- 1Department of Horticultural Biotechnology, Kyung Hee University, Yongin, South Korea
- 2Graduate School of Green-Bio Science, Kyung Hee University, Yongin, South Korea
Clavibacter michiganensis is a Gram-positive bacterium that causes bacterial canker and wilting in host plants like tomato. Two major virulence genes encoding a cellulase (celA) and a putative serine protease (pat-1) have been reported. Here we show that Nicotiana benthamiana, a commonly used model plant for studying molecular plant–pathogen interactions, is a surrogate host of C. michiganensis and C. capsici. When a low concentration of two Clavibacter species, C. michiganensis and C. capsici, were infiltrated into N. benthamiana leaves, they caused blister-like lesions closely associated with cell death and the generation of reactive oxygen species and proliferated significantly like a pathogenic bacterium. By contrast, they did not cause any disease symptoms in N. tabacum leaves. The celA and pat-1 mutants of C. michiganensis still caused blister-like lesions and cankers like the wild-type strain. When a high concentration of two Clavibacter species and two mutant strains were infiltrated into N. benthamiana leaves, all of them caused strong and rapid necrosis. However, only C. michiganensis strains, including the celA and pat-1 mutants, caused wilting symptoms when it was injected into stems. When two Clavibacter species and two mutants were infiltrated into N. tabacum leaves at the high concentration, they (except for the pat-1 mutant) caused a strong hypersensitive response. These results indicate that C. michiganensis causes blister-like lesions, canker, and wilting in N. benthamiana, and celA and pat-1 genes are not necessary for the development of these symptoms. Overall, N. benthamiana is a surrogate host of Clavibacter species, and their novel virulence factors are responsible for disease development in this plant.
Introduction
Nicotiana benthamiana is commonly used as a model plant in many studies of plant–microbe interactions (Goodin et al., 2008; Bombarely et al., 2012). This plant has many benefits: a rapid life cycle, ease of seed harvest, and relatively small plant size (which precludes any large space requirement) (Davarpanah et al., 2009). N. benthamiana has been shown to be susceptible via artificial inoculation against many kinds of pathogenic microorganisms, such as Gram-negative bacteria, fungi, oomycetes, and viruses. Thus, this plant has been used in studies of various molecular interactions with microbes mostly via virus-induced gene silencing and transient expression assay (Liu et al., 2002; Tran et al., 2016). However, pathogenic interactions between N. benthamiana and Gram-positive bacteria, including Clavibacter species, have not been well studied.
The genus Clavibacter belongs to the family Microbacteriaceae within Actinobacteria and includes seven Gram-positive and plant-pathogenic species (Li et al., 2018). C. michiganensis is an important bacterial pathogen of the tomato plant (Solanum lycopersicum). It can be transmitted by, and survive in, contaminated seeds and plant debris of tomatoes (de León et al., 2011; Tancos et al., 2013). When C. michiganensis invades the tomato plant through wounded stems, roots, leaves, and natural openings, it moves to plant xylem, eventually impairing xylem vessels and causing wilting. Moreover, it causes various disease symptoms in tomato, such as cankers on stems, blister lesions on leaves, bird’s eye lesions on fruits, and plant death (Medina-Mora et al., 2001; Sen et al., 2015; Chalupowicz et al., 2017). C. capsici causes bacterial canker in stems, fruits, and leaves of pepper plants. This bacterium was re-classified from C. michiganensis by biochemical, physiological, and phylogenetic analyses (Oh et al., 2016).
A few virulence factors of C. michiganensis have been reported. A pathogenicity island (PAI), a subset of genomic islands, is present on the chromosome (Gartemann et al., 2008). Approximately 129 kb of PAI carries the chp/tomA region, further subdivided into two subregions, namely, the chp and the tomA subregions. These subregions carry genes encoding putative serine proteases, such as chpC and chpG (Stork et al., 2008), and tomatinase (Kaup et al., 2005; Eichenlaub and Gartemann, 2011), respectively. C. michiganensis also harbors two major virulence genes in two plasmids, namely, pCM1 and pCM2; each plasmid carries celA and pat-1 genes, which encode cellulase (endo-β-1,4-glucanase) and a putative serine protease, respectively (Dreier et al., 1997; Jahr et al., 2000; Hwang et al., 2019). In a previous study, each plasmid-cured mutant strain showed reduced and/or lost pathogenicity, compared with the wild-type (WT) strain, whereas each maintained its proliferation ability (Chalupowicz et al., 2012), indicating that these two genes in plasmids are critical for virulence in tomatoes. In the case of C. capsici, its virulence factors have not been well-studied yet, but some chp genes, such as chpG and chpE genes, present in pCM1Cc plasmid have been reported (Hwang et al., 2018, 2020).
C. michiganensis has been shown to naturally infect other Solanum plants, such as eggplant (S. melongena), potato (S. tuberosum), and black nightshade (S. nigrum) (Bradbury, 1986; Ignatov et al., 2019), besides its well-known tomato host plant. Moreover, plants in other genera, such as Datura, Hyoscyamus, and Physalis, can be infected; disease symptoms can also develop via artificial inoculation with C. michiganensis (Thyr et al., 1975; Eichenlaub et al., 2007). After inoculation with C. michiganensis, N. benthamiana plant displayed canker symptoms on its stems (Balaji et al., 2011), indicating this plant’s potential as a surrogate host for C. michiganensis. Artificial or surrogate host plants displayed disease symptoms via artificial inoculation of the pathogens. For example, the model plant Arabidopsis thaliana is routinely used as a surrogate host plant to study molecular plant interactions with Pseudomonas syringae pv. tomato DC3000, which causes bacterial speck disease on tomato (Xin and He, 2013). N. benthamiana has been reported to be a surrogate host of plant pathogens including Acidovorax citrulli, which causes bacterial fruit blotch in cucurbits. This bacterium caused disease symptoms of water-soaking-like cell death, but not hypersensitive response (HR), on N. benthamiana leaves via syringe infiltration (Traore et al., 2019).
In this study, we conducted several experiments to investigate the pathogenic interactions between N. benthamiana and the representative species of genus Clavibacter, that is, C. michiganensis and C. capsici. We also examined whether the known important virulence genes of C. michiganensis in tomato are responsible for the development of disease symptoms in N. benthamiana. We found that N. benthamiana is a surrogate host plant of C. michiganensis and C. capsici, and different virulence genes of C. michiganensis are required for the development of blister-like lesions, rapid necrosis, canker, and wilting.
Materials and Methods
Plant Materials and Growth Conditions
Two tobacco species, N. benthamiana and N. tabacum, were grown in 32 cell seedling trays filled with sterile commercial soil (Baroker, Seoul Bio Co., Ltd., Eumseong, Korea) in a growth chamber at 26°C with a photoperiod of 14 h of light and 10 h of darkness. Five- to six-week-old N. benthamiana plants were used for virulence tests via syringe infiltration, stem inoculation, and spray inoculation. N. benthamiana plants at the four- to six-leaf stage (around 3 weeks old) were used for virulence tests via root-dip inoculation. Seven- to eight-week-old N. tabacum plants were used for virulence and bacterial growth tests on the leaves via syringe infiltration.
Bacterial Strains and Growth Conditions
C. michiganensis type strain LMG7333T (Hwang et al., 2019), C. capsici type strain PF008T (Oh et al., 2016), and A. citrulli strain Ac8 (Song et al., 2020) were cultured at 26°C for 48 h in the King’s B medium (20 g/l of protease peptone number 3, 1.5 g/l of K2HPO4, 6 ml/l of 1 M MgSO4, and 16 ml/l of 50% glycerol). Tn::celA (Hwang et al., 2019) and Tn::pat-1 mutants (Hwang et al., 2022) of C. michiganensis LMG7333T were streaked onto the King’s B medium with 10 mg/l of chloramphenicol at 26°C for 48 h.
Virulence Assay in Nicotiana Plants
For the leaf infiltration, five- to six-week-old N. benthamiana and seven- to eight-week-old N. tabacum plant leaves were infiltrated with either sterilized distilled water (mock) or 5×104 CFU/ml bacterial suspension or approximately 108 CFU/ml (OD600 = 0.1) bacterial suspension using a needleless syringe; at least three leaves were infiltrated per treatment. All inoculated plants were placed in a growth chamber at 26°C and 50% humidity. The development of blister-like symptoms and necrosis was observed for 8 days and 48 hours, respectively. This assay was repeated at least three times.
For stem inoculation, five- to six-week-old N. benthamiana plants were injected with 20 ul of 10 mM MgCl2 (mock) or 108 CFU/ml (OD600 = 0.1) bacterial suspension after wounding the stems with a pipette tip. All inoculated plants were placed in a growth chamber at 26°C and 50% humidity. Wilting development was observed for 3 weeks. Wilted leaves were counted and compared with the number of fully grown leaves above the inoculation site. The ratio between wilted leaves and fully grown leaves was calculated by percentage. Bacterial canker symptoms on stem inoculation sites were observed at 0, 5, 10, and 15 days after inoculation (dai), and their sizes were measured as lengths of their longitudinal crack using a ruler. This assay was repeated at least three times.
For the root-dip inoculation, N. benthamiana plants in the four- to six-leaf stage were pulled out and dipped in the bacterial suspension (approximately 109 CFU/ml, OD600 = 2.0) for 30 or 40 min, depending on plant size. Then, the inoculated plants were replanted into soil in a new tray. All inoculated plants were placed in a growth chamber at 26°C and 50% humidity. Wilting development was observed for 3 weeks. Root-inoculated N. benthamiana plants were analyzed by the number of plants displaying wilting using five categories defining the severity of wilting symptoms: (1) 0 = no visible wilting; (2) 1 = 1–25% wilting symptoms; (3) 2 = 26–50% wilting symptoms; (4) 3 = 51–75% wilting symptoms; (5) 4 = 76–100% wilting symptoms or dead at around 18 dai. The disease index used in this study was described in previous research (Shinohara et al., 2005). This assay was repeated at least three times.
