- 1College of Agriculture, Guizhou University, Guiyang, China
- 2Key Laboratory of Tobacco Quality in Guizhou Province, Guiyang, China
- 3College of Tobacco, Guizhou University, Guiyang, China
Potassium (K+) is essential for crop growth. Increasing the K+ content can often directly promote the improvement of crop yield and quality. Heterosis plays an important role in genetic improvement and leads to genetic gains. We found that the K+ content of tobacco showed significant heterosis, which is highly significant for cultivating tobacco varieties with high K+ content. However, the mechanism by which K+ content heterosis occurs in tobacco leaves is not clear. In this study, a comprehensive comparative transcriptome sequencing analysis of root samples from the hybrid G70 × GDH11 and its parental inbred lines G70 and GDH11 was performed to elucidate the importance of the root uptake capacity of K+ in the formation of heterosis. The results showed that 29.53% and 60.49% of the differentially expressed genes (DEGs) exhibited dominant and over-dominant expression patterns, respectively. These non-additive upregulated DEGs were significantly enriched in GO terms, such as metal ion transport and reaction, ion balance and homeostasis, ion channel activity, root meristem growth, and regulation of root hairs. The KEGG annotation results indicated that these genes were mainly involved in the pathways such as energy metabolism, carbohydrate formation, amino acid metabolism, and signal transduction. Further analysis showed that probable potassium transporter 17 (NtKT17) and potassium transporter 5-like (NtKT5), associated with potassium ion absorption, glutamate receptor 2.2-like and glutamate receptor 2.8-like, associated with ion channel activity, LOC107782957, protein detoxification 42-like, and probable glutamate carboxypeptidase 2, associated with root configuration, showed a significantly higher expression in the hybrids. These results indicated that the over-dominant expression pattern of DEGs played a key role in the heterosis of K+ content in tobacco leaves, and the overexpression of the genes related to K+ uptake, transport, and root development in hybrids helped to improve the K+ content of plants, thus showing the phenomenon of heterosis.
Introduction
The new “green revolution” includes crop genetic improvement, healthy plant production, and efficient utilization of water and fertilizer (Beddington, 2010). Among them, the genetic improvement of crops ensures sustainable agricultural development through the cultivation of new varieties and the production of genetic gains (Wan, 2018). For the genetic improvement of crops, heterosis plays an important role in breeding desirable varieties and has led to remarkable achievements (Wu et al., 2021). Since it can greatly improve the production and quality of animals and plants, it significantly benefits society and the economy (Zhang et al., 2020), and also plays an important role in solving issues regarding global food security (Li et al., 2016).
Heterosis is a common biological phenomenon in which the hybrid offspring have better phenotypic traits than their parents (Hochholdinger and Baldauf, 2018). In China, it was found that the hybridization between horses and donkeys produced stronger mules (Yu et al., 2021). However, the study of plant heterosis began in Europe. In the mid-18th century, Kolreute performed tobacco hybridization between different species to cultivate early maturing tobacco hybrids with excellent qualities (Murad et al., 2002). Dominance (Davenport, 1908), overdominance (East, 1936), and epistasis (Williams, 1959) can explain heterosis partially, and their effects have been studied to some extent. Crop heterosis is not only different among different crops and traits, but the same trait of the same crop may be different due to different hybrid combinations, hybridization methods, and the cultivation environment (Schnable and Springer, 2013). Therefore, the mechanism of heterosis formation lacks a general explanation; specifically, heteropolyploid heterosis is relatively under-studied (Tian et al., 2018).
Tobacco (Nicotiana tabacum L.) is a special economic crop that is widely cultivated and has the characteristics of a short growth cycle, clear genetic background, and easy genetic transformation. It is also a model plant to study and elucidate various biological mechanisms (Xu et al., 2020; Zhang et al., 2022). Potassium ion (K+) is a macroelement required for plant growth and affects the yield, quality, and stress resistance of plants by participating in activities such as protein synthesis, cell osmotic regulation, photosynthesis, stomatal movement, and enzyme activation (Pettigrew, 2008; Ragel et al., 2019). Tobacco is a potassium-loving crop, and potassium can not only significantly improve the color, combustion power, and firepower of tobacco leaves but also increase their flexibility and softness (Wang et al., 2022). Therefore, high potassium content in tobacco leaves increases the yield and quality of the leaves.
Tobacco directly absorbs K+ from the soil through roots and subsequently accumulates K+ in the leaves. Potassium ion is transported to leaves through the xylem due to the expression of potassium channel genes and potassium transporter genes (Sano et al., 2009). The potassium absorption capacity of tobacco roots is closely related to the physiological characteristics (root active absorption area, root activity, ATPase activity, etc.) and root morphology (Li and Li, 2001; Li and Ma, 2003), and there are also considerable differences in the capacity to absorb K+ among different genotypes (Chen et al., 2010). Various genes related to K+ absorption and transport have been isolated and cloned. These genes play various roles in different stages of life (Chai et al., 2019) and include influx potassium channel genes: AKT1 (Hirsch et al., 1998), KAT1 (Sottocornola et al., 2008), KC1 (Reintanz et al., 2002), etc.; outflow potassium channel genes: SKOR (Gaymard et al., 1998); and potassium transporter genes: KUP1 (Santa-María et al., 2018), HAK (Greiner et al., 2011), TPK1 (Gobert et al., 2007), etc. The mining of these key genes that regulate K+ absorption and transport is important for the directional improvement of potassium content in plants.
The potassium content of tobacco leaves increases in the topping period. After topping, the potassium content decreases rapidly, resulting in the low potassium content of mature tobacco leaves (Dai et al., 2009). Studies on improving tobacco quality focus on the strategies to effectively enhance the potassium content and select tobacco varieties with a high potassium content. In the early stages of research, we found that the heterosis of potassium content in tobacco leaves was prominent, and through heterosis utilization breeding, we obtained the hybrids Guiyan 202 and Guiyan 4 with high potassium content in tobacco leaves (Liu et al., 2006b, 2016). However, the mechanism of development of potassium content heterosis in tobacco leaves is unclear. Therefore, to determine the molecular components that might act on potassium heterosis, we performed transcriptome analysis of root samples of hybrids and their parents of the model plant common tobacco (N. tabacum L.) to elucidate the underlying mechanism of root potassium uptake capacity in the formation of potassium heterosis.
Materials and methods
Plant materials and planting
The tobacco hybrid F1 (G70 × GDH11) and two parental inbred lines (G70 and GDH11) selected for this study were provided by the Guizhou Key Laboratory of Tobacco Quality Research. We ensured that the collection of plant material and experimental research and field studies on plants complied with relevant institutional, national, and international guidelines and legislation. The seeds were sown in a special substrate for flue-cured tobacco and raised by floating the seedlings in a greenhouse. The seedlings were grown there till they had five true leaves, and then they were transplanted to the field. The field experiment was conducted in the tobacco scientific research and experimental base of Guizhou University in 2021, with three replicates and a row plant spacing of 110 cm × 55 cm. All plants were topped after 50% of the center flowers of the plants were open.
Samples were collected every 10 days between 60 and 90 days after transplantation. Three tobacco plants with the same growth performance were randomly selected from each plot, and their young fibrous roots and root tips were sampled and mixed together. The samples were first washed with clean water and then with PBS. Next, they were placed in separate sterilized centrifuge tubes, frozen with liquid nitrogen, and stored in a low-temperature refrigerator at −80°C at the earliest. The leaf samples were killed at 105°C for 30 min, then turned to 75°C to dry, ground into powder, bagged, and sealed for storage.
Determination of K+ content and analysis of heterosis
To determine the content of K+ in leaves, 0.5 g of the sample was soaked in 0.5 mol/L of dilute hydrochloric acid and filtered. Then, the K+ content was determined using a flame spectrophotometer (Munns et al., 2010). The K+ content was calculated according to the following formula:
Here, C indicates the K+ concentration (ppm) obtained from the standard curve; V indicates the volume of the liquid to be measured; G indicates the dry smoke sample weight (g); 106 is the weight conversion coefficient.
The values of over high-parent heterosis (OPH), mid-parent heterosis (MPH), and below low-parent heterosis (BPH) were calculated according to the following formulae: , , , where F1 represents the first hybrid generation, HP represents the high-value parent, MP represents the average parent value , and LP represents the low-value parent.
RNA isolation and library preparation
The biological samples of 70 days after transplanting were used as materials for RNA sequencing analysis. Total RNA from the root of nine samples was extracted using the TRIzol reagent following the manufacturer’s instructions (OE Biotech Co., Ltd., Item No.: T105096). The purity and quantity of RNA were evaluated using the NanoDrop 2000 spectrophotometer (Thermo Scientific, United States). The integrity of the RNA was assessed using the Agilent 2,100 Bioanalyzer (Agilent Technologies, Santa Clara, CA, United States). Then, the libraries were constructed using TruSeq Stranded mRNA LT Sample Prep Kit (Illumina, San Diego, CA, United States) following the manufacturer’s instructions.
