- 1College of Horticulture and Landscape, Henan Institute of Science and Technology, Xinxiang, China
- 2Henan Province Engineering Research Center of Horticultural Plant Resource Utilization and Germplasm Enhancement, Xinxiang, China
APETALA2/ETHYLENE RESPONSIVE FACTOR (AP2/ERF), a plant-specific transcription factor (TF) family, plays an essential role in the growth and development of plants, and in their response to biotic and abiotic stresses. However, information on AP2/ERF in Cucurbita moschata (pumpkin), an edible and medicinal vegetable used worldwide, is scarce. A total of 212 AP2/ERF genes were identified in the C. moschata genome (CmoAP2/ERFs). Based on phylogenetic analysis, they were divided into four groups—28 AP2s, 92 ERFs, 86 dehydration-responsive element-binding (DREB) factors, and 6 ABI3/VPs (RAV). The 212 AP2/ERF genes were unevenly distributed on the 20 chromosomes of C. moschata. The results of structural analysis showed the absence of introns on 132 CmoAP2/ERFs. Four pairs of tandem duplication and 155 pairs of segmental duplication events were identified, which indicated that segmental duplications might be the main reason for the expansion of the CmoAP2/ERF family. The analysis of cis-regulatory elements (CREs) showed that most of the CmoAP2/ERFs contained hormone response elements (ABREs, EREs) in their promoters, suggesting that AP2/ERFs could contribute to the processes regulated by ethylene and abscisic acid. By comparing the transcriptome of ethephon-treated and control plants, we found that 16 CmoAP2/ERFs were significantly upregulated after ethephon treatment. Furthermore, we determined the expression patterns of these genes at different developmental stages of female and male flowers. This study provides insights into the identification, classification, physicochemical property, phylogenetic analysis, chromosomal location, gene structure, motif identification, and CRE prediction of the AP2/ERF superfamily in C. moschata. Sixteen CmoAP2/ERF genes were identified as ethylene-inducible genes. The results of this study will be valuable for understanding the roles of CmoAP2/ERFs in ethylene response and should provide a foundation for elucidating the function of AP2/ERF TFs in C. moschata.
Introduction
Plants are exposed to various environmental factors during their growth and development, such as drought, extremes of temperature, salinity, and heavy metals. To combat the various stress, plants have developed complex signaling networks at molecular and cellular levels (Wang et al., 2003; Chinnusamy et al., 2004). Transcription factors (TFs) play important roles in converting stress signals into cellular responses though binding to cis-regulatory elements (CREs) of genes involved in stress response (Franco-Zorrilla et al., 2014). Therefore, TFs are important regulators of the growth and development of plants and are involved in their adaptation to environmental factors (Liu et al., 2001; Hir and Bellini, 2013).
The AP2/ERF family of TFs is the largest and unique TF family in plants, the members of which play important roles in regulating diverse processes of plant growth, development, and response to hormone and environmental stress (Riechmann and Meyerowitz, 1998). The AP2/ERF family has been identified in many plants, such as Arabidopsis, rice (Nakano et al., 2006), maize (Zhuang et al., 2010), wheat (Zhuang et al., 2011), radish (Karanja et al., 2019), cucumber (Hu and Liu, 2011), mung bean (Labbo et al., 2018), sugarcane (Li et al., 2020), durum wheat (Faraji et al., 2020), eggplant (Li D. et al., 2021), banana (Lakhwani et al., 2016), and oil palm (Zhou and Yarra, 2021). This family contains at least one highly conserved AP2/ERF DNA binding domain, which consists of 60–70 amino acids. Based on the number of AP2/ERF DNA binding domains and sequence similarities, the AP2/ERF superfamily can be divided into three categories (AP2, EREBP, and RAV). Members of the AP2 family contain two AP2/ERF domains, whereas those with a single AP2/ERF domain are grouped into the EREBP family. The RAV family members consist of two distinct DNA binding domains, the AP2/ERF domain and the B3 domain (Nakano et al., 2006). According to the difference in the 14th and 19th amino acid residues in the AP2/ERF domain, the EREBP family is further divided into ERF and DREB subfamilies. The 14th and 19th amino acids in the ERF subfamily are alanine (A) and aspartic acid (D), respectively, which can specifically bind to a cis-acting element, AGCCGCC, of the GCC-box in the promoter region and participate in the regulation of ethylene response and abiotic stress (Ohme-Takagi and Shinshi, 1995). The 14th and 19th amino acids in the DREB subfamily are valine (V) and glutamate (E), respectively, which can specifically bind DRE/CRT elements, and a few members bind GCC-box or CE1 elements. The functions of AP2/ERF family genes have been investigated in several studies. Among them, the members of the AP2 subfamily are involved in the development of floral organs and fruits, as well as in seed germination (Bowman et al., 1989; Elliott et al., 1996). In Arabidopsis, overexpression of soybean GmAP2 genes led to early flowering and increased the length, width, and area of seeds (Jiang et al., 2020). The EREBP family members mainly respond to abiotic or biotic stress; the DREB subfamily members specifically recognize and bind the dehydration responsive elements (DRE)/C-repeat element (CRT) at the promoter of target genes in response to drought (Dossa et al., 2016), low temperature (Sakuma et al., 2002), and salt stress (Zhao et al., 2018). The ERF family members are involved in ethylene response by binding to GCC-box elements (Ohme-Takagi and Shinshi, 1995), in a stress response-dependent or independent manner. Ectopic expression of GmERF3, which binds to the GCC box and DRE/CRT element, in tobacco plants was reported to increase resistance against Alternaria alternata, Ralstonia solanacearum, and tobacco mosaic virus, and enhanced the tolerance of plants to salinity and dehydration stresses (Zhang et al., 2009). Ectopic expression of CaERF5 in tobacco led to an upregulation of the expression of defense-related genes and enhanced resistance to R. solanacearum infection (Lai et al., 2014). The RAV family proteins are important in signal transduction of plant hormones, such as ethylene and brassinosteroids (Alonso et al., 2003), and participate in the regulation of plant growth and development (Lu et al., 2014; Shin and Nam, 2018; Osnato et al., 2020), and biological and abiotic stress response (Sohn et al., 2006; Li et al., 2011; Fu et al., 2014).
Cucurbita moschata is a widely cultivated vegetable crop used both for food and medicine (Lu et al., 2019). As a monoecious plant, sex differentiation of C. moschata could be regulated by multiple phytohormones. Among these, ethylene is the main regulator of sex determination in Cucurbitaceae (Manzano et al., 2011). Ethephon is widely used as a ethylene-releasing agent to induce more female flowers to obtain higher yields in Cucurbitaceae crops. As mentioned above, AP2/ERF family members are involved in ethylene response in other crops (Ohme-Takagi and Shinshi, 1995; Alonso et al., 2003). However, the AP2/ERF TF family has not been systematically identified in C. moschata, which severely limits the study of the genes in the family.
With the completion of its whole genome sequencing in 2017, the molecular biology research on C. moschata has entered a new stage. In this study, the AP2/ERF family members were identified in C. moschata. The phylogenetic analysis and characterization of gene structure, conserved motifs, chromosome localization, and CREs were performed. In addition, combined with the transcriptomic data for shoot apices of seedlings treated with ethephon, we analyzed the ethylene response members of the AP2/ERF family in C. moschata. The expression patterns of the ethylene-induced genes at different developmental stages of female and male flowers were determined. The results provide a foundation for further exploration of the biological functions of each AP2/ERF family gene and provide clues for unraveling the ethylene response mechanism in C. moschata.
Materials and Methods
Identification and Classification of AP2/ERF Family Genes in the Cucurbita moschata Genome
The C. moschata genome sequences1 were downloaded to identify the AP2/ERF family members. A Hidden Markov Model search (HMMsearch) was conducted using the HMM profile of the AP2/ERF domain (PF00847), which was obtained using the Pfam program.2 The preliminary screening of members was performed using HMMER3.1, with P < 0.001. All the searched sequences were then submitted to the SMART tool3 and NCBI CDD4 to detect the AP2/ERF domain and to remove the sequences without the AP2/ERF domain.
The protein features, including amino acid number, isoelectric point (pI), molecular weight (MW), aliphatic index, grand average of hydropathicity (GRAVY), and instability index, were predicted using ExPASy.5
Chromosomal Distribution, Gene Structure, Conserved Motif, and Phylogenetic Analysis
The information on chromosome distribution of the AP2/ERF genes was plotted with TBtools6 based on the C. moschata genome database. Collinearity analysis within C. moschata was performed using the TBtools and MCScanX (Wang et al., 2012; Chen et al., 2020). The values of non-synonymous (Ka) and synonymous (Ks) substitution for each duplicated AP2/ERF gene pair were calculated with KaKs_Calculator 2.0 (Wang et al., 2010). The exon–intron organization of the AP2/ERF family genes was displayed using GSDS7 (Hu et al., 2015). Conserved motifs were predicted using MEME,8 with the parameter of maximum number of motifs being 20. Phylogenetic tree was constructed using the maximum likelihood method and plotted with 1,000 bootstraps using MEGA X (Kumar et al., 2018).
Analysis of Cis-Regulatory Elemens
A sequence 2,000 bp upstream of the transcription start site of each CmoAP2/ERF gene was selected to predict CREs, using the PlantCARE tool9 (Lescot et al., 2002).
Ethylene Response Analysis of CmoAP2/ERF Genes
The RNA-Seq data of shoot apices subjected to ethephon (100 mg/L) treatment for 4 h, which were previously obtained by our group (NCBI accession number: PRJNA736171), were used to explore the expression patterns of CmoAP2/ERFs in response to ethylene. The transcript levels of CmoAP2/ERFs were calculated with the fragments per kilobase of exon per million fragments mapped (FPKM) method. The obtained FPKM values of CmoAP2/ERFs were Log2 normalized. Heatmap was drawn using the TBtools software for further visualization.
