- 1College of Agronomy, Shenyang Agricultural University, Shenyang, China
- 2Crop Research Institute, Anhui Academy of Agricultural Sciences, Hefei, China
- 3Institute of Economic Crop, Shanxi Academy of Agricultural Sciences, Fenyang, China
- 4Institute of Crop Germplasm Resources, Jilin Academy of Agricultural Sciences, Changchun, China
Seed germination is the sensitive period to salt stress. Calcium chloride (CaCl2) has been proved as an effective priming agent which can promote the sorghum germination under salt stress. However, there are few reports on CaCl2 priming to improve the salt tolerance during seed germination. The present study investigated the effects of CaCl2 priming on sorghum germination, antioxidant metabolism, osmotic regulation and ion balance under salt stress (150 mM NaCl). The results revealed that the salt stress inhibited the elongation of mesocotyl and root and reduced the germination rate of sorghum. While CaCl2 priming significantly promoted the elongation of mesocotyl and root, and increased the germination rate of sorghum under salt stress. CaCl2 priming notably increased the content of osmotic substances in mesocotyl and root of sorghum under salt stress, and increased the relative water content in these tissues. CaCl2 priming decreased Na+ content and increased K+, Ca2+ contents and the K+/ Na+ in mesocotyl and root, such effects might be induced by up-regulating the expression of NHX2, NHX4, SOS1, AKT1, AKT2, HKT1, HAK1, and KUP. CaCl2 priming reduced the antioxidant enzymes activities and related gene expression compared with untreated sorghum seeds under salt stress. In short, CaCl2 priming improved sorghum germination by enhancing osmotic regulation and ion balance instead of antioxidant enzyme activity. However, the molecular mechanisms of Ca2+ signaling induced by CaCl2 priming in association with the enhanced germination in primed sorghum seeds under salt stress need to be addressed in future studies.
Introduction
Worldwide, land salinization is a serious environmental problem. More than 6% of world land area is saline and 20% of irrigated lands are affected by salinity (Song and Wang, 2014; Yuan et al., 2016). sorghum is a highly resistant crop and has received extensive attention due to its huge production potential that can meet the challenges of global food security (Hao et al., 2021). However, sorghum is very sensitive to salt during the germination stage. Salt stress will affect emergence and establishment of early seedlings, and reduce the final yield (Zhu et al., 2018). Seed germination is critical to successful crop production (Hajihashemi et al., 2020). There are several reports about salt stress delaying and inhibiting seed germination and plant growth (Ning et al., 2019; Sheteiwy et al., 2020). Therefore, improving seed germination under salt stress is of great significance for improving the emergence and production of sorghum in saline soil.
Seed priming is a convenient, inexpensive, and low-risk means that can effectively improve the emergence of crops in saline soil. It is also the most commonly used technical intervention for farmers to improve seed germination (Hussain et al., 2018). Seed priming refers to initially exposing the seed to a promoter that makes the seed more resistant to future stress (Tanou et al., 2012). Partial hydration of seeds in a specific environment occurs during priming (Giri and Schillinger, 2003). Priming activates positive metabolic processes for seeds to resist different abiotic stresses (Jisha et al., 2013). Previous studies revealed that seed priming can significantly alleviate the inhibition of salt stress on crop seed germination (Shah et al., 2020; Chen et al., 2021).
Environmental changes lead to frequent occurrences of abiotic stress and severe damage to plant health (Abdoulaye et al., 2019). Changes of substances metabolism and gene expression occur in plant tissue and cell under abiotic stress especially salt stress (Ibrahim, 2016; He et al., 2017; Gao et al., 2020). The effects of salt stress on crops involve primary stress and secondary stress. The former includes osmotic stress and ion stress, and the latter includes the production of reactive oxygen species (ROS) (Wani et al., 2018; Yang and Guo, 2018). Under salt stress, high concentrations of salt ions reduce the free energy of water and the resulting decline in the water potential of the surrounding environment leads to osmotic stress in plant growth. Therefore, plants experience difficulty in absorbing water, leading to plant water deficit. The osmotic regulators, such as proline, amino acids, soluble sugars, and soluble proteins, plays a vital role during seed germination under salt stress, which can reduce cell osmotic potential and promote cell water absorption and seed germination (Ibrahim, 2016). Various studies suggest that seed priming can enhance the ability of osmotic adjustment in plants under salt stress (Tabassum et al., 2017; Bajwa et al., 2018; Rehman et al., 2021).
High concentration of sodium ions is the main cause of salt induced ion stress (Ismail et al., 2014). Seed germination is inhibited by salt due to the toxic effects of sodium ions on embryo activity, including structural damage to macromolecular substances and the cell membrane (Daszkowska-Golec, 2011). Salt is absorbed by roots and transported to all parts of the plant. The accumulation of sodium ions in cytoplasm will affect the absorption of other ions, such as potassium ions, which in turn affects the normal physiological metabolism of plants. For instance, potassium ions are crucial for the catalytic activity of many enzymes (Fu and Luan, 1998; Lazof and Bernstein, 1998; Munns and Tester, 2008). Calcium is an important component of cell structure and involved in cell elongation and cell division processes. It plays a significant role in regulating nutrient absorption across cell membranes and improving water absorption (Summart et al., 2010). In addition, Ca2+ can alleviate the negative effects of Na+ on plant growth (Gobinathan et al., 2009). Therefore, maintaining the ion homeostasis in plant cells under salt stress is essential to improve salt tolerance. The role of seed priming in maintaining ion homeostasis under salt stress has been reported in many literatures (Bajwa et al., 2018; Mahboob et al., 2019; Wang et al., 2019). However, there are fewer reports on the homeostasis of calcium and sodium ions than those on the homeostasis of potassium and sodium ions. Secondary stress induced by salt, such as excessive accumulation of ROS, can cause serious damage to cell structure and macromolecular substances (Genisel et al., 2015; Yang and Guo, 2018). An evolved complex antioxidant system in plants can remove excess ROS and reduce cellular oxidative damage. The ROS scavenging system includes non-enzymatic antioxidants, such as proline, and antioxidant enzymes, including the superoxide dismutase (SOD), peroxidase (POD), catalase (CAT), and ascorbate peroxidase (APX) (El-Beltagi et al., 2020). Antioxidant substances activity and synthesis are affected by abiotic stress (Sheteiwy et al., 2021; Yang et al., 2021). Although most studies have indicated that seed priming alleviates salt stress damage through enhanced antioxidant activity (Jiang et al., 2020; Soliman et al., 2020), a few studies have shown that seed priming did not enhance antioxidant enzyme activities. For example, Genisel et al. (2015) reported that cysteine priming reduced the production of ROS under salt stress and reduced oxidative damage, but the activity of antioxidant enzymes was significantly decreased. It was inferred that the reduction of cysteine alone led to a decrease in the demand for the antioxidant system. Therefore, further studies are needed to determine whether seed priming enhances the comprehensive resistance mechanism induced by salt stress, since the secondary stress is fundamentally caused by the primary stress.
Calcium chloride has been proved to be the most effective priming agent to promote sorghum germination under salt stress (Chen et al., 2021), but the physiological effects of calcium chloride are not well understood. The purpose of this study was to investigate (1) the effect of calcium chloride on osmotic adjustment, ion homeostasis. and antioxidant metabolism during sorghum germination under salt stress; and (2) the metabolic pathway(s) through which calcium chloride plays a key role in alleviating the inhibition of salt stress on sorghum germination.
Materials and Methods
Seed Material and Experimental Design
Sorghum (Sorghum bicolor (L.) Moench) seeds of Liaoza15 used in this study were provided by the Liaoning Academy of Agricultural Sciences. Uniform seeds were disinfected with 5% NaClO solution for 5 min, then rinsed with distilled water three to five times, and wiped the surface water. Seeds were primed with distilled water or CaCl2 solution for 12 h at 25°C in the dark, and the ratio of seed weight to solution volume was 1:5 (w/v). Then, the seeds were dried to the original moisture content at room temperature (25°C) after wiping the surface moisture. Preliminary test results showed that CaCl2 solution with a concentration of 6 g.L–1 had the best priming effect. The no primed seeds were, respectively, cultured under distilled water (NPN) or 150 mM NaCl (NPS). The seeds subjected to hydro-priming (HPS) and CaCl2 priming (CaPS) were both cultured under NaCl stress. The seeds were incubated in a Petri dish covered with double-layer filter paper, and 10 mL of distilled water or NaCl solution was added to each Petri dish. The Petri dish was placed in a completely dark climate-controlled incubator at a temperature of 25 ± 1°C and 70% humidity. Each treatment was repeated three times, and each replicate contained 50 seeds. The number of germinated seeds were counted on the 7th day and the germination rate was calculated. The germination was determined to have occurred once the radicle length was equal to the seed length and the bud was half the seed length. The mesocotyl length, root length, fresh weight, and dry weight were measured. The mesocotyl and root tissues were quickly placed in liquid nitrogen and stored at −80°C for the determination of physiological parameters.
Germination Rate and Growth Parameters
On the 7th day after germination, 10 plants were randomly selected from each replicate to determine the mesocotyl length, root length, fresh weight, and dry weight. The calculation formula for germination rate is as follows:
Moisture Status and Osmotic Adjustment Substances
Fresh mesocotyl and root tissues were weighed (FM), quickly immersed in distilled water for 24 h, the surface was wiped, the swollen mass (TM) weighed, and finally dried in an oven at 80°C to constant weight (DM). The formula for determining relative water content (RWC) is as follows (Hayat et al., 2007):
Proline content was determined using ninhydrin method (Bates et al., 1973). Soluble protein content was determined using coomassie brilliant blue (CCB) based on the method of Guzel and Terzi (2013). Soluble sugar content determination was conducted according to the method of Quan et al. (2004). Free amino acid content was measured according to the method described by Zhang et al. (2015).
