- 1Department of Agronomy, Muhammad Nawaz Shareef University of Agriculture, Multan, Multan, Pakistan
- 2Department of Environmental Science, COMSATS University Islamabad, Vehari, Pakistan
- 3Army Public School and College Mailsi Garrison, Mailsi, Pakistan
- 4Department of Horticulture, Ghazi University, Dera Ghazi Khan, Pakistan
- 5Chair of Climate Change, Environmental Development and Vegetation Cover, Department of Botany and Microbiology, College of Science, King Saud University, Riyadh, Saudi Arabia
- 6Department of Agronomy, University College of Dera Murad Jamali Naseerabad, Lasbela University of Agriculture, Water and Marine Sciences, Uthal, Pakistan
- 7Department of Agricultural Engineering, Khwaja Fareed University of Engineering and Information Technology, Rahim Yar Khan, Pakistan
- 8Tasmanian Institute of Agriculture, University of Tasmania, Burnie, TAS, Australia
- 9College of Life Science, Linyi University, Linyi, China
- 10Hainan Key Laboratory for Sustainable Utilization of Tropical Bioresource, College of Tropical Crops, Hainan University, Haikou, China
- 11Department of Agronomy, Faculty of Agricultural Sciences, The University of Haripur, Haripur, Pakistan
Climatic conditions significantly affect the maize productivity. Among abiotic factors, nitrogen (N) fertilizer and temperature are the two important factors which dominantly affect the maize (Zea mays L.) production during the early crop growth stages. Two experiments were conducted to determine the impact of N fertilizer and temperature on the maize growth and yield. In the first experiment, the maize hybrids were screened for their sensitivity to temperature variations. The screening was based on the growth performance of the hybrids under three temperatures (T1 = ambient open-air temperature, T2 = 1◦C higher than the ambient temperature, and T3 = 1◦C lower than the ambient temperature) range. The results showed that an increase in temperature was resulted less 50% emergence and mean emergence (4.1 and 6.3 days, respectively), while emergence energy and full emergence were higher (25.4 and 75.2%, respectively) under the higher temperature exposure. The results showed that Syngenta 7720 and Muqabla S 25W87 were temperature tolerant and sensitive maize hybrids, respectively. The second experiment was carried out to study the response of the two selected maize hybrids (Syngenta 7720 and Muqabla S 25W87) to four N fertilizer applications. The results revealed that the maximum N use efficiency (19.5 kg kg−1) was achieved in maize hybrids with low N application (75 kg N ha−1 equivalent to 1.13 g N plant−1). However, the maximum maize grain yield (86.4 g plant−1), dry weight (203 g plant−1), and grain protein content (15.0%) were observed in maize hybrids that were grown with the application of 300 kg N ha−1 (equivalent to 4.52 g N plant−1). Therefore, it is recommended that the application of 300 kg N ha−1 to temperature tolerant maize hybrid may be considered best agricultural management practices for obtaining optimum maize grain yield under present changing climate.
Introduction
The maize crop is ranked as the third major cereal crop after wheat and rice and similarly, it is the third most widely grown cereal crop in Pakistan (Hussain et al., 2011). The maize production is highly dependent on the climatological and pedological conditions and it is necessary to determine how changes in climate and soil conditions can maintain or improve the production. The meteorological parameters determined that local climate conditions have a great deal on agricultural productivity. Considering the possible climate change and its impact on regional agricultural systems are critical. In recent years, climate change has been affecting the global agricultural productivity and precisely these impacts are more toward the negative side of crop production (Joshi et al., 2015; Boonwichai et al., 2019; Zhang et al., 2020). Soil is another key factor in determining the plants growth and their productivity (Rodrigo-Comino et al., 2018). Soil characteristics are critical and require for the precise calculations of the number of fertilizers to be supplied, and N is the key element due to its impact on the plant water relations and biota in the rhizosphere (Basso et al., 2020; He et al., 2020).
The quality of grain crops and crop yield is strongly dependent on the N fertilizer. Numerous researchers reported that N fertilizers application generally has positive and significant impacts on the crop growth and yield (Gasim, 2001; Amanullah et al., 2016). The maize plant characteristic such as leaf number per plant is increased by the application of N which improved plant height (Akintoye, 1996) by increasing the distance among the internodes and length of the internodes (Gasim, 2001). Therefore, the application of N fertilizer is also good for increasing the height, leaf area, and stem diameter as well as the fresh and dry yields of maize (Koul, 1997). Although only small amounts of irrigation water and N fertilizers are required during early growth stages (Chen et al., 2020), higher concentration of N at that time in the root zone is beneficial for achieving the high-crop yields (Ritchie et al., 1993; Hammad et al., 2018). As N is an important source of nutrition but its losses during crop growth (Wang et al., 2019) not only results in N deficiency but also negatively affects the environment (Sutton et al., 2013).
The farming communities are not well familiar about the interaction of N fertilizer with other crop input sources especially temperature which are affecting the final grain yield. Similarly, N use efficiency (NUE) can be influenced with changes in ambient temperature and N application rates (Zhang et al., 2022). So, optimum utilization of N fertilizer by a plant is a major factor for good crop production (Sinclair and Horie, 1989; Yousaf et al., 2016). An optimum supply of N fertilizer increases total biomass production and crop yield but at lower N fertilizer supply, plant dry matter accumulation of reproductive parts decreases, resulting in the lower grain yield (Monneveux et al., 2005). Hence, N fertilizer management is a crucial need of the time while considering the enigma of global food insecurity to ensure adequate grain crop production across the World (Thompson et al., 2015).
