- 1Department of Plant Physiology, College of Agriculture Vellanikkara, Kerala Agricultural University, Thrissur, India
- 2Centre for Intellectual Property Rights, Technology Management and Trade, College of Agriculture Vellanikkara, Kerala Agricultural University, Thrissur, India
- 3Department of Vegetable Science, College of Agriculture Vellanikkara, Kerala Agricultural University, Thrissur, India
Vegetable production is a key determinant of contribution from the agricultural sector toward national Gross Domestic Product in a country like India, the second largest producer of fresh vegetables in the world. This calls for a careful scrutiny of the threats to vegetable farming in the event of climate extremes, environmental degradation and incidence of plant pests/diseases. Cucurbits are a vast group of vegetables grown almost throughout the world, which contribute to the daily diet on a global scale. Increasing food supply to cater to the ever-increasing world population, calls for intensive, off-season and year-round cultivation of cucurbits. Current situation predisposes these crops to a multitude of stressors, often simultaneously, under field conditions. This scenario warrants a systematic understanding of the different stress specific traits/mechanisms/pathways and their crosstalk that have been examined in cucurbits and identification of gaps and formulation of perspectives on prospective research directions. The careful dissection of plant responses under specific production environments will help in trait identification for genotype selection, germplasm screens to identify superior donors or for direct genetic manipulation by modern tools for crop improvement. Cucurbits exhibit a wide range of acclimatory responses to both biotic and abiotic stresses, among which a few like morphological characters like waxiness of cuticle; primary and secondary metabolic adjustments; membrane thermostability, osmoregulation and, protein and reactive oxygen species homeostasis and turnover contributing to cellular tolerance, appear to be common and involved in cross talk under combinatorial stress exposures. This is assumed to have profound influence in triggering system level acclimation responses that safeguard growth and metabolism. The possible strategies attempted such as grafting initiatives, molecular breeding, novel genetic manipulation avenues like gene editing and ameliorative stress mitigation approaches, have paved way to unravel the prospects for combined stress tolerance. The advent of next generation sequencing technologies and big data management of the omics output generated have added to the mettle of such emanated concepts and ideas. In this review, we attempt to compile the progress made in deciphering the biotic and abiotic stress responses of cucurbits and their associated traits, both individually and in combination.
Vegetable Production: The Cucurbit Context
The vegetable crops belonging to the family Cucurbitaceae are known as cucurbits or gourds. This important family of vegetables contains 950 species in over 90 genera and is mainly distributed in the tropics and subtropics (Schaefer and Renner, 2011). Cucurbit family includes several genera and represents the largest tropical vegetable group (Roy and Chakrabarti, 2003; Ebert et al., 2021), as summarized in Table 1
Wide variability is observed in the genetic makeup of the members with monoploid chromosome number ranging from seven (Cucumis sativus) to twenty (Cucurbita spp.) (Rai et al., 2007; Choudhary et al., 2016; Samadia and Haldhar, 2017). A great variability also exists in the utilization of these crops, viz., salads (cucumber, gherkins, long melon), sweet dishes (ash gourd, pointed gourd), pickles (gherkins), and desserts (melons). Cucurbit seeds are also high in oil and protein content attesting to their nutritive value (Rahman et al., 2008; Choudhary et al., 2015; Bhargava et al., 2016).
Despite their wide adaptability and varied uses in different parts of the world, their commercial cultivation is increasingly facing the threats of climate change and consequent biotic and abiotic stresses as well as genetic erosion. It is imperative that a holistic approach on the scientific management of various factors affecting the crop performance including development of stress tolerant types, manipulation of metabolic pathways for tolerance/resistance and their crosstalks, as well as propagation strategies for withstanding biotic and abiotic stresses be adopted for successful cucurbit production.
Biotic Stress Responses in Cucurbits
Cucurbits are often attacked by a wide range of pests including beetles, fruit flies, aphids, white flies, borers, mites etc. (Sharma et al., 2016; Srivastava and Joshih, 2021; Table 2). The pests include those affecting cucurbits worldwide as well as those which are more pronounced in certain regions of the globe, where they attain the status of primary pests. Cucumber moth, Diaphania indica, is a potentially damaging pest of cucurbitaceous vegetables worldwide (Chintha et al., 2002; Kinjo and Arakaki, 2002; Hosseinzade et al., 2014; Dai et al., 2018; Jalali et al., 2019; Capinera, 2020; Debnath et al., 2020; Khanzada et al., 2021). The attractiveness of cucurbitaceous host plants for D. indica was observed to depend on the species and condition of the plant (uninfested and infested), and sex, mating status and experience of the insect. Females that had experience of cucumber, squash and melon plants were significantly attracted to the same plant, and the larvae were attracted only to volatiles of uninfested cucumber, squash and melon (Jalali et al., 2019). Striped cucumber beetle (StCB) and the western striped cucumber beetle (WStCB) are native to North America and StCB is reported to have attained the status of primary pest in northeastern and midwestern United States and eastern Canada (Haber et al., 2021).
Although pests attack affects all stages of cucurbits, severity and susceptibility depends on the plant type and stage of incidence. Pests like red pumpkin beetle and leaf miner are serious at seedling stage (Bains and Prakash, 1985) while beetles [flea beetles (Phyllotreta cruciferae) and spotted cucumber beetles (Diabrotica undecimpunctata)] were identified as major pests of cucumber at vegetative stage (Alao et al., 2017). Plant growth promoting rhizobacteria (PGPR) induced resistance was reported to be more effective than insecticides for control of cucumber beetles on cucumber possibly by inducing altered production of allelochemicals acting as beetle attractants, repellents, or feeding stimulants (Zehnder et al., 1997). The fruit fly (Zeugodacus cucurbitae), a pest of summer squash, cucumber, pumpkin and bitter gourd (Subedi et al., 2021) attacks only flowers and fruits at crop maturity (Ram et al., 2009). Alao et al. (2017) also demonstrated that at vegetative stage of the plant, insect attack was considerably lower in cucumber compared to watermelon, and was attributed to the presence of antixenosis or antibiosis factors in cucumber. Some of the pests that attack cucurbits like whiteflies, thrips and mites, transmit viruses apart from causing feeding damage (Wisler et al., 1998; Park and Lee, 2005; Messelink et al., 2008; Turechek et al., 2014). Although cultivation under greenhouse conditions is reported to be favorable for cucumber production, it is conducive for the rapid development of insect and mite populations (Messelink et al., 2020).
Climate change has led to resurgence of pests and their spread to new areas and often the resistance of varieties breakdown with the evolution of the pest. The fact that pests often become resistant to commercial pesticide formulations in use necessitates a study on pests and their management as well as identification of resistant genotypes and the traits that confer the resistance response.
Cucurbits are found to be affected severely by several diseases including fungal, bacterial and viral diseases, and nematodes, among which the viruses were reported to cause the largest number of diseases (McCreight, 2011; Table 3). Oomycete pathogens like Pseudoperonospora cubensis, causing downy mildew, affects all major cucurbit crops, including cucumber, muskmelon, squashes, and watermelon and can assume epidemic proportions (Holmes et al., 2015). Virus diseases, apart from causing reduction in vegetative growth and crop yield, also results in poor fruit quality and makes the plant susceptible to other pathogens as well (Sastry, 2013). However, some studies have demonstrated that healthy wild gourd plants (Cucurbita pepo ssp. texana) contract bacterial wilt at significantly higher rates than virus infected plants. Prior infection by Zucchini yellow mosaic virus (ZYMV) was found to delay the subsequent onset and progression of bacterial wilt disease by Erwinia tracheiphila (Shapiro et al., 2013). Majority of the fungal diseases in cucurbits caused by Stagonosporopsis cucurbitacearum (gummy stem blight and black rot), Alternaria alternata (leaf spot), Fusarium solani (damping off and wilt), Alternaria cucumerina (leaf spot) and Myrothecium roridum (foliar and stem lesion) are seed borne (Gannibal, 2011; Farrag and Moharam, 2012; Fish et al., 2012). Bacterial pathogens like Acidovorax avenae subsp. citrulli which causes fruit blotch of cucurbits is also reported to be seed borne and contaminated seeds is the main source of the bacterial inoculum (Block and Shepherd, 2008). Cucurbits adopt various strategies to resist or tolerate diseases. Despite the fact that several genotypes showing resistance to fungal, bacterial, viral and oomycete diseases are available in the germplasm, long-term planting, variable adaptability of pathogens and suppression of host resistance mechanisms by the pathogens often leads to a gradual decline in plant resistance (Chen et al., 2021; Gao et al., 2021; Zhang S. et al., 2021).
Abiotic Stress Responses in Cucurbits
Cucurbits are a vast group of vegetables which contribute to the daily diet of a large portion of the world population and are grown almost throughout the world. These vegetables are a good source of nutrients and hence play a vital role in ensuring nutritional security to mankind. Increasing food demands call for intensive, offseason and year-round cultivation of cucurbits, thereby predisposing these crops to a multitude of stressors like high temperature, drought, salinity, heavy metal toxicity, nutrient deficiency/toxicity, soil pH etc. The climate change scenario has further intensified the predisposition to abiotic stressors- high temperature, drought and salinity being the major players in the global arena.
Plants support their growth and development even under adverse conditions by developing several tolerance and adaptation mechanisms. The biochemical, physiological and molecular responses elicited in response to abiotic stress are guided by common stress tolerance pathways, shared by most of the cultivated crops.
Heat Stress Response
Global warming is one of the most alarming effects of climate change with a long term impact on agriculture, particularly the vulnerable vegetable crops. Heat stress is a function of temperature, duration/period of stress and rate of increase in temperature. Cucurbits being warm season vegetables, are more likely to be exposed to heat stress particularly the summer crop. High temperatures can influence cell development, synthesis of cell wall, plant hormonal connections, amalgamation of proteins, stomatal regulation (thereby influencing photosynthesis, CO2 assimilation and respiration) etc. (Hasanuzzaman et al., 2012).
High temperatures adversely affect several physiological, biochemical, morphological and molecular processes and pathways in plants. Seed germination of cucumber and melon is reduced drastically at 45 and 42°C respectively (Kurtar, 2010). The ideal temperatures for crop growth and development are 18.3–23.8°C for squash, pumpkin, muskmelon and cucumber, and 23.8–29.4°C for watermelon. In cucumber (Cucumis sativus L.) or watermelon (Citrullus lanatus L.), temperatures above 35°C caused a reduction in flowers and sugar content (Lai et al., 2018). Heat stress resulted in reduced biomass, root growth and development, leaf area (Porter and Gawith, 1999; Al-Busaidi et al., 2012; Balal et al., 2016) and decreased fruit length, fruit diameter and reduced fruit weight (Balal et al., 2016). The alterations in cell division, cell elongation, water loss and reduced photosynthetic rate under heat stress resulted in reduced yield, leaf area, biomass etc. (Hasanuzzaman et al., 2013).
The photosynthetic rate is positively correlated with the chlorophyll content in the leaves (Lin et al., 2011). Plant growth and yield are adversely affected due to reduced chlorophyll under high temperature stress and subsequent reduction in photosynthetic rate. In cucumber, heat stress induced reduction in chlorophyll and photosynthetic rate has been observed (Balal et al., 2016; Zhou et al., 2016). Reactive oxygen species (ROS) levels are enhanced in the plant tissues in response to heat stress which results in oxidative stress (Suzuki and Mittler, 2006; Potters et al., 2007; Pucciariello et al., 2012). During the electron transport in photosynthetic process, electron leakage to oxygen molecule results in generation of ROS (Sharma et al., 2012). Plants have different mechanisms (enzymatic and non-enzymatic) to detoxify the ROS. Several antioxidative enzyme activities, i.e., superoxide dismutase (SOD), peroxidase (POD), catalase (CAT), ascorbate peroxidase (APX), guaiacol peroxidase (GPX), glutathione reductase (GTR), monodehydroascorbate reductase (MDHAR), etc. are generally reduced under heat stress (Balal et al., 2016) and are upregulated in response to stress particularly in tolerant species or in response to ameliorants (Balal et al., 2016).
Another strategy to counteract stress induced osmotic damage is the accumulation of various compatible solutes like proline, glycine betaine (GB), amino acids, sugars, quaternary ammonium and sulphonium compounds etc. (Majumder et al., 2009). In cucumber, increased levels of proline, glycine betaine and total soluble sugars were reported in response to heat stress (Balal et al., 2016).
Screening of cucurbit genotypes based on these traits is an effective strategy to identify stress tolerant lines/varieties. High temperature tolerant varieties have been developed in cucurbits such as AHW-19, AHW-65, Thar Manak (Watermelon), Thar Samridhi (bottle gourd), Thar Karni (ridge gourd), Thar Tapish (sponge gourd) etc. (Saroj and Choudhary, 2020).
Drought Stress Response
Cucurbits are warm season vegetable crops mostly cultivated in the summer season, hence prone to drought stress if not irrigated at critical stages of growth. Drought response is classified into three categories viz., drought escape (shortening the life cycle), drought avoidance (minimizing water loss or maximizing water uptake thereby preventing exposure to stress) and drought tolerance (helps the plant to withstand stress by osmoregulation, osmotic adjustment, stomatal regulation etc.). However, crop adaptation to drought may be achieved through a balance between these three strategies (Saroj and Choudhary, 2020). Hence, a combination of different traits should be used as a screening criterion for drought tolerance, rather than a single trait (Singh and Sarkar, 1991). The important traits to be considered while breeding for drought tolerance are early vigor, root depth and density, low and high temperature tolerance, carbon isotope discrimination, osmoregulation, low stomatal conductance, leaf posture, reflectance and duration, sugar accumulation etc. However, priority should be given to those traits which can maintain stability of yield in addition to overall yield (Parry et al., 2005). Some of the drought tolerant genotypes identified are AHW-65 and Thar Manak in watermelon, VRSM-58, AHS-10, AHS-82 in snapmelon etc. In cucumber, drought stress reduces photosynthetic rate, increases superoxide anion radicals (O2.–), electrolyte leakage and lipid peroxidation products like malondialdehyde (MDA), whereas the activities of key antioxidant enzymes superoxide dismutase (SOD) and peroxidase (POD) as well as soluble sugar and proline contents are decreased (Wang J. et al., 2012; Zhang et al., 2013; Fan et al., 2014; Sun et al., 2016).
Salinity Stress Response
Soil salinity has become a severe problem in agricultural production. It is one of the major factors limiting plant growth and productivity particularly in the arid and semi-arid regions of the world (Parida and Das, 2005). Under salinity conditions, stress is induced due to lower water potential of the root medium, toxic effects of Na+ and Cl− and nutrient imbalance by reduction in uptake or shoot transport (Colla et al., 2006). Salinity stress response is multigenic, as a number of processes involved in the tolerance mechanism are affected, such as various compatible solutes/osmolytes, polyamines, ROS and antioxidant defense mechanisms, ion transport and compartmentalization of injurious ions (Sairam and Tyagi, 2004).
In cucumber, the salt tolerance in a genotype was associated with higher relative water content (RWC), total chlorophyll content, and SOD, CAT, and APOX activities, together with the lower MDA and proline contents, and Na+ and Cl– concentrations (Furtana and Tipirdamaz, 2010). Sodium chloride stress induces reduction in biomass, photosynthetic pigments, and proline accumulation, while lipid peroxidation and K+, Na+, and Cl– contents are increased (Hawrylak-Nowak, 2009). The addition of 150 mM of NaCl to the nutrient solution of a floating system where 30 varieties of Cucurbitaceae species were cultivated, affected plant growth parameters (number of leaves, shoot length, diameter and dry weight, root length and dry weight) in a genotype-dependent manner (Modarelli et al., 2020). Salinity reduced chlorophylla content by up to 49% in some genotypes, whereas in others chlorophylla content increased by up to 61%. Similarly, chlorophyllb was reduced by salinity by up to 51% in some genotypes or increased by up to 64% in some others. The increase in photosynthetic pigments was considered as a consequence of the reduction of the leaf area and therefore of the dilution effect. Moreover, salinity increased electrolyte leakage by up to 509%, as compared to the non-salinized control.
