- 1Rice Research Institute of Sichuan Agricultural University, Chengdu, China
- 2State Key Laboratory of Crop Gene Exploration and Utilization in Southwest China, Sichuan Agricultural University, Chengdu, China
- 3Key Laboratory of Southwestern Chinese Medicine Resources, Chengdu University of Traditional Chinese Medicine, Chengdu, China
- 4Leshan Municipal Bureau of Agriculture and Rural Affairs, Leshan, China
The cooking and eating quality of rice grains is a major focus from a consumer’s perspective and is mainly determined by the apparent amylose content (AAC) of the starch. Waxy rice, a type of rice with an AAC of less than 2%, is an important goal for the breeding of high-quality rice. In recent years, the cloning of the Waxy (Wx) gene has revealed the molecular mechanism of the formation of waxy traits in rice. However, there have been limited studies on the physicochemical properties, such as gelatinization temperature, rapid viscosity analyzer profile, and amylopectin fine structure of wx mutants. In the current study, a rapid and highly efficient strategy was developed through the CRISPR/Cas9 gene-editing system for generating wx mutants in the background of five different rice varieties. The wx mutation significantly reduced the AAC and starch viscosity but did not affect the major agronomic traits (such as plant height, panicle number per plant, grain number per panicle, and seed-setting frequency). Incorporation of the wx mutation into varieties with low initial AAC levels resulted in further reduction in AAC, but without significantly affecting the original, desirable gelatinization traits and amylopectin structure types, suggesting that parents with low initial AAC should be preferred in breeding programs.
Introduction
Starch consists of two classes of α-polyglucans, amylose and amylopectin. Amylose is primarily a linear polymer, consisting of 1,4-D-glucopyranosyl units, while amylopectin is a highly branched polymer (Hizukuri et al., 1981; Hizukuri, 1986), with the amylose: amylopectin ratio in conventional rice being approximately 20: 80. The amylose content (AC) of the starch is arguably the most important quality indicator in rice grains, particularly with respect to cooking, processing, and eating qualities (Juliano, 1998). Rice cultivars can be divided into five categories according to AC: waxy (0–2%), very low (5–12%), low (13–20%), intermediate (21–25%), and high amylose (26–33%) (Juliano, 1992). Waxy rice (or glutinous rice) is regarded as high-quality rice, which is usually sticky when cooked (Juliano, 1998). Because of its unique, functional characteristics, waxy rice is widely used in processed food, medicines, and cosmetics (Bao et al., 2004; Puchongkavarin et al., 2005; Chun et al., 2010).
Amylose is proposed to have a significant influence on the physicochemical properties of waxy rice flour (Jane et al., 1999). Waxy rice starch with a certain amount of amylose as the donor substrate results in increases in the levels of crystallinity, solubility and paste clarity, gelatinization temperature (GT), enthalpy (ΔH), gel strength, and storage modulus (Lu et al., 2008; Guo et al., 2019). There is a reduction in the retrogradation of flour, a process in which disaggregated amylose and amylopectin chains in a gelatinized starch paste reassociate to form more ordered structures, when waxy rice flour is used, with an increase in AC (Sasaki et al., 2000). On the other hand, the fine structure of amylopectin is considered to affect the gelatinization, retrogradation, and rheology of waxy rice starch (Kalichevsky et al., 1990; Chung et al., 2008). During retrogradation of waxy rice starch, GT is positively correlated with the branch chain length of amylopectin (Jane et al., 1999; Singh et al., 2012), with the greater branch density of amylopectin being associated with increasing ΔH and solubility but with decreasing viscosity of waxy rice starch (Sorndech et al., 2015; Ren et al., 2017). These studies revealed the vital role played by amylopectin fine structure and AC in the physicochemical properties of waxy rice. However, there are no effective and rapid means for breeding waxy rice cultivars by targeting low AC or the fine structure of amylopectin, and this limitation has constrained the larger-scale production and consumption of waxy rice cultivars.
The Wx gene is located on rice chromosome 6 and encodes granule-bound starch synthase I (GBSSI), which mainly controls amylose synthesis in the seed endosperm and thus directly affects the quality of rice grains (Sano, 1984; Wang et al., 1995). The use of numerous allelic variants of Wx (e.g., Wxlv, Wxa, Wxin, Wxb, Wxop, Wxmp, and wx) in the rice breeding programs has led to regional differences in the AC of rice (Sano, 1984; Larkin and Park, 2003; Chen et al., 2008; Mikami et al., 2008; Dobo et al., 2010; Teng et al., 2011; Zhang et al., 2019) and allows rice breeders to control the expression of the Wx gene to obtain rice varieties with desired starch quality traits (Satoh and Omura, 1981; Liu et al., 2003; Liu et al., 2005; Kong et al., 2015). In recent years, the CRISPR/Cas9 gene-editing system has been used to knock out or fine-tune the expression of the Wx gene (Ma et al., 2015). The first exon of the Wx gene was edited to produce a null mutation via CRISPR/Cas9, to generate four japonica rice wx mutant lines with significantly reduced AC, but with an unaltered expression of agronomic traits (Zhang et al., 2018; Yunyan et al., 2019). Editing the TATA box of the Wxb promoter downregulated Wx expression and thus fine-tuned grain AC, which could improve the grain quality (Huang et al., 2020). However, the relationship between the physicochemical properties of rice starch in each wx mutant and its corresponding wild type (WT) remains unclear, and more information is needed before breeding for high-quality waxy starch by gene editing can be carried out effectively.
In the current study, we systematically analyzed the physicochemical properties of wx mutants generated in different genetic backgrounds by the CRISPR/Cas9 system. The results showed that the physicochemical properties of wx mutants are highly correlated with the properties of the WT parent, suggesting that parents with low initial AAC should be preferred for generating waxy rice mutants with desirable starch characteristics. Our work provides new insights into the breeding of waxy rice with high-quality starch and could contribute significantly to expanding the waxy rice germplasm resources.
Results
Mutant Isolation and Agronomic Trait Assessment
We first selected two cultivated rice varieties with different major Wx alleles, namely, QLD (Wxa) and YSZ (Wxb), which are both widely grown in the rice-producing region of Southwest China. We designed a CRISPR/Cas9 (Figure 1A) construct that accurately targeted the second exon (87–109 bp) of the Wx gene with the expectation of generating a null mutation (Figure 1B). In the genetic background of QLD (Figure 1C), we obtained four homozygous mutant alleles for the target site, with each of these mutations shifting the reading frame of the candidate gene in the 5’ coding region, indicating that they were most likely to be null alleles. Five homozygous mutant alleles of Wxb were also generated in the background of YSZ (Figure 1D). We observed that 80% of T0 transformants were mutants in QLD plants and 82.35% in YSZ plants, indicating that the CRISPR/Cas9 system exhibited a high mutagenesis efficiency (Supplementary Table 1). Meanwhile, our results showed that the wx mutations did not change the main agronomic traits of the T1 generation lines, including plant height, panicle number per plant, grain number per plant, and seed-setting frequency (Supplementary Figure 1).
