- 1Division of Agricultural Chemicals, ICAR-Indian Agricultural Research Institute, New Delhi, India
- 2Division of Entomology, ICAR-Indian Agricultural Research Institute, New Delhi, India
The present study aimed to develop nanoemulsions (NEs) of essential oil (EO) and lipid-soluble extract (HE) of Pogostemon cablin leaves using biosurfactant, saponin. Hydro-distilled EO and fat-soluble HE were analyzed using GC-MS, which revealed 38.7 ± 2.7% and 37.5 ± 2.1% patchoulol, respectively. EO and HE were formulated with saponin to prepare corresponding coarse emulsions (CEs); furthermore, high-speed homogenization for 2 min was followed by ultrasonication for 3 min with constant frequency of 50 kHz. of the CEs resulted in respective NEs. NEs were characterized for the physico-chemical properties such as emulsion intrinsic stability, particle size distribution, polydispersity index (PDI), and transmission electron microscopy (TEM) for morphology and accurate nanodroplet diameters. CEs and NEs were investigated for insecticidal efficacy against adults of Tetranychus urticae and larvae of Spodoptera litura. Stable NEs of EO and HE at 500 μg mL−1 concentration exhibited corresponding average particle size of 51.7 and 89.9 nm, while TEM image revealed spherical-shaped droplets with the average droplet diameters of 15.3 and 29.4 nm, respectively. NEs of EO and HE displayed highest efficacy in contact toxicity (LC50 43.2 and 58.4 μg mL−1) after 48 h and fumigant toxicity (LC50 9.3 and 13.6 μg mL−1) after 24 h against T. urticae. In addition, NEs of EO showed considerable antifeedant and feeding deterrent action (AI 99.21 ± 0.74 and FI 99.73 ± 1.24) against S. litura larvae.
Introduction
The present concept of green crop protection tools emphasized the exploitation of bioactive volatile and non-volatile phyto-constituents, which serve as potential sources of new molecules with a complex mechanism of action (Pavela and Benelli, 2016). In spite of huge versatility of the natural compounds, common constraints exist for their delivery systems, which are related to limited aqueous solubility and stability. Nanotechnological interventions are represented as one of the promising solutions of the problem (Nenaah et al., 2015; Campolo et al., 2017). Further increasing interests have been focused in recent studies regarding the development of NEs for encapsulation of volatile bioactive compounds/EOs for their promising application in agriculture (Khot et al., 2012). EOs have been recognized as eco-benign promising crop protection tool (Kundu et al., 2021); thus, nano-formulations of EOs are being designed, developed, and evaluated to manage many economically important pests (Heydari et al., 2020).
NEs provide a structural framework where bioactive ingredients are dispersed in the aqueous medium and stabilized with the help of surfactant particles. The stable oil-in-water NEs usually comprised of lipophilic active ingredient(s), surfactant, and water (Noori et al., 2018). It appears mostly transparent or slightly translucent in texture with the particle diameter within 100 nm (Balasubramani et al., 2017), since Sugumar et al. (2014) considered NEs having droplet size ranging between 20 and 200 nm. High-pressure homogenization has been used to prepare emulsions with reduced diameter; however, the process could only be sustained on high consumption of energy. Micro-fluidization is another energy-intensive technique, which shears the emulsion droplet size through molecular collision under microfluidic compartment (McClements, 2004). Related studies on the preparation of NEs using various techniques have indicated ultrasonic energy as competitive or even relatively superior employing rotor–stator dispersing to achieve uniform nanodroplets (Kentish et al., 2008).
Nano-sizing of lipophilic components including EOs helps to form kinetically stable emulsion with improved dispersibility in aqueous medium and higher degree of aqueous diffusion (Hashem et al., 2018). Furthermore, bioavailability improved as NEs with the increased surface area dimensions could easily penetrate the cell wall and reach the specific target binding sites; therefore, nano-emulsification reduced the application rate of active ingredients (Acevedo-Fani et al., 2015). However, comprehensive investigations are truly imperative to ascertain proper encapsulation and stability of nanodroplets. Thus, emulsifiers play a crucial role, and the critical micelle concentrations (CMC) of emulsifiers usually determine the kinetic stability of the emulsion (Kumar et al., 2019a). Naturally occurring green emulsifiers such as biosurfactants, amphiphilic proteins, and polysaccharides have been exploited to prepare nanoemulsions. Biosurfactants like saponins are preferred as these are required in small quantities to develop stable nanodroplets of lipophilic compounds (McClements and Gumus, 2016). Besides, steroidal saponins have been reported to possess insecticidal properties (Dolma et al., 2021).
Pogostemon species are perennial herbaceous plants, which belong to Lamiaceae family, are native to Philippines, and are widely distributed across warm and humid tropical climate of South Asian countries including India (Kusuma et al., 2018). Volatile EO of P. cablin is primarily constituted with sesquiterpenes, namely, patchoulene and patchouli alcohol (Sundaresan et al., 2012). Investigations on biological properties of the Pogostemon EO and phytochemicals revealed multidimensional pharmacological functions against a panel of targets (Hu et al., 2018; Roshan et al., 2022). Significant antifeedant activity of the EO has also been recorded against cosmopolitan pests (Huang et al., 2014). Furthermore, the oil was reported to exhibit LD50 0.2 μg/adult against Tribolium castaneum (Feng et al., 2019) and LD50 8.0 μg/insect against Choristoneura rosaceana (Machial et al., 2010). Based on the assumptions of higher efficacy of EO and extracts of Pogostemon, patchouli alcohol appeared as the key component responsible for broad-spectrum activities (Lima et al., 2013).
Hence, the hypothesis has been built with the proof of concept to utilize the EO and lipid-soluble fractions of P. cablin for the preparation of NEs with improved efficacy against acarid and insect. With these backgrounds, the present research was designed to profile volatile chemical constituents of EO and lipid-soluble fractions of P. cablin for the development of NE-based delivery system in an attempt to achieve potential bio-insecticide.
Materials and Methods
Plant Materials
Fresh leaves of P. cablin (5.0 kg) were collected from farmer's field, Hirisave village (12.9172° N and 76.4563° E) near Hassan district of Karnataka, India, during the month of April 2019. The voucher specimen (PC-2019-KHV-01) was authenticated from ICAR-National Bureau of Plant Genetic Resources, New Delhi, India. Fresh leaves were cleaned, gently washed with water, and used for isolation of EO. Shade dried leaves were powdered and used for extraction.
Distillation of EO
Fresh leaves of P. cablin (1.0 kg) were hydro-distilled in a Clevenger's apparatus (Borosil Glass Works Ltd., Mumbai, India) for continuous 12 h according to the method reported by Kundu et al. (2016). Pale yellowish-colored EO was collected from the apparatus. Furthermore, EO was partitioned with diethyl ether (3 × 50 mL) followed by passing through anhydrous sodium sulfate (20 g) using a glass funnel and stored. The yield of EO (%) was calculated as 1.43% (v/w).
Extraction
Coarsely powdered leaves (1.0 kg) of P. cablin were submerged with 2.5-L hexane (Merck® India Ltd, Mumbai, India) and sonicated for 2 h at 35°C using bath sonicator (PCI Analytics Ltd, Mumbai, India) following the method reported by Dutta et al. (2021). The extraction was repeated thrice with the same sample followed by filtration and concentrated to dryness under reduced pressure in a rotary evaporator (Heidolph, Germany) below 40°C to afford the crude HE (109.7 g).
GC-MS Analysis
Pogostemon EO and HE were analyzed in a 5590C GC-MS (Agilent Technologies®, USA) using a stationary phase column (30 m × 0.25 μm, 0.25 μm, Agilent Technologies®, USA) which was equipped to a mass spectrometer. Samples (1 μL, each) were injected through auto-injector under split-less mode. Helium was used as carrier gas with the flow rate of 1 mL min−1 and pressure of 10 psi. Then, oven condition was programed where temperature started at 30°C held for 1 min., then increased at the rate of 3°C min−1 to reach 60°C, and then held for 5 min. Hereafter, temperature was increased with the rate of 2°C min−1 to reach 150°C and with the hold time of 5 min. Next, temperature was again raised at the rate of 5°C min−1 to reach 220°C with the hold time of 5 min. At last, temperature increased to 280°C at the rate of 10°C min−1. Both the samples were analyzed with the runtime of 90 min. The MS acquisition parameters were programed with the ion source temperature of 170°C, electron ionization of 70 eV, transfer line temperature of 280°C, solvent delay of 3 min., and E.M. voltage of 1,419 V. The ionization energy (70 eV) was fixed with scanning rate of 1 s with the mass range of 50–550 amu. Volatile aromatic constituents were identified by matching their mass spectra, fragmentation pattern, reference standard, and retention index (literature and experimental) using Adams (2007), NIST, and WILEY libraries (Kumar et al., 2021).
