424 caused the lysis of M. incognita eggshells and resulted in the inhibition of hatching in vitro (Jung et al., 2002).
In addition to the observed dose effect of P. polymyxa on tomato susceptibility to M. incognita, a dose-dependent effect of the bacterium on tomato growth was also observed. Increased growth linked to the use of the endophytic bacterium has been observed in tomato (Katsenios et al., 2021), barley, cucumber, pepper, sesame, and Arabidopsis thaliana (Jeong et al., 2019). Plant growth-promoting rhizobacteria (PGPR) are known for production and degradation of the phytohormone auxin, leading to enhanced root growth (Leveau and Lindow, 2005; Spaepen et al., 2014; Finkel et al., 2020). The beneficial effect on growth relies on diverse mechanisms, such as acquiring limiting nutrients, that is, phosphate solubilization (Turan et al., 2012), oxidation of sulfur into sulfate (Grayston and Germida, 1991), production of siderophores to capture iron (Marschner et al., 2011), and nitrogen fixation (Hurek and Reinhold-Hurek, 2003; Iniguez et al., 2004). Paenibacillus polymyxa increases soil porosity, fixes nitrogen, and solubilizes phosphorus (Lal and Tabacchioni, 2009; Lal et al., 2016). The isolate MVY-024 was found to significantly increase the amount of ammonium and mineral N in the soil, thus promoting plant growth. However, phytotoxic effects have also been reported (Maes and Baeyen, 2003; Timmusk et al., 2005; Hong et al., 2016). In vitro studies showed that P. polymyxa LMG27872 inhibits maize seed germination in a dose-dependent manner (Maes and Baeyen, 2003).
We also showed a negative effect of P. polymyxa LMG27872 on root and shoot phenotype of tomato. However, in the pot assay, the negative effect on growth was absent when the plants were challenged with M. incognita. The mechanism observed here coincides with a faster and stronger defense response following pathogen attack, a phenomenon termed as defense priming (Conrath et al., 2015; Martinez-Medina et al., 2016; De Kesel et al., 2021). A primed defense response was observed in other studies using endophytic bacteria and fungi to control M. incognita (Vos et al., 2013; Hu et al., 2017; Dahlin et al., 2019; Abd El-Aal et al., 2021). Negative effects of BSs on growth could be linked to genes related to plant cell wall degradation. Endoglucanases, glycoside hydrolases, and xylanases were found in the genome of the bacterium used in this research (Langendries, 2022). Future experiments can be performed to test whether diffusible and/or VOCs affect plant growth.
The tests performed using P. polymyxa showed both direct (nematicidal effects) and indirect effects. This suggest that its mode of action for the biological control of M. incognita may be paralysis and antibiosis, which caused mortality, immobility, prevention of invasion, and inhibition of hatch. Based on this, we suggest double application: one application at seeding or planting, and a second application when egg masses are being formed. Given the fact that soil application will further dilute the BS, 100% BS is recommended.
Conclusion
In conclusion, results showed, for the first time, a biological efficacy of P. polymyxa LMG27872 on M. incognita attacking tomato, and this effect was dose-dependent. The effect of P. polymyxa on tomato growth was also dose-dependent. The highest dose of BS had a negative effect on plant growth. However, such negative effect was not observed when the BS-treated plants were challenged with M. incognita, suggesting tolerance or a defense priming mechanism. The promising results show the potential of P. polymyxa LMG27872 to protect plants from RKN parasitism and its implementation in integrated nematode management suitable for organic production.
Data availability statement
The raw data supporting the conclusions of this article will be made available by the authors, without undue reservation.
Author contributions
RS and WW planned and designed the research. RS conducted parasitism experiments and in vitro tests and wrote the manuscript. WW read, commented, and approved the manuscript. Both authors contributed to the article and approved the submitted version.
Funding
This work was financially supported by the FWO (grant GOE4821N) through “Bio-control of plant-parasitic nematodes on selected medicinal plants in Northern Vietnam.”
Acknowledgments
We would like to acknowledge the contributions of Cinzia Vanmalderghem, Niels Vermassen, and Anne-Marie Deeren of Plant Unit, ILVO.
Conflict of interest
The authors declare that the research was conducted in the absence of any commercial or financial relationships that could be construed as a potential conflict of interest.
Publisher’s note
All claims expressed in this article are solely those of the authors and do not necessarily represent those of their affiliated organizations, or those of the publisher, the editors and the reviewers. Any product that may be evaluated in this article, or claim that may be made by its manufacturer, is not guaranteed or endorsed by the publisher.