Determination of Na+, K+, Ca2+ Content
Concentrated sulfuric acid of 10 mL was added to 0.1 g of the dried sample and digested in a digestion furnace at 320°C for 30 min. During digestion, 30% hydrogen peroxide solution was added twice until the digestion solution was pellucid. The digestion solution was made up to 100 mL with distilled water and the flame photometer (AP1200, AOPU analytical instrument, Shanghai, China) was used for ion content determination. The ion content was calculated using the standard curves of Na+, K+, and Ca2+ (Shi et al., 2020).
Reactive Oxygen Species and Membrane Damage
The determination of hydrogen peroxide content was carried out based on the method of Velikova et al. (2000). Preparation of diaminobenzidine (DAB) staining solution: distilled water was added to an appropriate amount of DAB to make the concentration 1 mg.mL–1. Adjusting pH to 3.8 with concentrated hydrochloric acid dropwise to dissolve DAB, then the pH was adjusted to 5.8 with sodium hydroxide before use. Mesocotyl and root were incubated in DAB solution in the dark at 25°C for more than 8 h.
The determination of O2– was determined by referring to Tian et al. (2003). Preparation of nitrotetrazolium blue chloride (NBT) staining solution: an appropriate amount of NBT was dissolved in 10 mM potassium phosphate buffer (pH 7.6) to 0.5 mg.mL–1 and reserved at 4°C in the dark. The plant material was placed in the NBT solution for 3 h in the dark at room temperature. The electrolyte leakage rate was conducted according to Hniličková et al. (2019). The electrolyte leakage (EL) is determined according to the following formula:
Where the EC1 refers to the initial conductivity and EC2 represents the final conductivity.
Malondialdehyde (MDA) content determination is based on the method of Zhang et al. (2007).
Antioxidant Enzyme Activity and Electrophoresis
The extraction of antioxidant enzymes was conducted using the method of Gogorcena et al. (1995). This antioxidant extract can be used to determine its activity. The SOD activity was determined using the method of Beyer and Fridovich (1987). The POD activity was measured according to Fielding and Hall (1978). The determination of CAT activity refers to the method of Aebi (1984). The determination of APX activity refers to the method of Amako et al. (1994).
Antioxidant enzyme isoenzymes were separated on a 1 mm thick polyacrylamide gel (3% concentrated gel, 7% separating gel). Detailed electrophoresis methods refer to the description of Jin et al. (2009). The SOD isoenzyme was stained using the o-dianisidine staining method. The staining solution comprised 2 mM o-dianisidine, 0.1 mM riboflavin, 20% ethanol, and 10 mM pH 7.2 phosphate buffer, and the gel was immersed in the staining solution for 30 min, taken out, and rinsed with distilled water. The gel was then immersed in phosphate buffer solution and exposed to a fluorescent lamp for 30 min, and pictures were taken after the bands became clear. Benzidine staining method was adopted for POD isoenzyme staining. The gel was stained with 0.1% benzidine in acetate buffer (100 mM, pH 4.5) containing 2 mM H2O2, and then immersed in 7% (v/v) acetic acid to terminate the reaction. Starch method was adopted for CAT isoenzyme staining; 1% soluble starch was boiled until colorless and transparent. The gel was soaked in starch solution for 1 h and 40 min. Then the starch solution was discarded, 0.5% H2O2 was added to the gel for 1 min, and rinsed with distilled water. Finally, 0.5% potassium iodide solution was added. The background turned blue at 25°C and the areas with enzyme activity were colorless and transparent. APX staining was performed as follows: the gel was soaked in 50 mM PBS (pH 7.0) containing 2 mM ascorbic acid for 30 min, incubated for 20 min with 50 mM PBS containing 4 mM ascorbic acid and 2 mM H2O2, and then the gel was washed with 100 mM PBS (pH 7.5). The staining solution contained 28 mM TEMED and 2.45 mM NBT PBS (pH 7.8). The gel in staining solution was shaken gently and rinsed with distilled water. The color development was observed and photographs were taken.
RNA Extraction
Fresh samples were thoroughly ground with TRNzol Universal reagent in liquid nitrogen. The grinding process was carried out rapidly, preferably not more than 1 min, using 0.1 g of sample used 1 mL TRNzol Universal reagent. The homogenized sample was stored at 25°C for 10 min to make the nucleic acid-protein complex separated. The sample was centrifuged at 4°C and 12,000 rpm (approximately 13,400 × g) for 10 min. Chloroform (0.2 mL) was added to each 1 mL of TRNzol Universal reagent, and was vigorously shaken for 15 s, and stored at 25°C for 3 min. Then the sample was centrifuged at 12,000 rpm (∼13,400 × g) at 4°C for 15 min and separated into three layers: pink organic phase, middle layer, and upper colorless water phase. The water phase (about 500 μL) containing RNA was poured into a new centrifuge tube and an equal volume of isopropanol was added. After mixing, it was allowed to stand at 25°C for 10 min, then centrifuged at 4°C, 12,000 rpm (∼13,400 × g) for 10 min, and the supernatant was discarded. The RNA precipitate is usually invisible before centrifugation. The RNA forms a gel-like precipitate on the side and bottom of the tube after centrifugation. Then, 1 mL of 75% ethanol was used to wash the precipitate. For every 1 mL TRNzol Universal reagent, at least 1 mL of 75% ethanol should be used to disperse the precipitate, then centrifuged at 4°C and 10,000 rpm (∼9,391 × g) for 5 min. We poured out the liquid, but were careful not to pour out the precipitate. The remaining liquid was centrifuged briefly and was carefully aspirated without disturbing the precipitate. The sample was then dried at 25°C for 2–3 min (we were careful to avoid over-drying as it is difficult to dissolve the RNA after complete drying), according to the needs of the experiment, 30–100 μL RNase-free ddH2O was added to the sample and mixed by repeated pipetting to fully dissolved the RNA. The gene ID and primer information used for qRT-PCR are shown in Table 1.
Statistical Analysis
One-way analysis of variance (ANOVA) was carried out using SPSS 18.0 software (SPSS Inc., Chicago, IL, United States). Duncan’s multiple range test was used to assess significant differences (p < 0.05) among treatments using SPSS 18.0. Graph Pad Prism 8 (Graph Pad Software Inc., San Diego, CA, United States) is used for graphing, and the data in graphs are all expressed as mean ± standard deviation.
Rusults
Germination Parameters
The germination phenotypes of different treatments are shown in Figure 1. Salt stress inhibited the seed germination, which manifested as reduction in shoot and root length. Hydro-priming and CaCl2 priming promoted the germination of sorghum seeds under salt stress. The shoot and root length were significantly increased and the effect of CaCl2 priming was significantly better than that of hydro-priming.
Salt stress significantly reduced the germination rate, mesocotyl length, mesocotyl fresh weight, mesocotyl dry weight, root length, root fresh weight, and root dry weight by 21.53, 67.07, 66.49, 46.25, 74.38, 74.14, and 35.40%, respectively (Table 2). There was no significant difference between HPS treatment and NPS treatment in terms of germination rate and root dry weight. Mesocotyl length, mesocotyl fresh weight, mesocotyl dry weight, root length, and root fresh weight in HPS were significantly increased by 96.35, 95.26, 34.56, 164.94, and 154.97%, respectively, compared with NPS treatment. The germination rate, mesocotyl length, mesocotyl fresh weight, mesocotyl dry weight, root length, root fresh weight, and root dry weight of CaPS treatment were significantly increased by 27.45, 169.79, 206.05, 82.35, 219.48, 258.94, and 16.44%, respectively, compared with NPS treatment. It is observed that CaCl2 priming could effectively improve seed germination under salt stress.
Relative Water Content
Under salt stress, the relative water content of mesocotyl and root was significantly reduced by 39.70 and 39.76%, respectively. Compared with NPS treatment, the relative water content of mesocotyl and root was significantly increased by 37.32 and 35.00% in HPS treatment. Compared with NPS treatment, the relative water content of mesocotyl and root in CaPS treatment were significantly increased by 59.31 and 61.81%, respectively (Figure 2). These results indicated that CaCl2 priming significantly improved water status under salt stress.
