- 1Key Laboratory of Plant Functional Genomics of the Ministry of Education/Jiangsu Key Laboratory of Crop Genomics and Molecular Breeding, Agricultural College of Yangzhou University, Yangzhou, China
- 2Co-innovation Center for Modern Production Technology of Grain Crops of Jiangsu Province/Key Laboratory of Crop Genetics and Physiology of Jiangsu Province, Yangzhou University, Yangzhou, China
- 3Institute of Agricultural Sciences for Lixiahe Region in Jiangsu, Yangzhou, China
- 4State Key Laboratory of Rice Biology, China National Rice Research Institute, Hangzhou, China
- 5National Key Laboratory of Plant Molecular Genetics, CAS Center for Excellence in Molecular Plant Sciences, Institute of Plant Physiology and Ecology, Chinese Academy of Sciences, Shanghai, China
- 6Joint International Research Laboratory of Agriculture and Agri-Product Safety, The Ministry of Education of China, Institutes of Agricultural Science and Technology Development, Yangzhou University, Yangzhou, China
Rice blast, caused by Magnaporthe oryzae (M. oryzae), is one of the most destructive diseases threatening rice production worldwide. Development of resistant cultivars using broad-spectrum resistance (R) genes with high breeding value is the most effective and economical approach to control this disease. In this study, the breeding potential of Pigm gene in geng/japonica rice breeding practice in Jiangsu province was comprehensively evaluated. Through backcross and marker-assisted selection (MAS), Pigm was introduced into two geng rice cultivars (Wuyungeng 32/WYG32 and Huageng 8/HG8). In each genetic background, five advanced backcross lines with Pigm (ABLs) and the same genotypes as the respective recurrent parent in the other 13 known R gene loci were developed. Compared with the corresponding recurrent parent, all these ABLs exhibited stronger resistance in seedling inoculation assay using 184 isolates collected from rice growing regions of the lower region of the Yangtze River. With respect to panicle blast resistance, all ABLs reached a high resistance level to blast disease in tests conducted in three consecutive years with the inoculation of seven mixed conidial suspensions collected from different regions of Jiangsu province. In natural field nursery assays, the ABLs showed significantly higher resistance than the recurrent parents. No common change on importantly morphological traits and yield-associated components was found among the ABLs, demonstrating the introduction of Pigm had no tightly linked undesirable effect on rice economically important traits and its associated grain weight reduction effect could be probably offset by others grain weight genes or at least in the background of the aforementioned two varieties. Notably, one rice line with Pigm, designated as Yangnonggeng 3091, had been authorized as a new variety in Jiangsu province in 2021, showing excellent performance on both grain yield and quality, as well as the blast resistance. Together, these results suggest that the Pigm gene has a high breeding value in developing rice varieties with durable and broad-spectrum resistance to blast disease.
Introduction
Rice blast, caused by the fungus Magnaporthe oryzae (M. oryzae), is one of the most devastating diseases of rice worldwide (Dean et al., 2012). The yield loss caused by rice blast was 10–30% in epidemic years and reached 40–50% or even higher in serious cases (Skamnioti and Gurr, 2009; Li et al., 2019). For example, it brought approximately 566 thousand tons of yield loss in 2015 alone in China (Liu et al., 2016a). Developing resistant cultivars by marker-assisted selection (MAS) of resistance genes has been proven to be the most effective, economical and eco-friendly strategy to control blast disease (Xiao et al., 2020).
To date, more than 350 quantitative resistance loci (QRLs) and 100 qualitative resistance (R) genes conferring resistance to M. oryzae have been identified, of which at least 32 R genes and five QRL genes have been cloned (Li et al., 2017; Yin et al., 2021). Comparatively, QRL genes, like Pi21 and bsr-d1, generally have broad-spectrum and durable resistance because they mostly do not belong to ‘gene-for-gene’ resistance and do not exert strong selection pressure on pathogens. Through genome editing, Tao et al. (2021) and Zhou et al. (2021a) obtained some lines that contained either of the two genes, Pi21 and bsr-d1, or both. After inoculation, they found that the lines containing both QRL genes showed apparently stronger resistance than the lines possessing one of them, implying the pyramiding of QRL genes is a feasible approach to develop a broad-spectrum resistance variety. However, due to relatively small effects of QRL genes on resistance, especially on panicle resistance, as well as limited number of QRL genes isolated so far, deployment of R genes with broad-spectrum resistance is of particular importance in current rice breeding projects. To date, only a few R genes were considered conferring broad-spectrum resistance, such as Piz, Piz-t, Pi1, Pi2, Pi9, Pi33, Pi54, Pigm, and Pi40 (Li et al., 2020; Xiao et al., 2020). Among them, Pigm, isolated from a Chinese local variety Gumei 4 with broad-spectrum and durable resistance to blast, exhibited resistance to 50 isolates of M. oryzae from all over the world (Deng et al., 2006, 2017). It is particularly interesting since it contains a pair of antagonistic genes, PigmR and PigmS. PigmR gives rice broad-spectrum resistance, but it reduces rice 1000-seed weight and affects grain yield. PigmS, in contrast, offsets the adverse effect of PigmR on yield by improving the seed setting. More importantly, PigmS inhibits PigmR-mediated broad-spectrum disease resistance by competing with PigmR to form a heterodimer, which alleviates the selection pressure of the pathogenic evolution of M. oryzae races and so renders PigmR durable resistance (Deng et al., 2017), implying its importance in rice breeding program.
Leaf blast and panicle blast are two major types of rice blast diseases, and panicle blast is an important cause for yield loss because it occurs on the panicle neck, directly restricting grain filling (Titone et al., 2015). Resistance to panicle blast and leaf blast at the seedling stage is often inconsistent, which means that the resistance of some R genes is not effective across the development stages (Puri et al., 2009; Ishihara et al., 2014; Liu et al., 2016b). Therefore, it is particularly important to employ broad-spectrum R genes independent of the development stage in rice molecular breeding. However, most studies have focused on seedling blast resistance, rather than panicle blast resistance, because the panicle inoculation generally needs a large space and is time-consuming and easily affected by the environment compared to spraying inoculation at the seedling stage (Hao et al., 2011; Xiao et al., 2020). Most of the broad-spectrum R genes mentioned before have not been evaluated for panicle blast resistance. Recently, a set of near-isogenic lines carrying six resistance alleles (Piz, Piz-t, Pi2, Pi9, Pi40, and Pigm) at the Piz locus, respectively, under the background of xian/indica rice variety Yangdao 6 and geng/japonica rice line 07GY31, were developed by Wu et al. (2016, 2017). After inoculation with M. oryzae isolates from different ecological regions of China, they found that Pigm showed more broad-spectrum resistance to both seedling blast and panicle blast than the other R genes (Wu et al., 2016, 2017).
Geng rice is an important food source in China, and more than 85% of rice cultivation areas grow geng rice in Jiangsu province (Zhang et al., 2011). Recently, the occurrence of rice blast, especially for panicle blast, has presented an increasingly serious threat on rice production in Jiangsu province. For instance, around 70% of rice fields were destroyed by panicle blast in 2014, resulting in huge yield losses (Wei and Song, 2014; He et al., 2015). Studies have shown that some R genes, like Pib, Pita, Pish, Pik-h, Piz, and Pi54, have been more widely used in geng rice varieties authorized in Jiangsu province (Fan et al., 2014; Wu et al., 2015; Wang et al., 2020), while with the genetic variation of M. oryzae strains, these R genes are gradually losing their resistance (Zhu et al., 2018; Wang et al., 2020). For the R gene Pigm, however, to the best of our knowledge, almost no geng varieties currently released in Jiangsu province contain this gene. Recently, Zeng et al. (2018) introduced Pigm into three different geng rice varieties through MAS and found that the BC4F1 plants with heterozygous Pigm alleles showed strong panicle blast resistance against M. oryzae races from southern China. In addition, Wang et al. (2019) and Chen et al. (2020) employed two different geng rice varieties from Jiangsu province to cross or backcross with Gumei 4 and found that the plants carrying Pigm gene exhibited at least moderate resistance to panicle blast. These studies suggest that Pigm has a breeding potential in improving blast resistance of geng rice in Jiangsu province. However, due to the fact that the Pigm locus is from a landrace Gumei 4 and the adverse effect of PigmR on 1,000-seed weight reported by Deng et al. (2017), the breeding value of the Pigm locus in geng rice breeding project in Jiangsu province remains to be extensively evaluated.