Heavy Metal Toxicity
Heavy metal accumulation in soils is of great concern in agricultural production due to the adverse effects on food safety and marketability, crop growth due to phytotoxicity, and environmental health of soil organisms (Gill, 2014). Heavy metals cause irreversible damage to a number of vital metabolic constituents and important biomolecules including injury to plant cell walls and cell membranes. Mercury, lead, cadmium, vanadium, arsenic, chromium etc. are some of the heavy metals which are present as soil pollutants and cause severe damage to the crops raised. A common consequence of heavy metal toxicity is the excessive accumulation of ROS and methylglyoxal (MG), both of which can cause peroxidation of lipids, oxidation of protein, inactivation of enzymes, DNA damage and/or interact with other vital constituents of plant cells.
Mercury (Hg) and lead (Pb) heavy metal stress results in high peroxidase activity in cucumber, bottle gourd, sponge gourd and bitter gourd (Khan and Chaudhry, 2006, 2010). In melon, with increasing cadmium concentration, seedling growth, net photosynthetic rate (Pn), stomatal conductance (gs), transpiration rate (Tr), and stomatal limitation (Ls) decreased; meanwhile, intercellular carbon dioxide concentration (Ci) increased significantly (Zhang et al., 2016; Khan et al., 2020). Hg induces oxidative stress in cucumber seedlings, resulting in plant injury due to reduced activities of antioxidant enzymes (catalase and ascorbate peroxidase), reduced chlorophyll content, increased lipid peroxidation, protein oxidation etc. (Cargnelutti et al., 2006).
Trait Gene Discovery and Functional Annotation- Individual and Combined Stress Events
Trait based crop improvement assumes significance in the context of the highly variable environmental conditions, which often results in the co-existence of multiple stresses. Identification of suitable traits/trait-combinations conducive for conferring tolerance in different ecosystems is inevitable. There have been promising reports on the functional characterization of different stress responsive genes in cucurbits or the genes cloned from cucurbits in model crops (Parmar et al., 2017; Nanasato and Tabei, 2020). The High Affinity K+ Transporter (HKT) genes encode Na+ and/or K+ transport systems, active at the plasma membrane and play a crucial role in imparting salt tolerance to different plant species e.g., HKT 1;5 in barley (Hazzouri et al., 2018) and CmHKT1;1 in pumpkin (Fu et al., 2018). The YUCCA proteins are critical partners in auxin biosynthesis in plants, which have been reported to regulate response to abiotic stresses and flower development in cucumbers. CsYUC8 and CsYUC9 were specifically upregulated to elevate the auxin level under high temperature in Cucumis sativus. CsYUC10b was dramatically increased but CsYUC4 was repressed in response to low temperature. CsYUC10a and CsYUC11 act against the upregulation of CsYUC10b under salinity stress, suggesting that distinct YUC members participate in different stress responses, and may even antagonize each other to maintain the proper auxin levels in cucumber (Yan et al., 2016). A wholistic genomic and functional analysis of bHLH genes was attempted in cucumber to identify 142 bHLH genes, classified into 32 subfamilies, among which five CsbHLH genes were found to simultaneously respond to three abiotic stresses (NaCl, ABA and low-temperature treatments). Targeted promoter analysis also revealed many cis-elements responsive to multiple stresses and plant hormones (Li et al., 2020). Similar attempts targeting different traits have resulted in the identification of prospective candidate genes, which have been functionally characterized in either cucurbits or in model crops like Arabidopsis. There have been reports on novel candidates such as intrinsically disordered proteins belonging to Plant Group II LEA Proteins with possible roles in multiple stress responses (Abdul Aziz et al., 2021), which could be promising even in cucurbits. The functional annotation of candidate genes in cucurbits has been achieved by traditional over-expression or gene silencing approaches, with recent advancements leading to the adoption of advanced gene interference technologies and CRISPR/CAS mediated gene editing approaches. The successful trait-gene based crop improvement attempts in this direction in different cucurbits have been tabulated in Table 4.
Possible Crosstalk in Pathways/Mechanisms Under Combined Stresses
Challenges faced by plants come in multitudes and often a combinatorial response to the simultaneous occurrence of stresses, either abiotic or biotic or cross combinations, is actually displayed by plants. A concerted effort to study cucumber plants exposed to salt stress and thereafter infected with Pseudomonas syringae pv lachrymans (Psl), revealed that there were distinct changes in photochemistry, the antioxidant system, primary carbon metabolism, salicylic acid (SA) and abscisic acid (ABA) contents. The careful examination of hormonal and redox balance as well as the carboxylate metabolism and activities of some NADPH-generating enzymes indicated that salt-stressed plants were more prone to pathogen infection. There can be critical convergence points and master regulators for the characteristic response to specific abiotic factor-pathogen combination. In case of cucumber, the combinatorial stress response to salt stress and P. syringae is dominated by the abiotic factor. Modulation of SA-mediated defense, hormonal, ROS/redox and metabolic signals are responsible for predisposing cucumber plants to P. syringae after sequential salt stress episodes making them highly susceptible (Chojak-Koźniewska et al., 2018). Another important stressor is temperature which has profound influence on the occurrence of bacterial diseases caused by Ralstonia solanacearum (causal agent of wilt in tomato), Acidovorax avenae (causal agent of seedling blight and bacterial fruit blotch of cucurbits) and Burkholderia glumae (causal agent of bacterial panicle blight in rice) (Kudela, 2009; Pandey et al., 2017). Cucumber mosaic virus (CMV) as an important viral invader of cucurbits has significance in understanding its interactive specificities with other stressors. CMV infection was found to impart improved drought tolerance of Capsicum annum (pepper), S. lycopersicum, and Nicotiana tabacum (tobacco) (Xu et al., 2008; Pandey et al., 2017). The combinatorial effects between abiotic-abiotic and abiotic-biotic pairs can be starkly different. Generally abiotic stress combinations can have “only net effects and no stress interactions,” leading to additive deteriorative effects due to co-occurrence of two stresses together. It will be different for a plant-pathogen system wherein, it may lead to enhanced or reduced susceptibility to the pathogen; some pathogens also modulate abiotic stress tolerance. In case of heat–pathogen and drought–pathogen stress combinations, wherein multiple individual stresses or sequential stresses occur one after the other, either prior priming leading to stress memory or predisposition can be the consequence (Pandey et al., 2017). CsbHLH041 is an important regulator in response to multiple abiotic stresses like salinity and water deficit in cucumber (Li et al., 2020). Phytohormonal variations can result in a common response against both biotic and abiotic stressors such as similar morphological root changes under CMV infection and a heavy metal challenge like cadmium stress (Vitti et al., 2013). It was very recently identified that there are distinct metabolite signatures, with special reference to amino acids, associated with response to salt and drought stresses in Cucumis melo L. (Chevilly et al., 2021). High histidine contents and the ability to sequester salts in vacuoles are expected to confer salt stress tolerance capacity. However, varieties or cultivars with enhanced levels of isoleucine, glycine, serine and asparagine exhibited drought stress tolerance. There was a retardation in tolerance to abiotic stresses when the phenylalanine levels were high (Chevilly et al., 2021).
Possible Strategies to Achieve Combined Stress Tolerance
A. Propagation Methods as a Means for Combining Multiple Stress Tolerance Traits
Vegetable grafting is a unique horticultural technique used in the propagation of fruit vegetables due to the multitude of advantages over the conventional propagation methods. Vegetable grafting was primarily developed and practiced with an objective of avoiding the damage caused by soil borne pathogens and pests (Cohen et al., 2007). The scope for grafting has further widened for combating abiotic stress tolerance, with the advancement in our understanding of the rootstock mediated effect on superior performance of scion, exploiting the physiological stress tolerance reserved in the wild species (Colla et al., 2010, 2012). Grafting has emerged as a viable alternative to relatively slower breeding approaches for enhancing environmental stress tolerance in fruit vegetables (Flores et al., 2010). Grafting is a special method of adapting plants to counteract environmental stresses by grafting superior commercial cultivars onto specific vigorous rootstocks (Lee and Oda, 2003).
Cucurbits are the first group of vegetables where grafting was widely popularized to combat biotic stress particularly Fusarium wilt. Research on cucurbit grafting began in the 1920s with the use of Cucurbita moschata as a rootstock for watermelon in Japan. Grafting is a quick, less expensive and viable solution for combating soil borne pathogens and their novel races, in comparison to the tedious breeding approach adopted for developing resistant cultivars (Davis et al., 2008). Watermelon, cucumber and melons are the major cucurbits which are propagated using grafted seedlings in order to overcome biotic and abiotic stresses.
(a) Grafting for Biotic Stress Tolerance in Cucurbits
In cucurbits, grafting has proven to impart resistance/tolerance to several fungal, bacterial and nematode infections, soil borne pathogens and even some viral as well as foliar pathogens. The most devastating soil borne pathogen of cucurbits is Fusarium oxysporum causing Fusarium wilt (FW). In watermelon and cucumber, grafting is the most popular alternative for controlling Fusarium wilt. Some of the achievements in combating biotic stress in cucurbits through grafting has been summarized in Table 5.
(b) Grafting for Abiotic Stress Tolerance in Cucurbits
Grafting has also emerged as an effective adaptive technique to overcome abiotic stressors including drought, flooding, waterlogging, salinity, heavy metal contamination, suboptimal and supraoptimal temperatures, nutrient deficiencies, toxicities etc. When the plants are exposed to these abiotic factors beyond the threshold level for optimal biochemical/physiological activity or morphological development, it results in reduction of plant performance and subsequent yield reduction. In cucurbits, several studies involving different rootstocks have proven their efficiency in alleviating the adverse effects of a number of abiotic factors; some of these have been summarized in Table 6.
Under the present climate change scenario multiple stresses in combination or separately, pose severe threat to vegetable production including cucurbits. Use of rootstocks conferring multiple stress resistance could be a sustainable and eco-friendly alternative to the more complicated traditional/molecular breeding approaches to develop multiple stress resistant varieties (Table 7). The melon hybrid (Cucurbita maxima x Cucurbita moschata), figleaf gourd (Cucurbita ficifolia), pumpkin, bottle gourd and sponge gourd rootstocks have the potential to impart multiple stress tolerance to scions of different cucurbits. Other wild and cultivated species of cucurbits could also be explored for their capabilities to confer multiple stress resistance to susceptible species/varieties.
B. Genetic Manipulation Avenues for Developing Stress Tolerance (Conventional/Molecular Breeding/Biotechnological)
(a) Breeding for Biotic Stress Tolerance
Screening of germplasm for resistance, utilization of the identified resistant lines as donors for recombination breeding or backcross breeding, interspecific crosses, mutation breeding and manipulation using propagation strategies are the widely used conventional strategies for the development of biotic stress tolerant cucurbit genotypes. Cucurbits are widely affected by viral diseases and among them bottle gourd is found to be moderately resistant to viral disease caused by CMV and yellow mosaic virus (ZYMV) (Provvidenti and Gonsalves, 1984; Provvidenti, 1995; Ling and Levi, 2007). It also displays resistance to fungal diseases like Fusarium wilt (Yetişir and Sari, 2003) and powdery mildew (Kousik et al., 2008) and has been exploited in its use as rootstock for watermelon (Yetişir and Sari, 2003; Keinath and Hassell, 2014).
Resistance to viruses as well as other pests and diseases has also been identified in wild or semi-domesticated types of bitter gourd (M. charantia var. muricata) (Asna, 2018). Previous studies also demonstrated the utility of mutation breeding in the development of biotic stress tolerant genotypes. Bitter gourd cultivar MDU 1 developed through mutation breeding from the landrace MC 013, displayed tolerance to pumpkin beetle, fruit fly and leaf spot diseases (Rajasekharan and Shanmugavelu, 1984). The reported cucurbit genotypes resistant to specific pathogens/pests are tabulated in Table 8.
(b) Breeding for Abiotic Stress Tolerance
Genomic/genetic resources and plant transformation protocols have recently been developed and standardized for cucurbits (Nanasato et al., 2013; Sun et al., 2017; Montero-Pau et al., 2018, 2017). The characterization of the wild relatives of cucurbits have aided in finding their potential use in breeding and other related crop improvement initiatives (e.g., Holdsworth et al., 2016). It was also interesting to note that many wild relatives have multiple stress tolerance capacities, both biotic and abiotic. Potential and documented use of wild cucurbits in breeding with special emphasis on possession of traits such as abiotic and biotic stress tolerance is tabulated in Table 9. It has also been reported that many of the wild cucurbits are under the threat of being endangered, demanding conservation interventions owing to their tolerance potentials (Khoury et al., 2020).
Concerted efforts have been made to identify and characterize the potential species and genotypes, among the different cucurbits, considered tolerant to drought or heat or a combination of both stresses (Saroj and Choudhary, 2020; Mkhize et al., 2021). The potential tolerant sources have been tabulated in Table 10.
The successful strategy for identifying the candidate tolerant sources and developing elite donors is inclined towards a physiogenetic approach including careful analysis of the key physiological traits distinctly critical for each stress (Figure 1) and subsequent characterization of the genetic basis for the respective trait manifestation. However, it is pertinent that there may be common physiological traits that can be capable enough to confer tolerance to multiple stresses. Abiotic stress tolerance, in particular, is a complex trait; the component primary, secondary (constitutive or induced) and integrative traits will have their distinct individual relevance under different stresses, along with their contributory significance.
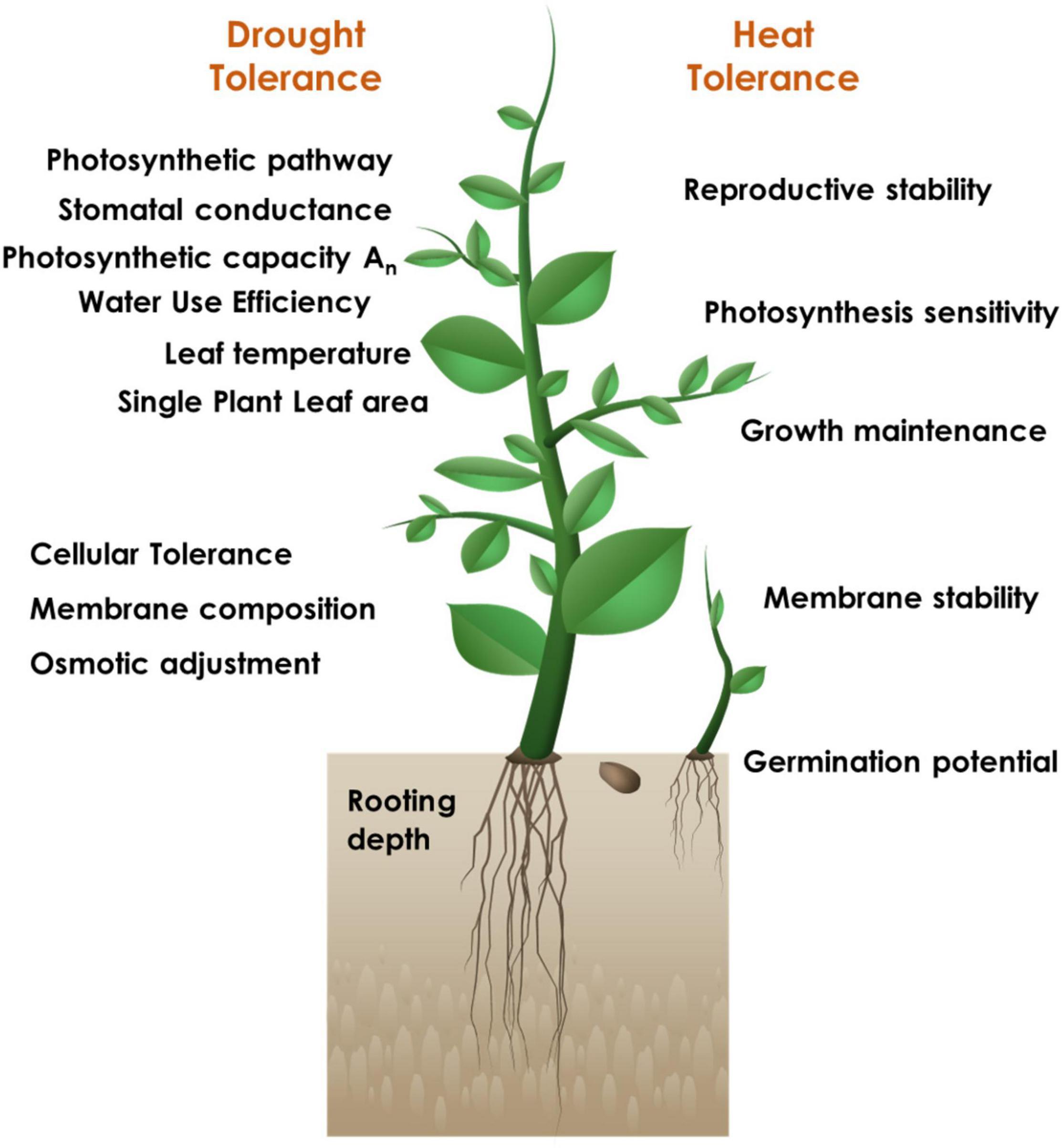
Figure 1. Physiological trait-based screening for identification of drought and heat stress tolerant sources.
