- Laboratory of Plant Breeding, Research Faculty of Agriculture, Hokkaido University, Sapporo, Japan
Hybrid sterility genes define species identities, setting reproductive barriers between distantly related Oryza relatives. They induce allelic-specific selective gametic abnormalities by killing pollens, embryo sacs, or both, and thus resulting in the male specific transmission ratio distortion (mTRD), female specific transmission ratio distortion (f TRD), and/or sex-independent transmission ratio distortion (siTRD) in hybrids. Although more than 50 hybrid sterility genes have been reported, comprehensive analysis on the distributional pattern of TRD systems in Oryza species is limited. In this review, we surveyed the TRD systems and the underlying possible mechanisms in these species. In rice, pollen killers which cause mTRD are often observed in higher frequency than egg killers and gamete eliminators, which are factors affecting f TRD and siTRD, respectively. Due to the rather massive population of pollen grains, their reduction in the number caused by hybrid sterility possesses a smaller selective disadvantage to the hybrid individuals, in contrast to female gamete abortion. The pattern of TRD distribution displays less abundancy in siTRD. It suggests that fixation of siTRD might require a certain time rather than single sex-specific factors. The presence of linked sterility factors worked for mTRD and f TRD, and strength of their linkage in chromosomal regions might determine the type of sterility and TRD. The study of TRD systems has a potential to reveal the relationships between selfish genes and their functions for reproductive isolation.
Introduction
Based on interbreeding, the biological species concept stated, “Species are groups of interbreeding natural populations that are reproductively isolated from other such groups.” (Mayr, 1996; Coyne and Orr, 2004). It literally means that the boundaries between the population of one species and that of another are defined by reproductive barriers, and the mechanism itself is referred to as “reproductive isolation”, in which genetically based intrinsic barriers prevent gene flow between populations of different species (Nosil, 2013). Reproductive isolation maintains species identity, and evolutionary biologists are highly interested in this area of study. Reproductive isolation is considered to be incidentally acquired as a by-product of other divergences between species' populations (Nosil, 2013). The genetic variations gradually increase over time, and the accumulation of those changes and divergences is probably either neutral or wholesome to its own genetic background while it works as a deleterious factor in other alternative genetic backgrounds, exclusively in a heterozygous state (Turelli, 1998). It is possible for hybrid dysfunction to appear due to a divergence in multiple genomic regions across the whole genome in a wide variety of species populations. The Bateson-Dobzhansky-Muller (BDM) model is a model in which negatively epistatic interaction between divergent alleles contributes deleterious effects within hybrid populations (Bateson, 1909; Dobzhansky, 1937; Muller, 1942; Kubo et al., 2008; Nosil, 2013; Xie et al., 2019a) and is a widely accepted model of the emergence of reproductive barriers. However, are genes for reproductive isolation always neutral or wholesome when they spread in a population? By summarizing genes for hybrid sterility, a form of reproductive barrier, we will discuss how selfish genes act to build species barriers in rice.
Reproductive Barriers in Rice
In plants, reproductive barriers can be basically divided into two categories, namely prezygotic reproductive isolation and postzygotic reproductive isolation, based on the developmental stage in which they give rise (Koide et al., 2008b; Ouyang and Zhang, 2013; Zin Mar et al., 2021). While prezygotic isolation occurs during the formation of the zygote, the latter restricts the introgressive gene flow in crossed populations, inducing hybrid arrest after fertilization at different developmental stages and/or other advanced generations (Chen et al., 2016). Hybrid sterility, the gametic disorder at their reproductive stage with the failure to produce fertile male and/or female gametes in normally grown hybrid plants, is one form of postzygotic reproductive isolation. The genus Oryza, which consists of two cultivated rice species and 22 wild rice relatives (Ammiraju et al., 2010), is a valuable pool for improvement of agricultural traits. In rice genetics, interspecific and intraspecific hybrid sterility, which is one of the most pronounced forms of postzygotic reproductive isolation, has been the most extensively investigated subject across a wide variety of genomic regions in a substantial number of different rice populations (Ouyang and Zhang, 2013; Li et al., 2020).
The Genetic Models of Hybrid Sterility
Based upon either sporophytic or gametophytic function and the number of loci involved, altogether four separate genic models underly rice hybrid sterility: namely (1) One-locus sporo-gametophytic interaction, (2) Duplicate gametic lethals, (3) One-locus sporophytic interaction, and (4) Complementary sporophytic interaction (Oka, 1957; Sano et al., 1979; Koide et al., 2008b). Among them, one-locus sporo-gametophytic interaction fits with genetic mechanisms for the majority of sterility loci in rice (Oka, 1957; Xie et al., 2019a). Therefore, we will focus on single or tightly linked sterility loci in this review (see Table 1). Hybrid sterility loci, such as S1, S2, and S5, can perfectly explain how one-locus sporo-gametophytic model works in rice sterility (Sano et al., 1979; Chen et al., 2009; Yang et al., 2012, 2016; Xie et al., 2017; Koide et al., 2018; Zin Mar et al., 2021). As an example, for S2 locus, although S2g (allele derived from oryza glaberrima) and S2s (allele derived from O. sativa) are neutral in their respective backgrounds, the incompatible interaction between these two alleles occurred in heterozygous hybrids. As a result, neither male nor female gametes which carry S2g allele survive, causing preferential transmission of selfish allele S2s in later generations (Sano et al., 1979; Zin Mar et al., 2021). Such a preferential transmission of one of the two alleles is referred to as transmission ratio distortion (TRD). Selfish genes causing TRD take advantage over their alternative alleles, and preferentially promote their own distribution in populations, with the help of incompatibilities that occur in heterozygous hybrids.
