- 1Guangdong Key Laboratory for Innovative Development and Utilization of Forest Plant Germplasm, Guangzhou, China
- 2College of Forestry and Landscape Architecture, South China Agricultural University, Guangzhou, China
Castanopsis hystrix is one of the main timber trees grown in China. However, severe shortage of natural seeds and the difficulty of explant regeneration has limited seedling supply. As such, there is a need for research on asexual multiplication of C. hystrix. This study established a rapid propagation technology system for C. hystrix genotypes, including explant treatment, proliferation, and rooting. HZ (a modified MS medium) supplemented with 4.4 μM BA and 0.5 μM IBA was found to be the optimal medium for shoot sprouting. The maximum proliferation coefficient and the number of effective shoots was obtained on HZ medium supplemented with 2.6 μM BA and 1.0 μM IBA, were 3.00 and 5.63, respectively. A rooting rate of 83.33% was achieved using half-strength HZ medium supplemented with 3.2 μM NAA. Adding vitamin C (80 mg⋅l–1) for 7 days in a dark environment reduced the browning rate, while increasing the proliferation rate. Additionally, through cytological observation, we established how and where adventitious roots occur. The survival rate of transplanted plantlets was > 90%. This is the first report of an in vitro regeneration technique that uses stem segments of mature C. hystrix as explants.
Introduction
Castanopsis hystrix belongs to the genus Castanopsis of the Fagaceae family, mainly distributed in the tropical and subtropical regions of southern China, and has very important economic, ecological and social value (Su et al., 2004; Wu et al., 2012; Liu et al., 2020; Souma and Ishikawa, 2020). As a native broad-leaved precious timber tree species, its seeds can be used to extract tanning agents and foods rich in tannins and starch (Chen et al., 1993a,b; Tanaka et al., 1993; Chang et al., 1995). Its rapid growth also has significant ecological functions such as water and soil conservation, disaster prevention, and mitigation. Moreover, they play a critical role in biodiversity and the global carbon budget (Xiaofang et al., 2015; You et al., 2018; Liang et al., 2019; Zhang et al., 2019).
Difficulties in breeding have restricted the application of superior genotypes of C. hystrix. Currently, seedling production is the primary breeding method. However, seedling production is difficult because of the phenomenon of “biennial fruiting” and difficulties in seed storage. The different genotypes have considerable growth rate, material, and seed yield variations. Moreover, the seedlings are not consistent with the growth characteristics of mother trees (San-José et al., 2010). Although the grafting method has been reported, the survival rate is only 44%, and there is a “partial crown” phenomenon (Baofu et al., 2018). A lack of sufficient branches also limits large-scale production (Ahuja and Jain, 2017).
Biotechnological methods may be valuable in the propagation and conservation of selected genotypes (Gaidamashvili and Benelli, 2021). In vitro propagation is not only less affected by the external environment but can also produce orderly and consistent young plants with ideal characteristics (Kumar et al., 2011; Martínez et al., 2021; Chakraborty et al., 2022). Adult trees are difficult to use as material because of the large number of endophytic bacteria, difficulty establishing an aseptic system, and shoot induction and differentiation, which dramatically limits in vitro propagation (Martínez et al., 2017a, 2021). Therefore, it is essential to establish an efficient propagation technology for mature C. hystrix. In a prior study, explored the technique of using the seeds of mature C. hystrix as explants for tissue culture (Ruchun et al., 2016; Chunyan and Suyun, 2017). Research using mature C. hystrix branches as a material for in vitro culture techniques is limited.
In the present study, we established an efficient and rapid propagation protocol to ensure the fixation of these ideal traits to the next generation. The findings of this study can be used for clonal propagation and germplasm conservation of superior genotypes of C. hystrix.
Materials and methods
Plant materials and culture conditions
Semi-lignified branches were collected from a miniature grafting nursery (Figure 2B) in the College of Forestry and Landscape Architecture’s greenhouse, South China Agricultural University (113°21 E, 23°09 N). The grafting material was obtained from a mature tree (over 100 years old, Figure 2A), having a favorable genotype. It has the advantages of firmness and high hardness of wood.
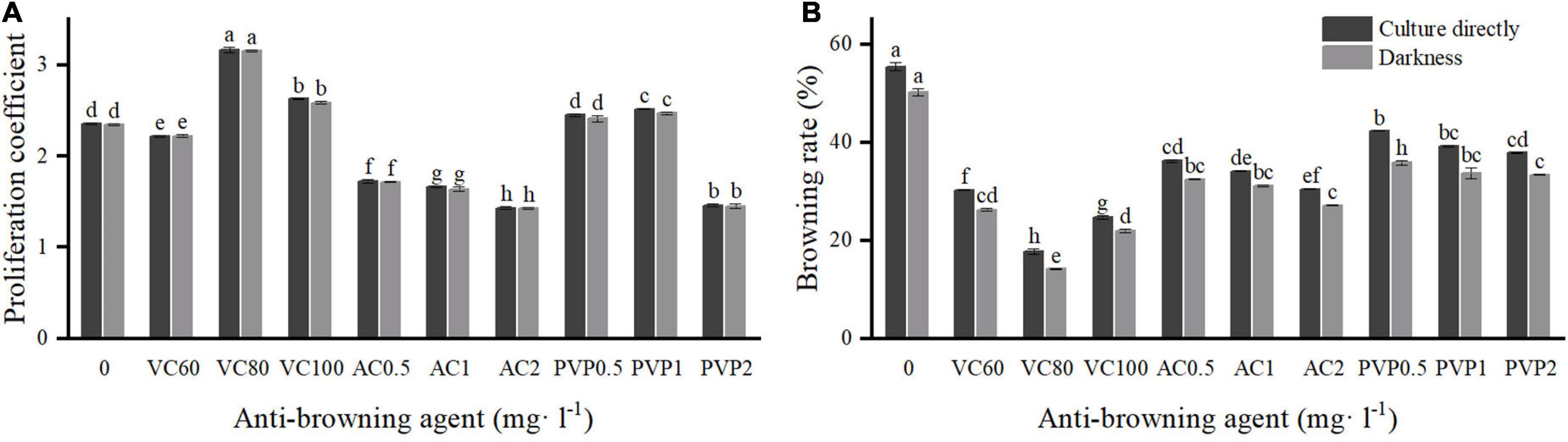
Figure 1. Effects of different types and concentrations of anti-browning agents on shoot proliferation and browning. Group a (left) was cultured directly under light. Group b (right) was transferred to light culture after a 7-day darkness treatment. (A) Proliferation coefficient; (B) browning rate. Different uppercase letters in the same column indicate a significant difference, as per Duncan’s multiple range test (p ≤ 0.05, n = 3 indicates three replicates).