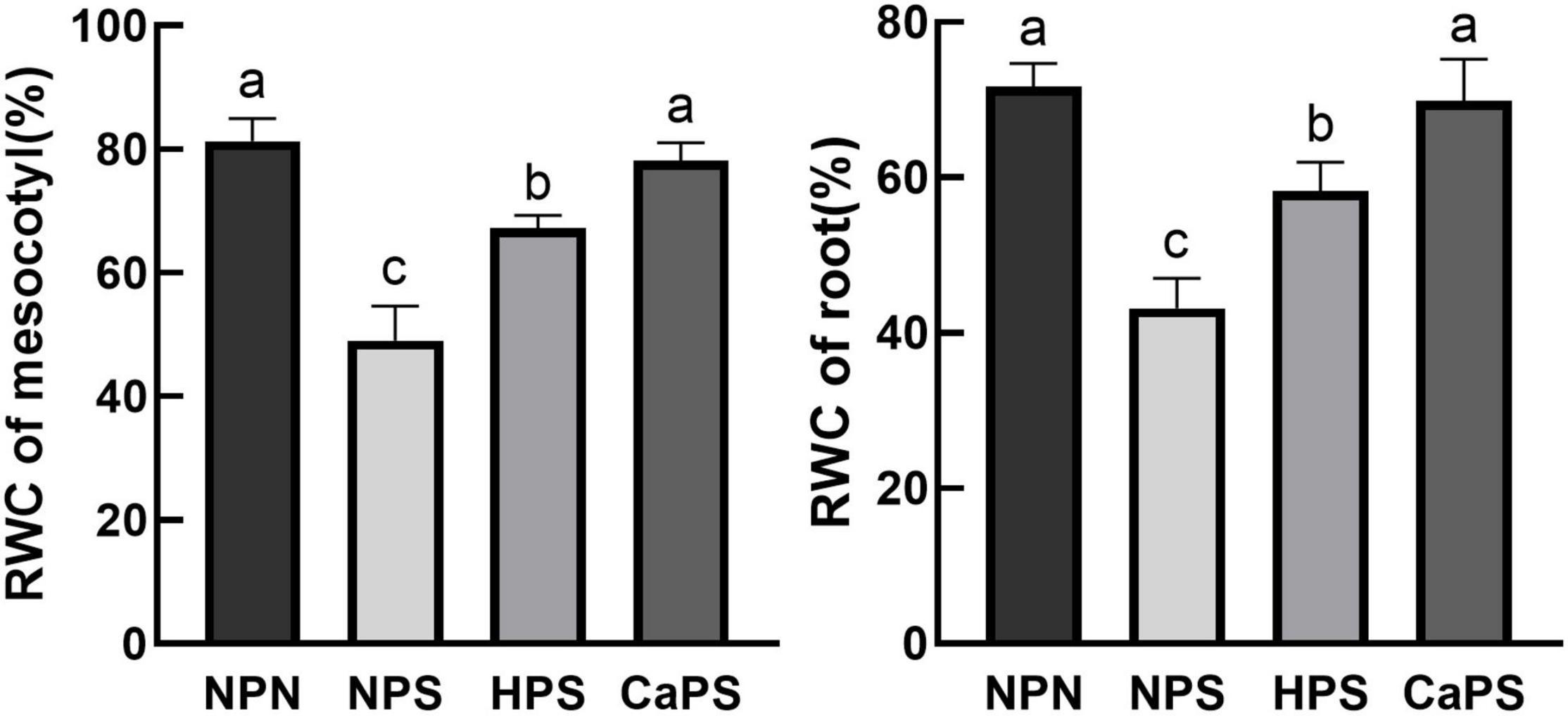
Figure 2. Effect of CaCl2 priming on relative water content of sorghum tissue under salt stress. RWC, relative water content.
Accumulation of Osmotic Adjustment Substance
Under salt stress, the content of soluble sugar, proline, free amino acid, and soluble protein in mesocotyl were significantly increased by 72.21, 60.50, 21.13, and 15.05%, respectively (Figure 3). The content of soluble sugar, proline, free amino acid, and soluble protein in root, respectively, increased 42.26, 68.79, 22.53, and 39.30%. Compared with NPS treatment, the soluble sugar, proline, free amino acid, and soluble protein content in mesocotyl were significantly increased by 22.21, 44.31, 14.64, and 9.25% in the HPS treatment, while they significantly increased in the root by 13.57, 10.71, 32.60, and 45.56%, respectively. Compared with NPS treatment, the content of soluble sugar, proline, free amino acid, and soluble protein increased significantly in CaPS treatment by 45.43, 110.69, 30.04, and 14.52%, and increased by 23.04, 28.61, 47.99, and 61.47% in root, respectively. Changes in these osmotic adjustment substances illustrated that CaCl2 priming induced improvement of osmotic adjustment ability.
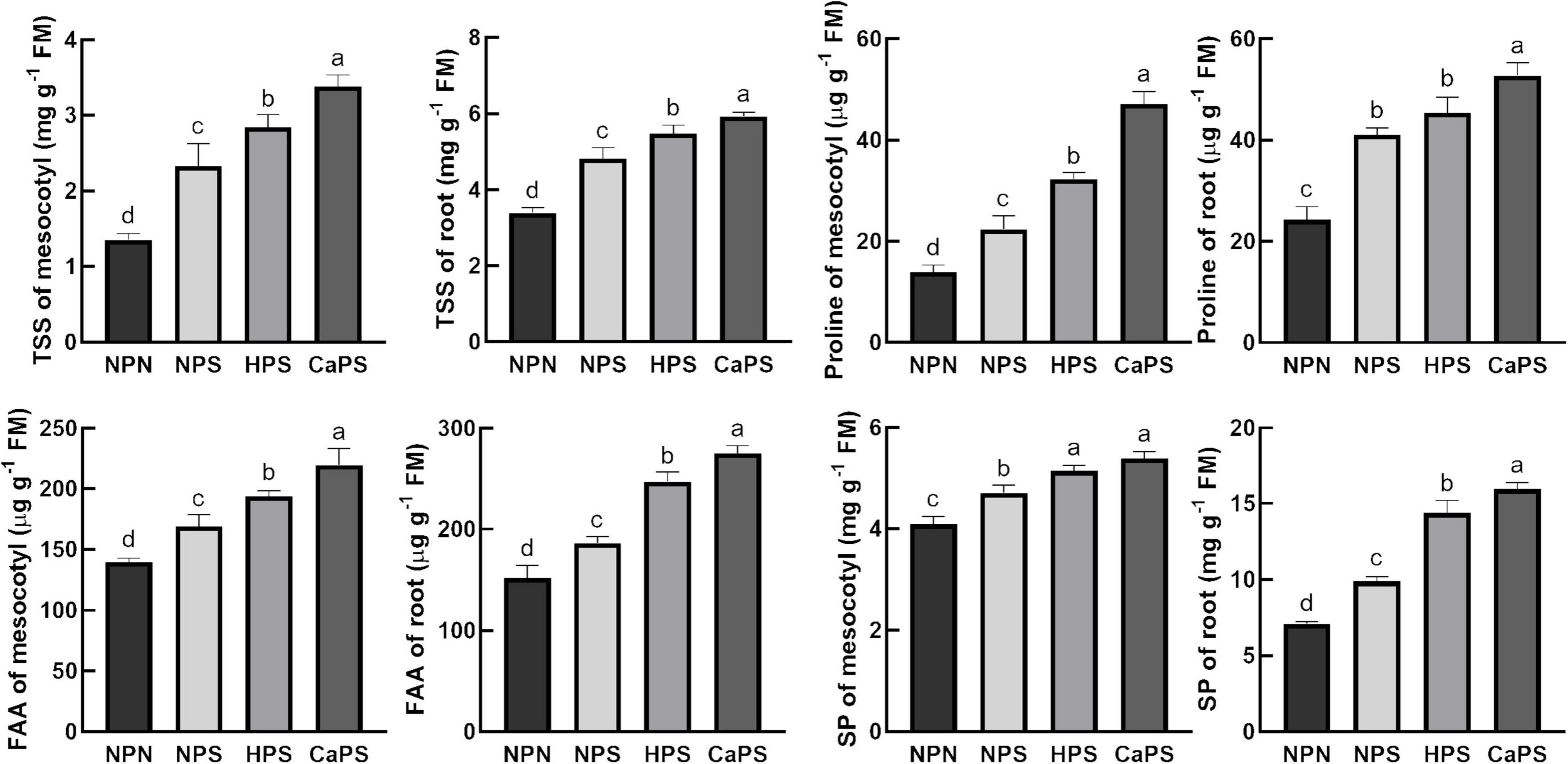
Figure 3. Effect of CaCl2 priming on osmotic adjustment substances in sorghum tissue under salt stress. TSS, total soluble sugar; FAA, free amino acid; SP, soluble protein.
Na+, K+, and Ca2+ Content
Under salt stress, Na+ content in mesocotyls and roots were significantly increased by 53.15 and 125.53%, respectively, while K+ content was significantly increased by 23.86 and 80.46%, respectively. Ca2+ content had no significant change under salt stress. K+/Na+ in mesocotyl and root were significantly reduced by 17.78 and 18.75%, while Ca2+/Na+ were significantly reduced by 42.99 and 58.14%, respectively. Compared with NPS, the Na+ content of mesocotyl and roots in HPS treatment was significantly reduced by 18.32 and 26.92%, respectively, and there was no significant difference in K+ content. Ca2+ content was significantly increased by 49.65 and 29.53%, and K+/Na+ was significantly increased by 34.46 and 45.56%, respectively. Ca2+/Na+ was increased significantly by 84.79 and 77.74%, respectively. Compared with NPS treatment, the Na+ content of mesocotyl and root was significantly reduced by 32.34 and 28.15% in CaPS treatment, respectively. K+ content was significantly increased by 34.54 and 37.81%, Ca2+ content was significantly increased by 170.04 and 113.50%, and K+/Na+ was significantly increased by 98.21 and 90.96%, Ca2+/Na+ was increased significantly by 302.27 and 197.74%, respectively (Table 3). These results showed that CaCl2 priming significantly improved ion balance under salt stress.
Accumulation of Reactive Oxygen
Salt stress notably increased the content of O2– and H2O2 in mesocotyl and root, which was increased by 65.15 and 105.40% in mesocotyl, and 72.69 and 79.11% in root, respectively (Figure 4). Compared with NPS, both hydro-priming and CaCl2 priming treatments significantly reduced the level of reactive oxygen species. The content of O2– and H2O2 in the mesocotyl were reduced by 22.86 and 23.61% in HPS treatment, and by 27.48 and 23.09% in root, respectively. The content of O2– and H2O2 in mesocotyl were reduced by 26.47 and 25.56%, respectively, in CaPS treatment, and by 37.89 and 34.52% in root, respectively. The results of reactive oxygen staining were also basically consistent with the quantitative results (Figure 5). It can be seen from these results that the ROS level was significantly reduced by CaCl2 priming.