RNA Extraction and Real-Time PCR Analysis
At the flowering stage of plants, the female and male flower buds with vertical diameters of 0.5, 1.0, and 2.0 cm, which represent different developmental stages, were collected, respectively, and immediately frozen in liquid nitrogen for RNA extraction. RNA was extracted from the samples using the MiniBEST Plant RNA Extraction Kit (Takara, Dalian, China) and then reverse transcribed into cDNA using the PrimeScript™ RT Master Mix (Perfect Real Time) Reagent Kit (Takara, Dalian, China). Real-time PCR was performed on a Bio-Rad IQ5 instrument (Foster City, CA, United States). Based on the results of ethylene response analysis of CmoAP2/ERF genes, 16 ethylene-induced genes have been selected to explore their expression patterns at different developmental stages of flower. The primers for CmoAP2/ERF genes were designed using the Primer Premier 5.0 software. The sequences of primers are listed in Additional File 1. The reactions were performed as described by the manufacturer. Real-time PCR conditions were as follows: 95 °C for 30 s and 40 cycles of 95 °C for 5 s and 61 °C for 30 s. The RNA expression levels were normalized to that of ACTIN. They were calculated using the 2–ΔΔCt method (Livak and Schmittgen, 2001). The expression of each gene was determined using three biological and three technical replicates.
Results
Genome-Wide Identification of CmoAP2/ERF Genes
With reference to the C. moschata genome database, members of the AP2/ERF family were searched using HMMsearch with a HMM profile of PF00847. After SMART tool and NCBI CDD verification, a total of 212 sequences were identified as candidate CmoAP2/ERF genes across the whole genome. The basic features, including amino acid number, aliphatic index, GRAVY, pI, MW, and instability index of the CmoAP2/ERF family genes, are listed in Additional File 2. The identified CmoAP2/ERF genes ranged in length from 416 to 22,786 bp. These genes were predicted to encode proteins with 132–1183 amino acids. The MW of CmoAP2/ERF proteins ranged from 14.79 (CmoERF42) to 130.61 (CmoERF36) kDa and the pI varied from 4.36 (CmoERF19) to 11.54 (CmoERF25). There are 73 CmoAP2/ERF proteins with pI > 7 and 139 with pI < 7, which indicates that most of these proteins have a higher proportion of acidic amino acids. The GRAVY score of CmoAP2/ERF proteins ranged from −1.104 (CmoERF68) to −0.018 (CmoDREB12). Most of the CmoAP2/ERF genes were predicted to encode unstable proteins with the instability index >40, and only 11 family members, namely CmoRAV2, CmoDREB38, CmoERF34, CmoERF39, CmoERF40, CmoRAV3, CmoDREB65, CmoERF54, CmoRAV5, CmoERF78, and CmoRAV6, were predicted to be stable proteins based on the instability index.
Phylogenetic Tree Analysis
To evaluate the evolutionary relationship of the CmoAP2/ERF genes, we constructed a phylogenetic tree using the amino acid sequences of proteins encoded by these genes (Figure 1). The CmoAP2/ERF genes were grouped into three major classes, EREBP, AP2, and RAV. Twenty-eight genes containing two identical AP2/ERF domains were assigned to the AP2 family, six genes having one AP2/ERF and one B3 domains were assigned to the RAV family, and 178 genes with a single AP2/ERF domain were assigned to the EREBP family. They were further grouped into ERF (92 members, with a conserved Ala and Asp residue located at the 14th and 19th position, respectively) and DREB (86 members, with a conserved Val and Gln residue located at the 14th and 19th position, respectively) subfamilies.
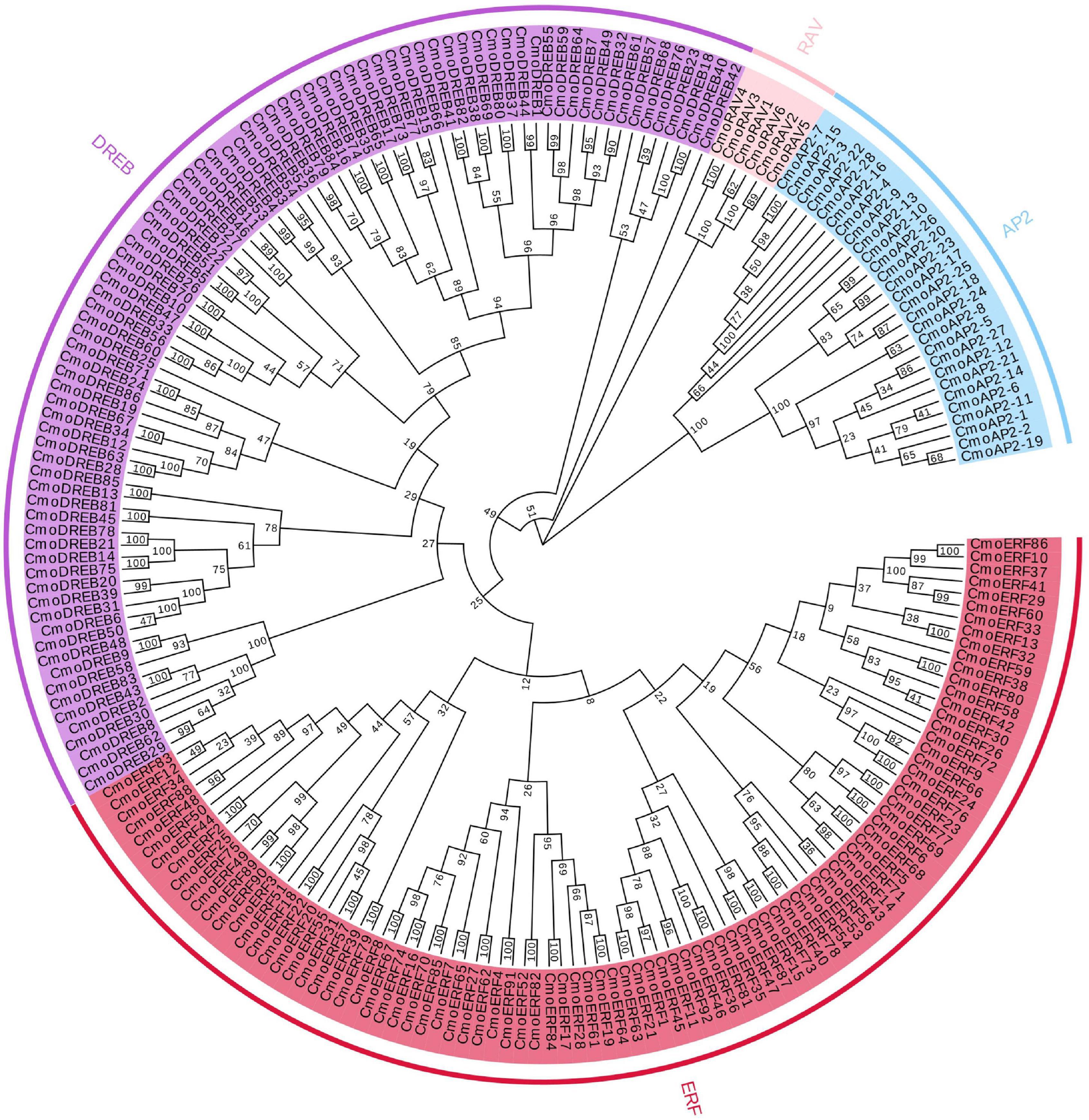
Figure 1. Phylogenetic tree of 212 AP2/ERF family proteins in Cucurbita moschata. Red highlighted area shows the ERF group; purple highlighted area shows the DREB group; blue highlighted area shows the AP2 group; pink highlighted area shows the RAV group. The numbers on the branch points represent bootstrap values; scale length represent genetic distance.
Gene Structure and Conserved Motif Analysis of the CmoAP2/ERF Family
To characterize the gene structure of CmoAP2/ERF members, the introns and exons of the CmoAP2/ERF genes were examined. As shown in Figure 2A and Additional File 3, in the ERF subfamily, 67 genes have no introns and 25 genes have 1–17 introns. Most of the DREB genes have no introns, except for 26 genes, in which the number of introns range from one to nine (Figure 2B). All the AP2 genes possess 4–12 introns (Figure 2C). Only one RAV gene, CmoRAV4, has 13 introns, whereas the remaining five RAV genes have one intron each (Figure 2D). Overall, 132 members of the CmoAP2/ERF family have no introns and 80 members have 1–17 introns. Among 212 CmoAP2/ERFs, 29 have 3′- and 5′-untranslated regions (UTRs); 9 genes lack 5′-UTR, and 10 genes lack 3′-UTR.