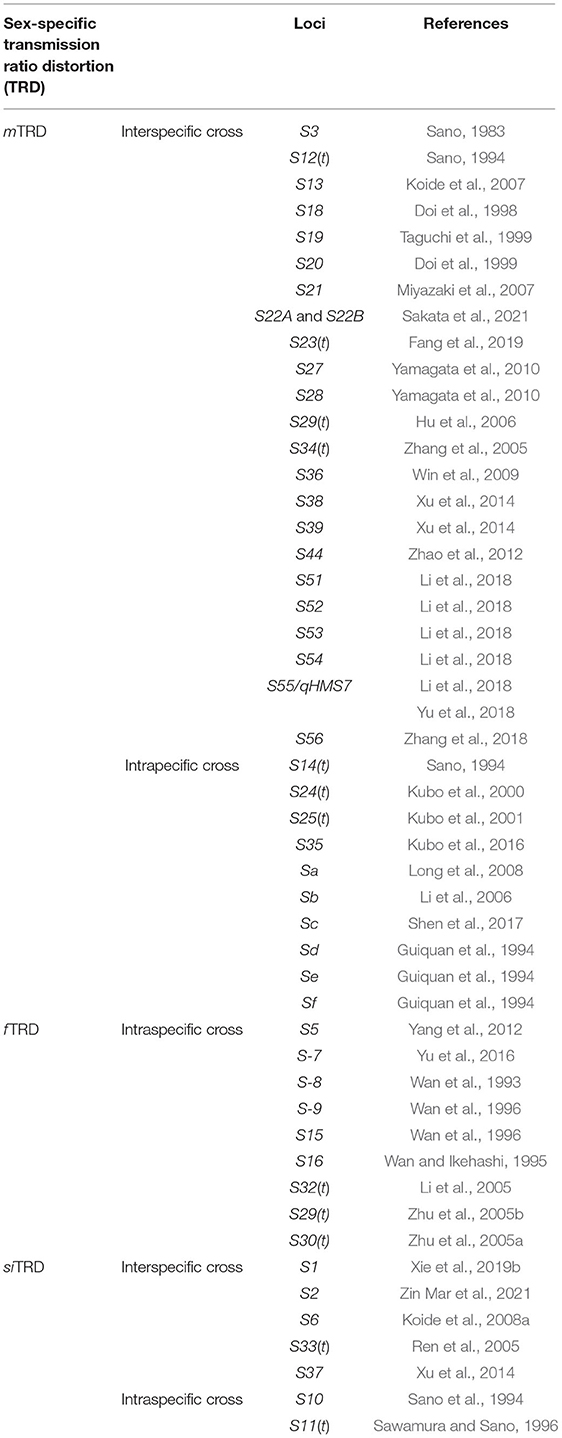
Table 1. Hybrid sterility loci in rice and their sex-specificity-related transmission ratio distortion.
Sex-Specificity of Trd in Hybrid Sterility in Rice
Although several underlying mechanisms behind the TRD phenomenon, including non-random segregation of chromosomes during meiosis (Pardo-Manuel de Villena and Sapienza, 2001; Birchler et al., 2003; Fishman and Willis, 2005; Koide et al., 2008a), unequal gametic success in fertilization (Price, 1997; Diaz and Macnair, 1999; Seymour et al., 2019), and embryo lethality (Lyttle, 1991; Silver, 1993; Price, 1997; Diaz and Macnair, 1999; Ubeda and Haig, 2005; Moyle, 2006) has been reported in plants, selective gametic abnormality (Lyttle, 1991; Silver, 1993; Ubeda and Haig, 2005; Moyle, 2006; Koide et al., 2008a) caused by hybrid sterility locus is the most frequently observed in Oryza species. The selective abnormality occurs in either male/female-gametes or sex-independently. In this report, the former and latter are termed mTRD (male specific transmission ratio distortion)/f TRD (female specific transmission ratio distortion) and siTRD (sex-independent transmission ratio distortion), respectively, (Maguire, 1963; Sano, 1983; Koide et al., 2008c; Ouyang and Zhang, 2013). Therefore, in general, hybrid sterility genes in rice can be divided into three subcategories: pollen killer (PK: which induces hybrid male sterility and mTRD), egg killer (EK: which results in hybrid female sterility and f TRD), and gamete eliminator (GE: which eliminates both pollen and embryo sac causing siTRD) (Figure 1).
