- 1African Sustainable Agriculture Research Institute (ASARI), Mohammed VI Polytechnic University (UM6P), Laâyoune, Morocco
- 2AgroBioSciences Department (AgBS), Mohammed VI Polytechnic University (UM6P), Ben Guerir, Morocco
Cacti are one of the most significant and diversified groups of angiosperms, distributed and cultivated globally, mostly in semi-arid, arid, and the Mediterranean climate regions. Conventionally, they are propagated by seeds or through vegetative propagation via rooted offshoots or grafting. However, these multiplication procedures remain insufficient for mass propagation. In vitro culture techniques are utilized to mass propagate endangered and commercial cacti species. These include somatic embryogenesis and plant regeneration through indirect or direct organogenesis. The latter is a promising tool for commercial clonal propagation of high-value species and has been successfully implemented for several species, such as Mammillaria, Hylocereus, Cereus, Echinocereus, and Ariocarpus. However, its success depends on explant type, basal nutrient formulation of culture medium, and types and concentrations of plant growth regulators. This study aimed to assess the potential of in vitro propagation methods applied to cacti species and discuss the different factors affecting the success of these methods. This study has also highlighted the insufficient work on Opuntia species for mass propagation through axillary buds' proliferation. The development of an efficient micropropagation protocol is thus needed to meet the supply of increasing demand of Opuntia species for human consumption as fruit, animal feed, and ecological restoration in semi-arid and arid zones.
Introduction
Cactaceae represents one of the most noticeable and diverse families of angiosperm available in nature and is cultivated mostly in warm and semi-arid and arid regions of the Americas, the Mediterranean countries, Africa, Asia, Europe, and Australia (Casas and Barbera, 2002). This family has nearly 130 genera, and around 2000 species present in different forms of growth and shapes such as columnar (Carnegiea, Pachycereus, Stenocereus, and Lophocereus), barrel (Ferocactus and Echinocactus), hedgehog (Echinocereus), pincushion (Mammillaria), cholla (Opuntia), and prickly pear (Opuntia and Nopalea) (Shedbalkar et al., 2010). Besides a remarkable diversity in growth forms, they are well-known for their great ability to grow and adapt under stressful environments, especially drought (Inglese et al., 2017; ICARDA, 2021). Cacti are often used to grow under marginal and sloppy lands as a barrier to soil erosion in West Asia (Jordan, Syria, Israel, and Yemen), Australia, and Europe (Italy, Portugal, and Spain), as a plant for ecological restoration and land reclamation, and also grown as a commercial crop such as fruit for human and feed for livestock (Khalafalla et al., 2007; Lema-Rumińska and Kulus, 2014; Inglese et al., 2017).
Opuntia is one of the well-known genera among the cacti, and Opuntia ficus-indica is the most important species cultivated for edible fruits and cladodes, vegetables, and animal feed, especially in the Mediterranean and the West and Central Asian countries (Glimn-Lacy and Kaufman, 2006; Shedbalkar et al., 2010; Nefzaoui et al., 2014; Inglese et al., 2017). Cacti are also known for their outstanding nutritional, medicinal, and biological properties conferred by the presence of high-value chemical compounds such as phenolic compounds, carotenoids, vitamins, flavonoids, and betalains (El-Mostafa et al., 2014; Díaz et al., 2017; Al-Khayri et al., 2018; González-Stuart and Rivera, 2019).
Cacti are propagated either from seeds or from vegetative cutting and grafting. Seed propagation is common and simple but difficult to obtain seedlings for some species due to the limitation of flowering, reproduction, and seed formation (Lema-Rumińska and Kulus, 2014). Cacti propagation through seeds is also time-consuming and requires specific light and temperature conditions (Monroy-Vázquez et al., 2017). Further, the use of seeds does not guarantee genetic stability and true-to-type progenies due to the predominance of cross-pollination and genetic diversity. In some cases, seed germination percentage is low due to the physical dormancy imposed by the hard coat. For O. ficus-indica, besides the low germination percentage (5.3%) recorded by Stambouli-Essassi et al. (2017), seeds evolved into cactus plants after 5 years of culture, while the first flowering can be achieved after seven years. In opposition to seed germination, conventional vegetative propagation is appropriate for the commercial production of cacti plants with high economical and agronomical values (Ghaffari et al., 2013). Even though vegetative propagation presents some advantages over seed germination, this method of propagation is mostly restricted to columnar species. The propagation rate can also be a limiting factor. The number of cladodes per plant has been estimated for vegetatively propagated cochineal carmine cactus pear forage-resistant species to be around 15.64 cladodes per plant after 540 days after planting (Lopes et al., 2013). Moreover, some cacti genera suffer from extreme extractivism, illegal trades and unsustainable harvesting, and the degradation of their natural habitat due to anthropic action (Jesus et al., 2019). These disturbances are largely responsible for the extinction threat of local populations and drastic changes in cacti distribution and abundance and can be exacerbated by slow growth and dependence on reproductive individuals for population maintenance (Hughes, 2017). Added to this, the limited number of plants produced using conventional techniques remains insufficient with the market need worldwide (Lema-Rumińska and Kulus, 2014). These difficulties related to market need supplying can partly be solved through the adoption of plant tissue culture techniques. Micropropagation is a powerful tool that allows the conservation of endangered species and mass-scale production of commercial varieties and species. For instance, through micropropagation, nearly 457,000 plants of O. ficus-indica can be produced from a single explant in a year (Rodriguez and Ramirez-Pantoja, 2020). However, this cannot be achieved without a better understanding of the different factors controlling the plant regeneration process in order to increase the efficiency of micropropagation techniques aiming the production and multiplication of superior genotypes of interest. This comprehensive review was carried out to assess the potential, advantages, and limitations of the available in vitro culture techniques applied for cacti micropropagation. Further, axillary buds' proliferation (the most important and specific method of in vitro culture), a promising Opuntia micropropagation technique, allows the development of new shoots and plants from preexisting meristems. A case study on micropropagation through axillary buds' proliferation, especially on the key parameters affecting its success, is required as it is one of the most rapid and reliable methods for in vitro clonal propagation and ensures the genetic homogeneity, epigenetic stability, and the production of true-to-type progenies.
Methodology of Research
For the review literature, a Web browsing was conducted in Scopus, ScienceDirect, Google Scholar, and Springer databases using keywords “cactus” or “cacti,” “propagation,” “in vitro” or “micropropagation,” “axillary buds,” or “areoles.” From the search, a total of 1,005 relevant studies were collected. From the collection, 457 duplicates were removed and 88 studies were preserved after screening the title, abstract, and full text. The outline of the literature search, database, and management is shown in Figure 1. Among the selected studies, “four” were about in vitro grafting, “eight” about somatic embryogenesis, and “49” related to regeneration through direct and indirect organogenesis.
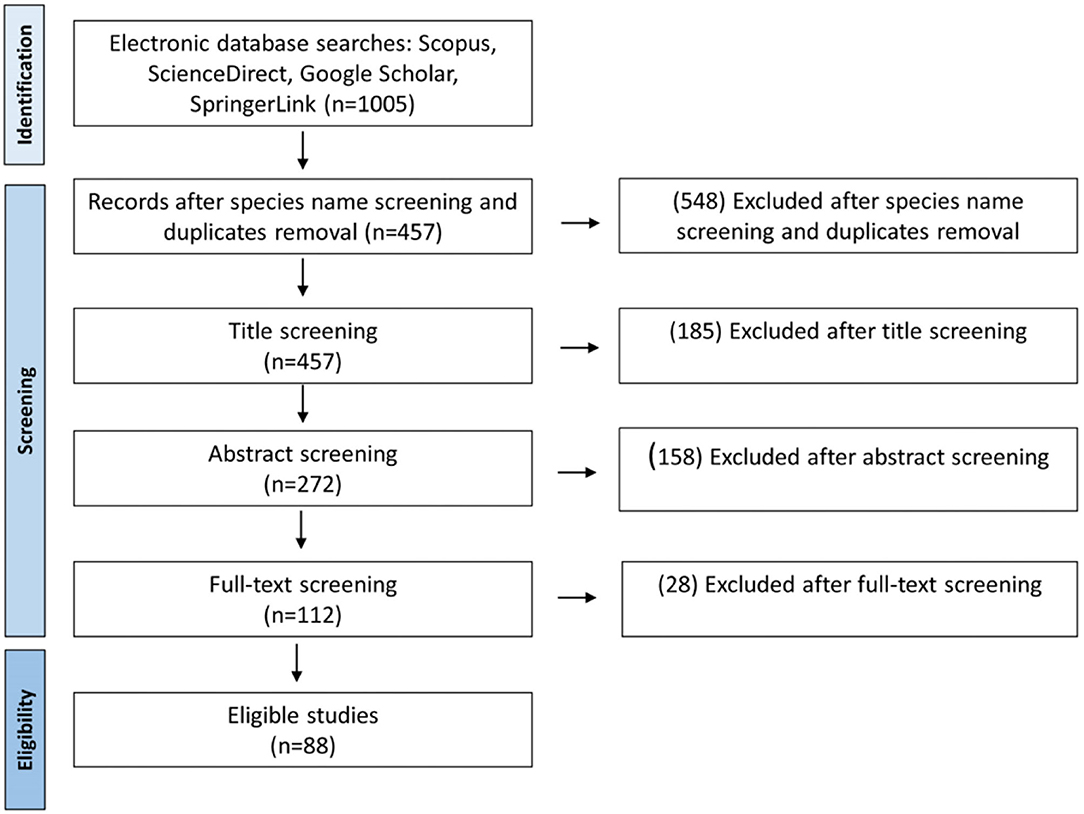
Figure 1. Literature search and selection process of the included studies of cacti and Opuntia species micropropagation.
In vitro Propagation Techniques Applied to Cacti
Micropropagation can be defined as a vegetative method of propagation accomplished under aseptic in vitro conditions using small portions of the plant, also called explants, such as stem segments, cotyledons, leaves, axillary and apical buds, immature seeds, and embryos. Cactus micropropagation methods have been developed for over 60 years (Lema-Rumińska and Kulus, 2014). Numerous micropropagation techniques have been applied such as regeneration through somatic embryogenesis, regeneration through direct or indirect organogenesis, and in vitro grafting (Figure 2). Those methods have been used for several species of cactus such as Mammillaria, Cereus, Hylocereus, Echinocereus, Astrophytum, Stenocactus, Schlumbergera, Coryphantha, and Copiapoa (Wakhlu and Bhau, 2000; Papafotiou et al., 2001; Mohamed-Yasseen, 2002; Al-Ramamneh et al., 2006; Lema-Rumińska and Kulus, 2012; Lema-Rumińska et al., 2013; Bhau and Wakhlu, 2015; Chornobrov and Bilous, 2021; Ivannikov et al., 2021). The regeneration through direct or indirect organogenesis appeared to be the most important and reliable method of in vitro propagation with about 80% of the conducted work on cacti (Figure 3).
