- 1Centre of Marine Sciences, University of Algarve, Faro, Portugal
- 2Laboratory of Antibiotics and Chemotherapeutics, Instituto de Biociências, Letras e Ciências Exatas (IBILCE), São Paulo State University, São José do Rio Preto, Brazil
- 3Department of Physical and Analytical Chemistry, Faculty of Experimental Sciences, University of Jaén, Jaén, Spain
- 4IHAP, Université de Toulouse, INRAE, ENVT, Toulouse, France
- 5Université de Toulouse, ENVT, Toulouse, France
The use of plants and their metabolites stands as a promising option to tackle parasitic infections by gastrointestinal nematodes (GIN) in integrated control strategies. Still, the influence of environmental and phenological factors, and their interactions, in the wild on the metabolomics and biological properties of target plant species, is often disregarded. In this work, we hypothesized that variations in the anthelmintic (AH) properties and chemical composition of extracts from the salt tolerant species Cladium mariscus L. Pohl (sawgrass) may be influenced by seasonal factors and organ-parts. To test this hypothesis, acetone/water extracts were prepared from dried biomass obtained from aerial organs collected from sawgrass in consecutive seasons and tested against Haemonchus contortus and Trichostrongylus colubriformis by the larval exsheathment inhibition assay (LEIA) and egg hatching inhibition assay (EHIA). To ascertain the role of plant organ, the activity of leaves and inflorescences extracts from summer samples was compared. The role of polyphenols in the anthelmintic activity depending on GINs and fluctuations across seasons and plant organs was assessed using polyvinylpolypyrrolidone (PVPP), coupled with an in-depth chemical profiling analysis using high-performance liquid chromatography completed with electrospray ionization mass spectrometric detection (HPLC-ESI-MSn). Main differences in anthelmintic activities were observed for summer and autumn samples, for both assays. Moreover, inflorescences’ extracts were significantly more active than those from leaves against both parasite species on EHIA and against H. contortus on LEIA. Application of PVPP totally inhibit the AH effects based on EHIA and only partly for LEIA. Non-treated PVPP extracts were predominantly composed of flavan-3-ols, proanthocyanidins, luteolin and glycosylated flavonoids, while two flavonoid glycosides were quantified in all PVPP-treated samples. Thus, the activity of such compounds should be further explored, although some unknown metabolites remain to be identified. This study reinforces the hypothesis of the AH potential of sawgrass and of its polyphenolic metabolites uses as nutraceutical and/or phytotherapeutic drugs.
Introduction
Parasitic infections by gastrointestinal nematodes (GIN) represent a serious economical and health threat to outdoor production systems of small ruminant worldwide, where Haemonchus contortus, Teladorsagia circumcincta, Trichostrongylus spp., and Nematodirus spp. are the most prevalent species (Charlier et al., 2018). As increasing anthelmintic resistances are reported, mainly due to the indiscriminate administration of available commercial synthetic drugs (Rose Vineer et al., 2020), it becomes peremptory to find novel GIN integrated control options. A promising suggested approach is the use of plants and their bioactive products, either as nutraceuticals, phytotherapeutic remedies, including essential oils as sources of secondary metabolites of veterinary interest to control parasites (Hoste et al., 2015).
Increasing evidence of in vitro and in vivo anthelmintic effects has been described for a range of botanical species and linked to a variety of bioactive metabolites, with particular emphasis on plants rich in polyphenolic compounds, specifically tannins (Hoste et al., 2006, 2015; Manolaraki, 2011; Spiegler et al., 2017; Santos et al., 2019; Liu et al., 2020). Such plants include both glycophytes, for example Legume forages (e.g., sainfoin: Onobrychis viciifolia Scop. and sulla: Hedysarum coronarium L.) and halophytes [e.g., mastic tree: Pistacia lentiscus L. and sawgrass: Cladium mariscus (L.) Pohl] (Oliveira et al., 2021c). Halophytes are high salt tolerant plants able to cope with several abiotic stressors, besides salinity, for example, high light intensity, high UV radiation, and drought/flood. That capacity is possible due several adaptation mechanisms, including the synthesis of high levels of antioxidant secondary molecules, such as polyphenols. Polyphenols are therefore produced as part of the plant defense machinery, and as such, the extent of its production is influenced by multiple complex abiotic and biotic interactions. Several studies on different models of bioactive plants rich in polyphenols have shown that the concentration of these compounds and, consequently, their pharmacological properties, rely to a range of factors either botanical (e.g., plant species, organs; plant cultivars and varieties, phenological stages), geographical and environmental (e.g., season, area of collection), as well as cultivation systems (Manolaraki, 2011). Similar factors have been described for halophytes wild plants. For example, high light intensity, UV irradiance, and saline stress enhanced the biosynthesis of antioxidant flavonoids in Ligustrum vulgare L., particularly quercetin and luteolin glycosides (Tattini et al., 2004; Agati et al., 2011). Variations on the chemical composition and bioactive properties of plant organs (e.g., leaves, stems, flowers) are described for different salt-tolerant species such as Eryngium maritimum L., Limoniastrum monopetalum L., and Limonium algarvense L. (Trabelsi et al., 2012; Rodrigues et al., 2015; Pereira et al., 2019). In addition, Azaizeh et al. (2013, 2015) observed that the polyphenol content and anthelmintic effects of P. lentiscus L. and Inula viscosa L. extracts on third stage (L3) mixed-species larvae exsheathment process changes across seasons. Thus, seasonality and organ-related variations should be taken into consideration when evaluating the anthelmintic value of botanical species aiming at its future use for GIN control strategies.
Cladium mariscus (L.) Pohl (Cyperaceae), also known as sawgrass, is a grass-like perennial halophytic herbaceous species distributed along the Mediterranean region, in low to moderate saline environments (Gerdol et al., 2018). Sawgrass lengthy and long-lasting leaves have characteristic saw-shaped margins and inflorescences rise above leaves (Castroviejo, 2008; Figure 1). In our previous investigations, sawgrass 80% acetone/water extracts exhibited high polyphenol content coupled with in vitro antioxidant and anti-inflammatory properties, with marked differences among seasons (Lopes et al., 2016; Oliveira et al., 2021a). Moreover, sawgrass aerial organs extract suppressed egg hatching and L3 larvae exsheathment processes of two models of GINs of sheep and goats, namely one abomasal species Haemonchus contortus and one intestinal species Trichostrongylus colubriformis (Oliveira et al., 2021b).
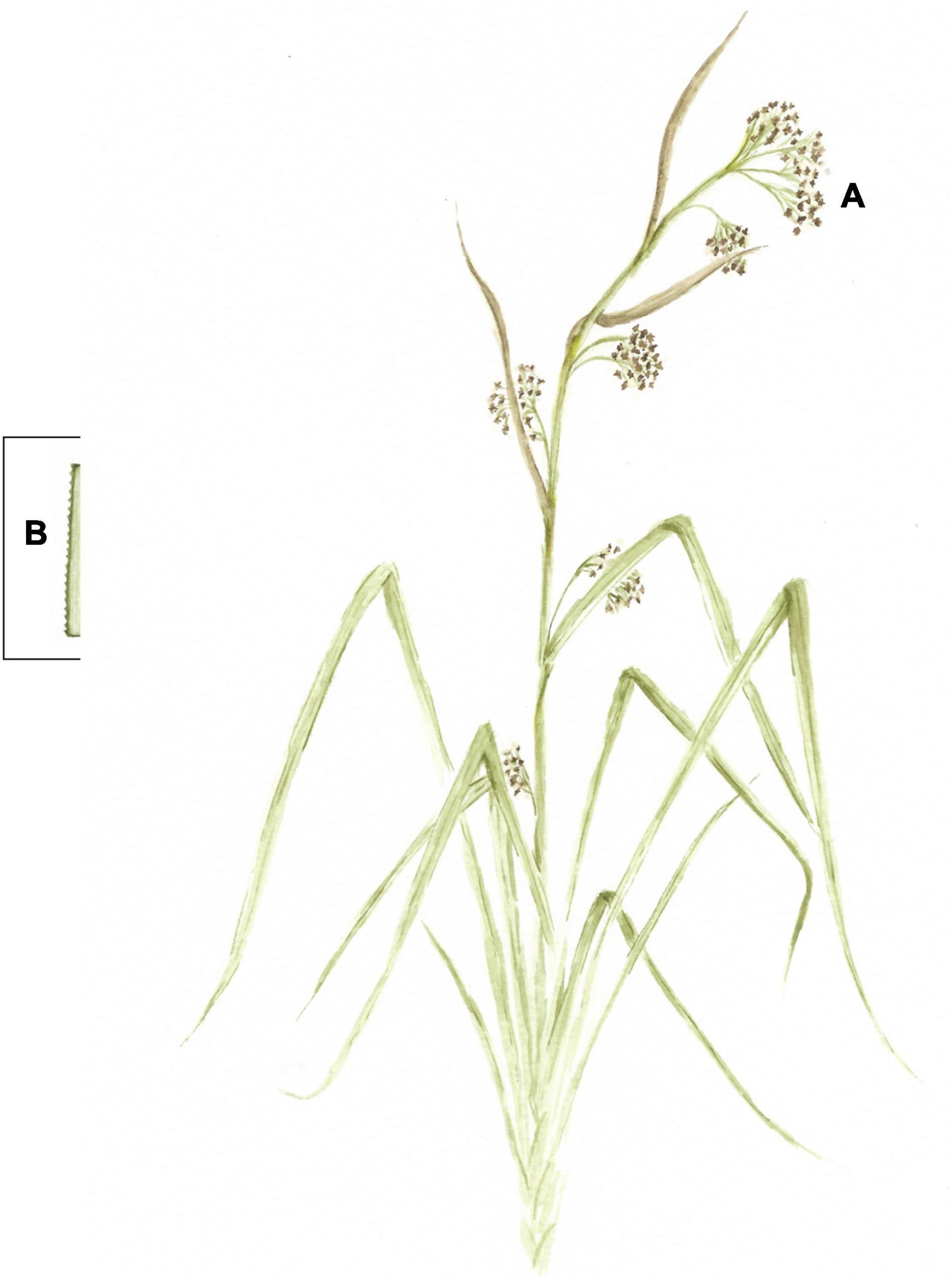
Figure 1. Illustration of Cladium mariscus (L.) Pohl (sawgrass) aerial organs: inflorescences (A) and detail of leaves (B). Illustration made by M. Oliveira.
