- 1The Key Laboratory for Silviculture and Conservation of Ministry of Education, Key Laboratory for Silviculture and Forest Ecosystem of State Forestry and Grassland Administration, Research Center for Urban Forestry, Beijing Forestry University, Beijing, China
- 2The Key Laboratory for Silviculture and Conservation of Ministry of Education, Beijing Forestry University, Beijing, China
- 3Faculty of Life Science, Kim Il Sung University, Pyongyang, North Korea
- 4Jilin Provincial Academy of Forestry Sciences Faculty of Life Science, Changchun, China
Tree thinning affects the light environment, which in turn affects the growth and survival of understory vegetation, thus improving species diversity and nutrient cycling, as well as the ecological habitat factors. However, the response of understory vegetation to the thinning intensity and short-time effects in the temperate broadleaf-conifer mixed forest is not completely clear. In this study, four permanent plots with a total area of 4 hm2 were established in a mixed broadleaf-conifer forest in northeast China, with thinning intensities of 20% (light thinning, LT), 35% (medium thinning, MT), 55% (heavy thinning, HT) and the unthinned plot (CK), respectively, in accordance with the basal area. The responses of species diversity to changes in understory vegetation were conducted by a structural equation model (SEM). The results showed that compared with CK, thinning significantly increased the photosynthetically active radiation (PAR) and the light quality (R/FR) (p < 0.05), while decreased the contents of soil total nitrogen (TN), total phosphorous (TP), organic matter (OM), nitrate nitrogen (NN), ammonia nitrogen (AN) and pH. The degree of fragmentation of light factors among the treatment plots gradually decreased as thinning intensity increased. Among all the thinning treatments, PAR and R/FR were found to be the optimal light condition when the forest thinning intensity was 55%. The light condition was found to have a significant negative correlation with soil TN, TP, OM, and AN. While the soil nutrients were positively correlated with herbaceous layer diversity but negatively correlated with shrub layer diversity. The soil nutrients were lost after thinning in a short time and herb diversity decreased, but shrub diversity increased significantly compared with unthinned plots. For the understory vegetation, the species diversity of shrub and herb layer were showed to be more sensitive to soil nutrients than light environment.
Introduction
Plants with a height lower than 1.3m in the forest are considered understory vegetation, playing a crucial role in the species diversity of the forest ecosystem. Moreover, understory vegetation is of great significance for the nutrient circulation and energy flow of the forest ecosystem, which can change the forest microclimate (Ramovs and Roberts, 2003; Hart and Chen, 2006; Rivaie, 2014; Su et al., 2022). The dynamic adaptation of understory species diversity to the environment can affect the composition and structure of the community and reflect the development stage of the community (Ares et al., 2010). Higher species diversity is conducive to improving the stability of the forest ecosystem (Rota et al., 2014). The availability and heterogeneity of resources have long been regarded as the factors that affect the species’ coexistence, thus altering species diversity (Stein et al., 2014). However, due to the asymmetric consumption of space, light, water, and soil nutrients by the overstory, understory vegetation often exists in a limited resource environment (Su et al., 2019). Previous studies have confirmed that light and soil conditions are generally considered to be the main limiting resources affecting the establishment and growth of understory vegetation (Nicotra et al., 1999; Stein et al., 2014; Zhou et al., 2016; Su et al., 2019; Liu et al., 2021). The reasons for these inconsistent results may be related to stand types, and for the mixed broadleaf-conifer forest, the response of vegetation diversity to light and soil environments was still unclear.
Thinning has been one of the most extensively used forest management by forest managers, and it is essential management to facilitate natural forest structure (Zhu et al., 2010; Verschuyl et al., 2011). Numerous studies have shown that selective logging is capable of reducing stand density, increasing canopy opening, and leading to significant changes in the microenvironment, which has been found as the major factors for forest development (Hale, 2003; Zu-gen et al., 2005; Sercu et al., 2017). Sunlight incident to the ground surface is blocked due to the high canopy density of the unthinned stand, while the canopy structure of the thinned stand can allow a greater amount of light to reach the forest ground surface (Zhu et al., 2003; Zu-gen et al., 2005). Similarly, Nicotra et al. (1999) suggested that thinning can change the availability and spatial patterns of the below-canopy light environment. Noguchi et al. (2017) reported that forest thinning could significantly increase the light intensity in the understory, and the ratio of red to far-red light (R/FR) increased as well. Except for the light environment, thinning has also been shown to affect the physical and chemical properties of soil conditions. Lots of studies argued that soil properties are improved through thinning, e.g., the improvement of soil aeration and water permeability (the increase of soil capillary porosity and total porosity), the contents of soil organic carbon (SOC), soil total nitrogen (TN), total phosphorous (TP), nitrate nitrogen (NN) are significantly increased compared with those of the stand without thinning (Zhou et al., 2016; Dang et al., 2018). However, thinning reduced litter and nutrient return, it may reduce the content of soil nutrients (Choe et al., 2021), especially for the content of nitrogen (N) and phosphorus (P), which were primarily input through litter and reported to be the most common limiting elements in terrestrial ecosystems (Zhou et al., 2021). Therefore, the time after thinning and the thinning intensity may matter most for analyzing how thinning affects soil nutrient content.
How does understory vegetation diversity respond to changes in light and soil environment caused by thinning? One long-standing generalization in ecological research is that thinning created forest open space, improved the light environment in the forest, and increased the plant available resources, which contributed to the changes in understory vegetation diversity (Nicotra et al., 1999; Lencinas et al., 2011; Noguchi et al., 2017; Goodwin et al., 2018; Rossman et al., 2018). Another opposing view is that the improvement of light after thinning will reduce the diversity of understory vegetation. There are two main explanations: (1) In the cases of light-intensity thinning, the light environment changes a little but also produced forest gaps, which would lead to the death of shade-tolerant species (Wang et al., 2021); (2) As for the high-intensity thinning, the understory light is significantly enhanced, while one or few responsive species would quickly monopolize resources, and the other species died from the competition (Newman, 1973; Alaback and Herman, 1988; Thomas et al., 1999). Meanwhile, Soil properties are an important factor driving the distribution pattern of small-scale vegetation species (Siefert et al., 2012), and its influence on plant species diversity is complicated and does not show consistent regularity (Ning et al., 2019). Some studies believed that soil organic matter (SOM) content is a good indicator of soil fertility and nutrient availability, which tend to positively influence plant diversity (Goodall, 1980; Fu et al., 2004). However, Lenière and Houle (2006) found a weak negative relationship between organic matter (OM), TN, and herbaceous layer plant diversity. Some suggest that soil pH affects the activities of many enzymes involved in the mineralization of essential nutrients (N, S, and P), which is related to understory (Palmer, 1991; Chytrý et al., 2003; Weiher et al., 2004). It is well known that in the short term after thinning, only the soil surface layer is significantly affected. Besides, the root system of the herb layer is shallower than that of the shrub layer (Agnelli et al., 2004). Therefore, Rodríguez-Loinaz et al. (2008) reported that soil physicochemical properties tend to significantly positively correlate with the diversity of the herb layer, but not with shrubs. How do habitat factors among light and soil environments affect the understory species diversity? And whether the responses of the herb layer and shrub layer to these factors consistent?
In this study, three different thinning intensities were designed in the mixed broadleaf-conifer forest in northeast China. After thinning for three years, the main habitat factors (e.g., light and soil) were monitored, and the species diversity of shrubs and herbaceous were also investigated. We have discussed the effects of thinning on the soil environment and understory herbaceous diversity before (Choe et al., 2021), but the changes in light environment and shrub diversity were still unclear. Based on the “Niche Partitioning Theory” (NPT), which indicates that abiotic factors have essential roles in regulating species diversity (Austin, 1989; Pacala et al., 1996; Sterck et al., 2011), we constructed our conceptual model guided by the theory and related previous studies (Supplementary Figure 1) (Gerhardt, 1996; Lee et al., 1997; Nicotra et al., 1999; Wetzel and Burgess, 2001; Ediriweera et al., 2008). The data were analyzed using a structural equation model (SEM) to explore (1) the response of both light and soil environment in the mixed broadleaf-conifer forest to different thinning intensities, as well as (2) the influencing mechanism of light and soil environment on the species diversity of understory vegetation after thinning.
Materials and methods
Site description
This study was carried out in the Jiaohe experimental forest (43°51′–44°05′N, 127°35′–127°51′ E, 459m a.s.l), in Jilin Province (Figure 1). The region is characterized by a typical temperate continental monsoon climate with a mean annual precipitation of 800 mm. The average annual temperature is 3.8°C, the hottest month is in July with the average temperature 23.3°C, average temperature of coldest month in January is −15°C. The soil is dark brown forest soil, and the depth range is 20–100 cm. The study site is in a mixed broad-leaved and conifer forest, dominated by Pinus koraiensis, Tilia amurensis, Quercus mongolica, Fraxinus mandshurica, Carpinus cordata, Acer mono and Ulmus davidiana var. japonica. The main shrubs are Philadelphus schrenkii, Deutzia amurensis, Corylus mandshurica, Lonicera japonica and Eleutherococcus Senticosus. The main herb species include Rosaceae Filipendula, Aegopodium alpestre, Impatiens no-litangere, Meehania urticaefolia, Brachybotrys paridiformis, Carex pilosa Scop, etc.
Study site and thinning treatment
In this study, four permanent plots of 1 hectare were set, which were interfered with by thinning with different intensities (the intensity of thinning was obtained in accordance with the sectional area of chest height) in the winter of 2011. The four treatments were no thinning (CK, control), low-intensity thinning (LT, 20% of the trees removed), moderate-intensity thinning (MT, 35% of the trees removed) and high-intensity thinning (HT, 55% of the trees removed). The four plots were near-mature forests, and the information about sampling plots before and after thinning see (Table 1). To acquire more effective information and to be consistent with the principle of model parameter estimation, the grid method (Diggle and Giorgi, 2016; Choe et al., 2021) was adopted to divide the 1-hectare fixed plot (100 m × 100 m) into 400 sub-plots with equal spacing of 5 m × 5 m. The respective small quadrat within each plot was numbered as 1–400, and then 200 quadrats were randomly selected by random number method from each plot to investigate the light factors and plant species. The relative coordinate positions of the respective sampling point were recorded.