Membrane Damage
Salt stress significantly increased MDA content and electrolyte leakage in the mesocotyl and root tissues (Figure 6). The MDA content increased significantly by 91.24 and 105.27%, and the electrolyte leakage increased by 112.83 and 39.85%, respectively. Compared with NPS, the MDA content of mesocotyl and root was significantly reduced by 25.26 and 26.20% in HPS treatment, while the electrolyte leakage of the mesocotyl was significantly reduced by 26.31% in HPS treatment. The MDA content of mesocotyl and root was significantly reduced by 42.67 and 39.05% in CaPS treatment, while electrolyte leakage was significantly reduced by 44.52 and 16.58% in CaPS treatment, respectively, indicating that CaCl2 priming could effectively protect plasma membranes under salt stress.
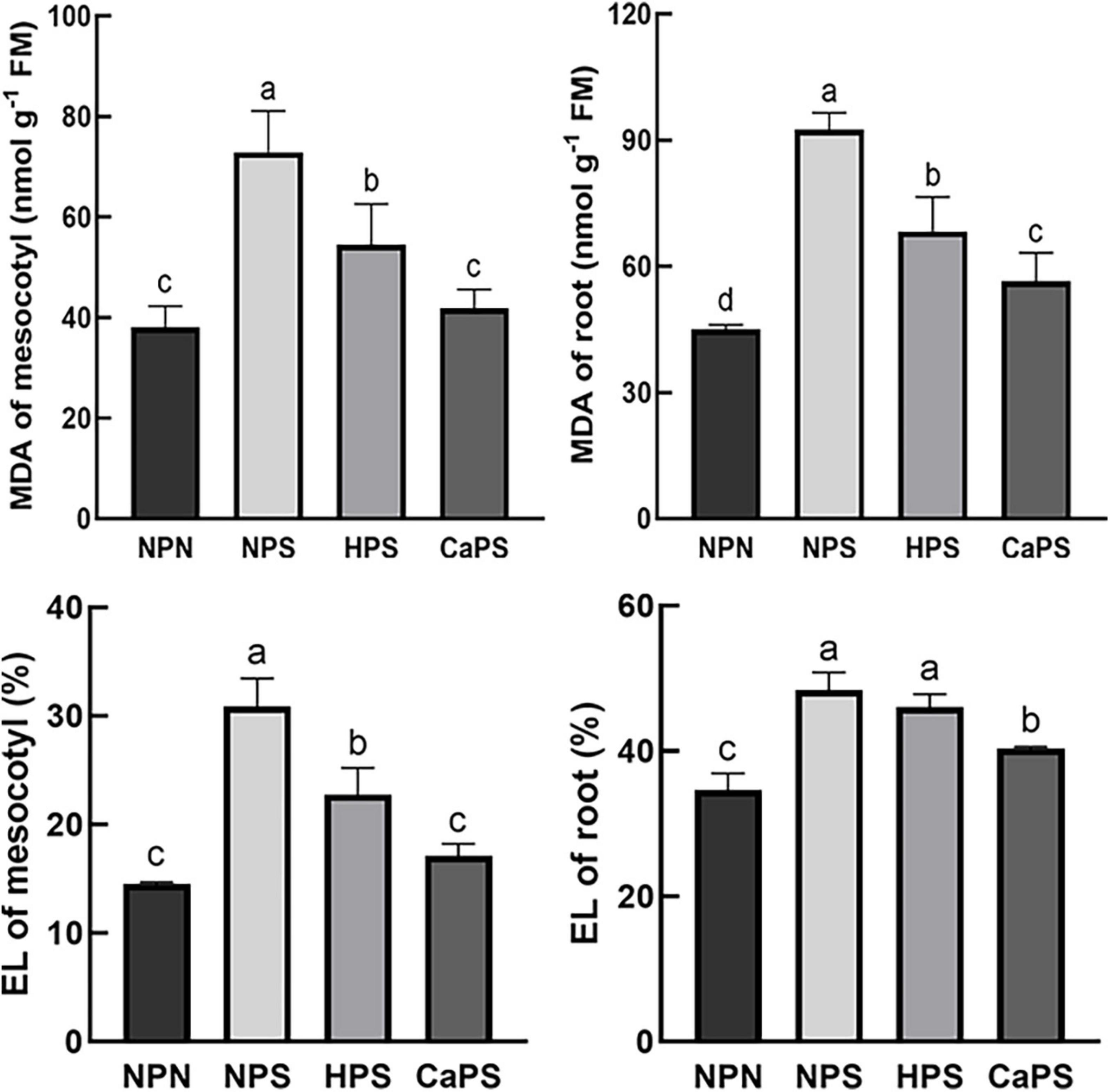
Figure 6. Effect of CaCl2 priming on MDA content and electrolyte leakage in sorghum tissue under salt stress. MDA, malondialdehyde; EL, electrolyte leakage.
Antioxidant Enzyme System
Salt stress significantly increased the activities of antioxidant enzymes in sorghum tissues (Figure 7). The activities of SOD, POD, CAT, and APX in mesocotyl were significantly increased by 97.84, 5.57, 76.10, and 29.40%, and increased by 81.56, 14.59, 83.37, and 44.86% in root. Compared with NPS treatment, the activities of SOD, CAT, and APX in mesocotyl were significantly reduced by 25.51, 23.12, and 14.97% in HPS treatment, and the activities of SOD, POD, CAT, and APX in roots were significantly decreased by 19.26, 7.85, 39.82, and 14.78%, respectively. Compared with NPS treatment, the activities of SOD, CAT, and APX in mesocotyl were significantly reduced by 48.59, 20.27, and 11.26% in the CaPS treatment, and the activities of SOD, POD, CAT, and APX in the roots were significantly decreased by 22.41, 10.56, 35.99, and 18.42%, respectively. The results of antioxidant enzyme staining are consistent with the quantitative results (Figure 8). These results suggested that seed priming did not further enhance antioxidant enzyme activity under salt stress.
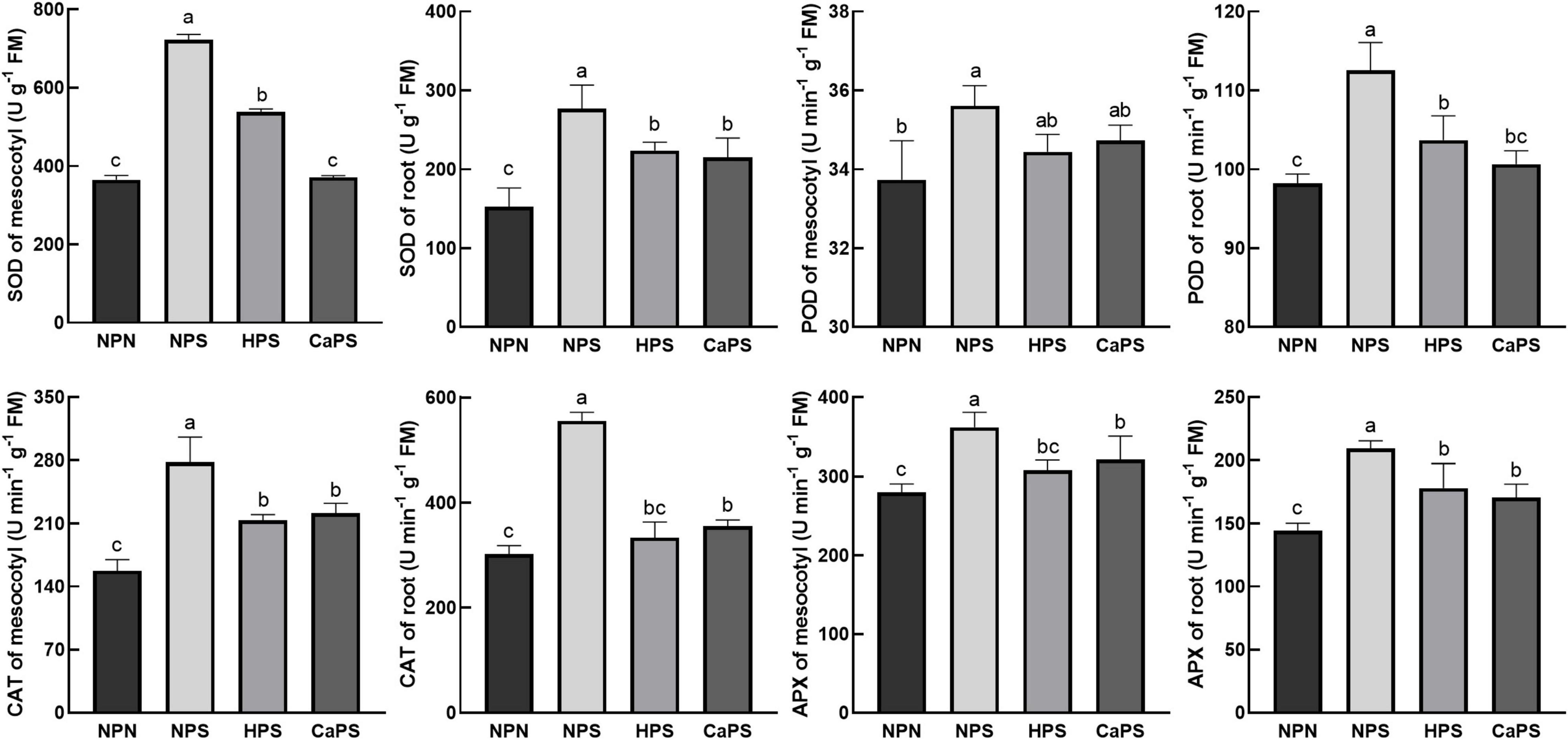
Figure 7. Effect of CaCl2 priming on antioxidant enzyme activities in sorghum tissue under salt stress.