In this work, we hypothesize that (1) differences in anthelmintic effects can occur among seasons and between aerial organs of sawgrass, and that (2) polyphenols could have a role on the detected anthelmintic effects. The first hypothesis was explored by using sawgrass extracts prepared from biomass collected from wild plants during the four seasons, and from different plant organs, namely leaves and inflorescences. Extracts were in vitro tested relying on two nematode stages (egg hatching and L3 larvae), and two key nematode species (H. contortus and T. colubriformis). To test the second hypothesis, polyvinylpolypyrrolidone (PVPP), a polyphenol-binding agent, was added to the extracts and the metabolites in non-treated and treated samples were profiled by high-performance liquid chromatography with electrospray ionization mass spectrometric detection (HPLC-ESI-MSn).
Materials and Methods
Plant Collection and Processing
Cladium mariscus (L.) Pohl aerial parts, including leaves and inflorescences (Figure 1), were manually harvested in Ludo, Faro, southern Portugal (37°01’03.3” N, 7°59’18.1”W, aprox. 7 m elevation) in spring (Sp; April, 2017: minimum temperature: 12°C, maximum temperature: 21°C, mean precipitation: 34 mm), summer (Su; July 2017: minimum temperature: 19°C, maximum temperature: 29°C; mean precipitation: 1 mm), autumn (Au; October, 2017: minimum temperature: 14°C, maximum temperature: 25°C, mean precipitation: 55 mm) and winter (Wi; January, 2018: minimum temperature: 8°C, maximum temperature: 16°C; mean precipitation: 210 mm) (source: WeatherSpark based on data obtained in the Faro International Airport). Inflorescences were present during summer and, to a lesser extent, in autumn, while green leaves were collected all year. In addition, only for summer season, samples of leaves (Le) and inflorescences (Inf) were also collected, for separate analysis. Afterward, samples were transported to the laboratory, washed, frozen at -20°C until freeze-dried (Lyoalfa 15) for 3 days and reduced to powder using a coffee and a ball miller (Retsch PM 100). For plant collection in the wild, mandatory licenses for the Portuguese territory were obtained, and the protocol for collection was followed according to the standard procedures recommended by “Instituto da Conservacão da Natureza e das Florestas (ICNF),” the national regulatory body. Voucher specimen (XBH03) were kept in the XtremeBio group herbarium, at Centre of Marine Sciences (CCMAR), University of Algarve (UAlg), Faro, Portugal, and the formal identification of the botanical material was made by Dr. Luiìsa Custoìdio (CCMAR).
Sample Preparation
Dried biomass was extracted with an 80% aqueous acetone solution (1:40, w/v) at room temperature (RT, 20–25°C), for 16 h, under stirring. Then, the residue was sieved using qualitative filters and concentrated in a rotary evaporator under reduced pressure and temperature (approximately 40°C), aiming acetone removal. Afterward, the aqueous residue was freeze-dried, and the extracts recovered for use in the further anthelmintic and chemical assays.
In vitro Anthelmintic Assays
Haemonchus contortus and Trichostrongylus colubriformis Parasites
The feces of caprine and ovine donors, kept indoors, monospecifically infected with susceptible strains of either H. contortus or T. colubriformis, were used to obtain third stage larvae (L3) and eggs. The facilities hosting the animals and trial performance met French ethical and welfare rules (agreement C 31 555 27 of August 19, 2010). Larvae were recovered with the Baermann method, stored in culture flasks at 4°C for one (H. contortus) or 4 months (T. colubriformis), before use in the larval exsheathment inhibition (LEIA) experiments. Eggs were collected on the day of the egg hatching inhibition (EHIA) experiments and used up to 2 h prior to collection.
Larval Exsheathment Inhibition Assay
Larval exsheathment inhibition assay (LEIA) protocol was performed as previously described by Bahuaud et al. (2006). The extracts were prepared in serial concentrations of 1,200, 600, 300, 150, and 75 μg/mL in phosphate buffered saline (PBS; 0.1 M phosphate, 0.05 M NaCl, pH 7.2) and incubated at 23°C for 3 h with L3 larvae (approx. 800 larvae/mL). After three times washed and centrifuged, the pellet was resuspended in 200 μL of PBS, and 40 μL were used to count the proportion of ensheathed/exsheathed larvae at 0 min. Then, the remaining 160 μL were exposed to a solution of Milton (2% w/v sodium hypochlorite, 16.5% w/v sodium chloride in PBS), aiming at the artificial induction of larvae exsheathment. The optimal concentration of Milton’s solution to achieve a gradual exsheathment process, i.e., reaching 100% exsheathment in 60 min, was tested for each batch before use. After 20, 40, and 60 min of exposure, the number of ensheathed and exsheathed larvae were counted under a microscope (400×). For each extract concentration and negative control (PBS) four replicates were performed and run in parallel. Percentage of larvae exsheathment (LE) for each replicate, was given by the formula: %LE = [(number of exsheathed larvae)/(number of exsheathed + ensheathed larvae) × 100].
Egg Hatching Inhibition Assay
Egg hatching inhibition assay (EHIA) protocol was performed as previously described in Oliveira et al. (2021b). Fecal samples were filtrated twice using a gaze hydrophile compress, sieved (25 μm) and washed with distilled water. The residues were subjected to three centrifugation steps using a saline saturated solution (d = 1.2), and the pellet recovered in PBS. After quantification, eggs (100 per well) were plated in 48-well sterile plates and treated with the extract solutions, prepared in PBS in concentrations ranging from 5,000 to 78 μg mL–1. After a 48 h incubation period at 27°C, the number of larvae and eggs, in each well, was registered after microscopic counting. Six replicates were performed for each extract concentration and the negative control, PBS, was run in parallel. The percentage of egg hatching (EH) for each well, was obtained as given by the following formula: %EH = [(number of larvae)/(number of eggs + larvae) × 100].
Polyvinylpolypyrrolidone Treatment
To appraise the possible role of polyphenols in the anthelmintic activity, PVPP, a polyphenol inhibitor (Doner et al., 1993) was added to extracts solutions (ratio 50:1; w/w) at the concentrations of 2,500 μg mL–1 and 1,200 μg mL–1, as previously described by Oliveira et al. (2021b). After overnight incubation at 4°C, the solution was centrifuged for 10 min at 4,500 rpm and the supernatant posteriorly used in the in vitro assays and for chemical analysis. The extracts not exposed to PVPP and the negative control, PBS, were run in parallel.
Chemical Profiling by High-Performance Liquid Chromatography With Electrospray Ionization Mass Spectrometric Detection
Chromatographic analyses were performed with an Agilent Series 1100 HPLC system with a G1315B diode array detector (Agilent Technologies, Santa Clara, CA, United States) and an ion trap mass spectrometer (Esquire 6000, Bruker Daltonics, Billerica, MA, United States) with an electrospray interface. Separation was performed in a Luna Omega Polar C18 analytical column (150 × 3.0 mm; 5 μm particle size) with a Polar C18 Security Guard cartridge (4 × 3.0 mm), both purchased from Phenomenex, Torrance, CA, United States. Detailed chromatographic conditions are available in Fernández-Poyatos et al. (2019). Compounds’ identification was performed by mass spectrometry data, whereas the quantification was carried out by UV using analytical standards of catechin (280 nm), apigenin (350 nm), kaempferol (350 nm) and luteolin (350 nm). Calibration graphs were constructed in the 0.5–100 mg/mL range. Repeatability (n = 9) and intermediate precision (n = 9, 3 consecutive days) were lower than 3 and 8%, respectively. Each analytical standard was used to quantify the corresponding compound or compounds of the same chemical family. The characterization of the metabolites was carried out by HPLC-ESI-MSn using the negative ion mode and identification was performed using analytical standards as well as bibliographic information. Compounds were numbered according to their elution order, keeping the same numbering in all extracts.
Statistical Analysis
Results are expressed either as effective concentration that inhibits 50% of the larval exsheathment and/or egg hatching (EC50) values (μg/mL) including 95% confidence intervals (CI). EC50 values and 95% CI were obtained by Probit analysis, while significant differences among groups were detected by relative median potency estimates, through IBM SPSS Statistics v. 26.0 software. PVPP results are expressed as average ± standard error of the mean (SEM).
Results
Season- and Organ-Related Effects on in vitro Anthelmintic Properties
Table 1 summarizes the in vitro anthelmintic activity results of sawgrass extracts prepared from biomass collected in successive seasons, against H. contortus and T. colubriformis larvae and eggs. Concerning the LEIA results, no significant seasonal effects were recorded for H. contortus, however, the summer sample was the most effective on inhibiting T. colubriformis L3 larvae exsheathment (EC50 = 77.8 μg mL–1), followed by the autumn extract (IC50 = 110.9 μg mL–1). Spring and winter samples were less active on T. colubriformis than H. contortus, while summer and autumn samples inhibited larvae exsheathment from both species in a similar manner. The extracts consistently presented lower EC50 values toward L3 larvae exsheathment than egg hatching processes and were more effective on impairing egg hatching of H. contortus than those of T. colubriformis (Table 1). While no seasonal effects were observed on EHIA using T. colubriformis parasites, the summer extract was the most active against H. contortus (EC50 = 1446.6 μg mL–1). The inflorescence extract was significantly more active to inhibit larvae exsheathment of H. contortus (EC50 = 60.0 μg mL–1) than leaves (EC50 = 87.7 μg mL–1). Indeed, the inflorescences extracts were also more effective than leaves against egg hatching, as illustrated by EC50 values for inflorescence representing 37% of those obtained for leaves, for both parasite species (Table 2). Interestingly, despite the high activity of inflorescences sample on EHIA, this is not reflected in the seasonal samples, particularly in summer as marked differences are not observed between samples collected in different seasons.