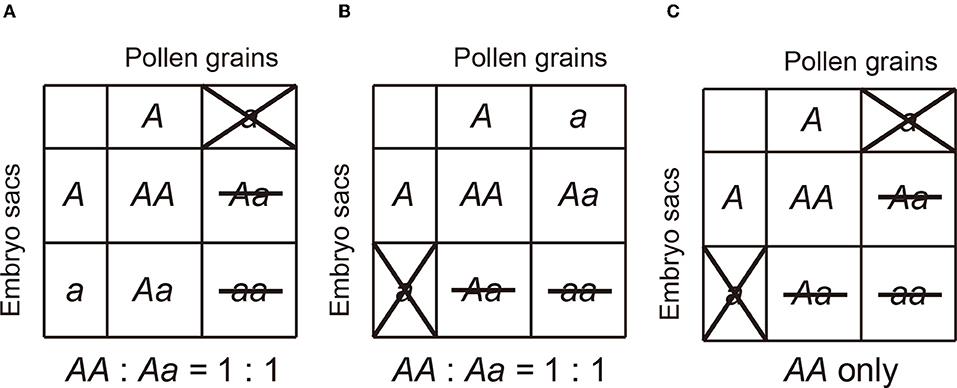
Figure 1. The genetic actions of three categories of hybrid sterility in rice. (A) Pollen killer, (B) Egg killer, (C) Gamete eliminator.
Several loci causing TRD have been cloned and their underlying mechanisms have been studied. The S5 locus is the most renowned hybrid sterility locus in the study of rice reproductive barriers. This locus is an example of “Killer-Protector system”, and its effect is shown in the failure of embryo sac fertility (We note that “Killer” in the Killer-Protector system and “Killer” in PK or EK have different actions. The former “Killer” induces the abortion of gametes with both alleles. The “Protector” selectively protects gametes with one of two alleles from the “Killer” function. As a result, the preferential dysfunction of gametes with one of two alleles occurs. The factor causing preferential function is referred to as PK, EK, or GE. Thus, PK and EK is a concept of a factor with the combined function of “Killer” and “Protector”.). Three different tightly linked genes (ORF3, ORF4, and ORF5) regulate the tripartite complex in indica-japonica crossed species. The indica-derived S5 allele (ORF3+, ORF4–, and ORF5+) contained both functionally active Protector and Killer genes, ORF3+ and ORF5+, while its allelic japonica-derived S5 region (ORF3–, ORF4+, and ORF5–) carries inactive Protector and Killer genes, ORF3– and ORF5–. However, the indica-derived Killer factor ORF5+ enacts its killing function with the assistance of its japonica-derived partner ORF4+ in the heterozygous combination. Meanwhile, ORF3+, which derives from the indica, selectively protects the embryo sac in which it resides. In the absence of stress responsive gene ORF3+, the unsolved endoplasmic reticulum (ER) stress induced by ORF5+ in partner with ORF4+, triggers programmed cell death (PCD), which results in embryo sac abortion (Yang et al., 2012).
The S1 locus is one of the major reproductive barriers between Asian and African rice species and a remarkable siTRD locus. The O. glaberrima allele (S1g) takes advantage over O. sativa allele (S1s) within populations, thus preferentially transmitting S1g and eliminating both male and female gametes when two alleles meet in a heterozygous state (S1s/S1g) (Koide et al., 2008c, 2018). According to Xie et al. (2017), CRISPR/Cas9-generated knockout mutants of OgTPR1 on S1g region (which encodes a protein with two trypsin-like peptidase domains and one ribosome biogenesis regulatory protein domain) produce normally fertile pollen and embryo sacs in crossing to O. sativa parent. The tripartite gamete Killer-Protector complex involving S1A4, S1TPR (OgTPR1), and S1A6 (SSP) of the S1g region, generates a sterility signal in sporophytic cells and protect itself with S1TPR in gametophytic cells, whereas its allelic S1s region lacks both functional killer and protector (Xie et al., 2019b).
The evolution of Killer-Protector system should be explained by models in which the deleterious effect of the Killer did not occur in a lineage (e.g., BDM model or parallel-sequential divergence model, Ouyang and Zhang, 2013), because without Protector, Killer induces abortion of gametes with both alleles highly reducing the chance of its fixation in a population. In the study of S5 sterility locus, the combination of non-functional Protector, and functional Killer and its partner (ORF3–, ORF4+, and ORF5+) was not found in the surveyed population (Yang et al., 2012). It may suggest that the combination that has Killer alone, without having functional Protector, cannot survive long in a population and also suggest that Killer can exist with functional Protector. However, after the emergence of Killer-Protector system, a single hybrid sterility locus with two tightly linked genes produces incompatibility when it meets the divergent allele in a different population. Then, the selfish nature of the system (i.e., TRD) caused by Killer-Protector system may facilitate its spread in a population.