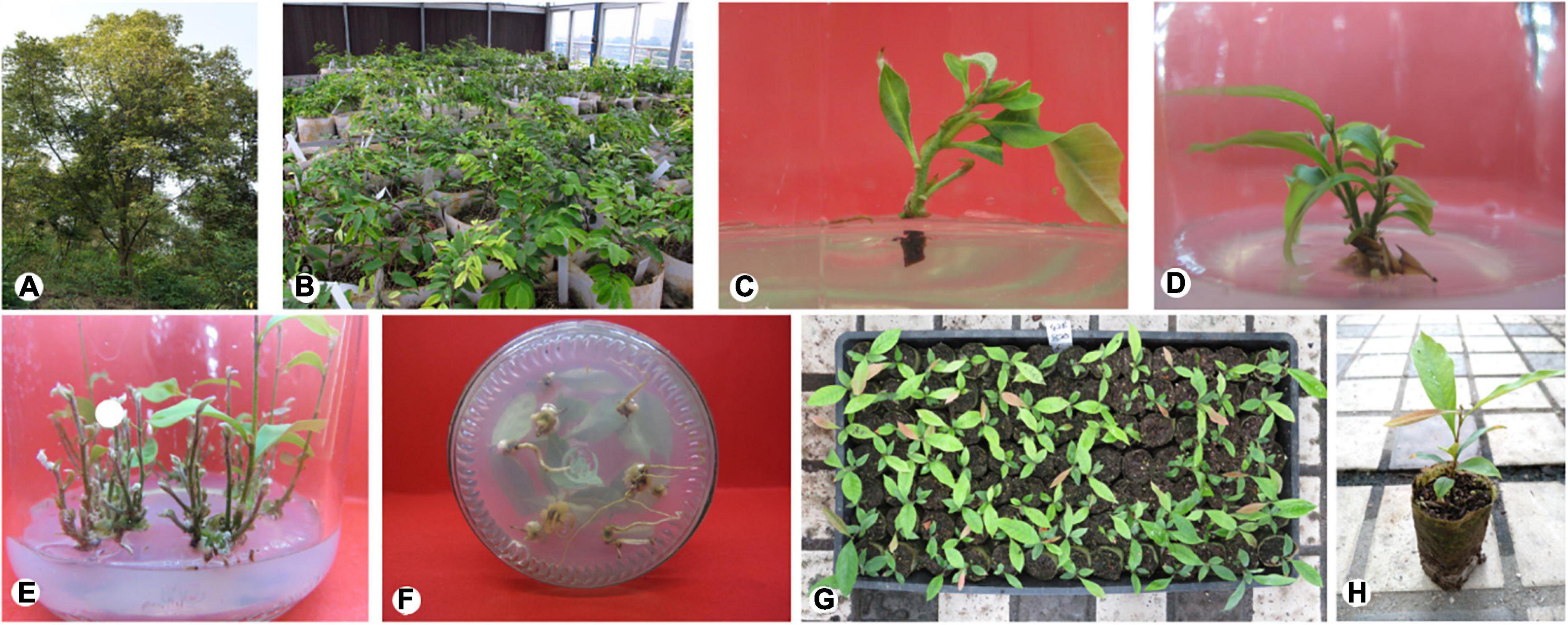
Figure 2. Micropropagation of mature Castanopsis hystrix trees using axillary node explants. (A) Mother plant; (B) the miniature grafting nursery; (C) shoot sprouting; (D,E) shoot proliferation; (F) rooting; (G) plantlet acclimatization for 30 days; (H) plantlet acclimatization for 90 days.
All culture media were adjusted to pH 5.7, solidified with 6 g⋅l–1 agar, and autoclaved at 121°C for 20 min (Corredoira et al., 2006). For the sprouting and proliferation, 30% sucrose was added in the regeneration medium while for root induction 15% sucrose was supplied (Purohit et al., 2002). After inoculation, the plantlets were incubated in a growth chamber with a 12 h/day photoperiod (provided by cool-white fluorescent lamps at 50–60 μmol m–2 s–1) at 23 ± 2°C (Martínez et al., 2017b).
Disinfection and shoot sprouting
Branches approximately 5–15 cm in length were collected as the initial material. After two-thirds of the leaves were cut off, they were washed with 5% (v: v) liquid detergent (consisting of surfactant and soft water) solution, rinsed under running water for 1 h, and then moved to a clean bench. Initial materials were cut into stem segments with 1–2 axillary shoots (1–2 cm in length) and then placed into sterile glass bottles according to the degree of lignification (semi-lignified). Thirty explants were placed in each bottle for surface disinfection. A total of 6 treatments of disinfection were used in this study (Table 1). After disinfection, both ends of the wound of explants were cut (0.5–1.5 cm) and inoculated on MS supplemented with 4.4 μM BA and 0.5 μM indole-3-butyric acid (IBA). The contamination, browning, and survival rates of each treatment were recorded after 30 days.
To optimize shoot sprouting, we chose five different basal media containing BA 4.4 μM and IBA 0.5 μM for comparison (Table 2). Among them, HZ is a medium that has been modified from MS. The macronutrient component of the HZ medium is composed of 520 mg⋅l–1 NH4NO3; 950 mg⋅l–1 KNO3, 495 mg⋅l–1 KH2PO4, 370 mg⋅l–1 MgSO4⋅7H2O, and 440 mg⋅l–1 CaCl2⋅2H2O, other mineral nutrients are the same in composition as in MS. On this basis, to further optimize the shoot sprouting, BA (2.2, 4.4 and 8.8 μM), α-naphthaleneacetic acid (NAA) (0, 0.5 and 2.7 μM), and IBA (0, 0.5 and 2.5 μM) with different combinations were added to medium. Nine treatments were designed based on the L9 (34) orthogonal test (Table 3). Observations were made after 30 days, including shoot sprouting rate, shoot length, and growth state.
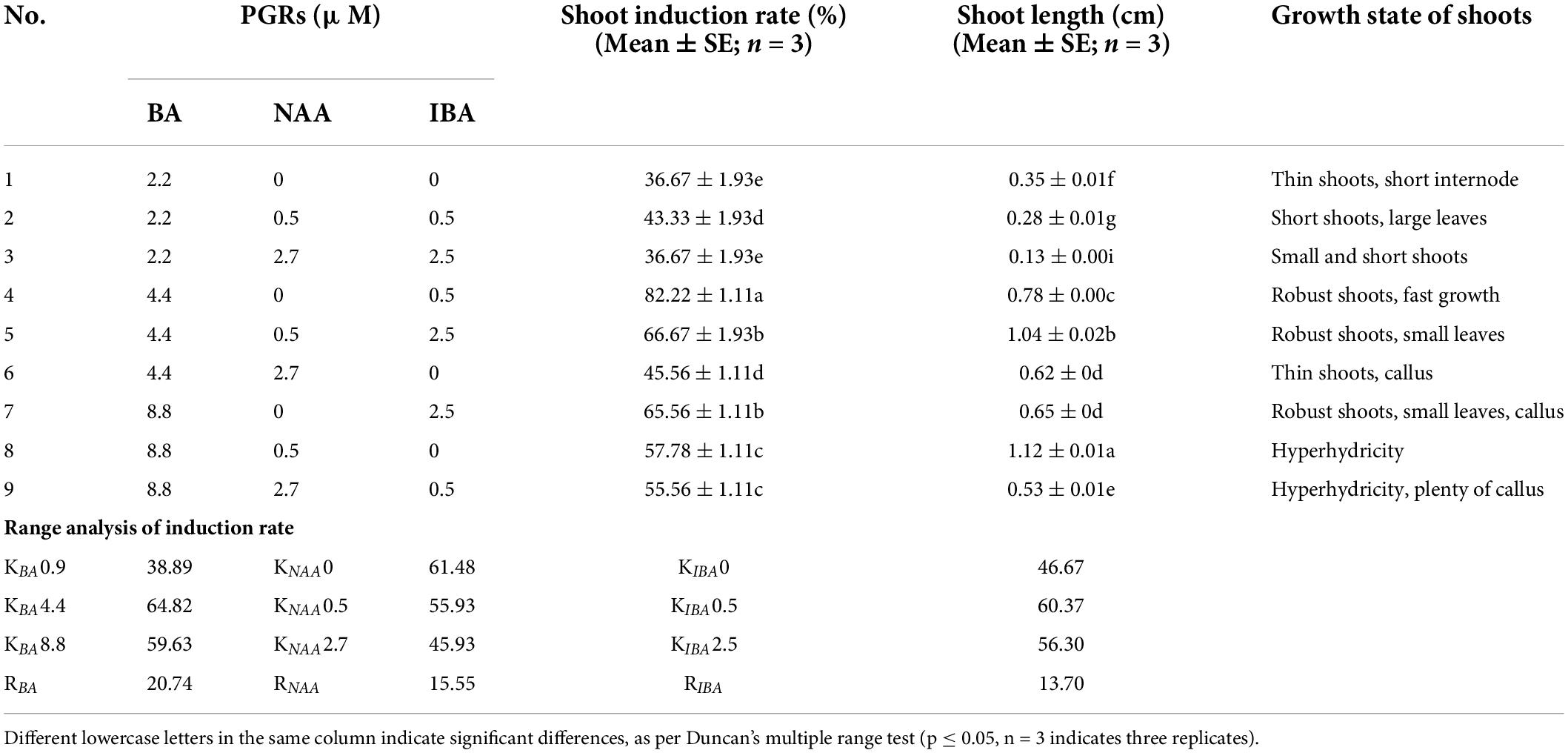
Table 3. Effects of different concentrations and compositions of BA, NAA, and IBA on shoot induction, and range analysis.