Gene Expression Level
Under salt stress, the expression of superoxide dismutase genes SOD2, peroxidase genes POD1, POD2, catalase genes CAT1, CAT2, and CAT3 were significantly up-regulated, which were up-regulated by 118.47, 68.07, 126.45, 129.79, 172.19, and 39.40%, respectively. CaCl2 priming treatment reduced the expression of these genes (Figure 9). Compared with NPS, the expression levels were reduced by 25.74, 20.86, 63.53, 42.24, 50.38, and 19.66%, respectively. The expression level of SOD4A was significantly reduced by 49.45%. These results suggested that changes in antioxidant enzyme activities were correlated with gene expression levels. Under salt stress, the expressions level of Na+/H+ antiporter genes, NHX2, NHX4, SOS1, and K+ transporter genes, AKT1, AKT2, HKT1, HAK1, and KUP were up-regulated by 81.78, 624.86, 2134.87, 394.22, 86.41, 584.78, 242.53, and 158.84%, respectively. Compared with NPS, CaCl2 priming treatment further increased the expression of these genes, which were increased by 30.23, 142.05, 163.52, 166.51, 29.95, 133.36, 58.23, and 163.52%, respectively, indicating that CaCl2 priming enhanced the genes expression of ion transporter to resist ion stress.
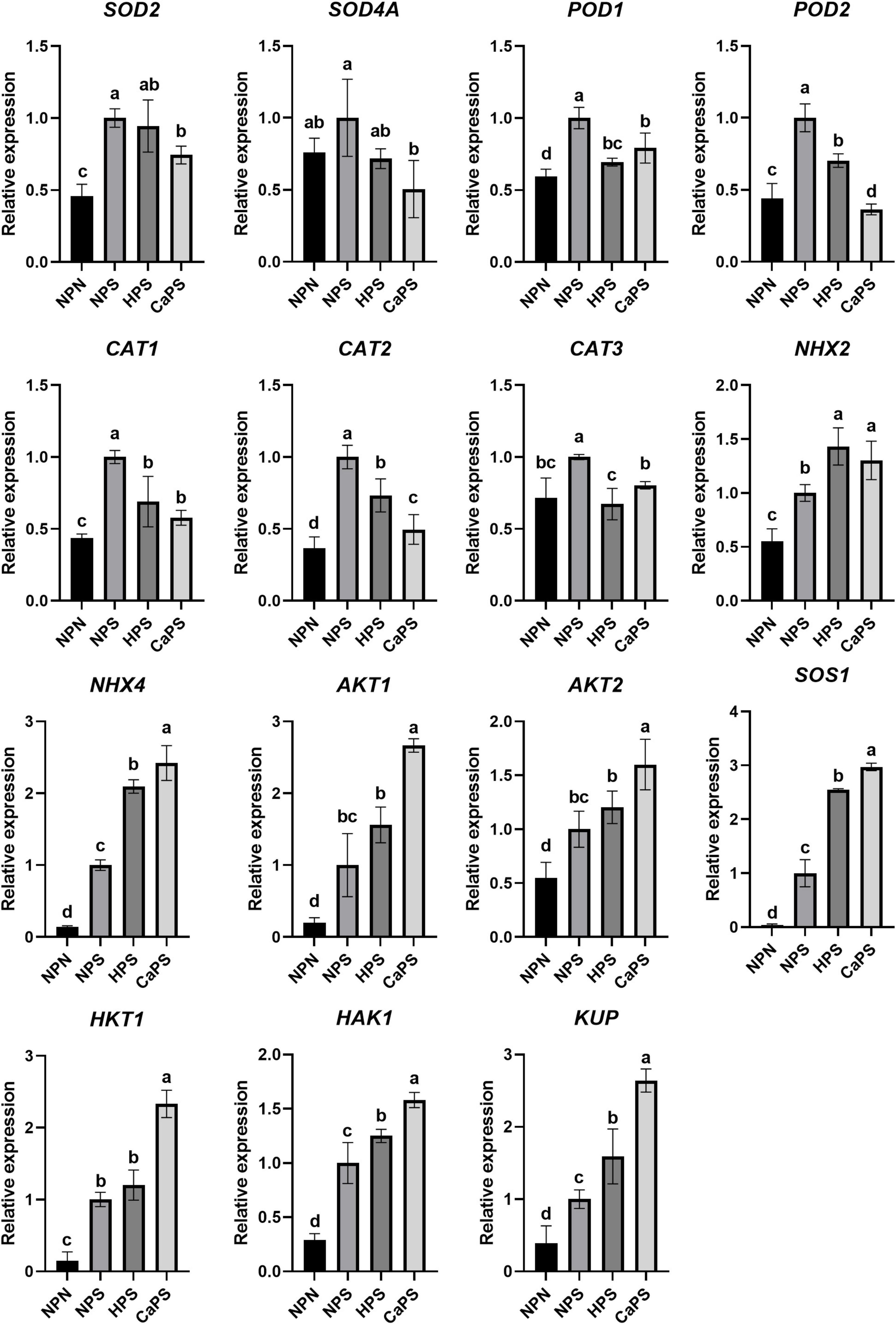
Figure 9. Effects of CaCl2 priming on the expression of antioxidant enzymes and ion transport genes in sorghum under salt stress.
Discussion
Physiological and molecular changes of plant tissues and cells under abiotic stress affect plant growth and development (He et al., 2017; Gao et al., 2020; Basit et al., 2022). Salt stress negatively affects many growth processes of plants, such as seed germination rate, germination induction, and seedling establishment (Ning et al., 2019; Sheteiwy et al., 2020). Previous studies have shown that calcium plays a key role in plant response to abiotic stress (Niu et al., 2017), and in plant growth and development, including seed germination (Kong et al., 2015). This study found that salt stress significantly reduced the germination rate and growth. Hydro-priming and CaCl2 priming promoted germination under salt stress to varying degrees, and the effect of CaCl2 priming treatment was significantly better than that of hydro-priming, corroborating the fact that Ca2+ may play a key role in this process.
Proline is an important osmotic regulator, which maintains osmotic balance in vivo by increasing cell osmotic potential under osmotic stress (Reddy et al., 2015). This study showed that sorghum mesocotyl and root accumulated more proline under salt stress, which was a self-regulating mechanism of plants in response to salt stress. These results are consistent with the results reported by Mulaudzi et al. (2020). Proline not only plays an important role in regulating osmotic balance, but also acts as a molecular chaperone to prevent membrane damage (Delauney and Verma, 1993). In addition to proline, other osmotic adjustment substances including free amino acids, soluble sugars, and soluble protein content changes showed results similar to that of proline. Heinemann et al. (2020) showed that the accumulation of free amino acids may be the result of proteolysis during osmotic adjustment. Therefore, the accumulation of free amino acids may be an adaptive mechanism for plants in response to osmotic stress. Seed priming can effectively enhance this physiological adaptation, which is similar to the results of Islam et al. (2015) who reported that hydro-priming and CaCl2 priming increased the proline content, improved salt tolerance, and that the effect of CaCl2 treatment was better than hydro-priming. The accumulation of osmotic adjustment substances can maintain a suitable water potential, thus offsetting the adverse effects of osmotic stress caused by increased salt concentration (Kumar et al., 2003). Although the osmotic adjustment substances increased under salt stress, the relative water content in sorghum tissues was low, indicating that the increased osmotic adjustment substances were not enough to alleviate the osmotic stress caused by increased salt concentration. Seed priming further increased the content of osmotic adjustment substances, so that the relative water content in the tissues increased, and the water state was improved.
Ion regulation is another key parameter that reflects the salt tolerance mechanism of plants. Therefore, the evaluation of ion accumulation, especially the accumulation of Na+, K+, and Ca2+ in different plant organs will aid in elucidating the salt tolerance mechanism (Noreen et al., 2010). The decrease in Na+ content and the increase in K+ content were important reasons for the improvement of plant salt tolerance caused by seed priming (Bajwa et al., 2018). In this study, salt stress increased the K+ content in sorghum tissues, because when exposed to salt stress, plants tried to maintain a higher K+/Na+ in the cytoplasm to maintain ion balance (Shabala and Pottosin, 2014). However, due to the increased accumulation of Na+ in tissues under salt stress and the limited ability of plants to absorb K+, plants were unable to increase K+/Na+ through their own ability to regulate ion balance under salt stress. Although hydro-priming has no significant effect on K+ content, it reduced the Na+ content in the tissues. Therefore, K+/Na+ was increased, while CaCl2 priming influenced both the increase of K+ content and the decrease of Na+ content. CaCl2 priming has a better effect in increasing K+/Na+ to maintain ion balance under salt stress. Manishankar et al. (2018) reported the important role of calcium signaling in plant K+ transport and balance. Therefore, the increased K+/Na+ concentration under salt stress by CaCl2 treatment may be related to the enhanced calcium signaling. Ca2+ signaling plays an important role in activating the core SOS pathway by promoting Na+ efflux and storage in vacuoles under salt stress (Manishankar et al., 2018). In addition, excess Na+ is known to affect the cell wall integrity. The latest evidence showed that the Ca2+ signaling plays an vital role in maintaining the integrity of the cell wall and alleviating the inhibition of salt stress on cell growth (Feng et al., 2018). Therefore, Ca2+ signal plays a key role in the process of CaCl2 priming by improving the growth of sorghum mesocotyl and root under salt stress and probably contributed to better performance of CaCl2 priming compared with hydro-priming. In this study, CaCl2 priming increased Ca2+/Na+ by more than three times in mesocotyl, and by nearly two times in roots. CaCl2 priming showed a stronger effect in regulating Ca2+/Na+ than in K+/Na+ in response to salt stress. Hydro-priming had similar results, but it was inferior to CaCl2 priming in regulating ion balance. Plants has several classes of Na+ transporters called salt overly sensitive 1 (SOS1), Na+/H+ exchangers (NHX), and high-affinity K+ transporters (HKT). Similarly, there are several K+ transporters reported, including class I HKT, K+ uptake (KUP), and high-affinity K+ transporter (HAK) transporters (Saddhe et al., 2021). Plants use Na+ antiporters to transport Na+ to apoplasts or vacuoles to maintain low levels of Na+ in the cytoplasm. K+ transporters are responsible for the absorption and transport of K+ (Zhao et al., 2021). In this study, the up-regulation of Na+ antiporter genes NHX2, NHX4, SOS1, and K+ transporter genes AKT1, AKT2, HKT1, HAK1, and KUP expression in the CaCl2 priming treatment may be responsible for the decrease of Na+ content and the increase of K+ content in tissues.