Discussion
Evolution of Sex-Specificity in TRD in Rice
Approximately 50 hybrid sterility loci were identified in diverged species from the genus Oryza, and so far, cloning and characterization at a molecular level were already implemented for 10 loci out of those sterility loci or pairs (Ouyang and Zhang, 2013; Li et al., 2020). Among 49 hybrid sterility loci surveyed in this review, the number of loci for mTRD is the largest, in contrast to that of fTRD and siTRD (Table 1). In addition, the sterility based on gamete specificity disproportionately distributes between interspecific and intraspecific hybrids (Table 1). The distribution of mTRD loci is much wider in interspecific hybrids. On the other hand, all the f TRD loci are detected exclusively in intraspecific crosses. With regards to siTRD, it takes <15% of the total number of hybrid sterility loci, and it is very few in proportions compared to the other two sterility factors, mTRD and f TRD. Such a bias in frequency of sex-specificity in TRD system observed in Oryza might reflect different evolutionary pressures acting on the system, suggesting that TRD systems are not driven only by mutation and genetic drift. Therefore, uncovering the mechanisms underlying the observed pattern of TRD systems may be a key to understanding the evolution of reproductive isolation in Oryza. Although there is no direct evidence, several conjectures are possible to explain these patterns.
The Abundance of mTRD
The simple difference between male and female gametes is the quantity of them produced by a single plant. In a single flower of rice, thousands of pollen grains are produced, while only one egg is produced. Such a difference in the quantity of gametes can cause differences in selection pressure acting on factors which induce the abortion of them. One can easily imagine that the reduction of pollen numbers causes less effect on fecundity than that of egg numbers. The PK, which is one factor causing mTRD, induces abortion of only pollen grains of a specific genotype. Therefore, mTRD caused by a PK can spread in a population with a small selective disadvantage. In contrast, female-specific TRD (f TRD) and sex-independent TRD (siTRD) induced by EK and GE, respectively, cause abortion of eggs. Thus, f TRD and siTRD offers a selective disadvantage on the individual. The abundance of male sterility in hybrids is also found in Drosophila (Presgraves and Meiklejohn, 2021). A much smaller selective disadvantage of male sterility genes might help them exist within diverse populations at a higher pace of frequency. However, we also note that other experimental factors might be able to explain the abundance of mTRD reported: pollen sterility is easier to detect/analyze than egg sterility because a large number of pollen grains are available for the assay.
Uncommonly Observed Sex-Independent TRD
Among the three types of TRD systems, siTRD is less frequently observed (Table 1). In addition, most of them are observed in interspecific cross combinations. These observations suggest that hybrid sterility genes causing both male and female sterility occur less frequently and more time is necessary for fixation than ones causing single sex-specific sterility.
Although the S1 locus for siTRD has been cloned, molecular mechanisms causing sex-independent sterility in pollen grains and eggs are unknown. Because pollen grains and eggs are developed in physically separated tissues (i.e., anthers and ovaries), it is difficult to imagine that abortion of gametes in one sex causes gametic abortion of another sex. The sex-independent abortion of gametes might be caused by disturbance of the biological/developmental process common in two sexes. Therefore, the rareness of siTRD might reflect less abundancy of the biological/developmental process common in two sexes than in one sex.
Another possible mechanism for the emergence of siTRD is a combination of factors for mTRD and f TRD. If the two genes, each of which causes mTRD and fTRD, respectively, are located in tightly linked regions on a chromosome, the region is expected to behave like a factor for siTRD. Because of the limited number of cloned loci for siTRD, it is still unknown how often such a “pseudo-siTRD” occurrs. In the case of the S1 locus, which causes siTRD in inter-specific hybrids, Koide et al. (2008c) reported the change of sex-specificity of TRD depending on the length of introgressed chromosomal segments. The line with a long introgressed segment on chromosome 6 from O. glaberrima in the genetic background of O. sativa causes both pollen and embryo sac abortion when crossed with O. sativa. As a result, siTRD is observed in the next generation of hybrids. In contrast, when the line with a short introgressed segment from O. glaberrima was crossed with O. sativa, only pollen abortion and mTRD were observed. These results suggested the presence of two linked factors responsible for mTRD and f TRD in the region (We note that no other research groups have reported the change of sex-specificity of TRD induced by the S1 locus.). Another locus, the S6 for siTRD, has been suggested to be a compound locus of f TRD and pollen competition (Koide et al., 2012). In the siTRD induced by the S6 locus, preferential abortion was observed in ovules, but not in pollen grains, suggesting that mTRD was caused by competition of pollens with different genotypes. The degree of TRD was altered only for male gametes when genetic background was changed. These results suggested the presence of two different genes for mTRD and f TRD in a closely linked region, though no direct evidence of these two factors were reported. As we described above, the gene for mTRD may be easier to evolve than that for f TRD, because of its small selective disadvantage on fecundity. Therefore, if the siTRD system originated via tight linkage between mTRD and f TRD, the rate of its emergence is dependent on how often f TRD evolved in the chromosomal region closely linked to the mTRD. It should also depend on the strength of recombination between these two factors in the chromosomal region.
Although the common genetic basis of siTRD (i.e., one factor or two factors) is still unknown, the evolution of siTRD is dependent on the balance between transmission advantage through pollen and disadvantage of female gamete abortion. Therefore, population size and outcrossing rate also affect the evolutionary process of siTRD. Uncovering the molecular basis and evolutionary trajectories of siTRD system will provide clearer insight into how selfish elements relate to the development of species' barriers as theorized by Frank (1991).