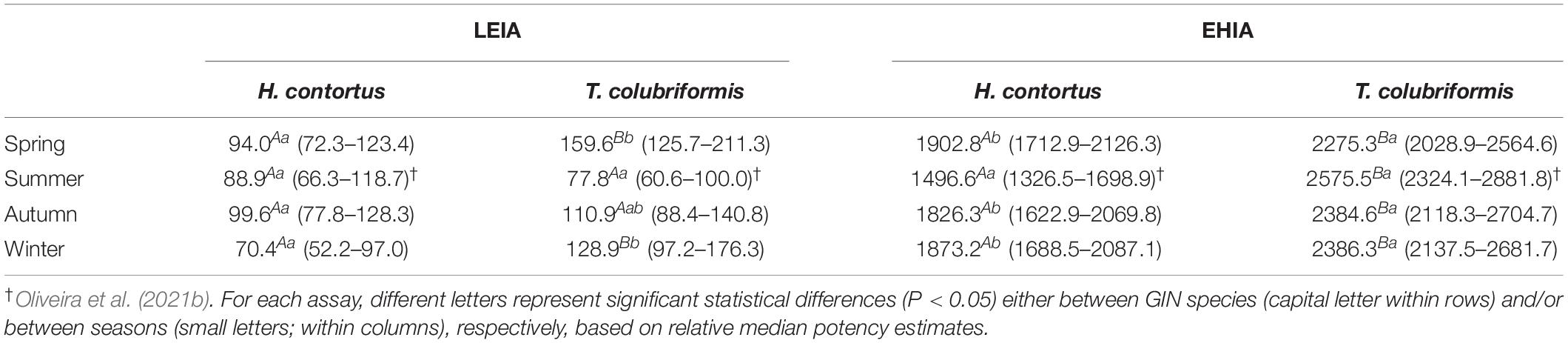
Table 1. Effective concentration that inhibits 50% of larval exsheathment or egg hatching (EC50 values, μg mL–1) and 95% confidence intervals (CI) obtained for Cladium mariscus (L.) Pohl (sawgrass) extracts on Haemonchus contortus and Trichostrongylus colubriformis L3 larvae exsheathment (LEIA) and egg hatching inhibition assays (EHIA).

Table 2. Effective concentration that inhibits 50% of larval exsheathment or egg hatching (EC50 values, μg mL–1) and 95% confidence intervals (CI) obtained for Cladium mariscus (L.) Pohl (sawgrass) organ extracts on Haemonchus contortus and Trichostrongylus colubriformis L3 larvae exsheathment (LEIA) and egg hatching inhibition assays (EHIA).
Role of Polyphenols in the Anthelmintic Activity: Polyvinylpolypyrrolidone as a Polyphenol Binding Agent
All PVPP-treated extracts, including those from leaves and inflorescences, still exhibit activity against the H. contortus and T. colubriformis L3 exsheathment in a similar manner (10–40% larvae exsheathment), while egg hatching was completely restored (Figures 2–4).
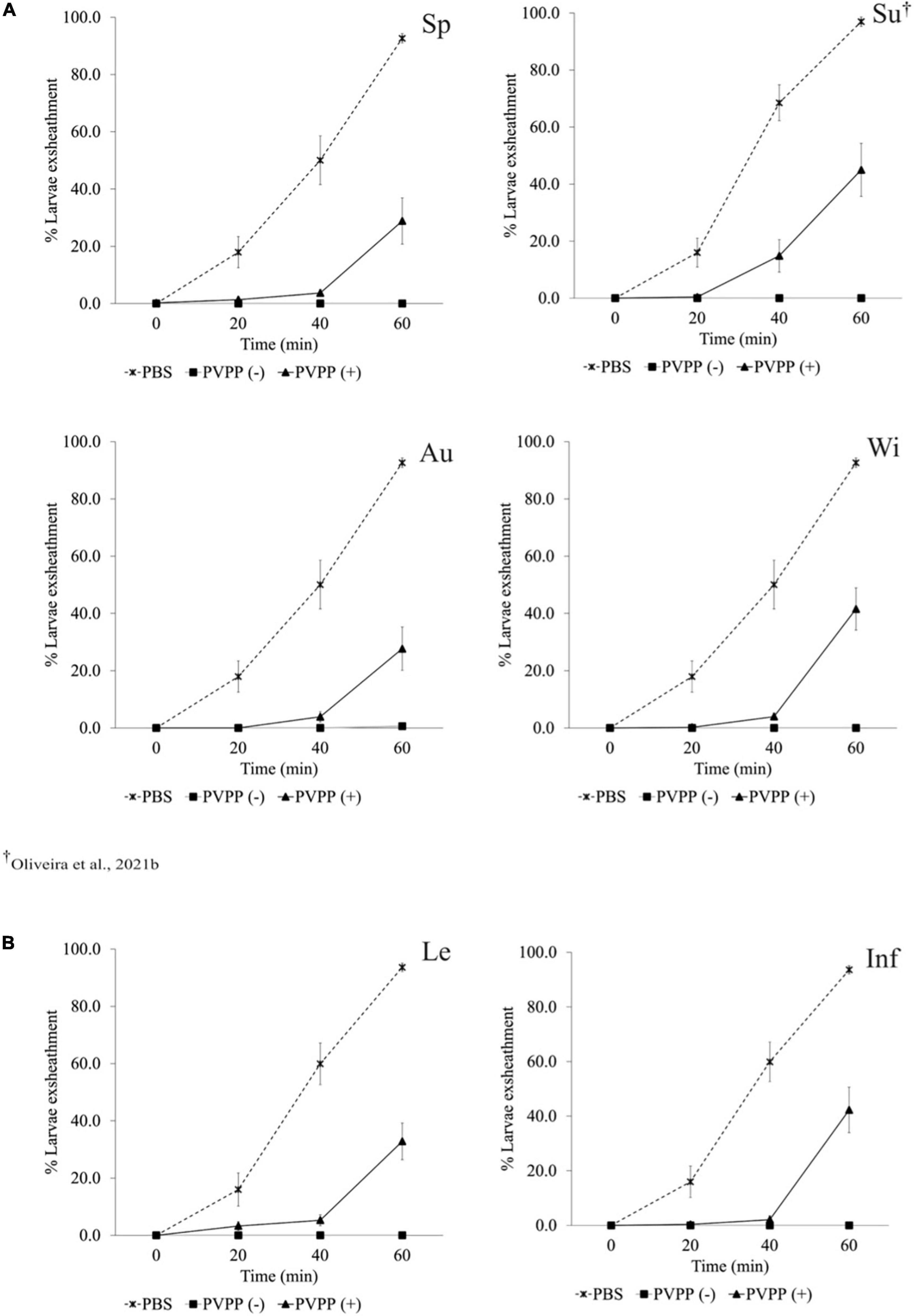
Figure 2. Anthelmintic effects of Cladium mariscus (L.) Pohl (sawgrass) seasonal (A) and organ (B) extracts, on LEIA for Haemonchus contortus at the concentration of 1,200 μg mL–1, either treated [PVPP(+)] or not [PVPP(-)] with PVPP. Sp, spring; Su, summer; Au, autumn; Wi, winter; Le, leaves; Inf, inflorescences.
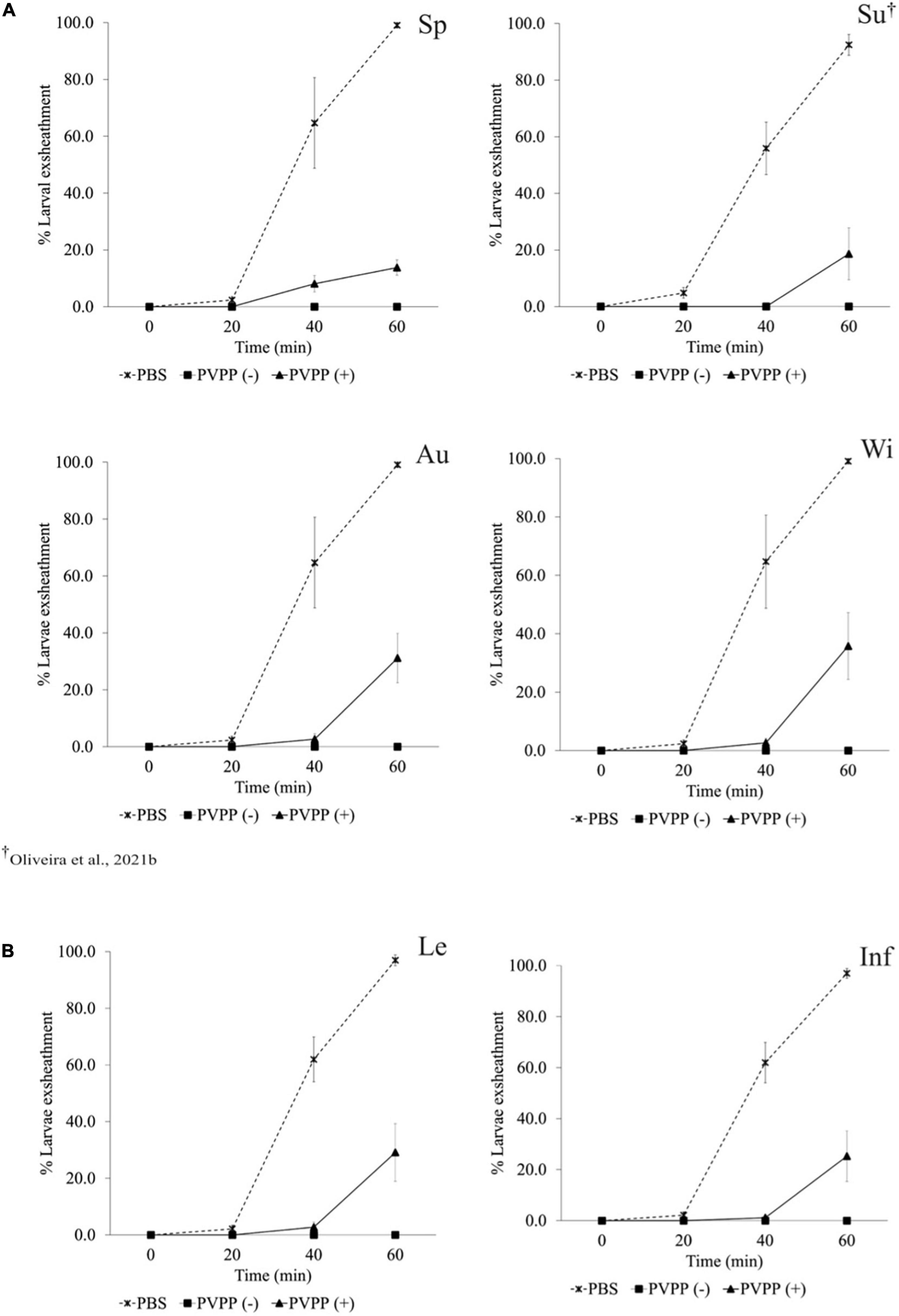
Figure 3. Anthelmintic effects of Cladium mariscus (L.) Pohl (sawgrass) seasonal (A) and organ (B) extracts, on LEIA for Trichostrongylus colubriformis at the concentration of 1,200 μg mL–1, either treated [PVPP(+)] or not [PVPP(-)] with PVPP. Sp, spring; Su, summer; Au, autumn; Wi, winter; Le, leaves; Inf, inflorescences.