For the spray inoculation, N. benthamiana plants were sprayed with 50 ml of bacterial suspensions (approximately 5×106 CFU/ml, OD600 = 0.005 and 108 CFU/ml, OD600 = 0.1) containing 0.02% Silwet L−77 (MOMENTIVE Co., Ltd., Seoul, Korea). Wilting development was observed for more than 3 weeks. All inoculated plants were placed in a growth chamber at 26°C and 50% humidity. This assay was repeated twice.
Measurement of Bacterial Growth in Nicotiana Leaves
To measure the bacterial growth inside both N. benthamiana and N. tabacum leaves after syringe infiltration with 5×104 CFU/ml bacterial suspension, three-leaf disks (1 cm in diameter) were collected from the infiltrated leaves of at least three different plants using a cork borer at 0, 2, 4, 6, and 8 dai. The collected leaf disks were washed with 70% ethanol for 30 s for surface sterilization and were rinsed with sterilized distilled water twice. Next, washed leaf disc samples were ground by vortexing them in 2 ml microtubes with two iron beads and 1 ml of sterilized distilled water. The homogenate was serially diluted and put onto a KB agar plate. The numbers of colony-forming units (CFU) were calculated after 48–72 h at 26°C.
Ion Conductivity Measurement in Nicotiana Leaves
For quantification of necrosis in N. benthamiana leaves, ion conductivity was measured as described in the previous study (Choi et al., 2012). At least 4 leaves selected from different plants were infiltrated with 108 CFU/ml (OD600 = 0.1) bacterial suspension using needleless syringe. After drying drenched leaves completely for 60 or 90 min, six-leaf disks (1 cm in diameter) were taken from each dried leaf using a cork borer. The leaf disks were moved to a 50 ml snap tube with 25 ml of sterilized distilled water to remove the initially leaked ion until 3 h after inoculation (hai). Then, water inside a 50 ml snap tube was removed completely, except for leaf disks, and 25 ml of sterilized distilled water was added to the snap tube again. Ion conductivity was measured using a conductivity meter (Acorn CON6 portable conductivity meter, Oakton Instrument, Vernon Hills, IL, USA) at 3, 12, 24, 36, and 48 hai. The snap tubes with leaf disks were incubated on the shaker at 60 rpm. Four of 50 ml snap tubes with six-leaf disks and 25 ml sterilized distilled water were used for each bacterial strain. The experiments were repeated at least twice.
To quantify the HR in N. tabacum leaves, six-leaf disks (1 cm in diameter) were collected from the infiltrated leaves with each bacterial strain at 3, 9, 15, and 21 hai. Leaf disks were moved to a 50 ml snap tube with 25 ml of sterilized distilled water and were washed for an hour. Then, the same volume of water was added to the snap tube. Next, those tubes were incubated for an hour in the shaking incubator at 26°C and 180 rpm, and ion conductivity was measured using a conductivity meter. The experiments were repeated at least twice.
Trypan Blue Staining in N. benthamiana Leaves
The trypan blue staining procedure was modified from a previous study (Guo et al., 2019). Briefly, the leaf disks (1 cm in diameter) from leaves of at least 3 different N. benthamiana plants infiltrated using a syringe with 5×104 CFU/ml of C. michiganensis LMG7333T and C. capsici PF008T were taken using a cork borer at 0, 3, 5, and 7 dai. Sterilized distilled water was used for mock treatment. Leaf disks were treated with trypan blue to stain damaged or dead cells. A 3 ml trypan blue solution (10 ml lactic acid, 10 g phenol, 10 ml glycerol, 10 ml distilled water, and 10 mg trypan blue) was added into a 5 ml tube with leaf disks. One day later, the trypan blue solution was exchanged with 4 ml of absolute ethanol to remove unstained trypan blue. After 1 day of ethanol bleaching, the absolute ethanol in the 5 ml tube was exchanged with 70% ethanol for storage until the time that leaf disks were observed using a microscope. Leaf disks were observed using an optical microscope (ECLIPSE E200LED MV R, Nikon Corporation, Tokyo, Japan) at a magnification x40.
3,3′-Diaminobenzidine Staining in N. benthamiana Leaves
The DAB staining method was followed and modified from a previous study (Shi et al., 2014). Briefly, leaf disks (1 cm in diameter) were collected from at least three different N. benthamiana plants infiltrated with 5×104 CFU/ml of C. michiganensis LMG7333T and C. capsici PF008T using a cork borer at 0, 1, 3, 5, and 7 dai. Sterilized distilled water was used for mock treatment. The collected leaf disks were placed in the petri dish and stained with 10 ml of 0.1% DAB solution for 1 day. Next, DAB solution was removed from the petri dish, and 10 ml of absolute ethanol was added to the petri dish to bleach the leaf disk chlorophyll. After 1 day of ethanol bleaching, absolute ethanol in the petri dish was exchanged with 70% ethanol for storage until the time that leaf disks were observed using a microscope. All DAB-stained N. benthamiana leaf disks were observed using an optical microscope at a magnification x40.
Detection of Clavibacter Bacteria in Plants via PCR Analysis
PCR analysis was conducted for the detection of Clavibacter bacteria in the plants after inoculation. PCR amplification proceeded according to the manufacturer’s manual for 2x Taqbasic PCR Master Mix 2 (Biofact, Daejeon, Korea). The total volume was 20 uL and consisted of 10 ul of 2X Taqbasic PCR Master Mix 2, 1 ul of template, 1ul of forward and reverse primers (10 pmol/ul), and 7 ul of sterilized distilled water.
Several specific primer pairs were used as follows. Primer pair CMR16S F (5′-gtgatgtcagagcttcctctggcggat-3) and CMR16S R (5′-gtacggctaccttgttacgacttagt-3′) were used for C. michiganensis LMG7333T and C. capsici PF008T, because they specifically target the 16S-rRNA gene of Clavibacter species (Yim et al., 2012). For confirmation of C. michiganensis Tn::celA and Tn::pat-1 mutant strains, the H1 (5′-atgacatttcgccaagttcgtgca-3′) and H2 (5′-tcagtgcacagggtagaagcg-3′) primer pair was used for celA gene. The EB15 (5′- actagtagaacgctccctgcggccttcg-3′) and EB16 (5′- aagcttacttgtcgtcatcgtctttgtagtcggaggtcgctaatatgtaatacggt-3′) primer pair was used for pat-1 gene.
Statistical Analysis
Bacterial growth was expressed as mean and standard deviations. The disease index of stem and root inoculation was calculated as the mean and standard error. Disease severity data were analyzed via non-parametric Kruskal–Wallis test (p < 0.05). Duncan’s multiple range test was conducted for statistical analysis of the parametric comparison between independent groups (p < 0.05). All experiments were repeated at least two or three times.
Results
Two C. Species Caused Blister-Like Lesions in Leaves of N. benthamiana, but Not N. tabacum
To investigate whether Gram-positive Clavibacter bacteria can cause any disease symptoms in model plants of Nicotiana species, both C. michiganensis LMG7333T and C. capsici PF008T were infiltrated into leaves of two Nicotiana species, N. benthamiana and N. tabacum. N. benthamiana leaves showed white granular particles, called blister-like lesions, when 5×104 CFU/ml of each bacterial species was infiltrated (Figure 1A). These lesions were generated on the leaf surface around 4 dai. The formation of blisters was originally reported in tomato, the host plant of C. michiganensis (Chalupowicz et al., 2017), and blister-like lesions on N. benthamiana appeared phenotypically similar to those in tomato leaves. Conversely, N. tabacum leaves did not display any disease symptoms by infiltration with the same titer of either C. michiganensis LMG7333T or C. capsici PF008T suspension (Figure 1B). In N. benthamiana leaves, the infiltrated bacterial pathogens grew significantly up to 106-fold more than the initial inoculum until 8 dai (Figure 1C); an increase was more than that by the known pathogen A. citrulli. However, the bacterial titer of both Clavibacter species increased only 102- to103-fold more in N. tabacum leaves from the initial inoculum without any symptoms, and this increase was higher than that by A. citrulli (Figure 1D). Moreover, the bacterial titer of C. capsici was significantly higher than C. michiganensis (Figure 1D). These results indicate that N. benthamiana appears to be a surrogate host plant of two Clavibacter species, whereas N. tabacum appears to be a non-host plant.