Measurement and sampling
Light factors (PAR, R/FR and NDVI), soil nutrients (TN, TP, TK, AN, NN, OM and pH) and understory species diversity (Shannon-Wiener Diversity index) were investigated in four plots with different thinning intensities. The restoration time of the thinning plots was 3 years, and the investigation of the four plots was from July 2014 to September 2015.
Light measurements
The light factors were determined using the 8-channel version of the SpectroSense2 Surface Vegetation Spectrometer (SpectroSense2) based on clear weather conditions (with cloudy or rainy weather avoided). Three optical sensor probes were used, which consisted of PAR, infrared and far-infrared, and these probes were fixed at a height of nearly 1m during the investigation. The measurement began at the same time after the quadrat was selected, and the observation position was obtained. Subsequently, the corresponding data of infrared, far-infrared, and PAR were read directly from the LED screen of the instrument.
The measured data could be exported by software. Moreover, the data could be edited and counted in Excel and Word. The average value of PAR, R/FR (red/far-red) was used for calculation (light quality). Next, the average value was obtained. Normalized vegetation index (NDVI) refers to a transformation form of near-infrared and RED channel reflectance ratio (SR = NIR/RED), NDVI = (NIR-R)/(NIR+R).
Soil sampling
We divided each 1-hectare plot (100 m × 100 m) into 4 sub-plots with equal spacing of 50 m × 50 m for soil samples, and 25 sampling sites were sited in each sub-plots. The samples of 0–20 cm soil layer were collected using a ring knife. Plant residues, gravel and other sundries in the unearthed samples were selected, and the fresh soil samples were dried and screened. The pH was obtained using the electrode potential method (Richards, 1954). Organic matter (OM) was obtained using the potassium dichromate volumetric method in combination with the external heating method (Walkley and Black, 1934). Total nitrogen (TN) was determined by the Kjeldahl method. Total phosphorus (TP) was determined by the Mo-Sb colorimetric method (Li and Wang, 2012). Total potassium (TK) was obtained through NaOH melting flame photometry. Soil ammonium nitrogen (AN) and nitrate nitrogen (NN) were extracted with potassium chloride and then measured with the flow analyzer (FIA) (Wilke et al., 2005).
Shrub and herb diversity
The understory species diversity in plots was investigated using the ShannonWiener Diversity index (H). The species diversity is expressed as:
Ni denotes the number of the i-th species; N represents the number of all intermediate individuals.
Statistical analyses
A Generalized Linear Mixed Model (GLMM) and One-way ANOVA to analyze the effect of thinning intensities on light condition, soil nutrients and understory species diversity. The Kolmogorov-Smirnov (K-S) test was performed to examine the normality of the data. The data not consistent with the normal distribution was converted. The habitat factors (PAR, R/FR, NDVI, TN, TP, TK, pH, OM, AN, NN) were extracted using the PCA into a few principal components and represented the light and soil environments in subsequent analyses first (García-Palacios et al., 2017; Ruiz-Benito et al., 2017; Hao et al., 2020). And a SEM (Supplementary Figure 1) were developed to explore how habitat factors (light and soil environment) affect the shrub and herb diversity. Besides, Bentler’s comparative fit index (CFI) and root mean square error of approximation (RMSEA) were employed as the fitting goodness indicator. The principal component analysis and the SEM were conducted with software SPSS26.0 and Amos, respectively. All statistical analyses were performed using the R software. The GLMM was implemented using the “lme4” package (Lingjun et al., 2019), and SEM using the “lavaan” package (Rosseel, 2012). Kriging interpolation mapping was generated using the software of ArcGIS10.2.
Results
Effects of thinning on light factors
Effects of different thinning intensities on light factors
The GLMM and ANOVA results showed that there were significant differences among the light factors in different thinning intensities (Figure 2 and Supplementary Table 1). The mean value of PAR ranged from 169.0 (CK) to 515.46 (HT), and the mean value increased with the increase in the thinning intensity, whereas no significant difference was found between the control group and the low-intensity thinning group (LT). Moreover, the control group and the low-intensity thinning group were significantly lower than the moderate and high thinning groups (MT and HT), and no significant difference was found in PAR between the moderate and high thinning groups. The mean value of R/FR ranged from 0.499 (CK) to 0.628 (HT), and it increased monotonically as the thinning intensity increased. The mean value of R/FR in the control group was significantly lower than that in the thinning group, whereas no significant difference was identified in the mean value of R/FR between the three thinning groups. The lowest Normalized vegetation index (NDVI) was 0.613 ± 0.0083 when the thinning intensity was 35%.
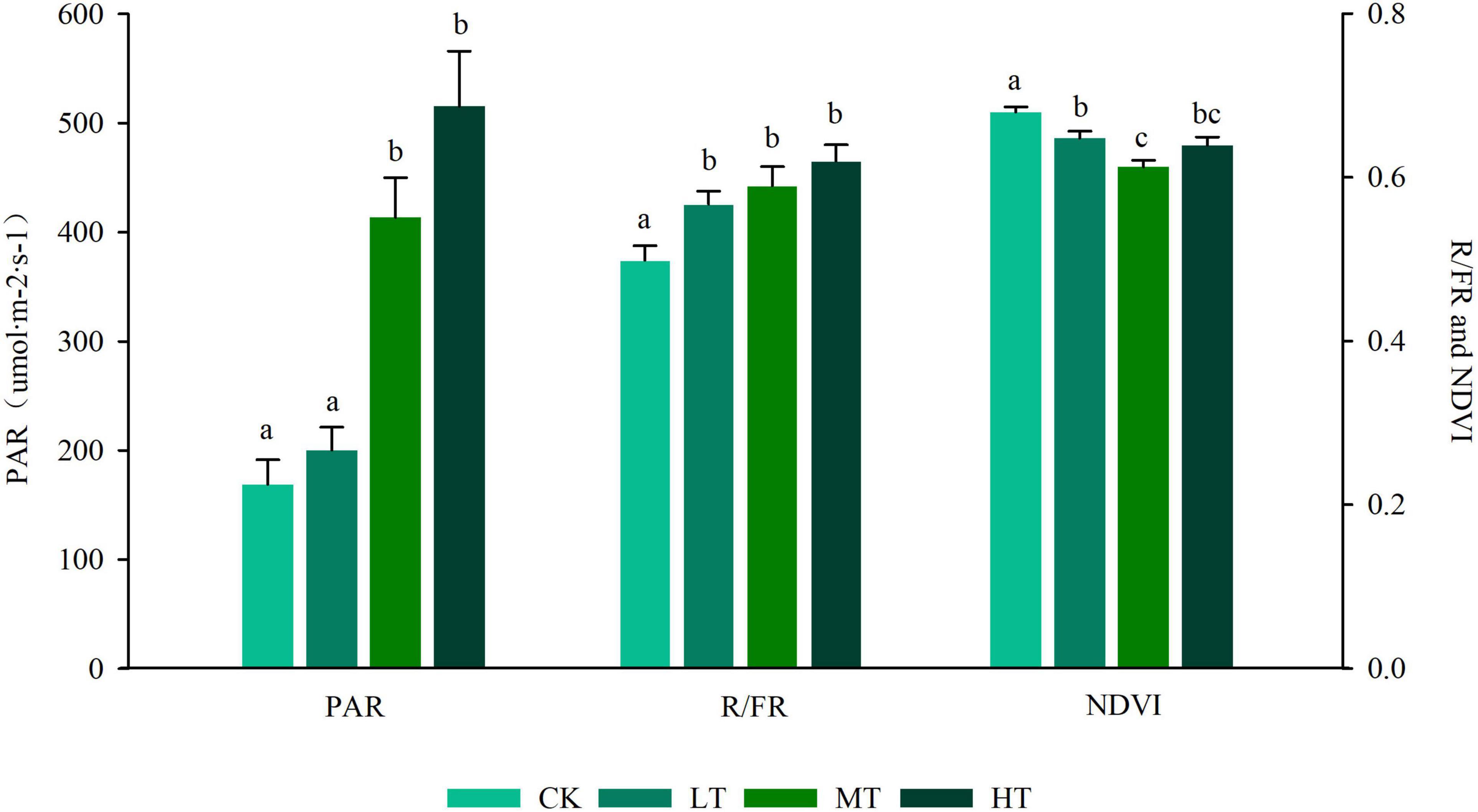
Figure 2. Response of light factors on the different strength of thinning. The left ordinate represents photosynthetically active radiation (PAR), and the right represents normalized vegetation index (NDVI) and R/FR. Different letters of the mean values represent significant differences (p < 0.05). CK, unthinned plot; LT, thinning intensity was 20%; MT, thinning intensity was 35%; HT, thinning intensity was 55%.
Spatial distribution pattern of light factors
In accordance with the semi-variogram theory and the structural analysis, the kriging method was used to optimize the interpolation of the sampling area and generate the isoline map of light factors in the stand with different thinning intensities. The distribution of PAR in the control group was patchy with a high fragmentation degree, and most areas of the stand received less PAR. With the increase of thinning intensity, the area that can receive light in the treatment stands gradually expands, and the degree of PAR fragmentation gradually decreases. The area of PAR higher than 460.945μmol⋅m–2⋅s–1 accounted for two thirds of the total area of the sample plot characterized by high-intensity thinning (Figure 3). The distribution of R/FR in different thinning intensities was patchy. The degree of R/FR fragmentation in the CK and the MT plots was higher than that of the LT and HT plots, while the area with high R/FR (greater than 0.848) in the MT plot was the largest among the four plots (Figure 4).