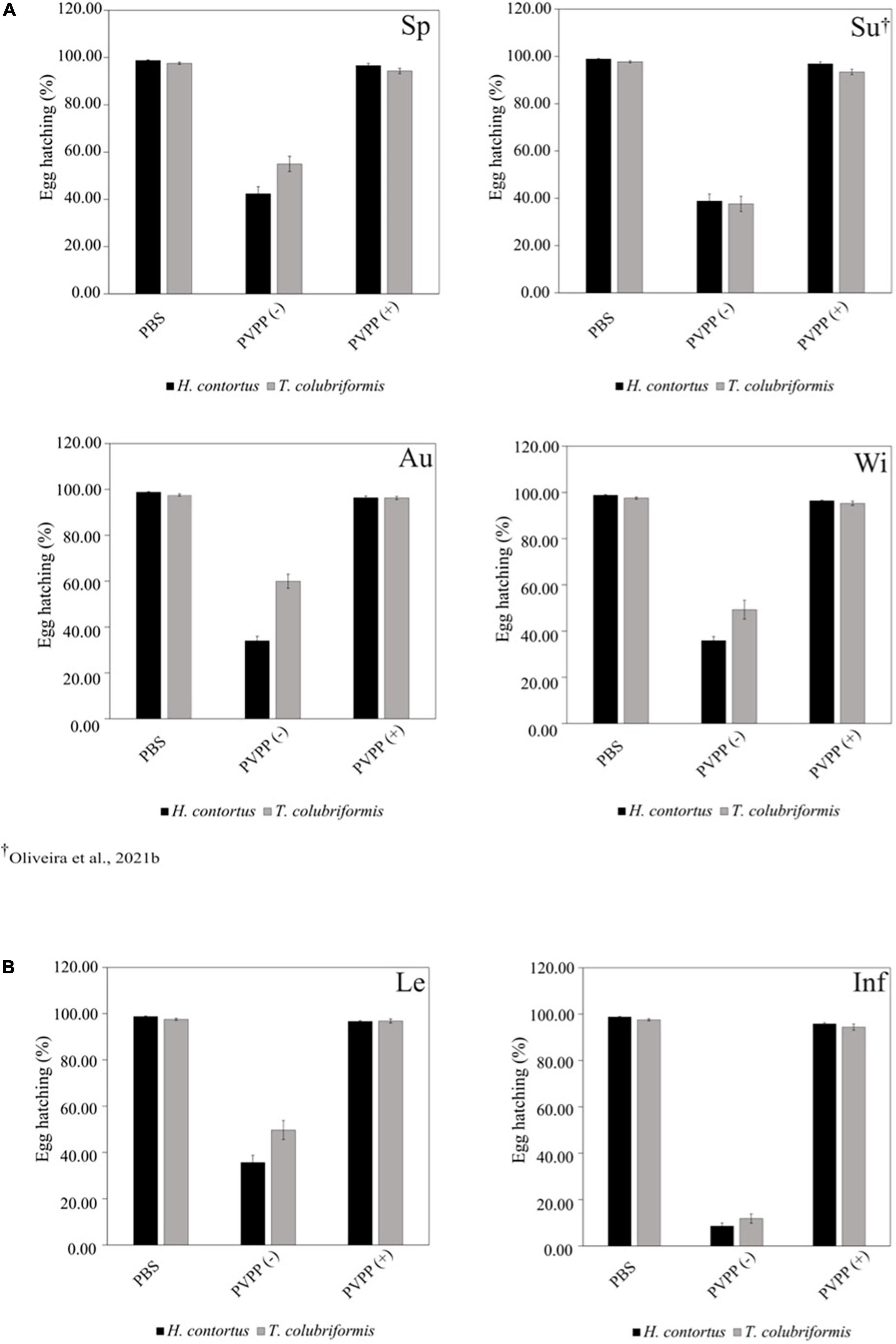
Figure 4. Anthelmintic effects of Cladium mariscus (L.) Pohl (sawgrass) seasonal (A) and organ (B) extracts on EHIA for Haemonchus contortus and Trichostrongylus colubriformis at the concentration of 2,500 μg mL–1, either treated [PVPP(+)] or not [PVPP(-)] with PVPP. Sp, spring; Su, summer; Au, autumn; Wi, winter; Le, leaves; Inf, inflorescences.
High-Performance Liquid Chromatography With Electrospray Ionization Mass Spectrometric Detection Comparative Analysis of the Chemical Profile of Polyvinylpolypyrrolidone Treated vs. Non-treated Extracts
The chemical characterization of the metabolites in each extract carried out by HPLC-ESI-MSn analysis is depicted in Table 3, while quantification of the main compounds of non-treated and treated PVPP samples is presented in Tables 4, 5. Compounds were numbered according to their elution order, keeping the same numbering in all extracts.
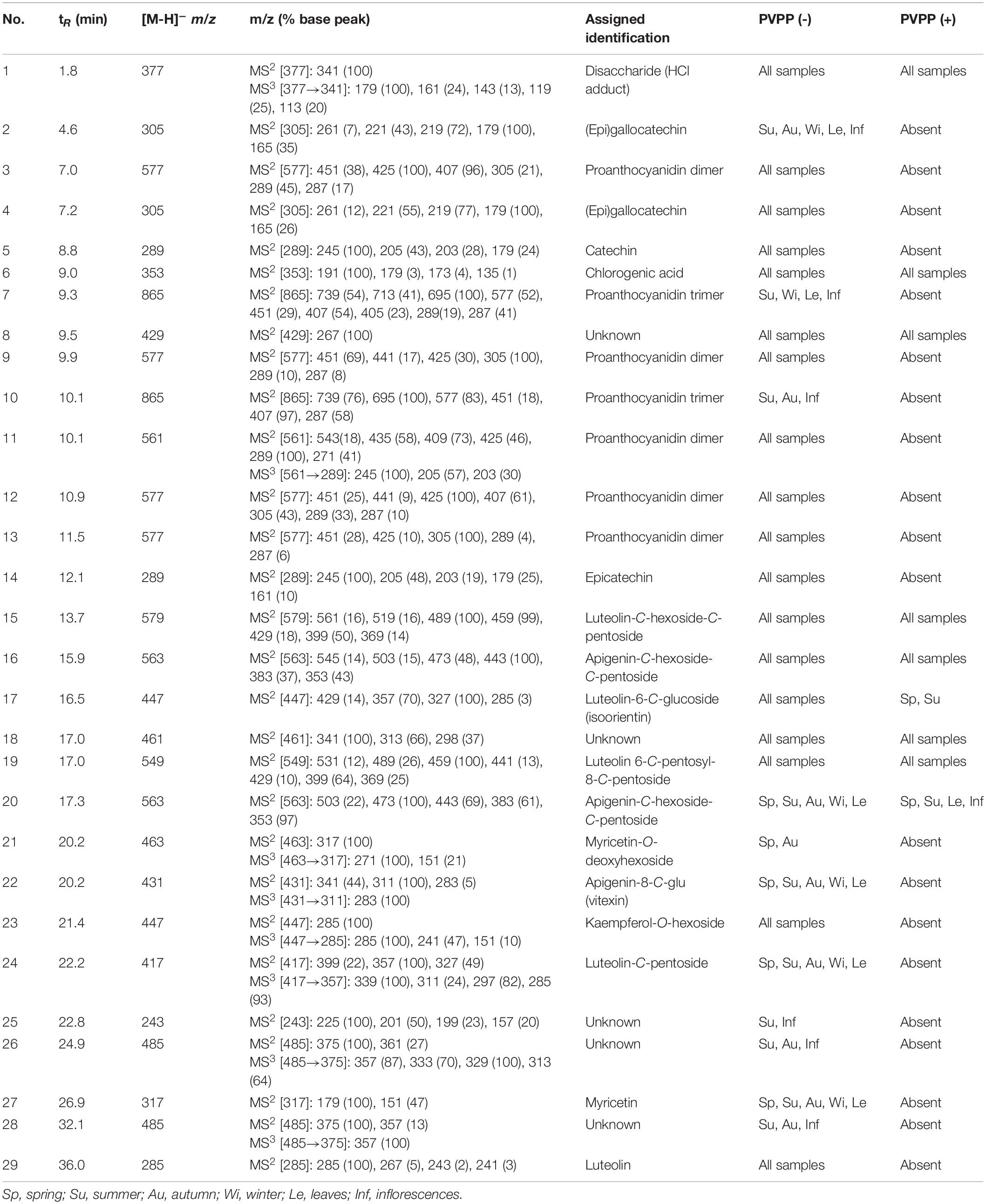
Table 3. Characterization of the polyphenolic compounds found in the analyzed extracts of Cladium mariscus (L.) Pohl (sawgrass).