Critical Micelle Concentration
Saponin (C36H54O11, sapogenin content 20–35%) sourced from Quillaja sp. (Merck® India Ltd. (Mumbai, India) was used as biosurfactant. CMC of saponin was determined from electrical conductance (EC) of different concentrations. For that, 100 to 12,000 μg mL−1 of saponin was prepared in aqueous medium and EC values were determined by a probe-type waterproof EC meter (HI 98304, Hanna, New Delhi, India). At first, the EC meter probe was calibrated with the ready-made KCl solution of known strength. Then, the prepared saponin aqueous solutions were measured by stirring and maintaining temperature equilibrium at 25 ± 1°C. The probe was washed thoroughly with de-ionized water after each measurement, starting from lower to higher concentrations of saponin.
Nanoemulsions (NEs) Preparation
At first, primary coarse emulsions (CEs) of EO and HE of P. cablin were prepared separately with the double concentration of pre-determined CMC values of saponin (0.5%). To prepare the CEs, EO (0.5g) and HE (0.5g) were separately mixed with the surfactant, and saponin (0.25 g) with minimum amount of deionized water, and finally, the volume was made up to 50 mL and vortexed for 5 min to obtain CEs (1%) of EO and HE. The freshly prepared CEs were thoroughly dispersed individually in high-speed homogenizer (IKA Ultra-Turrax T25, India) for 2 min. Thereafter, emulsion dilution technique was used to prepare nanoemulsions (NEs) from the CEs (Ghosh et al., 2013). Both the CEs (1%) were diluted serially with 0.5% aqueous solution of saponin to prepare secondary emulsions of lower concentrations (31.25–500 μg mL−1) of EO and HE. The diluted secondary emulsions were then subjected to ultrasonication using a probe ultrasonicator (MISONIX, Ultrasonic Liquid Processors, USA) for 3 min. at the amplitude of 50 kHz. to obtain NEs.
Stability of NEs
NEs of EO and HE were subjected to centrifugation at 10,000 rpm for 20 min. for checking any phase separation. Furthermore, the stability of the prepared emulsions was observed at room temperature at different time intervals and at accelerated storage condition (54 ± 1°C) for 14 days.
Particle Size of NEs
Average particle diameter, distribution, zeta potential, and polydispersity index (PDI) of the NEs and CEs of EO and HE were determined using a Zetasizer (Microtrac, Germany) following the principles of dynamic light scattering. Samples were measured with the help of a probe attached with the instrument with a laser light source. Microtrac FLEX data analysis program was used for the measurement of average droplet size. All the measurements were replicated thrice for each concentration.
Transmission Electron Microscopy
NEs were visualized using TEM (JEM 1011, JEOL, Japan) operated at an acceleration voltage of 80 kV to determine the morphology and droplet size at all the concentrations. The Cu-coated grid (200 mesh) of TEM was impregnated with each concentration of NE and kept for 15 min. for partial drying. The grids were further stained with 2% uranyl acetate and allowed to dry again for 3 h, and micrographs were acquired at the magnification of 80,000x at 100 nm under TEM.
Acaricidal Assays
Culturing of T. urticae
Adults of Tetranychus urticae were collected from tomato ecosystem which had not been exposed to any acaricide before. The acarids were reared on surface of the mulberry (Morus alba) leaves and kept on wet sponge in the laboratory at 27 ± 1°C, 65 ± 5% RH, and 13:11 h under L:D photoperiod till three generations before conducting the bioassay to obtain pure culture. Mites took 8–10 days during summer and 10–16 days during winter to complete one generation.
Leaf Dip Assay
CEs and NEs were tested against adults of T. urticae following leaf dip method. Test samples at the strength of 31.25–500 μg mL−1 were used for acaricidal assay. Mulberry (Morus alba) leaves were cleaned and treated separately with different concentration of the samples. Treated leaves were allowed to dry for 2 h, and 25 adults of T. urticae were transferred to the treated leaves. Saponin solution was used as negative control. All the Petri plates were incubated at ambient laboratory conditions under insect culture chamber maintaining 27 ± 1°C. Observations were taken after 24- and 48-h exposure. Mortality (%) was calculated, and probit analysis and LC50 values (μg mL−1) were determined using statistical software.
Fumigation Assay
CEs and NEs were tested for fumigant toxicity against adults of T. urticae. Using a stereomicroscope, 25 adults were transferred on the cleaned mulberry leaf holding on the dorsal part of the hysterosoma using a handling brush. The leaves were kept inside the glass jars. Each treatment (2.0 mL) of each sample was socked in cotton balls and hung with the help of lid inside the jar. Test concentrations of each sample were kept at 100–5.0 μg mL−1. Each treatment was replicated five times along with negative control, and the dead adults were counted after 24 h. The treated adults were considered dead if appendages did not respond even after touching with the brush. Mortality (%) was recorded, and further, LC50 values (μg mL−1) were determined.
Insecticidal Activity Against S. litura
Insect Culture
Eggs of S. litura were collected from tomato plants and incubated under laboratory conditions with high RH of 80 ± 5%. When eggs turned into dark color, matured (3–4 days), and were inoculated for hatching, freshly hatched larvae were fed on castor (Ricinus communis) leaf bouquets till larvae entered into pupation. Then, sex was identified, separated in different jars for emergence, and released for oviposition in jar, which consists of 15–20% honey solution dipped in a cotton wad for food and zig-zag folded paper strip for egg laying. Field collected cultures were reared in laboratory for 2–3 generations to obtain pure culture and used for testing.
Larvicidal Assay
Larvicidal activity of the CEs and NEs based on EO and HE was studied using potter tower spray at five different test concentrations (500–31.25 μg mL−1). Fresh castor leaf disk was kept in each Petri dish, and five third instar larvae of S. litura were released. Each test concentration (1.0 mL) was sprayed on the third instar larvae of S. litura kept in Petri dish. Each treatment was replicated thrice. Petri dishes were kept under laboratory ambient conditions, and observations were taken after 24 and 48 h. Mortality (%) was calculated, and lethal concentration in terms of LC50 values (μg mL−1) was determined.
Antifeedant Assay
CEs and NEs were evaluated using non-choice and choice leaf disk method (Sengonca et al., 2006). Briefly, fresh castor leaves were collected from field and cleaned thoroughly. Leaves were cut evenly maintaining disk desired size (3 × 3 cm2), were dipped in various test concentrations (500–31.25 μg mL−1) separately, and were allowed to air dry at room temperature for 3 h. Additionally, leaf disks dipped in saponin solution were used as negative control. In each Petri dish, one layer of wet filter paper was placed to avoid drying of the leaf disks, if any. One third instar larva was introduced into each treated plate and placed in an incubator at 27 ± 1°C with 65 ± 5% RH and a 14:10 (L: D) photoperiod. Each treatment was replicated eight times. Observation of the larvae was taken after 24-h exposure to determine the effect of on their feeding behavior. Larvae were found to be sterile and could not feed the leaves; however, most of the treated plates, close to complete consumption of leaves, were observed in control. Feeding of treated and control leaves was measured after 24 h. using a Leaf Area Meter (ADC Bioscientific Ltd., India), and the antifeedant index (AI) was determined by the following equation AI% = [(1 – T/C) × 100], where T is the average area of treated leaf consumed and C is the average leaf area consumed without treatment. The Feeding Index (FI) was calculated as [(C – T)/(C + T)] × 100.
Statistical Analysis
Data were measured using the Statistical Package for the Social Sciences (SPSS, Version 14.0, IBM, NY, USA). The results were expressed as mean±standard deviation (SD), and differences between variables were tested using one-way ANOVA. Statistically significant level was determined at p-value < 0.05.