RNA sequencing and analysis of differentially expressed genes
The libraries were sequenced on an Illumina HiSeq X Ten platform, and 150 bp paired-end reads were generated. An average of 8.67 million reads was obtained per sample. Raw data (raw reads) in the fastq format were initially processed using Trimmomatic (Bolger et al., 2014), and the low-quality reads were removed to obtain clean reads. Then, about 8.60 million clean reads for each sample were retained for subsequent analyses.
The clean reads were mapped to the tobacco genome1 using HISAT2 (Kim et al., 2015). The Fragments Per Kilobase of exon model per million mapped fragments (FPKM; Roberts et al., 2011) of each gene was calculated using Cufflinks (Trapnell et al., 2011), and the read counts of each gene were obtained using the HTSeq-count tool (Anders et al., 2015). Differential expression analysis was performed using the DESeq2 (Anders and Huber, 2012); the value of p < 0.05 and foldchange >2 or foldchange <0.5 were set as the threshold for significant differential expression. Hierarchical cluster analysis of differentially expressed genes (DEGs) was performed to determine the expression pattern of genes in different groups and samples. The GO enrichment and KEGG pathway enrichment analyses of the DEGs were performed using the R software, based on the hypergeometric distribution.
Real-time fluorescence quantitative PCR
To confirm the level of gene expression, based on the initial results of RNA sequencing, six genes were randomly selected for real-time fluorescence quantitative PCR (RT-qPCR) experiments. The experiments were performed using the SYBR Premix Ex Taq kit (Takara) and the Applied Biosystems 7500 Real-Time PCR system (Life Technologies Corporation, Beverly, MA, United States). The genes and corresponding primers used for the qPCR test are listed in Supplementary File 4. To calculate the relative expression level of each gene, the 2−ΔΔCt method was used (Livak and Schmittgen, 2001).
Statistical analyses
Duncan’s new multiple range tests were conducted to analyze the variation in the K+ content (p < 0.05) using the SPSS (version 16.0) software. Use Origin 2018 (95_64) and Adobe Illustrator CS6 for figure drawing.
Results
The performance of K+ content and its heterosis in tobacco leaves
To determine the difference in the K+ content between the hybrids and their parents in different growth periods, we took samples every 10 days between 60 and 90 days after transplantation and evaluated the K+ content in parents and their hybrids (Supplementary File 1). In the four growth stages studied, the K+ content of the hybrid G70 × GDH11 was significantly higher than that of the female parent G70 and the male parent GDH11 (Figure 1A). Between 60 and 90 days after transplantation, the K+ content of the three tested plant samples first increased and then decreased, and the shift (increase to decrease) in the K+ content in the plants occurred 70 days after transplantation (Figure 1B). Moreover, 70 days after transplantation, the K+ content in the two-parent samples decreased significantly more than that in the hybrid. Subsequently, we investigated the field agronomic traits of the hybrid and their parents (Supplementary Table S1), and found that the hybrid G70 × GDH11 performed significantly better over the two parents, indicating that the hybrids with strong K+ content advantage had more robust stalks, longer and wider leaves, and larger biomass weight. These results showed that the hybrid had a stronger K+ absorption capacity and a higher ability to retain K+ in the leaves.
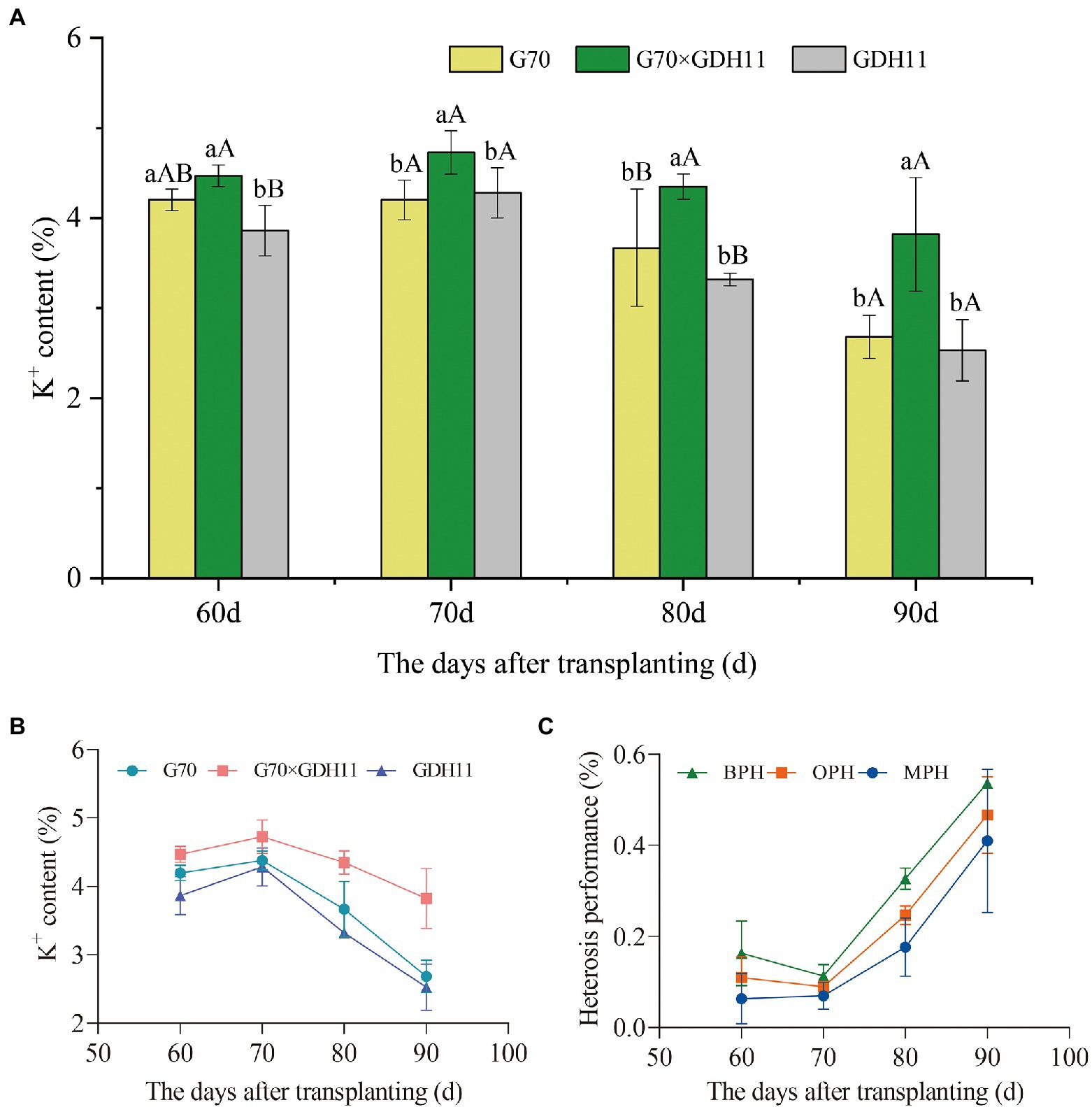
Figure 1. Potassium content of tobacco hybrids and their parents. (A) The K+ content of the hybrid G70 × GDH11 and its parents G70 and GDH11. (B) The changes in the K+ content in hybrid F1 and its parents between 60 and 90 days after transplantation. (C) The heterosis performance values of the hybrid G70 × GDH11. The average value of three biological replicates per material was used for mapping. Error bars represent significant differences among the three replicates. Significant differences in the K+ content at p < 0.05 and p < 0.01 were determined using Duncan’s new multiple range tests. The lowercase alphabets represent a significant difference (p < 0.05), while the uppercase alphabets represent a highly significant difference (p < 0.01).
The heterosis performance of G70 × GDH11 in different periods showed that K+ heterosis increased rapidly 70 days after transplantation (Figure 1C). Therefore, we took the plant material 70 days after transplantation as the experimental sample to investigate the key role of the absorptive capacity of tobacco roots in the formation of K+ content heterosis. Ninety days after transplantation, the OPH, MPH, and BPH of G70 × GDH11 were more than 40%, indicating that the K+ content of tobacco was higher in the hybrid offspring and heterosis breeding might produce hybrid plants with significantly higher K+ content than that in either parent plant.
