- 1Department of Botany, Stress Physiology Phenomics Centre, University of Karachi, Karachi, Pakistan
- 2Institute of Oceanography, Minjiang University, Fuzhou, China
- 3Environmental Horticulture Department and Mid-florida Research and Education Center, IFAS, University of Florida, Apopka, FL, United States
- 4Institute of Environmental Studies, University of Karachi, Karachi, Pakistan
- 5M.A.H. Qadri Biological Research Center, University of Karachi, Karachi, Pakistan
- 6Department of Microbiology, University of Karachi, Karachi, Pakistan
Environmental extremes such as hypersaline conditions are significant threats to agricultural productivity. The sustainable use of halophilic microbial strains was evaluated in plant in a salt stress environment. Oxygen-evolving complex (OEC), energy compartmentalization, harvesting efficiencies (LHE), specific energy fluxes (SEF), and nitrogen assimilation of oilseed crops (Sunflower cultivars) in a suboptimal environment was examined. Plants were grown in a plastic pot (15 ×18 cm2) containing sterilized (autoclaved at 120°C for 1 h) soil. Twenty-five ml suspension (107 CFU/ml) each of Bacillus cereus strain KUB-15 and KUB-27 (accession number NR 074540.1) and Bacillus licheniformis strain AAB9 (accession number MW362506), were applied via drenching method. Month-old plants were subjected to salt stress via gradual increment method. The energy compartmentalization of microbial inoculated plants exposed to salt stress revealed higher photosystem II (PSII) activity at the donor side, lesser photo-inhibition, and increased performance of oxygen-evolving complex compared to control. High potassium (K+) and low sodium (Na+) ions in treated leaves with the activated barricade of the antioxidant system stimulated by Bacillus strains favored enhanced photochemical efficiency, smooth electron transport, and lesser energy dissipation in the stressed plants. Moreover, the results reveal the increased activity of nitrite reductase (NiR) and nitrate reductase (NR) by microbial inoculation that elevated the nitrogen availability in the salt-stressed plant. The current research concludes that the application of bio-inoculants that reside in the hyper-saline environment offers substantial potential to enhance salt tolerance in sunflowers by modulating their water uptake, chlorophyll, nitrogen metabolism, and better photochemical yield.
Highlights
- Halophilic microbes were isolated from the saline environment.
- Bacterial inoculation enhanced the light-harvesting efficiency and energy compartmentalization.
- Better osmotic regulation and reduced energy dissipation enhance salt tolerance.
- Cultivar Agsun−5264 was more physiologically tolerant than S-278.
Introduction
Overpopulation, environmental extremes, and abiotic stressors have affected the agricultural soils around the globe (Hasanuzzaman et al., 2013). Environmental fluctuations affect the ecosystem in several ways, and hyper-saline landscapes threaten global food security by compromising agricultural productivity (Bharti et al., 2016). Salinity stress is considered a major threat that might limit productivity worldwide. It was noticed that exposure to soil salinity to plants initiates responses at both morph-physiological and molecular levels due to salt-induced ionic and osmotic stress. Understanding salt stress responses and tolerance mechanisms is vital for developing salt-tolerant crops and sustaining crop productivity in the future (Cho et al., 2014). Salt stress results in ionic imbalance, impaired cellular homeostasis, and hormonal imbalance in plants. This contributes to the excessive accumulation of reactive free radicals (ROS), and retarded plant growth leading to cell death (Mahajan and Tuteja, 2005). Yield loss of around 70% has been reported among various agro-economically vital crops across the globe, and one of them is the sunflower (Nolan et al., 2007; Hussain et al., 2019).
Sunflower (Helianthus annuus) is an oily seed crop and has significant importance in agricultural industries worldwide (Birck et al., 2017). Its cultivation is continuously expanding due to its premium oil and dietary fiber content (Khan et al., 2015). It is a moderate salt-tolerant crop and can adapt to salt stress to a certain extent, depending upon the species with their genetic modification. Nearly 522 cultivars of sunflowers are studied, and 30 are salt-resistant (Li et al., 2020). Salt stress is a greater concern in a low rainfall region as inadequate water does not leach out excessive salt from the root zone (Nolan et al., 2007). Therefore, biotechnologically favored approaches for sustainable agriculture to overcome the impact of climatic change to avert the menaces of saline stress. The formulation of bio-inoculant with the utilization of microbial resources is quite common to induce salt tolerance in crops (Mahanty et al., 2017).
A rhizobacterium exists in a saline soil and offers substantial potential to serve as a soil-friendly proxy for bio-fertilizers in a sub-optimal environment. These rhizobacteria are phyto-stimulants exhibit growth-promoting features on a wide range of hosts. They trigger nitrogen assimilation, potassium and phosphate solubilization, iron accessibility, and bioremediation to stimulate systemic resistance in the plant (Berg and Smalla, 2009; Drogue et al., 2012; Mahanty et al., 2017; Bhattacharyya et al., 2020).
A plethora of research spells out the importance of Bacillus as PGPMs in promoting plant growth and development against sub-optimal conditions (Chakraborty et al., 2013; Kasim et al., 2013; Radhakrishnan et al., 2017). However, PGPM-induced photochemical yield concerning photosynthetic performance and subsequent nitrogen metabolism in plants is yet to be explored. The rhizobacteria populated in brackish soil may have better tolerance and positively impact plants in water stress conditions. Consequently, it is assumed that the newly isolated bacterial strains mineralize the soil minerals into the bio-available form under stress conditions. It might cause an ultimate impact on light-harvesting efficiency and photosynthetic performance and thus boost nitrogen metabolism and physiological tolerance in plants. Light-harvesting efficiency, performance index, number of active reaction centers, and heat dissipation are the few attributes that are very important to demonstrate photosystem II functionality in an abiotic stress condition (Siddiqui et al., 2021). Similarly, the flow of electrons in the thylakoid membrane and the efficiency of the evolving oxygen complex were probed to understand the tolerance mechanisms aided by PGPM in a salt stress condition. Therefore, three novel strains, i.e., Bacillus cereus strain KUB-15, B. cereus strain KUB-27, and B. licheniformis strain AAB9, were isolated from the saline environment to scrutinize the sustainable effect of newly isolated bacterial strains on oxygen-evolving complex, energy harvesting compartmentalization, PS II efficiencies and nitrogen assimilation in two sunflower cultivars, i.e., Agsun-5264 and S-278 under salt stress conditions were studied.
Materials and Methods
Collection of Seeds
Seeds of sunflower (Helianthus annuus L.) in two cultivars, i.e., Agsun-5264 and S-278, were provided by the Department of Seed Certification, Government of Pakistan. The germplasm of both cultivars was sterilized for 10 min with a bleach solution (10% commercial bleach + 0.02% triton X-100) and then rinsed 3 times with distilled water. The experiment was performed under the greenhouse conditions (28–32/18–20°C of day and night temperature, respectively, and 60–70% of relative humidity).
Bacterial Strain
Microbial isolates were collected from the University of Karachi. Two microbial isolates, i.e., Bacillus cereus strain KUB-15 and KUB-27 (accession number NR 074540.1), were obtained from the Department of Botany. Bacillus licheniformis strain AAB9 (accession number MW362506) was collected from the Department of Microbiology, University of Karachi, Pakistan. Bacillus species were cultured and purified on a Nutrient Agar medium for 24 h at 37°C. The bacterial colonies were calculated by multiplying with the dilution factor (Yadav et al., 2010). The working stock was prepared by inoculating the Bacillus species into the sterile broth (25 ml), shaken vigorously, and raised at 37°C for the next 24 h. The bacterial suspension was adjusted to 107 Colony Forming Unit (CFU) /mL per 1 kg soil.
Soil Treatment With Bacillus Species (Drenching)
Ten Seeds of both cultivars were sown in a plastic pot (15 ×18 cm2) containing sterilized (autoclaved at 120°C for 1 h) soil. Twenty-five milliliter suspension (107 CFU/ml) of respective Bacillus species were applied via the drenching method and distilled water (bacteria-free) for control. The optimal concentration of Bacillus was selected by considering the preliminary evaluation of plants. Thirty-day-old plants were subjected to salt stress via the gradual increment method (Umar et al., 2019). Two different concentrations of NaCl were selected for salt stress, i.e., 100 and 200 mM. The plants irrigated with distilled water serve as a control among stress treatments. Soil composition includes silt (16%), organic matter (0.14%), clay (10%), sand (73%), and nitrogen (0.008%). The soil had 7.9 pH and 1.71 dSm−1 of EC.
All the treatments and the control were replicated four times and experienced a high light intensity of 28–35,000 lux, especially at noon. The greenhouse experiment includes the factorial design of seven treatments representing the application of bacterial isolates besides exposure to salt stress with their respective control (given in Figure 1). All the treatments and control were replicated four times and assessed for the growth and physiological aspects after harvesting.
Morphological Characteristics
After harvesting the plants, their growth and biomass were measured and are given in Table 1. Ten plants from each treatment and control were randomly selected and washed with tap water to measure the seedling length and number of leaves. The length was measured with the help of a stainless scale. After measurement, the plant parts were chopped into small pieces and stored at low temperature for further analysis. Morphological illustrations of each treated plant and control were noted and demonstrated in Supplementary Figure 4A.
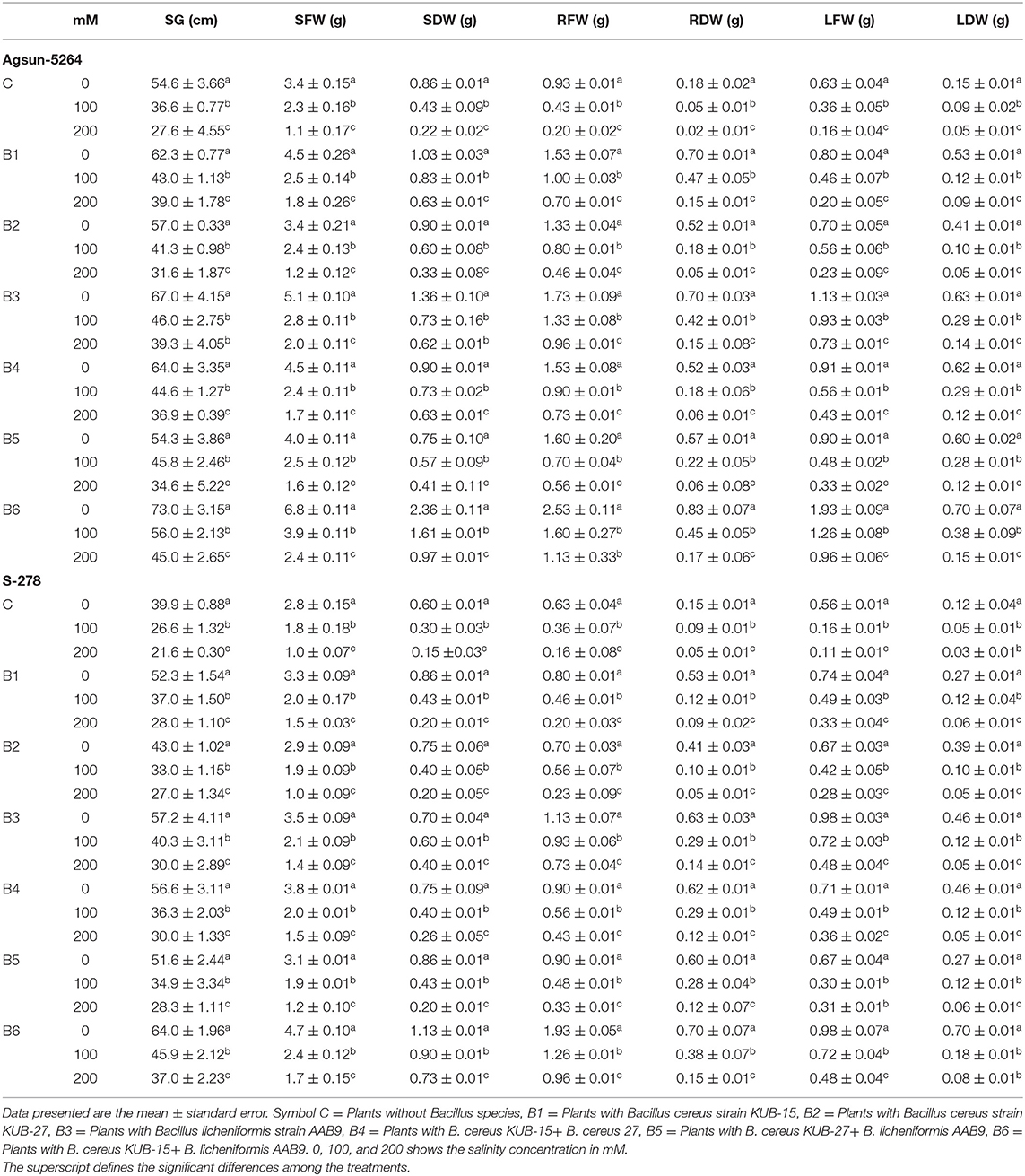
Table 1. Interactive effects of microbial strains on morphological traits of sunflower cultivars saline environment.