Supplementary material
The Supplementary Material for this article can be found online at: https://www.frontiersin.org/articles/10.3389/fpls.2022.961085/full#supplementary-material
References
Abd El Daim, I. A., Haggblom, P., Karlsson, M., Stenstrom, E., and Timmusk, S. (2015). Paenibacillus polymyxa A26 Sfp-type PPTase inactivation limits bacterial antagonism against Fusarium graminearum but not of F. Culmorum in kernel assay. Front. Plant Sci. 6:368. doi: 10.3389/fpls.2015.00368
Abd El-Aal, E. M., Shahen, M., Sayed, S., Kesba, H., Ansari, M. J., El-Ashry, R. M., et al. (2021). In vivo and in vitro management of Meloidogyne incognita (Tylenchida: Heteroderidae) using rhizosphere bacteria, Pseudomonas spp. and Serratia spp. compared with oxamyl. Saudi J. Biol. Sci. 28, 4876–4883. doi: 10.1016/j.sjbs.2021.06.078
Abd El-Rahman, A., Shaheen, H. A., Abd El-Aziz, R. M., and Ibrahim, D. S. (2019). Influence of hydrogen cyanide-producing rhizobacteria in controlling the crown gall and root-knot nematode, Meloidogyne incognita. Egypt. J. Biol. Pest Control 29, 1–11. doi: 10.1186/s41938-019-0143-7
Ali Siddiqui, I., and Ehteshamul-Haque, S. (2001). Suppression of the root rot–root knot disease complex by Pseudomonas aeruginosa in tomato: The influence of inoculum density, nematode populations, moisture and other plant-associated bacteria. Plant Soil 237, 81–89. doi: 10.1023/A:1013313103032
Backman, P. A., and Sikora, R. A. (2008). Endophytes: An emerging tool for biological control. Biol. Control 46, 1–3. doi: 10.1002/jsfa.11042
Beal, J., Farny, N. G., Haddock-Angelli, T., Selvarajah, V., Baldwin, G. S., Buckley-Taylor, R., et al. (2020). Robust estimation of bacterial cell count from optical density. Commun. Biol. 3, 1–29. doi: 10.1038/s42003-020-01127-5
Bui, H. X., Hadi, B. A., Oliva, R., and Schroeder, N. E. (2020). Beneficial bacterial volatile compounds for the control of root-knot nematode and bacterial leaf blight on rice. Crop Prot. 135, 104792. doi: 10.1016/j.cropro.2019.04.016
Byrd, D. Jr., Kirkpatrick, T., and Barker, K. (1983). An improved technique for clearing and staining plant tissues for detection of nematodes. J. Nematol. 15, 142.
Castillo, J. D., Lawrence, K. S., and Kloepper, J. W. (2013). Biocontrol of the reniform nematode by Bacillus firmus GB-126 and Paecilomyces lilacinus 251 on cotton. Plant Dis. 97, 967–976. doi: 10.1094/PDIS-10-12-0978-RE
Chávez-Ramírez, B., Kerber-Diaz, J. C., Acoltzi-Conde, M. C., Ibarra, J. A., Vasquez-Murrieta, M. S., and Estrada-De Los Santos, P. (2020). Inhibition of Rhizoctonia solani RhCh-14 and Pythium ultimum PyFr-14 by Paenibacillus polymyxa NMA1017 and Burkholderia cenocepacia CACua-24: A proposal for biocontrol of phytopathogenic fungi. Microbiol. Res. 230:126347. doi: 10.1016/j.micres.2019.126347
Chávez-Ramírez, B., Rodríguez-Velázquez, N. D., Mondragón-Talonia, C. M., Avendaño-Arrazate, C. H., Martínez-Bolaños, M., Vásquez-Murrieta, M. S., et al. (2021). Paenibacillus polymyxa NMA1017 as a potential biocontrol agent of Phytophthora tropicalis, causal agent of cacao black pod rot in Chiapas. Mexico. Antonie van Leeuwenhoek 114, 55–68. doi: 10.1007/s10482-020-01498-z
Cheng, W., Yang, J., Nie, Q., Huang, D., Yu, C., Zheng, L., et al. (2017). Volatile organic compounds from Paenibacillus polymyxa KM2501-1 control Meloidogyne incognita by multiple strategies. Sci. Rep. 7, 1–11. doi: 10.1038/s41598-017-16631-8
Choudhary, D. K., Prakash, A., and Johri, B. (2007). Induced systemic resistance (ISR) in plants: Mechanism of action. Indian J. Microbiol. 47, 289–297. doi: 10.1007/s12088-007-0054-2
Cohen, Y., Vaknin, M., and Mauch-Mani, B. (2016). BABA-induced resistance: Milestones along a 55-year journey. Phytoparasitica 44, 513–538. doi: 10.1007/s12600-016-0546-x
Conrath, U., Beckers, G. J., Langenbach, C. J., and Jaskiewicz, M. R. (2015). Priming for enhanced defense. Annu. Rev. Phytopathol. 53, 97–119. doi: 10.1146/annurev-phyto-080614-120132
Costa, A., Corallo, B., Amarelle, V., Stewart, S., Pan, D., Tiscornia, S., et al. (2021). Paenibacillus sp. strain UY79, isolated from a root nodule of Arachis villosa, displays a broad spectrum of antifungal activity. Appl.. Environ. Microbiol. 88, 1645–1621. doi: 10.1128/AEM.01645-21