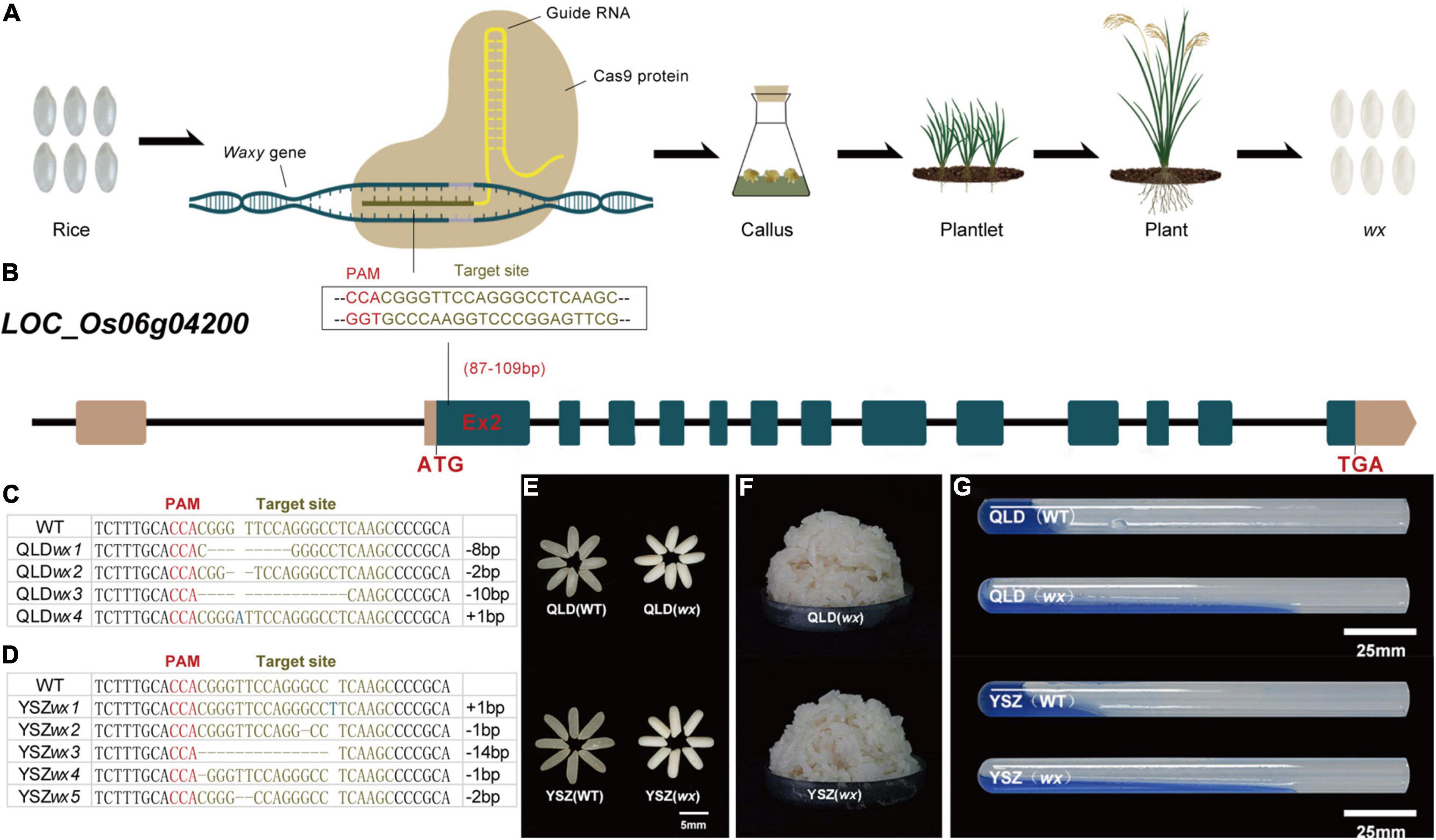
Figure 1. New waxy rice by CRISPR/Cas9-mediated mutagenesis of Waxy gene in two rice varieties. (A) Schematic experimental design. From left to right are wild-type rice, CRISPR/Cas9 system, callus, plantlet, plant, and waxy rice. (B) Schematic diagram of the targeted site in exon 2 of Waxy gene (LOC_Os06g04200). The numbers in brackets indicate the distance to the start codon (ATG). (C–D) Nucleotide variations at the targets (the protospacer adjacent motif in blank) of homozygous mutant lines from YSZwx1 and QLDwx4. “−”, base deletion; “+”, base insertion. The targeted sequence is highlighted in brown, and the protospacer adjacent motif (PAM) sequences are in red. (E) Grain phenotypes of wx mutants and their corresponding WTs. (F) The appearance of cooked waxy rice. (G) Gel consistency.
Assessment of the Grain Appearance and Cooking Quality of the wx Mutants
In addition, we identified two single-base homozygous mutants, YSZwx1 and QLDwx4 (T5 generation), and performed quality analysis on them (Supplementary Table 2). The results showed no significant difference in the major grain quality traits of the two wx mutants, except that the color of endosperm changed from transparent to milky white (Figure 1E) and the kernel weight decreased (Supplementary Figure 2). The cooking and eating quality of YSZwx1 rice was soft but not sticky, while that of the QLDwx4 rice was very sticky (Figure 1F), and the grains of both mutants exhibited a significantly higher gel consistency (GC) than that of flour of the corresponding WT parents (Figure 1G).
Analyses of Apparent Amylose Content, Total Protein, Total Starch, and Grain Yield in wx Mutants
We observed that the AAC values of YSZ and QLD were significantly decreased to 1.16 and 2.36%, respectively (Figure 2A) (reduced by 91.01 and 89.80%, respectively) in the corresponding mutants, leading to increased GC (Figure 2B). The total protein content of YSZ and QLD was increased by 26.01 and 6.37%, respectively, in the mutants (Figure 2C), but the total starch content showed no consistent effect in the mutants, increasing by 8.11% in the YSZ mutant and decreasing by 6.19% in the QLD mutant, compared with the corresponding WT (Figure 2D). Moreover, the rice yield values indicated that the grain yields of YSZwx1 and QLDwx4 were 8,195 and 9,050 kg/hm2 (= kg/ha), respectively, which were significantly higher than the yields of the corresponding wild types (Figure 2E). These results suggest that editing the Wx gene can significantly improve both the quantity and the quality of the rice produced by the mutants.
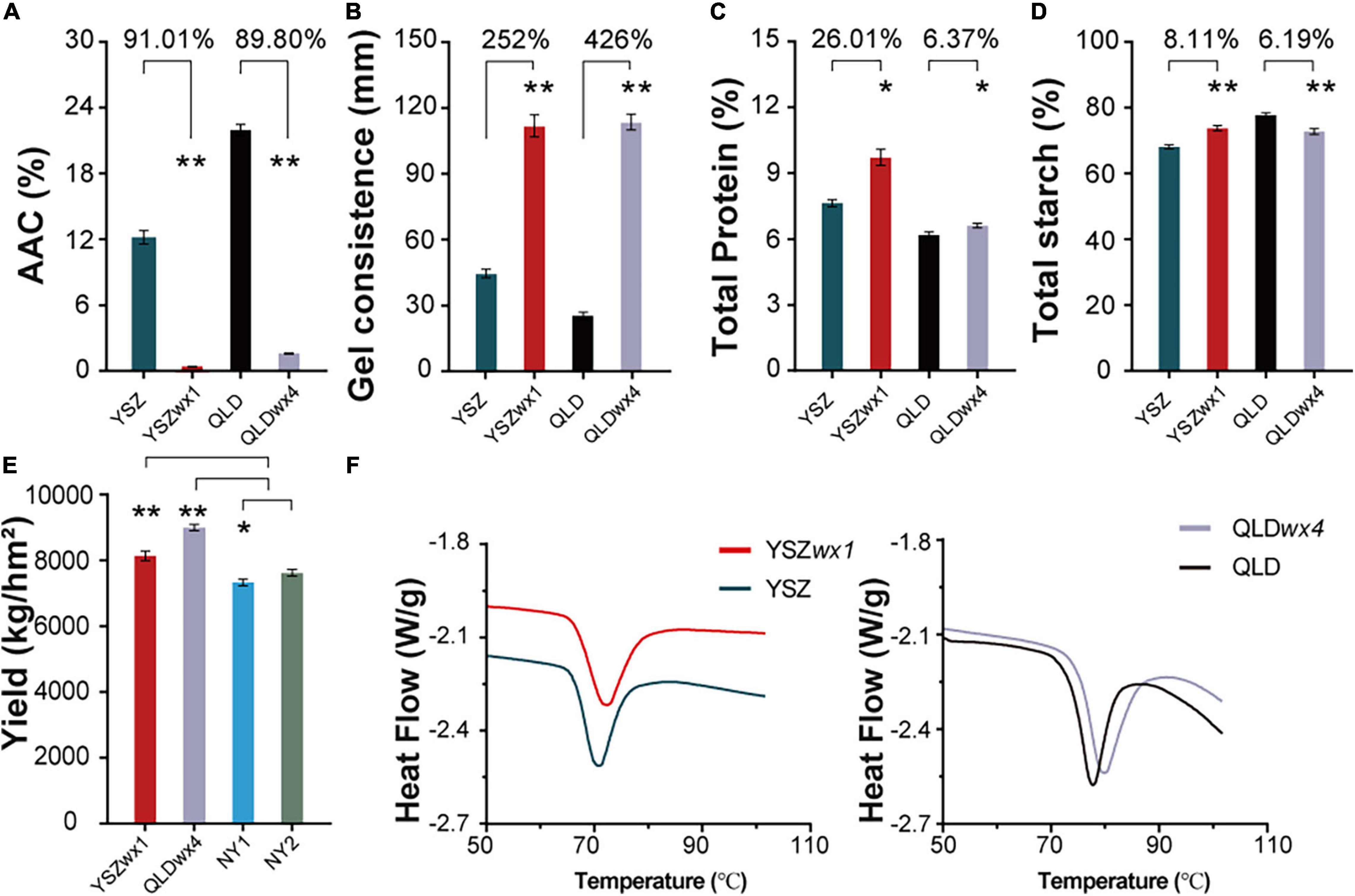
Figure 2. Apparent amylose content (AAC), gel consistency (GC), total protein, total starch, grain yield, and gelatinization properties of wx mutants and their corresponding WT lines. (A) AAC of wx mutants and their corresponding WTs. (B) GC of wx mutants and their corresponding WTs. (C) Total protein of wx mutants and their corresponding WTs. (D) Total starch of wx mutants and their corresponding WTs. (E) The grain yield of wx mutants of NY1 and NY2. NY1 is a japonica waxy rice (in blue), NY2 is an indica waxy rice (in green). (F) Gelatinization properties curve in wx mutants and their corresponding WTs. Error bars are mean ± SD (n = 3). Significant differences were determined by Student’s t-test (*P < 0.05, **P < 0.01).
