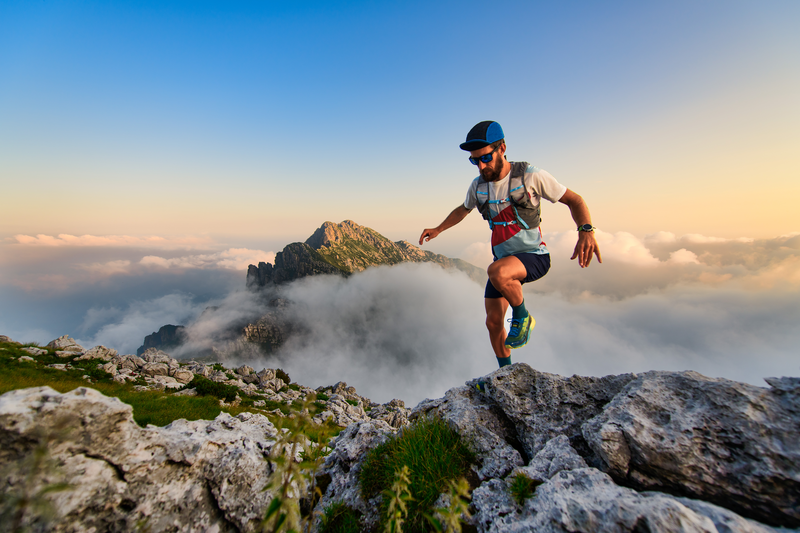
95% of researchers rate our articles as excellent or good
Learn more about the work of our research integrity team to safeguard the quality of each article we publish.
Find out more
REVIEW article
Front. Plant Sci. , 06 June 2022
Sec. Plant Breeding
Volume 13 - 2022 | https://doi.org/10.3389/fpls.2022.883470
This article is part of the Research Topic Insights in Plant Breeding: 2021 View all 6 articles
The formation of gametes with somatic chromosome number or unreduced gametes (2n gametes) is an important process involved in the origin of polyploid plants in nature. Unreduced gametes are the result of meiotic mutations occurring during micro- and mega-sporogenesis. 2n gametes have been identified or artificially induced in a large number of plant species. Breeding of plants through 2n gametes can be advantageous because it combines genetic effects of polyploidy with meiotic recombination and sexual hybridization to produce tremendous genetic variation and heterosis. 2n gametes also occur in ornamental plants, but the potential of using 2n gametes in ornamental plant breeding has not been extensively exploited. Ornamental plants are primarily produced for their esthetic appearance and novelty, not for food and yield, and they can be readily propagated through vegetative means. Triploids, tetraploids, and plants with even higher ploidy levels produced through 2n gametes can be propagated through tissue culture to fix their phenotypes, thus leading to the development of new cultivars. In this review article, we intend to discuss the mechanisms underlying the formation of 2n gametes, techniques for 2n gamete identification, methods for enhancing 2n gamete formation, and the current status in the use of 2n gametes for development of novel ornamental plants. We believe that polyploidy breeding through 2n gametes represents a viable way of developing new cultivars, new species, and even new genera of ornamental plants.
Unreduced gametes are referred to as male or female gametes that have somatic chromosome numbers, thus they are also known as 2n gametes. This phenomenon as an important evolutionary force, however, was not recognized until the early last century. Prior to the 1920s, it was widely accepted that polyploidy in plants resulted from hybridization followed by chromosome doubling (Winge, 1917); Karpechenko (1927) was probably among the first to notice the occurrence of unreduced gametes. He believed that tetraploid Oenothera, Primula, Solanum, and Datura were not from the result of hybridization followed by chromosome doubling. In his study of hybridization between Raphanus and Brassica, Karpechenko (1927) found that hexaploid plants were not derived from doubling of triploid zygotes but caused by unreduced gametes from meiotic failure. The formation of unreduced gametes was further explained by Buxton and Darlington (1931) on amphiploid Digitalis, and they stated that the omission of reduction during the meiosis was the cause of somatic chromosome numbers. Subsequently, unreduced gametes were documented in an increasing number of plant species. Harlan and DeWet (1975) reported that unreduced gametes occurred in 85 plant genera. Recent studies showed that 2n gametes occur in a wide range of plants.
Unreduced gametes occur not only in plants but also in green algae, insects, chickens, mammals, birds, fish, and amphibians and have been considered a primary mechanism for polyploid formation (Mason and Pires, 2015). In plants, polyploids are not blind alleys or evolutionary dead-ends as claimed by Mayrose et al. (2011). Unreduced gametes facilitate polyploid formation and interploidy gene flow in mixed ploidy populations, resulting in increased genetic variation, fitness, heterozygosity, and breeding success. Additionally, 2n gamete formation is an essential component of apomixis Ravi et al., 2008 and an important way for the restoration of F1 hybrid fertility (De Storme and Geelen, 2013). Furthermore, 2n gamete formation generates novel genetic and genomic variation including synthesizing polyploid species, promoting plants to explore new environmental niches, and outcompeting their diploid progenitors.
This article is intended to review the occurrence of 2n gamete in ornamental plants, mechanisms underlying 2n gamete formation, the identification and use of 2n gametes for development of new ornamental cultivars. Ornamental plants are those grown for decoration and beautification of indoor and outdoor environments, not for food; thus, they are valued for their esthetic appearance, not for their yield. A large number of plants are produced as ornamental plants, including floriculture crops, ornamental shrubs, trees, grasses, and bamboos as well as ornamental aquatic plants (Chen, 2021). A significant number of ornamental plants are propagated vegetatively through natural means, such as bulbs, corms, runners, or artificial means like cutting, grafting, layering, or tissue culture. Evidence shows that 2n gametes have played a fundamental role in the development of polyploid cultivars, species, and even genera of ornamental plants, demonstrating the viability of sexual polyploidization in plant evolution and speciation.
Unreduced gametes generally arise from meiotic defects. Meiosis is a process of producing haploid cells during which diploid cells undergo DNA replication, followed by two rounds of cell divisions known as meiosis I and meiosis II (Figure 1). In meiosis I, homologous chromosomes pair with each other and undergo genetic recombination, a process allowing to exchange genetic information through crossover. The homologous chromosomes are then separated, resulting in two haploid cells having half the number of chromosomes as the parental cell, thus meiosis I is a reductional division. Meiosis II resembles mitosis where sister chromatids are separated from each other, producing four cells with reduced chromosome number, this process is known as an equational division. However, meiotic defects can occur, including the omission of the first or second meiotic division, abnormal spindle morphology in the second division, or disturbed cytokinesis (Bretagnolle and Thompson, 1995; Ramanna and Jacobsen, 2003). Meiosis restitution is the predominant mechanism of 2n gamete formation in plants (Brownfield and Kohler, 2011; De Storme and Geelen, 2013). There are three main mechanisms underlying the formation of 2n gametes in plants: first division restitution (FDR), second division restitution (SDR), and indeterminant meiotic restitution (IMR).
Figure 1. Mechanisms underlying the formation of 2n gametes based on the genotypic outcome. For simplicity, the meiotic cell is diploid and only contains two chromosomes that are fully heterozygous (blue and red chromosomes obtained from genetically different parents). WT represents normal meiosis, resulting in four haploid cells. There are two types of first division restitution (FDR): (1) In strict FDR, there was no chromosome pairing and recombination, and chromosomes directly advance to the second division, resulting in 2n gametes that are genetically identical to the parent. (2) In broad FDR, meiosis I is not lost, chromosomes pair and undergo recombination, but the orientation and position of the spindles in meiosis II are disturbed, often being parallel (a), tripolar (b), or fused (c). As a result, the diploid cell is predicted to contain non-sister chromosome (d’Erfurth et al., 2008). In second division restitution (SDR), meiosis I occurs normally with homologous chromosomes pairing and recombination, they divide reductionally followed by cytokinesis to produce a dyad. In meiosis II, however, the centromeres of the half-bivalents divide, but the chromatids do not migrate to the poles, resulting two 2n gametes. Indeterminant meiotic restitution (IMR) shows characteristics similar to FDR and SDR. During the first meiotic division, some bivalents disjoin reductionally as in SDR, while some univalents divide equationally as in FDR.
In FDR, pairing and split-up of homologous chromosomes fail to occur or occur at a low frequency in meiosis I (Tang and Luo, 2002); but the second division proceeds normally, resulting in two sister chromatids of homologous chromosomes to move to opposite poles (Hermsen, 1984). There are two types of FDR: a strict sense and a broad sense (Sun et al., 2021). In the strict sense, there was no pairing and recombination, and chromosomes directly advance to the second division, resulting in 2n gametes that are genetically identical to the parent. Such an FDR fully maintains parental heterozygosity and epistasis. In the broad sense, meiosis I is not lost, chromosomes pair and undergo recombination, but the orientation and position of the spindles in meiosis II are disturbed (d’Erfurth et al., 2008), often being parallel (a), tripolar (b), or fused (c; Figure 1). As a result, the broad-sense FDR produces either two 2n gametes or one 2n gametes with two haploid gametes. In this case, 2n gametes partially retain the parental heterozygosity. However, the occurrence of crossover may increase genetic variation and also allow the introgression of genes of interest in breeding.
In contrast to FDR, the first meiotic division occurs normally in SDR. Homologous chromosomes pair with recombination, and they divide reductionally followed by cytokinesis to produce a dyad. In meiosis II, however, the centromeres of the half-bivalents divide, but the chromatids do not migrate to the poles (Figure 1). The resulting 2n gametes are homozygous from the centromere to the first crossover but maintain parental heterozygosity at the telomeric side (Ramanna and Jacobsen, 2003). As a result, 2n gametes derived from SDR have reduced heterozygosity and show a substantial loss of parental epistasis (Peloquin et al., 2008). In general, SDR is rare in hybrids because all chromosomes are not appropriately paired as bivalents, and it tends to occur only in hybrids with closely related genomes (Ramanna and Jacobsen, 2003). The presence of cytokinesis and the formation of a cell wall after the telophase I is characteristic for most of the monocot plants (Bielig et al., 2003; Boldrini et al., 2006).
The occurrence of IMR was first reported in interspecific hybrids of ornamental lily (Longiflorum × Asiatic lily; Lim et al., 2001). IMR shows characteristics similar to FDR and SDR in which both univalents and bivalents are formed at metaphase I. During the first meiotic division, some bivalents disjoin reductionally as in SDR, while some univalents divide equationally as in FDR (Figure 1), which give rise to 2n gametes with an odd number of parental chromosomes. Unreduced gametes produced in IMR only partially retain parental heterozygosity at the centromere (Zhou et al., 2008).
The origin of 2n gametes has profound effects on breeding of ornamental plants. In general, 2n gametes derived from FDR are more advantageous than those from SDR for transferring parental heterozygosity (Barcaccia et al., 2003; Zhang et al., 2009; Dewitte et al., 2012). In potato, FDR is known to be more than twice as effective as SDR in transmitting parental heterozygosity (Barone et al., 1995; Peloquin et al., 2008). Furthermore, progenies bred by FDR 2n gametes have more vigorous growth due to the higher allelic diversity (Yao et al., 2013). FDR is the basic mechanism of 2n pollen formation in Alstroemeria (Ramanna et al., 2003), Begonia (Dewitte et al., 2010a), Lilium (Lim et al., 2001; Barba-Gonzalez et al., 2008; Zhou et al., 2008; Khan et al., 2010), and Tulipa (Marasek-Ciolakowska et al., 2014). This is probably attributed to the chromosomal composition of FDR gametes that are more balanced and more viable than those from SDR and IMR. Additionally, 2n gametes derived from FDR or IMR with crossovers can increase genetic variation in polyploid progenies as well as the extent of introgressions Barba-Gonzalez et al., 2005. Genomic in situ hybridization (GISH) analysis confirmed the presence of recombinant chromosomes in FDR derived 2n gametes in meiotic polyploids in Lilium (Khan et al., 2009a,b; Xie et al., 2010) and Tulipa (Marasek-Ciolakowska et al., 2012, 2014).
The identification of 2n gametes is largely focused on pollen as it is more convenient to isolate than egg cells. Common methods include pollen size measurements, flow cytometric detection of pollen DNA content, analysis of the microsporogenesis, and ploidy analysis of the progeny (Loginova and Silkova, 2017; Hwang et al., 2020). The identification of 2n eggs is complicated, which is performed by cytological examination using paraffin section, along with the ploidy analysis of the progeny.
