- Key Laboratory of the Ministry of Education for Coastal and Wetland Ecosystems, College of the Environment and Ecology, Xiamen University, Xiamen, China
Seed germination is critical to the life history of plants, playing an important role in the successful recruitment, colonization, and even invasion of new individuals within and outside population distribution ranges. Cold stratification and temperature are the key factors affecting seed germination traits. Studying how these two factors drive geographical variation in seed germination is essential to analyze and predict the geographical distribution range of alien plants in novel habitats. Spartina alterniflora, native to the United States, was introduced into China in 1979 and has spread over 20° of latitude along the eastern coast of China. Germination plays a crucial role in S. alterniflora’s large-scale invasion and diffusion across latitude. To evaluate the effects of cold stratification and temperature on seed germination of S. alterniflora across latitude, we collected seeds at seven locations across latitude in China. We exposed these provenances to cold stratification at 4°C (0, 1, 3, and 5 months) and germination temperature (5°C, 15°C, 25°C, and 35°C) treatments in growth chambers. Seed germination was observed for 98 days, and we calculated germination rate, germination index, and germination time. Results indicated that longer cold stratification significantly promoted germination rate and germination index, but decreased germination time. Similarly, higher germination temperature significantly promoted germination rate and germination index, but decreased germination time. Moreover, there were significant interactive effects on germination traits between cold stratification and temperature. Seed germination traits showed linear relationships with latitude, indicating that S. alterniflora seeds from different provenances germinated at different times and adopted different germination strategies. The stratification and temperature are the most important factors regulating the dormancy and germination seeds, so they can be important drivers of this variation along latitude. Under scenarios of warmer regional temperature, seeds at higher latitudes could germinate earlier and have higher germination rate, which would favor a potential northern expansion of this invasive plant.
Introduction
Seed germination is a critical stage in the life cycle of plants and plays a key role in the establishment, naturalization, and spread of their populations (Donohue et al., 2010). Several factors that affect germination (including cold stratification and germination temperature; Baskin and Baskin, 1998; Gillard et al., 2017) vary across latitude and thus drive the geographical distribution of plants (Brändle et al., 2003; Leiblein-Wild et al., 2014; Gillard et al., 2017). Understanding the importance of cold stratification time and germination temperature for seed germination characteristics is, therefore, key to analyzing and predicting the distribution and range of plant species (Cavieres and Arroyo 2000; Gremer et al., 2020), especially in the light of anticipated changes to local and regional climates (Walck et al., 2011; Zogas et al., 2020).
Dormancy ensures that seeds avoid the abiotic stresses associated with extreme cold and drought (Wagmann et al., 2012; Baskin and Baskin, 2014) and germinate under suitable conditions for subsequent growth (Bewley et al., 2013). In temperate and boreal regions, stratification helps break dormancy for seeds after the cold, wet, winter period (Baskin and Baskin, 2014). For example, cold stratification has been shown to increase germination percentage and germination rate in species such as Arbutus unedo, Corylopsis coreana, Primula beesiana, and Sclerocarya birrea (Moyo et al., 2009; Pipinis, 2017; Kim et al., 2018; Yang et al., 2020). Although longer stratification times are usually needed by species that experience longer winters (Zhang et al., 2019), prolonged seed stratification may reduce germination and subsequent seedling survival (Plyler and Carrick, 1993; Plyler and Proseus, 1996). Temperature also affects seed germination (Baskin and Baskin, 1977). For example, higher temperatures increased the germination rates of Amaranthus tuberculatus, Setaria faberi, and Abutilon theophrasti seeds (Leon et al., 2004; Chamorro et al., 2018). In many cases, a rise in temperature is a signal for seeds to germinate, and a drop in temperature is a signal to go dormant (Watt and Bloomberg, 2012).
These environmental factors (cold winter and warming spring) that drive stratification and germination temperature vary predictably by latitude, but recent studies of seed germination at different latitudes have shown contrasting results. Cross-species analyses have found that some species germinate faster at higher latitudes (Chamorro et al., 2018) and some slower (Zhou et al., 2021), suggesting species-specific responses to their environment. Yet, few studies have examined variation in seed germination within species over their geographic distribution in response to these factors (Baskin and Baskin, 1998; Molina-Montenegro et al., 2018; Solé-Medina et al., 2020).
An excellent system to study the relative importance of drivers of seed germination is the cordgrass Spartina alterniflora in China, introduced from the United States in 1979. The distribution now covers a range of over 20° (19°N–40°N) of latitude after 40 years of deliberate planting and natural dispersal (An et al., 2007; Zhang et al., 2017), which poses a serious threat to the Chinese coastal and wetland ecosystem (Li et al., 2009). Germination appears to play a crucial role in S. alterniflora’s large-scale latitude invasion and diffusion (Daehler and Strong, 1994; Liu et al., 2016), because stratification time and germination temperature vary throughout its range (Liu et al., 2021). Spartina alterniflora has an optimal cold stratification time of 4 months at 4°C (Biber and Caldwell, 2008). Longer or shorter periods of stratification reduce germination rates (Stalter, 1973; Seneca, 1974). Further, the expansion of S. alterniflora populations was closely related to local temperature (Loebl et al., 2006). The germination rate of S. alterniflora increased with increasing temperature from 5°C to 25°C (Yuan and Shi, 2009). Similarly, other traits such as germination characteristics (Liu and Zhang, 2021), vegetative growth (Liu et al., 2020a,b), flowering phenology (Somers and Grant, 1981; Crosby et al., 2015; Chen et al., 2021), seed set (Liu et al., 2016, 2017a), and life-cycle biomass allocation (Seneca, 1974; Liu et al., 2022) are also related to latitude in both invasive and native areas. However, the effects of stratification time and temperature gradient on germination characteristics have not been considered over a large-scale latitude gradient for S. alterniflora.
To evaluate the effects of cold stratification time and temperature on seed germination characteristics of populations from different latitudes, we sampled populations of S. alterniflora along a large-scale latitudinal gradient in coastal China. We exposed collected seeds from these different provenances to a range of cold stratification and temperature treatments and recorded their germination characteristics. The experiments addressed the following three questions: (1) What are the relative effects of cold stratification and temperature on seed germination? (2) How does seed germination vary along latitudinal gradients? (3) How do the effects of cold stratification and temperature on germination vary based on latitude?
Materials and Methods
Study Species
Spartina alterniflora, native to North America, is self-incompatible and wind-pollinated. Since its introduction into China in 1979, this species is now widely distributed along China’s coastline from 19° N to 40° N latitude (Zhang et al., 2017). Spartina alterniflora expands mainly through clonal growth and sexually reproducing seeds and maintains established populations mainly through clonal growth and sexual reproduction, and sexual reproduction is the main way of long-distance transmission (Daehler and Strong, 1994). Seed germination characteristics will strongly influence the latitudinal gradient dispersal in China. In general, S. alterniflora has no persistent seed bank because seed survival time is less than a year. They remain dormant until the following spring after they mature (Wang et al., 2006). The adaptability of germination to the environment is one of the key driving factors affecting distribution (Liu et al., 2021).
Seed Collection and Cold Stratification
In September–November, 2020, we collected S. alterniflora seeds across a wide latitudinal gradient from the introduced range in China. We sampled seven locations between 38.15°N and 20.54°N (Figure 1; Table 1). According to the climate data (2009–2019) from China Meteorological Data Service Center,1 annual mean temperature at the locations varied from 14.1°C to 23.5°C, and annual maximum and minimum temperature varied from 10.0°C to 27.1°C. To explore seeds’ response to the nature temperature range and below or up the nature temperature range, we set up 4 temperature treatments of 5°C, 15°C, 25°C, or 35°C. And, days of daily average temperature below 4°C indicates the seeds cold stratification time (Biber and Caldwell, 2008; Wu et al., 2019). It can be seen that seeds under natural treatments go through 0 to over 3 months of temperature below 4°C. Therefore, we set up four treatments of 0/1/3/5-month cold stratification time.