Stomatal Conductance (SC) and Chlorophyll Content Index (CCI)
Ten intact leaves were randomly selected from each treatment and control to measure the stomatal conductance (SC), and chlorophyll content index (CCI). For CCI, portable and non-destructive device (CCM-200; Opti-Sciences Inc., Hudson, NH, USA) was used. Fully expanded leaves were taken for chlorophyll content measurements.
Decagon leaf Porometer (Model SC-1) was used to measure the stomatal conductance. The leaves were placed in between the sensor head of the portable device. The stomatal conductance (mmol m−2 s−1) and temperature were displayed on the device screen.
Fluorescence Observation
Sixteen fully extended leaves of each treatment and control were selected to observe fluorescence traits using an Opti-science Fluorometer (Model OS30p+; Hudson, USA). The leaves were dark-adapted for 30 min by attaching the leaf clips to the leaves. The actinic light source (660 nm) was used to illuminate the leaves, which have an intensity of 3,500 μmol m−2 s−1 for 1 s. The data were then transferred to the computer by a connecting cord. Fluorescence parameters were assessed and modified according to the protocols of Stirbet et al. (2018), Strasser et al. (2010), and Maxwell and Johnson (2000).
Osmotic Potential (O.P) and Relative Water Content (RWC)
Twenty Leaves from each control and treatment were randomly selected and frozen in liquid nitrogen to estimate the osmotic potential of the leaves. The grounded leaves (cell sap) were then centrifuged at 14,000 rpm for 15 min at 4°C. Osmotic potential of expressing cell sap of the tissues was noted by Osmometer type 6 Loser Messtechnik, Berlin, Germany. The samples' osmotic potential values were then converted into the unit bar (Turner, 2018). For relative water content measurement, fresh leaf samples were used. Five hundred mg of fresh weight was used. The samples were then kept in distilled water for 24 h to obtain the turgid weight (T.W) and then dried at 72°C for 48 h to obtain dry weight (D.W) to calculate relative water content (Barrs and Weatherley, 1962).
Electrolyte Leakage
0.5 g of leaf blades were cut into the small disc by using a cork borer and contained in a bolted tube having double distilled water (20 ml) for the determination of the electrolyte leakage (%) (Lutts et al., 1996). The sample was incubated at 25°C for 24 h, and then a conductivity meter (CDM), mentioned as EC1, recorded the sample's electrical conductivity. The sample was boiled at 95°C for 30 min to note the solution's electrical conductivity as EC2. The following equation was used to determine the Electrolyte Leakage (%) as the ratio of EC1 and EC2:
H2O2 Production and Thio-Barbituric Acid Reactive Substances (TBARS Content)
Production of hydrogen peroxide (H2O2) was estimated in leaf samples of each treatment and control plant (Velikova et al., 2000). One hundred milligram of fresh leaf samples were homogenized with 0.1% trichloroacetic acid (3%) in an ice bath and then centrifuged for 15 min at 12,000 g. Later in 0.5 ml of supernatant, 0.5 ml of phosphate buffer (10 mM) with pH 7.0, and 1 ml of potassium iodide (1 M) were added. The absorbance was recorded at 390 nm and the content of H2O2 was calculated using a standard curve expressed in μmol g−1 FW (Dhindsa et al., 1981). Protocols were used to estimate TBARS content. The frozen sample (100 g) was homogenized with trichloroacetic acid (TCA 0.5%). The supernatant was then subjected to centrifugation for 15 min at 12,600 g (rev/min). Three thousand microliters of reagent solution which includes TCA (20%) and Thio-barbituric acid (0.5%) were added to a clear supernatant (1,000 μl) and then kept for 30 min in a water bath (100°C) and cooled at RT. Thiobarbituric acid reactive substances (TBARS)-TBA extinction coefficient was recorded at 532 nm as 155 mmol/cm. The TBARS content was calculated using the formula:
Assay of Antioxidant Enzymes
Leaf samples (500 mg) were homogenized in liquid nitrogen. Later antioxidant enzymes were extracted with 12 mL of extraction buffer (Tris-HCl pH 6.8, 10 mL DDT, 0.1 mM EDTA, 50 mg PVP). The mixture was then centrifuged at 4C for 15 min at 3,354 g for total protein estimation (Bradford, 1976). The antioxidant enzymes, i.e., catalase (EC # 1.11.1.6) and Superoxide Dismutase (EC # 1.15.1.1), were measured by the method of Patterson et al. (1984) and Beyer and Fridovich (1987), respectively.
Nitrate Reductase Activity and Nitrite, Nitrate Estimation
Small pieces of leaves (200 mg) were cut and incubated with 5 mL of 0.1 M Phosphate buffer solution consisting of propanol (0.1%) and KNO3 (0.1 M), pH 7.2 under anaerobic and dark conditions. For assessing nitrate content in assay solution, 2,000 μL of color development reagent containing 1,000 μL each of 0.02% N-(1-naphthyl) ethylene diamine hydrochloride (NDD) and 1% sulfanilamide in HCL were added to 100 μL of the assay solution. The O.D of the samples was recorded at 540 nm (μmole NO2 h−1 g−1 fresh weight expressed the enzyme's activity). Nitrate reductase (NR) and nitrite (NIR) activity was measured as suggested by Srivastava (1975) and Losada and Paneque (1971) based on the nitrate reduction at 540 nm. Total nitrite and nitrate-N content were estimated by acid digestion (Nerdy and Putra, 2018).
Ion Analysis
A flame photometer (Jenway PFP7, UK) was used for ion analysis (Na+ and K+ ions). Ten milliliter of 2 N HCl (diluted with deionized water) was used to digest the oven-dried leaf samples, and which were then analyzed through a flame photometer suggested by Chapman and Pratt (1961)).
Statistical Analysis
All treatments and control were subjected to SPSS version 20 for the statistical analysis of the current data. The Bonferroni post-hoc test was applied to differentiate significant differences among the mean values of different treatments and presented as small alphabets on the bar graphs (p ≤ 0.05).
Results
Effect of Microbial Strain on Growth and Biomass Accumulation
Growth parameters and seedling biomass were retarded under salt stress. The plants were severely affected under 150 mM compared to 75 mM salt stress. It was observed that the inoculation of halophilic bacteria in salt-treated cultivars revealed a significant increase in plant growth and biomass accumulation. Table 1 showed that Bacillus licheniformis in single inoculation (B3) and in combination (B6 and B5) had more pronounced effects in sunflower cultivars than Bacillus cereus strains (B1, B2, and B4) under a salt-stress environment. The growth and biomass accumulation of cultivar Agsun-5264 was better than in S-278 under salt stress conditions. Leaf dry and fresh weight was greatly improved in both cultivars under salt stress conditions.
Physiological Response
The application of newly isolated bacterial strains substantially improved relative water content (RWC), stomatal conductance (SC), and chlorophyll content index (CCI) of both sunflower cultivars exposed to salt stress (Figure 2). Application of single/dual inoculation of bacterial strains showed a substantial increase in the physiological parameters of both sunflower cultivars compared to uninoculated cultivars in a saline environment. It was evident from the data that the application of B. licheniformis (B3) improved 36–40% in RWC, 258–228% in SC, and 54–109% in CCI of Agsun-5264 under salt stress conditions. While 35–43% RWC, 200–231% SC, and 63–95% CCI increase were observed in the combined treated sample (B6) (Supplementary Table 2). Similarly, the inoculation of B3 and B6 pointedly decreased the osmotic potential in cultivar Agsun-5264 compared to other treatments under salinity stress conditions (Figure 2).
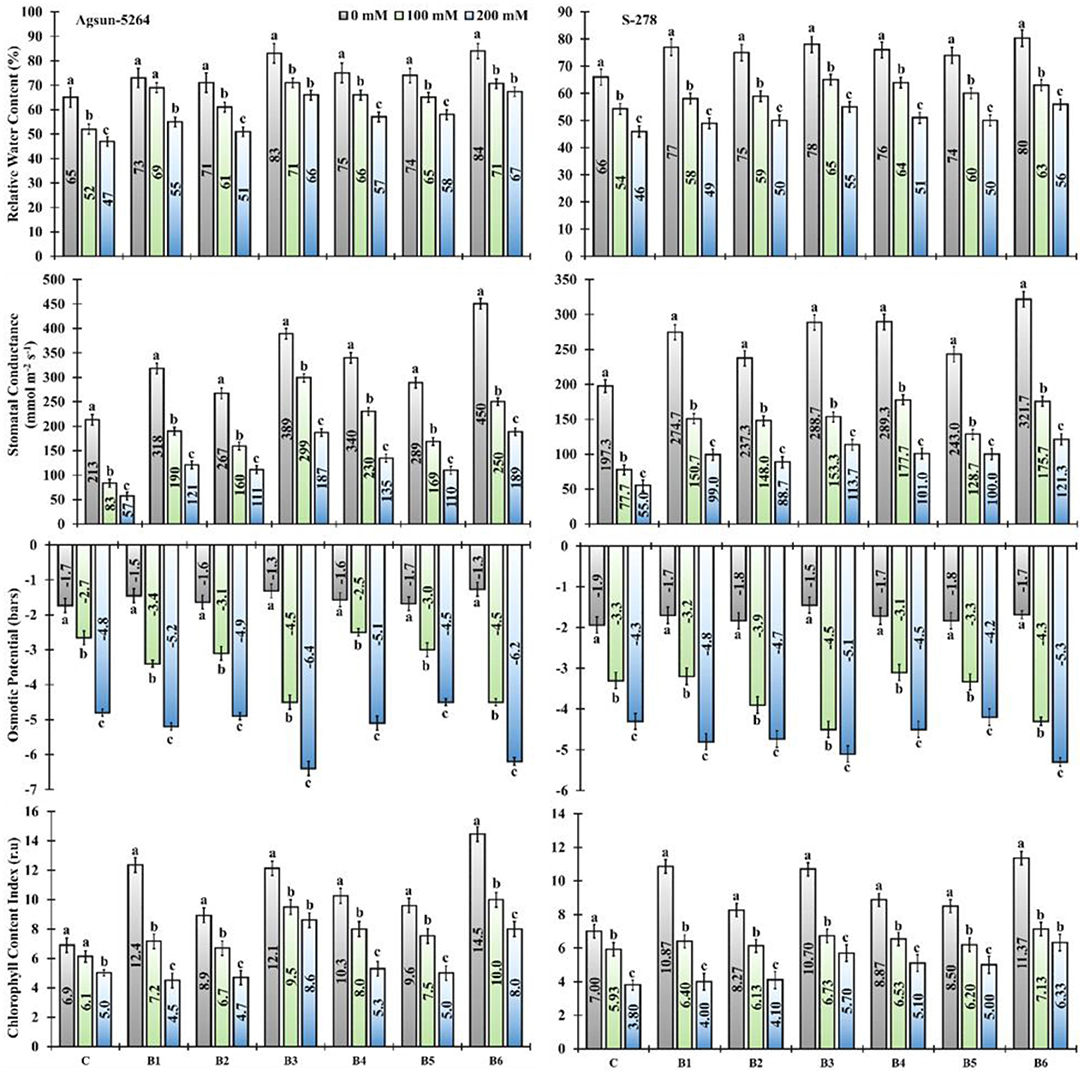
Figure 2. Effect of halophilic microbial strains on the relative water content (RWC), stomatal conductance (S.C), osmotic potential (O.P), and chlorophyll content index (CCI) of sunflower cultivars under salinity stress. Vertical line on the bar represents mean standard error (S.E). Similar alphabet shows non-significant difference at p ≤ 0.05 (Bonferroni post-hoc test) between treatments. Symbols on the horizontal axis represents plants: C = Without Bacillus species, B1 = Bacillus cereus strain KUB-15, B2 = Bacillus cereus strain KUB-27, B3 = Bacillus licheniformis strain AAB9, B4 = KUB-15 + KUB-27, B5 = KUB-27 + AAB9 and B6 = KUB-15 + AAB9. Legends demonstrate that 0 mM = Control plants without salinity stress, 100 mM = Plants treated with 100 mM NaCl, and 200 mM = Plants treated with 200 mM NaCl.