In this study, we introduced Pigm gene into two high-yield and good-quality geng rice cultivars in Jiangsu by MAS and obtained a series of advanced backcrossing lines (ABLs) with Pigm gene and excellent performance in field. Furthermore, we comprehensively evaluated the resistance of ABLs to both seedling and panicle blast diseases using 184 M. oryzae isolates collected from multiple places in the lower region of the Yangtze River, especially in Jiangsu province. In addition, no tightly linked undesirable effects on important economical and agronomical traits were found in the introduction of Pigm. Particularly, one of the advanced lines has been authorized as a new variety in Jiangsu province, which showed excellent blast resistance as well as high yield and good quality. Together, our data infer that Pigm gene has a high breeding value in the geng rice breeding project toward broad-spectrum and durable blast resistance, and the resistant ABLs generated in this study will be of high value in breeding practice in future.
Results
Development of Advanced Backcross Lines With Pigm by Marker-Assisted Selection
To determine the breeding potential of Pigm gene utilized in the geng rice breeding program in Jiangsu province, we introduced Pigm into two well-known geng rice cultivars WYG32 and HG8 by MAS, respectively (Figure 1). In BC4F5 generations, we obtained 13 and 16 ABLs, which contained homozygous Pigm gene and shared similar agronomic traits with the recurrent parents WYG32 and HG8, respectively. These lines were named WYG32-PG-1 to WYG32-PG-13 and HG8-PG-1 to HG8-PG-16 (Supplementary Table 1).
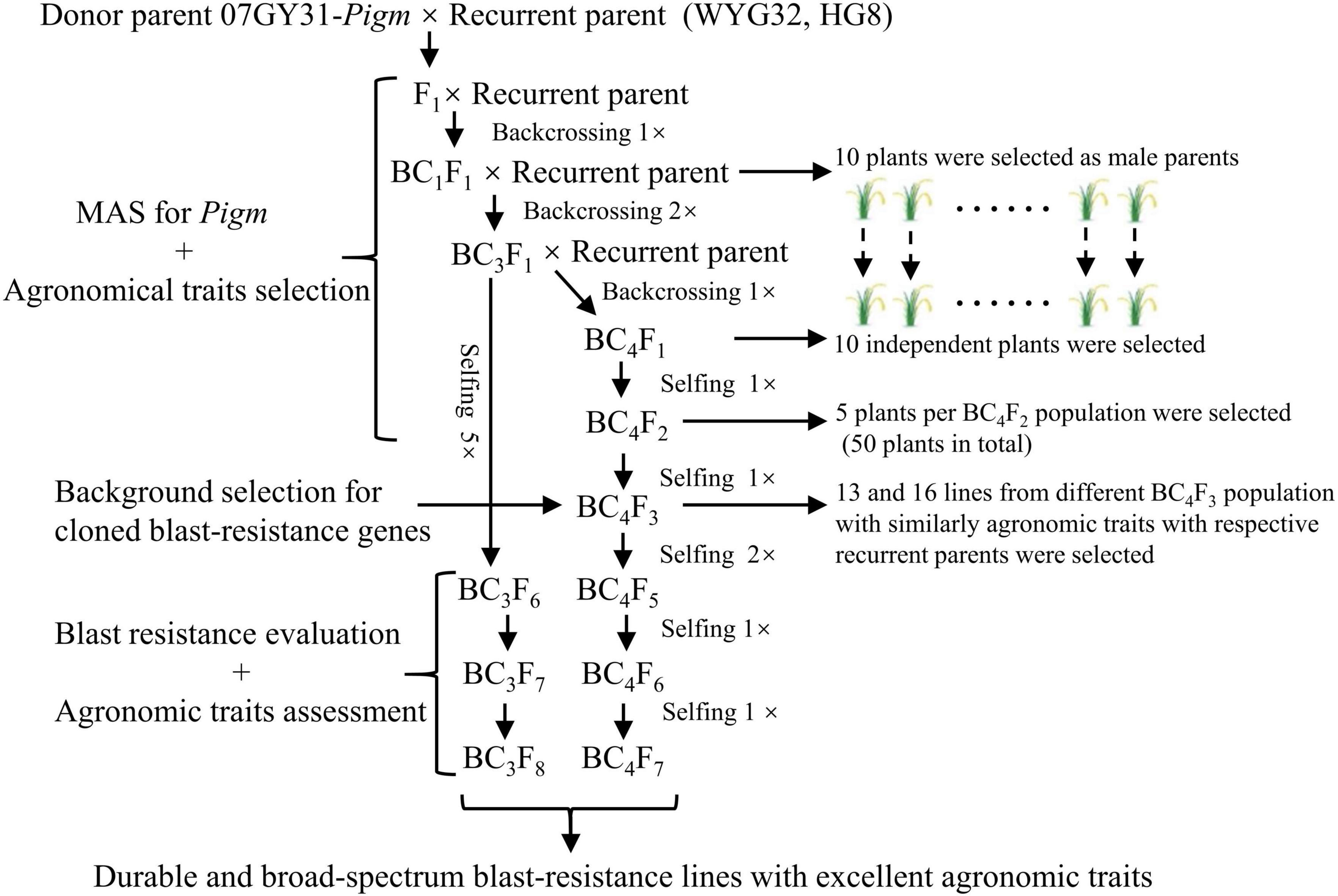
Figure 1. Marker-assisted selection (MAS) scheme for transferring Pigm and developing durable and broad-spectrum blast-resistant lines. WYG32, Wuyungeng 32; HG8, Huageng 8.
In order to exclude the influence of other rice blast R genes, we further detected the genotypes of these ABLs in other 13 known R gene loci (Pia, Pib, Pid2, Pid3, Pik, Pikm, Pikh, Pit, Pita, Pi1, Pi5, Pi25, and Pb1) using functional markers. We found that 11 of the 13 and 12 of the 16 ABLs carried same R gene combination as the recurrent parents WYG32 and HG8, respectively; and among them, five ABLs were selected from each background for subsequently resistant evaluation and agronomic trait assessment (Supplementary Table 1).
Advanced Backcross Lines With Pigm Showed More Broad-Spectrum Resistance at the Seedling Stage to M. oryzae Strains Collected From the Lower Region of the Yangtze River
To evaluate the resistance effect of Pigm gene, a total of 144 M. oryzae isolates were used in inoculation assay. Among them, 84 strains were collected from infected fields in almost all prefectures of Jiangsu province, and the remaining isolates were from major rice growing provinces in the lower region of the Yangtze River (Figure 2A). The results showed that the susceptible variety LTH was susceptible to all 144 isolates, with a resistance frequency of 0 (Figure 2B), indicating that our inoculation assays were successful. The resistance frequencies of the recurrent parents WYG32 and HG8 were 71.53 and 75.46%, respectively, while the ABLs were found to be resistant to almost all isolates, with resistance frequencies ranging from 98.61 to 100% (Figure 2B). This indicates the Pigm has broad-spectrum resistance to M. oryzae isolates from the lower region of the Yangtze River.