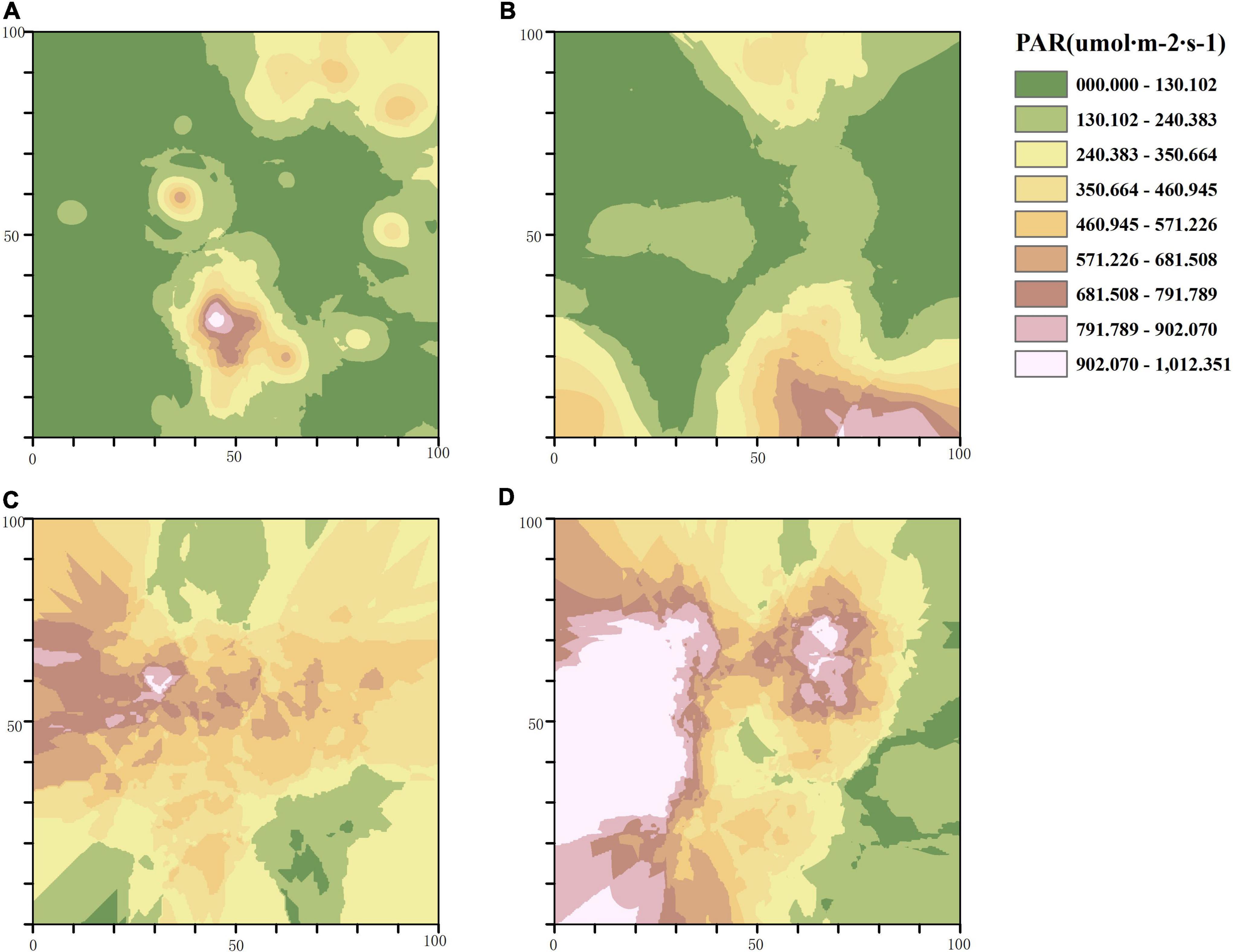
Figure 3. Spatial distribution pattern of photosynthetically active radiation (PAR). (A) Unthinned plot (CK), (B) light thinning plot (LT), (C) medium thinning plot (MT), (D) heavy thinning plot (HT).
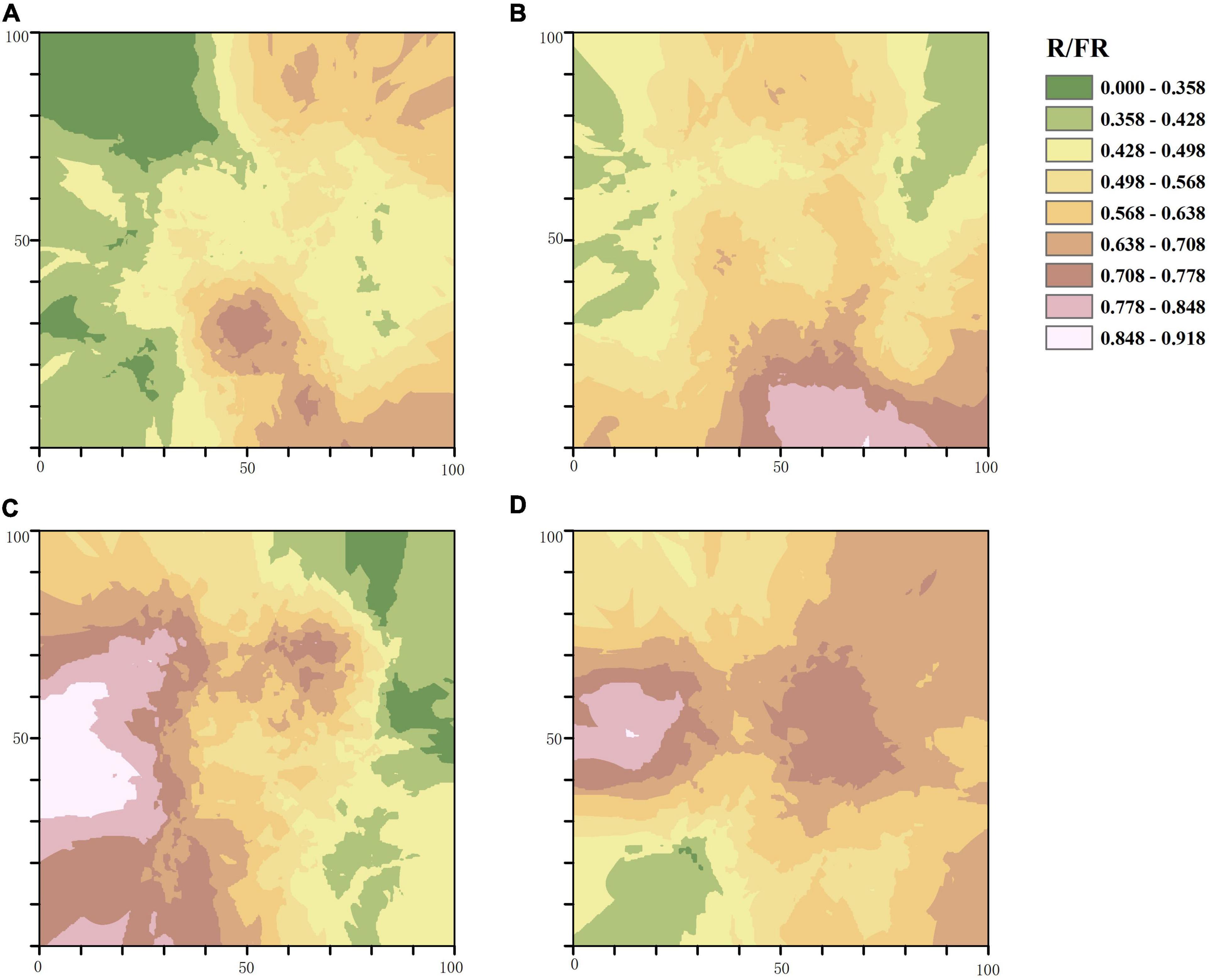
Figure 4. Spatial distribution pattern of R/FR. (A) Unthinned plot (CK), (B) light thinning plot (LT), (C) medium thinning plot (MT), (D) heavy thinning plot (HT).
Effects of thinning on soil conditions
Different thinning intensities exerted different effects on soil nutrient indexes (Table 2 and Supplementary Table 1). The TN content in soil tended to decrease with the increase of the cutting intensity. Besides, a significant difference was found between the CK group and thinning treatments, whereas there was no significant difference among the three treatment groups. Significant differences were found in TP and NN between the CK and thinning treatments. The F values of pH, OM and AN contents were 30.598, 31.856 and 25.017, respectively. Furthermore, Sig values were all lower than 0.01, thus suggesting that different thinning intensities significantly affected pH, OM and AN. The F value and Sig value of TK were 2.032 and 0.109, thus revealing that different thinning intensities did not significantly affect TK (p < 0.05).
Effect of thinning intensities on understory species diversity
Different thinning intensities exerted different effects on shrub and herb layers diversity (Table 3 and Supplementary Table 2). The Shannon-Wiener diversity index of herb layers in the CK group is highest, followed by the MT plot. As for the shrub layers, the CK group was the lowest compared with the thinned group (p < 0.05).
Effect of light and soil environment on understory species diversity
A principal component analysis (PCA) was conducted to compress the variation of light factors into several principal components. The first principal component (LE), which represented 43.8% of the total variation (Figure 5) was selected to represent the main information of the lighting environment. Other components with the eigenvalue less than 1 was abandoned in this analysis. Likewise, using the same procedure, we conducted a PCA to extract the main information from the seven soil properties. The first two principal components (SE1 and SE2), which jointly captured 64.4% of the total variation were retained (Figure 6). Through the principal component analysis (PCA), the dimension reduction analysis was conducted on the original habitat data. LE, SE1 and SE2 basically represented the information of light and soli environmental factors, respectively.