Conclusions
In Oryza genus, reproductive isolation is excessively influenced by PK rather than EK and GE, which results in preferential occurrence of mTRD in contrast to the other two types, f TRD and siTRD. Compared to single sex-specificity, factors controlling siTRD are less frequently observed, mostly in interspecific hybridizations. Unveiling the underlying cause(s) behind this disproportionate pattern of TRD systems will shed light on the evolutionary process of reproductive barriers between rice relatives. Since our understanding on TRD systems remains very limited with confined experimental factors, further efforts are required to extend our investigation on many other selfish genes that exist and their distribution in Oryza genus.
Author Contributions
Zin Mar Myint and YK contributed to conception, analyzed, and wrote the manuscript. All authors contributed to manuscript revision, read, and approved the submitted version.
Funding
YK was funded by JSPS KAKENHI Grant Number 21H02160.
Conflict of Interest
The authors declare that the research was conducted in the absence of any commercial or financial relationships that could be construed as a potential conflict of interest.
Publisher's Note
All claims expressed in this article are solely those of the authors and do not necessarily represent those of their affiliated organizations, or those of the publisher, the editors and the reviewers. Any product that may be evaluated in this article, or claim that may be made by its manufacturer, is not guaranteed or endorsed by the publisher.
Acknowledgments
We thank Prof. Y. Kishima for his support for the project.
References
Ammiraju, J. S. S., Song, X., Luo, M., Sisneros, N., Angelova, A., Kudrna, D., et al. (2010). The Oryza BAC resource: a genus-wide and genome scale tool for exploring rice genome evolution and leveraging useful genetic diversity from wild relatives. Breed. Sci. 60, 536–543. doi: 10.1270/jsbbs.60.536
Bateson, W. (1909). “Heredity and variation in modern lights,” in Darwin and Modern Science, ed A. C. Seward (Cambridge: Cambridge University Press), 85–101.
Birchler, J. A., Dawe, R. K., and Doebley, J. F. (2003). Marcus Rhoades, preferential segregation and meiotic drive. Genetics 164, 835–841. doi: 10.1093/genetics/164.3.835
Chen, C., E, Z., and Lin, H.-X. (2016). Evolution and molecular control of hybrid incompatibility in plants. Front. Plant Sci. 7, 01208. doi: 10.3389/fpls.2016.01208
Chen, Z., Hu, F., Xu, P., Li, J., Deng, X., Zhou, J., et al. (2009). QTL analysis for hybrid sterility and plant height in interspecific populations derived from a wild rice relative, Oryza longistaminata. Breed. Sci. 59, 441–445. doi: 10.1270/jsbbs.59.441
Diaz, A., and Macnair, M. R. (1999). Pollen tube competition as a mechanism of prezygotic reproductive isolation between Mimulus nasutus and its presumed progenitor M. guttatus. New Phytol. 144, 471–478. doi: 10.1046/j.1469-8137.1999.00543.x
Dobzhansky, T. (1937). Genetic nature of species differences. Am. Nat. 71, 404–420. doi: 10.1086/280726
Doi, K., Taguchi, K., and Yoshimura, A. (1999). RFLP mapping of S20 and S21 for F1 pollen semi-sterility found in backcross progeny of Oryza sativa and O. glaberrima. Rice Genet. Newsl. 16, 65–68.
Doi, K., Tagushi, K., and Yoshimura, A. (1998). A new locus affecting high F1 pollen sterility found in backcross progenies of japonica rice and African rice. Rice Genet. Newsl. 15, 146–148.
Fang, C., Li, L., He, R., Wang, D., Wang, M., Hu, Q., et al. (2019). Identification of S23 causing both interspecific hybrid male sterility and environment-conditioned male sterility in rice. Rice 12, 10. doi: 10.1186/s12284-019-0271-4
Fishman, L., and Willis, J. H. (2005). A novel meiotic drive locus almost completely distorts segregation in Mimulus (Monkeyflower) hybrids. Genetics 169, 347–353. doi: 10.1534/genetics.104.032789
Frank, S. A. (1991). Divergence of meiotic drive-suppression systems as an explanation for sex-biased hybrid sterility and inviability. Evolution. 45, 262–267. doi: 10.1111/j.1558-5646.1991.tb04401.x
Guiquan, Z., Yonggen, L., Hua, Z., Jinchang, Y., and Guifu, L. (1994). Genetic studies on the hybrid sterility in culitivated rice (Oryza sativa) IV: genotypes for F1 pollen sterility. Acta Genet. Sin. 21, 34–41.
Hu, F., Xu, P., Deng, X., Zhou, J., Li, J., and Tao, D. (2006). Molecular mapping of a pollen killer gene S29(t) in Oryza glaberrima and colinear analysis with S22 in O. glumaepatula. Euphytica 151, 273–278. doi: 10.1007/s10681-006-9146-z
Koide, Y., Ikegaya, T., Nishimoto, D., Kanazawa, A., and Sano, Y. (2007). Hybrid male sterility induced by S13 found in a distantly related rice species. Genes Genet. Syst. 82, 522–522.