Dahlin, P., Eder, R., Consoli, E., Krauss, J., and Kiewnick, S. (2019). Integrated control of Meloidogyne incognita in tomatoes using fluopyram and Purpureocillium lilacinum strain 251. Crop Prot. 124:104874. doi: 10.1016/j.cropro.2019.104874
De Kesel, J., Conrath, U., Flors, V., Luna, E., Mageroy, M. H., Mauch-Mani, B., et al. (2021). The induced resistance lexicon: Do’s and don’ts. Trends. Plant Sci. 26, 685–691. doi: 10.1016/j.tplants.2021.01.001
Devran, Z., and Baysal, Ö (2018). Induction of resistance to Meloidogyne incognita by DL-Beta amino butyric acid under salt stress condition. Australas. Plant Dis. Notes 13, 1–3. doi: 10.1007/s13314-018-0304-7
Dudeja, S. S., Suneja-Madan, P., Paul, M., Maheswari, R., and Kothe, E. (2021). Bacterial endophytes: Molecular interactions with their hosts. J. Basic Microbiol. 61, 475–505. doi: 10.1002/jobm.202000657
Ek-Ramos, M. J., Gomez-Flores, R., Orozco-Flores, A. A., Rodríguez-Padilla, C., González-Ochoa, G., and Tamez-Guerra, P. (2019). Bioactive products from plant-endophytic Gram-positive bacteria. Front. Microbiol. 10:463. doi: 10.3389/fmicb.2019.00463
Emami, S., Alikhani, H. A., Pourbabaee, A. A., Etesami, H., Motasharezadeh, B., and Sarmadian, F. (2020). Consortium of endophyte and rhizosphere phosphate solubilizing bacteria improves phosphorous use efficiency in wheat cultivars in phosphorus deficient soils. Rhizosphere 14, 100196. doi: 10.1016/j.rhisph.2020.100196
Finkel, O. M., Salas-González, I., Castrillo, G., Conway, J. M., Law, T. F., Teixeira, P. J. P. L., et al. (2020). A single bacterial genus maintains root growth in a complex microbiome. Nature 587, 103–108. doi: 10.1038/s41586-020-2778-7
Gamalero, E., and Glick, B. R. (2020). The use of plant growth-promoting bacteria to prevent nematode damage to plants. Biology 9, 381. doi: 10.3390/biology9110381
Giné, A., and Sorribas, F. J. (2017). Effect of plant resistance and BioAct WG (Purpureocillium lilacinum strain 251) on Meloidogyne incognita in a tomato–cucumber rotation in a greenhouse. Pest Manag. Sci. 73, 880–887. doi: 10.1002/ps.4357
Glick, B. R., Todorovic, B., Czarny, J., Cheng, Z., Duan, J., and Mcconkey, B. (2007). Promotion of plant growth by bacterial ACC deaminase. Crit. Rev. Plant Sci. 26, 227–242. doi: 10.1080/07352680701572966
Gómez-Lama Cabanás, C., Schilirò, E., Valverde-Corredor, A., and Mercado-Blanco, J. (2014). The biocontrol endophytic bacterium Pseudomonas fluorescens PICF7 induces systemic defense responses in aerial tissues upon colonization of olive roots. Front. Microbiol. 5:427. doi: 10.3389/fmicb.2014.00427
Grayston, S. J., and Germida, J. J. (1991). Sulfur-oxidizing bacteria as plant growth promoting rhizobacteria for canola. Can. J. Microbiol. 37, 521–529. doi: 10.1139/m91-088
Haggag, W. M., and Timmusk, S. (2008). Colonization of peanut roots by biofilm-forming Paenibacillus polymyxa initiates biocontrol against crown rot disease. J. Appl. Microbiol. 104, 961–969. doi: 10.1111/j.1365-2672.2007.03611.x
Hallmann, J., Davies, K. G., and Sikora, R. (2009). “Biological control using microbial pathogens, endophytes and antagonists,” in Root-knot nematodes, eds R. N. Perry, M. Moens, and J. L. Starr (Wallingford: CAB International), 380–441. doi: 10.1079/9781845934927.0380
Hallmann, J., Quadt-Hallmann, A., Miller, W., Sikora, R., and Lindow, S. (2001). Endophytic colonization of plants by the biocontrol agent Rhizobium etli G12 in relation to Meloidogyne incognita infection. Phytopathology 91, 415–422. doi: 10.1094/PHYTO.2001.91.4.415
Hallmann, J., Quadt-Hallmann, A., Rodrıguez-Kabana, R., and Kloepper, J. (1998). Interactions between Meloidogyne incognita and endophytic bacteria in cotton and cucumber. Soil Biol. Biochem. 30, 925–937. doi: 10.1016/S0038-0717(97)00183-1
Hallmann, J., Rodrıguez-Kábana, R., and Kloepper, J. (1999). Chitin-mediated changes in bacterial communities of the soil, rhizosphere and within roots of cotton in relation to nematode control. Soil Biol. Biochem. 31, 551–560. doi: 10.1016/S0038-0717(98)00146-1
Hallmann, J., Rodríguez-Kábana, R., Kloepper, J., Ogoshi, A., Kobayashi, K., Homma, Y., et al. (1997). “Nematode interactions with endophytic bacteria,” in Plant growth-promoting rhizobacteria-present status and future prospects, (Sapporo: Nakanishi Printing), 243–245.