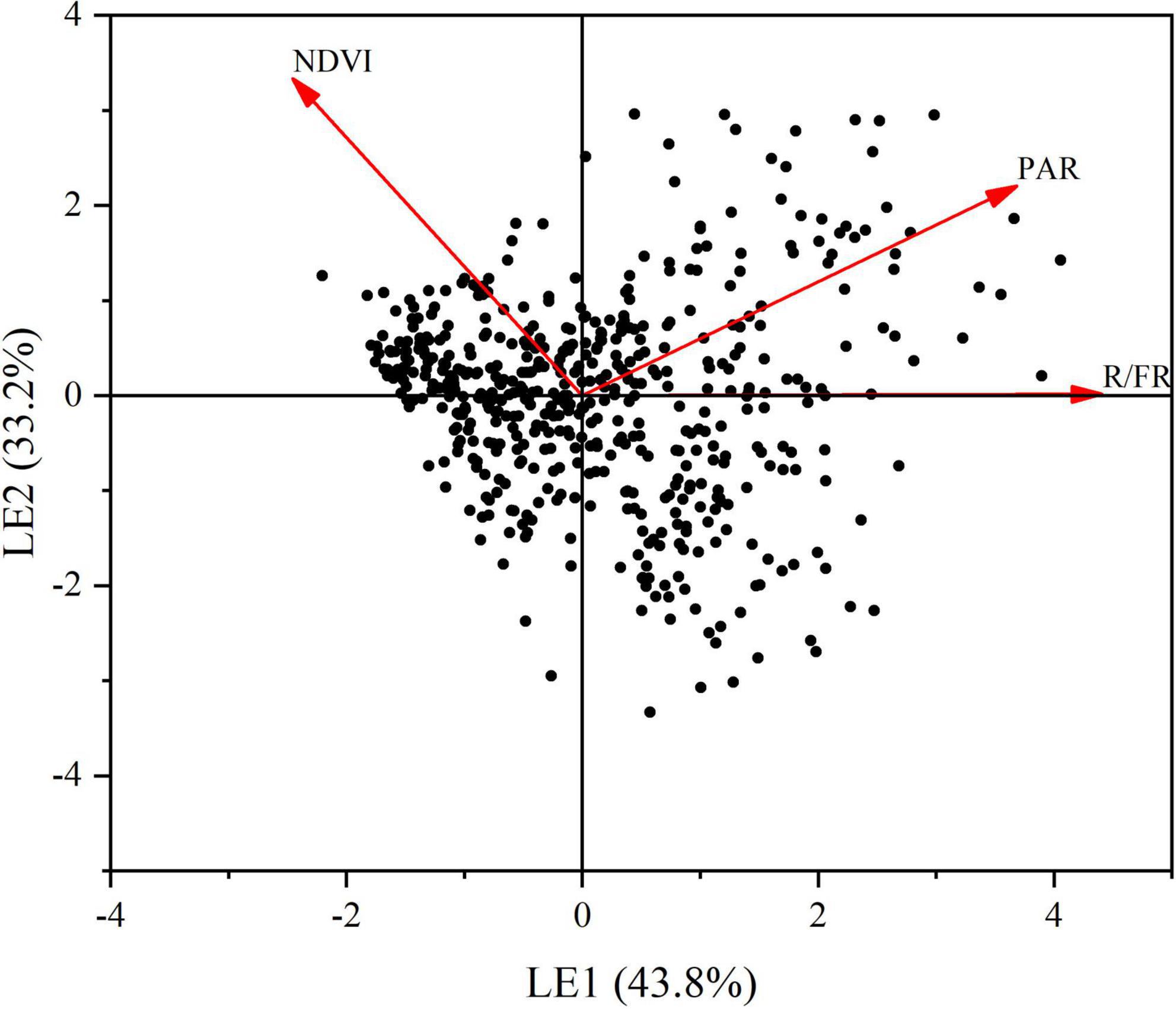
Figure 5. Principal component analysis (PCA) of light environment. Red arrows depict the loads of pearson correlations between light environmental variables including photosynthetically active radiation (PAR), R.FR and normalized vegetation index (NDVI). Variance explained by each principal component is shown in brackets. LE: light environment. LE1: the first axes of a principal component. LE2: the second axes of a principal component.
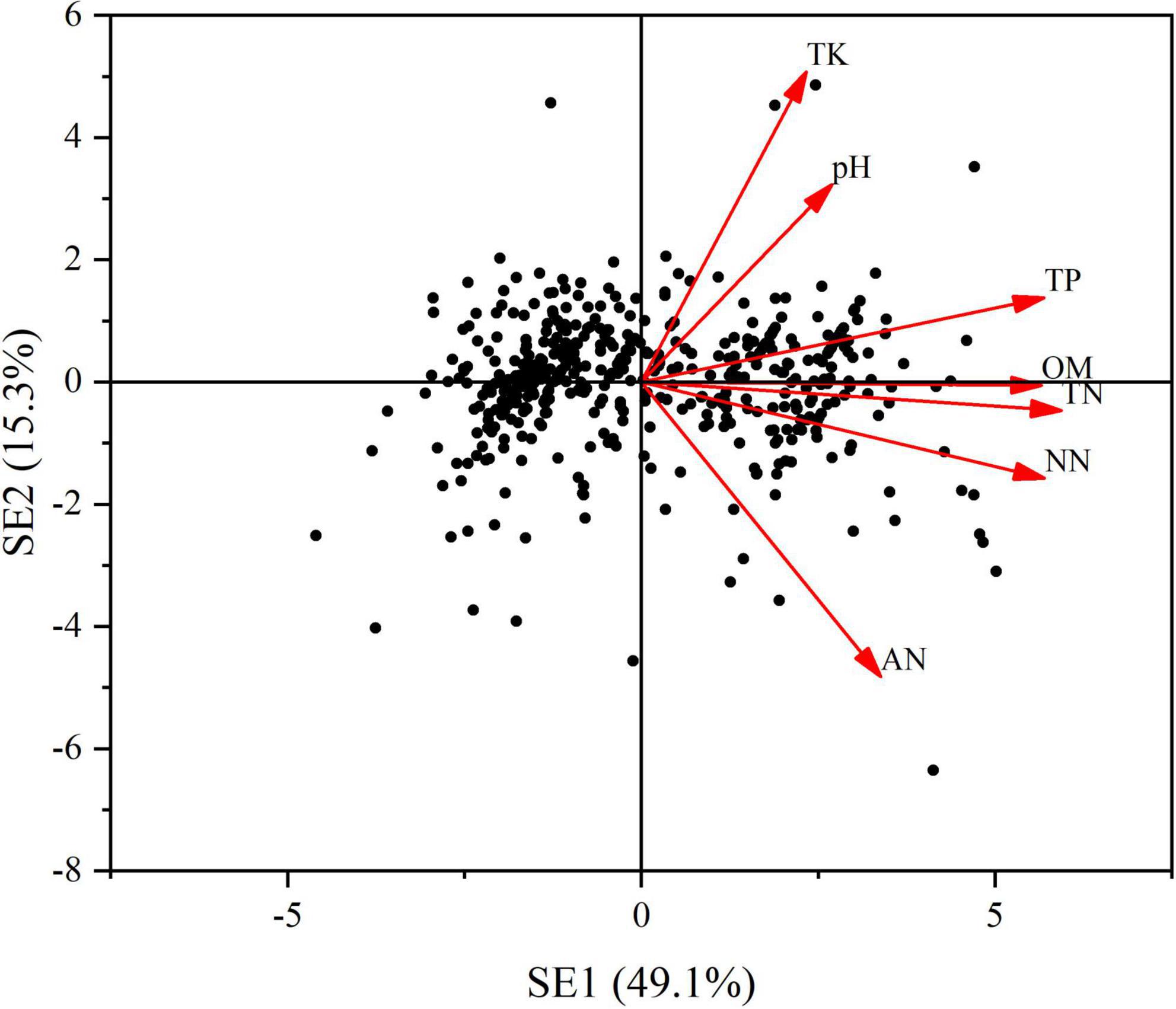
Figure 6. Principal component analysis (PCA) of soil nutrients. Red arrows depict the loads of pearson correlations between light environmental variables including TN, TP, TK, pH, OM, AN, and NN. Variance explained by each principal component is shown in brackets. SE: soil nutrients. SE1: the first axes of a principal component. SE2: the second axes of a principal component.
Thus, we used these principal components to build a SEM. The results confirmed that our structural equation model had a good fit to the data (CFI = 1.00, RMSEA = 0.00, and CMIN/DF = 0.183) (Figure 7). The light factor (LE) in the stand after thinning had a significant negative effect on SE1 (the standardized path coefficients: r = −0.308, p < 0.05), while LE did not significantly affect SE2 (r = −0.087, p > 0.05). The correlation between light and Shannon-Weiner index of understory were not significant. However, the soil nutrients (SE1 and SE2) showed a negative correlation with Shannon-Weiner index of shrub but a positive correlation with Shannon-Weiner index of herb. The relationship between shrubs and herbs was not significant. Furthermore, light indirect affects shrub and herb Shannon-Weiner index by directly changing soil conditions.
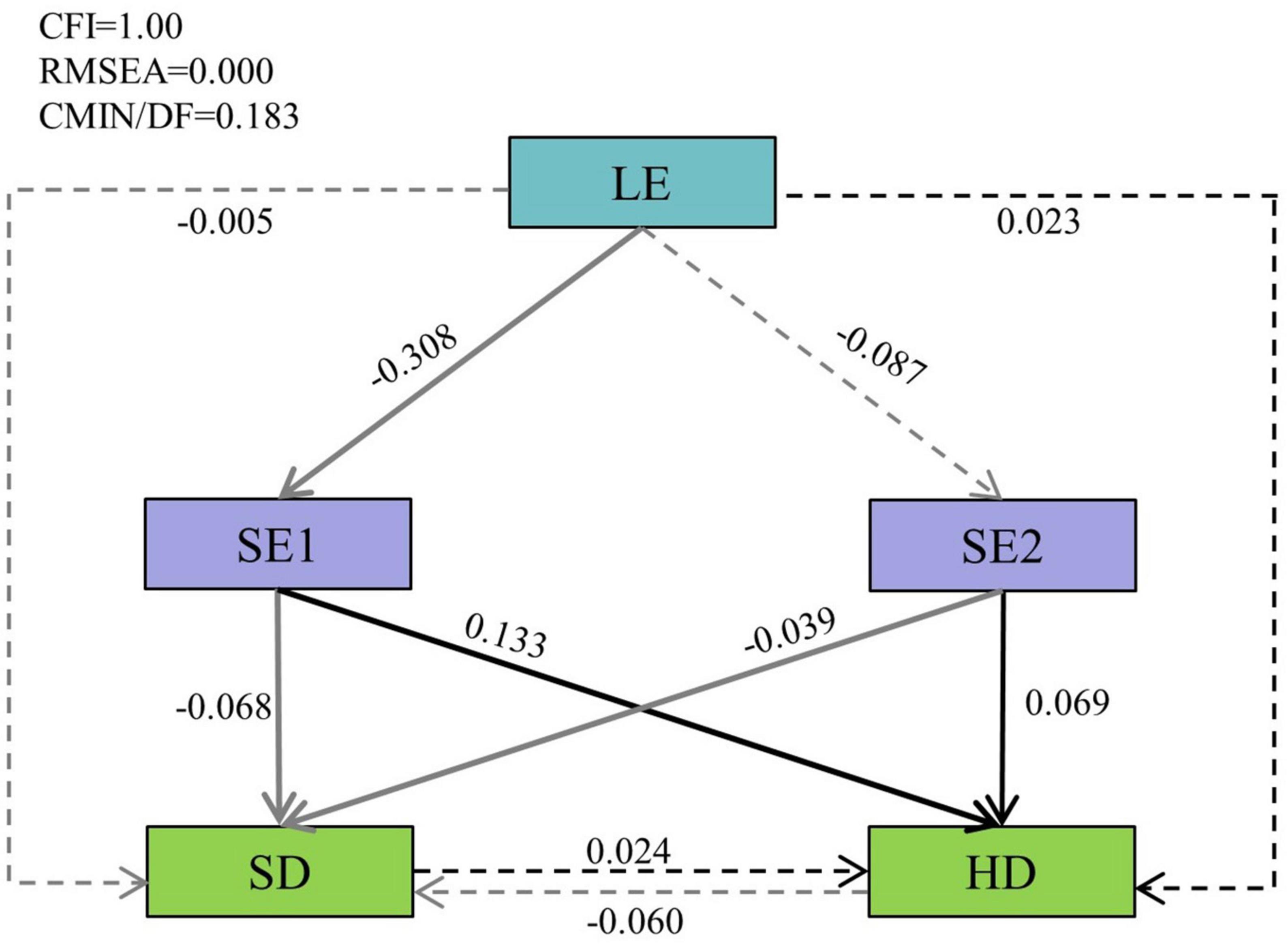
Figure 7. The structural equation model (SEM) results. The single-directional arrows represent the hypothesized cause-and-effect relationships between the variables. The standardized path coefficients are marked on the arrow lines. Residual errors related to the respective endogenous variable in the model are not presented. The solid lines represent significant relationships (p < 0.05), and dashed lines represent insignificant relationships (p > 0.05). Black arrows represent positive effects, and the gray arrows represent negative effects. Light factor (LE) represents the light factors extracted by the principal component analysis (PCA), the first axes of a principal component (SE1) and the second axes of a principal component (SE2) represent the first two soil factors extracted by the PCA, SD, and HD represent Shannon-Weiner index of shrub and herb, respectively.