Analyses of Starch Thermal Properties of the Mutants
The results of analyses of the thermal properties of the starch from the wx mutants suggested that editing of the Wx gene did not significantly change the GT, although the two wx mutants showed a slightly higher GT range than the WT lines (Figure 2F). For example, the onset temperature (To), peak temperature (Tp), conclusion temperature (Tc), and ΔH of YSZ and YSZ wx1 were 66.28 and 66.65°C, 70.70 and 71.66°C, 76.27 and 78.74°C, and ΔH of 11.6 and 12.55 J/g, respectively (Supplementary Table 3). Similar results were also obtained for QLD and QLDwx4 (Supplementary Table 3). The results suggest that To, Tp, Tc, and ΔH from the wx mutants were all higher, compared with the corresponding WT, which led to the mutant starch being gelatinized later than the WT starch. However, the mutant starch still belonged to the same GT type as the WT starch.
Analyses of Starch Pasting Properties of the Mutants
The pasting properties of all wx mutants were significantly different from those of the corresponding WT lines (P < 0.05) (Figure 3A). For example, the mean peak viscosity (PKV), hot paste viscosity (HPV), cool paste viscosity (CPV), breakdown viscosity (BDV = PKV-HPV), setback viscosity (SBV = CPV-HPV), peak time, and pasting temperature (PT) of QLD and QLDwx4 were 2,662 and 2,841 cp, 1,760 and 1,432 cp, 4,210 and 1,497 cp, 939 and 1,488 cp, 1,548 and -1,344 cp, 6.38 and 4.57 s, and 80.7 and 81.5°C, respectively (Supplementary Table 4). The results show that wx mutants have softer pasting properties than their corresponding WT lines (Figure 3B).
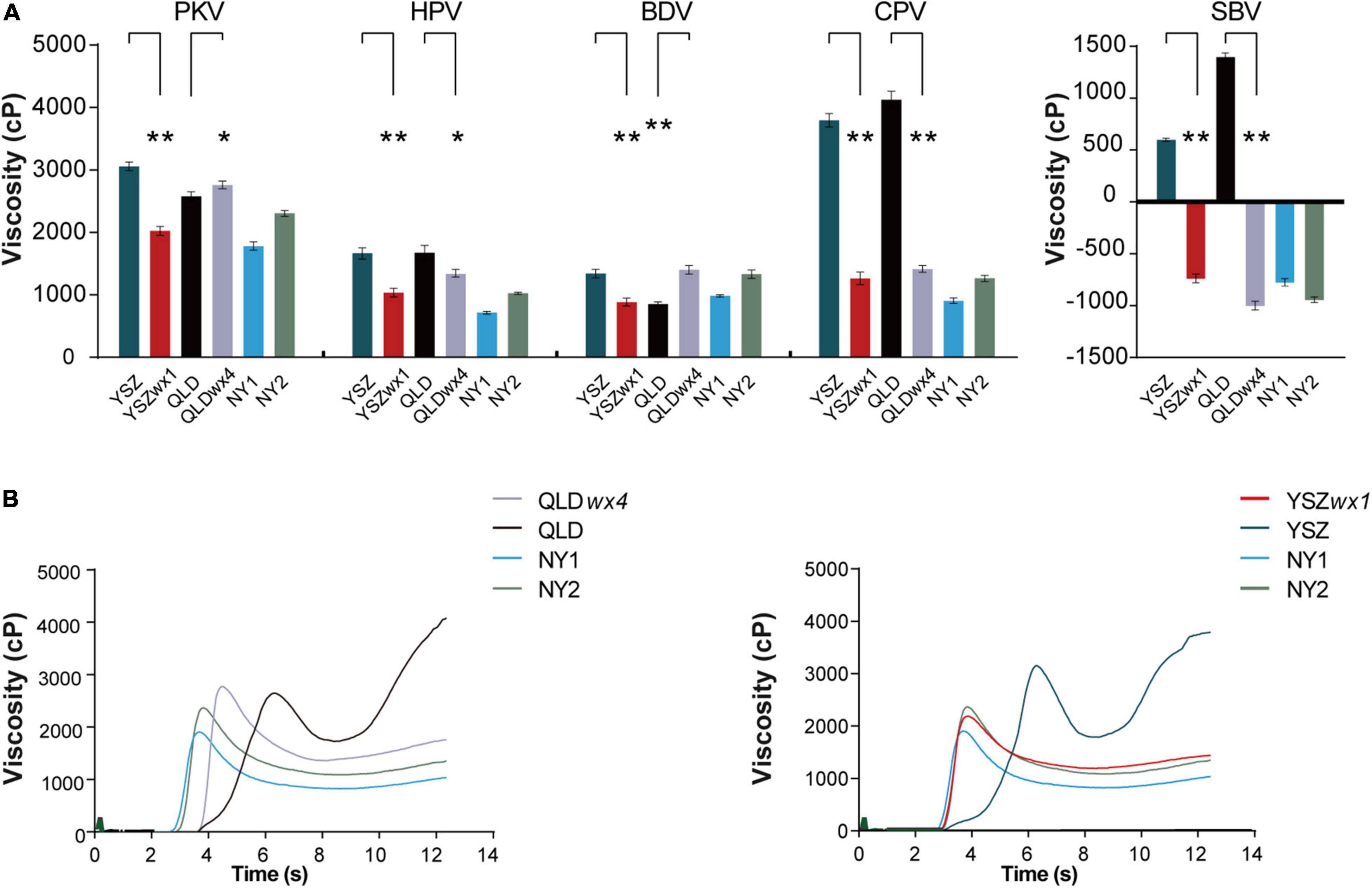
Figure 3. Rapid viscosity analyzer profiles of rice starch in wx mutants and their corresponding WTs. (A) Rapid viscosity analyzer (RVA) profiles of starch in wx mutants and their corresponding WTs. (B) Rapid viscosity analyzer profile curve of wx mutants and their corresponding WT flour samples. The date of peak viscosity (PKV), hot paste viscosity (HPV), cool paste viscosity (CPV), breakdown viscosity (BDV = PKV-HPV), and setback viscosity (SBV = CPV-HPV) were derived from the RVA profiles. NY1 is a japonica waxy rice variety (in blue), and NY2 is an indica waxy rice variety (in green). Error bars are mean ± SD (n = 3). Significant differences were determined by Student’s t-test (*P < 0.05, **P < 0.01).
Chain-Length Distribution of Amylopectin
Subsequently, we compared the chain-length distribution of amylopectin between the two wx mutants and their corresponding WT parents. The chain-length distributions of amylopectin in wx mutants were markedly different from those of the corresponding WT lines (Figure 4A). Wx mutants exhibited a higher percentage of short-chain amylopectin (degree of polymerization, DP 7–20) and a lower percentage of medium- to long-chain amylopectin (DP > 20) than the WT lines (Figure 4B). Based on the amylopectin chain ratio (ACR) [ACR = (ΣDP ≤ 10)/(ΣDP ≤ 24)], the amylopectin structure of the starch from cultivated rice can be classified into one of three types: L-type (ACR ≤ 0.200), M-type (ACR 0.201–0.239), and S-type (ACR ≥ 0.240) (Nakamura et al., 2002). Interestingly, even though the ACR values of the wx mutants were significantly lower than those of the corresponding WT lines, they still belonged to the same amylopectin structural type. The amylopectin structure of QLDwx4 and QLD belonged to the L-type, whereas YSZwx1 and YSZ belonged to the M-type (Figure 4C).