Hasky-Guenther, K., Hoffmann-Hergarten, S., and Sikora, R. A. (1998). Resistance against the potato cyst nematode Globodera pallida systemically induced by the rhizobacteria Agrobacterium radiobacter (G12) and Bacillus sphaericus (B43). Fundam. Appl. Nematol. 21, 511–517.
He, Z., Kisla, D., Zhang, L., Yuan, C., Green-Church, K. B., and Yousef, A. E. (2007). Isolation and identification of a Paenibacillus polymyxa strain that coproduces a novel lantibiotic and polymyxin. Appl. Environ. Microbiol. 73, 168–178. doi: 10.1128/AEM.02023-06
Hoang, H., Tran, L. H., Nguyen, T. H., Nguyen, D. A. T., Nguyen, H. H. T., Pham, N. B., et al. (2020). Occurrence of endophytic bacteria in vietnamese robusta coffee roots and their effects on plant parasitic nematodes. Symbiosis 80, 75–84. doi: 10.1007/s13199-019-00649-9
Hong, C. E., Kwon, S. Y., and Park, J. M. (2016). Biocontrol activity of Paenibacillus polymyxa AC-1 against Pseudomonas syringae and its interaction with Arabidopsis thaliana. Microbiol. Res. 185, 13–21. doi: 10.1016/j.micres.2016.01.004
Hu, H.-J., Chen, Y.-L., Wang, Y.-F., Tang, Y.-Y., Chen, S.-L., and Yan, S.-Z. (2017). Endophytic Bacillus cereus effectively controls Meloidogyne incognita on tomato plants through rapid rhizosphere occupation and repellent action. Plant Dis. 101, 448–455. doi: 10.1094/PDIS-06-16-0871-RE
Hurek, T., and Reinhold-Hurek, B. (2003). Azoarcus sp. strain BH72 as a model for nitrogen-fixing grass endophytes. J. Biotechnol. 106, 169–178. doi: 10.1016/j.jbiotec.2003.07.010
Iniguez, A. L., Dong, Y., and Triplett, E. W. (2004). Nitrogen fixation in wheat provided by Klebsiella pneumoniae 342. Mol. Plant Microbe Interact. 17, 1078–1085. doi: 10.1094/MPMI.2004.17.10.1078
Jeong, H., Choi, S.-K., Ryu, C.-M., and Park, S.-H. (2019). Chronicle of a soil bacterium: Paenibacillus polymyxa E681 as a tiny guardian of plant and human health. Front. Microbiol. 10:467. doi: 10.3389/fmicb.2019.00467
Jones, J. T., Haegeman, A., Danchin, E. G., Gaur, H. S., Helder, J., Jones, M. G., et al. (2013). Top 10 plant-parasitic nematodes in molecular plant pathology. Mol. Plant Pathol. 14, 946–961. doi: 10.1111/mpp.12057
Jung, W.-J., Jung, S.-J., An, K.-N., Jin, Y.-L., Park, R.-D., Kim, K.-Y., et al. (2002). Effect of chitinase-producing Paenibacillus illinoisensis KJA-424 on egg hatching of root-knot nematode (Meloidogyne incognita). J. Microbiol. Biotechnol. 12, 865–871.
Katsenios, N., Andreou, V., Sparangis, P., Djordjevic, N., Giannoglou, M., Chanioti, S., et al. (2021). Evaluation of plant growth promoting bacteria strains on growth, yield and quality of industrial tomato. Microorganisms 9, 2099. doi: 10.3390/microorganisms9102099
Khaliluev, M. R., Bogoutdinova, L. R., Raldugina, G. N., and Baranova, E. N. (2022). A simple and effective bioassay method suitable to comparative in vitro study of tomato salt tolerance at early development stages. Method Protoc. 5, 11. doi: 10.3390/mps5010011
Khan, Z., Kim, S., Jeon, Y., Khan, H., Son, S., and Kim, Y. (2008). A plant growth promoting rhizobacterium, Paenibacillus polymyxa strain GBR-1, suppresses root-knot nematode. Bioresour. Technol. 99, 3016–3023. doi: 10.1016/j.biortech.2007.06.031
Khanna, K., Jamwal, V. L., Kohli, S. K., Gandhi, S. G., Ohri, P., Bhardwaj, R., et al. (2019). Role of plant growth promoting Bacteria (PGPRs) as biocontrol agents of Meloidogyne incognita through improved plant defense of Lycopersicon esculentum. Plant soil 436, 325–345. doi: 10.1007/s11104-019-03932-2
Kiewnick, S., and Sikora, R. (2004). Optimizing the efficacy of Paecilomyces lilacinus (strain 251) for the control of root-knot nematodes. Commun. Agric. Appl. Biol. Sci. 69, 373–380.