A decrease in the losses of N fertilizer from the agricultural lands is a hot topic worldwide among the agricultural researchers. Besides, N optimization ensures the soil environmental safety. As the climate change (temperature) and N fertilizer effects on maize hybrids potential yields, however, interaction among the two critical factors, i.e., temperature with N utilization has not been explored yet especially under semi-arid environments. Therefore, this study was planned for the following objectives (i) to determine the temperature effect on the growth of maize hybrids and (ii) to optimize N fertilizer dose for the local maize hybrids under the changing climate.
Materials and Methods
The Design and Treatment of First Experiment
A pot experiment was conducted in glasshouse at COMSATS University Islamabad, Vehari Campus to study temperature effects on maize growth and yield. Soil analysis was carried out for determining NPK ratio in the soil. Prior to the experiment, the germination test was conducted to find out the seed germination percentage of maize hybrids. The experiment objective was to screen the 10 maize hybrids (detail shown in Table 1) against temperature sensitivity. These hybrids were grown under three different temperatures (T1 = Open-air ambient temperature, T2 = 1◦C higher than ambient temperature, and T3 = 1◦C lower than ambient temperature). The higher and lower temperatures were continually maintained in the glasshouse. A thermometer was placed among the pots for measuring the temperature. The completely randomized design with factorial arrangement having three replicates was used during the study. Two maize hybrids (H1 = heat tolerant and H2 = heat sensitive) were screened from the experiment on basis of germination and growth characteristics. Furthermore, a detail on daily open-air meteorological and green house data of experimental location is shown in Figure 1.
Observation
The experimental plants data were observed after each hour. The number of seeds emergence were noted according to the handbook of the Association of Official Seed Analysis (AOSA). (1990). Time taken to 50% seedlings (E50) emergence was calculated according to the formula of Coolbear et al. (1984) modified by Farooq et al. (2005).
Mean emergence time (MET) was calculated according to the equation of Ellis and Roberts (1981).
Energy of germination was recorded at 4th day after planting. It was the percentage of emerged seedlings 4 days after planting relative to the total number of seeds tested (Farooq et al., 2006).
The plants were harvested at maturity. The stem length (cm) was measured from base to tip of the plants. The plant shoots were cut from the pot and the soil was washed with water on iron grille to retrieve the roots. The root length (cm) was also measured from plant base to the root tip. The plants were separated into roots, shoots, and leaves. The plant each fraction was oven dried separately at 70◦C till constant weight. The dry weight (DW) of roots and shoots was measured in grams (g).
The Design and Treatments of Second Experiment
The two maize hybrids (H1 = Syngenta 7720 and Muqabla S H2 =25W87 temperature tolerant and sensitive, respectively) selected from the first experiment were tested under four different N rates with one control treatment (N1 = 0, N2 = 1.13 g plant−1 equal to 75 kg N ha−1, N3 = 2.26 g plant−1 equal to 150 kg N ha−1, N4 = 3.39 g plant−1 equal to 225 kg N ha−1, and N5 = 4.52 g plant−1 equal to 300 kg N ha−1). The completely randomized design with factorial arrangement having four replicates was used during this study.
Plant Sampling
Plant height (cm) was measured with the help of scale from base to tip of the plants at maturity. At physiological maturity, plants were extracted from the pot and the soil was washed with the water pressure on iron grille. The root length (cm) was measured with the help of scale from plant base to root tip. The harvested plants were thrashed manually for measuring the thousand grain weight and total yield. Thousand grains were taken from each plant and sun dried up to standard moisture content in the grains. The total grains and dry matter of each plant were calculated as g plant−1. The Micro-Kjeldhal method (Helrich, 1990) was used for determining the N contents in grains and then the crude protein contents were determined by using the following formula
The NUE was calculated by the formula derived by Dobermann (2007).
where, F is the amount of N fertilizer (kg ha−1), Y is the crop yield (kg ha−1) with N application, and Y◦ is the crop yield (kg ha−1) without application of N fertilizer.
All biological processes in plant respond to temperature, and all responses can be summarized in terms of cardinal temperatures (a base temperature). Therefore, the thermal time (growing degree days) of plant was calculated according to Gallagher et al. (1983) by using the following formula:
where Tb is cardinal or base temperature taken as 8◦C for maize (FAO, 1978).
Statistical Analysis
The effects of treatments on the studied variables were analyzed with ANOVA using the SAS statistical software. The least significant difference test was used for comparing the means of the treatments when the F-values were significant at p 0.05 (SAS Institute, 2004). The interaction among the factors (temperature, maize hybrids, and nitrogen [N]) was non-significant, so the results of the factors were described separately.
Results
First Experiment
Time taken to 50% emergence of seedling was significantly affected under different temperature conditions and the hybrids showed different response to the temperature to initiate 50% emergence (Table 1). The maize hybrids took minimum time (4.1 day) for 50% emergence when they were subjected to ≈1◦C higher temperature (T2) than the ambient temperature (T1) while the maximum time (6.2 day) to exhibit 50% emergence was taken by the maize seeds that were kept under T3 (≈1◦C less from normal temperature). Similarly, among the maize hybrid, minimum time (3.9 day) taken to 50% emergence was observed in H3 (Syngenta 7720) while the hybrid H7 (Muqabla S 25W87) took maximum time (6.8 day) for 50% emergence.