The traditional approach for identifying 2n pollen is based on pollen morphology. 2n pollen is commonly known as giant pollen (Bretagnolle and Thompson, 1995), which is defined as a pollen with a diameter greater than 1.5 times that of the normal one. This criterion is based on the assumption that the doubling of DNA content in 2n gametes would approximately double the pollen cell volume (Mason et al., 2011). In ornamental plants of Agave (Gomez-Rodriguez et al., 2012), Begonia (Dewitte et al., 2009), Dianthus (Zhou et al., 2015), Hibiscus (van Laere et al., 2009), and Rosa (Crespel et al., 2006), the diameter of the 2n pollen is about 30% larger than that of the haploid pollen. The presence of large 2n pollen grains results in a bimodal distribution of pollen sizes instead of a normal distribution (De Storme and Geelen, 2011). Although the size distribution between the pollen grains sometimes overlaps, a threshold value of the pollen grain size is often used to select individuals that produce 2n gametes (Sugiura et al., 2000; Crespel et al., 2006). However, caution should be given when using this method to guide 2n gamete identification because giant pollen does not necessarily prove doubled DNA content. Another disadvantage of this screening technique is the broad overlap in size distribution between small and large pollen in some genera, such as grasses. In these cases, the frequency of 2n pollen based on size is difficult to determine. Thus, other methods should be used to confirm the association between giant pollen and 2n pollen, and supplementary evaluation of pollen viability is necessary for breeding purposes.
Flow cytometry has been widely used to measure pollen nuclear DNA content in order to understand pollen development and detect the presence of 2n pollen (Bino et al., 1990; Dewitte et al., 2009; van Laere et al., 2009; Zhu et al., 2014; Zhang et al., 2021). Estimating male 2n gametes with flow cytometry entails extracting nuclei from a large number of pollen grains, staining them with a DNA-selective fluorochrome, and generating fluorescence histograms with peaks corresponding to groups of nuclei with different DNA content. Flow cytometric analysis compares the DNA content of pollen nuclei to the DNA content of somatic leaf tissue. Pollen nuclei are expected to have only half of the DNA content (1C) compared to nuclei from somatic cells (2C) of the same plant. Consequently, 2n pollen have a nuclear DNA content equal to that somatic cells.
The occurrence in 2n pollen is associated with the presence of monads, dyads, or triads during microsporogenesis (Negri et al., 1995; Fernandez et al., 2010; Chung et al., 2013; Nakato and Masuyama, 2021; Xu et al., 2021; Zhang et al., 2021) except 2n gamete formation that is the result of pre- or post-meiotic restitution. Analysis of microsporogenesis may therefore provide an alternative method to identify 2n pollen, but this method does not provide any information about pollen viability. Cytological staining is carried out with dyes, such as acetocarmine, aceto-orcein, or fuscin, resulting in the visualization of chromatins. With the advent of a new cytological methods, immunostaining, in combination with the use of propidium iodide (PI) and 4’,6’-diamidino-2-phenylindol and spindle (anti-α-tubulin antibodies), cellular components involved in the division can be more accurately determined. Recently, immunostaining using antibodies to phospho-histone H3 (Ser10), which is characterized by localization along the entire chromosome length in the first meiotic division and only in the centromeric region in the second division, has made it possible to distinguish the stages of meiotic division (Loginova and Silkova, 2016).
Ploidy analysis of progenies (usually using flow cytometry) can reveal the presence of 2n gametes in parent plants. This method has been used frequently in ornamental plant breeding. In breeding of Hibiscus, five F2 hexaploid plants were isolated from self-pollinated hybrids of tetraploid F1, which was developed from the cross between H. syriacus “Oiseau Bleu” (4x) and H. paramutabilis (4x). The occurrence of hexaploidy indicated that the F1 hybrids must produce unreduced eggs since no unreduced pollen could be detected in the F1 hybrids (van Laere et al., 2009); Zhou et al. (2017) identified seven tetraploid hybrid plants from 12 progenies obtained from five crossing combinations between a tetraploid Dianthus caryophyllus “Butterfly” and diploid cultivars, suggesting that 2n male gametes were involved in polyploid formation. In lily breeding, four odd-allotetraploid seedlings were obtained from an interploidy cross, Lilium LA × AAAA. This result implied that the intergenomic variation was caused by 2n eggs, which was confirmed by GISH (Xiao et al., 2021). Progeny analysis, however, is time consuming with no guarantee of information about the production frequency of 2n gametes in the parental plants (Bretagnolle and Thompson, 1995) due to the differences in pollen viability, germination speed, or pollen tube growth between haploid and 2n pollen.
The use of molecular cytological techniques, such as GISH and fluorescent in situ hybridization in combination with marker analysis, such as amplified fragment length polymorphism on meiocytes or polyploid progeny provides more accurate or additional information on the mechanisms behind 2n gamete formation (Barba-Gonzalez et al., 2005; Chung et al., 2013; Zhang et al., 2021). Molecular cytological approaches have been successfully used in the case of allopolyploids, where the constituent genomes can be clearly discriminated (Xi et al., 2015). Through DNA in situ hybridization, genomes of allopolyploids can be more critically assigned and intergenomic translocations and recombination can be detected, which has been used in Gasteria-Aloe hybrids (Takahashi et al., 1997), Alstroemeria species (Ramanna et al., 2003), and Lilium species (Karlov et al., 1999; Lim et al., 2001; Barba-Gonzalez et al., 2005). As such, GISH can also be used to discover the mechanism of 2n gamete formation (Karlov et al., 1999).
Unreduced gametes can occur naturally via the mechanisms described above and artificially through the manipulation of environmental conditions or use of specific chemicals. Thus, the natural occurrence is the formation of 2n gametes spontaneously without artificial intervention. Up to now, naturally occurring unreduced gametes have been reported in more than 40 genera across 60 species and hybrid progenies of ornamental plants (Table 1), and artificially induced 2n gamete formation has been reported in at least 10 genera of ornamental plants (Table 2).
Naturally occurring 2n gametes are mainly derived from two major sources: interspecific or intergeneric hybrids and odd-polyploids (Bretagnolle and Thompson, 1995). Interspecific and intergeneric hybrids have a greater chance to produce 2n gametes at a higher frequency than their parents (Ramsey and Schemske, 1998; Ramanna et al., 2003). The frequency of 2n male gamete formation in traditional cultivars of Cymbidium ranged from 0.5 to 1.0% but 2.5 to 4.03% in interspecific hybrids (Zeng et al., 2020). Unreduced gametes were produced in interspecific or intergeneric hybrids of Alstroemeria (Ramanna et al., 2003), Cyclamen (Ishizaka, 1998), Cymbidium (Zeng et al., 2020), Hibiscus (van Laere et al., 2009), Impatiens (Stephens, 1998), Lilium (Lim et al., 2001, 2004; Barba-Gonzalez et al., 2005), and red clover (Trifolium; Meredith et al., 1995; Table 1). Cytologically, interspecific hybrids either show no chromosome pairing or have abnormal pairing and the presence of lagging chromosomes, chromosome bridges, or univalent in meiosis (Trojak-Goluch and Berbec, 2003; Dewitte et al., 2012; Crespel and Morel, 2014; Wang et al., 2015). Two important features have been reported in 2n gamete formation among interspecific hybrids. First, 2n eggs and 2n pollen could be simultaneously produced by the same hybrid. Second, neither the two parents of the F1 hybrids nor their (F2) sexual polyploid progenies could produce 2n gametes in any notable frequencies (Ramanna and Jacobsen, 2003).
The second source for frequently producing 2n gametes is odd polyploids. Old polyploids are characterized by their karyotypic, genomic, and reproductive instability. As a result, their meiosis may produce gametes with different levels of ploidy, including 2n gametes. For example, triploid lily is generally sterile but can be used as a female parent to produce fertile progenies. In this case, there is an occurrence of 2n gametes. Thus, odd polyploids have been considered a source for 2n gamete formation, and triploids have been used as a bridge between diploids and tetraploids to produce higher polyploids (Köhler et al., 2010).
Unreduced gametes can be induced by manipulation of environmental factors, temperature in particular and treatment with chemicals, such as nitrous oxide (N2O), trifluralin, colchicine, and oryzalin (Table 2). The treatments, depending on the magnitude, may cause meiosis abnormalities in microspore mother cell, including chromosome separation failure (chromosomal adhesion or backward chromosomes) and spindle abnormalities (parallel, fused, and tripolar spindles; Li et al., 2016; Liao et al., 2016b; Wang et al., 2017b). Of these, parallel and fused spindles, and premature cytokinesis result in the formation of dyads, and the tripolar spindles create the triads during the tetrad period (Wu et al., 2011). These abnormalities could lead to the occurrence of 2n pollen.
Either high or low temperatures have been shown to triggers 2n gamete production (Mason et al., 2011; Pecrix et al., 2011; De Storme et al., 2012; Crespel et al., 2015; Zhou et al., 2015; Li et al., 2016; Wang et al., 2017b; Mai et al., 2019); Lokker et al. (2005) grew four complete sterile lily genotypes in a phytotron with an extreme temperature fluctuation regime: four alternating periods of 10 and 30°C each day for 6 weeks and found that three of the four genotypes became fertile as evidenced by the production of viable 2n gametes. Pecrix et al. (2011) observed that the frequency of 2n pollen in Rosa plants after exposure to a high temperature gradient was up to 24.5% compared to the control treatment at 24°C. The 2n pollen mainly resulted from temperature-induced spindle mis-orientations in meiosis II.
Low-temperature treatment can also induce 2n gamete formation. A short period of cold stress at 4–5°C induced the production of diploid and polyploid pollens in Arabidopsis (De Storme et al., 2012). In Datura and Achillea, the frequency of 2n pollen formation was higher at low temperatures (Ramsey and Schemske, 1998; Ramsey, 2007); Mason et al. (2011) demonstrated that cold stress significantly stimulated 2n pollen production in B. napus × B. carinata interspecific hybrids. Zhang et al. (2019b) showed that low temperatures increased the frequency of SDR-type 2n female gametes in the diploid rubber clone GT1.
Colchicine, oryzalin, trifluralin, N2O, and amiprophos-methyl have been commonly used for inducing polyploids and also 2n pollen formation (Younis et al., 2014). Colchicine has been used for inducing 2n pollen of Begonia, Dianthus, Tulipa, Lilium, and other ornamental plants (Okazaki et al., 2005; Akutsu et al., 2007; Wu et al., 2007; Sato et al., 2009; Dewitte et al., 2010a; Lai et al., 2015; Yang et al., 2016). N2O can inhibit microtubule polymerization, but not actin filament formation. It was reported that N2O effectively induced 2n gametes (both 2n pollen and 2n egg) in Tulipa (Okazaki et al., 2005), Lilium (Barba-Gonzalez et al., 2006; Akutsu et al., 2007), and Begonia (Dewitte et al., 2010a). N2O as a gas can readily penetrate tissue, thereby protecting the tissues from harmful aftereffects as soon as the gas is released (Ostergren, 1954; Kato and Geiger, 2002); Akutsu et al. (2007) showed that effects of N2O were optimal when treatments started during pollen mother cell progression to metaphase I. Using this technique, fertile 2n gametes were induced from sterile hybrids, but the efficiency of the treatment was genotype specific (Barba-Gonzalez et al., 2006; Dewitte et al., 2010b).
With the advance in molecular biology, genes specifically responsible for 2n gamete formation have been increasingly identified. An Arabidopsis gene DYAD/SWITCH1 (SWI1) was found to be responsible for the production of 2n female gametes, resulting in progenies with triploid plants. AtPS1 (Arabidopsis thaliana parallel spindle 1) is another gene involved in 2n gamete formation in Arabidopsis (d’Erfurth et al., 2008). AtPS1 mutants produce up to 65% of 2n pollens, pollination with the pollen resulted in a large number of triploid plants in the next generation. ASMC5/6 (structural maintenance of chromosome 5/6) complex has been identified to be a crucial factor for preserving genome stability (Yang et al., 2021). SMC5/6 mutants show an absence of chromosome segregation during the first and/or second meiotic division, producing 2n gametes. A comparison of the well-established meiotic mutants in alfalfa with the genes identified from Arabidopsis showed that nine proteins belonging to A. thaliana known for their involvement in 2n gamete production occurred in alfalfa, suggesting common lineage of genes implicated in 2n gamete formation (Palumbo et al., 2021). Molecular techniques, particular CRISPR/Cas9 could be used for engineering plants with increased production of either 2n pollen or 2n eggs and used for breeding of novel polyploid ornamental plants.