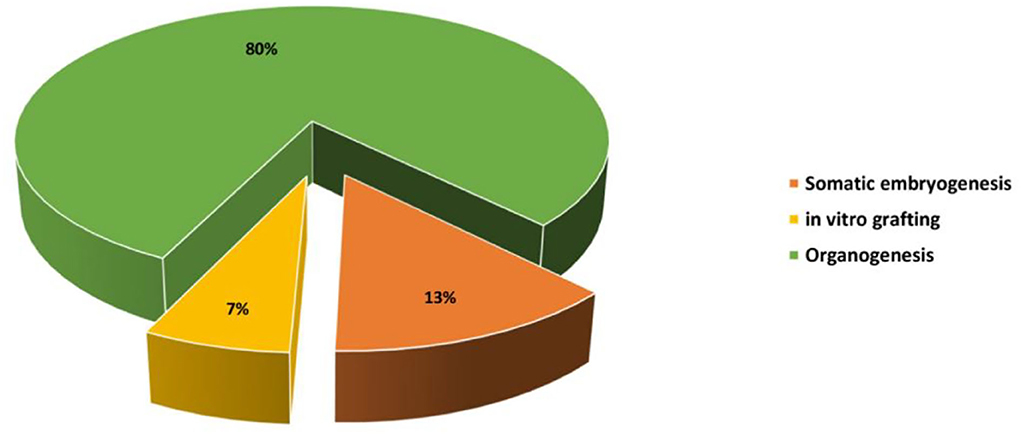
Figure 2. Percentage of the studies screened and reviewed for each method of in vitro propagation on cacti species.
Somatic Embryogenesis
Somatic embryogenesis is the process that allows the regeneration of whole plants from somatic embryos developed from somatic cells. Although these embryos are obtained from somatic cells, they show an identical pattern of embryo development commonly observed in zygotic embryogenesis (Gomes et al., 2006). Somatic embryogenesis has been applied in some cacti including prickly pear cactus (O. ficus-indica) (Gomes et al., 2006; Bouamama et al., 2011), Ariocarpus kotschoubeyanus (Moebius-Goldammer et al., 2003), Schlumbergera truncata (Al-Ramamneh et al., 2006), and Astrophytum asterias (Lema-Rumińska and Kulus, 2012).
In several cacti, somatic embryogenesis requires the presence of auxin or cytokinins alone. In O. ficus-indica, the addition of 4 mg/L of 4-amino 3,5,6-trichloropicolinic acid (picloram) to the semi-solid Murashige and Skoog (MS) basal medium allows the development of somatic embryos from shoot apices culture (Gomes et al., 2006). In Astrophytum asterias, somatic embryos derived from seeds were obtained using a higher concentration of 2,4-dichlorophenoxyacetic acid (2,4-D) (7 mg/L) (Lema-Rumińska and Kulus, 2012). Lema-Rumińska and Kulus (2014) succeeded in the induction of somatic embryos from areoles' culture of Copiapoa tenuissima by adding 2 mg/L of 2,4-D to the culture medium. For Schlumbergera truncata, somatic embryo culture was established from areoles' cultivation in a liquid Schenk and Hildebrandt (SH)-based medium supplemented with 1.5 mg/L of kinetin (Kin) (Al-Ramamneh et al., 2006). In some cases, somatic embryogenesis occurs as a result of the combined action of auxins and cytokinin. Tubercles of in vitro germinated seeds of Ariocarpus kotschoubeyanus evolved into somatic embryos in the presence of 6-benzylaminopurine (BA) (2–5 mg/L) and 1-naphthaleneaceticc acid (NAA) (0.1–1.1 mg/L) (Moebius-Goldammer et al., 2003). Indirect somatic embryogenesis can be initiated from immature anther culture in the presence of 2 mg/L 2,4-dichlorophenoxyacetic acid (2,4-D) and 2.5 mg/L of thidiazuron (TDZ) (Bouamama et al., 2011). MS basal medium supplementation with 5 mg/L of TDZ and 0.2 mg/L of NAA allowed the establishment of callus culture from Christmas cactus (S. truncata) axillary buds' culture and the proliferation of these callus cultures into somatic embryos (Chornobrov and Bilous, 2021). Somatic embryogenesis can also be trigged in the absence of auxin and cytokinins sources. Jedidi et al. (2013) were able to develop somatic embryos from immature ovule culture in a semi-solid MS medium supplemented with 1 mg/L of gibberellic acid (GA3).
In vitro Grafting
In vitro grafting is the process of grafting a micro-scion onto the stems of young rootstock plants generated through in vitro culture (Ashrafzadeh, 2020). This propagation technique has been adopted for cacti as an alternative solution for the problems encountered during the propagation through grafting, mostly the poor success, contamination issues, and dehydration stress of graft union surrounding tissues (Estrada-Luna et al., 2002). Besides the development of pathogen-free plants, the scion growth requirements are directly supplied from the rootstock (Badalamenti et al., 2016). In vitro grafting has previously been tested for the genus Opuntia. Estrada-Luna et al. (2002) succeeded in the in vitro grafting of O. ficus-indica on five different rootstocks (O. streptacantha, O. robusta, O. cochinera, O. leucotricha, and O. ficus-indica). They also recorded the highest percentage of viability (up to 90%) with horizontal grafting, while only 30% of micro-grafted plants were able to survive when the scion was wedge-shaped (Estrada-Luna et al., 2002). Gymnocalycium mihanovichii, an ornamental cactus lacking chlorophyll, was successfully in vitro grafted on Trichocereus spachianus, with a 100% percentage of viability in the presence of indole-3-butyric acid (IBA) at 100 mg/L (Moghadam et al., 2012). In vitro grafting was found effective to ensure the propagation of endangered cacti species such as Pelecyphora aselliformis using O. ficus-indica as a rootstock (Badalamenti et al., 2016). In vitro grafting offers many advantages for the in vitro culture of cacti species, even endangered ones, which can contribute to the ex vitro conservation of threatened species (Badalamenti et al., 2016).
Organogenesis
Organogenesis can occur directly from cells, tissues, or organs cultivated in vitro (direct organogenesis) or indirectly (indirect organogenesis) from a transitional step of callus formation (cell dedifferentiation) from which new plantlets are formed and developed from callus masses (Pérez-Molphe-Balch et al., 2015).
Indirect Organogenesis
Indirect organogenesis has been successfully implemented for several cacti species such as Astrophytum asterias, Ariocarpus Kotschoubenyanus, Blossfeldia liliputiana, Strombocactus disciformis, Coryphantha elephantidens, Mammillaria elongata, Mammillaria gracilis, Mammillaria mathildae, Mammillaria pectinifera, Escobaria minima, and Pelecyphora aselliformis (Wakhlu and Bhau, 2000; Papafotiou et al., 2001; Giusti et al., 2002; Poljuha et al., 2003; del Socorro Santos-Díaz et al., 2006; García-Rubio and Malda-Barrera, 2010; Ivannikov et al., 2021; Table 1). Callus-mediated regeneration commonly occurs when the culture medium is supplemented with high amounts of auxin or cytokinin during the initial step of callus formation (Pérez-Molphe-Balch et al., 2015). Newly formed buds are obtained after callus transfer to a culture medium with a high cytokinin/auxin ratio (Pérez-Molphe-Balch et al., 2015; Table 1). In Ariocarpus kotschoubeyanus, adventitious shoots have been obtained indirectly from tubercles (prominent cone-shaped protuberances, grooved on the upper surface and topped by an areole of spines) after 6 weeks of culture in the presence of BA alone or in combination with 1-naphthaleneacetic acid (NAA). The highest average numbers of buds per explant, that is, 6.2 and 6.3, were obtained with the application of BA (1 mg/L) and BA/NAA (3 mg/L BA and 1 mg/L NAA), underlining the efficiency of the technique. The highest shoot formation was also recorded within the same hormonal combination (Moebius-Goldammer et al., 2003). García-Rubio and Malda-Barrera (2010) established an efficient protocol of Mammillaria mathildae micropropagation through the culture of shoot basal segments in the presence of different concentrations of BA and indole-3-acetic acid (IAA). The cultivated explants developed callus on MS medium supplemented with different amounts of BA (0, 5, and 10 mg/L) and IAA (0, 0.25, 0.5, and 1 mg/L) (García-Rubio and Malda-Barrera, 2010). In Mammillaria albicoma, Wyka et al. (2006) obtained adventitious shoots from subsequent cultures of callus derived from flower buds culture in the presence of 0.1 mg/L of NAA and 5 mg/L of BA.
For cacti such as Astrophytum asterias, Blossfeldia liliputiana, and Strombocactus disciformis, the presence of auxin is not mandatory for indirect organogenesis. Indeed, the best organogenetic response was achieved in the absence of auxin (2,4-D) (Ivannikov et al., 2021). Kin or TDZ can also be used to induce indirect adventitious shoot formation instead of BA. Wakhlu and Bhau (2000) achieved Coryphantha elephantidens shoot regeneration through pith (storage tissue at the center of the vascular plant systems) tissue culture in MS medium enriched with 1.5 mg/L of Kin and 0.5 mg/L of 2,4-D. TDZ regenerative capacity was investigated by Giusti et al. (2002) for three different cacti: Pelecyphora aselliformis, Escobaria minima, and Mammillaria pectinifera. The authors found that the medium supplementation with 0.5 mg/L of TDZ prompted callus formation and then shoot regeneration of the three cacti species at a variable percentage (Giusti et al., 2002). Even though shoot can be regenerated from explant-derived callus, this method is less recommended than direct organogenesis, for clonal propagation due to the genetic instabilities favored by callus development. For instance, it has been previously reported that callus cultures or callogenesis promote somaclonal variation, which can contribute to genetic diversity (Sala et al., 2011). Therefore, indirect organogenesis is not recommended when aiming for the preservation of genetic integrity. However, it can be used in breeding programs, generating new varieties with new features, especially for ornamental, food, and medicinal purposes (Pérez-Molphe-Balch et al., 2015).
Direct Organogenesis
Direct organogenesis is defined as the direct process of the production of new buds from the tissue without intervening callus stage. During the last decades, micropropagation through direct organogenesis has been widely implemented to ensure the micropropagation of many endangered species, including those belonging to the Cactaceae family (Papafotiou et al., 2001; Pérez-Molphe-Balch and Dávila-Figueroa, 2002; Moebius-Goldammer et al., 2003; Dávila-Figueroa et al., 2005; de Medeiros et al., 2006; Ramirez-Malagon et al., 2007; Table 2). The success of this method of propagation relies mostly on the balance between cytokinin and auxin amounts. BA and NAA are commonly used to prompt direct shoot formation. For instance, an efficient protocol for direct organogenesis has been established for Ariocarpus kotschoubeyanus using BA alone or in combination with NAA. The highest number of buds forming explants was recorded in the presence of BA only (1, 3, and 5 mg/L) (Moebius-Goldammer et al., 2003).