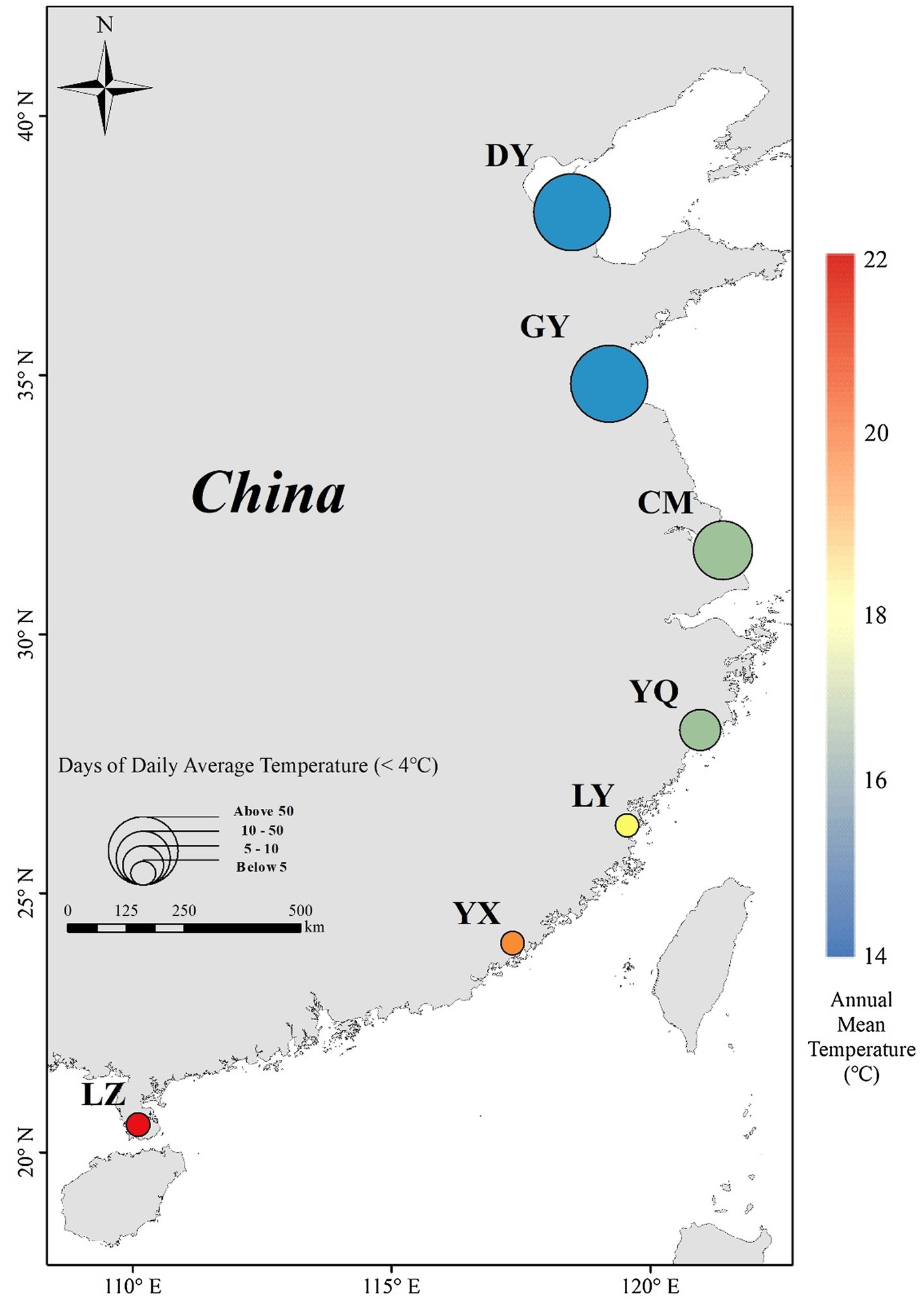
Figure 1. Spartina alterniflora seed collection locations in China (circle), and annual mean temperature (indicated by color) and days of average temperature < 4°C (indicated by size of circle) variation across latitude. See Table 1 for site code.
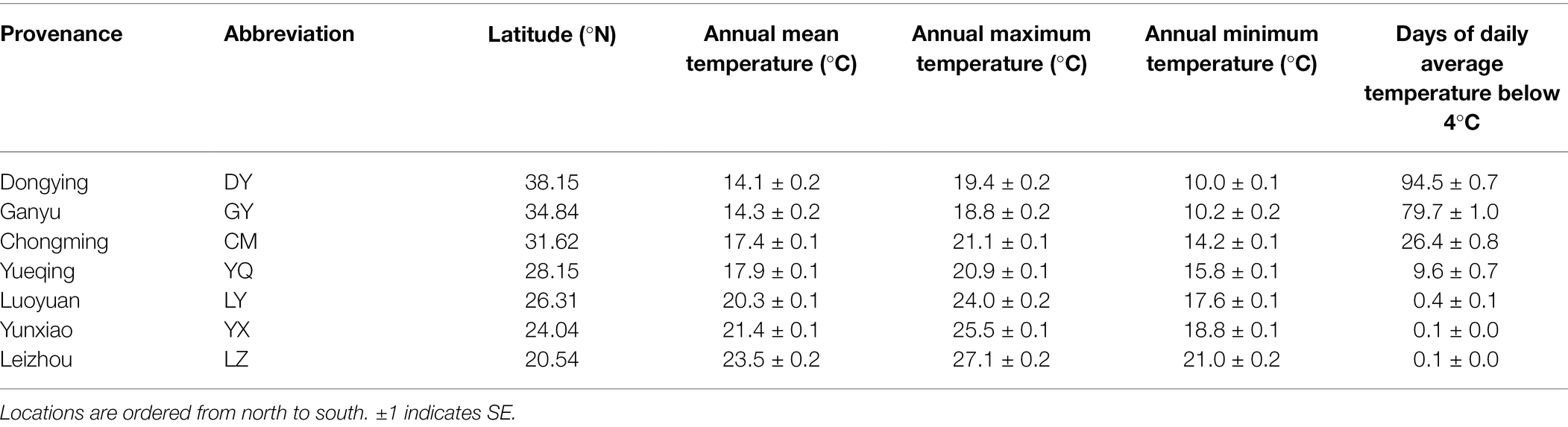
Table 1. Geographic locations and climate (annual mean temperature, annual maximum temperature, annual minimum temperature, and days of daily average temperature below 4°C of seven survey locations on the coast of China) of seven populations of Spartina alterniflora were used in this study.
Within each location we worked at two sites, separated by no <2 km (Liu et al., 2016; Figure 1). At each site, we established five randomly positioned quadrats of 0.5 m × 0.5 m. Within each quadrat, we randomly collected whole inflorescences from 10 individual plants. Previous studies have shown that filled seeds contain an embryo and endosperm, and can potentially germinate and grow; unfilled seeds have neither of these issues and cannot germinate or grow (Daehler and Strong, 1994; Ayres et al., 2007). We hand-sorted filled seeds from unfilled seeds and counted them (Daehler and Strong, 1994; Liu et al., 2016). Each provenance is with 10 samples, each sample contained more than 400 filled seeds. Before the germination experiments, the seeds were cleaned with ultra-pure water, cover with 10‰ seawater, placed in sealed and labeled plastic bags within 1 month of collection, and then stored in a refrigerator at 4°C for a 0-, 1-, 3-, or 5-month stratification period to break their dormancy (Plyler and Carrick, 1993; Koning, 2006). We removed the seeds that germinated before the germination experiment.
Germination Experiment
To monitor germination, seeds were placed into Petri dishes containing 1 sheet of filter paper and wetted with ultra-pure water to keep seeds moist. The filter paper was wetted or misted daily to prevent seeds from any drying that would cause mortality (Plyler and Carrick, 1993). Dishes were placed in a growth chamber under one of four temperature regimes: 5°C, 15°C, 25°C, or 35°C. Over the first 10 days, the number of germinated seeds was recorded daily. From day 11 to 98, observations were made every 2 days. The seed germination and flowering phenology varied across latitude in the field (Liu et al., 2017a; Qiu et al., 2018; Chen et al., 2021), so the stratification/storage time between seed maturation and germination is different. Samples that did not undergo stratification were placed to germinate in December. Those that experienced 1 month of stratification were placed to germinate at the end of January, those with 3 months of stratification at the end of March, and those with 5 months of stratification at the end of May. Each trial was followed for 98 days, by which time the most of seeds had reached the germination plateau. The final plateau was determined when no germination had occurred for 5 successive days for most of the dishes (Grime et al., 1981). In total, therefore, 16 germination trials were run for each location (four stratification treatments × four temperature treatments) with 20 seeds in each.
To compare germination among treatments and location, we calculated the germination rate (G%), germination index (GI), median germination time (T50), and mean germination time (MGT). Germination rate reflects seed activity, germination index reflects seed germination vigor, mean germination time and T50 reflect seed germination speed, and the four indices jointly reflect the germination potential of S. alterniflora seeds under the action of different environmental factors. The cumulative germination rate (G%) of S. alterniflora seeds was calculated as follows:
where n is the cumulative germination number at a specified time and N is the total number of tested seeds (Su et al., 2011; Yang et al., 2020).
The germination index (GI) was calculated as follows:
where Gt is the number of germinations at different times and Dt is the corresponding number of germination days (Zhou et al., 2021).
The median germination time (T50) was the time for 50% of final germination (Ranal and Santana, 2006).
The average germination time (MGT) was calculated as follows:
where Ni is the number of seeds germinating on day i, Di is the number of germination days (Ranal and Santana, 2006).
