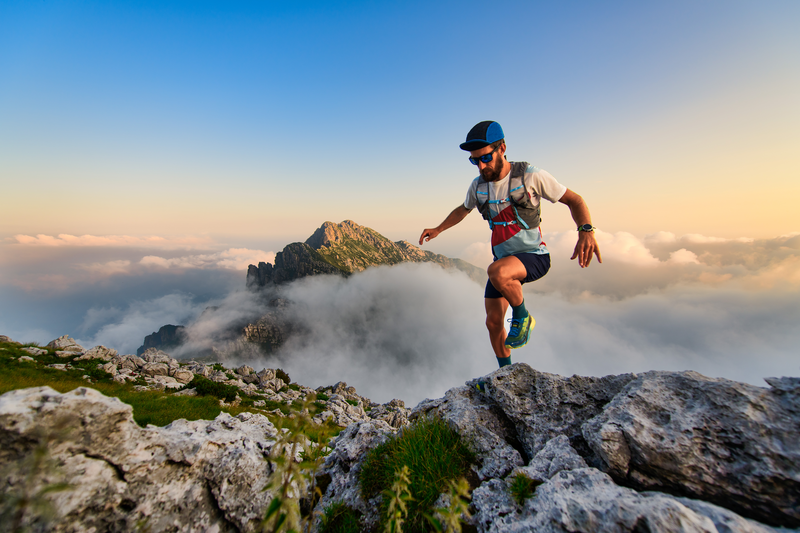
94% of researchers rate our articles as excellent or good
Learn more about the work of our research integrity team to safeguard the quality of each article we publish.
Find out more
ORIGINAL RESEARCH article
Front. Plant Sci. , 08 July 2022
Sec. Plant Metabolism and Chemodiversity
Volume 13 - 2022 | https://doi.org/10.3389/fpls.2022.899444
This article is part of the Research Topic XVII Spanish Portuguese Congress on Plant Biology (BP2021) - Plant Biochemistry and Metabolism View all 8 articles
Environmental conditions are key factors in the modulation of the epigenetic mechanisms regulating gene expression in plants. Specifically, the maintenance of cell cultures in optimal in vitro conditions alters methylation patterns and, consequently, their genetic transcription and metabolism. Paclitaxel production in Taxus x media cell cultures is reduced during its maintenance in in vitro conditions, compromising the biotechnological production of this valuable anticancer agent. To understand how DNA methylation influences taxane production, the promoters of three genes (GGPPS, TXS, and DBTNBT) involved in taxane biosynthesis have been studied, comparing the methylation patterns between a new line and one of ~14 years old. Our work revealed that while the central promoter of the GGPPS gene is protected from cytosine methylation accumulation, TXS and DBTNBT promoters accumulate methylation at different levels. The DBTNBT promoter of the old line is the most affected, showing a 200 bp regulatory region where all the cytosines were methylated. This evidence the existence of specific epigenetic regulatory mechanisms affecting the last steps of the pathway, such as the DBTNBT promoter. Interestingly, the GGPPS promoter, a regulatory sequence of a non-specific taxane biosynthetic gene, was not affected by this mechanism. In addition, the relationship between the detected methylation points and the predicted transcription factor binding sites (TFBS) showed that the action of TFs would be compromised in the old line, giving a further explanation for the production reduction in in vitro cell cultures. This knowledge could help in designing novel strategies to enhance the biotechnological production of taxanes over time.
As sessile organisms, plants must deal continuously with environmental fluctuations and external stressors. Therefore, they have developed a wide variety of mechanisms to ensure a strict gene expression regulation, allowing them to adapt their physiology efficiently. In the early ‘70s, the flow of biological information was suggested to follow from DNA to RNA and, finally, to protein (Crick, 1970). Years later, it was found that less than 5% of the genomic DNA encoded for proteins. The rest was composed of the so-called junk DNA, including transposable elements (TE) and highly repetitive DNA (Nowak, 1994). Nowadays, the initially considered “junk” DNA turned into the key to understanding how genes and genomes are regulated. A well-known example is gene promoters, which despite not being part of the coding sequence (CDS), contain the necessary elements to initiate and regulate the transcription process, representing a central role in gene regulation (Porto et al., 2014). In addition, a new layer of complexity has been found in gene regulation since not only the genetic sequences have been demonstrated to be relevant in gene transcription but also their epigenetic patterns.
Epigenetic marks include a vast range of regulatory events, such as chromatin structure remodeling, histone modifications, DNA methylation and small non-coding RNAs action, that do not involve changes in the DNA sequence (Springer and Schmitz, 2017). Epigenetic patterns have been believed to be transient and very dynamic. Nevertheless, some epigenetic variations have been proved to be meiotically heritable in plants without altering the DNA sequence (Kaeppler and Phillips, 1993), later defining the so-called epialleles. This distinctive mechanism supports the existence of an epigenetic memory in plant genomes, although the specific mechanisms are unexplored yet (Mutanda et al., 2021).
DNA methylation is an evolutionarily ancient covalent modification that involves the addition of a methyl group to the fifth position of the pyrimidine ring of cytosine bases to form 5-methylcytosine (5-mC; Zhang et al., 2018). Methylation of promoter regions is often associated with transcriptional repression, meaning gene silencing (Law and Jacobsen, 2010). In plant, cytosine methylation can take place in three different contexts: the symmetric CG and CHG and the asymmetric CHH (where H can correspond to A, T, or C; Karim et al., 2016). Regarding the establishment of the 5-mC patterns, two mechanisms have been identified: the de novo and the maintenance pathways, extensively described by He et al. (2011). The de novo mechanism establishes new patterns, while the maintenance mechanism conserves methylation patterns in the newly generated strands of DNA during the state of post-replicative DNA modification (Springer and Schmitz, 2017). Epigenetic patterns, and specifically DNA methylation, have been demonstrated to play a key role in controlling gene transcription to respond to environmental changes or stress (He et al., 2011; Zhang et al., 2018). These responses are linked with the production of a wide range of specialized metabolites to protect themselves from stress signals.
Natural products derived from plant specialized metabolism are highly demanded due to their effectiveness against a wide range of diseases, including taxanes to treat cancer (Changxing et al., 2020), tannins and flavonoids to treat diabetes (Mtewa et al., 2021) and flavonoids to treat hepatic alterations (Bachar et al., 2020), among others. However, both the low concentrations of specialized metabolites in their natural source and the over-harvesting of wild plants which have left several species endangered are impediments to meet this increasing demand (Georgiev et al., 2009). In this stage, plant cell cultures can be employed as biofactories to produce a great diversity of compounds, providing a promising, economical, and environmentally friendly solution. However, certain limitations still need to be overcome before their maximum potential is reached. One of these limitations is the gradual loss of specialized metabolite production during in vitro culture maintenance, which represents an important barrier in the development of large-scale production systems (Dougall et al., 1980; Deus-Neumann and Zenk, 1984; Beum et al., 2004). Why cell cultures lose the ability to produce high levels of specialized metabolites when cultured in optimized conditions while this is not happening in in vivo conditions, remains unclear.