Shoot proliferation
The induced shoots were cut into approximately 1-cm sections and transferred into HZ medium. BA (0.9, 2.6 and 4.4 μM), NAA (0, 0.5 and 1.1 μM), and IBA (0, 0.5 and 1.0 μM), in different concentrations, were added to the medium. Nine treatments were designed according to the L9 (34) orthogonal test (Table 4). After 7 weeks, the proliferation coefficient, number of effective shoots (≥0.5 cm) per explant, and growth state were recorded.
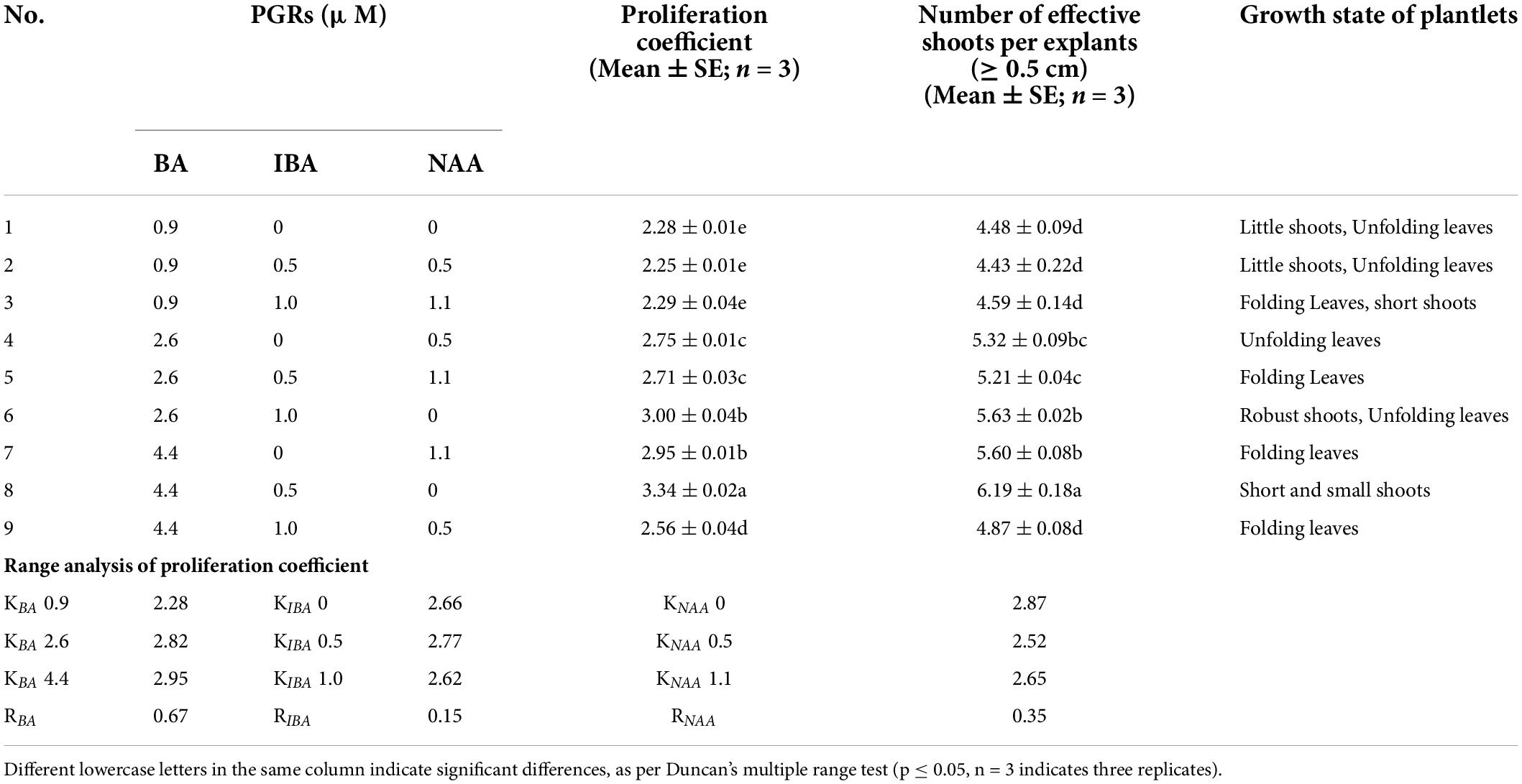
Table 4. Effects of different concentrations and compositions of BA, IBA, and NAA on shoot proliferation, and range analysis.
To reduce the browning rate, three anti-browning agents at different concentrations (Figure 1) were added to the proliferation medium for the experiment. There were a total of 10 treatments. Two groups of comparative experiments were set up. The first group was cultured directly under light conditions after inoculation, and the other group was cultured in the dark for 7 days and then transferred to a light culture. The browning rate was calculated after 14 days, and the proliferation coefficient was recorded after 7 weeks.
Rooting
Robust shoots (2–3 cm in length) were harvested and transferred to half-strength HZ medium supplemented with different concentrations of NAA (1.1, 2.2, 3.2, 4.3, 5.4, and 8.1 μM) and IBA (1.0, 2.0, 2.9, 3.9, 4.9, and 7.4 μM) (Table 5). After 30 days of culture, rooting rate and growth state were recorded.
Histology observation of differentiating root in microshoots
To investigate the rooting mechanism of C. hystrix plantlets, the formation of adventitious roots was observed by agarose sectioning. Starting from the induction of rooting culture, the bases of the 5–10 plantlets (0.5–1.0 cm in length) were cut and collected every 2 days and stored in a fixation medium [70% alcohol, acetic acid, and 38% formaldehyde (18: 1: 1 v: v: v)]. Then, the same volume of a mixture of TO (TO type biological tablet transparent agent) transparent agent and anhydrous ethanol, 30% anhydrous ethanol, and 70% TO transparent agent were used to treat the samples for 1 h. Finally, the samples were embedded in 3% agarose, cooled, solidified, and sliced with a vibratome (VT1000S, Leica, GER), with a thickness of 35 μm. They were then stained with toluidine blue, observed, and photographed using a microscope (BX43, Olympus, Japan).
Acclimatization and transplantation
After 30 days of rooting culture, 1,000 plantlets with well-developed roots were placed in the greenhouse for 7–10 days. The plantlets were removed from the culture vessels, and the attached medium was washed away. Subsequently, the plantlets were transplanted to containers (containing a mixture of peat soil, perlite, and coconut bran 3: 1: 1 v: v: v). Before transplanting, the substrate was disinfected with potassium permanganate (800–1,000 ppm). Plantlets were watered thoroughly and kept in a greenhouse (25 ± 1°C, 80% RH, 70% shading). One week after transplanting, spray with 1% carbendazim and 2 weeks later, they were moved outdoors, and water and fertilizer (N: 10 g⋅l–1, P2O5: 10 g⋅l–1, K2O: 10 g⋅l–1, Fe: 0.05 g⋅l–1, Mn: 0.05 g⋅l–1, Nutrient solution and water 1:1,000 v: v) management were conducted daily. Survival rates were recorded after 30 days.