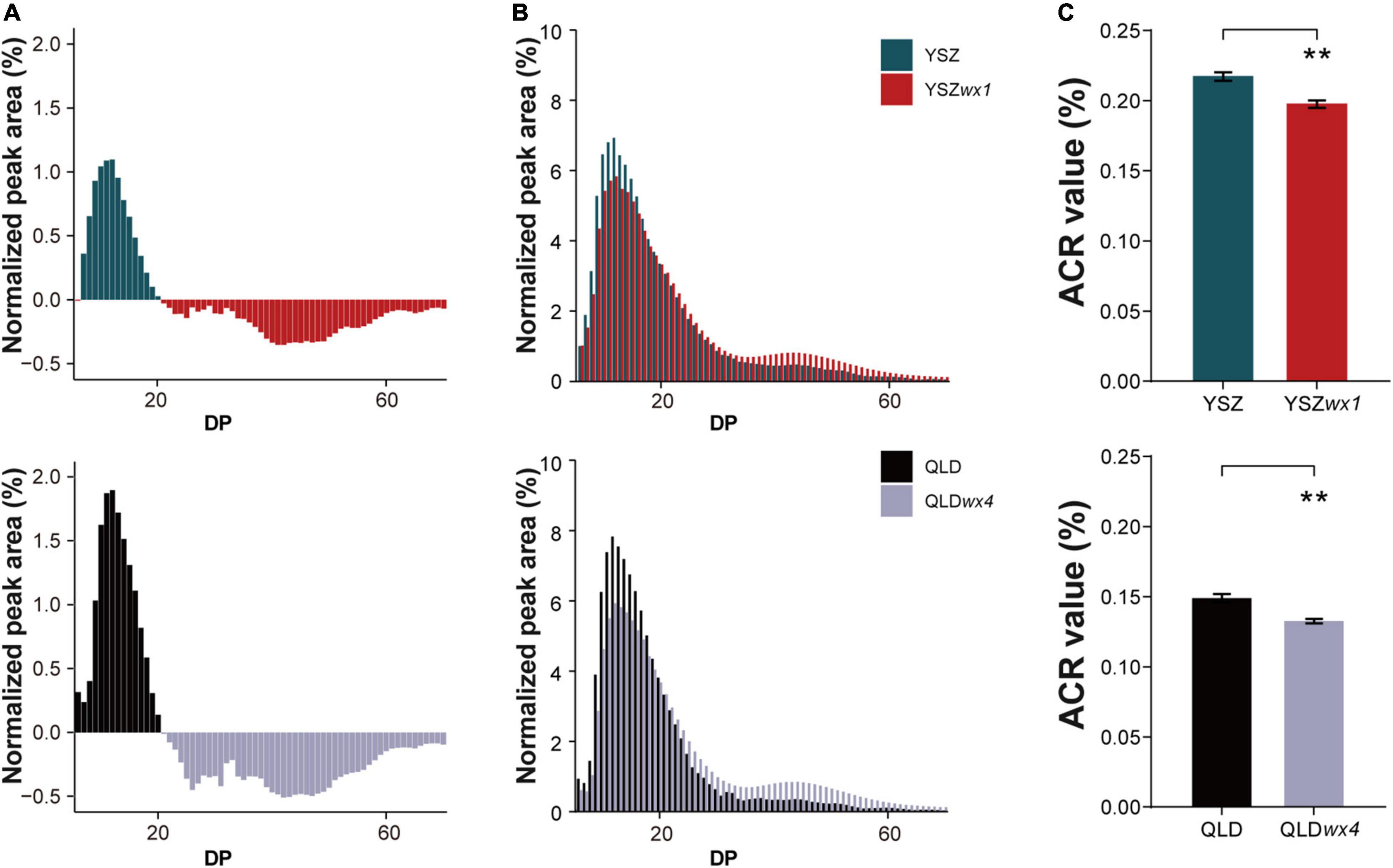
Figure 4. The fine structure of amylopectin in wx mutants and their corresponding WTs. (A) Comparison of percentage values of high-performance anion-exchange chromatography with pulsed amperometric detection (HPAEC-PAD) chromatograms of amylopectin chain length between wx mutants and their corresponding WTs. The plus values represent the WTs and the minus values represent the wx mutants. (B) The difference in the chain-length distribution of amylopectin between wx mutants and their corresponding WTs. (C) The ΣDP ≤ 10/ΣDP ≤ 24 values of wx mutants and their corresponding WTs. ACR: amylopectin chain ratio. Error bars are mean ± SD (n = 3). Significant differences were determined by Student’s t-test: **P < 0.01.
Correlation Analysis of Starch Samples
We used the same method for generating wx mutants with three other rice cultivars to find a potential link between gene-edited wx mutants and wild types (WTs) (Supplementary Figure 3 and Supplementary Table 5). These three rice cultivars all belonged to the Wxb genotype (Supplementary Table 6). The results showed that the cultivar (Figure 5A) with a low AAC would generate a wx mutant with a lower AAC (Figure 5B). Furthermore, the AAC of the wxa mutants was higher than that of the three wxb mutants, and the AAC values of QLDwx4 and SH789wx were greater than 2% (Supplementary Tables 2, 6). The Tc and ΔH values of the wx mutants were greater than those of the corresponding WTs, although their GT types were unchanged (Table 1). Similarly, the ACR values were altered by mutation but stayed within the same amylopectin structure type as the WT (Figure 5B). Further data analysis showed a positive linear correlation between ACR and GT (Figure 5C). In addition, two new starch types from the mutants were proposed, based on the amylopectin structure and the GT, namely, the HGT-type (high gelatinization temperature type, ACR < 0.18) and LGT-type (low gelatinization temperature type, ACR > 0.18). We demonstrated that the GT type and ACR type were not affected by the editing of the Wx gene, and that, to achieve wx mutants with a lower AAC, the WT parent exhibiting a lower AAC should be selected.
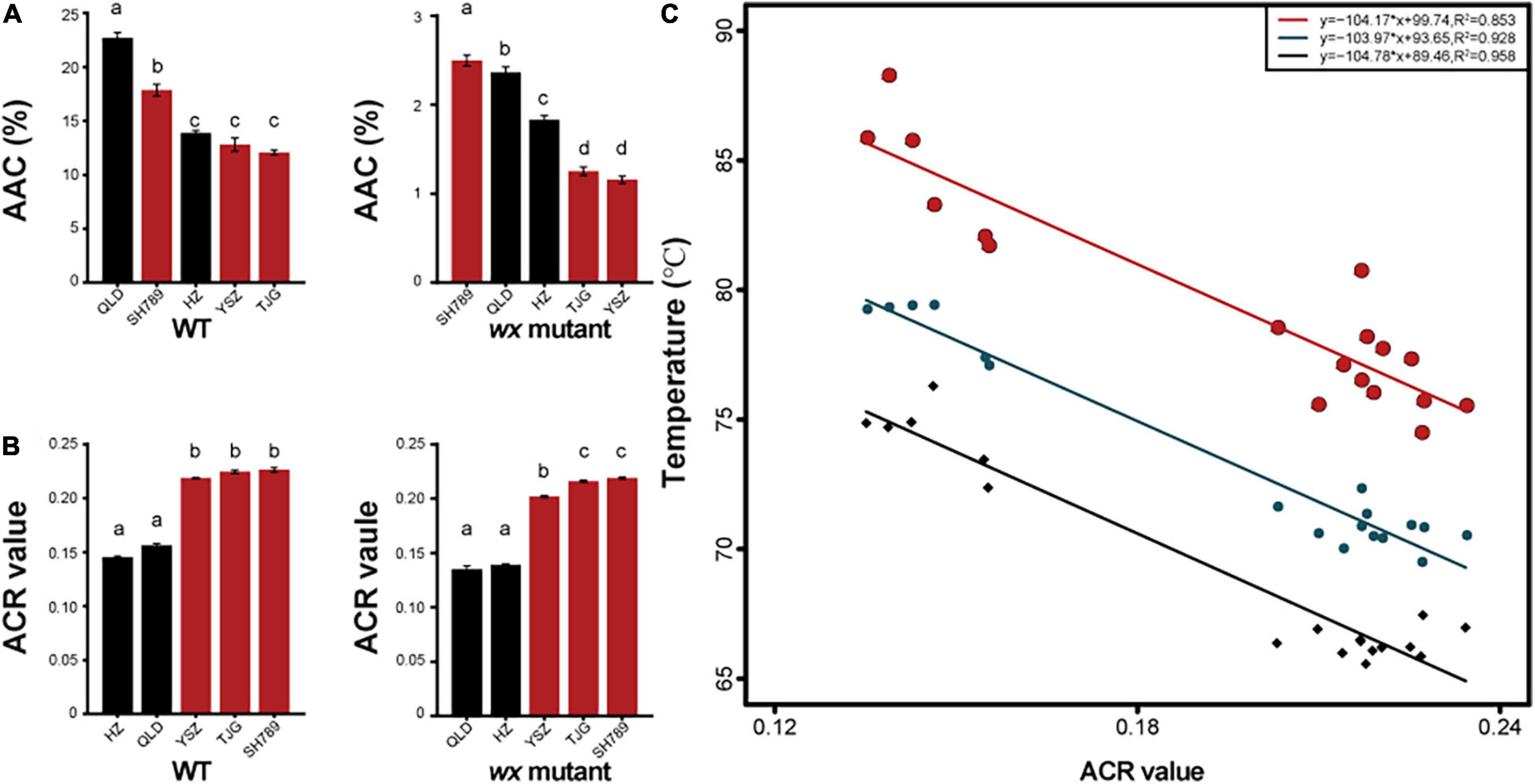
Figure 5. The apparent amylose content (AAC) and amylopectin chain ratio (ACR) value of five wx mutants and their corresponding WTs (A) AAC of five wx mutants and their corresponding WTs. (B) ACR value of five wx mutants and their corresponding WTs. AAC and ACR value of WTs (left-hand bar graph) and wx mutants (middle bar graph). (C) Relationships between ACR value and gelatinization temperature; each point represents the ACR value of a variety of rice or waxy rice. Black points represent the onset temperature (To), bluish-black points represent the peak temperature (Tp), and red points represent the conclusion temperature (Tc). Error bars are mean ± SD (n = 3). Values followed by different superscripts in the same column are significantly different (P < 0.05).