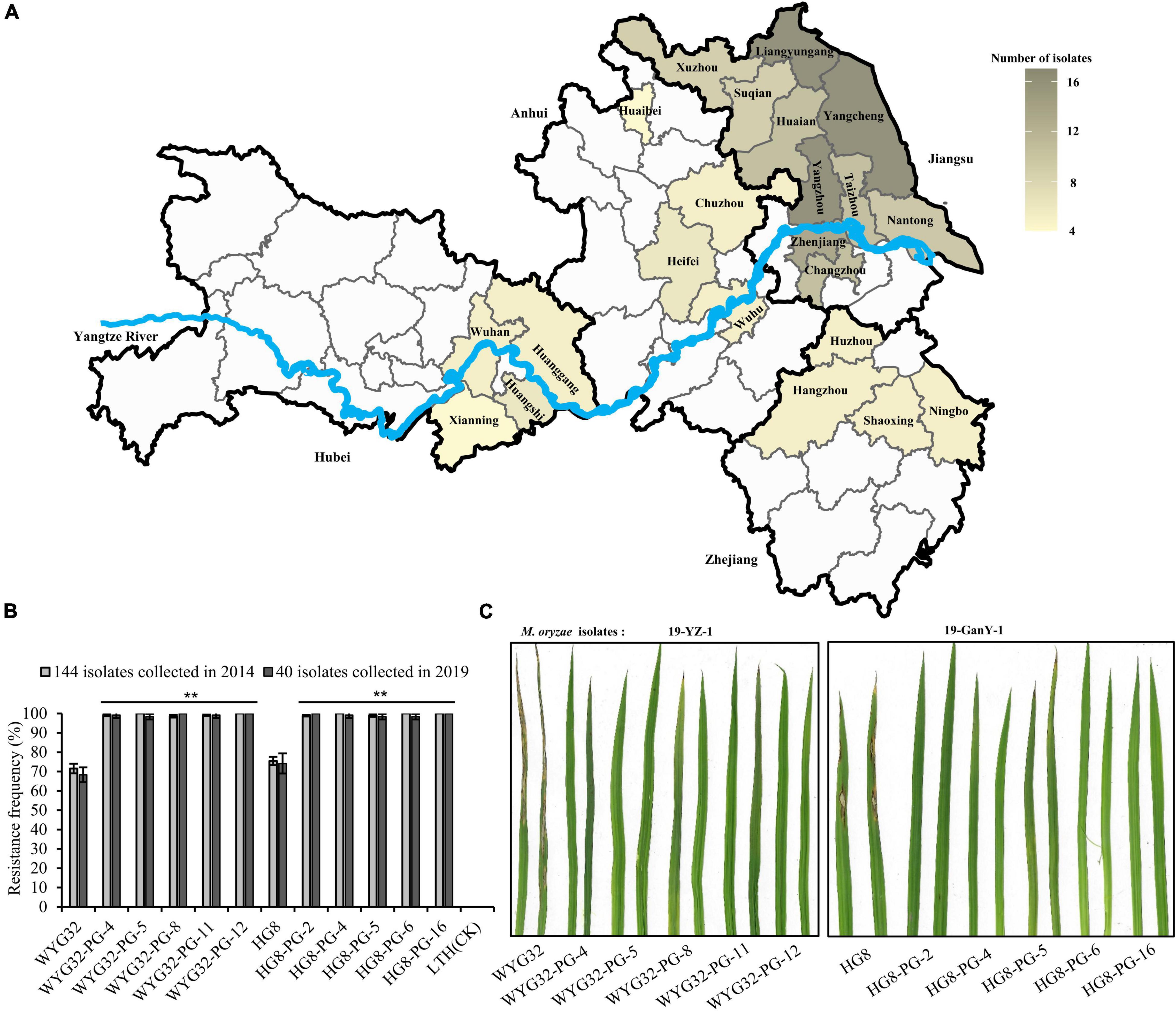
Figure 2. Blast resistance scores of ABLs and the recurrent parents at the seedling stage. (A) Distribution of 184 M. oryzae isolates used for seedling blast inoculation in the lower region of the Yangtze River. (B) Comparison of resistance frequencies among ABLs and the recurrent parents (WYG32 and HG8) against M. oryzae isolates. (C) Seedling blast disease reactions of ABLs against two M. oryzae isolates, 19-YZ-1 and 19-GanY-1. **P < 0.01 compared with the recurrent parent by Student’s t-test.
To further determine the potential of Pigm in the current rice breeding program in Jiangsu province, we collected 85 isolates in 2019 from the locations that have been previously collected. Through functional markers of the 10 known avirulence gene loci (Avr1-Co39, Avri-Pita, ACE1, Avr-Pib, PWL1, Avr-Pia, Avr-Pi9, Avr-Pizt, Avr-Pik, and Avr-Pii), we found that these isolates had significant variation at the 10 known avirulence gene loci (Supplementary Table 2). Based on the phylogenetic results from genotyping data at these avirulence gene loci, we classified these 85 isolates into four groups (RA, RB, RC, and RD) (Supplementary Table 3). After combining the location information, we found that each of the four groups contained the isolates from all different ecological regions, implying that the genetic variation of these isolates was not caused by the ecological regions. Then, in each group, 10 isolates from different locations were selected and used in seedling blast inoculation assay. We found that all ABLs showed high resistance to these isolates with resistant spectra from 98.33 to 100%, which were significantly higher than those of the corresponding recurrent parent and the susceptible control LTH which showed a resistant spectrum of 0% (Figures 2B,C). Taken together, these data demonstrate that introducing Pigm into geng rice varieties in Jiangsu province is able to greatly broaden their resistance spectrum to blast disease, indicating a great potential of Pigm in the rice breeding program, especially in Jiangsu province.
Advanced Backcross Lines With Pigm Exhibited High Resistance Against Panicle Blast
To further assess the panicle blast resistance of these ABLs, a total of seven mixed conidial suspensions of the isolates collected from Jiangsu were used to inoculate ABLs at the booting stage. The mixed conidial suspensions of M2018, M2019, and M2020-1 were provided by Professor Yongfeng Liu, which were used in the test region of Jiangsu rice varieties for identifying the resistant level of each candidate rice variety in 2018, 2019, and 2020, respectively; four other mixed suspensions (M2020-2, M2020-3, M2020-4, and M2020-5) were prepared in the present study, each comprising six isolates that were selected from each of the four groups (RA, RB, RC, and RD) based on genotypes and location distribution (Figure 3 and Supplementary Table 3). In these seven trials, the disease grades of the control WYG32 were 9 in four trials (M2018, M2020-3, M2020-4, and M2020-5) and 7.4 ∼ 8.6 in the other three trials (M2019, M2020-1, and M2020-2), showing high susceptibility to panicle blast. By contrast, all five ABLs in this background reached a high resistance level to panicle blast, with the disease grade ranging from 0 to 0.8. For another background, the disease grades of HG8 were in the range of 3 ∼ 5 in all seven trials, exhibiting moderate resistance to panicle blast. However, all five ABLs under the HG8 background displayed high resistance to panicle blast, with the disease grade ranging from 0 to 0.6. Taken together, these results indicated that transferring Pigm into geng rice varieties in Jiangsu province could greatly improve rice resistance to panicle blast.
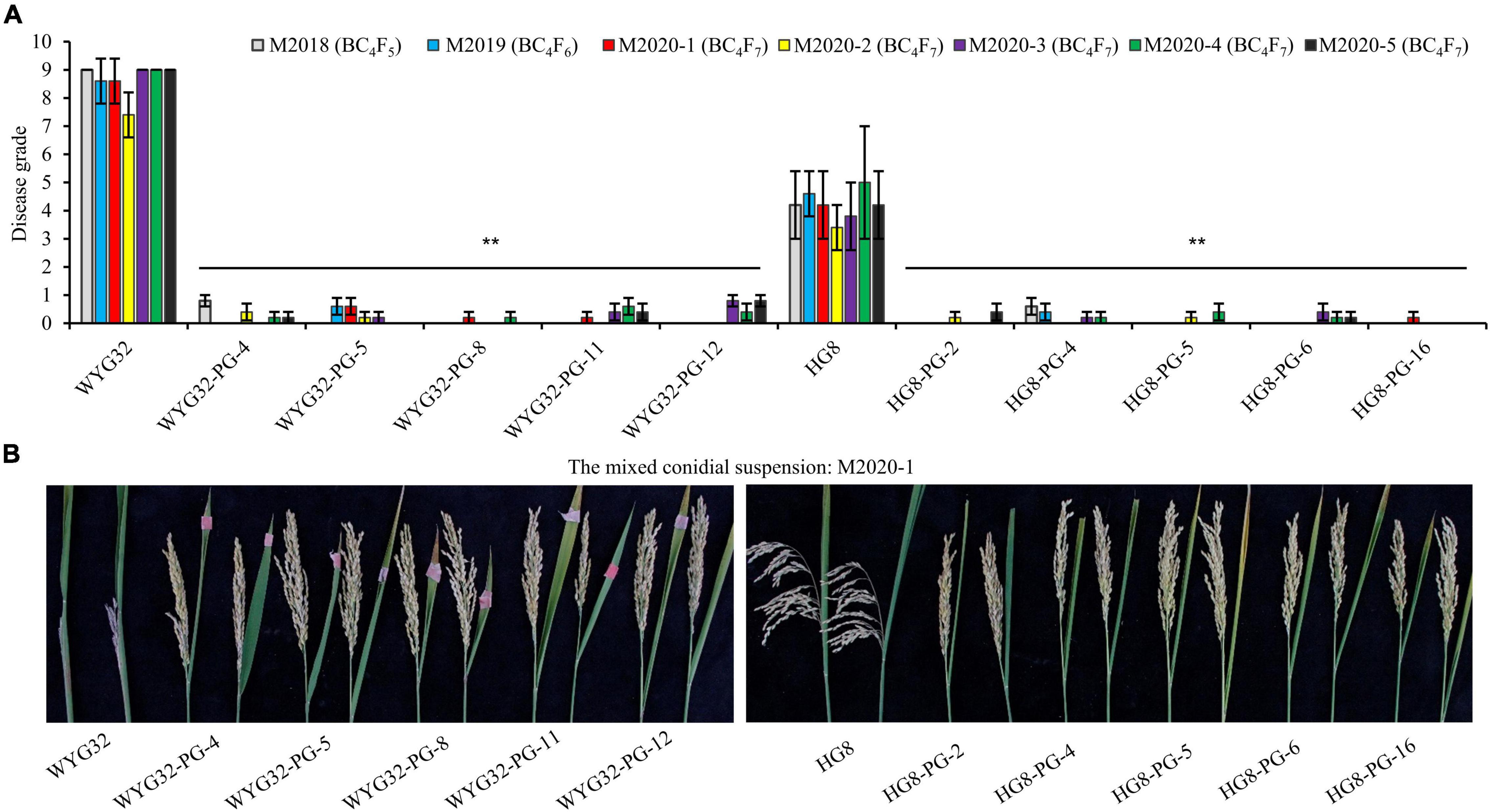
Figure 3. Panicle blast resistance scores of ABLs and the recurrent parents at the ripening stage. (A) Comparison of disease grades among ABLs and the recurrent parents (WYG32 and HG8) against the mixed conidial suspensions of M. oryzae isolates. (B) Panicle blast disease reactions of ABLs against the mixed conidial suspension M2020-1. **P < 0.01 compared with the recurrent parent by Student’s t-test.