Plants adapt to salt stress not only via osmotic regulation and ion balance adjustment, but also through antioxidant enzyme defense system to remove ROS produced by salt stress (Genisel et al., 2015). There are many reports that indicate that seed priming improved the salt tolerance of plants by enhancing the antioxidant enzyme system (Alves et al., 2020; Jiang et al., 2020). However, studies have shown that seed priming resulted in a partial decrease in the activity of antioxidant enzymes, but the level of reactive oxygen remained low. This is because the priming agent itself had the function of scavenging ROS, leading to a reduction in the anti-oxidation system activation (Genisel et al., 2015). The regulation of ROS metabolic homeostasis is critical for plant responses to abiotic stresses (Sheteiwy et al., 2021; Yang et al., 2021). In this study, seed priming treatment did not increase the activity of the antioxidant enzyme system, however, it decreased their activity. It should be noted that the priming agent used in this study had no effect on reactive oxygen scavenging. Therefore, the reduction in reactive oxygen level is mediated by the priming agent and not the antioxidant enzymes. The down-regulation of antioxidant enzyme genes could be attributed to the decrease in antioxidant enzyme activity. In addition, the balance between production and removal of reactive oxygen was less affected by salt stress due to seed priming, so the reactive oxygen level was lower in CaPS than in the control plants, and the stronger antioxidant enzyme system was not activated. Yang and Guo (2018) suggested that salt stress induced ion stress, osmotic stress, and secondary stress, especially oxidative stress. Oxidative stress was mainly caused by the excessive accumulation of ROS. Therefore, the accumulation of ROS may be related to the degree to which plants are subjected to ion stress and osmotic stress. However, seed priming is a seed pretreatment process carried out before the occurrence of stress. The reduction of reactive oxygen content caused by seed priming may be related to its enhanced osmotic adjustment ability and ion balance. The results of this study also confirmed to this conjecture. Moreover, the change of MDA content also synchronously confirmed that the degree of lipid peroxidation under seed priming treatment was low, but further studies should be conducted to explore the mechanisms of reactive oxygen species production under salt stress and their relationship to osmotic and ion balance regulation in plants.
Conclusion
In conclusion, this study revealed that CaCl2 priming increased the content of osmotic adjustment substances in mesocotyl and root of sorghum under salt stress compared with hydro-priming. CaCl2 priming regulated ion balance under salt stress by increasing K+/Na+, especially Ca2+/Na+. CaCl2 priming enhanced the salt tolerance during seed germination and alleviated the inhibition of salt stress on sorghum seed germination and growth (Figure 10). Ca2+ played an important role in improving the salt tolerance of sorghum induced by CaCl2.
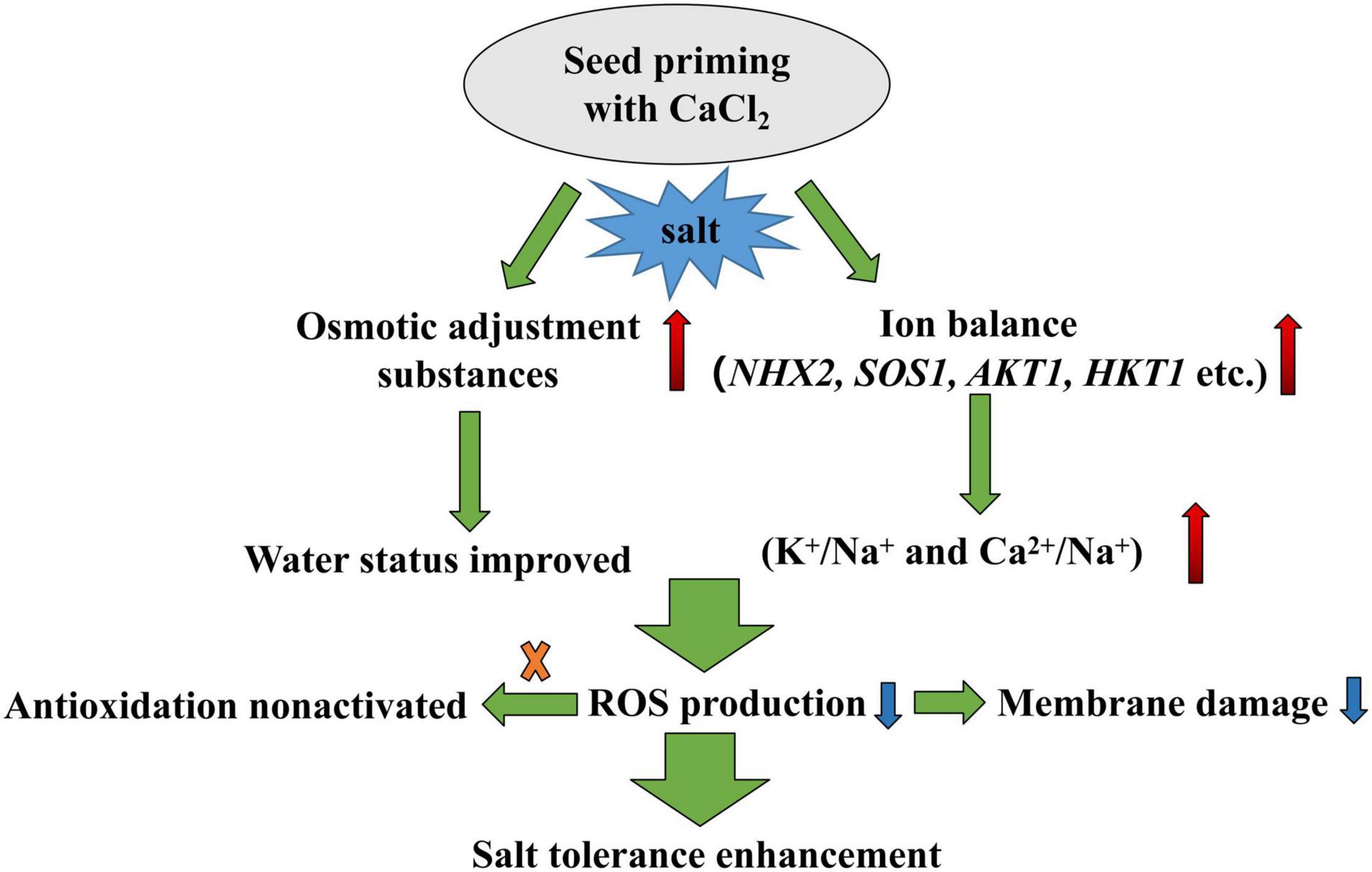
Figure 10. A flowchart of CaCl2 priming enhancing salt tolerance during sorghum germination. The red arrows indicate increase and blue arrows indicate decrease.
Data Availability Statement
The datasets presented in this study can be found in online repositories. The names of the repository/repositories and accession number(s) can be found in the article/Supplementary Material.
Author Contributions
XC performed the experiment, analyzed the data, and drafted the manuscript. YZ conducted the experiment and modified the manuscript. RZ and BL helped in performing the experiment. TC helped in modifying the manuscript. BC provided the resources. All authors contributed to the article and approved the submitted version.
Funding
This work was financially supported by the China Agriculture Research System of MOF and MARA (CARS-06-14.5-A17), and General Research Project of Education Department of Liaoning Province of China (LSNFW202006).
Conflict of Interest
The authors declare that the research was conducted in the absence of any commercial or financial relationships that could be construed as a potential conflict of interest.
Publisher’s Note
All claims expressed in this article are solely those of the authors and do not necessarily represent those of their affiliated organizations, or those of the publisher, the editors and the reviewers. Any product that may be evaluated in this article, or claim that may be made by its manufacturer, is not guaranteed or endorsed by the publisher.