A large number of polyploid ornamental cultivars have been developed through the use of 2n gametes (sexual polyploidization). Table 3 lists some of those across 21 genera, which can be summarized as follows: (1) triploids developed from the cross of diploid × diploid in Cymbidium, Hevea brasiliensis, Lilium, Phalaenopsis, Populus, Rosa, Vaccinium, and Zantedeschia; (2) tetraploids derived from the cross of diploid × diploid, diploid × tetraploid, or tetraploid × diploid in Alstroemeria, Calluna vulgaris, Chrysanthemum, Cymbidium, Dianthus, Lilium, Morus alba, Petunia hybrida, Phalaenopsis, Phegopteris, Rosa, Primula, Pyrus, Ranunculus cantoniensis, Trifolium pretense, and Zantedeschia; (3) pentaploids produced from the cross of diploid × triploid, triploid × diploid, tetraploid × diploid, tetraploid × triploid, or diploid × hexaploid in Fragaria, Lilium, Phalaenopsis, Rosa, Primula, and Phegopteris; (4) hexaploids obtained from the cross of diploid × tetraploid, tetraploid × diploid, tetraploid × tetraploid, triploid × triploid, or diploid × octaploid in Fragaria, Phalaenopsis, Primula, and Phegopteris; and (5) octaploids selected from the cross of tetraploid × tetraploid in Primula. Additionally, sexual triploids of Hevea (Zheng et al., 1983), Populus (Zhang et al., 1992; Li et al., 1994; Kang et al., 2000; Guo et al., 2017), and Zantedeschia (Wu et al., 2011), as well as tetraploids of Petunia (Cai et al., 2020) and Lilium (Barba-Gonzalez et al., 2004) were also successfully developed using artificially induced 2n gametes.
Polyploidization through 2n gametes represents a new trend in breeding of ornamental plants (Ramanna et al., 2012; Marasek-Ciolakowska et al., 2021). This is mainly due to the following factors: (1) Ornamental plants are prized by their novelty and esthetic appearance, including flower shape, size, and color; leaf shape, texture, color, and size; plant overall growth form and growth vigor (Henny and Chen, 2003). They are not cultivated for grain production; thus, there is little concern whether or not they are poor in seed production or sterile due to triploid block. Plants with unique phenotypes can be effectively propagated asexually using tissue culture technique to immediately increase the number of plants for commercial production. (2) An increasing number of ornamental plant species has been found to produce 2n gametes (Table 1). The frequency of 2n gamete occurrence typically ranges from 0.1 to 2.0%, but it could be much higher up to 10% in interspecific hybrids (Kreiner et al., 2017a,b; Sun et al., 2021). Many ornamental plants are actually interspecific hybrids (Kato and Mii, 2012). The higher frequencies offer a unique opportunity for breeders to manipulate chromosomes and develop new cultivars of ornamental plants (Table 3), which is described in the following subsections. (3) Polyploid plants, particularly those developed via 2n gametes generally have increased organ size, robust growth form, and improved tolerance to abiotic and biotic stresses. Sexual polyploidization using 2n gametes allows the introgression of desirable traits in interspecific breeding and results in genetic heterozygosity and heterosis (Barba-Gonzalez et al., 2004, 2005, 2006). Although polyploidization can be attained through mitotic chromosome doubling (Eng and Ho, 2019; Niazian and Nalousi, 2020), this approach does not result in introgression breeding due to the lack of intergenomic recombination. (4) The ornamental plant industry is a fast-growing sector in world agriculture (Chen, 2021). Ornamental plants represent the sixth largest agricultural commodity group in the United States European countries, such as Netherlands produces a large quantity of diverse floriculture crops. The ornamental plant industry in China is blooming. The total turnover for floriculture crops (excluding ornamental trees and shrubs and ornamental grasses and bamboo) was estimated to be $300 billion (Azadi et al., 2016). A key driving force for the continuous growth of the ornamental plant industry is the demand for new cultivars with novel esthetic value (Henny and Chen, 2003; Noman et al., 2017). Thus, polyploidization through 2n gametes has been increasingly used for improving ornamental plants, and this is particularly true in bulbous and orchid crops. Most single and double flowered cultivars of Hippeastrum on the market are tetraploid. The majority of modern intersectional cultivars of Lilium are triploids, and some commercial ones are aneuploids. In the genus Narcissus, nearly 75% of cultivars are tetraploid, but only 12% each for diploid and triploid cultivars. Many tulip, chrysanthemums, and cultivated orchids are polyploid. Most modern commercially valuable rose cultivars are tetraploids. In fact, the availability of these neopolyploids is largely attributed to the functionality of 2n gametes used in breeding, which are briefly discussed as follows:
Developing new cultivars through interploidy crosses is often difficult due to the difference in ploidy levels. However, the occurrence of 2n gametes can greatly facilitate interploidy crosses, resulting in the development of new polyploid cultivars. The interploidy crosses include 2x × 4x, 4x × 2x, or 2x × 3x. Hybridization of tetraploids with diploids or vice versa produced triploid semperflorens Begonia and Begonia rex (Horn, 2004; Marasek-Ciolakowska et al., 2016) as well as triploids Lilium (Lim et al., 2003; Zhou et al., 2008) and Tulipa (Kroon and Van Eijk, 1977; van Scheepen, 1996). Triploid Aloineae (Brandham, 1982), Dactylis (Jones and Borrill, 1962), Lilium (Lim et al., 2003), Primula (Hayashi et al., 2009), and Tulipa (Marasek-Ciolakowska et al., 2014) crossed with tetraploid counterparts resulted in pentaploid plants, respectively. Hydrangea macrophylla is one of the most economically important ornamental crops worldwide, with United States sales of Hydrangea species topping $120 million in 2014. A recent study showed that diploid (2n = 2x = 36), triploid (2n = 3x = 54), tetraploid (2n = 4x = 72), and even aneuploid H. macrophylla are most fertile and produce viable offspring in interploidy crosses. Triploid and tetraploid offspring can be produced by hybridization of diploid with diploid individuals or by crossing diploid with tetraploid plants, and even crossing triploids with either diploid or tetraploid plants. Such interploidy crosses are due to production of unreduced gametes (Trankner et al., 2020). Triploid hydrangeas have thicker stems, large flowers, and larger stoma compared to full-sibling diploids. These findings explained the origin of triploid hydrangeas and also why there are more triploid cultivars are on the market than diploid cultivars (Alexander, 2020).
The availability of 2n gametes facilitates interspecific and intergenic hybrid development in ornamental plants. The aims of such hybridizations are to broaden genetic variability or transfer valuable traits, such as disease resistance and novel ornamental characteristics for developing new cultivars. Begonia is one of the largest genera of floriculture crops with more than 2,000 species. It has been divided into several groups based on the origin and growth characteristics. Among them, Elatior-hybrids represent about 88% of the total begonia production (Haegeman, 1979; Kroon, 1993). Most “Elatior” begonia were developed from crosses between different tuberous hybrid species (B × tuberhydrida) and B. socotrana (Marasek-Ciolakowska et al., 2016). Both spontaneous and induced 2n gametes have played important role in the interspecific hybrid development. For example, most “Elatior” hybrids are triploids, and a few are tetraploids (Marasek-Ciolakowska et al., 2016). The occurrence of 2n pollen was common in Begonia with a frequency varied from 1% in Begonia “Rubaiyat” to 100% in “Florence Rita” and B276 (Dewitte et al., 2009), of which FDR was the major mechanism underlying the 2n pollen formation (Dewitte et al., 2010a,b).
Tulip (Tulipa L.) is one the most popular bulbous crops, and its breeding has been aimed at the introgression of new flower colors and shapes, flower longevity, resistance to tulip breaking virus (TBV), Botrytis tulipae, and Fusarium oxysporum into commercial cultivars (Marasek-Ciolakowska et al., 2016). Interspecific crosses were made between T. gesneriana and T. fosteriana (TBV resistant) resulting in a series of cultivars including Darwin hybrids that are highly resistant to BVT virus (van Eijk et al., 1991; van Raamsdonk et al., 1995). More than 50 Darwin hybrid cultivars were developed (van Scheepen, 1996), which were largely derived from sexual polyploidization. This is because some Darwin triploids were fertile and could be backcrossed to T. gesneriana, and some F1 Darwin hybrids could produce 2n and haploid gametes, allowing the generation of polyploids (Marasek-Ciolakowska et al., 2016).
The occurrence of 2n gametes has also led to the formation of new species and new genera. Classical examples are tetraploid species of Tragopogon mirus and T. miscellus in the sunflower family (Soltis and Soltis, 2009). T. mirus (2n = 4x = 24) was derived from the cross of diploid T. dubius with diploid T. porrifolius (2n = 2x = 12), while T. miscellus (2n = 4x = 24) was developed from the cross of T. dubius with diploid T. pratensis. The underlying mechanism for the formation of the two species was explained by unreduced gametes produced by the diploid parents. An early example of synthesized genus is × Aranda orchids (Lee and Tham, 1988). This genus represents a group of intergenic hybrids developed from crosses between Vanda (2n = 2x = 38) and Arachnis (2n = 2x = 38). The initial hybrids (F1) of the two genera had 2n = 2x = 38 but were sterile. However, some of the hybrids produced 2n gametes at a rate up to 10%, and they were fertile as maternal parents. Backcross with either Vanda or Arachnis resulted in × Aranda hybrids with chromosome of 2n = 3x = 57. The vanda parents provide flower color and shape with the stacked strap leaves, and arachnis parents contributes curved, thin petals and fast growth characteristic. They have been widely used as cut flowers due to their vigorous growth and very abundant flowers. More than 200 such hybrids were developed prior to 1985.
There are two types of polyploidy: autopolyploids and allopolyploids. The former display polysomic inheritance, and the latter in most cases show disomic inheritance. In general, allopolyploid plants show higher heterozygosity and heterosis. Lily as one of the most important floriculture crops with four popular genomes: Asiatic (A genome), Longiflorum (L genome), Oriental (O genome), and Trumpet (T genome; van Tuyl and Arens, 2011). Using 2n gametes, along with cut style pollination and embryo rescue, LA, OA, LO, and OT hybrids were developed (Asano and Myodo, 1977; van Tuyl et al., 2000). By somatic chromosome doubling, allotetraploid hybrids of LALA, OAOA, LOLO, OTOT, and LTLT were produced (Xiao et al., 2021). As 2n gametes occurs in those F1 hybrids, interploidy crosses of 2x × 4x produced LAA, OTO, LOO, and AOA cultivars. Additionally, three odd-allotetraploid cultivars, namely Honesty (LAAA; Zhou et al., 2013; Xiao et al., 2019), Original Love (LAAA; Yang et al., 2019; Zheng, 2019), and Santa Rosa (LLLO; Zhang et al., 2012) were developed. Since LA can produce a small number of 2n egg, “Honesty” was developed from a cross of LA × AAAA. LALA can produce a large number of 2n gametes, “Original Love” was selected from the cross of LALA × AAAA. “Santa Rosa” was derived from the cross of LOLO × LLLL or vice versa. Most functional 2n gametes were formed through FDR, and a few were derived from IMR. Compared to diploid, triploid, and other tetraploid plants, the odd-allotetraploid cultivars have taller and stronger stems, produce more bulbils, and resist diseases (Xiao et al., 2021). As 2n gametes are largely produced through FDR, the heterosis is probably attributed to intergenomic differences in the hybrids. Allopolyploidy can confer additional advantages: novel genetic variation, and phenotypes different to the parent species can be produced through transgressive segregation and allelic heterosis.
Triploid plants can be recovered from the cross of 2x × 2x, 2x × 4x, 4x × 2x, or 2x × 3x, of which the cross of one parent that produces 2n gametes with another diploid parent is one of the most common practices (Wang et al., 2016). Studies showed that 2n gametes produced by a female parent plays important role in the successful formation of triploid plant (Ramsey and Schemske, 1998), and this is in part attributed to the appropriate endosperm balance number (Lu et al., 2013). Many triploid cultivars of Narcissus (Brandham, 1986), Lilium (Noda, 1986), Crocus (Ørgaard et al., 1995), and Tulipa (van Scheepen, 1996; Marasek et al., 2006) were developed from unreduced gametes of diploid parents. The early cultivars from the subgenus Narcissus were diploid, from which triploid cultivars arose in the latter half of 19th century due to the occurrence of 2n gametes. Subsequently, tetraploid Narcissus were developed by the end of the 19th century (Brandham, 1986). Tulips are important bulbous flowering plants with more than 8,000 cultivars in the market. Among them, Darwin hybrids represent an important group of cultivars grown for cut flowers, and they are triploids (2n = 3x = 36) developed from interspecific cross of Tulipa gesneriana (2n = 2x = 24) and T. fosteriana (2n = 2x = 24). GISH and median chromosome analyses showed that 24 chromosomes were derived from T. gesneriana and 12 chromosomes were from T. fosteriana (Marasek et al., 2006), suggesting that the one of the most popular groups of tulips, Darwin triploid hybrids were developed through 2n gametes derived from T. gesneriana.