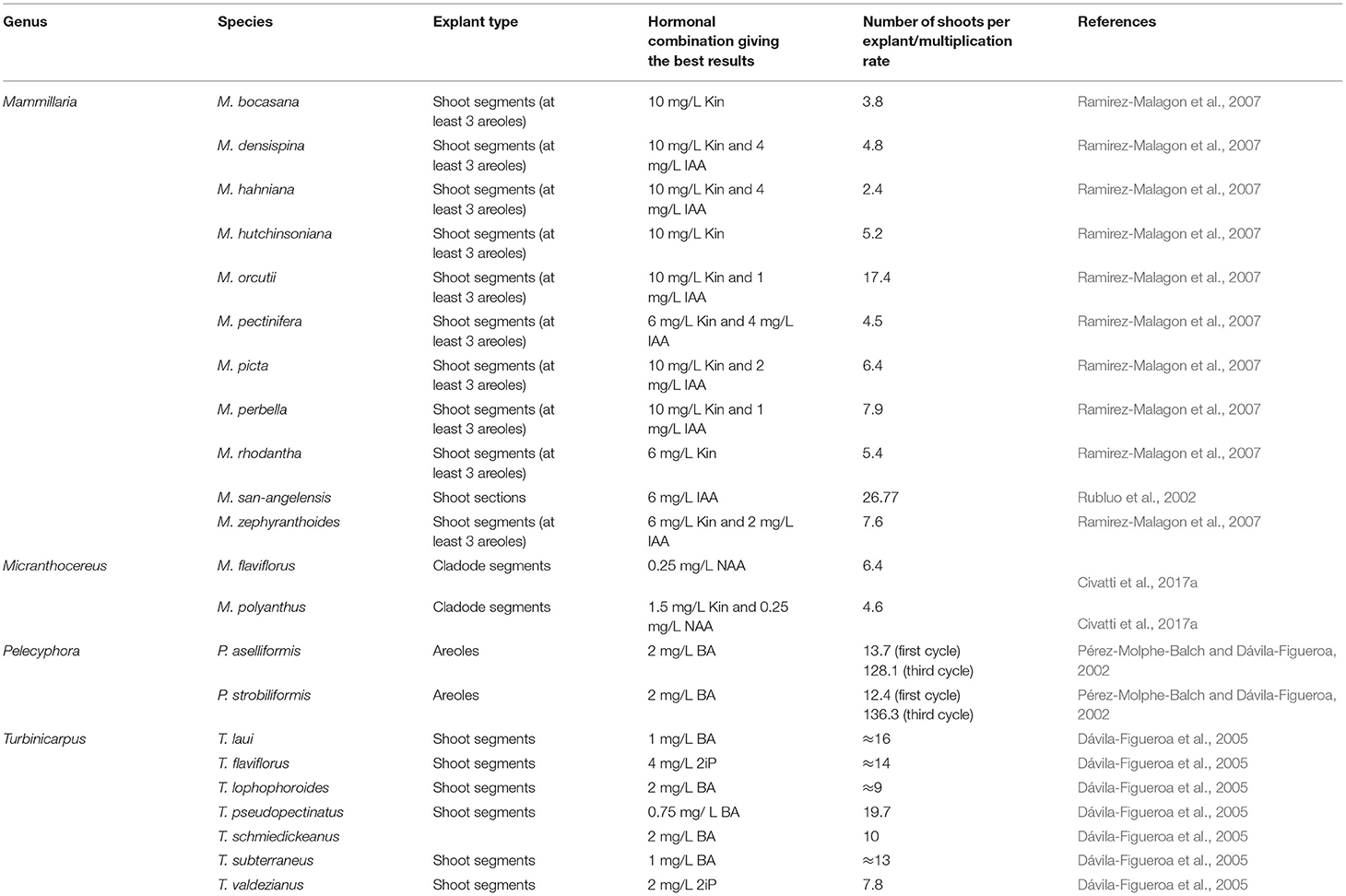
Table 2. In vitro clonal propagation of cacti species via direct organogenesis reported in previous studies.
In Mammillaria genus, culture medium supplementation with kinetin and IAA at different amounts stimulated adventitious shoot development (Ramirez-Malagon et al., 2007). The highest organogenic response was recorded in the presence of 10 mg/L of Kin for six different Mammillaria species (M. hutchisoniana, M. picta, M. orcutii, M. bocasana, M. hahniana, and M. perbella), while the lower concentration of kinetin (6 mg/L) was sufficient to induce the adventitious shoot formation for M. rhodantha, M. densispina, M. zephyranthoides, and M. pectinifera (Ramirez-Malagon et al., 2007). Nevertheless, the propagation rate was highly surpassed by combining IAA with Kin (4 mg/L IAA and 6 mg/L Kin) for this species (Ramirez-Malagon et al., 2007). However, other reports showed that the presence of cytokinin is not required for cacti in vitro propagation. It was previously underlined that adventitious shoot formation can also be stimulated in the absence of plant growth regulators or the presence of auxin alone (Papafotiou et al., 2001). In M. elongata, Papafotiou et al. (2001) recorded a better organogenic response in the absence of plant growth regulators or the presence of 0.1 mg/L of NAA. For M. sanangelensis, another member of the genus, a maximum proliferation rate was achieved in the presence of 6 mg/L of NAA (Rubluo et al., 2002). In M. flaviflorus and M. densiflorus, the best organogenic response was, however, observed in the presence of a lower NAA concentration (0.25 mg/L of NAA), which suggests that the presence of auxin is, in some cases, prerequisite for adventitious shoot induction (Civatti et al., 2017a,b).
Constraints of In Vitro Propagation in Cacti
Fungal and Bacterial Contamination
Cactus micropropagation is usually initiated by growing plants outdoors or under greenhouse conditions or from in vitro plants obtained from seeds (Lema-Rumińska and Kulus, 2014). Therefore, disinfection or surface sterilization of plant material (seeds or in vivo plant material) is mandatory to eliminate bacterial and fungal contamination present on the surface of plant material (Pérez-Molphe-Balch et al., 2015). Several disinfecting agents were applied for cacti surface sterilization, such as sodium hypochlorite (present in commercial bleach and other reagents), which has been widely used; however, its efficiency remains low. Combining sodium hypochlorite with ethanol appeared to be more effective to neutralize the exogenous microbiota of several cacti species; thus, this procedure has been widely employed (Estrada-Luna et al., 2008; Bhau and Wakhlu, 2015). In Coryphantha elephantidens, three-step disinfection was used for surface sterilization of young shoots, that is, thorough washing with tap water and 1% of detergent, a 1 min soaking in ethanol (70:30 v/v), and immersion in sodium hydrochloride for 15 min (Bhau and Wakhlu, 2015). The aseptic culture of Opuntia robusta cladodes was obtained by soaking in a biocide solution (comprising 3 mg/L benlate, 3 mg/L captan, 1 ml/L previcur, 0.5 g/L amoxicillin, and 0.4% of ketoconazole) before the application of hypochlorite solution. Within this disinfection protocol, the surface sterilization efficiency was nearby 90% (Astello-García et al., 2013). While using flowers as explants for micropropagation of Mammillaria genus (M. albicoma, M. carmenae, and M. schiedeana), Wyka et al. (2009) performed the surface sterilization of flowers by soaking them in 70% of ethanol for 1 min, followed by an active shaking in sodium hypochlorite solution (0.15% (v/w) available chlorine) for 5 min.
Besides sodium hypochlorite, other disinfecting agents, such as mercury chloride, have been tested in combination with alcohol for surface sterilization. For instance, Chornobrov and Bilous (2021) succeeded in the establishment of aseptic cultures from Christmas cactus (S. truncata) areoles (isolated from phylloclades), with more than 90% of decontaminated explants using a hydro-alcoholic solution of ethyl alcohol (70:30 v/v), followed by submersion of 0.1% of mercury chloride solution.
As an attempt to overcome the contamination issue encountered with the in vitro propagation of cacti using in vivo plant material, several authors initiated aseptic culture from in vitro germinated seeds. O. ficus-indica seeds were disinfected using 20% sodium hypochlorite combined with Tween 20 for 5–10 min, following a deep cleaning under running tap water (Heikal et al., 2021). Cortés-Olmos et al. (2018) used ethanol and sodium hypochlorite for Lophophora williamsii seeds' disinfection. For sweet cactus (Nopalea cochenillifera) species, the lowest contamination percentage was obtained when seeds were disinfected with 70% of ethanol for 1 min, followed by a deep soaking in a commercial bleach solution (sodium hypochlorite) at 1.5% for 10 min (Castro et al., 2011). Resende et al. (2021) applied a higher concentration of ethanol (96%) and sodium hypochlorite (2%) for the surface sterilization of Melocactus glaucescens seeds. The surface sterilization was established using 0.1% of commercial detergent (Extran) before the disinfection with 70% of ethanol for 1 and 25 min soaking in sodium hypochlorite solution at 2% (Dávila-Figueroa et al., 2005).
Explant Necrosis
Explant necrosis resulting from the phenolic compounds' release is another potential problem that has been reported with cacti micropropagation (Lema-Rumińska and Kulus, 2014). The incidence of explant necrosis in Mammillaria genus as the result of the intensive and widespread oxidation has been explained by Ramirez-Malagon et al. (2007). Phenolic compound secretion can be prevented or neutralized by the addition of antioxidant substances in the culture medium (Lema-Rumińska and Kulus, 2014). Ascorbic acid, citric acid, active charcoal, and polyvinylpolypyrrolidone (PVP) have widely been used for this purpose. For instance, Lázaro-Castellanos et al. (2018) used activated charcoal at 1 g/L for the in vitro propagation of three Mammillaria species to reduce explant oxidation. Explant browning has been controlled in H. undatus callus cultures using activated charcoal at 1 g/L (Cruz and Balendres, 2021). Mabrouk et al. (2021) also added 1 g/L of activated charcoal to the culture medium for Opuntia spp. micropropagation. A higher concentration of activated charcoal, that is, 3 g/L, was used for the in vitro germination and shoot development of Hamatocactus setispinus (Xavier and Jasmim, 2015). A lower concentration of activated charcoal was used for pitaya cultures. Lee and Chang (2022) were able to neutralize phenolic compounds secreted during the in vitro culture of pitaya (dragon fruit) through the addition of 200 mg/L of activated charcoal. Tissue oxidation was partially prevented in Nyctocereus serpentinus, Ferocactus, and Coryphantha by the addition of 2 g/L of PVP (Balch et al., 1998). A combination of antioxidant substances is required in some cases. Non-oxidized callus cultures from O. robusta have been initiated using a combination of antioxidant substances amended at different concentrations, namely activated charcoal (10 g/L), ascorbic acid (0.025 mg/L), citric acid (0.025 mg/L), and PVP (3 g/L) (Astello-García et al., 2013). It appears that many cacti produced high amounts of phenolic compounds, which lead to explant oxidation. Culture medium supplementation with the appropriate amounts of antioxidant substances prevents oxidative stress as a result of the accumulation of phenolic compounds (Lema-Rumińska and Kulus, 2014).
Hyperhydricity (Vitrification)
Hyperhydricity or vitrification is a morphological disorder occurring during the in vitro culture. In cacti, hyperhydrated plants showed an excessive accumulation of water within cells or tissues, low lignification in the inner part of the rind which enables cells to store high amounts of water causing cell enlargement, a signification reduction in photosynthetic performance and photosynthetic pigments, and impaired stomatal function resulting in a glassy transparent appearance and reduced mechanical strength (Balen et al., 2012; Borland et al., 2018; de Souza et al., 2019). Vitrified plants show a distinctive phenotype with a reduced number of spines and/or roots (Lema-Rumińska and Kulus, 2014). The vitrified shoots display structural alterations in chloroplasts and plastids such as chloroplasts dedifferentiation, thylakoid lump, plastid swelling, and phytoferritin accumulation (Balen et al., 2012; Lema-Rumińska and Kulus, 2014). As a result, hyperhydrated plants failed to survive in field conditions due to their reduced photosynthetic performances (Quiala et al., 2009; de Souza et al., 2019). This failure has been estimated in T. valdezianus up to 65% (Ht et al., 2011). This abnormal phenomenon, more likely observed in in vitro propagated plants, is seemingly linked with oxidative stress that occurs at the cellular level, as a result of stressful in vitro growth conditions such as light intensity and relative humidity, high amounts of plant growth regulators, and gas release by explants in the cultivation containers (such as petri dishes and jars) (Saher et al., 2004; Ht et al., 2011).