Results
Volatile Composition of EO and HE
Volatile constituents of EO of P. cablin leaves were identified in GC-MS which showed several peaks, corresponding to twenty-four mono- and sesquiterpenoids, representing 96.9% of the oil (Table 1). Sesquiterpene hydrocarbons (55.5%) were most abundant followed by oxygenated sesquiterpenes (40.6%). Patchouli alcohol (38.7 ± 2.7%) was found as the major oxygenated sesquiterpene followed by α-bulnesene (18.1 ± 1.2%) and α-guaiene (17.7 ± 1.2%). Other major sesquiterpenes of the oil were trans-β-caryophyllene (3.7 ± 0.5%), β-patchoulene (3.6 ± 0.5%), β-elemene (3.3 ± 0.4%), β-guaiene (3.2%), α-patchoulene (2.6 ± 0.3%), and α-salinene (2.5 ± 0.2%). Only three monoterpene hydrocarbons such as α-pinene (0.2 ± 0.0%), β-pinene (0.3 ± 0.1%), and dl-limonene (0.2 ± 0.0%) were identified accounting only 0.7% of the EO.
Mass spectrum of patchouli alcohol showed molecular ion [M]+ peak at m/z 222, which was further broken to give daughter ion peaks at m/z 207, 179, and 161 after sequentially losing methyl and hydroxyl moieties. Other peaks at m/z 138, 125, 98, 81, and 69 were also originated due to subsequent cleavage of hydrocarbons (Figure 1). Similarly, α-bulnesene was characterized from its characteristic [M]+ peak at m/z 204 and further fragmented to daughter ion peaks at m/z 189, 175, 161, 147, 135, 121, 107, 93, and 79 with removal of methylene and methyl groups (Figure 1).
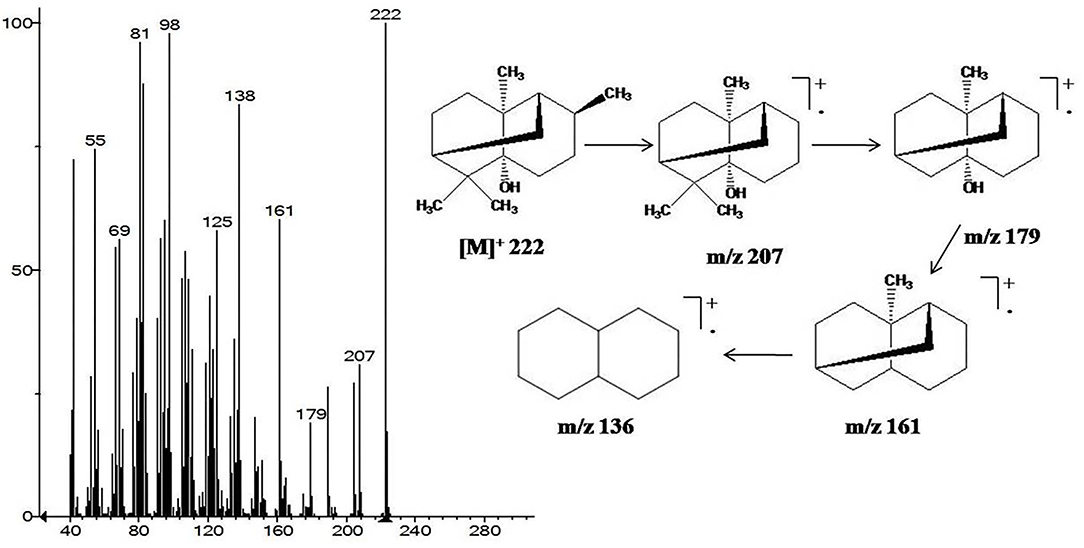
Figure 1. GC-MS fragmentation pattern of the most abundant patchouli alcohol characterized in EO and HE of P. cablin.
Nineteen aromatic compositions, representing 93.5% of the non-polar fat-soluble HE, have been identified in GC-MS and mentioned as per their elution in HP-5MS stationary phase (Table 1). However, total sesquiterpene hydrocarbon content of hexane soluble fraction was 34.1%, while oxygenated sesquiterpene was 38.7%. Most abundant patchouli alcohol (37.5 ± 2.1%) was identified as the sole oxygenated sesquiterpene. Besides, long-chain fatty acids and long-chain hydrocarbons were found in the HE, representing 10.9 and 5.0%, respectively. Among fatty acids, octadecenoic acid (6.4 ± 0.5%) was identified as the major compound followed by octadecanoic acid (2.4 ± 0.3%) and hexadecanoic acid (2.1 ± 0.2%). Similarly, long-chain hydrocarbons, mainly docosane (2.3 ± 0.3%), tetracosane (2.1 ± 0.3%), squalene (1.3 ± 0.2%), triacontane (0.9 ± 0.2%), and non-acosane (0.8 ± 0.2%), were identified.
Characterizations and Stability of NEs
Based on the recorded electrical conductance of various concentrations of saponin, sharp change was observed at 0.25% (Figure 2); thus, double concentration, 0.5%, has been selected for the final preparation of CEs and NEs. Primary CEs with the 1% strength of EO (w/w) and HE were prepared separately and diluted to get various test concentrations (500–31.25 μg mL−1). Subsequently, ultrasonication-assisted nano-emulsification of CEs of EO and HE in aqueous medium resulted in the preparation of respective NEs (Figure 3). Formation of cavitations in the liquid due to ultrasonic wave helped to utilize the energy for shearing of coarse droplets in nano size range which was further stabilized by the surfactant particles. The prepared NEs (31.25 to 125 μg mL−1) were found transparent; however, slight turbidity was recorded at 250–500 μg mL−1 concentrations. Here, non-toxic biosurfactant, saponin, was effectively used to stabilize the developed NEs at 0.5% concentration.
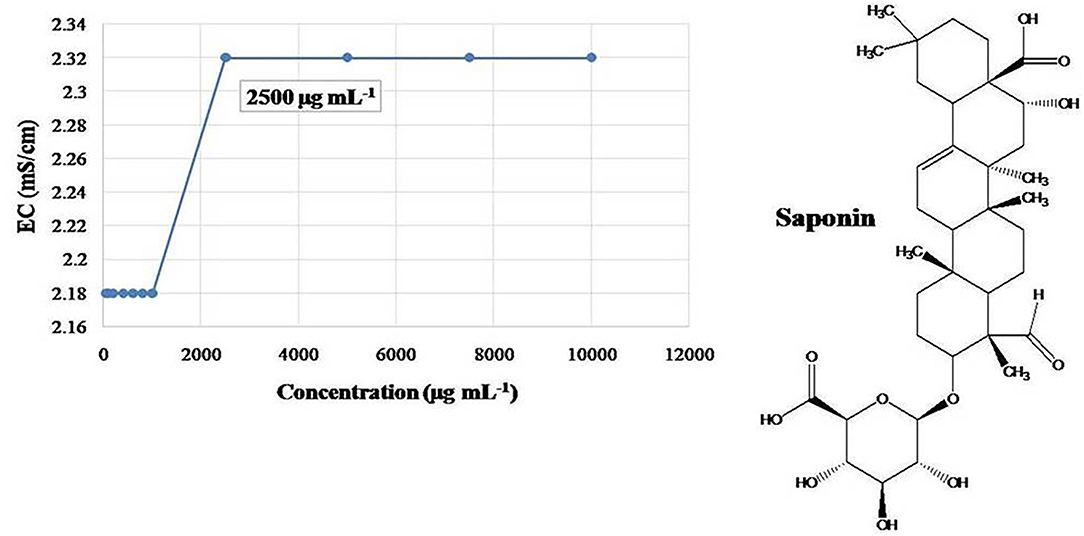
Figure 2. Critical micelle concentration (CMC) of biosurfactant, saponin as measured by electrical conductance (EC).