Advanced Backcross Lines With Pigm Displayed High Blast Resistance in Blast Nursery Field
To evaluate the resistance effect of ABLs under natural conditions with high blast disease pressure, field assays were performed in Ganyu, Jiangsu province, in 2018–2019. As shown in Figure 4, the recurrent parent WYG32 showed moderate susceptibility in 2018 and high susceptibility in 2019 with diseased panicle rates of 17.7 and 92.3%, respectively, indicating the severity of this region on blast disease. However, as expected, the diseased panicle rates of all five ABLs under WYG32 background was 0% in both years, reaching a high resistant level. For another background, HG8 showed moderately resistant levels in 2 years, with diseased panicle rates of 7.8% in 2018 and 9.8% in 2019, whereas all the ABLs in this background showed high resistance in 2 years, except the line HG8-PG-5 which reached a resistant level of 1.1% in 2019. The results in the blast nursery further demonstrated that transferring Pigm into geng rice varieties in Jiangsu province could apparently enhance rice resistance to blast.
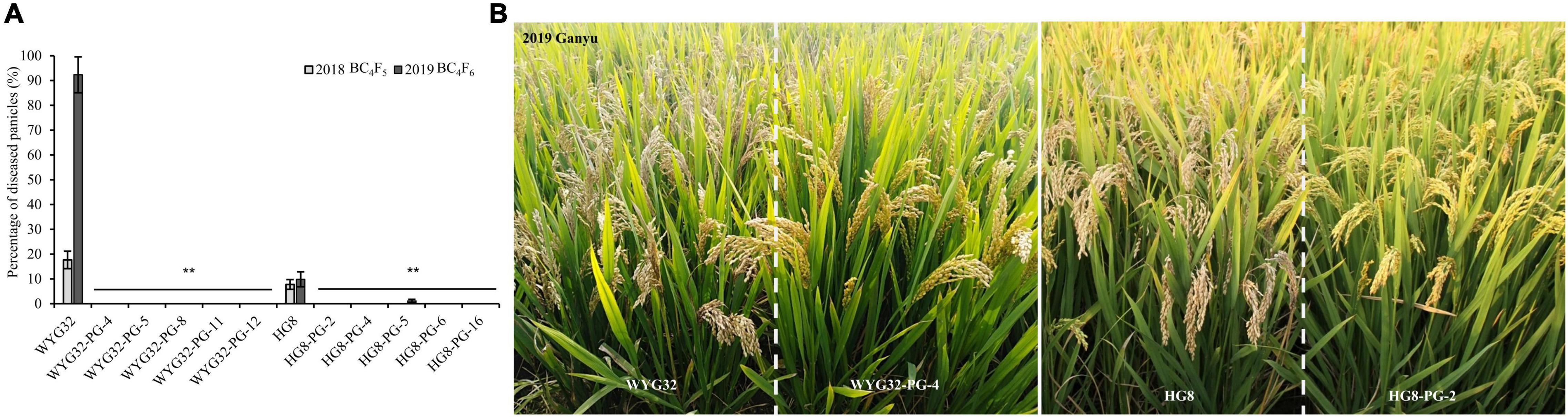
Figure 4. Resistance levels of ABLs to panicle blast in a blast nursery. (A) Comparison of diseased panicle rates among ABLs and the recurrent parents (WYG32 and HG8). (B) Panicle blast disease reactions of partial ABLs in the field in 2019. **P < 0.01 compared with the recurrent parent by Student’s t-test.
No Apparently Adverse Effects Were Detected Among Advanced Backcross Lines With Pigm Compared With the Recurrent Parent
To check whether the introduction of Pigm could affect agronomic traits and grain quality, we measured these traits for ABLs and the recurrent parents. As listed in Table 1, we found that in total, most ABLs displayed similar traits as the corresponding recurrent parent. A few traits were found to have significant differences between some lines and the control, whereas these were not common among different ABLs. For instance, ABLs WYG32-PG-4, WYG32-PG-8, WYG32-PG-11, and HG8-PG-6 showed higher plant height than the corresponding recurrent parents, whereas the other ABLs displayed a plant height similar to that of the controls, indicating these changed traits were not closely linked with Pigm. Together, these data allowed us to infer that the introduction of Pigm has no tightly linked adverse effects on rice agronomical traits and grain quality, at least for the varieties tested in our study.
The Pigm was reported previously to reduce grain weight but increase the seed setting ratio and ultimately balance grain yield and immunity (Deng et al., 2017). In the present study, however, we did not observe significant reduction on the grain weight of these ABLs. For these inconsistent results, we hypothesis that it could be due to various genes controlling grain weight distributed in these ABLs and Nipponbare, which were tested in a previous study. Therefore, we compared the genotypes of eight major genes controlling grain weight/shape (TGW6, TGW3, GW2, GW5, GW8, qGL3, GS3, and qLGY3) among ABLs Gumei 4 and Nipponbare by using functional markers (Gong et al., 2021). We found that all ABLs carried TGW6, TGW3, GW2, and GS3, whereas Gumei 4 and Nipponbare contained GW2, GS3, and qGL3 (Supplementary Figure 1). Comparatively, TGW6 and TGW3 were specifically harbored in all ABLs, suggesting that one of them or both may offset the negative effect of Pigm on grain weight, which is of interest for further verification.
A New Rice Variety Yangnonggeng 3091 Was Authorized With Strong Blast Resistance, High Grain Yield, and Good Quality
In addition to the MAS selection of Pigm, we also conducted agronomic trait selection and evaluation, which allowed us to obtain a new rice line in BC3F6 generation under HG8 background, designated as Yangnonggeng 3091(YNG3091, Figure 5A). In terms of panicle blast resistance, YNG3091 exhibited high resistance (disease grade, 0 ∼ 0.4) to all five mixed conidial suspensions (2020-1, 2020-2, 2020-3, 2020-4, and 2020-5), whereas the well-known control variety Huaidao 5 (HD5) in the rice region test of Jiangsu province showed moderately susceptible levels (disease grade, 5.4 ∼ 7) (Figure 5B). Moreover, YNG3091 recorded high resistance levels in natural blast disease nurseries in nine different cities of Jiangsu province (Figure 5B). For grain yield, we found that YNG3091 increased by 4.4% per 667 m2 compared with HD5 (Figure 5C). In addition, YNG3091 showed a slightly higher plant height than HD5 but had a similar total growth period. With respect to grain quality, we found that the chalkiness rate and chalkiness degree of YNG3091 were significantly lower than those of HD5, and the other traits were similar to those of HD5 (Figure 5D). Due to good performance on grain appearance, YNG3091 was evaluated as grade II of good quality rice according to the rice quality standard of China’s agricultural industry (MARAPRC, 2002). In 2021, YNG3091 was authorized as a new rice variety (Approval No.: Sushengdao 20210046) in Jiangsu province (Figure 5). This further confirmed that Pigm gene has great potential in developing new rice varieties with high blast resistance.
