- 1Department of Agronomy, College of Agriculture, University of Sargodha, Sargodha, Pakistan
- 2Future Regions Research Centre, Federation University Australia, Mount Helen, VIC, Australia
- 3Department of Agronomy, University of Agriculture Faisalabad, Faisalabad, Pakistan
- 4College of Agriculture, BZU, Bahadur Sub Campus, Layyah, Pakistan
- 5College of Agriculture, Fujian Agriculture and Forestry University, Fuzhou, China
- 6Department of Agronomy, Bahauddin Zakariya University, Multan, Pakistan
- 7Department of Plant Physiology, Institute of Biology, Warsaw University of Life Sciences SGGW, Warsaw, Poland
- 8Institute of Technology and Life Sciences, National Research Institute, Raszyn, Poland
- 9Department of Forestry, College of Agriculture, University of Sargodha, Sargodha, Pakistan
- 10Department of Bioenegineering, West Pomerania, University of Technology Szczecin, Szczecin, Poland
- 11Plant Production Department (Horticulture-Medicinal and Aromatic Plants), Faculty of Agriculture (Saba Basha), Alexandria University, Alexandria, Egypt
- 12Twój Swiat Jacek Mojski, Lukow, Poland
- 13Fundacja Zielona Infrastruktura, Lukow, Poland
Rising atmospheric CO2 concentrations are known to influence the response of many plants under drought. This paper aimed to measure the leaf gas exchange, water use efficiency, carboxylation efficiency, and photosystem II (PS II) activity of Datura stramonium under progressive drought conditions, along with ambient conditions of 400 ppm (aCO2) and elevated conditions of 700 ppm (eCO2). Plants of D. stramonium were grown at 400 ppm and 700 ppm under 100 and 60% field capacity in a laboratory growth chamber. For 10 days at two-day intervals, photosynthesis rate, stomatal conductance, transpiration rate, intercellular CO2 concentration, water use efficiency, intrinsic water use efficiency, instantaneous carboxylation efficiency, PSII activity, electron transport rate, and photochemical quenching were measured. While drought stress had generally negative effects on the aforementioned physiological traits of D. stramonium, it was found that eCO2 concentration mitigated the adverse effects of drought and most of the physiological parameters were sustained with increasing drought duration when compared to that with aCO2. D. stramonium, which was grown under drought conditions, was re-watered on day 8 and indicated a partial recovery in all the parameters except maximum fluorescence, with this recovery being higher with eCO2 compared to aCO2. These results suggest that elevated CO2 mitigates the adverse growth effects of drought, thereby enhancing the adaptive mechanism of this weed by improving its water use efficiency. It is concluded that this weed has the potential to take advantage of climate change by increasing its competitiveness with other plants in drought-prone areas, suggesting that it could expand into new localities.
Introduction
Since the Industrial Revolution, atmospheric CO2 has been increasing and is predicted to reach 800 ppm at the end of this century (Valone, 2021). Elevated CO2 (eCO2) stimulates global warming, causing rapid changes in the climate of the earth, affecting variations in temperature, precipitation amounts and intensity patterns (Skendžić et al., 2021). Moreover, it has been reported that global warming acts to cause deficits in atmospheric vapor pressure, resulting in drought stress in plants (Song et al., 2021). This rapid climate change may also alter the geographical ranges of species (Skendžić et al., 2021), and it is, therefore, predicted that species that can tolerate warmer and drier weather will have competitive benefits over non-tolerant species (Karimizadeh et al., 2021). Changes in precipitation patterns will also affect the success of species invasion and the nature of ecosystems in general. It has been reported that crop species under eCO2 act to enhance photosynthesis when other factors like light, water, and nutrients are not limiting (Boucher et al., 2009; AbdElgawad et al., 2015; AbdElgawad et al., 2016; Cruz et al., 2018; Alba et al., 2019). Dusenge et al. (2019) concluded that the current ambient CO2 concentration is a limiting factor to growth, so an increase in CO2 concentration clearly has the potential to increase the growth and yield of plants.
Elevated CO2 increases the activity rate of Rubisco, which results in increased plant growth (López-Calcagno et al., 2020). This will be beneficial for agriculture because of greater crop productivity, but the growth of weeds, especially invasive species, will also be enhanced (Wu et al., 2017). Physiologically, elevated CO2 increases biomass production by reducing water loss through the stomata, in conjunction with an increase in root growth and improved root structure (Li et al., 2020). Stomatal conductance is based on CO2, which reduces the transpiration rate of crop species grown under elevated CO2 with ample water supplies (Kimball, 2016). However, the response of stomata to eCO2 is dependent on the interactive effect of elevated CO2 and soil water content (Xu et al., 2016). It is well documented that an increase in CO2 concentration causes partial stomatal closure, which reduces leaf transpiration and increases net carbon assimilation (Dai, 2013; AbdElgawad et al., 2015; Cruz et al., 2018).
Elevated CO2 increases the net assimilation rate by up to 30%, which can lead to a significant increase in dry matter and yield of crops (Robredo et al., 2007; Cruz et al., 2018; Pan et al., 2018). Wheat crops grown under 550 ppm CO2 concentration have been shown to increase their yield by 15%, the canopy temperature by 0.85°C, and have reduced their evapotranspiration by 13%, consequently increasing the water use efficiency by 18%. Rising levels of CO2 have, therefore, potential beneficial effects on the growth and yield of plants, especially in areas where drought causes crop failure (Jin et al., 2019). According to AbdElgawad et al. (2016), eCO2 has been shown to mitigate the stress impact of drought, and they quoted examples of barley and alfalfa crop growth reduction under drought stress (Zeid and Shedeed, 2006; Robredo et al., 2007). However, despite these beneficial effects on crops, the rising level of atmospheric CO2 may have negative consequences for yield losses due to weed-crop competition (Ziska et al., 2019). Observations have shown that while vegetative growth of C3 crops is favored over C4 weeds under elevated CO2 (Ziska and Caulfield, 2000), C3 weeds may be favored over C3 crops (Ziska et al., 2019). It has recently been suggested that the effect of drought may be overcome by some compensatory mechanism within the plants (Qi et al., 2021). Numerous reports showed that plants that were grown under eCO2 dried more slowly as water was withheld due to lower stomatal conductance and transpiration rate (Yan et al., 2017; Dikšaitytė et al., 2019; Pastore et al., 2020). It is postulated that the benefit gained by weeds from eCO2 under drought conditions is linked to physiological traits like chlorophyll content, gas exchange, water use efficiency, and PSII activity of plants (Li et al., 2019). Elevated CO2 increased the growth and reproduction of both indigenous and non-indigenous weeds, which has resulted in an enhancement of their competitive ability. This outcome supports the hypothesis of Blossey and Notzold (1995), who hypothesized that there is an evolution of increased competitive ability in invasive plants. It is well documented that invasive species affect the growth, development, and reproduction of native species by altering the ecosystem and introducing direct competition for essential resources (Werner et al., 2008). Although gaining an understanding of the effect of increased atmospheric CO2 combined with drought on the growth and yield of crops has a priority over weed studies (Nguyen et al., 2017), weed infestation is, nevertheless, an important impediment to crop productivity and needs to be investigated in its own right.
Datura stramonium, commonly known as thorn apple or Jimson weed, is an invasive C3 weed species belonging to the family Solanaceae (Chadha et al., 2020). It is grown in subtropical and temperate regions worldwide (Chadha et al., 2020). It has fast seedling growth with a short vegetative stage and is characterized by indeterminate growth habits. Broad leaves and sympodial branches allow this weed to shade the surrounding areas and increase its competitive ability. It is a common weed found in soybean, potato, tomato, and tobacco crops. D. stramonium has narcotic properties and contains tropane alkaloids, mainly scopolamine, hyoscyamine, and atropine chemicals, which are known to be poisonous for humans, cattle, and horses (Baloch et al., 2017). These characters restrict the agricultural use of this species, but some studies have shown the potential of D. stramonium for bio-oil production (Aysu and Durak, 2015; Durak and Aysu, 2016). Therefore, it is important to develop a good understanding of how D. stramonium is likely to respond to changing environments, indicating that the lack of scientific literature regarding the effect of eCO2 concentration on the physiological processes of D. stramonium is a significant problem. The current study focuses on an understanding of the regulation of gas exchange, photosynthetic efficiency of PSII, and water use efficiency of D. stramonium at 400 and 700 ppm CO2 grown under normal irrigation and drought conditions, and reflects on the implications of these results for their growth and invasive characteristics.
Materials and methods
Seed collection
Seeds of D. stramonium were obtained by removing the heads from mature plants, and subsequently drying and threshing them to remove extraneous material. These heads were collected from more than 100 different plants in Ballarat, Victoria, Australia. The cleaned seeds were stored in a dried amber glass bottle at 19°C in the seed ecology laboratory of Federation University, Mt. Helen, Australia, until used in the experiment.
Condition of the experiment
Experiments were conducted at Federation University (37°37.39°S, 143°53.27°E) in two CO2 chambers (2.1 m length, 2.1 width, and 2.0 m height) (Steridium Pty. Ltd., Brendale, Qld, Australia). One CO2 chamber was set at 400 ppm CO2 concentration (aCO2), while the other was set at 700 ppm CO2 concentration (eCO2). The average chamber temperature was maintained at 22/18°C day/night alternating temperature with 60% humidity. Twenty plastic pots (13 cm wide and 14 cm high) were filled, each with 1.2 kg of a 2:1 mixture of garden soil and a commercially available potting mixture. Three seeds of D. stramonium were sown in the center of each pot. Out of the twenty pots, 10 randomly selected samples were kept in the cabinet maintained at an ambient CO2 level (400 ppm), and the remaining 10 pots were kept in the second cabinet maintained at an elevated CO2 level (700 ppm). The pots were watered daily, and seedlings were thinned at the four-leaf stage, leaving one seedling in each pot. Two moisture levels (well-watered and drought) were also maintained in each CO2 chamber. The well-watered treatments were maintained at 100% field capacity and drought treatments at 60% field capacity. Drought treatment commenced 25 days after sowing. These water regimes were selected as different plant species showed variable responses to eCO2 concentrations under drought conditions. Water holding capacity was determined according to Bajwa et al. (2019). Briefly, 10 kg of the soil was placed into three pots and saturated with tap water. The pot surface was covered with black plastic, and the pots were allowed to drain for 48 h to determine the water hole. After this time, the plastic sheet was removed and three soil samples (each weighing 300 g) were taken from the mid position of each pot. These samples were weighed (wet weight of soil, A) before being oven-dried (90°C for 72 h) and reweighed (dry weight of soil, B). The field capacity was then calculated by the formula (A − B) × 100/B. The 60% field capacity was determined based on that fraction of the water holding capacity.