Differences in transcriptome expression between the roots of parents and hybrids
Using the hybrid G70 × GDH11 and its parents G70 and GDH11, RNA sequencing was performed using IlluminaHiSeq2000 to obtain a comprehensive reference transcriptome of nine tobacco root samples. The sequencing results were subjected to quality control analysis and sequence alignment. Information on the quality control analysis of the sequencing data is presented in Table 1, and the results of the comparison between the original data and the reference genome after quality control are presented in Table 2. With an average error rate of 0.02% across all samples, Q20 > 98%, Q30 > 94%, and GC content >42%, the clean sequences accounted for more than 99% of the total sequences (Table 1). The total sequence numbers and the results of genome mapping of the three cDNA libraries showed that, on average, 65.9% of clean sequences were mapped to the tobacco K326 reference genome (Table 2). These results demonstrated that the sequencing data were reliable and could be used for further analyzing differentially expressed genes associated with target traits.
To evaluate the quality of the transcriptomics and gene differential expression level data, six genes were randomly selected for qRT-PCR analysis. The actin gene was used as an internal reference gene to analyze the level of expression of each gene. The genes and the corresponding primers used for qRT-PCR determination were listed in Supplementary Table S2. The changes in the expression of the selected genes based on the relative expression level followed a pattern similar to that found in the transcriptomics data (Figure 2), which indicated that the transcriptome profiling data were reliable. Therefore, we can carry out the next step of differential gene screening and expression analysis.
To analyze the causes of tobacco heterosis at the transcriptional level, we compared the transcriptional information of the F1 hybrid with that of G70 and GDH11, as well as the transcriptional information between the parents. In the subsequent analysis, we considered 18,245 genes that were simultaneously identified in the three genotypes (Supplementary File 2, Figure 3A). Using the threshold of p ≤ 0.05 and |log2 Fold Change| ≥ 2, we identified 226 upregulated and 367 downregulated transcripts between the hybrid and G70. Similarly, 400 upregulated and 287 downregulated transcripts were observed between the hybrid and GDH11, and 215 upregulated and 424 downregulated transcripts were observed between G70 and GDH11 (Figure 3C). The results of a Venn diagram analysis showed that only 24 DEGs co-existed in the three groups (Figure 3B), which indicated that there were significant differences in the transcriptional ubiquitous expression of the three genotypes. Therefore, we speculated that these DEGs might be the reason that tobacco hybrids show heterosis in some traits.
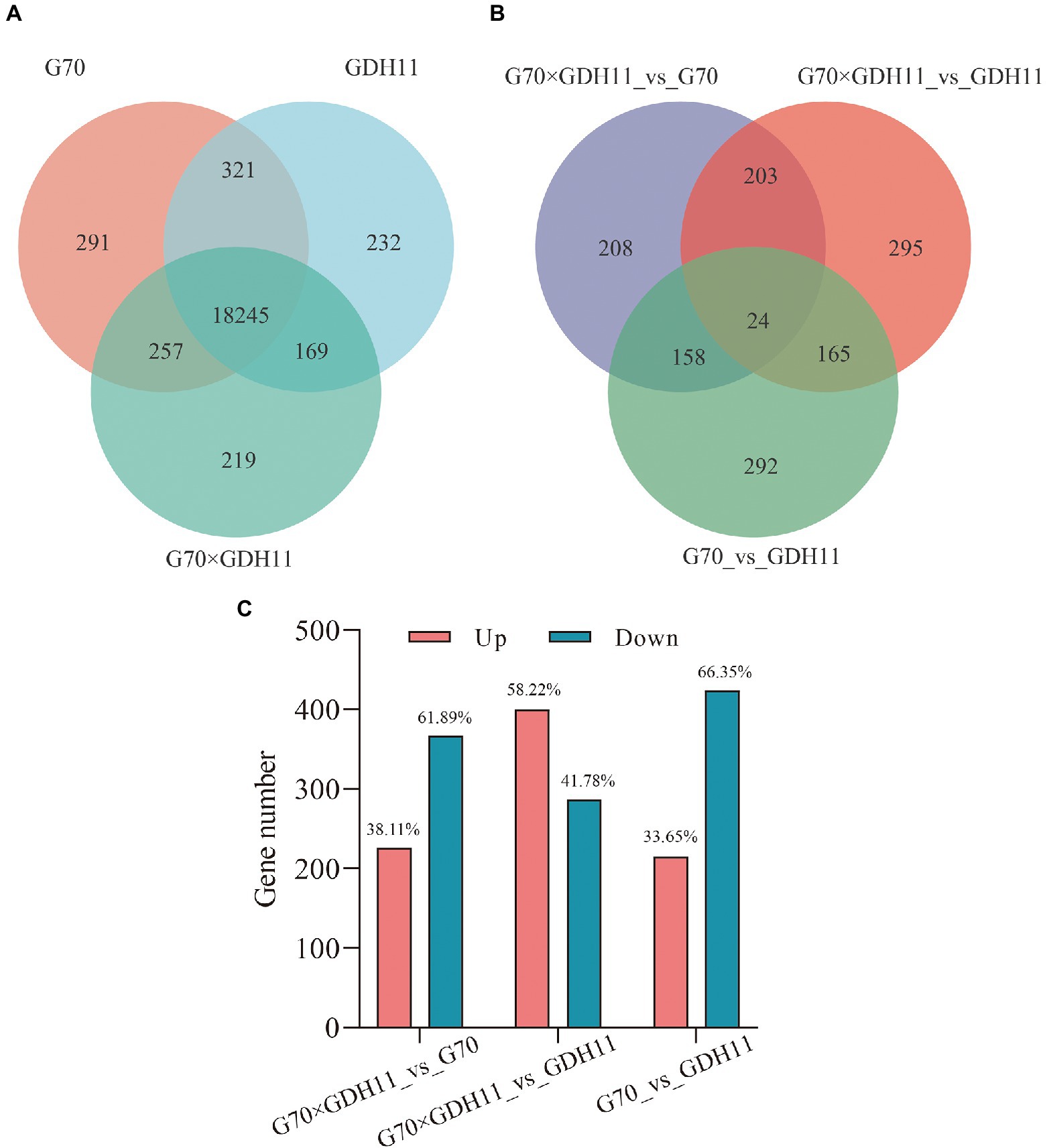
Figure 3. The transcriptional profiling statistics of different materials and comparison groups. (A) Venn diagram of the identified genes for the three tobacco varieties. (B) Venn diagram of the DEGs in the comparison groups G70_vs_GDH11, G70 × GDH11_vs_G70, and G70 × GDH11_vs_GDH11. (C) Statistics of up and down regulated differentiallyexpressed genes between hybrid and parental inbredlines.
Identification and analysis of expression patterns of DEGs in the hybrid
To further analyze the effects of these DEGs in the formation of K+ content heterosis in tobacco leaves, we divided the differential genes into 12 expression patterns (P1–P12, Figure 4A) and determined the effect of additive and non-additive expression of the genes on heterosis of K+ content. Genes in the P1 and P2 patterns were additively expressed. The genes in the P3–P6 pattern showed dominant expression, in which P3 and P4 showed partial paternal dominant expression, and P5 and P6 showed partial maternal dominant expression. P7–P9 showed low parental dominant expression, and P10–P12 showed over-high parental dominant expression. We divided these 12 expression patterns into five categories (Figure 4B), and different colors were used to indicate an expression pattern type.