Koide, Y., Ikenaga, M., Sawamura, N., Nishimoto, D., Matsubara, K., Onishi, K., et al. (2008a). The evolution of sex-independent transmission ratio distortion involving multiple allelic interactions at a single locus in rice. Genetics 180, 409–420. doi: 10.1534/genetics.108.090126
Koide, Y., Ogino, A., Yoshikawa, T., Kitashima, Y., Saito, N., Kanaoka, Y., et al. (2018). Lineage-specific gene acquisition or loss is involved in interspecific hybrid sterility in rice. Proc. Nat. Acad. Sci. U. S. A. 115, E1955–E1962. doi: 10.1073/pnas.1711656115
Koide, Y., Onishi, K., Kanazawa, A., and Sano, Y. (2008b). “Genetics of speciation in rice,” in Rice Biology in the Genomics Era. Biotechnology in Agriculture and Forestry, eds H. Y. Hirano, Y. Sano, A. Hirai, and T. Sasaki (Berlin, Heidelberg: Springer), 247–259.
Koide, Y., Onishi, K., Nishimoto, D., Baruah, A. R., Kanazawa, A., and Sano, Y. (2008c). Sex-independent transmission ratio distortion system responsible for reproductive barriers between Asian and African rice species. New Phytol. 179, 888–900. doi: 10.1111/j.1469-8137.2008.02490.x
Koide, Y., Shinya, Y., Ikenaga, M., Sawamura, N., Matsubara, K., Onishi, K., et al. (2012). Complex genetic nature of sex-independent transmission ratio distortion in Asian rice species: the involvement of unlinked modifiers and sex-specific mechanisms. Heredity. 108, 242–247. doi: 10.1038/hdy.2011.64
Kubo, T., Eguchi, M., and Yoshimura, A. (2000). A new gene for F1 pollen sterility in japonica/indica cross of rice. Rice Genet. Newsl. 17, 63–64.
Kubo, T., Eguchi, M., and Yoshimura, A. (2001). A new gene for F1 pollen sterility located on chromosome 12 in japonica/indica cross of rice. Rice Genet. Newsl. 18, 54.
Kubo, T., Yamagata, Y., Eguchi, M., and Yoshimura, A. (2008). A novel epistatic interaction at two loci causing hybrid male sterility in an inter-subspecific cross of rice (Oryza sativa L.). Genes Genet. Syst. 83, 443–453. doi: 10.1266/ggs.83.443
Kubo, T., Yoshimura, A., and Kurata, N. (2016). Pollen killer gene S35 function requires interaction with an activator that maps close to S24, another pollen killer gene in rice. G3. 6, 1459–1468. doi: 10.1534/g3.116.027573
Li, D., Zhu, S., Jiang, L., and Wan, J. (2005). Mapping for a new locus causing hybrid sterility in a China landrace (Oryza sativa L.). Rice Genet. Newsl. 22, 17–19.
Li, J., Zhou, J., Xu, P., Deng, X., Deng, W., Zhang, Y., et al. (2018). Mapping five novel interspecific hybrid sterile loci between Oryza sativa and Oryza meridionalis. Breed. Sci. 68, 516–523. doi: 10.1270/jsbbs.18001
Li, J., Zhou, J., Zhang, Y., Yang, Y., Pu, Q., and Tao, D. (2020). New insights into the nature of interspecific hybrid sterility in rice. Front. Plant Sci. 11, 555572. doi: 10.3389/fpls.2020.555572
Li, W., Zeng, R., Zhang, Z., Xiaohua, D., and Guiquan, Z. (2006). Fine mapping of locus S-b for F1 pollen sterility in rice (Oryza sativa L.). Chin. Sci. Bull. 51, 675–680. doi: 10.1007/s11434-006-0675-6
Long, Y., Zhao, L., Niu, B., Su, J, Wu, H., Chen, Y., et al. (2008). Hybrid male sterility in rice controlled by interaction between divergent alleles of two adjacent genes. Proc. Natl. Acad. Sci. U. S. A. 105, 18871–18876. doi: 10.1073/pnas.0810108105
Lyttle, T. W. (1991). Segregation distorters. Annu. Rev. Genet. 25, 511–581. doi: 10.1146/annurev.ge.25.120191.002455
Maguire, M. P. (1963). High transmission frequency of a tripsacum chromosome in corn. Genetics 48, 1185–1194. doi: 10.1093/genetics/48.9.1185
Miyazaki, Y., Doi, K., Yasui, H., and Yoshimura, A. (2007). Identification of a new allele of F1 pollen sterility gene, S21, detected from the hybrid between Oryza sativa and O. rufipogon. Rice Genet. Newsl. 23, 36–38.
Moyle, L. C. (2006). Genome-wide associations between hybrid sterility QTL and marker transmission ratio distortion. Mol. Biol. Evol. 23, 973–980. doi: 10.1093/molbev/msj112
Nosil, P. (2013). “Reproductive isolation,” in Brenner's Encyclopedia of Genetics, eds S. Molley and K. Hughes (San Diego, CA: Academic Press), 173–176.