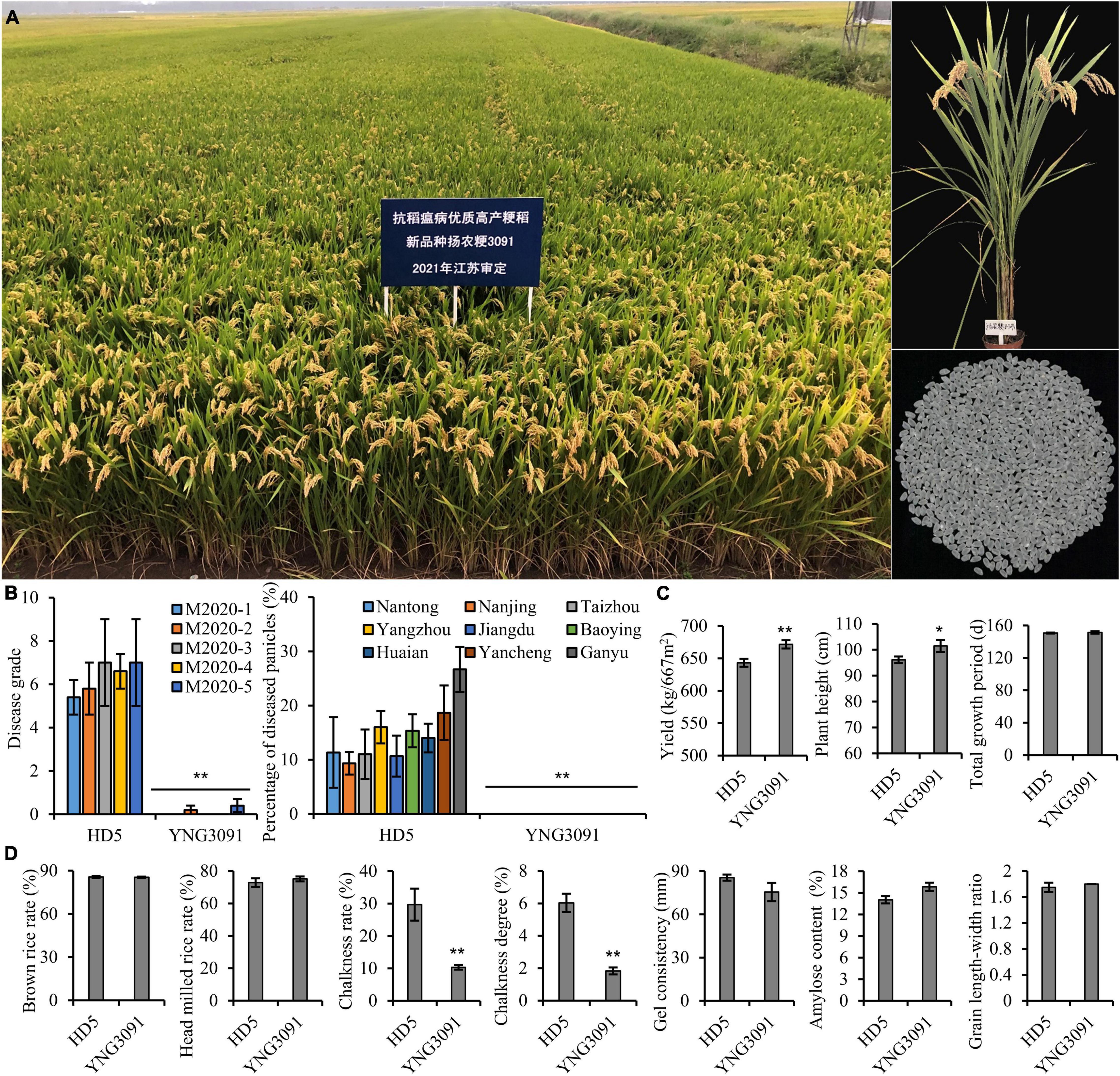
Figure 5. Breeding high blast-resistant rice variety Yangnonggeng 3091 (YNG 3091) with high yield and quality. (A) Field performance and milled rice grains of YNG3091. (B–D) Comparison of blast resistance levels (B), important agronomic traits (C), and grain quality traits (D) between YNG3091 and the control cultivar Huaidao 5 (HD5). *P < 0.05, **P < 0.01 compared with HD5 by Student’s t-test.
Discussion
Pigm Conferred Durable and Broad-Spectrum Resistance to Both Seedling and Panicle Blast
Deployment of broad-spectrum and durable resistance genes is of great importance to develop a broad-spectrum resistance variety. In this study, we developed several ABLs containing Pigm gene in the genetic background of two different geng rice varieties from Jiangsu province by MAS (Figure 1). Wang et al. (2020) found that in addition to the Pigm locus, 13 known R genes also distributed in geng rice varieties of Jiangsu province with various distribution degrees. A few R genes and R gene combinations showed apparently different contributions on rice blast resistance. Therefore, in order to reduce the effects from other R genes on the evaluation of Pigm resistance, we selected five ABLs in each background that showed same genotypes with the corresponding recurrent parent in the other 13 known R gene loci (Supplementary Table 1). After inoculation with 184 M. oryzae isolates in the seedling stage, we found that all ABLs showed significantly higher broad-spectrum resistance than the corresponding recurrent parent. These 184 isolates were from different locations and years in the lower region of the Yangtze River, especially in Jiangsu province, displayed a wide regional representative (Figure 2). It is expected that after years of planting different varieties, the major epidemic isolates in field might be changed. For further testing the resistance effect of Pigm on recent M. oryzae isolates, 40 of the 184 isolates were collected in 2019 from the locations that were previously collected in 2014, which showed an apparently genetic diversity based on the genotype analysis of known avirulence gene loci (Supplementary Tables 2,3). After combining the other studies using different isolates from different years and at least three different rice growing ecological regions (Tian et al., 2016; Dai et al., 2018; Zhou et al., 2021b), we infer that Pigm gene confers durable and broad-spectrum resistance to rice blast.
In terms of breeding practice, it is of great significance to regularly collect latest epidemic isolates to evaluate the resistance of rice lines to rice blast, especially panicle blast. The laboratory of Professor Yongfeng Liu (Plant Protection Institute of Jiangsu Academy of Agricultural Sciences) has been used to perform the evaluation experiment of blast resistance for the rice lines collected from the test region of new rice varieties in Jiangsu province. Therefore, they have to isolate a large number of M. oryzae isolates from all over the province each year, and through the pathogenicity test, six of the isolates that showed the strongest pathogenicity and genetic diversity were used for panicle inoculation next year (private communication with Prof. Liu). The mixed conidial suspensions M2018, M2019, and M2020-1 were from Liu’s laboratory, and we found that the ABLs showed a high resistance level to all these conidial suspensions in panicle blast inoculation assay. Similarly, in the inoculation assay using the other four mixed conidial suspensions, the ABLs reached high resistance levels (Figure 3 and Supplementary Tables 2,3). Meanwhile, the results of the natural evaluation in the blast nursery in Ganyu in 2 consecutive years (2018–2019) and nine different cities in 2020 showed a consistent trend with artificial inoculation (Figures 4, 5B). These results indicate that Pigm gene has strong effects in improving rice panicle blast resistance, at least for geng rice varieties in Jiangsu province. Collectively, although the fact that durable resistance of Pigm is still required to be monitored after a number of rice varieties with Pigm gene widely planted, the Pigm gene could be considered conferring durable resistance because it displayed very broad-spectrum resistance to both seedling and panicle blast.
Effect of Pigm Gene on Reduction of Rice Grain Weight Can Be Probably Eliminated by Combination With Other Excellent Grain Weight-/Shape-Related Alleles
Linkage between R genes and deleterious alleles (linkage drag) is one of the major reasons limiting their utilization in breeding practice. Many broad-spectrum R genes, such as Pi1, Pi9, Pi33, Pi40, Pi56, and Pigm, are mainly distributed in local special germplasms but not modern cultivars, and their cumbersome chain of linkage drag might produce negative effects on agronomic traits (Xiao et al., 2020). The Pigm gene was reported to achieve yield balance by reducing grain weight but increasing the seed setting (Deng et al., 2017), while a decrease in grain weight is generally an undesirable trait in breeding practice. In the present study, we obtained five ABLs in each of the two recurrent parents. Notably, when compared with the corresponding recurrent parent, we found that all ABLs displayed almost the same 1,000-grain weight with the control, except for HG8-PG-4 which showed a significant lower 1,000-grain weight than HG8 (Table 1). Grain weight is typical of a quantitative trait controlled by multiple genes, and so far, at least 44 genes have been confirmed to be involved in the regulation of grain weight in rice (Li et al., 2018). Specially, wide interactions among different grain weight-related genes and complex affection of hormone signaling on grain weight have been found (Zuo and Li, 2014), which will undoubtedly affect the expression of a minor effect gene in complex genetic background. In the breeding process for developing these ABLs, the plants without good performance on agronomic traits, including grain size, were in fact not selected. Based on this, we further found that compared with Gumei 4 and Nipponbare, ABLs specifically carried TGW6 and TGW3 genes among eight major genes controlling the grain weight/shape (Supplementary Figure 1), suggesting that probably one of them or both may counteract the negative effect of PigmR on grain weight in ABLs, which is worthy of further investigation. In addition, due to the fact that the backcross and self-pollinated populations in each generation do not contain a large number of plants (Figure 1), we inferred that a weak reduction of PigmR on grain weight could be easily eliminated by agronomical trait selection or at least in the varieties’ background in this study.