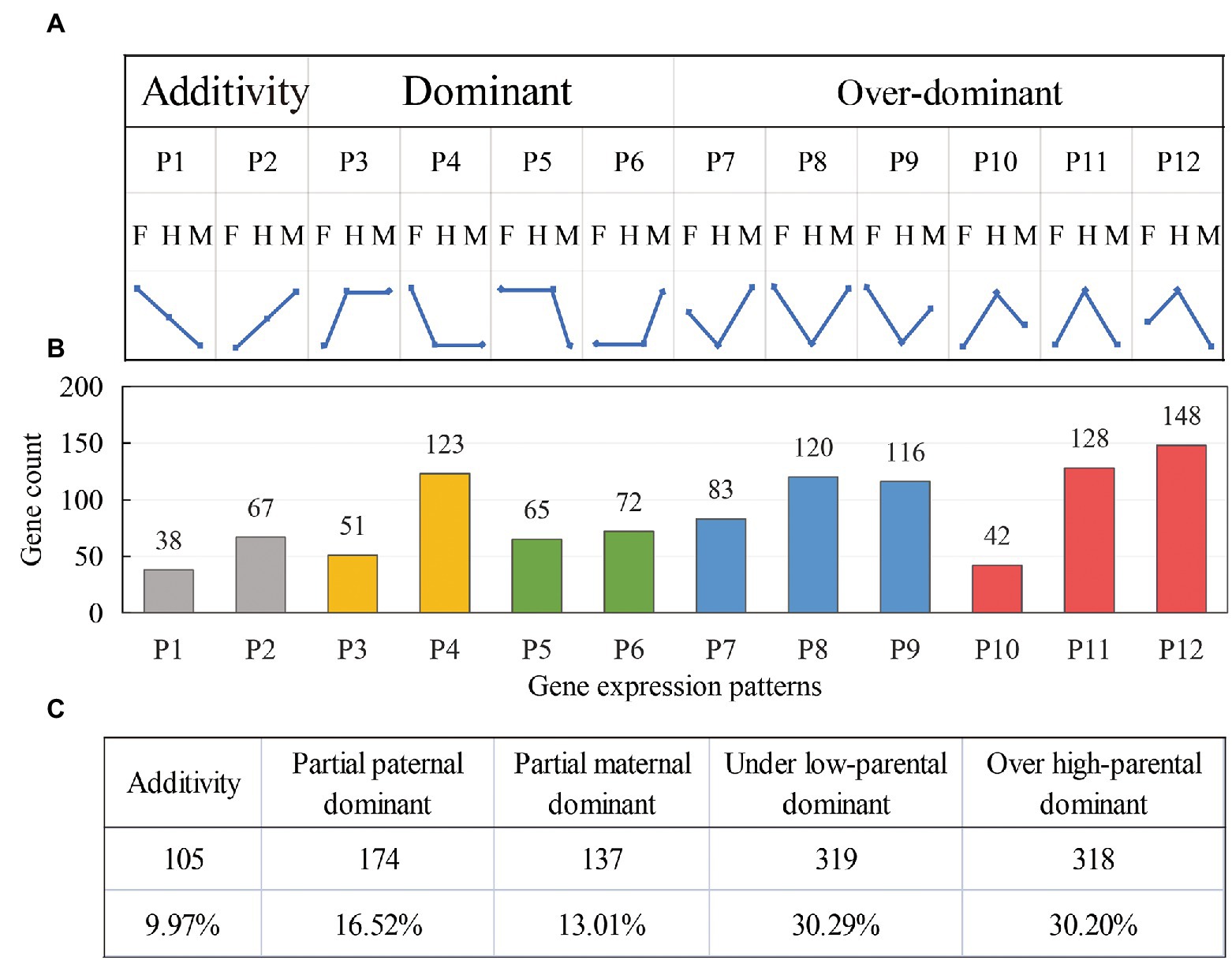
Figure 4. Identification and classification of differential gene expression patterns in the hybrids. (A) The division of 12 expression patterns; F: female parent, H: hybrid, and M: negative parent. (B) The distribution of DEGs in the hybrids among 12 expression patterns. (C) The number and proportion of DEGs in the five expression patterns.
In the hybrid G70 × GDH11, only 9.97% (P1 and P2) of the gene expression levels were between the parents, showing an additive expression pattern (Figure 4C). The remaining 90.03% of the genes showed a non-additive expression pattern, which indicated that the formation of K+ content heterosis was more affected by the non-additive effect of the genes. Among the non-additively expressed genes, 29.53% (P3-P6) of the genes showed a dominant expression pattern, and 60.49% of the genes (P7–P12) showed an over-dominant expression pattern. The results indicated that the over-dominant expression pattern of DEGs played a major role in the formation of K+ content heterosis.
Functional enrichment analysis of non-additively expressed genes
The degree of heterosis was found to depend on the non-additive effect of genes. Moreover, the non-additive expression of the genes affected the performance of hybrid offspring. To understand the biological functions of these non-additivity expressed genes, we performed the GO functional enrichment analysis on the gene sets with dominant (120 DEGs) and over-dominant upregulated (318 DEGs) expression. The GO enrichment analysis results of downregulated genes in non-additivity expression are shown in Supplementary Figures S1, S2. The results showed that the upregulated genes were mainly enriched in metal ion transport, calcium ion binding, potassium/sodium transporter activity, regulation of root morphogenesis, and ion channel activity (Figure 5A). The upregulated genes with over-dominant expression could be enriched in more GO functional terms, mainly including root defense response, metal ion transport and response, ion balance and homeostasis, ion channel activity, root meristem growth, and the regulation of root hair (Figure 5B). The results of the functional analysis indicated that these non-additively upregulated differential genes in the hybrid G70 × GDH11 were good sources of potassium uptake in the hybrid. Their overexpression facilitates root growth and regulation of the meristem. These genes are also involved in ion transport and the regulation of ion channel activity, which enables K+ in the soil to be more efficiently absorbed by the roots and transported to the tobacco leaves, thus causing the potassium content in the leaves of the hybrids to show heterosis.
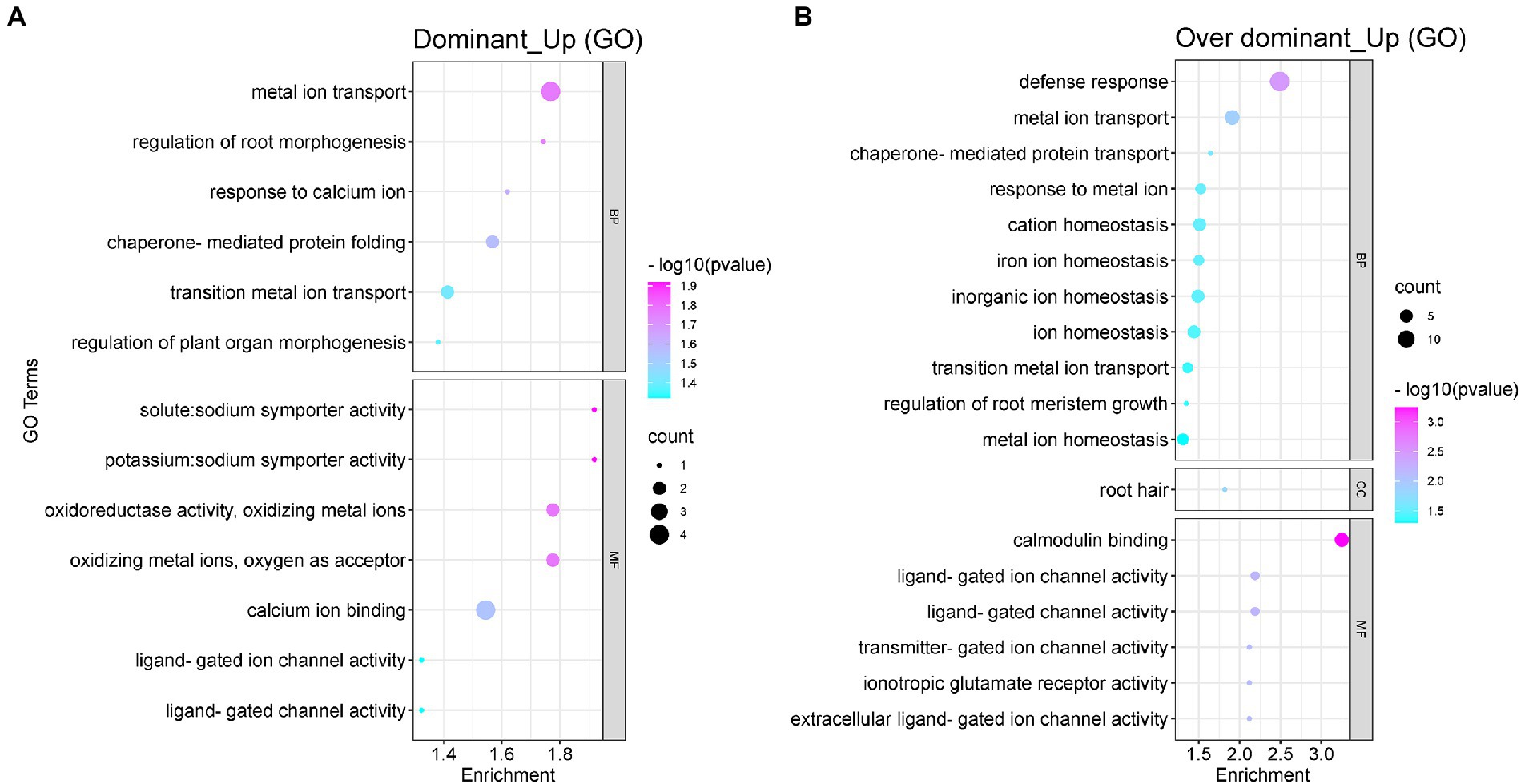
Figure 5. The GO functional enrichment analysis of the non-additive upregulated DEGs. (A) The GO functional enrichment analysis of the upregulated dominant DEGs. (B) The GO functional enrichment analysis of the upregulated over-dominant DEGs.