Oka, H.-I. (1957). Genic analysis for the sterility of hybrids between distantly related varieties of cultivated rice. J. Genet. 55, 397–409. doi: 10.1007/BF02984059
Ouyang, Y., and Zhang, Q. (2013). Understanding reproductive isolation based on the rice model. Annu. Rev. Plant Biol. 64, 111–135. doi: 10.1146/annurev-arplant-050312-120205
Pardo-Manuel de Villena, F., and Sapienza, C. (2001). Nonrandom segregation during meiosis: the unfairness of females. Mamm. Genome 12, 331–339. doi: 10.1007/s003350040003
Presgraves, D. C., and Meiklejohn, C. D. (2021). Hybrid sterility, genetic conflict and complex speciation: lessons from the Drosophila simulans clade species. Front. Genet. 12, 669045. doi: 10.3389/fgene.2021.669045
Price, C. S. C. (1997). Conspecific sperm precedence in Drosophila. Nature 388, 663–666. doi: 10.1038/41753
Ren, G., Xu, P., Deng, X., Zhou, J., Hu, F., Li, J., et al. (2005). A new gamete eliminator from Oryza glaberrima. Rice Genet. Newsl. 22, 45–47.
Sakata, M., Takano-Kai, N., Miyazaki, Y., Kanamori, H., Wu, J., Matsumoto, T., et al. (2021). Domain unknown function DUF1668-containing genes in multiple lineages are responsible for F1 pollen sterility in rice. Front. Plant Sci. 11, 632420. doi: 10.3389/fpls.2020.632420
Sano, Y. (1983). A new gene controlling sterility in F1 hybrids of two cultivated rice species. J. Hered. 74, 435–439. doi: 10.1093/oxfordjournals.jhered.a109832
Sano, Y., Chu, Y.-E., and Oka, H.-I. (1979). Genetic studies of speciation in cultivated rice. 1. Genic analysis for the F1 sterility between O. sativa L. and O. glaberrima Steud. Jpn. J. Genet. 54, 121–132. doi: 10.1266/jjg.54.121
Sano, Y., Sano, R., Eiguchi, M., and Hirano, H. (1994). Gamete eliminator adjacent to the wx locus as recealed by pollen analysis in rice. J. Hered. 85, 310–312. doi: 10.1093/oxfordjournals.jhered.a111465
Sawamura, N., and Sano, Y. (1996). Chromosomal location of gamete eliminator, S11(t), found in an indica-japonica hybrid. Rice Genet Newslett. 13, 70–71.
Seymour, D. K., Chae, E., Arioz, B. I., Koenig, D., and Weigel, D. (2019). Transmission ratio distortion is frequent in Arabidopsis thaliana controlled crosses. Heredity 122, 294–304. doi: 10.1038/s41437-018-0107-9
Shen, R., Wang, L., Liu, X., Wu, J., Jin, W., Zhao, X., et al. (2017). Genomic structural variation-mediated allelic suppression causes hybrid male sterility in rice. Nat. Commun. 8, 1–10. doi: 10.1038/s41467-017-01400-y
Silver, L. M. (1993). The peculiar journey of a selfish chromosome: mouse t haplotypes and meiotic drive. Trends Genet. 9, 250–254. doi: 10.1016/0168-9525(93)90090-5
Taguchi, K., Doi, K., and Yoshimura, A. (1999). RFLP mapping of S19, a gene for F1 pollen semi-sterility found in backcross progeny of Oryza sativa and O. glaberrima. Rice Genet. Newsl. 16, 70–71.
Turelli, M. (1998). The causes of Haldane's rule. Science. 282, 889–891. doi: 10.1126/science.282.5390.889
Ubeda, F., and Haig, D. (2005). On the evolutionary stability of Mendelian segregation. Genetics 170, 1345–1357. doi: 10.1534/genetics.104.036889
Wan, J., and Ikehashi, H. (1995). Identification of a new locus S-16 causing hybrid sterility in native rice varieties (Oryza sativa L.) from Tai hu Lake region and Yunnan province, China. Jpn. J. Breed. 45, 461–470. doi: 10.1270/jsbbs1951.45.461
Wan, J., Yamaguchi, Y., Kato, H., and Ikehashi, H. (1996). Two new loci for hybrid sterility in cultivated rice (Oryza sativa L.). Theoret. Appl. Genet. 92, 183–190. doi: 10.1007/BF00223375
Wan, J., Yanagihara, S., Kato, H., and Ikehashi, H. (1993). Multiple alleles at a new locus causing hybrid sterility between a Korean indica variety and a javanica variety in rice (Oryza sativa L.). Jpn. J. Breed. 43, 507–516. doi: 10.1270/jsbbs1951.43.507
Win, K., Kubo, T., Miyazaki, Y., Doi, K., Yamagata, Y., and Yoshimura, A. (2009). Identifification of two loci causing F1 pollen sterility in inter- and intraspecifific crosses of rice. Breed. Sci. 59, 411–418 doi: 10.1270/jsbbs.59.411
Xie, Y., Shen, R., Chen, L., and Liu, Y.-G. (2019a). Molecular mechanisms of hybrid sterility in rice. Sci. China Life Sci. 62, 737–743. doi: 10.1007/s11427-019-9531-7