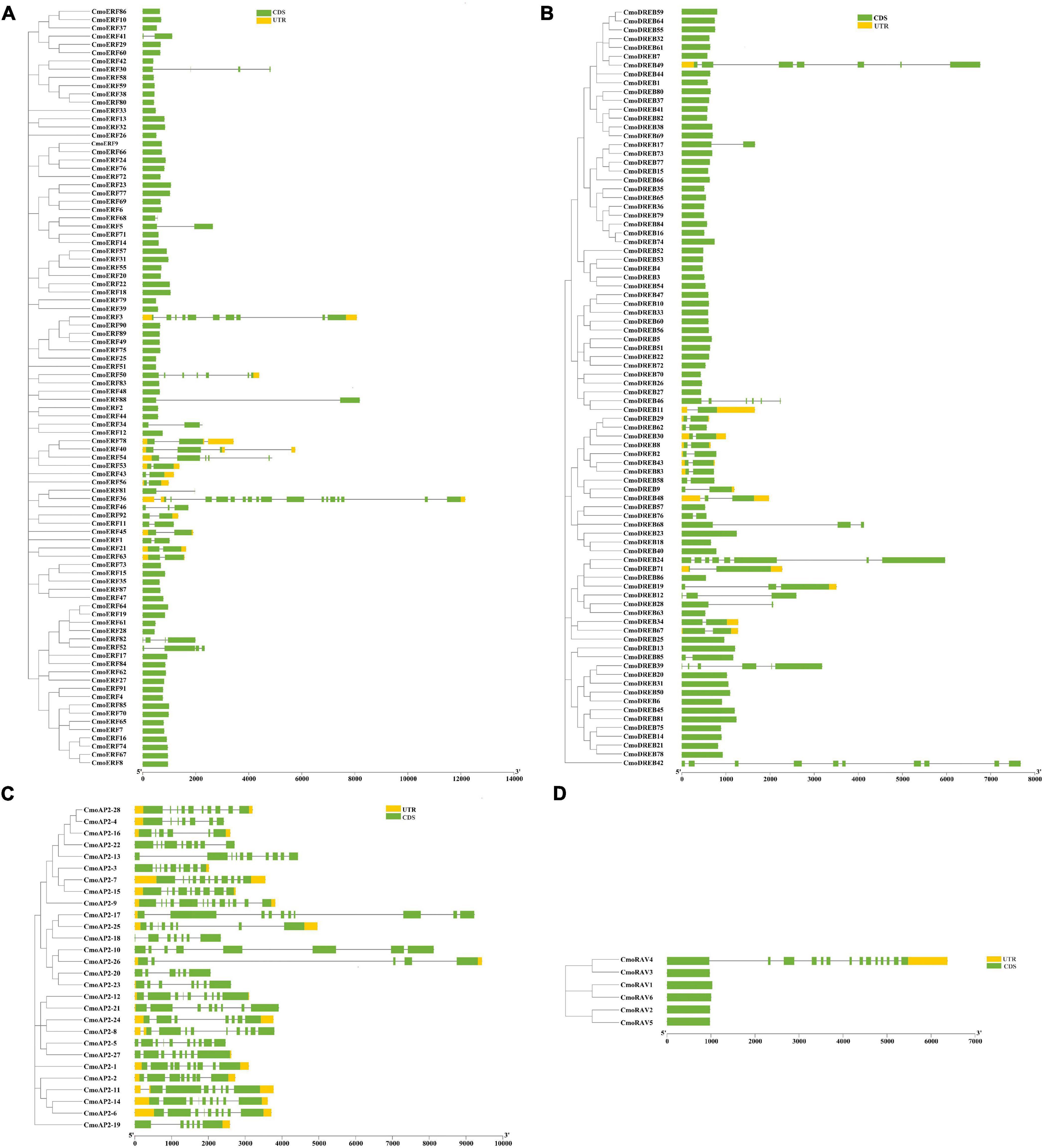
Figure 2. Phylogenetic relationship and gene structure of the 212 CmoAP2/ERF family proteins in Cucurbita moschata. CDS (coding sequence) and UTR are represented by the green box and yellow box, respectively, and the black line represents the intron in gene structure analyse. (A) ERF subfamily; (B) DREB subfamily; (C) AP2 subfamily; (D) RAV subfamily.
Twenty important conserved motifs in the CmoAP2/ERF proteins were analyzed (Figure 3 and Additional File 4). Motif-1 and -2 are generally present in all the CmoAP2/ERF protein sequences, representing the highly conserved AP2 domain. Besides Motif-1, -2, and -3 are present in most of the ERF protein sequences, except CmoERF64, CmoERF19, CmoERF61, CmoERF28, CmoERF82, CmoERF52, CmoERF17, and CmoERF84. Motif-1 is present in all the DREB protein sequences. Motif-2 is present in most of the DREB protein sequences, except CmoDREB17, CmoDREB73, CmoDREB66, CmoDREB35, CmoDREB65, CmoDREB36, CmoDREB79, CmoDREB84, CmoDREB16, CmoDREB74, CmoDREB53, CmoDREB4, and CmoDREB42. Motif-2, -4, and -6 are present in all the AP2 protein sequences. Motif-5 is commonly found in the AP2 protein, except for CmoAP2-9, whereas interestingly, CmoAP2-9 possess two repeated Motif-18, -19, -9, -3, -14, and -2. Motif-1, -2, and -3 are present in all the RAV protein sequences. The results of conserved motif analysis were generally consistent with the phylogenetic relationship.
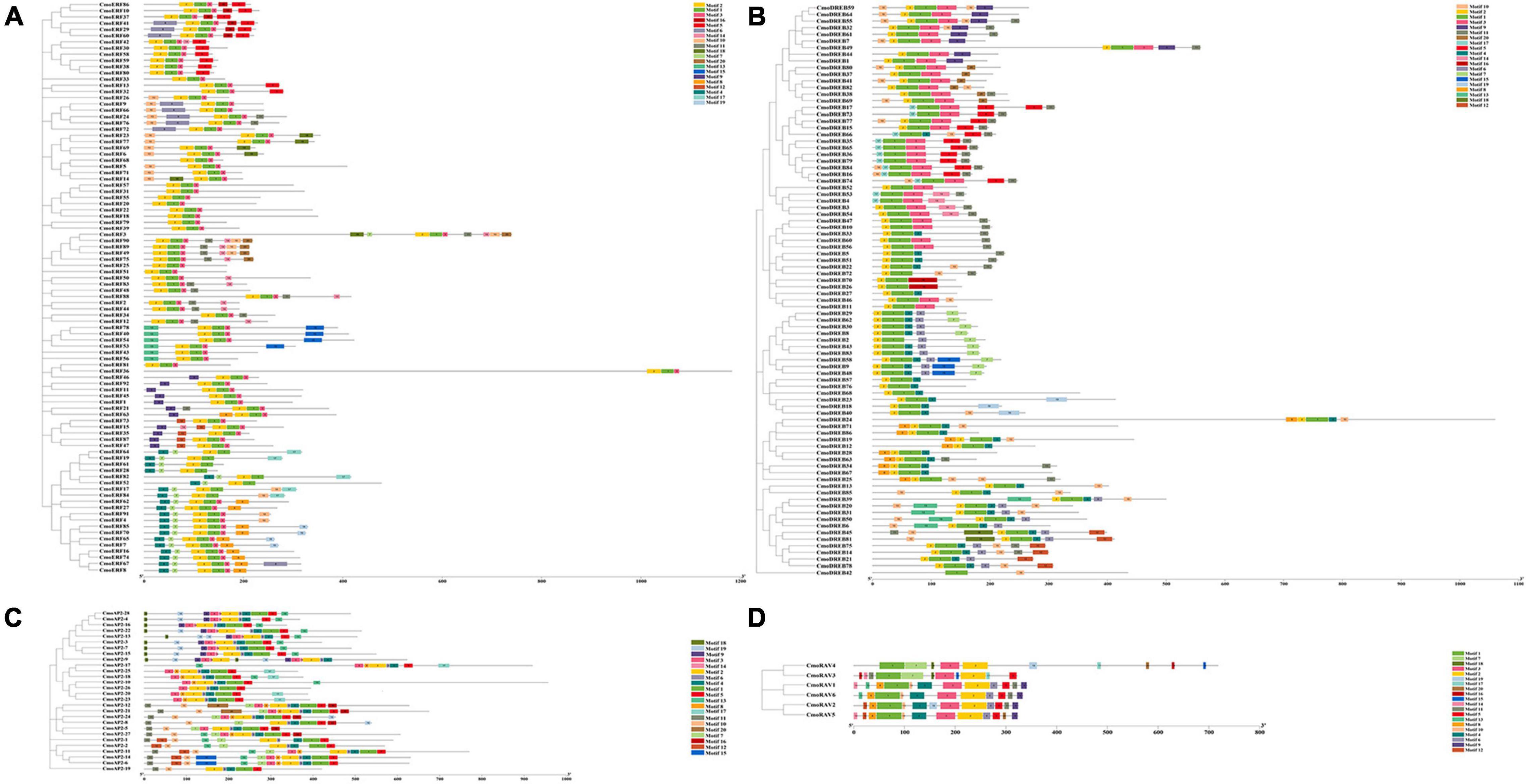
Figure 3. Phylogenetic relationship and conserved motif of the 212 CmoAP2/ERF family proteins in Cucurbita moschata. Boxes in different colors represent different conserved motifs. (A) ERF subfamily; (B) DREB subfamily; (C) AP2 subfamily; (D) RAV subfamily.
Chromosomal Distribution, Gene Duplication, and Collinearity Analysis of the CmoAP2/ERF Genes
To further investigate the gene duplication events in C. moschata, the chromosomal distribution of each CmoAP2/ERF gene was identified from the genomic data of C. moschata. A total of 212 CmoAP2/ERF genes were mapped onto 20 chromosomes (Figure 4). Chromosome 04 contained the highest number of CmoAP2/ERF genes (19 genes). The lowest number of CmoAP2/ERF genes was found on chromosome 13 (3 genes), which were all identified as ERF genes. Six RAV genes were found to be distributed on four chromosomes, chromosomes 04, 11, 15, and 18. Most of the chromosomes contained a mixture of different family genes, except chromosomes 09, 13, 19, and three chromosomes only contained the EREBP family (ERF and DREB subfamily) genes. The 212 CmoAP2/ERF genes were renamed from CmoERF1 through CmoERF92, CmoDREB1 through CmoDREB86, CmoAP2-1 through CmoAP2-28, and CmoRAV1 through CmoRAV6, respectively, based on their order on the chromosome. The precise location of each CmoAP2/ERF gene on the chromosomes of C. moschata is detailed in Additional File 3.