Of the 10 pots in each chamber, half were subjected to well-watered conditions and the remaining half were subjected to a drought regime. The pots were weighed to maintain an accurate amount of water field capacity levels, noting that the weight of the growing plant was much smaller than that of the soil in the pot. There were five replications (one pot for each replication) for each treatment and each replication consisted of one plant. In drought treatments, water was withheld until Day 8 in both CO2 chambers, after which drought treatment pots were re-watered to investigate the recovery response of the D. stramonium plants. The amount of water added was calculated based on pot weight, and 60% field capacity was maintained till Day 10. In the well-watered treatments, water was added on alternate days.
Gas exchange and fluoresence
To evaluate the effect of CO2 and drought on physiological parameters, the LI-COR portable infrared CO2 gas analyzer was used (LI-6400 XT portable photosynthesis system, LI-COR, Biosciences, Lincoln, Nebraska, USA). Measurements were recorded with the following adjustments by LI-COR: The block temperature was set at 20°C, the photosynthetic photon flux density (PPFD) was 1,000 µmol m−2 s−1 with a red-blue light source, the leaf cuvette area was set at 2 cm2 and the flow rate was adjusted to 500 µmol m−2 s−1. The light conditions in the chamber were set for 12 h and 12 h dark. The mean value of VPD in the leaf cuvette was 1.75 ± 0.03 kPa. The CO2 concentration in the chamber was noted before each measurement, and adjusted to within ±10 ppm of the stated level. On alternate days, net photosynthesis rate, stomatal conductance, transpiration rate, and intercellular CO2 concentration were measured and the parameters were started to measure at 9:30 am. Water use efficiency was calculated by dividing the net photosynthesis rate with the transpiration rate; intrinsic water use efficiency was calculated by dividing the net photosynthesis rate with stomatal conductance; and instantaneous carboxylation efficiency was calculated by dividing the net photosynthesis rate by the intercellular CO2 concentration. Photosystem II (PSII) activity, such as minimum fluorescence, maximum fluorescence, the effective quantum efficiency of PSII, photochemical efficiency of PSII, photochemical quenching, and photosynthetic electron transport rate, were calculated according to the methods described by Maxwell and Johnson (2000). Maximum fluorescence was determined by applying a saturating light pulse of 7,000 µmol m−2s−1. The effective quantum efficiency of PSII was measured under actinic light of 180 µmol m−2s−1.
The effective quantum efficiency of PSII was calculated as
Where ΦPSII is the effective quantum efficiency of PSII, is the maximum fluorescence and Fs is the steady state fluorescence prior to the flash.
Photochemical efficiency of PSII ( ) was determined in terms of efficiency of energy harvesting by oxidized PSII using the following equation
Where is the maximum fluorescence and is the minimum fluorescence.
The photochemical quenching was computed from
Where Fs is the steady-state fluorescence and is the minimum fluorescence.
Statistical analysis
Data for gas exchange parameters were presented in graphs along with the standard error of each mean, and the graphs were prepared using the SigmaPlot 11 software. Regression analysis was performed on the data of photosynthesis rate, stomatal conductance, and transpiration rate under 8 days of drought conditions. To investigate the effects of time of observation (time), water conditions (water), and CO2 levels (CO2), physiological parameters were analyzed with Statisix 8.1 using three-factor ANOVA. All the main effects and two- and three-way interactions were examined using Tukey’s HSD test at a 5% probability level.
Results
Gas exchange parameters
Photosynthesis activities were recorded for D. stramonium under two moisture conditions (well-watered and drought) at 400 ppm and 700 ppm CO2 concentrations (Figure 1A). These measurements were recorded for 10 days at two-day intervals. It is depicted in Figure 1A that elevated CO2 increased the photosynthesis rate by 2–3 µmol CO2 m−2 s−1 when compared to that with ambient CO2. However, the photosynthesis rate was variable under drought conditions with the two CO2 concentrations. Under drought conditions, the photosynthesis rate under aCO2 decreased from 14 to 1 µmol CO2 m−2 s−1 at Day 8, while with eCO2 this reduction was less, being 2.8 µmol CO2 m−2 s−1 photosynthesis rate at Day 8. This clearly indicates that eCO2 mitigated the adverse effects of drought on D. stramonium. After the measurement, water was added to the drought-treated plants to investigate the recovery response. The photosynthesis rate was seen to recover, showing a higher rate with eCO2 compared to that under aCO2. Figure 1 showed that the photosynthesis rate increased from 2.86 to 8.4 µmol CO2 m−2 s−1 with eCO2, while the corresponding recovery under aCO2 was 5.4 µmol CO2 m−2 s−1. The ANOVA table indicated that CO2 (p<0.001), time (p<0.001), water (p<0.001), CO2 × time (p<0.001), and time × water (p<0.001) interactions were significant for photosynthesis rate, while the interactions of CO2 × water and CO2 × time × water were non-significant for this parameter.
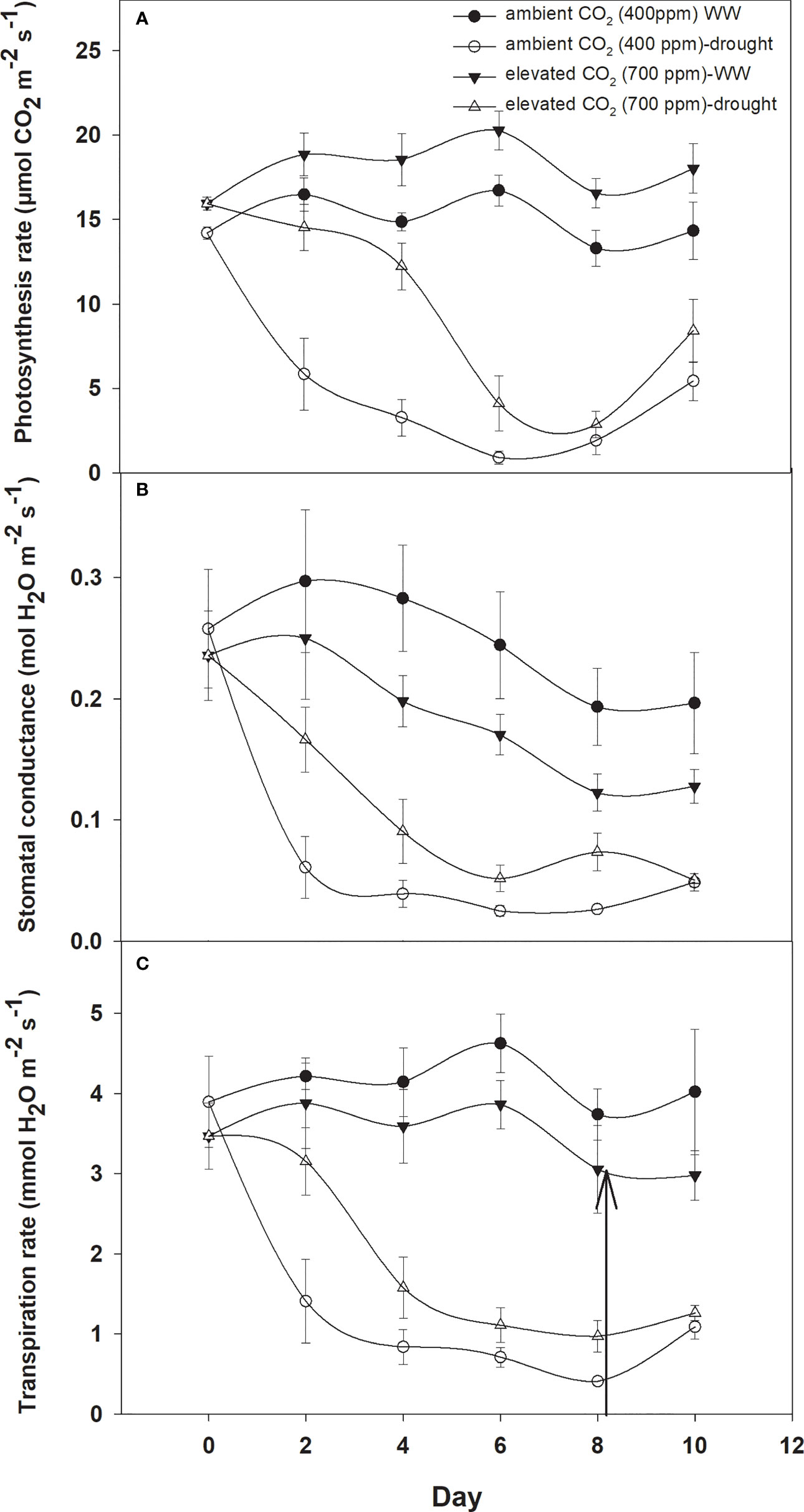
Figure 1 Effect of CO2 concentrations and moisture conditions on (A) photosynthesis rate, (B) stomatal conductance, and (C) transpiration rate, of Datura stramonium. The vertical line on the data point represents the standard error of the mean and the vertical arrow line after Day 8 represents the addition of water in the drought treatments. WW, well-watered.
When D. stramonium was grown under well-watered conditions, the stomatal conductance was higher with aCO2 compared to that with eCO2 (Figure 1B). Moreover, the stomatal conductance under drought conditions showed variable responses to both CO2 concentrations (400 ppm and 700 ppm). The decline in stomatal conductance due to drought conditions was higher with aCO2 and reached 0.03 mol H2O m−2 s−1 on Day 8. Stomatal conductance with eCO2 on Day 8 was 0.07 mol H2O m−2 s−1. Re-watering the D. stramonium sample after the Day 8 measurement did not significantly improve the stomatal conductance and recorded only 0.05 mol H2O m−2 s−1 conductance. The ANOVA results in Table 1 showed that time (p<0.001), water (p<0.001), CO2 × water (p<0.001), and time × water (p<0.001) were all significant for stomatal conductance. The rest of the effects were non-significant for this parameter.