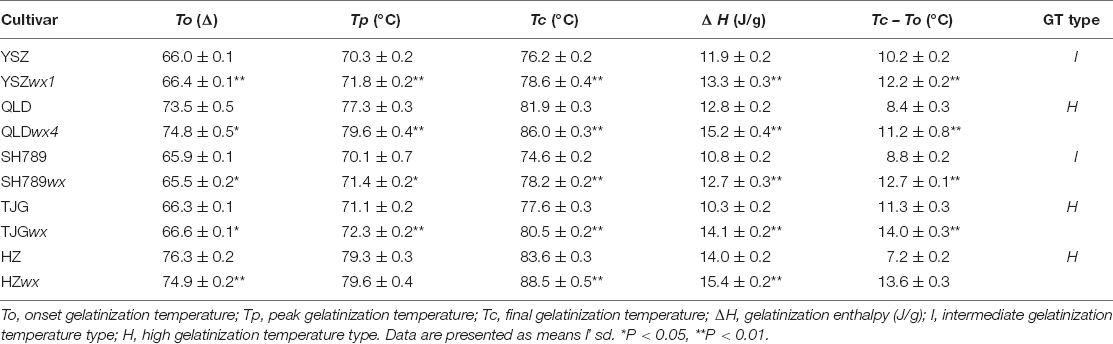
Table 1. Differential scanning calorimetry (DSC) and enthalpy (ΔH) values of wx mutants and their corresponding WTs.
In this study, we also separately analyzed the relationship between the physicochemical properties of the starch obtained from the WT and the wx mutants. Pearson’s linear correlation analysis indicated that the correlations between rice grain quality, amylopectin structure, and starch physicochemical properties differed between WTs and the wx mutants (Figure 6). The WT data revealed that GT was negatively correlated with both DP 6–12 and ACR but was positively correlated with DP 13–24. The AAC was positively correlated with peak time, CPV, HPV, and SBV, but was negatively correlated with BDV and GC. On the other hand, the data from wx mutants indicated that GT was negatively correlated with DP 6–12, DP 6–24, and ACR, but was positively correlated with DP 13–24, peak time, CL, and DP 25–100. The AAC of wx mutants showed a negative correlation with GC and a positive correlation with peak time, HPV, and CPV. The results indicated that amylopectin structure, AAC, GT, and pasting properties in different rice cultivars reflected different physicochemical properties.
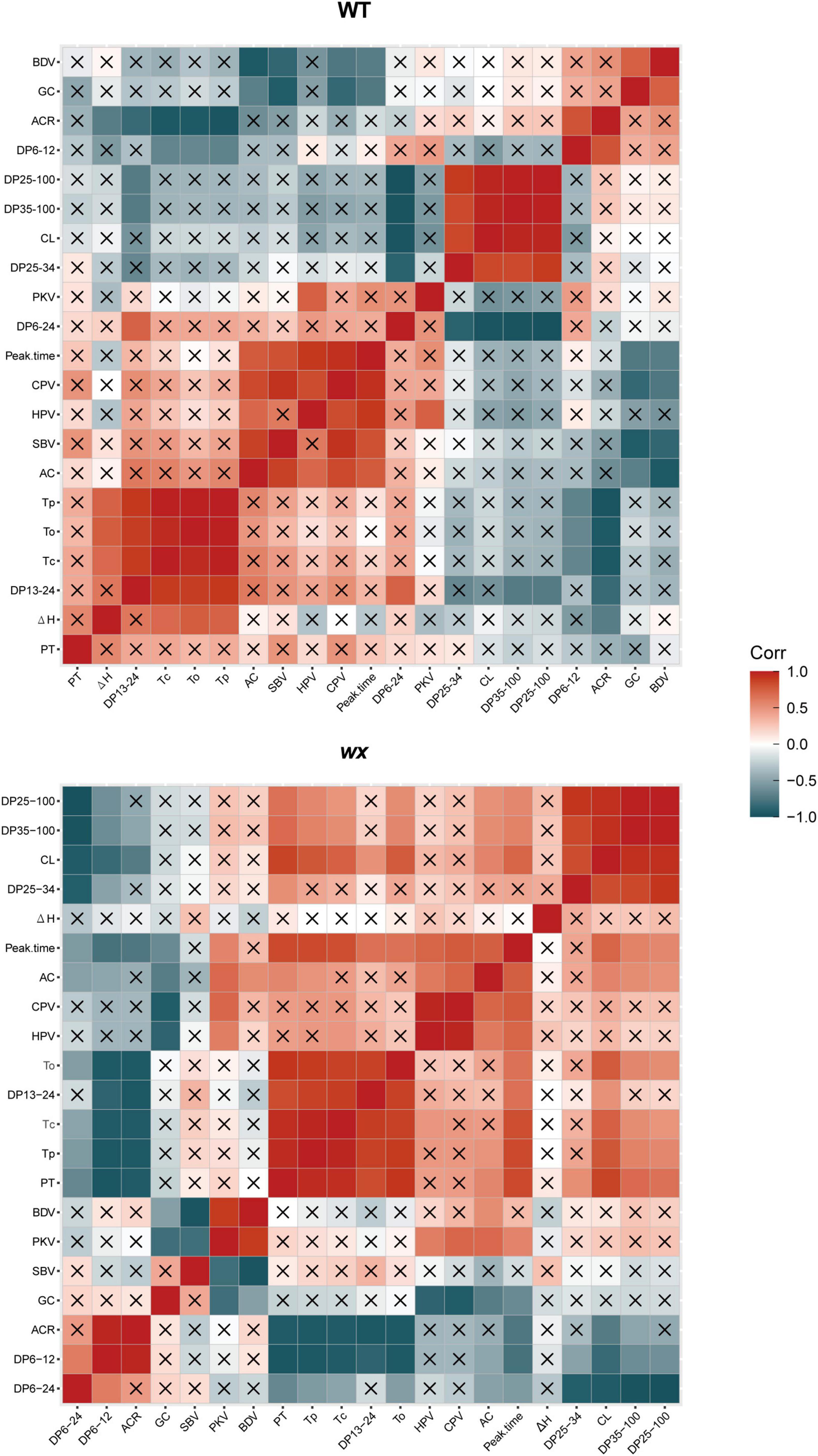
Figure 6. Pearson’s correlation analysis of the relationship between amylopectin structure of wx mutants and their corresponding WTs. The stronger the red color, the more significant the positive correlation; the stronger the bluish-black color, the more significant the negative correlation. “×” means no correlation. ΔH, gelatinization enthalpy; To, onset temperature; Tp, peak temperature; Tc, conclusion temperature; AC, amylose content; PKV, peak viscosity; HPV, hot paste viscosity; CPV, cool paste viscosity; BDV, breakdown viscosity; SBV, setback viscosity; PT, pasting temperature; ACR value, amylopectin chain ratio; CL, chain length; GC, gel consistency.
Discussion
Over the past few decades, many waxy rice cultivars have been developed by conventional plant breeding techniques and mutagenesis. However, these methods have the disadvantages of unpredictable starch quality, as well as being time-consuming and laborious (Toda, 1980; Deng, 1992; Olsen and Purugganan, 2002). Recently, the development of genome-editing technology, particularly the discovery and application of the CRISPR/Cas9 gene-editing system, has led to its widespread use in studying plant genome functions (Ran et al., 2013; Belhaj et al., 2015; Bortesi and Fischer, 2015). Several studies have reported that the Wx gene can be successfully edited with high efficiency in rice through the use of the CRISPR/Cas9 system, which shows a high average mutation rate of up to 80%, without affecting the major agronomic traits in the transgenic lines (Zhang et al., 2018; Yunyan et al., 2019; Huang et al., 2020). In agreement with previous studies, the wx mutation rate in the present study showed a high value of 80% and above, and wx mutants exhibited major agronomic traits similar to those of their corresponding WT lines. However, in this study, we identified the Wx allele and the starch quality of the WT lines before editing and compared the major starch quality traits of the wx mutants from parents with different Wx alleles, an approach that is different from that undertaken in previous studies.
Our results showed that the AAC values of three wxb mutants were lower than that of wxa mutants (Supplementary Tables 2, 6), and WT parents with a lower level of AAC will generate wx mutants with lower AAC values. The AAC of wxa mutants was higher than the 2% upper threshold, a characteristic that is widely believed to be unacceptable for the AAC of elite waxy rice grains (Juliano, 1992). Therefore, our results indicate that it is necessary to select parental rice varieties with a lower level of AAC to obtain high-quality waxy rice grains in the breeding program (conventional or mutagenesis breeding). A recent study reported that silencing the expression of the Wx gene regulates genes related to amylose synthesis, with upregulation of granule-bound starch synthase II (GBSSII) compensating for some loss of amylose in the rice endosperm (Pérez et al., 2019). This may explain why the same Wx parental genotype results in different AAC levels after gene editing (Figure 5A), and further studies are warranted to study the contribution of different GBSSII types to the amylose content in endosperm in order to develop new waxy rice varieties with more precise and desirable AAC levels.