To understand the biological pathways associated with the non-additive upregulated DEGs, we performed the KEGG pathway enrichment analysis for these genes. The results showed that the DEGs of the dominant and over-dominant gene sets were enriched in 36 and 62 pathways, respectively (Supplementary Files 3, 4). The first-level classification results of these pathways showed that most of them belonged to the categories of metabolism, genetic information processing, cellular processes, and environmental information processing (Figures 6A,B). The non-additive upregulated DEGs were mainly involved in energy metabolism, starch and sucrose metabolism, photosynthesis, carbohydrate formation, amino acid metabolism, plant hormone signal transduction, and signaling pathway, indicating that the significant expression of these biological processes in hybrids provides energy for the development of the roots and the absorption and transport of K+, which is helpful to the accumulation of K+ and the production of K+ content superiority in hybrids.
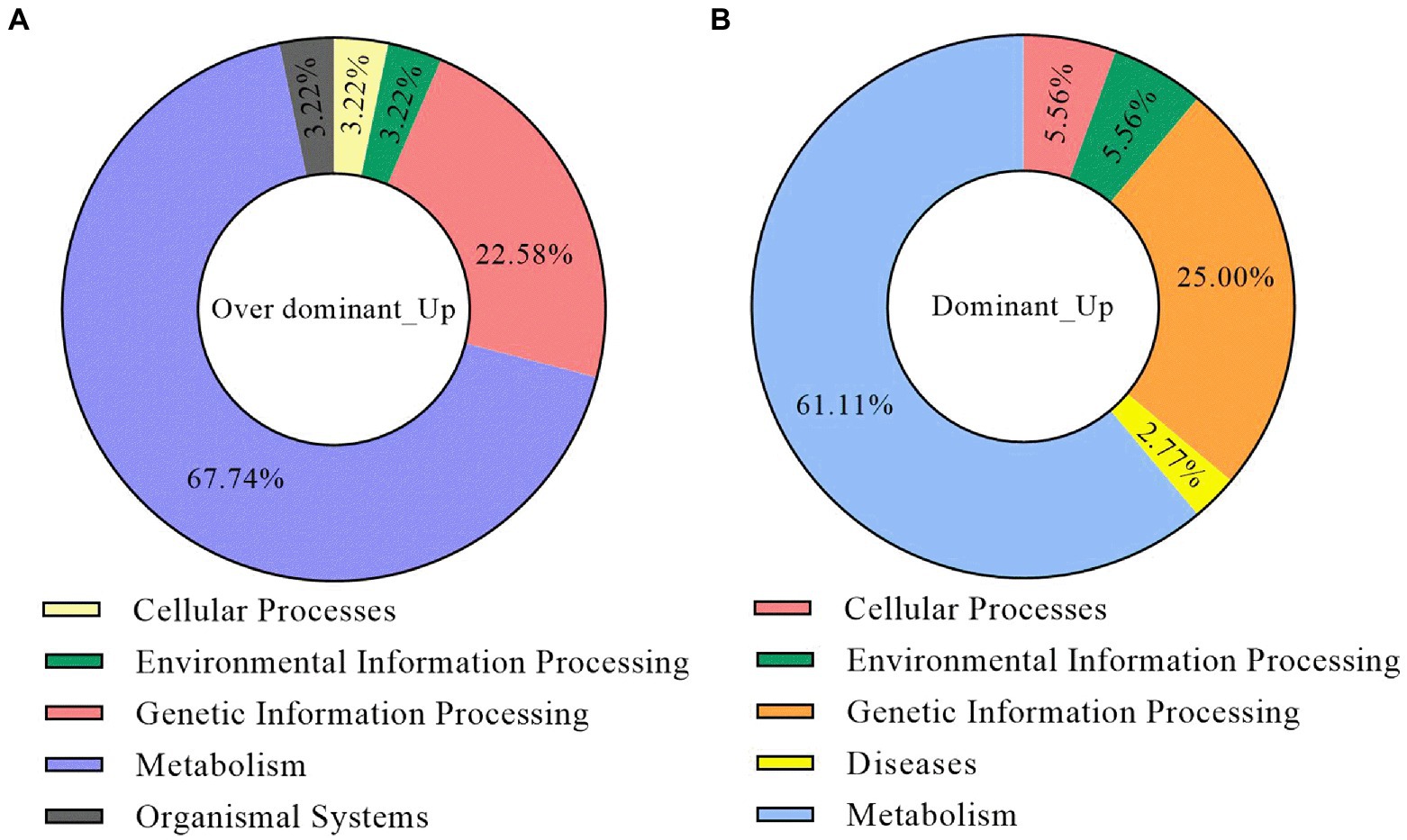
Figure 6. The KEGG pathway enrichment analysis of the non-additive upregulated DEGs. (A) The classification of the KEGG enrichment results for dominant upregulated DEGs. (B) The classification of the KEGG enrichment results for the over-dominant upregulated DEGs.
Mining of key genes regulating potassium absorption in hybrids
To further analyze the basis of the formation of tobacco K+ heterosis, we performed homology alignment and annotation analysis of the genes associated with the important GO entries (Table 3). The results showed that the probable potassium transporter 17 (NtKT17) and potassium transporter 5-like (NtKT5), associated with potassium transport, showed over-dominant upregulation and dominant expression in the hybrid G70 × GDH11, respectively. They play an important role in metal ion transport in plants. The glutamate receptor 2.2-like and glutamate receptor 2.8-like associated with ligand-gated channel activity showed an over-dominant upregulation expression in the hybrids. Some calcium-dependent protein kinase 28-like and calmodulin-binding protein 60 D-like related to the potassium ion absorption mechanism also showed a over-dominant upregulation. Furthermore, tabacum protein detoxification 42-like, involved in root hair development, and LOC107782957, involved in root morphology regulation, also showed over-dominant and dominant upregulation expression in the hybrids, respectively. We selected six genes related to K+ absorption and transport for the Real-Time Fluorescence Quantitative PCR detection (Figure 7). The genes and the corresponding primers are listed in Supplementary Table S3. The results showed that the expression level of these genes in hybrid G70 × GDH11 was significantly higher than that in two parental inbred lines, which indicated that these genes indeed have significant over-dominant and dominant expression patterns in hybrids. These results suggested that the over-dominant and dominant upregulation of key genes associated with K+ uptake and transport leads to the generation of K+ heterosis.
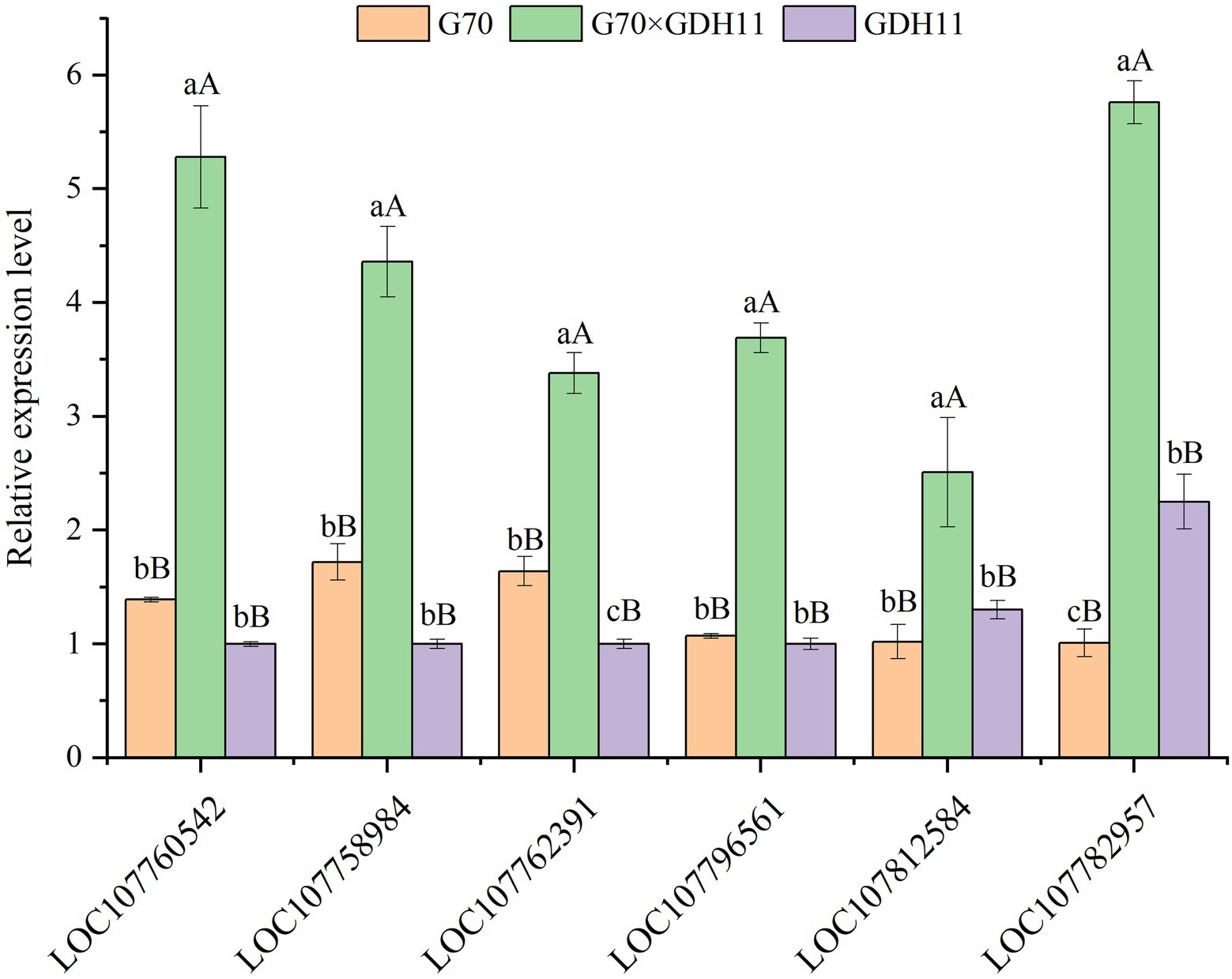
Figure 7. Expression level analysis of K+ related genes by qRT-PCR. The lowercase alphabets represent a significant difference (p < 0.05), while the uppercase alphabets represent a highly significant difference (p < 0.01).