Photochemical Efficiencies
It was noted that the performance index (PIABS), dark-adapted quantum yield (FV/FM ratio), the number of the active reaction center of photosynthetic apparatus (FV/FO) of salt-treated cultivars were greatly reduced (Figure 3). The salt stress did not only decrease the cultivars' performance index but also increased energy dissipation (FO/FM). It was evident from the data the magnitudes of the negative impact of salinity on photochemical traits like FV/FM ratio, PIABS, and FV/FO ratio was mitigated due to bacterial application, application of B. licheniformis (B3) in salt stress, which showed 11–20% in FV/FM, 346–343% in PIABS, and 34%, to 57% increase in Fv/FO. In comparison, 17–25% FV/FM, 292–343% PIABS, and 61–76% Fv/FO were increased in combined treatment (B6), compared to un-inoculated control plants (Supplementary Table 2). The single (B3) and dual (B6) application of newly isolated bacterial stain B. licheniformis made a more positive impact on Agsun-5264, showed substantial improvement in the FV/FM ratio, PIABS, FV/FO ratio, and declined in FO/FM under salt stress conditions (Figure 3).
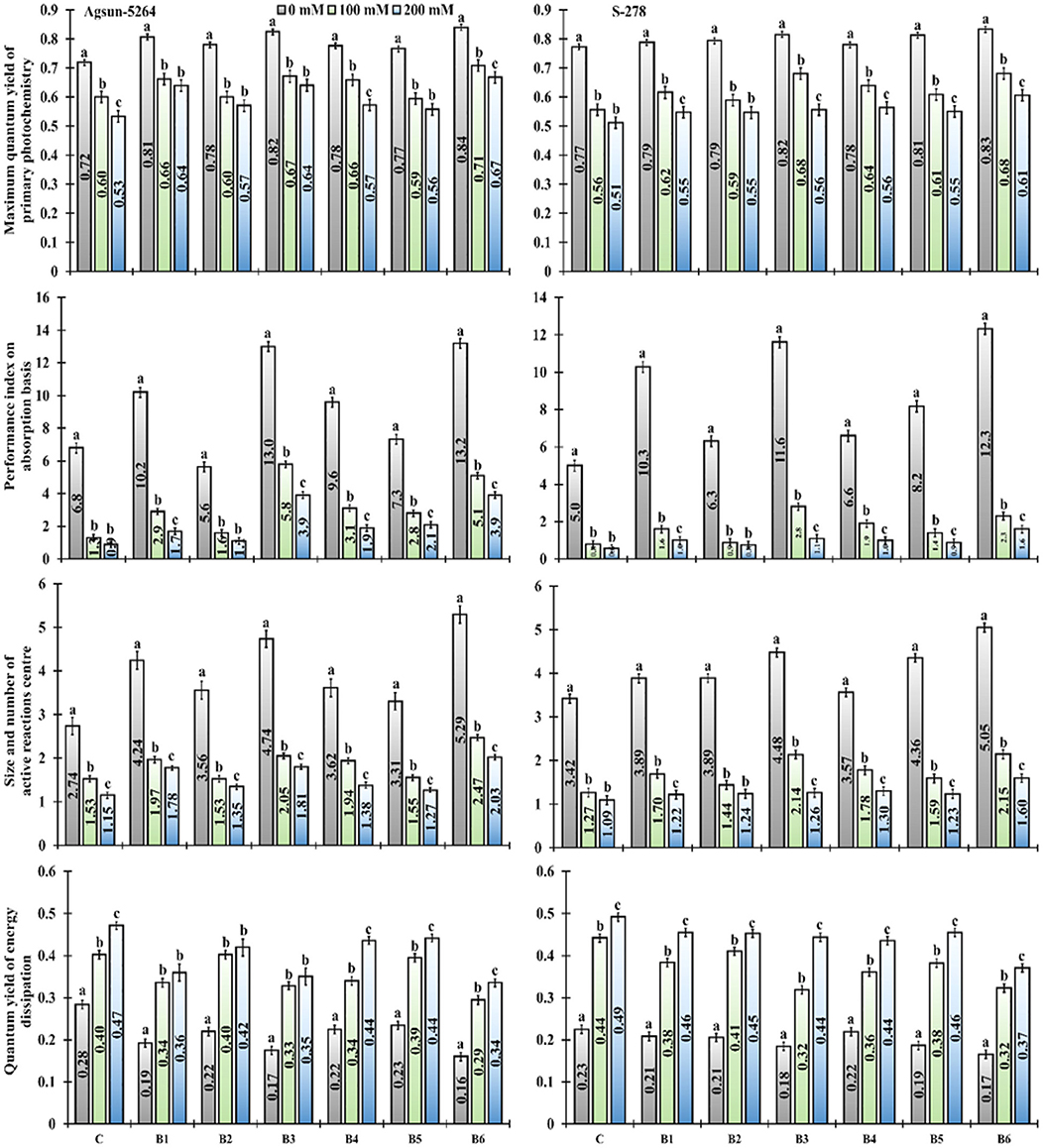
Figure 3. Effect of halophilic microbial strains on Maximum quantum yield of primary photochemistry (FV/FM), Performance index on absorption basis (PIABS), Size and number of active reaction center (FV/FO) and Quantum yield of energy dissipation (FO/FM) of sunflower cultivars during salinity stress. Vertical line on the bar represents mean standard error (S.E). Similar alphabet shows non-significant difference at p ≤ 0.05 (Bonferroni post-hoc test) between treatments.
Both treated cultivars' specific and phenomenological energy fluxes were calculated on reaction center (RC) and cross-section (CS) basis. It was evident from the data that electron transport per active reaction center (ETO/RC or CS), absorbance per reaction center (ABS/RC or CS), maximum trapped exciton flux per active PSII (TRO/RC or CS), and dissipated energy flux per active reaction center (DIO/RC or CS) of sunflower cultivars were greatly affected in saline condition, demonstrated significant differences among cultivars (Figure 4 and Supplementary Figure 1). Application of bacterial strains B3, and its combination B6 caused substantial improvement in ABS/CS, TRO/CS, and ETO/RC or CS under salt stress conditions. However, the magnitude of energy loss or energy dissipation was declined due to bacterial application B3 and B6 in particular. The bacterial application did a more pronounced effect on Agsun-5264 under a salt-stress environment.
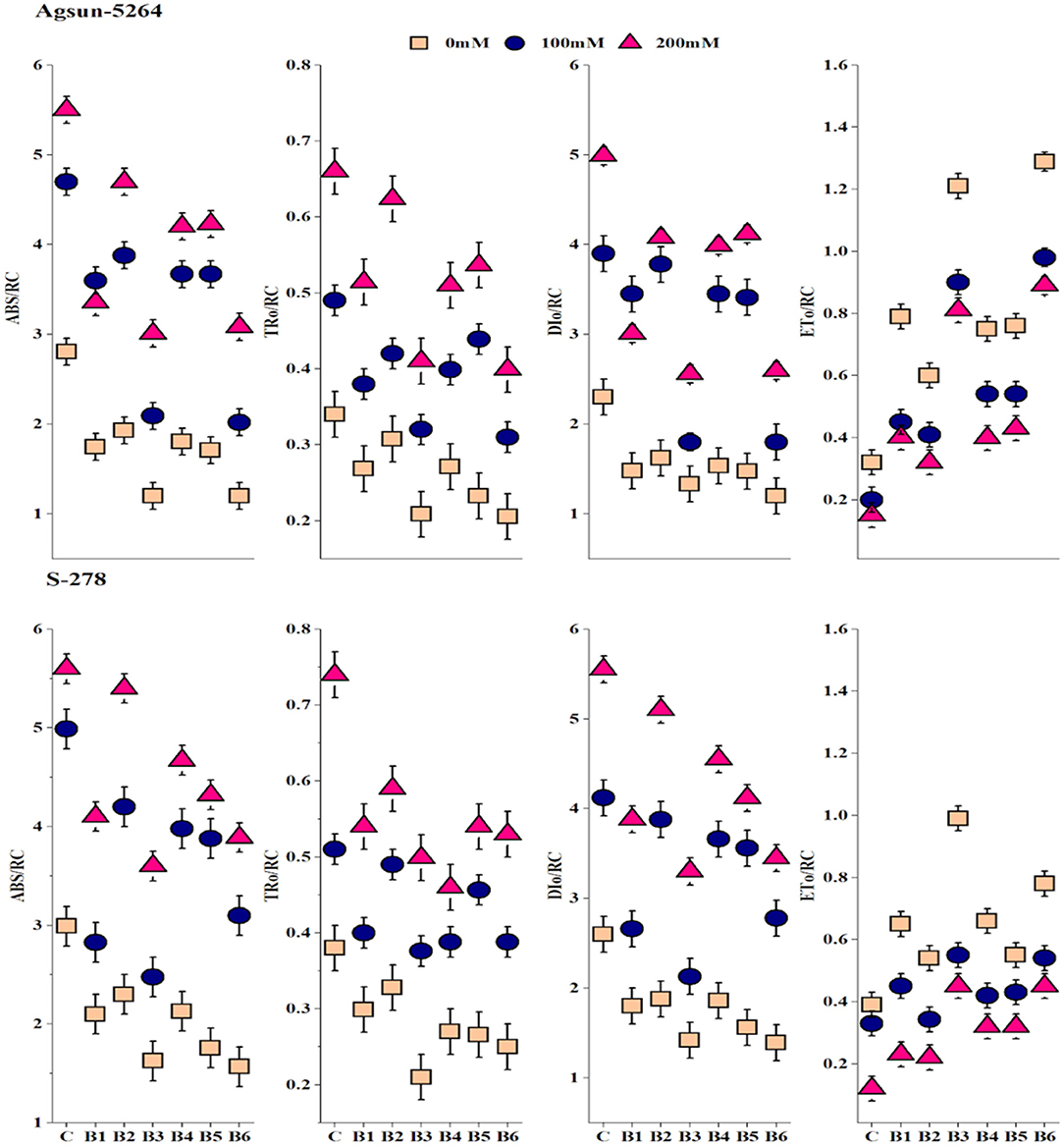
Figure 4. Effect of halophilic microbial strains on changes in specific energy fluxes per reaction center including Absorption per reaction center (ABS/RC), Trapping per reaction center (TRO/RC), Electron transport per reaction center (ETO/RC) and Dissipation per reaction center (DIO/RC) of sunflower cultivars during salinity stress. Vertical line on the bar represents mean standard error (S.E). Similar alphabet shows non-significant difference at p ≤ 0.05 (Bonferroni post-hoc test) between treatments.