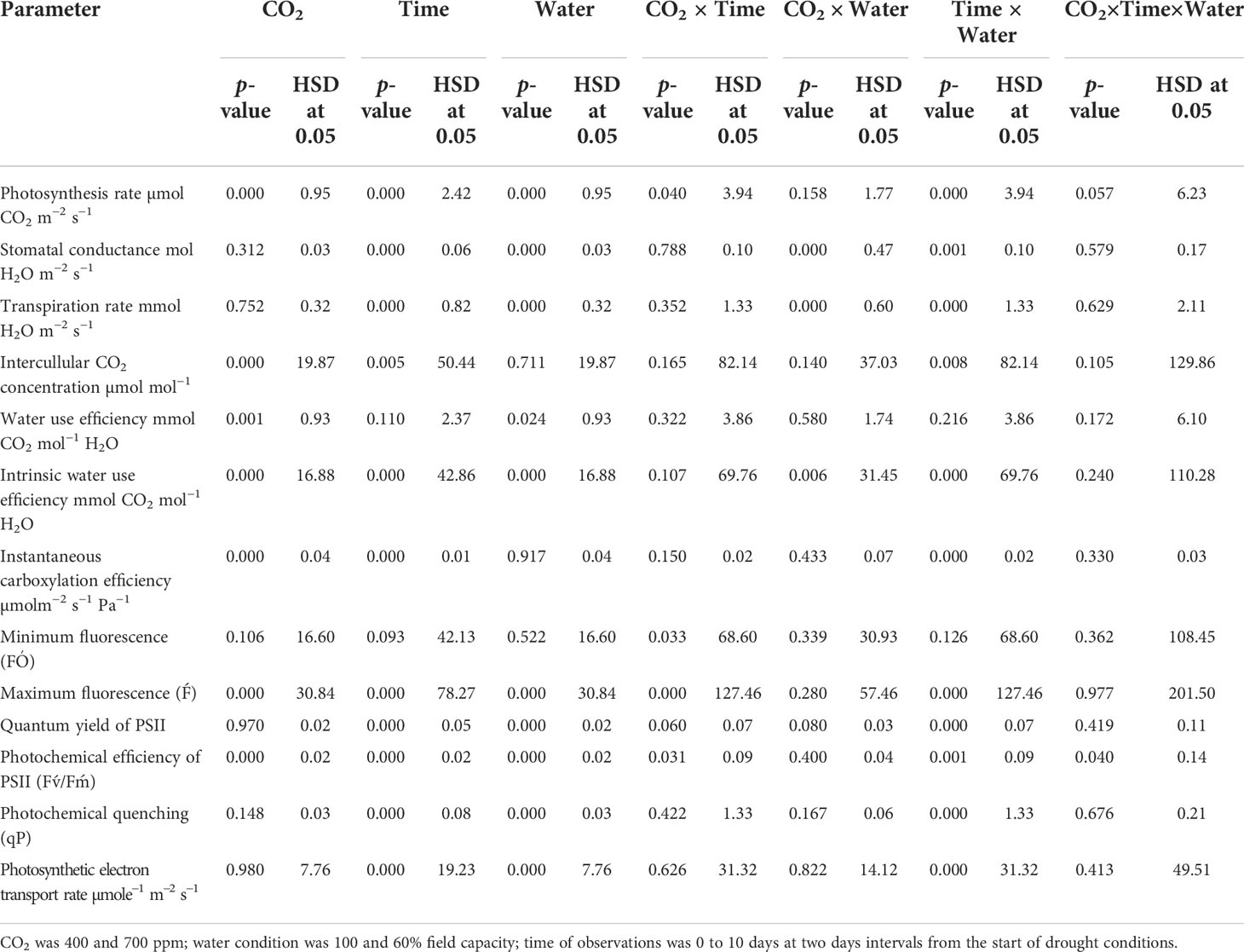
Table 1 Summary of the analysis variance for physiological parameters of Datura stramonium in response to CO2 levels, water condition and time of observation.
D. stramonium exhibited a significant difference in transpiration rate under eCO2 and aCO2 when grown under well-watered or drought conditions (Figure 1C). Elevated CO2 reduced the transpiration rate compared to that with aCO2 under well-watered conditions. Under this water regime, transpiration rate with aCO2 was recorded up to 4.6 mmol H2O m−2 s−1 while it was 3.8 mmol H2O m−2 s−1 with eCO2. Drought stress resulted in a progressive decline in transpiration rate under both concentrations of CO2 until Day 8, but this decline was significantly less with eCO2. The transpiration rate was 0.97 mmol H2O m−2 s−1 at Day 8 with eCO2, whereas it was 0.41 mmol H2O m−2 s−1 with aCO2. Moreover, recovery in transpiration was higher with eCO2 upon re-instating the water to drought treatments (Figure 1C). The main effects of time (p<0.001) and water (p<0.001) and the interaction of CO2 × water (p<0.001) and time × water (p<0.001) were significant for the transpiration rate.
Elevated CO2 concentration increased the intercellular CO2 concentration compared to that with aCO2 (Figure 2). D. stramonium grown under well-water conditions recorded about 430–586 µmol mol−1 intercellular CO2 concentration with aCO2 compared with eCO2, where it was 249–304 µmol mol−1. Drought conditions were seen to have drastic effects on intercellular CO2 concentration, and this was increased with an increase in drought duration. The ANOVA values in Table 1 showed that the main effects (CO2 and time) and interaction of time × water were significant for intercellular CO2 concentration (p<0.009).
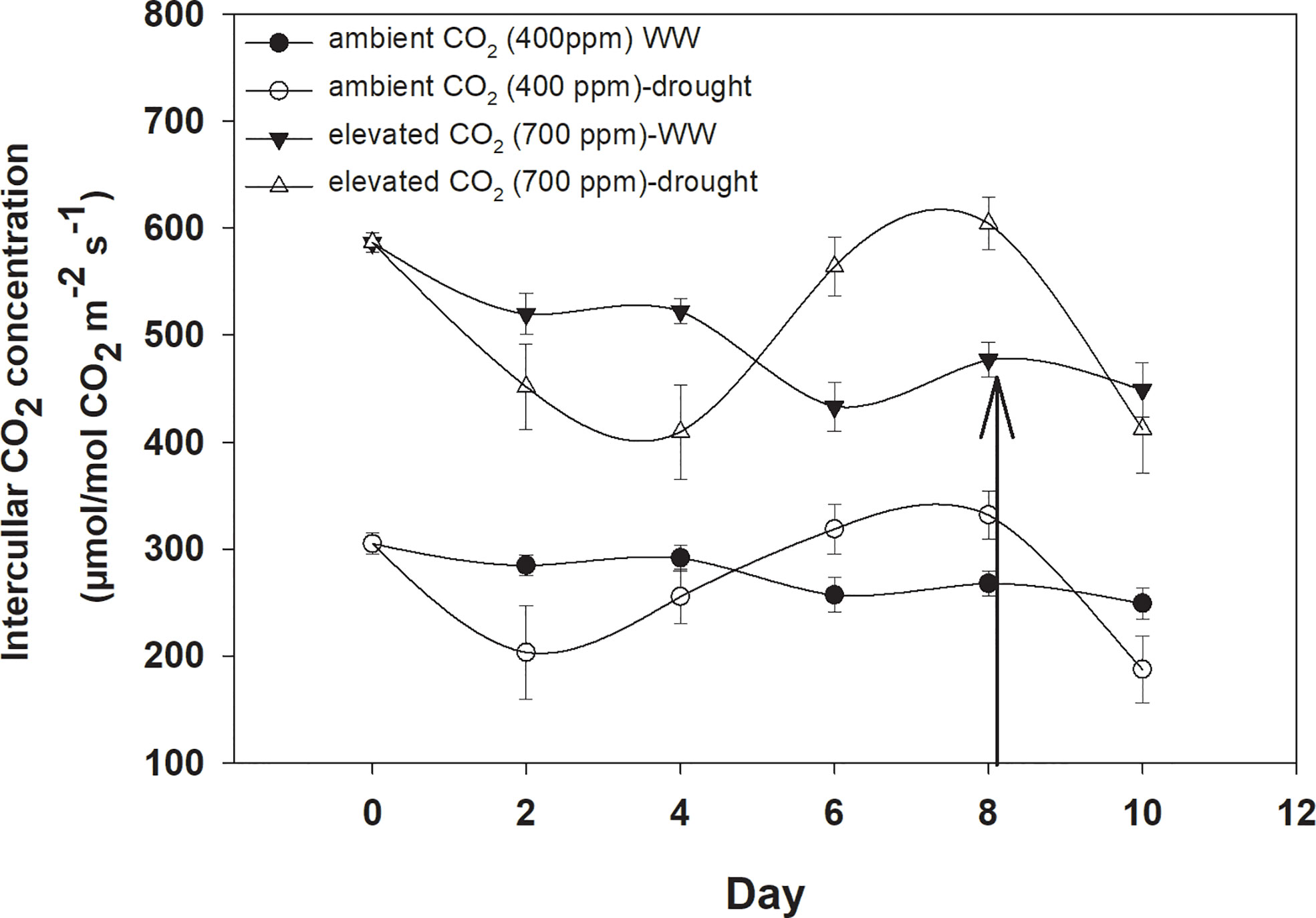
Figure 2 Effect of CO2 concentrations and moisture conditions on intercellular CO2 concentration of Datura stramonium. The vertical line on the data point represents the standard error of the mean and the vertical arrow line after Day 8 represents the addition of water in the drought treatments. WW, well-watered.
Water use efficiency
Under well-watered conditions, water use efficiency was 5–6 with eCO2 compared to that of aCO2, which recorded 3–4 mmol CO2 mol−1 H2O (Figure 3A). The response of D. stramonium towards water use efficiency under drought conditions was variable, with water use efficiency decreasing with an increase in drought duration until Day 8. The decline in water use efficiency with eCO2, was lower than that with aCO2. Water use efficiency was reduced from 4 to 1.6 mmol CO2 per mol of water under eCO2 whereas this decline was up to 2.1 mmol CO2 per mol of water with aCO2 concentration under drought conditions. The re-instatement of water in drought treatment at Day 8 led to a significant recovery in water use efficiency, reaching 4.8 mmol CO2 mol−1 H2O in the treatment where a 400 ppm CO2 concentration was maintained. By comparison, water use efficiency reached 5.7 mmol CO2 mol−1 H2O when the CO2 concentration was maintained at 700 ppm. Statistical analysis of water use efficiency in Table 1 showed that the mean effects of CO2 (p<0.001) and water (p<0.024) were significant, whereas the main effects of time and all interactions were non-significant.