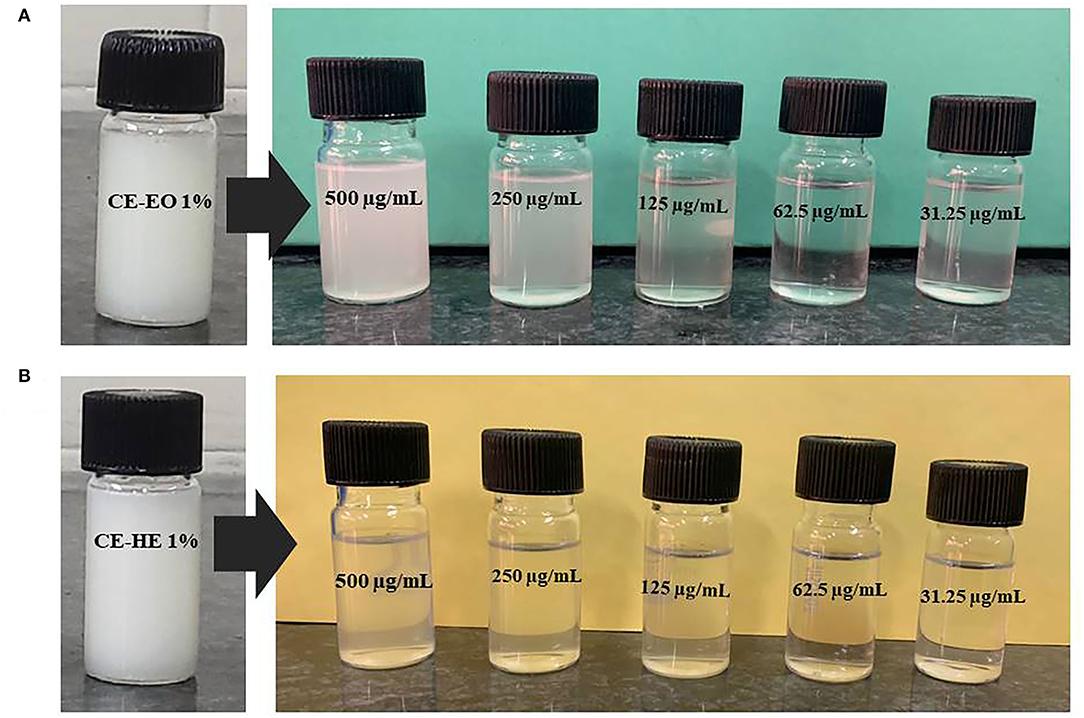
Figure 3. Visual appearance of CEs and different concentrations of NEs of (A) EO and (B) HE of P. cablin.
The properties of serially diluted NEs of EO and HE are shown in Table 2. The average droplet diameter at 500 μg mL−1 concentration of NE of EO as prepared by homogenization followed by ultrasonication was 71.68 ± 0.84 nm with the polydispersity index (PDI) of 0.49 ± 0.02, signifying narrow dimension of particle size distribution (Figure 4A). Likewise, average droplet diameter at the same concentration of NE-HE was 89.87 nm (Figure 4C) with corresponding PDI 0.51 ± 0.03. There was a clear indication that with decrease in concentration of EO and HE in the NEs, mean droplet size decreased. However, no significant relationship was observed between concentration and PDI as a narrow spectrum was maintained for PDI both in case of NEs of EO (0.49–0.69) and HE (0.51–0.67), suggesting uniform size distribution of the droplets irrespective of change in concentration. As 0.5% saponin concentration was maintained throughout the study for all the samples, there was always less chance of much variation in size distribution as the micelles remained same. However, with increased loading of EO and HE, the micelles got swelled by entrapment of EO and HE. Therefore, the mean droplet diameter was found to be more at higher loading concentrations. In the present study, zeta potential of the prepared NEs of EO and HE at 500 μg mL−1 was found to be −29.21 ± 0.49 mV and −29.06 ±0.21 mV (Figures 4B,D) at the native PH of 4.58 and 4.18, respectively. Thus, the absolute droplet charges were found very less. For all the samples, zeta potential was found to be higher than −20 mV, suggesting formation of stable NEs.
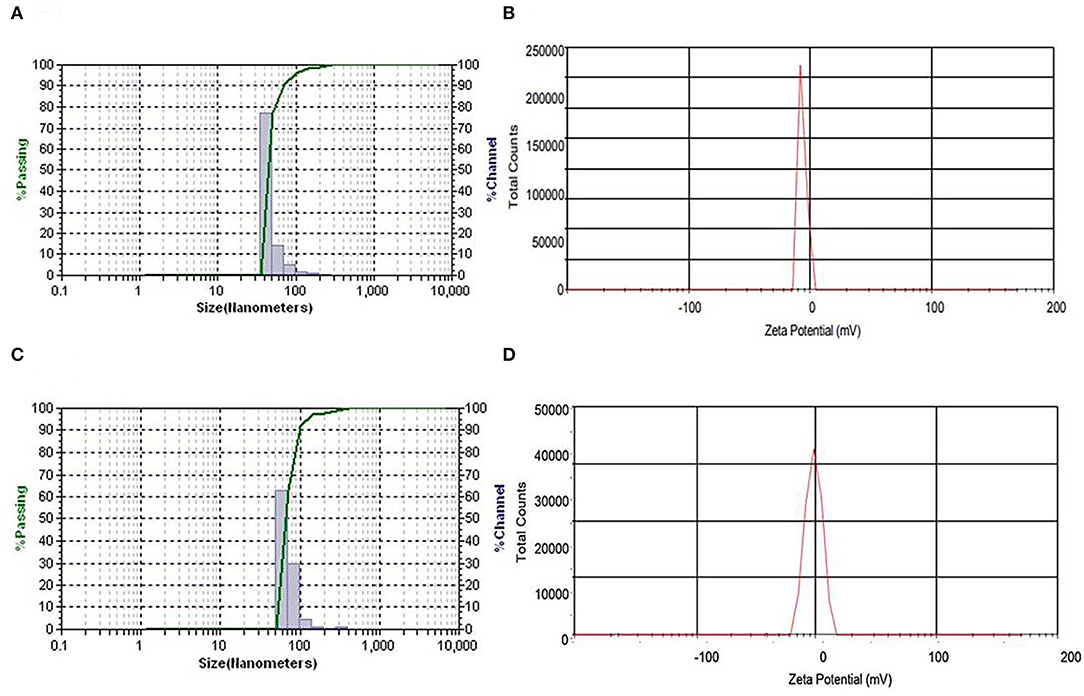
Figure 4. (A) Particle size distribution and (B) zeta potential of 500 μg mL−1 concentration of NEs of EO. (C) Particle size distribution and (D) zeta potential of 500 μg mL−1 concentration of NEs of HE.
Morphology and size of the droplets of NEs were visualized under TEM. Figure 5 displayed TEM images of the droplets of NEs of EO and HE. It was quite evident from the TEM images displaying spherical-shaped nanodroplets. The average diameter of the NE of EO droplets was 15.32 nm, which is relatively three times smaller than the average diameter determined obtained from particle size analyzer. Similarly, the average diameter of the NE of lipid-soluble HE droplets was 29.41 nm. However, droplet diameter varied within the range of 12.78 to 38.97 nm. The variation in droplet size could be attributed to the fact that TEM analyses of the droplets in the dry state gave accurate size based on the real morphology of the droplets, whereas average hydrodynamic diameter of the droplets was obtained from the particle size analyzer which was the average size of hydrated micelles. Furthermore, NEs were found to be stable with no phase separation even after 14 days of storage under accelerated storage condition at 54 ± 1°C and at room temperature. The average droplet diameters at 500 μg mL−1 concentrations of NEs of EO and HE after 14 days of accelerated storage were 91.22 ± 1.29 nm and 99.41 ± 0.72 nm, respectively. Furthermore, no aggregation of droplets was observed upon TEM analysis, indicating kinetic stability of the emulsions.
Acaricidal Action on T. urticae
Acaricidal activity of CEs and NEs of EO and HE of P. cablin against adults of T. urticae revealed significant mortality after 24 and 48 h of the treatment (Table 3). Both the CEs were effective after 48 h with the LC50 values <170 μg mL−1. Of T. urticae were found dead along the midrib of the treated leaves. CE-EO was comparatively more toxic than CE-HE. The magnitude of acaricidal efficacy was more after 48 h for the emulsions. Contact toxicity by leaf dip method with respect to time of application might contribute immensely to the actual acaricidal action. As hypotheses, NE-EO and NE-HE were very potent with higher effectiveness, LC50 values <100 μg mL−1. NE-EO exhibited highest efficacy with the LC50 89.7 μg mL−1 and 43.2 μg mL−1 after 24- and 48-h exposure, respectively. Likewise, NE-HE was relatively high toxic with their corresponding LC50 values of 97.2 μg mL−1 and 58.4 μg mL−1 after 24- and 48-h exposure.