Supplementary Material
The Supplementary Material for this article can be found online at: https://www.frontiersin.org/articles/10.3389/fpls.2022.881039/full#supplementary-material
References
Abdoulaye, A. O., Lu, H., Zhu, Y., Hamoud, Y. A., and Sheteiwy, M. (2019). The global trend of the net irrigation water requirement of maize from 1960 to 2050. J. Clim. 11:124. doi: 10.3390/cli7100124
Aebi, H. (1984). Catalase in vitro. Methods Enzymol. 105, 121–126. doi: 10.1016/S0076-6879(84)05016-3
Alves, R. D. C., Nicolau, M. C. M., Checchio, M. V., Junior, G. D. S. S., Oliveira, F. D. A. D., Prado, R. M., et al. (2020). Salt stress alleviation by seed priming with silicon in lettuce seedlings: an approach based on enhancing antioxidant responses. Bragantia 79, 1–11. doi: 10.1590/1678-4499.20190360
Amako, K., Chen, G. X., and Asada, K. (1994). Separate assays specific for ascorbate peroxidase and guaiacol peroxidase and for the chloroplastic and cytosolic isozymes of ascorbate peroxidase in plants. Plant Cell Physiol. 35, 497–504. doi: 10.4028/www.scientific.net/MSF.492-493.229
Bajwa, A. A., Farooq, M., and Nawaz, A. (2018). Seed priming with sorghum extracts and benzyl aminopurine improves the tolerance against salt stress in wheat (Triticum aestivum L.). Physiol. Mol. Biol. Plants 24, 239–249. doi: 10.1007/s12298-018-0512-9
Basit, F., Ulhassan, Z., Mou, Q., Nazir, M. M., Hu, J., Hu, W., et al. (2022). Seed priming with nitric oxide and/or spermine mitigate the chromium toxicity in rice (Oryza sativa) seedlings by improving the carbon-assimilation and minimising the oxidative damages. Funct. Plant Biol. doi: 10.1071/FP21268
Bates, L. S., Waldren, R. P., and Teare, I. D. (1973). Rapid determination of free proline for water-stress studies. Plant Soil 39, 205–207. doi: 10.1007/BF00018060
Beyer, W. F., and Fridovich, I. (1987). Assaying for superoxide dismutase activity: some large consequences of minor changes in conditions. Anal. Biochem. 161, 559–566. doi: 10.1016/0003-2697(87)90489-1
Chen, X., Zhang, R., Xing, Y., Jiang, B., Li, B., Xu, X., et al. (2021). The efficacy of different seed priming agents for promoting sorghum germination under salt stress. PLoS One 16:e0245505. doi: 10.1371/journal.pone.0245505
Daszkowska-Golec, A. (2011). Arabidopsis seed germination under abiotic stress as a concert of action of phytohormones. Omics 15, 763–774. doi: 10.1089/omi.2011.0082
Delauney, A. J., and Verma, D. P. S. (1993). Proline biosynthesis and osmoregulation in plants. Plant J. 4, 215–223. doi: 10.1046/j.1365-313X.1993.04020215.x
El-Beltagi, H. S., Mohamed, H. I., and Sofy, M. R. (2020). Role of ascorbic acid, glutathione and proline applied as singly or in sequence combination in improving chickpea plant through physiological change and antioxidant defense under different levels of irrigation intervals. Molecules 25:1702. doi: 10.3390/molecules25071702
Feng, W., Kita, D., Peaucelle, A., Cartwright, H. N., Doan, V., Duan, Q., et al. (2018). The FERONIA receptor kinase maintains cell-wall integrity during salt stress through Ca2+ signaling. Curr. Biol. 28, 666–675. doi: 10.1016/j.cub.2018.01.023
Fielding, J. L., and Hall, J. L. (1978). A biolchemical and cytochemical study of peroxidase activity in roots of Pisum sativum: I. a comparison of dab-peroxidase and guaiacol-peroxidase with particular emphasis on the properties of cell wall activity. J. Exp. Bot. 29, 969–981. doi: 10.1093/jxb/29.4.969
Fu, H. H., and Luan, S. (1998). AtKuP1: a dual-affinity K+ transporter from Arabidopsis. Plant Cell 10, 63–73. doi: 10.2307/3870629
Gao, C., Sheteiwy, M., Han, J., Dong, Z., Pan, R., Guan, Y., et al. (2020). Polyamine biosynthetic pathways and their relation with the cold tolerance of maize (Zea mays L.) seedlings. Plant Signal. Behav. 15:1807722. doi: 10.1080/15592324.2020.1807722
Genisel, M., Erdal, S., and Kizilkaya, M. (2015). The mitigating effect of cysteine on growth inhibition in salt-stressed barley seeds is related to its own reducing capacity rather than its effects on antioxidant system. Plant Growth Regul. 75, 187–197. doi: 10.1007/s10725-014-9943-7
Giri, G. S., and Schillinger, W. F. (2003). Seed priming winter wheat for germination, emergence, and yield. Crop Sci. 43, 2135–2141. doi: 10.2135/cropsci2003.2135
Gobinathan, P., Sankar, B., Murali, P. V., and Panneerselvam, R. (2009). Interactive effects of calcium chloride on salinity-induced oxidative stress in Pennisetum typoidies. Bot. Res. Int. 2, 143–148.
Gogorcena, Y., Iturbe-Ormaetxe, I., Escuredo, P. R., and Becana, M. (1995). Antioxidant defenses against activated oxygen in pea nodules subjected to water stress. Plant Physiol. 108, 753–759. doi: 10.1104/pp.108.2.753
Guzel, S., and Terzi, R. (2013). Exogenous hydrogen peroxide increases dry matter production, mineral content and level of osmotic solutes in young maize leaves and alleviates deleterious effects of copper stress. Bot. Stud. 54:26. doi: 10.1186/1999-3110-54-26
Hajihashemi, S., Skalicky, M., Brestic, M., and Vachová, P. (2020). Cross-talk between nitric oxide, hydrogen peroxide and calcium in salt-stressed Chenopodium quinoa Willd. at seed germination stage. Plant Physiol. Biochem. 154, 657–664. doi: 10.1016/j.plaphy.2020.07.022
Hao, H., Li, Z., Leng, C., Lu, C., Luo, H., Liu, Y., et al. (2021). Sorghum breeding in the genomic era: opportunities and challenges. Theor. Appl. Genet. 134, 1899–1924. doi: 10.1007/s00122-021-03789-z
Hayat, S., Ali, B., Hasan, S. A., Aiman, S., and Ahmad, H. A. (2007). Brassinosteroid enhanced the level of antioxidants under cadmium stress in Brassica juncea. Environ. Exp. Bot. 60, 33–41. doi: 10.1016/j.envexpbot.2006.06.002
He, F., Shen, H., Lin, C., Fu, H., Sheteiwy, M. S., Guan, Y., et al. (2017). Transcriptome analysis of chilling-imbibed embryo revealed membrane recovery related genes in maize. Front. Plant Sci. 7:1978. doi: 10.3389/fpls.2016.01978
Heinemann, B., Künzler, P., Eubel, H., Braun, H. P., and Hildebrandt, T. (2020). Estimating the number of protein molecules in a plant cell: protein and amino acid homeostasis during drought. Plant Physiol. 185(Suppl. 1) 385–404. doi: 10.1093/plphys/kiaa050
Hniličková, H., Hnilička, F., Orsák, M., and Hejnák, V. (2019). Effect of salt stress on growth, electrolyte leakage, Na+ and K+ content in selected plant species. Plant Soil Environ. 65, 90–96. doi: 10.17221/620/2018-PSE
Hussain, S., Khaliq, A., Tanveer, M., Matloob, A., and Hussain, H. A. (2018). Aspirin priming circumvents the salinity-induced effects on wheat emergence and seedling growth by regulating starch metabolism and antioxidant enzyme activities. Acta Physiol. Plant. 40:68. doi: 10.1007/s11738-018-2644-5
Ibrahim, E. A. (2016). Seed priming to alleviate salinity stress in germinating seeds. J. Plant Physiol. 192, 38–46. doi: 10.1016/j.jplph.2015.12.011
Islam, F., Yasmeen, T., Ali, S., Ali, B., Farooq, M. A., and Gill, R. A. (2015). Priming-induced antioxidative responses in two wheat cultivars under saline stress. Acta Physiol. Plant. 37:153. doi: 10.1007/s11738-015-1897-5
Ismail, A., Takeda, S., and Nick, P. (2014). Life and death under salt stress: same players, different timing? J. Exp. Bot. 65, 2963–2979. doi: 10.1093/jxb/eru159
Jiang, X. W., Zhang, C. R., Wang, W. H., Xu, G. H., and Zhang, H. Y. (2020). Seed priming improves seed germination and seedling growth of Isatis indigotica Fort. under salt stress. HortScience 55, 647–650. doi: 10.21273/HORTSCI14854-20
Jin, X., Huang, Y., Zeng, F., Zhou, M., and Zhang, G. (2009). Genotypic difference in response of peroxidase and superoxide dismutase isozymes and activities to salt stress in barley. Acta Physiol. Plant. 31, 1103–1109. doi: 10.1007/s11738-009-0328-x
Jisha, K. C., Vijayakumari, K., and Puthur, J. T. (2013). Seed priming for abiotic stress tolerance: an overview. Acta Physiol. Plant. 35, 1381–1396. doi: 10.1007/s11738-012-1186-5
Kong, D., Ju, C., Parihar, A., Kim, S., Cho, D., and Kwak, J. M. (2015). Arabidopsis glutamate receptor homolog3.5 modulates cytosolic Ca2+ level to counteract effect of abscisic acid in seed germination. Plant Physiol. 167, 1630–1642. doi: 10.1104/pp.114.251298
Kumar, S. G., Reddy, A. M., and Sudhakar, C. (2003). NaCl effects on proline metabolism in two high yielding genotypes of mulberry (Morus alba L.) with contrasting salt tolerance. Plant Sci. 165, 1245–1251. doi: 10.1016/S0168-9452(03)00332-7
Lazof, D. B., and Bernstein, N. (1998). The NaCl induced inhibition of shoot growth: the case for disturbed nutrition with special consideration of calcium. Adv. Bot. Res. 29, 113–189. doi: 10.1016/S0065-2296(08)60311-0
Mahboob, W., Khan, M. A., Shirazi, M. U., and Faisal, S. (2019). Seed priming modulates germination potential, osmoprotectants accumulation and ionic uptake in wheat seedlings under salt stress. Int. J. Agric. Biol. 22, 594–600. doi: 10.17957/IJAB/15.1104