Notably, a rice line YNG3091 from BC3F6 generation has been authorized as a new rice variety in Jiangsu province, which displays good resistance to both seedling blast and panicle blast, as well as excellent performance on both grain yield and quality (Figure 5). Most recently, a new geng variety Jinxiangyu 1 carrying Pigm was also developed via MAS in Jiangsu province, which also showed excellent blast resistance and high yield (Xiao et al., 2021). Together, these demonstrate that Pigm has a high breeding value in developing a blast-resistant variety and the reduction effect of PigmR on grain weight could be overcome by agronomical trait selection. In addition, this study provides several new rice lines including the variety YNG3091 carried Pigm, which can be directly used as new donors in the rice breeding project and ultimately speed up the development of blast resistant varieties in Jiangsu province and its surrounded region.
Materials and Methods
Plant Materials and Pathogens
The geng rice line 07GY31-Pigm, which carries Pigm from Gumei 4 and exhibits broad-spectrum resistance against rice blast (Wu et al., 2017), was used as the donor of Pigm, and two geng rice cultivars, Wuyungeng 32 (WYG32) and Huageng 8 (HG8), were authorized by Jiangsu province, which showed high yield and good grain quality, and selected as recurrent parents to develop blast-resistant materials/varieties with high breeding potential.
Totally, 144 and 85 M. oryzae isolates were collected and obtained from infected fields of 22 cities in the lower region of the Yangtze River (including Jiangsu, Anhui, Hubei, and Zhejiang provinces) in 2014 (Wu et al., 2017) and 13 different cities/counties in Jiangsu province in 2019, including Jingtan (JT), Wujin (WJ), Zhenjiang (ZJ), Yangzhou (YZ), Baoying (BY), Jiangdu (JD), Gaoyou (GY), Taizhou (TZ), Huaian (HA), Siyang (SiY), Ganyu (GanY), Sheyang (SheY), and Yancheng (YC). The isolation and cultivation of single-spore strains and inoculum preparation were carried out as described previously (Puri et al., 2009).
Molecular Marker Assay
Genomic DNA was extracted from 1-month-old rice leaves and the mycelium of M. oryzae strains using the CTAB method (Zhang et al., 1992). The tightly linked molecular marker ZJ58.7 (2013–2018) and the functional marker GMR-3 (2019–2020) were selected to monitor Pigm (Yu et al., 2013; Wang et al., 2019). The functional markers used to detect 13 cloned blast R genes (Pia, Pib, Pi-d2, Pi-d3, Pik, Pikm, Pik-h, Pit, Pi-ta, Pi1, Pi5, Pi25, and Pb1) in rice plants and 10 isolated avirulence genes (Avr1-Co39, Avri-Pita, ACE1, Avr-Pib, PWL1, Avr-Pia, Avr-Pi9, Avr-Pizt, Avr-Pik, and Avr-Pii) in M. oryzae strains referred to the primer sequences described by Shen et al. (2019) and Wang et al. (2020), respectively. The reaction mixture (20 μL) for PCR amplification consisted of 2 μL DNA template (about 40 ng μL–1), 1 μL of each primer (10 μmol L–1), 2 μL MgCl2 (25 mmol L–1), 0.4 μL dNTP (10 mmol L–1), 2 μL 10 × PCR buffer, 0.2 μL Taq polymerase enzyme (5 U μL–1), and 11.4 μL ddH2O. PCR amplification was carried out using the following protocol: a pre-denaturation for 5 min at 95°C, followed by 35 cycles of 30 s at 94°C, 30 s at the annealing temperature, 1min at 72°C, and a final extension 72°C for 10 min. The PCR products were separated by electrophoresis on 4% agarose gels with ethidium bromide and photographed using a gel imaging system (Tanon 2500).
Marker-Assisted Selection Procedure for Transferring Pigm and Developing New Lines
The MAS scheme for transferring Pigm into two genetic backgrounds is presented in Figure 1. Pigm was monitored in progenies from either backcrossed or self-pollinated plants using the molecular markers. The donor of Pigm (07GY31-Pigm) was crossed with each of the two recurrent parents (WYG32 and HG8) to obtain F1 plants in 2013. Then F1 plants of each cross were backcrossed with the corresponding recurrent parent till obtaining the 10 advanced independent BC4F1 plants. In 10 BC4F2 populations, five plants per BC4F2 population (50 plants in total) with a homozygous Pigm allele and good agronomic traits were screened for individually harvesting. In BC4F3 populations, a total of 13 and 16 lines with a similar agronomic phenotype with WYG32 and HG8 were selected, respectively. In each BC4F3 line, only one plant with excellent performance through visible selection was kept, and the same selection strategy was used in each BC4F4 line. Last, till BC4F5, the agronomic characters of each lines presented uniformity and were harvested together as a pure line. All BC4F5 homozygous lines were then examined for other 13 cloned blast R genes, Pia, Pib, Pi-d2, Pi-d3, Pik, Pikm, Pik-h, Pit, Pi-ta, Pi1, Pi5, Pi25, and Pb1, using functional markers. Finally, in each background, five BC4F5 ABLs with the same R gene combination on these 13 R gene loci as the recurrent parents were selected in 2018 for subsequent experiments (Supplementary Table 1). Meanwhile, in the BC3F6 generation, excellent lines carrying Pigm were screened to participate in the region test of rice new varieties in Jiangsu province.
Evaluation for Seedling Blast Resistance by Artificial Inoculation
The evaluation of seedling blast resistance by artificial inoculation was performed according to the method described by Wu et al. (2015) with slight modifications in BC4F6 generation in 2019 and BC4F7 generation in 2020. Totally, 12 plants of each line/cultivar were grown on a plastic tray (60 cm × 30 cm × 4 cm) in the greenhouse maintained at 26–32°C. The highly susceptible cultivar Lijangxintuanheigu (LTH) was used as the susceptible control. There were three replicate trays for each blast isolate. For the experiment, 3-week-old seedlings were placed in a glass box (70 cm × 45 cm × 33 cm) and were sprayed with 30 ml of an isolate conidial suspension using a vacuum pump (4–7.8 kg/cm2). The inoculated seedlings were cultured in the glass box maintained at 25°C in the dark for 24 h and then were transferred to a transparent plastic box, covered with fresh-keeping film to maintain humidity, and grown under 12-h light at 28°C/12-h darkness at 25°C for 7 days. Disease reactions were recorded 7 days post-inoculation in accordance with standard procedures (Mackill and Bonman, 1992). Resistance levels of tested materials were represented by resistance frequency, which was calculated as follows: number of incompatible isolates/total number of isolates inoculated × 100% (Wu et al., 2015).
Evaluation for Panicle Blast Resistance by Artificial Inoculation and Natural Induction
The artificial identification of panicle blast resistance in field was performed as described previously (Wu et al., 2017) using the mixed conidial suspension containing six representative M. oryzae isolates. A randomized complete block design was used with three biological replications. Each replication contained 10 rows and 10 plants per row, with a row spacing of 11.1 by 30 cm. At the booting stage, 10 panicles were injected with 1 mL of each mixed conidial suspension (5 × 104 conidia/mL). At the ripening stage, the panicle blast disease scores were evaluated according to a ‘0–9’ rating method (MARAPRC, 2014). According to disease scores, each rice line was then classified into differently resistant levels: highly resistant (0 ≤ disease grade < 1), resistant (1.0 ≤ disease grade < 3), moderately resistant (3.0 ≤ disease grade < 5.0), moderately susceptible (5.0 ≤ disease grade < 7.0), susceptible (7.0 ≤ disease grade < 9.0), and highly susceptible (disease grade = 9.0).