Discussion
The growth and development of plants are inseparable from the action of various essential elements. Among them, K+ is a major element that affects various aspects of plant growth (Sano et al., 2009). Especially for food crops and cash crops, an increase in the K+ content can often directly improve the yield and quality (Yang and Wang, 2017). Thus, the absorption and transport of K+ have gained attention in recent years (Pettigrew, 2008). The quality of the tobacco plant is affected by K+. However, studies on K+ channels and transporters in tobacco are limited, especially on the genes regulating K+ heterosis. Our study showed that the heterosis expression of K+ content in tobacco was prominent and affected by the non-additive effect of the genes, of which the over-dominant effect played a major role. The results showed that breeding new tobacco varieties with high K+ content through heterosis is feasible.
With the development of modern molecular biology and advancement in quantitative genetics, research on heterosis has progressed. Transcriptomics is commonly used to analyze the mechanism of crop heterosis formation and satisfactory results have also been achieved in many studies (Palareti et al., 2016; Jiang et al., 2017; Birdseye et al., 2021; Liu et al., 2021, 2022). A correlation between heterosis and gene expression levels was shown in many studies (Wei et al., 2009; He et al., 2010). However, studies on the heterosis of important traits in tobacco are limited. Our transcriptomics results revealed that 35,520 genes were transcribed in at least one genotype (Supplementary File 5), which showed the active genes that regulate K+ uptake and transport in tobacco.
Potassium ion is absorbed from the soil by root epidermal cells and cortical cells in plants, and ion channels and transport carriers play an important role in this process (Sánchez-Caldero et al., 2009). Liu et al. (2019) showed that the expression of NtTPK1 and NtORK1 might be related to the K+ content and the formation of heterosis. Previous studies found that the influx K+ channel genes AKT1, NtKC1, KAT1, and K+ transporter gene NtTPK1 showed dominant and over-dominant expression in the roots and leaves of the hybrids, indicating that these genes played a key role in the formation of K+ content heterosis in hybrid tobacco leaves (Luo et al., 2022). Some studies have shown that the K+ transporter plays a major role in absorbing K+ from the rhizosphere and can also be used as a low-affinity K+ transporter at high K+ concentrations (Kim et al., 1998; Ahn et al., 2004). In this study, probable potassium transporter 17 (NtKT17) and potassium transporter 5-like (NtKT5) showed upregulated over-dominant and dominant expression in the hybrids, respectively, with advantages in expression. The results further showed that these two genes were also involved in the absorption of K+ in the roots, and the absorption rate in the hybrids was higher than that in the parents.
By opening and closing, ion channels regulate the speed of movement of specific substances in and out of cells, required for various cell functions (Hedrich, 2012). K+ channel parenchyma is a transmembrane protein that is present on the cytoplasmic membrane or the inner membrane of plant cells (Hosy et al., 2003; Dreyer and Uozumi, 2011). Among these, ligand-gated channels, also known as chemical-gated ion channels, open after certain transmitters bind to the channel protein receptor molecule, thus allowing Na+, Ca2+, or K+ to pass through (Liu et al., 2006a; Xie et al., 2020). We found that the glutamate receptor 2.2-like and glutamate receptor 2.8-like associated with ligand-gated channel activity showed an upregulated over-dominant expression in the hybrid. Therefore, we speculated that these two types of glutamate receptors might also play an important role in the formation of heterosis in tobacco K+ content, thus contributing to K+ transport by the roots. However, their mechanism of action needs to be further analyzed and confirmed.
Plant root architecture plays an important role in nutrient absorption, and there are considerable differences in root architecture between different species of plants or different varieties of the same plant species (Hao, 2015). Studies showed that the number of roots was positively correlated with the K+ content of leaves in cotton (Hsu et al., 1978). Root hairs can absorb nutrients in the soil more effectively by increasing the contact area with the soil (Jungk, 2001; Wang et al., 2016). From the perspective of molecular biology, ion channels and transporters in root hairs greatly facilitate nutrient absorption (Libault et al., 2010). Our results showed that the uncharacterized LOC107782957 gene involved in the regulation of tobacco root morphogenesis upregulated dominant expression in the hybrids. Furthermore, protein detoxification 42-like, involved in root hair development, and probable glutamate carboxypeptidase 2, involved in the regulation of root meristem growth, showed an upregulated over-dominant expression pattern. The results indicated that the upregulated expression of these key genes in the hybrids promotes root growth and development, which is crucial for nutrient uptake by the roots. Many researchers argue that energy metabolism strongly affects the growth and development of plant roots (Yokota et al., 2003; Libault et al., 2010; Wang et al., 2016). Similar to the results of those studies, the KEGG enrichment results of this study also indicated that many upregulated non-additive DEGs are involved in energy metabolism and carbohydrate formation, thus enhancing the root absorption capacity of tobacco hybrids.
Crop heterosis formation is an extremely complex process. Our study provided a new perspective on the mechanism of K+ content heterosis at the transcriptional level and might provide new information for cultivating tobacco varieties with high K+ content. Potassium exists in plants in an ionic state, and its accumulation is jointly influenced by the growth and development of the root, the expression of K+ uptake genes and transporters, K+ channel activity, and the homeostasis of ions. Overexpression of the genes associated with these physiological functions in hybrids contributes to K+ absorption and accumulation, thus exhibiting K+ content heterosis.
Data availability statement
The data presented in the study are deposited in the NCBI Sequence Read Archive (SRA) repository, accession number PRJNA836042.
Author contributions
ZM, WL, and RL conceived and designed the research. WL, PW, and YK conducted field experiments. KP, SZ, and TL conducted the molecular biology experiments. ZM, LD, and RJ analyzed the data. ZM, KP, and YH wrote the manuscript. ZM and RL revised the manuscript. All authors contributed to the article and approved the submitted version.
Funding
This research was funded by the National Science Foundation of China (no. 32060510), the Talent Cultivation Project for High-Level Renovation Plan of Guizhou Province (no. [2016]5663), the Guizhou Provincial Key Foundation (no. [2019]1405), and Tobacco Company of Guizhou Province (nos. 2020XM07 and 201904).
Conflict of interest
The authors declare that the research was conducted in the absence of any commercial or financial relationships that could be construed as a potential conflict of interest.
Publisher’s note
All claims expressed in this article are solely those of the authors and do not necessarily represent those of their affiliated organizations, or those of the publisher, the editors and the reviewers. Any product that may be evaluated in this article, or claim that may be made by its manufacturer, is not guaranteed or endorsed by the publisher.
Supplementary material
The Supplementary Material for this article can be found online at: https://www.frontiersin.org/articles/10.3389/fpls.2022.940787/full#supplementary-material
Supplementary File 1 | The original data of Figure 1.
Supplementary File 2 | The number of genes and information on gene expression identified for the three genotype materials.
Supplementary File 3 | The KEGG annotation results of the dominant upregulated DEGs.
Supplementary File 4 | The KEGG annotation results of the over-dominant upregulated DEGs.
Supplementary Figure S1 | The GO functional enrichment analysis of the downregulated dominant DEGs.
Supplementary Figure S2 | The GO functional enrichment analysis of the downregulated over-dominant DEGs.