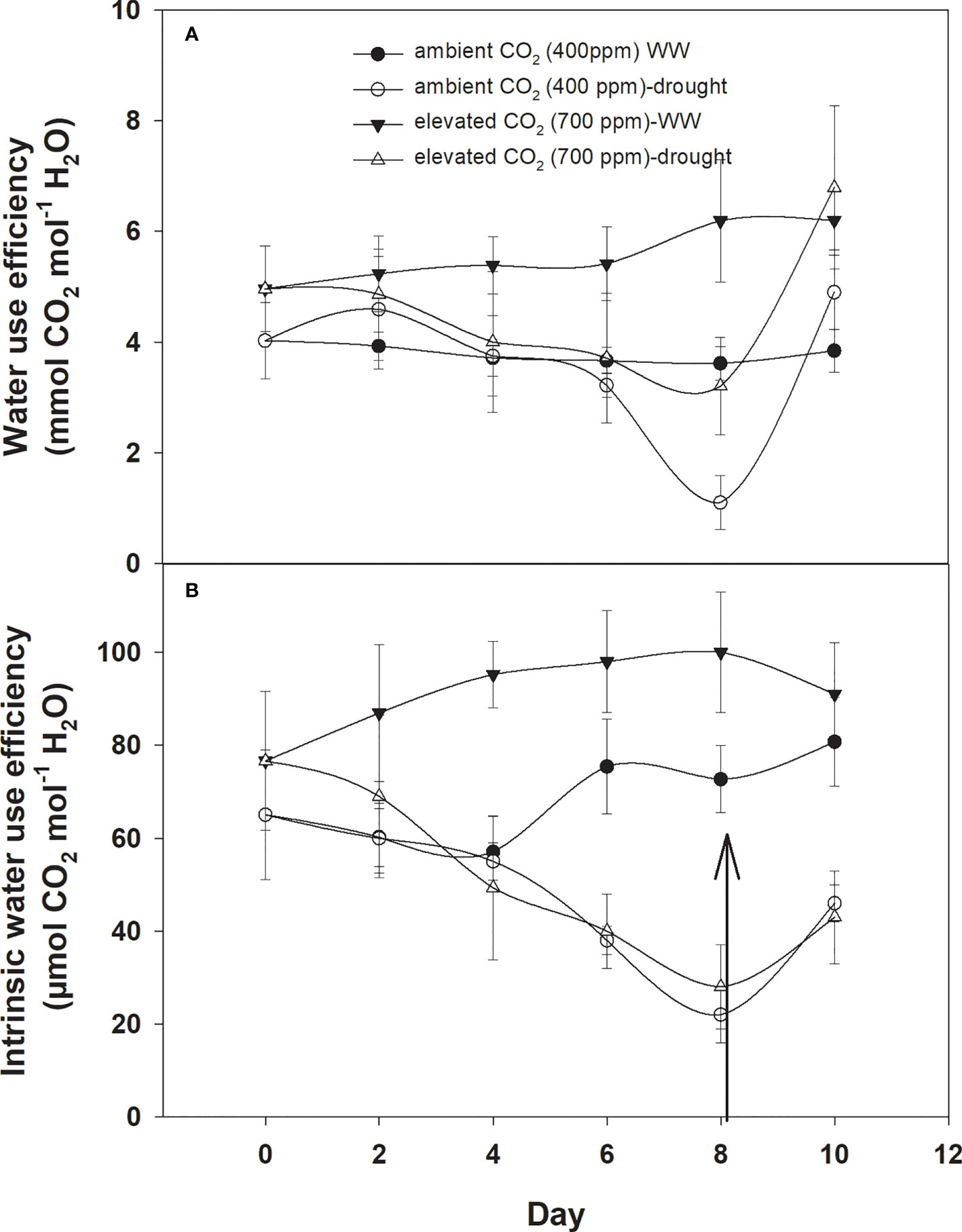
Figure 3 Effect of CO2 concentrations and moisture conditions on (A) water use efficiency and (B) intrinsic water use efficiency of Datura stramonium. The vertical line on the data point represents the standard error of the mean and the vertical arrow line after Day 8 represents the addition of water in the drought treatments. WW, well-watered.
Similar to water use efficiency, the intrinsic water use efficiency of D. stramonium was higher with eCO2 compared to that with aCO2 under well-watered conditions (Figure 3B), while the intrinsic water use efficiency under drought conditions with aCO2 or eCO2 was reduced at variable rates. A progressive decline in intrinsic water use efficiency under eCO2 in drought treatment conditions started at Day 6 and reached a minimum (49 mmol CO2 per mol of water) at Day 8. With aCO2 in drought conditions, the progressive decline started on Day 4 and reached 31 mmol CO2 per mol of water on Day 8. The addition of water at this date significantly recovered intrinsic water use efficiency. Elevated CO2 and aCO2 resulted in 162.2 and 110.5 mmol CO2 per mol of water as their intrinsic water use efficiency, respectively. Data from ANOVA showed that the main effects of CO2 (p<0.001), time (p<0.001), and water (p<0.0001), plus interaction of CO2 × water (p = 0.006), and time × water (p<0.001), were significant for the intrinsic water use efficiency of D. stramonium. The three-way interaction was non-significant for this parameter.
Carboxylation efficiency
Under well-watered conditions, elevated CO2 caused a significant reduction in instantaneous carboxylation efficiency when compared with aCO2 (Figure 5). The instantaneous carboxylation efficiency values were between 0.05 and 0.07 µmol m−2 s−1 Pa−1 under eCO2, whereas it was 0.03–0.04 µmol m−2 s−1 Pa−1 under aCO2. Drought conditions resulted in a variable response for instantaneous carboxylation efficiency for D. stramonium. Drought caused reductions in instantaneous carboxylation efficiency with an increase in the duration of drought, but this decline was much more progressive under aCO2 compared to that with eCO2. Figure 4 shows that the instantaneous carboxylation efficiency decline started at the second day of drought stress, compared with the decline starting at Day 4 with eCO2 under drought conditions. The addition of water at Day 8 to drought treatments increased the instantaneous carboxylation efficiency under both CO2 concentrations but instantaneous carboxylation efficiency reached 0.04 µmol m−2 s−1 Pa−1 with aCO2 after water addition to the drought treatment. The ANOVA results presented in Table 1 showed that the main effects of CO2 (p<0.001), time (p<0.000), water (p<0.000), and interaction of time × water (p<0.000) were significant for instantaneous carboxylation efficiency of D. stramonium, whereas the rest of the interactions were non-significant.
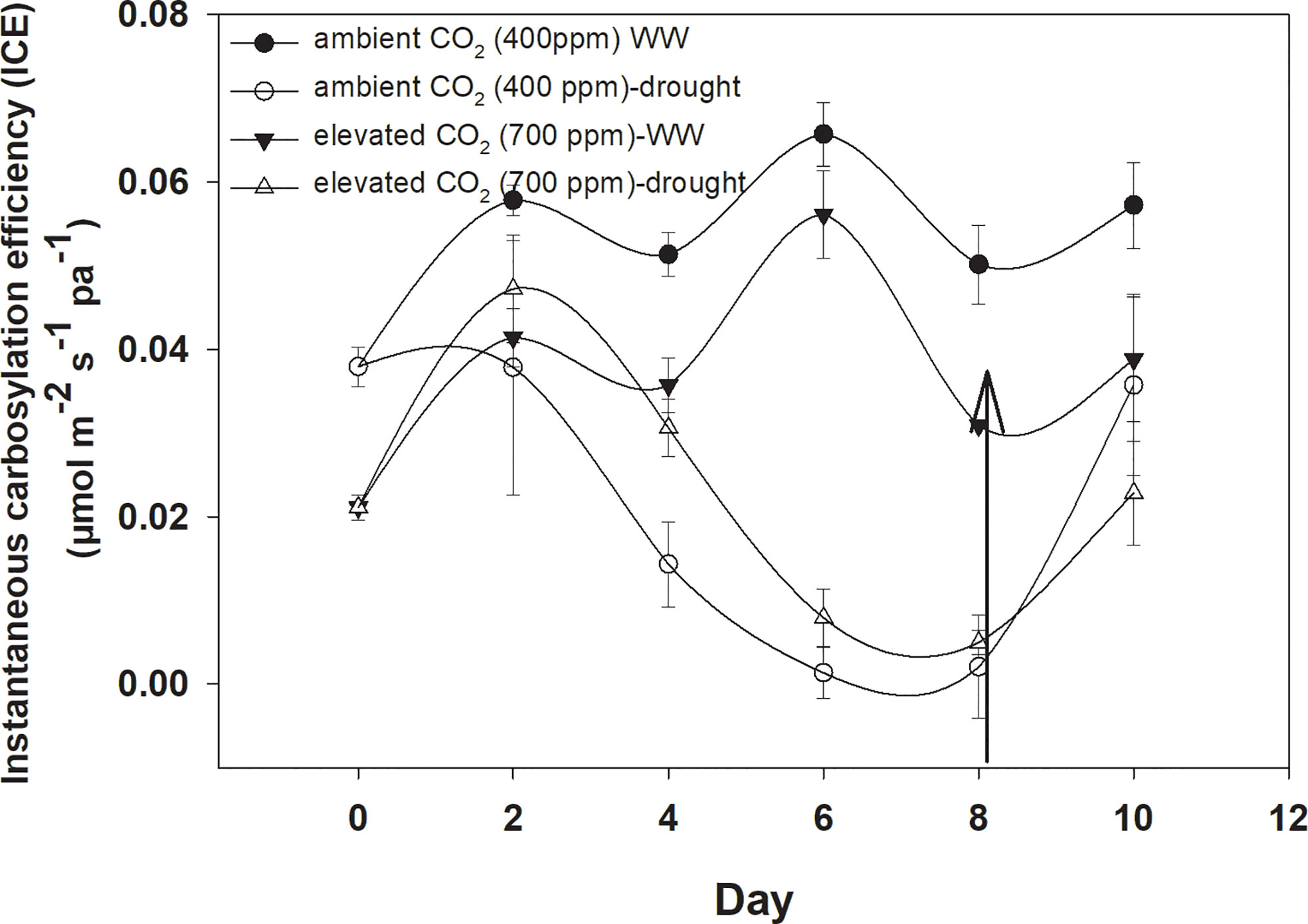
Figure 4 Effect of CO2 concentrations and moisture conditions on instantaneous carboxylation efficiency of Datura stramonium. The vertical line on the data point represents the standard error of the mean and the vertical arrow line after Day 8 represents the addition of water in the drought treatments. WW, well-watered.
Photosynthetic electron transport
Under well-watered conditions, the photosynthetic electron transport rate was higher with an eCO2 concentration than with aCO2 (Figure 5). Drought significantly affected the photosynthetic electron transport efficiency with every increment in the drought days and the photosynthetic electron transport rate reached a minimum value (44 µmol e−1 m−2 s−1 for aCO2 and 38 µmol e−1 m−2 s−1 for eCO2) on Day 8 of measurement under both CO2 concentrations. Water addition to drought treatments recovered the photosynthetic electron transport rate under both CO2 concentrations. The photosynthetic electron transport rate of D. stramonium was significant in response to time and water, and the interaction time × water. The rest of the interactions were seen to be non-significant (Table 1).