Discussion
Effects of thinning on habitat factors
Thinning is a vital measure to adjust stand structure. The change in canopy structure that arises from forest thinning is the main driving mechanism of the change in the understory light environment (Richards and Hart, 2011), which mainly alters the light flux density and spectral quality, and spatial distribution through the absorption, reflection and transmission of light by the upper leaves (Parker et al., 2002). Consistent with a large number of previous studies (Montgomery and Chazdon, 2001; Wetzel and Burgess, 2001; Sullivan et al., 2002), we found that the PAR and R/FR increased monotonically with the thinning intensity. Thinning shortens the canopy structure, expands the canopy space, and the light characteristics are sensitive to the change of forest layers (Wan and He, 2020), which will reduce the obstruction of incident light and increase the par under the forest PAR (Yamada et al., 2014; Franca et al., 2018). The NDVI is the normalized vegetation index, capable of fully indicating the vegetation coverage in the forest community (Liang et al., 2018), and we found that the mean value of NDVI in HT group was higher than in the other groups. Thus, the probable reason for the above result is that the sample plots with high thinning intensities have sufficient sunshine and more weeds, thus resulting in a large vegetation coverage area and a higher NDVI value. Our results indicated that the light factors in the CK group showed significant patch distribution in space, leading to the discontinuity of light factors. The thinning forests would have more uniform light than the original forest due to its more uniform canopy structure. Similarly, Nicotra et al. (1999) and Yamada et al. (2014) reported that selectively logged stands are more homogeneous with regard to variation in light than the original forest.
As for the soil environment, most studies have suggested that soil nutrient content decreases in a short term after thinning (Jennings et al., 2012; Choe et al., 2021). Binglin et al. (2020) argued that five years after the thinning, the soil TP and TK contents of the Birch plantation decreased significantly. Thus, an increase in the logging intensity could trigger the loss of soil nutrients (N, P, and K). This study was consistent with the above research, thinning significantly reduced the TN, TP, OM, NN, and AN in the soil, whereas the change of TK content in the soil was insignificant. The reasons for the above result were speculated as follows: (1) After thinning, the amount of nutrient return through the decomposition of litters decreases, so the soil nutrient content formed by the decomposition and the transformation of litters declines (Zhou et al., 2019). (2) The removal of residues from thinning can lead to the loss of nutrients (e.g., N, P, and K) from soil (Dong et al., 2021). Thinning produces a considerable amount of nutrient-rich residues that are broken down by organisms in the soil and can deliver nutrients to the soil (Garcia-Oliva et al., 2003; Semenov et al., 2012; Wang et al., 2012). Existing studies have suggested that soil total carbon and TN increased during residue retention in 12-24 months after thinning (Chen and Xu, 2005; Tutua et al., 2019). In contrast, removing the whole plant after thinning would lead to the loss of soil carbon and nitrogen and the decrease of unstable soil OM (Kumaraswamy et al., 2014). Accordingly, compared with the removal of forest residues, the retention of forest residues can improve the quality of resources and make up for the loss of soil nutrients caused by thinning, thus facilitating plant growth and maintaining the balance of forest nutrients (Fernandez et al., 2016; Rocha et al., 2018). (3) After thinning, the change in soil temperature and water content in the stand will facilitate the decomposition and consumption of soil OM, thus resulting in the decrease of soil nutrient content (Jandl et al., 2007; James and Harrison, 2016). (4) The investigation of this study was conducted 3 years after the thinning, and the forest recovery time was relatively short. As a result, the residual effect of forest interference by thinning may still exist. Thus, long-term observation should be conducted on the effects of thinning on soil nutrients (Choe et al., 2021).
Effect of light and soil environment on understory species diversity
The change of light conditions in the forest directly affects the physical and chemical properties of soil. Our results showed that there was a negative correlation between light and soil nutrients. Lu et al. (2020) have also drawn a similar conclusion. Light caused the formation of a large number of free radicals such as hydroxyl, peroxy, singlet oxygen and peroxide in the soil, which can accelerate the photolysis of organic matter (Mathew and Khan, 1996). And photochemical loss of organic matter can partly explain the 20–50% reduction in soil organic matter content in tillage layer, and these losses are concentrated at the top 10 cm of soil (Tiessen et al., 1982; Luo et al., 2010).
The amount of light reaching the understory is one of the most important limiting factors affecting the species of understory plants (Kirby, 1988; Van Calster et al., 2008; Márialigeti et al., 2016; Shi et al., 2021), which determines the coverage, diversity and species composition of undergrowth vegetation. Many studies have shown that improved understory light conditions caused by increased canopy openness can improve plant diversity, especially in the herbaceous layer (Lee et al., 1997; Beaudet and Messier, 2002; Vesala et al., 2005; Ligot et al., 2014; Wang et al., 2017; Ádám et al., 2018; Wan and He, 2020; Shi et al., 2021; Zhang et al., 2021; Su et al., 2022). Theoretically, the effects of light on vegetation are complex because the abundance or diversity of understory vegetation does not necessarily correlate positively with light availability. For example, in the study by Härdtle et al. (2003), light conditions were the most important driver of acid beech forests, whereas in more neutral forest sites, soil properties proved to be more decisive. In our research, we did not find significant light condition effects, and soil properties were turned out to contribute more to the diversity of undergrowth vegetation than light conditions. One supposed reason was that there were enough light resources in the understory after thinning, and the soil nutrient content became the main limiting factor for the survival of undergrowth plants.
According to the SEM obtained in this study, both SE1 and SE2 were negatively correlated with shrub layer diversity, while positively with herb layer, which means that the decrease of soil nutrients would lead to a decline in the shrub diversity, but not in herbs. Vegetation and soil are inseparable, and soil nutrients are important factors driving the species diversity of understory vegetation (Pohl et al., 2009). Previous studies had proved that understory vegetation diversity was mainly affected by soil factors, including soil pH (Tabatabai and Bremner, 1970; Acosta-Martínez and Tabatabai, 2000; Pärtel et al., 2004; Lenière and Houle, 2006), soil P contents (Sitters et al., 2013; Feng et al., 2018) and soil K contents, etc. (Potts et al., 2002; Bobbink et al., 2010; Santana et al., 2010), and there were different effects on the diversity of shrub and herb layers. In general, the growth of understory vegetation is related to the availability of essential soil nutrients, the solubility of toxic elements, and soil microbial communities (Weaver and Hamill, 1985). For the shrub layer, higher pH and soil water content can reduce the availability of soil nutrient elements (such as available phosphorus) and increase the dissolution of toxic elements, thus inhibiting the growth of soil microorganisms and some shrub plants that are alkali-resistant and sensitive to toxic elements (Ma et al., 2012). However, the herbaceous calcareous plants that prefer humidity habitats often have a tolerance to higher soil pH (Yibo et al., 2019). As for the soil P content, studies on plant niche differentiation along the gradient of soil nutrient resources found that niche differentiation only occurred when the P content was low, which was beneficial to the coexistence of more species (Steidinger, 2015; Xu et al., 2017). In addition, excessive soil K contents reduced the fine root biomass at the community level (Wright et al., 2011; Xu et al., 2017), which also caused damage to shrubs. All the above studies have obtained consistent results, that is, soil factors are positively correlated with herbs and negatively or not correlated with shrubs, which is consistent with our findings. Compared with a light environment, soil conditions showed a larger influence on the species diversity of understory vegetation in mixed broadleaf-conifer forest three years after thinning.
Conclusion
The results of this study suggested that forest thinning affected the light factors and the soil conditions in the stand, and changes in the above habitat factors would have further effects on the species diversity of understory vegetation. In the short period (3 years) after thinning, in the Broad-leaved Korean pine forest of northeast China, the light environment was found as the optimal light environment in the heavy thinning forest. However, TN, TK, OM, and NN decreased with the increase of the cutting intensity. After thinning, the light factor showed a significant negative correlation with the soil environment in the stand. The response of understory shrub and herbaceous diversity to the soil environment was more significant than that of the light condition. Lower soil nutrients were related to a higher shrub diversity but a lower herb diversity. This study was conducted 3 years after the thinning, and post-felling recovery is a long-term process with various habitat factors in the stand changing constantly, thus leading to the change of understory vegetation diversity. Accordingly, it is suggested that long-term dynamic monitoring should be conducted to lay a more solid theoretical basis for forest management.
Data availability statement
The original contributions presented in this study are included in the article/Supplementary material, further inquiries can be directed to the corresponding author.