Some fluorescence parameters like VI, 1-VI, VJ,1-VJ, and Wk were assessed in salinity stress. The value of relative variable Chl a fluorescence at I-step (VI), relative variable Chl a fluorescence at J-step (VJ), and ratio of variable fluorescence at K-step to the amplitude FJ-FO (Wk) were increased. At the same time, 1-VI and 1-VJ decreased in both the cultivars compared to the control (Supplementary Figure 2). But in comparison, among the single/dual inoculation of PGPMs, B3 and B6 are substantially lower down the VI, VJ, and Wk in Agsun-5264 compared to cultivar S-278 under a saline environment (Supplementary Figure 2). Meanwhile, PGPMs significantly increase the 1-VI and 1-VJ in Agsun-5264 than S-278 under stress.
Energy pipeline model showing the proportion of specific energy fluxes per active photosystem II. The model was developed to compare the efficacy of bacterial strain regarding the ABS/RC, ETO/RC, TRO/RC, DIO/RC, and NiR activity in the salt-stressed cultivar with or without PGPMs. In comparison, among the treatments of PGPMs, B3 and B6 greatly improved ETO/CS and NiR in cultivar Agsun-5264 than S-278. The membrane model also showed the application of B3 and B6 substantially maintained the ABS/RC, TRO/RC, and DIO/RC in cultivar Agsun-5264 than S-278.
OJIP-transient or induction curve was stable with PGPMs in both cultivars under a stress environment (Figure 5). However, compared to the single/dual inoculation of PGPMs, B3 and B6 highly retained OJIP-transient or induction curve in Agsun-5264, indicating an effective enhancement in photosynthetic rate S-278 under stress.
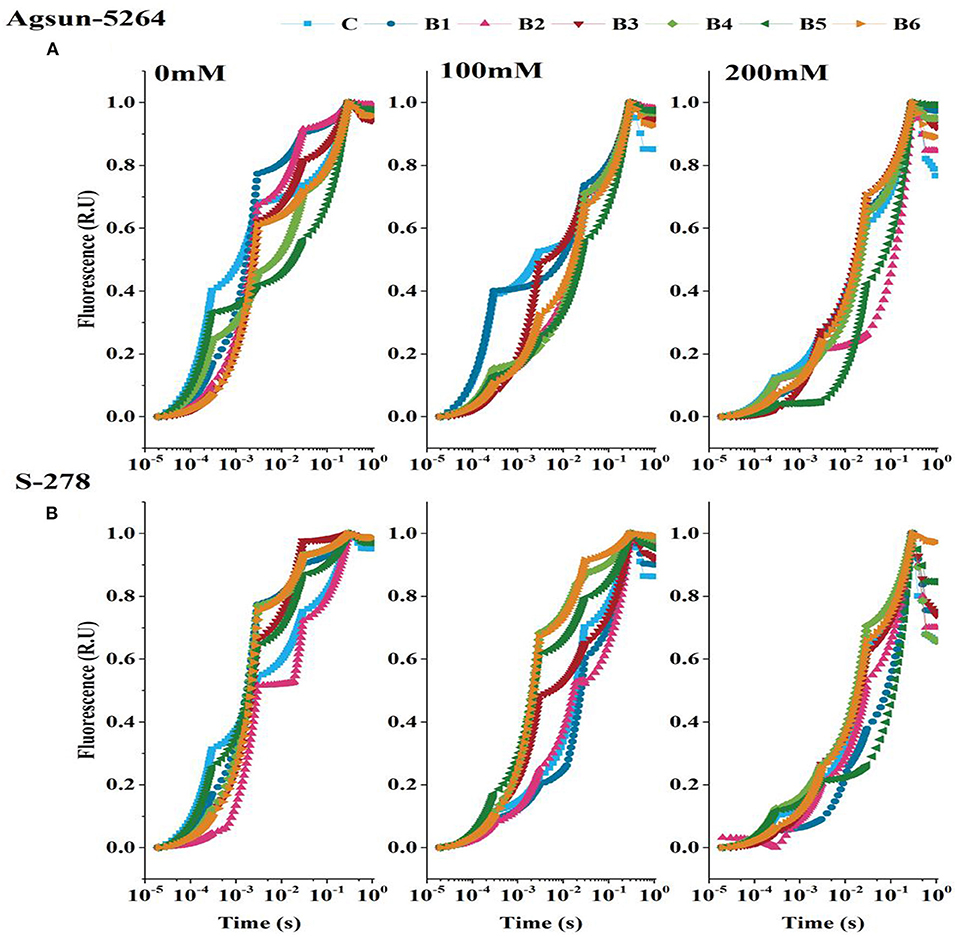
Figure 5. Effect of halophilic microbial strains on Chlorophyll a fluorescence induction curve (OJIP) of sunflower cultivars. (A) Agsun-5264 and (B) S-278 under salinity stress.
ROS Scavenging and Antioxidant System
In particular, usage of single/dual inoculation of PGPMs, B3, and B6 substantially lower the magnitude of MDA (53 and 51%) and H2O2 (43 and 42%) content in Agsun-5264 under salt stress condition, indicating lesser membrane damage compared to other treatments. Similar trend was observed among the cultivar S-278 (Supplementary Table 2). The application of PGPMs revealed that enzymes catalase (CAT) and superoxide dismutase (SOD) activities was elevated in both sunflower cultivars with the increase in NaCl concentration. However, Agsun-5264 showed maximum SOD and CAT activity (72–74% and 101–110%) than S-278 due to B3 and B6 inoculation. It has appeared that the presence of PGPMs in saline environments increased the antioxidant enzyme activity compared to saline medium without PGPMs.
Nitrogen Metabolism and Related Key Enzymes
The nitrite (NiR) and nitrate (NR) reductase activity significantly decreased under salt stress. It was noted that the magnitude of nitrite and nitrate content of sunflower cultivars decreased due to varying degrees of salt stress. However, PGPMs, B3, and B6 greatly increased the nitrite (NIR) and nitrate (NR) reductase activity under a salt stress environment. The nitrite and nitrate content in Agsun-5264 was increased compared to S-278 after PGPM inoculation. The significant enhancement in nitrogen assimilation was observed when salt stress plant were subjected to B3 and B6 bio-inoculant treatments (Figure 6).
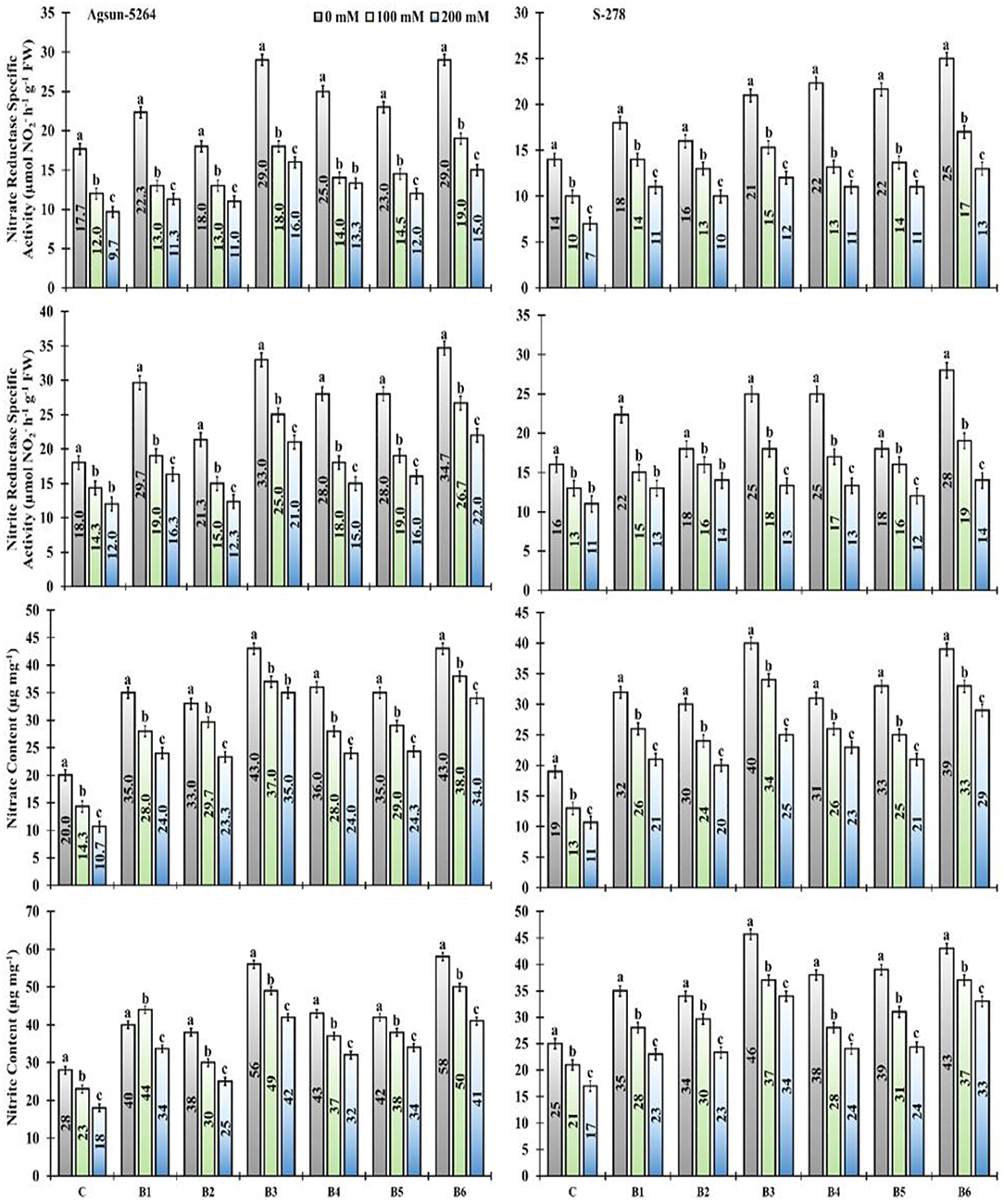
Figure 6. Effect of halophilic microbial strains on changes in Nitrate reductase specific activity (NR), Nitrite reductase specific activity (NiR), Nitrate content and Nitrite content of sunflower cultivars under salinity stress. Vertical line on the bar represents mean standard error (S.E). Similar alphabet shows non-significant difference at p ≤ 0.05 (Bonferroni post-hoc test) between treatments.
Ionic Regulation
PGPMs decreased the sodium (Na+) ion concentration and Na+/K+ ratio in both sunflower cultivars under salinity stress (Figure 7). However, in comparison among the single/dual inoculation of PGPMs, B3 and B6, in particular, greatly reduced the Na+ ion and Na+/K+ ratio in Agsun-5264 compared to other cultivar S-278 under saline environment (Supplementary Table 2). PGPMs significantly increase the K+ accumulation in both cultivars under stress environments (Figure 7). It was noticed that the utilization of PGPMs, like, B3 and B6, substantially improved the K+ ratio in Agsun-5264 compared to S-278 under stress conditions.