Manishankar, P., Wang, N., Köster, P., Alatar, A. A., and Kudla, J. (2018). Calcium signaling during salt stress and in the regulation of ion homeostasis. J. Exp. Bot. 69, 4215–4226. doi: 10.1093/jxb/ery201
Mulaudzi, T., Hendricks, K., Mabiya, T., Muthevhuli, M., Ajayi, R. F., Mayedwa, N., et al. (2020). Calcium improves germination and growth of Sorghum bicolor seedlings under salt stress. Plants 9:730. doi: 10.3390/plants9060730
Munns, R., and Tester, M. (2008). Mechanisms of salinity tolerance. Annu. Rev. Plant Biol. 59, 651–681. doi: 10.1146/annurev.arplant.59.032607.092911
Ning, L. H., Du, W. K., Song, H. N., Shao, H. B., Qi, W. C., Sheteiwy, M. S. A., et al. (2019). Identification of responsive miRNAs involved in combination stresses of phosphate starvation and salt stress in soybean root. Environ. Exp. Bot. 167:103823. doi: 10.1016/j.envexpbot.2019.103823
Niu, L., Yu, J., Liao, W., Yu, J., Zhang, M., and Dawuda, M. M. (2017). Calcium and calmodulin are involved in nitric oxide-induced adventitious rooting of cucumber under simulated osmotic stress. Front. Plant Sci. 8:1684. doi: 10.3389/fpls.2017.01684
Noreen, Z., Ashraf, M., and Akram, N. A. (2010). Salt-induced regulation of some key antioxidant enzymes and physio-biochemical phenomena in five diverse cultivars of turnip (Brassica rapa L.). J. Agron. Crop Sci. 196, 273–285. doi: 10.1111/j.1439-037X.2010.00420.x
Quan, R., Shang, M., Zhang, H., Zhao, Y., and Zhang, J. (2004). Improved chilling tolerance by transformation with betA gene for the enhancement of glycinebetaine synthesis in maize. Plant Sci. 166, 141–149. doi: 10.1016/j.plantsci.2003.08.018
Reddy, P. S., Jogeswar, G., Rasineni, G. K., Maheswari, M., Reddy, A. R., Varshney, R. K., et al. (2015). Proline over-accumulation alleviates salt stress and protects photosynthetic and antioxidant enzyme activities in transgenic sorghum [Sorghum bicolor (L.) Moench]. Plant Physiol. Biochem. 94, 104–113. doi: 10.1016/j.plaphy.2015.05.014
Rehman, H. U., Alharby, H. F., Bamagoos, A. A., Abdelhamid, M. T., and Rady, M. M. (2021). Sequenced application of glutathione as an antioxidant with an organic biostimulant improves physiological and metabolic adaptation to salinity in wheat. Plant Physiol. Biochem. 158, 43–52. doi: 10.1016/j.plaphy.2020.11.041
Saddhe, A. A., Mishra, A. K., and Kumar, K. (2021). Molecular insights into the role of plant transporters in salt stress response. Physiol. Plant. 173, 1–14. doi: 10.1111/ppl.13453
Shabala, S., and Pottosin, I. (2014). Regulation of potassium transport in plants under hostile conditions: implications for abiotic and biotic stress tolerance. Physiol. Plant. 151, 257–279. doi: 10.1111/ppl.12165
Shah, T., Latif, S., Saeed, F., Ali, I., Ullah, S., Alsahli, A. A., et al. (2020). Seed priming with titanium dioxide nanoparticles enhances seed vigor, leaf water status, and antioxidant enzyme activities in maize (Zea mays L.) under salinity stress. J. King Saud Univ. Sci. 33:101207. doi: 10.1016/j.jksus.2020.10.004
Sheteiwy, M. S., Elgawad, H. A., Xiong, Y. C., Macovei, A., Brestic, M., Skalicky, M., et al. (2021). Inoculation with Bacillus amyloliquefaciens and mycorrhiza confers tolerance to drought stress and improve seed yield and quality of soybean plant. Physiol. Plant. 172, 2153–2169. doi: 10.1111/ppl.13454
Sheteiwy, M. S., Shao, H., Qi, W., Daly, P., Sharma, A., Shaghaleh, H., et al. (2020). Seed priming and foliar application with jasmonic acid enhance salinity stress tolerance of soybean (Glycine max L.) seedlings. J. Sci. Food. Agric. 101, 2027–2041. doi: 10.1002/jsfa.10822
Shi, X. L., Zhou, D. Y., Guo, P., Zhang, H., and Yu, H. Q. (2020). External potassium mediates the response and tolerance to salt stress in peanut at the flowering and needling stages. Photosynthetica 58, 1141–1149. doi: 10.32615/ps.2020.070
Soliman, M., Elkelish, A., Souad, T., Alhaithloul, H., and Farooq, M. (2020). Brassinosteroid seed priming with nitrogen supplementation improves salt tolerance in soybean. Physiol. Mol. Biol. Plants 26, 501–511. doi: 10.1007/s12298-020-00765-7
Song, J., and Wang, B. (2014). Using euhalophytes to understand salt tolerance and to develop saline agriculture: Suaeda salsa as a promising model. Ann. Bot. 115, 541–553. doi: 10.1093/aob/mcu194
Summart, J., Thanonkeo, P., Panichajakul, S., Prathepha, P., and McManus, M. T. (2010). Effect of salt stress on growth, inorganic ion and proline accumulation in Thai aromatic rice, Khao Dawk Mali 105, callus culture. Afr. J. Biotechnol. 9, 145–152. doi: 10.1186/1475-2859-9-1
Tabassum, T., Farooq, M., Ahmad, R., Zohaib, A., and Wahid, A. (2017). Seed priming and transgenerational drought memory improves tolerance against salt stress in bread wheat. Plant Physiol. Biochem. 118, 362–369. doi: 10.1016/j.plaphy.2017.07.007
Tanou, G., Fotopoulos, V., and Molassiotis, A. (2012). Priming against environmental challenges and proteomics in plants: update and agricultural perspectives. Front. Plant Sci. 3:216. doi: 10.3389/fpls.2012.00216
Tian, M., Gu, Q., and Zhu, M. (2003). The involvement of hydrogen peroxide and antioxidant enzymes in the process of shoot organogenesis of strawberry callus. Plant Sci. 165, 701–707. doi: 10.1016/S0168-9452(03)00224-3
Velikova, V., Yordanov, I., and Edreva, A. (2000). Oxidative stress and some antioxidant systems in acid rain-treated bean plants – protective role of exogenous polyamines. Plant Sci. 151, 59–66. doi: 10.1016/s0168-9452(99)00197-1
Wang, N., Wang, X., Shi, J., Liu, X., Xu, Q., Zhou, H., et al. (2019). Mepiquat chloride-priming induced salt tolerance during seed germination of cotton (Gossypium hirsutum L.) through regulating water transport and K+ /Na+ homeostasis. Environ. Exp. Bot. 159, 168–178. doi: 10.1016/j.envexpbot.2018.12.024
Wani, S. H., Tripathi, P., Zaid, A., Challa, G. S., Kumar, A., Kumar, V., et al. (2018). Transcriptional regulation of osmotic stress tolerance in wheat (Triticum aestivum L.). Plant Mol. Biol. 97, 469–487. doi: 10.1007/s11103-018-0761-6
Yang, S., Ulhassan, Z., Shah, A. M., Khan, A. R., Azhar, W., Hamid, Y., et al. (2021). Salicylic acid underpins silicon in ameliorating chromium toxicity in rice by modulating antioxidant defense, ion homeostasis and cellular ultrastructure. Plant Physiol. Biochem. 166, 1001–1013. doi: 10.1016/j.plaphy.2021.07.013
Yang, Y., and Guo, Y. (2018). Elucidating the molecular mechanisms mediating plant salt-stress responses. New Phytol. 217, 523–539. doi: 10.1111/nph.14920
Yuan, F., Leng, B., and Wang, B. (2016). Progress in studying salt secretion from the salt glands in recretohalophytes: how do plants secrete salt? Front. Plant Sci. 7:977. doi: 10.3389/fpls.2016.00977
Zhang, F., Yu, J., Johnston, C. R., Wang, Y., Zhu, K., Lu, F., et al. (2015). Seed priming with polyethylene glycol induces physiological changes in sorghum (Sorghum bicolor L. Moench) seedlings under suboptimal soil moisture environments. PLoS One 10:e0140620. doi: 10.1371/journal.pone.0140620
Zhang, G., Tanakamaru, K., Abe, J., and Morita, S. (2007). Influence of waterlogging on some anti-oxidative enzymatic activities of two barley genotypes differing in anoxia tolerance. Acta Physiol. Plant. 29, 171–176. doi: 10.1007/s11738-006-0022-1
Zhao, S., Zhang, Q., Liu, M., Zhou, H., Ma, C., and Wang, P. (2021). Regulation of plant responses to salt stress. Int. J. Mol. Sci. 22:4609. doi: 10.3390/ijms22094609
Keywords: sorghum germination, seed priming, saline stress, osmotic adjustment, ion transport, calcium signaling
Citation: Chen X, Zhang R, Li B, Cui T, Liu C, Liu C, Chen B and Zhou Y (2022) Alleviation of Oxidative Damage Induced by CaCl2 Priming Is Related to Osmotic and Ion Stress Reduction Rather Than Enhanced Antioxidant Capacity During Germination Under Salt Stress in Sorghum. Front. Plant Sci. 13:881039. doi: 10.3389/fpls.2022.881039
Received: 22 February 2022; Accepted: 11 April 2022;
Published: 29 April 2022.
Edited by:
Marcelo Pompelli, Federal University of Pernambuco, BrazilCopyright © 2022 Chen, Zhang, Li, Cui, Liu, Liu, Chen and Zhou. This is an open-access article distributed under the terms of the Creative Commons Attribution License (CC BY). The use, distribution or reproduction in other forums is permitted, provided the original author(s) and the copyright owner(s) are credited and that the original publication in this journal is cited, in accordance with accepted academic practice. No use, distribution or reproduction is permitted which does not comply with these terms.
*Correspondence: Bingru Chen, chenbingru1979@163.com; Yufei Zhou, zhouyufei2002@aliyun.com; zhouyufei@syau.edu.cn