Statistical Analysis
To confirm the effects of temperature, cold stratification, and their interaction on germination traits, we used a mixed model with temperature, cold stratification, and their interaction as fixed factors, and with latitude (provenance) as random effects. To confirm the differences in latitudinal clines under different temperatures, we used general linear models, and the two-way ANOVA with latitude and temperature as main factors, to determine the main and interacting effects on germination traits under each cold stratification treatment. Data were cube-root (x)-transformed or cube-root [ln(x + 0.1)]-transformed or cube-root (x/10 + 1)-transformed or arcsine [cube-root (x/100)]-transformed to improve the normality of errors and homogeneity of variance when necessary. To explore germination variation across latitude, we used linear regressions to analyze the relationships between germination traits (germination rate, germination index, T50 and mean germination time) and latitude of origin. To identify the gradients in climate, a principal component analysis (PCA) was conducted with rda function in the vegan package (Oksanen et al., 2019). To determine the latitude effect variation under different treatments, we did the linear regressions between the slopes of germination traits vary by latitude under different cold stratification and temperature. We performed all analyses in R version 4.1.2 (R Core Team, 2021).
Results
Cumulative Germination Varied by Stratification Time and Temperature
Cumulative germination percentage varied considerably under different treatment conditions (Figure 2). Germination rate at 98 days ranged from <10% with 1-month stratification at 5°C (Figure 2E) to >75% germination with a 5-month stratification at 35°C (Figure 2 P). Seeds reached germination plateau most rapidly at 35°C and 5-month stratification treatment (Figure 2P). As we predicted, in general, with longer stratification time and at the higher temperature, germination occurred earlier and was more rapid. Even so, germination was also determined by latitude. Seeds from lower latitudes tended to have lower and slower germination rates.
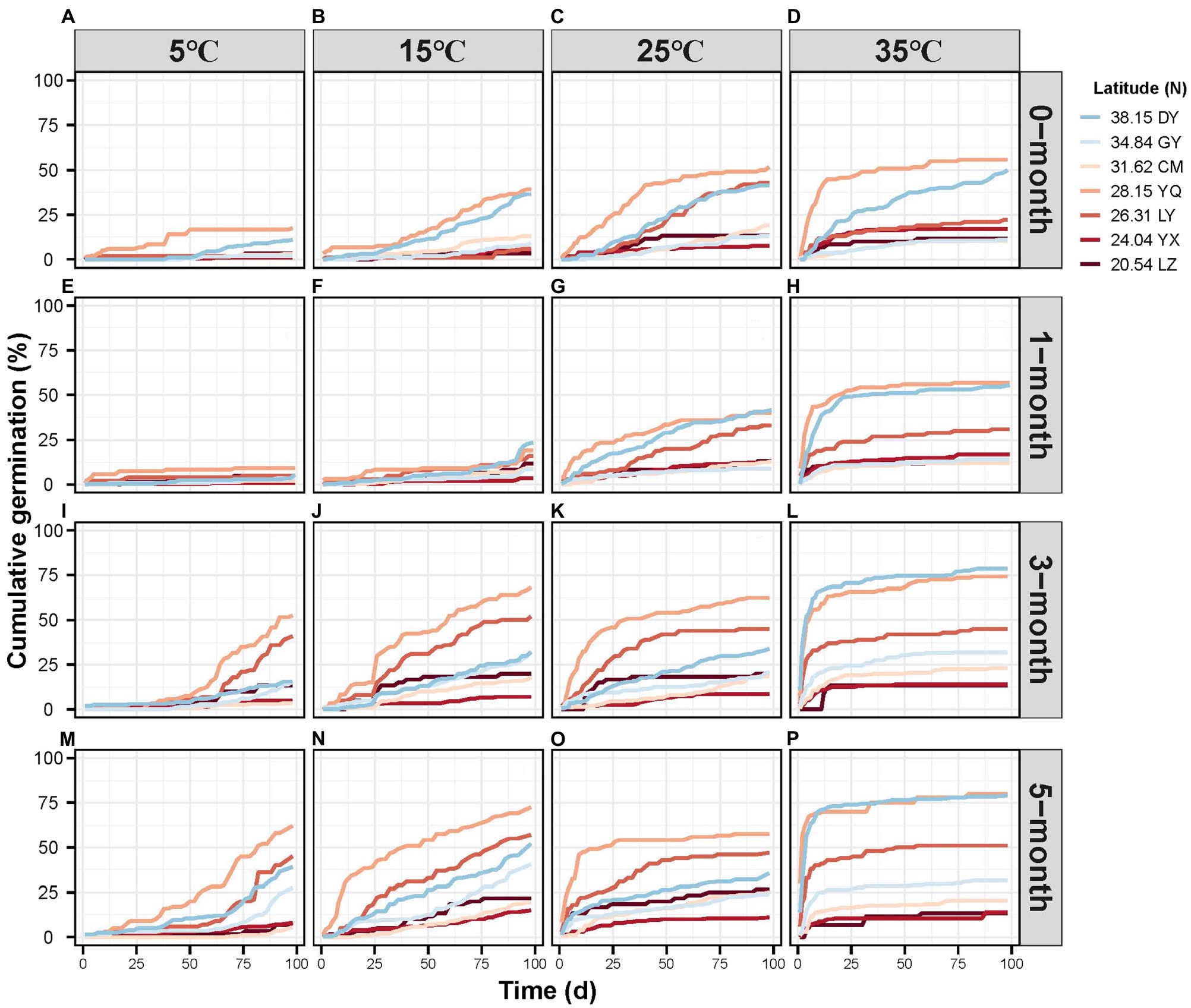
Figure 2. The cumulative germination percentage per observation day of different latitude sites under different stratification time (A-D 0-month; E-H 1-month; I-L 3-month; M-P 5-month) and temperature (A, E, I, M 5 °C; B, F, J, N 15 °C; C, G, K, O 25 °C; D, H, L, P 35 °C).
Germination Traits Response to Stratification Time and Temperature
In line with our predictions, longer stratification time and higher temperature both increased mean germination rate ( for stratification treatments; for temperature treatments) and germination index ( for stratification treatments; for temperature treatments; Figure 3A; Supplementary Figure S1A). Meanwhile, longer stratification and higher temperatures tended to decrease both mean germination time ( for stratification treatments; for temperature treatments) and T50 ( for stratification treatments; for temperature treatments; Figure 3B; Supplementary Figure S1B). Moreover, for germination rate, mean germination time, germination index and T50, there was a significant interaction between stratification and temperature ( for germination rate; for mean germination time; for germination index; for T50; Table 2).
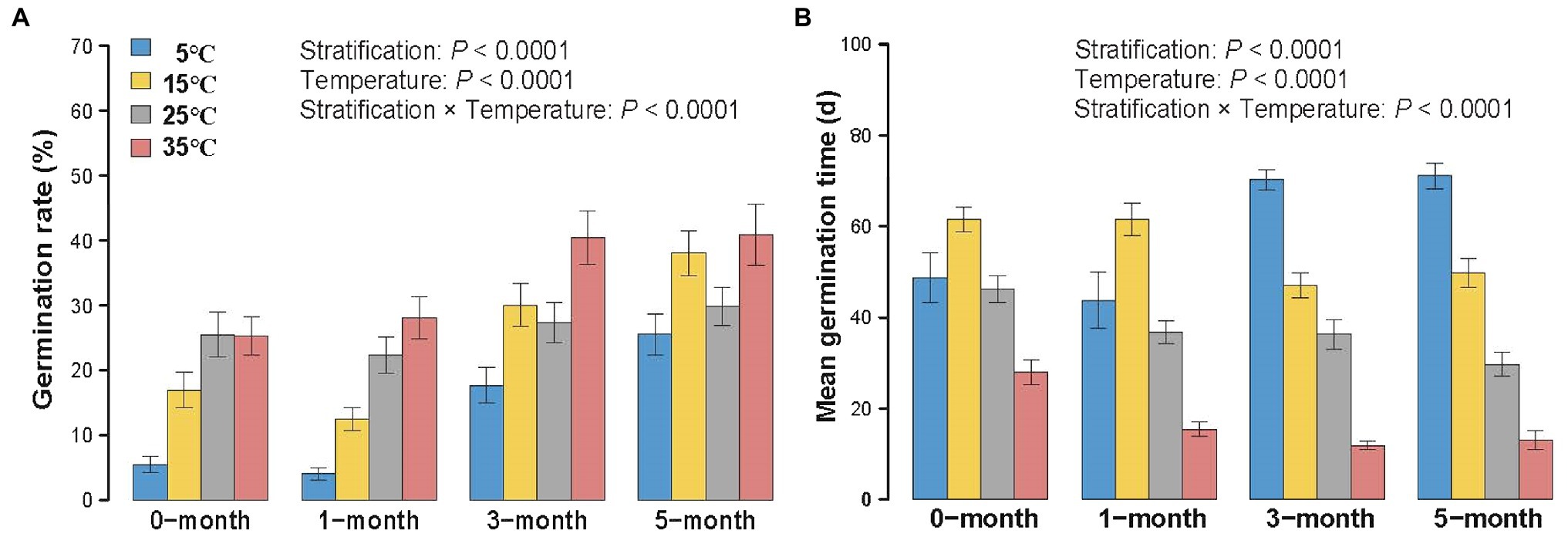
Figure 3. Variation of germination rate (A) and mean germination time (B) of 0/1/3/5-month-stratification Spartina alterniflora seeds under different temperatures (5°C–35°C). Values indicate means ± 1 SE.