Author contributions
XZ, MH, and CX: conceptualization. JY: methodology and writing—original draft preparation. XZ and JY: software. XZ, CX, and CC: validation. CC and HH: investigation. XZ: writing—review and editing, project administration, and funding acquisition. All authors have read and agreed to the published version of the manuscript.
Funding
This research was funded by “National Key R&D Program, grant number: 2021YFE0193200” and “National Natural Science Foundation of China grant number: 31901277”.
Conflict of interest
The authors declare that the research was conducted in the absence of any commercial or financial relationships that could be construed as a potential conflict of interest.
Publisher’s note
All claims expressed in this article are solely those of the authors and do not necessarily represent those of their affiliated organizations, or those of the publisher, the editors and the reviewers. Any product that may be evaluated in this article, or claim that may be made by its manufacturer, is not guaranteed or endorsed by the publisher.
Supplementary material
The Supplementary Material for this article can be found online at: https://www.frontiersin.org/articles/10.3389/fpls.2022.948648/full#supplementary-material
References
Acosta-Martínez, V., and Tabatabai, M. A. (2000). Enzyme activities in a limed agricultural soil. Biol. Fertil. Soils 31, 85–91. doi: 10.1007/s003740050628
Ádám, R., Ódor, P., Bidló, A., Somay, L., and Bölöni, J. (2018). The effect of light, soil pH and stand heterogeneity on understory species composition of dry oak forests in the north hungarian mountains. Community Ecol. 19, 259–271. doi: 10.1556/168.2018.19.3.7
Agnelli, A., Ascher, J., Corti, G., Ceccherini, M. T., Nannipieri, P., and Pietramellara, G. (2004). Distribution of microbial communities in a forest soil profile investigated by microbial biomass, soil respiration and DGGE of total and extracellular DNA. Soil Biol. Biochem. 36, 859–868. doi: 10.1016/j.soilbio.2004.02.004
Alaback, P. B., and Herman, F. R. (1988). Long-term response of understory vegetation to stand density in Picea-Tsuga forests. Can. J. For. Res. 18, 1522–1530. doi: 10.1139/x88-233
Ares, A., Neill, A. R., and Puettmann, K. J. (2010). Understory abundance, species diversity and functional attribute response to thinning in coniferous stands. For. Ecol. Manage. 260, 1104–1113. doi: 10.1016/j.foreco.2010.06.023
Austin, M. P. (1989). Plant strategies and the dynamics and structure of plant communities: by david tilman, princeton university press. Trends Ecol. Evol. 4, 28–29. doi: 10.1016/0169-5347(89)90015-3
Beaudet, M., and Messier, C. (2002). Variation in canopy openness and light transmission following selection cutting in northern hardwood stands: an assessment based on hemispherical photographs. Agric. For. Meteorol. 110, 217–228. doi: 10.1016/S0168-1923(01)00289-1
Binglin, P., Zhenyu, W., Mei, Y., and Shinan, L. (2020). Effects of thinning on tree growth and soil chemical properties of betula luminifera plantation. J. Beihua Univ. (Nat. Sci.) 21, 398–404. doi: 10.11713/j.issn.1009-4822.2020.03.024
Bobbink, R., Hicks, K., Galloway, J., Spranger, T., Alkemade, R., Ashmore, M., et al. (2010). Global assessment of nitrogen deposition effects on terrestrial plant diversity: a synthesis. J. Ecol. Appl. 20, 30–59. doi: 10.1890/08-1140.1
Chen, C. R., and Xu, Z. H. (2005). Soil carbon and nitrogen pools and microbial properties in a 6-year-old slash pine plantation of subtropical Australia: impacts of harvest residue management. For. Ecol. Manage. 206, 237–247. doi: 10.1016/j.foreco.2004.11.005
Choe, C.-H., Kim, J.-H., Xu, C.-Y., Choe, J.-S., Man, H.-S., and Jo, S.-M. (2021). Effect of soil nutrient spatial heterogeneity by cutting disturbance on understory plant diversity in broadleaved-Korean pine forest in changbai mountain, china. Eur. J. For. Res. 140, 603–613. doi: 10.1007/s10342-021-01360-5
Chytrý, M., Tichý, L., and Roleèek, J. (2003). Local and regional patterns of species richness in Central European vegetation types along the pH/calcium gradient. Folia Geobot. 38, 429–442. doi: 10.1007/BF02803250
Dang, P., Gao, Y., Liu, J., Yu, S., and Zhao, Z. (2018). Effects of thinning intensity on understory vegetation and soil microbial communities of a mature Chinese pine plantation in the Loess Plateau. Sci. Total Environ. 630, 171–180. doi: 10.1016/j.scitotenv.2018.02.197
Diggle, P. J., and Giorgi, E. (2016). Model-based geostatistics for prevalence mapping in low-resource settings rejoinder. J. Am. Stat. Assoc. 111, 1119–1120. doi: 10.1080/01621459.2016.1200919
Dong, X., Du, X., Sun, Z., and Chen, X. (2021). Effects of residue retention and removal following thinning on soil bacterial community composition and diversity in a larix olgensis plantation, northeast china. Forests 12:559. doi: 10.3390/f12050559
Ediriweera, S., Singhakumara, B. M. P., and Ashton, M. S. (2008). Variation in canopy structure, light and soil nutrition across elevation of a Sri Lankan tropical rain forest. For. Ecol. Manage. 256, 1339–1349. doi: 10.1016/j.foreco.2008.06.035
Feng, H., Xue, L., and Chen, H. J. (2018). Responses of decomposition of green leaves and leaf litter to stand density, N and P additions in Acacia auriculaeformis stands. Eur. J. For. Res. 137, 819–830. doi: 10.1007/s10342-018-1142-z
Fernandez, A. L., Sheaffer, C. C., Wyse, D. L., Staley, C., Gould, T. J., and Sadowsky, M. J. (2016). Associations between soil bacterial community structure and nutrient cycling functions in long-term organic farm soils following cover crop and organic fertilizer amendment. Sci. Total Environ. 566, 949–959. doi: 10.1016/j.scitotenv.2016.05.073
Franca, F., Louzada, J., and Barlow, J. (2018). Selective logging effects on ‘brown world’ faecal-detritus pathway in tropical forests: a case study from Amazonia using dung beetles. For. Ecol. Manage. 410, 136–143. doi: 10.1016/j.foreco.2017.12.027
Fu, B. J., Liu, S. L., Ma, K. M., and Zhu, Y. G. (2004). Relationships between soil characteristics, topography and plant diversity in a heterogeneous deciduous broad-leaved forest near Beijing, China. Plant Soil 261, 47–54. doi: 10.1023/B:PLSO.0000035567.97093.48
Garcia-Oliva, F., Sveshtarova, B., and Oliva, M. (2003). Seasonal effects on soil organic carbon dynamics in a tropical deciduous forest ecosystem in western Mexico. J. Trop. Ecol. 19, 179–188. doi: 10.1017/s0266467403003201
García-Palacios, P., Shaw, E. A., Wall, D. H., and Hättenschwiler, S. (2017). Contrasting mass-ratio vs. niche complementarity effects on litter C and N loss during decomposition along a regional climatic gradient. J. Ecol. 105, 968–978. doi: 10.1111/1365-2745.12730
Gerhardt, K. (1996). Effects of root competition and canopy openness on survival and growth of tree seedlings in a tropical seasonal dry forest. For. Ecol. Manage. 82, 33–48. doi: 10.1016/0378-1127(95)03700-4
Goodall, D. (1980). Plant strategies and vegetation processes. Landsc. Plan. 7, 192–193. doi: 10.1016/0304-3924(80)90019-2
Goodwin, M. J., North, M. P., Zald, H. S. J., and Hurteau, M. D. (2018). The 15-year post-treatment response of a mixed-conifer understory plant community to thinning and burning treatments. For. Ecol. Manage. 429, 617–624.