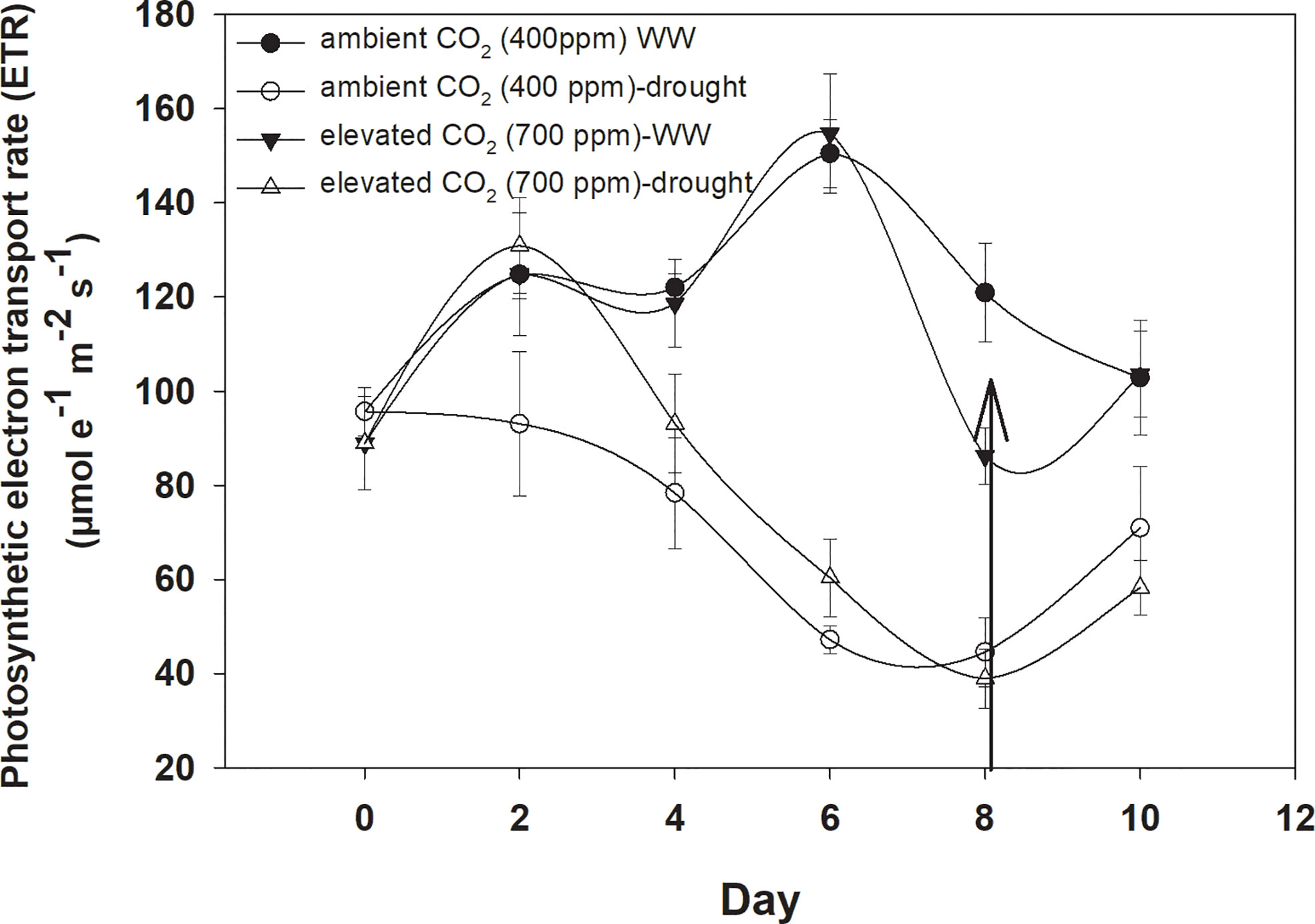
Figure 5 Effect of CO2 concentrations and moisture conditions on the photosynthetic electron transport rate of Datura stramonium. The vertical line on the data point represents the standard error of the mean and the vertical arrow line after Day 8 represents the addition of water in the drought treatments. WW, well-watered.
Fluorescence
The minimum and maximum fluorescence values of D. stramonium grown in well-watered conditions were higher with eCO2 compared to with aCO2 (Figures 7A, B). The effect of drought was more pronounced on minimum fluorescence under aCO2 as the minimum fluorescence decreased linearly until Day 8 of observation with an increase in drought duration. By comparison, eCO2 under drought conditions had no effect on minimum fluorescence until Day 6, and after that, it declined to 319 (Figure 6A). Maximum fluorescence under drought conditions was higher with eCO2 compared to that with aCO2, and a decline in maximum fluorescence occurred with both CO2 concentrations with an increase in drought conditions until Day 8 of the observation (Figure 6B). Water addition to drought treatment significantly recovered the minimum fluorescence under both CO2 concentrations, while water addition to drought treatments slightly recovered the maximum fluorescence. The ANOVA table results showed that the interaction of CO2 × time (p = 0.03) was significant for the minimum fluorescence of D. stramonium while the effects of CO2 (p<0.001), time (p<0.001), water (p<0.001), and the interaction of CO2 × time (p<0.001), and time × water (p<0.001) were significant for the maximum fluorescence of D. stramonium.
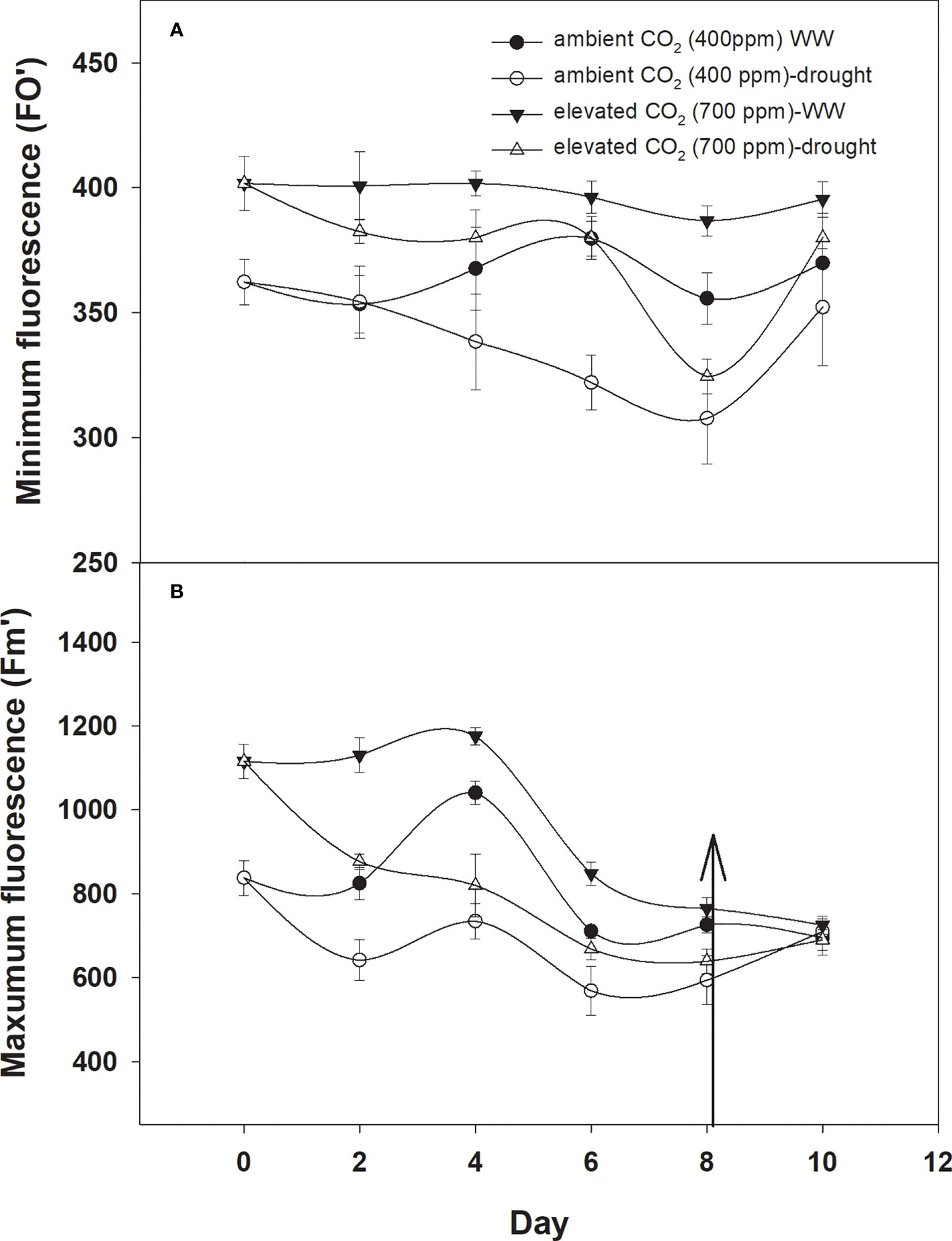
Figure 6 Effect of CO2 concentrations and moisture conditions on (A) minimum fluorescence and (B) maximum fluorescence of Datura stramonium. The vertical line on the data point represents the standard error of the mean and the vertical arrow line after Day 8 represents the addition of water in the drought treatments. WW, well-watered.
PSII activity
The effective quantum efficiency of PSII with both CO2 concentrations was similar when D. stramonium was grown under well-watered conditions (Figure 7A). However, drought conditions showed the variable response of the effective quantum efficiency of PSII under tested CO2 concentrations. Elevated CO2 concentration slightly mitigated the adverse effects of drought, and the effective quantum efficiency of PSII was sustained up to Day 4 with an increase in drought duration. By comparison, aCO2 linearly decreased the effective quantum efficiency of PSII with an increase in drought duration until Day 8. Addition of water to drought treatments recovered the effective quantum efficiency of PSII of D. stramonium in the same way under both CO2 concentrations. The main effects of time (p<0.001), water (p<0.001), and interaction of time × water (p<0.001) were non-significant for the effective quantum efficiency of PSII.
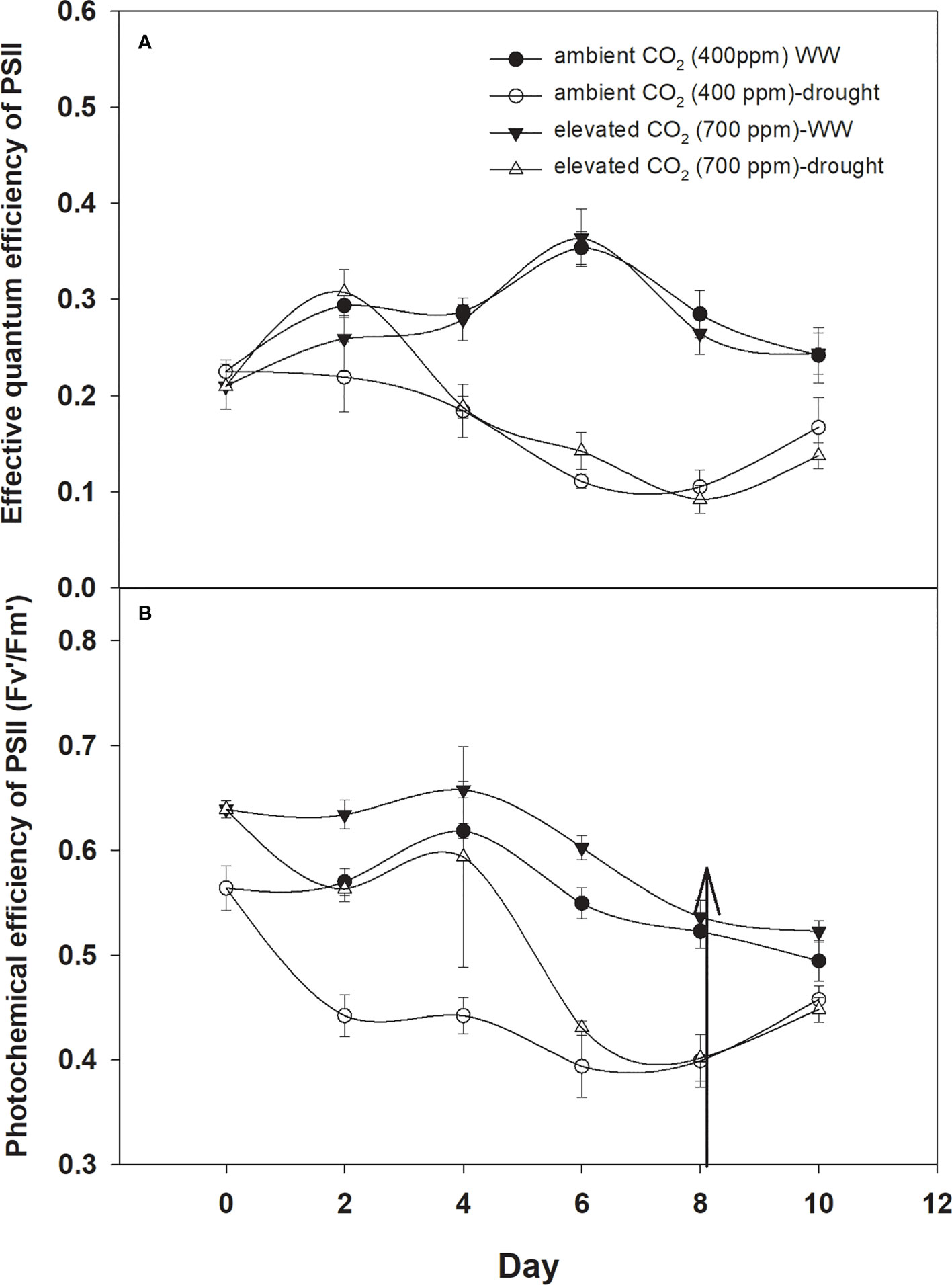
Figure 7 Effect of CO2 concentrations and moisture conditions on (A) effective quantum efficiency of PSII and (B) photochemical efficiency of PSII of Datura stramonium. The vertical line on the data point represents the standard error of the mean and the vertical arrow line after Day 8 represents the addition of water in the drought treatments. WW, well-watered.