Kiewnick, S., and Sikora, R. (2006). Biological control of the root-knot nematode Meloidogyne incognita by Paecilomyces lilacinus strain 251. Biol. Control 38, 179–187. doi: 10.1016/j.biocontrol.2005.12.006
Krämer, D., Wilms, T., and King, R. (2020). Model-based process optimization for the production of macrolactin D by Paenibacillus polymyxa. Processes 8, 752. doi: 10.3390/pr8070752
Kramer, J., Özkaya, Ö, and Kümmerli, R. (2020). Bacterial siderophores in community and host interactions. Nat. Rev. Microbiol. 18, 152–163. doi: 10.1038/s41579-019-0284-4
Kumar, K. K., and Dara, S. K. (2021). Fungal and bacterial endophytes as microbial control agents for plant-parasitic nematodes. Intl. J. Environ. Res. Public Health 18, 4269. doi: 10.3390/ijerph18084269
Lal, S., and Tabacchioni, S. (2009). Ecology and biotechnological potential of Paenibacillus polymyxa: A minireview. Indian J. Microbiol. 49, 2–10. doi: 10.1007/s12088-009-0008-y
Lal, S., Chiarini, L., and Tabacchioni, S. (2016). “New insights in plant-associated Paenibacillus species: Biocontrol and plant growth-promoting activity,” in Bacilli and agrobiotechnology, eds M. T. Islam, M. Rahman, P. Pandey, C. K. Jha, and A. Aeron (Cham: Springer International Publishing), 237–279. doi: 10.1007/978-3-319-44409-3_11
Langendries, S., and Goormachtig, S. (2021). Paenibacillus polymyxa, a Jack of all trades. Environ. Microbiol. 23, 5659–5669. doi: 10.1111/1462-2920.15450
Lee, B., Farag, M. A., Park, H. B., Kloepper, J. W., Lee, S. H., and Ryu, C.-M. (2012). Induced resistance by a long-chain bacterial volatile: Elicitation of plant systemic defense by a C13 volatile produced by Paenibacillus polymyxa. PLoS One 7:e48744. doi: 10.1371/journal.pone.0048744
Leveau, J. H., and Lindow, S. E. (2005). Utilization of the plant hormone indole-3-acetic acid for growth by Pseudomonas putida strain 1290. Appl. Environ. Microbiol. 71, 2365–2371. doi: 10.1128/AEM.71.5.2365-2371.2005
Li, X., Hu, H.-J., Li, J.-Y., Wang, C., Chen, S.-L., and Yan, S.-Z. (2019). Effects of the endophytic bacteria Bacillus cereus BCM2 on tomato root exudates and Meloidogyne incognita infection. Plant Dis. 103, 1551–1558. doi: 10.1094/PDIS-11-18-2016-RE
Li, Y., and Chen, S. (2019). Fusaricidin produced by Paenibacillus polymyxa WLY78 induces systemic resistance against Fusarium wilt of cucumber. Intl. J. Mol. Sci. 20, 5240. doi: 10.3390/ijms20205240
Luo, Y., Cheng, Y., Yi, J., Zhang, Z., Luo, Q., Zhang, D., et al. (2018). Complete genome sequence of industrial biocontrol strain Paenibacillus polymyxa HY96-2 and further analysis of its biocontrol mechanism. Front. Microbiol. 9:1520. doi: 10.3389/fmicb.2018.01520
Maes, M., and Baeyen, S. (2003). Experiences and perspectives for the use of a Paenibacillus strain as a plant protectant. Commun. Agric. Appl. Biol. Sci. 68, 457–462.
Mahmoud, M., and Jabaji, S. (2021). Genome sequence of Paenibacillus polymyxa strain HOB6, isolated from hemp seed oil. Microbiol. Resour. Ann. 10:e0034421. doi: 10.1128/MRA.00344-21
Mandyam, K. G., and Jumpponen, A. (2015). Mutualism–parasitism paradigm synthesized from results of root-endophyte models. Front. Microbiol. 5:776. doi: 10.3389/fmicb.2014.00776
Marschner, P., Crowley, D., and Rengel, Z. (2011). Rhizosphere interactions between microorganisms and plants govern iron and phosphorus acquisition along the root axis – model and research methods. Soil Biol. Biochem. 43, 883–894. doi: 10.1016/j.soilbio.2011.01.005
Martinez-Medina, A., Flors, V., Heil, M., Mauch-Mani, B., Pieterse, C. M., Pozo, M. J., et al. (2016). Recognizing plant defense priming. Trends Plant Sci. 21, 818–822. doi: 10.1016/j.tplants.2016.07.009
Mengistu, A. A. (2020). Endophytes: Colonization, behaviour, and their role in defense mechanism. Int. J. Microbiol. 30, 1–8. doi: 10.1155/2020/6927219
Moens, M., Perry, R. N., and Starr, J. L. (2009). “Meloidogyne species–a diverse group of novel and important plant parasites,” in Root-knot nematodes, eds R. N. Perry, M. Moens, and J. L. Starr (Wallingford: CABI International), 1–17. doi: 10.1079/9781845934927.0001
Mülner, P., Schwarz, E., Dietel, K., Herfort, S., Jähne, J., Lasch, P., et al. (2021). Fusaricidins, polymyxins and volatiles produced by Paenibacillus polymyxa DSM 32871 and M1. Pathogens 10:1485. doi: 10.3390/pathogens10111485
Munif, A., Hallmann, J., and Sikora, R. A. (2013). The influence of endophytic bacteria on Meloidogyne incognita infection and tomato plant growth. J. ISSAAS 19, 68–74.