Footnotes
References
Ahn, S. J., Shin, R., and Schachtman, D. P. (2004). Expression of KT/KUP genes in Arabidopsis and the role of root hairs in K+ uptake. Plant Physiol. 134, 1135–1145. doi: 10.1104/pp.103.034660
Anders, S., and Huber, W. (2012). Differential expression of RNA-Seq data at the gene level–the DESeq package. Biotechnol. Bull. 28, 1–28. doi: 10.13560/j.cnki.biotech.bull.1985.2018-0814
Anders, S., Pyl, P. T., and Huber, W. (2015). HTSeq-A python framework to work with high-throughput sequencing data. Bioinformatics 31, 166–169. doi: 10.1093/bioinformatics/btu638
Beddington, J. (2010). Food security: contributions from science to a new and greener revolution. Philos. Trans. R. Soc. B Biol. Sci. 365, 61–71. doi: 10.1098/rstb.2009.0201
Birdseye, D., De Boer, L. A., Bai, H., Zhou, P., Shen, Z., Schmelz, E. A., et al. (2021). Plant height heterosis is quantitatively associated with expression levels of plastid ribosomal proteins. Proc. Natl. Acad. Sci. U. S. A. 118, 1–7. doi: 10.1073/pnas.2109332118
Bolger, A. M., Lohse, M., and Usadel, B. (2014). Trimmomatic: a flexible trimmer for Illumina sequence data. Bioinformatics 30, 2114–2120. doi: 10.1093/bioinformatics/btu170
Chai, W. W., Wang, W. Y., Cui, Y. N., and Wang, S. M. (2019). Research progress of function on KUP/HAK/KT family in plants. Plant Physiol. J. 55, 1747–1761. doi: 10.13592/j.cnki.ppj.2019.0133
Chen, P., Shi, J., Zhao, L., Li, Y., Wen, D., and Deng, J. (2010). Study on the potassium of flue-cured tobacco among different variety and locations of Yunna. J. Yunnan Univ. Sci. Ed. 32, 42–46.
Dai, X. Y., Su, Y. R., Wei, W. X., Wu, J. S., and Fan, Y. K. (2009). Effects of top excision on the potassium accumulation and expression of potassium channel genes in tobacco. J. Exp. Bot. 60, 279–289. doi: 10.1093/jxb/ern285
Davenport, C. (1908). Degeneration, albinism and inbreeding. Science 28, 454–455. doi: 10.1126/science.28.718.454.c
Dreyer, I., and Uozumi, N. (2011). Potassium channels in plant cells. FEBS J. 278, 4293–4303. doi: 10.1111/j.1742-4658.2011.08371.x
Gaymard, F., Pilot, G., Lacombe, B., Bouchez, D., Bruneau, D., Boucherez, J., et al. (1998). Identification and disruption of a plant shaker-like outward channel involved in K+ release into the xylem sap. Cell 94, 647–655. doi: 10.1016/S0092-8674(00)81606-2
Gobert, A., Isayenkov, S., Voelker, C., Czempinski, K., and Maathuis, F. J. M. (2007). The two-pore channel TPK1 gene encodes the vacuolar K+ conductance and plays a role in K+ homeostasis. Proc. Natl. Acad. Sci. U. S. A. 104, 10726–10731. doi: 10.1073/pnas.0702595104
Greiner, T., Ramos, J., Alvarez, M. C., Gurnon, J. R., Kang, M., Van Etten, J. L., et al. (2011). Functional HAK/KUP/KT-like potassium transporter encoded by chlorella viruses. Plant J. 68, 977–986. doi: 10.1111/j.1365-313X.2011.04748.x
Hao, Y. (2015). Physiological mechanisms of high potassium efficiency and fertilization cffects of cotton.
He, G., Zhu, X., Elling, A. A., Chen, L., Wang, X., Guo, L., et al. (2010). Global epigenetic and transcriptional trends among two rice subspecies and their reciprocal hybrids. Plant Cell 22, 17–33. doi: 10.1105/tpc.109.072041
Hedrich, R. (2012). Ion channels in plants. Physiol. Rev. 92, 1777–1811. doi: 10.1152/physrev.00038.2011
Hirsch, R. E., Lewis, B. D., Spalding, E. P., and Sussman, M. R. (1998). A role for the AKT1 potassium channel in plant nutrition. Science 280, 918–921. doi: 10.1126/science.280.5365.918
Hochholdinger, F., and Baldauf, J. A. (2018). Heterosis in Plants. Curr. Biol. 28, R1089–R1092. doi: 10.1016/j.cub.2018.06.041
Hosy, E., Vavasseur, A., Mouline, K., Dreyer, I., Gaymard, F., Porée, F., et al. (2003). The Arabidopsis outward K+ channel GORK is involved in regulation of stomatal movements and plant transpiration. Proc. Natl. Acad. Sci. U. S. A. 100, 5549–5554. doi: 10.1073/pnas.0733970100
Hsu, H. H., Lancaster, J. D., and Jones, W. F. (1978). Potassium concentration in leaf-blades and petioles as affected by potassium fertilization and stage of maturity of cotton 1. Commun. Soil Sci. Plant Anal. 9, 265–277. doi: 10.1080/00103627809366807
Jiang, Y., Schmidt, R. H., Zhao, Y., and Reif, J. C. (2017). Quantitative genetic framework highlights the role of epistatic effects for grain-yield heterosis in bread wheat. Nat. Genet. 49, 1741–1746. doi: 10.1038/ng.3974
Jungk, A. (2001). Root hairs and the acquisition of plant nutrients from soil. J. Plant Nutr. Soil Sci. 164, 121–129. doi: 10.1002/1522-2624(200104)164:2<121::AID-JPLN121>3.0.CO;2-6
Kim, E. J., Kwak, J. M., Uozumi, N., and Schroeder, J. I. (1998). AtKUP1: An arabidopsis gene encoding high-affinity potassium transport activity. Plant Cell 10, 51–62. doi: 10.1105/tpc.10.1.51
Kim, D., Langmead, B., and Salzberg, S. L. (2015). HISAT: a fast spliced aligner with low memory requirements Daehwan HHS public access. Nat. Methods 12, 357–360. doi: 10.1038/nmeth.3317
Li, D., Huang, Z., Song, S., Xin, Y., Mao, D., Lv, Q., et al. (2016). Integrated analysis of phenome, genome, and transcriptome of hybrid rice uncovered multiple heterosis-related loci for yield increase. Proc. Natl. Acad. Sci. U. S. A. 113, E6026–E6035. doi: 10.1073/pnas.1610115113
Li, Z., and Li, J. (2001). Study on difference in morpholofical and physiological characters of wheat varieties to potassium. J. Plant Nutr. Fertil. 7, 36–43.
Li, T., and Ma, G. (2003). Physiological and morphological characteristics of roots in grain amaranth genotypes enrichment in potassium. Zuo wu xue bao. 30, 1145–1151. Available at: http://europepmc.org/abstract/cba/470214 (Accessed November 2004).
Libault, M., Brechenmacher, L., Cheng, J., Xu, D., and Stacey, G. (2010). Root hair systems biology. Trends Plant Sci. 15, 641–650. doi: 10.1016/j.tplants.2010.08.010
Liu, W., He, G., and Deng, X. W. (2021). Biological pathway expression complementation contributes to biomass heterosis in Arabidopsis. Proc. Natl. Acad. Sci. U. S. A. 118, 1–9. doi: 10.1073/pnas.2023278118
Liu, R., Huang, N., Deng, G., Feng, Y., and Ding, W. (2006b). The study on technique of nitrogen rates, density and leaves of tobacco remained Guiyan 4. J. Mt. Agric. Biol. 25, 95–100.