Hale, S. E. (2003). The effect of thinning intensity on the below-canopy light environment in a Sitka spruce plantation. For. Ecol. Manag. 179, 341–349. doi: 10.1016/s0378-1127(02)00540-6
Hao, M., Messier, C., Geng, Y., Zhang, C., Zhao, X., and von Gadow, K. (2020). Functional traits influence biomass and productivity through multiple mechanisms in a temperate secondary forest. Eur. J. For. Res. 139, 959–968. doi: 10.1007/s10342-020-01298-0
Härdtle, W., von Oheimb, G., and Westphal, C. (2003). The effects of light and soil conditions on the species richness of the ground vegetation of deciduous forests in northern Germany (Schleswig-Holstein). For. Ecol. Manage. 182, 327–338. doi: 10.1016/S0378-1127(03)00091-4
Hart, S. A., and Chen, H. Y. H. (2006). Understory vegetation dynamics of North American boreal forests. Crit. Rev. Plant Sci. 25, 381–397. doi: 10.1080/07352680600819286
James, J., and Harrison, R. (2016). The effect of harvest on forest soil carbon: a meta-analysis. Forests 7:308. doi: 10.3390/f7120308
Jandl, R., Lindner, M., Vesterdal, L., Bauwens, B., Baritz, R., Hagedorn, F., et al. (2007). How strongly can forest management influence soil carbon sequestration? Geoderma 137, 253–268. doi: 10.1016/j.geoderma.2006.09.003
Jennings, T. N., Smith, J. E., Cromack, K., Sulzman, E. W., McKay, D., Caldwell, B. A., et al. (2012). Impact of postfire logging on soil bacterial and fungal communities and soil biogeochemistry in a mixed-conifer forest in central Oregon. Plant Soil 350, 393–411. doi: 10.1007/s11104-011-0925-5
Kirby, K. J. (1988). Changes in the ground flora under plantations on ancient woodland sites. Forestry 61, 317–338. doi: 10.1093/forestry/61.4.317
Kumaraswamy, S., Mendham, D. S., Grove, T. S., O’Connell, A. M., Sankaran, K. V., and Rance, S. J. (2014). Harvest residue effects on soil organic matter, nutrients and microbial biomass in eucalypt plantations in Kerala, India. For. Ecol. Manage. 328, 140–149. doi: 10.1016/j.foreco.2014.05.021
Lee, D. W., Oberbauer, S. F., Krishnapilay, B., Mansor, M., Mohamad, H., and Yap, S. K. (1997). Effects of irradiance and spectral quality on seedling development of two Southeast Asian Hopea species. Oecologia 110, 1–9. doi: 10.1007/s004420050126
Lencinas, M. V., Pastur, G. M., Gallo, E., and Cellini, J. M. (2011). Alternative silvicultural practices with variable retention to improve understory plant diversity conservation in southern Patagonian forests. For. Ecol. Manage. 262, 1236–1250. doi: 10.1016/j.foreco.2011.06.021
Lenière, A., and Houle, G. (2006). Response of herbaceous plant diversity to reduced structural diversity in maple-dominated (Acer saccharum Marsh.) forests managed for sap extraction. For. Ecol. Manage. 231, 94–104. doi: 10.1016/j.foreco.2006.05.024
Li, B., and Wang, Z. Q. J. (2012). Estimation of nitrogen and phosphorus release rates at sediment-water interface of nansi lake, china. Adv. Mater. Res. 573–574, 573–577. doi: 10.4028/www.scientific.net/AM
Liang, L., Chen, F., Shi, L., and Niu, S. (2018). NDVI-derived forest area change and its driving factors in China. PLoS One 13:e0205885. doi: 10.1371/journal.pone.0205885
Ligot, G., Balandier, P., Courbaud, B., Jonard, M., Kneeshaw, D., and Claessens, H. (2014). Managing understory light to maintain a mixture of species with different shade tolerance. For. Ecol. Manage. 327, 189–200. doi: 10.1016/j.foreco.2014.05.010
Lingjun, M., Chunyu, Z., Jie, Y., and Xiuhai, Z. (2019). Effects of density and habitat on arbor seedling survival in a mixed conifer and broad-leaved forest in jiaohe, jilin province. Sci. Silvae Sin. 55, 172–180. doi: 10.11707/j.1001-7488.20191119
Liu, S., Yin, H., Li, X., Li, X., Fan, C., Chen, G., et al. (2021). Short-term thinning influences the rhizosphere fungal community assembly of Pinus massoniana by altering the understory vegetation diversity. Front. Microbiol. 12:620309. doi: 10.3389/fmicb.2021.620309
Lu, Y., Guo, Z., and Wang, Y. (2020). Study on soil nutrient activation process by different bands of light. Hans J. Soil Sci. 8, 15–22. doi: 10.12677/HJSS.2020.81003
Luo, Z., Wang, E., and Sun, O. J. (2010). Soil carbon change and its responses to agricultural practices in australian agro-ecosystems: a review and synthesis. Geoderma 155, 211–223. doi: 10.1016/j.geoderma.2009.12.012
Ma, M., Zhou, X., Ma, Z., and Du, G. (2012). Composition of the soil seed bank and vegetation changes after wetland drying and soil salinization on the Tibetan Plateau. Ecol. Eng. 44, 18–24. doi: 10.1016/j.ecoleng.2012.03.017
Márialigeti, S., Tinya, F., Bidló, A., and Ódor, P. (2016). Environmental drivers of the composition and diversity of the herb layer in mixed temperate forests in hungary. Plant Ecol. 217, 549–563. doi: 10.1007/s11258-016-0599-4
Mathew, R., and Khan, S. U. (1996). Photodegradation of metolachlor in water in the presence of soil mineral and organic constituents. J. Agric. Food Chem. 44, 3996–4000. doi: 10.1021/jf960123w
Montgomery, R. A., and Chazdon, R. L. (2001). Forest structure, canopy architecture, and light transmittance in tropical wet forests. Ecology 82, 2707–2718. doi: 10.2307/2679955
Newman, E. I. (1973). Competition and diversity in herbaceous vegetation. Nature. 244:310. doi: 10.1038/244310a0
Nicotra, A., Chazdon, R., and Iriarte, S. (1999). Spatial heterogeneity of light and woody seedling regeneration in tropical wet forests. Ecology 80, 1908–1926. doi: 10.2307/176668
Ning, Z. Y., Li, Y. L., Yang, H. L., Zhang, Z. Q., and Zhang, J. P. (2019). Stoichiometry and effects of carbon, nitrogen, and phosphorus in soil of desertified grasslands on community productivity and species diversity (in Chinese). Acta Ecol. Sin. 39, 3537–3546. doi: 10.5846/stxb201711242094
Noguchi, M., Miyamoto, K., and Okuda, S. (2017). Heavy thinning in hinoki plantations in Shikoku (southwestern Japan) has limited effects on recruitment of seedlings of other tree species. J. For. Res. 22:141. doi: 10.1080/13416979.2017.1290324
Pacala, S. W., Canham, C. D., Saponara, J., Silander, J. A. Jr., Kobe, R. K., and Ribbens, E. (1996). Forest models defined by field measurements: estimation, error analysis and dynamics. Ecol. Monogr. 66, 1–43. doi: 10.2307/2963479
Palmer, M. W. (1991). Patterns of species richness among North Carolina hardwood forests: tests of two hypotheses. J. Veg. Sci. 2, 361–366. doi: 10.2307/3235928
Parker, G. G., Davis, M. M., and Chapotin, S. M. (2002). Canopy light transmittance in Douglas-fir–western hemlock stands. Tree Physiol. 22, 147–157. doi: 10.1093/treephys/22.2-3.147
Pärtel, M., Helm, A., Ingerpuu, N., Reier, Ü, and Tuvi, E.-L. (2004). Conservation of Northern European plant diversity: the correspondence with soil pH. Biol. Conserv. 120, 525–531. doi: 10.1016/j.biocon.2004.03.025
Pohl, M., Alig, D., Koerner, C., and Rixen, C. (2009). Higher plant diversity enhances soil stability in disturbed alpine ecosystems. Plant Soil 324, 91–102. doi: 10.1007/s11104-009-9906-3
Potts, M., Ashton, P., Kaufman, L., and Plotkin, J. (2002). Habitat patterns in tropical rain forests: a comparison of 105 plots in Northwest Borneo. Ecology 83, 2782–2797. doi: 10.1890/0012-9658(2002)083[2782:HPITRF]2.0.CO;2
Ramovs, B. V., and Roberts, M. R. (2003). Understory vegetation and environment responses to tillage, forest harvesting, and conifer plantation development. Ecol. Appl. 13, 1682–1700. doi: 10.1890/02-5237
Richards, J. D., and Hart, J. L. (2011). Canopy gap dynamics and development patterns in secondary quercus stands on the cumberland plateau, alabama, USA. For. Ecol. Manage. 262, 2229–2239. doi: 10.1016/j.foreco.2011.08.015
Richards, L. A. (1954). Diagnosis and improvement of saline and alkali soils. AIBS Bull. 4:14. doi: 10.1093/aibsbulletin/4.3.14-a
Rivaie, A. A. (2014). The effects of understory vegetation on P availability in Pinus radiata forest stands: a review. J. For. Res. 25, 489–500. doi: 10.1007/s11676-014-0488-4
Rocha, J. H. T., Goncalves, J. L. D., Brandani, C. B., Ferraz, A. D., Franci, A. F., Marques, E. R. G., et al. (2018). Forest residue removal decreases soil quality and affects wood productivity even with high rates of fertilizer application. For. Ecol. Manage. 430, 188–195. doi: 10.1016/j.foreco.2018.08.010
Rodríguez-Loinaz, G., Onaindia, M., Amezaga, I., Mijangos, I., and Garbisu, C. (2008). Relationship between vegetation diversity and soil functional diversity in native mixed-oak forests. Soil Biol. Biochem. 40, 49–60. doi: 10.1016/j.soilbio.2007.04.015
Rosseel, Y. (2012). lavaan: an R package for structural equation modeling. J. Stat. Softw. 48, 1–36. doi: 10.18637/jss.v048.i02
Rossman, A. K., Halpern, C. B., Harrod, R. J., Urgenson, L. S., Peterson, D. W., and Bakker, J. D. (2018). Benefits of thinning and burning for understory diversity vary with spatial scale and time since treatment. For. Ecol. Manage. 419, 58–78. doi: 10.1016/j.foreco.2018.03.006
Rota, E., Caruso, T., and Bargagli, R. (2014). Community structure, diversity and spatial organization of enchytraeids in Mediterranean urban holm oak stands. Eur. J. Soil Biol. 62, 83–91. doi: 10.1016/j.ejsobi.2014.02.016
Ruiz-Benito, P., Ratcliffe, S., Zavala, M. A., Martínez-Vilalta, J., Vilà-Cabrera, A., Lloret, F., et al. (2017). Climate- and successional-related changes in functional composition of European forests are strongly driven by tree mortality. Glob. Change Biol. 23, 4162–4176. doi: 10.1111/gcb.13728
Santana, V. M., Bradstock, R. A., Ooi, M. K. J., Denham, A. J., Auld, T. D., and Jaime Baeza, M. (2010). Effects of soil temperature regimes after fire on seed dormancy and germination in six Australian Fabaceae species. Aust. J. Bot. 58, 539–545. doi: 10.1071/bt10144
Semenov, A. V., Silva, M., Szturc-Koestsier, A. E., Schmitt, H., Salles, J. F., and van Elsas, J. D. (2012). Impact of incorporated fresh C-13 potato tissues on the bacterial and fungal community composition of soil. Soil Biol. Biochem. 49, 88–95. doi: 10.1016/j.soilbio.2012.02.016