Taxanes have been demonstrated to be a group of particular interest due to their unique anticancer mechanism of action. Among taxanes, paclitaxel, the active principle of Taxol®, is a phytopharmaceutical anticancer drug used to treat several types of cancer, including metastatic carcinoma of ovary and breast cancer, and tumors in lungs, skin, colon, kidneys, and prostate (Vidal-Limon et al., 2018). This natural antitumoral agent is a diterpenoid specialized metabolite that was first isolated in the late ‘60s. Paclitaxel blocks the mitosis progression and the activation of the mitosis checkpoint, leading to apoptosis or to the reversion of cancer cells to G0 phase (Mutanda et al., 2021). Its biosynthetic pathway comprises 19 enzyme-catalyzed steps, 15 of which are known, starting from the precursor geranylgeranyl diphosphate (GGPP) and leading to paclitaxel itself (Croteau et al., 2006). At the moment, some genes have been highlighted as putative flux-limiting genes, generating a highly controlled synthesis of taxanes (Onrubia et al., 2013; Lenka et al., 2015; Escrich et al., 2021). Nevertheless, the specific mechanism regulating taxanes biosynthesis is still not fully raveled, hindering the development of novel strategies to reach high yields of paclitaxel using rational approaches.
Available evidence shows that altered cytosine methylation patterns can be found in in vitro systems, leading to discrete phenotypic changes (Finnegan et al., 1996; Stelpflug et al., 2014; Ghosh et al., 2021). In addition, higher DNA methylation levels have been correlated with a low yield of specialized metabolites in vitro plant cell cultures (Tyunin et al., 2016; Sanchez-Muñoz et al., 2019). In our previous study, the transcriptomic and phytochemical profiling of the long-term in vitro maintained cell culture were performed (Sanchez-Muñoz et al., 2018). These results show that the taxane production decreases 4 times under elicited conditions. Accordingly, the genes of the taxane biosynthetic pathway present a reduced transcription activity, even in the presence of the elicitor MeJA. The longer the cell cultures had been maintained in optimized in vitro conditions, the greater was the number of methylated cytosines found throughout the BAPT promoter.
The production of certain compounds, in this case taxanes, is mainly regulated by the transcription process, and is principally controlled by the promoter structure. In plants, the transcription process is triggered by the initiation complex, recruiting the RNA polymerase near the transcription start site (TSS). Plant promoters can be structured in three regions; the core promoter, situated from −35 to +35 of the TSS (Porto et al., 2014), and the proximal and distal promoter regions, situated further from the TSS, from 200 to −100 bp and up to thousands of bp from the TSS, respectively (Peremarti et al., 2010). These regions usually contain cis elements that can act as enhancers or silencers by the binding of specific TFs and, specifically in the core promoter, both transcription initiators [namely the TATA-box, the Initiation Region (INR) and the Downstream Promoter Elements (DPE)] and facilitators (such as the CAAT-box and the Y-patch region) can be found. Some studies focused on transcription factor binding sites (TFBSs) demonstrated that different MeJA-responsive motifs were located in all key genes of the taxane biosynthetic pathway (Lenka et al., 2015; Yanfang et al., 2018; Zhou et al., 2019). The relation between TFBSs and gene accessibility to the RNA transcription machinery could be modified and influenced by DNA methylation, affecting gene expression and, therefore, various cellular processes (Law and Jacobsen, 2010).
The aim of this study is to provide new insights into the gradual loss of taxane production in Taxus spp. cell cultures maintained in optimized in vitro conditions and the specific methylation mechanisms involved. Therefore, a low producer Taxus x media cell line (of ~14 years old) and a new cell culture recently induced from fresh plant material were compared to reveal specific methylation changes during its maintenance in optimal conditions. The promoter region of three taxane biosynthetic genes was studied: the geranylgeranyl diphosphate synthase (GGPPS), the first gene of the diterpene pathway and, therefore, a non-specific taxane gene; the taxadiene synthase (TXS) gene, involved in the first step of the taxane biosynthesis; and the 3′-N-debenzoyl-2′-deoxytaxol-N-bezoyltransferase (DBTNBT) gene, the last gene of the pathway. With this specific set of genes, we aim to distinguish methylation patterns between three differential steps in taxane biosynthesis. The bisulfite sequencing technique was used to find different methylation patterns in these three promoters between the two age-different cell cultures, since it allows the study of methylation patterns genome-wide or in specific sequences (Cokus et al., 2008). The methylation level was determined at a single-cytosine resolution to find specific changes in the regulation of the different parts of taxane biosynthesis, as well as hotspots of DNA methylation in the three studied promoter regions. This analysis differentiates between the three possible cytosine contexts: CG, CHG, and CHH (being H any nucleotide except G). Furthermore, in silico analysis were carried out to identify cis-acting regulatory element involved in the MeJA-responsiveness. The integration of both processes could provide novel insights into taxane regulation, clarifying the specific mechanism influencing paclitaxel production.
Taxus × media callus tissue was obtained by dedifferentiation of Taxus × media young stem fragments (Expósito et al., 2009) and maintained for more than 10 years by biweekly subcultures on solid Gamborg’s B5 callus growing media (Gamborg et al., 1968) supplemented with 0.5% sucrose, 0.5% fructose, growth regulators [picloram (2 mg L−1), kinetin (0.1 mg L−1), and gibberellic acid (0.5 mg L−1)], and 1 ml of an antioxidant cocktail containing L-glutamine (29.23 g L−1), L-ascorbic acid (5.02 g L−1), and citric acid (4.99 g L−1). To obtain new callus cultures, T. × media sterilized explants were dedifferentiated on Gamborg’s B5 solid media (Gamborg et al., 1968) supplemented with 3% sucrose, growth regulators [2.4-dichlorophenoxyacetic acid (4 mg L−1), kinetin (1 mg L−1), and gibberellic acid (0.5 mg L−1)], and 1 ml of the antioxidant cocktail. The new callus tissue was maintained as above. In order to differentiate both calli tissues, the approximately 14-year-old callus tissue was called the old cell line (old line) and the freshly obtained was called the new cell line (new line).
The bisulfite (BS) method has been used for detecting changes in the genome-wide methylation patterns or specific sequence context in tissue culture plants. BS treatment implies the treatment of genomic DNA with sodium bisulfite which causes cytosine conversion to uracil, while methylated cytosine (5-mC) is not converted to uracil (Cokus et al., 2008). Dellaporta protocol for plant DNA extraction was adapted to extract genomic DNA (gDNA) from 400 mg of T. × media callus tissue (Dellaporta et al., 1983). One μg of DNA was treated using the EpiTect Bisulfite Kit (Qiagen, Hilden, Germany). Demethylated DNA by PCR amplification was used as a control to check the conversion efficiency of treated DNA. Specific degenerated primers (Supplementary Material 1), containing Y or R instead of C or G in the forward and the reverse primers, respectively, were used to amplify overlapping segments of 150–300 bp of the promotor and the early CDS regions of GGPPS, TXS, and DBTNBT genes, covering from the core promoter to the starting region of the CDS. 0.5% agarose gel was used to visualize the amplified DNA fragments and purify them with the Wizard® SV Gel and PCR Clean-Up System (Promega, Madison, WI, United States). Amplified and cleaned-up samples were sequenced using an ABI3730 DNA analyzer (Applied Biosystems™, ThermoFisher Scientific, Waltham, MS, United States).