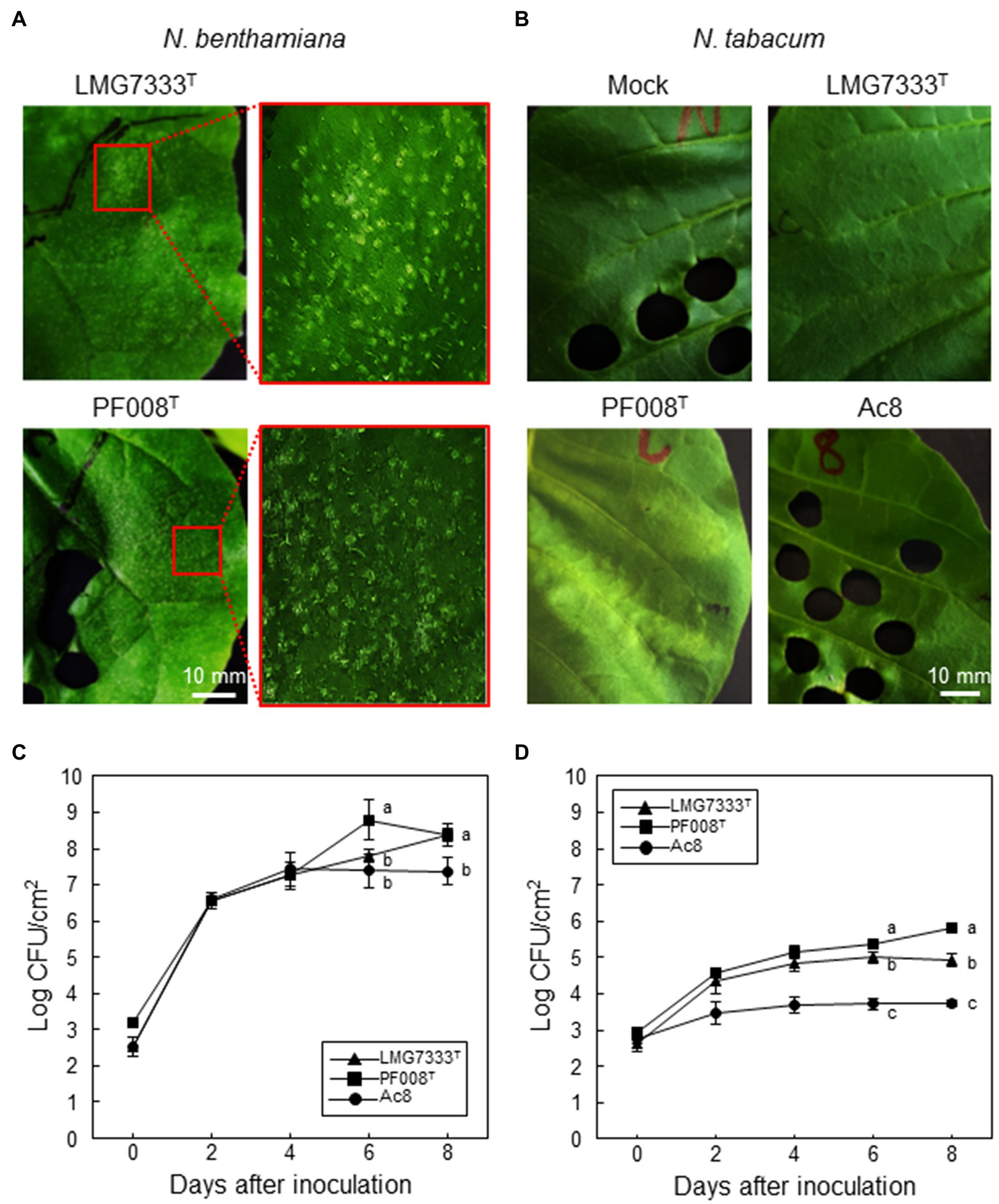
Figure 1. Disease symptoms and bacterial growth in N. benthamiana and N. tabacum leaves. Both Clavibacter species induced blister-like symptoms on N. benthamiana (A), whereas no visible symptoms were induced on N. tabacum (B), when 5×104 CFU/ml of C. michiganensis LMG7333T and C. capsici PF008T bacterial suspensions were infiltrated. Photos were taken at 4 days after infiltration (dai). Mock, 10 mM MgCl2. Bacterial growth in N. benthamiana (C) and N. tabacum (D) leaves was measured at the indicated time points. The A. citrulli strain Ac8 was used as a positive control. An average and standard deviation (n = 3) of each bacterial titer are shown in the figures. The different letters at 6 and 8 dai in the graphs indicate a statistically significant difference analyzed via Duncan’s multiple range test (p < 0.05). Similar results were obtained from three independent assays. Scale bar = 10 mm.
Blister-Like Lesions on N. benthamiana Leaves Are Closely Associated With Cell Death and the Generation of Reactive Oxygen Species
To investigate features of blister-like lesions on N. benthamiana leaves after infiltration with C. michiganensis LMG7333T and C. capsici PF008T, leaf disks from infiltrated N. benthamiana leaves were collected and treated with trypan blue solution 0, 1, 3, 5, and 7 dai (Figure 2). Dead cells turned to blue after staining, as trypan blue is normally used for observation of such cells. Blue color spots began to appear from 3 dai, and the number and size of those spots were gradually increased (Figure 2). These patterns were exactly matched with blister-like lesions, indicating that plant cells in blister-like lesions are undergoing death.
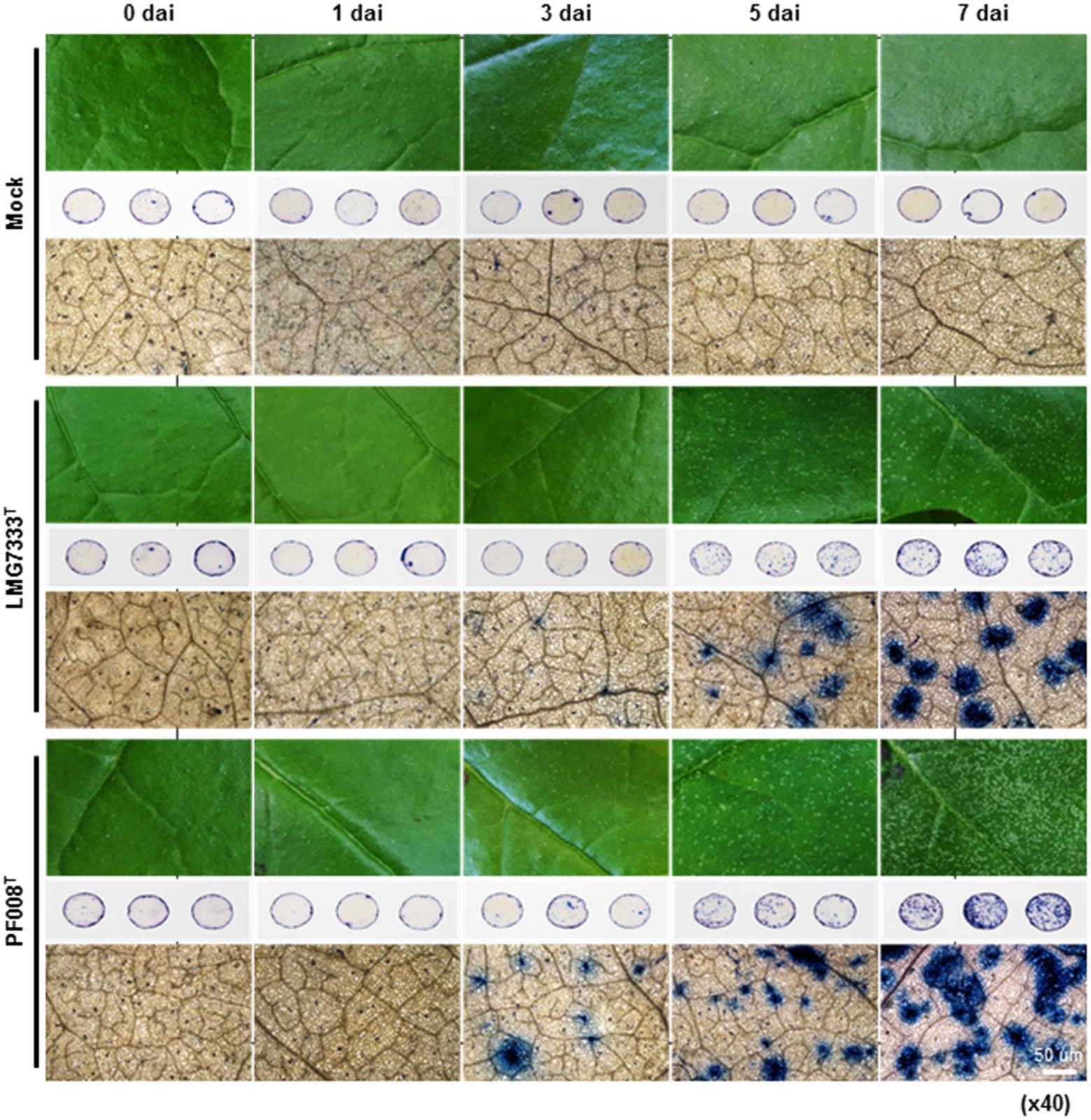
Figure 2. Trypan blue staining of N. benthamiana leaves showing blister-like symptoms, after infiltration with 5×104 CFU/ml bacterial suspensions of C. michiganensis LMG7333T and C. capsici PF008T. Three-leaf disks (1 cm in diameter) per treatment were collected for trypan blue staining at 0, 1, 3, 5, and 7 days after inoculation (dai). Stained leaf disks were observed using an optical microscope at magnification x40. Sterilized distilled water was used for mock treatment. Scale bar = 50 um.
Generally, ROS generation is accompanied by cell death (Balint-Kurti, 2019). Thus, the 3,3′-diaminobenzidine (DAB) staining was conducted with leaf disks collected from N. benthamiana leaves after infiltration with two Clavibacter species (Supplementary Figure S1). The stained spots by DAB began to appear from 3 dai, and the intensity gradually increased (Supplementary Figure S1). Like trypan blue staining, DAB-stained spots exactly matched with the areas of blister-like lesions, indicating that blister-like lesions are generated by cell death, and accompanied by the generation of ROS, such as hydroxy peroxide.
Two Important Virulence Genes of C. michiganensis in Tomatoes Are Not Required for the Development of Blister-Like Lesions and Rapid Necrosis on N. benthamiana Leaves
Previously, it was shown that celA and pat-1 genes of C. michiganensis are critical for disease development in tomatoes (Gartemann et al., 2003; Hwang et al., 2019). Thus, we tried to determine whether these two important virulence genes are required for the development of blister-like lesions on N. benthamiana leaves. For this, Tn::celA and Tn::pat-1 mutant strains of C. michiganensis LMG7333T were infiltrated into N. benthamiana leaves, and the formation of blister-like lesions was monitored. Intriguingly, when 5×104 CFU/ml of each mutant strain was infiltrated, each caused as many blister-like lesions on leaves as the WT strain (Figure 3A). Moreover, those mutant strains grew as much as the WT strain (Figure 3B). These results indicate that two known important virulence genes are not required for the formation of blister-like lesions in N. benthamiana.