The photochemical efficiency of PSII was significantly higher with eCO2 compared to that with aCO2 (Figure 7B). Drought had significant effects on this parameter and effects were more pronounced on the photochemical efficiency of PSII under aCO2, significantly decreasing with the initiation of drought and declining from 0.56 to 0.40 from drought Day 1 to Day 8. On the other hand, drought effects were slight on the photochemical efficiency of PSII until Day 4 of the drought, and after that, it declined to 0.40 at Day 8 of the drought duration. Water addition to drought treatment grown under both CO2 concentrations recovered the photochemical efficiency of PSII in the same way (Figure 7). Results of the ANOVA showed that time (p<0.001), water (p<0.001), CO2 (p<0.001), and time × water (p = 0.001) have significant effects on the photochemical efficiency of PSII (Table 1).
Photochemical quenching
Photochemical quenching was higher with aCO2 compared to that with eCO2 when D. stramonium was grown under well-watered conditions (Figure 8). Drought stress significantly reduced photochemical quenching with an increase in drought duration, and this decline was in a similar way under both CO2 concentrations. The addition of water to drought treatments on Day 8 recovered photochemical quenching, with this recovery being higher with aCO2. The ANOVA results indicated that the effects of time (p<0.001), water (p<0.001), and time × water (p<0.001) were significant for photochemical quenching of D. stramonium (Table 1).
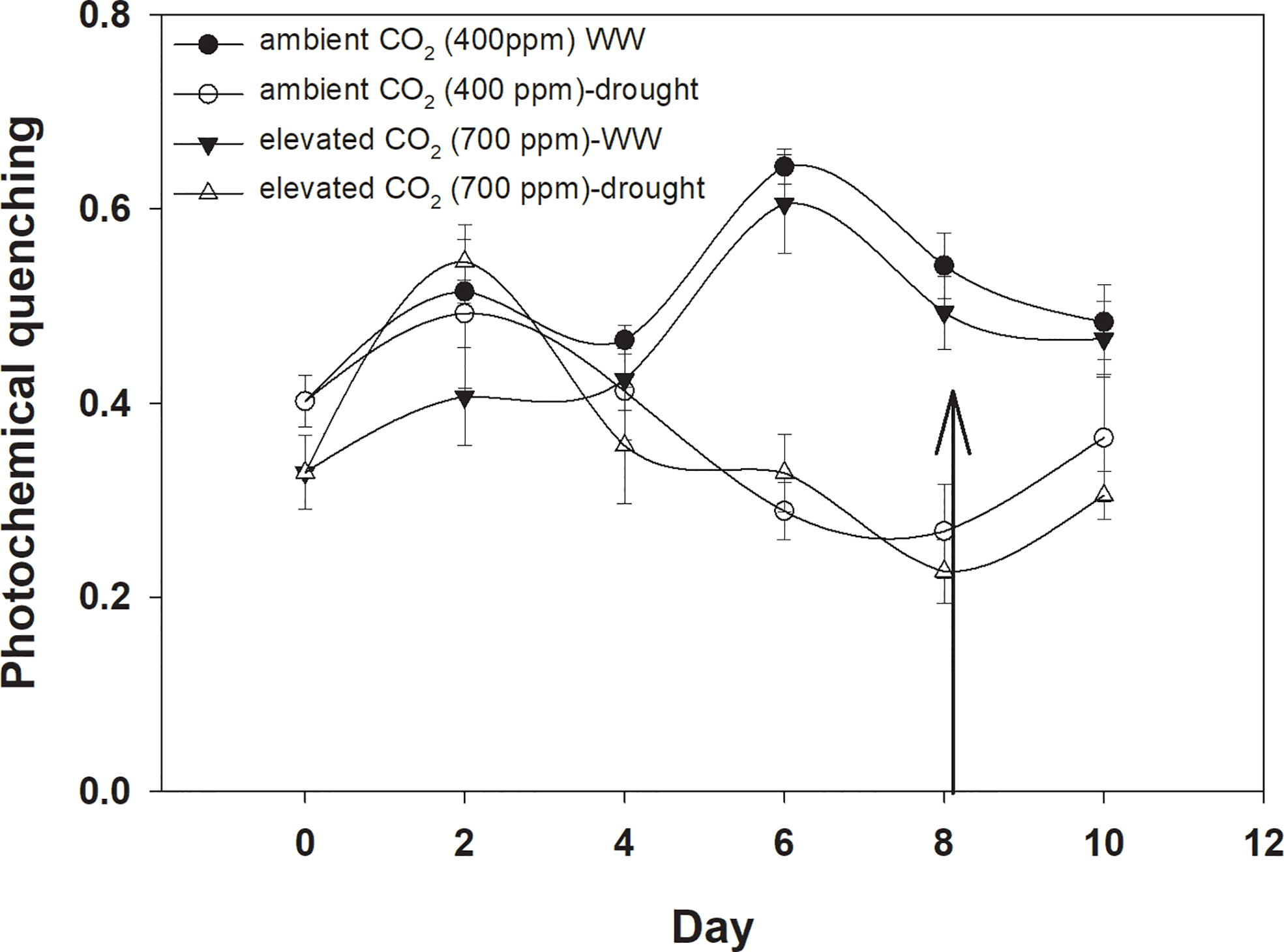
Figure 8 Effect of CO2 concentrations and moisture conditions on photochemical quenching of Datura stramonium. A nail on the data point represents the standard error of the mean and the vertical arrow line after Day 8 represents the addition of water in the drought treatments. WW, well-watered.
Discussion
Climate change has led to significant variation in rainfall patterns, and this, together with increased atmospheric CO2 concentrations, will increase wheat-crop competition for available resources (Ramesh et al., 2017; Bajwa et al., 2019; Chadha et al., 2020). This study revealed that atmospheric CO2 concentrations (400 ppm and 700 ppm) and limited water conditions had variable effects on gas exchange parameters, photosynthetic electron transport rate, and water use efficiency of D. stramonium. Drought conditions had negative effects on the photosynthesis rate of D. stramonium, but elevated CO2 concentrations mitigated the adverse effects of drought on the photosynthesis rate of D. stramonium. Moreover, eCO2 increased the photosynthetic rate under normal irrigation conditions. It is well documented that drought causes a range of responses in photosynthesis mechanisms, depending upon species and season (Alba et al., 2019; Dusenge et al., 2019). Ji et al. (2015) reported that eCO2 increased the leaf photosynthetic rate. In our study under well-watered conditions, the photosynthesis rate was increased by about 25% with eCO2 compared to that of ambient CO2 concentration. Similarly, eCO2 also mitigated the adverse effects of drought and increased the net photosynthesis rate by 12% under drought conditions, and the net photosynthesis rate was 50% higher with elevated CO2 under severe drought conditions. Furthermore, it is generally believed that photosynthesis increases with eCO2, even under stressful environments (Ghahramani et al., 2019; De Kauwe et al., 2021). D. stramonium grown under eCO2 exhibited lower stomatal conductance compared to that which was grown under aCO2. According to Habermann et al. (2019), plants grown under eCO2 in drought conditions resulted in greater stomatal closure than those grown under aCO2. Stomatal conductance is considered to decrease both photosynthesis rate and CO2 concentration in intercellular spaces of the leaf which inhibits metabolism (Cornic, 2000). However, the relative importance of stomatal conductance in restricting the supply of CO2 to metabolism (stomatal limitation) and impairment, which decreases the potential rate, of photosynthesis rate is unclear. Moreover, metabolic limitation is often observed and correlates with loss of ATP, which stands to decrease with mild water loss (Lawlor, 2002). In our study, elevated CO2 maintained stomatal functioning under water stress.
The study by Khalid et al. (2019) found that the stomatal conductors of D. alba decreased due to an increase in CO2 concentration along roadsides. Our results showed a progressive decline in transpiration rate under both CO2 concentrations, but this decline was significantly less with eCO2. The decline in transpiration rate might be due to stomatal closure, stimulated by a combination of eCO2 and drought stress. A study by Walia et al. (2022) showed that elevated CO2 decreased stomatal and transpiration rates by partial closure of stomata, resulting in increased water use efficiency and lower water stress in the plant. Robredo et al. (2007) found that plants grown under eCO2 have more water use efficiency and developed water stress more slowly than those grown under ambient CO2 concentration. Moreover, eCO2 reduced the drought-induced damage in D. stramonium, with plant recovery being significant upon re-commencement of water and drought condition treatments. In previous work, it was noted that the degree of recovery depends upon species and drought duration (Duan et al., 2022). Robredo et al. (2007) also noted that barley plants were not able to recover stomatal closure upon re-watering after 16 days of drought stress. In our study, intercellular CO2 concentration increased with an increase in CO2 concentration, and also that drought stress has drastic effects on intercellular CO2, which increases with an increase in drought duration. Our findings are in line with those of Wang et al. (2018) and Dregulo (2022), who reported that stomatal closure and reduced transpiration rate were found under an enriched CO2 environment. In another study, it was clearly shown that limitations on photosynthesis rate due to drought stress are based on the significant decrease in stomatal conductance and intercellular concentration (Salmon et al., 2020).