A distinct characteristic of triploid plants is their sterility, known as triploid block, this is a phenomenon resulting in the formation of non-viable progeny after hybridization of plants with different ploidy. This is mainly due to the unbalanced meiotic chromosome segregation and endosperm imbalance (Köhler et al., 2010; Wang et al., 2017a). In ornamental plant breeding, triploid plants can be maintained through vegetative means, such as bulbous propagation in Crocus, Lilium, Narcissus, and Tulipa as well as micropropagation through tissue culture. Triploid ornamental plants generally have higher growth vigor, large flower size, sturdier stem, broader and thicker leaves, or more compact plants compared to their diploid progenitors because the energy that is normally devoted to seed production is used for the growth of flowers and other organs (Miyashita et al., 2009; Tiku et al., 2014). Furthermore, the sterility could be particularly useful for reducing the invasiveness of some ornamental plants. Some important ornamental plants are classified as invasive, as their seed production and dispersal by birds and other means could result in potential colonization of natural habitats that break the balance of native flora (Li et al., 2004). For example, Lantana camara is a popular ornamental plant but is considered an invasive species because its pollen can hybridize with an endangered relative L. depressa in Florida. Studies showed that triploid cultivars of L. camara had lowest pollen stainability at 9.3% compared to 64.6% in diploid and 45.1% in tetraploid cultivars (Czarnecki et al., 2014). Meanwhile, 2n female gametes were found to produce in diploid, triploid, and tetraploid cultivars (Czarnecki and Deng, 2009). Thus, the authors acknowledge that to develop triploid lantana, appropriate parental plants should be carefully selected (Czarnecki et al., 2012).
Triploid sterility, however, may not be completely correct. Increasing evidence shows that many triploids can be used as male or female parent in cross breeding programs (Lim et al., 2003; Zhou et al., 2008; Hayashi et al., 2009; Nakato and Masuyama, 2021). Pentaploids and hexaploids were produced by using of triploid as the parents in Phalaenopsis and Primula. In Phalaenopsis, no hybrids were produced from the cross of triploid × triploid; however, hexaploid was obtained from the self-pollinated progeny of triploid P. decursivepinnata. Numerous reports conform that triploid lily is usually sterile and can be used as a female parent to cross with suitable male parents (Lim et al., 2003; Barba-Gonzalez et al., 2006; Khan et al., 2009b; Xie et al., 2010; Zhou et al., 2011, 2012, 2014; Chung et al., 2013; Xi et al., 2015; Dhiman et al., 2019); Cui et al. (2022) showed that all triploid lilies are partially fertile when used as female parents even they are completely sterile as male parents. The triploid lilies as female parents can be used to cross with appropriate diploid or tetraploid males to produce aneuploid cultivars.
Sexual polyploidization has significantly advanced ornamental plant breeding, resulting in a variety of novel cultivars in the market, including those from Alstroemeria, Begonia, Chrysanthemum, Cymbidium, Lilium, Phalaenopsis, Tulipa, and others. These cultivars have either unique or larger flowers, different colors, robust growth form, and resistance to different abiotic and biotic stresses. Furthermore, sexual polyploidization has resulted in not only new cultivars, but also new species and new genera, accelerating plant speciation. More than 40 years ago, Roose and Gottlieb (1976) demonstrated that T. mirus and T. miscellus, two relatively new allotetraploid ornamental plant species had combined allozyme profiles of the diploid parents (T. dubius and T. porrifolius for T. mirus; T. dubius and T. pratensis for T. miscellus). This report documented the link between genotype and biochemical phenotype as well as enzyme additivity. Now, we are in the age of genomics, polyploidization, particularly through the 2n gamete route, can cause genomic rearrangement (Soltis et al., 2004), changes in gene content and gene number, alternation in gene expression in combination with actions of transposal elements, small RNAs, and epigenetic regulation (Moghe and Shiu, 2014; Li et al., 2019; Williams, 2021). It is truly believed that such changes and interactions will result in different gene expression profiles, metabolism alternation, and morphology differences. With the art of selection, new cultivars and new plants will be developed. We are not concerned about the evolutionary dead-end of polyploidy, rather we are pursuing a better understanding how polyploidization has rearranged the genetic makeup of plants, how the changes alter gene expression, and subsequently phenotypic variation, and how we can better use 2n gametes as a means for developing novel plants and new cultivars.
The exploitation of 2n gametes creates a plethora of opportunities for practical breeding in ornamental plants. Spontaneous production of 2n gametes was found in more than 211 accessions belonging to 37genera, 25 families in ornamental plants. The occurrence frequency of 2n gametes ranges from 0.03 to 100.00%, depending on genetic and environmental factors. In general, the occurrence percentage of 2n gamete in interspecific or intergeneric hybrids was higher than traditional cultivars, but not all of the hybrids produce more 2n gametes. Both diploids and polyploids can produce 2n gametes, which can be used for producing polyploids with different ploidy levels. Triploids are generally thought to be an evolutionary dead-end, but in practice, they can be used as either the male or female parent in a cross-breeding program to produce sexual polyploids. 2n gametes can also be artificially induced by treatment with colchicine, N2O, and trifluralin and by manipulation of temperature. Artificial productions of 2n gametes are successfully achieved in 61 accessions belonging to 10 genera, nine families with the occurrence frequency ranging from 0.1 to 100%. Triploid, tetraploid, pentaploid, hexaploidy, and octaploid ornamental plants were created by the use of 2n gametes. Information gathered from this review shows that polyploid breeding with 2n gametes is an efficient and reliable method for ornamental plant breeding. With ongoing research at the molecular level and research toward efficient methods for inducing 2n gametes, the importance of 2n gametes for ornamental plant breeding will continue to increase in the future.
LX, JC, and Z-SZ conceived the idea, edited and refined the manuscript. L-ZK and X-QL conducted literature search and wrote the initial draft. All authors read, corrected, and approved the manuscript.
This study was supported in part by the Science and Technology Plan of Guangzhou with the project number of 202002030068 and Special Project of Agriculture Science Independent Innovation of Guangzhou Agricultural Bureau, China (21102422).
The authors declare that the research was conducted in the absence of any commercial or financial relationships that could be construed as a potential conflict of interest.
All claims expressed in this article are solely those of the authors and do not necessarily represent those of their affiliated organizations, or those of the publisher, the editors and the reviewers. Any product that may be evaluated in this article, or claim that may be made by its manufacturer, is not guaranteed or endorsed by the publisher.
We would like to thank Terri A. Mellich for critical review of this manuscript.
Abd El-Twab, M. H., and Kondo, K. (2007). Rapid genome reshuffling induced by allopolyploidization in F1 hybrid in Chrysanthemum remotipinnum (formerly Ajania remotipinna) and Chrysanthemum chanetii (formerly Dendranthema chanetii). Chromosome Bot. 2, 1–9. doi: 10.3199/iscb.2.1
Akutsu, M., Kitamura, S., Toda, R., Miyajima, I., and Okazaki, K. (2007). Production of 2n pollen of Asiatic hybrid lilies by nitrous oxide treatment. Euphytica 155, 143–152. doi: 10.1007/s10681-006-9317-y
Alexander, L. (2020). Ploidy level influences pollen tube growth and seed viability in interploidy crosses of Hydrangea macrophylla. Front. Plant Sci. 11:100. doi: 10.3389/fpls.2020.00100
Asano, Y., and Myodo, H. (1977). Studies on crosses between distantly related species of lilies. II. The culture of immature hybrid embryos. J. Japan. Soc. Hort. Sci. 46, 267–273. doi: 10.2503/jjshs.46.267
Azadi, P., Bagheri, H., Nalousi, A. M., Nazari, F., and Chandler, S. F. (2016). Current status and biotechnological advances in genetic engineering of ornamental plants. Biotechnol. Adv. 34, 1073–1090. doi: 10.1016/j.biotechadv.2016.06.006
Barba-Gonzalez, R., Lim, K. B., Ramanna, M. S., Visser, R. G. F., and van Tuyl, J. M. (2005). Occurrence of 2n gametes in the F1 hybrids of oriental × Asiatic lilies (Lilium): relevance to intergenomic recombination and backcrossing. Euphytica 143, 67–73. doi: 10.1007/s10681-005-2657-1
Barba-Gonzalez, R., Lim, K. B., Zhou, S., Ramanna, M. S., and Van Tuyl, J. M. (2008). “Interspecific hybridization in lily: the use of 2n gametes in interspecific lily hybrids,” in Floriculture, Ornamental and Plant Biotechnology, Vol. V, ed. J. A. Teixeira da Silva (London: Global Science Books), 138–145.
Barba-Gonzalez, R., Lokker, A. C., Lim, K. B., Ramanna, M. S., and van Tuyl, J. M. (2004). Use of 2n gametes for the production of sexual polyploids from sterile Oriental × Asiatic hybrids of lilies (Lilium). Theor. Appl. Genet. 109, 1125–1132. doi: 10.1007/s00122-004-1739-0
Barba-Gonzalez, R., Miller, C. T., Ramanna, M. S., and van Tuyl, J. M. (2006). Nitrous oxide (N2O) induces 2n gametes in sterile F-1 hybrids between oriental x Asiatic lily (Lilium) hybrids and leads to intergenomic recombination. Euphytica 148, 303–309. doi: 10.1007/s10681-005-9032-0
Barcaccia, G., Tavoletti, S., Mariani, A., and Fabio, V. (2003). Occurrence, inheritance and use of reproductive mutants in alfalfa improvement. Euphytica 133, 37–56. doi: 10.1023/A:1025646523940
Barone, A., Gebhardt, C., and Frusciante, L. (1995). Heterozygosity in 2n gametes of potato evaluated by RFLP markers. Theor. Appl. Genet. 91, 98–104. doi: 10.1007/BF00220864
Belling, J., and Blakeslee, A. F. (1923). The reduction division in haploid, diploid, triploid and tetraploid Daturas. Proc. Natl. Acad. Sci. U.S.A. 9, 106–111. doi: 10.1073/pnas.9.4.106
Bielig, L. M., Mariani, A., and Berding, N. (2003). Cytological studies of 2n male gamete formation in sugarcane Saccharum L. Euphytica 133, 117–124.
Bino, R. J., Van Tuyl, T. J. M., and De Vroes, J. N. (1990). Flow cytometric determination of relative nuclear DNA contents in bicellulate and tricellulate pollen. Ann. Bot. 65, 3–8. doi: 10.2307/2444802
Boldrini, K. R., Pagliarini, M. S., and DoValle, C. B. (2006). Abnormal timing of cytokinesis in microsporogenesis in Brachiaria humidicola (Poaceae: Paniceae). J. Genet. 85, 225–228. doi: 10.1007/BF02935337
Brandham, P. E. (1982). Inter-embryo competition in the progeny of autotriploid Aloineae (Liliaceae). Genetica 59, 29–42. doi: 10.1007/bf00130812
Brandham, P. E. (1986). Evolution of polyploidy in cultivated Narcissus subgenus Narcissus. Genetica 68, 161–167. doi: 10.1007/BF02424439
Bretagnolle, F., and Thompson, J. D. (1995). Gametes with the somatic chromosome number: mechanisms of their formation and role in the evolution of autopolyploid plants. New Phytol. 129, 1–22. doi: 10.1111/j.1469-8137.1995.tb03005.x
Brownfield, L., and Kohler, C. (2011). Unreduced gamete formation in plants: mechanisms and prospects. J. Exp. Bot. 62, 1659–1668. doi: 10.1093/jxb/erq371
Buxton, B. H., and Darlington, C. D. (1931). Behaviour of a new species, Digitalis mertonensis. Nature 127:94. doi: 10.1038/127094b0
Cai, S. Y., Wei, Y., She, H. L., Zhang, Y., Wei, W., and Lu, J. Q. (2020). Study on creation of tetraploid Petunia hybrida by sexual polyploidization. Southern Hort. 31, 12–16.
Cao, Y., Huang, L., Li, S., and Yang, Y. (2002). Genetics of ploidy and hybridized types for polyploidy breeding in pear. Acta Hort. 587, 207–211. doi: 10.17660/actahortic.2002.587.24
Chambers, K. L. (1955). A biosystematic study of the annual species of Microseris. Contrib. Dudley Herb. 4, 207–312.
Chen, J. (2021). Ornamental plant research inaugural editorial. Ornam. Plant Res. 1, 1–2. doi: 10.48130/opr-2021-0001
Chung, M. Y., Chung, J. D., Ramanna, M., van Tuyl, J. M., and Lim, K. B. (2013). Production of polyploids and unreduced gametes in Lilium auratum x L. henryi hybrid. Int. J. Biol. Sci. 9, 693–701.
Clausen, J., Keck, D. D., and Hiesey, W. M. (1945). Experimental Studies on the Nature of Species II. Plant Evolution Through Amphiploidy and Autopolyploidy, with Examples from the Madiinae. Washington, DC: Carnegie Institute Washington.