From each sequenced sample, two Ab1 files as sequencing output were obtained, one for the forward and the other for the reverse strand. The chromatograms contained in the Ab1 files were used to determine the percentage of methylation for single-cytosine in the sequences described by Parrish et al. (2012). The relationship of T and C signals and G and A signals represented the percentage of methylation of each cytosine (mC) calculated with the formula: mC = C/(C + T) × 100, for sequences obtained using forward primers, and mC = G/(G + A) × 100, for reverse primers. With this data, we differentiate between general and specific methylation levels. For the general methylation state, we establish a cytosine as methylated when its specific methylation value is more than 50% in the analyzed sample. On the other hand, the specific cytosine methylation percentage is directly obtained from the processed Ab1 file and represents the average of the exact level of methylation in the complete cell culture. The sequences were aligned using Clustal Omega from EMBL-EBI tools (Li et al., 2015) and graphically represented by Cymate software (Hetzl et al., 2007). The master sequence was obtained from the NCBI database. As a negative control, the BS conversion of PCR amplified fragments (where the methylation of all the cytosines is lost) was used. T-test was performed using GraphPad Prism version 7 for Windows, GraphPad Software, California, United States. A p value of <0.05 (*), <0.005 (**), and < 0.001 (***) was assumed for significant differences.
The percentage of pyrimidine (Y) presented in the promoter was represented using the sequence annotation plotting library of the DNA Features Viewer with a window size of 30 pb (Zulkower and Rosser, 2020). The promoter structure was annotated including the cis elements previously detected (see section Analysis of Y Percentage). The count of each base and the pyrimidine and purine percentages of the studied regions were obtained by Unipro Ugene software (v. 40.0; Okonechnikov et al., 2012).
Regulatory Sequence Analysis Tools for Plants (RSAT Plants; Medina-Rivera et al., 2015) and PlantCARE (Lescot et al., 2002) were used to predict the cis-regulatory elements present in the core promoter. The online tool RNA polymerase II promoters in plant DNA sequences TSSPlant (Softberry Inc.; Solovyev et al., 2010) was used to predict the transcription start sites (TSSs) and the presence of TATA-box in the selected promoters. The cis-regulatory elements involved in methyl-jasmonate (MeJA) and ethylene responses were predicted using PlantPAN 3.0 (Chow et al., 2019).
The analyzed genes were obtained from the NCBI database and are freely accessible under the following accession numbers: AY566309.1 (GGPPS complete gene, Taxus × media), EF153471.1 (TXS promoter and partial CDS, Taxus cuspidata), FJ603644.1 (DBTNBT promoter, T. cuspidata), and AY563629.1 (DBTNBT partial promoter and complete CDS, T. cuspidata).
In order to discriminate different methylation patterns through the taxane biosynthetic pathway we selected three genes involved in different points of it: GGPPS as a non-specific taxane biosynthetic gene and shared by other plant physiological processes, TXS as the first specific taxane biosynthetic gene, and DBTNBT, the last step of taxane biosynthesis. The general methylation state where the cytosines were represented as methylated or not was defined to detect methylation hotspots within the sequences. In Figure 1, it is represented the distribution of the methylated cytosines through the selected promoter regions in both the old and new cell line, clearly showing notable differences between the three genes.
Figure 1. Bisulfite-sequencing methylation analysis of the partial promoter region in the old and the new Taxus x media cell line. (A) GGPPS partial promoter. (B) TXS partial promoter. (C) DBTNBT partial promoter 352 nucleotides before TSS, fragment 1. (D) DBTNBT partial promoter from TSS to CDS, fragment 2. Master and negative sequences correspond to the untreated and the unmethylated sequences, respectively. The three different cytosine contexts are indicated in red spheres (CG), blue squares (CHG) and green triangles (CHH), colored (methylated) or empty (not methylated).
The analyzed GGPPS promoter region did not present methylated cytosines in any context, neither in the new line nor the old line (Figure 1A). On the other hand, the TXS promoter region presented a slightly higher methylation level, presenting a differential methylation pattern comparing the new line and the old line (Figure 1B). While the new line only showed four methylated cytosines, the old line had 13 methylated cytosines, seven of them were in the 100 nucleotides upstream of the CDS. Interestingly, the study of the cytosine content in the TXS promoter region shows a completely different composition due to the lack of CHG context (Figure 1B).
The DBTNBT promoter was split up into two different representations due to the distance between the TSS and the CDS (see section Analysis of Y Percentage). Its cytosine composition and methylation pattern are represented by a region of 352 nucleotides before TSS, and a region of 202 nucleotides from the TSS to the CDS (Figures 1C,D, respectively). Methylated cytosines were found in the DBTNBT promoter in both the new and the old cell lines in all three contexts. The cytosine methylation pattern before the TSS showed that almost all CG and CHG cytosine contexts were methylated in both new and old cell lines with 92.31% and 96.51% of methylated cytosines, respectively. This methylation pattern was not detected in the other two genes previously studied, suggesting a differential methylation regulation focused on this specific part of the pathway.
Moreover, in the region represented before TSS, the old line showed more methylated CHH than the new line. The study of the DBTNBT promoter region after the TSS showed a non-homogeneous (methylated and unmethylated) cytosine distribution, showing an unequal cytosine accumulation through its sequence. In addition, the methylated cytosines were found in a specific region of approximately 180 nucleotides (Figure 1D). Furthermore, the distribution of the cytosines (methylated or not) were different from position 108 until 179, being clustered in groups of 4–5 cytosines.
Table 1 represents the study of the general state of methylation in each promoter, as well as the specific methylation levels of each cytosine for each cell line (for more details, see section Analysis of Position-Specific DNA Methylation). The GGPPS promoter showed unmethylated cytosines along the studied region, and, consequently, the general methylation state is zero in all three contexts (Figure 1A; Table 1). Concordantly, the specific cytosine methylation percentage was low in all three cytosine contexts for both cell lines. The TXS promoter region presented differences in the general and specific cytosine methylation states between both cell lines. The CG context and the total percentage of specific methylated cytosines showed a significant difference between the two cell lines, although the differences showed in the total amount could be attributed to the CG methylation context.
The analysis of the specific methylation state in the DBTNBT promoter revealed that the three cytosine contexts presented significant differences, although the CHH context presented the most significant differences (Table 1). On the DBTNBT fragment from the TSS to CDS, the new line showed few methylated cytosines compared with the old line, which showed a significant highly methylated cytosine region whose mainly correspond to CHH cytosines (Figures 1C,D). Surprisingly, in the DBTNBT promoter region, before the TSS, both lines had at least more than 90% of CG and CHG methylated cytosines (Table 1; Figure 1C). However, in the region from the TSS to the CDS no CG methylated cytosines were found, and less than 50% of the CHG cytosines were methylated in the new line (Figure 1D). Interestingly, these results highlighted that the differential epigenetic regulation through the DBTNBT promoter region, as well as that the significant difference in the total amount of specific methylation could be mainly attributed, as expected, to the changes in the CHH context (Table 1).