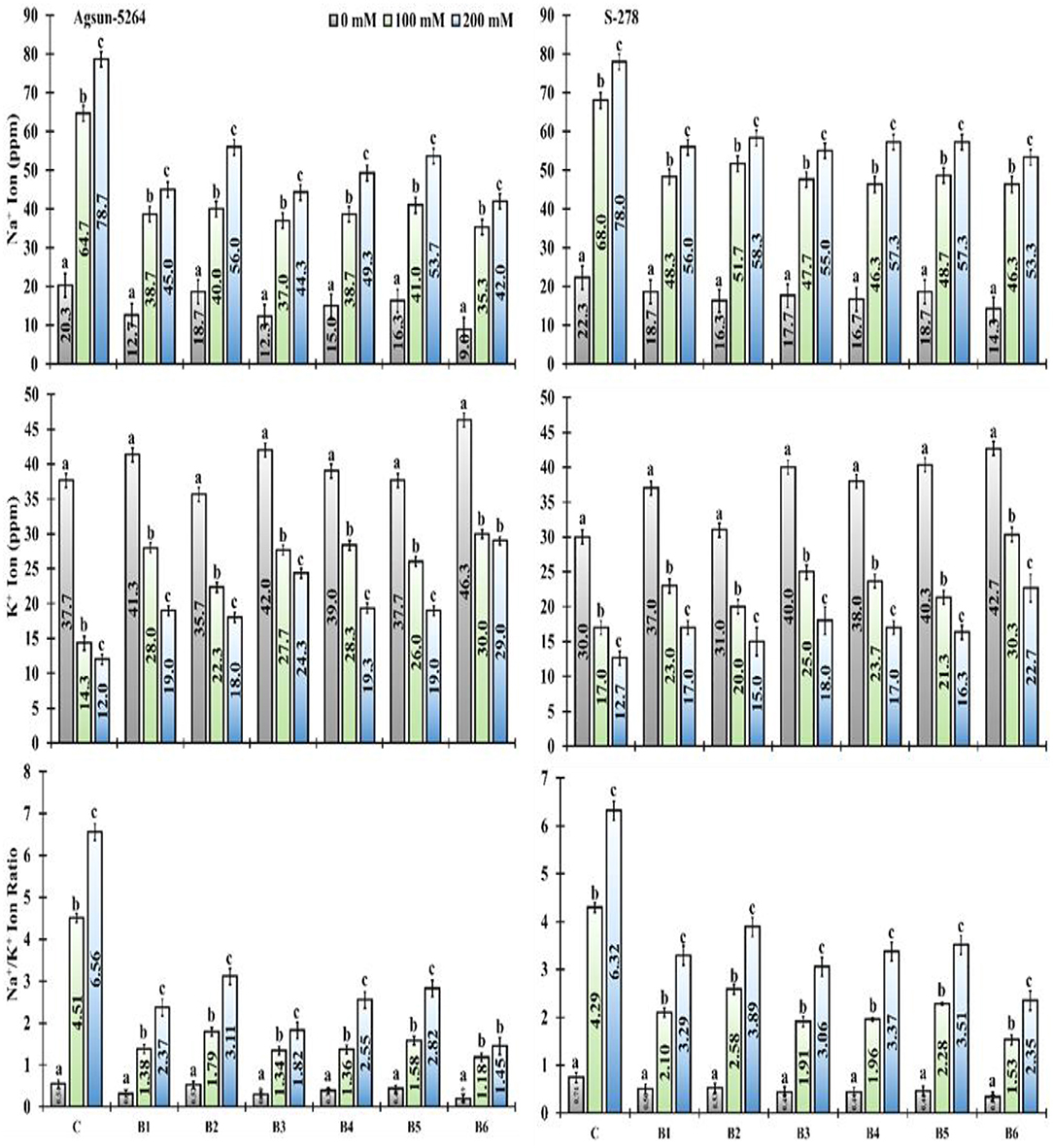
Figure 7. Effect of halophilic microbial strains on changes in sodium ion (Na+), potassium ion (K+), and Na+/K+ ratio of sunflower cultivars under salinity stress. Vertical bar lines express standard error (S.E) and similar letters on the error bar represent non-significant difference at p ≤ 0.05 (Bonferroni post-hoc test) between treatments.
Discussion
The exploitation of halotolerant bio-inoculants such as PGPMs to deal with stress has emerged to improve the productivity of crops (Grover et al., 2011). The present study investigates the potential use of PGPMs in hypersaline conditions to induce salt tolerance in crop plants. Salt tolerance was assessed using photochemical features and subsequent nitrogen metabolism.
Sunflower cultivars showed significant variation in growth characteristics under stress condition (Table 1). Apparently application of newly isolated bacterial strains B. licheniformis (B3) or in dual condition (B6) lessens the negative impact of salt stress in sunflower cultivars. The results revealed that single/dual inoculation of B. licheniformis enhanced the growth and biomass in Agsun-5264. It was supported by the literature that the dual inoculants such as Azospirillum brasilense and Pseudomonas fluorescens enhanced height, biomass, yield, and antioxidant enzyme activity in potato plants under abiotic stress conditions (Trdan et al., 2019). Moreover, single or dual PGPMs inoculation increased RWC in plants indicating the microbial association with plant that can weaken abiotic stress conditions (Igiehon and Babalola, 2021). The investigation showed that Agsun-5264 had greater stomatal conductance with the use of B. licheniformis under salinity stress, proving a fast-growth strategy to deal with stress. Sustaining a significant quantity of relative water content and stomatal conductance in leaves is a vital strategy for tackling abiotic stress, and stress-tolerant species also maintain adequate relative water content in a stress environment (Chartzoulakis et al., 2002; Cho et al., 2014; Siddiqui et al., 2014; Yao et al., 2018). Similarly higher chlorophyll content in Agsun-5264 due to B. licheniformis application under saline conditions is likely to lead to robust photosynthesis. The higher photosynthesis in cultivar Agsun-5264 due to B. licheniformis indicates that salinity did not significantly damage the photosynthetic apparatus (Gong et al., 2014). The osmotic potential is enhanced in non-inoculated plants compared to the inoculated ones (Figure 2). The results endorse the findings of Siddiqui et al. (2014), suggesting higher Na+ ions accumulation due to higher osmotic potential that causes osmotic stress in crops. High osmotic potential reduces the plants' ability to absorb water from the soil (Machado and Serralheiro, 2017). The study showed lower osmotic potentials of Agsun-5264 due to the application of PGPMs B3 and B6, indicating retention of water from the soil under salinity stress conditions. Moreover, there might be the possibility of bacterial symbiosis with Agsun-5264 providing tolerance to the salt-treated plants that result in an alignment of the osmotic potential for cellular water retention and turgor maintenance, thus reducing the detrimental effects of salt stress by stabilizing the solute potential (Bai et al., 2019).
The cultivar Agsun-5264 divulged the higher FV/FM ratio value, PIABS, and FV/FO, representing the lower disruption in antenna complex and RuBP regeneration capability due to single/dual inoculations of B3 and B6 under salinity stress. It was evident from the data that the electron transport rate from QA to QB remained unaffected in sunflower cultivar under salt stress due to bio-inoculant. Furthermore, vibrant reaction centers, larger size, and the quantity of photosynthetic apparatus leads to lesser photodamage in saline environment (Umar et al., 2019). It further indicates that the application of B. licheniformis (B3) in salt stress conditions eliminates all reservations at the oxidation sides of PSI and PSII (Zaghdoudi et al., 2011). The present study reveals that B. licheniformis improves CCI value, which finally raised the PIABS under salinity stress conditions. Increased PIABS improved photochemical efficiency or smoothed electron transport chain, revealing that the system structure, photoinhibition of photosynthesis, potential activity, and function of PSII were not affected during severe stress conditions (Appenroth et al., 2003; Oukarroum et al., 2009; Kalaji et al., 2018). Similarly, B. licheniformis treated cultivar Agsun-5264 divulged low values of FO/FM, signifying a lower disruption in electron transport at the PSII donor side, and the plastoquinone reduction (QA) rate was lower than the oxidation rate (QB) (De Lucena et al., 2012; Umar et al., 2019).
Specific energy fluxes per active reaction center (RC) including ABS/RC, ETO/RC, TRO/RC, and DIO/RC divulged absorption, trapping, electron transfer to QA− to PQ, and energy flux dissipated into heat (Figure 4). A rapid decrease in DIO/RC and DIO/CS implied the bulk of energies was not dissipated in a heat form (Meng et al., 2016). B. licheniformis decreased the photoinhibition in Agsun-5264 associated with the lesser DIO/RC related to the higher FV/FM ratio during salinity stress. Further, B. licheniformis treated Agsun-5264 reflected better ETO/RC. The absorption rate is higher than the energy utilization rate, suggesting that PSII may be quenched non-photochemically to prevent photodamage (Siddiqui et al., 2021). Meanwhile, phenomenological energy fluxes per CS, including ABS/CS, ETO/CS, and TRO/CS increased in cultivar Agsun-5264 due to utilization of B3 and B6, indicating that increases in the density of vibrant reaction centers finally improve the trapping efficiency of PSII (Siddiqui et al., 2021).
It is evident (Supplementary Figure 2) that Agsun-5264 treated with B. licheniformis enhances photochemical performance and has better tolerance against salt stress. Application of bio-inoculant B3 and B6 increased 1-VI and 1-VJ and decreased VI and VJ in Agsun-5264 under stress conditions. These results showed a smooth electrons flow from QA to QB and reduced assemblage of QA in PSII reaction centers (Da Browski et al., 2016). Further, B3 and B6 decreased 'K' step (WK) demonstrated the lowest harm in the oxygen-evolving complex (OEC), which led to a rise in the electron donation ability of the PSII donor side in cultivar Agsun-5264. The Wk values are associated with the temperature of the stress plants (Wen et al., 2005; Van Heerden et al., 2007).
Model (Figure 8) showed the tolerance level of the sunflower cultivar Agsun-5264 due to B. licheniformis by regulating the activities of PSII reaction centers and enhancing the chloroplasts' ability to absorb and dissipate energy is a key to increasing the salt tolerance. In this model, B. licheniformis increase of ETO/RC and NiR in cultivar Agsun-5264 indicates that the function and organization of PSII were less damaged and may be a defensive strategy to cope with salt stress. In contrast, B. licheniformis reduced ABS/RC and TRO/RC during salt stress conditions implying that the effective average absorption of antenna and trapping per active RC are lower due to some RC activation. B. lichaniformis decreased the photoinhibition in Agsun-5264 linked to lesser DIO/RC related to higher TRo/RC during salinity stress and increased regulation of PSII activities. The results demonstrate that average absorption and trapping per active RC rise due to the deactivation of a few RCs, and the total dissipation to the number of active RCs rises due to the high dissipation of dormant RCs. B. licheniformis enhanced nitrogen assimilation, improving chlorophyll formation and raising photosynthetic activity in cultivars Agsun-5264 during stress. The data regarding better OJIP transient curve and accumulation of active reaction centers of PSII in Agsun-5264 than S-278 (Figure 5), further strengthening the idea concerning adequate photosynthesis performance under stress conditions (Strasser et al., 2004; Umar et al., 2019).