Sercu, B. K., Baeten, L., van Coillie, F., Martel, A., Lens, L., Verheyen, K., et al. (2017). How tree species identity and diversity affect light transmittance to the understory in mature temperate forests. Ecol. Evol. 7, 10861–10870. doi: 10.1002/ece3.3528
Shi, X.-P., Bai, Y.-F., Song, P., Liu, Y.-Y., Zhang, Z.-W., Zheng, B., et al. (2021). Clonal integration and phosphorus management under light heterogeneity facilitate the growth and diversity of understory vegetation and soil fungal communities. Sci. Total Environ. 767:144322. doi: 10.1016/j.scitotenv.2020.144322
Sitters, J., Edwards, P. J., and Olde Venterink, H. (2013). Increases of soil C, N, and P pools along an acacia tree density gradient and their effects on trees and grasses. Ecosystems 16, 347–357. doi: 10.1007/s10021-012-9621-4
Siefert, A., Ravenscroft, C., Althoff, D., Alvarez-Yepiz, J. C., Carter, B. E., Glennon, K. L., et al. (2012). Scale dependence of vegetation-environment relationships: a meta-analysis of multivariate data. J. Veg. Sci. 23, 942–951. doi: 10.1111/j.1654-1103.2012.01401.x
Stein, A., Gerstner, K., and Kreft, H. (2014). Environmental heterogeneity as a universal driver of species richness across taxa, biomes and spatial scales. Ecol. Lett. 17, 866–880. doi: 10.1111/ele.12277
Steidinger, B. (2015). Qualitative differences in tree species distributions along soil chemical gradients give clues to the mechanisms of specialization: why boron may be the most important soil nutrient at Barro Colorado Island. New Phytol. 206, 895–899. doi: 10.1111/nph.13298
Sterck, F., Markesteijn, L., Schieving, F., and Poorter, L. (2011). Functional traits determine trade-offs and niches in a tropical forest community. Proc. Natl. Acad. Sci. U.S.A. 108, 20627–20632. doi: 10.1073/pnas.1106950108
Su, X., Wang, M., Huang, Z., Fu, S., and Chen, H. Y. H. (2019). Forest understorey vegetation: colonization and the availability and heterogeneity of resources. Forests 10:944. doi: 10.3390/f10110944
Su, X., Zheng, G., and Chen, H. Y. H. (2022). Understory diversity are driven by resource availability rather than resource heterogeneity in subtropical forests. For. Ecol. Manage. 503:119781. doi: 10.1016/j.foreco.2021.119781
Sullivan, T. P., Sullivan, D. S., Lindgren, P. M. F., and Boateng, J. O. (2002). Influence of conventional and chemical thinning on stand structure and diversity of plant and mammal communities in young lodgepole pine forest. For. Ecol. Manage. 170, 173–187. doi: 10.1016/S0378-1127(01)00775-7
Tabatabai, M. A., and Bremner, J. M. (1970). Arylsulfatase activity of soils. Soil Sci. Soc. Am. J. 34, 225–229. doi: 10.2136/sssaj1970.03615995003400020016x
Thomas, S. C., Halpern, C. B., Falk, D. A., Liguori, D. A., and Austin, K. A. (1999). Plant diversity in managed forest: understory responses to thinning and fertilization. Ecol. Appl. 9, 864–879. doi: 10.2307/2641335
Tiessen, H., Stewart, J. W. B., and Bettany, J. R. (1982). Cultivation effects on the amounts and concentration of carbon, nitrogen, and phosphorus in grassland soils. Agron. J. 74, 831–835. doi: 10.2134/agronj1982.00021962007400050015x
Tutua, S., Zhang, Y. L., Xu, Z. H., and Blumfield, T. (2019). Residue retention mitigated short-term adverse effect of clear-cutting on soil carbon and nitrogen dynamics in subtropical Australia. J.Soils Sediments 19, 3786–3796. doi: 10.1007/s11368-019-02412-5
Van Calster, H., Baeten, L., Verheyen, K., De Keersmaeker, L., Dekeyser, S., Rogister, J. E., et al. (2008). Diverging effects of overstorey conversion scenarios on the understorey vegetation in a former coppice-with-standards forest. For. Ecol. Manage. 256, 519–528. doi: 10.1016/j.foreco.2008.04.042
Verschuyl, J., Riffell, S., Miller, D., and Wigley, T. B. (2011). Biodiversity response to intensive biomass production from forest thinning in North American forests – a meta-analysis. For. Ecol. Manage. 261, 221–232. doi: 10.1016/j.foreco.2010.10.010
Vesala, T., Suni, T., Rannik, U., Keronen, P., Markkanen, T., Sevanto, S., et al. (2005). Effect of thinning on surface fluxes in a boreal forest. Glob. Biogeochem. Cycles 19:GB2001. doi: 10.1029/2004gb002316
Walkley, A. J., and Black, I. A. (1934). An examination of the degtjareff method for determining soil organic matter, and a proposed modification of the chromic acid titration method. Soil Sci. 37, 29–38. doi: 10.1097/00010694-193401000-00003
Wan, P., and He, R. (2020). Canopy structure and understory light characteristics of a natural Quercus aliena var. acuteserrata forest in China northwest: influence of different forest management methods. Ecol. Eng. 153:105901. doi: 10.1016/j.ecoleng.2020.105901
Wang, G., Sun, Y., Zhou, M., Guan, N., Wang, Y., Jiang, R., et al. (2021). Effect of thinning intensity on understory herbaceous diversity and biomass in mixed coniferous and broad-leaved forests of Changbai Mountain. For. Ecosyst. 8, 53. doi: 10.1186/s40663-021-00331-x
Wang, J. J., Li, X. Y., Zhu, A. N., Zhang, X. K., Zhang, H. W., and Liang, W. J. (2012). Effects of tillage and residue management on soil microbial communities in North China. Plant Soil Environ. 58, 28–33. doi: 10.17221/416/2011-pse
Wang, Z., Yang, H., Dong, B., Zhou, M., Ma, L., Jia, Z., et al. (2017). Effects of canopy gap size on growth and spatial patterns of Chinese pine (Pinus tabulaeformis) regeneration. For. Ecol. Manage. 385, 46–56. doi: 10.1016/j.foreco.2016.11.022
Weaver, S. E., and Hamill, A. S. (1985). Effects of soil pH on competitive ability and leaf nutrient content of corn (Zea mays L.) and three weed species. Weed Sci. 33, 447–451. doi: 10.1017/S0043174500082631
Weiher, E., Forbes, S., Schauwecker, T.B., and Grace, J. (2004). Multivariate control of plant species richness and community biomass in blackland prairie. OIKOS 106, 151–157. doi: 10.1111/j.0030-1299.2004.12545.x
Wetzel, S., and Burgess, D. (2001). Understorey environment and vegetation response after partial cutting and site preparation in Pinus strobus L. stands. For. Ecol. Manage. 151, 43–59. doi: 10.1016/S0378-1127(00)00695-2
Wilke, B.-M., Margesin, R., and Schinner, F. (2005). “Determination of chemical and physical soil properties,” in Manual for Soil Analysis, Monitoring and Assessing, Soil Bioremediation, eds R. Margesin and F. Schinner (Berlin: Springer-Verlag), 47–95.
Wright, S. J., Yavitt, J. B., Wurzburger, N., Turner, B. L., Tanner, E. V. J., Sayer, E. J., et al. (2011). Potassium, phosphorus, or nitrogen limit root allocation, tree growth, or litter production in a lowland tropical forest. Ecology 92, 1616–1625. doi: 10.1890/10-1558.1
Xu, Y. J., Lin, D. M., Shi, M., Xie, Y. J., Wang, Y. Z., Guan, Z. H., et al. (2017). Spatial heterogeneity and its causes in evergreen broad-leaved forests in the Ailao Mountains, Yunnan Province. (in Chinese with English abstract). Biodivers. Sci. 25, 23–33. doi: 10.17520/biods.2016199
Yamada, T., Yoshioka, A., Hashim, M., Liang, N., and Okuda, T. (2014). Spatial and temporal variations in the light environment in a primary and selectively logged forest long after logging in Peninsular Malaysia. Trees Struct. Funct. 28, 1355–1365. doi: 10.1007/s00468-014-1040-z
Yibo, T., Wenhui, S., Zi, F., Wei, Z., Zhiyang, O., Zhangqiang, T., et al. (2019). Effect of environmental factors on understory species diversity in Southwest Guangxi Excentrodendron tonkinense forests (in Chinese). Biodivers. Sci. 27, 970–983. doi: 10.17520/biods.2019133
Zhang, Y., Liu, T., Guo, J., Tan, Z., Dong, W., and Wang, H. (2021). Changes in the understory diversity of secondary Pinus tabulaeformis forests are the result of stand density and soil properties. Glob. Ecol. Conserv. 28:e01628. doi: 10.1016/j.gecco.2021.e01628
Zhou, Z., Wang, C., Jin, Y., and Sun, Z. (2019). Impacts of thinning on soil carbon and nutrients and related extracellular enzymes in a larch plantation. For. Ecol. Manage. 450:117523. doi: 10.1016/j.foreco.2019.117523
Zhou, L., Cai, L., He, Z., Wang, R., Wu, P., and Ma, X. (2016). Thinning increases understory diversity and biomass, and improves soil properties without decreasing growth of Chinese fir in southern China. Environ. Sci. Pollut. Res. 23, 24135–24150. doi: 10.1007/s11356-016-7624-y
Zhou, T., Wang, C., and Zhou, Z. (2021). Thinning promotes the nitrogen and phosphorous cycling in forest soils. Agric. For. Meteorol. 311:108665. doi: 10.1016/j.agrformet.2021.108665
Zhu, J., Yang, K., Yan, Q., Liu, Z., Yu, L., and Wang, H. (2010). Feasibility of implementing thinning in even-aged Larix olgensis plantations to develop uneven-aged larch-broadleaved mixed forests. J. For. Res. 15, 71–80. doi: 10.1007/s10310-009-0152-6
Zhu, J. J., Matsuzaki, T., Lee, F. Q., and Gonda, Y. (2003). Effect of gap size created by thinning on seedling emergency, survival and establishment in a coastal pine forest. For. Ecol. Manage. 182, 339–354. doi: 10.1016/s0378-1127(03)00094-x
Keywords: mixed broadleaf-conifer forest, thinning intensity, light environment, soil nutrient content, understory species diversity
Citation: Yu J, Zhang X, Xu C, Hao M, Choe C and He H (2022) Thinning can increase shrub diversity and decrease herb diversity by regulating light and soil environments. Front. Plant Sci. 13:948648. doi: 10.3389/fpls.2022.948648
Received: 20 May 2022; Accepted: 04 July 2022;
Published: 05 August 2022.
Edited by:
Qing-Lai Dang, Lakehead University, CanadaReviewed by:
Ye Tao, Xinjiang Institute of Ecology and Geography (CAS), ChinaLei Wang, Lakehead University, Canada
Copyright © 2022 Yu, Zhang, Xu, Hao, Choe and He. This is an open-access article distributed under the terms of the Creative Commons Attribution License (CC BY). The use, distribution or reproduction in other forums is permitted, provided the original author(s) and the copyright owner(s) are credited and that the original publication in this journal is cited, in accordance with accepted academic practice. No use, distribution or reproduction is permitted which does not comply with these terms.
*Correspondence: Xinna Zhang, emhhbmd4aW5uYUBiamZ1LmVkdS5jbg==