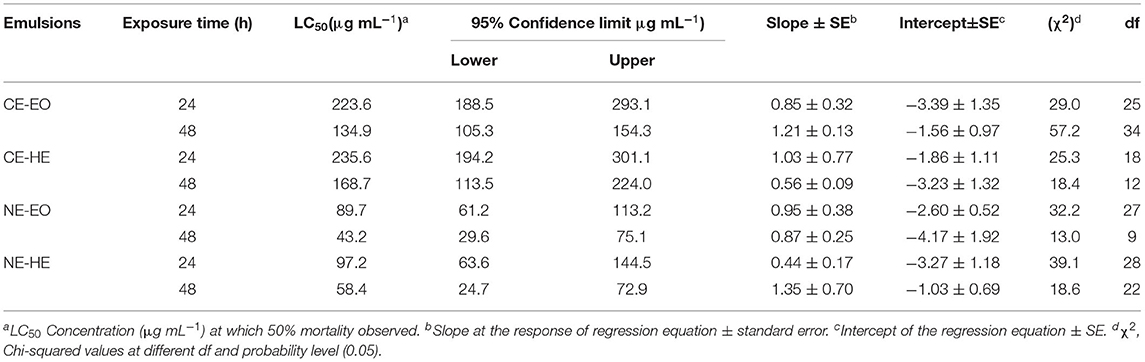
Table 3. Contact toxicity of CEs and NEs against the adults of T. urticae after 24 and 48 h of treatment.
Fumigant toxicity of the emulsions showed promising action at the sublethal concentrations after 24-h exposure (Table 4). Adults were found dead outside the border of the treated leaves. Among the tested emulsions, NE-EO (LC50 9.3 μg mL−1) displayed maximum fumigant action, while similar trend of fumigant action was observed for NE-HE (LC50 13.6 μg mL−1). NEs of the oil and non-polar fractions were equally effective in fumigant toxicity, even more potent than the contact toxicity assay.
Insecticidal Action Against S. litura
Larvicidal assay of NEs of EO and HE against third instar larvae revealed moderate action. CEs were found less effective with the LC50 values >400 μg mL−1. However, NEs-EO were effective, performing LC50 values 125.8 and 145.9 μg mL−1 after 48 and 24 h, respectively (Table 5). Similar findings were noticed for the NEs-HE, which exhibited LC50 values of 167.5 μg mL−1 and 190.6 μg mL−1 after 48- and 24-h exposure, respectively.
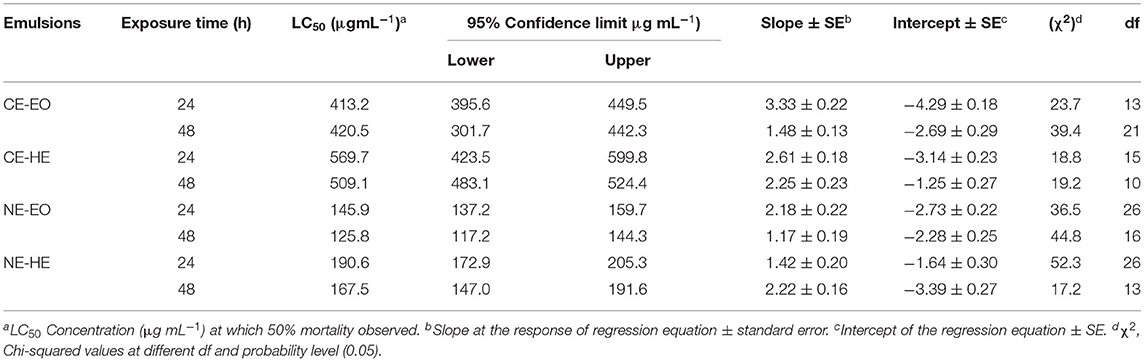
Table 5. Contact toxicity of CEs and NEs against third instar larvae of S. litura using leaf dip assay after 24 and 48 h.
Antifeedant activity of CEs and NEs of EO and HE demonstrated sufficient antifeedant activity at all the test concentrations in both no-choice and choice assays against larvae of S. litura (Figure 6). At the highest concentration of 500 μg mL−1, CE-EO and CE-HE showed the maximum AI value of 89.75 ± 2.12 and 87.55 ± 2.45, respectively, while NE-EO and NE-HE at the same concentration possessed AI value of 99.21 ± 0.74 and 98.75 ± 1.02, respectively (Table 6). Indeed, antifeedant activity of the CEs has been improved nearly 10% at the higher test concentrations and over 20% at the lower concentrations. On the contrary, NE-EO and NE-HE exhibited FI values of 99.73 ± 1.24 and 97.34 ± 1.0, respectively.
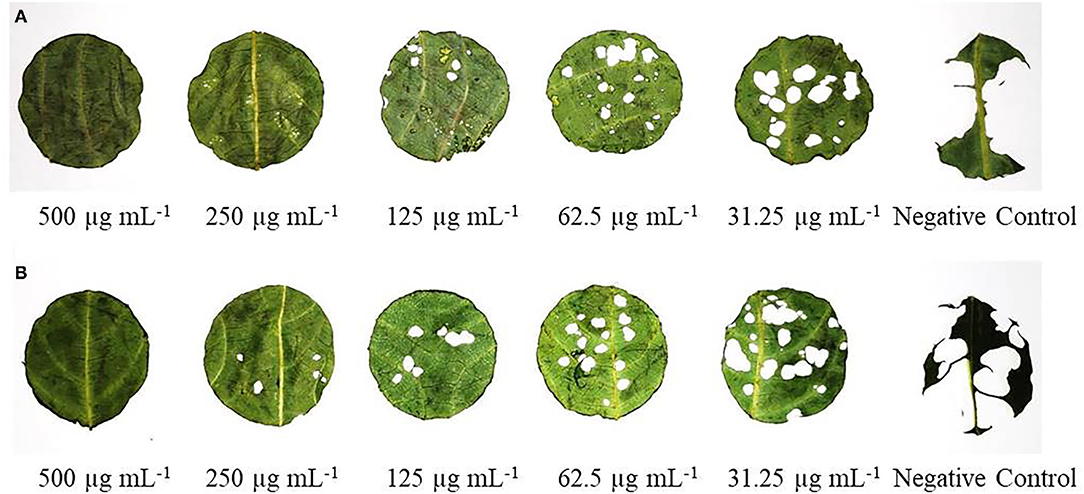
Figure 6. Visual display of antifeedant action of NEs of (A) EO and (B) HE against S. litura third instar larvae after 24 h.
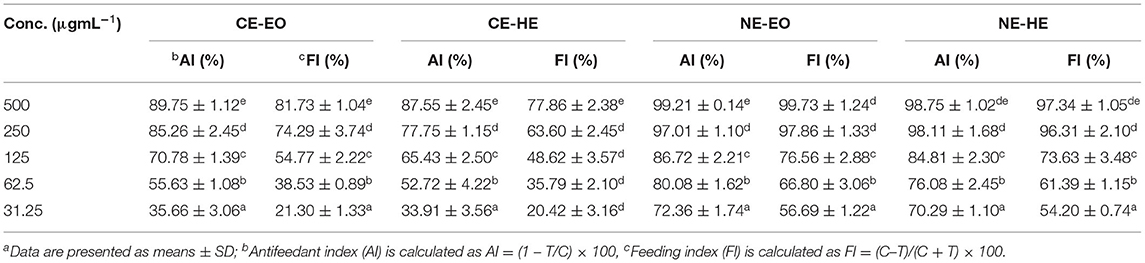
Table 6. Evaluationa of CEs and NEs of EO and HE of P. cablin for determination of antifeedant index (AI) and feeding index (FI) after 24 h.