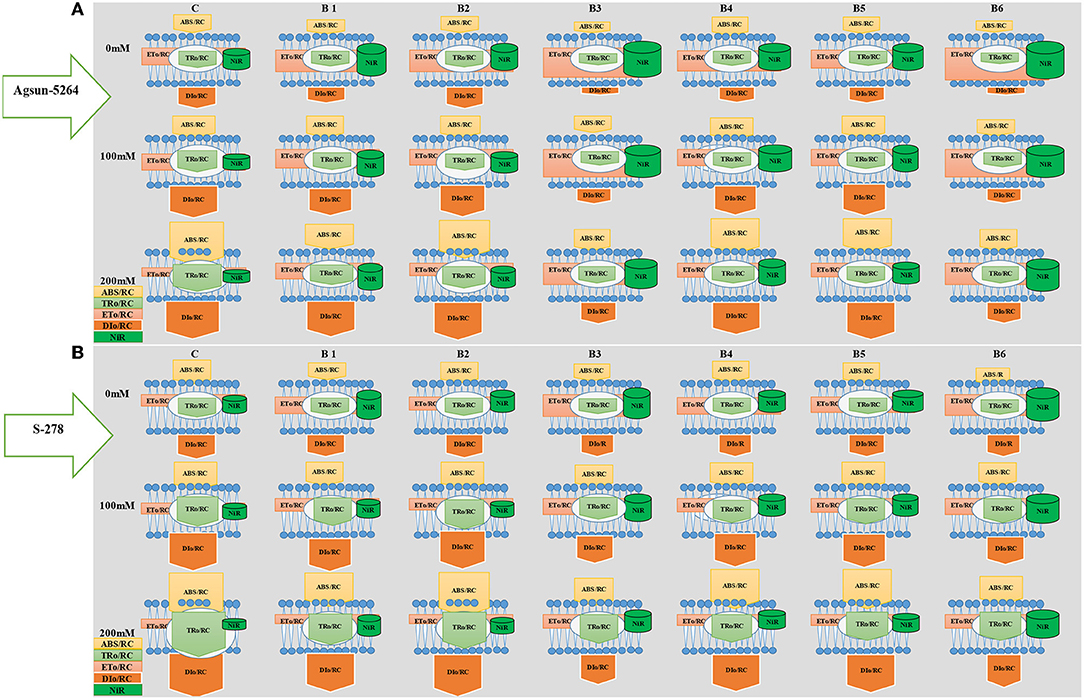
Figure 8. Energy pipeline model showing the proportion of specific energy fluxes per active PSII and NiR activity in the membrane of salt-stressed sunflower cultivars Agsun-5264 with or without microbial strains. In the membrane, ABS/RC, TRO/RC, ETO/RC, and DIO/RC indicate absorption, maximum trapped exciton flux per active PSII, electron transport, and dissipation flux, respectively. The value of each parameter can be seen in relative changes in the width of each arrow (see the color legend). The diagram exhibits the variation of ABS/RC, TRO/RC, ETO/RC, and DIO/RC, for seven treatments, namely, C = Without Bacillus species, B1 = Bacillus cereus strain KUB-15, B2 = Bacillus cereus strain KUB-27, B3 = Bacillus licheniformis strain AAB9, B4 = KUB-15 + KUB-27, B5 = KUB-27 + AAB9, and B6 = KUB-15 + AAB9. The model was developed by the values of four fluxes and NIR activity. The values of each of the four fluxes and NIR activity can be compared between cultivars and among treatments. The model displays fluxes and NIR activity in different shapes; the size of each shape is proportional to the corresponding normalized value. (A) Agsun-5264 and (B) S-278.
In the present study, inoculated plants exhibited lower values of MDA, H2O2 content, and electrolyte leakage (E.L) under salt-stress conditions. Their values were distinctly higher in the non-inoculated plants (Figure 9 and Supplementary Figure 3). The present findings are similar to those of Ahmad et al. (2012), who revealed membrane damage in mustard cultivars due to NaCl treatment, which led to modified membrane functioning. However, in comparison among the single/dual inoculation of PGPMs, B3 and B6 reduced the ROS production in Agsun-5264, leading to a significant decrease in membrane lipid peroxidation and enhanced resistibility against stress environment (Han et al., 2014; Lee et al., 2016; Siddiqui et al., 2021). Moreover, the inoculated plants (Agsun-5264) had a higher ROS-detoxifying enzyme activity than non-inoculated plants (Figure 9). Sufficient information indicates that the increased antioxidant enzyme activity plays a significant role in modulating ROS levels in plants during detrimental stress (Gong et al., 2014; Wei et al., 2015; Zhou et al., 2016). Many reports confirm that the capability of microbes and plant cells to reduce the influence of oxidative burst occurs by enhancing the accumulation of antioxidant enzymes and osmolytes (Khan and Bano, 2019; Khanna et al., 2019; Rajput et al., 2019; Vaishnav et al., 2019; Zahir et al., 2019; Nagpal et al., 2020). The findings indicate that the inoculation with B3 and B6 can mitigate the oxidative harm caused by salinity stress. In the present study, cultivar Agsun-5264 inoculated with the isolates B3 and B6 raised SOD and CAT activities during saline stress reduced lipid peroxidation and H2O2. The result divulges the favorable influence of microbes on the antioxidative enzymes' positive balance, which detoxifies ROS metabolism (Santos et al., 2018).
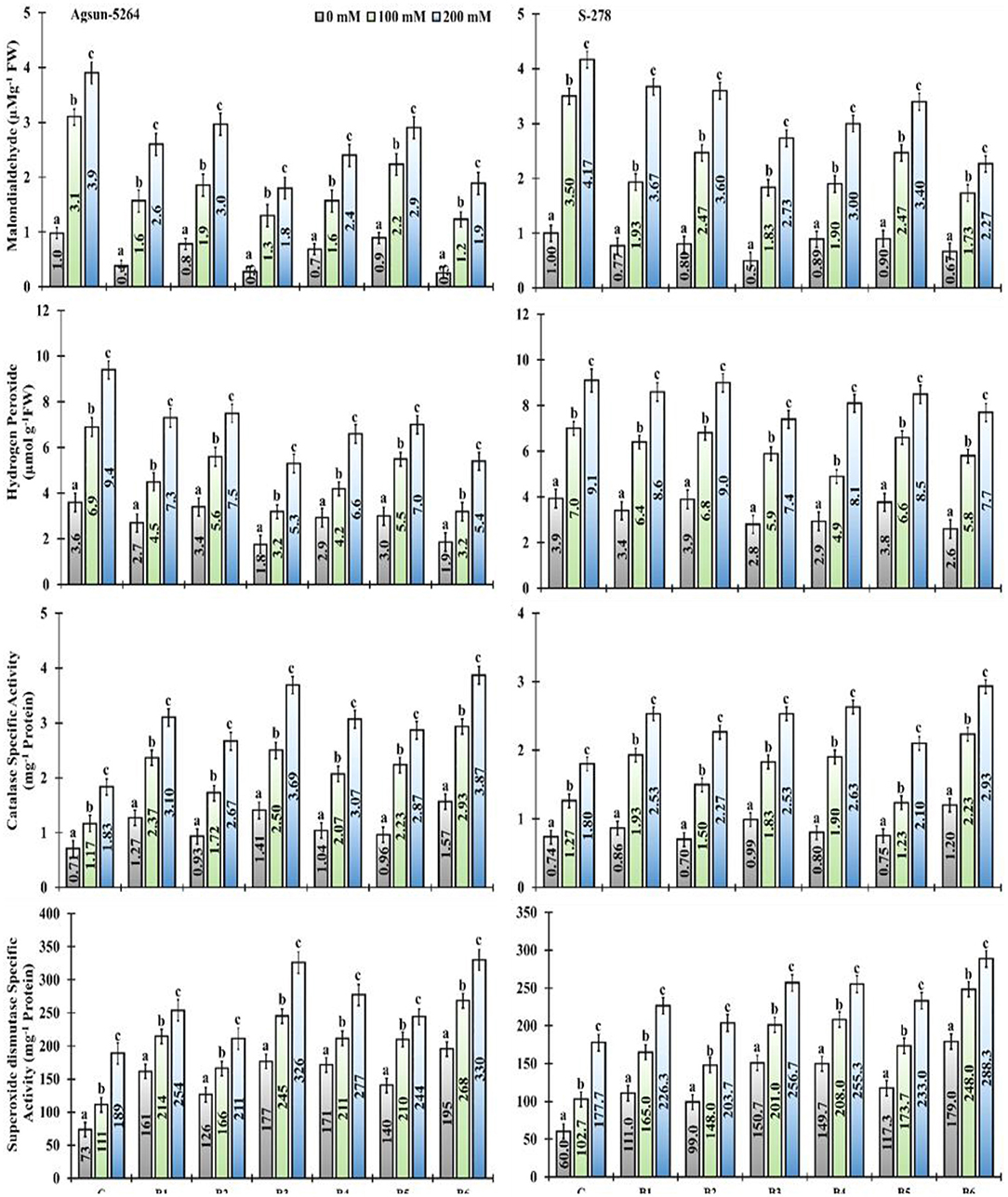
Figure 9. Effect of halophilic microbial strains on changes in Malondialdehyde (MDA), Hydrogen peroxide (H2O2), Catalase specific activity (CAT), and Superoxide dismutase specific activity (SOD) of sunflower cultivars under salinity stress. Vertical line on the bar represents mean standard error (S.E). Similar alphabet shows non-significant difference at p ≤ 0.05 (Bonferroni post-hoc test) between treatments.
Higher NiR and NR reductase activity in cultivar Agsun-5264 treated with B3 and B6 supported that the isolated strains are good for crop growth promotion. High nitrogen increases chlorophyll formation and photosynthetic activities (Wu et al., 2021). Likewise, the application of B3 and B6 also raised the nitrite and nitrate content in cultivar Agsun-5264 under salinity stress compared to other treatments. Similar results were observed in leguminous plants while treated with PGPR during abiotic stress (Siddiqui et al., 2021).
The cultivar Agsun-5264 with single/dual inoculation of B3 and B6 demonstrated the highest K+ and the Na+ absorption under a salt-stress environment. It is assumed that the PGPMs stimulated the root system that could increase the plant's ability to utilize higher nutrients, thus rhizosphere pH changes (organic acids), and facilitating K+ availability (Lugtenberg et al., 2013; Setiawati and Mutmainnah, 2016). Growth-promoting microorganisms secrete exopolysaccharide compounds, which bind with Na+ ions in the root, through which the plant's Na+ accretion reduces (Ashraf et al., 2004). Further, PGPMs balance the ion homeostasis and high K+/Na+ ratios in shoots by decreasing Na+ buildup in leaves, rising Na+ exclusion through roots, and lifting the high-affinity K+ transporter's activity (Ilangumaran and Smith, 2017). Many investigators stated a rise in K+ ions uptake in the existence of PGPMs inoculation in alfalfa (Younesi et al., 2013), maize (Rojas-Tapias et al., 2004), and cucumber (Kang et al., 2014). Besides this, Na+/K+ ratio is a salt tolerance index for plants (Cui et al., 2016). We observed that cultivar Agsun-5264 treated with B3 and B6 had a lower Na+/K+ ratio under salinity stress conditions, demonstrating that the increased tolerance by the Bacillus strain was partially attributable to minimizing Na+ accumulation. Our results agreed with the finding of Zhou et al. (2017).
Conclusion
The current research concludes that the application of bio-inoculants enhances salt tolerance in sunflowers by modulating their water uptake, improving nitrogen assimilation, and better photochemical yield. The smooth electron flow from PSII to photochemical yield, lower photo-inhibition, and reclamation of perturbation in photosynthetic apparatus against salt stress guarded by antioxidant system barricade. Demonstrated essential tolerance mechanisms induced by PGPMs.
Data Availability Statement
The original contributions presented in the study are included in the article/Supplementary Materials, further inquiries can be directed to the corresponding authors.
Author Contributions
FA conducted the entire experiment work and prepared illustrations, figures, and write-up. ZSi supervised the research work and guided the manuscript write-up. All authors equally contributed to the research work, manuscript preparation, and illustration.
Conflict of Interest
The authors declare that the research was conducted in the absence of any commercial or financial relationships that could be construed as a potential conflict of interest.