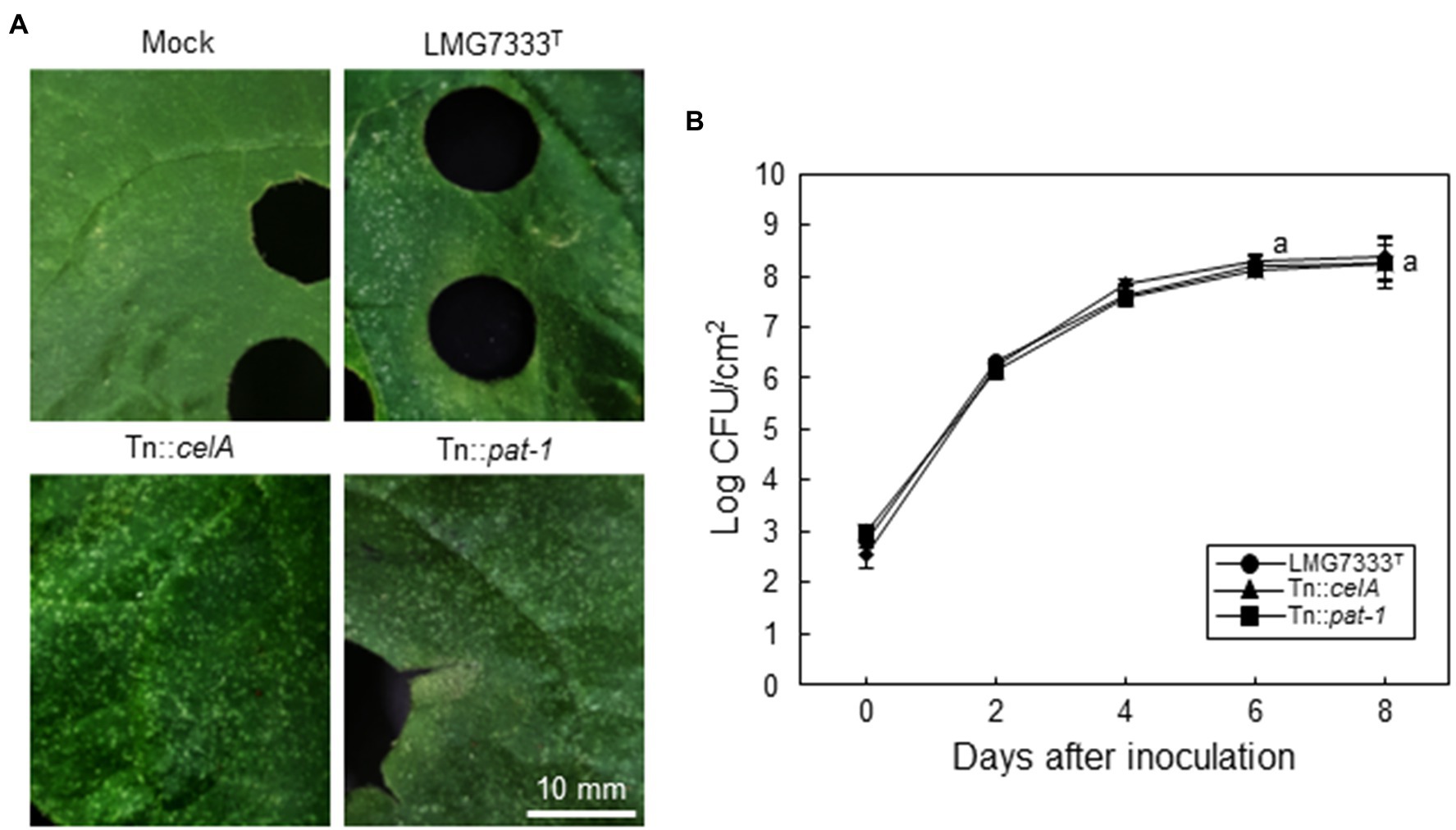
Figure 3. No contribution of two major virulence genes of C. michiganensis to the development of blister-like symptoms on N. benthamiana leaves. (A) Blister-like symptoms on N. benthamiana leaves by infiltration of 5×104 CFU/ml C. michiganensis LMG7333T and its Tn::celA or Tn::pat-1 mutant strains. The leaves were photographed at 8 days after inoculation (dai). (B) The bacterial growth of C. michiganensis LMG7333T, Tn::celA, and Tn::pat-1 strains in N. benthamiana leaves at the indicated time points. An average and standard deviation (n = 3) of each bacterial titer are shown in the figure. The letters at 6 and 8 dai in the graphs indicate a statistically significant difference analyzed via Duncan’s multiple range test (p < 0.05). Similar results were obtained from three independent assays. Scale bar = 10 mm.
To examine whether different bacterial concentrations cause different symptoms, N. benthamiana leaves were infiltrated with a higher bacterial titer (108 CFU/ml) of C. michiganensis LMG7333T or C. capsici PF008T. In this condition, the infiltrated regions by both bacterial pathogens rapidly displayed water-soaking symptoms, followed by typical necrosis by 48 h after inoculation (hai) (Figure 4A). Ion conductivity was measured in infiltrated N. benthamiana leaves to determine the speed of necrosis onset. The ion conductivity began to significantly increase from 12 hai and continuously increased until 36 hai in N. benthamiana leaves infiltrated with both Clavibacter species (Figure 4B). These results indicate that infiltration of N. benthamiana leaves with a high concentration of Clavibacter species causes rapid necrosis without blister-like lesions.
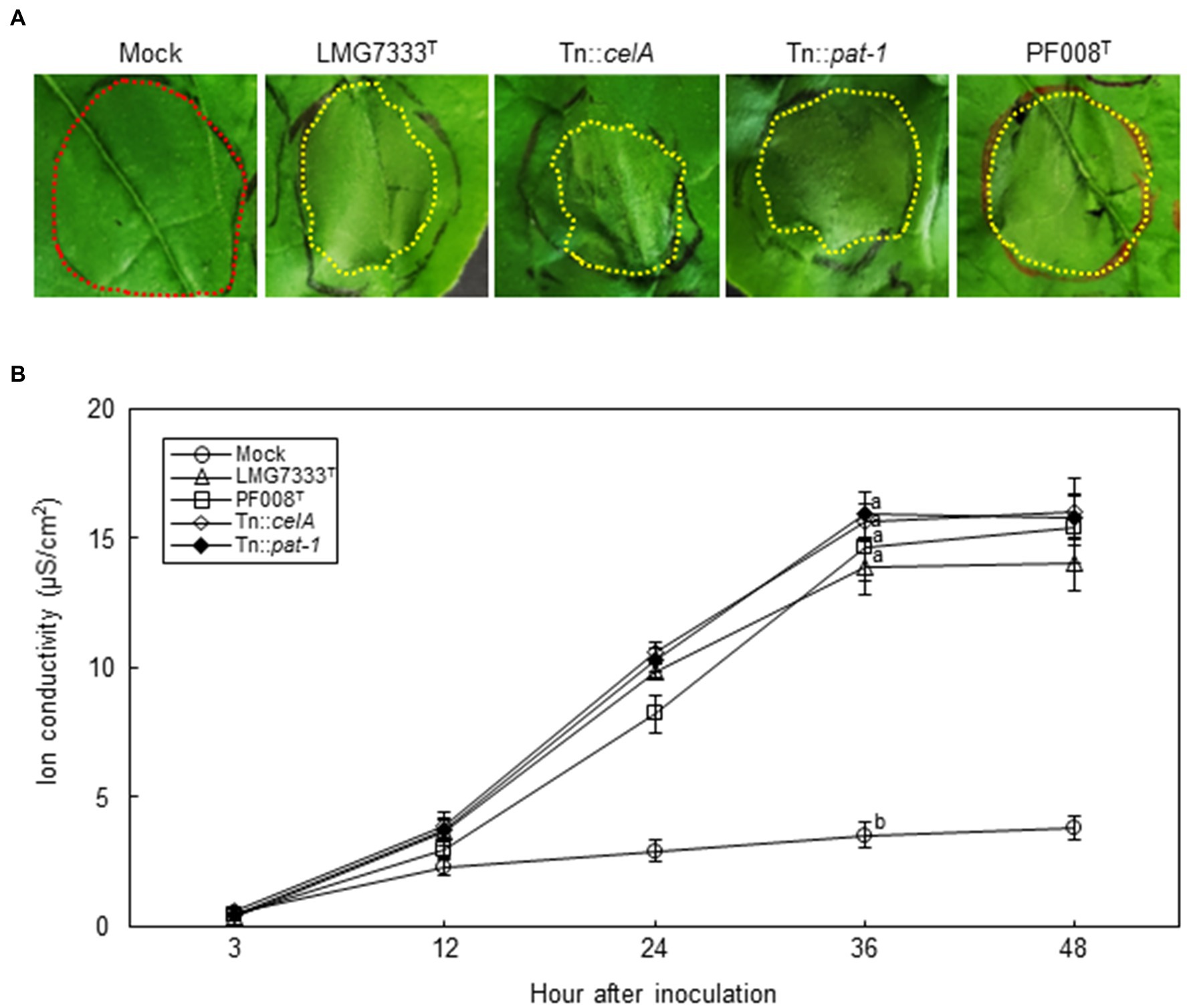
Figure 4. Influence of two major virulence genes of C. michiganensis for necrosis on N. benthamiana leaves. (A) The necrosis symptoms on N. benthamiana leaves by infiltration with 108 CFU/ml of C. capsici PF008T, C. michiganensis LMG7333T and its mutant strains. Each red and yellow dotted line indicates no symptom and necrotic parts, respectively. Photos showing necrosis symptoms were taken at 48 hours after infiltration (hai), respectively. (B) The measurement of ion conductivity on N. benthamiana leaves after inoculation with 108 CFU/ml of the bacterial strains. The letters at the time points in the graphs indicate a statistically significant difference analyzed via Duncan’s multiple range test (p < 0.05). Similar results were obtained from two independent assays. Mock, 10 mM MgCl2.
Next, to examine whether celA and pat-1 genes are required for the development of necrosis, 108 CFU/ml of Tn::celA and Tn::pat-1 mutant strains were infiltrated into N. benthamiana leaves. Results showed that these mutants caused necrosis like the WT strain (Figure 4A) and increased ion conductivity as much as the WT (Figure 4B), indicating that these two virulence genes in tomatoes are not critical for necrosis in N. benthamiana.