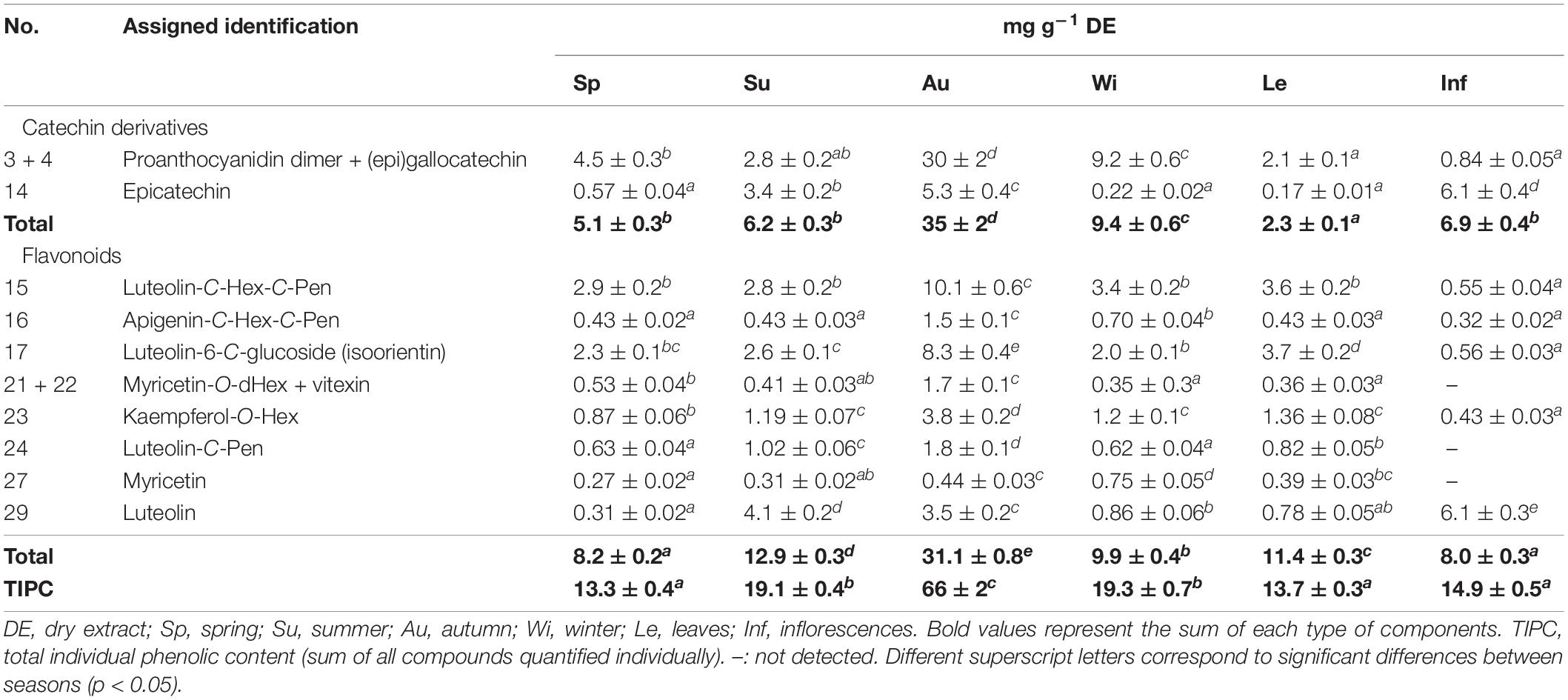
Table 4. Quantification of the main compounds detected in Cladium mariscus (L.) Pohl (sawgrass) before PVPP sample treatment.
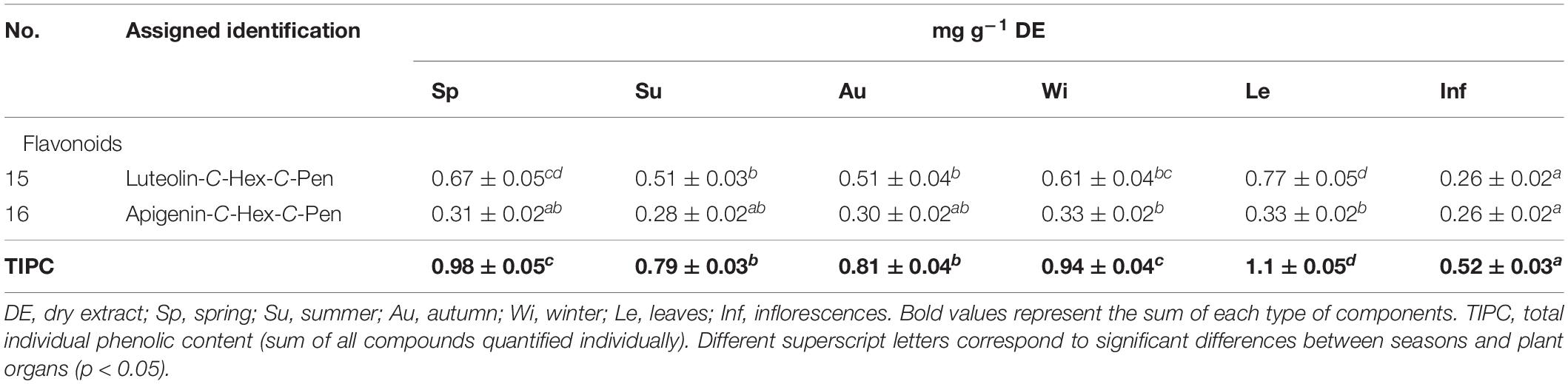
Table 5. Quantification of the main compounds detected in Cladium mariscus (L.) Pohl (sawgrass) after PVPP sample treatment.
All extracts were mainly composed of flavonoids, specifically flavan-3-ols (epigallocatechin, epicatechin 14) and proanthocyanidins (condensed tannins; 2.3–35.3 mg g-1, DW), luteolin (0.31–6.1 mg g-1, DW) and glycosylated flavonoids (1.9–27.6 mg g-1 DW; Table 4). Summer and autumn samples had the highest amounts of epicatechin 14 (3.4–5.3 mg g -1, DW) and of luteolin 29 (3.5–4.1 mg g-1, DW). Compound 5 was identified as catechin by comparison with an analytical standard. Compound 14, with the same fragmentation pattern, was thus identified as epicatechin. Several compounds were characterized as (epi)catechin derivatives. Compounds 2 and 4 were characterized as (epi)gallocatechin isomers. Compounds 3, 7, and 9–13 were characterized as proanthocyanidin dimers and trimers (Kajdžanoska et al., 2010; Hamed et al., 2014).
Compounds 16 and 20, with [M-H]- at m/z 563, were characterized as apigenin-C-hexoside-C-pentoside isomers due to the fragment ions observed at [M-H- 60]-, [M-H- 90]-, [M-H- 120]-, [M-H- 180]-, and [M-H- 210]-, characteristic of di-C-glycoside flavonoids (Han et al., 2008). Compound 22 was characterized as apigenin-8-C-glucoside (vitexin) based on bibliographic information (Waridel et al., 2001). Compound 23 was characterized as kaempferol-O-hexoside due to the neutral loss of 162 Da to yield kaempferol aglycone at m/z 285. The fragmentation of kaempferol was compared with an analytical standard. Compound 27 was identified as myricetin, with deprotonated molecular ion at m/z 317 and fragment ions at m/z 179 and 151. Compound 21 was characterized as myricetin-O-deoxyhexoside. Compound 29 was identified as luteolin by comparison with an analytical standard. Compounds 15, 17, 19, and 24 were luteolin-C-glycosides. Compound 17 was characterized as isoorientin due to the fragment ion at m/z 429 (absent in orientin) (Algamdi et al., 2011). Besides flavonoids, one phenolic acid, compound 6 was identified as chlorogenic acid by comparison with an analytical standard. Moreover, compound 1 suffered the neutral loss of 36 Da (HCl) to yield the base peak at m/z 341. Its fragmentation pattern was consistent with a disaccharide formed by two hexosides (probably glucose) (Brudzynski and Miotto, 2011).
The inflorescences extract (from the summer sample) exhibited increased amounts of compounds 14 and 29 (6.1 and 6.1 mg g–1 DW), in contrast to leaves (0.17 and 0.78 mg g–1 DW). The proanthocyanidin dimer + (epi)gallocatechin 3 + 4 reached a maximum of 30 mg g–1, dry weight (DW) in autumn. High amounts of 3 + 4 are noted in all extracts ranging from 2.1 up to 30 mg g–1 DW, except for inflorescences (0.84 mg g–1 DW). Proanthocyanidins 7 and 9–13 were identified but not quantified due to low signal.
Discussion
Salt-tolerant plants (halophytes) have ethnomedicinal and ethnoveterinary reported uses and are considered important sources of compounds and products with multiple commercial uses. Such plants may also represent an important resource for animal management and veterinary purposes, in view of the growing need to identify alternatives to chemical ingredients in livestock production and increasing concern for animal health and better welfare practices (Oliveira et al., 2021c). In our previous work (Oliveira et al., 2021a), sawgrass aqueous/acetone extracts were rich in total phenolics and tannins, and exhibited a significant activity, especially those made from biomass collected in summer and autumn. Such results, especially the tannins levels, prompt us to evaluate the antiparasitic properties of such extracts collected along the year toward larvae and eggs from two relevant GINs, namely H. contortus and T. colubriformis. Haemonchus contortus reside in the abomasum, while T. colubriformis exist in the small intestine, and both reduce voluntary feed intake and nutrient absorption, thus reducing drastically the production of small ruminants (Hoste et al., 2016).
The extracts were more active toward larvae than eggs, most probably due to structural dissimilarities between the eggshell and the larval sheath (Mansfield et al., 1992; Hoste et al., 2015). Differences in susceptibility between eggs and adults are also attributed to the chemical components in the extracts (Araújo-Filho et al., 2018). The extracts were also more active toward H. contortus, which is consistent with the higher susceptibility of H. contortus in contrast to T. colubriformis, reported previously by other authors (Paolini et al., 2004; Brunet and Hoste., 2006; Quijada et al., 2015). Seasonal differences in bioactivity were observed mainly for summer and autumn samples, we questioned whether it could be (1) due to environmental effects, since the production of these metabolites is part of the plant defense machinery, to cope with the harsh settings of the dry Mediterranean climate (Di Ferdinando et al., 2014); or (2) due to differences in biochemical contents associated to the plant organs, viz. leaves and inflorescences. Thus, we proceed to investigate the in vitro anthelmintic properties of extracts made from these organs collected in summer (Table 2). The inflorescences extracts were more effective than leaves against egg hatching, for both parasite species (Table 2). Interestingly, despite the high activity of inflorescences sample on EHIA, this is not reflected in the seasonal samples, particularly in summer as marked differences are not observed between samples collected in different seasons. Having in mind that an extract is a complex mixture of compounds, the latter observations can be a result of synergistic/antagonist interactions between the present metabolites or perhaps due to a dilution of the compounds of interest in the mixture (leaves and inflorescences in the summer sample) in comparison to the inflorescences extract alone.