Hyperhydricity is a serious problem limiting in vitro propagation of many Cactaceae species (de Souza et al., 2019). de Souza et al. (2019) have reported a high percentage of hyperhydricity (88–100%) in O. stricta in vitro plants regenerated in the presence of BA. About 75% of hyperhydricity was obtained in T. valdezianus shoots regenerated through cladodes' segment culture in the presence of BA (Ht et al., 2011). Hyperhydrated shoots were also obtained while cultivating Pelecyphora aselliformis areoles in the presence of ~2 mg/L of BA (Santos-Díaz et al., 2003). A concentration of 3 mg/L of BA increased hyperhydration incidence in Pilosocereus robinii in vitro shoots (Quiala et al., 2009). In other cacti, in shoots regenerated through areoles culture, the incidence of hyperhydricity estimated was 64.3%, 71%, and 85.2% for Mammillaria pectinifera, Escobaria manima, and Pelecyphora aselliformis, respectively (Giusti et al., 2002). For Micranthocereus, Civatti et al. (2017a) reported any hyperhydrated shoots when explants were cultivated in half-strength MS/2 supplemented with various NAA and Kin concentrations.
Several attempts have been made to reduce the occurrence of hyperhydricity in cacti species. Santos-Díaz et al. (2022) have found that the hyperhydricity incidence can be minimized by decreasing the salt concentration of the medium (the use of quarter-strength MS medium for example). The reduction in salt concentration, more precisely ammonium (NH4)/nitrate (NO3) ratio, is also effective to decrease shoot hyperhydricity in T. mombergeri, and a similar finding was also reported in Cereus jamacaru. For instance, vitrified and abnormal shoots of C. jamacaru recovered with the reduction in basal salt, vitamins, and sugar concentrations (Monostori et al., 2010). The increase in calcium amount (double-dose) also prevented the hyperhydration of regenerated shoots (Santos-Díaz et al., 2022). This positive effect of calcium was previously explained by White and Broadley (2003) as the putative role of calcium in cell wall reinforcement. The high affinity of calcium is linked with the pectic chains, which provide additional rigidity to cell walls (White and Broadley, 2003). Calcium is an important element for cactus nutrition and a prerequisite for proper shoot development (Santos-Díaz et al., 2022). Vitrification can also be reduced using high amounts of gelling agents (ex. 10 g/L of Agar) that reduce water availability in the medium culture (Pérez-Molphe-Balch et al., 2002). Polyethylene glycol addition to the medium culture lowers shoot hyperhydration in several cacti species such as A. kotschoubeyanus, T. laui, T. valdezianus, and P. aselliformis (del Socorro Santos-Díaz et al., 2003, 2006; Ht et al., 2011). Besides polyethylene glycol, other chemical substances, such as gibberellic acid synthesis inhibitor: paclobutrazol, were successfully used for this purpose (Ht et al., 2011). Paclobutrazol reduces endogenous gibberellin production and results in the inhibition of cell elongation (Hedden, 2020). The use of paclobutrazol in T. valdezianus allowed the development of normal shoots without any hyperhydricity symptoms (Ht et al., 2011). Overall, minimizing the excess accumulation of water that occurs in the in vitro culture (due to high basal salt concentrations, high cytokinins, and endogenous plant hormone amounts along with high water availability) is an efficient way to minimize hyperhydricity incidence in cacti in vitro shoot development (de Souza et al., 2019).
Micropropagation Through Axillary Buds' Proliferation in Opuntia Species: a Case of Study
Genus Opuntia belongs to the Cactaceae family, subfamily Opuntioideae, tribe Opuntieae (Omar et al., 2021). Opuntia genus comprises nearby 181 species and 10 naturally occurring hybrids (Anderson, 2001). The most widespread, cultivated, well-known, and economically important species that belong to this genus is O. ficus-indica. Besides O. ficus-indica, a few species such as O. robusta, O. hyptiacantha, O. streptacantha, O. albicarpa, O. amyclaea, O. megacantha, and O. cochenillifera are cultivated in different areas of the world (Omar et al., 2021). In semi-arid land of Morocco and other Mediterranean regions, several spiny and spineless Opuntia species are cultivated, especially for fruit production or animal feeding (e.g., O. ficus-indica and O. stricta) or for ornamental purposes (O. macrocantha and O. microdasys) (Figure 4). However, the production of many Opuntia species in nature is now at risk due to overexploitation, reduction in their natural areas, and cochineal infestation (Mabrouk et al., 2021). Thus, the establishment of reliable methods for mass propagation of prickly pear cultivated species is required to meet the high market demand and, ultimately, ensure the restoration and the rehabilitation of these species in their natural environment (Mabrouk et al., 2021).
Micropropagation through axillary buds sprouting is widely used to ensure the genetic homogeneity and stability and produce true clones of plants. Axillary bud growth is mostly controlled by the shoot apex or apical dominance (Bredmose and Costes, 2017). Activation of apical dominance is triggered by the action of growth regulators such as cytokinins for areoles (axillary buds) and dormant axillary buds' sprouting and multiplication, and synergetic cytokinin and auxin combinations to prompt shoot multiplication (Ngezahayo and Liu, 2014). This process relies on the activation of dormant axillary buds' development, which involved proliferation and development of plants from lateral meristems (Ngezahayo and Liu, 2014). The shoots then can be cut off and subcultured to a rooting medium (Figure 5). This propagation is most accurate since this method involves the initiation and development of new shoots from preexisting meristems rather than cell dedifferentiation of already differentiated cells (Grafi et al., 2011).
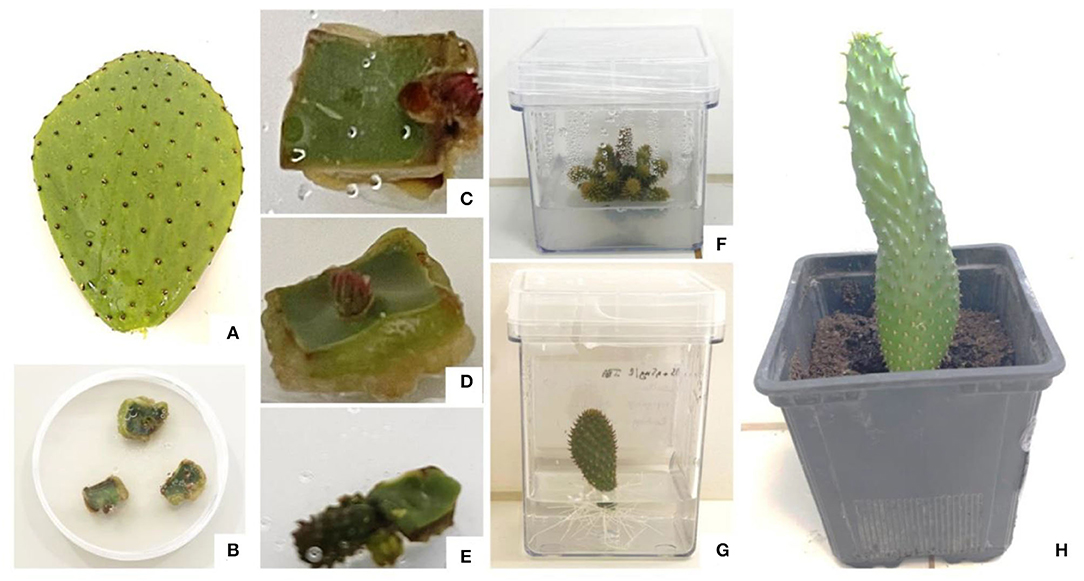
Figure 5. In vitro regeneration of Opuntia spp through axillary buds' proliferation. (A) Opuntia spp cladode collected from in vivo propagated plant, (B) areole isolation and aseptic culture establishment, (C,D) areole activation after 3 and 4 weeks of culture, respectively, (E) shoot initiation after 5 weeks of culture, (F) shoot multiplication from a single shoot, (G) in vitro rooting of in vitro shoots, and (H) acclimatization of in vitro regenerated plantlet in peat. The pictures were taken from micropropagation assays conducted at the African Sustainable Agriculture Research Institute, Mohammed VI Polytechnic University (ASARI-UM6P), Laâyoune, Morocco.
Despite several advantages offered by this method of micropropagation, there is limited research regarding the application of this method in Opuntia genus. In vitro shoot organogenesis can be applied successfully for Opuntia micropropagation, giving a high percentage of regeneration. Figure 6 demonstrates the research gaps in the use of this propagation method for prickly pear species. Till now, a limited number of studies (16 published articles from 1975 to 2021) were carried out on Opuntia species in vitro multiplication through axillary buds' sprouting and 65% of these studies were carried out on O. ficus-indica (Figure 6). Regarding geographical location, the previous works on axillary buds' proliferation were mainly conducted in Mexico and North African countries and the number of published works remains low. The success of micropropagation through axillary buds' proliferation relies on many factors, such as the explant type, age, and origin of the Opuntia plant, the mineral composition of the culture medium, and the addition of plant growth regulators in the culture medium and their concentrations.
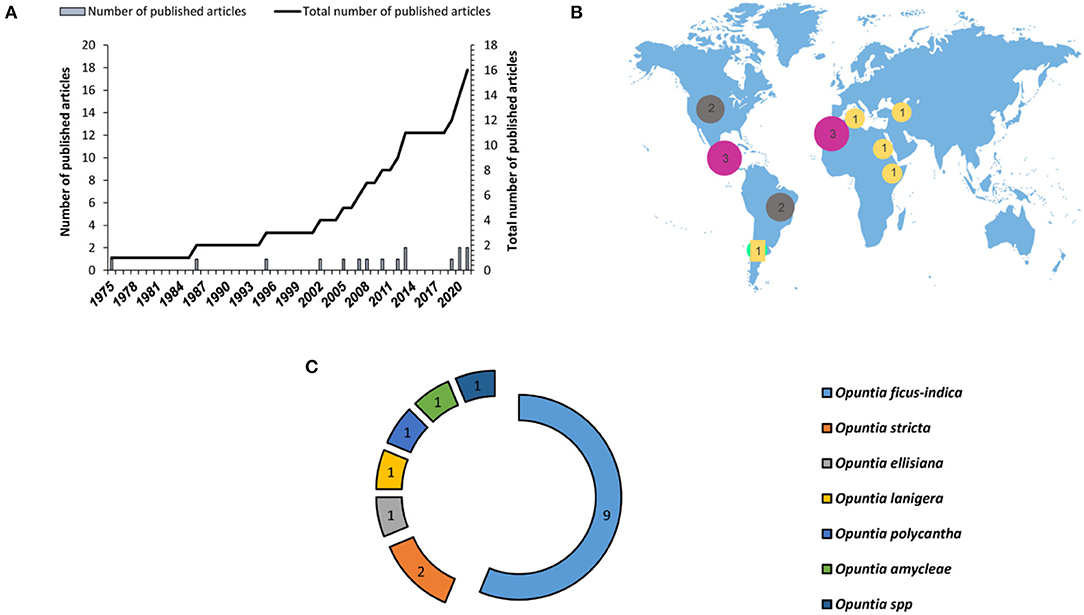
Figure 6. Number of published research articles on Opuntia micropropagation through axillary buds' proliferation. (A) Number of published articles worldwide during the last three decades, (B) geographical distribution of the published research articles, and (C) number of research studies depending on the plant species.