Oka, Y., and Cohen, Y. (2001). Induced resistance to cyst and root-knot nematodes in cereals by DL-β-amino-n-butyric acid. Eur. J. Plant Pathol. 107, 219–227. doi: 10.1023/A:1011278717976
Oka, Y., Cohen, Y., and Spiegel, Y. (1999). Local and systemic induced resistance to the root-knot nematode in tomato by DL-β-amino-n-butyric acid. Phytopathology 89, 1138–1143. doi: 10.1094/PHYTO.1999.89.12.1138
Oleńska, E., Małek, W., Wójcik, M., Swiecicka, I., Thijs, S., and Vangronsveld, J. (2020). Beneficial features of plant growth-promoting rhizobacteria for improving plant growth and health in challenging conditions: A methodical review. Sci. Total Environ. 743, 140682. doi: 10.1016/j.scitotenv.2020.140682
Park, K. Y., Seo, S. Y., Oh, B.-R., Seo, J.-W., and Kim, Y. J. (2018). 2,3-butanediol Induces systemic acquired resistance in the plant immune response. J. Plant Biol. 61, 424–434. doi: 10.1007/s12374-018-0421-z
Rat, A., Naranjo, H. D., Krigas, N., Grigoriadou, K., Maloupa, E., Alonso, A. V., et al. (2021). Endophytic bacteria from the roots of the medicinal plant Alkanna tinctoria Tausch (Boraginaceae): Exploration of plant growth promoting properties and potential role in the production of plant secondary metabolites. Front. Microbiol. 12:633488. doi: 10.3389/fmicb.2021.633488
Raza, W., Makeen, K., Wang, Y., Xu, Y., and Qirong, S. (2011). Optimization, purification, characterization and antioxidant activity of an extracellular polysaccharide produced by Paenibacillus polymyxa SQR-21. Bioresour. Technol. 102, 6095–6103. doi: 10.1016/j.biortech.2011.02.033
Raza, W., Yang, X., Wu, H., Wang, Y., Xu, Y., and Shen, Q. (2009). Isolation and characterisation of fusaricidin-type compound-producing strain of Paenibacillus polymyxa SQR-21 active against Fusarium oxysporum f. sp. nevium. Eur. J. Plant Pathol.S 125, 471–483. doi: 10.3389/fmicb.2021.633488
Raza, W., Yuan, J., Ling, N., Huang, Q., and Shen, Q. (2015). Production of volatile organic compounds by an antagonistic strain Paenibacillus polymyxa WR-2 in the presence of root exudates and organic fertilizer and their antifungal activity against Fusarium oxysporum f. sp. niveum. Biol. Control 80, 89–95. doi: 10.1016/j.biocontrol.2014.09.004
Reitz, M., Rudolph, K., Schroder, I., Hoffmann-Hergarten, S., Hallmann, J., and Sikora, R. (2000). Lipopolysaccharides of Rhizobium etli strain G12 act in potato roots as an inducing agent of systemic resistance to infection by the cyst nematode Globodera pallida. Appl. Environ. Microbiol. 66, 3515–3518. doi: 10.1128/AEM.66.8.3515-3518.2000
Rybakova, D., Rack-Wetzlinger, U., Cernava, T., Schaefer, A., Schmuck, M., and Berg, G. (2017). Aerial warfare: A volatile dialogue between the plant pathogen Verticillium longisporum and its antagonist Paenibacillus polymyxa. Front. Plant Sci. 8:1294. doi: 10.3389/fpls.2017.01294
Sahebani, N., and Hadavi, N. (2009). Induction of H2O2 and related enzymes in tomato roots infected with root-knot nematode (M. javanica) by several chemical and microbial elicitors. Biocontrol Sci. Technol. 19, 301–313. doi: 10.1080/09583150902752012
Schindelin, J., Arganda-Carreras, I., Frise, E., Kaynig, V., Longair, M., Pietzsch, T., et al. (2012). Fiji: An open-source platform for biological-image analysis. Nat. Methods 9, 676–682. doi: 10.1038/nmeth.2019
Schulz, B., and Boyle, C. (2006). “What are endophytes?,” in Microbial root endophytes, eds B. J. E. Schulz, C. J. C. Boyle, and T. N. Sieber (Berlin: Springer-Verlag), 1–13. doi: 10.1007/3-540-33526-9_1
Seid, A., Fininsa, C., Mekete, T., Decraemer, W., and Wesemael, W. M. (2015). Tomato (Solanum lycopersicum) and root-knot nematodes (Meloidogyne spp.)–a century-old battle. Nematology 17, 995–1009. doi: 10.1163/15685411-00002935
Siddiqui, I. A., and Shaukat, S. S. (2002b). Mixtures of plant disease suppressive bacteria enhance biological control of multiple tomato pathogens. Biol. Fertil. Soils 36, 260–268. doi: 10.1094/PDIS-04-17-0478-RE
Siddiqui, I., and Ehteshamul-Haque, S. (2000). Use of Pseudomonas aeruginosa for the control of root rot-root knot disease complex in tomato. Nematologia Mediterranea 28, 189–192.