C. michiganensis, but Not C. capsici, Caused Wilting on N. benthamiana
C. michiganensis causes not only blister-like lesions, but also wilting and canker symptoms in tomatoes (de León et al., 2011). To examine whether both C. michiganensis LMG7333T and C. capsici PF008T cause wilting in N. benthamiana, 108 CFU/ml bacterial suspension of two Clavibacter species was injected into the main stems via the stem inoculation method, and wilting development was monitored. Notably, C. michiganensis LMG7333T caused severe wilting in N. benthamiana, as in tomatoes, whereas C. capsici PF008T did not (Figure 5A). Wilting symptoms began to develop on the unilateral side of inoculation sites in N. benthamiana. After 3 weeks, severe wilting and necrosis were observed on the whole N. benthamiana plant only by C. michiganensis LMG7333T (Figure 5B).
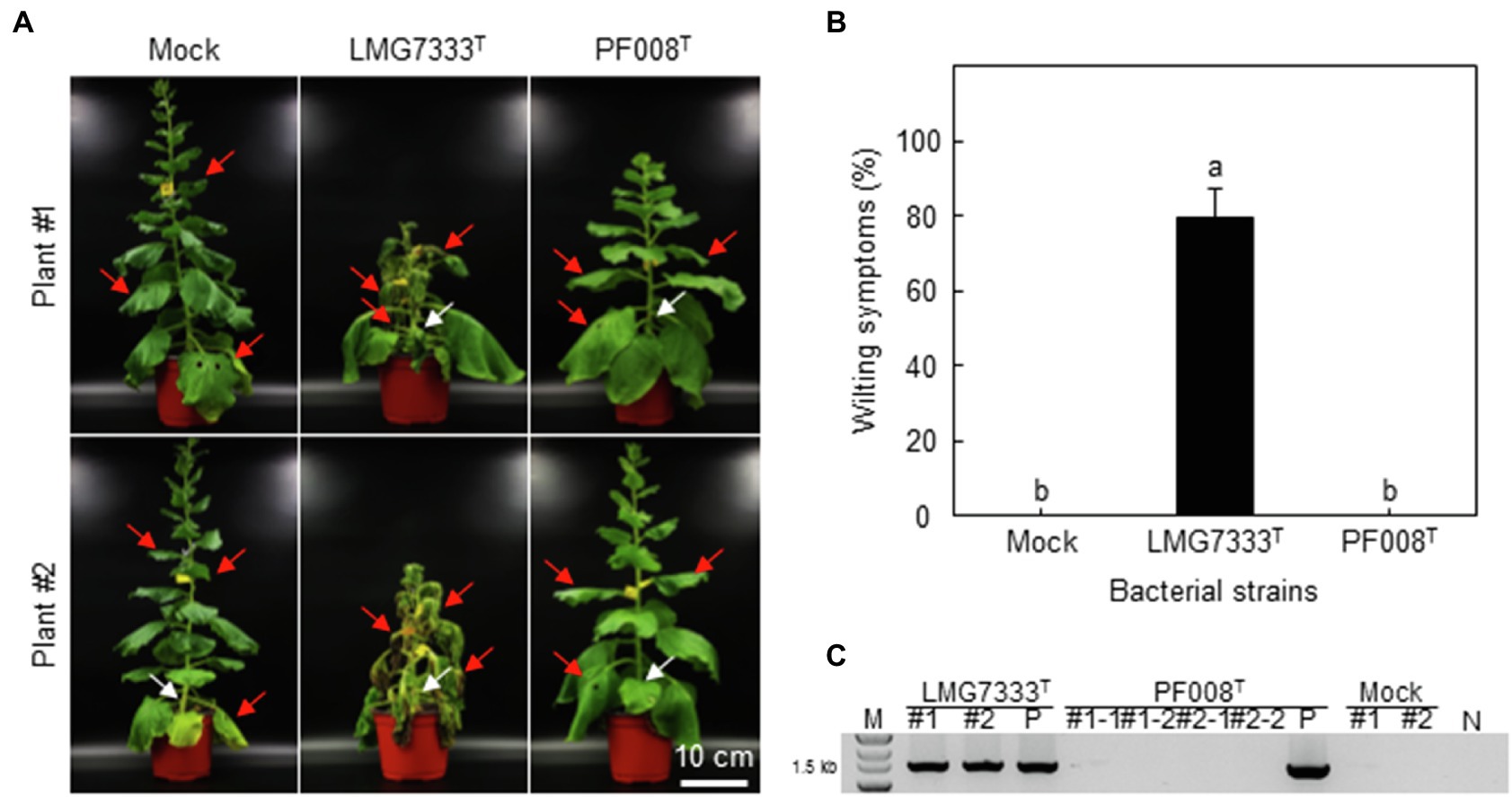
Figure 5. Wilting symptoms caused after stem inoculation with C. michiganensis LMG7333T, but not after C. capsici PF008T inoculation in N. benthamiana plants. (A) Wilting symptoms caused in N. benthamiana plants after injection with 20 ul of 108 CFU/ml of C. michiganensis LMG7333T and C. capsici PF008T. Injection sites in stems are indicated by white arrows. The inoculated plants were photographed at 21 days after inoculation (dai). (B) Quantification of wilting severity in N. benthamiana plants shown in (A). An average and standard deviation (n = 4) of wilting severity were obtained from two independent assays. The different letters on top of each bar indicate a statistically significant difference analyzed via Kruskal–Wallis test (p < 0.05). (C) Identification of inoculated bacteria in two wilted N. benthamiana plants (#1 and #2) via PCR analysis. The leaf disks (1 cm in diameter) were collected at three leaves (indicated by red arrows) from the inoculated plants at 21 dai. M, 1 kb DNA marker; P, bacterial cells of each Clavibacter species as positive control; N, no DNA. Scale bar = 10 cm.
Another main disease symptom of Clavibacter species in host plants is bacterial canker. Bacterial canker development on N. benthamiana stems by C. michiganensis infection has been previously reported (Balaji et al., 2011). Consistently, in this experiment, both C. michiganensis LMG7333T and C. capsici PF008T caused significant bacterial canker on inoculated N. benthamiana stems (Supplementary Figure S2).
To confirm that wilting was caused by C. michiganensis bacterium, the polymerase chain reaction (PCR) test was conducted for identification of this bacterium using leaf samples that were taken from locations near and above the inoculation site. PCR results showed that C. michiganensis LMG7333T could move to the entire plant, likely through its xylem vessels, whereas C. capsici PF008T could not move to nearby leaves nor in an upper direction in N. benthamiana (Figure 5C).
Clavibacter species normally invade the host plants through wounds and natural openings (Carlton et al., 1998; de León et al., 2011; Tancos et al., 2013). Thus, we sprayed a bacterial suspension of both Clavibacter species onto the N. benthamiana plants to mimic the natural invasion process. However, neither bacterial species caused any visible symptoms (Supplementary Figure S3A). PCR results also showed no evidence of the bacterial presence of both Clavibacter species inside plants (Supplementary Figure S3B). These results indicate that even C. michiganensis LMG7333T fails to actively infect N. benthamiana through stomata.
Two Important Virulence Genes of C. michiganensis in Tomatoes Are Partially Required for the Development of Wilting on N. benthamiana
To examine whether celA and pat-1 genes are required for the development of wilting in the N. benthamiana plant, 108 CFU/ml of C. michiganensis WT, Tn::celA and Tn::pat-1 mutant strains were inoculated using the stem inoculation method, and the development of wilting was monitored. Both mutants caused delayed wilting in N. benthamiana (Figure 6A), and the wilting severity was approximately 70% of that caused by WT C. michiganensis (Figure 6B). The presence of mutant strains in wilting N. benthamiana plants was confirmed via PCR test (Figure 6C). When N. benthamiana was inoculated using the root-dipping method with 109 CFU/ml of the bacterial suspensions, wilting results were similar to those of the stem inoculation method (Supplementary Figure S4). These results indicate that, like tomatoes, celA and pat-1 genes are partially required for wilting development in N. benthamiana.