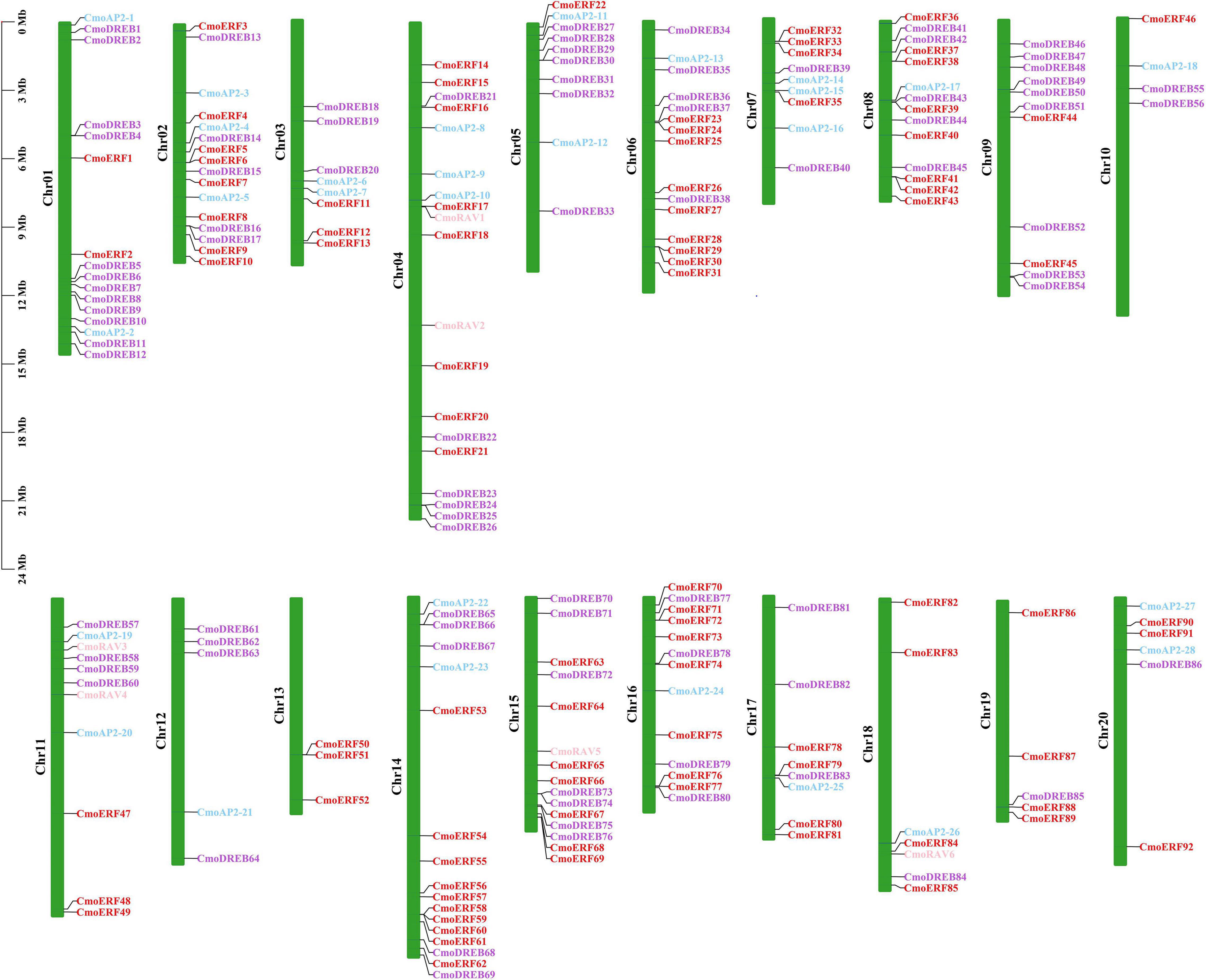
Figure 4. Distribution of CmoAP2/ERF genes in Cucurbita moschata chromosomes. Different colors distinguish subfamily genes. The number of chromosome was indicated on the left of each chromosome.
Gene duplication usually plays a key role in the evolution of gene families. To explore the evolution of CmoAP2/ERF genes, we analyzed tandem and segmental duplication events of these genes. Four pairs of tandem duplication genes were detected, of which two pairs were of ERF genes, and two were of DREB genes (Figure 5 and Additional File 5). A total of 155 pairs of 166 CmoAP2/ERF segmental duplication genes was detected, containing AP2 (21 pairs), RAV (2 pairs), DREB (53 pairs), and ERF (79 pairs) genes (Figure 5 and Additional File 5). These genes accounted for 78.3% of the CmoAP2/ERF genes. In addition, Ka/Ks values for CmoAP2/ERF genes in tandem and segmental duplications were calculated to determine the selection type that promoted the evolution of the CmoAP2/ERF family. The Ka/Ks values of the four tandem duplication gene pairs varied from 0.12 to 0.21 and those for segmental duplication gene pairs ranged from 0.07 to 0.52, showing that all the gene pairs have a Ka/Ks ratio <1. These results indicate that the evolution of CmoAP2/ERF genes is mainly affected under purification selection pressure.
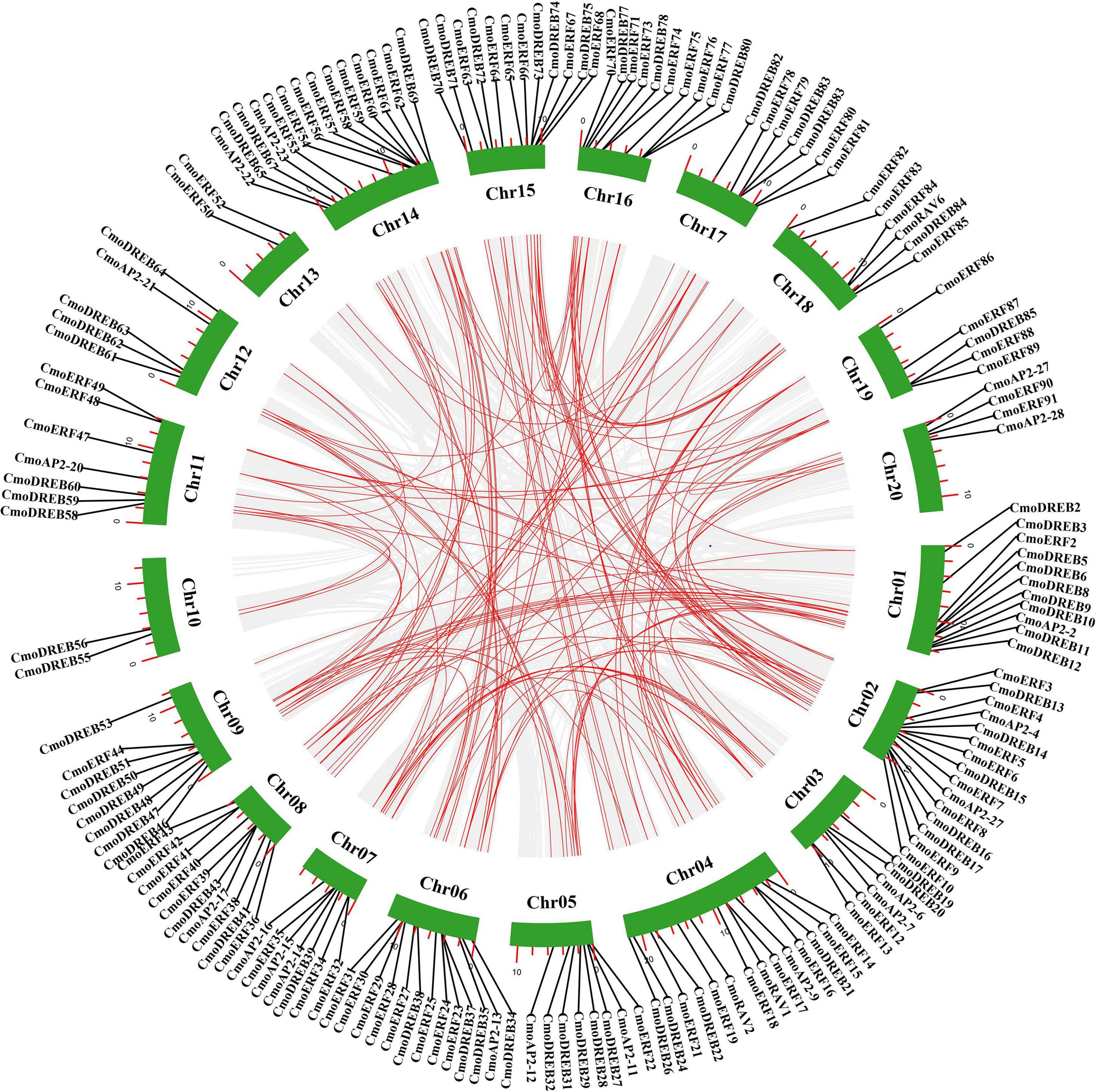
Figure 5. Schematic representations of segmental duplications of CmoAP2/ERFs. Gray lines indicate all syntenic blocks in the Cucurbita moschata genome, and the red lines indicate duplicated CmoAP2/ERF gene pairs. The number of chromosome is shown at the bottom of each chromosome. The red scale bar marked on the chromosome represent the length of the chromosome (Mb).
Cis-Regulatory Elemens in the Promoters of CmoAP2/ERF Family Genes
To better understand the regulatory mechanisms of CmoAP2/ERF genes, CREs of the promoter regions were studied using PlantCARE. The number of CREs and their functional classification are shown in Figure 6. A total of 5643 elements were predicted in the promoter regions of CmoAP2/ERF genes. The results indicated that the CREs mainly included plant hormone responsive elements, stress responsive elements, and plant growth and development elements. Among them, 2458 (43.56%) elements were involved in the response of plants to hormones, such as ethylene (ERE), abscisic acid (ABRE), auxin (TGA-element, AuxRR-core), methyl jasmonate (CGTCA-motif, TGACG-motif), gibberellin (GARE-motif, P-box, TATC-box), and salicylic acid (TCA-element). Among 212 CmoAP2/ERF genes, 183 contained abscisic acid responsive elements, ABREs, which accounted for 9.04% of the total number of CREs in the CmoAP2/ERF family. A total of 166 CmoAP2/ERF genes contained ethylene responsive elements, EREs, which accounted for 13.34% of the total number of CREs in the CmoAP2/ERF family. The results indicated that most of the CmoAP2/ERFs might play a key role in phytohormone regulation, especially for abscisic acid and ethylene. The promoter regions of many CmoAP2/ERF genes contain different binding sites involved in stress response. A total of 1121 (19.87%) elements, such as ARE, W box, TC-rich repeats, LTR, MBS, WUN-motif, GC-motif, were involved in stress response. Among 212 CmoAP2/ERF genes, 174 contained ARE element, which accounted for 7.02% of the total number of CREs in the CmoAP2/ERF family. A total of 121 CmoAP2/ERF genes contained the W box element, which accounted for 3.49% of the total number of CREs in the CmoAP2/ERF family. Overall, 2064 (36.58%) elements, such as G-box, Box 4, O2-site, CAT-box, CCGTCC-box, MRE, circadian, were involved in plant growth and development. G-box and Box 4 were commonly found in the promoter regions of CmoAP2/ERF genes. The number of CmoAP2/ERF genes that possess G-box and Box 4 elements in the promoter regions was 189 and 188, respectively. This suggests that some members of the CmoAP2/ERF family might play important regulatory roles in the growth and development of plants.