All tested materials were also subjected to the determination of blast resistance in blast nursery in the field of Ganyu county, Lianyungang city, Jiangsu, with high blast disease pressure in 2018–2019. A randomized complete block design was used with three biological replications. Each replication contained 10 rows and 10 plants per row, with a row spacing of 11.1 by 30 cm. The resistance level in the blast nursery was evaluated based on the diseased-panicle rate, which was calculated as the number of diseased panicles divided by the total number of panicles and multiplied by 100 (Ministry of Agriculture and Rural Affairs of the People’s Republic of China, 2014). According to the diseased panicle rates, the tested materials with a diseased panicle rate of 0% were considered highly resistant, 0.1–5% were resistant, 5.1–10% were moderately resistant, 10.1–25% were moderately susceptible, 25.1–50% were susceptible, and 50.1–100% were highly susceptible.
Examination of Importantly Agronomic and Grain Quality Traits
The ABLs were planted in the field at Yangzhou University, Jiangsu, China. The planting method was the same as described earlier (evaluation for panicle blast resistance). Overall, 10 plants between the second and ninth rows were randomly selected for measuring the importantly agronomic traits, which included plant height, days to heading, panicle number, panicle length, grain number per panicle, 1,000-seed weight, seed setting rate, and grain quality-associated traits, including chalkiness rate and amylose content according to the previous description (IRRI, 2002).
Data Analysis
Data of blast disease severity and agronomic traits were sorted and plotted by Excel 2016. Significant differences between ABLs and the recurrent parents were analyzed using Student’s t-test in the SPSS 19.0 program. The cluster analysis of 271 M. oryzae isolates was performed by using SPSS 19.0.
Data Availability Statement
The original contributions presented in this study are included in the article/Supplementary Material, further inquiries can be directed to the corresponding authors.
Author Contributions
SZ, ZC, and ZF designed the research. ZF, MYL, ZX, PG, YW, KW, JZ, XW, JW, MCL, and KH performed the experiments and analyzed the data. ZF wrote the manuscript. SZ, ZC, AL, YD, and HC revised the manuscript. All authors read and approved the manuscript.
Funding
This study was supported by the Key Studying and Developing Project of Jiangsu Province for Modern Agriculture (BE2019339), the Major Project of Jiangsu Province for Significant New Varieties Development (PZCZ201703), the Key Studying and Developing Project of Yangzhou City for Modern Agriculture (YZ2020031), the Natural Science Foundation of China (32000362) and of Jiangsu province (BK20200930), and a project funded by the Priority Academic Program Development of Jiangsu Higher Education Institutions (PAPD) and the Scientific Research Innovation Practice Project for postgraduate students of Jiangsu (KYCX20_2985), respectively.
Conflict of Interest
The authors declare that the research was conducted in the absence of any commercial or financial relationships that could be construed as a potential conflict of interest.
Publisher’s Note
All claims expressed in this article are solely those of the authors and do not necessarily represent those of their affiliated organizations, or those of the publisher, the editors and the reviewers. Any product that may be evaluated in this article, or claim that may be made by its manufacturer, is not guaranteed or endorsed by the publisher.
Acknowledgments
We are thankful to Yongfeng Liu (Plant Protection Institute of Jiangsu Academy of Agricultural Sciences, Jiangsu, China) for providing M. oryzae isolates.
Supplementary Material
The Supplementary Material for this article can be found online at: https://www.frontiersin.org/articles/10.3389/fpls.2022.937767/full#supplementary-material
Abbreviations
M. oryzae, Magnaporthe oryzae; R, resistance; MAS, marker-assisted selection; WYG32, Wuyungeng 32; HG8, Huageng 8; ABLs, advanced backcross lines with Pigm; QRLs, quantitative resistance loci; YNG3091, Yangnonggeng 3091; HD5, Huaidao 5.
References
Chen, T., Sun, X. C., Zhang, S. L., Liang, W. H., Zhou, L. H., Zhao, Q. Y., et al. (2020). Development and verification of specific molecular markers for Pigm gene associated with broad-spectrum resistance to rice blast. Chin. J. Rice Sci. 34, 28–36.
Dai, X. J., He, C., Zhou, L., Liang, M. Z., Fu, X. C., Qin, P., et al. (2018). Identification of a specific molecular marker for the rice blast-resistant gene Pigm and molecular breeding of thermo-sensitive genic male sterile leaf-color marker lines. Mol. Breed. 38:72. doi: 10.1007/s11032-018-0821-2
Dean, R., Van Kan, J. A., Pretorius, Z. A., Hammond-Kosack, K. E., Di Pietro, A., Spanu, P. D., et al. (2012). The top 10 fungal pathogens in molecular plant pathology. Mol. Plant Pathol. 13, 414–430. doi: 10.1111/j.1364-3703.2011.00783.x
Deng, Y. W., Zhai, K. R., Xie, Z., Yang, D. Y., Zhu, X. D., Liu, J. Z., et al. (2017). Epigenetic regulation of antagonistic receptors confers rice blast resistance with yield balance. Science 355, 962–965. doi: 10.1126/science.aai8898
Deng, Y. W., Zhu, X. D., Shen, Y., and He, Z. H. (2006). Genetic characterization and fine mapping of the blast resistance locus Pigm(t) tightly linked to Pi2 and Pi9 in a broad-spectrum resistant Chinese variety. Theor. Appl. Genet. 113, 705–713. doi: 10.1007/s00122-006-0338-7
Fan, F. J., Wang, F. Q., Liu, Y. F., Wang, J., Zhu, J. Y., Li, W. Q., et al. (2014). Evaluation of resistance to rice panicle blast with resistant genes Pi-b. Pi-ta, Pikm and Pi54. Acta. Agric. Boreali Occident Sin. 29, 221–226.
Gong, K., Xue, P., Weng, X. X., Liao, F. F., Sun, B., Peng, Z. Q., et al. (2021). Distribution of grain shape related genes in rice big grain Germplasm BG1 and elite restorer line Huazhan and development of relevant functional markers. Chin. J. Rice Sci. 35, 543–553.
Hao, Z. N., Wang, L. P., Liang, J. E., and Tao, R. X. (2011). Response of the panicles exserted from the caulis and from various effective tillers at four stages of panicle development to neck blast in rice. Eur. J. Plant Pathol. 131, 269–275. doi: 10.1007/s10658-011-9806-2
He, H. M., Wu, X. W., Shi, L., Tai, D. L., and Mei, A. Z. (2015). Characters and control countermeasures of rice panicle blast occurrence in Dongtai city in 2014. Mod. Agric. Sci. Technol. 15, 157–158. doi: 10.5958/0976-4615.2015.00001.0
IRRI (2002). Standard Evaluation System for Rice (SES), 4th Ed. Los Banos, Philippines: International Rice Research Institute (IRRI).
Ishihara, T., Hayano-Saito, Y., Oide, S., Ebana, K., La, N. T., Hayashi, K., et al. (2014). Quantitative trait locus analysis of resistance to panicle blast in the rice cultivar Miyazakimochi. Rice 7:2. doi: 10.1186/s12284-014-0002-9
Li, N., Xu, R., Duan, P. G., and Li, Y. H. (2018). Control of grain size in rice. Plant Reprod. 31, 237–251. doi: 10.1007/s00497-018-0333-6
Li, W., Deng, Y. W., Ning, Y. S., Hu, Z. H., and Wang, G. L. (2020). Exploiting broad-spectrum disease resistance in crops: from molecular dissection to breeding. Ann. Rev. Plant Biol. 71, 575–603. doi: 10.1146/annurev-arplant-010720-022215
Li, W. T., Chern, M. S., Yin, J. J., Wang, J., and Chen, X. W. (2019). Recent advances in broad-spectrum resistance to the rice blast disease. Curr. Opin. Plant Biol. 50, 114–120. doi: 10.1016/j.pbi.2019.03.015
Li, W. T., Zhu, Z. W., Chern, M., Yin, J. J., Yang, C., Ran, L., et al. (2017). A natural allele of a transcription factor in rice confers broad-spectrum blast resistance. Cell 170, 114–126. doi: 10.1016/j.cell.2017.06.008
Liu, Q., Yang, J. Y., Zhang, S. H., Zhao, J. L., Feng, A. Q., Yang, T. F., et al. (2016a). OsGF14b positively regulates panicle blast resistance but negatively regulates leaf blast resistance in rice. Mol. Plant Microbe Interact. 29, 46–56. doi: 10.1094/MPMI-03-15-0047-R
Liu, W. C., Liu, Z. D., Huang, C., Lu, M. H., Liu, J., and Yang, Q. B. (2016b). Statistics and analysis of crop yield losses caused by main diseases and insect pests in recent 10 years. Plant Prot. 42, 1–9.