Aiming to elucidate the role of polyphenols in the anthelmintic properties, the extracts were retested after and before treatment with PVPP, a polyphenol-binding agent. All PVPP-treated extracts retained activity toward H. contortus and T. colubriformis larvae, and egg hatching was completely restored (Figures 2–4), which agrees with previous results obtained with the summer sample (Oliveira et al., 2021b). Thus, polyphenols seem to be the main metabolites involved for the egg hatching inhibitory properties of the extracts at the highest concentration (2500 μg mL–1). In contrast, besides polyphenols, other compounds not adsorbed by PVVP seem also effective on L3 larvae exsheathment at 1,200 μg mL–1.
An HPLC-ESI-MSn comparative analysis coupled with the use of PVPP was conducted in an attempt to answer the following questions: (1) which major metabolites, removed after PVPP treatment, may be identified to exert the egg hatching inhibitory effects?; (2) which compounds may be remaining after PVPP treatment that can account for the larvae exsheathment activity?; and (3) what chemical variations occur between leaves and inflorescences extracts that may justify the significant higher anthelmintic activity of the latter samples ? Our previous results obtained for the summer samples (Oliveira et al., 2021b) provided some hints to address the first two questions, yet seasonal fluctuations and organ related variations were not priorly considered. We expected that an in-depth comparative analysis of the chemical assets of seasonal and organ extracts, in combination with the biological data herein presented, enables a clarification of the bioactive metabolites of interest for AH properties and its production dynamics. Flavonoids were the dominant compounds identified in all the extracts, especially flavan-3-ols (epigallocatechin, epicatechin 14), proanthocyanidins (condensed tannins), luteolin, and glycosylated flavonoids (Table 4). Summer and autumn samples had the highest amounts of epicatechin 14 and luteolin 29. Differences on the polyphenolic composition of plants have been demonstrated to be correlated to environmental changes, particularly in Mediterranean plants subjected to drought, high temperature and solar irradiance, high UV intensity and salt stress conditions, expected during dry seasons such as summer and autumn (Hernández et al., 2004; Di Ferdinando et al., 2014; Gori et al., 2020). This data sustains the role of polyphenols in plant defensive and adaptative strategies to cope with the challenging Mediterranean environmental settings. For example, quercetin and luteolin derivatives act as photoprotector compounds and accumulate in response to UV radiation and increased sun irradiance (Tattini et al., 2004; Agati et al., 2011). Nevertheless, in the wild, besides environmental variations, other abiotic and biotic factors interact concomitantly, which can also affect the production of these metabolites, e.g., the phenological stage of the plant. In this sense, we suspected that organ-related variations may also account for the increased 14 and 29 levels observed for these dry seasons, since the inflorescences extract (from the summer sample) also exhibit increased amounts of 14 and 29, in contrast to leaves, supporting the former observed variations in the anthelmintic activity. Of interest is the proanthocyanidin dimer + (epi)gallocatechin 3 + 4 content in autumn, but it did not influence greatly the anthelmintic properties. In fact, high amounts of 3 + 4 are noted in all extracts except for inflorescences. Since the inflorescences extract was particularly active against GIN egg hatching, most probably these compounds are not significantly impacting the anthelmintic effects of the extracts. On the other hand, the anthelmintic value of proanthocyanidins is well-recognized in the scientific community (Mueller-Harvey et al., 2018).
Proanthocyanidins 7 and 9–13 were identified but not quantified due to low signal, limiting a complete elucidation of the contribution of tannins to the anthelmintic effects, which were present in all samples, and particularly in the summer and inflorescences extracts. Moreover, other unidentified metabolites, particularly present in inflorescences extract might also contribute to the observed effects. The in vitro anthelmintic effects of flavan-3-ols, proanthocyanidins and luteolin are well-described in the literature (Molan et al., 2003; Klongsiriwet et al., 2015; Quijada et al., 2015). Epicatechin and epigallocatechin suppress T. colubriformis larvae development by 50% at 43 μg mL–1, but were less effective on egg hatching, inhibiting less than 20% up to 1 mg mL–1 (Molan et al., 2003). Moreover, Soldera-Silva et al. (2018) reported that epicatechin exhibit an IC50 value of 10 μg mL–1 in the larval migration test, using H. contortus parasites (Soldera-Silva et al., 2018). Regarding proanthocyanidins, several plant extracts rich in these metabolites have documented anthelmintic effects, influenced by the concentration, polymer size, and structural composition of these complex molecules. The most common monomers of proanthocyanidins are catechin and epicatechin [proacyanidin (PC)-type tannins] and gallocatechin and epigallocatechin [prodelphinidin (PD)-type tannins]. Interestingly, it has been demonstrated that PD are more potent inhibitors than PC on L3 exsheathment of H. contortus and T. colubriformis (Brunet and Hoste, 2006; Quijada et al., 2015; Mueller-Harvey et al., 2018) or egg hatching of T. colubriformis (Molan et al., 2003), and that the addition of a galloyl group enhances activity (Molan et al., 2003). In addition to these catechin derivatives, the flavonoid luteolin 29 exhibited an IC50 value of 17.1 μg mL–1 against L3 larvae exsheathment of H. contortus (Klongsiriwet et al., 2015). Synergistic interactions between procyanidins and luteolin (30 μM) have been demonstrated previously, resulting in a reduced IC50 value of 75.9 μg mL–1, in comparison to the isolated procyanidin fraction (IC50 = 356 μg mL–1; Klongsiriwet et al., 2015). However, more recently, 29 was ineffective as a larvicidal agent at 2.5 mg mL–1, against L3 larvae of H. contortus (Delgado-Núñez et al., 2020). These former results emphasize that flavan-3-ols, proanthocyanidins and luteolin are most likely the bioactive metabolites of C. mariscus extracts, as they are some of the main metabolites present. However, the contribution of flavonoid glycosides cannot be excluded, particularly because they were detected in high amounts in all extracts, except for inflorescences. Still, the considerably lower amount of flavonoid glycosides in the inflorescences extract perhaps may have unmasked the interactions with other metabolites present (e.g., proanthocyanidins and luteolin) leading to improved anthelmintic effects. Flavonoid glycosides have been identified in the chemical profile of several plant species with proven anthelmintic effects (Barrau et al., 2005; Mengistu et al., 2017; Araújo et al., 2019; Romero et al., 2020), and some authors have posed the possibility of occurring interactions of this type of compounds with GIN (Barrau et al., 2005; Alonso-Díaz et al., 2008). Barrau et al. (2005) determined that 3 flavonol glycosides (quercetin-3-O-rutinoside or rutin, kaempferol-3-rutinoside or nicotiflorin and isorhamnetin-3-rutinoside or narcissin) reduced the migration of H. contortus L3 larvae in 25–35% when applied at 1,200 μg mL–1. Moreover, Sprengel Lima et al. (2021) observed that the addition of rutinose to the quercetin structure resulted in a 2-fold increase in the larvicidal activity. In addition, two flavone-C-glycosides (isoschaftoside and schaftoside) exhibited high toxicity against the parasitic nematode Meloidogyne incognita, leading to the death of 50% of the worms at 114.66 and 323.09 μg mL–1, respectively (Du et al., 2011). Despite these limited studies, the anthelmintic value of glycosylated forms of polyphenols remains to be elucidated as well as their interactions with aglycone molecules.
The partial inhibition of L3 larvae exsheathment in the PVPP-treated samples could be, in fact, due to the remaining content of flavonoid glycosides identified in the chemical analysis (15–17, 19–20). Two C-glycosyl flavonoids were quantified in higher amounts i.e., luteolin-C-Hex-C-Pen 15 and apigenin-C-Hex-C-Pen 16 (Table 5), suggesting its potential involvement in the anthelmintic effects. The quantification of these metabolites in PVPP-treated samples did not vary greatly, except for the inflorescences extract (Table 5). Still, other metabolites present after PVPP treatment remain to be identified (e.g., compound 8). PVPP is widely used to adsorb polyphenolic structures from plant extracts and consequently to assess its impact on the biological activity (Barrau et al., 2005; Alonso-Díaz et al., 2008; Manolaraki et al., 2010; Vargas-Magaña et al., 2014; Mengistu et al., 2017). However, it is important to keep in mind that PVPP is generally more efficient in binding with molecules with a higher number of hydroxyl groups and that this binding is also influenced by structural substitution patterns (Doner et al., 1993). For example, Laborde et al. (2006) showed that the association of PVPP with quercetin aglycone was 4 to 5-fold stronger than that with its glucoside (quercetin-3-O-glucoside).
Conclusion
Altogether, the results of this work emphasize (1) that many factors can influence the variations in the bioactive compounds explaining the anthelminthic properties of plants; (2) the relevance of considering abiotic factors when surveying botanical resources from the wild; (3) the potential of sawgrass as a source of bioactive metabolites against gastrointestinal nematodes, and (4) a confirmation of the anthelmintic value of a range of polyphenols in the search for alternative control options for GIN infections of ruminants. The current obtained results encouraged future work to focus on the complete elucidation of biological effects of polyphenolic glycosides, but also to explore further the interactions with other phenolic compounds and their contribution for the anthelmintic effects when mixed. In fact, we would suggest that one should be careful when assuming that the remaining activity on anthelmintic assays using PVPP treated samples is not only associated with polyphenolic compounds, and a detailed biochemical characterization by high power analyzing methods before and after the use of PVPP could help to elucidate the bioactive metabolites of interest. In addition, the egg hatching properties of luteolin could be appraised, for a better understanding of the efficient inhibition of egg hatching by the inflorescence extracts.
Data Availability Statement
The raw data supporting the conclusions of this article will be made available by the authors, without undue reservation.
Author Contributions
MO performed the design of the study, collection and extraction of plant material, preparation of the extracts, in vitro assays, and wrote the first draft of the manuscript. CL assisted in the anthelmintic assays. EL-M performed the HPLC-ESI-MSn analysis. HH performed the design of the study and review of the manuscript. LC performed the design of the study, review and final approval of the manuscript, and was responsible for funding acquisition. All authors contributed to the article and approved the submitted version.