Amylopectin is also proposed to have a significant influence on the physicochemical properties of waxy rice flour (Man et al., 2013; Huang and Lai, 2014). Previous studies had shown that some starch-synthesizing enzymes influence the structure of amylopectin, such as soluble starch synthases (SSs), branching enzymes (BEs), debranching enzymes (DBEs), and isoamylase 1 (ISAI) (Umemoto et al., 1995; Man et al., 2013; Shufen et al., 2019; Zhu et al., 2020). These observations may rationally explain the differences in chain-length distributions of amylopectin in different wx mutants obtained from the same Wx allele (Figures 4, 5 and Supplementary Figure 4). The expression of the major starch synthesis-related genes is upregulated following the silencing of the expression of the Wx gene (Pérez et al., 2019), leading to the differences in chain-length distributions of amylopectin observed in wx mutants and their corresponding WTs (Figure 4 and Supplementary Figure 4). Although the chain-length distributions of amylopectin were changed, the range of ACR values was not significantly different (Figure 5). Based on the ACR values, three types of amylopectin could be distinguished, namely L-type (ACR < 0.2), M-type (0.2–0.24), and S-type (ACR > 0.24). The GT of rice can also be divided into three types: low-type (<70°C), intermediate-type (70–74°C), or high-type (>74°C), and is negatively correlated with ACR (Kongseree and Juliano, 1972; Nakamura et al., 2002; Vandeputte et al., 2003). In the current study, we found that wx mutants exhibited ACR values and amylopectin types similar to those of their corresponding WTs, and that the ACR value was linearly correlated with GT, which means that the breeder can obtain a waxy rice genotype with a GT and an amylopectin structural type similar to those of the corresponding WT. We also distinguished two amylopectin structure types based on ACR values: HGT-type (ACR < 0.18) and LGT-type (ACR > 0.18). The GT of the HGT-type is more than 77°C, in contrast to that of the LGT-type, which is less than 72°C (Table 1). Collectively, it can be easier to discriminate between amylopectin structure types selected on the basis of GT, which could benefit breeding for rice with specific waxy starch characteristics.
Previous studies had tended to indicate that, in all rice cultivars, the GT was negatively correlated with short amylopectin chains (DP 6–12) and ACR values, but positively correlated with the medium (12 ≤ DP ≤ 24) and long amylopectin chains (DP > 37) (Wang and Wang, 2002; Vandeputte et al., 2003; Wong et al., 2003). However, our study indicated that GT was only significantly negatively correlated with ACR and DP 6–12 when the genotypes were WTs, but was significantly positively correlated with DP 25–100 when all were wx mutants or waxy rice genotypes (Figure 6). Therefore, carrying out a statistical analysis of starch quality data combined from both non-waxy and waxy genotypes may not be the best way to identify a relationship between the physicochemical properties. On the basis of the above-mentioned results, we suggest that analyzing the relationship between the physicochemical properties of the same variety (waxy or non-waxy) can provide greater accuracy, and that selection on the basis of ACR value can more effectively identify genotypes with specific GT values.
Conclusion
In the current study, five new waxy rice mutants were generated, using the CRISPR/Cas9 system to edit the Wx gene. Detailed analysis of the two wx mutants from QLD and YSZ showed a significant decrease in AAC and viscosity compared with the corresponding WT, and a significant increase in GC and total protein content, with the grain yields of both mutants being higher than those achieved by the two parental elite cultivated rice WTs. Based on the ACR value, two types of amylopectin structure are proposed: HGT-type (ACR < 0.18; GT > 77°C) and LGT-type (ACR > 0.18; GT < 72°C). Our study also found that WTs with a low level of AAC generated wx mutants with lower AAC, with the wx mutants also having values of ACR, GT, and amylopectin structure types similar to those of their corresponding WTs, suggesting that parents with targets of specific GT values and low initial AAC should be selected for in breeding programs. In conclusion, we propose a novel strategy for generating waxy rice genotypes directly, and provide new insights into expanding waxy rice germplasm resources for use in breeding programs.
Materials and Methods
Plant Materials and Growth Conditions
We selected five elite rice varieties with different genetic backgrounds, including four indica varieties (YSZ, QLD, SH789, and HZ) and one japonica variety (TJG), which were mainly planted in eastern Asia, central China, and western China. The seed was supplied by the Rice Research Institute of Sichuan Agricultural University. The japonica waxy line NY1 and the indica waxy line NY2 were used as controls. Unless indicated, all rice lines were grown in paddy fields in Chengdu, China, during the normal rice-growing seasons.
CRISPR/Cas9 Gene-Editing and Gene Cloning
The CRISPR/Cas9-targeted genome editing tool was constructed and operated as previously described (Feng et al., 2013). The primer sequences used to construct the vector are shown in Supplementary Table 7.
The polymerase chain reaction (PCR) amplification conditions were as follows: initial denaturation at 94°C for 2 min, followed by 35 cycles of denaturation at 94°C for 30 s, annealing at 56°C for 30 s, extension at 72°C for 30 s, followed by a final extension at 72°C for 5 min, with the PCR mix being based on the Foregene PCR Fast Mix. The PCR products were sequenced by the Sanger method. The primers used for Wx sequences, the Wx allele genotype, target sequences, and reporter gene detection are listed in Supplementary Table 7.
Tasting Properties of Waxy Rice
A sample (30 g) of milled waxy rice grains was cooked in an electric rice cooker to determine the tasting properties (model MDFBZ02ACM, Xiaomi, China). The tasting quality of milled waxy rice was determined with an RCTA-11A Taste Analyzer (Satake, Japan) (Zhang et al., 2016).
Measurements of Waxy Rice Starch
Grains of a single wx mutant with stable inheritance in the T5 generation were dried at 37°C for 2 weeks. The dried grains were shelled, polished, and milled by a pearling rice mill, and finally screened through a 74-μ mesh. Starch was prepared by the method of Precha-Atsawanan et al. (2018). The waxy flour was steeped in 0.35% sodium hydroxide solution and incubated at 4°C for 48 h. The precipitants in the suspension were collected by centrifugation at 3,000 g for 15 min. The starch layer was re-mixed with water to which was added 1% alkaline protease (Sigma), and the pH was adjusted to 9 with sodium hydroxide and incubated at 37°C for 8 h. The washing step was repeated at least three times or until the pH value reached 7. The final precipitant was collected by centrifuging at 3,000 g for 30 min, and the starch was dried in a warm air oven at 40°C for 48 h. The starch was ground with a mortar and pestle, using a 0.08-mm sieve to collect the starch powder.
Quantification of Starch and Amylose
The rice flour was equilibrated in a constant temperature and humidity cabinet for 7 days. The starch content was determined according to the method of Smith and Zeeman (2006). D-glucose was stained with the GOPOD reagent (glucose oxidase plus peroxide and 4-aminoantipyrin) and determined by absorbance at 510 nm. Amylose content was measured as described in GB/T 15683-2008/ISO 6647-1: 2007. The amylase-iodine blue color was determined at 720 nm. Standard curves were plotted with standardized samples of rice amylose and amylopectin (China National Rice Research Institute, Zhejiang, China).
Quantification of Gel Consistency and Total Protein
The gel consistency (GC) of grains was evaluated as described previously (Cagampang et al., 1973). A sample (100 ± 1 mg) of the starch powder was placed into a 13 × 100 mm tube and wetted with 0.2 ml of 95% ethanol containing 0.025% thymol blue, and then 2.0 ml of 0.2 M KOH was added and allowed to boil for 3 min. GC was measured in ml as the length (mm) of the gel spreading in the tubes when laid flat on the graph for 1 h. The total protein content of the grain was assayed by the Kjeldahl method according to the Comin amylose assay procedure (Suzhou Comin Biotechnology Co., Ltd).1
Waxy Rice Powder Pasting Properties
To the white rice flour (3 g at 14% moisture content) obtained from the T5 transformant generation was added 25 ml of ddH2O2. The setting procedure included the following steps: incubation for 1 min at 50°C, increasing the temperature at a rate of 12°C/min to 95°C (over 3.75 min), maintaining the temperature at 95⋅C (for 2.5 min), then decreasing it to 50°C (over a period of 3.75 min) at 12°C/min, and maintaining the temperature at 50°C (for 2 min). The initial speed was set to 960 r/min for 10 s and then adjusted to 160 r/min. The pasting parameters of waxy rice flour, such as the peak viscosity (PKV), hot paste viscosity (HPV), cool paste viscosity (CPV), breakdown viscosity (BDV = PKV − HPV), setback viscosity (SBV = CPV − HPV), and pasting temperature (PT), were evaluated using a rapid viscosity analyzer (RVA, Newport Scientific, Australia) according to the methods of Chung et al. (2011).