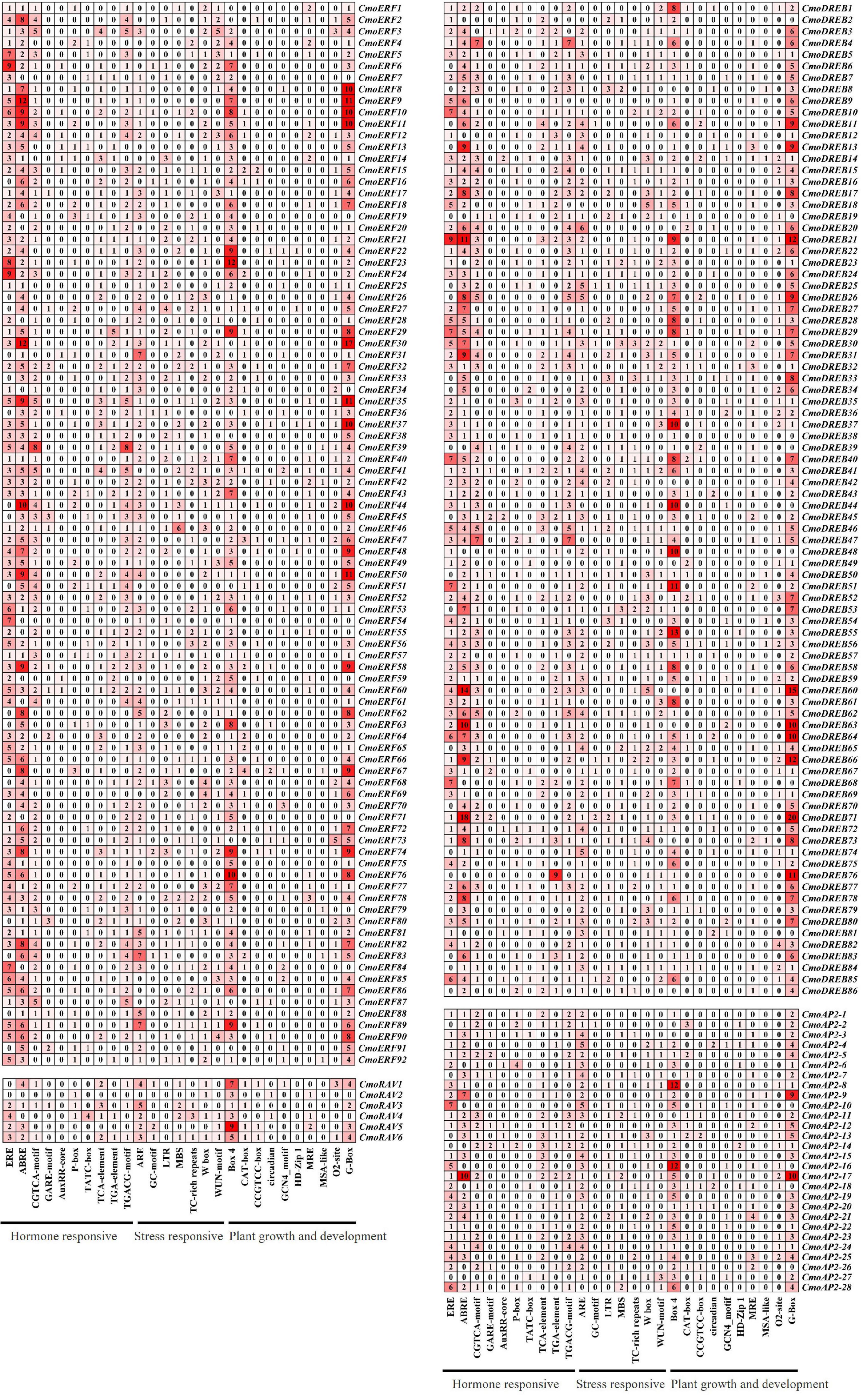
Figure 6. Cis-regulatory elements (CREs) in promoter region of CmoAP2/ERF genes in Cucurbita moschata. The number and the shade of red indicate the number of CREs.
Expression of CmoAP2/ERF Genes in Response to Ethylene Treatment
The results of CRE analysis showed that most of the CmoAP2/ERF genes respond to ethylene. To explore the roles of those genes in the response to ethylene, the RNA-Seq data of shoot apical meristem of pumpkin at the seedling stage after ethephon treatment were used to research their expression patterns. The results showed that 16 CmoAP2/ERF genes, including CmoERF24, CmoERF29, CmoERF53, CmoERF69, CmoERF76, CmoERF81, CmoDREB20, CmoDREB38, CmoDREB39, CmoDREB50, CmoDREB59, CmoDREB83, CmoRAV1, CmoRAV5, CmoRAV6, and CmoAP2-22, were significantly induced (P-value < 0.05, fold change ≥1.5). Notably, all these 16 CmoAP2/ERF genes were upregulated after ethephon treatment (Figure 7). These 16 ethylene-responsive genes were divided into DREB (6 genes), ERF (6 genes), RAV (3 genes), and AP2 (1 gene) subfamilies. They all have different plant hormone responsive elements. Twelve of the sixteen genes possess EREs, and the remaining four genes have other plant hormone responsive elements.
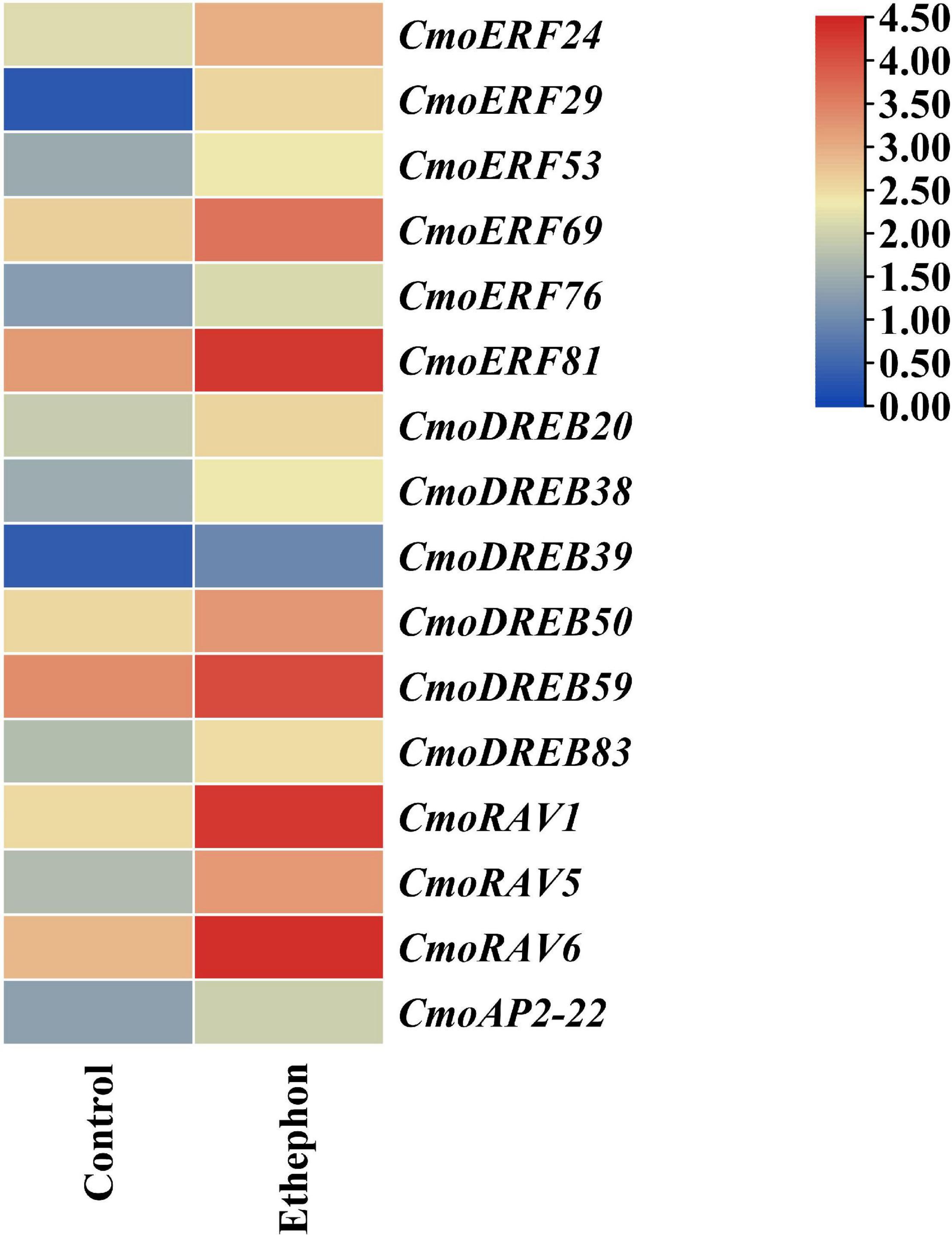
Figure 7. The expression pattern of sixteen CmoAP2/ERF genes in response to ethephon treatment in Cucurbita moschata. Expression levels are normalized according to the FPKM and the color scale refers to the deduced FPKM value normalized with Log2.
Expression Patterns of 16 Ethylene Responsive Genes at Different Developmental Stages of Flower
The results of real-time PCR showed that 16 ethylene-induced genes expressed at different developmental stages of female and male flowers and had different expression patterns (Figure 8). The expression levels of 7 ethylene responsive genes, namely CmoAP2-22, CmoRAV1, CmoRAV5, CmoRAV6, CmoERF29, CmoDREB20, and CmoDREB50 in the three developmental stages of female flowers were significantly higher than that in male flowers. These genes may be more essential in female flowers than in male flowers. In the three stages of female flowers, the expression levels of 10 ethylene responsive genes, namely CmoAP2-22, CmoRAV5, CmoRAV6, CmoERF24, CmoERF29, CmoERF53, CmoERF69, CmoDREB20, CmoDREB38, CmoDREB50, at 0.5 cm stage of female flowers was significantly higher than that in the 1.0 and 2.0 cm stages. Among them, the expression levels of CmoAP2-22, CmoRAV5, CmoERF53, CmoERF76, CmoERF81, CmoDREB20, and CmoDREB38 showed an obvious downward trend from the 0.5 to 2.0 cm stages of female flowers. In the three stages of male flowers, the expression levels of CmoAP2-22, CmoRAV1, CmoERF24, CmoERF29, CmoERF69, CmoERF76, CmoDREB20, and CmoDREB50 at the 0.5 cm stage of male flowers was significantly higher than that in the 1.0 and 2.0 cm stages. Most of the ethylene responsive genes, except CmoAP2-22, CmoERF81, CmoDREB38, CmoDREB39, and CmoDREB83, showed the lowest expression in the 1.0 cm stage. This indicates that these genes are expressed in a time-sensitive manner.