Time taken to mean emergence of maize was significantly influenced by the exposure of crop to various temperatures (Table 1). Maize grown in approximately one-degree higher temperature (T2) from normal temperature (T1) showed significantly lower time (6.3 day) for the plant mean emergence. Precisely evaluating the performance of subjected maize hybrids, the minimum time (5.7 day) taken for mean emergence was observed in H3 (Syngenta 7720) and the maximum time (9.1 day) was observed in H7 (Muqabla S 25W87).
The results showed (Table 1) that the exposure of maize seeds to various temperatures significantly influenced the time taken by emergence energy of various maize hybrids. The maize grown under T2 treatment showed the maximum emergence energy (25.4%) and the minimum emergence energy (21.0%) was observed in maize hybrids that were kept under T3. Maximum emergence energy (34.9%) among the hybrids was observed in H3 (Syngenta 7720) while less emergence energy (21.1%) was observed in H7 (Muqabla S 25W87).
In similar terms, the time taken for full emergence of maize hybrids was also affected by various temperatures (Table 1). Seeds exposed to approximately one-degree higher (T2) temperature from ambient showed higher emergence (75.2%). The minimum time (66.9%) taken to full emergence was observed in maize hybrids that were grown in T3 unit. The hybrids used in this study showed significant response to the temperature for time taken to full emergence. The maximum full emergence (82.4%) was observed in H3 (Syngenta 7720) and the minimum time taken for full emergence (42.3%) was recorded in H7 (Muqabla S 25W87).
The stem length is an important parameter, and the results showed that the seedlings exposed to various temperatures significantly differ in stem length (Table 2). The maximum stem length (102.4cm) was recorded in maize hybrid that was grown under the ambient conditions. However, among the maize hybrid, a significant difference was observed for stem length. The maximum stem length (111.6 cm) was observed in H3 (Syngenta 7720), while the minimum stem length (78.4 cm) was recorded in H7 (Muqabla S 25W87).
The plant DW is an important characteristic for determining the plant biomass accumulation. The results showed that the various temperatures distinctively influenced the plant DW of maize hybrids (Table 2). The maximum plant DW (69.9 g plant−1) was observed in maize grown under T1; however, maize hybrids that were kept under T3-exhibited minimum plant DW (50.2 g plant−1). Among the hybrids, the maximum plant DW (73.2 g plant−1) was recorded in H5 (Sohni-Dharti 626) which was statistically similar with maize hybrid H4, while the minimum plant DW (43.6 g plant−1) was recorded in the H7 (Muqabla S25W87).
The results showed that various temperatures significantly influenced the root length of maize (Table 2). The maximum root length (54.8 cm) was recorded in maize that was kept under the ambient temperature and the results were showed the root significantly decreased by decreasing the temperature. The root length was significantly varied among the maize hybrid and the maximum root length (65.3 cm) was observed in H3 (Syngenta 7720), while the minimum root length (28.1 cm) was recorded in H7 (Muqabla S25W87).
The root DW is an another important parameter that was evaluated under our prescribed temperature ranges in this study. The results showed that the root DW of maize hybrids was also affected by different temperature regimes (Table 2). The maximum root DW (11.18 g plant−1) was observed in maize hybrid which was grown under the ambient temperature. In case of different hybrids, the maximum root DW (13.78 g plant−1) was observed in H3 (Syngenta 7720) and the minimum plant DW (4.78 g plant−1) was recorded in H7 (Muqabla S25W87).
The results of first experiment showed the maize hybrid Syngenta 7720 was temperature tolerant while the hybrid Muqabla S25W87 was temperature sensitive. These two selected hybrids were evaluated with four N fertilizer rates including one control treatments during the second experiment.
Second Experiment
The results showed temperature tolerant maize hybrid (Syngenta 7720) attained the higher stem length (161 cm) as compared to temperature sensitive maize hybrid (Muqabla S25W87). The application of N was significantly affected the plant growth. There was a linear relationship between plant height and N application rates during the study (Table 3). The maximum plant height (191 cm) was observed by the application of 300 kg N ha−1 while the minimum plant height (107 cm) was recorded in the control treatment. The application of N fertilizer was enhanced 78% higher stem length when compared with the control treatment (191 vs. 107).
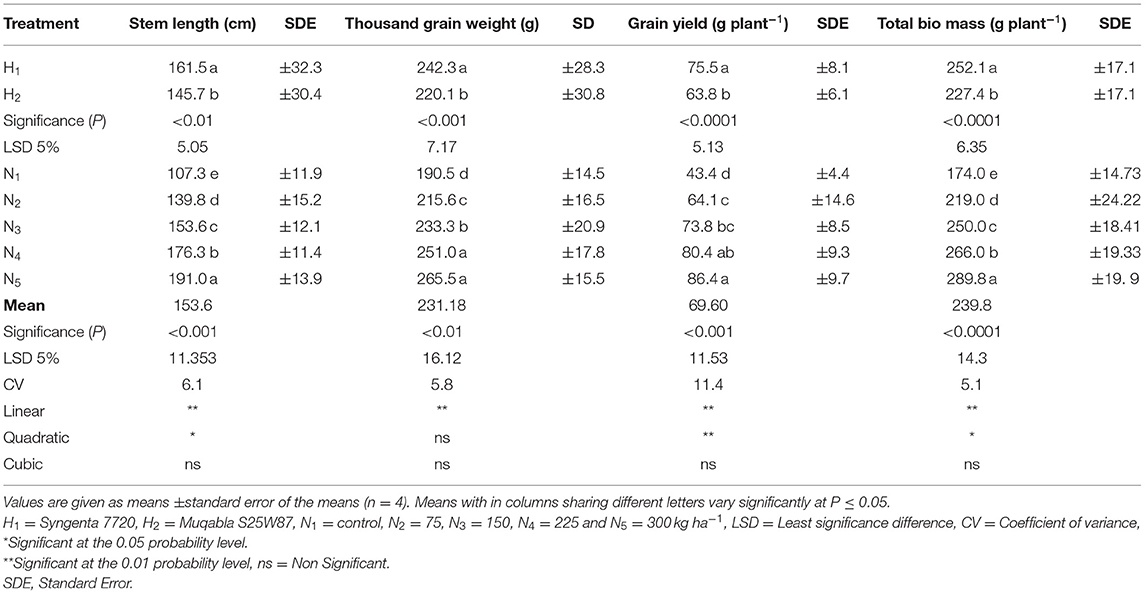
Table 3. Effect of nitrogen (N) application on temperature tolerant (H1) and sensitive (H2) maize hybrid growth and yield.