Table 2. Mixed model analysis of germination rate, mean germination time, germination index and T50 of Spartina alterniflora, cold stratification and temperature as fixed effect with latitude (provenance) as random effect.
Germination Variation Across Latitude Relating to Stratification Time and Temperature
Germination rate increased with latitude, which interacted with stratification time and temperature (Figures 4A–D). It can be seen that the slope of germination rate and germination index to latitude increased with temperature, while germination time decreased (Figure 5; Supplementary Figure S3). Seeds exposed to the higher temperature and longer stratification had higher germination rates than seeds that were exposed to shorter stratification times and lower temperatures, and the effects of this were greater in seeds from higher latitudes due to the higher slope of germination rate to latitude (Figure 5A). A similar interaction was observed for germination index (Supplementary Figures S2C,D, S3A), while opposite for T50 and germination time with decreasing trends (Figure 5B, Supplementary Figure S3B). Seeds exposed to colder temperatures took longer to germinate than those exposed to warmer temperatures, but those from lower latitudes were faster to germinate than seeds from higher latitudes at the coldest temperature at short stratification times (Figures 4E–H, 5B; Supplementary Figures S2E-H, S3B). The combination of latitude, cold stratification and the temperature contributed to the final germination. Principal component analysis of the five climate variables across latitude that the first PC axes explained almost all variation in climate gradients (94.67%) and were mainly associated with annual min temperature and days of daily average temperature below 4°C (Supplementary Figure S4).
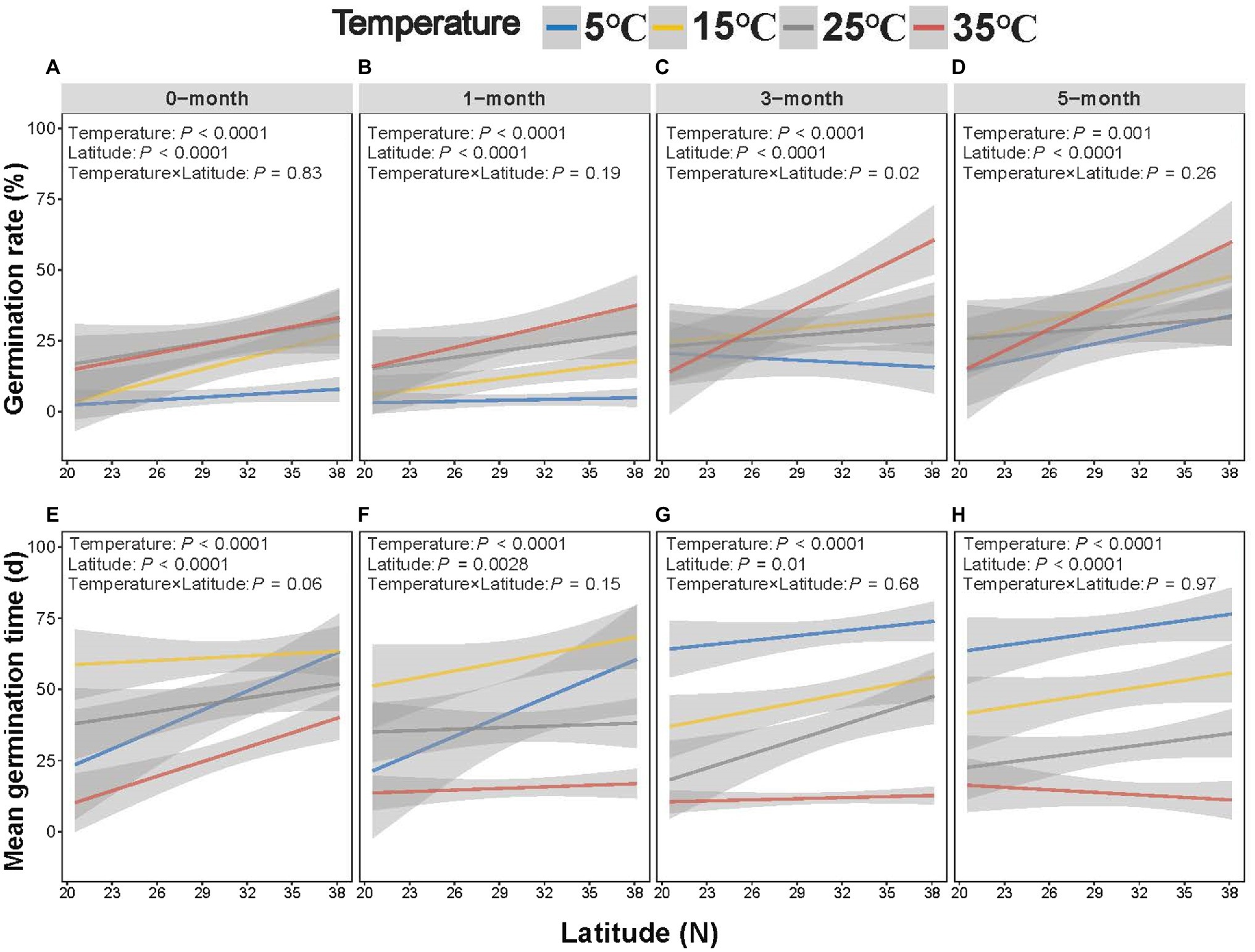
Figure 4. Effect of temperature/stratification treatments on the germination rate (A–D) and mean germination time (E–H) of Spartina alterniflora seeds from different latitudinal provenances (shaded area indicates 95% CI).
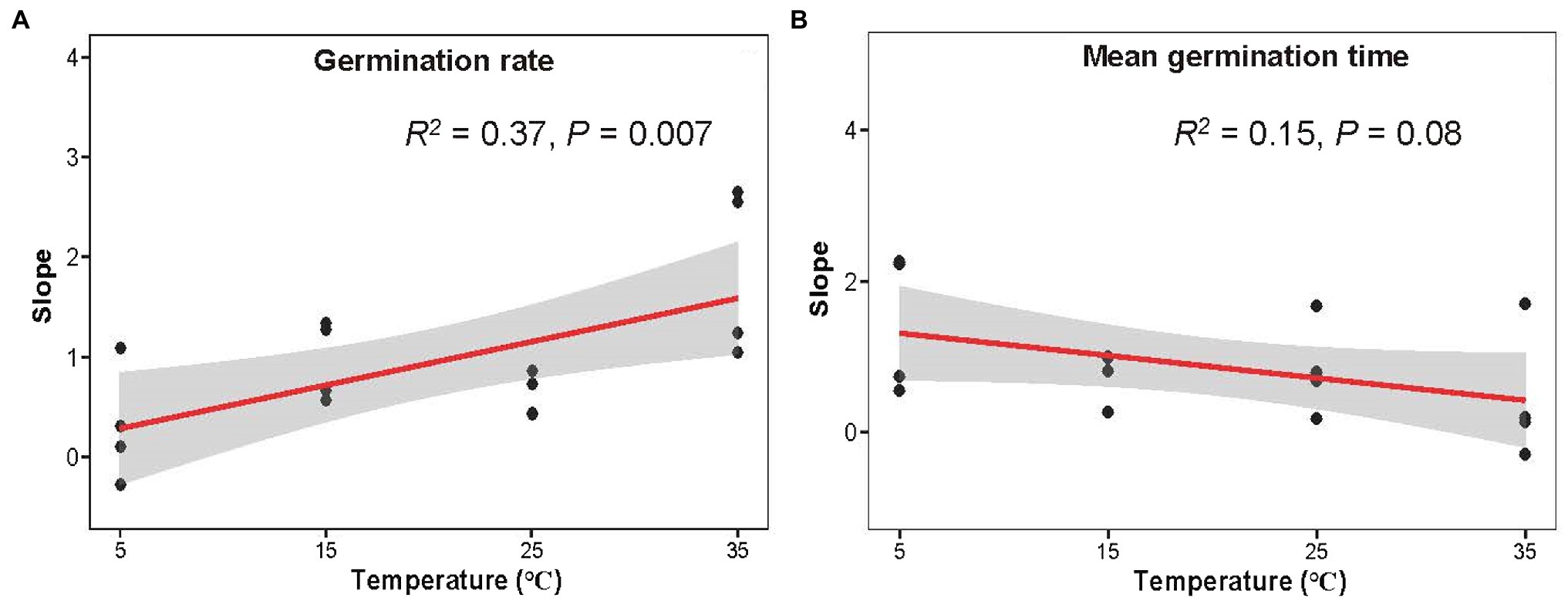
Figure 5. Variation of germination rate (A) and mean germination time slopes (B) of latitude influence of 0/1/3/5-month-stratification Spartina alterniflora seeds under different temperatures (shaded area indicates 95% CI). Slope was from Figure 4.