Explant Type
Micropropagation through axillary buds' sprouting can be initiated using any portion of plant tissue comprising areoles. As shown in Table 3, the lower shoot induction or multiplication efficiencies range from 2.0 to 2.8 shoots by a single explant, while the highest recorded was nearby 26.5 shoots per explant in one single cycle. However, if the explants are maintained on a cytokinin-enriched medium, areoles of newly developed shoots can serve as secondary explants from which new shoots can be developed. Therefore, it is possible to deploy the production of in vitro plants on large scale. For O. ficus-indica, the in vitro deployment estimated was 1,294 plants from a single explant in four cycles per year and has a potential of 456,976 plants production in a year (Rodriguez and Ramirez-Pantoja, 2020). Explants' age and origin also determine the success of this propagation method. The use of juvenile explants was proven to be highly efficient for in vitro propagation of Opuntia species. Bougdaoua and El Mtili (2020) had recently established a reliable protocol for Opuntia ficus-indica propagation using seedlings (20 mm length) using lower amounts of cytokinin (0.5 mg/L BA). Further, micropropagation efficiency can also be impacted by the explant position or orientation. For example, in O. lanigera, the vertical orientation (4.98 shoots per explant) developed more shoots per explant than in a horizontal orientation (3.69 shoots per explant) (Estrada-Luna et al., 2008). However, for O. amyclaea, the number of new shoots produced per explant was higher with explants oriented horizontally (Escobar et al., 1986).
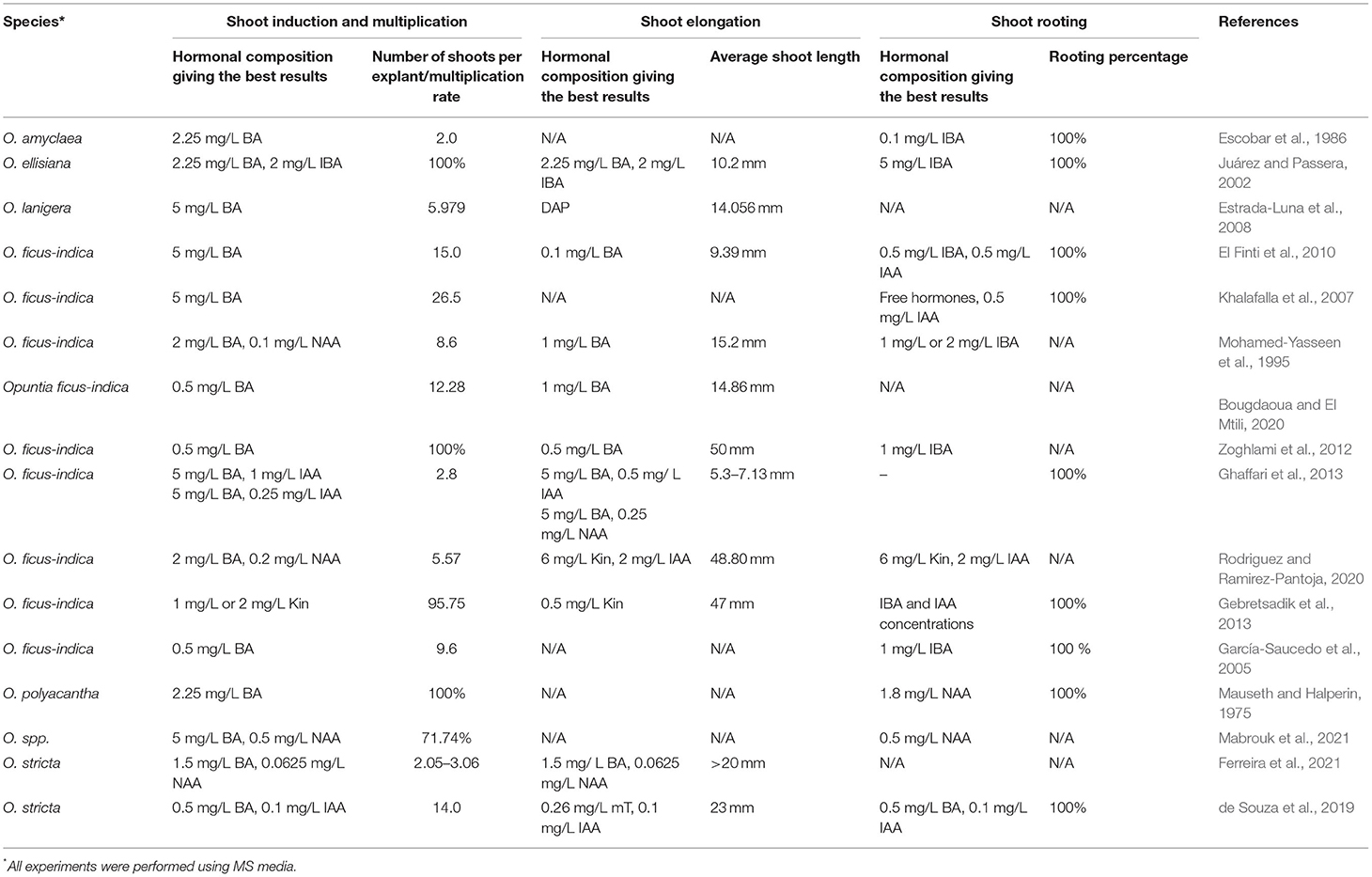
Table 3. Overview of the media culture and hormonal composition allowing the best responses for shoot induction, multiplication, and rooting reported in previous studies on Opuntia micropropagation through axillary buds' sprouting.
Aseptic Culture Establishment
The success of micropropagation through axillary buds' proliferation also depends on the disinfection procedure. The presence of glochids and thorns makes the disinfection process and the application of aseptic cultures extremely challenging. Earlier, sodium or calcium hypochlorite has been used for the surface sterilization of Opuntia species. Mabrouk et al. (2021) suggested a combination of 4.23% of calcium hypochlorite and 0.4% Tween 20 for 10 min as an efficient procedure for Opuntia spp aseptic culture establishment. Juárez and Passera (2002) succeeded in O. ellisiana surface sterilization by soaking cladodes in 20% sodium hypochlorite–Tween 80 for 10 min, followed by immersion in 1% benzalkonium chloride for 30 min. Two-step disinfection of O. amyclaea cladodes was performed by soaking in 70% alcohol for 1 min, followed by an active immersion in 60% calcium chloride for 10 min (Escobar et al., 1986). For O. lanigera, a spiny ornamental cactus, aseptic cultures have been established using 70% of ethanol for 5 min, followed by cladodes' deep immersion in a commercial bleach solution (6% of sodium hypochlorite) for 30 min (Estrada-Luna et al., 2008). However, the sodium hypochlorite–ethanol combination was not efficient for the disinfection of O. robusta and O. ficus-indica cladodes' surfaces due to the wide range of pathogens encountered in spines, areolas, and glochids. This contamination issue was overcome using biocide solution (García-Saucedo et al., 2005) or oxygenated water (30%) (Rodriguez and Ramirez-Pantoja, 2020) in combination with ethanol and commercial bleach.
Culture Media Formulation
Culture media formulation usually designates salt, vitamins, sugar, and growth regulators. Numerous culture media formulations have been used for plant micropropagation through axillary bud proliferation. The few earlier studies conducted on Opuntia genus have used MS medium (Estrada-Luna et al., 2008; Angulo-Bejarano and Paredes-López, 2011; Zoghlami et al., 2012; Ghaffari et al., 2013; Vidican, 2015; Ferreira et al., 2021; Mabrouk et al., 2021). It is widely admitted that salt formulation plays a crucial role in the prickly pear shoot initiation and development. Potassium nitrate, a major salt constituent of MS medium, is required for areoles' activation process. Potassium nitrate triggers the induction and the differentiation process of the aerial parts, and it plays the role of osmoregulator. Ferreira et al. (2021) induced shoot formation in O. stricta using a commercial nitrogen fertilizer instead of potassium nitrate and showed that MS medium can be amended with 1 g/L of a potassium nitrate-based fertilizer (Ferreira et al., 2021). Clayton et al. (1990) suggested the potential of other media formulations. These authors tested the efficiency of five different mineral formulations of culture medium (L2, Gamborg B5 (B5), SH, MS, and half-strength MS) on the number of new shoots produced by explant. They found that L2 medium was the most effective, yielding 5.5 axillary shoots per explant on average, while no significant differences in axillary shoot proliferation have been recorded between the four remaining mediums. Clayton et al. (1990) linked this great explant response with the chemical composition of the media culture, mainly high magnesium and calcium ions concentrations. In contrast, the use of a half-strength MS medium yielded the lowest axillary bud proliferation due to low salts concentration. Given the high axillary buds' proliferation obtained using the L2 medium for many cacti species including endangered ones, it could be interesting to explore the potential of this medium for in vitro shoot organogenesis.
Plant Growth Regulators
As mentioned above, the presence of specific plant growth regulators is necessary to stimulate the development of axillary buds. The latter can be assessed by removing the inhibitory effect exerted by the apical meristem on lateral or axillary buds (Bredmose and Costes, 2017). Apical dominance suppression can be triggered by cytokinin application. It was previously established that cytokinin removes apical dominance and activation of lateral buds, and Opuntia genus is not excluded from this rule. The use of cytokinin at high or moderate concentrations (1–5 mg/L BA or 1–2 mg/L Kin) was efficient to induce areoles sprouting (Table 3). For O. ficus-indica, the presence of BA is mandatory (Khalafalla et al., 2007). These authors found that MS medium supplementation with 5 mg/L BA yielded an average of 26.5 shoots per explant. Axillary buds' activation was also BA-dependent for O. polyacantha, at a concentration of 2.25 mg/L (Mauseth and Halperin, 1975). Even though the presence of cytokinin seems to be sufficient for areoles activation, several studies emphasize that the presence of auxin at low concentrations (0.0625 mg/L−0.5 mg/L NAA or 0.1 μM−1 mg/L IAA) in combination with moderate or high amounts of BA increases axillary shoot production in some prickly pear species (Table 3). For O. ellisiana, areoles activation can exclusively be stimulated using either a combination of zeatin and NAA (Clayton et al., 1990) or BA and IBA (Juárez and Passera, 2002). Shoot proliferation in O. ficus-indica was positively correlated with BA concentrations only, while no positive effect of NAA was reported during this study (El Finti et al., 2012). For other species, higher BA amounts inhibit axillary shoot development (Mohamed-Yasseen et al., 1995). Mabrouk et al. (2021) recently reported that the absence of BA reduced the caulogenesis to 0%, while it reached 100% in the presence of moderately high amounts of BA (4–6 mg/L). Media culture supplementation with both BA and Kin has an inhibitive effect on axillary shoots formation, which clearly demonstrates that the balance between endogenous and exogenous cytokinins is crucial for in vitro axillary buds' activation as previously reported by Pérez-Molphe-Balch et al. (2015).