Funding
This work was supported by Foundation for Science and Technology (FCT), the Portuguese National Budget funding (GreenVet-ALG-01-0145-FEDER-028876 and UIDB/04326/2020 projects). This work was also made under the frame of the project HaloFarMs, which was part of the Partnership on Research and Innovation in the Mediterranean Area (PRIMA) Programme supported by the European Union and funded by the national funding bodies of Participating States (FCT in Portugal). MO acknowledged FCT for the Ph.D. grant SFRH/BD/123658/2016. LC was sustained by FCT Scientific Employment Stimulus (CEECIND/00425/2017). CL acknowledged CAPES (finance code 001) for the scholarship and the Doctoral Program Sandwich Abroad (Grant no. 88881.187940/2018-01).
Conflict of Interest
The authors declare that the research was conducted in the absence of any commercial or financial relationships that could be construed as a potential conflict of interest.
Publisher’s Note
All claims expressed in this article are solely those of the authors and do not necessarily represent those of their affiliated organizations, or those of the publisher, the editors and the reviewers. Any product that may be evaluated in this article, or claim that may be made by its manufacturer, is not guaranteed or endorsed by the publisher.
Acknowledgments
The help provided by Dr. Nicolas Fabre and Valérie Cristofolli (Faculty of Pharmacy, Université Paul Sabatier Toulouse III, France) is gratefully acknowledged. In addition, technical and human support provided by CICT of Universidad de Jaén (UJA, MINECO, Junta de Andalucía, FEDER) and Setha Ketavong (UMR IHAP 1225 INRAE/ENVT Toulouse) are sincerely recognized and thanked.
References
Agati, G., Biricolti, S., Guidi, L., Ferrini, F., Fini, A., and Tattini, M. (2011). The biosynthesis of flavonoids is enhanced similarly by UV radiation and rootzone salinity in L. vulgare leaves. J. Plant Physiol. 168, 204–212. doi: 10.1016/j.jplph.2010.07.016
Algamdi, N., Mullen, W., and Crozier, A. (2011). Tea prepared from Anastatica Hirerochuntica seeds contains a diversity of antioxidant flavonoids, chlorogenic acids and phenolic compounds. Phytochemistry 72, 248–254. doi: 10.1016/j.phytochem.2010.11.017
Alonso-Díaz, M. A., Torres-Acosta, J. F. J., Sandoval-Castro, C. A., Capetillo-Leal, C., Brunet, S., and Hoste, H. (2008). Effects of four tropical tanniniferous plant extracts on the inhibition of larval migration and the exsheathment process of Trichostrongylus colubriformis infective stage. Vet. Parasitol. 153, 187–192. doi: 10.1016/j.vetpar.2008.01.011
Araújo, A. C. M., Almeida, E. B. Jr., Rocha, C. Q., Lima, A. S., Silva, C. R., Tangerina, M. M. P., et al. (2019). Antiparasitic activities of hydroethanolic extracts of Ipomoea imperati (Vahl) Griseb. (Convolvulaceae). PLoS One 14:e0211372. doi: 10.1371/journal.pone.0211372
Araújo-Filho, J. V., Ribeiro, W. L. C., André, W. P. P., Cavalcante, G. S., Guerra, M. C. M., Muniz, C. R., et al. (2018). Effects of Eucalyptus citriodora essential oil and its major component, citronellal, on Haemonchus contortus isolates susceptible and resistant to synthetic anthelmintics. Ind. Crops Prod. 124, 294–299.
Azaizeh, H., Halahleh, F., Abbas, N., Markovics, A., Muklada, H., Ungar, E. D., et al. (2013). Polyphenols from Pistacia lentiscus and Phillyrea latifolia impair the exsheathment of gastro-intestinal nematode larvae. Vet. Parasitol. 191, 44–50. doi: 10.1016/j.vetpar.2012.08.016
Azaizeh, H., Mreny, R., Markovics, A., Muklada, H., Glazer, I., and Landau, S. Y. (2015). Seasonal variation in the effects of Mediterranean plant extracts on the exsheathment kinetics of goat gastrointestinal nematode larvae. Small Rumin. Res. 131, 130–135. doi: 10.1016/j.smallrumres.2015.08.004
Bahuaud, D., Martinez-Ortiz-de-Montellano, C., Chauveau, S., Prevot, F., Torres-Acosta, J. F. J., Fouraste, I., et al. (2006). Effects of four tanniferous plant extracts on the in vitro exsheathment of third-stage larvae of parasitic nematodes. Parasitology. 132, 545–554. doi: 10.1017/S0031182005009509
Barrau, E., Fabre, N., Fouraste, I., and Hoste, H. (2005). Effect of bioactive compounds from Sainfoin (Onobrychis viciifolia Scop.) on the in vitro larval migration of Haemonchus contortus: role of tannins and flavonol glycosides. Parasitology. 131, 531–538. doi: 10.1017/S0031182005008024
Brudzynski, K., and Miotto, D. (2011). Honey melanoidins: analysis of the compositions of the high molecular weight melanoidins exhibiting radical-scavenging activity. Food Chem. 127, 1023–1030. doi: 10.1016/j.foodchem.2011.01.075
Brunet, S., and Hoste, H. (2006). Monomers of condensed tannins affect the larval exsheathment of parasitic nematodes of ruminants. J. Agric. Food Chem. 54, 7481–7487. doi: 10.1021/jf0610007
Castroviejo, S. (2008). “Cladium P. Browne,” in Flora Iberica, eds S. Castroviejo, C. Aedo, M. Laínz, Garmendia Muñoz, Feliner Nieto, J. Paiva, et al. (Madrid: Real Jardín Botánico, CSIC). 102–104.
Charlier, J., Thamsborg, S. M., Bartley, D. J., Skuce, P. J., Kenyon, F., Geurden, T., et al. (2018). Mind the gaps in research on the control of gastrointestinal nematodes of farmed ruminants and pigs. Transbound. Emerg. Dis. 65, 217–234. doi: 10.1111/tbed.12707
Delgado-Núñez, E. J., Zamilpa, A., González-Cortazar, M., Olmedo-Juárez, A., Cardoso-Taketa, A., Sánchez-Mendoza, E., et al. (2020). Isorhamnetin: a nematocidal flavonoid from Prosopis laevigata leaves against Haemonchus contortus eggs and larvae. Biomolecules. 10:773. doi: 10.3390/biom10050773
Di Ferdinando, M., Brunetti, C., Agati, G., and Tattini, M. (2014). Multiple functions of polyphenols in plants inhabiting unfavorable Mediterranean areas. Environ. Exp. Bot. 103, 107–116. doi: 10.1016/j.envexpbot.2013.09.012
Doner, W. L., Becard, G., and Irwin, L. P. (1993). Binding of flavonoids by polyvinylpolypyrrolidone. J. Agric. Food Chem. 41, 753–757. doi: 10.1021/jf00029a014
Du, S. S., Zhang, H. M., Bai, C. Q., Wang, C. F., Liu, Q. Z., Liu, Z. L., et al. (2011). Nematocidal flavone-C-glycosides against the root-knot nematode (Meloidogyne incognita) from Arisaema erubescens tubers. Molecules. 16, 5079–5086. doi: 10.3390/molecules16065079
Fernández-Poyatos, M. P. Ruiz-Medina, A.Llorent-Martínez, E. J. (2019). Phytochemical profile, mineral content, and antioxidant activity of Olea europaea L. cv. Cornezuelo table olives. Influence of in vitro simulated gastrointestinal digestion. Food Chem. 297:124933. doi: 10.1016/j.foodchem.2019.05.207
Gerdol, R., Brancaleoni, L., Lastrucci, L., Nobili, G., Pellizzari, M., Ravaglioli, M., et al. (2018). Wetland plant diversity in a Coastal nature reserve in Italy: relationships with salinization and eutrophication and implications for nature conservation. Estuaries Coasts 41, 2079–2091. doi: 10.1007/s12237-018-0396-5
Gori, A., Nascimento, L. B., Ferrini, F., Centritto, M., and Brunetti, C. (2020). Seasonal and diurnal variation in leaf phenolics of three medicinal Mediterranean wild species: what is the best harvesting moment to obtain the richest and the most antioxidant extracts? Molecules 25:956. doi: 10.3390/molecules25040956
Hamed, A. I., Al-Ayed, A. S., Moldoch, J., Piacente, S., Oleszek, W., and Stochmal, A. (2014). Profiles analysis of proanthocyanidins in the argun nut (Medemia argun – an ancient Egyptian palm) by LC-ESI-MS/MS. J. Mass Spectrom. 49, 306–315. doi: 10.1002/jms.3344
Han, J., Ye, M., Qiao, X., Xu, M., Wang, B.-R., and Guo, D.-A. (2008). Characterization of phenolic compounds in the Chinese herbal drug Artemisia annua by liquid chromatography coupled to electrospray ionization mass spectrometry. J. Pharm. Biomed. Anal. 47, 516–525. doi: 10.1016/j.jpba.2008.02.013
Hernández, I., Alegre, L., and Munné-Bosch, S. (2004). Drought-induced changes in flavonoids and other low molecular weight antioxidants in Cistus clusii grown under Mediterranean field conditions. Tree Physiol. 24, 1303–1311. doi: 10.1093/treephys/24.11.1303
Hoste, H., Jackson, F., Athanasiadou, S., Thamsborg, S. M., and Hoskin, S. O. (2006). The effects of tannin-rich plants on parasitic nematodes in ruminants. Trends Parasitol. 22, 253–261. doi: 10.1016/j.pt.2006.04.004
Hoste, H., Torres-Acosta, J. F., Sandoval-Castro, C. A., Mueller-Harvey, I., Sotiraki, S., Louvandini, H., et al. (2015). Tannin containing legumes as a model for nutraceuticals against digestive parasites in livestock. Vet. Parasitol. 212, 5–17. doi: 10.1016/j.vetpar.2015.06.026
Hoste, H., Torres-Acosta, J. F. J., Quijada, J., Chan-Perez, I., Dakheel, M. M., Kommuru, D. S., et al. (2016). Interactions between nutrition and infections with Haemonchus contortus and related gastrointestinal nematodes in small ruminants. Adv. Parasitol 93, 239–351. doi: 10.1016/bs.apar.2016.02.025
Kajdžanoska, M., Gjamovski, V., and Stefova, M. (2010). HPLC-DAD-ESI-MSn identification of phenolic compounds in cultivated strawberries from Macedonia. Maced. J. Chem. Chem. Eng. 29, 181–194.