Discussion
Comprehensive information on EO of P. cablin displayed abundance of either patchoulene or patchouli alcohol (Sundaresan et al., 2012). However, α-guaiene has been reported as the major constituent of Pogostemon EO (Tsai et al., 2007). Recently, forty-seven volatile constituents consisting of twenty sesquiterpenes were reported from the EO, which mentioned abundance of curzerene followed by epi-cadinol and acetophenone (Kumar et al., 2019b). Exceptionally, aciphyllene and acetophenone are often identified in higher content in the commercially available EO, which was further authenticated to be found as an adulterant, the major constituent of P. heyneanus (Murugan et al., 2010). Another literature report, from South Indian sample of P. cablin, suggested high content of acetophenone, β-pinene, and (E)-nerolidol in the EO (Anjana and Thoppil, 2013). Such variations in chemical compositions of EO could be attributed due to the distillation techniques, associated temperature on extraction, agroclimatic factors along with genetic variations of the planting materials, etc. (Kundu et al., 2013b; Dutta et al., 2020). A recent report suggested quality control and regulation aspects of patchouli EO are highly dependent on the variable composition of patchoulol and other sesquiterpenes (Pandey et al., 2022).
Saponin was used as emulsifier to develop the NEs which certainly contributed in positive manner to enhance the efficacy of the developed emulsions. It is the first report on the use of natural polymer for the preparation of NEs of Pogostemon bioactives, though most of the previous studies have been reported on the use of various non-ionic surfactants for the fabrication of NEs of EOs (Balasubramani et al., 2017; Campolo et al., 2017). The wide availability and relatively lower cost of the non-ionic surfactants could be responsible for its higher use. Contrastingly, xanthan gum along with subcritical water has been used to develop oil-in-water NEs (Ahmadi and Jafarizadeh-Malmiri, 2021a). They also suggested the application of natural gums and/or saponin using subcritical water for the preparation of EO-based NEs (Ahmadi and Jafarizadeh-Malmiri, 2021b). Thus, the use of green biopolymer has an edge over the excessive use of conventional emulsifiers in sustainable agriculture. In addition, saponin-like biopolymeric emulsifiers may enhance the biofunctional properties of the component.
In the present study, stable NEs were developed with saponin employing ultrasonication. Low energy subcritical water-based green method has been reported recently for the preparation of thyme oil-in-water NEs (Ahmadi and Jafarizadeh-Malmiri, 2020). The developed NEs exhibited better stability over a period of 14 days. Zeta potential of any emulsion has been recommended as the key indicator for its stability, which is related to electrostatic repulsion among the nanodroplets (Zainol et al., 2012). The stability of the NEs will be maintained with the limited or no coagulation of droplets, and therefore, the colloidal system should retain its droplet diameter in nano size range (<100 nm), resulting in better Brownian motion of the particles in the system (Heydari et al., 2020).
Previously, a homogenization method consisting of multiple adjuvants has been reported for the preparation of NEs of Pogostemon EO with non-ionic surfactant, propylene glycol as co-surfactant, and lecithin as emulsifier at 5% concentration; however, concomitant data on the physico-chemical properties have not been generated (Adhavan et al., 2017). Likewise, another study also reported the use of Tween 80 and/or Triton X-100 to stabilize geranium EO maintaining the oil surfactant ratio ranging from 5:1 to 1:5, suggesting better stability of the emulsion with higher amount of surfactant (Jesser et al., 2020). In the present context, NEs were prepared using ultrasound-assisted nano-emulsification of EO and lipid-soluble HE of P. cablin leaves with only biosurfactant, saponin at 0.5% concentration, without any use of co-surfactant, and/or co-emulsifier. In addition, better droplet size and emulsion stability of these NEs have been ascertained with rigorous physico-chemical characterizations and process validations.
A preliminary investigation on NEs of P. cablin oil (18%) with polyoxyethylene suggested excellent formicidal action on Atta opaciceps and Atta sexdens (Rocha et al., 2018). Furthermore, P. cablin oil-based tincture, candle, and crystal cake have been found effective as mosquito repellent (Das et al., 2016). Recently, patchouli oil has been nano-encapsulated on chitosan and evaluated for enhanced shelf life of maize seeds (Roshan et al., 2022). Recent past, the EO has been reported very effective in repellent action and fumigant toxicity against T. cinnabarinus (Cheng et al., 2020). Furthermore, better performance of NEs has been reported in literature against various insects (Badawy et al., 2018; Abdelgaleil et al., 2019). Current study revealed tremendous performance of the NEs over CEs against T. urticae both in fumigant and contact toxicity assays. However, required concentration of the emulsions was found to be very less to cause lethal action in fumigant assay, causing suffocation to death due to volatile nature of the chemical constituents. Nevertheless, acaricidal action in contact toxicity has been found satisfactory.
Plant volatiles have been found effective against many agriculturally important pests (Kundu et al., 2013a; Ahluwalia et al., 2014; Keerthiraj et al., 2021). Comprehensive studies have been reported on insecticidal activity of P. cablin oil. In the present study, NEs were found to be highly effective to cause larval mortality. Comparative assessment of the insecticidal activity of CEs and NEs revealed higher effectiveness of NEs due to nano sizing of the delivery system with higher surface area and better penetration through insect cell wall. Strong antifeedant and larvicidal action of the plant have been reported in literature against noctuid insects (Huang et al., 2014). NE-EO and NE-HE displayed considerable action with respect to AI and FI against S. litura larvae even at the lowest concentration of 31.25 μg mL−1. The salient finding of the antifeedant action certainly corroborates the literature report describing more than 80% feeding deterrent action of the oil (Huang et al., 2014). Major chemical components such as patchouli alcohol, α-bulnesene, and α-guaiene in both EO and HE could be responsible for higher efficacy. Though it is unclear whether the most abundant constituent, patchouli alcohol, is only responsible for the acaricidal and insecticidal action, further mechanism of action needs to be studied.
Conclusions
In summary, patchouli alcohol has been identified as the major constituent of EO and HE of P. cablin leaves. Stable NEs have been prepared with only 0.5% saponin either for EO or HE. The NEs of EO and HE were stable even after 30 days at room temperature with satisfactory qualitative characteristics. The results of acaricidal and antifeedant activities have demonstrated promising effect of NEs of EO and HE against T. urticae and S. litura following a dose-dependent trend, though NEs of EO have been performed slightly better than HE. As far as our literature survey could ascertain, this is the first report on potential activity of NEs of P. cablin against T. urticae. The output of the study has been well justified with the generation of key information for the development and utilization of NEs. However, future research regarding basic and fundamental studies on the mechanism of action of the compositional terpenoids is still needed for the development of next-generation bio-acaricides.
Data Availability Statement
The raw data supporting the conclusions of this article will be made available by the authors, without undue reservation.
Author Contributions
KM conducted the experiments. AK conceptualized the research work, analyzed the data, and wrote the manuscript. AD performed the analysis. SS supervised the experiments. BN analyzed the data and edited the manuscript. All authors contributed to the article and approved the submitted version.
Funding
This work was supported by the Indian Council of Agricultural Research (ICAR) and the Ministry of Agriculture and Farmers Welfare, Government of India (Grant No. CRSCIARISIL 2014035267), India.
Conflict of Interest
The authors declare that the research was conducted in the absence of any commercial or financial relationships that could be construed as a potential conflict of interest.
Publisher's Note
All claims expressed in this article are solely those of the authors and do not necessarily represent those of their affiliated organizations, or those of the publisher, the editors and the reviewers. Any product that may be evaluated in this article, or claim that may be made by its manufacturer, is not guaranteed or endorsed by the publisher.
Acknowledgments
We wish to thank Director, Indian Council of Agricultural Research–Indian Agricultural Research Institute (ICAR-IARI), New Delhi, India. The work has been performed by the student under his postgraduate program, PG School, ICAR-IARI, New Delhi, India, awarded with the fellowship under Junior Research Fellowship scheme, Indian Council of Agricultural Research (ICAR), New Delhi, India.