(c) Molecular Breeding and Biotechnological Approaches for Crop Improvement in Cucurbits
Strategies adopted for development of stress tolerant genotypes in cucurbits including conventional breeding, propagation techniques, mitigation strategies etc. have contributed immensely to the successful cultivation of members of the cucurbit family. However, traditional approaches are often time consuming and restricted by the available variation in the gene pool. Biotechnological interventions can result in rapid and sustainable development of crop varieties having high quality and stress tolerance.
(i) Genome Sequencing, Mapping and Marker Assisted Selection
Genome sequencing facilitates all subsequent analyses of genome structure, organization and function. Genome sequences have been published for major cucurbit family members like cucumber (Huang et al., 2009; Osipowski et al., 2020), melon (Garcia-Mas et al., 2012; Ruggieri et al., 2018), water melon (Guo et al., 2013), zucchini (Montero-Pau et al., 2018; Xanthopoulou et al., 2019), C. maxima (Sun et al., 2017), C. moschata (Sun et al., 2017), bottle gourd (Wu et al., 2017), wax gourd (Xie et al., 2019) etc. Genomic information has facilitated the discovery of genes and pathways associated with several stress response pathways leading to the development of stress tolerant varieties or genotypes.
In cucumber, several quantitative trait loci (QTLs) associated with resistance to virus, fungi and bacteria have been mapped. QTLs associated with abiotic stress tolerance like cold, water stress, temperature (Dong et al., 2020; Liu et al., 2021c), drought, salt (Liu et al., 2021a) etc. have also been identified in cucumber which can be utilized in breeding programs. Phytophthora crown rot resistance in C. moschata. was detected on chromosome 4 (QtlPC-C04), 11 (QtlPC-C11), and 14 (QtlPC-C14) by bulk segregant analysis and potential linked markers for utilization in marker assisted selection (MAS) (Ramos et al., 2020). A genome-wide association study (GWAS) based on 5,330 single-nucleotide polymorphisms (SNPs) in bottle gourd accessions detected HG_GLEAN_10011803 to be likely the major-effect candidate gene for resistance against FW in bottle gourd (Yanwei et al., 2021). Wang Y. et al. (2018) identified three major-effect contributing QTLs for downy mildew resistance viz., dm5.1, dm5.2, and dm5.3 and a major-effect QTL pm5.1 for powdery mildew resistance in cucumber. CsGy5G015660, encoding a putative leucine-rich repeat receptor-like serine/threonine-protein kinase (RPK2), was identified as a strong powdery mildew resistance candidate gene in a Korean cucumber inbred line, by genome wide SNP profiling and corresponding RT-PCR analyses (Zhang C. et al., 2021).
SSR marker ECM230 linked to the major QTL in melon (Cucumis melo L.) was reported to be useful in selection for resistance to CCYV (Cucurbit chlorotic yellows virus) (Kawazu et al., 2018). Two additive QTLs affected the whitefly attack and a major QTL that reduces acceptance by Aphis gossypii and 10 genome locations on five linkage groups involved in resistance to hemipterans in melon have been identified (Boissot et al., 2010). In cucumber, resistance to Watermelon mosaic virus (WMV) is controlled by a single recessive gene designated as wmv02245 and was mapped to chromosome 6 (Chr.6) (Tian et al., 2016). The bottle gourd genome sequence has facilitated the mapping of Prs, conferring Papaya ring-spot virus (PRSV) resistance, on chromosome 1 and the potential of a CAPS marker tightly linked to the Prs locus in marker-assisted selection of PRSV resistance in bottle gourd has been demonstrated (Wu et al., 2017). Zhang et al. (2014) identified SSR17631 marker, which could be used to screen cucumber resources with Fusarium wilt resistance in molecular marker-assisted selection breeding. The identified QTLs and associated markers can be effectively utilized in screening, selection and gene pyramiding for multiple stress tolerance in cucurbits.
(ii) Transgenic Development for Crop Improvement
Majority of the transgenics developed in cucurbits are for development of virus resistance (Gaba et al., 2004). Transgenic watermelon carrying a single chimeric transgene comprising a silencer DNA from the partial N gene of Watermelon silver mottle virus (WSMoV) fused to the partial coat protein (CP) gene sequences of CMV, Cucumber green mottle mosaic virus (CGMMV) and WMV demonstrated that fusion of different viral CP gene fragments in transgenic watermelon contributed to multiple virus resistance via RNA-mediated post-transcriptional gene silencing (PTGS) (Lin et al., 2012). Transgenic cucumber and melon lines harboring a hairpin construct of the Zucchini yellow mosaic potyvirus (ZYMV) HC-Pro gene displayed resistance to systemic ZYMV infection (Leibman et al., 2011). Transgenic oriental melon carrying untranslatable chimeric DNA with partial CP sequences of ZYMV and PRSV caused RNA-mediated PTGS conferring high degrees of resistance to ZYMV and PRSV W in C. melo (Wu et al., 2010). Transgenic watermelon with resistance to CMV infection was developed by expressing artificial microRNAs that target CMV 2a/2b genes (Liu et al., 2016).
(iii) Non-transgenic Biotechnological Approaches
Heavy restrictions placed on genetically modified organisms (GMOs) have resulted in the adoption of non-transgenic approaches in crop plants. CRISPR/Cas9, the novel and efficient tool for genome editing, was used in cucumber for the disruption of the eIF4E for the development of virus-resistant plants without otherwise affecting the plant genome (Chandrasekaran et al., 2016). Use of CRISPR/Cas9-mediated gene modification for Clpsk1 loss-of-function in watermelon seedlings made them more resistant to infection by Fusarium oxysporum f. sp. niveum indicating its effectiveness for watermelon improvement (Zhang et al., 2020). Strategies using the ability of dsRNAs to activate the plant RNA silencing mechanism has also been exploited in cucurbits. Exogenous application of in vitro-produced dsRNA molecules derived from the HC-Pro and CP genes of ZYMV, conferred significant protection ZYMV in watermelon and cucumber (Kaldis et al., 2018).
C. Novel Stress Tolerance Pathways and Mechanisms- the “Omics” Way
The evolution of high throughput next generation sequencing technologies have aided in the generation of immense omics resources for unraveling the more complex stress acclimation responses in cucurbits as has been demonstrated in many other crop species in the past two decades. The Cucurbit Genome Database (CuGenDB) developed by the Fei Lab at Boyce Thompson Institute, United States, serves as the integral portal for functional and comparative genomics (Zheng et al., 2019). The team has added on more tools to their armory with the development of CucCAP (Grumet et al., 2020), which helps in harnessing genomic resources for disease resistance and management in cucurbit crops. The expression repertoire in terms of transcriptomic and proteomic studies have also found place in the cucurbit quest for tolerance to abiotic and biotic stresses. RNA sequencing attempts have been made to prospect genes involved in long-term waterlogging tolerance in cucumber, unraveling transcript abundance specified to “plant hormone signal transduction pathway” in the “environmental information processing” category (Kreska et al., 2021). Salt stress specific transcriptomic analysis revealed the differential regulation of genes associated with carbon metabolism, biosynthesis of amino acids, carbon fixation in photosynthesis, nitrogen metabolism and fatty acid degradation in cucumber (Jiang et al., 2020). This study assumes significance in the context of the role of H2S in alleviating salinity stress wherein, proteome analysis indicated differential regulation of proteins involved in sulfur metabolism such as Cysteine synthase 1, Glutathione S-transferase U25-like, Protein disulfide-isomerase, and Peroxidase 2 (Jiang et al., 2020). WRKY transcription factors were reported to regulate downy mildew resistance in cucumber as evidenced by the higher expression of pattern recognition receptor (PRR) proteins unravelled by transcriptome analysis (Gao et al., 2021). Phenylpropanoid biosynthesis pathway emerged as a key regulator of resistance to Corynespora cassiicola stress in cucumber as revealed by transcriptome and miRNA analysis (Wang X. et al., 2018). Sucrose biosynthesis and ABA signal transduction were reported to be the key molecular regulations under drought stress, specifically induced after 4 days of drought stress in cucumber (Wang M. et al., 2018). Organellar genome influence, with special emphasis to chloroplastic and mitochondrial genomes in regulation of multiple traits have been highlighted by interventions brought about by next generation sequencing and omics in cucumber (Cucumis sativus L.) (Pawełkowicz et al., 2016).
In Cucurbita pepo subsp. pepo, down regulation of SA precursor related enzyme, CpPAL (Phenyl ammonia lyase) was found to be associated with susceptibility, while defensin overexpression was found to be related to tolerance (Ayala-Doñas et al., 2021). Targeted metabolomics studies have been attempted to understand the response of cucumber to silver and silver nanoparticles (Zhang et al., 2018), sulfur (Liu et al., 2021b) and elevated atmospheric CO2 (Li et al., 2018). An interdisciplinary approach involving different fields of plant sciences to culminate in adopting ionomics as an integrated assessment of elemental accumulation, will hold potential because molybdenum and iron are reported to mutually govern their homeostasis in cucumber (Cucumis sativus) plants (Vigani et al., 2017; Pita-Barbosa et al., 2019).
D. Stress Mitigation Strategies for Cucurbits by Exogenous Amelioration
Cucurbits are a class of vegetables often grown in hot and dry tropics, making them vulnerable to the exposure of multiple stresses. The knowledge and information on the different stress adaptive traits and mechanisms have paved the way for employment of different biostimulants and chemical ameliorants for sustainable management of different stressors. Mycorrhizal associations in cucurbits have been proven to be beneficial both under optimal and stressful conditions. The symbiotic interaction with arbuscular mycorrhizal fungi (AMF) has profound influence when multiple stresses occur at the same time. There are commercial examples of mycorrhizal consortia such as MycoApply ®, a four-species consortium, which facilitates nutrient and water uptake1. A consortium of three plant growth-promoting rhizobacterium (PGPR) strains (Bacillus cereus AR156, Bacillus subtilis SM21, and Serratia sp. XY21), has been reported to confer systemic tolerance to drought stress in cucumber, by maintaining assimilation and growth vigor and offering protection against oxidative stress damage (Wang C. J. et al., 2012). Humic acid is a highly beneficial biostimulant used in different crops to stimulate shoot and root growth and enhance tolerance to stresses, which has also been demonstrated in cucumber to influence yield and mineral nutrient uptake under salinity stress exposure (Demir et al., 1999). Foliar spray of Moringa leaf extract was found to be beneficial in enhancing growth, harvest index, WUE, photosynthetic stability, osmoregulation and membrane stability in Cucurbita pepo under drought stress (Abd El-Mageed et al., 2017). Similarly, under salinity stress, seed treatment/irrigation with a bacterial consortia of Bacillus species, Bacillus pumilis, Trichoderma harzianum, Paenibacillus azotoformans, and Polymyxa plays a role in maintaining growth by regulating ion homeostasis in Cucurbita pepo (Yildirim et al., 2006). Soil amelioration with Ascophyllum nodosum was beneficial in Cucumis sativus against salinity stress, which helped in maintaining fruit yield (Demir et al., 1999). Resistance against Fusarium oxysporum induced wilt in cucumber was effectively enhanced by a combination treatment with GAWDA ® (an antioxidant formulation designed and patented in Egypt) and an AMF consortia (Elwakil et al., 2013), and exogenous nitrate nutrition operating through modulation of photorespiration (Sun et al., 2021).
Brassinosteroids (BR) are naturally occurring plant steroids with growth regulatory potential, which has been reported to impart chilling stress tolerance in cucumber (Cucumis sativus) by a chemico-genetic regulation of oxidative stress management. BR-induced activation of plasma membrane-bound NADPH oxidase (RBOH) results in the upregulation of signaling molecules in the form of H2O2, which has a role in activating subsequent stress response pathways (Xia et al., 2009). Exogenous application of 24-Epibrassinolide was found to alleviate the detrimental effects of root zone temperature fluctuations in cucumber seedlings by regulating hormonal and ion homeostasis (Anwar et al., 2018, 2019a,b; Anwar and Kim, 2020). Amelioration of chilling stress in cucumber seedlings by triadimefon (Feng et al., 2003), selenium (Hawrylak-Nowak et al., 2010), and SA (Kang and Saltveit, 2002) have also been reported. Drought tolerance was found to be enhanced in cucumber plants treated with natural carbon materials like shungite, which led to an increase in antioxidant potential thereby reducing cellular damage (Kim et al., 2019). Although there are many strategies employed to combat stress incidence in cucurbits, development of novel technologies in the form of ameliorative treatments can be effective, provided the right traits and target mechanisms come under the purview of the mitigation strategy.