Shoot elongation can be easily triggered by the presence of cytokinin alone or in combination with low amounts of auxins. The presence of BA alone amended is sufficient to promote shoot elongation in O. ficus-indica. The presence of BA at low concentrations (0.1–0.5 mg/L) promoted the length of regenerated shoots to a maximum of 9.39–50 mm (El Finti et al., 2010; Zoghlami et al., 2012). Higher BA concentrations (1–5 mg/L) less promoted shoot elongation in O. ficus-indica. Ghaffari et al. (2013) recorded an average of 3 mm of shoot elongation in the presence of 5 mg/L BA. Meanwhile, the combination of BA (5 mg/L) with 0.25 mg/L of NAA significantly increased shoot length (by 7.13 mm) in O. ficus-indica. The increase in shoot length was attributed to the presence of auxins, widely known for their stimulatory role in cell division and elongation (Rodriguez and Ramirez-Pantoja, 2020). Shoot elongation can also be stimulated in the presence of low Kin concentrations alone (Gebretsadik et al., 2013) or by the combination of high Kin and moderate IAA amounts (Rodriguez and Ramirez-Pantoja, 2020). Beneficial effects of cytokinin–auxin on shoot elongation have also been reported in other Opuntia species. Ferreira et al. (2021) recorded the highest O. stricta shoot length values while cultivating newly formed shoots in the presence of 1.5 mg/L of BA and 0.0625 mg/L of NAA. Thus, the combination of low-to-moderate concentrations of cytokinins and low auxin amounts is required for a great shoot elongation for Opuntia genus. Besides plant growth regulator concentrations, shoot elongation was found to be species-dependent (Table 3) and sprout lengths are highly variable (Table 3). For O. ficus-indica, the best PGR treatments allowed the elongation of newly formed shoots to reach a maximum of 50 mm (Zoghlami et al., 2012), while the highest shoot elongation was 60% and 80% lower for O. stricta and O. ellisiana shoots (i.e., 20 and 10.2 mm, respectively) (Juárez and Passera, 2002; Ferreira et al., 2021).
The final step of micropropagation through axillary buds' proliferation is related to adventitious root formation or rhizogenesis. This step is usually initiated when newly formed shoots reached a specific length. For cacti, rooting can start once the length of the produced shoots is >5 mm (Lema-Rumińska and Kulus, 2014). Rooting induction medium commonly comprises auxin sources. IBA and IAA or NAA are widely used to trigger rhizogenesis in Opuntia species (Escobar et al., 1986; Mohamed-Yasseen et al., 1995; El Finti et al., 2010; Zoghlami et al., 2012; Mabrouk et al., 2021). One hundred percent of rooting has been recorded in O. ficus-indica, O. robusta, O. amyclaea, and Opuntia spp. in the presence of low-to-moderate amounts of IBA or IAA or NAA (0.5–2 mg/L) (Table 3). However, in O. ellisiana, rhizogenesis can only be triggered in the presence of high auxin amounts (5 mg/L IBA) (Juárez and Passera, 2002). In some cases, the presence of auxin in the media culture can inhibit root formation due to the high amounts of endogenous auxins. Thus, rooting in O. ficus-indica can occur spontaneously without PGRs (Khalafalla et al., 2007; Ghaffari et al., 2013).
Conclusion
An appropriate micropropagation method is required for the mass propagation for the commercial purpose, for the conservation of endangered species, and for ecosystem conservation and restoration. Conventional propagation methods (sexual propagation through seeds, vegetative propagation by cuttings, and grafting) have been used for centuries to propagate cacti species with great interest. However, these traditional techniques cannot be implemented to mass propagate and the scale-up production of commercial species given the limited number of plants that appears to be scarce with market needs. In this context, micropropagation has been proved as a powerful tool in both experimental biotechnology (regeneration and conservation biology) and horticultural production aiming food and fruit production and for ornamental and medicinal purposes. We found that micropropagation through axillary buds' proliferation has been adopted for several cacti genera, including Mammillaria, Echinocereus, Hylocereus, and Ariocarpus; however, less research has been conducted on the Opuntia genus. Based on this review, the following prospect can be proposed:
First, one of the factors affecting the success of micropropagation is related to the removal of fungal and bacterial contaminants from plant material surfaces. Despite the wide range of disinfection procedures (cited in section Fungal and Bacterial Contamination) that have been developed, the success of these procedures is mostly dependent on the species, the nature of plant material, and its growing conditions. Significant work has to be made to establish a reliable protocol for cacti surface sterilization. In the end, the developed method should allow the removal of or the reduction in fungal and bacterial infections without affecting explants.
Second, micropropagation through axillary buds' proliferation can supply a great asset to meet market needs for human uses (fruits) and animal forage. However, the success of this propagation method depends on the growth conditions (media culture composition). The previous studies were focused on the use of one single mineral culture formulation, that is, MS medium in combination with plant growth regulators, such as BA, NAA, IAA, and IBA. Better results can be achieved using other media culture formulations as demonstrated by Clayton et al. (1990) in other cacti species. Therefore, future research is required on testing the impact of the mineral formulation of the culture medium on the regeneration process.
Third, the overexploitation and the cochineal infestation of Opuntia species limit considerably the maintenance and the preservation of these species in their natural areas, one of the solutions that can be improved brought to explore genetic diversity. Some of the presented methods of propagation can contribute to and/or increase genetic diversity. Those methods should be used to develop tolerant/resistant genotypes and ecotypes.
Last, Opuntia and other cacti display great nutritional and medicinal properties conferred by the presence of bioactive compounds. In vitro culture techniques can be used to increase the production of these high-value chemical compounds. Cell dedifferentiation (callogenesis) and organogenesis can offer a promising pathway to produce high amounts of plant-derived secondary metabolites. Cell suspensions (derived from cell dedifferentiation) should be used for the large-scale production of these bioactive molecules (in bioreactors) to meet the increasing demand for plant-derived secondary metabolites for human health.
Author Contributions
SB: conceptualization, methodology, investigation, data analysis, interpretation, writing–original draft, and review and editing. EE, LK, KD, HB, and MS: methodology and review and editing. YE: conceptualization, methodology, validation, review and editing, project administration, and supervision. All authors have read and approved the final version of the document.
Funding
This research was funded by OCP Phosboucraâ Foundation, Laâyoune, Grant No. PR008.
Conflict of Interest
The authors declare that the research was conducted in the absence of any commercial or financial relationships that could be construed as a potential conflict of interest.
Publisher's Note
All claims expressed in this article are solely those of the authors and do not necessarily represent those of their affiliated organizations, or those of the publisher, the editors and the reviewers. Any product that may be evaluated in this article, or claim that may be made by its manufacturer, is not guaranteed or endorsed by the publisher.
References
Al-Khayri, J. M., Jain, S. M., and Johnson, D. V. (2018). Advances in Plant Breeding Strategies: Fruits. Cham: Springer. doi: 10.1007/978-3-319-91944-7
Al-Ramamneh, E. A.-D., Sriskandarajah, S., and Serek, M. (2006). Plant regeneration via somatic embryogenesis in Schlumbergera truncata. Plant Cell Tissue Organ Cult. 84, 333–342 doi: 10.1007/s11240-005-9042-6
Angulo-Bejarano, P. I., and Paredes-López, O. (2011). Development of a regeneration protocol through indirect organogenesis in prickly pear cactus [Opuntia ficus-indica (L.) Mill]. Sci. Hortic. 128, 283–288. doi: 10.1016/j.scienta.2011.01.030
Ashrafzadeh, S.. (2020). In vitro grafting–twenty-first century's technique for fruit tree propagation. Acta Agric. Scand. Sect. B Soil Plant Sci. 70, 404–405. doi: 10.1080/09064710.2020.1754452
Astello-García, M. G., Robles-Martínez, M., Barba-de la Rosa, A. P., and del Socorro Santos-Díaz, M. (2013). Establishment of callus from Opuntia robusta Wendl., a wild and medicinal cactus, for phenolic compounds production. Afr. J. Biotechnol. 12, 3204–3207
Badalamenti, O., Carra, A., Oddo, E., Carimi, F., and Sajeva, M. (2016). Is in vitro micrografting a possible valid alternative to traditional micropropagation in Cactaceae? Pelecyphora aselliformis as a case study. SpringerPlus 5, 1–4. doi: 10.1186/s40064-016-1901-6
Balch, E. P. M., Reyes, M. E. P., Amador, E. V., Rangel, E. M., Ruiz, L. R. M., and Viramontes, H. J. L. (1998). Micropropagation of 21 species of Mexican cacti by axillary proliferation. Vitro Cell. Dev. Biol. Plant. 34, 131–135. doi: 10.1007/BF02822777
Balen, B., Tkalec, M., Štefanić, P. P., Vidaković-Cifrek, Ž., and Krsnik-Rasol, M. (2012). In vitro conditions affect photosynthetic performance and crassulacean acid metabolism in Mammillaria gracilis Pfeiff tissues. Acta Physiol. Plant 34, 1883–1893. doi: 10.1007/s11738-012-0986-y
Bhau, B., and Wakhlu, A. (2015). A highly efficient in vitro propagation protocol for elephant tusk cactus: Coryphantha elephantidens (Lem.) Lem. J. Genet. Eng. Biotechnol. 13, 215–219. doi: 10.1016/j.jgeb.2015.07.003
Borland, A. M., Leverett, A., Hurtado-Castano, N., Hu, R., and Yang, X. (2018). “Functional anatomical traits of the photosynthetic organs of plants with crassulacean acid metabolism,” in The Leaf: A Platform for Performing Photosynthesis. Cham: Springer, 281–305. doi: 10.1007/978-3-319-93594-2_10
Bouamama, B., Ben Salem, A., Zoghlami, N., Zemni, H., Mliki, A., and Ghorbel, A. (2011). Somatic embryogenesis and plantlet regeneration from immature anthers of Opuntia ficus-indica. J. Hortic. Sci. Biotechnol. 86, 313–318. doi: 10.1080/14620316.2011.11512766
Bougdaoua, H., and El Mtili, N. (2020). In vitro regeneration of two northern Moroccan Opuntia ficus-indica (L.) Mill. genotypes. Moroccan J Biol. 17, 36–42.
Bredmose, N., and Costes, E. (2017). Axillary bud growth. Reference Module in Life Sciences, 1–10. doi: 10.1016/B978-0-12-809633-8.05056-1
Casas, A., and Barbera, G. (2002). Mesoamerican domestication and diffusion. Cacti Biol. Uses 143, 62. doi: 10.1525/california/9780520231573.003.0009
Castro, J. P., Araújo, E. R., Rêgo, M. M., and Rêgo, E. R. (2011). In vitro germination and disinfestation of sweet cactus (Nopalea cochenillifera (L.) Salm Dyck). Acta Sci. Agron. 33, 509–512. doi: 10.4025/actasciagron.v33i3.6275
Chornobrov, O., and Bilous, S. (2021). In vitro plant regeneration of Christmas cactus ((Haw.) Moran) by indirect morphogenesis. Folia For. Pol. 63, 68–73. doi: 10.2478/ffp-2021-0007
Civatti, L., Marchi, M., Schnadelbach, A., and Bellintani, M. (2017b). In vitro multiplication and genetic stability of two species of Micranthocereus Backeb. (Cactaceae) endemic to Bahia, Brazil. Plant Cell Tissue Organ. Cult. 131, 537–545. doi: 10.1007/s11240-017-1304-6
Civatti, L. M., Marchi, M. N. G., and Bellintani, M. C. (2017a). Micropropagation of two species of Micranthocereus (Cactaceae) with ornamental potential native to Bahia, Brazil. Afr. J. Biotechnol. 16, 749–762. doi: 10.5897/AJB2016.15901
Clayton, P. W., Hubstenberger, J. F., Phillips, G. C., and Butler-Nance, S. A. (1990). Micropropagation of members of the Cactaceae subtribe Cactinae. J. Am. Soc. Hortic. Sci. 115, 337–343. doi: 10.21273/JASHS.115.2.337
Cortés-Olmos, C., Gurrea-Ysasi, G., Prohens, J., Rodríguez-Burruezo, A., and Fita, A. (2018). In vitro germination and growth protocols of the ornamental Lophophora williamsii (Lem.) Coult. as a tool for protecting endangered wild populations. Sci. Hortic. 237, 120–127. doi: 10.1016/j.scienta.2018.03.064
Cruz, M. A., and Balendres, M. A. (2021). Dragon fruit calli development and fungal contamination as influenced by activated charcoal. J. Prof. Assoc. Cactus Dev. 23, 58–73.