Crespel, L., Le Bras, C., Relion, D., Roman, H., and Morel, P. (2015). Effect of high temperature on the production of 2n pollen grains in diploid roses and obtaining tetraploids via unilateral polyploidization. Plant Breed. 134, 356–364. doi: 10.1111/pbr.12271
Crespel, L., and Morel, P. (2014). Pollen viability and meiotic behaviour in intraspecific hybrids of Hydrangea aspera subsp aspera Kawakami group x subsp sargentiana. Plant Breed. 133, 536–541. doi: 10.1111/pbr.12170
Crespel, L., Ricci, S. C., and Gudin, S. (2006). The production of 2n pollen in rose. Euphytica 151, 155–164. doi: 10.1007/s10681-006-9136-1
Cui, L., Sun, Y., Xiao, K., Wan, L., Zhong, J., Liu, Y., et al. (2022). Analysis on the abnormal chromosomal behaviour and the partial female fertility of allotriploid Lilium – ‘Triumphator’ (LLO) is not exceptional to the hypothesis of lily interploid hybridizations. Sci. Hortic. 293:110746. doi: 10.1016/j.scienta.2021.110746
Czarnecki, D. M., and Deng, Z. N. (2009). Unreduced gametes and polyploidization in Lantana camara. Hortscience 44:1054.
Czarnecki, D. M., Hershberger, A. J., Robacker, C. D., Clark, D. G., and Deng, Z. A. (2014). Ploidy levels and pollen stainability of Lantana camara cultivars and breeding lines. Hortscience 49, 1271–1276. doi: 10.21273/hortsci.49.10.1271
Czarnecki, D. M., Wilson, S. B., Knox, G. W., Freyre, R., and Deng, Z. A. (2012). UF-T3 and UF-T4: two sterile Lantana camara cultivars. Hortscience 47, 132–137. doi: 10.21273/hortsci.47.1.132
De Storme, N., Copenhaver, G. P., and Geelen, D. (2012). Production of diploid male gametes in Arabidopsis by cold-induced destabilization of postmeiotic radial microtubule arrays. Plant Physiol. 160, 1808–1826. doi: 10.1104/pp.112.208611
De Storme, N., and Geelen, D. (2011). The Arabidopsis mutant jason produces unreduced first division restitution male gametes through a parallel/fused spindle mechanism in meiosis II. Plant Physiol. 155, 1403–1415. doi: 10.1104/pp.110.170415
De Storme, N., and Geelen, D. (2013). Sexual polyploidization in plants–cytological mechanisms and molecular regulation. New Phytol. 198, 670–684. doi: 10.1111/nph.12184
d’Erfurth, I., Jolivet, S., Froger, N., Catrice, O., Novatchkova, M., Simon, M., et al. (2008). Mutations in AtPS1 (Arabidopsis thaliana Parallel spindle 1) lead to the production of diploid pollen grains. PLoS Genet 4:e1000274. doi: 10.1371/journal.pgen.1000274
Dewitte, A., Eeckhaut, T., van Huylenbroeck, J., and van Bockstaele, E. (2009). Occurrence of viable unreduced pollen in a Begonia collection. Euphytica 168, 81–94. doi: 10.1007/s10681-009-9891-x
Dewitte, A., Eeckhaut, T., van Huylenbroeck, J., and van Bockstaele, E. (2010a). Induction of 2n pollen formation in Begonia by trifluralin and N2O treatments. Euphytica 171, 283–293. doi: 10.1007/s10681-009-0060-z
Dewitte, A., Eeckhaut, T., van Huylenbroeck, J., and van Bockstaele, E. (2010b). Meiotic aberrations during 2n pollen formation in Begonia. Heredity 104, 215–223. doi: 10.1038/hdy.2009.111
Dewitte, A., van Laere, K., and van Huylenbroeck, J. (2012). “Use of 2n gametes in plant breeding,” in Plant Breeding, ed. I. Abdurakhmonov (Rijeka: InTech), 59–86. doi: 10.1007/s00299-013-1534-y
Dhiman, M. R., Moudgil, S., Parkash, C., Kumar, R., Kumar, S., Sindhu, S. S., et al. (2019). Analysis of nuclear DNA content and ploidy levels in 3x × 4x Lilium (Lilium × elegans L.) progenies by flow cytometry. Isr. J. Plant Sci. 66, 127–135. doi: 10.1163/22238980-00001054
Eckenwalder, J. E., and Brown, B. P. (1986). Polyploid speciation in hybrid morning glories of Ipomoea L. sect. Quamoclit Griseb. Can. J. Genet. Cytol. 28, 17–20. doi: 10.1139/g86-004
El Mokadem, H., Crespel, L., Meynet, J., and Gudin, S. (2002a). The occurrence of 2n-pollen and the origin of sexual polyploids in dihaploid roses (Rosa hybrida L.). Euphytica 125, 169–177. doi: 10.1023/a:1015830803459
El Mokadem, H., Meynet, J., and Crespel, L. (2002b). The occurrence of 2n eggs in the dihaploids derived from Rosa hybrida L. Euphytica 124, 327–332. doi: 10.1023/a:1015781606511
Eng, W. H., and Ho, W. S. (2019). Polyploidization using colchicine in horticultural plants: a review. Sci. Hortic. 246, 604–617. 2018.11.010 doi: 10.1016/j.scienta
Fedorova, N. J. (1934). Polyploid inter-specific hybrids in the genus Fragaria. Genetica 16, 524–541. doi: 10.1007/BF01984746
Feng, Z., Liu, R. F., and Jia, G. X. (2012). Induction of 2n pollens by Trifluralin in Longiflorum x Asiatic hybrid (Lillium). Acta Agri. Boreali Occidentallis Sin. 21, 153–157.
Fernandez, A., Rey, H., and Solis Neffa, V. G. (2010). Evolutionary relationships between the diploid Turnera grandiflora and the octoploid T. fernandezii (Turneraceae). Ann. Bot. Fennici. 47, 321–329. doi: 10.5735/085.047.0501
Gajewski, W. (1953). A fertile amphipolyploid hybrid of Geitn1 rivale with G. muacroplylutin. Acta Soc. Bot. Pol. 22, 411–439. doi: 10.5586/asbp.1953.027
Gomez-Rodriguez, V. M., Rodriguez-Garay, B., and Barba-Gonzalez, R. (2012). Meiotic restitution mechanisms involved in the formation of 2n pollen in Agave tequilana Weber and Agave angustifolia Haw. Springerplus 1:17. doi: 10.1186/2193-1801-1-17
Guo, H. R., Du, G. H., Huang, Z. T., Xie, L., Wei, Q., and Zhang, Z. S. (2021). 2n male gametogenesis and its cytological mechanism in hybrids of Cymbidium sinense x C. lancifolium. J. Huazhong Agri. Univ. 40, 152–158.
Guo, L., Xu, W., Zhang, Y., Zhang, J., and Wei, Z. (2017). Inducing triploids and tetraploids with high temperatures in Populus sect Tacamahaca. Plant Cell Rep. 36, 313–326. doi: 10.1007/s00299-016-2081-0
Haan, A. D. E., Maceira, N. O., Lumaret, R., and Delay, J. (1992). Production of 2n gametes in diploid subspecies of Dactylis glomerata L. 2. Occurrence and frequency of 2n eggs. Ann. Bot. 4:4. doi: 10.1093/oxfordjournals.aob.a088351
Harlan, J. R., and DeWet, J. M. J. (1975). On ö. Winge and a prayer: the origins of polyploidy. Bot. Rev. 41, 361–390. doi: 10.1007/bf02860830
Hayashi, M., Kato, J., Ohashi, H., and Mii, M. (2007). Variation of ploidy level in inter-section hybrids obtained by reciprocal crosses between tetraploid Primula denticulata (2n = 4x = 44) and diploid P. rosea (2n = 2x = 22). J. Hortic. Sci. Biotechnol. 82, 5–10. doi: 10.1080/14620316.2007.11512191
Hayashi, M., Kato, J., Ohashi, H., and Mii, M. (2009). Unreduced 3x gamete formation of allotriploid hybrid derived from the cross of Primula denticulata (4x) x P. rosea (2x) as a causal factor for producing pentaploid hybrids in the backcross with pollen of tetraploid P. denticulata. Euphytica 169, 123–131. doi: 10.1007/s10681-009-9955-y
Henny, R. J., and Chen, J. (2003). Cultivar development of ornamental foliage plants. Plant Breed. Rev. 23, 245–290. doi: 10.1002/9780470650226.ch6
Hermsen, J. G. (1984). Mechanisms and genetic implications of 2n gamete formation. Iowa State J. Res. 58, 421–434.
Horn, W. (2004). The patterns of evolution and ornamental plant breeding. Acta Hortic. 651, 19–31. doi: 10.17660/ActaHortic.2004.651.1
Hwang, Y., Cabahug, R. A., Mancia, F. H., and Lim, K. (2020). Molecular cytogenetics and its application to major flowering ornamental crops. Hortic. Environ. Biotechnol. 61, 1–9. doi: 10.1007/s13580-019-00198-6
Ishizaka, H. (1998). Production of microspore-derived plants by anther culture of an interspecific F1 hybrid between Cyclamen persicum and C. purpurascens. Plant Cell Tiss. Org. Cult. 54, 21–28. doi: 10.1023/A:1006138704856
Jones, K., and Borrill, M. (1962). Chromosome status, gene exchange and evolution in Dactylis: 3, the role of the interploid hybrids. Genetica 32, 269–322.
Kagawa, F., and Nakajima, G. (1933). Genetic and cytological studies on species hybrids in Quamoclit. Jpn. J. Bot. 6, 315–326.
Kang, X. Y., and Zhu, Z. T. (1997). A study on the 2n pollen vitality and germinant characteristics of white Populus. Acta Bot. Yunnanica 19, 402–406.
Kang, X. Y., Zhu, Z. T., and Zhang, Z. Y. (2000). Breeding of triploids by the reciprocal crossing of Populus alba × P. glandulosa and P. tomentosa × P. bolleana. J. Beijing Forest. Univ. 22, 8–11.
Karlov, G. I., Khrustaleva, L. I., Lim, K. B., and van Tuyl, J. M. (1999). Homoeologous recombination in 2n-gametes producing interspecific hybrids of Lilium (Liliaceae) studied by genomic in situ hybridization (GISH). Genome 42, 681–686. doi: 10.1139/gen-42-4-681
Karpechenko, G. D. (1927). The production of polyploid gametes in hybrids. Hereditas 9, 349–368. doi: 10.1111/j.1601-5223.1927.tb03536.x
Kato, A., and Geiger, H. H. (2002). Chromosome doubling of haploid maize seedling using nitrous oxide gas at the flower primordial stage. Plant Breed. 121, 370–377.
Kato, J., Ishikawa, R., and Mii, M. (2001). Different genomic combinations in inter-section hybrids obtained from the crosses between Primula sieboldii (Section Cortusoides) and P. obconica (Section Obconicolisteri) by the embryo rescue technique. Theor. Appl. Genet. 102, 1129–1135. doi: 10.1007/s001220000516
Kato, J., and Mii, M. (2000). Differences in ploidy levels of inter-specific hybrids obtained by reciprocal crosses between Primula sieboldii and P. kisoana. Theor. Appl. Genet. 101, 690–696. doi: 10.1007/s001220051532
Kato, J., and Mii, M. (2012). Production of interspecific hybrids in ornamental plants. Method Mol. Biol. 877, 233–245. doi: 10.1007/978-1-61779-818-4_18
Kato, J., Ohashi, H., Ikeda, M., Fujii, N., Ishikawa, R., Horaguchi, H., et al. (2008). Unreduced gametes are the major causal factor for the production of polyploid interspecific hybrids in Primula. Plant Biotechnol. 25, 521–528. doi: 10.5511/plantbiotechnology.25.521
Khan, N. Barba-Gonzalez, R., Ramanna, M. S., Arens, P., and Visser, R. G. F.van Tuyl, J. M. (2010). Relevance of unilateral and bilateral sexual polyploidization in relation to intergenomic recombination and introgression in Lilium species hybrids. Euphytica 171, 157–173. doi: 10.1007/s10681-009-9998-0
Khan, N., Barba-Gonzalez, R., Ramanna, M. S., Visser, R. G. F., and Van Tuyl, J. M. (2009a). Construction of chromosomal recombination maps of three genomes of lilies (Lilium) based on GISH analysis. Genome 52, 238–251. doi: 10.1139/g08-122
Khan, N., Zhou, S., Ramanna, M. S., Arens, P., Herrera, J., Visser, R. G. F., et al. (2009b). Potential for analytic breeding in allopolyploids: an illustration from longiflorum x Asiatic hybrid lilies (Lilium). Euphytica 166, 399–409. doi: 10.1007/s10681-008-9824-0
Köhler, C., Scheid, O. M., and Erilova, A. (2010). The impact of the triploid block on the origin and evolution of polyploid plants. Trend. Genet. 26, 142–148. doi: 10.1016/j.tig.2009.12.006
Konyar, S. T. (2017). Ultrastructural aspects of pollen ontogeny in an endangered plant species, Pancratium maritimum L. (Amaryllidaceae). Protoplasma 254, 881–900. doi: 10.1007/s00709-016-0998-3
Koutecky, P., Badurova, T., Stech, M., Kosnar, J., and Karasek, J. (2011). Hybridization between diploid Centaurea pseudophrygia and tetraploid C. jacea (Asteraceae): the role of mixed pollination, unreduced gametes, and mentor effects. Biol. Linn. Soc. 104, 93–106. doi: 10.1111/j.1095-8312.2011.01707.x
Kovalsky, I. E., and Neffa, V. G. S. (2012). Evidence of 2n microspore production in a natural diploid population of Turnera sidoides subsp. carnea and its relevance in the evolution of the T. sidoides (Turneraceae) autopolyploid complex J. Plant Res. 125, 725–734. doi: 10.1007/s10265-012-0493-7
Kreiner, J. M., Kron, P., and Husband, B. C. (2017a). Evolutionary dynamics of unreduced gametes. Trend. Genet. 33, 583–593. doi: 10.1016/j.tig.2017.06.009
Kreiner, J. M., Kron, P., and Husband, B. C. (2017b). Frequency and maintenance of unreduced gametes in natural plant populations: associations with reproductive mode, life history and genome size. New Phytol. 214, 879–889. doi: 10.1111/nph.14423
Kroon, G. H. (1993). Breeding research in Begonia. Acta Hortic. 337, 53–58. doi: 10.17660/actahortic.1993.337.6
Kroon, G. H., and Van Eijk, J. P. (1977). Polyploidy in Tulips (Tulipa L.). The occurrence of diploid gametes. Euphytica 26, 63–66. doi: 10.1007/bf00032069
Lai, H. G., Chen, X., Chen, Z., Ye, J. Q., Li, K. M., and Liu, J. P. (2015). Induction of female 2n gametes and creation of tetraploids through sexual hybridization in cassava (Manihot esculenta). Euphytica 201, 265–273. doi: 10.1007/s10681-014-1207-0
Lee, Y. H., and Tham, F. Y. (1988). An advanced generation of Aranda orchids. Genome 30, 608–611. doi: 10.1139/g88-102
Li, J. Y., Wu, H. Z., Chen, X., and Zhou, D. (2011). Primary studies on breeding techniques by 2n gametes in colored Zantedeschia hybrid. Chinese Agri. Sci. Bull. 27, 108–113.