Thermal Properties of Waxy Rice Starch
The thermal properties of waxy rice starch were measured using differential scanning calorimetry (DSC Q2000; TA Instruments Ltd., Crawley, United Kingdom) (Yang et al., 2018). The onset temperature (To), peak temperature (Tp), conclusion temperature (Tc), and gelatinization enthalpy (ΔH) were recorded using a Universal Analysis 2000 (TA Instruments Ltd., Crawley, United Kingdom). A sample (3 mg) of starch was mixed with 6 μl of distilled water, and the paste was added to an aluminum pan and incubated at 4°C for 24 h. The specimens were then heated from 30 to 100°C at a rate of increase of 10°C/min.
Amylopectin Chain-Length Distribution
The chain-length distribution of amylopectin was analyzed according to the method of Kowittaya and Lumdubwong (2014). Waxy rice starch was suspended in 95% (v/v) ethanol and boiled for 60 min. Hydrolyzed polyglucan was treated with Pseudomonas amyloderamosa isoamylase (1,000 U/μl, Sigma). The chain-length distribution of waxy rice starch was analyzed by high-performance anion-exchange chromatography with pulsed amperometric detection (HPAEC-PAD). There were three mobile phases: A (aqueous solution), B (100 mM NaOH and 1 M NaAC), and C (100 mM NaOH). The flow rate was controlled at 0.4 ml/min, and the column temperature was controlled at 30°C.
Data Analysis
All experiments were independently repeated three times. The results were expressed as the mean ± standard deviation. The correlation was assessed using Pearson’s correlation coefficient. Data analysis was performed with SPSS 25.0, and the significance was defined as P < 0.05 (* or lowercase letters) and P < 0.01 (** or uppercase letters). SnapGene 5.2 was used to visualize PCR and cloning.
Data Availability Statement
The original contributions presented in the study are included in the article/Supplementary Material, further inquiries can be directed to the corresponding author.
Author Contributions
YF, TL, and JZ designed the strategy. YH, XY, XL, YL, YPL, BZ, RL, and ZZ completed part of the experiments. YF and JZ organized the figures and article modification. YF, TL, and JZ analyzed the data and wrote the manuscript. All authors commented on the manuscript.
Funding
We acknowledge funding from the Sichuan Science and Technology Program (Grant Nos. 2021YFN0043, 2020ZHFP0017, and 2020YFN0006) and the Chengdu Science and Technology Program (Grant No. 2021-YF05-02115-SN).
Conflict of Interest
The authors declare that the research was conducted in the absence of any commercial or financial relationships that could be construed as a potential conflict of interest.
Publisher’s Note
All claims expressed in this article are solely those of the authors and do not necessarily represent those of their affiliated organizations, or those of the publisher, the editors and the reviewers. Any product that may be evaluated in this article, or claim that may be made by its manufacturer, is not guaranteed or endorsed by the publisher.
Acknowledgments
We thank the Sichuan Science and Technology Program and the Chengdu Science and Technology Program for supporting this work.
Supplementary Material
The Supplementary Material for this article can be found online at: https://www.frontiersin.org/articles/10.3389/fpls.2022.881964/full#supplementary-material
Footnotes
References
Bao, J., Corke, H., and Sun, M. (2004). Genetic diversity in the physicochemical properties of waxy rice (Oryza sativa L) starch. J. Sci. Food Agric. 84, 1299–1306.
Belhaj, K., Chaparro-Garcia, A., Kamoun, S., Patron, N. J., and Nekrasov, V. (2015). Editing plant genomes with CRISPR/Cas9. Curr. Opin. Biotechnol. 32, 76–84.
Bortesi, L., and Fischer, R. (2015). The CRISPR/Cas9 system for plant genome editing and beyond. Biotechnol. Adv. 33, 41–52. doi: 10.1016/j.biotechadv.2014.12.006
Cagampang, G. B., Perez, C. M., and Juliano, B. O. (1973). A gel consistency test for eating quality of rice. J. Sci. Food Agric. 24, 1589–1594. doi: 10.1002/jsfa.2740241214
Chen, M. H., Bergman, C., Pinson, S. R. M., and Fjellstrom, R. G. (2008). Waxy gene haplotypes associations with pasting properties in an international rice germplasm collection. J. Cereal Sci. 48, 781–788.
Chun, A. R., Kim, D. J., Yoon, M. R., Oh, S. K., and Ju, S. C. (2010). Variation in quality and preference of sogokju (Korean traditional rice wine) from waxy rice varieties. Korean J. Cropence 55, 177–186.
Chung, H. J., Liu, Q. A., Lee, L., and Wei, D. Z. (2011). Relationship between the structure, physicochemical properties and in vitro digestibility of rice starches with different amylose contents. Food Hydrocoll. 25, 968–975. doi: 10.1111/jpn.13512
Chung, J. H., Han, J. A., Yoo, B., Seib, P. A., and Lim, S. T. (2008). Effects of molecular size and chain profile of waxy cereal amylopectins on paste rheology during retrogradation. Carbohydr. Polym. 71, 365–371.
Dobo, M., Ayres, N., Walker, G., and Park, W. D. (2010). Polymorphism in the GBSS gene affects amylose content in US and European rice germplasm. J. Cereal Sci. 52, 450–456.
Feng, Z., Zhang, B., Ding, W., Liu, X., Yang, D. L., Wei, P., et al. (2013). Efficient genome editing in plants using a CRISPR/Cas system. Cell Res. 23, 1229–1232. doi: 10.1038/cr.2013.114
Guo, L., Li, J., Gui, Y., Zhu, Y., and Cui, B. (2019). Improving waxy rice starch functionality through branching enzyme and glucoamylase: role of amylose as a viable substrate. Carbohydr. Polym. 230:115712. doi: 10.1016/j.carbpol.2019.115712
Hizukuri, S. (1986). Polymodal distribution of the chain lengths of amylopectins, and its significance. Carbohydr. Res. 147, 342–347.
Hizukuri, S., Takeda, Y., Yasuda, M., and Suzuki, A. (1981). Multi-branched nature of amylose and the action of debranching enzymes. Carbohydr. Res. 94, 205–213.
Huang, L. C., Li, Q. F., Zhang, C. Q., Chu, R., Gu, Z. W., Tan, H. Y., et al. (2020). Creating novel Wx alleles with fine-tuned amylose levels and improved grain quality in rice by promoter editing using CRISPR/Cas9 system. Plant Biotechnol. J. 18, 2164–2166. doi: 10.1111/pbi.13391
Huang, Y. C., and Lai, H. M. (2014). Characteristics of the starch fine structure and pasting properties of waxy rice during storage. Food Chem. 152, 432–439. doi: 10.1016/j.foodchem.2013.11.144
Jane, J., Chen, Y. Y., Lee, L. F., McPherson, A. E., Wong, K. S., Radosavljevic, M., et al. (1999). Effects of amylopectin Branch Chain length and amylose content on the gelatinization and pasting properties of starch. Cereal Chem. J. 76, 629–637.
Juliano, B. O. (1992). Structure, chemistry, and function of the rice grain and its fractions. Cereal foods world 37, 772–779.
Juliano, B. O. (1998). Varietal impact on rice quality. Cereal Foods World 43, 207–211,214–216,218–222. doi: 10.1007/s11306-020-01670-6
Kalichevsky, M. T., Orford, P. D., and Ring, S. G. (1990). The retrogradation and gelation of amylopectins from various botanical sources. Carbohydr. Res. 198, 49–55.
Kong, X., Kasapis, S., and Bao, J. (2015). Viscoelastic properties of starches and flours from two novel rice mutants induced by gamma irradiation. LWT Food Sci. Technol. 60, 578–582.
Kongseree, N., and Juliano, B. O. (1972). Physicochemical properties of rice grain and starch from lines differing in amylose content and gelatinization temperature. J. Agric. Food Chem. 20, 714–718.
Kowittaya, C., and Lumdubwong, N. (2014). Molecular weight, chain profile of rice amylopectin and starch pasting properties. Carbohydr. Polym. 108, 216–223. doi: 10.1016/j.carbpol.2014.02.081
Larkin, P., and Park, W. (2003). Association of waxy gene single nucleotide polymorphisms with starch characteristics in rice (Oryza sativa L.). Molecular Breeding 12, 335–339.
Liu, Q., Wang, Z., Chen, X., Cai, X., Tang, S., Yu, H., et al. (2003). Stable inheritance of the antisense Waxy gene in transgenic rice with reduced amylose level and improved quality. Transgenic Res. 12, 71–82. doi: 10.1023/a:1022148824018
Liu, Q. Q., Yu, H. X., Chen, X. H., Cai, X. L., Tang, S. Z., Wang, Z. Y., et al. (2005). Field performance of transgenicindicahybrid rice with improved cooking and eating quality by down-regulation ofWxGene expression. Mol. Breed. 16, 199–208.