The thousand grain weight is an important maize characteristic which plays a significant role for determining the maize yield. The temperature tolerant maize hybrid (H1) showed comparatively higher 1000 grain weight (242 g) as compared to temperature sensitive maize hybrid (H2) which suggests that the increasing trend of temperature might be a factor in decreasing the maize 1000 grain weight as increasing temperature decrease the growing degree days of the plant (Table 3). The results showed that there was a linear relationship between N application rates and 1000 grain weight. The maximum 1000 grain weight (265 g) was observed in the N4 treatment (300 kg N ha−1). This increase was 39.4% than the control treatment (265.5 vs. 190.5) and the minimum 1000 grain weight (190 g) was recorded in the control treatment.
The temperature tolerant maize hybrid (H1) showed higher grain yield (75.5 g plant−1) as compared to temperature sensitive maize hybrid (H2). There was a linear and quadratic relationship between N application rates and grain yield (Table 3). The maximum grain yield (86.4 g plant−1) was observed in the maize hybrids that were kept in N4 treatment (300 kg N ha−1). However, the minimum grain yield (43.4 g plant−1) was recorded in control treatment. Maize grown in N4 treatment resulted in 99% higher grain yield (86.4 vs. 43.4) as compared to zero N application (control). The grain yield was significantly and positively correlated (R2 = 0.92) with the N application rates (Figure 2A).
The results showed (Table 3) temperature tolerant maize hybrid (H1) showed significantly higher total biomass production (252 g plant−1) as compared to temperature sensitive maize hybrid (H2). The results revealed that there was a linear relationship between total biomass production and N application. The maximum total biomass (289.8 g plant−1) was observed in maize that was grown in N4 treatment and the minimum total biomass (174 g plant−1) was recorded in control treatment. The application of 300 kg N ha−1 (N4) resulted in 66.5% higher total biomass production (289.8 vs. 174) as compared to the control treatment.
The temperature tolerant maize hybrid showed higher root length (61.1 cm) as compared to temperature sensitive (H1) maize hybrid (Table 4). In addition, the N application showed linear and quadratic effects on root length. The maximum root length (71.5 cm) was observed by the application of 225 kg N ha−1 (N4) and beyond this rate, there was no significance increase in root length and the minimum root length (47.6 cm) was recorded in control treatment (N0). Similarly, the temperature tolerant maize hybrid (H1) showed the maximum root DW (17.8 g plant−1) as compared to temperature sensitive maize hybrid (Table 4). In case of N application, the results showed that the root DW was increased by increasing N application rate up to 300 kg ha−1 (N5). There was a linear and cubic effect of N application rates on root DW. The maximum root DW (20.3 g plant−1) was recorded in the N5 treatment. However, the minimum root weight (13.4 g plant−1) was recorded in unfertilized treatment, i.e., control treatment (N1). The application of N 300 kg ha−1 (N5) was resulted 51% (20.3 vs. 13.4) higher root DW as compared to control treatment.
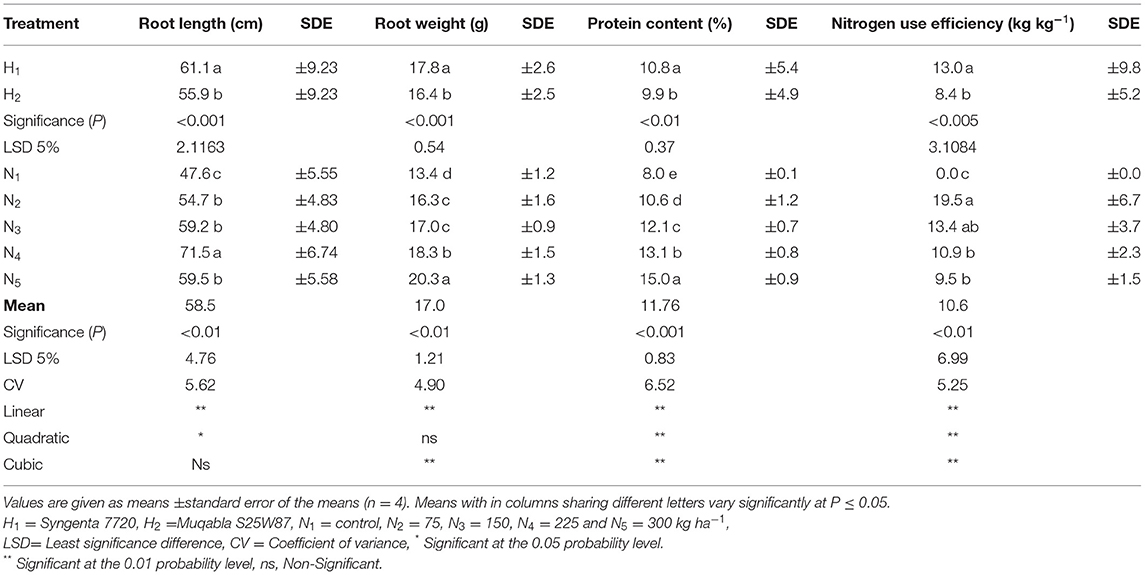
Table 4. Effect of N application on temperature tolerant (H1) and sensitive (H2) maize hybrid characteristic.