Future Perspectives in Crop Improvement for Multiple Stress Tolerance in Cucurbits
Cucurbits are vulnerable to simultaneous exposure of multiple stressors and hence interventions at various levels are imperative to achieve sustained crop production, even under adverse climatic conditions. The choice of the apt component trait for achieving tolerance to a stress episode is the key towards effective crop improvement. Crop improvement relies on the available diversity or creation of diversity as the source of desirable traits, including abiotic and biotic stress resistance. Genetic diversity is facing serious threat due to habitat loss owing to human intervention and climate change. Efforts for collection and conservation of cucurbit germplasm including related species, distant species, wild relatives and landraces needs to be expedited for their utilization in breeding programs, biotechnological approaches and propagation methods. Grafting has evolved as a relatively cheap and quick option for managing the biotic and abiotic stressors. Although a good number of commercial rootstocks have been identified, particularly in the temperate regions, these attempts are very rare in the tropical regions. Hence, systematic testing of genotypes and wild relatives of cucurbits for their potential use as rootstocks against multiple stresses should be a research priority. Rootstock breeding in cucurbits leading to development of vigorous intra and interspecific hybrid rootstocks conferring tolerance to multiple stresses needs urgent attention. Hence, it is very crucial to screen for the right plant traits/characters/mechanisms for employing any of the prospective strategies discussed in this review. A comprehensive account of the different plant adaptive traits/mechanisms critical under each stressor (Figure 2) can aid in the adoption of the correct trait(s) combination and the approach in the event of a multiple stress exposure. With climate change posing novel, varied and often multiple stresses to the crops, strategies for screening for multiple stress resistance needs to be evolved and employed. Even a moderate level of resistance for multiple stress factors can confer tolerance to stresses through cross-talk between various pathways and mitigate their damaging effect. Targeted manipulation of tolerance traits will be possible if a better understanding of the combined stress effects is realized. Breeding approaches should focus on gene pyramiding for multiple stress tolerance in cucurbits through a combination of conventional and biotechnological approaches. Robust markers, genomic prediction tools and phenotyping facilities need to be developed for rapid screening and identification of stress tolerant genotypes in cucurbits. Previous attempts in this direction in vegetable crops like onion, including gene prospecting for drought tolerance (Kutty et al., 2012) and molecular marker identification by bulk segregant analysis of drought tolerance in F2 population (Kutty et al., 2013), concerted with physiological, morphological and biochemical characterization of drought stress response in different onion cultivars (Kutty et al., 2014) have been promising. Genes/gene products regulating multiple molecular mechanisms thus identified can be combined together by novel gene stacking approaches to alter multiple traits required for combined stress tolerance, as has been demonstrated in model crop plants (Vemanna et al., 2013; Pruthvi et al., 2014; Parvathi et al., 2015). Multiple trait manipulation employing a ‘super regulatory gene/QTL’ capable of coordinating numerous trait-related genes can help in developing genotypes tolerant to multiple stresses (Parvathi and Nataraja, 2017; Parvathi et al., 2021). Such initiatives would help in congregating the indispensable traits in an ideal genotype/accession, that would be critical for crop acclimation under a combination of stress challenges. Advanced gene editing technologies can be of great value in the view of multigenic control over tolerance in a multiple stress scenario. A careful and concerted trait-specific approach for each stress combination is imperative to achieve optimal realizable crop performance.
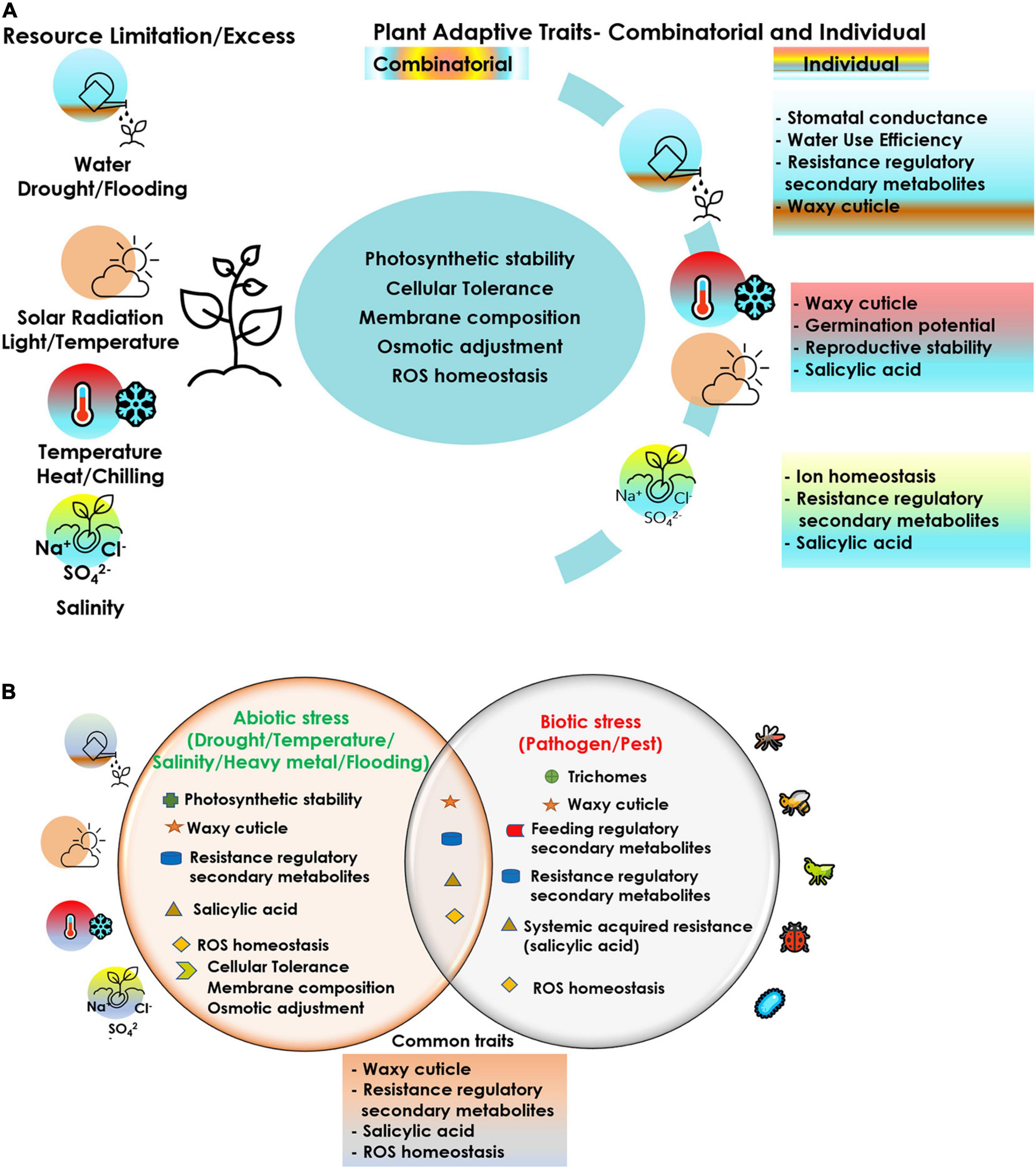
Figure 2. Plant adaptive traits/mechanisms critical under each stressor and prospective trait-stress combinations under co-occurrence of different stresses in cucurbits; (A) abiotic–abiotic, (B) abiotic–biotic.
Author Contributions
All authors listed have made an equal, direct, and intellectual contribution to the work, and approved it for publication.
Conflict of Interest
The authors declare that the research was conducted in the absence of any commercial or financial relationships that could be construed as a potential conflict of interest.
Publisher’s Note
All claims expressed in this article are solely those of the authors and do not necessarily represent those of their affiliated organizations, or those of the publisher, the editors and the reviewers. Any product that may be evaluated in this article, or claim that may be made by its manufacturer, is not guaranteed or endorsed by the publisher.
Footnotes
- ^ https://www.valentbiosciences.com/soilhealth/solutions/abiotic-stress-mitigation-for-cucurbit-vegetables/
References
Abd El-Mageed, T. A., Semida, W. M., and Rady, M. M. (2017). Moringa leaf extract as biostimulant improves water use efficiency, physio-biochemical attributes of squash plants under deficit irrigation. Agric. Water Manag. 193, 46–54. doi: 10.1016/j.agwat.2017.08.004
Abdul Aziz, M., Sabeem, M., Mullath, S. K., Brini, F., and Masmoudi, K. (2021). Plant group II LEA proteins: intrinsically disordered structure for multiple functions in response to environmental stresses. Biomolecules 11:1662. doi: 10.3390/biom11111662
Adkins, S., Webb, S. E., Baker, C. A., and Kousik, C. S. (2008). Squash vein yellowing virus detection using nested polymerase chain reaction demonstrates that the cucurbit weed Momordica charantia is a reservoir host. Plant Dis. 92, 1119–1123. doi: 10.1094/PDIS-92-7-1119
Alao, F. O., Adebayo, T. A., Odewole, A. F., and Emmanuel, O. O. (2017). Comparative assessment of insect pests population densities of three selected cucurbit crops. Acta Fytotechn Zootechn 20, 78–83. doi: 10.15414/afz.2017.20.04.78-83
Al-Busaidi, A., Ahmed, M., and Chikara, J. (2012). The impact of heat and water stress conditions on the growth of the biofuel plant Jatropha curcas. Int. J. Environ. Stud. 69, 273–288. doi: 10.1080/00207233.2012.663204
Al-Sadi, A. M., Al-Said, F. A., Al-Kaabi, S. M., Al-Quraini, S. M., Al-Mazroui, S. S., Al-Mahmooli, I. H., et al. (2011). Occurrence, characterization and management of fruit rot of immature cucumbers under greenhouse conditions in oman. Phytopathol. Mediter. 50, 421–429.
Al-Sadi, A. M., Deadman, M. L., Al-Said, F. A., and Khan, I. (2008). First report of pythium splendens associated with severe wilt of muskmelon (Cucumis melo) in oman. Plant Dis. 92:2. doi: 10.1094/PDIS-92-2-0313C
Anwar, A., and Kim, J.-K. (2020). Transgenic breeding approaches for improving abiotic stress tolerance: recent progress and future perspectives. Int. J. Mol. Sci. 2020:2695. doi: 10.3390/ijms21082695
Anwar, A., Bai, L., Miao, L., Liu, Y., Li, S., Yu, X., et al. (2018). 24-epibrassinolide ameliorates endogenous hormone levels to enhance low-temperature stress tolerance in cucumber seedlings. Int. J. Mol. Sci. 19:2497. doi: 10.3390/ijms19092497
Anwar, A., Di, Q., Yan, Y., He, C., Li, Y., and Yu, X. (2019a). Exogenous 24-epibrassinolide alleviates the detrimental effects of suboptimal root zone temperature in cucumber seedlings. Arch. Agron. Soil Sci. 65, 1927–1940. doi: 10.1080/03650340.2019.1582768
Anwar, A., Li, Y., He, C., and Yu, X. (2019b). 24-epibrassinolide promotes NO3– and NH4+ ion flux rate and NRT1 gene expression in cucumber under suboptimal root zone temperature. BMC Plant Biol. 19:225.
Arocha, Y., Vigheri, N., Nkoy-Florent, B., Bakwanamaha, K., Bolomphety, B., Kasongo, M., et al. (2008). First report of the identification of moroccan watermelon mosaic virus in papaya in Democratic Republic of Congo. Plant Pathol. 57, 387–387. doi: 10.1111/j.1365-3059.2007.01658.x
Asna, K. C. (2018). Characterization and Distant Hybridization for Biotic Stress Tolerance in Bitter Gourd (Momordica charantia L.). Ph. D., Thesis.
Ayala-Doñas, A., Gómez, P., and de Cara-García, M. (2021). Gene expression in cucurbita spp. root and crown during phytophthora capsici infection. Plants 10:2718. doi: 10.3390/plants10122718
Ayo-John, E. I., Olorunmaiye, P. M., Odedara, O. O., Dada, O. B., Abiola, K. O., and Oladokun, J. O. (2014). Assessment of field-grown cucurbit crops and weeds within farms in South-west Nigeria for viral diseases. Notulae Sci. Biol. 6, 321–325. doi: 10.15835/nsb639315
Azadi, P., Otang, N. V., Supaporn, H., Khan, R. S., Chin, D. P., Nakamura, I., et al. (2011). Increased resistance to cucumber mosaic virus (CMV) in Lilium transformed with a defective CMV replicase gene. Biotechnol. Lett. 33, 1249–1255. doi: 10.1007/s10529-011-0550-7
Bains, S. S., and Prakash, V. (1985). Susceptibility of different cucurbits to pseudoperonospora cubensis under natural and artificial epiphytotic conditions. Indian Phytopathol. 38, 138–139.
Balal, R. M., Shahid, M. A., Javaid, M. M., Iqbal, Z., Anjum, M. A., Garcia-Sanchez, F., et al. (2016). The role of selenium in amelioration of heat-induced oxidative damage in cucumber under high temperature stress. Acta Physiol. Plant 38, 1–14.
Bayoumi, Y., Abd-Alkarim, E., El-Ramady, H., El-Aidy, F., Hamed, E. S., Taha, N., et al. (2021). Grafting improves fruit yield of cucumber plants grown under combined heat and soil salinity stresses. Horticulturae 7:61. doi: 10.3390/horticulturae7030061
Berg, J. A. (2020). Cucumber Mildew Resistance: Identification of Cucumber Genes Involved in Susceptibility and Resistance to Powdery and Downy Mildew Ph. D., Thesis. Wageningen University & Research.
Bhargava, R., Gurjar, K., Haldhar, S. M., Singh, R. S., and Sharma, B. D. (2016). Impact of water stress on photosynthesis and secondary metabolites in snap melon and musk melon. Indian J. Arid Hortic. 11, 30–35.
Bhat, N. A., Bhat, K. A., Zargar, M. Y., Teli, M. A., Nazir, M., and Zargar, S. M. (2010). Current status of angular leaf spot (Pseudomonas syringae pv. lachrymans) of cucumber: a review. Int. J. Curr. Res. 8, 1–11.
Bikdeloo, M., Colla, G., Rouphael, Y., Hassandokht, M. R., Soltani, F., Salehi, R., et al. (2021). Morphological and physio-biochemical responses of watermelon grafted onto rootstocks of wild watermelon [Citrullus colocynthis (L.) Schrad] and commercial interspecific cucurbita hybrid to drought stress. Horticulturae 7:359. doi: 10.3390/horticulturae7100359
Block, C. C., and Reitsma, K. R. (2005). Powdery mildew resistance in the US national plant germplasm system cucumber collection. HortScience 40, 416–420. doi: 10.21273/hortsci.40.2.416
Block, C. C., and Shepherd, L. M. (2008). Long-term survival and seed transmission of Acidovorax avenae subsp. citrulli in melon and watermelon seed. Plant Health Prog. 9:36.
Boissot, N., Thomas, S., Sauvion, N., Marchal, C., Pavis, C., and Dogimont, C. (2010). Mapping and validation of QTLs for resistance to aphids and whiteflies in melon. Theor. Appl. Genet. 121, 9–20. doi: 10.1007/s00122-010-1287-8
Cargnelutti, D., Tabaldi, L. A., Spanevello, R. M., de Oliveira Jucoski, G., Battisti, V., Redin, M., et al. (2006). Mercury toxicity induces oxidative stress in growing cucumber seedlings. Chemosphere 65, 999–1006. doi: 10.1016/j.chemosphere.2006.03.037
Chandrasekaran, J., Brumin, M., Wolf, D., Leibman, D., Klap, C., Pearlsman, M., et al. (2016). Development of broad virus resistance in non-transgenic cucumber using CRISPR/Cas9 technology. Mol. Plant Pathol. 17, 1140–1153. doi: 10.1111/mpp.12375
Chen, Q., Yu, G., Wang, X., Meng, X., and Lv, C. (2021). Genetics and resistance mechanism of the cucumber (Cucumis sativus L.) against powdery mildew. J. Plant Growth Regul. 40, 147–153.
Chevilly, S., Dolz-Edo, L., Martínez-Sánchez, G., Morcillo, L., Vilagrosa, A., López-Nicolás, J. M., et al. (2021). Distinctive traits for drought and salt stress tolerance in melon (Cucumis melo L.). Front. Plant Sci. 12:777060. doi: 10.3389/fpls.2021.777060
Chintha, R. M., Das, R. K., and Mandal, S. K. (2002). Pests and beneficial arthropods occurring on pointed gourd, Trichosanthes dioica roxb.(Cucurbitaceae). J. Interacad. 6, 174–179.
Chojak-Koźniewska, J., Kuźniak, E., and Zimny, J. (2018). The effects of combined abiotic and pathogen stress in plants: insights from salinity and Pseudomonas syringae pv lachrymans interaction in cucumber. Front. Plant Sci. 9:1691. doi: 10.3389/fpls.2018.01691
Choudhary, B. R., Haldhar, S. M., Maheshwari, S. K., and Bhargava, R. (2016). Sources of variation in sponge gourd breeding. J. Agric. Eng. Food Technol. 3:58.
Choudhary, B. R., Haldhar, S. M., Maheshwari, S. K., Bhargava, R., and Sharma, S. K. (2015). Phytochemicals and antioxidants in watermelon (Citrullus lanatus) genotypes under hot arid region. Indian J. Agric. Sci. 85, 414–417.