Klongsiriwet, C., Quijada, J., Williams, A. R., Mueller-Harvey, I., Williamson, E., and Hoste, H. (2015). Synergistic inhibition of Haemonchus contortus exsheathment by flavonoid monomers and condensed tannins. Int. J. Parasitol. Drugs Drug Resist. 5, 127–134. doi: 10.1016/j.ijpddr.2015.06.001
Laborde, B., Moine-Ledoux, V., Richard, T., Saucier, C., Dubourdieu, D., and Monti, J.-P. (2006). PVPP-Polyphenol complexes: a molecular approach. J. Agric. Food Chem. 54, 4383–4389. doi: 10.1021/jf060427a
Liu, M., Panda, S. K., and Luyten, W. (2020). Plant-based natural products for the discovery and development of novel anthelmintics against nematodes. Biomolecules 10:426. doi: 10.3390/biom10030426
Lopes, A., Rodrigues, M. J., Pereira, C., Oliveira, M., Barreira, L., Varela, J., et al. (2016). Natural products from extreme marine environments: searching for potential industrial uses within extremophile plants. Ind. Crop. Prod. 94, 299–307. doi: 10.1016/j.indcrop.2016.08.040
Manolaraki, F. (2011). Propriétés Anthelminthiques du Sainfoin (Onobrychis viciifoliae): Analyse des Facteurs de Variations et du Rôle des Composés Phénoliques Impliqués. Ph.D. thesis. Toulouse: Institut National Polytechnique de Toulouse, 185.
Manolaraki, F., Sotiraki, S., Stefanakis, A., Skampardonis, V., Volanis, M., and Hoste, H. (2010). Anthelmintic activity of some Mediterranean browse plants against parasitic nematodes. Parasitology. 137, 685–696. doi: 10.1017/S0031182009991399
Mansfield, L. S., Gamble, H. R., and Fetterer, R. H. (1992). Characterization of the eggshell of Haemonchus contortus – I. structural components. Comp. Biochem. Physiol. B. 103, 681–686. doi: 10.1016/0305-0491(92)90390-d
Mengistu, G., Hoste, H., Karonen, M., Salminen, J.-P., Hendriks, W. H., and Pellikaan, W. F. (2017). The in vitro anthelmintic properties of browse plant species against Haemonchus contortus is determined by the polyphenol content and composition. Vet. Parasitol. 237, 110–116. doi: 10.1016/j.vetpar.2016.12.020
Molan, A. L., Meagher, L. P., Spencer, P. A., and Sivakumaran, S. (2003). Effect of flavan-3-ols on in vitro egg hatching, larval development and viability of infective larvae of Trichostrongylus colubriformis. Int. J. Parasitol. 33, 1691–1698. doi: 10.1016/s0020-7519(03)00207-8
Mueller-Harvey, I., Bee, G., Dohme-Meier, F., Hoste, H., Karonen, M., and Kölliker, R. (2018). Benefits of condensed tannins in forage legumes fed to ruminants: importance of structure, concentration, and diet composition. Crop Sci. 58:861. doi: 10.2135/cropsci2017.06.0369
Oliveira, M., Hoste, H., and Custódio, L. (2021c). A systematic review on the ethnoveterinary uses of mediterranean salt-tolerant plants: exploring its potential use as fodder, nutraceuticals or phytotherapeutics in ruminant production. J. Ethnopharmacol. 267:113464. doi: 10.1016/j.jep.2020.113464
Oliveira, M., Rodrigues, M. J., Neng, N. R., Nogueira, J. F., Bessa, R., and Custódio, L. (2021a). Seasonal variations of the nutritive value and phytotherapeutic potential of Cladium mariscus L. (Pohl.) targeting ruminant’s production. Plants 10:556. doi: 10.3390/plants10030556
Oliveira, M., Sprengel Lima, C., Ketavong, S., Llorent-Martínez, E., Hoste, H., and Custódio, L. (2021b). Disclosing the bioactive metabolites involved in the in vitro anthelmintic effects of salt-tolerant plants through a combined approach using PVPP and HPLC-ESI-MSn. Sci. Rep. 11:24303. doi: 10.1038/s41598-021-03472-9
Paolini, V., Fouraste, I., and Hoste, H. (2004). In vitro effects of three woody plant and sainfoin extracts on 3rd-stage larvae and adult worms of three gastrointestinal nematodes. Parasitology 129, 69–77. doi: 10.1017/s0031182004005268
Pereira, C. G., Locatelli, M., Innosa, D., Cacciagrano, F., Polesná, L., Santos, T. F., et al. (2019). Unravelling the potential of the medicinal halophyte Eryngium maritimum L.: in vitro inhibition of diabetes-related enzymes, antioxidant potential, polyphenolic profile and mineral composition. South Afr. J. Bot. 120, 204–212. doi: 10.1016/j.sajb.2018.06.013
Quijada, J., Fryganas, C., Ropiak, H. M., Ramsay, A., Mueller-Harvey, I., and Hoste, H. (2015). Anthelmintic activities against Haemonchus contortus or Trichostrongylus colubriformis from small ruminants are influenced by structural features of condensed tannins. J. Agric. Food Chem. 63, 6346–6354. doi: 10.1021/acs.jafc.5b00831
Rodrigues, M. J., Soszynski, A., Martins, A., Rauter, A. P., Neng, N. R., Nogueira, J. M. F., et al. (2015). Unravelling the antioxidant potential and the phenolic composition of different anatomical organs of the marine halophyte Limonium algarvense. Ind Crop Prod. 77, 315–322. doi: 10.1016/j.indcrop.2015.08.061
Romero, N., Areche, C., Cubides-Cárdenas, J., Escobar, N., García-Beltrán, O., Simirgiotis, M. J., et al. (2020). In vitro anthelmintic evaluation of Gliricidia sepium, Leucaena leucocephala, and Pithecellobium dulce: fingerprint analysis of extracts by UHPLC-Orbitrap mass spectrometry. Molecules. 25:3002. doi: 10.3390/molecules25133002
Rose Vineer, H., Morgan, E. R., Hertzberg, H., Bartley, D. J., Bosco, A., Charlier, J., et al. (2020). Increasing importance of anthelmintic resistance in European livestock: creation and meta-analysis of an open database. Parasite. 27:69. doi: 10.1051/parasite/2020062
Santos, F. O., Cerqueira, A. P. M., Branco, A., Batatinha, M. J. M., and Botura, M. B. (2019). Anthelmintic activity of plants against gastrointestinal nematodes of goats: a review. Parasitology 146, 1233–1246. doi: 10.1017/S0031182019000672
Soldera-Silva, A., Syfried, M., Campestrini, L. H., Zawadzki-Baggio, S. F., Minho, A. P., Molento, M. B., et al. (2018). Assessment of anthelmintic activity and bio-guided chemical analysis of Persea americana seed extracts. Vet. Parasitol. 251, 34–43. doi: 10.1016/j.vetpar.2017.12.019
Spiegler, V., Liebau, E., and Hensel, A. (2017). Medicinal plant extracts and plant-derived polyphenols with anthelmintic activity against intestinal nematodes. Nat. Prod. Rep. 34, 627.–643. doi: 10.1039/c6np00126b
Sprengel Lima, C., Pereira, M. H., Gainza, Y. A., Hoste, H., Regasini, L. O., and Chagas, A. C. S. (2021). Anthelmintic effect of Pterogyne nitens (Fabaceae) on eggs and larvae of Haemonchus contortus: analyses of structure-activity relationships based on phenolic compounds. Ind. Crop. Prod. 164:113348. doi: 10.1016/j.indcrop.2021.113348
Tattini, M., Calardi, C., Pinelli, P., Massai, R., Remorini, D., and Agati, G. (2004). Differential accumulation of flavonoids and hydroxycinnamates in leaves of Ligustrum vulgare under excess light and drought stress. New Phytologist. 163, 547–561. doi: 10.1111/j.1469-8137.2004.01126.x
Trabelsi, N., Falleh, H., Jallali, I., Daly, A. B., Hajlaoui, H., Smaoui, A., et al. (2012). Variation of phenolic composition and biological activities in Limoniastrum monopetalum L. organs. Acta Physiol. Plant. 34, 87–96. doi: 10.1007/s11738-011-0807-8
Vargas-Magaña, J. J., Torres-Acosta, J. F. J., Aguilar-Caballero, A. J., Sandoval-Castro, C. A., Hoste, H., and Chan-Pérez, J. I. (2014). Anthelmintic activity of acetone–water extracts against Haemonchus contortus eggs: interactions between tannins and other plant secondary compounds. Vet. Parasitol. 206, 322–327. doi: 10.1016/j.vetpar.2014.10.008
Waridel, P., Wolfender, J.-L., Ndjoko, K., Hobby, K. R., Major, H. J., and Hostettmann, K. (2001). Evaluation of quadrupole time-of-flight tandem mass spectrometry and ion-trap multiple-stage mass spectrometry for the differentiation of C-glycosidic flavonoid isomers. J. Chrom. 926, 29–41. doi: 10.1016/s0021-9673(01)00806-8
Keywords: halophytes, salt tolerant plants, anthelmintic, polyphenols, gastrointestinal nematodes, small ruminants
Citation: Oliveira M, Lima CS, Llorent-Martínez EJ, Hoste H and Custódio L (2022) Impact of Seasonal and Organ-Related Fluctuations on the Anthelmintic Properties and Chemical Profile of Cladium mariscus (L.) Pohl Extracts. Front. Plant Sci. 13:934644. doi: 10.3389/fpls.2022.934644
Received: 06 May 2022; Accepted: 06 June 2022;
Published: 23 June 2022.
Edited by:
Jens Rohloff, Norwegian University of Science and Technology, NorwayReviewed by:
Agustín Olmedo-Juárez, Instituto Nacional de Investigaciones Forestales, Agrícolas y Pecuarias (INIFAP), MexicoKamel Msaada, Center of Biotechnology of Borj Cedria (CBBC), Tunisia
Copyright © 2022 Oliveira, Lima, Llorent-Martínez, Hoste and Custódio. This is an open-access article distributed under the terms of the Creative Commons Attribution License (CC BY). The use, distribution or reproduction in other forums is permitted, provided the original author(s) and the copyright owner(s) are credited and that the original publication in this journal is cited, in accordance with accepted academic practice. No use, distribution or reproduction is permitted which does not comply with these terms.
*Correspondence: Luísa Custódio, lcustodio@ualg.pt