References
Abdelgaleil, S. A., Badawy, M. E., Mahmoud, N. F., and Marei, A. E. S. M. (2019). Acaricidal activity, biochemical effects and molecular docking of some monoterpenes against two-spotted spider mite (Tetranychus urticae Koch). Pestic. Biochem. Physiol. 156, 105–115. doi: 10.1016/j.pestbp.2019.02.006
Acevedo-Fani, A., Salvia-Trujillo, L., Rojas-Gra,ü, M. A., and Martín-Belloso, O. (2015). Edible films from essential-oil-loaded nanoemulsions: physicochemical characterization and antimicrobial properties. Food Hydrocoll. 47, 168–177. doi: 10.1016/j.foodhyd.2015.01.032
Adhavan, P., Kaur, G., Princy, A., and Murugan, R. (2017). Essential oil nanoemulsions of wild patchouli attenuate multi-drug resistant gram-positive, gram-negative and Candida albicans. Ind. Crop Prod. 100, 106116. doi: 10.1016/j.indcrop.2017.02.015
Ahluwalia, V., Sisodia, R., Walia, S., Sati, O. P., Kumar, J., and Kundu, A. (2014). Chemical analysis of essential oils of Eupatorium adenophorum and their antimicrobial, antioxidant and phytotoxic properties. J. Pest Sci. 87, 341–349. doi: 10.1007/s10340-013-0542-6
Ahmadi, O., and Jafarizadeh-Malmiri, H. (2020). Green approach in food nanotechnology based on subcritical water: effects of thyme oil and saponin on characteristics of the prepared oil in water nanoemulsions. Food Sci. Biotechnol. 29, 783–792. doi: 10.1007/s10068-019-00727-0
Ahmadi, O., and Jafarizadeh-Malmiri, H. (2021a). Intensification and optimization of the process for thyme oil in water nanoemulsions preparation using subcritical water and xanthan gum. Z. Phys. Chem. 235, 629–648. doi: 10.1515/zpch-2020-0001
Ahmadi, O., and Jafarizadeh-Malmiri, H. (2021b). Intensification process in thyme essential oil nanoemulsion preparation based on subcritical water as green solvent and six different emulsifiers. Green Process. Synth. 10, 430–439. doi: 10.1515/gps-2021-0040
Anjana, S., and Thoppil, J. E. (2013). Analysis of cytotoxic potential of the aqueous leaf extracts of Pogostemon auricularius (l.) Hassk. using Allium cepa root tip assay. Int. J. Res. Development Pharm. Life Sci. 2, 562–566.
Badawy, M. E., Abdelgaleil, S. A., Mahmoud, N. F., and Marei, A. E. S. M. (2018). Preparation and characterizations of essential oil and monoterpene nanoemulsions and acaricidal activity against two-spotted spider mite (Tetranychus urticae Koch). Int. J. Acarology 44, 330–340. doi: 10.1080/01647954.2018.1523225
Balasubramani, S., Rajendhiran, T., Moola, A. K., and Diana, R. K. B. (2017). Development of nanoemulsion from Vitex negundo L. essential oil and their efficacy of antioxidant, antimicrobial and larvicidal activities (Aedes aegypti L.). Environ. Sci. Pollut. Res. 24(17), 15125–15133. doi: 10.1007/s11356-017-9118-y
Campolo, O., Cherif, A., Ricupero, M., Siscaro, G., Grissa-Lebdi, K., Russo, A., et al. (2017). Citrus peel essential oil nanoformulations to control the tomato borer, Tuta absoluta: chemical properties and biological activity. Sci. Rep. 7, 13036. doi: 10.1038/s41598-017-13413-0
Cheng, Z. H., Duan, H. J., Zhu, X. R., Fan, F. F., Li, R., Li, S. C., et al. (2020). Effects of patchouli and wormwood oils on the bioassays and behaviors of Tetranychus cinnabarinus (Boisduval)(Acari: Tetranychidae). Int. J. Pest Manag. 66, 271–278. doi: 10.1080/09670874.2019.1636155
Das, K., Vasudeva, C., and Dang, R. (2016). Economical novel formulation and evaluation of herbal oils for mosquito and house fly repellent activities. Ann. Phytomed. 5, 91–96. doi: 10.21276/ap.2016.5.2.11
Dolma, S. K., Suresh, P. S., Singh, P. P., Sharma, U., and Reddy, S. G. (2021). Insecticidal activity of the extract, fractions, and pure steroidal saponins of Trillium govanianum W all. ex D. D on for the control of diamondback moth (Plutella xylostella L.) and aphid (Aphis craccivora K och). Pest. Manag. Sci. 77, 956–962. doi: 10.1002/ps.6107
Dutta, S., Kundu, A., Dutta, A., Saha, S., and Banerjee, K. (2021). A comprehensive chemical profiling of phytochemicals from Trachyspermum ammi and encapsulation for sustained release. LWT. 147, 111577. doi: 10.1016/j.lwt.2021.111577
Dutta, S., Kundu, A., Saha, S., Prabhakaran, P., and Mandal, A. (2020). Characterization, antifungal properties and in silico modelling perspectives of Trachyspermum ammi essential oil. LWT. 131, 109786. doi: 10.1016/j.lwt.2020.109786
Feng, Y. X., Wang, Y., You, C. X., Guo, S. S., Du, Y. S., and Du, S. S. (2019). Bioactivities of patchoulol and phloroacetophenone from Pogostemon cablin essential oil against three insects. Int. J. Food Prop. 22, 1365–1374. doi: 10.1080/10942912.2019.1648508
Ghosh, V., Mukherjee, A., and Chandrasekaran, N. (2013). Ultrasonic emulsification of food-grade nanoemulsion formulation and evaluation of its bactericidal activity. Ultrason. Sonochem. 20, 338–344. doi: 10.1016/j.ultsonch.2012.08.010
Hashem, A. S., Awadalla, S. S., Zayed, G. M., Maggi, F., and Benelli, G. (2018). Pimpinella anisum essential oil nanoemulsions against Tribolium castaneum—insecticidal activity and mode of action. Environ. Sci. Pollut. Res. 25, 18802-18812. doi: 10.1007/s11356-018-2068-1
Heydari, M., Amirjani, A., Bagheri, M., Sharifian, I., and Sabahi, Q. (2020). Eco-friendly pesticide based on peppermint oil nanoemulsion: Preparation, physicochemical properties, and its aphicidal activity against cotton aphid. Environ. Sci. Pollut. Res. 27, 6667–6679. doi: 10.1007/s11356-019-07332-y
Hu, G. Y., Peng, C., Xie, X. F., Xiong, L., Zhang, S. Y., and Cao, X. Y. (2018). Patchouli alcohol isolated from Pogostemon cablin mediates endothelium-independent vasorelaxation by blockade of Ca2+ channels in rat isolated thoracic aorta. J. Ethnopharmacol. 220, 188–196. doi: 10.1016/j.jep.2017.09.036
Huang, S. H., Xian, J. D., Kong, S. Z., Li, Y. C., Xie, J. H., Lin, J., et al. (2014). Insecticidal activity of pogostone against Spodoptera litura and Spodoptera exigua (Lepidoptera: Noctuidae). Pest Manag. Sci. 70, 510–516. doi: 10.1002/ps.3635
Jesser, E., Lorenzetti, A. S., Yeguerman, C., Murray, A. P., Domini, C., and Werdin-González, J. O. (2020). Ultrasound assisted formation of essential oil nanoemulsions: Emerging alternative for Culex pipienspipiens Say (Diptera: Culicidae) and Plodia interpunctella Hübner (Lepidoptera: Pyralidae) management. Ultrason. Sonochem. 61, 104832. doi: 10.1016/j.ultsonch.2019.104832
Keerthiraj, M., Mandal, A., Dutta, T. K., Saha, S., Dutta, A., Singh, A., et al. (2021). Nematicidal and molecular docking investigation of essential oils from Pogostemon cablin ecotypes against Meloidogyne incognita. Chem. Biodivers. 18, e2100320. doi: 10.1002/cbdv.202100320
Kentish, S., Wooster, T. J., Ashokkumar, M., Balachandran, S., Mawson, R., and Simons, L. (2008). The use of ultrasonics for nanoemulsion preparation. Innov. Food Sci. Emerg. Technol. 9, 170–175. doi: 10.1016/j.ifset.2007.07.005
Khot, L. R., Sankaran, S., Maja, J. M., Ehsani, R., and Schuster, E. W. (2012). Applications of nanomaterials in agricultural production and crop protection: a review. Crop Prot. 35, 64–70. doi: 10.1016/j.cropro.2012.01.007