Cohen, R., Burger, Y., Horev, C., Koren, A., and Edelstein, M. (2007). Introducing grafted cucurbits to modern agriculture: the Israeli experience. Plant Dis. 91, 916–923. doi: 10.1094/PDIS-91-8-0916
Colla, G., Roupahel, Y., Cardarelli, M., and Rea, E. (2006). Effect of salinity on yield, fruit quality, leaf gas exchange, and mineral composition of grafted watermelon plants. HortScience 41, 622–627.
Colla, G., Rouphael, Y., Jawad, R., Kumar, P., Rea, E., and Cardarelli, M. (2013). The effectiveness of grafting to improve NaCl and CaCl2 tolerance in cucumber. Sci. Hortic. 164, 380–391. doi: 10.1016/j.scienta.2013.09.023
Colla, G., Rouphael, Y., Leonardi, C., and Bie, Z. (2010). Role of grafting in vegetable crops grown under saline conditions. Sci. Hortic. 127, 147–155. doi: 10.1016/j.scienta.2010.08.004
Colla, G., Rouphael, Y., Rea, E., and Cardarelli, M. (2012). Grafting cucumber plants enhance tolerance to sodium chloride and sulfate salinization. Sci. Hortic. 135, 177–185. doi: 10.1016/j.scienta.2011.11.023
Colle, M., Straley, E. N., Makela, S. B., Hammar, S. A., and Grumet, R. (2014). Screening the cucumber plant introduction collection for young fruit resistance to Phytophthora capsici. HortScience 49, 244–249. doi: 10.21273/hortsci.49.3.244
Crino, P., Bianco, C. L., Rouphael, Y., Colla, G., Saccardo, F., and Paratore, A. (2007). Evaluation of rootstock resistance to fusarium wilt and gummy stem blight and effect on yield and quality of a grafted ‘inodorus’ melon. HortScience 42, 521–525. doi: 10.21273/hortsci.42.3.521
Dai, L. S., Zhou, X. D., Kausar, S., Abbas, M. N., Wu, L., and Zhou, H. L. (2018). Mitochondrial genome of Diaphania indica (Saunders)(Lepidoptera: Pyraloidea) and implications for its phylogeny. Int. J. Biol. Macromol. 108, 981–989. doi: 10.1016/j.ijbiomac.2017.11.011
Davis, A. R., Perkins-Veazie, P., Sakata, Y., Lopez-Galarza, S., Maroto, J. V., Lee, S. G., et al. (2008). Cucurbit grafting. Crit. Rev. Plant Sci. 27, 50–74.
Deadman, M. L., Al Sadi, A. M., Al Said, F. A., and Al Maawali, Q. (2009). The use of cucurbit hybrid rootstocks in the management of Pythium-induced damping-off of cucumber seedlings. Int. Sym. Cucurbits 871, 483–490. doi: 10.17660/actahortic.2010.871.67
Debnath, R., Mobarak, S. H., Mitra, P., and Barik, A. (2020). Comparative performance and digestive physiology of Diaphinia indica (Lepidoptera: Crambidae) on Trichosanthes anguina (Cucurbitaceae) cultivars. Bull. Entomol. Res. 110, 756–766. doi: 10.1017/S0007485320000255
Demir, K., Günes, A., Inal, A., and Alpaslan, M. (1999). E_ects of humic acids on the yield and mineral nutrition of cucumber (Cucumis sativus, L.) grown with different salinity levels. Acta Hortic. 492, 95–104. doi: 10.17660/actahortic.1999.492.11
Dhillon, N. P., Sanguansil, S., Srimat, S., Schafleitner, R., Manjunath, B., Agarwal, P., et al. (2018). Cucurbit powdery mildew-resistant bitter gourd breeding lines reveal four races of Podosphaera xanthii in Asia. HortScience 53, 337–341. doi: 10.21273/hortsci12545-17
Dong, S., Zhang, S., Wei, S., Liu, Y., Li, C., Bo, K., et al. (2020). Identification of quantitative trait loci controlling high-temperature tolerance in cucumber (Cucumis sativus L.) seedlings. Plants 9:1155. doi: 10.3390/plants9091155
Ebert, A. W., Drummond, E. B. M., Giovannini, P., and van Zonneveld, M. (2021). A Global Conservation Strategy for Crops in the Cucurbitaceae Family. Bonn, Germany: Global Crop Diversity Trust.
Elwakil, M. A., Baka, Z. A., Soliman, H. M., and Sadek, M. S. (2013). A modern tactic for reducing the biotic stress on cucumber plants caused by Fusarium oxysporum. Plant Pathol. J. 12, 26–31. doi: 10.3923/ppj.2013.26.31
Fan, H. F., Ding, L., Du, C. X., and Wu, X. (2014). Effect of short-term water deficit stress on antioxidative systems in cucumber seedling roots. Bot. Stud. 55, 1–7. doi: 10.1186/s40529-014-0046-6
Farrag, E. S., and Moharam, M. H. (2012). Pathogenic fungi transmitted through cucumber seeds and safely elimination by application of peppermint extract and oil. Notulae Sci. Biol. 4, 83–91. doi: 10.15835/nsb437969
Feng, Z., Guo, A., and Feng, Z. (2003). Amelioration of chilling stress by triadimefon in cucumber seedlings. Plant Growth Regul. 39, 277–283. doi: 10.1023/A:1022881628305
Fish, W. W., Bruton, B. D., and Popham, T. W. (2012) Cucurbit host range of Myrothecium roridum isolated from watermelon. Am. J. Plant Sci. 3, 353-359. doi: 10.4236/ajps.2012.33042
Flores, F. B., Sanchez-Bel, P., Estañ, M. T., Martinez-Rodriguez, M. M., Moyano, E., Morales, B., et al. (2010). The effectiveness of grafting to improve tomato fruit quality. Sci. Hortic. 125, 211–217. doi: 10.1016/j.scienta.2010.03.026
Fu, Q., Zhang, X., Kong, Q., Bie, Z., and Wang, H. (2018). Grafting onto pumpkin rootstock is an efficient alternative to improve melon tolerance to NaCl stress. Eur. J. Hortic. Sci. 83, 337–344. doi: 10.17660/ejhs.2018/83.6.1
Fu, X., Feng, Y.-Q., Zhang, X.-W., Zhang, Y.-Y., Bi, H.-G., and Ai, X.-Z. (2021). Salicylic acid is involved in rootstock–scion communication in improving the chilling tolerance of grafted cucumber. Front. Plant Sci. 12:693344. doi: 10.3389/fpls.2021.693344
Fuchs, M., and Gonsalves, D. (1995). Resistance of transgenic hybrid squash ZW-20 expressing the coat protein genes of Zucchini yellow mosaic virus and watermelon mosaic virus 2 to mixed infections by both potyviruses. Bio. Tech. 13, 14466–14473.
Fuchs, M., and Gonsalves, D. (1997). “Genetic engineering,” in Environmentally Safe Approcahes to Crop Disease Control, eds N. A. Rechcigl and J. E. Rechcigl (Boca Raton, FL: CRC Press), 333–368.
Furtana, G. B., and Tipirdamaz, R. (2010). Physiological and antioxidant response of three cultivars of cucumber (Cucumis sativus L.) to salinity. Turkish J. Biol. 34, 287–296.
Gaba, V., Zelcer, A., and Gal-On, A. (2004). Cucurbit biotechnology-the importance of virus resistance. Vitro Cell. Dev. Biol. Plant 40, 346–358. doi: 10.1079/ivp2004554
Gannibal, P. B. (2011). Alternaria cucumerina causing leaf spot of pumpkin newly reported in North Caucasus (Russia). New Dis. Rep. 23, 2044–2588.
Gao, X., Guo, P., Wang, Z., Chen, C., and Ren, Z. (2021). Transcriptome profiling reveals response genes for downy mildew resistance in cucumber. Planta 253, 1–18. doi: 10.1007/s00425-021-03603-6
Garcia-Mas, J., Benjak, A., Sanseverino, W., Bourgeois, M., Mir, G., González, V. M., et al. (2012). The genome of melon (Cucumis melo L.). Proc. Natl. Acad. Sci. U.S.A. 109, 11872–11877.
Grumet, R., Fei, Z., Levi, A., Mazourek, M., McCreight, J. D., Schultheis, J., et al. (2020). The CucCAP project: leveraging applied genomics to improve disease resistance in cucurbit crops. Acta Hortic. 1294, 91–104. doi: 10.17660/actahortic.2020.1294.12
Guan, W., Zhao, X., Dickson, D. W., Mendes, M. L., and Thies, J. (2014). Root-knot nematode resistance, yield, and fruit quality of specialty melons grafted onto Cucumis metulifer. HortScience 49, 1046–1051. doi: 10.1002/jsfa.7050
Guo, S., Zhang, J., Sun, H., Salse, J., Lucas, W. J., Zhang, H., et al. (2013). The draft genome of watermelon (Citrullus lanatus) and resequencing of 20 diverse accessions. Nat. Genet. 45, 51–58. doi: 10.1038/ng.2470
Gupta, N., Rathore, M., Goyary, D., Khare, N., Anandhan, S., Pande, V., et al. (2012). Marker free transgenic cucumber expressing Arabidopsis cbf1 gene confers chilling stress tolerance. Biol. Plant. 56, 57–63. doi: 10.1007/s10535-012-0016-3
Haber, A. I., Wallingford, A. K., Grettenberger, I. M., Ramirez Bonilla, J. P., Vinchesi-Vahl, A. C., and Weber, D. C. (2021). Striped cucumber beetle and western striped cucumber beetle (Coleoptera: Chrysomelidae). J. Int. Pest Manage. 12:1.
Haldhar, S. M. (2013). First Record of the Flower Beetles, Mylabris macilenta, Anthicus crinitus and Anthrenus subclaviger in Watermelon in arid Region of Rajasthan. Bikaner: ICAR-CIAH News Letter, 2–3.
Haldhar, S. M. (2016). New Report of Beet Armyworm, Spodoptera Litura in Ridge Gourd (Luffa Acutangula). Bikaner: ICAR-CIAH News Letter.
Haldhar, S. M., Choudhary, B. R., Bhargava, R., and Sharma, S. K. (2014). Development of an organic integrated pest management (IPM) module against insect-pests of muskmelon in arid region of Rajasthan, India. J. Exp. Biol. Agric. Sci. 2, 19–24.
Haldhar, S. M., Samadia, D. K., and Choudhary, B. R. (2018). Biotic stress (insect) of cucurbits in arid region of Rajasthan: a review. JOJ Hortic. Arboric. 1:555570.
Haldhar, S. M., Samadia, D. K., Bhargava, R., and Singh, D. (2017). Host plant genotypes determine bottom-up effect of Cucumis melo var. callosus against melon fruit fly. Crop Protect. 98, 157–165. doi: 10.1016/j.cropro.2017.03.028
Hasanuzzaman, M., Hossain, M. A., da Silva, J. A. T., and Fujita, M. (2012). “Plant response and tolerance to abiotic oxidative stress: antioxidant defense is a key factor,” in Crop Stress and Its Management: Perspectives and Strategies, eds B. Venkateswarlu, A. Shanker, C. Shanker, and M. Maheswari (Dordrecht: Springer), 261–315. doi: 10.1007/978-94-007-2220-0_8
Hasanuzzaman, M., Nahar, K., and Fujita, M. (2013). Extreme temperature responses, oxidative stress and antioxidant defense in plants. Abiotic Stress Plant Res. Appl. Agric. 13, 169–205.
Hawrylak-Nowak, B. (2009). Beneficial effects of exogenous selenium in cucumber seedlings subjected to salt stress. Biol. Trace Element Res. 132, 259–269. doi: 10.1007/s12011-009-8402-1
Hawrylak-Nowak, B., Matraszek, R., and Szyma’nska, M. (2010). Selenium modifies the effect of short-term chilling stress on cucumber plants. Biol. Trace Elem. Res. 138, 307–315. doi: 10.1007/s12011-010-8613-5
Hazzouri, K. M., Khraiwesh, B., Amiri, K., Pauli, D., Blake, T., Shahid, M., et al. (2018). Mapping of HKT1; 5 gene in barley using GWAS approach and its implication in salt tolerance mechanism. Front. Plant Sci. 9:156. doi: 10.3389/fpls.2018.00156
Holdsworth, W. L., LaPlant, K. E., Bell, D. C., Jahn, M. M., and Mazourek, M. (2016). Cultivar-based introgression mapping reveals wild species-derived Pm-0, the major powdery mildew resistance locus in squash. PLoS One 11:e0167715. doi: 10.1371/journal.pone.0167715
Holmes, G. J., Ojiambo, P. S., Hausbeck, M. K., Quesada-Ocampo, L., and Keinath, A. P. (2015). Resurgence of cucurbit downy mildew in the United States: a watershed event for research and extension. Plant Dis. 99, 428–441. doi: 10.1094/PDIS-09-14-0990-FE
Hosseinzade, S., Izadi, H., Namvar, P., and Samih, M. A. (2014). Biology, temperature thresholds, and degree-day requirements for development of the cucumber moth, Diaphania indica, under laboratory conditions. J. Insect Sci. 14:61. doi: 10.1093/jis/14.1.61
Hu, B., Li, D., Liu, X., Qi, J., Gao, D., Zhao, S., et al. (2017). Engineering non-transgenic gynoecious cucumber using an improved transformation protocol and optimized CRISPR/Cas9 system. Mol. Plant 10, 1575–1578. doi: 10.1016/j.molp.2017.09.005
Huang, S., Li, R., Zhang, Z., Li, L. I., Gu, X., Fan, W., et al. (2009). The genome of the cucumber. Cucumis sativus L. Nat. Genet. 41, 1275–1281.
Huang, Y., Bie, Z., He, S., Hua, B., Zhen, A., and Liu, Z. (2010). Improving cucumber tolerance to major nutrients induced salinity by grafting onto Cucurbita ficifolia. Environ. Exp. Bot. 69, 32–38. doi: 10.1016/j.envexpbot.2010.02.002
Huang, Y., Cao, H., Yang, L., Chen, C., Shabala, L., Xiong, M., et al. (2019). Tissue-specific respiratory burst oxidase homolog-dependent H2O2 signaling to the plasma membrane H+-ATPase confers potassium uptake and salinity tolerance in Cucurbitaceae. J. Exp. Bot. 70, 5879–5893. doi: 10.1093/jxb/erz328
Islam, S., Munshi, A. D., Mandal, B., Kumar, R., and Behera, T. K. (2010). Genetics of resistance in Luffa cylindrica roem. against tomato leaf curl New Delhi virus. Euphytica 174, 83–89. doi: 10.1007/s10681-010-0138-7
Jalali, M. A., Gharaei, A. M., Ziaaddini, M., Jalali, M., and Frerot, B. (2019). Oviposition preference and olfactory response of Diaphania indica (Lepidoptera: Pyralidae) to volatiles of uninfested and infested cucurbitaceous host plants. Eur. J. Entomol. 116, 392–401. doi: 10.14411/eje.2019.040
Jiang, J., Ren, X., Li, L., Hou, R., Sun, W., Jiao, C., et al. (2020). H2S regulation of metabolism in cucumber in response to salt-stress through transcriptome and proteome analysis. Front. Plant Sci. 2020:1283. doi: 10.3389/fpls.2020.01283
Kaldis, A., Berbati, M., Melita, O., Reppa, C., Holeva, M., Otten, P., et al. (2018). Exogenously applied dsRNA molecules deriving from the Zucchini yellow mosaic virus (ZYMV) genome move systemically and protect cucurbits against ZYMV. Mol. Plant Pathol. 19, 883–895. doi: 10.1111/mpp.12572
Kang, H. M., and Saltveit, M. E. (2002). Chilling tolerance of maize cucumber and rice seedling leaves and roots are differentially affected by salicylic acid. Physiol. Plant. 115, 571–576. doi: 10.1034/j.1399-3054.2002.1150411.x
Kaur, M., Varalakshmi, B., and Pitchaimuthu, M. (2021). Screening luffa germplasm and advanced breeding lines for resistance to tomato leaf curl New Delhi virus. J. Gen. Plant Pathol. 87, 287–294. doi: 10.1007/s10327-021-01010-z
Kawazu, Y., Shimomura, K., Maeda, S., Yamato, Y., Ueda, S., Okuda, S., et al. (2018). QTL mapping for resistance to cucurbit chlorotic yellows virus in melon (Cucumis melo L.). Euphytica 214, 1–11.