Xie, Y., Tang, J., Xie, X., Li, X., Huang, J., Fei, Y., et al. (2019b). An asymmetric allelic interaction drives allele transmission bias in interspecific rice hybrids. Nat. Commun. 10, 2501. doi: 10.1038/s41467-019-10488-3
Xie, Y., Xu, P., Huang, J., Ma, S., Xie, X., Tao, D., et al. (2017). Interspecific hybrid sterility in rice is mediated by OgTPR1 at the S1 locus encoding a peptidase-like protein. Mol. Plant. 10, 1137–1140. doi: 10.1016/j.molp.2017.05.005
Xu, P., Zhou, J., Li, J., Hu, F., Deng, X., Feng, S., et al. (2014). Mapping three new interspecific hybrid sterile loci between Oryza sativa and O. glaberrima. Breed. Sci. 63, 476–482. doi: 10.1270/jsbbs.63.476
Yamagata, Y., Yamamoto, E., Aya, K., Win, K., Doi, K., Sobrizal Ito, T., et al. (2010). Mitochondrial gene in the nuclear genome induces reproductive barrier in rice. Proc. Natl. Acad. Sci. U. S. A. 107, 1494–1499. doi: 10.1073/pnas.0908283107
Yang, J., Zhao, X., Cheng, K., Du, H., Ouyang, Y., Chen, J., et al. (2012). A Killer-Protector system regulates both hybrid sterility and segregation distortion in rice. Science 337, 1336–1340. doi: 10.1126/science.1223702
Yang, Y., Zhou, J., Li, J., Xu, P., Zhang, Y., and Tao, D. (2016). Mapping QTLs for hybrid sterility in three AA genome wild species of Oryza. Breed. Sci. 66, 367–371. doi: 10.1270/jsbbs.15048
Yu, X., Zhao, Z., Zheng, X., Zhou, J., Kong, W., Wang, P., et al. (2018). A selfish genetic element confers non-Mendelian inheritance in rice. Science 360, 1130–1132. doi: 10.1126/science.aar4279
Yu, Y., Zhao, Z., Shi, Y., Tian, H., Liu, L., Bian, X., et al. (2016). Hybrid sterility in rice (Oryza sativa L.) involves the tetratricopeptide repeat domain containing protein. Genetics 203, 1439–1451. doi: 10.1534/genetics.115.183848
Zhang, Y., Zhou, J., Li, J., Yang, Y., Xu, P., and Tao, D. (2018). Mapping of S56(t) responsible for interspecific hybrid sterility between Oryza sativa and Oryza glumaepatula. Breed. Sci. 68, 242–247. doi: 10.1270/jsbbs.17116
Zhang, Z., Xu, P., Hu, F., Zhou, J., Li, J., Deng, X., et al. (2005). A new sterile gene from Oryza glaberrima on chromosome 3. Rice Genet. Newsl. 22, 26–28.
Zhao, J., Li, J., Xu, P., Zhou, J., Hu, F., Deng, X., et al. (2012). A new gene controlling hybrid sterility between Oryza sativa and Oryza longistaminata. Euphytica 187, 339–344. doi: 10.1007/s10681-012-0691-3
Zhu, S., Jiang, L., Wang, C., Zhai, H., Li, D., and Wan, J. (2005a). The origin of weedy rice Ludao in China deduced by genome wide analysis of its hybrid sterility genes. Breed. Sci. 55, 409–414. doi: 10.1270/jsbbs.55.409
Zhu, S., Wang, C., Zheng, T., Zhao, Z., Ikehashi, H., and Wan, J. (2005b). A new gene located on chromosome 2 causing hybrid sterility in a remote cross of rice. Plant Breed. 124, 440–445. doi: 10.1111/j.1439-0523.2005.01143.x
Zin Mar, M., Koide, Y., Ogata, M., Kuniyoshi, D., Tokuyama, Y., Hikichi, K., et al. (2021). Genetic mapping of the gamete eliminator locus, S2, causing hybrid sterility and transmission ratio distortion found between Oryza sativa and Oryza glaberrima cross combination. Agriculture 11, 268. doi: 10.3390/agriculture11030268
Keywords: rice, hybrid sterility, selfish genes, reproductive isolating barrier, transmission ratio distortion
Citation: Zin Mar Myint and Koide Y (2022) Influence of Gender Bias on Distribution of Hybrid Sterility in Rice. Front. Plant Sci. 13:898206. doi: 10.3389/fpls.2022.898206
Received: 17 March 2022; Accepted: 11 May 2022;
Published: 12 July 2022.
Edited by:
Mallikarjuna Swamy, International Rice Research Institute (IRRI), PhilippinesReviewed by:
Jing Li, Yunnan Academy of Agricultural Sciences, ChinaAris Hairmansis, Indonesian Center for Rice Research, Indonesia
Copyright © 2022 Zin Mar Myint and Koide. This is an open-access article distributed under the terms of the Creative Commons Attribution License (CC BY). The use, distribution or reproduction in other forums is permitted, provided the original author(s) and the copyright owner(s) are credited and that the original publication in this journal is cited, in accordance with accepted academic practice. No use, distribution or reproduction is permitted which does not comply with these terms.
*Correspondence: Yohei Koide, eWtvaWRlQGFicy5hZ3IuaG9rdWRhaS5hYy5qcA==