Statistical analysis
The following formulas were used to calculate different plant regeneration parameters:
• Contamination rate (%) = number of contaminated explants/total number of explants × 100
• Browning rate (%) = number of browned explants/total number of explants × 100
• Survival rate (%) = number of surviving explants/total number of explants × 100
• Shoot sprouting rate (%) = number of sprouted explants/total number of explants × 100
• Proliferation coefficient = Total number of shoot ≥ 0.5 cm (in length)/Total number of regenerating explants
• Number of effective shoots per explant = total number of shoots ≥ 0.5 cm
• Rooting rate (%) = number of rooted explants/total number of explants × 100.
• Average root number per explant = total number of roots/numbers of rooted explants
All experiments were conducted in a completely randomized design with three replicates, and each treatment contained 30 explants, except for acclimatization. The results are presented as mean ± standard error (SE). The mean and SE values were calculated using Microsoft Excel 2019. IBM SPSS Statistics v26 (Armonk, NY, United States) was used for the statistical analyses. The significance of differences among the mean values was calculated using Duncan’s multiple range test at p ≤ 0.05. The results are presented as mean ± standard error of three replicates.
Results
Establishment of an aseptic system
Among the six treatments (Table 1), the best result was obtained with the combination of 1% (v: v) benzalkonium bromide for 2–4 min and 0.1% (w: v) mercuric chloride containing 2–3 drops of Tween 80 for 1–4 min. The survival rate of explants was up to 75%, and the contamination and browning rates were the lowest at 17 and 11%, respectively. This shows that multistage disinfection can significantly reduce the contamination rate of explants. This is consistent with the results of other researchers (Kuppusamy et al., 2019). Compared with treatment No. 5, the contamination rate of explants in treatment No. 4 without the addition of Tween 80 was significantly higher. Treatment No. 1, in which NaOCl alone was used as a disinfectant, had the highest contamination rate. The contamination rate of explants in treatments No. 3 and 6, in which 75% (v: v) ethanol was used, was significantly lower than that in treatment No. 1, but the browning rate was highest, at 25 and 27%, respectively.
Shoot sprouting
Among the five media (Table 2), modified MS medium (HZ) was the optimal medium. We found that explants cultured on MS, 1/2 MS, and Gamborg’s B5 basal medium (B5) had a very low induction rate, and the induced shoots were short, thin and gradually died after 2–3 cycles of culturing. Shoots cultured on MS and B5 media showed hyperhydricity. The effect of shoot sprouting cultured on 1/4 MS was moderate; the shoots were thin and weak, and the stem segments were yellow-green. In contrast, the HZ medium positively influenced shoot sprouting; the shoot sprouting rate reached 71.11%, and the shoot length reached 0.95 cm. Moreover, the shoots grown on HZ medium were robust and green in color and elongated faster.
The results (Table 3) showed that different concentrations and combinations of the plant growth regulators (PGRs) significantly affected shoot sprouting. The optimal combination of PGRS for C. hystrix was BA 4.4 μM and IBA 0.5 μM, wherein the shoot sprouting rate reached 82.22%, and the induced shoots were robust and grew rapidly (Figure 2C). In the culture medium containing 2.2 μM BA, the shoot sprouting rate was low, and the induced shoots were short. However, when BA reached 8.8 μM, hyperhydricity was observed in the shoots. Moreover, excessive auxin may lead to callus formation, in addition to inhibition of shoot sprouting.
Shoot proliferation
The findings of this study suggest that different concentrations and ratios of PGRs (BA, IBA, and NAA) can promote the proliferation of C. hystrix plantlets to different degrees (Table 4). In the range of 0.9–4.4 μM of BA, the combination of BA and a single auxin can significantly improve the proliferation of plantlets. However, when the concentration of BA was 0.9 μM, the overall proliferation coefficient was the lowest. Range analysis showed that the influence of the three PGRs on shoot proliferation was BA > NAA > IBA. According to the range values, when the concentration of BA was 4.4 μM and that of IBA was 0.5 μM, the proliferation coefficient of shoots reached the highest (Figures 2D,E, 3.34). However, under these conditions, the cultured shoots were short, the leaves were folded, and fewer shoots were suitable for rooting. By contrast, the shoots cultured on a medium provided with BA (2.6 μM) and IBA (1.0 μM) were robust, the leaves were extended, and there were many shoots suitable for rooting, with the proliferation coefficient reaching 3.00 and the number of effective shoots reaching 5.63.
We observed that the proliferation coefficient of C. hystrix plantlets cultured in darkness for 7 days decreased slightly, but the browning rate was significantly lower compared with culturing directly under light after inoculation. Furthermore, the addition of vitamin C (VC), activated charcoal (AC), and polyvinyl pyrrolidone (PVP) at different concentrations affected the proliferation and browning of plantlets to different degrees (Figure 1). Compared with the control treatment (54.44%), the browning rate of plantlets was significantly reduced after adding anti-browning agents into the medium. VC showed the best anti-browning effect. When the concentration of VC was 80 mg⋅l–1, the browning rate of the plantlets was the lowest (13.33%) after dark treatment for 7 days, and there was a higher proliferation rate than in the control treatments. Under such culture conditions, the plantlets grew well, and the shoots were healthy. However, when the concentration of VC was 60 and 100 mg⋅l–1, the growth of the plantlets was poor. Different concentrations also reduced the browning rate and increased the proliferation rate. Under such culture conditions, plantlets generally grew with weak shoots and slow growth. AC had the worst anti-browning effect on C. hystrix plantlets. The addition of AC significantly reduced the proliferation coefficient, and most of the shoots died and showed poor growth.
Rooting
The results showed that the addition of different concentrations of NAA and IBA significantly improved the rooting effect of C. hystrix plantlets compared with the control treatment (Table 5). The rooting rate first increased and then decreased with the increase in auxin concentration in treatments 2–7 and 8–13. We found that when auxin concentrations were too high, there were more calluses at the base of plantlets, which affected rooting quality. When auxin concentrations were too low, the roots were thin and the rooting rate was low. In terms of the rooting rate, rooting number, and growth state of plantlets, the overall rooting effect of NAA was better than that of IBA. Among them, when the concentration of NAA was 3.2 μM, the rooting rate was the highest (83.33%), the roots were strong, and the plantlets showed a substantial growth state (Figure 2F).
Histology observation of differentiating root in microshoots
No significant change was observed after the C. hystrix plantlets were transferred into the rooting medium for 4 days. Starting from the day 5, the cambium outer cells showed periclinal division in succession and formed a ring band consisting of cells with thin walls, dense mass, and strong meristematic ability (Figures 3a,b). On day 6, local swelling at the base of the stem segment of the plantlets gradually formed calluses. These calluses were formed by resuming the division of cells surrounding the medullary rays (Figure 3c). On day 7, some active cells at the junction of the original cambium and medullary rays continued to divide into cell clusters deeper than other regions, forming the adventitious root primordium (Figure 3d). As the cells divided, the root primordium showed tissue differentiation after approximately 9 days. From the anatomical observation, the cells located at the morphological upper end of the root primordium gradually formed growth points and root caps, whereas the cells located at the lower morphological end of the root primordium were stained lighter, elongated, and formed oblong parenchymatous cells (Figures 3e,f). Around day 11, with the elongation, division, and differentiation of the root primordia cells, the root primordium penetrated the cortex and epidermis, grew out of the stem (Figure 3g), and then continued to penetrate the callus wrapped by the base of adventitious roots (Figure 3h), while the vascular cells differentiated from the adventitious root were connected to the vascular system of the stem segment (Figure 3i).