Keinath, A. P., and Hassell, R. L. (2014). Control of fusarium wilt of watermelon by drafting onto bottle gourd or interspecific hybrid squash despite colonization of rootstocks by Fusarium. Plant Dis. 98, 255–266. doi: 10.1094/PDIS-01-13-0100-RE
Kreska, K., Szcześniak, M. W., Makałowska, I., and Czernicka, M. (2021). Long-term waterlogging as factor contributing to hypoxia stress tolerance enhancement in cucumber: comparative transcriptome analysis of waterlogging sensitive and tolerant accessions. Genes 12:189. doi: 10.3390/genes12020189
Khan, A. S., and Chaudhry, N. Y. (2006). Peroxidase activity (POX) in some cucurbits under the stress of mercury and lead. J. Food Agric. Environ. 4:274.
Khan, A. S., and Chaudhry, N. Y. (2010). Florigenic effects of IAA for improving pistillate and staminate flowering in some cucurbits under Pb stress. Pakistan J. Bot. 42, 1835–1840.
Khan, J., Yang, Y., Fu, Q., Shao, W., Wang, J., Shen, L., et al. (2020). Screening of watermelon varieties for lead tolerance at the seedling stage. HortScience 55, 858–869. doi: 10.21273/hortsci14855-20
Khan, M. M. H., Alam, M. Z., Rahman, M. M., Miah, M. I. H., and Hossain, M. M. (2012). Influence of weather factors on the incidence and distribution of pumpkin beetle infesting cucurbits. Bangl. J. Agric. Res. 37, 361–367. doi: 10.3329/bjar.v37i2.11241
Khanzada, B., Shoukat, U., Khanzada, K. K., and Lodhi, N. (2021). Survival capability and effect of selected insecticides against pumpkin caterpillar, Diaphania indica on watermelon. J. Entomol. Zool. Stud. 9, 1–5.
Khoury, C. K., Carver, D., and Kates, H. R. (2020). Distributions, conservation status, and abiotic stress tolerance potential of wild cucurbits (Cucurbita L.). Plants People Planet 2, 269–283. doi: 10.1002/ppp3.10085
Kim, T. Y., Sang-Hyo, L., Hara, K., and Seung-Yop, L. (2019). Enhancement of drought tolerance in cucumber plants by natural carbon materials. Plants 11:446. doi: 10.3390/plants8110446
Kinjo, K., and Arakaki, N. (2002). Effect of temperature on development and reproductive characteristics of Diaphania indica (Saunders)(Lepidoptera: Pyralidae). Appl. Entomol. Zool. 37, 141–145. doi: 10.1303/aez.2002.141
Kousik, C. S., Donahoo, R. S., and Hassell, R. (2012). Resistance in watermelon rootstocks to crown rot caused by Phytophthora capsici. Crop Protect. 39, 18–25. doi: 10.1016/j.cropro.2012.04.004
Kousik, C. S., Levi, A., Ling, K., and Wechter, W. P. (2008). Potential sources of resistance to cucurbit powdery mildew in U.S. plant introductions of bottle gourd. HortScience 43, 1359–1364. doi: 10.21273/hortsci.43.5.1359
Kudela, V. (2009). Potential impact of climate change on geographic distribution of plant pathogenic bacteria in central Europe. Plant Prot. Sci. 45, S27–S32.
Kumar, P., Khapte, P. S., Saxena, A., Singh, A., Panwar, N. R., and Kumar, P. (2019). Intergeneric grafting for enhanced growth, yield and nutrient acquisition in greenhouse cucumber during winter. J. Environ. Biol. 40, 295–301. doi: 10.22438/jeb/40/3/mrn-918
Kurtar, E. S. (2010). Modelling the effect of temperature on seed germination in some cucurbits. Afr. J. Biotechnol. 9, 1343–1353.
Kutty, M. S., Gowda, R. V., and Rao, N. K. S. (2014). Genotypic variability for physiological, biochemical and morphological responses to drought stress in onion (Allium cepa L.). Trop. Agric 41, 40202–40218.
Kutty, M. S., Saiprasad, G. V. S., Gowda, R. V., and Anand, L. (2012). Isolation and characterization of cDNA clones in Allium cepa L.(onion) corresponding to genes expressed in drought tolerance. Trop. Agric. 41, 10007–10011.
Kutty, M. S., Saiprasad, G. V. S., Gowda, R. V., and Anand, L. (2013). Phenotypic characterization and bulked segregant analysis of drought tolerance in F2 population of onion (Allium cepa L.). Trop. Agric. 90, 129–139.
Lai, Y., Shen, D., Zhang, W., Zhang, X., Qiu, Y., Wang, H., et al. (2018). Temperature and photoperiod changes affect cucumber sex expression by different epigenetic regulations. BMC Plant Biol. 18:268. doi: 10.1186/s12870-018-1490-3
Lebeda, A., and Cohen, Y. (2011). Cucurbit downy mildew (Pseudoperonospora cubensis) biology, ecology, epidemiology, host-pathogen interaction and control. Eur. J. Plant Pathol. 129, 157–192. doi: 10.1007/s10658-010-9658-1
Lebeda, A., Křístková, E., Sedláková, B., and McCreight, J. D. (2016). “Initiative for international cooperation of researchers and breeders related to determination and denomination of cucurbit powdery mildew races,” in Proceedings of the Cucurbitaceae 2016, XIth Eucarpia Meeting on Genetics and Breeding of Cucurbitaceae, Warsaw, Poland, 148–152.
Lecoq, H., and Desbiez, C. (2012). “Virus of cucurbit crops in mediterranean region: an ever-changing: loebenstein,” in Viruses and Virus Diseases of Vegetables in the Mediterranean Basin, Vol. 84, ed. G. Lecoq (Advanced Virus Research), 67–126. doi: 10.1016/B978-0-12-394314-9.00003-8
Lee, J. M. (1994). Cultivation of grafted vegetables. I. current status, grafting methods, and benefits. HortScience 29, 235–239. doi: 10.21273/hortsci.29.4.235
Lee, J. M., and Oda, M. (2003). Grafting of herbaceous vegetable and ornamental crops. Hortic. Rev. 28, 61–124. doi: 10.1002/9780470650851.ch2
Leibman, D., Wolf, D., Saharan, V., Zelcer, A., Arazi, T., Yoel, S., et al. (2011). A high level of transgenic viral small RNA is associated with broad potyvirus resistance in cucurbits. Mol. Plant Microbe Interact. 24, 1220–1238. doi: 10.1094/MPMI-05-11-0128
Li, B., Gao, W., Shi, Y. X., and Xie, X. W. (2012). Progress in researches on Corynespora leaf spot. Acta Phytophyl. Sin. 39, 171–176. doi: 10.1094/PHYTO-10-14-0283-R
Li, J., Wang, T., and Han, J. (2020). Genome-wide identification and characterization of cucumber bHLH family genes and the functional characterization of CsbHLH041 in NaCl and ABA tolerance in Arabidopsis and cucumber. BMC Plant Biol. 20:272. doi: 10.1186/s12870-020-02440-1
Li, J., Yang, Q., Zhang, S. M., Wang, Y. X., and Zhao, X. Y. (2009). Evaluation of biocontrol efficiency and security of a Bacillus subtilis strain B29 against cucumber Fusarium wilt in field. China Veget. 2, 30-33
Li, M., Li, Y., Zhang, W., Li, S., Gao, Y., Ai, X., et al. (2018). Metabolomics analysis reveals that elevated atmospheric CO2 alleviates drought stress in cucumber seedling leaves. Anal. Biochem. 559, 71–85. doi: 10.1016/j.ab.2018.08.020
Liao, C. T., and Lin, C. H. (1996). Photosynthetic responses of grafted bitter melon seedlings to flood stress. Environ. Exp. Bot. 36, 167–172. doi: 10.1016/0098-8472(96)01009-x
Lin, C. Y., Ku, H. M., Chiang, Y. H., Ho, H. Y., Yu, T. A., and Jan, F. J. (2012). Development of transgenic watermelon resistant to cucumber mosaic virus and Watermelon mosaic virus by using a single chimeric transgene construct. Trans. Res. 21, 983–993. doi: 10.1007/s11248-011-9585-8
Lin, D. D., Zhang, G. F., Yu, J. B., and Feng, J. (2011). Analyses of photosynthetic pigment content and chlorophyll fluorescence parameter in leaves of different clones of Cinnamomum camphora. J. Plant Res. Environ. 20, 56–61.
Lin, Y. S., Hwang, C. H., and Soong, S. C. (1998). Resistance of bitter gourd-loofah grafts to Fusarium oxysporum f. sp. momordicae and their yield. Plant Prot. Bull. (TW) 40, 121–132.
Ling, K., and Levi, A. (2007). Sources of resistance to Zucchini yellow mosaic virus in Lagenaria siceraria germplasm. Hortscience 42, 1124–1126. doi: 10.21273/HORTSCI.42.5.1124
Ling, K. S., Levi, A., Adkins, S., Kousik, C. S., Miller, G., Hassell, R., et al. (2013). Development and field evaluation of multiple virus-resistant bottle gourd (Lagenaria siceraria). Plant Dis. 97, 1057–1062. doi: 10.1094/PDIS-07-12-0639-RE
Liu, B., Ren, J., Zhang, Y., An, J., Chen, M., Chen, H., et al. (2015). A new grafted rootstock against root-knot nematode for cucumber, melon, and watermelon. Agron. Sust. Dev. 35, 251–259. doi: 10.1007/s13593-014-0234-5
Liu, Y., Du, Q., Bai, L., Sun, M., Li, Y., He, C., et al. (2021d). Interference of CsGPA1, the α-submit of G protein, reduces drought tolerance in cucumber seedlings. Hortic. Plant J. 7, 209–220. doi: 10.1016/j.hpj.2021.02.003
Liu, Y., Dong, S., Wei, S., Wang, W., Miao, H., Bo, K., et al. (2021c). QTL mapping of heat tolerance in cucumber (Cucumis sativus L.) at adult stage. Plants 10:324. doi: 10.3390/plants10020324
Liu, D., Dong, S., Bo, K., Miao, H., Li, C., Zhang, Y., et al. (2021a). Identification of QTLs controlling salt tolerance in cucumber (Cucumis sativus L.) seedlings. Plants 2021:85. doi: 10.3390/plants10010085
Liu, J., Gao, Y., Gong, F., Hou, F., Zhang, Z., Cheng, X., et al. (2021b). The transcriptome and metabolome reveal stress responses in sulfur-fumigated cucumber (Cucumis sativus L.). Front. Plant Sci. 12:778956. doi: 10.3389/fpls.2021.778956
Liu, L., Gu, Q., Ijaz, I., Zhang, J., and Ye, Z. (2016). Generation of transgenic watermelon resistance to cucumber mosaic virus facilitated by an effective agrobacterium-mediated transformation method. Sci. Hortic. 205, 32–38. doi: 10.1016/j.scienta.2016.04.013
Liu, S. (2016). Grafting cucumber onto luffa improves drought tolerance by increasing ABA biosynthesis and sensitivity. Sci. Rep. 6:20212. doi: 10.1038/srep20212
Majumder, A. L., Sengupta, S., and Goswami, L. (2009). “Osmolyte regulation in abiotic stress,” in Abiotic Stress Adaptation in Plants, eds A. Pareek, S. Sopory, and H. Bohnert (Dordrecht: Springer), 349–370. doi: 10.1007/978-90-481-3112-9_16
Martín-Hernández, A. M., and Picó, B. (2021). Natural resistances to viruses in cucurbits. Agronomy 11:23. doi: 10.3390/agronomy11010023
McCreight, J. D. (2011). Melon trait and germplasm resources survey 2011. Cucurbit Genet Coop. Rep. 3, 29–31.
Messelink, G. J., Calvo, F. J., Marín, F., and Janssen, D. (2020). “Cucurbits,” in Integrated Pest and Disease Management in Greenhouse Crops, eds M. L. Gullino, R. Albajes, and P. C. Nicot (New York, NY: Springer International Publishing), 537–566.
Messelink, G. J., van Maanen, R., van Steenpaal, S. E., and Janssen, A. (2008). Biological control of thrips and whiteflies by a shared predator: two pests are better than one. Biol. Control 44, 372–379. doi: 10.1016/j.biocontrol.2007.10.017
Mkhize, P., Mashilo, J., and Shimelis, H. (2021). Progress on genetic improvement and analysis of bottle gourd [lagenaria siceraria (molina) standl.] for agronomic traits, nutrient compositions, and stress tolerance: a review. front. sustain. Food Syst. 5:683635. doi: 10.3389/fsufs
Modarelli, G. C., Rouphael, Y., De Pascale, S., Öztekin, G. B., Tüzel, Y., Orsini, F., et al. (2020). Appraisal of salt tolerance under greenhouse conditions of a cucurbitaceae genetic repository of potential rootstocks and scions. Agronomy 10:967. doi: 10.3390/agronomy10070967
Molad, O., Smith, E., and Luria, N. (2021). New early phenotypic markers for cucumber green mottle mosaic virus disease in cucumbers exposed to fluctuating extreme temperatures. Sci. Rep. 11:19060. doi: 10.1038/s41598-021-98595-4
Montero-Pau, J., Blanca, J., Bombarely, A., Ziarsolo, P., Esteras, C., Martí-Gómez, C., et al. (2018). De novo assembly of the zucchini genome reveals a whole-genome duplication associated with the origin of the cucurbita genus. Plant Biotechnol. J. 16, 1161–1171. doi: 10.1111/pbi.12860
Montero-Pau, J., Blanca, J., Esteras, C., Martínez-Pérez, E. M., Gómez, P., Monforte, A. J., et al. (2017). An SNP-based saturated genetic map and QTL analysis of fruit-related traits in zucchini using genotyping- by-sequencing. BMC Genom. 18:3439. doi: 10.1186/s12864-016-3439-y
Nanasato, Y., and Tabei, Y. (2020). A method of transformation and current progress in transgenic research on cucumbers and cucurbita species. Plant Biotechnol. 37, 141–146. doi: 10.5511/plantbiotechnology.20.0225a
Nanasato, Y., Okuzaki, A., and Tabei, Y. (2013). Improving the transformation efficiency of cucurbita species: factors and strategy for practical application. Plant Biotechnol. 30, 287–294. doi: 10.5511/plantbiotechnology.13.0331a
Nawaz, M. A., Chen, C., Shireen, F., Zheng, Z., Jiao, Y., Sohail, H., et al. (2018). Improving vanadium stress tolerance of watermelon by grafting onto bottle gourd and pumpkin rootstock. Plant Growth Regul. 85, 41–56. doi: 10.1007/s10725-018-0372-x
Nawaz, M. A., Wang, L., Jiao, Y., Chen, C., Zhao, L., Mei, M., et al. (2017). Pumpkin rootstock improves nitrogen use efficiency of watermelon scion by enhancing nutrient uptake, cytokinin content, and expression of nitrate reductase genes. Plant Growth Regul. 2, 233–246. doi: 10.1007/s10725-017-0254-7
Ng, J. C. K., and Perry, K. L. (2004). Transmission of plant viruses by aphid vectors. Mol. Plant Pathol. 5, 505–511. doi: 10.1111/j.1364-3703.2004.00240.x
Nisini, P. T., Colla, G., Granati, E., Temperini, O., Crino, P., and Saccardo, F. (2002). Rootstock resistance to fusarium wilt and effect on fruit yield and quality of two muskmelon cultivars. Sci. Hortic. 93, 281–288. doi: 10.1016/s0304-4238(01)00335-1
Niu, M., Sun, S., Nawaz, M. A., Sun, J., Cao, H., Lu, J., et al. (2019). Grafting cucumber onto pumpkin induced early stomatal closure by increasing ABA sensitivity under salinity conditions. Front. Plant Sci. 10:1290. doi: 10.3389/fpls.2019.01290
Omar, A. F., El-Kewey, S. A., Sidaros, S. A., and Shimaa, A. K. (2011). Egyptian isolates of papaya ringspot virus form a molecularly distinct clade. J. Plant Pathol. 93:1224.