Li, N., Xu, C., Zhang, A., Lv, R., Meng, X., Lin, X., et al. (2019). DNA methylation repatterning accompanying hybridization, whole genome doubling and homoeolog exchange in nascent segmental rice allotetraploids. New Phytol. 223, 979–992. doi: 10.1111/nph.15820
Li, Y., Cheng, Z., Smith, W., Ellis, D., Chen, Y., Zheng, X., et al. (2004). Invasive ornamental plants: problems, challenges, and molecular tools to neutralize their invasiveness. Crit. Rev. Plant Sci. 23, 381–389. doi: 10.1080/07352680490505123
Li, Y., Wang, Y., Wang, P. Q., Yang, J., and Kang, X. Y. (2016). Induction of unreduced megaspores in Eucommia ulmoides by high temperature treatment during megasporogenesis. Euphytica 212, 515–524. doi: 10.1007/s10681-016-1781-4
Li, Y. H., Kang, X. Y., Wang, S. D., Zhang, Z. H., and Chen, H. W. (2008). Triploid induction in Populus alba × P. glandulosa by chromosome doubling of female gametes. Silvae Genet 57, 37–40. doi: 10.1515/sg-2008-0006
Li, F. L., Zhang, Z. Y., and Zhang, M. X. (1994). Studies on chromosome doubling and triploid breeding of white poplar II: the observation of some morphological characters in triploid of white poplar. J. Beijing For. Univ. 16, 15–18. doi: 10.13332/j.1000-1522.1994.02.003
Liao, T., Cheng, S., Zhu, X., Min, Y., and Kang, X. (2016a). Effects of triploid status on growth, photosynthesis, and leaf area in Populus. Trees 30, 1137–1147. doi: 10.1007/s00468-016-1352-2
Liao, X. S., Wu, Q. Q., Zhang, Z. J., Zheng, X. X., and Wang, L. Y. (2016b). Study on 2n pollen induction and its identification of Lillium oriental. Northern Hortic 8, 56–60.
Lim, K.-B., Barba-Gonzalez, R., Zhou, S., Ramanna, M. S., and Van Tuyl, J. M. (2005). Meiotic polyploidization with homoeologous recombination induced by caffeine treatment in interspecific lily hybrids. Korean J. Genet. 27, 219–226. doi: 10.1007/s001220100638
Lim, K. B., Ramanna, M. S., de Jong, J. H., Jacobsen, E., and van Tuyl, J. M. (2001). Indeterminate meiotic restitution (IMR): a novel type of meiotic nuclear restitution mechanism detected in interspecific lily hybrids by GISH. Theo. Appl. Genet. 103, 219–230.
Lim, K. B., Ramanna, M. S., Jacobsen, E., and van Tuyl, J. M. (2003). Evaluation of BC2 progenies derived from 3x-2x and 3x-4x crosses of Lilium hybrids: a GISH analysis. Theor. Appl. Genet. 106, 568–574. doi: 10.1007/s00122-002-1070-6
Lim, K.-B., Shen, T.-M., Barba-Gonzalez, R., Ramanna, M. S., and van Tuyl, J. M. (2004). occurrence of SDR 2n-gametes in Lillium hybrids. Breed. Sci. 54, 13–18. doi: 10.1270/jsbbs.54.13
Liu, Z., Seiler, G. J., Gulya, T. J., Feng, J. H., Rashid, K. Y., Cai, X. W., et al. (2017). Triploid production from interspecific crosses of two diploid perennial Helianthus with diploid cultivated sunflower (Helianthus annuus L.). G3 Genes Genomes Genet. 7, 1097–1108. doi: 10.1534/g3.116.036327
Loginova, D. B., and Silkova, O. G. (2016). Phosphorylation of histone H3ser10 in plant cell division. Russ. J. Genet. Appl. Res. 20, 87–95. doi: 10.18699/vj16.132
Loginova, D. B., and Silkova, O. G. (2017). Mechanisms of unreduced gamete formation in flowering plants. Russ. J. Genet. 53, 741–756. doi: 10.1134/s1022795417070080
Lokker, A. C., Barba-Gonzalez, R., Lim, K.-B., Ramanna, M. S., and van Tuyl, J. M. (2005). Genotypic and environmental variation in production of 2n gametes of Oriental x Asiatic lily hybrids. Acta Hortic. 673, 453–456. doi: 10.17660/ActaHortic.2005.673.58
Lu, M., Zhang, P. D., and Kang, X. Y. (2013). Induction of 2n female gametes in Populus adenopoda Maxim by high temperature exposure during female gametophyte development. Breed. Sci. 63, 96–103. doi: 10.1270/jsbbs.63.96
Luo, J. R., Arens, P., Niu, L. X., and van Tuyl, J. M. (2016). Induction of viable 2n pollen in sterile Oriental × Trumpet Lilium hybrids. J. Hortic. Sci. Biotechnol. 91, 258–263. doi: 10.1080/14620316.2016.1148371
Maceira, N. O., Haan, A. A. D., Lumaret, J. R., Billon, M., and Delay, J. (1992). Production of 2n gametes in diploid subspecies of Dactylis glomerata L.1.occurrence and frequency of 2n pollen. Ann. Bot. 69, 335–343. doi: 10.1093/oxfordjournals.aob.a088350
Mai, Y. N., Li, H. W., Suo, Y. J., Fu, J. M., Sun, P., Han, W. J., et al. (2019). High temperature treatment generates unreduced pollen in persimmon (Diospyros kaki Thunb.). Sci. Hortic. 258:108774. doi: 10.1016/j.scienta.2019.108774
Malik, R. A., Gupta, R. C., Kumari, S., and Malik, A. H. (2014). Cytomictic anomalous male meiosis and 2n pollen grain formation in Mertensia echioides Benth. (Boraginaceae) from Kashmir Himalaya. TheScientificWorld J. 2014:134192. doi: 10.1155/2014/134192
Marasek, A., Mizuochi, H., and Okazaki, K. (2006). The origin of Darwin hybrid tulips analyzed by flow cytometry, karyotype analyses and genomic in situ hybridization. Euphytica 151, 279–290. doi: 10.1007/s10681-006-9147-y
Marasek-Ciolakowska, A., Arens, P. F. P., and van Tuyl, J. M. (2016). “The role of polyploidization and interspecific hybridization in the breeding of ornamental crops,” in Polyploidy and Hybridization for Crop Improvement, ed. A. Mason (Boca Raton, FL: CRC Press), 159–181. doi: 10.1270/jsbbs.17097
Marasek-Ciolakowska, A., He, H., Bijman, P., Ramanna, M. S., Arens, P., and van Tuyl, J. M. (2012). Assessment of intergenomic recombination through GISH analysis of F1, BC1 and BC2 progenies of Tulipa gesneriana and T. fosteriana. Plant Syst. Evol. 298, 887–899. doi: 10.1007/s00606-012-0598-4
Marasek-Ciolakowska, A., Sochacki, D., and Marciniak, P. (2021). Breeding aspects of selected ornamental bulbous crops. Agronomy 11:1709. doi: 10.3390/agronomy11091709
Marasek-Ciolakowska, A., Xie, S. L., Arens, P., and van Tuyl, J. M. (2014). Ploidy manipulation and introgression breeding in Darwin hybrid Tulips. Euphytica 198, 389–400. doi: 10.1007/s10681-014-1115-3
Mason, A. S., Nelson, M. N., Yan, G., and Cowling, W. A. (2011). Production of viable male unreduced gametes in Brassica interspecific hybrids is genotype specific and stimulated by cold temperatures. BMC Plant Biol. 11:103. doi: 10.1186/1471-2229-11-103
Mason, A. S., and Pires, J. C. (2015). Unreduced gametes: meiotic mishap or evolutionary mechanism? Trend. Genet. 31, 5–10. doi: 10.1016/j.tig.2014.09.011
Mayrose, I., Zhan, S. H., Rothfels, C. J., Magnuson-Ford, K., Barker, M. S., Rieseberg, L. H., et al. (2011). Recently formed polyploid plants diversify at lower rates. Science 333, 1257–1257. doi: 10.1126/science.1207205
Meredith, M. R., Michaelson-Yeates, T. P. T., Ougham, H. J., and Thomas, H. (1995). Trifolium ambiguum as a source of variation in the breeding of white clover. Euphytica 82, 185–191. doi: 10.1007/bf00027065
Miyashita, T., Ohashi, T., Shibata, F., Araki, H., and Hoshino, Y. (2009). Plant regeneration with maintenance of the endosperm ploidy level by endosperm culture in Lonicera caerulea var. emphyllocalyx. Plant Cell Tiss. Org. Cult. 98, 291–301. doi: 10.1007/s11240-009-9562-6
Moghe, G. D., and Shiu, S. H. (2014). The causes and molecular consequences of polyploidy in flowering plants. Ann. N. Y. Acad. Sci. 1320, 16–34. doi: 10.1111/nyas.12466
Nakato, N., and Masuyama, S. (2021). Polyploid progeny from triploid hybrids of Phegopteris decursivepinnata (Thelypteridaceae). J. Plant Res. 134, 195–208. doi: 10.1007/s10265-021-01255-x
Negri, V., Lorenzetti, S., and Lemmi, G. (1995). Identification and cytological analysis of 2n pollen producers in Lotus tenuis Wald. et Kit. Plant Breed. 114, 86–88. doi: 10.1111/j.1439-0523.1995.tb00767.x
Negri, V., and Veronesi, F. (1989). Evidence for the existence of 2n gametes in Lotus tenuis Wald. et Kit. (2n = 2x = 12): their relevance in evolution and breeding of Lotus corniculatus L. (2n = 4x = 24). Theor. Appl. Genet. 78, 400–404. doi: 10.1007/bf00265303
Niazian, M., and Nalousi, A. M. (2020). Artificial polyploidy induction for improvement of ornamental and medicinal plants. Plant Cell Tiss. Organ. Cult. 142, 447–469. doi: 10.1007/s11240-020-01888-1
Nimura, M., Kato, J., Mii, M., and Katoh, T. (2006). Amphidiploids produced by natural chromosome-doubling in inter-specific hybrids between Dianthus x isensis Hirahata et Kitam. and D. japonicus Thunb. J. Hortic. Sci. Biotechnol. 81, 72–77. doi: 10.1080/14620316.2006.11512031
Noda, S. (1986). Cytogenetic behavior, chromosomal differences, and geographic distribution in L. lancifolium (Liliaceae). Plant Species Biol. (Kyoto) 1, 69–78. doi: 10.1111/j.1442-1984.1986.tb00016.x
Noman, A., Aqeel, M., Deng, J., Khalid, N., Sanaullah, T., and Shuilin, H. (2017). Biotechnological advancements for improving floral attributes in ornamental plants. Front. Plant Sci. 8:530. doi: 10.3389/fpls.2017.00530
Nukui, S., Kitamura, S., Hioki, T., Ootsuka, H., Miyoshi, K., Satou, T., et al. (2011). N2O induces mitotic polyploidization in anther somatic cells and restores fertility in sterile interspecific hybrid lilies. Breed. Sci. 61, 327–337. doi: 10.1270/jsbbs.61.327
Okada, H. (1984). Polyphyletic allopolyploid origin of Ranunculus cantoniensis (4x) from R. silerifolius (2x) × R. chinensis (2x). Plant Syst. Evol. 148, 89–102. doi: 10.1007/BF00984571
Okazaki, K., Kurimoto, K., Miyajima, I., Enami, A., Mizuochi, H., Matsumoto, Y., et al. (2005). Induction of 2n pollen in Tulips by arresting the meiotic process with nitrous oxide gas. Euphytica 143, 101–114. doi: 10.1007/s10681-005-2910-7
Ørgaard, M., Jacobsen, N., and Heslop-Harrison, I. S. (1995). The hybrid origin of two cultivars of Crocus (Iridaceae) analyzed by molecular cytogenetics including genomic southern and in situ hybridization. Ann. Bot. 76, 253–262. doi: 10.1006/anbo.1995.1094
Ostergren, G. (1954). Polyploids and aneuploids of Crepsis capillaris produced by treatment with nitrous oxide. Genetica 27, 54–64. doi: 10.1007/bf01664154
Palumbo, F., Pasquali, E., Albertini, E., and Barcaccia, G. (2021). A review of unreduced gametes and neopolyploids in alfalfa: how to fill the gap between well-established meiotic mutants and next-generation genomic resources. Plants (Basel) 10:999. doi: 10.3390/plants10050999
Parrott, W. A., and Smith, R. R. (1986). Recurrent selection for 2n pollen formation in red clover. Crop Sci. 26, 1132–1135. doi: 10.2135/cropsci1986.0011183x002600060009x
Parrott, W. A., Smith, R. R., and Smith, M. M. (1985). Bilateral sexual tetraploidization in red clover. Can. J. Genet. Cytol. 27, 64–68. doi: 10.1139/g85-011
Pecrix, Y., Rallo, G., Folzer, H., Cigna, M., Gudin, S., and Le Bris, M. (2011). Polyploidization mechanisms: temperature environment can induce diploid gamete formation in Rosa sp. J. Exp. Bot. 62, 3587–3597. doi: 10.1093/jxb/err052
Peloquin, S. J., Boiteux, L. S., Simon, P. W., and Jansky, S. H. (2008). A chromosome-specific estimate of transmission of heterozygosity by 2n gametes in potato. J. Hered. 99, 177–181. doi: 10.1093/jhered/esm110
Piao, M. L., Jia, G. X., and Zhang, D. M. (2020). Fertility analysis and 2n gametes induction of Lilium FA hybrids ‘Jiaoyang’ in short growth period. J. Beijing Forest. Univ. 42, 106–112.