Like other parameters, the higher protein content in grains (10.8%) was recorded in temperature tolerant maize hybrid (H1) as compared to temperature sensitive maize hybrid (Table 4). The results showed that the protein content in grain increased by increasing the N application rate up to 300 kg ha−1 (N5). There was a cubic effect of N application rates that was observed on the protein content in grains. The maximum protein content in grain (15.0%) was observed in the N5 treatment, while the minimum protein content in grain (8.0%) was recorded in the control treatment. The application of N 300 kg ha−1 resulted in 47% higher protein content (15.0 vs. 8.0) in grains as compared to the control treatment. The protein contents were significantly correlated (R2 = 0.97) with N application rates (Figure 2B).
The results revealed temperature tolerant maize hybrid (H1) attained significantly higher NUE (13.0 kg kg−1) as compared to H2 maize hybrid (Table 4). The results showed that the NUE was decreased by increasing N application rate. The maximum NUE (19.5 kg kg−1) was observed by the application of N at rate of 75 kg ha−1 (N2) and the minimum NUE (9.5 kg kg−1) was recorded in N5 treatment.
The crop development of second experiment is showed in Table 5 which represented calendar days and thermal time (growing degree days) from sowing to emergence. It has also been seen that the maize hybrids did not show any response to different N fertilizer application doses to thermal time from emergence to silking. However, the response was observed significant during the later crop growth stages. The temperature tolerant maize hybrid (H1) and higher doses of N fertilizer application attained more thermal time than temperature sensitive (H2) and other maize units which received lower N fertilizer rates. The application of 225 and 300 kg N ha−1 resulted in the maximum thermal time (1975◦C days) in temperature tolerant maize hybrid and the minimum thermal time (1937◦C days) was observed in control treatment (no N application).
Discussion
The results revealed that an increase in temperature accelerated seed germination of maize hybrids. As during the spring season under semi-arid conditions, sometime air temperature is low at initial stages from maize base temperature (8◦C). The response of maize seed emergence to temperature was best with minimum cardinal temperatures ranging from 8 to 37.5◦C, and optimum temperatures of 25.9 to 37.0◦C (Edalat and Kazemeini, 2014). Hence, an increased temperature enhanced the germination capacity coupled with the indication that optimum temperature is the pre-requirement for the maize germination process (Meng et al., 2022). It is predicted in the scenarios, air temperature will increase due to climate change, which could limit the length of the maize-growing season if other resources are not limited (Odgaard et al., 2011).
Morroco et al. (2005) stated that the high level of deviation, in response to changing temperatures, was recorded among the maize hybrids concerning physiological and morphological characteristics of the plant, which indicated that alleles are present which can improve the plant adoptability to lower and higher temperature conditions. Being a complex phenomenon, i.e., chilling stress, cold tolerance is generally controlled with the influence of multiple genes. Hence, to understand the responses of maize to low temperature stress at emergence stage, a multidisciplinary approach comprising physiology, genetics, and molecular biology will be the best way. The result from our study revealed that an increase in temperature resulted in the fast germination mechanism in the seeds. Even though there was a slight difference of temperature among the treatments; it was still enough to create differences observable for the maize hybrids tolerance potential under varying temperature conditions. However, higher temperature at later crop growth stages especially at reproductive stage resulted in lower grain yield due to decrease in pollen development (Ji et al., 2010). Furthermore, higher temperature during the whole growing season was also resulted in early plant maturity as the plant attained required thermal time early and they have less time for dry matter accumulation through photosynthesis and nutrients from the soil (Hammad et al., 2013; Hatfield and Prueger, 2015). Khaliq et al. (2008) studied the thermal time and calendar days of maize different cultivars and varying rate of N application and they found significant effects of N fertilizer on maize hybrid. Similar effects were also observed by Hammad et al. (2013) and the authors have concluded that the N application timing and rates were affected the maize growth and development. The observed lower yield and yield components including stem and root length, root weight, and thousand grain weight in maize with one-degree higher temperature are due to the less duration of developmental phases, diminish the photosynthetic potential coupled with increased respiration, lower light interception, and pollen sterility (Cairns et al., 2013). In T2 treatment units (1◦ higher temperature than ambient), the pollen viability was negatively affected by the heat stress as pollen desiccation is severely affected by the surrounding air temperature (Fonseca and Westgate, 2005).
Our results were also in accordance with the study of Ordóñez et al. (2015) highlighting that higher temperature is the main reason for the reduction in maize crop yield by influencing the developmental trends in ear growth. The exposure of maize hybrids toward higher temperature (T2) at silking stages is a strong factor for lower final yield (Cicchino et al., 2010) through affecting the distribution of photosynthates among the sources and sinks in maize plants. Moreover, this source capacity is in fact influenced by a decline in carbohydrate synthesis (Barnabás and Fehér, 2008) which is due to the downscaled photosynthesis plus higher respiration rates (Ordóñez et al., 2015). In addition, the sink capacity is negatively affected by the deterioration in the synchrony of anthesis-silking phenomena that leads to decrease the ovule fertilization and increased the kernel abortion. Eventually, these impacts cause the disturbance in the process of pollination and kernel set, hence, causing severe yield losses (Edreira et al., 2011).