The heterogeneous distribution of cytosines through the selected promoters motivated the further study of the pyrimidine composition of these regions. Pyrimidine (Y) accumulation is often related to the presence of a Y-patch region, usually located in the proximal promoter between the TSS and the CDS (Yamamoto et al., 2007a). In addition, a prediction of cis-regulatory elements was performed to identify possible targets of the Y accumulation. As the first result of this approach, the three genes studied showed to be TATA-less promoters (Figure 2). Therefore, they required of other cis elements to finely regulate gene transcription. As expected, the three promoters showed the minimal cis-motifs for transcriptional activity regulation near the TSS: the INR, several DPEs and the CAAT-box. The putative Y-patch region (orange box) is defined as a region, rich in pyrimidine content, that involves at least 100 nucleotides upstream of the CDS and comprises the core promoter and the TSS (Figure 2).
Figure 2. Percentage of pyrimidine presence in the promoter. It is represented the distal, proximal, and core promoter. The predicted cis elements are detailed in the zoomed core promoter. (A) GGPPS promoter, (B) TXS promoter and (C) DBTNBT promoter.
The determination of pyrimidine content of the three promoters under study (Figures 2A–C) predicted the location of the putative Y-Patch region. The selected accumulations of %Y were located from the position 1,113 to 1,240 for the GGPPS promoter (Figure 2A); from 1,067 to 1,221 for the TXS promoter (Figure 2B) and from 1,796 to 1,891 for the DBTNBT promoter gene (Figure 2C). These three regions were defined as Y-regions.
Table 2 shows that the three promoters, GGPPS, TXS and DBTNBT, present a nearly equal ratio of pyrimidines and purines, around 50%. On average, the difference of pyrimidine and purine percentage in GGPPS and TXS oscillates ±2.3 and 2%, respectively. The deep study of the nucleotide composition showed that GGPPS promoter composition remarkably presented only two and four cytosines in the core promoter and putative Y-region, respectively, whose are clearly underrepresented in relation with the other nucleotides (Table 2). That reduces the potential methylation sites due to the shortage of cytosines. The core promoter of TXS presented a variance of ±4.1% in the composition of pyrimidine and purine and, as above-mentioned, there was an accumulation reaching 66% of Y content. Nevertheless, observing the single nucleotide composition, this pyrimidine percentage is due to the quantity of thymine located in this region rather than cytosines (Table 2). As occurs with the GGPPS promoter, this fact significantly reduces potential methylation sites. Pyrimidine levels in the DBTNBT promoter present the highest rate of Y content compared to GGPPS and TXS promoters (Table 2). In this case, the prediction of cis elements in the DBTNBT promoter locates the TSS at position 1,689, showing a long distance from the TSS to the CDS (~200 bp; Figure 2C). This distance was highlighted by an orange box containing two separate pyrimidine accumulations. The valley between these two accumulations corresponds to the cytosine shortage located in positions 1 to 100 in the previous graphical representation (Figure 1D).
Table 2. Pyrimidine and purine percentage and single nucleotide composition of GGPPS, TXS, and DBTNBT promoters.
The nucleotide composition of the whole DBTNBT promoter sequence oscillated ±4.4 from the balanced value of 50% (Table 2). However, along the proximal promoter and core promoter, the composition of purine and pyrimidine was balanced. The Y-region of the DBTNBT promoter presents the highest unbalanced pyrimidine–purine percentage, reaching a variance of ±7.3%. Surprisingly, in discordance with GGPPS and TXS promoters, the main pyrimidine base in this Y-region is cytosine representing more than 50%. The high rate of cytosines in this specific region of the DBTNBT promoter sequence indicates that this region could be highly regulated by DNA methylation mechanisms.
To study the role of DNA methylation in plant stress responses, cis-regulatory elements in response to MeJA and ethylene signals have been predicted and located through promoters due to their established relationship with plant specialized metabolism. From these data, we integrated the predicted cis elements and the methylation patterns found in the GGPPS, TXS, and DBTNBT promoters (Figure 3).
Figure 3. cis-acting regulatory elements involved in defense and stress responsiveness in the partial promoter regions of Taxus x media cell lines. (A) GGPPS partial promoter. (B) TXS partial promoter. (C) DBTNBT promoter region before the TSS, fragment 1. (D) DBTNBT promoter region from the TSS to the initial region of the CDS, fragment 2. The three different cytosine contexts are indicated in red spheres (CG), blue squares (CHG) and green triangles (CHH), colored (methylated) or empty (not methylated). The transcription factors studied are AP2/ERF, MYC, WRKI, and LHY. The distance between the TSS and CDS is highlighted by a gray line.
First, we found that independently of the promoter studied, the vast majority of predicted TFBSs were clustering in the proximal zone of the CDS. Despite this, a differential presence of cis-acting regulatory elements through the studied promoters was found. The TXS and DBTNBT promoters presented the conserved motifs, CGTCA-motifs, G-boxes, and E-boxes, all related to responsiveness to MeJA. Discordantly, the GGPPS promoter did not show any of these cis elements in its promoter sequence, excepting a low affinity E-box (Supplementary Material 2).
In order to detect binding sites for specific transcription factors, we focused on AP2/ERF, MYC, LHY, and WRKY transcription factors binding sites (TFBSs). Regarding to MYC TFBSs, the GGPPS promoter region did not present any of them, but TXS and DBTNBT did. The GGPPS promoter showed the richest sequence in WRKY TFBSs, presenting the highest number of W-boxes (Figure 3A). MeJA responses had been also related to the overexpression of ethylene-responsive transcription factors such as the AP2/ERF, a cis-regulatory element that can be found through all the studied promoters.
The effect of the cytosine methylation points over the predicted TFBSs show relevant information when both lines, the new and the old, were compared. First, and due to the lack of methylated cytosines in the GGPPS promoter region, no TFBS were related to methylation accumulations. The methylation points in the TXS promoter of the new line did not seem to overlap any TFs involved in the MeJA response. However, the old line methylation pattern presented methylated cytosines in the same position as LHY and MYC TFBSs (Figure 3B). Interestingly, 3 of 5 MYC TFBSs overlap with methylation hotspots in the old line TXS promoter. WRKY and AP2/ERF TFs, on the other hand, were not showing any associated methylation accumulation.
In the case of the DBTNBT promoter region, most of the predicted TFBSs are located in a region of 40 nucleotides around the start of the CDS, matching with the distribution previously observed in the previous promoters. Nevertheless, the presence of the TFBSs in the DBTNBT region is clustering at a specific point of the sequence. The WRKY TFBSs were not associated with methylated cytosines in the new line, but these positions were methylated in the old line. The cis elements related to the ethylene response transcription factors (AP2/ERFs) were affected in the new and the old line. As expected, the MYC TFBSs in the DBTNBT promoter shown methylation accumulation in the old line. Clearly, the distribution of cis elements in response to MeJA and ethylene signaling along the three promoters studied was not homogeneous, also showing a differential accumulation of methylation cytosines through their sequences. In addition, and, surprisingly, the promoter region showing a higher methylation accumulation, the DBTNBT promoter, also showed a cluster of TFBSs overlapping with the above-mentioned region of the methylation hotspot.