Pringle, G. J., and Murray, B. G. (1992). Polyploidy and aneuploidy in the tomatillo Cyphomandra betacea (Cav.) Sendt (Solanaceae). I. Spontaneous polyploidy and feature of the euploids. Plant Breed. 108, 132–138. doi: 10.1111/j.1439-0523.1992.tb00112.x
Przybyla, A., Behrend, A., Bornhake, C., and Hohe, A. (2014). Breeding of polyploid heather (Calluna vulgaris). Euphytica 199, 273–282. doi: 10.1007/s10681-014-1117-1
Rabe, E. W., and Haufler, H. H. (1992). Incipient polyploid speciation in the maidenhair fern. Am. J. Bot. 79, 701–707. doi: 10.1002/j.1537-2197.1992.tb14611.x
Ramanna, M. S., and Jacobsen, E. (2003). Relevance of sexual polyploidization for crop improvement – a review. Euphytica 133, 3–18. doi: 10.1023/a:1025600824483
Ramanna, M. S., Kuipers, A. G. J., and Jacobsen, E. (2003). Occurrence of numerically unreduced (2n) gametes in Alstroemeria interspecific hybrids and their significance for sexual polyploidisation. Euphytica 133, 95–106. doi: 10.1023/a:1025652808553
Ramanna, M. S., Marasek-Ciolakowska, A., Xie, S., Khan, N., and van Tuyl, J. M. (2012). “The significance of polyploidy for bulbous ornamentals: a molecular cytogenetic assessment,” in Floriculture and Ornamental Biotechnology. Special Issue: Bulbous Ornamentals, Vol. 1, eds J. van Tuyl and P. Arens (London, UK: Global Science Books Ltd), 116–121.
Ramsey, J. (2007). Unreduced gametes and neopolyploids in natural populations of Achillea borealis (Asteraceae). Heredity 98, 143–150. doi: 10.1038/sj.hdy.6800912
Ramsey, J., and Schemske, D. W. (1998). Pathways, mechanisms, and rates of polyploidy formation in flowering plants. Annu. Rev. Ecol. Syst. 29, 467–501. doi: 10.1146/annurev.ecolsys.29.1.467
Ravi, M., Marimuthu, M. P. A., and Siddiqi, I. (2008). Gamete formation without meiosis in Arabidopsis. Nature 451, 1121–1124. doi: 10.1038/nature06557
Roose, M. L., and Gottlieb, L. D. (1976). Genetic and biochemical consequences of polyploidy in Tragopogon. Evolution 30, 818–830. doi: 10.2307/2407821
Sato, T., Miyoshi, K., and Okazaki, K. (2009). “Induction of 2n gametes and 4n embryo in Lilium (Lilium x formolongi hort.) by nitrous oxide gas treatment,” in Proceedings of the 23rd International Eucarpia Symposium, Section Ornamentals – Colourful Breeding and Genetics (Leiden).
Sheidai, M., Azanei, N., and Attar, F. (2009). New chromosome number and unreduced pollen formation in Achillea species (Asteraceae). Acta Biol. Szeged. 53, 39–43.
Soltis, D. E., Soltis, P. S., and Tate, J. A. (2004). Advances in the study of polyploidy since plant speciation. New Phytol. 161, 173–191. doi: 10.1046/j.1469-8137.2003.00948.x
Soltis, P. S., and Soltis, D. E. (2009). The role of hybridization in plant speciation. Annu. Rev. Plant Biol. 60, 561–588. doi: 10.1146/annurev.arplant.043008.092039
Stephens, L. C. (1998). Formation of unreduced pollen by an Impatiens hawkeri x platypetala interspecific hybrid. Hereditas 128, 251–255. doi: 10.1111/j.1601-5223.1998.00251.x
Sugiura, A., Ohkuma, T., Choi, Y. A., Tao, R., and Tamura, M. (2000). Production of nonaploid (2n = 9x) Japanese persimmons (Diospyros kaki) by pollination with unreduced (2n = 6x) pollen and embryo rescue culture. J. Am. Soc. Hortic. Sci. 125, 609–614. doi: 10.21273/jashs.125.5.609
Sun, P., Nishiyama, S., Asakuma, H., Voorrips, R. E., Fu, J., and Tao, R. (2021). Genomics-based discrimination of 2n gamete formation mechanisms in polyploids: a case study in nonaploid Diospyros kaki ‘Akiou’. G3 Genes Genom. Genet. 11:jkab188. doi: 10.1093/g3journal/jkab188
Takahashi, C., Leitch, I. J., Ryan, A., Bennett, M. D., and Brandham, P. E. (1997). The use of genomic in situ hybridization (GISH) to show transmission of recombinant chromosomes by a partially fertile bigeneric hybrid, Gasteria lutzii x Aloe aristata (Aloaceae), to its progeny. Chromosoma 105, 342–348. doi: 10.1007/s004120050193
Takamura, T., and Miyajima, I. (1996). Cross-compatibility and the ploidy of progenies in crosses between diploid and tetraploid cyclamen (Cyclamen persicum Mill.). J. Jap. Soc. Hortic. Sci. 64, 883–889. doi: 10.2503/jjshs.64.883
Talluri, R. S. (2011). Gametes with somatic chromosome number and their significance in interspecific hybridization in Fuchsia. Biol. Plant. 55, 596–600. doi: 10.1007/s10535-011-0133-4
Tang, X., and Luo, Z. (2002). Cytology of 2n pollen formation in nonastringent persimmon. Sci. Agric. Sin. 35, 585–588.
Tiku, A. R., Razdan, M. K., and Raina, S. N. (2014). Production of triploid plants from endosperm cultures of Phlox drummondii. Biol. Plant. 58, 153–158. doi: 10.1007/s10535-013-0372-7
Trankner, C., Gunther, K., Sahr, P., Engel, F., and Hohe, A. (2020). Targeted generation of polyploids in Hydrangea macrophylla through cross-based breeding. BMC Genet 21:147. doi: 10.1186/s12863-020-00954-z
Trojak-Goluch, A., and Berbec, A. (2003). Cytological investigations of the interspecific hybrids of Nicotiana tabacum L. x N. glauca Grah. J. Appl. Genet. 44, 45–54.
van Eijk, J. P., Van Raamsdonk, L. W. D., Eikelboom, W., and Bino, R. J. (1991). Interspecific crosses between Tulipa gesneriana cultivars and wild Tulipa species: a survey. Sex. Plant Reprod. 4, 1–5. doi: 10.1007/bf00194563
van Laere, K., Dewitte, A., Van Huylenbroeck, J., and Van Bockstaele, E. (2009). Evidence for the occurrence of unreduced gametes in interspecific hybrids of Hibiscus. J. Hortic. Sci. Biotechnol. 84, 240–247. doi: 10.1080/14620316.2009.11512511
van Raamsdonk, L. W. D., van Eijk, J. P., and Eikelboom, W. (1995). Crossability analysis in subgenus Tulipa of the genus Tulipa L. Bot. J. Linn. Soc. 117, 147–158. doi: 10.1111/j.1095-8339.1995.tb00449.x
van Scheepen, J. (1996). Classified list and International Register of Tulip Names. Hillegom: Royal General Bulbgrowers’ Association KAVB.
van Tuyl, J. M., and Arens, P. (2011). Lilium: breeding history of the modern cultivar assortment. Acta Hortic. 900, 223–230. doi: 10.17660/ActaHortic.2011.900.27
van Tuyl, J. M., De Vries, J. N., Bino, R. J., and Kwakkenbos, T. A. M. (1989). Identification of 2n-pollen producing interspecific hybrids of Lilium using flow cytometry. Cytologia 54, 737–745. doi: 10.1508/cytologia.54.737
van Tuyl, J. M., Dijken, A. V., Chi, H. S., Lim, K. B., Villemoes, S., and Van Kronenburg, B. C. E. (2000). Breakthroughs in interspecific hybridization of lily. Acta Hort. 508, 83–88. doi: 10.17660/actahortic.2000.508.10
Veronesi, F., Mariani, A., and Bingham, E. T. (1986). Unreduced gametes in diploid Medicago and their importance in alfalfa breeding. Theor. Appl. Genet. 72, 37–41. doi: 10.1007/bf00261451
Wang, J., Huo, B. B., Liu, W. T., Li, D. L., and Liao, L. (2017a). Abnormal meiosis in an intersectional allotriploid of Populus L. and segregation of ploidy levels in 2x x 3x progeny. PLoS One 12:e0181767. doi: 10.1371/journal.pone.0181767
Wang, J., Li, D. L., Shang, F. N., and Kang, X. Y. (2017b). High temperature-induced production of unreduced pollen and its cytological effects in Populus. Sci. Rep. 7:5281. doi: 10.1038/s41598-017-05661-x
Wang, J., You, H. L., Tian, J., Wang, Y. F., Liu, M. H., and Duan, W. L. (2015). Abnormal meiotic chromosome behavior and gametic variation induced by intersectional hybridization in Populus L. Tree Genet. Genomes 11, 1–11. doi: 10.1007/s11295-015-0880-z
Wang, X. L., Cheng, Z. M., Zhi, S., and Xu, F. X. (2016). Breeding triploid plants: a review. Czech J. Genet. Plant Breed. 52, 41–54. doi: 10.17221/151/2015-cjgpb
Williams, J. H. (2021). Consequences of whole genome duplicaiton for 2n pollen performance. Plant Reprod. 4, 321–334. doi: 10.1007/s00497-021-00426-z
Winge, Ö (1917). The chromosomes: their numbers and general importance. C. R. Trav. Lab. Carlsberg 13, 131–275.