The crop phenology and growth notably responded to varying rate of N fertilizer. An increased rate of N fertilizer up to the optimum dose is a sound approach to attain the highest yield. The best possible development and maize grain yield were accomplished by the application of N at the rate of 300 kg N ha−1. The application of N increased cell counts and volume per leaf as well as it accelerated the formation of chlorophyll and increased plants biomass during the early stages of crop growth. The results were supported by the findings of Amanullah et al. (2009) and Hammad et al. (2011) who reported that the application of N fertilizer was increased the crop duration in maize.
Hammad et al. (2013) also observed that 2072◦C thermal days with the application of 250 kg N ha−1 were required to obtain optimum maize yield. The crop phenology was influenced by temperature and N fertilizer application rate. Furthermore, it has also been evaluated that a continuous enhancement in N application is not so beneficial in term of NUE parameter (Gheysari et al., 2009). Paolo and Rinaldi (2008) concluded that NUE could be improved only at a specific N fertilizer rate. The fact that the highest water use efficiency was attained with the application of more water and N discloses that both water and N are yield-limiting factors in semiarid environments. However, mostly in developing countries, each farmer's main objective is final the economic yield of the crop. The maximum grain yield of the maize crop was achieved by the application of N up to 300 kg ha−1 (equivalent to 4.52 g N plant−1). Therefore, this rate of N should be considered as a best fertilizer management practice under the semiarid regions in Pakistan and similar regions in the rest of the World.
Conclusion
The increase in temperature decrease the time required for germination time as well as for maturity in maize with less growing degree days which resulted in lower grain yield. The results showed that the increase in the N application rate up to 300 kg ha−1 (equivalent to 4.52 g N plant−1) resulted maximum grain yield and total dry matter production, but increase is in N application rate is not a sound strategy for attaining maximum N use efficiency. Therefore, it is concluded the applications of 300 kg N ha−1 by selecting temperature resilient maize hybrids should be considered a good agricultural practice for achieving optimum maize grain yield, in semiarid region of Pakistan. Furthermore, future global warming will result in a reduction in maize grain yield. Therefore, it is suggested that plant breeders policy makers should develop new temperature tolerant maize hybrids for achieving the high-crop productivity under changing climate.
Data Availability Statement
The original contributions presented in the study are included in the article/supplementary material, further inquiries can be directed to the corresponding author/s.
Author Contributions
HH: conceptualization and supervision. MC, RJ, AA, FK, HB, WF, MM, and AS: methodology and formal analysis. SF: writing—original draft preparation. SS, KL, MH, AA, FK, and SF: writing—review and editing. AA: funding acquisition. All authors contributed to the article and approved the submitted version.
Conflict of Interest
The authors declare that the research was conducted in the absence of any commercial or financial relationships that could be construed as a potential conflict of interest.
Publisher's Note
All claims expressed in this article are solely those of the authors and do not necessarily represent those of their affiliated organizations, or those of the publisher, the editors and the reviewers. Any product that may be evaluated in this article, or claim that may be made by its manufacturer, is not guaranteed or endorsed by the publisher.
Acknowledgments
The authors are grateful to the Deanship of Scientific Research, King Saud University for funding through Vice Deanship of Scientific Research Chairs.
References
Akintoye (1996). Thesis Abstract, International Institute of Tropical Agri Research, March 12. p. 25–27. doi: 10.1162/thld_a_00575
Amanullah, Iqbal, A., Ali, A., Fahad, S., and Parmar, B. (2016). Nitrogen source and rate management improve maize productivity of smallholders under semiarid climates. Front. Plant Sci. 7:1773. doi: 10.3389/fpls.2016.01773
Amanullah, Khattak, A. R., and Khalil, S. K. (2009). Plant density and nitrogen effects on maize phenology and grain yield. J. Plant. Nutri. 32, 246–260. doi: 10.1080/01904160802592714
Association of Official Seed Analysis (AOSA). (1990). Rules for testing seeds. J. Seed Technol. 12: 1–112.
Barnabás, J. K, and Fehér, A. (2008). The effect of drought and heat stress on reproductive processes in cereals. Plant. Cell Environ. 31:11–38. doi: 10.1111/j.1365-3040.2007.01727.x
Basso, M. F., Arraes, F. B. M., Grossi-de-Sa, M., Moreira, V. J. V., Alves-Ferreira, M., and Grossi-de-Sa, M. F. (2020). Insights into genetic and molecular elements for transgenic crop development. Front. Plant Sci. 11, 509. doi: 10.3389/fpls.2020.00509
Boonwichai, S., Shrestha, S., Babel, M. S., Weesakul, S., and Datta, A. (2019). Evaluation of climate change impacts and adaptation strategies on rainfed rice production in Songkhram River Basin, Thailand. Sci. Total Environ. 652:189–201. doi: 10.1016/j.scitotenv.2018.10.201
Cairns, J. E., Crossa, J., Zaidi, P. H., Grudloyma, P., Sanchez, C., Araus, J. L., et al. (2013). Identification of drought, heat, and combined drought and heat tolerant donors in maize. Crop Sci. 53:1335–1346. doi: 10.2135/cropsci2012.09.0545
Chen, X., Thorp, K. R., van Oel, P. R., Xu, Z., Zhou, B., and Li, Y. (2020). Environmental impactassessment of water-saving irrigation systems across 60 irrigation construction projects in northern China. J. Clean Prod. 245:118883. doi: 10.1016/j.jclepro.2019.118883
Cicchino, M., Rattalino Edreira, J. I., Uribelarrea, M., and Otegui, M. E. (2010). Heat stress in field-grown maize: response of physiological determinants of grain yield. Crop Sci. 50:1438–1448. doi: 10.2135/cropsci2009.10.0574
Coolbear, P., Francis, A., and Grierson, D. (1984). The effect of low temperature pre-sowing treatment on the germination performance and membrane integrity of artificially aged tomato seeds. J. Experi. Bot. 35, 1609–1617. doi: 10.1093/jxb/35.11.1609
Dobermann, A (2007). Nutrient use efficiency—measurement and management: In proceedings of IFA. International Workshop on Fertilizer Best Management Practices, Brussels, Belgium.