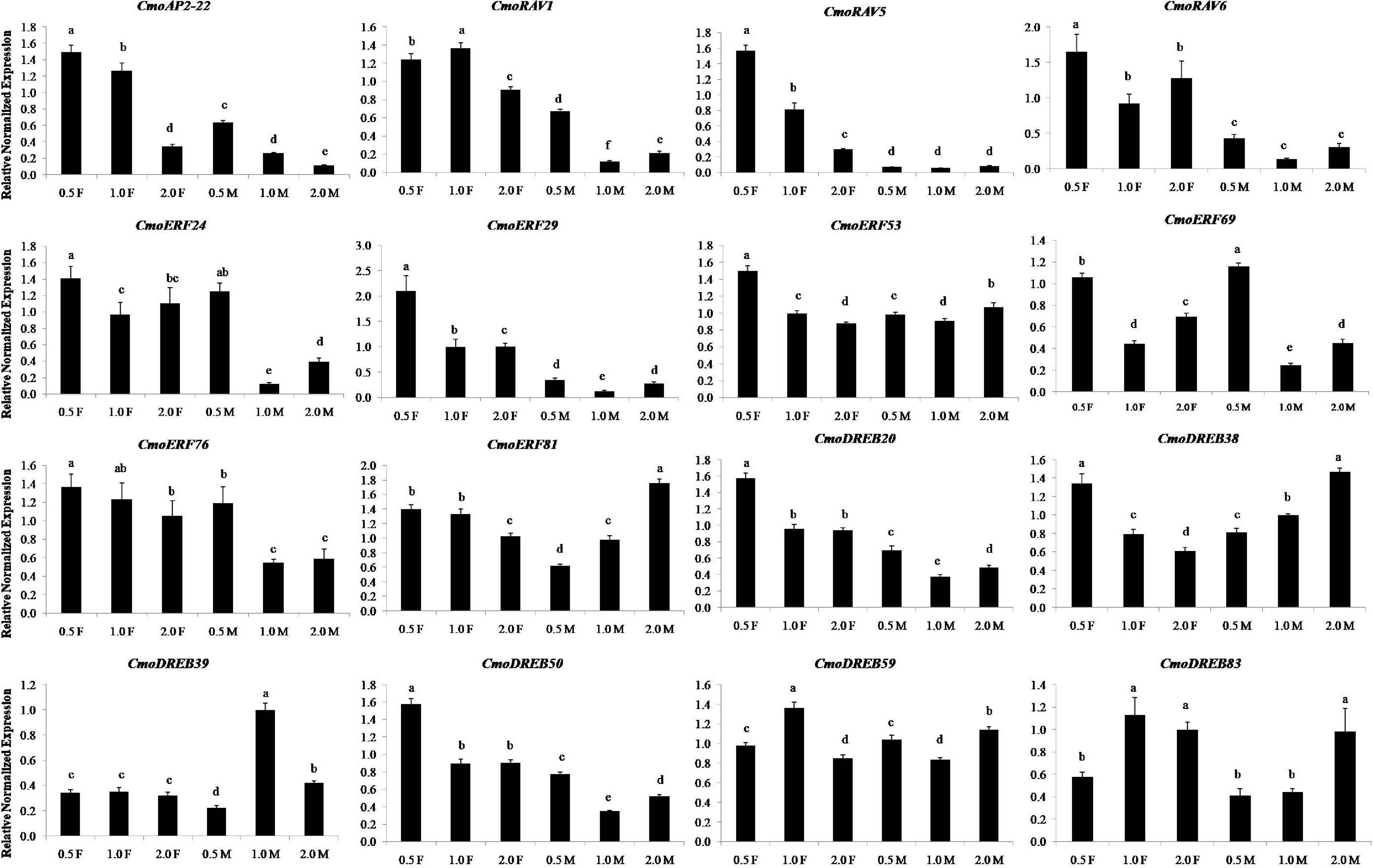
Figure 8. The relative expression levels of the sixteen genes at different developmental stages of flowers in Cucurbita moschata. 0.5, 1.0, and 2.0 represent the vertical diameter of flowers and they were measured in centimeters. The F and M represent female flower and male flower, respectively. Relative expression levels are shown as the mean ± SD from three replications. Different letters in a column indicate significant differences between the stages at p < 0.05 level.
Discussion
As largest plant-specific gene family, the AP2/ERF family plays an important role in regulating plant growth and reproduction, and in coping with environmental stress (Feng et al., 2020). Identification and analysis of gene families provide a basis for systematic study of biological functions of the family members. The information in this regard about the AP2/ERF members of C. moschata is limited. In this study, we identified 212 AP2/ERF members in C. moschata, benefiting from the publication of the C. moschata genome sequence. The number of AP2/ERF family members in C. moschata is greater than that in other Cucurbitaceae species, such as cucumber, melon, watermelon, bitter gourd (Lee et al., 2017), which may be due to the complexity of the evolutionary process.
Gene duplication events play key roles in the evolution of a gene family. Tandem duplications usually produce gene clusters or hot regions and segmental duplications generate homologous genes, which combine to bring about the expansion of the gene number (Alonso et al., 2003; Cannon et al., 2004; Zhang and Li, 2018). Four pairs of tandem duplication genes and 155 pairs of segmental duplication events were identified in C. moschata chromosomes. The high segmental duplications, which accounted for 78.3% of the CmoAP2/ERF genes, may have played important roles in the expansion of the CmoAP2/ERF gene family. In addition, we have found that not all segmentally duplicated genes showed similar functions and stable expressions, which agree with the previous finding (Abdullah et al., 2021). Only three pairs of segmentally duplicated genes (CmoDREB20-CmoDREB39, CmoRAV1-CmoRAV6, CmoERF24-CmoERF76) showed similar expression that they were up-regulated genes in the treatment of ethephon (Figure 7). 21 pairs of segmentally duplicated genes showed a different expression in the treatment of ethephon, as some genes were found up-regulated while some genes did not express. The expression levels of other segmentally duplicated genes were not affected by ethephon treatment. The paralogous numbers (159) of AP2/ERF genes in C. moschata was much higher than that in several plant species, including strawberry (14), jujube (18), and pineapple (27). This may be one of the reasons for the large number of AP2/ERF genes in C. moschata. In addition to tandem duplications and segmental duplications, whole-genome duplication events also affect the number of family members. Studies have found that C. moschata may have experienced whole-genome duplication events, core-eudicot common hexaploidization (ECH), cucurbit-common tetraploidization (CCT), cucurbita-specific tetraploidization (CST) (Jiao et al., 2011; Wang et al., 2018; Yu et al., 2021). Therefore, the large gene number of CmoAP2/ERF family can be attributed to tandem duplications, segmental duplications and whole-genome duplication events.
By analyzing the exon–intron structure of the CmoAP2/ERF genes, we found that 132 members of the CmoAP2/ERF family, including 67 ERF genes, 60 DREB genes, and five of the six RAV genes, had on introns (Figure 2). Similar results have been found in melon which has been indicated that most ERF family genes have no introns (Ma et al., 2015). It may have relations with the 155 pairs of segmental duplication events in the present study, which further confirmed that segmental duplication results in greater opportunities for losing, rather than acquiring, introns (Lin et al., 2006). These results indicate that ERF, DREB, and RAV gene differentiation might have occurred later in the C. moschata evolution. It seems that evolutionary events such as tandem and segmental events in the gene structure as well as in regulatory regions caused a high diversity in functions of AP2/ERF genes (Abdullah et al., 2021; Musavizadeh et al., 2021). Conserved motif analyses could provide more information about functional differences among the family members. The results of conserved motifs showed that Motif-1 and -2 are generally present in all the CmoAP2/ERF protein sequences, which indicates that AP2/ERF family genes are highly conserved in the evolution process of different species. Except Motif-1 and -2, the conserved motifs of the CmoAP2/ERF proteins in different subfamilies were different. Motif-2, -4, and -6 were present in all the AP2 protein sequences. Motif-1, -2, and -3 were present in all the RAV protein sequences. These results indicate that the functions of AP2 and RAV gene members may be comparatively conservative. Most of the genes in the same subfamily usually contain similar gene structures and conserved motifs.
Previous study has shown that many AP2/ERF genes respond to the plant hormones, such as ethylene and ABA (Xie et al., 2019). It is confirmed by our results of CREs analysis, which indicated that 166 of the 212 CmoAP2/ERF genes contained ethylene-responsive elements, EREs, and the ERF subfamily genes contained more number of EREs. The remaining CmoAP2/ERF genes possess other hormone response elements (Figure 6). These genes may play key roles in hormone signaling networks. In this study, we found that 16 CmoAP2/ERF genes could be positively regulated by ethylene. Among them, 12 genes were of the EREBP family, three were RAV genes, and only one gene belonged to the AP2 subfamily. Thus, EREBP family genes may play important roles in the response to ethylene. Similar results have been found in cucumber and melon (Tao et al., 2018). Except for some EREBP family genes, the RAV subfamily members are also involved in ethylene signaling (Alonso et al., 2003) and in the regulation of time of anthesis (Lu et al., 2014). In addition, we found that not all the 16 ethylene-induced genes contain EREs; CmoRAV1, CmoDREB39, CmoDREB50, and CmoDREB83 contain other plant hormone response elements in their promoter regions. The induction of 16 CmoAP2/ERF genes by ethylene might be due to complex plant hormone interactions and network regulatory relationships.
Several studies have shown that AP2/ERF genes are involved in flower development. For example, RAV genes in rice control the heading date and gynoecium development (Osnato et al., 2020). CsERF110 has been related to ethylene signaling and floral sex differentiation in cucumber (Tao et al., 2018). An application of exogenous ethylene could induce more female flower in C. moschata (Li Q. et al., 2021). The results of qRT-PCR analysis showed that 16 ethylene-induced CmoAP2/ERF genes expressed at different developmental stages of female and male flowers. Most of the genes were highly expressed in tender buds (0.5 cm stage). This indicates that these genes may play important roles in flower development. The expression levels of CmoAP2-22, CmoRAV1, CmoRAV5, CmoRAV6, CmoERF29, CmoDREB20, and CmoDREB50 in female flowers were significantly higher than that in male flowers. Furthermore, these 7 genes were up-regulated during ethylene induction of female flowers. Thus, they may post-ively regulate the formation of female flowers during floral sex differentiation of C. moschata. Further researches are required to understand the role of the ethylene-induced genes playing in the process of ethylene induction of female flowers. The results of this study confirm that some of the AP2/ERF genes play important roles in flower development.