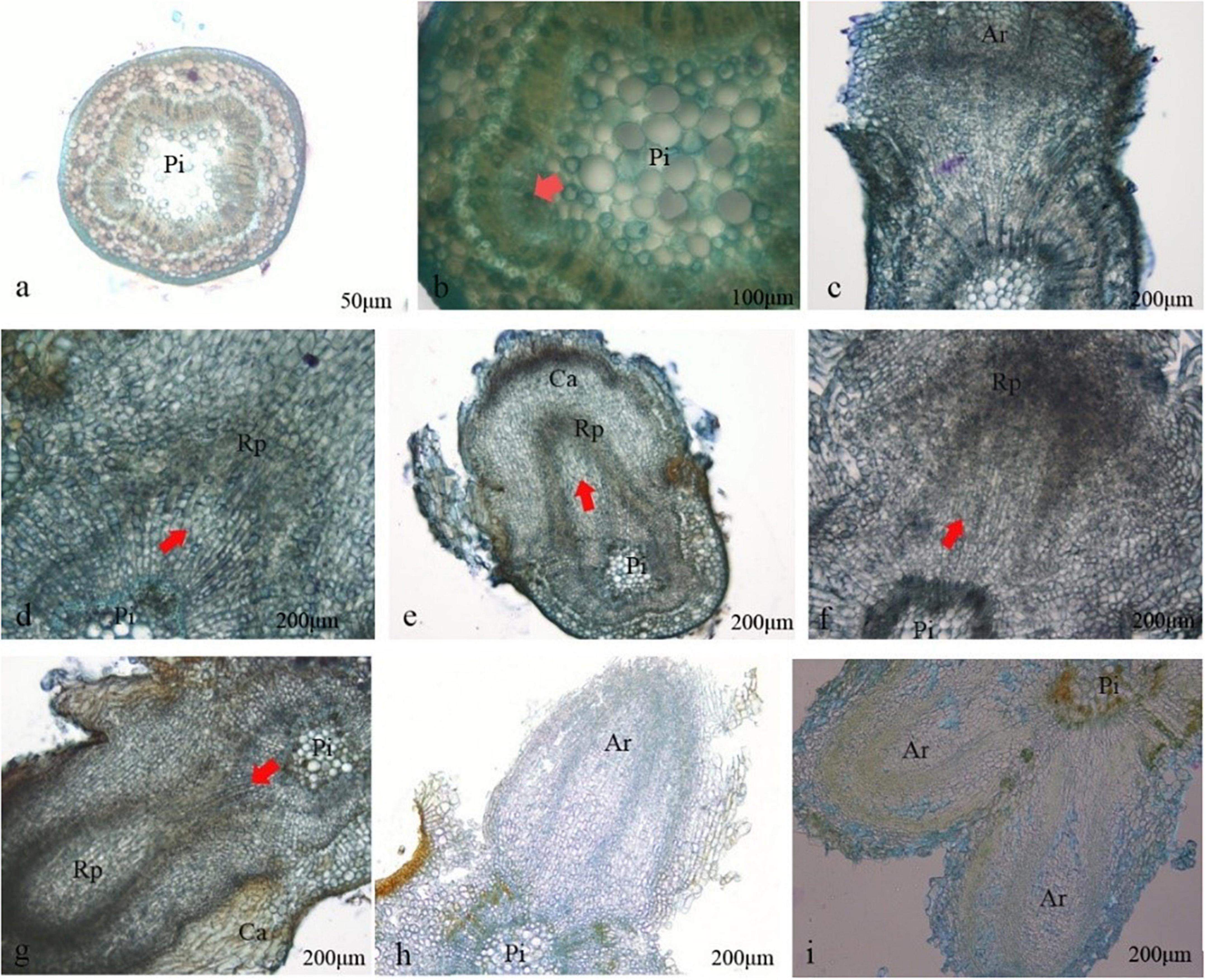
Figure 3. Histology observation on differentiating roots formation of Castanopsis hystrix plantlets. Ri, pith; Rp, root primordium; Ar, adventitious root; Ca, callus. (a) Rooted on day 4; (b) the cambium cells were active on day 5; (c) callus appeared; (d) root primordium appeared on day 7; (e,f) root primordium differentiated; (g) adventitious root broken through the cortex on day 11; (h) adventitious root broken through callus on days 12–13; (i) the adventitious root vascular connected to the stem vascular.
Acclimation and transplantation
The regenerated plantlets transplanted into plastic cups had a survival rate of over 90% after 30 days, with bright green leaves and good growth (Figures 2G,H). The lignified plants that were transferred to the large container bags grew well. The regenerated plants were phenotypically identical to the parent plants and the leaves and dark green mature leaves.
Discussion
The fuzziness of explants exposed outdoors for a long time is contaminated by various air pollution sources (Kang et al., 2020). In particular, adult trees in the wild have high levels of endophytic bacteria, resulting in tissue culture failure. Our preliminary experiments found that explants collected directly from mature trees in the field were heavily contaminated, making it difficult to establish an aseptic system. Therefore, we obtained a large amount of aseptic material by grafting superior genotypic branches as scions. Plants were cultivated in a greenhouse and regularly sprayed with 0.1% carbendazim. This approach has also been reported in other studies (Griebeler et al., 2014). The combination of Tween 80 and HgCl2 can also improve sterilization, and its superiority has been reported in previous studies (San-José et al., 2010; Martínez et al., 2017a). Our results are consistent with these findings.
During in vitro tissue culture, growth and morphology are mainly controlled by the composition of the basic culture medium (Carl and Richard, 2002; Kothari et al., 2004; Phillips and Garda, 2019). This study found that during shoot induction, except for culture on 1/4 MS and HZ, shoots induced from axillary shoots were shorter and gradually died after 2–3 cycles of culturing. It has been reported that inappropriate mineral nutrition in the medium, especially the proportion of macroelements such as N, P, and K, can affect the normal metabolism of plantlets (Nezami-Alanagh et al., 2019; Hameg et al., 2020). The experimental results showed that the low-salt medium is more conducive to the induction and growth of C. hystrix, which is the same as tissue culture research in Quercus rubra (Vengadesan and Pijut, 2009). High salt concentrations in the medium may cause hyperhydricity in plantlets (Oberschelp and Gonçalves, 2016; Nezami-Alanagh et al., 2018), which leads to chlorophyll deficiency in plantlet leaves, accumulation of water in intracellular spaces and walls, poorly developed cell walls, and reduction in cuticle thickness (Sen and Alikamanoglu, 2013; Tabart et al., 2015), as well as causes plantlets to exhibit a controlled emergency response with a phenomenon called hyperhydricity. Other nutrients can also affect plantlet growth. For example, Halloran and Adelberg (2011) found that nutrients such as Mg, Mn, and Ca affect the reproduction of Curcuma longa, and the effect of other elements on C. hystrix needs to be studied further. The optimization of a basic medium is a complex process; it can be optimized using computer technology, such as algorithm-based machine learning (artificial neural networks, fuzzy logic, and genetic algorithms) (Gago et al., 2010; Arteta et al., 2018; Nezami-Alanagh et al., 2018).
PGRs are important at all stages of plant tissue culture (Bidabadi and Jain, 2020) and are added to stimulate the formation of primordial roots in tissues presenting a rooting predisposition (Griebeler et al., 2014). In this study, BA had the most apparent effect on the induction and proliferation of C. hystrix shoots, confirming that BA plays a significant role in tissue culture (Namli et al., 2010). Cytokinins and auxins must be balanced during regeneration from explants (Skoog and Miller, 1957; Zhang et al., 2020); the concentration of PGRs was lower or higher and not conducive to the growth of shoots (Gonbad et al., 2014). The type and concentration of auxins are stricter in rooting cultures (Phillips and Garda, 2019). NAA was shown to stimulate rooting better than IBA, which is the opposite of Panax ginseng on rooting, indicating that the effect of auxins on roots varies from species to species (Kim et al., 2003). The combination of NAA and IBA has a better rooting effect (Khosh-Khui and Sink, 1982; Martínez et al., 2021); whether it applies to C. hystrix requires further verification.