Edalat, M., and Kazemeini, S. A. (2014). Estimation of cardinal temperatures for seedling emergence in corn. Aust J Crop Sci. 8, 1072–1078.
Edreira, J. I. R., Carpici, E. B., Sammarro, D., and Otegui, M. E. (2011). Heat stress effects around flowering on kernel set of temperate and tropical maize hybrids. Field Crops Res. 123, 62–73. doi: 10.1016/j.fcr.2011.04.015
Ellis, R. A., and Roberts, E. H. (1981). The quantification of ageing and survival in orthodox seeds. Seed Sci. Technol. 9: 373–409.
FAO (1978). Agro-ecological zones. Report on the project, Vol. 1. Methodology and Results for Africa, Rome.
Farooq, M., Basra, S. M. A., Ahmad, N., and Hafeez, K. (2005). Thermal hardening: a new seed vigor enhancement tool in rice. J. Integrat. Plant Bio. 47, 187–193. doi: 10.1111/j.1744-7909.2005.00031.x
Farooq, M., Basra, S. M. A., Khalid, M., Tabassum, R., and Mehmood, T. (2006). Nutrient homeostasis, reserves metabolism and seedling vigor as affected by seed priming in coarse rice. Can. J. Bot. 84, 1196–1202. doi: 10.1139/b06-088
Fonseca, A. E., and Westgate, M. E. (2005). Relationship between desiccation and viability of maize pollen. Field Crop Res. 94:114–125. doi: 10.1016/j.fcr.2004.12.001
Gallagher, J. N., Biscoe, P. V., and Jones, R. D. (1983). Environmental influence on the development, growth and yield of barley. In: Barley Production and Marketing. Agron. Soc. N. Z. 200, 21–50.
Gasim, S. H (2001). Effect of nitrogen, phosphorus and seed rate on growth, yield and quality of forage maize (Zea mays L.). M.Sc. Thesis, Faculty of Agric., Univ. of Khartoum.
Gheysari M. Mirlatifi S. M. Homaee M. Asadi and, M. E. Hoogenboom G. (2009). Nitrate leaching in a silage maize field under different irrigation and nitrogen fertilizer rates. Agri. Water Manag. 96, 946–954. doi: 10.1016/j.agwat.2009.01.005
Hammad, H. M., Abbas, F., Ahmad, A., Farhad, W., Wilkerson, C. J., and Hoogenboom, G. (2018). Evaluation of timing and rates for nitrogen application for optimizing maize growth and development and maximizing yield. Agron. J. 110, 565–571. doi: 10.2134/agronj2017.08.0466
Hammad, H. M., Ahmad, A., Farhad, W., Abbas, F., Qasim, K., and Saeed, S. (2013). Nitrogen stimulates phenological traits, growth and growing degree days of maize. Pak. J. Agri. Sci. 50: 337–343.
Hammad, H. M., Ahmad, A., Khaliq, T., Farhad, W., and Mubeen, M. (2011). Optimizing rate of nitrogen application for higher yield and quality in maize under semiarid environment. Crop Environ. 2, 38–41.
Hatfield, J. L., and Prueger, J. H. (2015). Temperature extremes: Effect on plant growth and development. Weather. Clim. Extremes. 10: 4–10. doi: 10.1016/j.wace.2015.08.001
He, G., Wang, Z., and Cui, Z. (2020). Managing irrigation water for sustainable rice production in China. J. Clean. Prod. 245, 118928 doi: 10.1016/j.jclepro.2019.118928
Helrich, K (1990). Official Methods of Analysis, Volume 1 and 2, 15th Education Association of Official Analytical Chemists, Arlington, Virginia.
Hussain, N., Khan, M. Y., and Baloch, M. S. (2011). Screening of maize varieties for grain yield at Dera Ismail Khan. J. Anim. Plant Sci. 21, 626–628.
Ji, X., Shiran, B., Wan, J., Lewis, D. C., Jenkins, C. L., Condon, A. G., et al. (2010). Importance of pre-anthesis anther sink strength for maintenance of grain number during reproductive stage water stress in wheat. Plant Cell Environ. 33, 926–942. doi: 10.1111/j.1365-3040.2010.02130.x
Joshi, N., Singh, A., and Madramootoo, C. (2015). Corn yield simulation under different nitrogen loading and climate change scenarios. J. Irrig. Drain. Eng. 10.1061/(ASCE)IR.1943 4774.0000895 04015013. doi: 10.1061/(ASCE)IR.1943-4774.0000895
Khaliq, T., Ahmad, A., Hussain, A., Ranjha, A. M., and Ali, M. A. (2008). Impact of nitrogen rates on growth, yield and radiation use efficiency of maize under varying environments. Pak. J. Agri. Sci. 45, 1–7
Koul, G. G (1997). Effect of sowing methods, nitrogen levels and seed rates on yield and quality of fodder maize (Zea mays L.) M.Sc. Thesis, Univ. of Khartoum, Faculty of Agric.