Methylation patterns alterations in specialized metabolism genes have been demonstrated to lead to an important reduction in the yield of plant in vitro cell cultures over time, as the expression of these genes can be drastically reduced. The identification of promoters accumulating high ratios of methylation can be indicative of regulatory points of a biosynthetic pathway, as well as key transcription factor binding sites. In this study, with the aim to clarify the role of the methylation mechanisms in the previously demonstrate transcriptional alterations of taxane biosynthetic genes, we selected three genes of this pathway, one of them belonging to the primary metabolism, the GGPPS gene, and the other two, the first (TXS) and the last gene (DBTNBT) involved specifically in the paclitaxel biosynthetic pathway. The study, using two aged-different Taxus × media cell cultures, could lead us to demonstrate the correlation between the methylation increment in key biosynthetic genes and the gradual loss of taxane production during its long-term maintenance in optimal in vitro conditions.
The methylation study performed reveals differential methylation patterns between the three promoters, being the last gene of the pathway (DBTNBT) the most methylated. Moreover, the accumulation of methylation points was considerable in the old line. As it was demonstrated by Sanchez-Muñoz et al. (2018), the in vitro maintenance of T. x media cultures increases the cytosine methylation in BAPT gene, a key gene involved in taxane production. The optimal growing conditions could cause a lack of stressing stimulus; therefore, the cell would silence the taxane biosynthetic pathway.
The deep study of each promoter reveals that the GGPPS promoter is the least affected by methylation mechanisms. We demonstrated that GGPPS promoter presented low levels of methylated cytosines and, in addition, a scarce cytosine content in the core promoter (Figure 1A; Table 2). This region correspond with the presence of the putative Y-patch (Yamamoto et al., 2007a).The unusual cytosine content prevents methylation accumulation and, consequently, avoids gene silencing. Therefore, the GGPPS, a non-specific taxane biosynthetic gene, is expected to not be affected by methylation, since it is a common precursor of the primary plant metabolism, such as the gibberellin biosynthetic pathway. In addition, the T. x media cell culture in vitro maintenance along the time does not alter the methylation pattern of the GGPPS, allowing the plant development.
In contrast, the TXS gene is specifically involved in the taxane biosynthetic pathway. It is observed that it is more affected by methylation mechanisms, especially in the old line. In this case, the maintenance in in vitro conditions increases the methylation levels of our cell cultures, as previously demonstrated in the BAPT promoter (Sanchez-Muñoz et al., 2018). Moreover, the highest accumulation of methylated cytosines, ~60%, was located in the putative Y-patch region. This region has a characteristic sequence, TCTCTCTTC, located from position 303 to 311 (Figure 1B), corresponding to patterns associated with the presence of Y-patch Yamamoto et al. (2007b). The methylation of these regulatory patterns in the old line indicates that the TXS gene is regulated by Y-patch motifs and the methylation of these motifs in the old line demonstrate that the gene expression is affected by in vitro culture maintenance.
In addition to the methylation mechanisms and the Y-patch motifs, other factors influence the regulation of gene expression, as well as the cytosine patterns. The studied TXS promoter region does not present the CHG context, reducing the methylation context possibilities. Further studies could be focused on the effect of the lack of CHG context and its relationship with the methylation mechanisms.
Surprisingly, the DBTNBT promoter structure differs from the other two promoters studied. On the one hand, the TSS is located almost 7 times further upstream of the CDS than the other promoters. On the other hand, there is a notable presence of cytosines in the DBTNBT promoter (24%) compared to the GGPPS and TXS promoters (14 and 16%, respectively), thus increasing the putative methylation points and the possible presence of Y-patch. In relation to cytosines, the three contexts are present in this promoter. The CHH context was the least methylated on the new line and showed the most significant differences between the two lines. Hence, the CHH context, only methylated by the novo mechanism is known to be the most dynamic one (He et al., 2011). It explains its implication in the adaptative response of cell cultures in in vitro conditions. Consequently, it is not surprising that the new line has very low levels of CHH-methylation relative to the old one, showing that the culture time increases the level of de novo methylation in DBTNBT promoter in Taxus spp., cell cultures, and therefore modifying the pathway productivity.
Regarding the possible presence of Y-patch in the DBTNBT promoter, this was located after the TSS with a characteristic distribution of cytosines. The study of pyrimidine composition in DBTNBT promoter (Figure 2) revealed that Y accumulations before the CDS belonged to the presence of cytosine (79%) instead of thymine, as opposed to the GGPPS and TXS promoters (Table 2). This analysis justifies the presence of the Y-patch region in the DBTNBT and its susceptibility to being affected by methylation mechanisms. Interestingly, this region of the old line was completely methylated. These facts imply that the DBNTBT gene is highly regulated, and its expression is affected by the time the cells are exposed to in vitro conditions. Therefore, these results could be linked with the low presence of paclitaxel, almost undetectable, in the taxane profiling of the old cell line (Sanchez-Muñoz et al., 2018). The high quantity of cytosines and the high methylation ratio found in the DBTNBT promoter, together with the results of the study of the BAPT promoter by Sanchez-Muñoz et al., 2018, showed that the last steps of the taxane pathway are strongly regulated.
Particularly on the CG cytosine contexts, the three promoters studied showed some non-CG methylated, especially in the new line. In the studies reviewed by Kenchanmane Raju et al. (2019), it was reported that plant defense responses are enhanced in non-CG methylated mutants, highlighting the limiting role of DNA methylation in stress responses (Luna and Ton, 2012; Slaughter et al., 2012; Kenchanmane Raju et al., 2018). The GGPPS promoter did not present any methylated CG. The CGs along the TXS promoter were only methylated in the old line. Regarding the DBTNBT promoter in the region after the TSS, all CGs are methylated in the old line. This could be explained by the fact that in in vitro conditions, plant cells progressively reduce the need for cell defense responses, and concordantly there is a progressive methylation accumulation in specific points of promoter related to specialized metabolism. The region between the TSS and the CDS in the DBTNBT promoter seems to have a pivotal role in taxane production regulation.
To understand how the expression of the three studied genes is regulated, the location of transcription factors is also a key aspect. The presence of cis-acting regulatory elements through GGPPS, TXS, and DBTNBT promoters was predicted in relation with MeJA and ethylene responses. Previous studies of taxane gene expression demonstrated that MeJA increases the taxane production (Cusido et al., 2014). The TXS and DBTNBT promoters present elements related to the ability to respond to MeJA, such as the CCGTCA motifs, G-box, and E-boxes, demonstrating a relevant putative regulatory role in taxane biosynthesis. The study of these cis elements showed that the distance between the TSS and the CDS in the DBTNBT promoter is longer than the other taxane biosynthetic genes studied (Figure 2C). As mentioned by Yamamoto et al. (2011), the core type affects the gene structure as well as the expression profile; the longer distance between TSS and CDS, the lower the expression ratio of the gene. This finding is also supported by the fact that the DBTNBT promoter is a TATA-less promoter and is differently regulated than the BAPT gene, a TATA-type promoter that shows a different promoter structure. The TATA-type promoter has a shorter distance between the TSS and the CDS, while in TATA-less types, this distance can be extended (Yamamoto et al., 2011). Considering that BAPT and DBTNBT are the last steps of the taxane biosynthetic pathway, it is expected that their expression would be strictly controlled.