The water use efficiency of D. stramonium was 40% higher with eCO2 compared to that with aCO2 under well-watered conditions, suggesting that eCO2 mitigates the adverse effects of drought on the water use efficiency of D. stramonium. This might be due to the reduced transpiration rate as stomatal closure is seen under eCO2. Indeed, as is broadly understood from our results together with other research, water use efficiency enhancement is the result of reduced stomatal conductance and enhanced net photosynthesis rate under a CO2-enriched environmental context (Wei et al., 2022; Wu et al., 2022). Similar to water use efficiency, the intrinsic water use efficiency of D. stramonium shows similar trends under eCO2 and drought conditions. Soil moisture conservation of many species has been reported when they are grown under elevated CO2 concentrations. Our results are further supported by Hassan et al. (2022), who reported that eCO2 reduced the impact of limited water conditions on the physiological traits of a number of plants. Drought conditions caused a reduction in instantaneous carboxylation efficiency with an increase in the duration of drought, but this decline was much lower with eCO2 when compared to that of aCO2. Our findings are in line with those of Wang et al. (2015) and Pérez-López et al. (2013), who showed a significant decline in instantaneous carboxylation efficiency with eCO2. Under well-watered conditions, photosynthetic electron transport rate was higher with aCO2, whereas photosynthetic electron transport rate decreased with the length of drought duration. This suggests that a higher rate of electron transport occurs in drought-stress environments coupled with elevated CO2 concentrations. These results are in line with the studies of the responses of Japanese white birch (Betula platyphylla var. japonica) to elevated carbon dioxide and drought, where photosynthetic electron transport rate was decreased by elevated CO2 but was elevated under drought in ambient CO2 conditions (Kitao et al., 2007). The minimum and maximum fluorescence values of D. stramonium grown in well-watered conditions were higher with eCO2 compared to those with aCO2. The results are in line with those of Chadha et al. (2020) and Weller et al. (2021), who suggested that fluorescence increased with an increase in atmospheric CO2.
The effective quantum efficiency of PSII was decreased in D. stramonium under drought in both CO2 treatments. However, elevated CO2 slightly mitigated the adverse effects on the effective quantum efficiency of PSII. Such trends have been observed in previous studies (Perveen et al., 2010; Wang et al., 2018), where it was concluded that elevated CO2 and drought decreased the effective quantum efficiency of PSII. However, the photochemical efficiency of PSII of D. stramomium was slightly increased with elevated CO2, indicating that a CO2-enriched environment reduces the risk of damage to PSII by abiotic stress. Similarly, Zhao et al. (2010) showed an increase in the photochemical efficiency of PSII in Betula platyphylla seedlings under 700 ppm CO2.
In conclusion, drought affects different physiological traits of D. stramonium. Nevertheless, eCO2 generally increased the leaf gas exchange parameters, water use efficiency, and PSII activity of D. stramonium, by comparison with ambient CO2. This would have contributed to maintaining a higher leaf water potential, so the improved water status of drought-treated plants at elevated CO2. As a result, plants exposed to high CO2 increased their net photosynthesis rate, which, when coupled with reduced stomatal closure and transpiration rate, led to increased water use efficiency. It therefore appears that when D. stramonium grows under elevated CO2 conditions, this mitigates or delays the effects of water stress. Therefore, under the anticipated changes in drought and rainfall patterns, this species may be found to be more competitive, thereby increasing its invasive range, which will result in its having a more serious agricultural impact in the future.
Data availability statement
The raw data supporting the conclusions of this article will be made available by the authors, without undue reservation.
Author contributions
SF conceived and designed the study. MJ conducted the experiments. All authors were involved in writing and reviewing the manuscript. All authors contributed to the article and approved the submitted version.
Conflict of interest
JM was employed by companies Twój Swiat Jacek Mojski and Fundacja Zielona Infrastruktura.
The remaining authors declare that the research was conducted in the absence of any commercial or financial relationships that could be construed as a potential conflict of interest.
Publisher’s note
All claims expressed in this article are solely those of the authors and do not necessarily represent those of their affiliated organizations, or those of the publisher, the editors and the reviewers. Any product that may be evaluated in this article, or claim that may be made by its manufacturer, is not guaranteed or endorsed by the publisher.
Supplementary material
The Supplementary Material for this article can be found online at: https://www.frontiersin.org/articles/10.3389/fpls.2022.929378/full#supplementary-material
References
AbdElgawad, H., Farfan-Vignolo, E. R., De Vos, D., Asard, H. (2015). Elevated CO2 mitigates drought and temperature-induced oxidative stress differently in grasses and legumes. Plant Sci. 231, 1–10. doi: 10.1016/j.plantsci.2014.11.001
AbdElgawad, H., Zinta, G., Beemster, G. T., Janssens, I. A., Asard, H. (2016). Future climate CO2 levels mitigate stress impact on plants: increased defense or decreased challenge? Front. Plant Sci. 7, 556. doi: 10.3389/fpls.2016.00556
Alba, C., Fahey, C., Flory, S. L. (2019). Global change stressors alter resources and shift plant interactions from facilitation to competition over time. Ecology 100, e02859. doi: 10.1002/ecy.2859
Aysu, T., Durak, H. (2015). Thermochemical conversion of datura stramonium l. by supercritical liquefaction and pyrolysis processes. J. Supercritical Fluids 102, 98–114. doi: 10.1016/j.supflu.2015.04.008
Bajwa, A. A., Wang, H., Chauhan, B. S., Adkins, S. W. (2019). Effect of elevated carbon dioxide concentration on growth, productivity and glyphosate response of parthenium weed (Parthenium hysterophorus l.). Pest Manage. Sci. 75, 2934–2941. doi: 10.1002/ps.5403
Baloch, A. H., Baloch, I. A., Ahmed, I., Ahmed, S. (2017). A study of poisonous plants of balochistan, Pakistan. Pure Appl. Biol. (PAB) 6, 989–1001. doi: 10.19045/bspab.2017.600105
Blossey, B., Notzold, R. (1995). Evolution of increased competitive ability in invasive nonindigenous plants: a hypothesis. J. Ecol. 83, 887–889. doi: 10.2307/2261425
Boucher, O., Friedlingstein, P., Collins, B., Shine, K. P. (2009). The indirect global warming potential and global temperature change potential due to methane oxidation. Environ. Res. Lett. 4, 044007. doi: 10.1088/1748-9326/4/4/044007
Chadha, A., Florentine, S., Javaid, M., Welgama, A., Turville, C. (2020). Influence of elements of climate change on the growth and fecundity of Datura stramonium. Environ. Sci. pollut. Res. 27, 35859–35869. doi: 10.1007/s11356-020-10251-y
Cornic, G. (2000). Drought stress inhibits photosynthesis by decreasing stomatal aperture–not by affecting ATP synthesis. Trends Plant Sci. 5, 187–188. doi: 10.1016/S1360-1385(00)01625-3
Cruz, J. L., Lecain, D. R., Alves, A. A., Coelho Filho, M. A., Coelho, E. F. (2018). Elevated CO2 reduces whole transpiration and substantially improves root production of cassava grown under water deficit. Arch. Agron. Soil Sci. 64, 1623–1634. doi: 10.1080/03650340.2018.1446523
Dai, A. (2013). Increasing drought under global warming in observations and models. Nat. Climate Change 3, 52–58. doi: 10.1038/nclimate1633
De Kauwe, M. G., Medlyn, B. E., Tissue, D. T. (2021). To what extent can rising [CO2] ameliorate plant drought stress? New Phytol. 231, 2118–2124. doi: 10.1111/nph.17540
Dikšaitytė, A., Viršilė, A., Žaltauskaitė, J., Januškaitienė, I., Juozapaitienė, G. (2019). Growth and photosynthetic responses in brassica napus differ during stress and recovery periods when exposed to combined heat, drought and elevated CO2. Plant Physiol. Biochem. 142, 59–72. doi: 10.1016/j.plaphy.2019.06.026
Dregulo, A. M. (2022). Climate-neutral waste management in The Russian Federation: New approach to sludge treatment on drying beds under climate change. Journal of Water and Land Development 52 (I-III), 95–100.
Duan, H., Resco De Dios, V., Wang, D., Zhao, N., Huang, G., Liu, W., et al. (2022). Testing the limits of plant drought stress and subsequent recovery in four provenances of a widely distributed subtropical tree species. Plant Cell Environ. 45, 1187–1203. doi: 10.1111/pce.14254
Durak, H., Aysu, T. (2016). Structural analysis of bio-oils from subcritical and supercritical hydrothermal liquefaction of datura stramonium l. J. Supercritical Fluids 108, 123–135. doi: 10.1016/j.supflu.2015.10.016
Dusenge, M. E., Duarte, A. G., Way, D. A. (2019). Plant carbon metabolism and climate change: elevated CO2 and temperature impacts on photosynthesis, photorespiration and respiration. New Phytol. 221, 32–49. doi: 10.1111/nph.15283
Ghahramani, A., Howden, S. M., Del Prado, A., Thomas, D. T., Moore, A. D., Ji, B., et al. (2019). Climate change impact, adaptation, and mitigation in temperate grazing systems: a review. Sustainability 11, 7224. doi: 10.3390/su11247224
Habermann, E., Dias De Oliveira, E. A., Contin, D. R., San Martin, J. A., Curtarelli, L., Gonzalez-Meler, M. A., et al. (2019). Stomatal development and conductance of a tropical forage legume are regulated by elevated [CO2] under moderate warming. Front. Plant Sci. 10, 609. doi: 10.3389/fpls.2019.00609
Hassan, I.F., Gaballah, M.S., Ogbaga, C.C., Murad, S.A., Brysiewicz, A., Bakr, B.M.M., et al (2022). Does melatonin improve the yield attributes of field-droughted banana under Egyptian semi-arid conditions. Journal of Water and Land Development 52 (I–III), 221–231.