Siddiqui, I., and Shaukat, S. (2002a). Rhizobacteria-mediated induction of systemic resistance (ISR) in tomato against Meloidogyne javanica. J. Phytopathol. 150, 469–473. doi: 10.1046/j.1439-0434.2002.00784.x
Sikora, R. A., Pocasangre, L., Zum Felde, A., Niere, B., Vu, T. T., and Dababat, A. (2008). Mutualistic endophytic fungi and in-planta suppressiveness to plant parasitic nematodes. Biol. Control 46, 15–23. doi: 10.1016/j.biocontrol.2008.02.011
Son, S., Khan, Z., Kim, S., and Kim, Y. (2009). Plant growth-promoting rhizobacteria, Paenibacillus polymyxa and Paenibacillus lentimorbus suppress disease complex caused by root-knot nematode and fusarium wilt fungus. J. Appl. Microbiol. 107, 524–532. doi: 10.1111/j.1365-2672.2009.04238.x
Spaepen, S., Bossuyt, S., Engelen, K., Marchal, K., and Vanderleyden, J. (2014). Phenotypical and molecular responses of Arabidopsis thaliana roots as a result of inoculation with the auxin-producing bacterium Azospirillum brasilense. New Phytol. 201, 850–861. doi: 10.1111/nph.12590
Su, L., Shen, Z., Ruan, Y., Tao, C., Chao, Y., Li, R., et al. (2017). Isolation of antagonistic endophytes from banana roots against Meloidogyne javanica and their effects on soil nematode community. Front. Microbiol. 8:2070. doi: 10.3389/fmicb.2017.02070
Terefe, M., Tefera, T., and Sakhuja, P. (2009). Effect of a formulation of Bacillus firmus on root-knot nematode Meloidogyne incognita infestation and the growth of tomato plants in the greenhouse and nursery. J. Invertebr. Pathol. 100, 94–99. doi: 10.1016/j.jip.2008.11.004
Timmusk, S., and Wagner, E. G. H. (1999). The plant-growth-promoting rhizobacterium Paenibacillus polymyxa induces changes in Arabidopsis thaliana gene expression: A possible connection between biotic and abiotic stress responses. Mol. Plant Microbe Interact. 12, 951–959. doi: 10.1094/MPMI.1999.12.11.951
Timmusk, S., Copolovici, D., Copolovici, L., Teder, T., Nevo, E., and Behers, L. (2019). Paenibacillus polymyxa biofilm polysaccharides antagonise Fusarium graminearum. Sci. Rep. 9:662. doi: 10.1038/s41598-018-37718-w
Timmusk, S., Grantcharova, N., and Wagner, E. G. H. (2005). Paenibacillus polymyxa invades plant roots and forms biofilms. Appl. Environ. Microbiol. 71, 7292–7300. doi: 10.1128/AEM.71.11.7292-7300.2005
Timmusk, S., Kim, S.-B., Nevo, E., Abd El Daim, I., Ek, B., Bergquist, J., et al. (2015). Sfp-type PPTase inactivation promotes bacterial biofilm formation and ability to enhance wheat drought tolerance. Front. Microbiol. 6:387. doi: 10.3389/fmicb.2015.00387
Timmusk, S., Van West, P., Gow, N., and Huffstutler, R. P. (2009). Paenibacillus polymyxa antagonizes oomycete plant pathogens Phytophthora palmivora and Pythium aphanidermatum. J. Appl. Microbiol. 106, 1473–1481. doi: 10.1111/j.1365-2672.2009.04123.x
Topalović, O., Bredenbruch, S., Schleker, A. S. S., and Heuer, H. (2020). Microbes attaching to endoparasitic phytonematodes in soil trigger plant defense upon root penetration by the nematode. Front. Plant Sci. 11:138. doi: 10.3389/fpls.2020.00138
Topalović, O., Elhady, A., Hallmann, J., Richert-Pöggeler, K. R., and Heuer, H. (2019). Bacteria isolated from the cuticle of plant-parasitic nematodes attached to and antagonized the root-knot nematode Meloidogyne hapla. Sci. Rep. 9, 1–13. doi: 10.1038/s41598-019-47942-7
Turan, M., Gulluce, M., Von Wirén, N., and Sahin, F. (2012). Yield promotion and phosphorus solubilization by plant growth–promoting rhizobacteria in extensive wheat production in Turkey. J. Plant Nutr. Soil Sci. 175, 818–826. doi: 10.1002/jpln.201200054
Turan, M., Kıtır, N., Alkaya, Ü, Günes, A., Tüfenkçi, Ş, Yıldırım, E., et al. (2016). Making soil more accessible to plants: The case of plant growth promoting rhizobacteria. Plant Growth 1, 61–69. doi: 10.5772/64826
Vanlommel, W., Moerkens, R., Bosmans, L., Lievens, B., Rediers, H., Wittemans, L., et al. (2020). Management of hairy root disease in protected tomato crops: A biological and chemical approach. Acta Hortic. 1269, 41–50. doi: 10.17660/ActaHortic.2020.1269.6
Vargas, P., Bosmans, L., Van Calenberge, B., Van Kerckhove, S., Lievens, B., and Rediers, H. (2021a). Bacterial community dynamics of tomato hydroponic greenhouses infested with hairy root disease. FEMS Microbiol. Ecol. 97, fiab153. doi: 10.1093/femsec/fiab153