Kumar, R., Kundu, A., Dutta, A., Saha, S., Das, A., and Bhowmik, A. (2021). Chemo-profiling of bioactive metabolites from Chaetomium globosum for biocontrol of Sclerotinia rot and plant growth promotion. Fungal Biol. 125, 167–176. doi: 10.1016/j.funbio.2020.07.009
Kumar, S., Nehra, M., Dilbaghi, N., Marrazza, G., Hassan, A., and Kim, K. (2019a). Nano-based smart pesticide formulations: emerging opportunities for agriculture. J. Control Release. 294, 131–153. doi: 10.1016/j.jconrel.2018.12.012
Kumar, V., Shriram, V., Bhagat, R., Khare, T., Kapse, S., and Kadoo, N. (2019b). Phytochemical profile, anti-oxidant, anti-inflammatory, and anti-proliferative activities of Pogostemon deccanensis essential oils. 3 Biotech 9, 31. doi: 10.1007/s13205-018-1560-0
Kundu, A., Dutta, A., Mandal, A., Negi, L., Malik, M., Puramchatwad, R., et al. (2021). A comprehensive in vitro and in silico analysis of nematicidal action of essential oils. Front. Plant Sci. 11, 614142. doi: 10.3389/fpls.2020.614143
Kundu, A., Saha, S., Walia, S., Ahluwalia, V., and Kaur, C. (2013a). Antioxidant potential of essential oil and cadinene sesquiterpenes of Eupatorium adenophorum. Toxicol. Environ. Chem. 95, 127–137. doi: 10.1080/02772248.2012.759577
Kundu, A., Saha, S., Walia, S., and Dutta, T. K. (2016). Antinemic potentiality of chemical constituents of Eupatorium adenophorum Spreng leaves against Meloidogyne incognita. Nat. Acad. Sci. Lett. 39, 145–149. doi: 10.1007/s40009-016-0439-z
Kundu, A., Saha, S., Walia, S., Shakil, N. A., Kumar, J., and Annapurna, K. (2013b). Cadinene sesquiterpenes from Eupatorium adenophorum and their antifungal activity. J. Environ. Sci. Health B. 48, 516–522. doi: 10.1080/03601234.2013.761921
Kusuma, H. S., Altway, A., and Mahfud, M. (2018). Solvent-free microwave extraction of essential oil from dried patchouli (Pogostemon cablin Benth) leaves. J. Ind. Eng. Chem. 58, 343–348. doi: 10.1016/j.jiec.2017.09.047
Lima, J. K., Albuquerque, E. L., Santos, A. C. C., Oliveira, A. P., Araújo, A. P. A., Blank, A. F., et al. (2013). Biotoxicity of some plant essential oils against the termite Nasutitermes corniger (Isoptera: Termitidae). Ind. Crop Prod. 47, 246–251. doi: 10.1016/j.indcrop.2013.03.018
Machial, C. M., Shikano, I., Smirle, M., Bradbury, R., and Isman, M. B. (2010). Evaluation of the toxicity of 17 essential oils against Choristoneura rosaceana (Lepidoptera: Tortricidae) and Trichoplusia ni (Lepidoptera: Noctuidae). Pest Manag. Sci. 66, 1116–1121. doi: 10.1002/ps.1988
McClements, D. J.. (2004). Food Emulsions: Principles, Practices, and Techniques (2nd edition). Boca Raton, Florida: CRC Press. doi: 10.1201/9781420039436
McClements, D. J., and Gumus, C. E. (2016). Natural emulsifiers-Biosurfactants, phospholipids, biopolymers, and colloidal particles: molecular and physicochemical basis of functional performance. Adv. Colloid. Interface Sci. 234, 3–26. doi: 10.1016/j.cis.2016.03.002
Murugan, R., Mallavarapu, G. R., Padmashree, K. V., Rao, R. R., and Livingstone, C. (2010). Volatile oil composition of Pogostemon heyneanus and comparison of its composition with patchouli oil. Nat. Prod. Commun. 5, 1961–1964. doi: 10.1177/1934578X1000501228
Nenaah, G. E., Ibrahim, S. I., and Al-Assiuty, B. A. (2015). Chemical composition, insecticidal activity and persistence of three Asteraceae essential oils and their nanoemulsions against Callosobruchus maculatus (F.). J. Stored Prod. Res. 61, 9–16. doi: 10.1016/j.jspr.2014.12.007
Noori, S., Zeynali, F., and Almasi, H. (2018). Antimicrobial and antioxidant efficiency of nanoemulsion-based edible coating containing ginger (Zingiber officinale) essential oil and its effect on safety and quality attributes of chicken breast fillets. Food Cont. 84, 312–320. doi: 10.1016/j.foodcont.2017.08.015
Pandey, S. K., Bhandari, S., Sarma, N., Begum, T., Munda, S., Baruah, J., et al. (2022). Essential oil compositions, pharmacological importance and agro technological practices of Patchouli (Pogostemon cablin Benth.): A review. J. Essent. Oil Bearing Plants. 24, 1212–1226. doi: 10.1080/0972060X.2021.1995511
Pavela, R., and Benelli, G. (2016). Essential oils as ecofriendly biopesticides? Challenges and constraints. Trends Plant Sci. 21, 1000–1007. doi: 10.1016/j.tplants.2016.10.005
Rocha, A. G., Oliveira, B. M. S., Melo, C. R., Sampaio, T. S., Blank, A. F., Lima, A. D., et al. (2018). Lethal effect and behavioral responses of leaf-cutting ants to essential oil of Pogostemon cablin (Lamiaceae) and its nanoformulation. Neotrop. Entomol. 47, 769–779. doi: 10.1007/s13744-018-0615-6
Roshan, A. B., Dubey, N. K., and Mohana, D. C. (2022). Chitosan nanoencapsulation of Pogostemon cablin (Blanco) Benth. essential oil and its novel preservative effect for enhanced shelf life of stored Maize kernels during storage: Evaluation of its enhanced antifungal, antimycotoxin, antioxidant activities and possible mode of action. Int. J. Food Sci.Technol. 57, 2195–2202. doi: 10.1111/ijfs.15289
Sengonca, C., Liu, B., and Zhu, Y. J. (2006). Insecticidal activity and antifeedant effect of a new type biocide GCSC-BtA against Plutella xylostella L. (Lep., Plutellidae). J. Pest Sci. 79, 3. doi: 10.1007/s10340-005-0117-2
Sugumar, S., Clarke, S. K., Nirmala, M. J., Tyagi, B. K., Mukherjee, A., and Chandrasekaran, N. (2014). Nanoemulsion of eucalyptus oil and its larvicidal activity against Culex quinquefasciatus. Bull Entomol. Res. 104, 393–402. doi: 10.1017/S0007485313000710
Sundaresan, V., Sahni, G., Verma, R. S., Padalia, R. C., Mehrotra, S., and Thul, S. T. (2012). Impact of geographic range on genetic and chemical diversity of Indian valerian (Valeria najatamansi) from northwestern Himalaya. Biochem. Genet. 50, 797–808. doi: 10.1007/s10528-012-9521-5
Tsai, Y. C., Hsu, H. C., Yang, W. C., Tsai, W. J., Chen, C. C., and Watanabe, T. (2007). α-Bulnesene, a PAF inhibitor isolated from the essential oil of Pogostemon cablin. Fitoterapia 78, 7–11. doi: 10.1016/j.fitote.2006.09.016
Keywords: biopolymer, volatile oil, ultrasonication, acaricidal, patchouli, Tetranychus urticae
Citation: Manjesh K, Kundu A, Dutta A, Saha S and Neelakanthaiah BS (2022) Bio-Insecticidal Nanoemulsions of Essential Oil and Lipid-Soluble Fractions of Pogostemon cablin. Front. Plant Sci. 13:874221. doi: 10.3389/fpls.2022.874221
Received: 11 February 2022; Accepted: 28 March 2022;
Published: 29 April 2022.
Edited by:
Jouda Mediouni-Ben Jemâa, Institut National de la Recherche Agronomique de Tunisie (INRAT), TunisiaReviewed by:
Abhishek Kumar Dwivedy, Banaras Hindu University, IndiaAgnieszka Synowiec, University of Agriculture in Krakow, Poland
Omid Ahmadi, Sahand University of Technology, Iran
Copyright © 2022 Manjesh, Kundu, Dutta, Saha and Neelakanthaiah. This is an open-access article distributed under the terms of the Creative Commons Attribution License (CC BY). The use, distribution or reproduction in other forums is permitted, provided the original author(s) and the copyright owner(s) are credited and that the original publication in this journal is cited, in accordance with accepted academic practice. No use, distribution or reproduction is permitted which does not comply with these terms.
*Correspondence: Aditi Kundu, chem.aditi@gmail.com; aditi.kundu@icar.gov.in