Conclusion
In this study, a total of 212 CmoAP2/ERF genes were identified from C. moschata and their basic biochemical information, phylogenetic relationships, chromosome locations, gene structure, conserved motifs, expression profiles under ethylene treatment were determined. The expression levels of 16 ethylene-induced CmoAP2/ERF genes at different developmental stages of female and male flowers were further analyzed. These results should provide an opportunity to understand the roles of CmoAP2/ERFs in ethylene responses and in flower development.
Data Availability Statement
The datasets presented in this study can be found in online repositories. The names of the repository/repositories and accession number(s) can be found in the article/Supplementary Material.
Author Contributions
QL and LZ conceived the study. QL and PC conducted the molecular experiments. CW, HZ, and JY provided software support. XL and JZ provided plant materials. QL wrote the manuscript. XL revised the manuscript. All authors have read and approved the final manuscript.
Funding
This work was supported by the Key Science and Technology Program of Henan Province (212102110128), the Major Public Welfare Projects of Henan Province (201300111300). Funders play roles in the experiment design, data analysis and preparation of the manuscript.
Conflict of Interest
The authors declare that the research was conducted in the absence of any commercial or financial relationships that could be construed as a potential conflict of interest.
Publisher’s Note
All claims expressed in this article are solely those of the authors and do not necessarily represent those of their affiliated organizations, or those of the publisher, the editors and the reviewers. Any product that may be evaluated in this article, or claim that may be made by its manufacturer, is not guaranteed or endorsed by the publisher.
Supplementary Material
The Supplementary Material for this article can be found online at: https://www.frontiersin.org/articles/10.3389/fpls.2022.847754/full#supplementary-material
Abbreviations
NCBI, national center for biotechnology information; TFs, transcription factors; ERF, ethylene responsive factor; DREB, dehydration-responsive element-binding; CDD, conserved domain database; SMART, simple modular architecture research tool; pI, isoelectric point; MW, molecular weight; GRAVY, grand average hydrophobicity; MEME, multiple EM for motif elicitation; MEGA, molecular evolutionary genetics analysis; Ka/Ks, nonsynonymous substitution rate/synonymous substitution rate; FPKM, fragments per kilobase of transcript per million fragments mapped values; FDR, false discovery rate.
Footnotes
- ^ http://cucurbitgenomics.org/organism/9
- ^ https://pfam.xfam.org/
- ^ http://smart.embl.de/
- ^ https://www.ncbi.nlm.nih.gov/Structure/bwrpsb/bwrpsb.cgi
- ^ http://web.expasy.org/protparam/
- ^ https://github.com/CJ-Chen/TBtool
- ^ http://gsds.gao-lab.org/index.php
- ^ http://meme-suite.org/tools/meme
- ^ http://bioinformatics.psb.ugent.be/webtools/plantcare/html/
References
Abdullah Faraji, S., Mehmood, F., Malik, H. M. T., Ahmed, I., Heidari, P., et al. (2021). The GASA gene family in cacao (Theobroma cacao, Malvaceae): Genome wide identification and expression analysis. Agronomy 11:1425. doi: 10.3390/agronomy11071425
Alonso, J. M., Stepanova, A. N., Solano, R., Wisman, E., Ferrari, S., Ausubel, F. M., et al. (2003). Five components of the ethylene-response pathway identified in a screen for weak ethylene-insensitive mutants in Arabidopsis. Proc. Natl. Acad. Sci. U.S.A. 100, 2992–2997. doi: 10.1073/pnas.0438070100
Bowman, J. L., Smyth, D. R., and Meyerowitz, E. M. (1989). Genes directing flower development in Arabidopsis. Plant Cell 1, 37–52. doi: 10.1105/tpc.1.1.37
Cannon, S. B., Mitra, A., Baumgarten, A., Young, N. D., and May, G. (2004). The roles of segmental and tandem gene duplication in the evolution of large gene families in Arabidopsis thaliana. BMC Plant Biol. 4:10. doi: 10.1186/1471-2229-4-10
Chen, C., Chen, H., Zhang, Y., Thomas, H. R., Frank, M. H., He, Y., et al. (2020). TBtools: an integrative toolkit developed for interactive analyses of big biological data. Mol. Plant 13, 1194–1202. doi: 10.1016/j.molp.2020.06.009
Chinnusamy, V., Schumaker, K., and Zhu, J. (2004). Molecular genetic perspectives on cross-talk and specificity in abiotic stress signalling in plants. J. Exp. Bot. 55, 225–236. doi: 10.1093/jxb/erh005
Dossa, K., Wei, X., Li, D., Fonceka, D., Zhang, Y., Wang, L., et al. (2016). Insight into the AP2/ERF transcription factor superfamily in sesame and expression profiling of DREB subfamily under drought stress. BMC Plant Biol. 16:171. doi: 10.1186/s12870-016-0859-4
Elliott, R. C., Betzner, A. S., Huttner, E., Oakes, M. P., Tucker, W. Q., Gerentes, D., et al. (1996). AINTEGUMENTA, an APETALA2-like gene of Arabidopsis with pleiotropic roles in ovule development and floral organ growth. Plant Cell 8, 155–168. doi: 10.2307/3870261
Faraji, S., Filiz, E., Kazemitabar, S. K., Vannozzi, A., Palumbo, F., Barcaccia, G., et al. (2020). The AP2/ERF gene family in Triticum durum: genome-wide identification and expression analysis under drought and salinity stresses. Genes. 11:1464. doi: 10.3390/genes11121464
Feng, K., Hou, X., Xing, G., Liu, J., Duan, A., Xu, Z., et al. (2020). Advances in AP2/ERF super-family transcription factors in plant. Crit. Rev. Biotechnol. 40, 1–27. doi: 10.1080/07388551.2020.1768509
Franco-Zorrilla, J. M., López-Vidriero, I., Carrasco, J. L., Godoy, M., Vera, P., and Solano, R. (2014). DNA-binding specificities of plant transcription factors and their potential to define target genes. Proc. Natl. Acad. Sci. U.S.A. 111, 2367–2372. doi: 10.1073/pnas.1316278111
Fu, M., Kang, H. K., Son, S., Kim, S., and Nam, K. H. (2014). A subset of Arabidopsis RAV transcription factors modulates drought and salt stress responses independent of ABA. Plant Cell Physiol. 55, 1892–1904. doi: 10.1093/pcp/pcu118
Hir, R. L., and Bellini, C. (2013). The plant-specific Dof transcription factors family: new players involved in vascular system development and functioning in Arabidopsis. Front. Plant Sci. 4:164. doi: 10.3389/fpls.2013.00164
Hu, B., Jin, J., Guo, A., Zhang, H., Luo, J., and Gao, G. (2015). GSDS 2.0: an upgraded gene feature visualization server. Bioinformatics 31, 1296–1297. doi: 10.1093/bioinformatics/btu817
Hu, L., and Liu, S. (2011). Genome-wide identification and phylogenetic analysis of the ERF gene family in cucumbers. Genet. Mol. Biol. 34, 624–633. doi: 10.1590/s1415-47572011005000054
Jiang, W., Zhang, X., Song, X., Yang, J., and Pang, Y. (2020). Genome-wide identification and characterization of APETALA2/Ethylene-responsive element binding factor superfamily genes in soybean seed development. Front. Plant Sci. 11:566647. doi: 10.3389/fpls.2020.566647
Jiao, Y., Wickett, N. J., Ayyampalayam, S., Chanderbali, A. S., Landherr, L., Ralph, P. E., et al. (2011). Ancestral polyploidy in seed plants and angiosperms. Nature 473, 97–100. doi: 10.1038/nature09916
Karanja, B. K., Xu, L., Wang, Y., Tang, M., Muleke, E. M., Dong, J., et al. (2019). Genome-wide characterization of the AP2/ERF gene family in radish (Raphanus sativus L.): unveiling evolution and patterns in response to abiotic stresses. Gene 718:144048. doi: 10.1016/j.gene.2019.144048
Kumar, S., Stecher, G., Li, M., Knyaz, C., and Tamura, K. (2018). MEGA X: molecular evolutionary genetics analysis across computing platforms. Mol. Biol. Evol. 35, 1547–1549. doi: 10.1093/molbev/msy096
Labbo, A. M., Mehmood, M., Akhtar, M. N., Khan, M. J., Tariq, A., and Sadiq, I. (2018). Genome-wide identification of AP2/ERF transcription factors in mungbean (Vigna radiata) and expression profiling of the VrDREB subfamily under drought stress. Crop Pasture Sci. 69, 1009–1019. doi: 10.1071/CP18180
Lai, Y., Dang, F., Lin, J., Yu, L., Lin, J., Lei, Y., et al. (2014). Overexpression of a pepper CaERF5 gene in tobacco plants enhances resistance to Ralstonia solanacearum infection. Funct. Plant Biol. 41, 758–767. doi: 10.1071/FP13305
Lakhwani, D., Pandey, A., Dhar, Y. V., Bag, S. K., Trivedi, P. K., and Asif, M. H. (2016). Genome-wide analysis of the AP2/ERF family in Musa species reveals divergence and neofunctionalisation during evolution. Sci. Rep. 6:18878. doi: 10.1038/srep18878
Lee, W. K., Lee, S. C., Ali, A., Kumar, M., Yang, T. J., and Song, K. (2017). Genome-Wide identification and classification of the AP2/EREBP gene family in the Cucurbitaceae Species. Plant Breed. Biotechnol. 5, 122–133. doi: 10.9787/PBB.2017.5.2.123