Lu, T.-J., Duh, C.-S., Lin, J.-H., and Chang, Y.-H. (2008). Effect of granular characteristics on the viscoelastic properties of composites of amylose and waxy starches. Food Hydrocoll. 22, 164–173.
Ma, X., Zhang, Q., Zhu, Q., Liu, W., Chen, Y., Qiu, R., et al. (2015). A robust CRISPR/Cas9 system for convenient, high-efficiency multiplex genome editing in monocot and dicot plants. Mol. Plant 8, 1274–1284. doi: 10.1016/j.molp.2015.04.007
Man, J., Yang, Y., Huang, J., Zhang, C., Chen, Y., Wang, Y., et al. (2013). Effect of simultaneous inhibition of starch branching enzymes I and IIb on the crystalline structure of rice starches with different amylose contents. J. Agric. Food Chem. 61, 9930–9937. doi: 10.1021/jf4030773
Mikami, I., Uwatoko, N., Ikeda, Y., Yamaguchi, J., Hirano, H. Y., Suzuki, Y., et al. (2008). Allelic diversification at thewxlocus in landraces of Asian rice. Theor. Appl. Genet. 116, 979–989.
Nakamura, Y., Sakurai, A., Inaba, Y., Kimura, K., Iwasawa, N., and Nagamine, T. (2002). The fine structure of amylopectin in endosperm from Asian cultivated rice can be largely classified into two classes. Starch Stärke 54, 117–131.
Olsen, K. M., and Purugganan, M. D. (2002). Molecular evidence on the origin and evolution of glutinous rice. Genetics 162, 941–950. doi: 10.1093/genetics/162.2.941
Pérez, L., Soto, E., Farré, G., Juanos, J., Villorbina, G., Bassie, L., et al. (2019). CRISPR/Cas9 mutations in the rice Waxy/GBSSI gene induce allele-specific and zygosity-dependent feedback effects on endosperm starch biosynthesis. Plant Cell Rep. 38, 417–433. doi: 10.1007/s00299-019-02388-z
Precha-Atsawanan, S., Puncha arnon, S., Wandee, Y., Uttapap, D., Puttanlek, C., and Rungsardthong, V. (2018). Physicochemical properties of partially debranched waxy rice starch. Food Hydrocoll. 79, 71–80.
Puchongkavarin, H., Varavinit, S., and Bergthaller, W. (2005). Comparative study of pilot scale rice starch production by an alkaline and an enzymatic process. Starch 57, 134–144.
Ran, F. A., Hsu, P. D., Wright, J., Agarwala, V., Scott, D. A., and Zhang, F. (2013). Genome engineering using the CRISPR-Cas9 system. Nat. Protoc. 8, 2281–2308.
Ren, J., Li, Y., Li, C., Gu, Z., Cheng, L., Hong, Y., et al. (2017). Pasting and thermal properties of waxy corn starch modified by 1,4-alpha-glucan branching enzyme. Int. J. Biol. Macromol. 97, 679–687. doi: 10.1016/j.ijbiomac.2017.01.087
Sano, Y. (1984). Differential regulation of waxy gene expression in rice endosperm. Theor. Appl. Genet. 68, 467–473. doi: 10.1007/BF00254822
Sasaki, T., Yasui, T., and Matsuki, J. (2000). Effect of amylose content on gelatinization, retrogradation, and pasting properties of starches from Waxy and Nonwaxy Wheat and their F1 seeds. Cereal Chem. 77, 58–63.
Satoh, H., and Omura, T. (1981). New endosperm mutations induced by chemical mutagens in rice Oryza sativa L. Jap. J. Breed. 31, 316–326.
Shufen, C., Yicong, C., Baobing, F., Guiai, J., Zhonghua, S., Ju, L., et al. (2019). Editing of rice isoamylase gene ISA1 provides insights into its function in starch formation. Rice Sci. 26, 77–87.
Singh, H., Lin, J. H., Huang, W. H., and Chang, Y. H. (2012). Influence of amylopectin structure on rheological and retrogradation properties of waxy rice starches. J. Cereal Sci. 56, 367–373. doi: 10.1016/j.carbpol.2015.12.025
Smith, A. M., and Zeeman, S. C. (2006). Quantification of starch in plant tissues. Nat. Protoc. 1, 1342–1345. doi: 10.1038/nprot.2006.232
Sorndech, W., Meier, S., and Jansson, A. (2015). Synergistic amylomaltase and branching enzyme catalysis to suppress cassava starch digestibility. Carbohydr. Polym. 132, 409–418. doi: 10.1016/j.carbpol.2015.05.084
Teng, B., Zeng, R-z, Wang, Y., Liu, Z., Zhang, Z., Zhu, H., et al. (2011). Detection of allelic variation at the Wx locus with single-segment substitution lines in rice (Oryza sativa L.). Mol. Breed. 30, 583–595.
Umemoto, T., Nakamura, Y., and Ishikura, N. (1995). Activity of starch synthase and the amylose content in rice endosperm. Phytochemistry 40, 1613–1616.
Vandeputte, G. E., Derycke, V., Geeroms, J., and Delcour, J. A. (2003). Rice starches. II. Structural aspects provide insight into swelling and pasting properties. J. Cereal Sci. 38, 53–59.
Wang, Y.-J., and Wang, L. (2002). Structures of four waxy rice starches in relation to thermal, pasting, and textural properties. Cereal Chem. 79, 252–256.
Wang, Z. Y., Zheng, F. Q., Shen, G. Z., Gao, J. P., Snustad, D. P., Li, M. G., et al. (1995). The amylose content in rice endosperm is related to the post-transcriptional regulation of the waxy gene. Plant J. 7, 613–622. doi: 10.1046/j.1365-313x.1995.7040613.x
Wong, K.-S., Kubo, A., Jane, J-l, Harada, K., Satoh, H., and Nakamura, Y. (2003). Structures and properties of amylopectin and phytoglycogen in the endosperm of sugary-1 mutants of rice. J. Cereal Sci. 37, 139–149.
Yang, T., Tan, X., Huang, S., Pan, X., Shi, Q., Zeng, Y., et al. (2018). Effects of experimental warming on physicochemical properties of indica rice starch in a double rice cropping system. Food Chem. 310:125981.
Yunyan, F., Jie, Y., Fangquan, W., Fangjun, F., Wenqi, L., Jun, W., et al. (2019). Production of two elite glutinous rice varieties by editing Wx gene. Rice Sci. 26, 118–124.
Zhang, C., Zhou, L., Zhu, Z., Lu, H., Zhou, X., Qian, Y., et al. (2016). Characterization of grain quality and starch fine structure of two japonica rice (Oryza sativa) cultivars with good sensory properties at different temperatures during the filling stage. J. Agric. Food Chem. 64, 4048–4057. doi: 10.1021/acs.jafc.6b00083
Zhang, C., Zhu, J., Chen, S., Fan, X., Li, Q., Lu, Y., et al. (2019). Wx(lv), the ancestral allele of rice Waxy gene. Mol. Plant 12, 1157–1166. doi: 10.1016/j.molp.2019.05.011
Zhang, J., Zhang, H., Botella, J. R., and Zhu, J.-K. (2018). Generation of new glutinous rice by CRISPR/Cas9-targeted mutagenesis of the Waxy gene in elite rice varieties. J. Integr. Plant Biol. 60, 369–375. doi: 10.1111/jipb.12620
Keywords: apparent amylose content, waxy rice, amylopectin structure, Waxy gene, CRISPR/Cas9
Citation: Fu Y, Luo T, Hua Y, Yan X, Liu X, Liu Y, Liu Y, Zhang B, Liu R, Zhu Z and Zhu J (2022) Assessment of the Characteristics of Waxy Rice Mutants Generated by CRISPR/Cas9. Front. Plant Sci. 13:881964. doi: 10.3389/fpls.2022.881964
Received: 24 February 2022; Accepted: 13 May 2022;
Published: 10 June 2022.
Edited by:
Tofazzal Islam, Bangabandhu Sheikh Mujibur Rahman Agricultural University, BangladeshReviewed by:
Mohd. Kamran Khan, Selcuk University, TurkeySulaiman Ahmed, Institute of Plant Physiology and Ecology, Shanghai Institutes for Biological Sciences (CAS), China
Copyright © 2022 Fu, Luo, Hua, Yan, Liu, Liu, Liu, Zhang, Liu, Zhu and Zhu. This is an open-access article distributed under the terms of the Creative Commons Attribution License (CC BY). The use, distribution or reproduction in other forums is permitted, provided the original author(s) and the copyright owner(s) are credited and that the original publication in this journal is cited, in accordance with accepted academic practice. No use, distribution or reproduction is permitted which does not comply with these terms.
*Correspondence: Jun Zhu, Wmh1anVuOTg3QDEyNi5jb20=
†These authors have contributed equally to this work