As mentioned above, specialized metabolism is closely related to stress and defense responses, of which the best characterized are those related to MeJA and ethylene signals. Both signals have been demonstrated to influence taxane biosynthesis (Cusido et al., 2014). And the action of specific transcription factors such as MYC (Lenka et al., 2015; Cui et al., 2019), AP2/ERF (Zhang et al., 2021), LHY and WRKY (Zhou et al., 2019) has been shown to be responsible for the effects in taxane production. According to our results, the GGPPS promoter has the highest amount of WRKY TFBSs located along the studied region (Figure 3A). The WRKY TFs, aside from being related to the MeJA response, have also been demonstrated to be a gibberellic acid (GA3) repressor. This correlates with the crosslink between GGPP, GA3 and taxanes synthesis. The WRKY TFs might be an on–off switch control to regulate the primary and specialized metabolism, hence avoiding the competition between the two metabolisms for GGPP. Regarding the TXS and the DBTNBT promoter, the distribution of the cis-acting regulatory elements shows TFBSs with a putative altered accessibility due to the methylation of the cytosines in the old cell line (Figure 3). Contrary, in the new line, the action of TFs should not be affected by methylation in the TXS promoter (Figure 3B). It should be pointed out the interesting results obtained in the DBTNBT promoter in the old line, where almost all MYC TFBSs predicted are accumulated between the TSS and the CDS, being all of them affected by methylation accumulation (Figure 3D). This evidence is in the line with Niederhuth and Schmitz (2017), where their study in A. thaliana showed that the majority (72%) of TFs binding is inhibited by methylation. According to these results, altogether with the transcriptomic and production alterations showed in previous study (Sanchez-Muñoz et al., 2018), it can be concluded that the production of taxanes could be epigenetically modulated by the methylation of these TFBSs, especially in the last steps of the pathway.
After performing an exhaustive study through the promoters, new insights about taxane biosynthesis regulation regarding epigenetic mechanisms have been provided. First, the GGPPS promoter has the region from the TSS to the CDS protected against methylation accumulation, since its cytosine distribution may prevent gene silencing caused by 5’mC methylation. The TXS promoter does not present the CHG context in the region analyzed. Consequently, only two of the three cysteine contexts could be methylated, offering the possibility of a slightly regulation in this first step of the pathway. Finally, the DBTNBT, as the last gene of the taxane pathway, seems to be strongly regulated. This seems to be achieved by two independent mechanisms. On the one hand, by the distance between the TSS and the CDS that the structure of the promoter is showing and, on the other hand, by the high rate of cytosines presented in this region, making a hotspot of cytosines that can be potentially methylated. In addition, the distribution of cis elements showed that the region between the TSS and the CDS is a key transcriptional regulator in this gene. These results, provide strong evidences to confirm that the last steps of taxane biosynthesis are key for the regulation of paclitaxel production. Moreover, it proves that epigenetic mechanisms are directly involved in silencing these genes, being our results for the last gene of the pathway perfectly correlated with the results in the BAPT promoter shown by this author. Last but not least, our study provides convincing evidence that the long-term effects of the in vitro conditions differentially affect the gene regulation of plant primary and specialized metabolism.
In conclusion, epigenetic methylation has shown to be an essential player in the expression of the last steps of taxane biosynthesis, mainly due to its close relationship with their promoter structure, particularly evident in the DBTNBT promoter. In this study, it has been demonstrated that epigenetic control depends not only on the gene and its function but also on the in vitro culture maintenance time. Besides, it is shown that methylation accumulation is a specific and directed mechanism rather than a general methylation accumulation through the entire genome. Furthermore, the transcription factors and cis elements are strategically located along the promoter over regions easily methylated by the de novo mechanism. All this evidence is not only providing new knowledge about the specific mechanism under the epigenetic regulation of specialized metabolites but also novel insights that will ease the design of strategies for the rational improvement of paclitaxel production, such as the modification of DBTNBT or BAPT promotor sequences by genome editing technologies as CRISPR.
The original contributions presented in the study are included in the article/Supplementary Material, further inquiries can be directed to the corresponding authors.
JP, RS-M, and EM conceived the project and designed the research plan. AE performed the bisulfite method and computational work. RC and MB supervised the DNA methylation analysis. RS-M and EM supervised the computational work. AE, RS-M, and EM wrote the manuscript. MB, RC, and JP supervised and complemented the writing. All authors contributed to the article and approved the submitted version.
This work has been carried out at the Plant Physiology Laboratory (Universitat de Barcelona). It was financially supported by the Spanish PID2020-113438RB-I00/AEI/10.13039/501100011033 and the Generalitat de Catalunya 2017SGR242. AE was supported by a fellowship from the Universitat Pompeu Fabra.
The authors declare that the research was conducted in the absence of any commercial or financial relationships that could be construed as a potential conflict of interest.
All claims expressed in this article are solely those of the authors and do not necessarily represent those of their affiliated organizations, or those of the publisher, the editors and the reviewers. Any product that may be evaluated in this article, or claim that may be made by its manufacturer, is not guaranteed or endorsed by the publisher.
The Supplementary Material for this article can be found online at: https://www.frontiersin.org/articles/10.3389/fpls.2022.899444/full#supplementary-material
Bachar, S. C., Bachar, R., Jannat, K., Jahan, R., and Rahmatullah, M. (2020). Hepatoprotective natural products. Annu. Rep. Med. Chem. 55, 207–249. doi: 10.1016/bs.armc.2020.06.003