Omar, N. H., and Adam, M. A. M. (2018). Survey of plant parasitic nematode associated with cucumber plant (Cucumis sativus L.) in Al-Jabal Al-Akther region. Al Mukhtar J. Sci. 33, 36–44.
Osipowski, P., Pawełkowicz, M., Wojcieszek, M., Skarzyńska, A., Przybecki, Z., and Pląder, W. (2020). A high-quality cucumber genome assembly enhances computational comparative genomics. Mol. Genet. Genom. 295, 177–193. doi: 10.1007/s00438-019-01614-3
Otani, T., and Seike, N. (2007). Rootstock control of fruit dieldrin concentration in grafted cucumber (Cucumis sativus). J. Pesticide Sci. 2007:30007.
Pal, S., Rao, S. E., Hebbar, S. S., Sriram, S., Pitchaimuthu, M., and Rao, K. V. (2020). Assessment of fusarium wilt resistant Citrullus sp. rootstocks for yield and quality traits of grafted watermelon. Sci. Hort. 272:109497. doi: 10.1016/j.scienta.2020.109497
Pandey, P., Irulappan, V., Bagavathiannan, M. V., and Senthil-Kumar, M. (2017). impact of combined abiotic and biotic stresses on plant growth and avenues for crop improvement by exploiting physio-morphological traits. Front. Plant Sci. 8:537. doi: 10.3389/fpls.2017.00537
Parida, A. K., and Das, A. B. (2005). Salt tolerance and salinity effects on plants: a review. Ecotoxicol. Environ. Safety 60, 324–349. doi: 10.1016/j.ecoenv.2004.06.010
Park, Y. L., and Lee, J. H. (2005). Impact of twospotted spider mite (Acari: Tetranychidae) on growth and productivity of glasshouse cucumbers. J. Econ. Entomol. 98, 457–463. doi: 10.1093/jee/98.2.457
Parmar, N., Singh, K. H., Sharma, D., Singh, L., Kumar, P., Nanjundan, J., et al. (2017). Genetic engineering strategies for biotic and abiotic stress tolerance and quality enhancement in horticultural crops: a comprehensive review. 3Biotech 7:239. doi: 10.1007/s13205-017-0870-y
Parry, M. A. J., Flexas, J., and Medrano, H. (2005). Prospects for crop production under drought: research priorities and future directions. Ann. Appl. Biol. 147, 211–226. doi: 10.1111/j.1744-7348.2005.00032.x
Parvathi, M. S., and Nataraja, K. N. (2017). “Simultaneous expression of stress regulatory genes for abiotic stress tolerance,” in Plant Tolerance to Individual and Concurrent Stresses, ed. S. K. Muthappa (Springer).
Parvathi, M. S., Dhanyalakshmi, K. H., and Nataraja, K. N. (2021). “Molecular mechanisms associated with drought and heat tolerance in plants and options for crop improvement for combined stress tolerance,” in Agronomic Crops, ed. M. Hasanuzzaman (Springer), 481–502. doi: 10.1007/978-981-15-0025-1_23
Parvathi, M. S., Sreevathsa, R., Rama, N., and Nataraja, K. N. (2015). Simultaneous expression of AhBTF3, AhNFYA7and EcZF modulates acclimation responses to abiotic stresses in rice (Oryza sativa L). Proc. Environ. Sci. 29, 236–237. doi: 10.1016/j.proenv.2015.07.290
Pawełkowicz, M. Zieliński, K. Zielińska, D. Pląder, W., Yagi, K., Wojcieszek, M., et al. (2016). Next generation sequencing and omics in cucumber (Cucumis sativus L.) breeding directed research. Plant Sci. 242, 77–88. doi: 10.1016/j.plantsci.2015.07.025
Peng, Y. Q., Zhu, J., Li, W. J., Gao, W., Shen, R. Y., and Meng, L. J. (2020). Effects of grafting on root growth, anaerobic respiration enzyme activity and aerenchyma of bitter melon under waterlogging stress. Sci. Hortic. 261:108977. doi: 10.1016/j.scienta.2019.108977
Pita-Barbosa, A., Ricachenevsky, F. K., and Flis, P. M. (2019). One “OMICS” to integrate them all: ionomics as a result of plant genetics, physiology and evolution. Theor. Exp. Plant Physiol. 31, 71–89. doi: 10.1007/s40626-019-00144-y
Porter, J. R., and Gawith, M. (1999). Temperatures and the growth and development of wheat: a review. Eur. J. Agron. 10, 23–36. doi: 10.1016/s1161-0301(98)00047-1
Potters, G., Pasternak, T. P., Guisez, Y., Palme, K. J., and Jansen, M. A. (2007). Stress-induced morphogenic responses: growing out of trouble? Trends Plant Sci. 12, 98–105. doi: 10.1016/j.tplants.2007.01.004
Provvidenti, R. (1995). A multi-viral resistant cultivar of bottle gourd (Lagenaria siceraria) from Taiwan. Cucurbit Genet Coop. Rep. 18, 65–67.
Provvidenti, R., and Gonsalves, D. (1984). Occurrence of zucchini yellow mosaic virus in cucurbits from Connecticut, New York, Florida and California. Plant Dis. 68, 443–446. doi: 10.1094/PD-69-443
Pruthvi, V., Narasimhan, R., and Nataraja, K. N. (2014). Simultaneous expression of abiotic stress responsive transcription factors, AtDREB2A, AtHB7 and AtABF3 improves salinity and drought tolerance in peanut (Arachis hypogaea L.). PLoS One 9:e111152. doi: 10.1371/journal.pone.0111152
Pucciariello, C., Banti, V., and Perata, P. (2012). ROS signaling as common element in low oxygen and heat stresses. Plant Physiol. Biochem. 59, 3–10. doi: 10.1016/j.plaphy.2012.02.016
Rahman, A. H. M. M., Anisuzzaman, M., Ahmed, F., Rafiul Islam, A. K. M., and Naderuzzaman, A. T. M. (2008). Study of nutritive value and medicinal uses of cultivated cucurbits. J. Appl. Sci. Res. 4, 555–558.
Rai, M., Singh, M., Pandey, S., Singh, B., and Yadav, D. S. (2007). IIVR: Fifteen Years of Accomplishments. Varanasi: Indian Institute of Vegetable Research Publication.
Rajasekharan, K. R., and Shanmugavelu, K. G. (1984). MDU-I bitter gourd. South Indian Hortic. 32:47.
Ram, H. H., Prasad, L., Singh, D. K., Yadav, R. S., and Singh, B. (2009). Screening of cucurbit germplasm against insect-pests and diseases under natural conditions. Prog. Agric. 9, 229–234.
Ramos, A., Fu, Y., Michael, V., and Meru, G. (2020). QTL-seq for identification of loci associated with resistance to Phytophthora crown rot in squash. Sci. Rep. 10, 1–8. doi: 10.1038/s41598-020-62228-z
Reyad, N. E. H. A., El-sayed, S. F., and Azoz, S. N. (2021). Evaluation of grafting using cucurbit interspecific hybrids to control fusarium wilt in cucumber. Plant Cell Biotechnol. Mol. Biol. 2021, 50–63.
Rostami, F., Alaei, H., Karimi, H. R., and Abad, A. B. (2015). Controlling the root and stem rot of cucumber, caused by Pythium aphanidermatum, using resistance cultivars and grafting onto the cucurbit rootstocks. Azarian J. Agric. 1, 19–24.
Rouphael, Y., Cardarelli, M., Rea, E., and Colla, G. (2008b). Grafting of cucumber as a means to minimize copper toxicity. Environ. Exp. Bot. 63, 49–58. doi: 10.1016/j.envexpbot.2007.10.015
Rouphael, Y., Cardarelli, M., Colla, G., and Rea, E. (2008a). Yield, mineral composition, water relations, and water use efficiency of grafted mini-watermelon plants under deficit irrigation. HortScience 43, 730–736. doi: 10.21273/hortsci.43.3.730
Rouphael, Y., Rea, E., Cardarelli, M., Bitterlich, M., Schwarz, D., and Colla, G. (2016). Can adverse effects of acidity and aluminum toxicity be alleviated by appropriate rootstock selection in cucumber? Front. Plant Sci. 7:1283. doi: 10.3389/fpls.2016.01283
Roy, S. K.Chakrabarti A. K. (2003). “VEGETABLES OF TROPICAL CLIMATES | commercial and dietary importance,” in Encyclopedia of Food Sciences and Nutrition, ed. B. Caballero, 5925–5932.
Ruggieri, V., Alexiou, K. G., Morata, J., Argyris, J., Pujol, M., Yano, R., et al. (2018). An improved assembly and annotation of the melon (Cucumis melo L.) reference genome. Sci. Rep. 8, 1–9. doi: 10.1038/s41598-018-26416-2
Sairam, R. K., and Tyagi, A. (2004). Physiology and molecular biology of salinity stress tolerance in plants. Curr. Sci. 2004, 407–421.
Sakata, Y., Ohara, T., and Sugiyama, M. (2008). The history of melon and cucumber grafting in Japan. Acta Hortic. 767, 217–228. doi: 10.17660/actahortic.2008.767.22
Samadia, D. K., and Haldhar, S. M. (2017). Breeding strategies and scope of improvement in arid zone fruit crop plants under abiotic stressed agro-climate: an analysis. J. Agric. Ecol. 4, 1–13.
Saroj, P. L., and Choudhary, B. R. (2020). Improvement in cucurbits for drought and heat stress tolerance—a review. Curr. Hortic. 8, 3–13. doi: 10.5958/2455-7560.2020.00013.8
Sastry, K. S. (2013). Plant Virus and Viroid Diseases in the Tropics. Introduction of Plant Viruses and Sub-Viral Agents, Classification, Assessment of Loss, Transmission and Diagnosis. New York: Springer.
Savvas, D., Ntatsi, G., and Barouchas, P. (2013). Impact of grafting and rootstock genotype on cation uptake by cucumber (Cucumis sativus L.) exposed to Cd or Ni stress. Sci. Hortic. 149, 86–96. doi: 10.1016/j.scienta.2012.06.030
Schaefer, H., and Renner, S. S. (2011). Phylogenetic relationships in the order cucurbitales and a new classification of the gourd family (Cucurbitaceae). Taxon 60, 122–138. doi: 10.1002/tax.601011
Schwarz, D., Rouphael, Y., Colla, G., and Venema, J. H. (2010). Grafting as a tool to improve tolerance of vegetables to abiotic stresses: thermal stress, water stress and organic pollutants. Sci. Hortic. 127, 162–171. doi: 10.1016/j.scienta.2010.09.016
Shapiro, L. R., Salvaudon, L., Mauck, K. E., Pulido, H., De Moraes, C. M., Stephenson, A. G., et al. (2013). Disease interactions in a shared host plant: effects of pre-existing viral infection on cucurbit plant defense responses and resistance to bacterial wilt disease. PLoS One 8:e77393. doi: 10.1371/journal.pone.0077393
Shapiro, L. R., Seidl-Adams, I., De Moraes, C. M., Stephenson, A. G., and Mescher, M. C. (2014). Dynamics of short and long term association between a bacterial plant pathogen and its arthropod vector. Sci. Rep. 4:4155. doi: 10.1038/srep04155
Sharma, A., Rana, C., and Shiwani, K. (2016). “Important insect pests of cucurbits and their management,” in Handbook of Cucurbits: Growth, Cultural Practices and Physiology, ed. M. (Pessarakli CRC Press) pp. 327–359.
Sharma, P., Jha, A. B., Dubey, R. S., and Pessarakli, M. (2012). Reactive oxygen species, oxidative damage, and antioxidative defense mechanism in plants under stressful conditions. J. Bot. 2012, 1–26. doi: 10.1155/2012/217037
Sharma, S., Kooner, R., and Arora, R. (2017). “Insect pests and crop losses,” in Breeding Insect Resistant Crops for Sustainable Agriculture, eds R. Arora and S. Sandbu (Singapore: Springer), 45–66. doi: 10.1007/978-981-10-6056-4_2
Shibuya, T., Tokuda, A., Terakura, R., Shimizu-Maruo, K., Sugiwaki, H., Kitaya, Y., et al. (2007). Short-term bottom-heat treatment during low-air-temperature storage improves rooting in squash (Cucurbita moschata duch.) cuttings used for rootstock of cucumber (Cucumis sativus L.). J. Japanese Soc. Hort. Sci. 76, 139–143.
Shirani-Bidabadi, S., Abolghasemi, R., and Zheng, S. J. (2018). Grafting of watermelon (Citrullus lanatus cv. mahbubi) onto different squash rootstocks as a means to minimize cadmium toxicity. Int. J. Phytoremed. 20, 730–738. doi: 10.1080/15226514.2017.1413338
Singh, J., and Raghuraman, M. (2011). Emerging scenario of important mite pests in north India. Zoosymposia 6, 170–179. doi: 10.11646/zoosymposia.6.1.27
Singh, N. N., and Sarkar, K. R. (1991). Physiological, Genetical Basis of Drought Tolerance in Maize. In Golden Jubilee Symp. on Genetic Res. and Education: Current Trends and the Next Fifty Years. Organised by the Indian Social. New Delhi: Genetics and Plant Breeding, IARI.
Srivastava, R. M., and Joshih, S. (2021). “Integrated pest management for cucurbits in cucumber (Cucumis sativus L.),” in Cucumber Economic Values and Its Cultivation and Breeding, ed. H. Wang (IntechOpen).
Suárez-Hernández, ÁM., Grimaldo-Juárez, O., Ceceña-Durán, C., Bazante-González, I., Núñez-Ramirez, F., and González-Mendoza, D. (2021). Plant growth and quality of cucumber grafted with Lagenaria siceraria in soil infested with nematodes. Emirates J. Food Agric. 33, 67–72. doi: 10.9755/ejfa.2021.v33.i1.2565
Subedi, K., Regmi, R., Thapa, R. B., and Tiwari, S. (2021). Evaluation of net house and mulching effect on cucurbit fruit fly (Bactrocera cucurbitae Coquillett) on cucumber (Cucumis sativus L.). J. Agric. Food Res. 3:100103. doi: 10.1016/j.jafr.2021.100103
Sun, H., Wu, S., Zhang, G., Jiao, C., Guo, S., and Ren, Y. (2017). Karyotype stability and unbiased fractionation in the paleoallotetraploid cucurbita genomes. Mol. Plant 10, 1293–1306. doi: 10.1016/j.molp.2017.09.003
Sun, Y., Li, Y., Li, Y., Wang, M., Mur, L. A. J., Shen, Q., et al. (2021). Nitrate mediated resistance against fusarium infection in cucumber plants acts via photorespiration. Plant Cell Environ. 44, 3412–3431.