Publisher's Note
All claims expressed in this article are solely those of the authors and do not necessarily represent those of their affiliated organizations, or those of the publisher, the editors and the reviewers. Any product that may be evaluated in this article, or claim that may be made by its manufacturer, is not guaranteed or endorsed by the publisher.
Supplementary Material
The Supplementary Material for this article can be found online at: https://www.frontiersin.org/articles/10.3389/fpls.2022.913825/full#supplementary-material
References
Ahmad, P., Hakeem, K. R., Kumar, A., Ashraf, M., and Akram, N. A. (2012). Salt induced changes in photosynthetic activity and oxidative defense system of three cultivars of mustard (Brassica juncea L.). Afr. J. Biotech. 11, 2694–2703. doi: 10.5897/AJB11.3203
Appenroth, K. J., Keresztes, A., Sárvári, E., Jaglarz, A., and Fischer, W. (2003). Multiple effects of chromate on Spirodela polyrhiza: electron microscopy and biochemical investigations. Plant. Biol. 5, 315–323. doi: 10.1055/s-2003-40801
Ashraf, M., Hasnain, S., Berge, O., and Mahmood, T. (2004). Inoculating wheat seedling with exopolysaccharide-producing bacteria restricts sodium uptake and stimulates plant growth under salt stress. Biol. Fertil. Soils 40, 152–157. doi: 10.1007/s00374-004-0766-y
Bai, X., Dai, L., Sun, H., Chen, M., and Sun, Y. (2019). Effects of moderate soil salinity on osmotic adjustment and energy strategy in soybean under drought stress. Plant Physiol. Biochem. 139, 307–313. doi: 10.1016/j.plaphy.2019.03.029
Barrs, H. D., and Weatherley, P. E. (1962). A re-examination of relative turgidity technique for estimation of water deficit in leaves. Aust. J. Biol. Sci. 15, 418–428. doi: 10.1071/BI9620413
Berg, G., and Smalla, K. (2009). Plant species and soil type cooperatively shape the structure and function of microbial communities in the rhizosphere. FEMS Microbial. Ecol. 68, 1–13. doi: 10.1111/j.1574-6941.2009.00654.x
Beyer, W. F., and Fridovich, I. (1987). Assaying for superoxide dis-mutase activity: some large consequences of minor changes in condition. Ann. Biochem. 161, 559–566. doi: 10.1016/0003-2697(87)90489-1
Bharti, N., Pandey, S. S., Barnawal, D., Patel, V. K., and Kalra, A. (2016). Plant growth-promoting rhizobacteria Dietzia natronolimnaea modulates the expression of stress-responsive genes protecting wheat from salinity stress. Scien. Rep. 6, 1–16. doi: 10.1038/srep34768
Bhattacharyya, C., Roy, R., Tribedi, P., Ghosh, A., and Ghosh, A. (2020). “Biofertilizers as substitute to commercial agrochemicals,” in Agrochemicals Detection, Treatment and Remediation (Science direct), 263–290.
Birck, M., Dalchiavon, F. C., Stasiak, D., Silva locca, A. F., Hiolanda, R., Guilherme, C., et al. (2017). Performance of sunflower cultivars at different seeding periods in central Brazil. Ciênc. Agrotecnologia 41, 42–51. doi: 10.1590/1413-70542017411021216
Bradford, M. M.. (1976). A rapid and sensitive method for the quan-titation of microgram quantities of protein utilizing the princi-ple of protein-dye binding. Ann. Biochem. 72, 248–254. doi: 10.1016/0003-2697(76)90527-3
Chakraborty, U., Chakraborty, B. N., Chakraborty, A. P., and Dey, P. L. (2013). Water stress amelioration and plant growth promotion in wheat plants by osmotic stress tolerant bacteria. World J. Microbiol. Biotechnol. 29, 789–803. doi: 10.1007/s11274-012-1234-8
Chapman, H. D., and Pratt, P. F. (1961). Methods for Analysis of Soil, Plants and Waters. Berkeley, CA: University of California.
Chartzoulakis, K., Patakas, A., Kofidis, G., Bosabalodis, A., and Nastou, A. (2002). Water stress affects leaf anatomy, gas exchange, water relations and growth of two avocado cultivars. J. Hortic Sci. 95, 39–50. doi: 10.1016/S0304-4238(02)00016-X
Cho, J. I., Lim, H. M., Siddiqui, Z. S., Park, S. H., Kim, A. R., Kwon, T. R., et al. (2014). Over-expression of PsGPD, a mushroom glyceraldehyde-3-phosphate dehydrogenase gene, enhances salt tolerance in rice plants. Biotechnol. Lett. 36, 1641–1648. doi: 10.1007/s10529-014-1522-5
Cui, P., Liu, H., Islam, F., Li, L., Farooq, M. A., Ruan, S., et al. (2016). OsPEX11, a peroxisomal biogenesis factor 11, contributes to salt stress tolerance in Oryza sativa. Front. Plant Sci. 7, 1357. doi: 10.3389/fpls.2016.01357
Da Browski, P., Baczewska, A. H., and Pawlu skiewicz, B. (2016). Prompt chlorophyll a fluorescence as a rapid tool for diagnostic changes in PS II structure inhibited by salt stress in Perennial ryegrass. J. Photochem. Photobiol. B Biol. 157, 22–31. doi: 10.1016/j.jphotobiol.2016.02.001
De Lucena, C. C., de Siqueira, D. L., Martinez, H. E. P., and Cecon, P. R. (2012). Salt stress change chlorophyll fluorescence in mango. Rev. Bras. Frutic. 34, 1245–1255. doi: 10.1590/S0100-29452012000400034
Dhindsa, R. A., Plumb-Dhindsa, P., and Thorpe, P. A. (1981). Leaf senescence: correlated with increased permeability and lipid peroxidation and decreases levels of superoxide dismutase and catalase. J. Exp. Bot. 126, 93–101. doi: 10.1093/jxb/32.1.93
Drogue, B., Dore, H., Borland, S., Wisniewski-Dyé, F., and Prigent-Combaret, C. (2012). Which specificity in cooperation between phytostimulating rhizobacteria and plants? Res. Microbiol. 163, 500–510. doi: 10.1016/j.resmic.2012.08.006
Gong, B., Li, X., Vanden Langenberg, K. M., Wen, D., Sun, S., Wei, M., et al. (2014). Overexpression of S-adenosyl-L-methionine synthetase increased tomato tolerance to alkali stress through polyamine metabolism. Plant Biotechnol. J. 12, 694–708. doi: 10.1111/pbi.12173
Grover, M., Ali, S. Z., Sandhya, V., Rasul, A., and Venkateswarlu, B. (2011). Role of microorganisms in adaptation of agriculture crops to abiotic stresses. World J. Microbiol. Biotechnol. 27, 1231–1240. doi: 10.1007/s11274-010-0572-7
Han, Q. Q., Lu, X. P., Bai, J. P., Qiao, Y., Pare, P. W., Wang, S. M., et al. (2014). Beneficial soil bacterium Bacillus subtilis (GB03) augments salt tolerance of white clover. Front. Plant Sci. 5, 1928. doi: 10.3389/fpls.2014.00525
Hasanuzzaman, M., Nahar, K., Alam, M. M., Roychowdhury, R., and Fujita, M. (2013). Physiological, biochemical, and molecular mechanisms of heat stress tolerance in plants. Int. J. Mol. Sci. 14, 9643–9684. doi: 10.3390/ijms14059643
Hussain, H. A., Men, S., Hussain, S., Chen, Y., Ali, S., Zhang, S., et al. (2019). Interactive effects of drought and heat stresses on morpho-physiological attributes, yield, nutrient uptake and oxidative status in maize hybrids. Sci. Rep. 9, 1–12. doi: 10.1038/s41598-019-40362-7
Igiehon, N. O., and Babalola, O. O. (2021). Rhizobium and Mycorrhizal fungal species improved soybean yield under drought stress conditions. Curr. Microbiol. 78, 1615–1627. doi: 10.1007/s00284-021-02432-w
Ilangumaran, G., and Smith, D. (2017). Plant growth promoting rhizobacteria in amelioration of salinity stress: a systems biology perspective. Front. Plant Sci. 8, 1768. doi: 10.3389/fpls.2017.01768
Kalaji, H. M., Rastogi, A., Zivcak, M., Brestic, M., Daszkowska-Golec, A., Sitko, K., et al. (2018). Prompt chlorophyll fluorescence as a tool for crop phenotyping: an example of barley landraces exposed to various abiotic stress factors. Photosynthetica 56, 953–961. doi: 10.1007/s11099-018-0766-z
Kang, S., Khan, A., Waqas, M., You, Y., Kim, J., Kim, J., et al. (2014). Plant growth-promoting rhizobacteria reduce adverse effects of salinity and osmotic stress by regulating phytohormones and antioxidants in Cucumis sativus. J. Plant Interact. 9, 673–682. doi: 10.1080/17429145.2014.894587
Kasim, W. A., Osman, M. E., Omar, M. N., Abd El-Daim, I. A., Bejai, S., and Meijer, J. (2013). Control of drought stress in wheat using plant-growth-promoting bacteria. J. Plant Growth Reg. 32, 122–130. doi: 10.1007/s00344-012-9283-7
Khan, A. L., Waqas, M., Hamayun, M., Shahzad, R., Kim, Y. H., Choi, K. S., et al. (2015). Endophytic infection alleviates biotic stress in sunflowers through regulation of defense hormones, antioxidants and functional amino acids. Eur. J. Plant Path. 141, 803–824. doi: 10.1007/s10658-014-0581-8
Khan, N., and Bano, A. (2019). Exopolysaccharide producing rhizobacteria and their impact on growth and drought tolerance of wheat grown under rainfed conditions. PLoS ONE. 14, e0222302. doi: 10.1371/journal.pone.0222302
Khanna, K., Jamwal, V. L., Kohli, S. K., Gandhi, S. G., Ohri, P., and Bhardwaj, R. (2019). Plant growth promoting rhizobacteria induced Cd tolerance in Lycopersicon esculentum through altered antioxidative defense expression. Chemosphere 217, 463–474. doi: 10.1016/j.chemosphere.2018.11.005
Lee, T. Y., Woo, S. Y., Kwak, M. J., Inkyin, K., Lee, K. E., Jang, J. H., et al. (2016). Photosynthesis and chlorophyll fluorescence responses of Populus sibirica to water deficit in a desertification area in Mongolia. Photosynthetica 54, 317–320. doi: 10.1007/s11099-015-0180-8
Li, W., Zhang, H., Zeng, Y., Xiang, L., Lei, Z., Huang, Q., et al. (2020). A salt tolerance evaluation method for sunflower (Helianthus annuus L.) at the seed germination stage. Sci. Rep. 10, e10626. doi: 10.1038/s41598-020-67210-3
Losada, M., and Paneque, A. (1971). Nitrite reductase. Meth. Enzymol. 23, 487–491. doi: 10.1016/S0076-6879(71)23120-7
Lugtenberg, B., Malfanova, N., Kamilova, F., and Berg, G. (2013). “Plant growth promotion by microbes,” in: Molecular Microbial Ecology of the Rhizosphere, 1st Edn., Vol. 2, ed F. J. de Bruijn (RA Leiden: John Wiley & Sons, Inc.), 559–573. doi: 10.1002/9781118297674.ch53
Lutts, S., Kinet, J. M., and Bouharmont, J. (1996). NaCl-induced senescence in leaves of rice (Oryza sativa L.) cultivars differing in salinity resistance. Ann. Bot. 78, 389–398. doi: 10.1006/anbo.1996.0134
Machado, R., and Serralheiro, R. (2017). Soil salinity: effect on vegetable crop growth. Management practices to prevent and mitigate soil salinization. Horticulture 3, 30. doi: 10.3390/horticulturae3020030
Mahajan, S., and Tuteja, N. (2005). Cold, salinity and drought stresses: an overview. Rev. Arch. Biochem. Biophys. 444, 139–158. doi: 10.1016/j.abb.2005.10.018
Mahanty, T., Bhattacharjee, S., Goswami, M., Bhattacharyya, P., Das, B., Ghosh, A., et al. (2017). Biofertilizers: a potential approach for sustainable agriculture development. Environ. Sci. Pollut. Res. 24, 3315–3335. doi: 10.1007/s11356-016-8104-0
Maxwell, K., and Johnson, G. (2000). Chlorophyll fluorescence - a practical guide. J. Exp. Bot. 51, 659–668. doi: 10.1093/jexbot/51.345.659
Meng, L. L., Song, J. F., Wen, J., Zhang, J., and Wei, J. H. (2016). Effects of drought stress on fluorescence characteristics of photosystem II in leaves of Plectranthus scutellarioides. Photosynthetica 54, 414–421. doi: 10.1007/s11099-016-0191-0
Nagpal, S., Sharma, P., Sirari, A., and Gupta, R. K. (2020). Coordination of Mesorhizobium sp. and endophytic bacteria as elicitor of biocontrol against Fusarium wilt in chickpea. Eur. J. Plant. Pathol. 158, 143–161. doi: 10.1007/s10658-020-02062-1
Nerdy, N., and Putra, E. D. L. (2018). Spectrophotometric method for determination of nitrite and nitrate levels in broccoli and cauliflower with different fertilization treatment. Orient J. Chem. 34. doi: 10.13005/ojc/340639
Nolan, B. T., Healy, R. W., Taber, P. E., Perkins, K., Hitt, K. J., and Wolock, D. M. (2007). Factors influencing ground-water recharge in the eastern United States. J. Hydrol. 332, 187–205. doi: 10.1016/j.jhydrol.2006.06.029
Oukarroum, A., Schansker, G., and Strasser, R. J. (2009). Drought stress effects on photosystem I content and photosystem II thermotolerance analyzed using Chl a fluorescence kinetics in barley varieties differing in their drought tolerance. Physiol. Plant. 137, 188–199. doi: 10.1111/j.1399-3054.2009.01273.x
Patterson, B. D., Macrae, E. A., and Ferguson, I. B. (1984). Estimation of hydrogen peroxide in plant extracts using titanium (IV). Ann. Biochem. 139, 487–492. doi: 10.1016/0003-2697(84)90039-3
Radhakrishnan, R., Hashem, A., and Abd Allah, E. F. (2017). Bacillus: a biological tool for crop improvement through bio-molecular changes in adverse environments. Front. Physiol. 8, 667. doi: 10.3389/fphys.2017.00667
Rajput, R. S., Ram, R. M., Vaishnav, A., and Singh, H. B. (2019). “Microbe based novel biostimulants for sustainable crop production,” in Microbial Diversity in Ecosystem Sustainability and Biotechnological Applications, ed T. Satynarayan (Singapore: Springer), 109–144.