Meng, A., Wen, D., and Zhang, C. (2022). Maize seed germination under low-temperature stress impacts seedling growth under normal temperature by modulating photosynthesis and antioxidant metabolism. Front. Plant Sci. 13:843033. doi: 10.3389/fpls.2022.843033
Monneveux, P., Zaidi, P. H., and Sanchez, C. (2005). Population density and low nitrogen affects yield-associated traits in tropical maize. Crop Sci. 45:535–545. doi: 10.2135/cropsci2005.0535
Morroco, A., Lorenzoni, C., and Fracheboud, Y. (2005). Chilling stress in maize. Maydica. 50: 571–580
Odgaard, M. V., Bøcher, P. K., Dalgaard, T., and Svenning, J. C. (2011). Climatic and non-climatic drivers of spatiotempo-ral maize-area dynamics across the northern limit for maize production - A case study from Denmark. Agri. Eco. Environ. 142, 291–302. doi: 10.1016/j.agee.2011.05.026
Ordóñez, R. A., Savin, R., Cossani, C. M., and Slafer, G. A. (2015). Yield response to heat stress as affected by nitrogen availability in maize. Field Crops Res. 183, 184–203. doi: 10.1016/j.fcr.2015.07.010
Ritchie, W. S., John, J., and Hanway, Garreno, B. (1993). How Acorn Plant Develop. Special Report No. 48, Iowa State Univ. of Science and Technology, Cooperative Extension Service
Rodrigo-Comino, J., Keesstra, S., and Cerdà, A. (2018). Soil erosion as an environmental concern in vineyards: The case study of celler del roure, Eastern Spain, by means of rainfall simulation experiments. Beverages 4, 31. doi: 10.3390/beverages4020031
Sinclair, T. R., and Horie, T. (1989). Leaf nitrogen, photosynthesis, and crop radiation use efficiency: a review. Crop Sci. 29:90–98. doi: 10.2135/cropsci1989.0011183X002900010023x
Sutton, M. A., Bleeker, A., Howard, C. M., Bekunda, M., Grizzetti, B., de Vries, W., et al (2013). Our Nutrient World. The Challenge to Produce More Food and Energy with Less Pollution. Edinburgh: Centre for Ecology and Hydrology. Available online at: https://edepot.wur.nl/249094
Thompson, A. M., Yu, J., Timmermans, M. C., Schnable, P., Crants, J. C., Scanlon, M. J., et al. (2015). Diversity of maize shoot apical meristem architecture and its relationship to plant morphology. G3: Genes| Genomes|. 5, 819–827. doi: 10.1534/g3.115.017541
Wang, S., Feng, X., Wang, Y., Zheng, Z., Li, T., He, S., et al. (2019). Characteristics of nitrogen loss in sloping farmland with purple soil in southwestern China during maize (Zea mays L.) growth stages. Catena. 182, 104169. doi: 10.1016/j.catena.2019.104169
Yousaf, M., Li, X., Zhang, Z., Ren, T., Cong, R., Ata-Ul-Karim, S. T., et al. (2016). Nitrogen fertilizer management for enhancing crop productivity and nitrogen use efficiency in a rice-oilseed rape rotation system in China. Front. Plant Sci. 7, 1496. doi: 10.3389/fpls.2016.01496
Zhang, L., Zhang, Z., Luo, Y., Cao, J., and Li, Z. (2020). Optimizing genotype-environment-management interactions for maize farmers to adapt to climate change in different agro-ecological zones across China. Sci. Total Environ. 728:138614. doi: 10.1016/j.scitotenv.2020.138614
Keywords: best management practices, climate variability, maize yield production, protein content, nitrogen
Citation: Hammad HM, Chawla MS, Jawad R, Alhuqail A, Bakhat HF, Farhad W, Khan F, Mubeen M, Shah AN, Liu K, Harrison MT, Saud S and Fahad S (2022) Evaluating the Impact of Nitrogen Application on Growth and Productivity of Maize Under Control Conditions. Front. Plant Sci. 13:885479. doi: 10.3389/fpls.2022.885479
Received: 28 February 2022; Accepted: 14 April 2022;
Published: 18 May 2022.
Edited by:
Mukhtar Ahmed, Pir Mehr Ali Shah Arid Agriculture University, PakistanReviewed by:
Ayman EL Sabagh, Siirt University, TurkeyZulfiqar Ahmad Saqib, University of Agriculture, Faisalabad, Pakistan
Copyright © 2022 Hammad, Chawla, Jawad, Alhuqail, Bakhat, Farhad, Khan, Mubeen, Shah, Liu, Harrison, Saud and Fahad. This is an open-access article distributed under the terms of the Creative Commons Attribution License (CC BY). The use, distribution or reproduction in other forums is permitted, provided the original author(s) and the copyright owner(s) are credited and that the original publication in this journal is cited, in accordance with accepted academic practice. No use, distribution or reproduction is permitted which does not comply with these terms.
*Correspondence: Hafiz Mohkum Hammad, aGFmaXptb2hrdW1AZ21haWwuY29t; Shah Fahad, c2hhaF9mYWhhZDgwQHlhaG9vLmNvbQ==
†These authors have contributed equally to this work