Jin, J., Armstrong, R., Tang, C. (2019). Impact of elevated CO2 on grain nutrient concentration varies with crops and soils–a long-term FACE study. Sci. Total Environ. 651, 2641–2647. doi: 10.1016/j.scitotenv.2018.10.170
Ji, G., Xue, H., Seneweera, S., Ping, L., Zong, Y.-Z., Qi, D., et al. (2015). Leaf photosynthesis and yield components of mung bean under fully open-air elevated [CO2]. J. Integr. Agric. 14, 977–983. doi: 10.1016/S2095-3119(14)60941-2
Karimizadeh, R., Keshavarzi, K., Karimpour, F., Peyman, S. (2021). Analysis of screening tools for drought tolerance in chickpea (Cicer arietinum l.) genotypes. Agric. Sci. Digest A Res. J. 41, 531–541. doi: 10.18805/ag.D-255
Khalid, N., Masood, A., Noman, A., Aqeel, M., Qasim, M. (2019). Study of the responses of two biomonitor plant species (Datura alba & Ricinus communis) to roadside air pollution. Chemosphere 235, 832–841. doi: 10.1016/j.chemosphere.2019.06.143
Kimball, B. A. (2016). Crop responses to elevated CO2 and interactions with H2O, n, and temperature. Curr. Opin. Plant Biol. 31, 36–43. doi: 10.1016/j.pbi.2016.03.006
Kitao, M., Lei, T. T., Koike, T., Kayama, M., Tobita, H., Maruyama, Y. (2007). Interaction of drought and elevated CO2 concentration on photosynthetic down-regulation and susceptibility to photoinhibition in Japanese white birch seedlings grown with limited n availability. Tree Physiol. 27, 727–735. doi: 10.1093/treephys/27.5.727
Lawlor, D. W. (2002). Limitation to photosynthesis in water-stressed leaves: stomata vs. metabolism and the role of ATP. Ann. Bot. 89, 871–885. doi: 10.1093/aob/mcf110
Li, S., Li, X., Wei, Z., Liu, F. (2020). ABA-mediated modulation of elevated CO2 on stomatal response to drought. Curr. Opin. Plant Biol. 56, 174–180. doi: 10.1016/j.pbi.2019.12.002
Li, D., Zhang, X., Li, L., Aghdam, M. S., Wei, X., Liu, J., et al. (2019). Elevated CO2 delayed the chlorophyll degradation and anthocyanin accumulation in postharvest strawberry fruit. Food Chem. 285, 163–170. doi: 10.1016/j.foodchem.2019.01.150
López-Calcagno, P. E., Brown, K. L., Simkin, A. J., Fisk, S. J., Vialet-Chabrand, S., Lawson, T., et al. (2020). Stimulating photosynthetic processes increases productivity and water-use efficiency in the field. Nat. Plants 6, 1054–1063. doi: 10.1038/s41477-020-0740-1
Maxwell, K., Johnson, G. N. (2000). Chlorophyll fluorescence–a practical guide. J. Exp. Bot. 51, 659–668. doi: 10.1093/jexbot/51.345.659
Nguyen, M. C., Zhang, Y., Li, J., Li, X., Bai, B., Wu, H., et al. (2017). A geostatistical study in support of CO2 storage in deep saline aquifers of the shenhua CCS project, ordos basin, China. Energy Proc. 114, 5826–5835. doi: 10.1016/j.egypro.2017.03.1720
Pan, C., Ahammed, G. J., Li, X., Shi, K. (2018). Elevated CO2 improves photosynthesis under high temperature by attenuating the functional limitations to energy fluxes, electron transport and redox homeostasis in tomato leaves. Front. Plant Sci. 9, 1739. doi: 10.3389/fpls.2018.01739
Pastore, M. A., Lee, T. D., Hobbie, S. E., Reich, P. B. (2020). Interactive effects of elevated CO2, warming, reduced rainfall, and nitrogen on leaf gas exchange in five perennial grassland species. Plant Cell Environ. 43, 1862–1878. doi: 10.1111/pce.13783
Pérez-López, U., Miranda-Apodaca, J., Muñoz-Rueda, A., Mena-Petite, A. (2013). Lettuce production and antioxidant capacity are differentially modified by salt stress and light intensity under ambient and elevated CO2. J. Plant Physiol. 170, 1517–1525. doi: 10.1016/j.jplph.2013.06.004
Perveen, S., Shahbaz, M., Ashraf, M. (2010). Regulation in gas exchange and quantum yield of photosystem II (PSII) in salt-stressed and non-stressed wheat plants raised from seed treated with triacontanol. Pakistan J. Bot. 42, 3073–3081.
Qi, M., Liu, X., Li, Y., Song, H., Yin, Z., Zhang, F., et al. (2021). Photosynthetic resistance and resilience under drought, flooding and rewatering in maize plants. Photosynthesis Res. 148, 1–15. doi: 10.1007/s11120-021-00825-3
Ramesh, K., Matloob, A., Aslam, F., Florentine, S. K., Chauhan, B. S. (2017). Weeds in a changing climate: vulnerabilities, consequences, and implications for future weed management. Front. Plant Sci. 8, 95. doi: 10.3389/fpls.2017.00095
Robredo, A., Pérez-López, U., de la Maza, H. S., González-Moro, B., Lacuesta, M., Mena-Petite, A., et al. (2007). Elevated CO2 alleviates the impact of drought on barley improving water status by lowering stomatal conductance and delaying its effects on photosynthesis. Environ. Exp. Bot. 59, 252–263. doi: 10.1016/j.envexpbot.2006.01.001
Salmon, Y., Lintunen, A., Dayet, A., Chan, T., Dewar, R., Vesala, T., et al. (2020). Leaf carbon and water status control stomatal and nonstomatal limitations of photosynthesis in trees. New Phytol. 226, 690–703. doi: 10.1111/nph.16436
Skendžić, S., Zovko, M., Živković, I. P., Lešić, V., Lemić, D. (2021). The impact of climate change on agricultural insect pests. Insects 12, 440. doi: 10.3390/insects12050440
Song, X., Bai, P., Ding, J., Li, J. (2021). Effect of vapor pressure deficit on growth and water status in muskmelon and cucumber. Plant Sci. 303, 110755. doi: 10.1016/j.plantsci.2020.110755
Valone, T. F. (2021). Study of a possible global environmental forecast and roadmap based on 420 ky of paleoclimatology. Modern Adv. Geography Environ. Earth Sci. 5, 130–140. doi: 10.9734/bpi/magees/v5/2845F
Walia, S., Rathore, S., Jamwal, S., Kumar, R. (2022). The combined response of elevated CO2 and temperature on Valeriana jatamansi Jones with worm manuring in the western himalaya: Integrating growth, biomass, and quality. Horticult. Environ. Biotechnol. 63, 161–172. doi: 10.1007/s13580-021-00386-3
Wang, A., Lam, S. K., Hao, X., Li, F. Y., Zong, Y., Wang, H., et al. (2018). Elevated CO2 reduces the adverse effects of drought stress on a high-yielding soybean (Glycine max (L.) merr.) cultivar by increasing water use efficiency. Plant Physiol. Biochem. 132, 660–665. doi: 10.1016/j.plaphy.2018.10.016
Wang, M., Xie, B., Fu, Y., Dong, C., Hui, L., Guanghui, L., et al. (2015). Effects of different elevated CO2 concentrations on chlorophyll contents, gas exchange, water use efficiency, and PSII activity on C3 and C4 cereal crops in a closed artificial ecosystem. Photosynthesis Res. 126, 351–362. doi: 10.1007/s11120-015-0134-9
Wei, Z., Abdelhakim, L. O. A., Fang, L., Peng, X., Liu, J., Liu, F. (2022). Elevated CO2 effect on the response of stomatal control and water use efficiency in amaranth and maize plants to progressive drought stress. Agric. Water Manage. 266, 107609. doi: 10.1016/j.agwat.2022.107609
Weller, S., Florentine, S., Javaid, M. M., Welgama, A., Chadha, A., Chauhan, B. S., et al. (2021). Amaranthus retroflexus L.(Redroot pigweed): Effects of elevated CO2 and soil moisture on growth and biomass and the effect of radiant heat on seed germination. Agronomy 11, 728. doi: 10.3390/agronomy11040728
Werner, C., Peperkorn, R., Máguas, C., Beyschlag, W. (2008). “Competitive balance between the alien invasive acacia longifolia and native Mediterranean species,” in Plant invasions: human perception, ecological impacts and management (Leiden, The Netherlands: Backhuys Publishers), 261–275.
Wu, H., Ismail, M., Ding, J. (2017). Global warming increases the interspecific competitiveness of the invasive plant alligator weed, Alternanthera philoxeroides. Sci. Total Environ. 575, 1415–1422. doi: 10.1016/j.scitotenv.2016.09.226
Wu, Y., Zhong, H., Li, J., Xing, J., Xu, N., Zou, H. (2022). Water use efficiency and photosynthesis of calamagrostis angustifolia leaves under drought stress through CO2 concentration increase. J. Plant Interact. 17, 60–74. doi: 10.1080/17429145.2021.2011444
Xu, Z., Jiang, Y., Jia, B., Zhou, G. (2016). Elevated-CO2 response of stomata and its dependence on environmental factors. Front. Plant Sci. 7, 657. doi: 10.3389/fpls.2016.00657
Yan, F., Li, X., Liu, F. (2017). ABA signaling and stomatal control in tomato plants exposure to progressive soil drying under ambient and elevated atmospheric CO2 concentration. Environ. Exp. Bot. 139, 99–104. doi: 10.1016/j.envexpbot.2017.04.008
Zeid, I., Shedeed, Z. (2006). Response of alfalfa to putrescine treatment under drought stress. Biol. Plantarum 50, 635–640. doi: 10.1007/s10535-006-0099-9
Zhao, X., Mao, Z., Xu, J. (2010). Gas exchange, chlorophyll and growth responses of Betula platyphylla seedlings to elevated CO2 and nitrogen. Int. J. Biol. 2, 143. doi: 10.5539/ijb.v2n1p143
Ziska, L. H., Blumenthal, D. M., Franks, S. J. (2019). Understanding the nexus of rising CO2, climate change, and evolution in weed biology. Invasive Plant Sci. Manage. 12, 79–88. doi: 10.1017/inp.2019.12
Keywords: gas exchange, photosystem II activity, fluorescence, water use efficiency, electron transport rate
Citation: Javaid MM, Florentine S, Mahmood A, Wasaya A, Javed T, Sattar A, Sarwar N, Kalaji HM, Ahmad HB, Worbel J, Ahmed MAA, Telesiński A and Mojski J (2022) Interactive effect of elevated CO2 and drought on physiological traits of Datura stramonium. Front. Plant Sci. 13:929378. doi: 10.3389/fpls.2022.929378
Received: 26 April 2022; Accepted: 20 September 2022;
Published: 26 October 2022.
Edited by:
Tanveer Alam Khan, Leibniz Institute of Plant Genetics and Crop Plant Research (IPK), GermanyReviewed by:
Halil Durak, Yüzüncü Yıl University, TurkeyHossein Sabouri, Gonbad Kavous University, Iran
Copyright © 2022 Javaid, Florentine, Mahmood, Wasaya, Javed, Sattar, Sarwar, Kalaji, Ahmad, Worbel, Ahmed, Telesiński and Mojski. This is an open-access article distributed under the terms of the Creative Commons Attribution License (CC BY). The use, distribution or reproduction in other forums is permitted, provided the original author(s) and the copyright owner(s) are credited and that the original publication in this journal is cited, in accordance with accepted academic practice. No use, distribution or reproduction is permitted which does not comply with these terms.
*Correspondence: Muhammad Mansoor Javaid, mmansoorjavaid@gmail.com; Athar Mahmood, athar.mahmood@uaf.edu.pk