Liu, G., Wang, Y., Sun, Y., and Wang, W. (2006a). Proteins for Transpor t of potassium in higher plants. Biotechnol. Bull. 21, 13–18. doi: 10.13560/j.cnki.biotech.bull.1985.2006.05.003
Liu, M., Zhang, W., Chi, X., Ding, F., Xu, X., Zeng, L., et al. (2019). Effect of potassium supply level on potassium content and formation of heterosis in tobacco leaves. J. Henan Agric. Univ. 53, 839–846. doi: 10.16445/j.cnki.1000-2340.2019.06.001
Liu, W., Zhang, Y., He, H., He, G., and Deng, X. W. (2022). From hybrid genomes to heterotic trait output: challenges and opportunities. Curr. Opin. Plant Biol. 66:102193. doi: 10.1016/j.pbi.2022.102193
Liu, R., Zhu, J., Yu, Q., Ding, F., Nie, Q., Huang, Y., et al. (2016). Breeding of a novel tobacco variety Guiyan 202 and its characteristics. Chinese Tob. Sci. 37, 8–13. doi: 10.13496/j.issn.1007-5119.2016.06.002
Livak, K. J., and Schmittgen, T. D. (2001). Analysis of relative gene expression data using real-time quantitative PCR and the 2-ΔΔCT method. Methods 25, 402–408. doi: 10.1006/meth.2001.1262
Luo, W., Jiang, C., Yu, Q., Xiong, J., Duan, L., Mo, Z., et al. (2022). Analysis of Heterosis of potassium content in tobacco leaf and breeding. Mol. Plant 2, 1–24. doi: 10.13271/j.mpb.020.001788
Munns, R., Wallace, P. A., Teakle, N. L., and Colmer, T. D. (2010). Measuring soluble ion concentrations (Na(+), K(+), cl(−)) in salt-treated plants. Methods Mol. Biol. 639, 371–382. doi: 10.1007/978-1-60761-702-0_23
Murad, L., Yoong Lim, K., Christopodulou, V., Matyasek, R., Lichtenstein, C. P., Kovarik, A., et al. (2002). The origin of tobacco’s T genome is traced to a particular lineage within Nicotiana tomentosiformis (Solanaceae). Am. J. Bot. 89, 921–928. doi: 10.3732/ajb.89.6.921
Palareti, G., Legnani, C., Cosmi, B., Antonucci, E., Erba, N., Poli, D., et al. (2016). Comparison between different D-dimer cutoff values to assess the individual risk of recurrent venous thromboembolism: analysis of results obtained in the DULCIS study. Int. J. Lab. Hematol. 38, 42–49. doi: 10.1111/ijlh.12426
Pettigrew, W. T. (2008). Potassium influences on yield and quality production for maize, wheat, soybean and cotton. Physiol. Plant. 133, 670–681. doi: 10.1111/j.1399-3054.2008.01073.x
Ragel, P., Raddatz, N., Leidi, E. O., Quintero, F. J., and Pardo, J. M. (2019). Regulation of K+ nutrition in plants. Front. Plant Sci. 10:281. doi: 10.3389/fpls.2019.00281
Reintanz, B., Szyroki, A., Ivashikina, N., Ache, P., Godde, M., Becker, D., et al. (2002). AtKC1, a silent Arabidopsis potassium channel α-subunit modulates root hair K+ influx. Proc. Natl. Acad. Sci. U. S. A. 99, 4079–4084. doi: 10.1073/pnas.052677799
Roberts, A., Trapnell, C., Donaghey, J., Rinn, J. L., and Pachter, L. (2011). Improving RNA-Seq expression estimates by correcting for fragment bias. Genome Biol. 12, R22–R14. doi: 10.1186/gb-2011-12-3-r22
Sánchez-Caldero, L., Chacó-López, A., Alatorre-Cobos, F., Leyva-González, M. A., and Herrera-Estrella, L. (2009). Signaling and Communication in Plants. Transporters and Pumps in Plant Signaling.
Sano, T., Kutsuna, N., Becker, D., Hedrich, R., and Hasezawa, S. (2009). Outward-rectifying K+ channel activities regulate cell elongation and cell division of tobacco BY-2 cells. Plant J. 57, 55–64. doi: 10.1111/j.1365-313X.2008.03672.x
Santa-María, G. E., Oliferuk, S., and Moriconi, J. I. (2018). KT-HAK-KUP transporters in major terrestrial photosynthetic organisms: a twenty years tale. J. Plant Physiol. 226, 77–90. doi: 10.1016/j.jplph.2018.04.008
Schnable, P. S., and Springer, N. M. (2013). Progress toward understanding heterosis in crop plants. Annu. Rev. Plant Biol. 64, 71–88. doi: 10.1146/annurev-arplant-042110-103827
Sottocornola, B., Gazzarrini, S., Olivari, C., Romani, G., Valbuzzi, P., Thiel, G., et al. (2008). 14-3-3 proteins regulate the potassium channel KAT1 by dual modes. Plant Biol. 10, 231–236. doi: 10.1111/j.1438-8677.2007.00028.x
Tian, M., Nie, Q., Li, Z., Zhang, J., Liu, Y., Long, Y., et al. (2018). Transcriptomic analysis reveals overdominance playing a critical role in nicotine heterosis in Nicotiana tabacum L. BMC Plant Biol. 18, 48–10. doi: 10.1186/s12870-018-1257-x
Trapnell, C., Williams, B. A., Pertea, G., Mortazavi, A., Kwan, G., Van Baren, M. J., et al. (2011). Transcript assembly and abundance estimation from RNA-Seq reveals thousands of new transcripts and switching among isoforms. Nat. Biotechnol. 28, 511–515. doi: 10.1038/nbt.1621.Transcript
Wan, J. (2018). Genetic crop improvement: a guarantee for sustainable agricultural production. Engineering 4, 431–432. doi: 10.1016/j.eng.2018.07.019
Wang, H., Lan, P., and Shen, R. F. (2016). Integration of transcriptomic and proteomic analysis towards understanding the systems biology of root hairs. Proteomics 16, 877–893. doi: 10.1002/pmic.201500265
Wang, X., Wang, B., Song, Z., Zhao, L., Ruan, W., Gao, Y., et al. (2022). A spatial–temporal understanding of gene regulatory networks and NtARF-mediated regulation of potassium accumulation in tobacco. Planta 255, 9–15. doi: 10.1007/s00425-021-03790-2
Wei, G., Tao, Y., Liu, G., Chen, C., Luo, R., Xia, H., et al. (2009). A transcriptomic analysis of superhybrid rice LYP9 and its parents. Proc. Natl. Acad. Sci. U. S. A. 106, 7695–7701. doi: 10.1073/pnas.0902340106
Williams, W. (1959). Heterosis and the genetics of complex characters. Heredity (Edinb). 15, 327–328. doi: 10.1038/hdy.1960.88
Wu, X., Liu, Y., Zhang, Y., and Gu, R. (2021). Advances in research on the mechanism of Heterosis in plants. Front. Plant Sci. 12:745726. doi: 10.3389/fpls.2021.745726
Xie, Z. Q., Tian, X. T., Zheng, Y. M., Zhan, L., Chen, X. Q., Xin, X. M., et al. (2020). Antiepileptic geissoschizine methyl ether is an inhibitor of multiple neuronal channels. Acta Pharmacol. Sin. 41, 629–637. doi: 10.1038/s41401-019-0327-4
Xu, J., Zhou, Y., Xu, Z., Chen, Z., and Duan, L. (2020). Combining physiological and metabolomic analysis to unravel the regulations of coronatine alleviating water stress in tobacco (Nicotiana tabacum L.). Biomol. Ther. 10, 1–16. doi: 10.3390/biom10010099
Yang, X., and Wang, Y. (2017). Current Progress of potassium nutrition on quality in Chinese herbs. J. Zhejiang Chinese Med. Univ. 41, 711–714. doi: 10.16466/j.issn1005-5509.2017.08.019
Yokota, E., Izeki, T., and Shimmen, T. (2003). Possible involvement of energy metabolism in the change of cytoplasm organization induced by a protein phosphatase inhibitor, calyculin A, in root hair cells of Limnobium stoloniferum. Protoplasma 221, 217–226. doi: 10.1007/s00709-002-0055-2
Yu, D., Gu, X., Zhang, S., Dong, S., Miao, H., Gebretsadik, K., et al. (2021). Molecular basis of heterosis and related breeding strategies reveal its importance in vegetable breeding. Hortic. Res. 8, 120–117. doi: 10.1038/s41438-021-00552-9
Zhang, D., Zhang, Y., Li, L., Song, D., Shi, S., Lei, C., et al. (2020). The formation mechanism of Heterosis and its application in animal genetic breeding. Genomics Appl. Biol. 39, 104–108. doi: 10.13417/j.gab.039.000104
Keywords: potassium, heterosis, transcriptomics, Nicotiana tabacum, quality
Citation: Mo Z, Luo W, Pi K, Duan L, Wang P, Ke Y, Zeng S, Jia R, Liang T, Huang Y and Liu R (2022) Comparative transcriptome analysis between inbred lines and hybrids provides molecular insights into K+ content heterosis of tobacco (Nicotiana tabacum L.). Front. Plant Sci. 13:940787. doi: 10.3389/fpls.2022.940787
Edited by:
Andrew H. Paterson, University of Georgia, United StatesReviewed by:
Ming Zheng, Oil Crops Research Institute (CAAS), ChinaXueyong Yang, Institute of Vegetables and Flowers (CAAS), China
Copyright © 2022 Mo, Luo, Pi, Duan, Wang, Ke, Zeng, Jia, Liang, Huang and Liu. This is an open-access article distributed under the terms of the Creative Commons Attribution License (CC BY). The use, distribution or reproduction in other forums is permitted, provided the original author(s) and the copyright owner(s) are credited and that the original publication in this journal is cited, in accordance with accepted academic practice. No use, distribution or reproduction is permitted which does not comply with these terms.
*Correspondence: Renxiang Liu, cnhsaXVAZ3p1LmVkdS5jbg==