Discussion
Experimental exposure to cold stratification and temperature treatments affected the germination traits of S. alterniflora seeds of different provenances along a latitudinal gradient in China. Prolonging stratification time and higher temperature both promoted seed germination rate and shortened seed germination time. Moreover, these two factors interacted with each other to influence the germination characteristics of seeds. We found that germination rate and index increased linearly with latitude, but germination time decreased with latitude. Cold stratification interacted with temperature to influence this relationship. With the greatest slope in five-month stratification, lower slope in one or three-month stratification, and no relationship in zero stratification, germination rate shows the latitude effect. And with higher temperature and longer stratification time, the latitude effect becomes more significant. The adaptability of S. alterniflora seed germination to temperature and cold stratification across latitude is different, which reflects the ecological adaptability of S. alterniflora to the coastal ecosystem and its invasion potential to large-scale latitude in China. With climate change and global warming, it may therefore have the potential of evolution. This study predicts global warming is likely to accelerate the establishment, naturalization, and spread of invasive plants of S. alterniflora in higher latitudes in China.
Effects of Cold Stratification on Seed Germination
Cold and wet storage (stratification) is essential to break dormancy and promote the germination of seeds of S. alterniflora. A minimum of 4–8 weeks at 4°C appears necessary (Biber and Caldwell, 2008). Our findings confirm that cold stratification is an effective agent for breaking the seed dormancy and improving germination in S. alterniflora. The importance of cold stratification has been confirmed in many species, including Primula beesiana (Yang et al., 2020), Aster tripolium, and Triglochin maritimum (Al-Hawija et al., 2012), and Guizotia scabra, Parthenium hysterophorus, and Verbesina encelioides (Karlsson et al., 2008); while a moderate thermal stratification has a positive influence on seed germination in Cirsium arvense (Bochenek et al., 2009). This requirement for cold stratification also helps to prevent winter germination.
Cold stratification appears to have several benefits. First, it can improve superoxide dismutase (SOD) activity in seeds, removing any accumulated O2−, an adaptation of seeds to the low-temperature environment in winter. Second, levels of abscisic acid (which inhibits germination) decrease significantly during cold stratification, coincident with an increase in germination capacity (Feurtado et al., 2004). In addition, cold stratification can also break seed dormancy in halophytes (Kołodziejek and Patykowski, 2015) and allow germination at the right time to escape direct salt stress. For example, S. alterniflora seeds from North Carolina, United States, have a dormancy period of 6–12 months (Stalter, 1973) and germination of invasive Spartina in China coincides with spring rains and rises in temperature (Yuan and Shi, 2009). In contrast to previous studies (Stalter, 1973; Seneca, 1974), we found no negative effect of a stratification period over 4 months, but that germination rate and index were even greater at 5 months. This difference may be because our data are from invasive populations, which may be different from native populations. Stratification shortened T50 and MGT above 15°C but showed the opposite pattern at 5°C. Further research is needed to support and analyze this condition.
Effects of Temperature on Seed Germination
The role of temperature in regulating the seed dormancy of halophytes is known for some species (Ajmal Khan et al., 2000), such as Halogeton glomeratus, Lepidium latifolium, and Peganum harmala (Ahmed and Khan, 2010), and Puccinellia nuttalliana and Puccinellia distans (Tarasoff et al., 2007). S. alterniflora is also known to be sensitive to changes in temperature (Kirwan et al., 2009). The invasive spread of S. alterniflora is closely related to local temperature (Loebl et al., 2006; Liu et al., 2016). It was found that number of shoots which indicated clonal growth increased slightly with temperature (Liu et al., 2016). Our results show that germination responds positively to higher temperatures, with or without a period of cold stratification, consistent with previous studies (Zhu et al., 2011; Hayasaka et al., 2020). In future climate scenarios, the increase with temperature on both clonal growth and sexual reproduction may lead to population expansion.
Seeds undergo active metabolic reactions during germination. As such, within a certain range, germination processes are accelerated as temperature increases (Zhang et al., 2012), but too low or too high a temperature will affect germination due to membrane permeability, membrane-binding activity, and enzyme denaturation (Gul and Weber, 1999; Gulzar et al., 2001). The optimal temperature for seed germination is closely related to the original habitat of the population (Zhang et al., 2012). Our experimental temperature treatments (5°C–35°C) coincided with the suitable range experienced in nature, and the field temperatures experienced by S. alterniflora are rarely (if ever) more than 35°C (Table 1; Figure 1). In subsequent experiments, we can set higher temperature treatments. Temperature cues are particularly important for the release of dormancy to allow germination and may be particularly affected by rising temperatures due to climate change (Loarie et al., 2008; Footitt et al., 2013, 2018; Baskin and Baskin, 2014). Also, it is noteworthy that cold days is important which can delay the germination (Milbau et al., 2009; Chamorro et al., 2017; Gremer et al., 2020).
Latitudinal Variation in Seed Germination of Spartina alterniflora
Our results show an apparent increase in germination rate in seeds from higher latitudes, consistent with the cross-species analysis of herbs by Graae et al. (2009). Moreover, our results demonstrate that germination traits of seeds of S. alterniflora from different provenances at different latitudes differ significantly in response to stratification and ambient temperature. This effect of latitude was especially strong in terms of germination index with higher temperatures (Chamorro et al., 2018; Wang et al., 2021), which indicates that seed germination of populations at higher latitude was more sensitive to temperatures (Cochrane et al., 2015). Therefore, population recruitment at higher latitude may benefit from warmer climate accelerates germination (Wu et al., 2019). The responses of seeds from different latitudes to stratification were significantly different (Fowler and Dwight, 1964). This is because low-latitude populations lack a cold stratification requirement, and high-latitude populations experience cold temperatures and need cold stratification to release dormancy (Weng et al., 2006; Cortés-Fernández et al., 2021). Reproductive traits, such as seed set and seed survival of S. alterniflora also increase with latitude (Liu et al., 2017a). Invasive plant populations are likely very plastic and can adapt to different local environments (Feng et al., 2009; Liu et al., 2017a), and their traits will co-vary with these conditions that vary with latitude and altitude (Colautti et al., 2009; Castillo et al., 2014). Although it is difficult to determine the balance of genetic vs. environmental influence (Weng et al., 2006; Cortés-Fernández et al., 2021), our results indicate that S. alterniflora, which is distributed over a wide latitudinal range, may have significant adaptive variation in seed germination.
With global warming, this variation may lead to a mismatch between the optimal germination time of S. alterniflora seeds and the environmental cues of previous adaptation (Walck et al., 2011; Cochrane, 2019; Sentinella et al., 2020). These variations in space and time suggest that a warmer climate may improve the long-term survival of S. alterniflora, as well as its competitive abilities (Kirwan et al., 2009; Clements and Ditommaso, 2011). High-latitude provenances have existing high adaptability to a cold environment (Mao et al., 2015). Climate warming may affect the population dynamics of species by altering seed germination patterns, especially for frontier populations near the boundaries of their distribution (Wu et al., 2019), which should be a focus of future research.
Interaction Effects
Cold stratification requirements are especially common in species from high latitudes (Wagner and Simons, 2008), as our results demonstrated. Previous studies have found that seeds from high-latitude populations that experience more severe climates have deeper dormancies that require longer periods of cold stratification to overcome (Cavieres and Arroyo, 2000, 2001; Bernareggi et al., 2016). In contrast, low-latitude populations lack a cold stratification requirement and experience temperatures conducive for germination (Graae et al., 2009). Our finding that cold stratification improves seed germination from high latitudes is consistent with the results of previous work on arctic and alpine species (Cavieres and Arroyo, 2000; Liu et al., 2017b), which all experience a severe abiotic environment.
Local climate will differentially affect populations of S. alterniflora across its invasive range (Liu et al., 2017a). Seeds of populations from cooler climates (high-latitude sites) could germinate earlier under warmer temperatures as a result of the earlier onset of the warm season or regional warming (Picciau et al., 2019). In a climate change context, this response would favor a potential expansion toward northern or higher elevation sites. On the other hand, similar changes to local climates would negatively affect germination in lower latitude sites, which may lead to a range reduction at the warmer end of the distribution. Within the temperature gradient, we set up, germination of S. alterniflora was always promoted, showing that the existing invasion region of S. alterniflora is a suitable range of temperature for it. Future work could examine germination potential at even higher temperatures (including soil temperature) and conduct field observations and real reciprocal experiments in the field.
Our results show that with longer stratification time, temperature plays a more important role in seed germination. Stratification and temperature, alongside latitude, are thus important drivers of population and community dynamics (Huang et al., 2016), by their influence on plant fitness, habitat selection, or niche construction (Donohue et al., 2005, 2010). Including more than one variable to more fully capture the germination process can facilitate an understanding of how local climate characteristics can affect germination across the distribution of a species. The germination responses of the seeds we tested provide insights into possible future effects of climate change on S. alterniflora distribution. This study suggests that global warming may accelerate the expansion and spread of invasive S. alterniflora in higher latitude coastal environments of China.
Data Availability Statement
The data presented in the study are deposited in the Dryad repository at https://doi.org/10.5061/dryad.rbnzs7hdd.
Author Contributions
WL, YZ, and JC conceived and designed the research. JC, YZ, WH, XW, and WL performed the experiments. HH, JC, and WL analyzed the data. JC and WL wrote the manuscript. WL and YZ provided the funding. All authors contributed to the article and approved the submitted version.