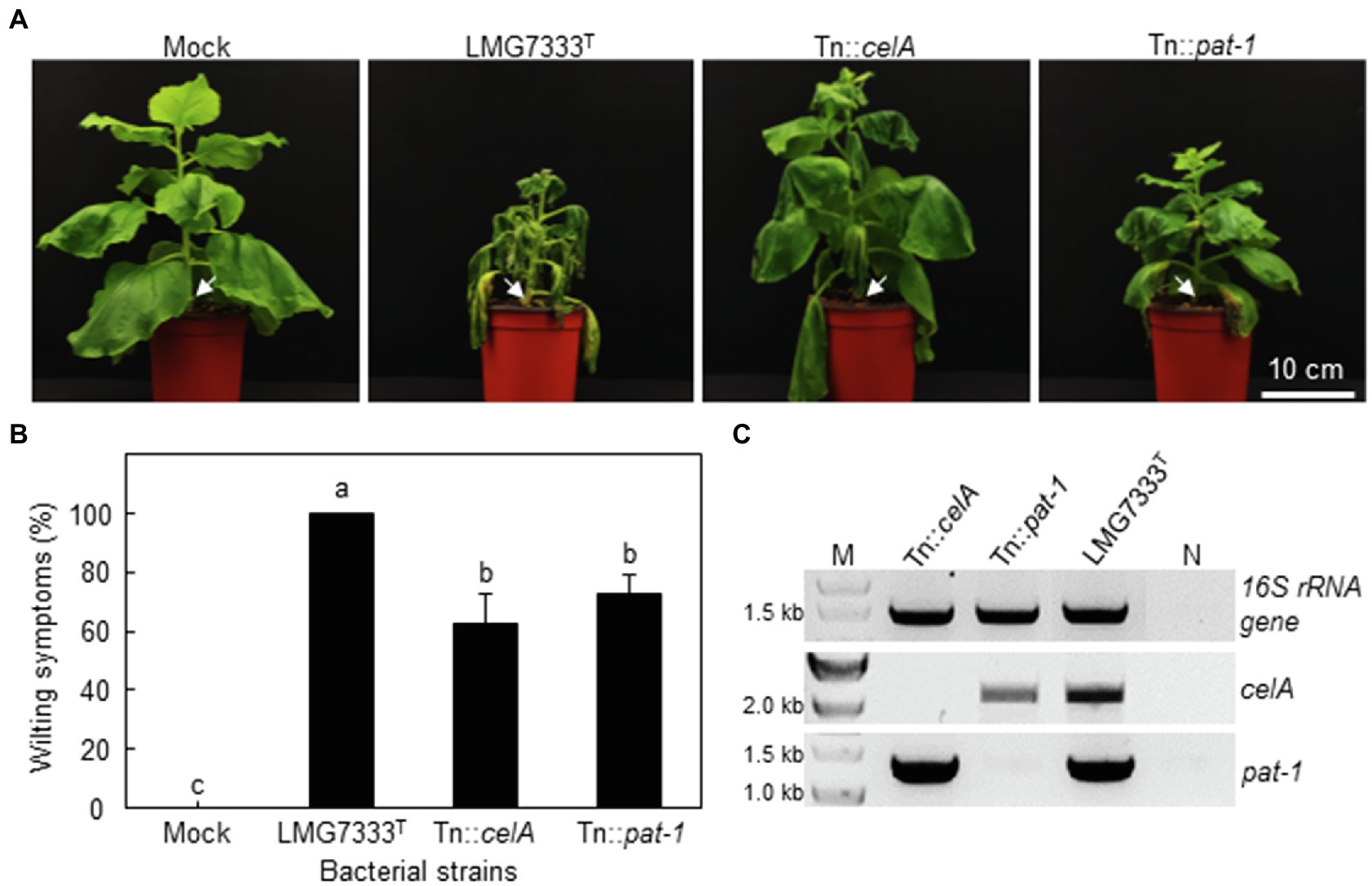
Figure 6. Influence of two major virulence genes of C. michiganensis in N. benthamiana plants on the development of wilting symptoms. (A) Wilting symptoms in N. benthamiana after stem inoculation with 108 CFU/ml of C. michiganensis LMG7333T and its Tn::celA and Tn::pat-1 mutant strains. Inoculation sites are indicated by white arrows. The inoculated plants were photographed at 21 days after inoculation (dai). (B) Quantification of wilting severity in N. benthamiana plants shown in (A). An average and standard deviation (n = 4) of wilting severity are shown in the figures. The different letters on top of each bar indicate a statistically significant difference analyzed via Kruskal–Wallis test (p < 0.05). Similar results were obtained from three independent assays. (C) Confirmation of inoculated bacteria via PCR analysis with primer sets specific to the indicated genes. M, 1 kb DNA marker; N, no DNA. Scale bar = 10 cm.
HR-Like Cell Death Was Induced on N. tabacum Leaves by Clavibacter Species
We showed that no disease symptoms were observed after infiltration of a low bacterial concentration (5×104 CFU/ml) of two Clavibacter species into N. tabacum leaves (Figure 1). To examine whether these Clavibacter species can induce HR-like cell death in N. tabacum, 108 CFU/ml of C. capsici PF008T and C. michiganensis LMG7333T were infiltrated. Results showed that both induced HR-like cell death within 18 hai (Figure 7A), and ion conductivity began to increase from 9 hai until 15 hai (Figure 7B). These results indicate that both Clavibacter species can induce HR-like cell death in N. tabacum, presenting another clue that N. tabacum is a non-host plant of these bacteria.
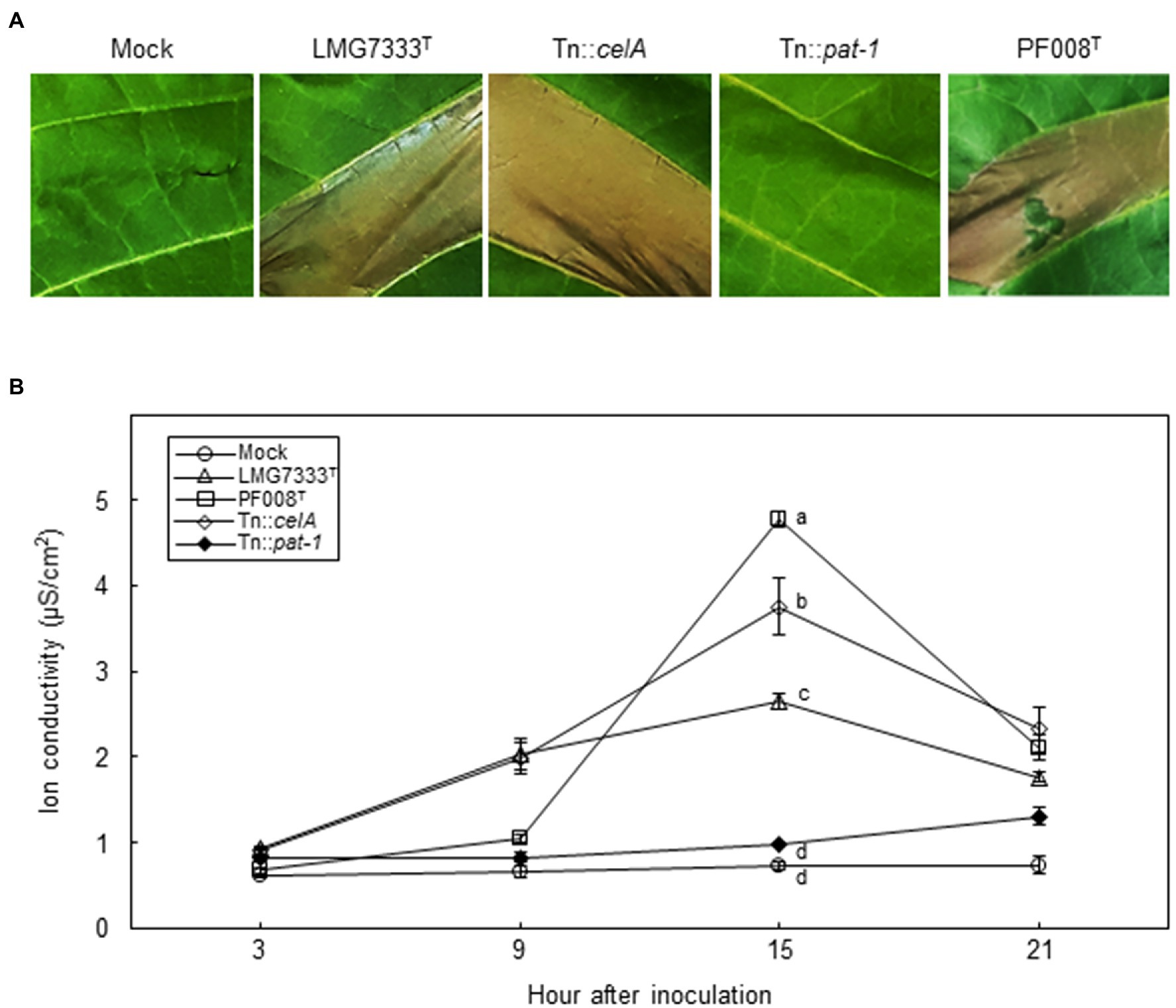
Figure 7. Influence of two major virulence genes of C. michiganensis for HR-like cell death on N. tabacum leaves. (A) The HR-like cell death on N. tabacum leaves by infiltration with 108 CFU/ml of C. capsici PF008T, C. michiganensis LMG7333T, and its mutant strains. Photos showing HR-like cell death were taken at 18 h after infiltration (hai), respectively. (B) The measurement of ion conductivity on N. tabacum leaves after inoculation with 108 CFU/ml of the bacterial strains. The letters at the time points in the graphs indicate a statistically significant difference analyzed via Duncan’s multiple range test (p < 0.05). Similar results were obtained from two independent assays. Mock, 10 mM MgCl2.
To determine whether celA and pat-1 genes are required for induction of HR-like cell death, mutant strains were infiltrated. The Tn::celA mutant induced HR-like cell death like the WT strain, whereas the Tn::pat-1 mutant failed (Figure 7A). The ion conductivity was consistent with these phenotypes (Figure 7B). These results indicate that the pat-1 gene, but not celA, is critical for HR induction in N. tabacum.
Discussion
In this study, we show that N. benthamiana displays blister-like lesions and rapid necrosis in leaves after infection with Gram-positive and plant-pathogenic bacteria C. michiganensis and C. capsici and wilting after infection with C. michiganensis (Table 1). On the basis of these results and as reported previously (Balaji et al., 2011), we propose that N. benthamiana is a surrogate host plant of Clavibacter pathogens, particularly, C. michiganensis. N. benthamiana as well as N. tabacum have been widely used as model plants for diverse research on plant–microbe interactions (Goodin et al., 2008). Unlike N. benthamiana, N. tabacum appears to be a non-host plant of Clavibacter pathogens because those pathogens induced HR-like cell death and grew much less in N. tabacum than in N. benthamiana.