Rojas-Tapias, D., Moreno-Galvan, A., Pardo-Díaz, S., Obando, M., Rivera, D., and Bonilla, R. (2004). Effect of inoculation with plant growth-promoting bacteria (PGPB) on amelioration of saline stress in maize (Zea mays). Agric. Ecosyst. Environ. Appl. Soil Ecol. 61, 264–272. doi: 10.1016/j.apsoil.2012.01.006
Santos, A. D. A., Silveira, J. A. G. D., Bonifacio, A., Rodrigues, A. C., and Figueiredo, M. D. V. B. (2018). Antioxidant response of cowpea co-inoculated with plant growth-promoting bacteria under salt stress. Braz. J. Microbiol. 49, 513–521. doi: 10.1016/j.bjm.2017.12.003
Setiawati, C., and Mutmainnah, L. (2016). Solubilization of potassium containing minerals by microorganisms from sugarcane rhizosphere. Agric. Sci. Proc. 9, 108–117. doi: 10.1016/j.aaspro.2016.02.134
Siddiqui, Z. S., Ali, F., and Uddin, Z. (2021). Sustainable effect of a symbiotic nitrogen-fixing bacterium Sinorhizobium meliloti on nodulation and photosynthetic traits of four leguminous plants under low moisture stress environment. Lett. Appl. Microbiol. 72, 714–724. doi: 10.1111/lam.13463
Siddiqui, Z. S., Cho, J. I., Park, S. H., Kwon, T. R., Ahn, B. O., Lee, K. S., et al. (2014). Physiological mechanism of drought tolerance in transgenic rice plants expressing Capsicum annuum Methionine sulfoxide reductase B2 (CaMsrB2) gene. Acta Physiol. Plant. 36, 1143–1153. doi: 10.1007/s11738-014-1489-9
Srivastava, H. S.. (1975). Distribution of nitrate reductase in aging bean seedlings. Plant Cell Physiol. 16, 995–999. doi: 10.1093/oxfordjournals.pcp.a075245
Stirbet, A., Lazar, D., Kromdijk, J., and Govindjee (2018). Chlorophyll a fluorescence induction: can just a one-second measurement be used to quantify abiotic stress responses? Photosynthetica 56, 86–104. doi: 10.1007/s11099-018-0770-3
Strasser, R. J., Tsimilli-Michael, M., Qiang, S., and Goltsev, V. (2010). Simultaneous in vivo recording of prompt and delayed fluorescence and 820-nm reflection changes during drying and after rehydration of the resurrection plant Haberlea rhodopensis. Biochim. Biophys. Acta Bioenerg. 1797, 1313–1326. doi: 10.1016/j.bbabio.2010.03.008
Strasser, R. J., Tsimilli-Michael, M., and Srivastava, A. (2004). “Analysis of the chlorophyll a fluorescence transient,” in Chlorophyll a Fluorescence (Dordrecht: Springer), 321–362.
Trdan, S., Vučajnk, F., Bohinc, T., and Vidrih, M. (2019). The effect of a mixture of two plant growth-promoting bacteria from Argentina on the yield of potato, and occurrence of primary potato diseases and pest-short communication. Acta Agricult. Scand. Sect. B Soil Plant Sci. 69, 89–94. doi: 10.1080/09064710.2018.1492628
Turner, N. C.. (2018). Turgor maintenance by osmotic adjustment: 40 years of progress. J. Exp. Bot. 69, 3223–3233. doi: 10.1093/jxb/ery181
Umar, M., Uddin, Z., and Siddiqui, Z. S. (2019). Responses of photosynthetic apparatus in sunflower cultivars to combined drought and salt stress. Photosynthetica 57, 627–639. doi: 10.32615/ps.2019.043
Vaishnav, A., Shukla, A. K., Sharma, A., Kumar, R., and Choudhary, D. K. (2019). Endophytic bacteria in plant salt stress tolerance: current and future prospects. J. Plant Growth Reg. 38, 650–668. doi: 10.1007/s00344-018-9880-1
Van Heerden, P. D. R., Swanepoel, J. W., and Kruger, G. H. J. (2007). Modulation of photosynthesis by drought in two desert scrub species exhibiting C3-mode CO2 assimilation. Environ. Exp. Bot. 61, 124–136. doi: 10.1016/j.envexpbot.2007.05.005
Velikova, V., Yordanov, I., and Edreva, A. (2000). Oxidative stress and some antioxidant systems in acid rain treated bean plants: pro-tective role of exogenous polyamines. Plant Sci. 151, 59–66. doi: 10.1016/S0168-9452(99)00197-1
Wei, L., Wang, L., Yang, Y., Wang, P., Guo, T., and Kang, G. (2015). Abscisic acid enhances tolerance of wheat seedlings to drought and regulates transcript levels of genes encoding ascorbate-glutathione biosynthesis. Front. Plant Sci. 6, 458. doi: 10.3389/fpls.2015.00458
Wen, X. G., Qiu, N. W., Lu, Q. T., and Lu, C. (2005). Enhanced thermos tolerance of photosystem II in salt-adapted plants of the halophyte Artemisia anethifolia. Planta 220, 486–497. doi: 10.1007/s00425-004-1382-7
Wu, Z., Wang, X., Song, B., Zhao, X., Du, J., and Huang, W. (2021). Responses of Photosynthetic Performance of Sugar Beet Varieties to Foliar Boron Spraying. Sugar tech. 23, 1332–1339. doi: 10.1007/s12355-021-01008-z
Yadav, R. K. P., Karamanoli, K., and Vokou, D. (2010). Estimating bacterial population on the phyllosphere by serial dilution plating and leaf imprint methods. Ecoprint 17, 47–52. doi: 10.3126/eco.v17i0.4105
Yao, J., Sun, D., Cen, H., Xu, H., Weng, H., Yuan, F., et al. (2018). Phenotyping of Arabidopsis drought stress response using kinetic chlorophyll fluorescence and multicolor fluorescence imaging. Front. Plant Sci. 9, 603. doi: 10.3389/fpls.2018.00603
Younesi, O., Chaichi, M., and Postini, K. (2013). Salt tolerance in alfalfa following inoculation with Pseudomonas. Middle East J. Sci. Res. 16, 101–107. doi: 10.5829/idosi.mejsr.2013.16.01.11626
Zaghdoudi, M., Msilini, N., Govindachary, S., Lachal, M., Ouerghi, Z., and Carpentier, R. (2011). Inhibition of photosystems I and II activities in salt stress-exposed Fenugreek (Trigonella foenum graecum). J. Photochem. Photobiol. B Biol. 105, 14–20. doi: 10.1016/j.jphotobiol.2011.06.005
Zahir, Z. A., Nadeem, S. M., Khan, M. Y., Binyamin, R., and Waqas, M. R. (2019). “Role of halotolerant microbes in plant growth promotion under salt stress conditions,” in Saline Soil Based Agriculture by Halotolerant Microorganisms, ed M. Kumar (Singapore: Springer), 209–253.
Zhou, C., Ma, Z., Zhu, L., Xiao, X., Xie, Y., Zhu, J., et al. (2016). Rhizobacterial Strain Bacillus megaterium BOFC15 induces cellular polyamine changes that improve plant growth and drought resistance. Int. J. Mol. 48, 109–112. doi: 10.3390/ijms17060976
Keywords: light harvesting, energy, bio-inoculation, halotolerant microbial strains, photochemical efficiencies
Citation: Ali F, Wei X, Siddiqui ZS, Chen J, Ansari HH, Wajid D, Shams ZI, Abbasi MW and Zafar U (2022) Scrutinizes the Sustainable Role of Halophilic Microbial Strains on Oxygen-Evolving Complex, Specific Energy Fluxes, Energy Flow and Nitrogen Assimilation of Sunflower Cultivars in a Suboptimal Environment. Front. Plant Sci. 13:913825. doi: 10.3389/fpls.2022.913825
Received: 06 April 2022; Accepted: 24 June 2022;
Published: 18 July 2022.
Edited by:
Hamada AbdElgawad, Beni-Suef University, EgyptReviewed by:
Mahmoud M. Y. Madany, Cairo University, EgyptNaleeni Ramawat, Agriculture University, Jodhpur, India
Copyright © 2022 Ali, Wei, Siddiqui, Chen, Ansari, Wajid, Shams, Abbasi and Zafar. This is an open-access article distributed under the terms of the Creative Commons Attribution License (CC BY). The use, distribution or reproduction in other forums is permitted, provided the original author(s) and the copyright owner(s) are credited and that the original publication in this journal is cited, in accordance with accepted academic practice. No use, distribution or reproduction is permitted which does not comply with these terms.
*Correspondence: Xiangying Wei, xiangyingwei@mju.edu.cn; Zamin Shaheed Siddiqui, zaminss@uok.edu.pk