Funding
This study was supported by the National Natural Science Foundation of China (grant nos. 32001234, 32025026, and 31971500), the Fundamental Research Funds for the Central Universities of China (grant no. 20720210075), and XMU Training Program of Innovation and Enterpreneurship for Undergraduates (grant no. 202010384136).
Conflict of Interest
The authors declare that the research was conducted in the absence of any commercial or financial relationships that could be construed as a potential conflict of interest.
Publisher’s Note
All claims expressed in this article are solely those of the authors and do not necessarily represent those of their affiliated organizations, or those of the publisher, the editors and the reviewers. Any product that may be evaluated in this article, or claim that may be made by its manufacturer, is not guaranteed or endorsed by the publisher.
Acknowledgments
We thank L. Li, Y. Xue, R. Xie, and Y. Jia for help in the field and germination experiments, and F. Cheng for help with the map.
Supplementary Material
The Supplementary Material for this article can be found online at: https://www.frontiersin.org/articles/10.3389/fpls.2022.911804/full#supplementary-material
Footnotes
References
Ahmed, M. Z., and Khan, M. A. (2010). Tolerance and recovery responses of playa halophytes to light, salinity, and temperature stresses during seed germination. Flora-Morphol. Distrib. Funct. Ecol. Plants 205, 764–771. doi: 10.1016/j.flora.2009.10.003
Ajmal Khan, M., Gul, B., and Weber, D. J. (2000). Germination responses of Salicornia rubra to temperature and salinity. J. Arid Environ. 45, 207–214. doi: 10.1006/jare.2000.0640
Al-Hawija, B. N., Partzsch, M., and Hensen, I. (2012). Effects of temperature, salinity and cold stratification on seed germination in halophytes. Nord. J. Bot. 30, 627–634. doi: 10.1111/j.1756-1051.2012.01314.x
An, S. Q., Gu, B. H., Zhou, C. F., Wang, Z. S., Deng, Z. F., Zhi, Y. B., et al. (2007). Spartina invasion in China: implications for invasive species management and future research. Weed Res. 47, 183–191. doi: 10.1111/j.1365-3180.2007.00559.x
Ayres, D. R., Zaremba, K., Sloop, C. M., and Strong, D. R. (2007). Sexual reproduction of cordgrass hybrids (Spartina foliosa x alterniflora) invading tidal marshes in San Francisco Bay: sexual reproduction of cordgrass hybrids. Divers. Distrib. 14, 187–195. doi: 10.1111/j.1472-4642.2007.00414.x
Baskin, J. M., and Baskin, C. C. (1977). Role of temperature in the germination ecology of three summer annual weeds. Oecologia 30, 377–382. doi: 10.1007/BF00399768
Baskin, J. M., and Baskin, C. C. (1998). Seed dormancy and germination in the rare plant species Amaranthus pumilus. Castanea 63, 493–494.
Baskin, J. M., and Baskin, C. C. (2014). What kind of seed dormancy might palms have? Seed Sci. Res. 24, 17–22. doi: 10.1017/S0960258513000342
Bernareggi, G., Carbognani, M., Mondoni, A., and Petraglia, A. (2016). Seed dormancy and germination changes of snowbed species under climate warming: the role of pre- and post-dispersal temperatures. Ann. Bot. 118, 529–539. doi: 10.1093/aob/mcw125
Bewley, J. D., Bradford, K. J., Hilhorst, H. W. M., and Nonogaki, H. (2013). Seeds. New York, NY: Springer.
Biber, P. D., and Caldwell, J. D. (2008). Seed germination and seedling survival of Spartina alterniflora Loisel. Am. J. Agric. Biol. Sci. 3, 633–638. doi: 10.3844/ajabssp.2008.633.638
Bochenek, A., Golaszewski, J., Piotrowicz-Cieslak, A. I., and Gorecki, R. J. (2009). The effects of temperature on the dormancy and germination of Cirsium arvense (L.). Scop. Seeds. Acta Soc. Bot. Pol. 78, 105–114. doi: 10.5586/asbp.2009.014
Brändle, M., Stadler, J., Klotz, S., and Brandl, R. (2003). Distributional range size of weedy plant species is correlated to germination patterns. Ecology 84, 136–144. doi: 10.1890/0012-9658(2003)084[0136:DRSOWP]2.0.CO;2
Castillo, J. M., Grewell, B. J., Pickart, A., Bortolus, A., Peña, C., Figueroa, E., et al. (2014). Phenotypic plasticity of invasive Spartina densiflora (Poaceae) along a broad latitudinal gradient on the Pacific coast of North America. Am. J. Bot. 101, 448–458. doi: 10.3732/ajb.1400014
Cavieres, L. A., and Arroyo, M. T. K. (2000). Seed germination response to cold stratification period and thermal regime in Phacelia secunda (Hydrophyllaceae). Plant Ecol. 149, 1–8. doi: 10.1023/a:1009802806674
Cavieres, L. A., and Arroyo, M. T. K. (2001). Persistent soil seed banks in Phacelia secunda (Hydrophyllaceae): experimental detection of variation along an altitudinal gradient in the Andes of Central Chile (33 S): soil seed banks in Phacelia secunda. J. Ecol. 89, 31–39. doi: 10.1046/j.1365-2745.2001.00514.x
Chamorro, D., Luna, B., and Moreno, J. M. (2017). Germination responses to current and future temperatures of four seeder shrubs across a latitudinal gradient in western Iberia. Am. J. Bot. 104, 83–91. doi: 10.3732/ajb.1600278
Chamorro, D., Luna, B., and Moreno, J. M. (2018). Local climate controls among-population variation in germination patterns in two Erica species across western Iberia. Seed Sci. Res. 28, 112–122. doi: 10.1017/S0960258518000041
Chen, X., Liu, W., Pennings, S. C., and Zhang, Y. (2021). Plasticity and selection drive hump-shaped latitudinal patterns of flowering phenology in an invasive intertidal plant. Ecology 102:e03311. doi: 10.1002/ecy.3311
Clements, D. R., and Ditommaso, A. (2011). Climate change and weed adaptation: can evolution of invasive plants lead to greater range expansion than forecasted? Weed Res. 51, 227–240. doi: 10.1111/j.1365-3180.2011.00850.x
Cochrane, J. A., Hoyle, G. L., Yates, C. J., Wood, J., and Nicotra, A. B. (2015). Climate warming delays and decreases seedling emergence in a Mediterranean ecosystem. Oikos 124, 150–160. doi: 10.1111/oik.01359
Cochrane, A. (2019). Multi-year sampling provides insight into the bet-hedging capacity of the soil-stored seed reserve of a threatened acacia species from Western Australia. Plant Ecol. 220, 241–253. doi: 10.1007/s11258-019-00909-0
Colautti, R. I., Maron, J. L., and Barrett, S. C. H. (2009). Common garden comparisons of native and introduced plant populations: latitudinal clines can obscure evolutionary inferences: evolution in invasive plants. Evol. Appl. 2, 187–199. doi: 10.1111/j.1752-4571.2008.00053.x
Cortés-Fernández, I., Cerrato, M. D., Ribas-Serra, A., Cardona, C., González, C., and Gil, L. (2021). Evidence of interpopulation variation in the germination of Eryngium maritimum L. (Apiaceae). Plant Ecol. 222, 1101–1112. doi: 10.1007/s11258-021-01164-y
Crosby, S. C., Ivens-Duran, M., Bertness, M. D., Davey, E., Deegan, L. A., and Leslie, H. M. (2015). Flowering and biomass allocation in U.S. Atlantic coast Spartina alterniflora. Am. J. Bot. 102, 669–676. doi: 10.3732/ajb.1400534
Daehler, C. C., and Strong, D. R. (1994). Variable reproductive output among clones of Spartina alterniflora (Poaceae) invading San Francisco Bay, California: the influence of herbivory, pollination, and establishment site. Am. J. Bot. 81, 307–313. doi: 10.1002/j.1537-2197.1994.tb15448.x
Donohue, K., Dorn, L., Griffith, C., Kim, E., Aguilera, A., Polisetty, C. R., et al. (2005). The evolutionary ecology of seed germination of Arabidopsis thaliana: variable natural selection on germination timing. Evolution 59, 758–770. doi: 10.1111/j.0014-3820.2005.tb01751.x
Donohue, K., Rubio de Casas, R., Burghardt, L., Kovach, K., and Willis, C. G. (2010). Germination, postgermination adaptation, and species ecological ranges. Annu. Rev. Ecol. Evol. Syst. 41, 293–319. doi: 10.1146/annurev-ecolsys-102209-144715
Feng, Y., Liao, Z., Zhang, R., Zheng, Y., Li, Y., and Lei, Y. (2009). Adaptive evolution in response to environmental gradients and enemy release in invasive alien plant species. Biodivers. Sci. 17, 340–352. doi: 10.3724/SP.J.1003.2009.09115 (In Chinese with English abstract).