Lescot, M., D’ehais, P., Thijs, G., Marchal, K., Moreau, Y., Peer, Y. V. D., et al. (2002). Plantcare, a database of plant cis-acting regulatory elements and a portal to tools for in silico analysis of promoter sequences. Nucleic Acids Res. 30, 325–327. doi: 10.1093/nar/30.1.325
Li, C. W., Su, R. C., Cheng, C. P., Sanjaya, You, S. J., Hsieh, T. H., et al. (2011). Tomato RAV transcription factor is a pivotal modulator involved in the AP2/EREBP mediated defense pathway. Plant Physiol. 156, 213–227. doi: 10.1104/pp.111.174268
Li, D., He, Y., Li, S., Shi, S., Li, L., Liu, Y., et al. (2021). Genome-wide characterization and expression analysis of AP2/ERF genes in eggplant (Solanum melongena L.). Plant Physiol. Biochem. 167, 492–503. doi: 10.1016/j.plaphy.2021.08.006
Li, P., Chai, Z., Lin, P., Huang, C., Huang, G., Xu, L., et al. (2020). Genome-wide identification and expression analysis of AP2/ERF transcription factors in sugarcane (Saccharum spontaneum L.). BMC Genomics 21:685. doi: 10.1186/s12864-020-07076-x
Li, Q., Guo, W., Chen, B., Pan, F., Yang, H., Zhou, J., et al. (2021). Transcriptional and hormonal responses in ethephon-induced promotion of femaleness in pumpkin. Front. Plant Sci. 12:715487. doi: 10.3389/fpls.2021.715487
Lin, H., Zhu, W., Silva, J. C., Gu, X., and Buell, C. R. (2006). Intron gain and loss in segmentally duplicated genes in rice. Genome Biol. 7:R41. doi: 10.1186/gb-2006-7-5-r41
Liu, Q., Zhang, G., and Chen, S. (2001). Structure and regulatory function of plant transcription factors. Chinese Sci. Bull. 46, 271–278. doi: 10.1007/BF03187184
Livak, K. J., and Schmittgen, T. D. (2001). Analysis of relative gene expression data using real-time quantitative PCR and the 2(-Delta Delta C(T)) method. Methods 25, 402–408. doi: 10.1006/meth.2001.1262
Lu, A., Yu, M., Fang, Z., Xiao, B., Guo, L., Wang, W., et al. (2019). Preparation of the controlled acid hydrolysates from pumpkin polysaccharides and their antioxidant and antidiabetic evaluation. Int. J. Biol. Macromol. 121, 261–269. doi: 10.1016/j.ijbiomac.2018.09.158
Lu, Q., Zhao, L., Li, D., Hao, D., Zhan, Y., and Li, W. A. (2014). GmRAV ortholog is involved in photoperiod and sucrose control of flowering time in soybean. PLoS One 9:e89145. doi: 10.1371/journal.pone.0089145
Ma, Y., Zhang, F. Y., Bade, R., Daxibater, A., Men, Z., and Hasi, A. (2015). Genome-wide identification and phylogenetic analysis of the ERF gene family in melon. J. Plant Growth Regul. 34, 66–77. doi: 10.1007/s00344-014-9443-z
Manzano, S., Martínez, C., Megías, Z., Gómez, P., Garrido, D., and Jamilena, M. (2011). The role of ethylene and brassinosteroids in the control of sex expression and flower development in Cucurbita pepo. Plant Growth Regul. 65, 213–221. doi: 10.1007/s10725-011-9589-7
Musavizadeh, Z., Najafi-Zarrini, H., Kazemitabar, S. K., Hashemi, S. H., Faraji, S., Barcaccia, G., et al. (2021). Genome-wide analysis of potassium channel genes in rice: expression of the OsAKT and OsKAT genes under salt stress. Genes 12:784. doi: 10.3390/genes12050784
Nakano, T., Suzuki, K., Fujimura, T., and Shinshi, H. (2006). Genome-wide analysis of the ERF gene family in Arabidopsis and rice. Plant Physiol. 140, 411–432. doi: 10.1104/pp.105.073783
Ohme-Takagi, M., and Shinshi, H. (1995). Ethylene-inducible DNA binding proteins that interact with an ethylene-responsive element. Plant Cell 7, 173–182. doi: 10.2307/3869993
Osnato, M., Matias-Hernandez, L., Aguilar-Jaramillo, A. E., Kater, M. M., and Pelaz, S. (2020). Genes of the RAV family control heading date and carpel development in rice. Plant Physiol. 183, 1663–1680. doi: 10.1104/pp.20.00562
Riechmann, J. L., and Meyerowitz, E. M. (1998). The AP2/EREBP family of plant transcription factors. Biol. Chem. 379, 633–646. doi: 10.1515/bchm.1998.379.6.633
Sakuma, Y., Liu, Q., Dubouzet, J. G., Abe, H., Shinozaki, K., and Yamaguchi-Shinozaki, K. (2002). DNA-binding specificity of the ERF/AP2 domain of Arabidopsis DREBs, transcription factors involved in dehydration-and cold inducible gene expression. Biochem. Biophys. Res. Commun. 290, 998–1009. doi: 10.1006/bbrc.2001.6299
Shin, H., and Nam, K. H. (2018). RAV1 negatively regulates seed development by directly repressing MINI3 and IKU2 in Arabidopsis. Mol. Cells 41, 1072–1080. doi: 10.14348/molcells.2018.0259
Sohn, K. H., Lee, S. C., Jung, H. W., Hong, J. K., and Hwang, B. K. (2006). Expression and functional roles of the pepper pathogen-induced transcription factor RAV1 in bacterial disease resistance, and drought and salt stress tolerance. Plant Mol. Biol. 61, 897–915. doi: 10.1007/s11103-006-0057-0
Tao, Q. Y., Niu, H. H., Wang, Z. Y., Zhang, W. H., Wang, Hu, Wang, S. H., et al. (2018). Ethylene responsive factor ERF110 mediates ethylene-regulated transcription of a sex determination-related orthologous gene in two Cucumis Species. J. Exp. Bot. 69, 2953–2965. doi: 10.1093/jxb/ery128
Wang, D., Zhang, Y., Zhang, Z., Zhu, J., and Yu, J. (2010). KaKs_calculator 2.0:a toolkit incorporating gamma-series methods and sliding window strategies. Genomics Proteomics Bioinformatics 8, 77–80. doi: 10.1016/s1672-0229(10)60008-3
Wang, J. P., Sun, P. C., Li, Y. X., Liu, Y. Z., Yang, N. S., Yu, J. G., et al. (2018). An overlooked paleo-tetraploidization in Cucurbitaceae. Mol. Biol. Evol. 35, 16–26. doi: 10.1093/molbev/msx242
Wang, W., Vinocur, B., and Altman, A. (2003). Plant responses to drought, salinity and extreme temperatures: towards genetic engineering for stress tolerance. Planta 218, 1–14. doi: 10.1007/s00425-003-1105-5
Wang, Y., Tang, H., DeBarry, J. D., Tan, X., Li, J., Wang, X., et al. (2012). MCScanX: a toolkit for detection and evolutionary analysis of gene synteny and collinearity. Nucleic Acids Res. 40:e49. doi: 10.1093/nar/gkr1293
Xie, Z., Nolan, T. M., Jiang, H., and Yin, Y. (2019). AP2/ERF transcription factor regulatory networks in hormone and abiotic stress responses in Arabidopsis. Front. Plant Sci. 10:228. doi: 10.3389/fpls.2019.00228
Yu, J. G., Wang, J. Y., Wang, J. Y., and Wang, J. P. (2021). Comparative genomic analysis of pumpkin, grape and wax gourd. Mol. Plant Breed. 19, 4525–4535. doi: 10.13271/j.mpb.019.004525
Zhang, G., Chen, M., Li, L., Xu, Z., Chen, X., Guo, J., et al. (2009). Overexpression of the soybean GmERF3 gene, an AP2/ERF type transcription factor for increased tolerances to salt, drought, and diseases in transgenic tobacco. J. Exp. Bot. 60, 3781–3796. doi: 10.1093/jxb/erp214
Zhang, Z., and Li, X. (2018). Genome-wide identification of AP2/ERF superfamily genes and their expression during fruit ripening of Chinese jujube. Sci. Rep. 8:15612. doi: 10.1038/s41598-018-33744-w
Zhao, H., Zhao, X., Li, M., Jiang, Y., Xu, J., Jin, J., et al. (2018). Ectopic expression of Limonium bicolor (Bag.) Kuntze DREB (LbDREB) results in enhanced salt stress tolerance of transgenic Populus ussuriensis Kom. Plant Cell Tissue Organ Cult. (PCTOC). 132, 123–136. doi: 10.1007/s11240-017-1317-1
Zhou, L., and Yarra, R. (2021). Genome-wide identification and characterization of AP2/ERF transcription factor family genes in oil palm under abiotic stress conditions. Int. J. Mol. Sci. 22:2821. doi: 10.3390/ijms22062821
Zhuang, J., Chen, J., Yao, Q., Xiong, F., Sun, C., Zhou, X., et al. (2011). Discovery and expression profile analysis of AP2/ERF family genes from Triticum aestivum. Mol. Biol. Rep. 38, 745–753. doi: 10.1007/s11033-010-0162-7
Keywords: pumpkin (Cucurbita moschata), AP2/ERF family, genome-wide identification, ethylene response, transcription factors
Citation: Li Q, Zhang L, Chen P, Wu C, Zhang H, Yuan J, Zhou J and Li X (2022) Genome-Wide Identification of APETALA2/ETHYLENE RESPONSIVE FACTOR Transcription Factors in Cucurbita moschata and Their Involvement in Ethylene Response. Front. Plant Sci. 13:847754. doi: 10.3389/fpls.2022.847754
Received: 03 January 2022; Accepted: 11 February 2022;
Published: 15 March 2022.
Edited by:
Mehar Hasan Asif, National Botanical Research Institute (CSIR), IndiaReviewed by:
Ling Xu, Zhejiang Sci-Tech University, ChinaParviz Heidari, Shahrood University of Technology, Iran
Copyright © 2022 Li, Zhang, Chen, Wu, Zhang, Yuan, Zhou and Li. This is an open-access article distributed under the terms of the Creative Commons Attribution License (CC BY). The use, distribution or reproduction in other forums is permitted, provided the original author(s) and the copyright owner(s) are credited and that the original publication in this journal is cited, in accordance with accepted academic practice. No use, distribution or reproduction is permitted which does not comply with these terms.
*Correspondence: Xinzheng Li, lxz2283@126.com