Beum, J. K., Gibson, D. M., and Shuler, M. L. (2004). Effect of subculture and elicitation on instability of Taxol production in Taxus sp. suspension cultures. Biotechnol. Prog. 20, 1666–1673. doi: 10.1021/bp034274c
Changxing, L., Galani, S., Hassan, F., Rashid, Z., Naveed, M., Fang, D., et al. (2020). Biotechnological approaches to the production of plant-derived promising anticancer agents: An update and overview. Biomed. Pharmacother. 132:110918. doi: 10.1016/j.biopha.2020.110918
Chow, C. N., Lee, T. Y., Hung, Y. C., Li, G. Z., Tseng, K. C., Liu, Y. H., et al. (2019). Plantpan3.0: A new and updated resource for reconstructing transcriptional regulatory networks from chip-seq experiments in plants. Nucleic Acids Res. 47, D1155–D1163. doi: 10.1093/nar/gky1081
Cokus, S. J., Feng, S., Zhang, X., Chen, Z., Merriman, B., Haudenschild, C. D., et al. (2008). Shotgun bisulphite sequencing of the Arabidopsis genome reveals DNA methylation patterning. Nature 452, 215–219. doi: 10.1038/nature06745
Croteau, R., Ketchum, R. E. B., Long, R. M., Kaspera, R., and Wildung, M. R. (2006). Taxol biosynthesis and molecular genetics. Phytochem. Rev. 5, 75–97. doi: 10.1007/s11101-005-3748-2
Cui, Y., Mao, R., Chen, J., and Guo, Z. (2019). Regulation mechanism of MYC family transcription factors in jasmonic acid signalling pathway on taxol biosynthesis. Int. J. Mol. Sci. 20:1843. doi: 10.3390/ijms20081843
Cusido, R. M., Onrubia, M., Sabater-Jara, A. B., Moyano, E., Bonfill, M., Goossens, A., et al. (2014). A rational approach to improving the biotechnological production of taxanes in plant cell cultures of Taxus spp. Biotechnol. Adv. 32, 1157–1167. doi: 10.1016/j.biotechadv.2014.03.002
Dellaporta, S. L., Wood, J., and Hicks, J. B. (1983). A plant DNA minipreparation: version II. Plant Mol. Biol. Rep. 1, 19–21. doi: 10.1007/BF02712670
Deus-Neumann, B., and Zenk, M. (1984). Instability of Indole alkaloid production in Catharanthus roseus cell suspension cultures. Planta Med. 50, 427–431. doi: 10.1055/s-2007-969755
Dougall, D. K., Johnson, J. M., and Whitten, G. H. (1980). A clonal analysis of anthocyanin accumulation by cell cultures of wild carrot. Planta 149, 292–297. doi: 10.1007/BF00384569
Escrich, A., Almagro, L., Moyano, E., Cusido, R. M., Bonfill, M., Hosseini, B., et al. (2021). Improved biotechnological production of paclitaxel in Taxus media cell cultures by the combined action of coronatine and calix[8]arenes. Plant Physiol. Biochem. 163, 68–75. doi: 10.1016/j.plaphy.2021.03.047
Expósito, O., Bonfill, M., Onrubia, M., Jané, A., Moyano, E., Cusidó, R. M., et al. (2009). Effect of taxol feeding on taxol and related taxane production in Taxus baccata suspension cultures. N. Biotechnol. 25, 252–259. doi: 10.1016/j.nbt.2008.11.001
Finnegan, E. J., Peacock, W. J., and Dennis, E. S. (1996). Reduced DNA methylation in Arabidopsis thaliana results in abnormal plant development. Proc. Natl. Acad. Sci. U. S. A. 93, 8449–8454. doi: 10.1073/pnas.93.16.8449
Gamborg, O. L., Miller, R. A., and Ojima, K. (1968). Nutrient requirements of suspension cultures of soybean root cells. Exp. Cell Res. 50, 151–158. doi: 10.1016/0014-4827(68)90403-5
Georgiev, M. I., Weber, J., and MacIuk, A. (2009). Bioprocessing of plant cell cultures for mass production of targeted compounds. Appl. Microbiol. Biotechnol. 83, 809–823. doi: 10.1007/s00253-009-2049-x
Ghosh, A., Igamberdiev, A. U., and Debnath, S. C. (2021). Tissue culture-induced DNA methylation in crop plants: a review. Mol. Biol. Rep. 48, 823–841. doi: 10.1007/s11033-020-06062-6
He, X. J., Chen, T., and Zhu, J. K. (2011). Regulation and function of DNA methylation in plants and animals. Cell Res. 21, 442–465. doi: 10.1038/cr.2011.23
Hetzl, J., Foerster, A. M., Raidl, G., and Scheid, O. M. (2007). CyMATE: A new tool for methylation analysis of plant genomic DNA after bisulphite sequencing. Plant J. 51, 526–536. doi: 10.1111/j.1365-313X.2007.03152.x
Kaeppler, S. M., and Phillips, R. L. (1993). Tissue culture-induced DNA methylation variation in maize. Proc. Natl. Acad. Sci. U. S. A. 90, 8773–8776. doi: 10.1073/pnas.90.19.8773
Karim, R., Nuruzzaman, M., Khalid, N., and Harikrishna, J. A. (2016). Importance of DNA and histone methylation in in vitro plant propagation for crop improvement: a review. Ann. Appl. Biol. 169, 1–16. doi: 10.1111/aab.12280
Kenchanmane Raju, S. K., Ritter, E. J., and Niederhuth, C. E. (2019). Establishment, maintenance, and biological roles of non-CG methylation in plants. Essays Biochem. 63, 743–755. doi: 10.1042/EBC20190032
Kenchanmane Raju, S. K., Shao, M. R., Wamboldt, Y., and Mackenzie, S. (2018). Epigenomic plasticity of Arabidopsis msh1 mutants under prolonged cold stress. Plant Direct 2:e00079. doi: 10.1002/pld3.79
Law, J. A., and Jacobsen, S. E. (2010). Establishing, maintaining and modifying DNA methylation patterns in plants and animals. Nat. Rev. Genet. 11, 204–220. doi: 10.1038/nrg2719
Lenka, S. K., Ezekiel Nims, N., Vongpaseuth, K., Boshar, R. A., Roberts, S. C., and Walker, E. L. (2015). Jasmonate-responsive expression of paclitaxel biosynthesis genes in Taxus cuspidata cultured cells is negatively regulated by the bHLH transcription factors TcJAMYC1, TcJAMYC2, and TcJAMYC4. Front. Plant Sci. 6:115. doi: 10.3389/fpls.2015.00115
Lescot, M., Déhais, P., Thijs, G., Marchal, K., Moreau, Y., Van De Peer, Y., et al. (2002). PlantCARE, a database of plant cis-acting regulatory elements and a portal to tools for in silico analysis of promoter sequences. Nucleic Acids Res. 30, 325–327. doi: 10.1093/nar/30.1.325
Li, W., Cowley, A., Uludag, M., Gur, T., McWilliam, H., Squizzato, S., et al. (2015). The EMBL-EBI bioinformatics web and programmatic tools framework. Nucleic Acids Res. 43, W580–W584. doi: 10.1093/nar/gkv279
Luna, E., and Ton, J. (2012). The epigenetic machinery controlling transgenerational systemic acquired resistance. Plant Signal. Behav. 7, 615–618. doi: 10.4161/psb.20155
Medina-Rivera, A., Defrance, M., Sand, O., Herrmann, C., Castro-Mondragon, J. A., Delerce, J., et al. (2015). RSAT 2015: regulatory sequence analysis tools. Nucleic Acids Res. 43, W50–W56. doi: 10.1093/nar/gkv362
Mtewa, A. G., Egbuna, C., Beressa, T. B., Ngwira, K. J., and Lampiao, F. (2021). “Phytopharmaceuticals: efficacy, safety, and regulation,” in Preparation of Phytopharmaceuticals for the Management of Disorders. eds. C. Chukwuebuka Egbuna, A. P. Mishra, and M. R. Goyal (Cambridge: Academic Press), 25–38.