Wongprichachan, P., Huang, K.-L., Chou, Y.-M., Hsu, S.-T., Liu, T.-Y., and Okubo, H. (2013). Induction of unreduced gamete in Phalaenopsis by N2O treatments. J. Fac. Agric. Kyushu Univ. 58, 27–31. doi: 10.5109/26157
Wu, H. Z., Shi, F. F., Zheng, S. X., Zhang, J. L., and Zhou, D. (2011). Induction of 2n pollen in coloured Zantedeschia hybrid and obtaining of its triploid. J. Agri. Biotechnol. 19, 662–668. doi: 10.3969/j.issn.1674-7968.2011.04.010
Wu, H. Z., Zheng, S. X., He, Y. Q., Yan, G. J., Bi, Y. F., and Zhu, Y. Y. (2007). Diploid female gametes induced by colchicine in oriental lilies. Sci. Hortic. 114, 50–53. doi: 10.1016/j.scienta.2007.04.004
Xi, M., van Tuyl, J. M., and Arens, P. (2015). GISH analyzed progenies generated from allotriploid lilies as female parent. Sci. Hortic. 183, 130–135. doi: 10.7150/ijbs.6427
Xiao, K., Zheng, W., Zeng, J., Wu, L., Cui, L., Liu, Y., et al. (2019). Analysis of abnormal meiosis and progenies of an odd allotetraploid Lilium ‘Honesty’. Sci. Hortic. 253, 316–321. doi: 10.1016/j.scienta.2019.04.012
Xiao, K. Z., Cui, L. M., Wan, L., Zhong, J., Liu, Y. M., Sun, Y. N., et al. (2021). A new way to produce odd-allotetraploid lily (Lilium) through 2n gametes. Plant Breed. 140, 711–718. doi: 10.1111/pbr.12932
Xie, S., Khan, N., Ramanna, M. S., Niu, L., Marasek-Ciolakowska, A., Arens, P., et al. (2010). An assessment of chromosomal rearrangements in neopolyploids of Lilium hybrids. Genome 53, 439–446. doi: 10.1139/g10-018
Xu, L. Q., Zhang, Q. L., and Luo, Z. R. (2008). Occurrence and cytological mechanism of 2n pollen formation in Chinese Diospyros spp. (Ebenaceae) staminate germplasm. J. Hortic. Sci. Biotechnol. 83, 668–672. doi: 10.1080/14620316.2008.11512441
Xu, W., Luo, G., Lian, X., Yu, F., Zheng, Y., Lei, J., et al. (2021). Meiotic behaviour and pollen fertility of F-1, F-2 and BC1 progenies of Iris dichotoma and I. domestica. Folia Hortic. 33, 173–183. doi: 10.2478/fhort-2021-0013
Yamaguchi, S. (1980). Identification of ploid level by pollen characters in Primula sieboldii E. Morren. Jap. J. Breed. 30, 293–300. doi: 10.1270/jsbbs1951.30.293
Yanagi, T., Hummer, K. E., Iwata, T., Sone, K., Nathewet, P., and Takamura, T. (2010). Aneuploid strawberry (2n = 8x+2 = 58) was developed from homozygous unreduced gamete (8x) produced by second division restitution in pollen. Sci. Hortic. 125, 123–128. doi: 10.1016/j.scienta.2010.03.015
Yang, F., Fernandez-Jimenez, N., Tuckova, M., Vrana, J., Capal, P., Diaz, M., et al. (2021). Defects in meiotic chromosome segregation lead to unreduced male gametes in Arabidopsis SMC5/6 complex mutants. Plant Cell 33, 3104–3119. doi: 10.1093/plcell/koab178
Yang, J., Yao, P. Q., Li, Y., Mo, J. Y., Wang, J. Z., and Kang, X. Y. (2016). Induction of 2n pollen with colchicine during microsporogenesis in Eucalyptus. Euphytica 210, 69–78. doi: 10.1007/s10681-016-1699-x
Yang, Y. X., Zheng, W., Xiao, K. Z., Wu, L. K., Zeng, J., and Zhou, S. J. (2019). Transcriptome analysis reveals the different compatibility between LAAA x AA and LAAA x LL in Lilium. Breed. Sci. 69, 297–307. doi: 10.1270/jsbbs.18147
Yao, H., Dogra, G. A., Auger, D. L., and Birchler, J. A. (2013). Genomic dosage effects on heterosis in triploid maize. Proc. Natl. Acad. Sci. U.S.A. 110, 2665–2669. doi: 10.1073/pnas.1221966110
Yao, P.-Q., Li, G.-H., Long, Q.-Y., He, L.-G., and Kang, X.-Y. (2016). Male parent identification of triploid rubber trees (Hevea brasiliensis) and the mechanism of 2n gametes formation. Forests 7:301. doi: 10.3390/f7120301
Yasui, K. (1931). Cytological studies in artifi- cially raised interspecific hybrids of Papaver. III. Unusual cases of cytokinesis in pollen mother cells in an F1 plant. Cytologia 2, 402–419. doi: 10.1508/cytologia.2.402
Younis, A., Hwang, Y., and Lim, K. (2014). Exploitation of induced 2n-gametes for plant breeding. Plant Cell Rep. 33, 215–223.
Zang, S. Z., Yang, J. M., Zhao, X. H., Fu, B., Zhang, D. X., and Qu, L. W. (2010). Colchicine induced by different processing methods and the concentration of 2n gametes of Lilium. Northern Hortic. 11, 98–100.
Zeng, R. Z., Zhu, J., Xu, S. Y., Du, G. H., Guo, H. R., Chen, J. J., et al. (2020). Unreduced male gamete formation in Cymbidium and its use for developing sexual polyploid cultivars. Front. Plant Sci. 11:558. doi: 10.3389/fpls.2020.00558
Zhang, F., Yang, M. H., Gong, J. Q., Feng, H., Gao, S. M., and Zhou, Y. (2019a). Study on 2n pollen induction by colchicine in rose variety ‘Old Brush’. Acta Bot. Boreali Occident. Sin. 39, 620–629.
Zhang, J. F., Wei, Z. Z., Li, D., and Li, B. (2009). Using SSR markers to study the mechanism of 2n pollen formation in Populus euramericana (Dode) Guinier and P. x popularis. Ann. For. Sci. 66:506. doi: 10.1051/forest/2009032
Zhang, X., Ren, G., Li, K., Zhou, G., and Zhou, S. (2012). Genomic variation of new cultivars selected from distant hybridization in Lilium. Plant Breed. 131, 227–230. doi: 10.1111/j.1439-0523.2011.01906.x
Zhang, X. Y., Tong, H. L., Han, Z. Q., Huang, L., Tian, J., Fu, Z. X., et al. (2021). Cytological and morphology characteristics of natural microsporogenesis within Camellia oleifera. Physiol. Mol. Biol. Plants 27, 959–968. doi: 10.1007/s12298-021-01002-5
Zhang, Y. Y., Zhang, X. F., Huang, X., Zhang, X. Y., Yao, P. Q., and Li, W. G. (2019b). Low temperature increases the frequency of 2n female gametes in the diploid rubber tree (Hevea brasiliensis (Willd. ex A.Juss.) Müll. Arg.). Tree Genet. Genomes 15:23. doi: 10.1007/s11295-019-1330-0
Zhang, Z. Y., Li, F. L., and Zhu, Z. T. (1992). Chromosome doubling and triploid breeding of Populus tomentosa Carr.and its hybrid. J. Beijing For. Univ. 14, 52–58. doi: 10.1007/s11632-005-0022-z
Zheng, S. X., Liao, X. S., Lin, Q. D., Ling, W. B., Gong, J. H., Long, B., et al. (2017). “Initial study on 2n gametes induction of strelitzia reginae,” in I International Symposium on Tropical and Subtropical Ornamentals, Vol. 1167, eds K. Thammasiri, N. Panvisavas, and R. E. Paull (Leuven: International Society for Horticultural Science), 157–162. doi: 10.17660/actahortic.2017.1167.24
Zheng, W. (2019). Analysis on the difference of ‘Original Love’ and ‘Tiny Skyline’, ‘White Fox’ in lily ploidy Hybrids and their Progenies. (MS). Nanchang: Jiangxi Agricultural University.
Zheng, X. Q., Zeng, X. S., Chen, X. M., and Yang, G. L. (1983). A new method for inducing triploid of Hevea. Chin. J. Trop. Crops 4, 1–4. doi: 10.9734/ajraf/2018/42469
Zhou, J. J., Zeng, R. Z., Liu, F., Yi, M. S., Li, Y. H., and Zhang, Z. S. (2009). Investigation on chromosome ploidy of the hybrids of Phalaenopsis polyploids. Acta Hortic. 36, 1491–1497. doi: 10.16420/j.issn.0513
Zhou, Q., Wu, J., Sang, Y. R., Zhao, Z. Y., Zhang, P. D., and Liu, M. Q. (2020). Effects of colchicine on Populus canescens ectexine structure and 2n pollen production. Front. Plant Sci. 11:295. doi: 10.3389/fpls.2020.00295
Zhou, S., Li, K., and Zhou, G. (2012). Analysis of endosperm development of allotriploid × diploid/tetraploid crosses in Lilium. Euphytica 184, 401–412. doi: 10.1007/s10681-011-0609-5
Zhou, S., Ramanna, M. S., Visser, R. G. F., and van Tuyl, J. M. (2008). Genome composition of triploid lily cultivars derived from sexual polyploidization of Longiflorum x Asiatic hybrids (Lilium). Euphytica 160, 207–215. doi: 10.1007/s10681-007-9538-8
Zhou, S., Yuan, G., Xu, P., and Gong, H. (2014). Study on lily introgression breeding using allotriploids as maternal parents in interploid hybridizations. Breed. Sci. 64, 97–102. doi: 10.1270/jsbbs.64.97
Zhou, S., Zhou, G., and Li, K. (2011). Euploid endosperm of triploid × diploid/tetraploid crosses results in aneuploid embryo survival in Lilium. HortScience 46, 558–562. doi: 10.21273/hortsci.46.4.558
Zhou, S. J., Tan, X., Fang, L. Q., Jian, J., Xu, P., and Yuan, G. L. (2013). Study of the female fertility of an odd-tetraploid of Lilium and its potential breeding significance. J. Am. Soc. Hortic. Sci. 138, 114–119. doi: 10.21273/jashs.138.2.114
Zhou, X., Mo, X., Gui, M., Wu, X., Jiang, Y., Ma, L., et al. (2015). Cytological, molecular mechanisms and temperature stress regulating production of diploid male gametes in Dianthus caryophyllus L. Plant Physiol. Biochem. 97, 255–263. doi: 10.1016/j.plaphy.2015.10.003
Zhou, X. H., Su, Y., Yang, X. M., Zhang, Y. P., Li, S. C., Gui, M., et al. (2017). The biological characters and polyploidy of progenies in hybridization in 4x-2x crosses in Dianthus caryophyllus. Euphytica 213:118. doi: 10.1007/s10681-017-1898-0
Zhu, J., Liu, Y. Y., Zeng, R. Z., Li, Y. H., Guo, H. R., Xie, L., et al. (2014). Preliminarily study on formation and cytological mechanism of unreduced male gametes in different ploidy Phalaenopsis. Acta Hortic. Sin. 41, 2132–2138.
Keywords: unreduced gametes, sexual polyploidization, plant breeding, ornamental plants, polyploid cultivars
Citation: Xie L, Ke L-z, Lu X-q, Chen J and Zhang Z-s (2022) Exploiting Unreduced Gametes for Improving Ornamental Plants. Front. Plant Sci. 13:883470. doi: 10.3389/fpls.2022.883470
Received: 25 February 2022; Accepted: 27 April 2022;
Published: 06 June 2022.
Edited by:
Diego Rubiales, Institute for Sustainable Agriculture (CSIC), SpainReviewed by:
Annalisa Giovannini, Council for Agricultural and Economics Research (CREA), ItalyCopyright © 2022 Xie, Ke, Lu, Chen and Zhang. This is an open-access article distributed under the terms of the Creative Commons Attribution License (CC BY). The use, distribution or reproduction in other forums is permitted, provided the original author(s) and the copyright owner(s) are credited and that the original publication in this journal is cited, in accordance with accepted academic practice. No use, distribution or reproduction is permitted which does not comply with these terms.
*Correspondence: Jianjun Chen, ampjaGVuQHVmbC5lZHU=; Zhi-sheng Zhang, enN6aGFuZ0BzY2F1LmVkdS5jbg==
Disclaimer: All claims expressed in this article are solely those of the authors and do not necessarily represent those of their affiliated organizations, or those of the publisher, the editors and the reviewers. Any product that may be evaluated in this article or claim that may be made by its manufacturer is not guaranteed or endorsed by the publisher.
Research integrity at Frontiers
Learn more about the work of our research integrity team to safeguard the quality of each article we publish.