Dávila-Figueroa, C. A., de la Rosa-Carrillo, L., and Pérez-Molphe-Balch, E. (2005). In vitro propagation of eight species or subspecies of Turbinicarpus (Cactaceae). Vitro Cell. Dev. Biol. Plant 41, 540–545. doi: 10.1079/IVP2005668
de Medeiros, L. A., de Ribeiro, R. C. S., Gallo, L. A., De Oliveira, E. T., and Demattê, M. E. S. P. (2006). In vitro propagation of Notocactus magnificus. Plant Cell Tissue Organ Cult. 84, 165–169. doi: 10.1007/s11240-005-9014-x
de Souza, L. M., Barbosa, M. R., Zárate-Salazar, J. R., Lozano-Isla, F., and Camara, T. R. (2019). Use of meta-Topolin, an unconventional cytokinin in the in vitro multiplication of Opuntia stricta haw. Biotecnol. Veg. 19, 85–97.
del Socorro Santos-Díaz, M., Elizalde-Rodríguez, C., and de Lourdes Santos-Díaz, M. (2006). Effect of coconut water, darkness and auxins on morphogenesis of Ariocarpus kotschoubeyanus (Cactaceae). Bradleya 2006, 83–88. doi: 10.25223/brad.n24.2006.a8
del Socorro Santos-Díaz, M., Méndez-Ontiveros, R., Arredondo-Gómez, A., and de Lourdes Santos-Díaz, M. (2003). Clonal propagation of Turbinicarpus laui Glass and Foster, a cactus threatened with extinction. Bradleya 2003, 7–12. doi: 10.25223/brad.n21.2003.a3
Díaz, M., del, S. S., de la Rosa, A.-P. B., Héliès-Toussaint, C., Guéraud, F., and Nègre-Salvayre, A. (2017). Opuntia spp.: characterization and benefits in chronic diseases. Oxid. Med. Cell. Longev. 2017, 1–17. doi: 10.1155/2017/8634249
El Finti, A., El Boullani, R., Belayadi, M., Ait Aabd, N., and El Mousadik, A. (2010). Micropropagation in vitro of Opuntia ficus-indica in Southern Morocco. Acta Hortic. 995, 93–98. doi: 10.17660/ActaHortic.2013.995.11
El Finti, A., El Boullani, R., El Ayadi, F., Ait Aabd, N., and El Mousadik, A. (2012). Micropropagation in vitro of Opuntia ficus-indica in south of Morocco. Int. J. Biol. Chem. Sci. 1, 6–10.
El-Mostafa, K., El Kharrassi, Y., Badreddine, A., Andreoletti, P., Vamecq, J., El Kebbaj, M. S., et al. (2014). Nopal cactus (Opuntia ficus-indica) as a source of bioactive compounds for nutrition, health and disease. Molecules 19, 14879–14901. doi: 10.3390/molecules190914879
Escobar, A. H. A, Villalobos, A. V. M, and Villegas, M. A. (1986). Opuntia micropropagation by axillary proliferation. Plant Cell Tissue Organ. Cult. 7, 269–277. doi: 10.1007/BF00037744
Estrada-Luna, A., Lopez-Peralta, C., and Cardenas-Soriano, E. (2002). In vitro micrografting and the histology of graft union formation of selected species of prickly pear cactus (Opuntia spp.). Sci. Hortic. 92, 317–327. doi: 10.1016/S0304-4238(01)00296-5
Estrada-Luna, A. A., de Jesús Martínez-Hernández, J., Torres-Torres, M. E., and Chable-Moreno, F. (2008). In vitro micropropagation of the ornamental prickly pear cactus Opuntia lanigera Salm-Dyck and effects of sprayed GA3 after transplantation to ex vitro conditions. Sci. Hortic. 117, 378–385. doi: 10.1016/j.scienta.2008.05.042
Ferreira, C. C. S., Paz, C. D., Souza, J. C., Peixoto, A. R., Rios, L. S., Nascimento, A. R., et al. (2021). Development of the prickly pear cactus Opuntia stricta (Haw.) Haw. (Cactaceae) in vitro in response to the replacement of potassium nitrate for a commercial kno 3 fertilizer. Ciênc. Rural 52, 1–6. doi: 10.1590/0103-8478cr20200122
García-Rubio, O., and Malda-Barrera, G. (2010). Micropropagation and reintroduction of the endemic Mammillaria mathildae (Cactaceae) to its natural habitat. HortScience 45, 934–938. doi: 10.21273/HORTSCI.45.6.934
García-Saucedo, P. A., Valdez-Morales, M., Valverde, M. E., Cruz-Hernandez, A., and Paredes-Lopez, O. (2005). Plant regeneration of three Opuntia genotypes used as human food. Plant Cell Tissue Organ Cult. 80, 215–219. doi: 10.1007/s11240-004-9158-0
Gebretsadik, H., Mekbib, F., and Abraha, E. (2013). Influence of cytokinin and auxin types and concentrations on in vitro shoot and root regeneration of cactus pear Opuntia ficus-indica (L.) mill.. J. Sci. Sustain. Dev. 1, 85–96. doi: 10.20372/au.jssd.1.1.2013.011
Ghaffari, A., Hasanloo, T., and Nekouei, M. K. (2013). Micropropagation of tuna (Opuntia ficus–indica) and effect of medium composition on proliferation and rooting. Int J Biosci. 3, 129–139. doi: 10.12692/ijb/3.11.129-139
Giusti, P., Vitti, D., Fiocchetti, F., Colla, G., Saccardo, F., and Tucci, M. (2002). In vitro propagation of three endangered cactus species. Sci. Hortic. 95, 319–332. doi: 10.1016/S0304-4238(02)00031-6
Glimn-Lacy, J., and Kaufman, P. B. (2006). Botany Illustrated: Introduction to Plants, Major Groups, Flowering Plant Families. New York: Springer.
Gomes, F. L. A. F., Heredia, F. F., Silva, P. B., Facó, O., and de Paiva Campos, F. A. (2006). Somatic embryogenesis and plant regeneration in Opuntia ficus-indica (L.) Mill. (Cactaceae). Sci. Hortic. 108, 15–21. doi: 10.1016/j.scienta.2005.12.007
González-Stuart, A. E., and Rivera, J. O. (2019). “Nutritional and therapeutic applications of prickly pear cacti,” in Bioactive Food as Dietary Interventions for Diabetes (2nd ed.), eds R. R. Watson and V. R. Preedy (Cambridge: Academic Press), 349–360. doi: 10.1016/B978-0-12-813822-9.00023-0
Grafi, G., Florentin, A., Ransbotyn, V., and Morgenstern, Y. (2011). The stem cell state in plant development and in response to stress. Front. Plant Sci. 2, 53. doi: 10.3389/fpls.2011.00053
Hedden, P.. (2020). The current status of research on gibberellin biosynthesis. Plant Cell Physiol. 61, 1832–1849. doi: 10.1093/pcp/pcaa092
Heikal, A., Abd El-Sadek, M. E., Salama, A., and Taha, H. S. (2021). Comparative study between in vivo-and in vitro-derived extracts of cactus (Opuntis ficus-indica L. Mill) against prostate and mammary cancer cell lines. Heliyon 7, e08016. doi: 10.1016/j.heliyon.2021.e08016
Ht, G. O., Mendoza, A. B., Bocardo, L. E., Quintanilla, J. V., and Oviedo, E. C. (2011). Hyperhydricity control of in vitro shoots of Turbinicarpus valdezianus (Möller) GL and F. Phyton 80, 175. doi: 10.32604/phyton.2011.80.175
Hughes, F. M.. (2017). Spatial pattern and cover effect on the abundance of a currently obliterated population of Melocactus violaceus: a threatened species in Brazilian sandy coastal plain. Rev. Bras. Bot. 40, 915–922. doi: 10.1007/s40415-017-0402-3
ICARDA (2021). “Cactus pear, a drought-tolerant crop grown by millions of farmers in dry areas for nutritional and income generating purposes,” in Panorama Solutions for Healthy Planet. Available online at: https://panorama.solutions/en/solution/cactus-pear-drought-tolerant-crop-grown-millions-farmers-dry-areas-nutritional-and-income#:~:text=Cactus%20is%20a%20multifunctional%20crop,farmer%20can%20generate%20more%20income (accessed May 29, 2022).
Inglese, P., Liguori, G., and De La Barrera, E. (2017). Ecophysiology and reproductive biology of cultivated cacti. Crop ecology, cultivation and uses of cactus pear crop ecology, cultivation. Food and Agriculture Organization of the United Nations and the International Center for Agricultural Research in the Dry Areas Rome, 43–50.
Ivannikov, R., Lobova, O., Ivannikova, N., and Krasnienkova, I. (2021). Micropropagation and organogenesis of Astrophytum asterias (Zuccà Lem. (Cactaceae Juss.), Blossfeldia liliputiana Werderm and Strombocactus disciformis (DC. Britton & Rose. J. Microbiol. Biotechnol. Food Sci. 11, e2201. doi: 10.55251/jmbfs.2201
Jedidi, E., Mahmoud, K., Kaaniche-Elloumi, N., and Jemmali, A. (2013). SEM and histological analysis of somatic embryogenesis performed on cactus pear (Opuntia ficus-indica (L.) mill.) ovules explants. Acta Hortic. 1067, 231–238 doi: 10.17660/ActaHortic.2015.1067.32
Jesus, I. S. D. D., Conceição, L. P., Schnadelbach, A. S., Assis, J. G. D. A., and Carvalho, M. L. S. D. (2019). Genetic evidence of multiple reproductive strategies in a microendemic and threatened cactus (Cactaceae: Discocactus pfeiff ) in Bahia, Brazil. Acta Bot. Bras. 33, 437–445. doi: 10.1590/0102-33062018abb0393
Juárez, M. C., and Passera, C. B. (2002). In vitro propagation of Opuntia ellisiana Griff. and acclimatization to field conditions. Biocell 26, 319–324. doi: 10.32604/biocell.2002.26.319
Khalafalla, M. M., Abdellatef, E., Mohameed Ahmed, M. M., and Osman, M. G. (2007). Micropropagation of cactus (Opuntia ficus-indica as strategic tool to combat desertification in arid and semi arid regions. Int. J. Sustain. Crop Prod. 2, 1–8.
Lázaro-Castellanos, J. O., Mata-Rosas, M., González, D., Arias, S., and Reverchon, F. (2018). In vitro propagation of endangered Mammillaria genus (Cactaceae) species and genetic stability assessment using SSR markers. In Vitro Cell. Dev. Biol. Plant. 54, 518–529. doi: 10.1007/s11627-018-9908-z
Lee, Y.-C., and Chang, J.-C. (2022). Development of an improved micropropagation protocol for red-fleshed pitaya ‘Da Hong' with and without activated charcoal and plant growth regulator combinations. Horticulturae 8, 104. doi: 10.3390/horticulturae8020104
Lema-Rumińska, J., Goncerzewicz, K., and Gabriel, M. (2013). Influence of abscisic acid and sucrose on somatic embryogenesis in cactus Copiapoa tenuissima Ritt. forma mostruosa. Sci. World J. 2013, 1–7 doi: 10.1155/2013/513985
Lema-Rumińska, J., and Kulus, D. (2012). Induction of somatic embryogenesis in Astrophytum asterias (Zucc.) Lem. in the aspect of light conditions and auxin 2, 4-D concentrations. Acta Sci. Pol. Hortorum Cult. 11, 77–87.