Mutanda, I., Li, J., Xu, F., and Wang, Y. (2021). Recent advances in metabolic engineering, protein engineering, and Transcriptome-guided insights Toward synthetic production of Taxol. Front. Bioeng. Biotechnol. 9:34. doi: 10.3389/fbioe.2021.632269
Niederhuth, C. E., and Schmitz, R. J. (2017). Putting DNA methylation in context: from genomes to gene expression in plants. Biochim. Biophys. Acta Gene Regul. Mech. 1860, 149–156. doi: 10.1016/J.BBAGRM.2016.08.009
Nowak, R. (1994). Mining treasures from junk DNA. Science 263, 608–610. doi: 10.1126/science.7508142
Okonechnikov, K., Golosova, O., Fursov, M., Varlamov, A., Vaskin, Y., Efremov, I., et al. (2012). Unipro UGENE: A unified bioinformatics toolkit. Bioinformatics 28, 1166–1167. doi: 10.1093/bioinformatics/bts091
Onrubia, M., Cusido, R. M., Ramirez, K., Hernandez-Vazquez, L., Moyano, E., Bonfill, M., et al. (2013). Bioprocessing of plant in vitro systems for the mass production of pharmaceutically important metabolites: paclitaxel and its derivatives. Curr. Med. Chem. 20, 880–891. doi: 10.2174/0929867311320070004
Parrish, R. R., Day, J. J., and Lubin, F. D. (2012). Direct bisulfite sequencing for examination of DNA methylation with gene and nucleotide resolution from brain tissues. Curr. Protoc. Neurosci. 60:7.24.1-7.24.12. doi: 10.1002/0471142301.ns0724s60
Peremarti, A., Twyman, R. M., Gómez-Galera, S., Naqvi, S., Farré, G., Sabalza, M., et al. (2010). Promoter diversity in multigene transformation. Plant Mol. Biol. 73, 363–378. doi: 10.1007/s11103-010-9628-1
Porto, M. S., Pinheiro, M. P. N., Batista, V. G. L., Dos Santos, R. C., De Albuquerque Melo Filho, P., and De Lima, L. M. (2014). Plant promoters: An approach of structure and function. Mol. Biotechnol. 56, 38–49. doi: 10.1007/s12033-013-9713-1
Sanchez-Muñoz, R., Bonfill, M., Cusidó, R. M., Palazón, J., and Moyano, E. (2018). Advances in the regulation of In vitro paclitaxel production: methylation of a Y-patch promoter region alters BAPT gene expression in Taxus cell cultures. Plant Cell Physiol. 59, 2255–2267. doi: 10.1093/pcp/pcy149
Sanchez-Muñoz, R., Moyano, E., Khojasteh, A., Bonfill, M., Cusido, R. M., and Palazon, J. (2019). Genomic methylation in plant cell cultures: a barrier to the development of commercial long-term biofactories. Eng. Life Sci. 19, 872–879. doi: 10.1002/elsc.201900024
Slaughter, A., Daniel, X., Flors, V., Luna, E., Hohn, B., and Mauch-Mani, B. (2012). Descendants of primed Arabidopsis plants exhibit resistance to biotic stress. Plant Physiol. 158, 835–843. doi: 10.1104/pp.111.191593
Solovyev, V. V., Shahmuradov, I. A., and Salamov, A. A. (2010). Identification of promoter regions and regulatory sites. Methods Mol. Biol. 674, 57–83. doi: 10.1007/978-1-60761-854-6_5
Springer, N. M., and Schmitz, R. J. (2017). Exploiting induced and natural epigenetic variation for crop improvement. Nat. Rev. Genet. 18, 563–575. doi: 10.1038/nrg.2017.45
Stelpflug, S. C., Eichten, S. R., Hermanson, P. J., Springer, N. M., and Kaeppler, S. M. (2014). Consistent and heritable alterations of DNA methylation are induced by tissue culture in maize. Genetics 198, 209–218. doi: 10.1534/genetics.114.165480
Tyunin, A. P., Ageenko, N. V., and Kiselev, K. V. (2016). Effects of 5-azacytidine-induced DNA demethylation on polyketide synthase gene expression in larvae of sea urchin Strongylocentrotus intermedius. Biotechnol. Lett. 38, 2035–2041. doi: 10.1007/s10529-016-2191-3
Vidal-Limon, H., Sanchez-Muñoz, R., Khojasteh, A., Moyano, E., Cusido, R. M., and Palazon, J. (2018). “Taxus cell cultures: an effective biotechnological tool to enhance and gain new biosynthetic insights into taxane production,” in Bioprocessing of Plant in vitro Systems. Reference Series in Phytochemistry. eds. A. Pavlov and T. Bley (Cham: Springer), 295–316.
Yamamoto, Y., Ichida, H., Abe, T., Suzuki, Y., Sugano, S., and Obokata, J. (2007a). Differentiation of core promoter architecture between plants and mammals revealed by LDSS analysis. Nucleic Acids Res. 35, 6219–6226. doi: 10.1093/nar/gkm685
Yamamoto, Y. Y., Ichida, H., Matsui, M., Obokata, J., Sakurai, T., Satou, M., et al. (2007b). Identification of plant promoter constituents by analysis of local distribution of short sequences. BMC Genomics 8, 1–23. doi: 10.1186/1471-2164-8-67/FIGURES/9
Yamamoto, Y. Y., Yoshioka, Y., Hyakumachi, M., and Obokata, J. (2011). Characteristics of core promoter types with respect to gene structure and expression in arabidopsis thaliana. DNA Res. 18, 333–342. doi: 10.1093/dnares/dsr020
Yanfang, Y., Kaikai, Z., Liying, Y., Xing, L., Ying, W., Hongwei, L., et al. (2018). Identification and characterization of MYC transcription factors in Taxus sp. Gene 675, 1–8. doi: 10.1016/j.gene.2018.06.065
Zhang, K., Jiang, L., Wang, X., Han, H., Chen, D., Qiu, D., et al. (2021). Transcriptome-wide analysis of AP2/ERF transcription factors involved in regulating Taxol biosynthesis in Taxus × media. Ind. Crop Prod. 171:113972. doi: 10.1016/J.INDCROP.2021.113972
Zhang, H., Lang, Z., and Zhu, J. K. (2018). Dynamics and function of DNA methylation in plants. Nat. Rev. Mol. Cell Biol. 19, 489–506. doi: 10.1038/s41580-018-0016-z
Zhou, T., Luo, X., Yu, C., Zhang, C., Zhang, L., Song, Y., et al. (2019). Transcriptome analyses provide insights into the expression pattern and sequence similarity of several taxol biosynthesis-related genes in three Taxus species. BMC Plant Biol. 19:33. doi: 10.1186/s12870-019-1645-x
Keywords: DNA methylation, taxane biosynthesis, epigenetic regulation, Taxol, cis-elements, promotors, paclitaxel
Citation: Escrich A, Cusido RM, Bonfill M, Palazon J, Sanchez-Muñoz R and Moyano E (2022) The Epigenetic Regulation in Plant Specialized Metabolism: DNA Methylation Limits Paclitaxel in vitro Biotechnological Production. Front. Plant Sci. 13:899444. doi: 10.3389/fpls.2022.899444
Received: 18 March 2022; Accepted: 20 June 2022;
Published: 08 July 2022.
Edited by:
Rogelio Santiago, University of Vigo, SpainReviewed by:
Vsevolod Jurievich Makeev, Vavilov Institute of General Genetics (RAS), RussiaCopyright © 2022 Escrich, Cusido, Bonfill, Palazon, Sanchez-Muñoz and Moyano. This is an open-access article distributed under the terms of the Creative Commons Attribution License (CC BY). The use, distribution or reproduction in other forums is permitted, provided the original author(s) and the copyright owner(s) are credited and that the original publication in this journal is cited, in accordance with accepted academic practice. No use, distribution or reproduction is permitted which does not comply with these terms.
*Correspondence: Raul Sanchez-Muñoz, cmF1bC5zYW5jaGV6bXVub3pAdWdlbnQuYmU=; Elisabeth Moyano, ZWxpc2FiZXRoLm1veWFub0B1cGYuZWR1
†These authors have contributed equally to this work
Disclaimer: All claims expressed in this article are solely those of the authors and do not necessarily represent those of their affiliated organizations, or those of the publisher, the editors and the reviewers. Any product that may be evaluated in this article or claim that may be made by its manufacturer is not guaranteed or endorsed by the publisher.
Research integrity at Frontiers
Learn more about the work of our research integrity team to safeguard the quality of each article we publish.