The celA and pat-1 genes of C. michiganensis, encoding a cellulase and a putative serine protease as apoplastic effectors, are critical in the development of disease symptoms in tomatoes (Gartemann et al., 2003; Hwang et al., 2019). However, these genes were unnecessary for the formation of blister-like lesions and rapid necrosis in N. benthamiana leaves (Figures 3, 4) and were partially necessary for complete wilting (Figure 6; Supplementary Figure S4). Previously, it was shown that C. michiganensis strain Cmm100, which lacks plasmids pCM1 and pCM2, formed blisters in tomato leaves (Chalupowicz et al., 2017); celA and pat-1 genes are located in pCM1 and pCM2, respectively (Dreier et al., 1997; Jahr et al., 2000). Moreover, C. capsici does not have celA but carries the pat-1 ortholog. However, this pat-1 ortholog failed to complement the pathogenicity function of the C. michiganensis pat-1 gene in tomatoes, whereas another pat-1 ortholog, chp-7 of C. sepedonicus, could partially complement it (Hwang et al., 2022). Although both Pat-1 and Chp-7 could elicit HR in N. tabacum (Nissinen et al., 2009; Lu et al., 2015), chp-7 failed to complement HR-eliciting ability of the Tn::pat-1 mutant of C. michiganensis (Hwang et al., 2022), indicating that these orthologs might use different mechanisms for HR elicitation in N. tabacum. Nevertheless, C. michiganensis caused blister-like lesions and rapid necrosis in N. benthamiana leaves (Figures 1, 4). These results collectively imply that celA and pat-1 genes are not connected with the formation of blister-like lesions. These findings reveal that novel pathogenicity or virulence factors of C. michiganensis are minimally required for the formation of blister-like lesions and rapid necrosis in leaves, and more virulence factors are necessary for complete wilting. Notably, the chpC gene in the chromosomal PAI region of C. michiganensis and other genes, such as stbA, pgaA, and endX/Y, appears to contribute to the formation of blisters in tomato leaves (Chalupowicz et al., 2017). Revealing the novel pathogenicity or virulence factors of C. michiganensis for the formation of blister-like lesions and rapid necrosis in leaves will help us understand the virulence mechanisms of this bacterium in N. benthamiana. Based on previous literature (Jacques et al., 2012; Tancos et al., 2015; Thapa et al., 2017), variation in the virulence of C. michiganensis natural isolates exists in tomato. It will be worthwhile to examine whether there are natural isolates of C. michiganensis showing different virulence patterns in tomato and N. benthamiana.
C. michiganensis caused severe wilting in N. benthamiana, whereas C. capsici did not (Figure 5). C. capsici causes bacterial canker in pepper stems without wilting symptom (Hwang et al., 2018), consistent with no wilting development in N. benthamiana. Previously, we showed that the introduction of celA into C. capsici resulted in increased ability to cause wilting in tomatoes (Hwang et al., 2018). However, the wilting severity was much less than that caused by C. michiganensis, implying that more factors, which might be missing in C. capsici, are required for severe wilting. It will be useful to study N. benthamiana plants to reveal those factors using both Clavibacter species.
Although blisters were shown in tomato leaves after infection with C. michiganensis (Chalupowicz et al., 2017), its features have not been studied. We showed that blister-like lesions caused by two Clavibacter species in N. benthamiana leaves were closely associated with cell death and the generation of ROS (Figure 2; Supplementary Figure S1). The color of blisters in tomatoes and the blister-like lesions in N. benthamiana leaves appear pale green, indicating that chlorophyll might be degraded during blister formation, and infected cells may eventually die. ROS might be responsible for these processes. It will be worthwhile to determine how blister-like lesions are formed in N. benthamiana leaves, which will help us understand the formation of blisters in tomato leaves.
Taken together, our results suggest that N. benthamiana is a surrogate host of C. michiganensis and C. capsici and N. tabacum is a non-host plant of both Clavibacter species. Although disease phenotypes in N. benthamiana after inoculation with two Clavibacter species appear very similar to those in natural host plants, such as tomato and pepper, different virulence factors might be necessary. Therefore, N. benthamiana can be used to understand the novel molecular mechanisms of Clavibacter pathogens for virulence or pathogenicity in the future.
Data Availability Statement
The original contributions presented in the study are included in the article/Supplementary Material, and further inquiries can be directed to the corresponding author.
Author Contributions
IP, IH, E-JO, C-TK, and C-SO conducted experiments, analyzed the data, and wrote the manuscript. All authors contributed to the article and approved the submitted version.
Funding
This work was supported by the National Research Foundation of Korea (NRF) grant funded by the Korean government (MIST; 2019R1A2C2004568 and 2018R1A5A1023599).
Conflict of Interest
The authors declare that the research was conducted in the absence of any commercial or financial relationships that could be construed as a potential conflict of interest.
Publisher’s Note
All claims expressed in this article are solely those of the authors and do not necessarily represent those of their affiliated organizations, or those of the publisher, the editors and the reviewers. Any product that may be evaluated in this article, or claim that may be made by its manufacturer, is not guaranteed or endorsed by the publisher.
Supplementary Material
The Supplementary Material for this article can be found online at: https://www.frontiersin.org/articles/10.3389/fpls.2022.876971/full#supplementary-material
Supplementary Figure S1 | 3,3′-Diaminobenzidine (DAB) straining of N. benthamiana leaves showing blister-like symptoms, after infiltration with 5×104 CFU/ml bacterial suspensions of C. michiganensis LMG7333T and C. capsici PF008T.
Supplementary Figure S2 | Canker development on N. benthamiana stems via the stem inoculation with 108 CFU/ml bacterial suspensions of C. michiganensis LMG7333T, C. capsici PF008T, and C. michiganensis LMG7333 Tn::celA and Tn::pat-1 mutant strains.
Supplementary Figure S3 | No symptoms after spray inoculation with C. michiganensis LMG7333T and C. capsici PF008T in N. benthamiana.
Supplementary Figure S4 | Influence of two major virulence genes of C. michiganensis in N. benthamiana plants for the development of wilting symptoms.
References
Balaji, V., Sessa, G., and Smart, C. D. (2011). Silencing of host basal defense response-related gene expression increases susceptibility of Nicotiana benthamiana to Clavibacter michiganensis subsp. michiganensis. Phytopathology 101, 349–357. doi: 10.1094/PHYTO-05-10-0132
Balint-Kurti, P. (2019). The plant hypersensitive response: concepts, control and consequences. Mol. Plant Pathol. 20, 1163–1178. doi: 10.1111/mpp.12821
Bombarely, A., Rosli, H. G., Vrebalov, J., Moffett, P., Mueller, L. A., and Martin, G. B. (2012). A draft genome sequence of Nicotiana benthamiana to enhance molecular plant-microbe biology research. Mol. Plant-Microbe Interact. 25, 1523–1530. doi: 10.1094/MPMI-06-12-0148-TA
Bradbury, J.F. (1986). Guide to Plant Pathogenic Bacteria. Wallingford, UK: CAB International Mycological Institute.
Carlton, W. M., Braun, E. J., and Gleason, M. L. (1998). Ingress of Clavibacter michiganensis subsp. michiganensis into tomato leaves through hydathodes. Phytopathology 88, 525–529. doi: 10.1094/PHYTO.1998.88.6.525
Chalupowicz, L., Barash, I., Reuven, M., Dror, O., Sharabani, G., Gartemann, K. H., et al. (2017). Differential contribution of Clavibacter michiganensis ssp. michiganensis virulence factors to systemic and local infection in tomato. Mol. Plant Pathol. 18, 336–346. doi: 10.1111/mpp.12400
Chalupowicz, L., Zellermann, E.-M., Fluegel, M., Dror, O., Eichenlaub, R., Gartemann, K.-H., et al. (2012). Colonization and movement of GFP-labeled Clavibacter michiganensis subsp. michiganensis during tomato infection. Phytopathology 102, 23–31. doi: 10.1094/PHYTO-05-11-0135
Choi, D. S., Hwang, I. S., and Hwang, B. K. (2012). Requirement of the cytosolic interaction between PATHOGENESIS-RELATED PROTEIN10 and LEUCINE-RICH REPEAT PROTEIN1 for cell death and defense signaling in pepper. Plant Cell 24, 1675–1690. doi: 10.1105/tpc.112.095869
Davarpanah, S. J., Jung, S. H., Kim, Y. J., Park, Y.-I., Min, S. R., Liu, J. R., et al. (2009). Stable plastid transformation in Nicotiana benthamiana. J. Plant Biol. 52, 244–250. doi: 10.1007/s12374-009-9023-0
de León, L., Siverio, F., López, M. M., and Rodríguez, A. (2011). Clavibacter michiganesis subsp. michiganensis, a seedborne tomato pathogen: healthy seeds are still the goal. Plant Dis. 95, 1328–1338. doi: 10.1094/PDIS-02-11-0091
Dreier, J., Meletzus, D., and Eichenlaub, R. (1997). Characterization of the plasmid encoded virulence region pat-1 of phytopathogenic Clavibacter michiganensis subsp. michiganensis. Mol. Plant-Microbe Interact. 10, 195–206. doi: 10.1094/MPMI.1997.10.2.195
Eichenlaub, R., and Gartemann, K.-H. (2011). The Clavibacter michiganensis subspecies: molecular investigation of gram-positive bacterial plant pathogens. Annu. Rev. Phytopathol. 49, 445–464. doi: 10.1146/annurev-phyto-072910-095258
Eichenlaub, R., Gartemann, K.-H., and Burger, A. (2007). “Clavibacter michiganensis, a group of Gram-positive phytopathogenic bacteria,” in Plant-Associated Bacteria. ed. S. Gnanamanickam (Dordrecht: Springer), 385–421.