Mackill, D., and Bonman, J. (1992). Inheritance of blast resistance in near-isogenic lines of rice. Phytopathology 82, 746–749. doi: 10.1094/Phyto-82-746
MARAPRC (2002). Cooking Rice Variety Quality. Beijing: Ministry of Agriculture and Rural Affairs of the People’s Republic of China (MARAPRC).
MARAPRC (2014). Technical Specification for Identification and Evaluation of Blast Resistance in Rice Variety Regional Test. Beijing: Ministry of Agriculture and Rural Affairs of the People’s Republic of China (MARAPRC).
Puri, K. D., Shrestha, S. M., Chhetri, G. B. K., and Joshi, K. D. (2009). Leaf and neck blast resistance reaction in tropical rice lines under greenhouse condition. Euphytica 165, 523–532. doi: 10.1007/s10681-008-9771-9
Shen, L. R., Qi, Z. Q., Du, Y., Yu, J. J., Yu, M. N., and Liu, Y. F. (2019). Pathogenicity differentiation and composition analysis of avirulence genes of Magnaporthe oryzae in Jiangsu province. Jiangsu J. Agric. Sci. 35, 42–47.
Skamnioti, P., and Gurr, S. J. (2009). Against the grain: safeguarding rice from rice blast disease. Trends Biotechnol. 27, 141–150. doi: 10.1016/j.tibtech.2008.12.002
Tao, H., Shi, X., He, F., Wang, D., Xiao, N., Fang, H., et al. (2021). Engineering broad-spectrum disease-resistant rice by editing multiple susceptibility genes. J. Integr. Plant Biol. 63, 1639–1648. doi: 10.1111/jipb.13145
Tian, H. G., Cheng, H. Q., Hu, J., Lei, C. L., Zhu, X. D., and Qian, Q. (2016). Effect of introgressed Pigm gene on rice blast resistance and yield traits of japonica rice in cold area. J. Shenyang Agric. Univ. 47, 520–526.
Titone, P., Mongiano, G., and Tamborini, L. (2015). Resistance to neck blast caused by Pyricularia oryzae in Italian rice cultivars. Eur. J. Plant Pathol. 142, 49–59. doi: 10.1007/s10658-014-0588-1
Wang, F. Q., Chen, Z. H., Xu, Y., Wang, J., Li, W. Q., Fan, F. J., et al. (2019). Development and application of the functional marker for the broad-spectrum blast resistance gene PigmR in rice. Sci. Agri. Sin. 52, 955–967.
Wang, X. Q., Du, H. B., Chen, X. J., Li, M. Y., Wang, J. N., Xu, Z. W., et al. (2020). Analysis of blast resistance genes and neck blast resistance of geng rice varieties/lines recently developed in Jiangsu province. Chin. J. Rice Sci. 34, 413–424.
Wei, F., and Song, Z. D. (2014). Characters and control countermeasures of rice panicle blast occurrence in Ganyu city in 2014. Mod. Agric. Sci. Technol. 24, 147–149.
Wu, Y. Y., Chen, Y., Pan, C. H., Xiao, N., Yu, L., Li, Y. H., et al. (2017). Development and evaluation of near-isogenic lines with different blast resistance alleles at the Piz locus in geng rice from the lower region of the Yangtze River. China Plant Dis. 101, 1283–1291. doi: 10.1094/PDIS-12-16-1855-RE
Wu, Y. Y., Xiao, N., Yu, L., Pan, C. H., Li, Y. H., Zhang, X. X., et al. (2015). Combination patterns of major R genes determine the level of resistance to the M. oryzae in rice (Oryza sativa L.). PLoS One 10:e0126130. doi: 10.1371/journal.pone.0126130
Wu, Y. Y., Yu, L., Pan, C. H., Dai, Z. Y., Li, Y. H., Xiao, N., et al. (2016). Development of near-isogenic lines with different alleles of Piz locus and analysis of their breeding effect under Yangdao 6 background. Mol. Breed. 36:12. doi: 10.1007/s11032-016-0433-7
Xiao, N., Pan, C. H., Li, Y. H., Wu, Y. Y., Cai, Y., Lu, Y., et al. (2021). Genomic insight into balancing high yield, good quality, and blast resistance of japonica rice. Genome Biol. 22:283. doi: 10.1186/s13059-021-02488-8
Xiao, N., Wu, Y. Y., and Li, A. H. (2020). Strategy for use of rice blast resistance genes in rice molecular breeding. Rice Sci. 27, 263–277. doi: 10.1016/j.rsci.2020.05.003
Yin, J., Zou, L., Zhu, X., Cao, Y., He, M., and Chen, X. (2021). Fighting the enemy: how rice survives the blast pathogen’s attack. Crop J. 9, 543–552. doi: 10.1016/j.cj.2021.03.009
Yu, M. M., Dai, Z. Y., Pan, C. H., Chen, X. J., Yu, L., Zhang, X. X., et al. (2013). Resistance spectrum difference between two broad-spectrum blast resistance genes. Pigm and Pi2, and their interaction effect on Pi1. Acta. Agron. Sin. 39, 1927–1934. doi: 10.3724/SP.J.1006.2013.01927
Zeng, S. Y., Li, C., Du, C. C., Sun, L. T., Jing, D. D., Lin, T. Z., et al. (2018). Development of specific markers for Pigm in marker-assisted breeding of panicle blast resistant geng rice. Chin. J. Rice Sci. 32, 453–461.
Zhang, Q. F., Saghai-Maroof, M. A., Lu, T. Y., and Shen, B. Z. (1992). Genetic diversity and differentiation of indica and geng rice detected by RFLP analysis. Theor. Appl. Genet. 83, 495–499. doi: 10.1007/BF00226539
Zhang, Z. J., Zhang, H. X., Yang, J. C., Song, Y. S., Zhao, B. H., Ji, H. J., et al. (2011). Changes of safe dates for full heading in geng rice over past 50 years in Jiangsu Province. Acta. Agron. Sin. 37, 146–151. doi: 10.3724/SP.J.1006.2011.00146
Zhou, Y. B., Xu, S. C., Jiang, N., Zhao, X. H., Bai, Z. N., Liu, J. L., et al. (2021a). Engineering of rice varieties with enhanced resistances to both blast and bacterial blight diseases via CRISPR/Cas9. Plant Biotech. J. 20, 876–885. doi: 10.1111/pbi.13766
Zhou, Z., Sun, L., Hu, W. B., Zhou, B., Tao, S. H., Zhang, S. H., et al. (2021b). Breeding high-grain quality and blast resistant rice variety using combination of traditional breeding and marker-assisted selection. Rice Sci. 28, 422–426. doi: 10.1016/j.rsci.2021.07.002
Zhu, Y. L., Fan, F. J., Xie, Y. L., Wu, Y. B., Qiao, Z. Y., and Zhang, J. D. (2018). Detection and evaluation of resistance genes of rice blast in late ripening middle geng strain in Jiangsu Province. Jiangsu Agric. Sci. 46, 106–109.
Keywords: rice, blast resistance, Pigm gene, marker-assisted selection, geng rice in Jiangsu
Citation: Feng Z, Li M, Xu Z, Gao P, Wu Y, Wu K, Zhao J, Wang X, Wang J, Li M, Hu K, Chen H, Deng Y, Li A, Chen Z and Zuo S (2022) Development of Rice Variety With Durable and Broad-Spectrum Resistance to Blast Disease Through Marker-Assisted Introduction of Pigm Gene. Front. Plant Sci. 13:937767. doi: 10.3389/fpls.2022.937767
Received: 06 May 2022; Accepted: 06 June 2022;
Published: 22 July 2022.
Edited by:
Zhengguang Zhang, Nanjing Agricultural University, ChinaReviewed by:
Wen-Ming Wang, Sichuan Agricultural University, ChinaYi Zhen Deng, South China Agricultural University, China
Copyright © 2022 Feng, Li, Xu, Gao, Wu, Wu, Zhao, Wang, Wang, Li, Hu, Chen, Deng, Li, Chen and Zuo. This is an open-access article distributed under the terms of the Creative Commons Attribution License (CC BY). The use, distribution or reproduction in other forums is permitted, provided the original author(s) and the copyright owner(s) are credited and that the original publication in this journal is cited, in accordance with accepted academic practice. No use, distribution or reproduction is permitted which does not comply with these terms.
*Correspondence: Zongxiang Chen, Y3p4QHl6dS5lZHUuY24=; Shimin Zuo, c216dW9AeXp1LmVkdS5jbg==
†These authors have contributed equally to this work