Lema-Rumińska, J., and Kulus, D. (2014). Micropropagation of cacti-a review. Haseltonia. 2014, 46–63. doi: 10.2985/026.019.0107
Lopes, E. B., Costa, L. B., Cordeiro Junior, A. F., and Brito, C. H. (2013). Rendimentoe aspectos fenológicos de espécie de palma forrageira em relação ao cultivo com dois tipos de cladódios. Cien. Tecnol. Agropecuaria 7, 59–61.
Mabrouk, A., Abbas, Y., El Goumi, Y., El Maaiden, E., El Kharrassi, Y., Antry-Tazi, S. E., et al. (2021). Optimization of prickly pear cacti (Opuntia spp.) micropropagation using an experimental design method. J. Microbiol. Biotechnol. Food Sci. 11, e1577. doi: 10.15414/jmbfs.1577
Mauseth, J. D., and Halperin, W. (1975). Hormonal control of organogenesis in Opuntia polyacantha (Cactaceae). Am. J. Bot. 62, 869–877. doi: 10.1002/j.1537-2197.1975.tb14127.x
Moebius-Goldammer, K. G., Mata-Rosas, M., and Chávez-Avila, V. M. (2003). Organogenesis and somatic embryogenesis in Ariocarpus kotschoubeyanus (Lem.) K. Schum. (Cactaceae), an endemic and endangered Mexican species. Vitro Cell. Dev. Biol. Plant 39, 388–393. doi: 10.1079/IVP2003427
Moghadam, A. R. L., Ardebili, Z. O., and Rezaie, L. (2012). Effect of indole butyric acid on micrografting of cactus. Afr. J. Biotechnol. 11, 6484–6493. doi: 10.5897/AJB11.2672
Mohamed-Yasseen, Y.. (2002). Micropropagation of pitaya (Hylocereus undatus Britton et Rose). Vitro Cell. Dev. Biol.-Plant. 38, 427–429. doi: 10.1079/IVP2002312
Mohamed-Yasseen, Y., Barringer, S. A., Splittstoesser, W. E., and Schnell, R. J. (1995). Rapid propagation of tuna (Opuntia ficus-indica) and plant establishment in soil. Plant Cell Tissue Organ Cult. 42, 117–119. doi: 10.1007/BF00037690
Monostori, T., Tanács, L., and Mile, L. (2010). Studies on in vitro propagation methods in cactus species of the genera Melocactus, Cereus and Lobivia. Acta Hortic. 937, 255–261. doi: 10.17660/ActaHortic.2012.937.31
Monroy-Vázquez, M. E., Peña-Valdivia, C. B., García-Nava, J. R., Solano-Camacho, E., Campos, H., and García-Villanueva, E. (2017). Imbibition, viability and vigor of seeds of four Opuntia species with different degree of domestication. Agrociencia 51, 27–42. doi: 10.1080/01919512.2016.1261010
Nefzaoui, A., Louhaichi, M., and Ben Salem, H. (2014). Cactus as a tool to mitigate drought and to combat desertification. J. Arid Land Stud. 24, 121–124.
Ngezahayo, F., and Liu, B. (2014). Axillary bud proliferation approach for plant biodiversity conservation and restoration. Int. J. Biodivers. 2014, 727025. doi: 10.1155/2014/727025
Omar, A. A., ElSayed, A. I., and Mohamed, A. H. (2021). “Genetic diversity and ecotypes of Opuntia spp.,” in Opuntia spp.: Chemistry, Bioactivity and Industrial Applications. Cham :Springer, 181–199. doi: 10.1007/978-3-030-78444-7_8
Papafotiou, M., Balotis, G. N., Louka, P. T., and Chronopoulos, J. (2001). In vitro plant regeneration of Mammillaria elongata normal and cristate forms. Plant Cell Tissue Organ Cult. 65, 163–167. doi: 10.1023/A:1010601328667
Pérez-Molphe-Balch, E., and Dávila-Figueroa, C. A. (2002). In vitro propagation of Pelecyphora aselliformis Ehrenberg and P. strobiliformis Werdermann (Cactaceae). Vitro Cell. Dev. Biol.-Plant 38, 73–78. doi: 10.1079/IVP2001248
Pérez-Molphe-Balch, E., Pérez-Reyes, M. E., Dávila-Figueroa, C. A., and Villalobos-Amador, E. (2002). In vitro propagation of three species of columnar cacti from the Sonoran Desert. HortScience 37, 693–696. doi: 10.21273/HORTSCI.37.4.693
Pérez-Molphe-Balch, E., Santos-Díaz, M. S., Ramírez-Malagón, R., and Ochoa-Alejo, N. (2015). Tissue culture of ornamental cacti. Sci. Agric. 72, 540–561. doi: 10.1590/0103-9016-2015-0012
Poljuha, D., Balen, B., Bauer, A., Ljubeši,ć, N., and Krsnik-Rasol, M. (2003). Morphology and ultrastructure of Mammillaria gracillis (Cactaceae) in in vitro culture. Plant Cell Tissue Organ Cult. 75, 117–123. doi: 10.1023/A:1025030115905
Quiala, E., Matos, J., Montalvo, G., de Feria, M., Chávez, M., Capote, A., et al. (2009). In vitro propagation of Pilosocereus robinii (Lemaire) Byles et Rowley, endemic and endangered cactus. J. Prof. Assoc. Cactus Dev. 11, 18–25.
Ramirez-Malagon, R., Aguilar-Ramirez, I., Borodanenko, A., Perez-Moreno, L., Barrera-Guerra, J., Nuñez-Palenius, H., et al. (2007). In vitro propagation of ten threatened species of Mammillaria (Cactaceae). Vitro Cell. Dev. Biol. Plant 43, 660–665. doi: 10.1007/s11627-007-9076-z
Resende, S. V., Lima-Brito, A., Silva, G. T., and Santana, J. R. F. D. (2021). In vitro seed germination and plant growth of “Cabeça-de-frade” (Cactaceae) Rev. Caatinga 34, 1–8. doi: 10.1590/1983-21252021v34n101rc
Rodriguez, O. J. L., and Ramirez-Pantoja, P. E. (2020). Micropropagation of selected materials of Opuntia ficus indica L through culture in vitro of areola. J Appl Biotechnol Bioeng. 7, 121–126 doi: 10.15406/jabb.2020.07.00225
Rubluo, A., Marin-Hernández, T., Duval, K., Vargas, A., and Márquez-Guzmán, J. (2002). Auxin induced morphogenetic responses in long-term in vitro subcultured Mammillaria san-angelensis Sánchez-Mejorada (Cactaceae). Sci. Hortic. 95, 341–349. doi: 10.1016/S0304-4238(02)00040-7
Saher, S., Piqueras, A., Hellin, E., and Olmos, E. (2004). Hyperhydricity in micropropagated carnation shoots: the role of oxidative stress. Physiol. Plant 120, 152–161. doi: 10.1111/j.0031-9317.2004.0219.x
Sala, J., Mangolin, C. A., Franzoni, J., and Machado, Mde F. (2011). Esterase polymorphism and the analysis of genetic diversity and structure in cactus populations descended from Cereus peruvianus plants regenerated in vitro. Biochem. Genet. 49, 270–282. doi: 10.1007/s10528-010-9405-5
Santos-Díaz, M. D. S., Méndez-Ontiveros, R., Arredondo-Gómez, A., and Santos-Diaz, M. D. L. (2003). In vitro organogenesis of Pelecyphora aselliformis Erhenberg (Cactaceae). Vitro Cell. Dev. Biol. Plant 39, 480–484. doi: 10.1079/IVP2003456
Santos-Díaz, M. D. S., Santos-Díaz, M., and Alvarado-Rodríguez, J. (2022). In vitro regeneration of the endangered cactus Turbincarpus mombergeri (Riha), a hybrid of T. laui × T. pseudopectinatus. Plant Cell Tissue Organ Cult. (PCTOC) 148, 271–279. doi: 10.21203/rs.3.rs-742548/v1
Shedbalkar, U. U., Adki, V. S., Jadhav, J. P., and Bapat, V. A. (2010). Opuntia and other cacti: applications and biotechnological insights. Trop. Plant Biol. 3, 136–150. doi: 10.1007/s12042-010-9055-0
Stambouli-Essassi, S., Zakraoui, M., Bouzid, S., and Harzallah-Skhiri, F. (2017). Sexual Propagation of the tunisian spinescent opuntia ficus-indica (L.) Mill., morphogenetic deployment and polymorphism. Notulae Botan. Horti Agrobot. Cluj Napoca 45, 50–58. doi: 10.15835/nbha45110600
Vidican, I.. (2015). Study on ability to regenerative organogenesis Opuntia (Tourney.) Mill. fragilis var. fragilis in vitro currently grown in substrate a mixture of equal amounts of 3-indolebutyric (IBA) and benzyladenine (BA). Analele Univ. Din Oradea Fasc. Protectia Mediu. 24, 111–118.
Wakhlu, A., and Bhau, B. S. (2000). Callus formation and plant regeneration from tubercles of Coryphantha elephantidens (Lem.) Lem. Vitro Cell. Dev. Biol. Plant 36, 211–214. doi: 10.1007/s11627-000-0039-x
White, P. J., and Broadley, M. R. (2003). Calcium in plants. Ann. Bot. 92, 487–511. doi: 10.1093/aob/mcg164
Wyka, T., Wróblewska, M., and Hamerska, M. (2009). Use of cactus flowers as explants for micropropagation. J. Hortic. Sci. Biotechnol. 84, 454–458. doi: 10.1080/14620316.2009.11512548
Wyka, T. P., Hamerska, M., and Wroblewska, M. (2006). Organogenesis of vegetative shoots from in vitro cultured flower buds of Mammillaria albicoma (Cactaceae). Plant Cell Tissue Organ Cult. 87, 27–32. doi: 10.1007/s11240-006-9128-9
Xavier, P. B., and Jasmim, J. M. (2015). In vitro germination and acclimatization of Hamatocactus setispinus. Ornam. Hortic. 21, 193–200. doi: 10.14295/aohl.v21i2.589
Keywords: areoles, Cactaceae, in vitro culture, multiplication, Opuntia
Citation: Bouzroud S, El Maaiden E, Sobeh M, Devkota KP, Boukcim H, Kouisni L and El Kharrassi Y (2022) Micropropagation of Opuntia and Other Cacti Species Through Axillary Shoot Proliferation: A Comprehensive Review. Front. Plant Sci. 13:926653. doi: 10.3389/fpls.2022.926653
Received: 22 April 2022; Accepted: 08 June 2022;
Published: 07 July 2022.
Edited by:
Joydeep Banerjee, Indian Institute of Technology Kharagpur, IndiaReviewed by:
Jean Carlos Cardoso, Federal University of São Carlos, BrazilSofia D. Carvalho, Universidad San Francisco de Quito, Ecuador
Copyright © 2022 Bouzroud, El Maaiden, Sobeh, Devkota, Boukcim, Kouisni and El Kharrassi. This is an open-access article distributed under the terms of the Creative Commons Attribution License (CC BY). The use, distribution or reproduction in other forums is permitted, provided the original author(s) and the copyright owner(s) are credited and that the original publication in this journal is cited, in accordance with accepted academic practice. No use, distribution or reproduction is permitted which does not comply with these terms.
*Correspondence: Youssef El Kharrassi, eW91c3NlZi5lbGtoYXJyYXNzaUB1bTZwLm1h