The browning phenomenon is mainly caused by the action of oxidative enzymes, such as polyphenol oxidase (PPO) and phenylalanine ammonia lyase (PAL), with phenolic acid to produce toxic dark brown tissue culture plantlets (Shi et al., 2013; Zhou et al., 2018), leading to explant death and failure of regeneration (Tang and Newton, 2004; Zhenzhu et al., 2016; Shang et al., 2017). The factors affecting browning are complex and include the culture itself and the culture environment. VC was the best choice to inhibit the browning of C. hystrix plantlets, and darkness treatment for 7 days after inoculation resulted in a low browning rate. In contrast, PVP and AC had a negative effect on C. hystrix plantlets after culturing. This means that different types of anti-browning agents have different effects on the prevention of browning (González-Rodríguez et al., 2004). Another reason may be that PVP and AC act as adsorbents and compete with plantlets for nutrition in long-term culture (Cai et al., 2020). However, as an antioxidant, VC has less impact on plantlets and can even provide nutrition (Joy et al., 1988; Vijayalakshmi and Shourie, 2016). In addition, darkness treatments after inoculation can effectively reduce browning (Chavan et al., 2015; Serain et al., 2021) because proper dark handling increases endogenous phenol levels and the utilization of carbohydrates, which in turn leads to a higher rooting rate (Druart et al., 1982; Kang et al., 2020).
The root is an important organ for plants to absorb water and nutrients (Tanimoto et al., 1995). The formation of adventitious roots is a complex process controlled by many environmental and physiological factors (Deng et al., 2017), and it is essential to understand the formation and physiology of adventitious roots for breeding (Steffens and Rasmussen, 2016). In rooting cultures, plantlets are usually found at the base of hard-to-root plants, often called callus rooting. However, histological observation of adventitious roots showed that the root primordium of C. hystrix was produced from the interoperative formation layer, and no root primordia were formed in the callus, confirming that callus production was not directly related to the formation of root primordia. Some studies have shown that auxin-induced calluses share some characteristics with the root development pathway, which may promote the development of adventitious roots (Xu et al., 2018). Whether callus production promotes adventitious root growth in C. hystrix remains unknown. Once the callus has been proven to inhibit the growth of C. hystrix adnate roots, we can reduce the number of calluses during rooting by optimizing the rooting medium.
Previous studies have reported low survival rates of Quercus rubra during acclimation (Vengadesan and Pijut, 2009). However, in this study, more than 90% of the plantlets were preserved after acclimatization. According to their growth performance, these plantlets grew uniformly without excessive differences, showing a high degree of homogeneity between the regenerants. On the leaves, it was observed that the color of the regenerated plants was essentially the same as that of the parent leaves. Apical leaves were buff, whereas other leaves were dark green. Thus, in vitro propagation can maintain the excellent traits of the parent.
Conclusion
This is the first report of the in vitro regeneration of mature C. hystrix. In this study, the obstacle of low survival rate of tissue culture of C. hystrix was successfully confirmed. We also solved other problems, such as the maximum number of shoots per explant was obtained on HZ containing 4.4 μM BA and 0.5 μM IBA, and markedly increased the shoot number and growth. For the optimal multiplication medium was on HZ containing 2.6 μM BA and 1.0 μM NAA, and for the rooting medium, the optimal hormone concentration was half-strength HZ + 3.2 μM NAA. The regenerated plants, which were propagated in this system, showed good growth, lush foliage, and well-developed roots. These results indicate that the regeneration protocol of C. hystrix can be used for clonal propagation of the superior genotype to lay the foundation for its popularization, preservation of germplasm, and genetic transformation.
Data availability statement
The original contributions presented in the study are included in the article/supplementary material, further inquiries can be directed to the corresponding author/s.
Author contributions
HZ: formal analysis, validation, and writing–original draft. MG: methodology and writing—original draft. QW, RL, and MZ: data curation and formal analysis. XD: supervision. RX: conceptualizations and resources, and writing–review and editing. All authors contributed to the article and approved the submitted version.
Funding
This study was supported by the Guangdong Forestry Science and Technology Innovation Special Funds Project (2014KJCX002) and the Provincial Science and Technology Plan Project of Guangdong Province (2016B020233001).
Acknowledgments
We are grateful to test platform and assistance provided by the School of Forestry and Landscape Architecture, South China Agricultural University.
Conflict of interest
The authors declare that the research was conducted in the absence of any commercial or financial relationships that could be construed as a potential conflict of interest.
Publisher’s note
All claims expressed in this article are solely those of the authors and do not necessarily represent those of their affiliated organizations, or those of the publisher, the editors and the reviewers. Any product that may be evaluated in this article, or claim that may be made by its manufacturer, is not guaranteed or endorsed by the publisher.
References
Ahuja, M. R., and Jain, S. M. (2017). Biodiversity and Conservation of Woody Plants. Cham: Springer.
Arteta, T. A., Hameg, R., Landin, M., Gallego, P. P., and Barreal, M. E. (2018). Neural networks models as decision-making tool for in vitro proliferation of hardy kiwi. Eur. J. Hortic. Sci. 83, 259–265. doi: 10.17660/eJHS.2018/83.4.6
Baofu, L., Junbao, Z., Guobiao, C., Wei, Z., Xincai, S., Sijing, L., et al. (2018). Castanopsis hystrix Grafting Method. C.N. Patent No.1,079,502,20A. Beijing: C.N. Patent and Trademark Office.
Bidabadi, S. S., and Jain, S. M. (2020). Cellular, molecular, and physiological aspects of in vitro plant regeneration. Plants 9:702. doi: 10.3390/plants9060702
Cai, X., Wei, H., Liu, C., Ren, X., Thi, L. T., and Jeong, B. R. (2020). Synergistic effect of NaCl pretreatment and P.V.P. on browning suppression and callus induction from petal explants of paeonia lactiflora pall. ‘festival maxima’. Plants 9:346. doi: 10.3390/plants9030346
Carl, M. R., and Richard, R. W. (2002). Mineral nutrition and plant morphogenesis. In Vitro Cell. Dev. Biol. Plant. 38:9. doi: 10.2307/20065020
Chakraborty, T., Chaitanya, K. V., and Akhtar, N. (2022). Analysis of regeneration protocols for micropropagation of Pterocarpus santalinus. Plant Biotechnol. Rep. 16, 1–15. doi: 10.1007/S11816-021-00728-8
Chang, C. W., Lin, M. T., Lee, S. S., Liu, K. C., Hsu, F. L., and Lin, J. (1995). Differential inhibition of reverse transcriptase and cellular D.N.A polymerase-α activities by lignans isolated from Chinese herbs, Phyllanthus myrtifolius Moon, and tannins from Lonicera japonica Thunb. and Castanopsis hystrix. Antiviral Res. 27, 367–374. doi: 10.1016/0166-3542(95)00020-M
Chavan, J. J., Ghadage, D. M., Bhoite, A. S., and Umdale, S. D. (2015). Micropropagation, molecular profiling and R.P-H.P.L.C determination of mangiferin across various regeneration stages of Saptarangi (Salacia chinensis L.). Ind. Crops Prod. 76, 1123–1132. doi: 10.1016/j.indcrop.2015.08.028
Chen, H. F., Tanaka, T., Nonaka, G. I., Fujioka, T., and Mihashi, K. (1993a). Hydrolysable tannins based on a triterpenoid glycoside core, from Castanopsis hystrix. Phytochemistry 32, 1457–1460. doi: 10.1016/0031-9422(93)85159-O
Chen, H. F., Tanaka, T., Nonaka, G. I., Fujioka, T., and Mihashi, K. (1993b). Phenylpropanoid-substituted Catechins from Castanopsis hystrix and structure revision of cinchonains. Phytochemisty 33, 183–187. doi: 10.1016/0031-9422(93)85419-R
Chunyan, T., and Suyun, C. (2017). Castanopsis hystrix Tender Branch Tissue Culture and Propagation Method. C.N. Patent No.1,067,978,89A. Beijing: C.N. Patent and Trademark Office.