Vargas, P., Bosmans, L., Van Kerckhove, S., Van Calenberge, B., Raaijmakers, J. M., Lievens, B., et al. (2021b). Optimizing biocontrol cctivity of Paenibacillus xylanexedens for management of hairy root disease in tomato grown in hydroponic greenhouses. Agronomy 11, 817. doi: 10.3390/agronomy11050817
Vejan, P., Abdullah, R., Khadiran, T., Ismail, S., and Nasrulhaq Boyce, A. (2016). Role of plant growth promoting rhizobacteria in agricultural sustainability—a review. Molecules 21, 573. doi: 10.3390/molecules21050573
Viljoen, J. J., Labuschagne, N., Fourie, H., and Sikora, R. A. (2019). Biological control of the root-knot nematode Meloidogyne incognita on tomatoes and carrots by plant growth-promoting rhizobacteria. Trop. Plant Pathol. 44, 284–291. doi: 10.1007/s40858-019-00283-2
Vos, C., Schouteden, N., Van Tuinen, D., Chatagnier, O., Elsen, A., De Waele, D., et al. (2013). Mycorrhiza-induced resistance against the root–knot nematode Meloidogyne incognita involves priming of defense gene responses in tomato. Soil Biol. Biochem. 60, 45–54. doi: 10.1016/j.soilbio.2013.01.013
Vos, C., Tesfahun, A., Panis, B., De Waele, D., and Elsen, A. (2012). Arbuscular mycorrhizal fungi induce systemic resistance in tomato against the sedentary nematode Meloidogyne incognita and the migratory nematode Pratylenchus penetrans. Appl. Soil Ecol. 61, 1–6. doi: 10.1016/j.apsoil.2012.04.007
Walia, A., Guleria, S., Chauhan, A., and Mehta, P. (2017). “Endophytic bacteria: Role in phosphate solubilization,” in Endophytes: Crop productivity and protection, eds D. K. Maheshwari and K. Annapurna (Cham: Springer), 61–93. doi: 10.1007/978-3-319-66544-3_4
Wesemael, W., Perry, R., and Moens, M. (2006). The influence of root diffusate and host age on hatching of the root-knot nematodes. Meloidogyne chitwoodi and M. fallax. Nematology 8, 895–902. doi: 10.1163/156854106779799204
Wesemael, W., Viaene, N., and Moens, M. (2011). Root-knot nematodes (Meloidogyne spp.) in Europe. Nematology 13, 3–16. doi: 10.1163/138855410X526831
Yang, G., Zhou, B., Zhang, X., Zhang, Z., Wu, Y., Zhang, Y., et al. (2016). Effects of tomato root exudates on Meloidogyne incognita. PLoS One 11:e0154675. doi: 10.1371/journal.pone.0154675
Yi, J., Zhang, D., Cheng, Y., Tan, J., and Luo, Y. (2019). The impact of Paenibacillus polymyxa HY96-2 luxS on biofilm formation and control of tomato bacterial wilt. Appl. Microbiol. Biotechnol. 103, 9643–9657. doi: 10.1007/s00253-019-10162-0
Zhao, L., Xu, Y., Lai, X.-H., Shan, C., Deng, Z., and Ji, Y. (2015). Screening and characterization of endophytic Bacillus and Paenibacillus strains from medicinal plant Lonicera japonica for use as potential plant growth promoters. Brazilian J. Microbiol. 46, 977–989. doi: 10.1590/S1517-838246420140024
Zhao, L.-J., Yang, X.-N., Li, X.-Y., Wei, M., and Feng, L. (2011). Antifungal, insecticidal and herbicidal properties of volatile components from Paenibacillus polymyxa strain BMP-11. Agric. Sci. China 10, 728–736. doi: 10.1016/S1671-2927(11)60056-4
Keywords: root-knot nematode, Solanum lycopersicum, hatching, biocontrol, pest management
Citation: Singh RR and Wesemael WML (2022) Endophytic Paenibacillus polymyxa LMG27872 inhibits Meloidogyne incognita parasitism, promoting tomato growth through a dose-dependent effect. Front. Plant Sci. 13:961085. doi: 10.3389/fpls.2022.961085
Received: 03 June 2022; Accepted: 04 August 2022;
Published: 14 September 2022.
Edited by:
Ivânia Esteves, University of Coimbra, PortugalReviewed by:
Aurelio Ciancio, Istituto per la Protezione Sostenibile delle Piante, ItalyChristoph Engl, Queen Mary University of London, United Kingdom
Copyright © 2022 Singh and Wesemael. This is an open-access article distributed under the terms of the Creative Commons Attribution License (CC BY). The use, distribution or reproduction in other forums is permitted, provided the original author(s) and the copyright owner(s) are credited and that the original publication in this journal is cited, in accordance with accepted academic practice. No use, distribution or reproduction is permitted which does not comply with these terms.
*Correspondence: Wim M. L. Wesemael, wim.wesemael@ilvo.vlaanderen.be