Sun, Y., Wang, H., Liu, S., and Peng, X. (2016). Exogenous application of hydrogen peroxide alleviates drought stress in cucumber seedlings. South Afr. J. Bot. 106, 23–28. doi: 10.1016/j.sajb.2016.05.008
Suzuki, N., and Mittler, R. (2006). Reactive oxygen species and temperature stresses: a delicate balance between signaling and destruction. Physiol. Plant. 126, 45–51. doi: 10.1111/j.0031-9317.2005.00582.x
Sydänmetsä, M., and Mbanzibwa, D. R. (2016). Occurrence of cucumber mosaic virus, zucchini yellow mosaic virus and watermelon mosaic virus in cultivated and wild cucurbits in the coastal areas of Tanzania. Afr. J. Agric. Res. 11, 4062–4069. doi: 10.5897/ajar2016.11462
Tamilselvi, N. A., Pugalendhi, L., and Sivakumar, M. (2017). Response of cucurbitaceous rootstocks and bitter gourd scions to root knot nematode Meloidogyne incognita Kofoid and White. Int. J. Hortic. Floric. 5, 247–251.
Tetteh, A. Y., Wehner, T. C., and Davis, A. R. (2010). Identifying resistance to powdery mildew race 2W in the USDA-ARS watermelon germplasm collection. Crop Sci. 50, 933–939. doi: 10.2135/cropsci2009.03.0135
Thies, J. A., Ariss, J. J., Hassell, R. L., Olson, S., Kousik, C. S., and Levi, A. (2010). Grafting for management of southern root-knot nematode, Meloidogyne incognita, in watermelon. Plant Dis. 94, 1195–1199. doi: 10.1094/PDIS-09-09-0640
Tian, G., Miao, H., Yang, Y., Zhou, J., Lu, H., Wang, Y., et al. (2016). Genetic analysis and fine mapping of watermelon mosaic virus resistance gene in cucumber. Mol. Breed. 36, 1–11.
Tsai, W. S., Abdourhamane, I. K., Knierim, D., Wang, J. T., and Kenyon, L. (2010). First report of zucchini yellow mosaic virus associated with leaf crinkle and yellow mosaic diseases of cucurbit plants in Mali. Plant Dis. 94, 923–923. doi: 10.1094/PDIS-94-7-0923B
Turechek, W., Roberts, P. D., Stansly, P. A., Webster, C. G., Kousik, C. S., and Adkins, S. T. (2014). Spatial and temporal analysis of multiple whitefly transmitted virus infections in watermelon. Plant Dis. 98, 1024–1165.
Vemanna, R. S., Babitha, K. C., Rao, H. M. H., Sathyanarayanagupta, S. K., Sarangi, S. K., Nataraja, K. N., et al. (2013). Modified multisite gateway cloning strategy for consolidation of genes in plants. Mol. Biotechnol. 53, 129–138. doi: 10.1007/s12033-012-9499-6
Vigani, G., Di Silvestre, D., Agresta, A. M., Donnini, S., Mauri, P., Gehl, C., et al. (2017). Molybdenum and iron mutually impact their homeostasis in cucumber (Cucumis sativus) plants. New Phytol. 213, 1222–1241. doi: 10.1111/nph.14214
Vitti, A., Nuzzaci, M., Scopa, A., Tataranni, G., Remans, T., Vangronsveld, J., et al. (2013). Auxin and cytokinin metabolism and root morphological modifications in Arabidopsis thaliana seedlings infected with cucumber mosaic virus (CMV) or exposed to cadmium. Int. J. Mol. Sci. 14, 6889–6902. doi: 10.3390/ijms14046889
Wang, C. J., Yang, W., Wang, C., Gu, C., Niu, D. D., Liu, H. X., et al. (2012). Induction of drought tolerance in cucumber plants by a consortium of three plant growth-promoting rhizobacterium strains. PLoS One 7:e52565. doi: 10.1371/journal.pone.0052565
Wang, J., Shang, S., Tian, L., Zhou, M., Pan, Q., Zou, K., et al. (2018). Effects of low temperature stress on antioxidant system of grafted bitter gourd seedlings. Chin. J. Trop. Crops 39, 237–245.
Wang, J., Zhang, L., Cao, Y., Qi, C., Li, S., Liu, L., et al. (2012). CsATAF1 positively regulates drought stress tolerance by an ABA-dependent pathway and by promoting ROS scavenging in cucumber. Plant Cell Physiol. 59, 930–945. doi: 10.1093/pcp/pcy030
Wang, M., Jiang, B., Peng, Q., Liu, W., He, X., Liang, Z., et al. (2018). Transcriptome analyses in different cucumber cultivars provide novel insights into drought stress responses. Int. J. Mol. Sci. 19:2067. doi: 10.3390/ijms19072067
Wang, M., Zhou, S., Lu, J., Xu, A., Huang, Y., Bie, Z., et al. (2021). CmRCC1 gene from pumpkin confers cold tolerance in tobacco by modulating root architecture and photosynthetic activity. Front. Plant Sci. 12:765302. doi: 10.3389/fpls.2021.765302
Wang, W., Liu, X., Gai, X., Ren, J., Liu, X., Cai, Y., et al. (2015). Cucumis sativus L. WAX2 plays a pivotal role in wax biosynthesis, influencing pollen fertility and plant biotic and abiotic stress responses. Plant Cell Physiol. 56, 1339–1354. doi: 10.1093/pcp/pcv052
Wang, X., Zhang, D., Cui, N., Yu, Y., Yu, G., and Fan, H. (2018). Transcriptome and miRNA analyses of the response to Corynespora cassiicola in cucumber. Sci. Rep. 8, 1–16. doi: 10.1038/s41598-018-26080-6
Wang, Y., Van den Langenberg, K., Wen, C., Wehner, T. C., and Weng, Y. (2018). QTL mapping of downy and powdery mildew resistances in PI 197088 cucumber with genotyping-by-sequencing in RIL population. Theor. Appl. Genet. 131, 597–611. doi: 10.1007/s00122-017-3022-1
Wehner, T. C., and Amand, P. C. (1995). Anthracnose resistance of the cucumber germplasm collection in North Carolina field tests. Crop Sci. 35, 228–236. doi: 10.2135/cropsci1995.0011183x003500010042x
Wintermantel, W. M., Hladky, L. L. J., Fashing, P., Ando, K., and McCreight, J. D. (2019). First report of cucurbit chlorotic yellows virus infecting melon in the new world. Plant Dis. 103:778. doi: 10.1094/PDIS-07-21-1473-PDN
Wisler, G. C., Duffus, J. E., Liu, H. Y., and Li, R. H. (1998). Ecology and epidemiology of whitefly-transmitted closteroviruses. Plant Dis. 82, 270–280. doi: 10.1094/PDIS.1998.82.3.270
Wu, H. W., Yu, T. A., Raja, J. A., Christopher, S. J., Wang, S. L., and Yeh, S. D. (2010). Double-virus resistance of transgenic oriental melon conferred by untranslatable chimeric construct carrying partial coat protein genes of two viruses. Plant Dis. 94, 1341–1347.
Wu, S., Shamimuzzaman, M. D., Su, H., Salse, J., Sui, X., Wilder, A., et al. (2017). The bottle gourd genome provides insights into Cucurbitaceae evolution and facilitates mapping of a papaya ring-spot virus resistant locus. Plant J. 92, 963–975. doi: 10.1111/tpj.13722
Wu, S., Wang, X., Reddy, U., Sun, H., Bao, K., Gao, L., et al. (2019). Genome of ‘charleston gray’, the principal american watermelon cultivar, and genetic characterization of 1,365 accessions in the US national plant germplasm system watermelon collection. Plant Biotechnol. J. 17, 2246–2258.
Xanthopoulou, A., Montero-Pau, J., Mellidou, I., Kissoudis, C., Blanca, J., Picó, B., et al. (2019). Whole-genome resequencing of Cucurbita pepo morphotypes to discover genomic variants associated with morphology and horticulturally valuable traits. Hortic. Res. 6, 1–17. doi: 10.1038/s41438-019-0176-9
Xia, Y. J., Zhou, Y. H., Tao, Y., Mao, W. H., Shi, K., Asami, T., et al. (2009). Reactive oxygen species are involved in brassinosteroid-induced stress tolerance in cucumber. Plant Physiol. 150, 801–814. doi: 10.1104/pp.109.138230
Xie, D., Xu, Y., Wang, J., Liu, W., Zhou, Q., Luo, S., et al. (2019). The wax gourd genomes offer insights into the genetic diversity and ancestral cucurbit karyotype. Nat. Commun. 10, 1–12. doi: 10.1038/s41467-019-13185-3
Xu, P., Chen, F., Mannas, J. P., Feldman, T., Sumner, L. W., and Roossinck, M. J. (2008). Virus infection improves drought tolerance. New Phytol. 180, 911–921. doi: 10.1111/j.1469-8137.2008.02627.x
Xu, Y., Yuan, Y., Du, N., Wang, Y., Shu, S., Sun, J., et al. (2018). Proteomic analysis of heat stress resistance of cucumber leaves when grafted onto Momordica rootstock. Hortic. Res. 5, 1–18. doi: 10.1038/s41438-018-0060-z
Yamaguchi, T., and Iwadate, Y. (2009). Adaptability of several cucurbit plants for use as rootstocks to prevent cucumber black root rot caused by Phomopsis sclerotioides. Ann. Rep. Soc. Plant Protect. North Japan 60, 96–101.
Yan, S., Che, G., Ding, L., Chen, Z., Liu, X., and Wang, H. (2016). Different cucumber CsYUC genes regulate response to abiotic stresses and flower development. Sci. Rep. 6:20760. doi: 10.1038/srep20760
Yanwei, L., Ying, W., Xinyi, W., Jian, W., Xiaohua, W., Baogen, W., et al. (2021). Novel genomic regions of fusarium wilt resistance in bottle gourd [Lagenaria siceraria (mol.) standl.] discovered in genome-wide association study. Front. Plant Sci. 12:650157. doi: 10.3389/fpls.2021.650157
Yetişir, H., and Sari, N. (2003). Effect of different rootstock on plant growth, yield and quality of watermelon. Austr. J. Exp. Agric. 43, 1269–1274. doi: 10.1002/jsfa.10149
Yetisir, H., Caliskan, M. E., Soylu, S., and Sakar, M. (2006). Some physiological and growth responses of watermelon [Citrullus lanatus (Thunb.) Matsum. and Nakai] grafted onto Lagenaria siceraria to flooding. Environ. Exp. Bot. 58, 1–8. doi: 10.1016/j.envexpbot.2005.06.010
Yildirim, E., Taylor, A. G., and Spittler, T. D. (2006). Ameliorative effects of biological treatments on growth of squash plants under salt stress. Sci. Hortic. (Amst.) 111, 1–6. doi: 10.1016/j.scienta.2006.08.003
Yu, B., Yan, S., Zhou, H., Dong, R., Lei, J., Chen, C., et al. (2018). Overexpression of CsCaM3 improves high temperature tolerance in cucumber. Front. Plant Sci. 9:797. doi: 10.3389/fpls.2018.00797
Yu, T. A., Chiang, C. H., Wu, H. W., Li, C. M., Yang, C. F., Chen, J. H., et al. (2011). Generation of transgenic watermelon resistant to zucchini yellow mosaic virus and papaya ringspot virus type W. Plant Cell Rep. 30, 359–371. doi: 10.1007/s00299-010-0951-4
Zehnder, G., Kloepper, J., Yao, C., and Wei, G. (1997). Induction of systemic resistance in cucumber against cucumber beetles (Coleoptera: Chrysomelidae) by plant growth-promoting rhizobacteria. J. Econ. Entomol. 90, 391–396. doi: 10.1093/jee/90.2.391
Zhang, C., Badri Anarjan, M., Win, K. T., Begum, S., and Lee, S. (2021). QTL-seq analysis of powdery mildew resistance in a Korean cucumber inbred line. Theor. Appl. Genet. 134, 435–451. doi: 10.1007/s00122-020-03705-x
Zhang, H., Du, W., Peralta-Videa, J. R., Gardea-Torresdey, J. L., White, J. C., Keller, A., et al. (2018). Metabolomics reveals how cucumber (Cucumis sativus) reprograms metabolites to cope with silver ions and silver nanoparticle-induced oxidative stress. Environ. Sci. Technol. 52, 8016–8026. doi: 10.1021/acs.est.8b02440
Zhang, M., Liu, Q., and Yang, X. (2020). CRISPR/Cas9-mediated mutagenesis of Clpsk1 in watermelon to confer resistance to Fusarium oxysporum f.sp. niveum. Plant Cell Rep. 39, 589–595. doi: 10.1007/s00299-020-02516-0
Zhang, M., Yang, X., Xu, J., Liu, G., Yao, X., and Ren, R. (2021). Characterization of fusarium root rot disease in grafted watermelon. Eur. J. Plant Pathol. 159, 1–11. doi: 10.1007/s10658-020-02013-w
Zhang, N., Zhao, B., Zhang, H. J., Weeda, S., Yang, C., Yang, Z. C., et al. (2013). Melatonin promotes water-stress tolerance, lateral root formation, and seed germination in cucumber (Cucumis sativus L.). J. Pineal Res. 54, 15–23. doi: 10.1111/j.1600-079X.2012.01015.x
Zhang, S., Liu, J., Xu, B., and Zhou, J. (2021). Differential responses of Cucurbita pepo to Podosphaera xanthii reveal the mechanism of powdery mildew disease resistance in pumpkin. Front. Plant Sci. 12:306. doi: 10.3389/fpls.2021.633221
Zhang, S., Miao, H., and Yang, Y. (2014). A major quantitative trait locus conferring resistance to Fusarium wilt was detected in cucumber by using recombinant inbred lines. Mol. Breed. 34, 1805–1815. doi: 10.1007/s11032-014-0140-1
Zhang, Y., Chen, Y., and Gu, W. (2016). “Effects of cadmium stress on growth, reactive oxygen metabolism, and photosynthesis of melon seedlings [conference poster],” in Cucurbitaceae 2016, XIth Eucarpia Meeting on Cucurbit Genetics & Breeding, July 24-28, 2016, Warsaw, Poland, 250–254.
Zheng, Y., Wu, S., Bai, Y., Sun, H., Jiao, C., Guo, S., et al. (2019). Cucurbit genomics database (CuGenDB): a central portal for comparative and functional genomics of cucurbit crops. Nucleic Acids Res. 47, D1128–D1136. doi: 10.1093/nar/gky944
Zhou, H., Guo, S., An, Y., Shan, X., Wang, Y., Shu, S., et al. (2016). Exogenous spermidine delays chlorophyll metabolism in cucumber leaves (Cucumis sativus L.) under high temperature stress. Acta Physiol. Plant. 38, 1–12.
Keywords: cucurbits, stress tolerance, biotic stress, abiotic stress, metabolic pathways breeding, grafting, mitigation
Citation: Parvathi MS, Antony PD and Kutty MS (2022) Multiple Stressors in Vegetable Production: Insights for Trait-Based Crop Improvement in Cucurbits. Front. Plant Sci. 13:861637. doi: 10.3389/fpls.2022.861637
Received: 24 January 2022; Accepted: 14 March 2022;
Published: 03 May 2022.
Edited by:
Mostafa Abdelwahed Abdelrahman, Aswan University, EgyptReviewed by:
Suresh Gawande, Directorate of Onion and Garlic Research (ICAR), IndiaPradeep Kumar, Central Arid Zone Research Institute (ICAR), India
Copyright © 2022 Parvathi, Antony and Kutty. This is an open-access article distributed under the terms of the Creative Commons Attribution License (CC BY). The use, distribution or reproduction in other forums is permitted, provided the original author(s) and the copyright owner(s) are credited and that the original publication in this journal is cited, in accordance with accepted academic practice. No use, distribution or reproduction is permitted which does not comply with these terms.
*Correspondence: M. S. Parvathi, parvathi.cph@gmail.com, parvathi.m@kau.in
†These authors have contributed equally to this work and share first authorship