Corredoira, E., Valladares, S., and Vieitez, A. M. (2006). Morphohistological analysis of the origin and development of somatic embryos from leaves of mature Quercus robur. In Vitro Cell. Dev. Biol. Plant 42, 525–533. doi: 10.1079/IVP2006827
Deng, K., Dong, P., Wang, W., Feng, L., Xiong, F., Wang, K., et al. (2017). The T.O.R pathway is involved in adventitious root formation in Arabidopsis and potato. Front. Plant Sci. 8:784. doi: 10.3389/fpls.2017.00784
Druart, P., Kevers, C., Boxus, P., and Gaspar, T. (1982). In vitro promotion of root formation by apple shoots through darkness effect on endogenous phenols and peroxidases. Z. Pflanzenphysiol. Bd. 76, 429–436.
Gago, J., Landín, M., and Gallego, P. P. (2010). Artificial neural networks modeling the in vitro rhizogenesis and acclimatization of Vitis vinifera L. J. Plant Physiol. 167, 1226–1231. doi: 10.1016/j.jplph.2010.04.008
Gaidamashvili, M., and Benelli, C. (2021). Threatened woody plants of Georgia and micropropagation as a tool for in vitro conservation. Agronomy 11:1082. doi: 10.3390/agronomy11061082
Gonbad, R. A., Sinniah, U. R., Aziz, M. A., and Mohamad, R. (2014). Influence of cytokinins in combination with G.A3 on shoot multiplication and elongation of tea clone Iran 100 (Camellia sinensis (L.) O. Kuntze). Sci. World J. 2014:943054. doi: 10.1155/2014/943054
González-Rodríguez, A., Arias, D. M., Valencia, S., and Oyama, K. (2004). Morphological and R.A.P.D. analysis of hybridization between Q. affinis and Q. laurina (fagaceae), two Mexican red oaks. Am. J. Bot. 91, 401–409. doi: 10.3732/ajb.91.3.401
Griebeler, A. G., Consatti, G., Freitas, E. M. D., and Sperotto, R. A. (2014). Optimal culture conditions for the initial development of Ilex paraguariensis a.St.-Hil. Explants. Acta Bot. Bras. 28, 548–551. doi: 10.1590/0102-33062014abb3465
Halloran, S. M., and Adelberg, J. (2011). A macronutrient optimization platform for micropropagation and acclimatization: using turmeric (Curcuma longa L.) as a model plant. In Vitro Cell. Dev. Biol. Plant. 47, 257–273. doi: 10.1007/s11627-011-9364-5
Hameg, R., Arteta, T. A., Landin, M., Gallego, P. P., and Barreal, M. E. (2020). Modeling and optimizing culture medium mineral composition for in vitro propagation of Actinidia arguta. Front. Plant Sci. 11:554905. doi: 10.3389/fpls.2020.554905
Joy, R. W., Patel, K. R., and Thorpe, T. A. (1988). Ascorbic acid enhancement of organogenesis in tobacco callus. Plant Cell Tiss. Organ Cult. 13, 219–228. doi: 10.1007/BF00043670
Kang, L., Zheng, K., Xie, Y., Deng, Y., Yu, Y., Zhu, M., et al. (2020). Efficient tissue culture protocol for magnolia lucida (Magnoliaceae) and confirmation of genetic stability of the regenerated plants. Plants 9:997. doi: 10.3390/plants9080997
Khosh-Khui, M., and Sink, K. C. (1982). Rooting-enhancement of Rosa hybrida for tissue culture propagation. Sci. Hortic. 17, 371–376. doi: 10.1016/0304-4238(82)90118-2
Kim, Y. S., Hahn, E. J., and Paek, Y. K. Y. (2003). Lateral root development and saponin accumulation as affected by IBA or NAA in adventitious root cultures of panax ginseng Ca. Meyer. In Vitro Cell. Dev. Biol. Plant 39, 245–249. doi: 10.1079/IVP2002397
Kothari, S. L., Agarwal, K., and Kumar, S. (2004). Inorganic nutrient manipulation for highly improved in vitro plant regeneration in finger millet—Eleusine coracana (L.) Gaertn. In Vitro Cell. Dev. Biol. Plant. 40, 515–519. doi: 10.1079/IVP2004564
Kumar, N., Vijay Anand, K. G., and Reddy, M. P. (2011). In vitro regeneration from petiole explants of non-toxic Jatropha curcas. Ind. Crops Prod. 33, 146–151. doi: 10.1016/j.indcrop.2010.09.013
Kuppusamy, S., Ramanathan, S., Sengodagounder, S., Senniappan, C., Shanmuganathan, R., Brindhadevi, K., et al. (2019). Optimizing the sterilization methods for initiation of the five different clones of the Eucalyptus hybrid species. Biocatal. Agric. Biotechnol. 22:101361. doi: 10.1016/j.bcab.2019.101361
Liang, X., He, P., Liu, H., Zhu, S., Uyehara, I. K., Hou, H., et al. (2019). Precipitation has dominant influences on the variation of plant hydraulics of the native Castanopsis fargesii (Fagaceae) in subtropical China. Agric. Forest Meteorol. 271, 83–91. doi: 10.1016/j.agrformet.2019.02.043
Liu, B., Lin, R., Jiang, Y., Jiang, S., Xiong, Y., Lian, H., et al. (2020). Transcriptome analysis and identification of genes associated with starch metabolism in Castanea henryi seed (Fagaceae). Int. J. Mol. Sci. 21:1431. doi: 10.3390/ijms21041431
Martínez, M. T., Arrillaga, I., Sales, E., Pérez-Oliver, M. A., González-Mas, M. D. C., and Corredoira, E. (2021). Micropropagation, characterization, and conservation of Phytophthora cinnamomi-Tolerant holm oak mature trees. Forests 12:1634. doi: 10.3390/f12121634
Martínez, M. T., Corredoira, E., Viéitez, M. A. M., Cernadas, M. J., Montenegro, R., Ballester, A., et al. (2017a). Micropropagation of mature Quercus ilex L. Trees by axillary budding. Plant Cell Tiss. Organ Cult. 131, 499–512. doi: 10.1007/s11240-017-1300-x
Martínez, M. T., San José, M. C., Vieitez, A. M., Cernadas, M. J., Ballester, A., and Corredoira, E. (2017b). Propagation of mature Quercus ilex L. (Holm oak) trees by somatic embryogenesis. Plant Cell Tiss. Organ Cult. 131, 321–333. doi: 10.1007/s11240-017-1286-4
Namli, S., Akbas, F., Isikalan, Ç, Tilkat, E. A., and Basaran, D. (2010). The effect of different plant hormones (PGRs) on multiple shoots of Hypericum retusum Aucher. Plant OMICS 3, 12–17. doi: 10.1007/s11103-009-9563-1
Nezami-Alanagh, E., Garoosi, G. A., Landín, M., and Gallego, P. P. (2018). Combining D.O.E with neurofuzzy logic for healthy mineral nutrition of pistachio rootstocks in vitro culture. Front. Plant Sci. 9:1474. doi: 10.3389/fpls.2018.01474
Nezami-Alanagh, E., Garoosi, G. A., Landín, M., and Gallego, P. P. (2019). Computer-based tools provide new insight into the key factors that cause physiological disorders of pistachio rootstocks cultured in vitro. Sci. Rep. 9:9740. doi: 10.1038/s41598-019-46155-2
Oberschelp, G. P. J., and Gonçalves, A. N. (2016). Assessing the effects of basal media on the in vitro propagation and nutritional status of Eucalyptus dunnii Maiden. In Vitro Cell. Dev. Biol. Plant 52, 28–37. doi: 10.1007/s11627-015-9740-7
Phillips, G. C., and Garda, M. (2019). Plant tissue culture media and practices: an overview. In Vitro Cell. Dev. Biol. Plant. 55, 242–257. doi: 10.1007/s11627-019-09983-5
Purohit, V. K., Tamta, S., Chandra, S., Vyas, P., Palni, L. M. S., and Nandi, S. K. (2002). In vitro multiplication of Quercus leucotrichophora and Q. Glauca: important himalayan oaks. Plant Cell Tissue Organ Cult. 69, 121–133. doi: 10.1023/A:1015296713243
Ruchun, X., Ruiping, L., Xiaomei, D., Jinfeng, J., and Mengqiu, Z. (2016). Castanopsis hystrix High Efficiency Isolated Culture Plant Regeneration Method. C.N. Patent No.1,054,751,30B. Beijing: C.N. Patent and Trademark Office.