Gartemann, K.-H., Abt, B., Bekel, T., Burger, A., Engemann, J., Flügel, M., et al. (2008). The genome sequence of the tomato-pathogenic actinomycete Clavibacter michiganensis subsp. michiganensis NCPPB382 reveals a large island involved in pathogenicity. J. Bacteriol. 190, 2138–2149. doi: 10.1128/JB.01595-07
Gartemann, K.-H., Kirchner, O., Engemann, J., Gräfen, I., Eichenlaub, R., and Burger, A. (2003). Clavibacter michiganensis subsp. michiganensis: first steps in the understanding of virulence of a gram-positive phytopathogenic bacterium. J. Biotechnol. 106, 179–191. doi: 10.1016/j.jbiotec.2003.07.011
Goodin, M. M., Zaitlin, D., Naidu, R. A., and Lommel, S. A. (2008). Nicotiana benthamiana: its history and future as a model for plant–pathogen interactions. Mol. Plant-Microbe Interact. 21, 1015–1026. doi: 10.1094/MPMI-21-8-1015
Guo, W.-L., Chen, B.-H., Guo, Y.-Y., Yang, H.-L., Mu, J.-Y., Wang, Y.-L., et al. (2019). Improved powdery mildew resistance of transgenic Nicotiana benthamiana overexpressing the Cucurbita moschata CmSGT1 gene. Front. Plant Sci. 10:955. doi: 10.3389/fpls.2019.00955
Hwang, I. S., Lee, H. M., Oh, E. J., Lee, S., Heu, S., and Oh, C. S. (2020). Plasmid composition and the chpG gene determine the virulence level of Clavibacter capsici natural isolates in pepper. Mol. Plant Pathol. 21, 808–819. doi: 10.1111/mpp.12932
Hwang, I. S., Oh, E.-J., Lee, H. B., and Oh, C.-S. (2019). Functional characterization of two cellulase genes in the gram-positive pathogenic bacterium Clavibacter michiganensis for wilting in tomato. Mol. Plant-Microbe Interact. 32, 491–501. doi: 10.1094/MPMI-08-18-0227-R
Hwang, I. S., Oh, E.-J., Song, E., Park, I. W., Lee, Y. Y., Sohn, K. H., et al. (2022). An apoplastic effector Pat-1Cm of the gram-positive bacterium Clavibacter michiganensis acts as both a pathogenicity factor and an immunity elicitor in plants. Front. Plant Sci. 13:888290. doi: 10.3389/fpls.2022.888290
Hwang, I. S., Oh, E. J., Kim, D., and Oh, C. S. (2018). Multiple plasmid-borne virulence genes of Clavibacter michiganensis ssp. capsici critical for disease development in pepper. New Phytol. 217, 1177–1189. doi: 10.1111/nph.14896
Ignatov, A. N., Spechenkova, N. A., Taliansky, M., and Kornev, K. P. (2019). First report of Clavibacter michiganensis subsp. michiganensis infecting potato in Russia. Plant Dis. 103:147. doi: 10.1094/PDIS-04-18-0691-PDN
Jacques, M.-A., Durand, K., Orgeur, G., Balidas, S., Fricot, C., Bonneau, S., et al. (2012). Phylogenetic analysis and polyphasic characterization of Clavibacter michiganensis strains isolated from tomato seeds reveal that nonpathogenic strains are distinct from C. michiganensis subsp. michiganensis. Appl. Environ. Microbiol. 78, 8388–8402. doi: 10.1128/AEM.02158-12
Jahr, H., Dreier, J., Meletzus, D., Bahro, R., and Eichenlaub, R. (2000). The endo-β-1, 4-glucanase CelA of Clavibacter michiganensis subsp. michiganensis is a pathogenicity determinant required for induction of bacterial wilt of tomato. Mol. Plant-Microbe Interact. 13, 703–714. doi: 10.1094/MPMI.2000.13.7.703
Kaup, O., Gräfen, I., Zellermann, E.-M., Eichenlaub, R., and Gartemann, K.-H. (2005). Identification of a tomatinase in the tomato-pathogenic actinomycete Clavibacter michiganensis subsp. michiganensis NCPPB382. Mol. Plant-Microbe Interact. 18, 1090–1098. doi: 10.1094/MPMI-18-1090
Li, X., Tambong, J., Yuan, K. X., Chen, W., Xu, H., Lévesque, C. A., et al. (2018). Re-classification of Clavibacter michiganensis subspecies on the basis of whole-genome and multi-locus sequence analyses. Int. J. Syst. Evol. Microbiol. 68, 234–240. doi: 10.1099/ijsem.0.002492
Liu, Y., Schiff, M., Marathe, R., and Dinesh-Kumar, S. P. (2002). Tobacco Rar1, EDS1 and NPR1/NIM1 like genes are required for N-mediated resistance to tobacco mosaic virus. Plant J. 30, 415–429. doi: 10.1046/j.1365-313X.2002.01297.x
Lu, Y., Hatsugai, N., Katagiri, F., Ishimaru, C. A., and Glazebrook, J. (2015). Putative serine protease effectors of Clavibacter michiganensis induce a hypersensitive response in the apoplast of Nicotiana species. Mol. Plant-Microbe Interact. 28, 1216–1226. doi: 10.1094/MPMI-02-15-0036-R
Medina-Mora, C. M., Hausbeck, M. K., and Fulbright, D. W. (2001). Bird's eye lesions of tomato fruit produced by aerosol and direct application of Clavibacter michiganensis subsp. michiganensis. Plant Dis. 85, 88–91. doi: 10.1094/PDIS.2001.85.1.88
Nissinen, R., Xia, Y., Mattinen, L., Ishimaru, C. A., Knudson, D. L., Knudson, S. E., et al. (2009). The putative secreted serine protease Chp-7 is required for full virulence and induction of a nonhost hypersensitive response by Clavibacter michiganensis subsp. sepedonicus. Mol. Plant-Microbe Interact. 22, 809–819. doi: 10.1094/MPMI-22-7-0809
Oh, E.-J., Bae, C., Lee, H.-B., Hwang, I. S., Lee, H.-I., Yea, M. C., et al. (2016). Clavibacter michiganensis subsp. capsici subsp. nov., causing bacterial canker disease in pepper. Int. J. Syst. Evol. Microbiol. 66, 4065–4070. doi: 10.1099/ijsem.0.001311
Sen, Y., van der Wolf, J., Visser, R. G. F., and van Heusden, S. (2015). Bacterial canker of tomato: current knowledge of detection, management, resistance, and interactions. Plant Dis. 99, 4–13. doi: 10.1094/PDIS-05-14-0499-FE
Shi, W., Hao, L., Li, J., Liu, D., Guo, X., and Li, H. (2014). The Gossypium hirsutum WRKY gene GhWRKY39-1 promotes pathogen infection defense responses and mediates salt stress tolerance in transgenic Nicotiana benthamiana. Plant Cell Rep. 33, 483–498. doi: 10.1007/s00299-013-1548-5
Shinohara, R., Kanda, A., Ohnishi, K., Kiba, A., and Hikichi, Y. (2005). Contribution of folate biosynthesis to Ralstonia solanacearum proliferation in intercellular spaces. Appl. Environ. Microbiol. 71, 417–422. doi: 10.1128/AEM.71.1.417-422.2005
Song, Y.-R., Hwang, I. S., and Oh, C.-S. (2020). Natural variation in virulence of Acidovorax citrulli isolates that cause bacterial fruit blotch in watermelon, depending on infection routes. Plant Pathol. J. 36, 29–42. doi: 10.5423/PPJ.OA.10.2019.0254
Stork, I., Gartemann, K. H., Burger, A., and Eichenlaub, R. (2008). A family of serine proteases of Clavibacter michiganensis subsp. michiganensis: chpC plays a role in colonization of the host plant tomato. Mol. Plant Pathol. 9, 599–608. doi: 10.1111/j.1364-3703.2008.00484.x
Tancos, M. A., Chalupowicz, L., Barash, I., Manulis-Sasson, S., and Smart, C. D. (2013). Tomato fruit and seed colonization by Clavibacter michiganensis subsp. michiganensis through external and internal routes. Appl. Environ. Microbiol. 79, 6948–6957. doi: 10.1128/AEM.02495-13
Tancos, M. A., Lange, H. W., and Smart, C. D. (2015). Characterizing the genetic diversity of the Clavibacter michiganensis subsp. michiganensis population in New York. Phytopathology 105, 169–179. doi: 10.1094/PHYTO-06-14-0178-R
Thapa, S. P., Pattathil, S., Hahn, M. G., Jacques, M.-A., Gilbertson, R. L., and Coaker, G. (2017). Genomic analysis of Clavibacter michiganensis reveals insight into virulence strategies and genetic diversity of a gram-positive bacterial pathogen. Mol. Plant-Microbe Interact. 30, 786–802. doi: 10.1094/MPMI-06-17-0146-R
Thyr, B. D., Samuel, M. J., and Brown, P. G. (1975). New solanaceous host records for Corynebacterium michiganense. Plant Dis. Rep. 59, 595–598.
Tran, P.-T., Choi, H., Choi, D., and Kim, K.-H. (2016). Virus-induced gene silencing reveals signal transduction components required for the Pvr9-mediated hypersensitive response in Nicotiana benthamiana. Virology 495, 167–172. doi: 10.1016/j.virol.2016.05.011
Traore, S. M., Eckshtain-Levi, N., Miao, J., Castro Sparks, A., Wang, Z., Wang, K., et al. (2019). Nicotiana species as surrogate host for studying the pathogenicity of Acidovorax citrulli, the causal agent of bacterial fruit blotch of cucurbits. Mol. Plant Pathol. 20, 800–814. doi: 10.1111/mpp.12792
Xin, X.-F., and He, S. Y. (2013). Pseudomonas syringae pv. Tomato DC3000: a model pathogen for probing disease susceptibility and hormone signaling in plants. Annu. Rev. Phytopathol. 51, 473–498. doi: 10.1146/annurev-phyto-082712-102321
Keywords: Clavibactermichiganensis, Nicotiana benthamiana, surrogate host, virulence factors, virulence mechanisms
Citation: Park IW, Hwang IS, Oh E-J, Kwon C-T and Oh C-S (2022) Nicotianabenthamiana, a Surrogate Host to Study Novel Virulence Mechanisms of Gram-Positive Bacteria, Clavibacter michiganensis, and C. capsici in Plants. Front. Plant Sci. 13:876971. doi: 10.3389/fpls.2022.876971
Edited by:
Sang-Wook Han, Chung-Ang University, South KoreaReviewed by:
Doron Teper, Agricultural Research Organization (ARO), IsraelHo Won Jung, Dong-A University, South Korea
Copyright © 2022 Park, Hwang, Oh, Kwon and Oh. This is an open-access article distributed under the terms of the Creative Commons Attribution License (CC BY). The use, distribution or reproduction in other forums is permitted, provided the original author(s) and the copyright owner(s) are credited and that the original publication in this journal is cited, in accordance with accepted academic practice. No use, distribution or reproduction is permitted which does not comply with these terms.
*Correspondence: Chang-Sik Oh, co35@khu.ac.kr