Feurtado, J. A., Ambrose, S. J., Cutler, A. J., Ross, A. R. S., Abrams, S. R., and Kermode, A. R. (2004). Dormancy termination of western white pine (Pinus monticola Dougl. Ex D. Don) seeds is associated with changes in abscisic acid metabolism. Planta 218, 630–639. doi: 10.1007/s00425-003-1139-8
Footitt, S., Huang, Z., Clay, H. A., Mead, A., and Finch-Savage, W. E. (2013). Temperature, light and nitrate sensing coordinate A. rabidopsis seed dormancy cycling, resulting in winter and summer annual phenotypes. Plant J. 74, 1003–1015. doi: 10.1111/tpj.12186
Footitt, S., Huang, Z., Ölcer-Footitt, H., Clay, H., and Finch-Savage, W. E. (2018). The impact of global warming on germination and seedling emergence in Alliaria petiolata, a woodland species with dormancy loss dependent on low temperature. Plant Biol. 20, 682–690. doi: 10.1111/plb.12720
Fowler, D. P., and Dwight, T. W. (1964). Provenance differences in the stratification requirements of white pine. Can. J. Bot. 42, 669–675. doi: 10.1139/b64-062
Gillard, M., Grewell, B. J., Futrell, C. J., Deleu, C., and Thiébaut, G. (2017). Germination and seedling growth of water primroses: a cross experiment between two invaded ranges with contrasting climates. Front. Plant Sci. 8:1677. doi: 10.3389/fpls.2017.01677
Graae, B. J., Verheyen, K., Kolb, A., Van Der Veken, S., Heinken, T., Chabrerie, O., et al. (2009). Germination requirements and seed mass of slow- and fast-colonizing temperate forest herbs along a latitudinal gradient. Écoscience 16, 248–257. doi: 10.2980/16-2-3234
Gremer, J. R., Chiono, A., Suglia, E., Bontrager, M., Okafor, L., and Schmitt, J. (2020). Variation in the seasonal germination niche across an elevational gradient: the role of germination cueing in current and future climates. Am. J. Bot. 107, 350–363. doi: 10.1002/ajb2.1425
Grime, J. P., Mason, G., Curtis, A. V., Rodman, J., and Band, S. R. (1981). A comparative study of germination characteristics in a local Flora. J. Ecol. 69:1017. doi: 10.2307/2259651
Gul, B., and Weber, D. J. (1999). Effect of salinity, light, and temperature on germination in Allenrolfea occidentalis. Can. J. Bot. 77:7.
Gulzar, S., Khan, M. A., and Ungar, I. A. (2001). Effect of salinity and temperature on the germination of Urochondra setulosa (Trin.) C. E. Hubbard. Seed Sci. Technol. 29:10.
Hayasaka, D., Nakagawa, M., Maebara, Y., Kurazono, T., and Hashimoto, K. (2020). Seed germination characteristics of invasive Spartina alterniflora Loisel in Japan: implications for its effective management. Sci. Rep. 10:2116. doi: 10.1038/s41598-020-58879-7
Huang, L., Kuang, J., and Shu, W. (2016). Microbial ecology and evolution in the acid mine drainage model system. Trends Microbiol. 24, 581–593. doi: 10.1016/j.tim.2016.03.004
Karlsson, L. M., Tamado, T., and Milberg, P. (2008). Inter-species comparison of seed dormancy and germination of six annual Asteraceae weeds in an ecological context. Seed Sci. Res. 18, 35–45. doi: 10.1017/S0960258508888496
Kim, J. H., Suh, J. K., Yoon, S.-T., Jourdan, P., and Roh, M. S. (2018). Germination of fully developed corylopsis seeds influenced by harvest date and cold stratification. HortScience 53, 1360–1363. doi: 10.21273/HORTSCI13032-18
Kirwan, M. L., Guntenspergen, G. R., and Morris, J. T. (2009). Latitudinal trends in Spartina alterniflora productivity and the response of coastal marshes to global change. Glob. Chang. Biol. 15, 1982–1989. doi: 10.1111/j.1365-2486.2008.01834.x
Kołodziejek, J., and Patykowski, J. (2015). Germination and Dormancy in Annual Halophyte Juncus Ranarius. Song & Perr. Bot Horti Agrobo, 8.
Koning, C. O. (2006). Propagation of wetland plants: herbaceous plants, shrubs and trees. Restor. Ecol. 14, 321–322. doi: 10.1111/j.1526-100X.2006.00137.x
Leiblein-Wild, M. C., Kaviani, R., and Tackenberg, O. (2014). Germination and seedling frost tolerance differ between the native and invasive range in common ragweed. Oecologia 174, 739–750. doi: 10.1007/s00442-013-2813-6
Liu, W., Chen, X., Strong, D. R., Pennings, S. C., Kirwan, M. L., Chen, X., et al. (2020a). Climate and geographic adaptation drive latitudinal clines in biomass of a widespread salt marsh plant in its native and introduced ranges. Limnol. Oceanogr. 65, 1399–1409. doi: 10.1002/lno.11395
Liu, W., Zhang, Y., Chen, X., Maung‐Douglass, K., Strong, D. R., Pennings, S. C., et al. (2020b). Contrasting plant adaptation strategies to latitude in the native and invasive range of Spartina alterniflora. New Phytol. 226, 623–634. doi: 10.1111/nph.16371
Leon, R. G., Knapp, A. D., and Owen, M. D. K. (2004). Effect of temperature on the germination of common waterhemp (Amaranthus tuberculatus), giant foxtail (Setaria faberi), and velvetleaf (Abutilon theophrasti). Weed Sci. 52, 67–73. doi: 10.1614/P2002-172
Li, B., Liao, C., Zhang, X., Chen, H., Wang, Q., Chen, Z., et al. (2009). Spartina alterniflora invasions in the Yangtze River estuary, China: an overview of current status and ecosystem effects. Ecol. Eng. 35, 511–520. doi: 10.1016/j.ecoleng.2008.05.013
Liu, W., Maung-Douglass, K., Strong, D. R., Pennings, S. C., and Zhang, Y. (2016). Geographical variation in vegetative growth and sexual reproduction of the invasive Spartina alterniflora in China. J. Ecol. 104, 173–181. doi: 10.1111/1365-2745.12487
Liu, W., Strong, D. R., Pennings, S. C., and Zhang, Y. (2017a). Provenance-by-environment interaction of reproductive traits in the invasion of Spartina alterniflora in China. Ecology 98, 1591–1599. doi: 10.1002/ecy.1815
Liu, X., Xu, D., Yang, Z., and Zhang, N. (2017b). Geographic variations in seed germination of Dalbergia odorifera T. Chen in response to temperature. Ind. Crop Prod. 102, 45–50. doi: 10.1016/j.indcrop.2017.03.027
Liu, W., and Zhang, Y. (2021). Geographical variation in germination traits of the salt-marsh cordgrass Spartina alterniflora in its invasive and native ranges. J. Plant Ecol. 14, 348–360. doi: 10.1093/jpe/rtaa094
Liu, W., Chen, X., Wang, J., and Zhang, Y. (2022). Does the effect of flowering time on biomass allocation across latitudes differ between invasive and native salt marsh grass Spartina alterniflora?. Ecol. Evol. 12:e8681. doi: 10.1002/ece3.8681
Loarie, S. R., Carter, B. E., Hayhoe, K., McMahon, S., Moe, R., Knight, C. A., et al. (2008). Climate change and the future of California’s endemic flora. PLoS One 3:e2502. doi: 10.1371/journal.pone.0002502
Loebl, M., van Beusekom, J. E. E., and Reise, K. (2006). Is spread of the neophyte Spartina anglica recently enhanced by increasing temperatures? Aquat. Ecol. 40, 315–324. doi: 10.1007/s10452-006-9029-3
Mao, D., Yu, L., Chen, D., Li, L., Zhu, Y., Xiao, Y., et al. (2015). Multiple cold resistance loci confer the high cold tolerance adaptation of Dongxiang wild rice (Oryza rufipogon) to its high-latitude habitat. Theor. Appl. Genet. 128, 1359–1371. doi: 10.1007/s00122-015-2511-3
Milbau, A., Graae, B. J., Shevtsova, A., and Nijs, I. (2009). Effects of a warmer climate on seed germination in the subarctic. Ann. Bot. 104, 287–296. doi: 10.1093/aob/mcp117
Molina-Montenegro, M. A., Acuna-Rodriguez, T., Flores, S. M., Hereme, R., Lafon, A., Atala, C., et al. (2018). Is the success of plant invasions the result of rapid adaptive evolution in seed traits? Evidence from a latitudinal rainfall gradient. Front. Plant Sci. 9. doi: 10.3389/fpls.2018.00208
Moyo, M., Kulkarni, M. G., Finnie, J. F., and Van Staden, J. (2009). After-ripening, light conditions, and cold stratification influence germination of Marula [Sclerocarya birrea (A. rich.) Hochst. Subsp. caffra (Sond.) Kokwaro] seeds. HortScience 44, 119–124. doi: 10.21273/HORTSCI.44.1.119
Oksanen, J., Blanchet, F. G., Friendly, M., Kindt, R., Legendre, P., Dan, M., et al. (2019). Vegan: Community Ecology Package. R package version 2.5–4. Retrieved April 20, 2019, from https://CRAN.R-project.org/package=vegan
Picciau, R., Pritchard, H. W., Mattana, E., and Bacchetta, G. (2019). Thermal thresholds for seed germination in Mediterranean species are higher in mountain compared with lowland areas. Seed Sci. Res. 29, 44–54. doi: 10.1017/S0960258518000399
Pipinis, D. (2017). Punitive white welfare bureaucracies: examining the link between white presence within welfare bureaucracies and sanction exits in the United States. Soc. Serv. Rev. 91, 71–105. doi: 10.1086/690831
Plyler, D. B., and Carrick, K. M. (1993). Site-specific seed dormancy in Spartina alterniflora (poaceae). Am. J. Bot. 80, 752–756. doi: 10.1002/j.1537-2197.1993.tb15290.x
Plyler, D. B., and Proseus, T. E. (1996). A comparison of the seed dormancy characteristics of Spartina patens and Spartina alterniflora (Poaceae). Am. J. Bot. 83, 11–14. doi: 10.1002/j.1537-2197.1996.tb13868.x
Qiu, S., Xu, X., Liu, S., Liu, W., Liu, J., Nie, M., et al. (2018). Latitudinal pattern of flowering synchrony in an invasive wind-pollinated plant. Proc. R. Soc. B Biol. Sci. 285, 20181072. doi: 10.1098/rspb.2018.1072
Ranal, M. A., and Santana, D. G. D. (2006). How and why to measure the germination process? Rev. Brasil. Bot. 29, 1–11. doi: 10.1590/S0100-84042006000100002
R Core Team (2021). R: A language and environment for statistical computing. R Foundation for Statistical Computing, Vienna, Austria.