San-José, M. C., Corredoira, E., Martínez, M. T., Vidal, N., Valladares, S., Mallón, R., et al. (2010). Shoot apex explants for induction of somatic embryogenesis in mature Quercus robur L. Trees Plant Cell Rep. 29, 661–671. doi: 10.1007/s00299-010-0852-6
Sen, A., and Alikamanoglu, S. (2013). Antioxidant enzyme activities, malondialdehyde, and total phenolic content of P.E.G-induced hyperhydric leaves in sugar beet tissue culture. In Vitro Cell. Dev. Biol. Plant. 49, 396–404. doi: 10.1007/s11627-013-9511-2
Serain, A. F., Silvério, S. E. B., De Lourenço, C. C., Nunes, V. K., Corrêa, W. R., Stefanello, M. E. A., et al. (2021). Development of Sinningia magnifica (Otto & A. Dietr.) Wiehler (Gesneriaceae) tissue culture for in vitro production of quinones and bioactive molecules. Ind. Crops Prod. 159:113046. doi: 10.1016/j.indcrop.2020.113046
Shang, W., Wang, Z., He, S., He, D., Liu, Y., and Fu, Z. (2017). Research on the relationship between phenolic acids and rooting of tree peony (Paeonia suffruticosa) plantlets in vitro. Sci. Hortic. 224, 53–60. doi: 10.1016/j.scienta.2017.04.038
Shi, R., Shuford, C. M., Wang, J. P., Sun, Y. H., Yang, Z., Chen, H. C., et al. (2013). Regulation of phenylalanine ammonia-lyase (P.A.L) gene family in wood forming tissue of Populus trichocarpa. Planta 238, 487–497. doi: 10.1007/s00425-013-1905-1
Skoog, F., and Miller, C. O. (1957). Chemical regulation of growth and organ formation in plant tissues cultured in vitro. Symp. Soc. Exp. Biol. 11, 118–130. doi: 10.1002/chin.199621020
Souma, J., and Ishikawa, T. (2020). Heissiella donguri sp. Nov. (Hemiptera: Heteroptera: Tingidae) feeding on Quercus gilva (Fagaceae): first representative of the genus from Japan. Zootaxa 4751, 377–385. doi: 10.11646/zootaxa.4751.2.12
Steffens, B., and Rasmussen, A. (2016). The physiology of adventitious roots. Plant Physiol. 170, 603–617. doi: 10.1104/pp.15.01360
Su, Z., Chen, B., Chang, Y., and Yang, J. (2004). Environmental correlates of distribution of the 25 broad-leaved tree species indigenous to Guangdong Province, China. For. Stud. China 6, 23–28. doi: 10.1007/s11627-011-9364-5
Tabart, J., Franck, T., Kevers, C., and Dommes, J. (2015). Effect of polyamines and polyamine precursors on hyperhydricity in micropropagated apple shoots. Plant Cell Tissue Organ Cult. 120, 11–18. doi: 10.1007/s11240-014-0568-3
Tanaka, T., Abbas, H. K., and Duke, S. O. (1993). Structure-dependent phytotoxicity of fumonisins and related compounds in a duckweed bioassay. Phytochemistry 33, 779–785.
Tang, W., and Newton, R. J. (2004). Increase of polyphenol oxidase and decrease of polyamines correlate with tissue browning in Virginia pine (Pinus virginiana Mill.). Plant Sci. 167, 621–628. doi: 10.1016/j.plantsci.2004.05.024
Tanimoto, M., Roberts, K., and Dolan, L. (1995). Ethylene is a positive regulator of root hair development in Arabidopsis thaliana. Plant J. 8, 943–948. doi: 10.1046/j.1365-313X.1995.8060943.x
Vengadesan, G., and Pijut, P. M. (2009). In vitro propagation of Northern Red Oak (Quercus rubra L.). In Vitro Cell. Dev. Biol. Plant. 45, 474–482.
Vijayalakshmi, U., and Shourie, A. (2016). Remedial effect of ascorbic acid and citric acid on oxidative browning of Glycyrrhiza glabra callus cultures. Biotechnologia 3, 179–186. doi: 10.5114/bta.2016.62355
Wu, B., Zhang, X., and Wu, X. (2012). New lignan glucosides with tyrosinase inhibitory activities from exocarp of Castanea henryi. Carbohydr. Res. 355, 45–49. doi: 10.1016/j.carres.2012.04.009
Xiaofang, D., Wenjuan, H., Shizhong, L., Honglang, D., and Juxiu, C. (2015). Nitrogen and phosphorus productivities of five subtropical tree species in response to elevated CO2 and N addition. Eur. J. Forest Res. 134, 845–856.
Xu, C., Cao, H., Zhang, Q., Wang, H., Xin, W., Xu, E., et al. (2018). Control of auxin-induced callus formation by bZIP59–L.B.D complex in Arabidopsis regeneration. Nat. Plants 4, 108–115. doi: 10.1038/s41477-017-0095-4
You, Y., Huang, X., Zhu, H., Liu, S., Liang, H., Wen, Y., et al. (2018). Positive interactions between Pinus massoniana and Castanopsis hystrix species in the uneven-aged mixed plantations can produce more ecosystem carbon in subtropical China. For. Ecol. Manag. 410, 193–200. doi: 10.1016/j.foreco.2017.08.025
Zhang, P., He, Y., Feng, Y., De La Torre, R., Jia, H., Tang, J., et al. (2019). An analysis of potential investment returns of planted forests in South China. New Forests 50, 943–968. doi: 10.1007/s11056-019-09708-x
Zhang, Y., Bozorov, T. A., Li, D. X., Zhou, P., Wen, X. J., Ding, Y., et al. (2020). An efficient in vitro regeneration system from different wild apple (Malus sieversii) explants. Plant Methods 16:56.
Zhenzhu, F., Jun, D., Songlin, H., Liming, W., and Yuee, M. (2016). The influence of phenols on tissue browning of tree Penoy (Paeonia suffruticosa Andr). J. Nucl. Agric. 30, 201–207.
Keywords: Castanopsis hystrix, mature tree, disinfection, in vitro regeneration, browning, adventitious roots
Citation: Zhang H, Guo M, Wu Q, Zhao M, Li R, Deng X and Xi R (2022) Efficient regeneration of mature castanopsis hystrix from in vitro stem explants. Front. Plant Sci. 13:914652. doi: 10.3389/fpls.2022.914652
Received: 07 April 2022; Accepted: 18 July 2022;
Published: 12 August 2022.
Edited by:
Paloma Moncaleán, Neiker Tecnalia, SpainReviewed by:
Anwar Shahzad, Aligarh Muslim University, IndiaVinod Kataria, Jai Narain Vyas University, India
E. A. Siril, University of Kerala, India
Aloka Kumari, University of KwaZulu-Natal, South Africa
Copyright © 2022 Zhang, Guo, Wu, Zhao, Li, Deng and Xi. This is an open-access article distributed under the terms of the Creative Commons Attribution License (CC BY). The use, distribution or reproduction in other forums is permitted, provided the original author(s) and the copyright owner(s) are credited and that the original publication in this journal is cited, in accordance with accepted academic practice. No use, distribution or reproduction is permitted which does not comply with these terms.
*Correspondence: Xiaomei Deng, eG1kZW5nX3NjYXVAMTYzLmNvbQ==; Ruchun Xi, eGlyYzIwMDNAMTI2LmNvbQ==