Seneca, E. D. (1974). Germination and seedling response of Atlantic and gulf coasts populations of Spartina alterniflora. Am. J. Bot. 61, 947–956. doi: 10.1002/j.1537-2197.1974.tb14034.x
Sentinella, A. T., Warton, D. I., Sherwin, W. B., Offord, C. A., and Moles, A. T. (2020). Tropical plants do not have narrower temperature tolerances, but are more at risk from warming because they are close to their upper thermal limits. Glob. Ecol. Biogeogr. 29, 1387–1398. doi: 10.1111/geb.13117
Solé-Medina, A., Heer, K., Opgenoorth, L., Kaldewey, P., Danusevicius, D., Notivol, E., et al. (2020). Genetic variation in early fitness traits across European populations of silver birch (Betula pendula). AOB Plants. 12:plaa019. doi: 10.1093/aobpla/plaa019
Somers, G. F., and Grant, D. (1981). Influence of seed source upon phenology of flowering of Spartina alterniflora Loisel. And the likelihood of cross pollination. Am. J. Bot. 68, 6–9. doi: 10.1002/j.1537-2197.1981.tb06349.x
Stalter, R. (1973). Seed viability in two Atlantic Coast populations of Spartina alterniflora. Castanea 38, 110–113.
Su, J., Li, C., Chen, L., Liu, X., Chen, S., and He, L. (2011). Effects of different pretreatment methods on seed germination of Rhododendron aureum and R.parvifolium. J. Plant Resour. Environ. 20, 64–69. (In Chinese with English abstract)
Tarasoff, C. S., Ball, D. A., and Mallory-Smith, C. A. (2007). After ripening requirements and optimal germination temperatures for Nuttall’s alkaligrass (Puccinellia nuttalliana) and weeping alkaligrass (Puccinellia distans). Weed Sci. 55, 36–40. doi: 10.1614/WS-06-050.1
Wagmann, K., Hautekèete, N.-C., Piquot, Y., Meunier, C., Schmitt, S. E., and Van Dijk, H. (2012). Seed dormancy distribution: explanatory ecological factors. Ann. Bot. 110, 1205–1219. doi: 10.1093/aob/mcs194
Wagner, I., and Simons, A. M. (2008). Intraspecific divergence in seed germination traits between high- and low-latitude populations of the Arctic-alpine annual Koenigia islandica. Arct. Antarct. Alp. Res. 40, 233–239. doi: 10.1657/1523-0430(07-003)[WAGNER]2.0.CO;2
Walck, J. L., Hidayati, S. N., Dixon, K. W., Thompson, K., and Poschlod, P. (2011). Climate change and plant regeneration from seed: climate change and plant regeneration. Glob. Chang. Biol. 17, 2145–2161. doi: 10.1111/j.1365-2486.2010.02368.x
Wang, Q., An, S. Q., Ma, Z. J., Zhao, B., Chen, J. K., and Li, B. (2006). Invasive Spartina alterniflora: biology, ecology and managementtude populations in relation to temperature. Acta Phytotaxon. Sin. 44, 559–588. doi: 10.1360/aps06044
Wang, X., Zhou, Y., Xue, Y., Cheng, J., Liu, W., and Zhang, Y. (2021). Seed germination characteristics of Spartina alterniflora from high and low latitude populations in relation to temperature. Chin. J. Ecol. 40, 763–2772. doi: 10.13292/j.1000–4890.202109.007
Watt, M. S., and Bloomberg, M. (2012). Key features of the seed germination response to high temperatures. New Phytol. 196, 332–336. doi: 10.1111/j.1469-8137.2012.04280.x
Weng, J.-H., and Hsu, F.-H. (2006). Variation of germination response to temperature in Formosan lily (Lilium formosanum wall.) collected from different latitudes and elevations in Taiwan. Plant Prod. Sci. 9, 281–286. doi: 10.1626/pps.9.281
Wu, H., Wang, S., Wei, X., and Jiang, M. (2019). Sensitivity of seed germination to temperature of a relict tree species from different origins along latitudinal and altitudinal gradients: implications for response to climate change. Trees 33, 1435–1445. doi: 10.1007/s00468-019-01871-0
Yang, L.-E., Peng, D.-L., Li, Z.-M., Huang, L., Yang, J., and Sun, H. (2020). Cold stratification, temperature, light, GA3, and KNO3 effects on seed germination of Primula beesiana from Yunnan. China. Plant Divers. 42, 168–173. doi: 10.1016/j.pld.2020.01.003
Yuan, Z., and Shi, F. (2009). Ecological adaptability of alien species Spartina alterniflora Loisel seed germination. Chin. J. Ecol. 197, 2466–2470. doi: 10.13292/j.1000-4890.2009.0412
Zhang, D., Hu, Y., Liu, M., Chang, Y., Yan, X., Bu, R., et al. (2017). Introduction and spread of an exotic plant, Spartina alterniflora, Along coastal marshes of China. Wetlands 37, 1181–1193. doi: 10.1007/s13157-017-0950-0
Zhang, H., Irving, L. J., Tian, Y., and Zhou, D. (2012). Influence of salinity and temperature on seed germination rate and the hydrotime model parameters for the halophyte, Chloris virgata, and the glycophyte, Digitaria sanguinalis. South Afr. J. Bot. 78, 203–210. doi: 10.1016/j.sajb.2011.08.008
Zhang, K., Yao, L., Zhang, Y., Baskin, J. M., Baskin, C. C., Xiong, Z., et al. (2019). A review of the seed biology of Paeonia species (Paeoniaceae), with particular reference to dormancy and germination. Planta 249, 291–303. doi: 10.1007/s00425-018-3017-4
Zhou, L., Yu, H., Yang, K., Chen, L., Yin, W., and Ding, J. (2021). Latitudinal and longitudinal trends of seed traits indicate adaptive strategies of an invasive plant. Front. Plant Sci. 12:657813. doi: 10.3389/fpls.2021.657813
Zhu, Z., Zhang, L., and Xiao, D. (2011). Seed production of Spartina alterniflora and its response of germination to temperature at Chongming Dongtan, Shanghai. Acta Ecol. Sin. 31, 1574–1581. (In Chinese with English abstract)
Keywords: invasive plants, cold stratification, seed germination, temperature, latitude, global warming
Citation: Cheng J, Huang H, Liu W, Zhou Y, Han W, Wang X and Zhang Y (2022) Unraveling the Effects of Cold Stratification and Temperature on the Seed Germination of Invasive Spartina alterniflora Across Latitude. Front. Plant Sci. 13:911804. doi: 10.3389/fpls.2022.911804
Edited by:
Suprasanna Penna, Bhabha Atomic Research Centre (BARC), IndiaCopyright © 2022 Cheng, Huang, Liu, Zhou, Han, Wang and Zhang. This is an open-access article distributed under the terms of the Creative Commons Attribution License (CC BY). The use, distribution or reproduction in other forums is permitted, provided the original author(s) and the copyright owner(s) are credited and that the original publication in this journal is cited, in accordance with accepted academic practice. No use, distribution or reproduction is permitted which does not comply with these terms.
*Correspondence: Wenwen Liu, lww@xmu.edu.cn